- 1Key Laboratory of Marine Biotechnology of Zhejiang Province, School of Marine Sciences, Ningbo University, Ningbo, China
- 2Key Lab of Mariculture and Enhancement of Zhejiang Province, Zhejiang Marine Fisheries Research Institute, Zhoushan, China
This study examined the effects of extracts of hijiki (Sargassum fusiforme) on the growth and physiology of three species of red tide microalgae (Prorocentrum donghaiensis, Skeletonema costatum, and Heterosigma akashiwo) that commonly grow in the East China Sea. The red tide algae were cultivated with the hijiki extracts at different concentrations to investigate the effects of the extracts on cells growth, chlorophyll a content, maximum quantum yield of PSII (Fv/Fm), the activities of four oxidoreductases including peroxidase (SOD), glutathione S-transferase (GST), glutathione peroxidase (GSH-Px), glutathione reductase (GR), and the level of the membrane lipid peroxidation product, malondialdehyde (MDA). The sensitivity of red tide algae to the extracts varied among the strains, with P. donghaiensis being the most sensitive, followed by S. costatum, and then H. akashiwo. Furthermore, the extracts had a rapid lethal effect on P. donghaiensis at over 1.6 g/L and on S. costatum and P. donghaiensis at over 8 g/L. From that concentration, increasing amounts of the extracts in cultures of S. costatum promoted a reduction in Chla contents and Fv/Fm values. In addition, the oxidoreductase activity of S. costatum was reduced at 4 and 6 g/L, as shown by the reduced activity of SOD, GR, GSH-Px, GST and MDA content in the cells. The results presented herein will be useful to the development and utilization of hijiki on red tide control, and marine environmental protection.
Introduction
Due to the impact of human activities and changes in the natural environment, eutrophication is increasing along the coast of East China Sea, which is harmful for the biodiversity and ecological functions of the marine ecosystem and resulting in frequent occurrence of red tides. Red tide is a disastrous abnormal marine ecological phenomenon, in which some planktonic microalgae, protozoa, or bacteria suddenly proliferate or gather in a short period of time under certain environmental conditions, resulting in a change in water color. Red tide often leads to water hypoxia, resulting in the deaths of a large number of fish, shellfish, and other marine organisms, threatening marine ecology and aquaculture. In addition, microcystins produced by harmful algae accumulate in fish and ultimately endanger human health through the food chain (Yu and Chen, 2019). In the first decade of the twenty-first century, the frequency and scale of red tides in China were three times greater than in the late 1950s. During the period between 2000 and 2017, the cumulative area of red tides in China reached 210,000 square kilometers, with the red tide problems in the East China Sea being by far the most serious as compared to that in South China Sea and Bohai Sea (Yu and Chen, 2019). Red tide has become a constraint on the offshore economy development (León-Muñoz et al., 2018; Mascareño et al., 2018), a threat to human food safety (Van Dolah, 2000; McCabe et al., 2016; Daguer et al., 2018), and an ecological disaster damaging marine ecosystems (Yu and Chen, 2019). Consequently, red tide is now an ecological problem that needs to be addressed urgently (Berdalet et al., 2017). Red tide of the Raphidophyta alga Pseudochattonella cf. verruculosa during the 2016 austral summer (February–March) killed nearly 12% of the Chilean salmon production, causing the worst mass mortality of fish and shellfish ever recorded in the coastal waters of western Patagonia, and the direct economic loss was more than one billion US dollars (León-Muñoz et al., 2018). In October 2017, a red tide of Karenia brevis (formerly known as Gymnodinium breve), which lasted for 15 months, broke out off the coast of Florida in the United States (Soto et al., 2018). To date, more than 200 toxins have been isolated from red tide species including dinoflagellates, diatoms, and cyanobacteria, resulting in toxic effects including diarrheic shellfish poisoning, azaspiracid poisoning, neurotoxic shellfish poisoning, ciguatera fish poisoning, paralytic shellfish poisoning, tetrodotoxin, and amnesic shellfish poisoning. These toxins pose a great threat to human beings and other organisms (Daguer et al., 2018).
Controlling red tides by means of physical and chemical methods may exert adverse effects on the marine ecosystem (Jeong et al., 2000). Some scholars have proposed that the large-scale cultivation of macroalgae might lead to absorption of nitrogen and phosphorus in water (Ahn et al., 1998), regulating the marine ecosystem and inhibiting the occurrence of red tides (Nakai et al., 1999). Macroalgae cultivation may also provide a spawning ground for fish by providing food and attachment bases for fish eggs; therefore, this activity has the potential to play an important role in ecosystem restoration and increasing the diversity of fishery resources (Yang et al., 2015). Studies have confirmed that seaweeds such as Gracilaria confervoides, Gracilariopsis lemaneiformis, Corallina pilulifera, Enteromorpha prolifera, Ulva lactuca, U. fasciata, U. pertusa, and Sargassum thunbergii have allelopathic inhibitory effects on red tide microalgae (Jeong et al., 2000; An et al., 2008; Liu et al., 2011; Lu et al., 2011; Tang and Gobler, 2011). Moreover, the growth (Della et al., 2000), cell function, and cell membrane structure (An et al., 2008; Lu et al., 2011; Tang and Gobler, 2011) of red tide algae such as Skeletonema costatum, Amphidinum sp., and Karenia mikimoto can be inhibited and damaged by the dry power, methanol extracts, filtrate and water-soluble extracts obtained from the water used to cultivate these seaweeds. It has been shown that S. fusiforme reduced the chlorophyll a and maximum chlorophyll fluorescence (Fv/Fm) levels in Karenia mikimotoi (Ma et al., 2017). Although the inhibitory effects of seaweed extracts on the growth of microalgae have been studied, little is known about their mechanisms of inhibition.
Environmental stress may cause an increase in oxygen free radicals such as superoxide anion radicals, hydrogen peroxide, hydroxyl radicals, and singlet oxygens in plant cells. The radicals usually participate in degrading chloroplasts and reducing the content of ascorbic acid and the activity of ascorbate peroxidase, leading to membrane lipid peroxidation. Some of the free radicals are closely related to the bleaching of photosynthetic pigments and membrane lipid peroxidation. Singlet oxygens in cells react with many macromolecular substances, thereby damaging the normal growth and proliferation of cells (Gill and Tuteja, 2010). During evolution, living organisms have developed an antioxidant system to resist external stressors, including a number of enzymes—superoxide dismutase (SOD), catalase (CAT), ascorbate peroxidase (APX), glutathione reductase (GR), glutathione peroxidase (GPX), and glutathione-S-transferase (GST), and non-enzymatic antioxidants—ascorbic acid (ASH), glutathione (GSH), phenolic compounds, alkaloids, non-protein amino acids, a-tocopherols, and malondialdehyde (MDA) (Gill and Tuteja, 2010). The system can reduce the levels of free radicals caused by environmental stress to protect the cells (Gross, 2003; Hejl and Koster, 2004; Gill and Tuteja, 2010). However, excessively high levels of free radicals cannot be eliminated by the antioxidant system, which can reduce the activity of the enzymes in the antioxidant system. Peroxidation damage is one of the main effects of stressors on living organisms.
Sargassum fusiforme is an edible brown algae with high medicinal value that is widely distributed along the coasts of China, South Korea, and Japan (Hu et al., 2016; Sun et al., 2019; Zhang et al., 2020). In recent years, hijiki has been cultivated on a large scale in Dongtou, Wenzhou, Zhejiang Province. We conducted several investigations in April and June of 2018 and 2019 (data not published), and found that there were significant differences in the community structure of phytoplankton between cultivation and non-cultivation areas, and that the abundance and dominance of Skeletonema costatum in the cultivation areas were significantly lower than in non-cultivation areas. In this study, we analyzed the effects of hijiki extracts on the growth, Chla content, maximum quantum yield, and enzyme activity of the red tide algae Heterosigma akashiwo, S. costatum and Prorocentrum donghaiensis, which commonly grow in the East China Sea (Liu et al., 2013; Jiang et al., 2017). We sought to determine the inhibitory effects of S. fusiforme on marine microalgae, identify the red tide microalgae that are inhibited by it, as well as to understand the biological mechanism of the inhibitory effects of hijiki on microalgae.
Materials and Methods
Microalgae Strains and Cultivation Conditions
The red tide microalgae Heterosigma akashiwo, Skeletonema costatum, and Prorocentrum donghaiensis were purchased from Shanghai Guangyu Biotechnology Co., Ltd. (Zhejiang, China). These microalgae were cultivated in f/2(+Si) medium and transferred to fresh medium every week for proliferation. Algae cultivation was performed in a smart light growth chamber (Ningbo Southeast Instrument Co., Ltd., Zhejiang, China). The temperature was 23°C ± 0.1 for H. akashiwo and S. costatum, and 20°C ± 0.1 for P. donghaiensis. Light intensity was 4,000 umol.m–2⋅s–1 with a light:dark photoperiod of 12 h:12 h.
Preparation of Seaweed Samples and Extracts
S. fusiforme was collected from Banping Island hijiki culture area, Dongtou, Wenzhou (N 120°59′45″–121°15′58″, E 27°41′19″–28°01′10″). The samples were then washed with pure water and dried at room temperature to a constant weight. The dried hijiki was subsequently pulverized into powder and stored at −20°C until use. Prior to use, the powder was soaked in sterilized seawater for 48 h at a powder-to-seawater ratio of 100 g:1 L. Next, the extracts were filtered through a 400 mesh silk net sieve and centrifuged (15 min at 12,000 rpm). After centrifugation, the supernatant was collected as the stock solution of the hijiki extracts with a concentration of 100 g/L. Both sterilized (121°C, 20 min) and non-sterilized stock solutions were utilized in this study. The concentration of the extracts was expressed as dry weight (g) of S. fusiforme per volume (L) of seawater.
Sterilization on the Inhibitory Potential of the Hijiki Extracts Against Microalgae Growth
Either the sterilized or unsterilized hijiki extracts, microalgae in the exponential phase, and enriched f/2 medium prepared with sterilized seawater were added into a 2,000 mL culture flask to a working volume of 1,000 mL with 6 g/L extracts. No extract was added in the control group. The densities of the P. donghaiensis, S. costatum, and H. akashiwo were 68.3 × 106, 70.8 × 106, and 65.2 × 106 cells/L, respectively. The cultivation of the three strains was performed using both sterilized and unsterilized hijiki extracts; and each treatment was replicated thrice. This gave a total of 6 treatments and 18 experimental runs (Table 1). During cultivation, 1 mL samples were collected at 48, 96, and 144 h to measure the density of microalgae. Lugol’s solution (0.05 mL) was added to stain the cells. Using a 0.1 mL Sedgewick Rafter cell (Figure 1), the density of microalgae was counted under an optical microscope, after which the inhibitory rate (IR) was calculated using the following equation:
where N0 and Nt are control density of microalgae and the density at time t of culture, respectively.
Effect of the Concentration of the Hijiki Extracts on Microalgae Growth
Culture systems with 0, 0.4, 0.8, 1.2, 1.6, 2.0, 4.0, 8.0, and 10.0 g/L extracts were prepared, t to study whether the extracts had the same inhibitory effect on three types of red tide microalgae grown at different densities. The culture systems with 0.4, 0.8, 1.2, and 1.6 g/L extracts were designated as the low-concentration group, in which microalgae grew at normal density. The initial densities of P. donghaiensis, S. costatum, and H. akashiwo in the low-concentration extract groups were 420, 650, and 610 cells/L, respectively. The culture systems with 2.0, 4.0, 8.0, and 10.0 g/L extracts were designated as the high-concentration group, in which the microalgae grew at red tide density. The initial densities of P. donghaiensis, S. costatum, and H. akashiwo in this group were 75.2 × 106, 78.5 × 106, and 72.2 × 106 cells/L, respectively. The treatment with 0 g/L of hijiki extract was considered as the control group. At 24, 48, 72, 96, 120, 144, and 168 h, 1 mL of culture solution was collected into a centrifuge tube and stained to measure the density of microalgae and calculate the IR and the relative growth rate (μ). Another 1 mL of the culture solution was collected to observe the morphology of living cells.
The sample needed to be diluted in order to count it accurately when the cell density was high. The approximate cell density was estimated before dilution. Then, 0.1 ml solution was dropped into the counting plate, and the three squares shown in the green triangle in Figure 1 were selected to count the number of cells to estimate the approximate cell density because the cell density in the Sedgewick Rafter cell often decreases from the middle to the edge. The dilution factor (d) was selected according to the principle that there should be about 10 cells each square after dilution. The number of cells (n) in 0.1 mL solution after dilution was calculated, and was multiplied by d to obtain the cell density of the solution before dilution.
With <1 cell per 0.1 mL solution, the sample needed to be concentrated to count it accurately. In a test tube, Lugol’s reagent was used to stain the cells in 10 mL of solution, and the cells were allowed to settle for 24 h. The stained cells settled to the bottom of the test tube due to the gravity effect. A pipet was used to carefully discard the supernatant until the volume was 1, 0.5, or 0.1 mL for counting. The concentration factor was c. The number of cells (n) in 0.1 mL solution after concentration was calculated, and was divided by c to obtain the cell density of the solution before concentration.
The half inhibitory concentrations (IC50) of the extracts on microalgae at day 5 were calculated using the linear interpolation method.
In this study, the IC50 was calculated by the linear interpolation method (Han et al., 2013) between two given points as follows:
The cell densities of the two given points were (a, Na) and (b, Nb).
Solving this equation gives
If y = 0.5 NC,
In the equation, a and b are the two given concentrations and Na and Nb are the cell densities at the given concentrations, respectively; x is the unknown concentration; y is the cell density at the unknown concentration; Nc is the cell density of the control at Nh. In this study, the cell density of the control at 144 h was used.
The relative growth rate (μ) was calculated using the following equation:
where μ is the relative growth rate, h–1; Xn is the density of microalgae at time tn; and X(n−1) is the density of microalgae at time t(n−1).
Determination of Chla and Fv/Fm
The culture systems were prepared in the same way as described in the section “Sterilization on the Inhibitory Potential of the Hijiki Extracts against Microalgae Growth,” and the final concentrations of the extracts were set to 0, 2.0, 4.0, 8.0, and 10.0 g/L. Each culture system was prepared in triplicate. At 0, 4, 8, 12, 24, 48, 72, 96, 120, 144, and 168 h, 50 mL of the culture solutions were collected into centrifuge tubes and placed in the dark for adaption for 15 min, after which the maximum quantum yield (Fv/Fm) (Davide et al., 2016; Guan and Li, 2017) was measured using a handheld chlorophyll fluorometer (AquaPen-C AP-C 100) and the Chla content was determined using a spectrophotometer. Next, the culture solution was sampled and filtered through a 0.45 μm nitrocellulose filter membrane, after which the filter membrane was placed in a centrifuge tube and 10 mL of 90% acetone was added. After being held for 24 h at 4°C, the tube was centrifuged at 3,000 rpm for 10 minutes and the absorbance of the supernatants was then measured at 750, 664, 647, and 630 nm using 90% acetone as a reference. The content of Chla was calculated using the equation modified by Jeffrey and Humphrey (1975):
where Chla is the concentration (μg/L) of chlorophyll a; v is the volume (mL) of the sampled culture solution; V is the actual volume (L) of seawater used; L is the pathlength (cm) of the cuvette; and E664, E647 and E630 are the absorbance values of the solution at 664, 647, and 630 nm, respectively.
Determination of Enzyme Activity
The culture system was prepared in the same way as described in the section “Sterilization on the Inhibitory Potential of the Hijiki Extracts against Microalgae Growth,” except that the working volume was 1,250 mL. At 0, 4, 8, 12, 24, 48, 72, 96, 120, 144, and 168 h, 100 mL aliquots of the culture solutions were collected. Before sampling, the culture flask was shaken to evenly distribute the algal cells in the culture solution. After sampling, the culture solution was centrifuged at 1,000 rpm/min for 10 min and pellet cells were collected. Commercial kits A001-1-2, A003-1-3, A004-1-1, A005-1-2, and A062-1-1 were used to determine the activity or content of SOD, MDA, GST, GSH-Px, and GR, respectively.
SPSS Statistical Analysis of Data
Excel 2016 was used to plot the data. SPSS 20.0.0 was used to conduct one-way ANOVA analysis and a p < 0.05 was considered to be significant.
Results
The pH Values of the Cultures
The pH values of both sterilized and non-sterilized extracts were 4.967 ± 0.003. The pH values of the high- concentration groups (0, 2, 4, 6, 8, and 10 g/L) were 8.309 ± 0.008, 8.179 ± 0.017, 8.044 ± 0.009, 7.815 ± 0.064, 7.708 ± 0.057, and 7.634 ± 0.065, respectively. The pH values of the low- concentration groups (0, 0.4, 0.8, 1.2, and 1.6 g/L) were 8.293 ± 0.004, 8.281 ± 0.006, 8.267 ± 0.014, 8.246 ± 0.016, and 8.235 ± 0.011, respectively. Therefore, the addition of hijiki extracts reduced the pH value of algae cultures (Figure 2).
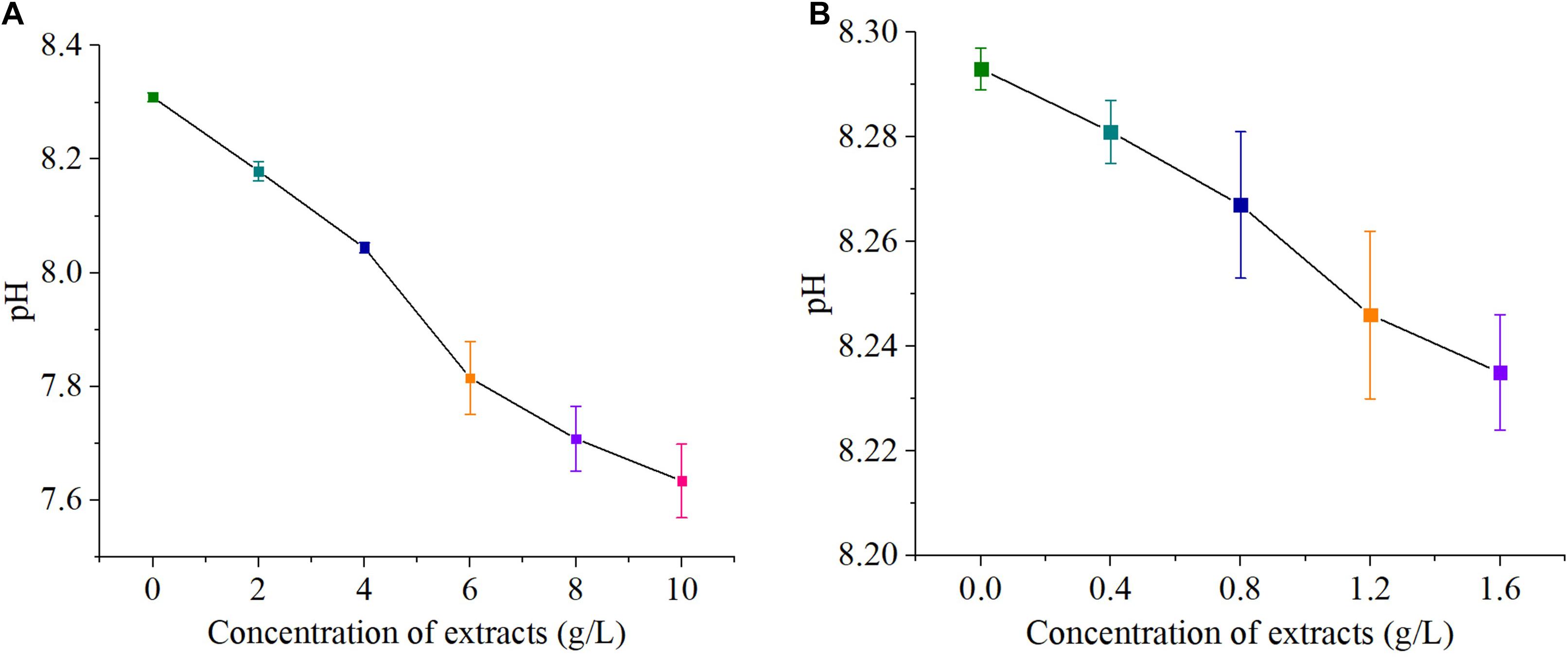
Figure 2. pH value of concentration S. fusiforme extracts, high concentration (A) and low concentration (B).
Inhibitory Effect of Sterilized and Unsterilized Hijiki Extracts on Microalgae Growth
The effects of the sterilized and unsterilized 6 g/L hijiki extracts on the daily growth rates of P. donghaiensis, S. costatum, and H. akashiwo were analyzed. The daily growth rate was determined at 48, 96, and 144 h of culture. The average daily growth rates of three microalgae at different times were slightly higher in the cultures with sterilized extracts than in those with unsterilized extracts, but these differences were not significant (p > 0.05) (Figure 3), indicating that high temperature and high pressure sterilization did not change the inhibitory effects of the extracts on the red tide microalgae. To prevent contamination by bacteria and protists, the extracts used for all experiments in this study were sterilized.
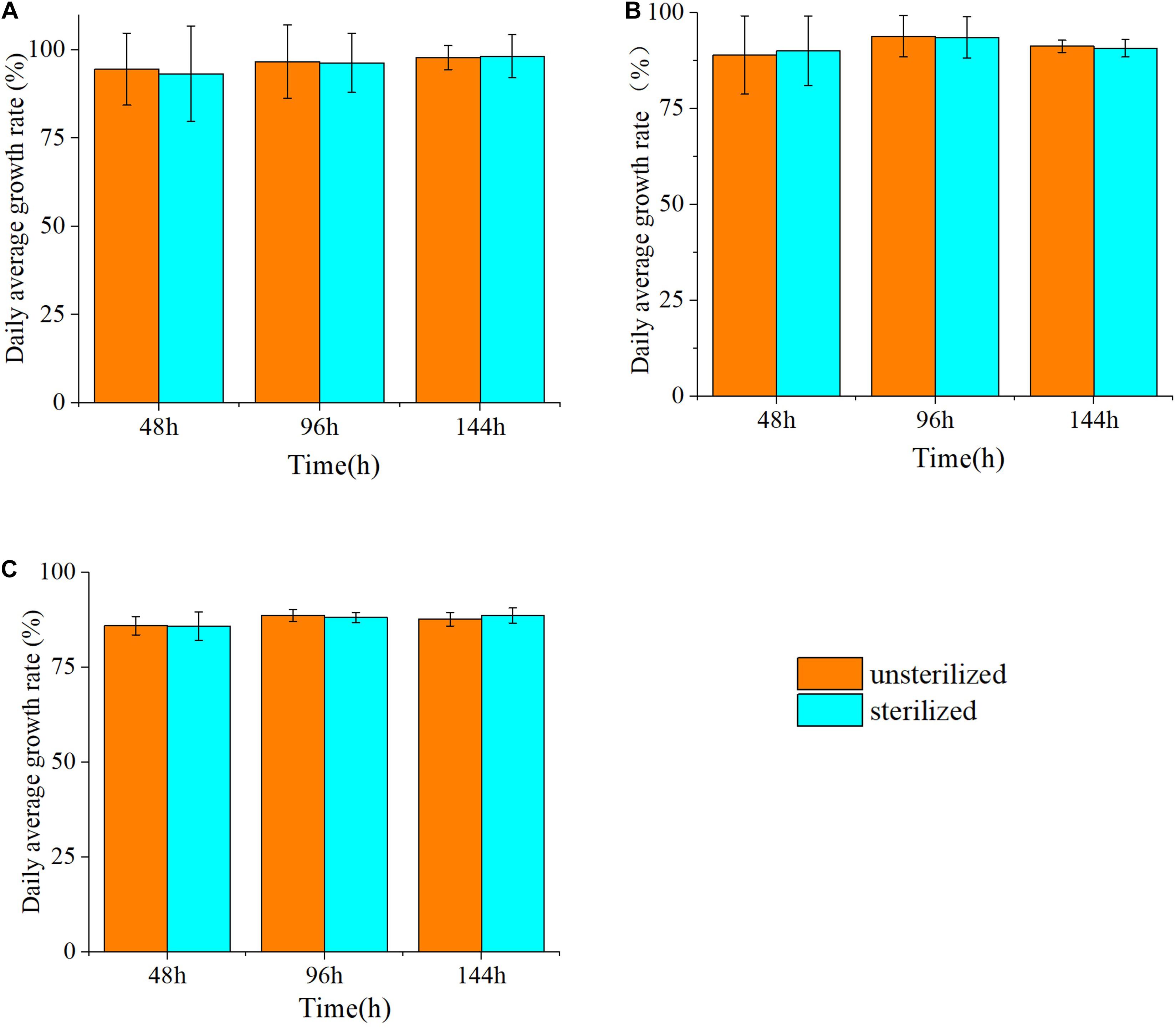
Figure 3. The inhibitory rate (%) of three species of microalgae cultured with unsterilized and sterilized 6 g/L hijiki extract for 48, 96, and 144 h. (A) Prorocentrum donghaiensis; (B) Skeletonema costatum; (C) Heterosigma akashiwo.
IC50 of Hijiki Extracts on Different Concentration of Microalgae
Based on the linear interpolation method, the IC50 of the extracts for inhibiting the growth of three microalgae of high density and low density within 144 h was analyzed. The IC50 on high density of P. donghaiensis, S. costatum, and H. akashiwo were 2.0 ± 0.5 g/L, 2.3 ± 0.4 g/L and 2.9 ± 0.7 g/L, respectively, while those on low density P. donghaiensis, S. costatum, and H. akashiwo were 0.19 ± 0.06 g/L, 0.25 ± 0.05 g/L, and 0.35 ± 0.08 g/L (Table 1). Therefore, the sequence of the inhibitory effect of the hijiki extracts on three microalgae at both density levels was P. donghaiensis > S. costatum > H. akashiwo.
Effect of Hijiki Extract Concentration on the Growth and Morphology of Microalgae
Effect on the Growth of Microalgae at Low Cell Densities
As the concentration of Hijiki extracts increased, the inhibitory effects on the growth of P. donghaiensis, S. costatum, H. akashiwo gradually increased. Extract concentrations over 1.6 g/L showed lethal effects on P. donghaiensis in 48 h. After 24 h of cultivation, the cell densities of P. donghaiensis, S. costatum, and H. akashiwo cultured with 0.4, 0.8, 1.2, and 1.6 g/L extracts were significantly lower than that of the control groups (p < 0.05) (Figures 4A–C). The relative growth rates of P. donghaiensis (0.0 g/L: 0.014 ± 0.005, 0.4 g/L: 0.010 ± 0.006, 0.8 g/L: 0.004 ± 0.001, 1.2 g/L: −0.023 ± 0.009, and 1.6 g/L: −0.075 ± 0.012), S. costatum (0.0 g/L: 0.013 ± 0.005, 0.4 g/L: −0.047 ± 0.010, 0.8 g/L: −0.058 ± 0.011, 1.2 g/L: −0.074 ± 0.015, and 1.6 g/L: −0.078 ± 0.021), and H. akashiwo (0.0 g/L: 0.017 ± 0.009, 0.4 g/L: 0.012 ± 0.004, 0.8 g/L: −0.005 ± 0.001, 1.2 g/L: −0.008 ± 0.002, and 1.6 g/L: −0.018 ± 0.008) cultured with 0.4, 0.8, 1.2, and 1.6 g/L extracts at 0–24 h were significantly lower than the control (p < 0.05), decreased with the increase in the concentration of the extracts, and recovered after 24 h (Table 2). The P. donghaiensis cultured with 0.4 and 0.8 g/L maintained a rapid growth during culture, while that cultured with 1.2 g/L extracts grew slowly (Table 2). No living cells of P. donghaiensis were found at 48 h cultured with 1.6 g/L extracts (Figure 4A). The S. costatum and H. akashiwo cultured with 0.4, 0.8, and 1.2 extracts underwent a rapid growth during culture (μ > 0), while those cultured with 1.6 g/L extracts grew slowly (Table 2).
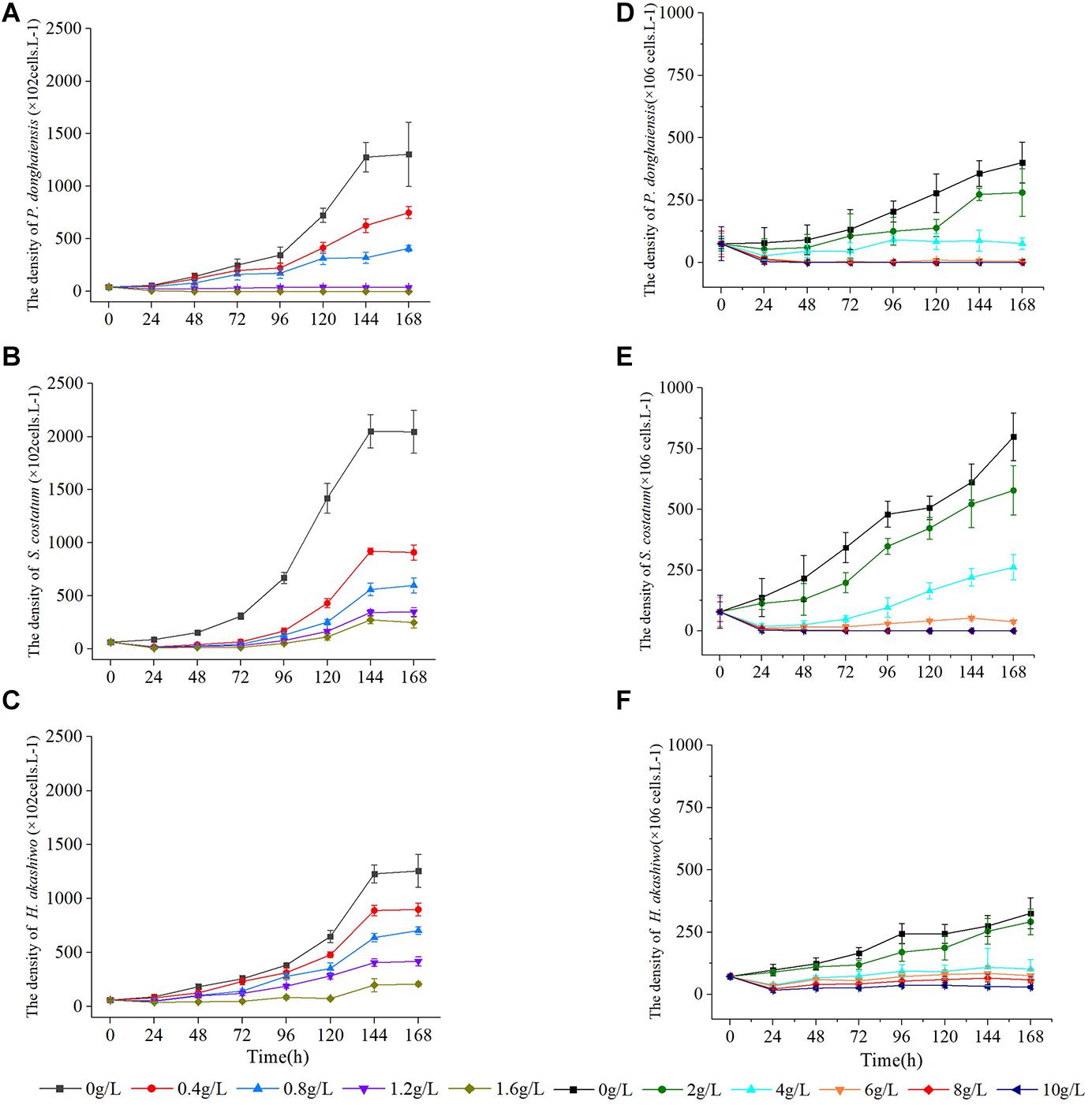
Figure 4. Effect of hijiki extracts on, Prorocentrum donghaiensis, Skeletonema costatum, and Heterosigma akashiw Low density (A–C) and high density (D–F).
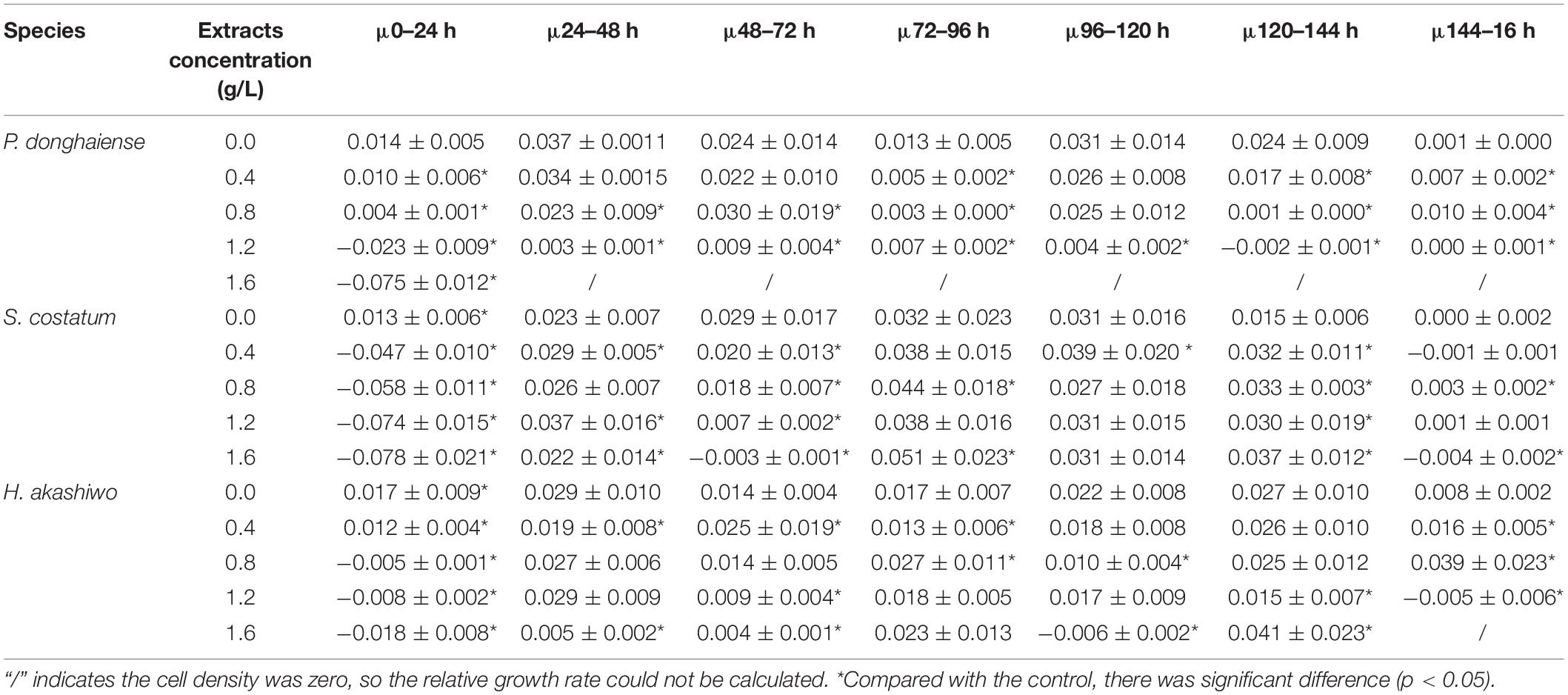
Table 2. The relative growth rate of low density microalgae under the influence of different concentrations of extracts (μ) (h–1).
Effect on the Growth of Microalgae at High Cell Densities
As the concentration increased, the inhibitory effects of hijiki extracts on the growth of P. donghaiensis, S. costatum, and H. akashiwo increased. After 24 h of cultivation, the cell densities of P. donghaiensis, S. costatum, and H. akashiwo cultured with 2, 4, 6, 8, and 10 g/L extracts were significantly lower than that of the control groups (p < 0.05) (Figures 4D–F). The inhibitory effect of extracts on P. donghaiensis was stronger than on S. costatum and H. akashiwo. When cultured with 2, 4, 6, 8, and 10 g/L extracts for 24 h, the relative growth rates of P. donghaiensis (0 g/L: 0.002 ± 0.001, 2 g/L: −0.014 ± 0.006, 4 g/L: −0.043 ± 0.020, 6 g/L: −0.067 ± 0.033, 8 g/L: −0.078 ± 0.035, and 10 g/L: −0.128 ± 0.086), S. costatum (0 g/L: 0.023 ± 0.008, 2 g/L: 0.015 ± 0.009, 4 g/L: −0.061 ± 0.023, 6 g/L: −0.081 ± 0.025, 8 g/L: −0.090 ± 0.039, and 10 g/L: 0.153 ± 0.061), and H. akashiwo (0 g/L: 0.013 ± 0.005, 2 g/L: 0.009 ± 0.004, 4 g/L: −0.027 ± 0.011, 6 g/L: −0.031 ± 0.012, 8 g/L: −0.050 ± 0.019, and 10 g/L: −0.060 ± 0.020) were significantly lower than that of the control (p < 0.05), and decreased with the increase in the concentration of the extracts, indicating that those extract concentrations had very strong inhibitory effects on the growth of the three microalgae (Table 3). The relative growth rate of P. donghaiensis cultured with 2 and 4 g/L extracts recovered after 24 h, and the growth of P. donghaiensis cultured with 6 g/L extracts decreased during culture. The growth of S. costatum cultured with 4 and 6 g/L extracts recovered after 24 h, and that of H. akashiwo cultured with 4, 6, 8, and 10 g/L recovered after 24 h (Table 3). S. costatum and H. akashiwo cultured with 2 g/L grew rapidly during culture. When cultured with 8 and 10 g/L of the extracts, a small number of living cells of P. donghaiensis was observed at 24 h, but no living cells were found at 48 h. Similarly, a small number of living cells of S. costatum was observed at 24 h, but no living cells were found at 96 h (Figure 4).
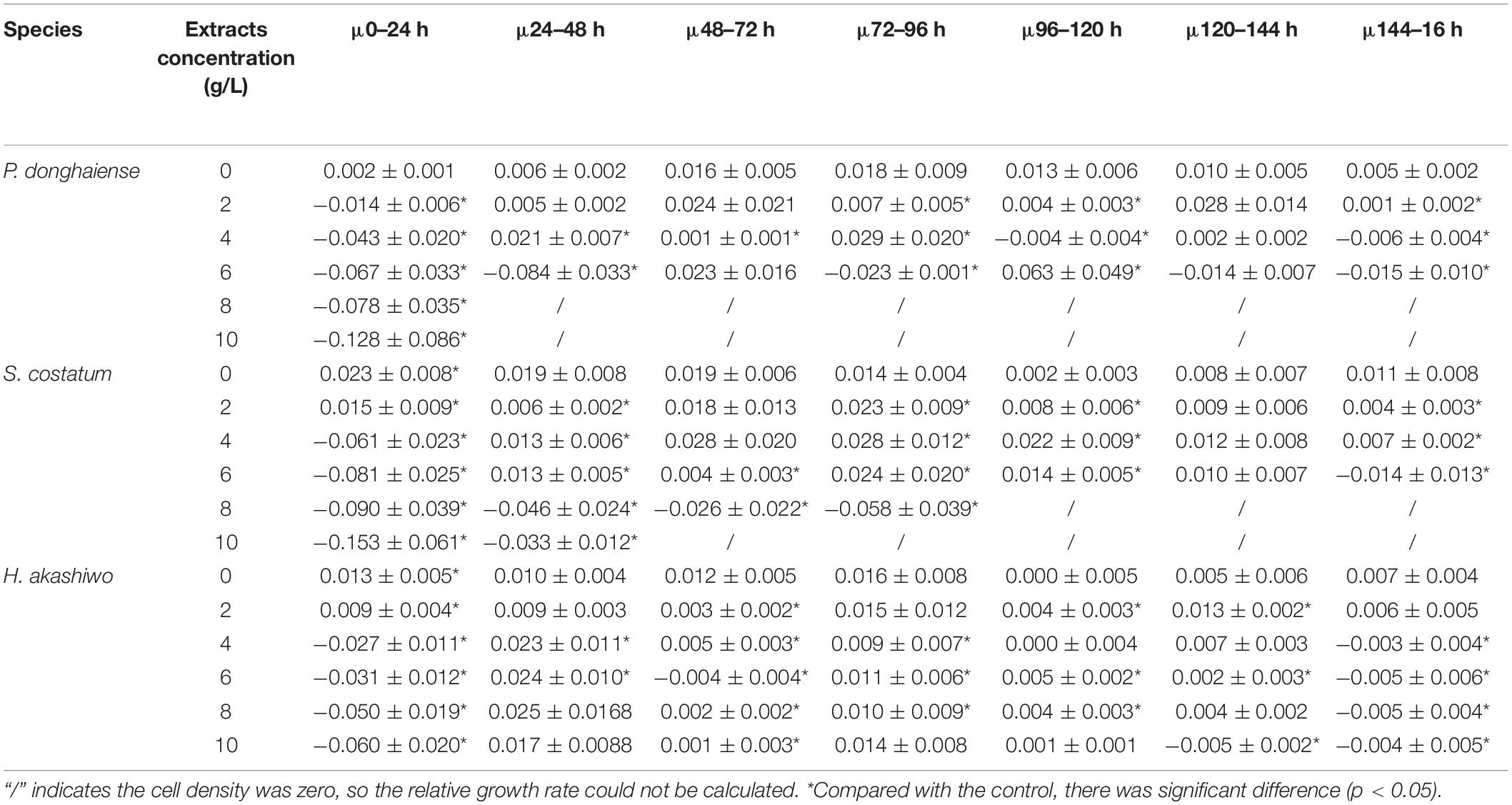
Table 3. The relative growth rate of high density microalgae under the influence of different high concentrations of extracts (μ) (h–1).
Effect on the Morphology of Microalgae
The hijiki extracts affected the morphology of P. donghaiensis and the growth of S. costatum. Normally, P. donghaiensis has fusiform cells. However, in this study, cells of P. donghaiensis cultured with seaweed extracts showed varying degrees of enlargement. The number of enlarged cells increased with increasing concentrations of hijiki extracts and duration of culture. By day 9 of culture with 6 g/L extracts, almost all algal cells had round or square shapes with enlarged nuclei, some cells grew exospores, and no dividing cells were observed. No living cells were found on day 10 of culture (Figure 5). Most of the S. costatum cells in the control grew into filaments and were distributed evenly in culture solution, while in the culture with hijiki extracts the cells gathered and grew in clusters that were difficult to disperse. Moreover, higher concentrations of hijiki extracts resulted in more clustering and lower cells survival. For example, at 96 h, living algal cells were still common in the culture with 4 g/L extracts, while they were sporadic in the treatment with 8 g/L extracts and not present in the 10 g/L extract treatment (Figure 6).
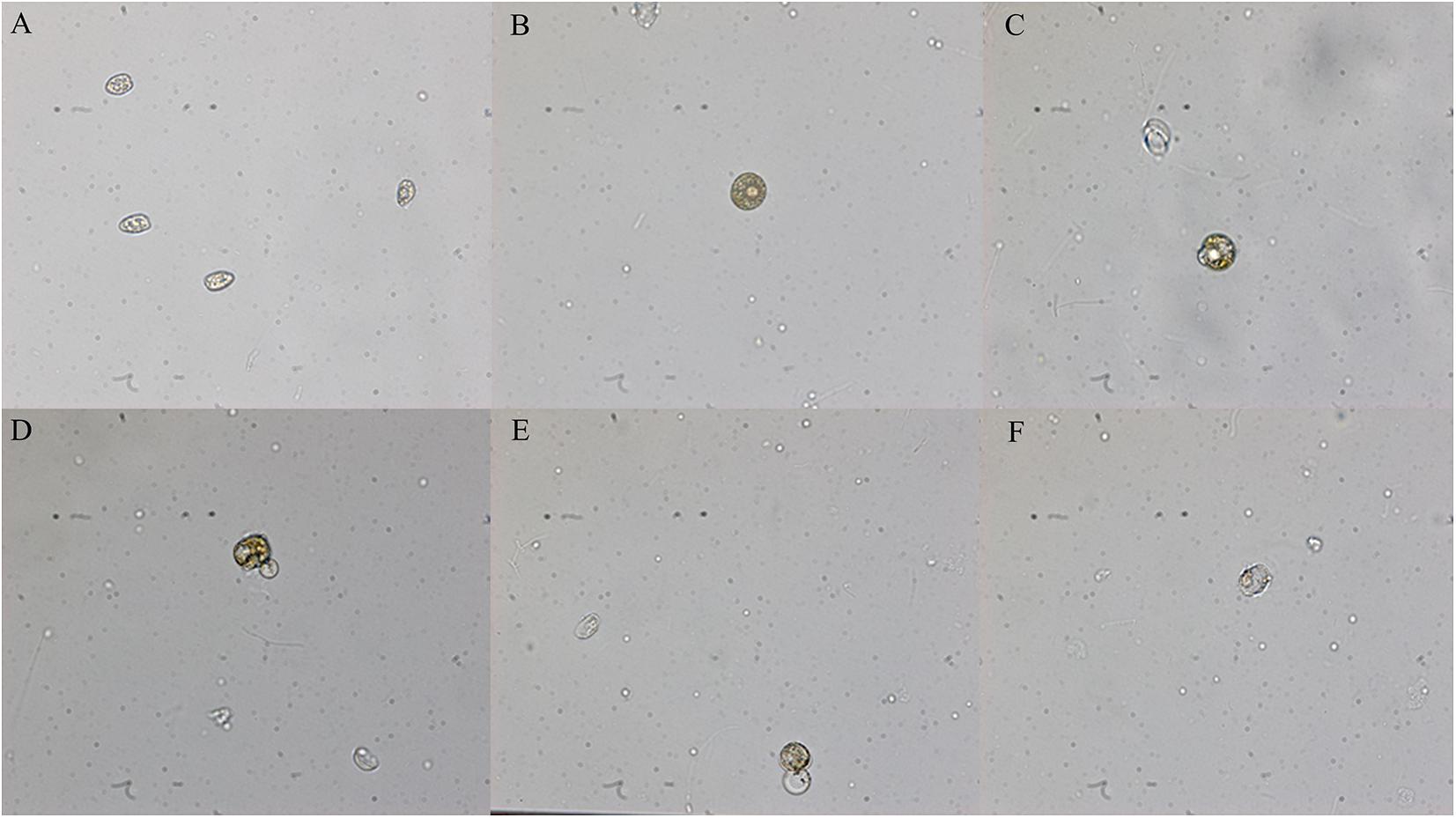
Figure 5. Morphological changes of Prorocentrum donghaiensis caused by hijiki extract (40× objective lens). (A) Normal cells; (B) round cells; (C) small exospores; (D) medium exospores; (E) large exospores; (F) dead cells.
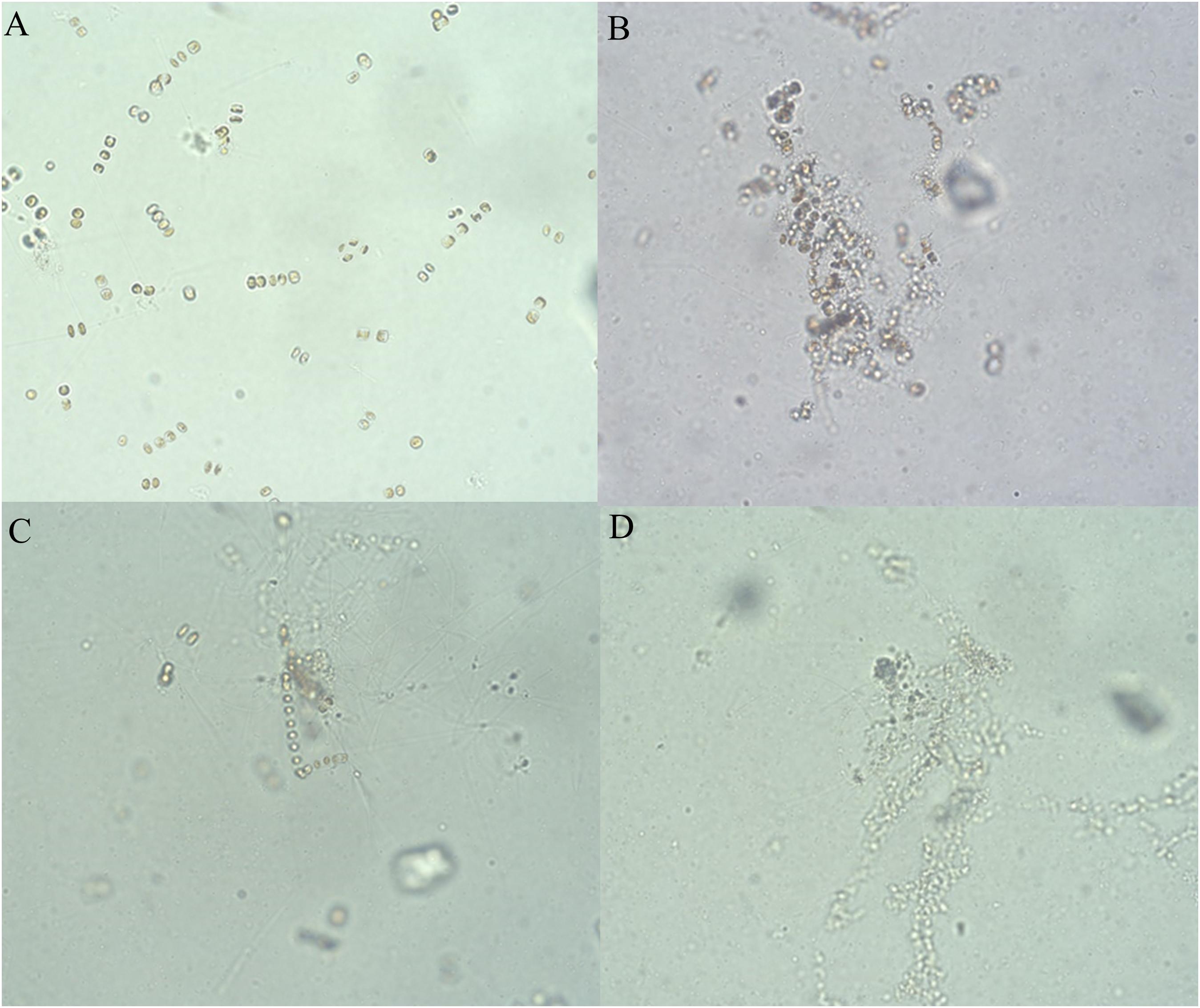
Figure 6. Growth of Skeletonema costatum cultured at different concentrations of hijiki extracts for 96 h (20× objective lens). (A) Control; (B) extract concentration of 4 g/L; (C) extract concentration of 8 g/L; (D) extract concentration of 10 g/L.
Effect on Chla Content and Maximum Quantum Yield (Fv/Fm)
As the concentration of extracts increased, the Chla content of S. costatum gradually decreased. At 12 h of starting the cultivation, there was no significant difference in algal Chla content between the treatments with 2, 4, and 6 g/L extracts and the control (p > 0.05), while after 12 h, the algal Chla contents were all significantly lower than that of the control (p < 0.05). In the culture with 8 and 10 g/L extracts, Chla content dropped to 0 at 24 h (Figure 7A). Additionally, the Fv/Fm was significantly higher than that of the control (p < 0.05) at 4, 8, and 12 h, after which it gradually decreased relative to the control. At 24 h, the Fv/Fm decreased to 0 in the culture with 8 g/L extracts. At 48 h, the Fv/Fm in the culture with 10 g/L extracts decreased to 0. In the culture with 2, 4, and 6 g/L extracts, the Fv/Fm decreased first, and then gradually increased (Figure 7B), but was significantly lower than that of the control at all levels (p < 0.05). After 12 h, the Fv/Fm value was basically consistent with that of the Chla content.
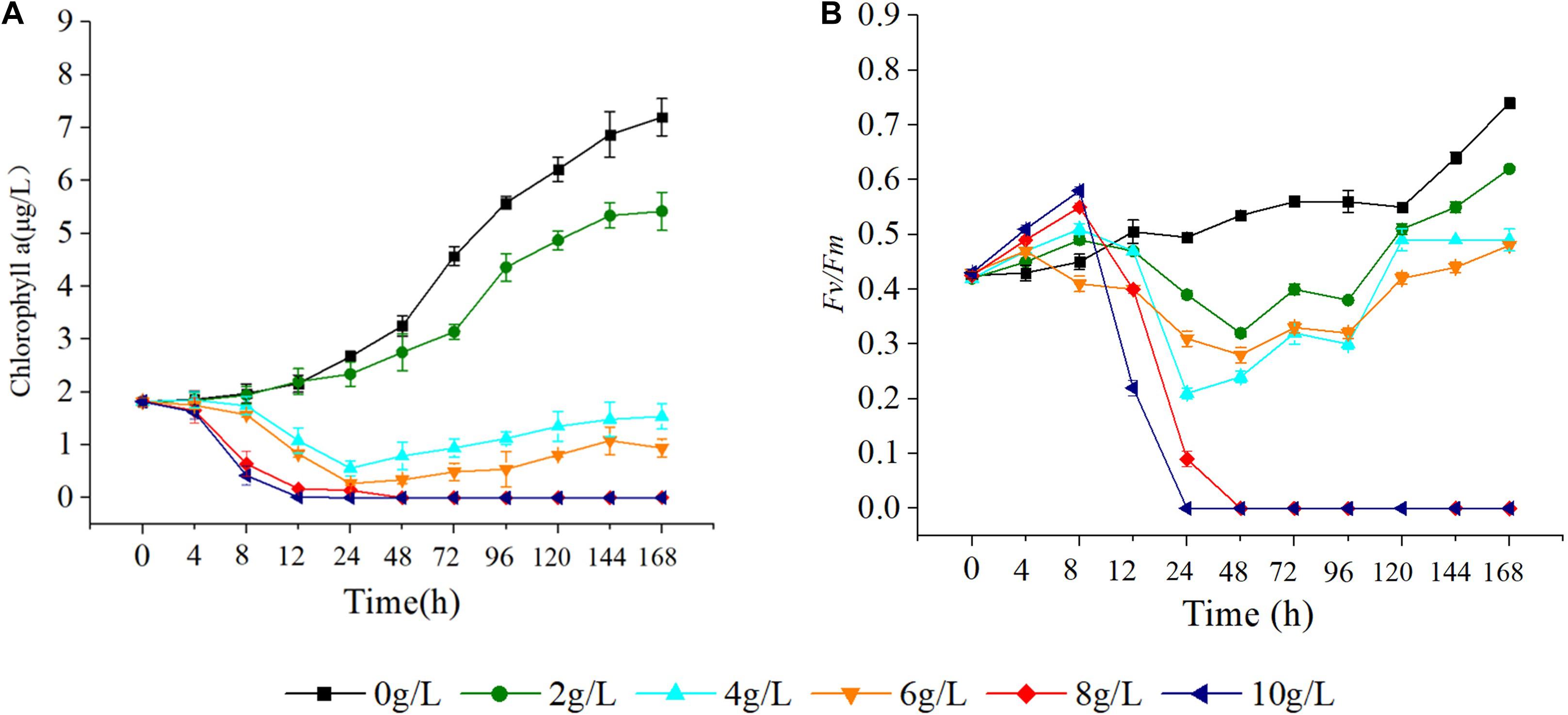
Figure 7. Effect of hijiki extract on chlorophyll a content (A) and maximum quantum yield (B) of Skeletonema costatum.
Effect of Hijiki Extracts on Enzyme Activity
Evaluation of the effects of 4 and 6 g/L extracts on the activity of oxidoreductase of S. costatum revealed significant effects on SOD, MDA, GSH-Px, GST, and GR relative to the control. Specifically, the activities of SOD, GR, and GSH-Px all increased, then decreased in response to extract treatment. Moreover, the S. costatum culture with 4 g/L extracts showed an increase in SOD activity within 12 h, but this difference was not significant. The activity of SOD was significantly reduced at 24 h (p < 0.05), then dropped to the lowest value at 48 h, which was only 33.3% of the control. At 168 h, the activity of SOD increased to about 50% of the control (Figure 8A). The hijiki extracts caused an initial increase in the activity of GR of S. costatum. At 4 h, the activity of GR in cultures treated with 4 and 6 g/L extracts was significantly higher than that of the control (p < 0.05), after which it decreased. At 12 h, the activity of GR in samples treated with 6 g/L extracts was significantly lower than that of the control (p < 0.05). At 24 h, the activity of GR in cultures treated with 4 g/L extracts was significantly lower than that of the control (p < 0.05) (Figure 8B). Cultures treated with 4 and 6 g/L extracts showed significant increases in the activity of GSH-Px at 4 and 8 h compared with that of the control (p < 0.05), while they decreased to the same level of the control (p < 0.05) at 12 h, and were significantly lower than that of the control at 24 h (p < 0.05) (Figure 8C). The contents of MDA and GST were lower than that of the control in cultures treated with hijiki extracts. At 4 h, the contents of MDA in the cultures treated with 4 and 6 g/L extracts were significantly lower than that of the control (p < 0.05) (Figure 8D). The activity of GST in the culture treated with 4 and 6 g/L extracts was also significantly lower than that of the control (p < 0.05) (Figure 8E).
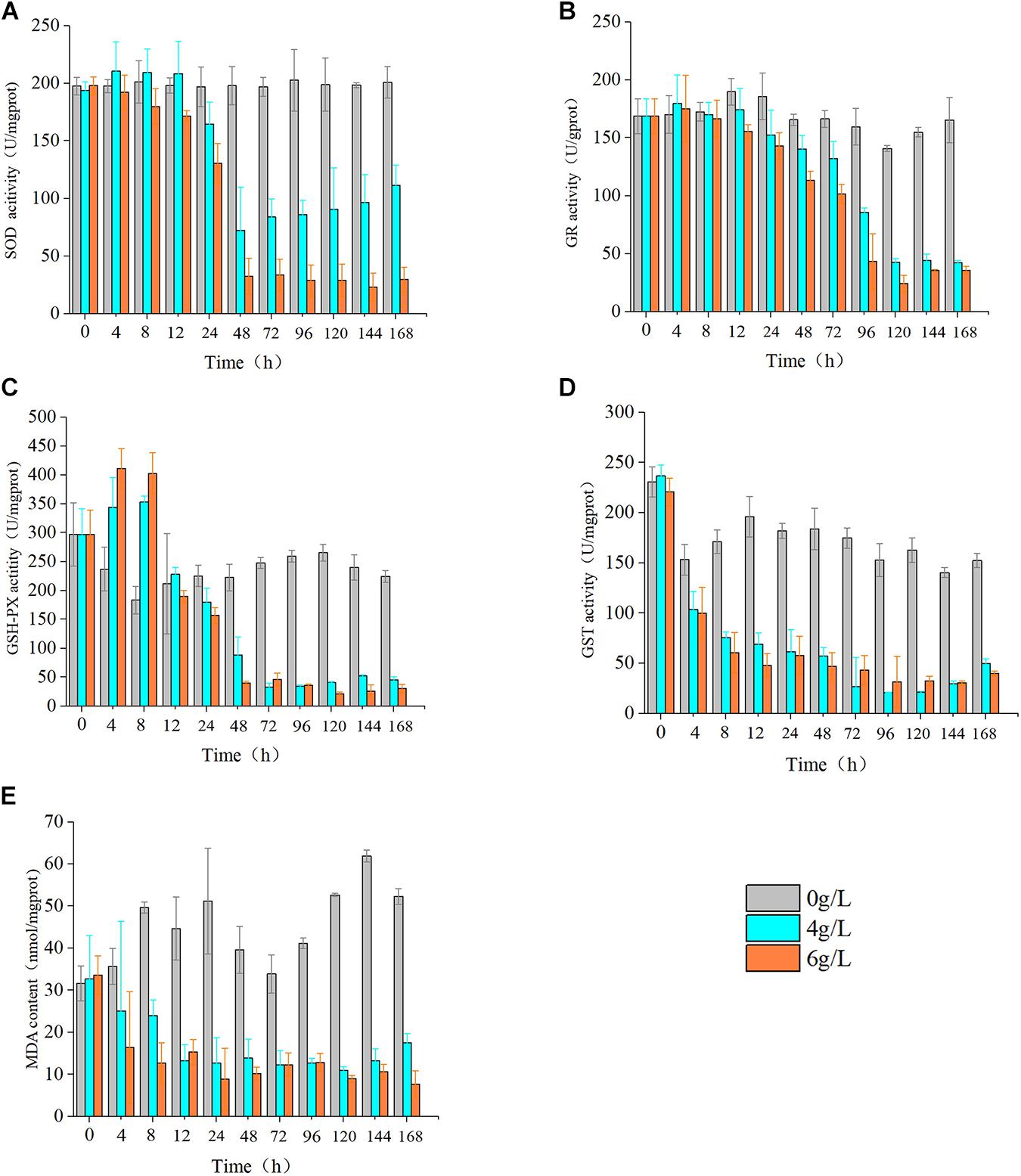
Figure 8. Effect of hijiki extract on the activity of enzymes. (A) SOD, (B) GR, (C) GSH, (D) GST, and non-enzymatic antioxidants (E) MDA.
Discussion
Effect of Hijiki Extracts on the Growth of Red Tide Miroalgae
The hijiki extracts used in the experiment were autoclaved to eliminate the influence of microbes on the growth of microalgae. The IC50 of the extracts on growth of the red tide microalgae with both normal and red tide density showed an order of P. donghaiensis > S. costatum > H. akashiwo, indicating that the inhibitory effects of the extracts on the red tide microalgae varied with species and growing densities of the microalgae. Hijiki extracts not only inhibited the growth and proliferation of the microalgae, but also their morphology and dispersion.
Effect of Hijiki Extracts on Chla Content and Fv/Fm of S. costatum
In this study, hijiki extracts had a significant effect on the Chla content and Fv/Fm of S. costatum. Although the addition of hijiki extracts reduced the pH value of algae cultures, they were all within the pH range suitable for microalgae growth (Hansen, 2002). Therefore, the inhibitory substances in the extracts are presumably secondary metabolites that have allelopathic effects. The allelochemicals reduced Chla content and damaged the photosynthetic system II (PSII), which prevented the plant from obtaining energy through photosynthesis, ultimately leading to reduced cell proliferation rate and inhibited growth (Gross, 2003). Moreover, the effects of allelochemicals on the photosynthesis of terrestrial plants, freshwater algae, and seaweeds were reflected by reduced Chla contents and Fv/Fm values (Körner and Nicklisch, 2002; Sukenik et al., 2002; Şliwińska-Wilczewska et al., 2017; Xu et al., 2019; Zhao et al., 2019). Eucalyptus leaf extracts significantly reduced the Fv/Fm of Microcystis aeruginosa on the third day of treatment (Zhao et al., 2019). Also, allelochemicals of picocyanobacterium Synechococcus sp. significantly reduced the Chla and Fv/Fm of Phormidium sp. in 3 days (Şliwińska-Wilczewska et al., 2017). Additionally, exudates of Myriophyllum spicatum were able to inhibit the PSII of cyanobacteria, green algae, and diatoms (Körner and Nicklisch, 2002). Moreover, myristic acid (C14H28O2), acrylic acid (C3H4O2), and linolenic acid (C18H302) isolated from Enteromorpha prolifera caused a reduction of Chla content in S. costatum and H. akashiwo (An et al., 2008). In a recent study, Cylcellrotheca closterium extracts reduced the Chla content and Fv/Fm of P. donghaiensis (Xu et al., 2019).
Chlorophyll a is essential for photosynthesis of algal cells. Results from the present study showed that hijiki extracts seriously damaged the photosynthetic system, caused a reduction of Chla content, and inhibited the growth of S. costatum, indicating that the allelochemicals of hijiki could directly affect the photosynthetic system.
As a natural probe for photosynthetic energy conversion, Fv/Fm contains rich information about photosynthesis and is widely used to analyze the effects of stress on plant photosynthesis (Baker, 2004). Fv/Fm denotes the maximum quantum yield of PSII and can be used to indicate the integrity of PSII. When the value of Fv/Fm is greater than 0.44, PSII activity decreases with decreasing values of Fv/Fm. If the Fv/Fm value is less than 0.44, the reaction center is inactivated or destroyed (Schansker and Jack, 1999). In our study, cultures grown with 8 g/L extracts for 24 h or 10 g/L extracts for 48 h caused the Fv/Fm of S. costatum to decrease to 0 (Figure 4). This implied that PSII was damaged, which prevented photosynthesis from proceeding, thereby inhibiting the growth and proliferation of cells or causing the cells to die. The Fv/Fm of the alga cultured with 4, 8, and 10 g/L extracts showed a trend that was initially decreasing and then increasing, which implied that PSII might have a negative feedback regulation that repaired and restored the photosynthetic system. Therefore, it would be useful to determine the effectiveness and effective duration of red tide controlling measures by using a chlorophyll fluorometer to quickly measure the Chla content and Fv/Fm value of algae.
Effect of Hijiki Extracts on Oxidoreductase Activity
During culture with hijiki extracts, the contents of SOD, GR, and GSH-Px in microalgae all showed a trend of initial increasing and then decreasing, which indicated that the stress exerted by low concentrations of extracts increased ROS level in the algal cells, while the antioxidant enzymes were unable to eliminate the excessive oxidation products that in turn damaged the enzyme system. The content of GST and MDA decreased throughout the culture, indicating that the algal cells were damaged to a great degree. Therefore, the hijiki extracts had the dual characteristics of enhancing and inhibiting the activity of enzymes. Hijiki extracts damaged the activity of oxidoreductase in the cells of S. costatum to varying degrees, which led to a failed removal of ROS over time. The accumulated ROS led to lipid peroxidation, which might have destroyed the cell structure and caused damage or death of algal cells. The performance of oxidoreductase in our study was basically consistent with that reported in terrestrial plants under stress conditions (Gill and Tuteja, 2010). Under abiotic stresses such as salt, drought, water, light, and heavy metal stresses, SOD, GSH-PX, GST, and GR activity of many terrestrial plants increases significantly, and MDA content significantly decreases, to effectively reduce oxidative stress and lipid peroxidation damage (Gill and Tuteja, 2010; Xu et al., 2013; Yousefzadeh-Najafabadi and Ehsanzadeh, 2017; Cengiz et al., 2019).
Conclusion
Large-scale cultivation of seaweed can provide potential solutions to global marine environmental problems such as ocean acidification, hypoxia, eutrophication, and harmful algal blooms (Yang et al., 2021). This study was conducted under controlled conditions in the laboratory, and the effects of environmental factors such as temperature and light on the experimental results were excluded. The effects of hijiki extracts on the growth, Chla content, maximum quantum yield, activity of antioxidant enzymes (SOD, GSH-Px, GST, and GR), and content of MDA of red tide microalgae were analyzed. The results showed that the extracts of hijiki dry powder contains substances that have inhibitory effects on the three species of microalgae, and the inhibitory effect increases with the concentration of the extracts. The inhibitory substances could directly affect the photosynthetic system and oxidoreductase system of S. costatum, inhibiting the growth of the red tide microalgae. Overall, this research shows a new prospect for the utilization of hijiki on the red tide control, and marine environmental protection.
Data Availability Statement
The raw data supporting the conclusions of this article will be made available by the authors, without undue reservation, to any qualified researcher.
Author Contributions
YZ and NX designed the research and wrote the manuscript. YZ performed the research. All authors read and approved the final version of the manuscript.
Funding
This work was supported by the Natural Science Foundation of Zhejiang Province (LGF19D060002 and LZ17D060001) and the National Key R&D Program of China (2018YFD0900702).
Conflict of Interest
The authors declare that the research was conducted in the absence of any commercial or financial relationships that could be construed as a potential conflict of interest.
References
Ahn, O., Petrell, R. J., and Harrison, P. J. (1998). Ammonium and nitrate uptake by Laminaria saccharina and Nereocystis leuetkeana originating from a salmon seacage farm. J. Appl. Phycol. 10, 333–340. doi: 10.1023/A:1008092521651
An, Z., Wang, Z. Y., Li, F. M., Tian, Z. J., and Hu, H. Y. (2008). Allelopathic inhibition on red tide microalgae Skeletonema costatum by five macroalgal extracts. Front. Env. Sci. Eng. 2:297–305. doi: 10.1007/s11783-008-0055-3
Baker, R. (2004). Applications of chlorophyll fluorescence can improve crop production strategies: an examination of future possibilities. J. Exp. Bot. 55, 1607–1621. doi: 10.1093/jxb/erz535
Berdalet, E., Kudela, R., Urban, E., Enevoldsen, H., Banas, N. S., Bresnan, E., et al. (2017). Global HAB: a new program to promote international research, observations, and modeling of harmful algal blooms in aquatic systems. Oceanography 30, 70–81. doi: 10.5670/oceanog.2017.111
Cengiz, K., Nudrat, A. A., Abdulkadir, S., and Muhammad, A. (2019). Alleviating effect of nitric oxide on oxidative stress and antioxidant defence system in pepper (Capsicum annuum L.) plants exposed to cadmium and lead toxicity applied separately or in combination. Sci. Hortic. 255, 52–60. doi: 10.1016/j.scienta.2019.05.029
Daguer, H., Hoff, R. B., Molognoni, L., Kleemann, C. R., and Felizardo, L. V. (2018). Outbreaks, toxicology, and analytical methods of marine toxins in seafood. Curr. Opin. Food Sci. 24, 43–55. doi: 10.1016/j.cofs.2018.10.006
Davide, I., Alicia, G., Ismael, M., José, M., and Rossella, P. (2016). Outdoor production of Tisochrysis lutea in pilot-scale tubular photobioreactors. J. Appl. Phycol. 28, 3159–3166. doi: 10.1007/s10811-016-0856-x
Della, G., Antonio, F., and Isidori, M. (2000). Antialgal ent-labdane diterpenes from Ruppia maritime. Phytochemistry 55, 909–913. doi: 10.1016/S0031-9422(00)00253-3
Gill, S. S., and Tuteja, N. (2010). Reactive oxygen species and antioxidant machinery in abiotic stress tolerance in crop plant. Plant Physiol. Bioch. 48, 909–930. doi: 10.1016/j.plaphy.2010.08.016
Gross, E. M. (2003). Allelopathy of aquatic autotrophs. Crit. Rev. Plant Sci. 22, 3–4. doi: 10.1080/713610859
Guan, W. C., and Li, L. (2017). Atomic ratio of N to P influences the impact of UV irradiance on photosynthesis and growth in a marine dinoflagellate, Alexandriu tamarense. Photosynthetica 55, 501–509. doi: 10.1007/s11099-016-0670-3
Han, X. R., Gao, S., Hou, J. N., Li, H. M., and Shi, X. Y. (2013). Allelopathic effects of extracts from Ulva prolifera powders on the growth of Prorocentrum donghaiense and Skeletonema costatum. Acta Ecol. Sin. 33, 7417–7429. doi: 10.1351/PAC-REP-10-07-02
Hansen, P. J. (2002). Effect of high pH on the growth and survival of marine phytoplankton: implications for species succession. Aquat. Microbial. Ecol. 28, 279–288.
Hejl, A. M., and Koster, K. L. (2004). Juglone disrupts root plasma membrane H+-ATPase activity and impairs water uptake, root respiration, and growth in soybean (Glycine max) and corn (Zea mays). J. Chem. Ecol. 30, 453–471. doi: 10.1023/B:JOEC.0000017988.20530.d5
Hu, P., Li, Z. X., Chen, M. C., Sun, Z. L., Ling, Y., Jiang, J., et al. (2016). Structural elucidation and protective role of a polysaccharide from Sargassum fusiforme on ameliorating learning and memorydeficiencies in mice. Carbohyd. Polym. 139, 150–158. doi: 10.1016/j.carbpol.2015.12.019
Jeffrey, S. W., and Humphrey, G. F. (1975). New spectrophotometric equations for determining chlorophylls a, b, c1 and c2 in higher plants, algae and natural phytoplankton. Biochem. Physiol. Pflanz. 167, 191–194. doi: 10.1016/S0015-3796(17)30778-3
Jeong, J. H., Jin, H. J., Sohn, C. H., Suh, K. H., and Hong, Y. K. (2000). Algicidal activity of the seaweed Corallina pilulifera against red tide. J. Appl. Phycol. 12, 37–43. doi: 10.1023/A:1008139129057
Jiang, B., Li, H., Teng, G., Yan, J., Li, B. X., Li, J. B., et al. (2017). Using GOCI extracting information of red tide for time-series analysing in East China Sea. J. Zhejiang Univ. SC A 44, 576–583. doi: 10.3785/j.issn.1008-9497.2017.05.013
Körner, S., and Nicklisch, A. (2002). Allelopathic growth inhibition of selected phytoplankton species by submerged macrophytes. J. Phycol. 32, 862–871. doi: 10.1046/j.1529-8817.2002.t01-1-02001.x
León-Muñoz, J., Urbina, M. A., Garreaud, R., and Iriarte, J. L. (2018). Hydroclimatic conditions trigger record harmful algal bloom in western Patagonia (summer 2016). Sci. Rep. 8:1330. doi: 10.1038/s41598-018-19461-4
Liu, H. M., Xie, H. M., and Gong, Y. M. (2011). Secondary metabolites from the seaweed Gracilaria lemaneiformis and their allelopathic effects on Skeletonema costatum. Biochem. Syst. Ecol. 39, 397–400.
Liu, L., Zhou, J., Zheng, B. H., Cai, W. Q., Lin, K. X., and Tang, J. L. (2013). Temporal and spatial distribution of red tide outbreaks in the Yangtze River Estuary and adjacent waters. China. Mar. Pollut. Bull. 72, 213–221. doi: 10.1016/j.marpolbul.2013.04.002
Lu, H. M., Xie, H. H., Gong, Y. X., Wang, Q., and Yang, Y. F. (2011). Secondary metabolites from the seaweed Gracilaria lemaneiformis and their allelopathic effects on Skeletonema costatum. Biochem. Sys. Eco. 39, 397–400. doi: 10.1016/j.bse.2011.05.015
Ma, Z., Wu, M., and Lin, L. (2017). Allelopathic interactions between the macroalga Hizikia fusiformis(Harvey) and the harmful blooms-forming dinoflagellate Karenia mikimotoi. Harmful Algae 65, 19–26. doi: 10.1016/j.hal.2017.04.003
Mascareño, A., Cordero, R., Azócar, G., Billi, M., Henríquez, P. A., and Ruz, G. A. (2018). Controversies in social-ecological systems: lessons from a major red tide crisis on Chiloe Island, Chile. Ecol. Soc. 23:15. doi: 10.5751/ES-10300-230415
McCabe, R. M., Barbara, M., Hickey, Raphae, M., Kudela Kathi, A., et al. (2016). An unprecedented coastwide toxic algal bloom linked to anomalous ocean conditions. Geophys. Res. Lett. 43, 10366–10376. doi: 10.1002/2016GL070023
Nakai, S., Inoue, Y., Hosomi, M., and Murakami, A. (1999). Growth inhibition of blue-green algae by allelopathic effects of macrophytes. Water Sci. Technol 39, 47–53. doi: 10.1016/S0273-1223(99)00185-7
Schansker, G., and Jack, J. S. (1999). Performance of active photosystemII centers in photoinhibited pea leaves. Photosynthesis Res. 62, 175–184. doi: 10.1023/A:1006374707722
Şliwińska-Wilczewska, S., Maculewicz, J., Felpeto, A. B., Vasconcelos, V., and Latala, A. (2017). Allelopathic activity of picocyanobacterium Synechococcus sp. on filamentous cyanobacteria. J. Exp. Ma. Bio. Ecol 496, 16–21. doi: 10.1016/j.jembe.2017.07.008
Soto, I. M., Cambazoglu, M. K., and Boyette, A. D. (2018). Advection of Karenia brevis blooms from the Florida panhandle towards Mississippi coastal waters. Harmful Algae 72, 46–64. doi: 10.1016/j.hal.2017.12.008
Sukenik, A., Eskhol, R., Livne, A., Ora, H., Meir, M., Dani, T., et al. (2002). Inhibition of growth and photosynthesis of the dinoflagellate Peridinium gatunense by Microcystis sp. (cyanobacteria): a novel allelopathic mechanism. Limnol. Oceanogr. 47, 1656–1663. doi: 10.4319/lo.2002.47.6.1656
Sun, Y., Chen, X., Zhang, L., Liu, H., and Li, P. C. (2019). The antiviral property of Sargassum fusiforme polysaccharide for avian leukosis virus subgroup in vitro and in vivo. Int. J. Biol. Macromol. 138, 70–78. doi: 10.1016/j.ijbiomac.2019.07.073
Tang, Y., and Gobler, C. (2011). The green macroalga, Ulva lactuca, inhibits the growth of seven common harmful algal bloom species via allelopathy. Harmful Algae 10, 480–488. doi: 10.1016/j.hal.2011.03.003
Van Dolah, F. M. (2000). Marine algal toxins: origins, health effects, and their increased occurrence. Environ. Health Persp. 108 Suppl, 133–141. doi: 10.2307/3454638
Xu, R., Yamada, M., and Fujiyama, H. (2013). Lipid peroxidation and antioxidative enzymes of two turfgrass species under salinity stress. Pedosphere 23, 213–222. doi: 10.1016/S1002-0160(13)60009-0
Xu, W. J., Wang, J. T., Tan, L. J., Guo, X., and Xue, Q. N. (2019). Variation in allelopathy of extracellular compounds produced by Cylindrotheca closterium against the harmful-algal-bloom dinoflagellate Prorocentrum donghaiense. Mar. Environ. Res. 148, 19–25. doi: 10.1016/j.marenvres.2019.05.005
Yang, Y., Luo, H., Wang, Q., He, Z., and Long, A. (2021). Large-scale cultivation of seaweed is effective approach to increase marine carbon sequestration and solve coastal environmental problems. Chin. Acad. Sci. 36, 1–11. doi: 10.16418/j.issn.1000-3045.20210217103
Yang, Y. F., Liu, Q., Chai, Z. Y., and Tang, Y. Z. (2015). Inhibition of marine coastal bloom-forming phytoplankton by commercially cultivated Gracilaria lemaneiformis (Rhodophyta). J. Appl. Phycol. 27, 2341–2352. doi: 10.1007/s10811-014-0486-0
Yousefzadeh-Najafabadi, M., and Ehsanzadeh, P. (2017). Photosynthetic and antioxidative upregulation in drought-stressed sesame (Sesamum indicum L.) subjected to foliar applied salicylic acid. Photosynthetica 4, 611–622. doi: 10.1007/s11099-017-0673-8
Yu, Z. M., and Chen, N. S. (2019). Emerging trends in red tide and major research progresses. Ocean. Limnol. Sin. 50, 474–486. doi: 10.11693/hyhz20190200041
Zhang, R., Zhang, X. X., Tang, Y. X., and Mao, J. L. (2020). Composition, isolation, purification and biological activities of Sargassum fusiforme polysaccharides: A review. Carbohyd. Polym. 228:115381. doi: 10.1016/j.carbpol.2019.115381
Keywords: Sargassum fusiforme, red tide microalgae, Chla, Fv/Fm, oxidoreductase
Citation: Zhang Y, Xu N and Li Y (2021) Effect of the Extracts of Sargassum fusiforme on Red Tide Microalgae in East China Sea. Front. Mar. Sci. 8:628095. doi: 10.3389/fmars.2021.628095
Received: 11 November 2020; Accepted: 15 June 2021;
Published: 22 July 2021.
Edited by:
Keith Davidson, Scottish Association For Marine Science, United KingdomReviewed by:
Nor Azman Kasan, University of Malaysia Terengganu, MalaysiaEileen Bresnan, Marine Scotland, United Kingdom
Copyright © 2021 Zhang, Xu and Li. This is an open-access article distributed under the terms of the Creative Commons Attribution License (CC BY). The use, distribution or reproduction in other forums is permitted, provided the original author(s) and the copyright owner(s) are credited and that the original publication in this journal is cited, in accordance with accepted academic practice. No use, distribution or reproduction is permitted which does not comply with these terms.
*Correspondence: Nianjun Xu, eHVuaWFuanVuQG5idS5lZHUuY24=