Corrigendum: Abundance and Potential Biological Removal of Common Dolphins Subject to Fishery-Impacts in South Australian Waters
- 1Cetacean Ecology, Behaviour and Evolution Laboratory (CEBEL), College of Science and Engineering, Flinders University, Adelaide, SA, Australia
- 2Molecular Ecology Laboratory, College of Science and Engineering, Flinders University, Adelaide, SA, Australia
- 3Department of Biological Sciences, Macquarie University, Sydney, NSW, Australia
- 4Cetacean Ecology Research Group, School of Natural Sciences, Massey University, Auckland, New Zealand
- 5Evolutionary Genetics Group, Department of Anthropology, University of Zurich, Zurich, Switzerland
- 6Global Ecology Partuyarta Ngadluku Wardli Kuu, College of Science and Engineering, Flinders University, Adelaide, SA, Australia
Conservation management of wildlife species should be underpinned by knowledge of their distribution and abundance, as well as impacts of human activities on their populations and habitats. Common dolphins (Delphinus delphis) are subject to incidental capture in a range of Australia’s commercial fisheries including gill netting, purse seining and mid-water trawling. The impact these fishery interactions have on common dolphin populations is uncertain, as estimates of abundance are lacking, particularly for the segments of the populations at risk of bycatch and in greater need of protection. Here we used double-observer platform aerial surveys and mark-recapture distance sampling methods to estimate the abundance of common dolphins in 2011 over an area of 42,438 km2 in central South Australia, where incidental mortality of common dolphins due to fisheries bycatch is the highest. We also used the potential biological removal (PBR) method to estimate sustainable levels of human-caused mortality for this segment of the population. The estimated abundance of common dolphins was 21,733 (CV = 0.25; 95% CI = 13,809–34,203) in austral summer/autumn and 26,504 in winter/spring (CV = 0.19; 95% CI = 19,488–36,046). Annual PBR estimates, assuming a conservative maximum population growth rate of Rmax = 0.02 and a recovery factor of Fr = 0.5 for species of unknown conservation status, ranged from 95 (summer/autumn) to 120 dolphins (winter/spring), and from 189 (summer/autumn) to 239 dolphins (winter/spring) with an Rmax = 0.04. Our results indicate that common dolphins are an abundant dolphin species in waters over the central South Australian continental shelf (up to 100 m deep). Based on the 2011 abundance estimates of this species, the highest estimated bycatch of common dolphins (423 mortalities in 2004/05) in the southern Australian region exceeded the precautionary PBR estimates for this population segment. Recent bycatch levels appear to be below PBR estimates, but low observer coverage and underreporting of dolphin mortalities by fishers means that estimates of dolphin bycatch rates are not robust. The effects of cumulative human impacts on common dolphins are not well understood, and thus we recommend a precautionary management approach to manage common dolphin bycatch based on local abundance estimates.
Introduction
Understanding the impacts of fisheries bycatch on marine megafauna species is one of the biggest challenges facing their conservation and management. Cetacean mortality in fishing gear is a global conservation issue, particularly for small odontocetes (Read et al., 2006; Reeves et al., 2013; Brownell et al., 2019). Unsustainable bycatch in local fisheries caused the extinction of the Yangtze River dolphin or baiji (Lipotes vexillifer) (Turvey et al., 2007), and is rapidly driving the vaquita (Phocoena sinus), one of the most endangered mammals in the world, toward the same fate (Taylor et al., 2017; Jaramillo-Legorreta et al., 2019). The development of effective conservation and management measures to address impacts of bycatch relies on our ability to identify affected population(s), monitor population trends, and determine sustainable levels of incidental mortality (Harwood, 1999; Milner-Gulland and Akçakaya, 2001).
Evaluating the sustainability of marine mammal bycatch is difficult as robust data on population size and cumulative bycatch are often unavailable. Impacts of bycatch mortality in fisheries must be addressed with respect to the population or stock (i.e., a demographically independent biological population) being affected and its size. However, obtaining robust and unbiased estimates of abundance of marine mammals remains challenging due to the large geographic expanses that often characterize their distribution and the many factors that introduce detection bias (Marsh and Sinclair, 1989; Taylor et al., 2007; Buckland et al., 2015). Furthermore, bycatch of marine mammals is not evenly distributed across a population or species geographical range but concentrated in particular hotspots (Lewison et al., 2014; Tulloch et al., 2020). Such concentrated mortality can lead to the fragmentation of populations, reduction in annual survival and population growth rates, genetic diversity and gene flow among fragments; local extinctions of detected or undetected populations; and cascading ecological changes on the structure and function of marine ecosystems (Pichler and Baker, 2000; Lewison et al., 2004; Heppell et al., 2005). Therefore, careful consideration needs to be given to the estimation of the levels of impact that the populations can sustain in regard to: (i) the spatial scale at which human-caused mortality occurs, (ii) the area used to estimate abundance in relation to the population’s range, and (iii) the potential limitations of the method depending on the biological scenario at hand. As a precautionary approach, it has been recommended that in the absence of wide scale abundance estimates for a marine mammal population or stock, and where human-caused mortality is concentrated over a portion of a stock’s range, calculations of sustainable levels of human-caused mortality be allocated to the geographic region occupied by that stock, until a range-wide abundance estimate for the stock is possible (NMFS, 2005; Moore and Merrick, 2011). Such an approach has been used to evaluate sustainable levels of human-caused mortality on a wide diversity of marine mammals (e.g., López et al., 2003; Marsh et al., 2004; Slooten and Dawson, 2008; Cagnazzi et al., 2013; Parra and Cagnazzi, 2016).
In Australian waters, dolphins are susceptible to bycatch from a range of commercial fisheries including gill netting, purse seining and mid-water trawling (Shaughnessy et al., 2003; Tulloch et al., 2020). Among the species most heavily impacted are bottlenose dolphins (Tursiops spp.) off Western Australia (Allen et al., 2014), spinner dolphins (Stenella longirostris) off northern Australia (Harwood and Hembree, 1987), and common dolphins (Delphinus delphis) off southern Australia (Hamer et al., 2008). Although there is considerable concern about the effect that fisheries interactions may have on the respective populations of these species, very little is known about their distribution, demography, and cumulative incidental mortality across fisheries and jurisdictions to assess population consequences of bycatch mortality.
All cetaceans in Australian waters are protected under the Australian Environment Protection and Biodiversity Conservation Act 1999 (EPBC Act), and commercial fisheries must report interactions that result in their death or injury. Commercial fisheries operating within the Australian Fishing Zone (extending from 3 to 200 nautical miles off the coast) are managed by the Commonwealth government’s Australian Fisheries Management Authority (AFMA). State and territory governments have the responsibility of administering Australia’s fisheries within three nautical miles from the coastline. Australia’s Commonwealth, state and territory governments receive advice on the assessment and management of bycatch by advisory committees including a wide variety of stakeholder groups (industry, policy, conservation, state and territory governments, recreational and research).
Incidental bycatch of common dolphins in southern Australia is known to occur in the South Australian Sardine Fishery (SASF) (Hamer et al., 2008), the Gillnet Hook and Trap (GHAT) sector of the Southern and Eastern Scalefish and Shark Fishery (SESSF) (AFMA, 2011), and the mid-water trawl sector of the Small Pelagic Fishery (SPF) (DEH, 2006). Among these fisheries, the SASF, which operates mainly in state waters adjacent to the state of South Australia, has recorded the highest number of common dolphin mortalities (Table 1). An initial fisheries observer program of the SASF in 2004/05 revealed an estimated 1728 common dolphins encircled and 377 dolphin mortalities across the fishing fleet over a 7-month period (Hamer et al., 2008). Further extrapolation from observer data covering 3.9% of the total net-sets in 2004/05 financial year, indicated that a total of 449 net-set events resulted in common dolphin encirclements and 423 dolphin mortalities occurred in the fishery during this time (Table 1; Ward et al., 2018). As a result, a Code of Practice (CoP) for mitigating interactions with common dolphins was developed by the South Australia Sardine Industry Association in collaboration with the South Australian Research and Development Institute (Hamer et al., 2008; Ward et al., 2018). The CoP involves avoidance and delay procedures designed to reduce dolphin encirclements and release procedures to minimize dolphin mortalities. Extrapolation from observer data (3.9 to 25.2% net sets observed per annum) collected between 2004/05 and 2014/15 suggested that since the implementation of the CoP, total dolphin encirclements and mortality have been reduced by 87 and 97%, respectively (Ward et al., 2018). However, in 2018/19 the estimated common dolphin mortality increased to 113, the highest reported since 2004/05 (Table 1; Goldsworthy et al., 2019). Since its inception, concerns about the long-term efficacy of the CoP, including low observer coverage and undereporting of common dolphin mortalities by fishers (Bilgmann et al., 2009), have led to continued independent monitoring of the SASF through fishery logbooks and an observer program to estimate the magnitude of ongoing interactions (Ward et al., 2018).
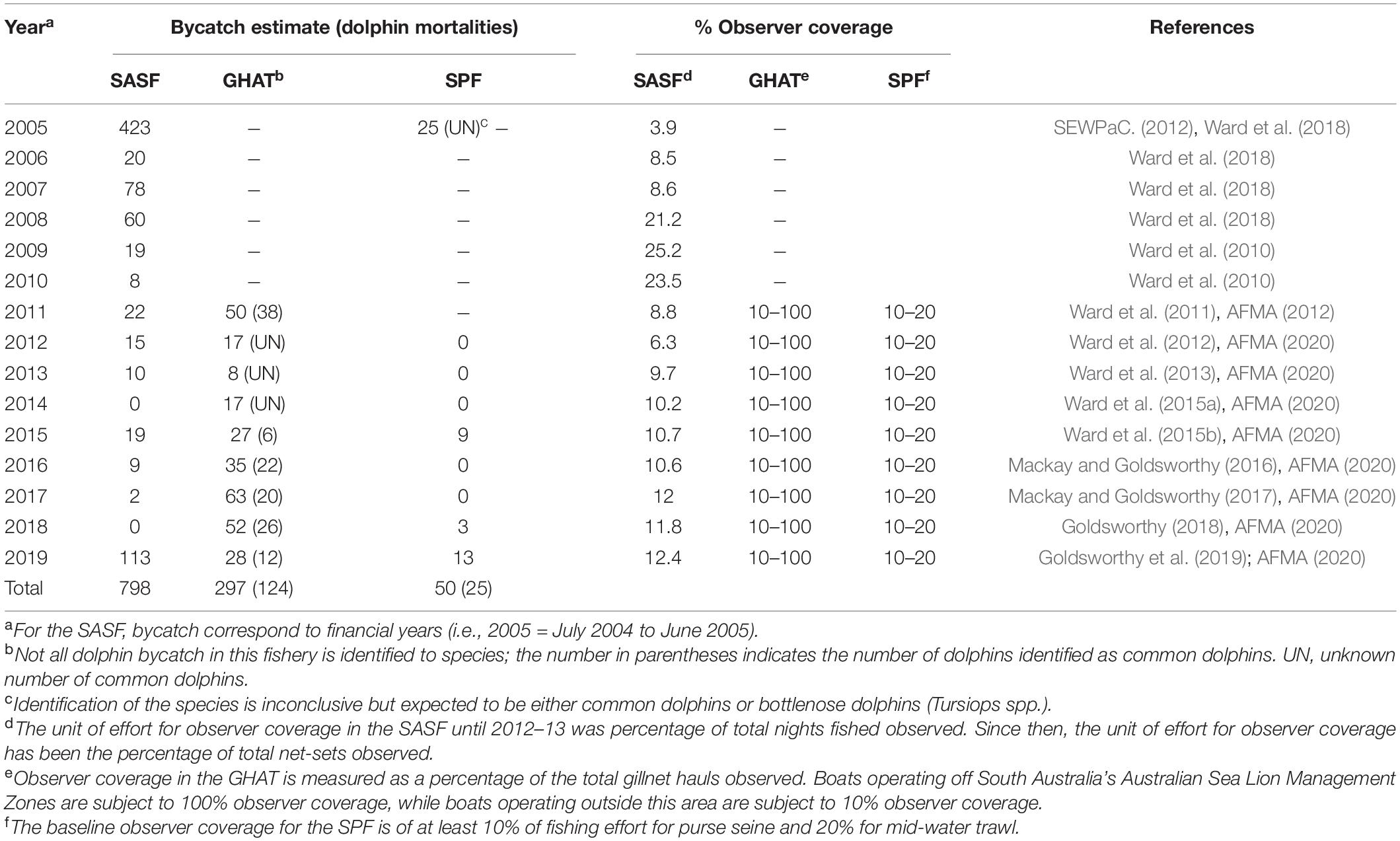
Table 1. Annual bycatch estimates of common dolphins (Delphinus delphis) from observer programs in the South Australian Sardine Fishery (SASF), the Gillnet Hook and Trap (GHAT) sector of the Southern and Eastern Scalefish and Shark Fishery and the mid-water trawl sector of the Small Pelagic Fishery (SPF) off southern Australia.
The GHAT fishery operates in Commonwealth waters from the New South Wales/Victorian border westward to the South Australian/Western Australian border, including the waters around Tasmania. Although this gillnet fishery has been operating since the 1970s, high bycatch rates of dolphins in the GHAT only became known to AFMA in 2010 (AFMA, 2011). A total of 52 dolphin interactions were reported between September 2010 and September 2011, with 50 leading to mortalities, of which 38 were common dolphins (AFMA, 2012). The interactions mainly occurred south of the Coorong off South Australia in the area from Kangaroo Island to Cape Jaffa (the Coorong Zone). This region was then temporarily closed to gillnet fishing by AFMA to mitigate interactions, with gillnet fishing in adjacent waters requiring 100% monitoring by observers or electronic monitoring systems (AFMA, 2013). AFMA subsequently implemented its Gillnet Dolphin Mitigation Strategy, which permitted fishing with gillnets in the Coorong Zone when operating in accordance with a suite of new measures. These measures included monitoring and reporting of dolphin interactions, implementation of a dolphin mitigation plan, and adherence to performance criteria and escalating management responses to ensure fishing operations do not continue unchecked if dolphin bycatch occurred (AFMA, 2014). As a result, dolphin interactions in the fishery decreased to <30 (range: 9–29) between 2012 and 2015, but increased to 35–67 (with at least 12–22 identified as common dolphins) between 2016 and 2019 (Table 1; AFMA, 2020). AFMA introduced the second stage of its mitigation strategy in 2017 (updated in 2019) which applies to all gillnet fishing in the SESSF and established the South Australian Dolphin Zone (i.e., recognizing all South Australian waters as high risk for dolphin interactions) (AFMA, 2019a). Electronic monitoring continued to be mandatory with temporary closures for fishers with six or more dolphin interactions in a review period, rather than closing zones for the entire gillnet fishery (AFMA, 2019a).
The SPF fishery operates typically in Commonwealth waters and extends from the Queensland/New South Wales border, around southern Australia, to north of Perth in Western Australia. Dolphin interactions in mid-water trawling of the SPF were first noted in 2004/05, when 25 dolphin mortalities (unidentified species, likely common dolphins, D. delphis, or common bottlenose dolphins, Tursiops truncatus) occurred off Tasmania in the western zone of the fishery (Table 1; SEWPaC., 2012). In response, AFMA introduced a mitigation framework including a Dolphin Mitigation Strategy, Bycatch and Discard Work Plan, bycatch reduction devices, electronic monitoring across all trawl vessels, mandatory Vessel Management Plans, and temporary closures for individual fishers with six or more dolphin interactions in a review period (DEE, 2018; AFMA, 2019b). From 2005 to 2014 no dolphin interactions were recorded, but a total of 25 common dolphin mortalities were reported between 2015 and 2019 (Table 1; AFMA, 2020).
While acknowledging the efforts made by the fishing industry in conjunction with the fisheries management authorities to reduce mortalities of common dolphins caused by fishing activities, these fisheries continue to have interactions, and the population-level impacts of bycatch on common dolphins are unknown due to the lack of information on dolphin demographic data in the region. In all, the few studies conducted on common dolphins in South Australia emphasize the urgent need for population demographic studies toward the assessment, reduction and mitigation of fisheries interactions (Kemper and Gibbs, 2001; Bilgmann et al., 2008, 2014; Hamer et al., 2008; Mackay et al., 2016). In the absence of abundance estimates, it is difficult to assess the potential demographic impacts of bycatch on local dolphin population(s) and to determine sustainable levels of bycatch.
Genetic studies of common dolphins have found that Australasian common dolphins exhibit complex patterns of hierarchical metapopulation structure with nested subpopulations within regional populations (Bilgmann et al., 2014; Barceló et al., 2021). The most recent study using a powerful genome-wide dataset identified three highly distinct and genetically diverse regional populations of common dolphins with low migration rates (2–9%) across Australasia, represented by the southern coast of Australia, the east coast of Australia, and Tasmania and New Zealand combined (Barceló et al., 2021). Within the southern coast of Australia regional population, two to four local subpopulations were identified with moderate migration rates (11%) between adjacent subpopulations (Barceló et al., 2021). Fisheries-caused mortality of common dolphins in the southern coast of Australia regional population is highest in the SASF fishery, whose fishing areas are in the Spencer Gulf, Gulf St. Vincent, Investigator Strait, and central shelf waters of South Australia. All mortalities and most encirclements of common dolphins in the SASF have been reported for this region (Ward et al., 2018).
Here we use double-observer aerial surveys and mark-recapture distance sampling methods to estimate the density and abundance of common dolphins inhabiting Spencer Gulf, Gulf St. Vincent, Investigator Strait and central shelf waters of central South Australia, where bycatch interactions and mortality of these dolphins in the SASF are concentrated. We also estimate sustainable levels of human-caused mortality using the potential biological removal (PBR) method.
Materials and Methods
Survey Design and Data Collection
Aerial surveys of common dolphins were conducted in the austral summer/autumn (March to June) and winter/spring (August to October) of 2011 over the core SASF purse seine fishing area in central South Australia including Spencer Gulf, Gulf St. Vincent, Investigator Strait and central shelf waters (Figure 1). The timing of the survey was planned to capture seasonality of dolphin distribution and abundance, to cover the high (March to June) and low season (August to October) of the SASF, and to coincide with the time of highest dolphin encirclements and mortalities reported in the SASF (March to June) (Ward et al., 2018). The study area was subdivided into four strata based on aircraft flying range and the availability of refueling and landing locations within the study area. We used automated survey design algorithms (Strindberg and Buckland, 2004) implemented in the software program DISTANCE (Thomas et al., 2009) to design a systematic line transect survey with regular line spacing within each survey stratum, and with a random start point to allow for homogeneous coverage probability over the selected area. The survey design consisted of parallel line transects aligned systematically and spaced 7–8 km apart (Figure 1). Gulf St. Vincent and Spencer Gulf (Stratum 2 and 3) were surveyed with transects aligned east-west extending from coast to coast, and central shelf waters and Investigator Strait (stratum 1 and 4) with transects aligned north-south, covering up to the 100 m depth isobath or the northern coast of Kangaroo Island. Transect orientation, east-west and north-south, was chosen to allow for transects to be approximately perpendicular to the coast in each stratum, thus facilitate sampling across different water depths and habitat types.
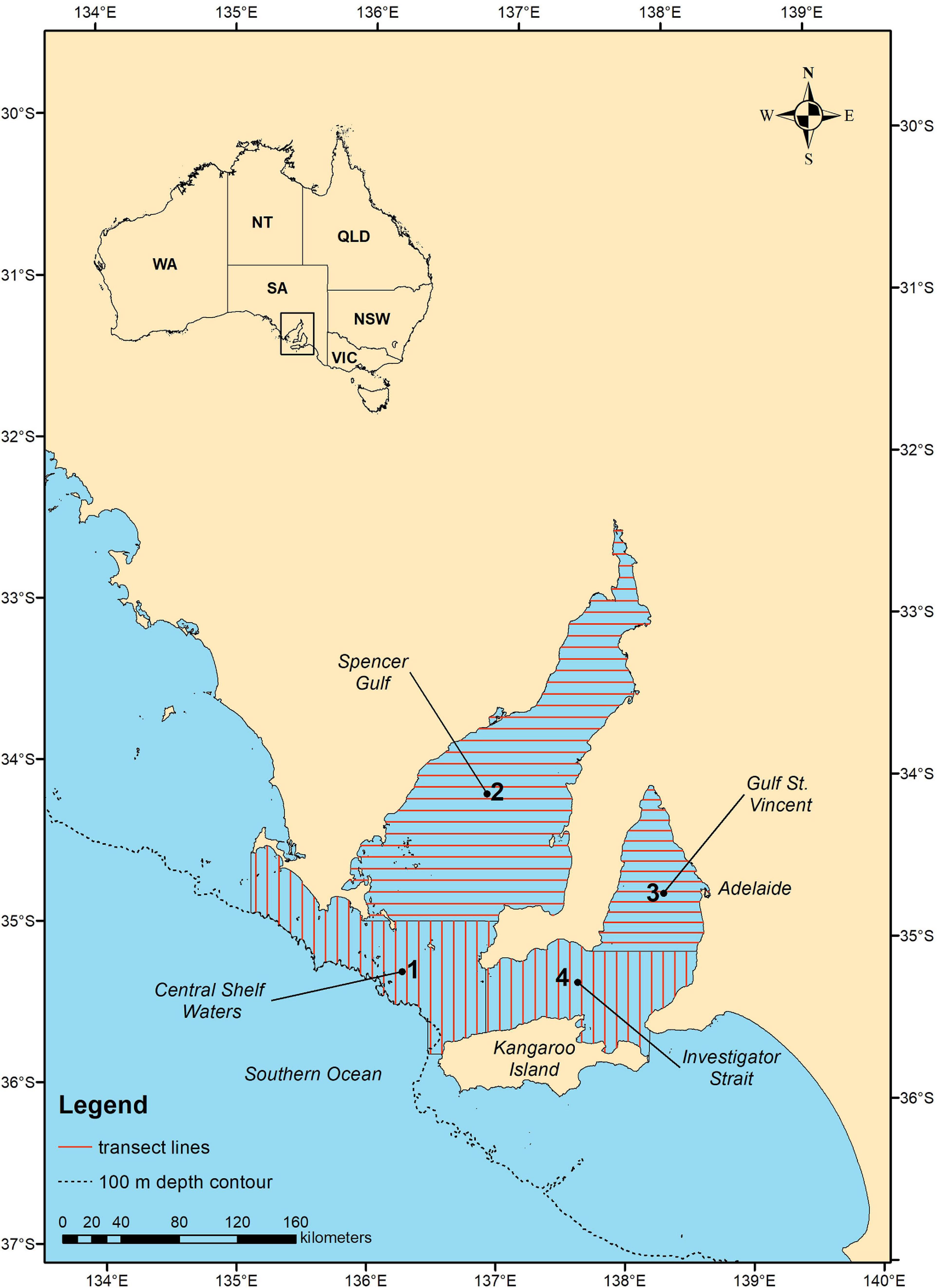
Figure 1. Map of the study area indicating the four aerial survey strata (numbers in bold), transect line layout (red lines). Black lines indicate the coastline, the divisions between strata, and for the southern section of stratum 1 the 100 m depth contour of the Australian continental shelf.
We conducted surveys from a Partenavia twin-engine, six-seat, high-wing aircraft commonly used in aerial surveys of cetaceans (e.g., Slooten et al., 2004; Panigada et al., 2011; Salgado Kent et al., 2012; Bilgmann et al., 2018). All survey lines were flown at a speed of 100 knots (185.2 km/h), at an altitude of 500 feet (152.4 m) and in good sighting conditions (i.e., winds < 15 knots and Beaufort Sea state ≤ 3). Surveys were conducted in “passing mode” (i.e., survey effort was not suspended to deviate from the transect line when a sighting was made), except when species identification or school size were uncertain. In these cases, survey effort was suspended to circle the animals, make further observations, and take digital photographs to confirm species identification and school size. A school was defined as individuals that were within a 100 m radius of each other and traveling in the same direction or exhibiting the same behavioral state. Survey effort then resumed at the same location where line transect effort was suspended. Transects were flown once in summer/autumn, and once in winter/spring.
Line transect surveys were conducted using double-observer methods with independent observation platforms at the middle and rear of the aircraft. The survey team consisted of six people: the pilot and data recorder in the cockpit, two observers, one on either side of the aircraft, sitting in the middle seats, just behind the cockpit, and two observers sitting in the rear on either side of the aircraft. Mid and rear-seat observers searched independently of each other and were visually (with a curtain) and acoustically (wearing headphones) isolated from each other while on effort. The mid-seat observers had bubble windows that allowed them to view the track line directly below the aircraft, while rear observers had flat windows that allowed them to view down to a 65-degree declination angle. The observers and the data recorder communicated via aviation headsets connected to two intercoms, so that the rear observers could be isolated acoustically from the mid-seat observers while on effort. Each intercom was connected to a separate track of a two-track digital voice recorder. This arrangement allowed both mid- and rear-seat observer data to be recorded independently. The observers recorded declination angles to dolphin sightings when abeam using inclinometers (Suunto PM-5/360PC) and collected data on species identification, school sizes and sighting conditions including Beaufort sea state, turbidity, cloud cover and glare severity (Supplementary Table 1). The data recorder entered survey effort data, sighting conditions (at the commencement of each transect leg and whenever any of these values changed), and all sighting data called by the mid seat observers, together with time stamp signals of position from a GPS system. All observers were trained in how to search for dolphins, correctly identifying the species of interest, estimating school size, and accurately measuring declination angles. Sightings with uncertain or unknown species identification, declination angles and or school sizes were not considered for analysis.
Data were entered and stored in a handheld computer, with integrated GPS, using the software CYBERTRACKER1, and a sequence developed specifically for dolphin aerial surveys. For analysis, declination angles to dolphin sightings recorded by the observers were converted to their respective perpendicular distance of the animal to the transect line using formulae which take into account the curvature of the earth (Lerczak and Hobbs, 1998). For sightings of dolphin schools, the approximate center of the school was used to measure the declination angle and therefore the perpendicular distance was representative of the distance from the approximate center of the school to the transect line.
Data Analysis
Estimates of abundance of common dolphins in summer/autumn and winter/spring were derived for each survey stratum using mark-recapture distance sampling (MRDS) methods (Laake and Borchers, 2004; Borchers et al., 2006) implemented in the DISTANCE software, version 7.1 (Thomas et al., 2010). Aerial surveys of cetaceans are subject to visibility bias, because animals may be submerged and therefore not available for detection (‘availability bias’), and they may be available for detection but the observers fail to detect them (‘perception bias’) (Marsh and Sinclair, 1989; Buckland et al., 2015). Perception bias can be accounted for by MRDS applied to data collected using double-observer line transect methods. In this analysis, the two observers in the mid seats of the plane were considered to be one platform (observer 1), and the two observers in the rear seats were considered as a second platform (observer 2). Dolphin detections by either observer serve as a set of trials for the other, in which one observer either succeeds to detect or ‘recapture’ the same animals as the other, or they fail. Sightings detected by both rear and mid-seat observers (duplicates) were assigned based on: (i) similarity in in the timing of both observations (±10 s), (ii) side of the aircraft that the observation was made, (iii) species identified, (iv) similarity in the distance from the track line (±30 m), and (v) resemblance in school sizes (±5 individuals) (Buckland et al., 2015; Panigada et al., 2017). When perpendicular distances and school sizes differed between duplicates, the average distance and school size of the duplicate pair was used for analysis (Burt et al., 2014). Analysis of the sightings made by observer 1 and 2 on the same side of the aircraft allows the estimation of the proportion of dolphin schools that were missed by observers, the probability of detection on the transect line, and the shape of the detection function away from the transect line as a function of perpendicular distance. We acknowledge that a probabilistic method to identify duplicate observations in MRDS has been proposed, but it has not been applied widely and still relies on an arbitrary definition of sighting discrepancy as the basis of the probabilistic analysis (Hamilton et al., 2018).
Although observers were acting independently, variables that affect detection probability of the target species (e.g., school size, Beaufort sea state) will usually affect the detection probabilities of both observers (Borchers et al., 2006). Thus detections by observers are not entirely independent, resulting in unmodelled heterogeneity in detection probability, which may lead to a positive bias in the estimated probability of detection and a negative bias in abundance estimates (Burt et al., 2014; Buckland et al., 2015). To account for this unmodelled heterogeneity, we used a point independence MRDS model to estimate detection probability (Laake and Borchers, 2004; Borchers et al., 2006). Point independence MRDS models are designed to deal with unmodelled heterogeneity by estimating two detection functions, a multiple covariate distance sampling (DS) detection function for detections pooled across platforms (mid and rear-seat observers), and a mark-recapture (MR) detection function to estimate the probability of detection on the transect line (i.e., at distance zero, Burt et al., 2014).
Mark-recapture distance sampling models were fitted using various permutations of both the half-normal and hazard-rate functional forms, and covariates (i.e., perpendicular distance, school size, Beaufort sea state, turbidity, cloud cover, glare severity, and side of the plane) thought to affect the scale of the detection function of the distance sampling and mark-recapture model components. Following distance sampling guidelines (Buckland et al., 2015), the data for summer/autumn and winter/spring aerial surveys were right truncated at a perpendicular distance of 900 m as very few observations were made past this distance. The 65-degree declination angle limiting the rear observers’ field of view (due to the lack of bubble windows), equates to a distance of 71 m from the transect line, thus this was set as our left truncation distance on each side of the plane (Slooten et al., 2004; Bilgmann et al., 2019; Sucunza et al., 2020). Sightings made by front and rear observers over the same region (after truncation) were used for MRDS analyses. We used a forward stepping procedure based on minimum Akaike Information Criterion (AIC) for model selection (Buckland et al., 2015).
The abundance of individual animals in each stratum () was estimated as (Buckland et al., 2015):
where Ai is the size of stratum i, ni is the total number of schools sighted in stratum i, Si is the average school size in stratum i, Li is the total length of transect line surveyed in stratum i, ESW is the effective half strip width, and g(0) is the estimated probability of detecting a school directly on the transect line. The variance of the density and abundance estimates was calculated based on Innes et al. (2002).
Correcting for Availability Bias
Mark-recapture distance sampling addresses perception bias but is not able to correct for availability bias. To adjust abundance estimates for availability bias, we estimated the proportion of time that dolphins were visible at or just below the water surface (Barlow, 1988; Laake et al., 1997; Slooten et al., 2004; Heide-Jørgensen et al., 2010). Here we used a helicopter survey approach modified from Slooten et al. (2004) to conduct 19 focal follows of common dolphin schools in the study area during 8–9 of February 2012. Focal follows were conducted at the same altitude (152.4 m) that the plane surveys were flown, and in similar distances from the shore and water clarity conditions than those encountered during the aerial survey. Once we sighted a school of dolphins from the helicopter, the school was circled for 10–15 min, and an observer recorded the amount of time the dolphins were visible (at or just below the water surface) and not visible (too far below the surface to be seen) between dives using the CyberTracker data application on a handheld computer with integrated GPS. We estimated that the average proportion of time common dolphin schools were visible from the air was 0.94 (CV = 0.11) (Bilgmann et al., 2018). Thus, abundance estimates were corrected to account for availability bias using (Heide-Jørgensen et al., 2010):
where is the estimated proportion of time dolphins are available for detection at or just below the water surface. The coefficient of variation (CV) of was calculated as (Heide-Jørgensen et al., 2010):
Estimating Potential Biological Removal
One of the most widely used approaches to assess/manage bycatch of marine mammal and other long-lived marine vertebrates is to calculate the maximum number of animals, not including natural mortalities, that may be removed from a marine mammal stock while allowing that stock to reach or maintain its optimum sustainable population (the Potential Biological Removal, PBR, Wade, 1998). The PBR method aims to ensure that human-caused mortality is below levels that could lead to population depletion while considering uncertainty and potential biases in the available information. We calculated the PBR using the following formula:
where Nmin is the 20th percentile of the estimated population size, Rmax the maximum theoretical or estimated annual population growth rate of the population in question, and Fr is a recovery factor between 0.1 and 1.0 that allocates a fraction of expected net production toward population growth and accounts for uncertainties in Rmax, Nmin, or errors in the determination of stock boundaries that might prevent population recovery (Wade, 1998).
Nmin was estimated as the lower percentile of a log-normal distribution as:
Where is the total population estimate selected for the analysis, CV is the coefficient of variation and Z is the standard normal deviate corresponding to a specific percentile, fixed at 0.842 for the 20th percentile (Wade, 1998).
As no estimates of the maximum annual population growth rates are available for common dolphins in South Australia, we set Rmax at 0.04 and 0.02. The value of Rmax = 0.04 is considered to be a standard value for cetaceans, particularly delphinids, which show maximum population growth rates in the order of 2 to 4% per year (Perrin and Reilly, 1984; Wade, 1998). A maximum growth rate of 4.5 ± 0.09% per year has been estimated for common dolphins in the Eastern North Atlantic where the species is subject to bycatch in several fisheries (Mannocci et al., 2012). We also used Rmax = 0.02 as a conservative approach to account for potential reduced maximum productivity rates in the dolphin population caused by factors during and post dolphin encirclements or other unidentified factors. These include encirclements causing mother-calf separations (Archer et al., 2001), declines in numbers of calves (Wade et al., 2007), and high fetal mortalities (Perrin, 1968), all of which are known population-level effects. These are likely major contributors to the lack of recovery of two closely related delphinid populations with similar ecology in the tuna purse seine fishery of the Eastern Tropical Pacific (Wade et al., 2007). Lastly, to derive PBR estimates we used recovery factors (Fr) values expected for endangered species or stocks known to be declining (Fr = 0.1), species of unknown conservation status (Fr = 0.5), and for species not at risk (Fr = 1) (Wade, 1998; Taylor et al., 2000). At species level, common dolphins are currently listed globally as Least Concern by the IUCN (Hammond et al., 2008), but as Data Deficient by The Action Plan for Australian Mammals 2012 (Woinarski et al., 2014).
Results
Survey Effort and Sightings
A total of 5,234.9 km of transect line was flown during the summer/autumn surveys and 5,284 km during the winter/spring, covering an area of 42,437.8 km2 (Table 2). Common dolphins were sighted in both seasons and in all strata, except during summer/autumn in Gulf St. Vincent (stratum 3) (Figure 2). We sighted a total of 223 common dolphin schools during summer/autumn and 584 schools during winter/spring, of which 147 and 401 were unique sightings (i.e., not duplicates), respectively (Table 2). School sizes varied from 1 to 100, with larger groups sighted during the summer/autumn (mean ± SD = 17.4 ± 19.9) than in the winter/spring period (mean ± SD = 7.6 ± 9.8) (Table 2).
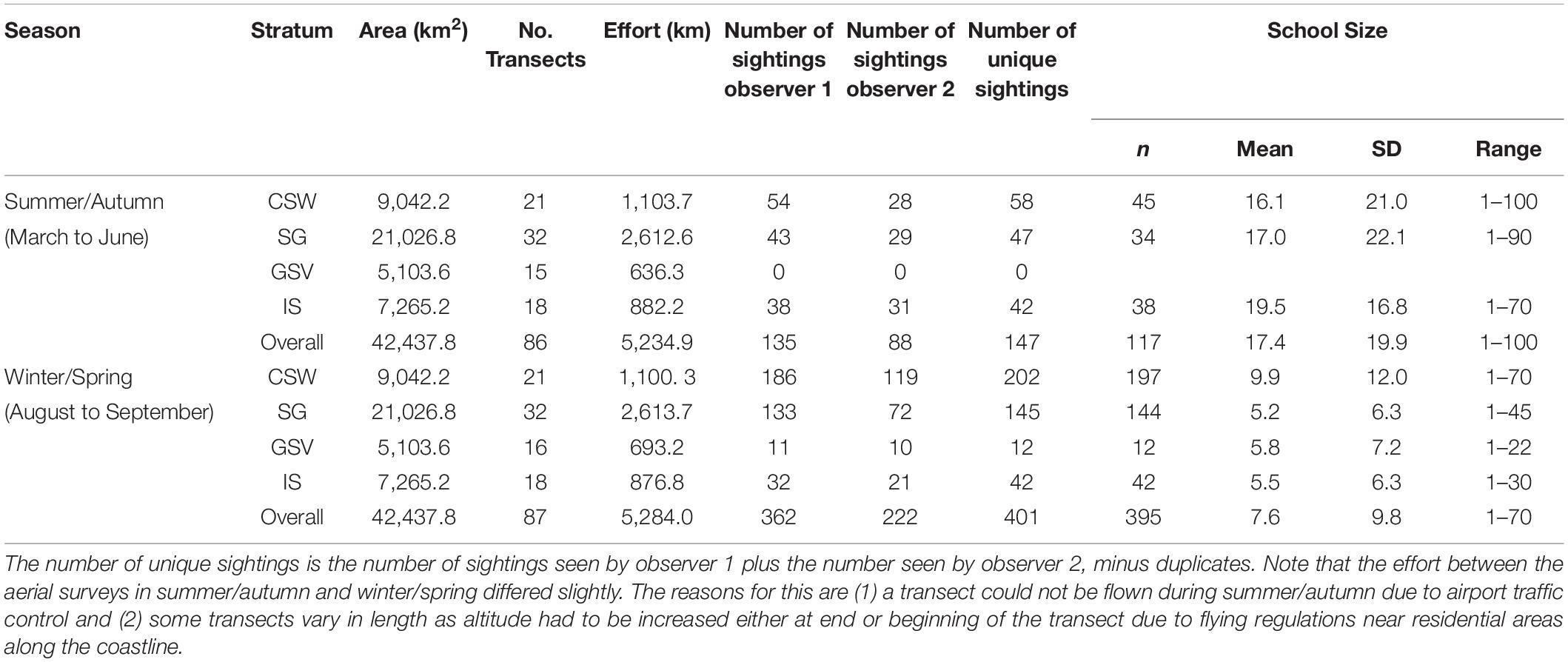
Table 2. Summary of aerial survey effort in 2011 in central South Australia by season and stratum (CSW, Central shelf waters; SG, Spencer Gulf; GSV, Gulf St. Vincent; IS, Investigator Strait), number of common dolphin (Delphinus delphis) schools sighted (before truncation) by mid (observer 1) and rear (observer 2) platforms, and estimates of school sizes.
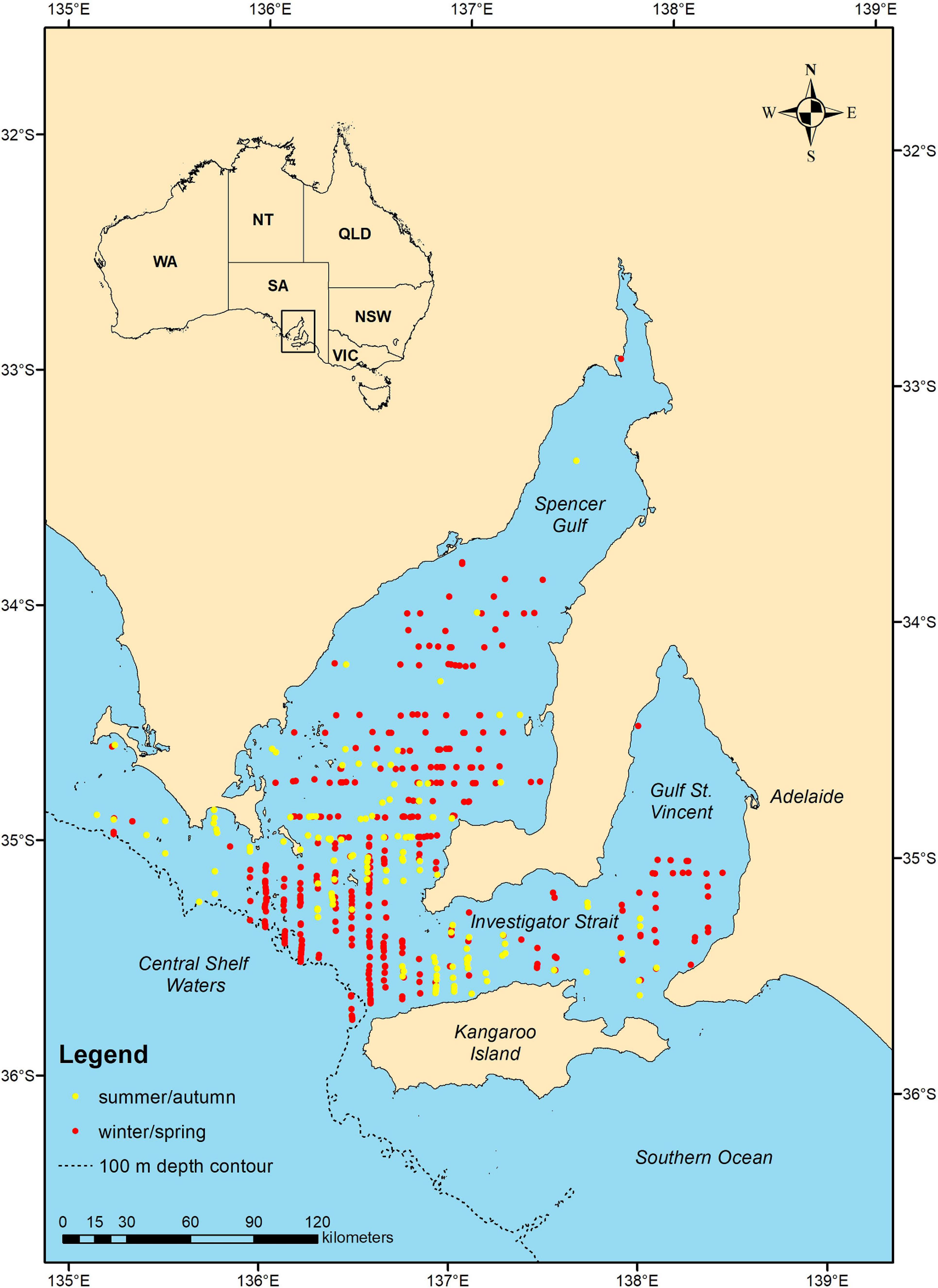
Figure 2. Map showing the distribution of common dolphin (Delphinus delphis) schools sighted (unique sightings, no truncation) in summer/autumn (yellow dots) and winter/spring (red dots) of 2011 during aerial line transect surveys in central South Australia.
Abundance and Density Estimates
After right truncation at 900 m and left truncation at 71 m, sightings were reduced to n = 120 and n = 337 for the summer/autumn and winter/spring period, respectively. The hazard-rate key function had a lower AIC than the half-normal key function for both summer/autumn and winter/spring surveys, and thus was selected for subsequent abundance estimation with distance sampling models (Supplementary Table 1). The average detection probability at the track-line [i.e., g(0)] for both mid and rear-seat observers was 0.89 (CV = 0.04) in summer/autumn and 0.72 (CV = 0.06) in winter/spring (Figure 3). The pooled probability of detection (i.e., that at least one observer in the double-platform configuration would see a sighting if it was on the track line) was 0.99 (CV = 0.01) in summer/autumn and 0.91 (CV = 0.03) in winter/spring (Figure 3).
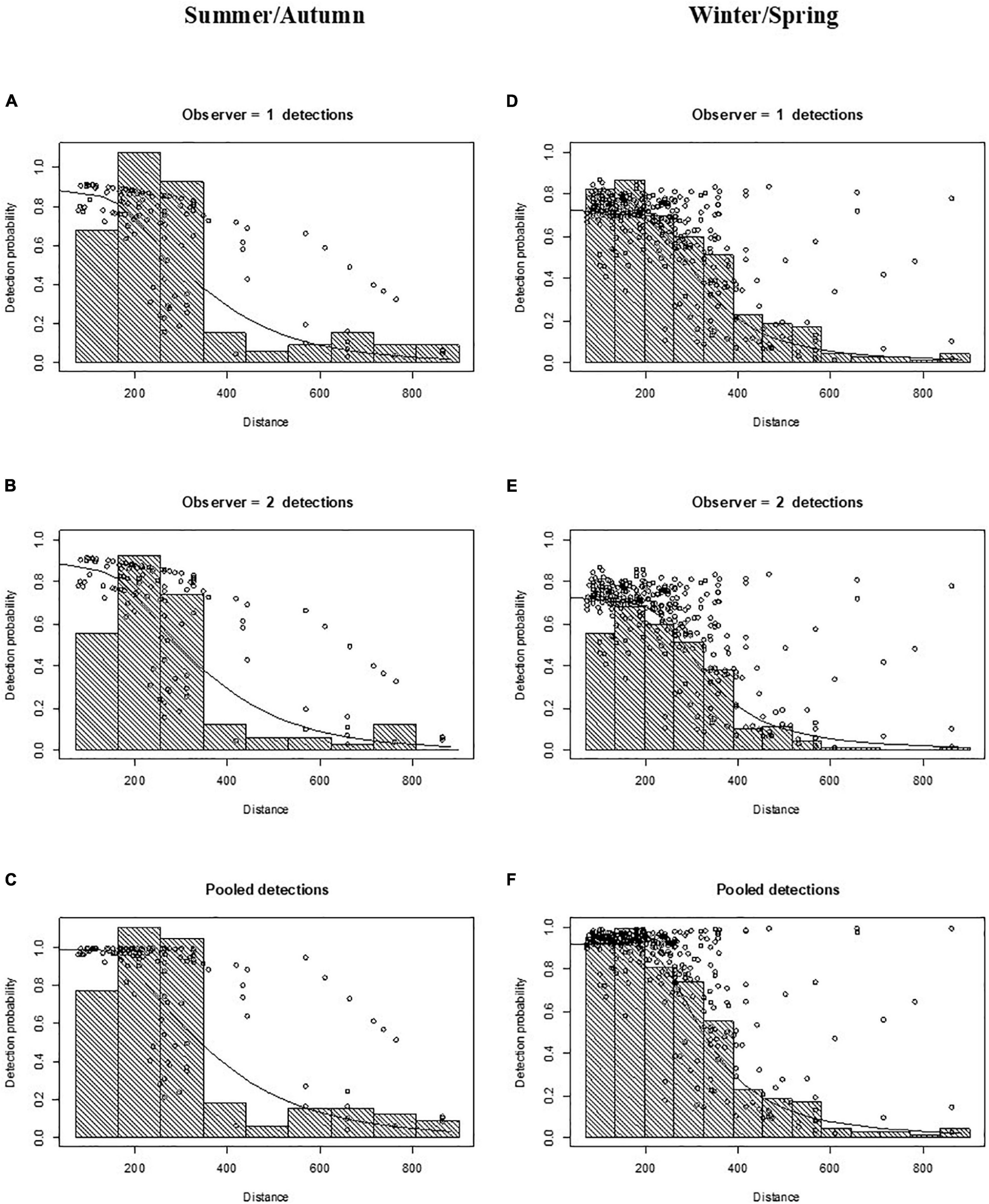
Figure 3. Detection function plots for the 2011 aerial survey of common dolphins (Delphinus delphis) in central South Australia in summer/autumn (A–C) and winter/spring (D–F). Points (circles) are the probability of detection for each sighting given its perpendicular distance and other covariate values. Lines are the fitted models. (A,D) Observer 1 detections (mid-seat observers); (B,E) observer 2 detections (rear-seat observers); and (C,F) pooled detections. Data were left truncated at 71 m (to adjust for obstructed view of the transect line) and right truncated at 900 m (to remove outliers at long distances).
The best MRDS model for the summer/autumn on the basis of AIC, involved a DS model with a hazard-rate key function scaled with covariates perpendicular distance + Beaufort sea state + turbidity + cloud cover + glare + side of the plane, and a MR model specified with perpendicular distance + cloud cover (Supplementary Table 2). The MRDS model with the lowest AIC for the winter/spring period included a DS model with a hazard-rate key function and perpendicular distance + Beaufort sea state + school size + cloud cover + glare + side of the plane as explanatory covariates, and a mark-recapture model including the same covariates (Supplementary Table 2).
Estimated abundance of common dolphins based on the selected models and corrected for availability and perception bias over the whole study area was 21,733 (CV = 0.25; 95% CI = 13,809–34,203) for summer/autumn and 26,504 for winter/spring (CV = 0.19; 95% CI = 19,488–36,046) (Table 3). Abundance and density estimates of common dolphins were highest in central continental shelf waters and lowest in Gulf St. Vincent across both seasons (Table 3). Except for the Investigator Strait, all strata showed higher abundance and density estimates of common dolphins during winter/spring than in summer/autumn (Table 3). Despite these differences, confidence intervals of abundance and density estimates for most survey regions and the overall study area overlapped broadly between seasons (Table 3).
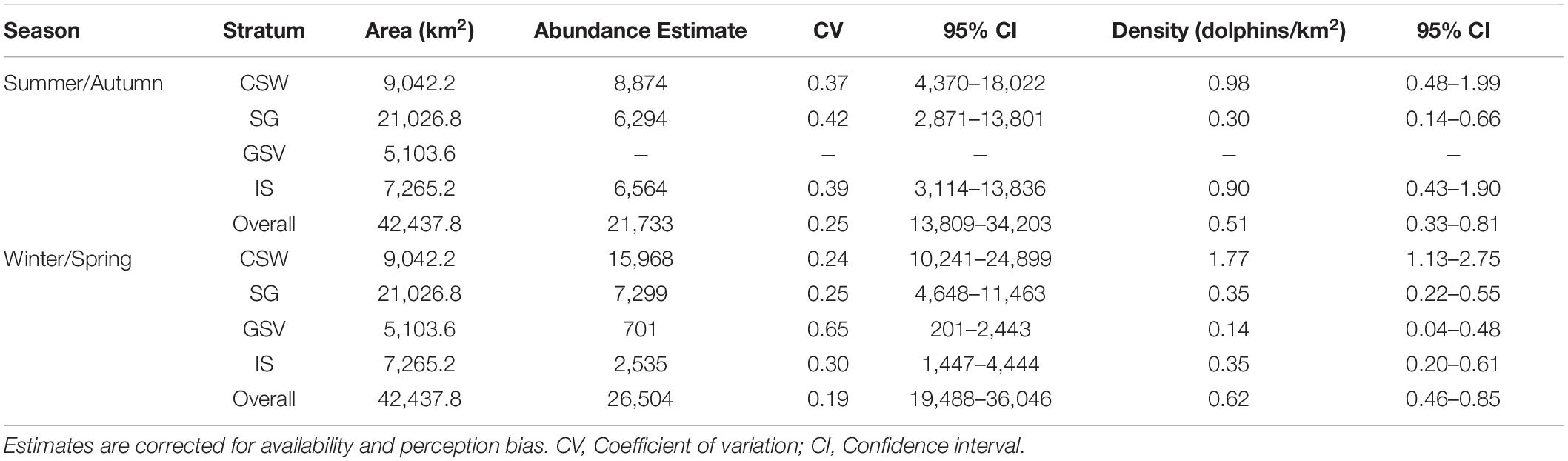
Table 3. Estimates of abundance and density of common dolphins (Delphinus delphis) from the 2011 summer/autumn and winter/spring aerial surveys in central South Australia.
Estimates of Potential Biological Removal
Estimates of the annual PBR of common dolphins in the study area, assuming a conservative maximum population growth rate of Rmax = 0.02 and a recovery factor of Fr = 0.5 for species of unknown conservation status, ranged from 95 (summer/autumn) to 120 dolphins (winter/spring) (Table 4). Using a maximum rate of population increase of Rmax = 0.04 and an Fr = 0.5 resulted in annual PBR estimates of 189 (summer/autumn) and 239 dolphins (winter/spring) (Table 4).
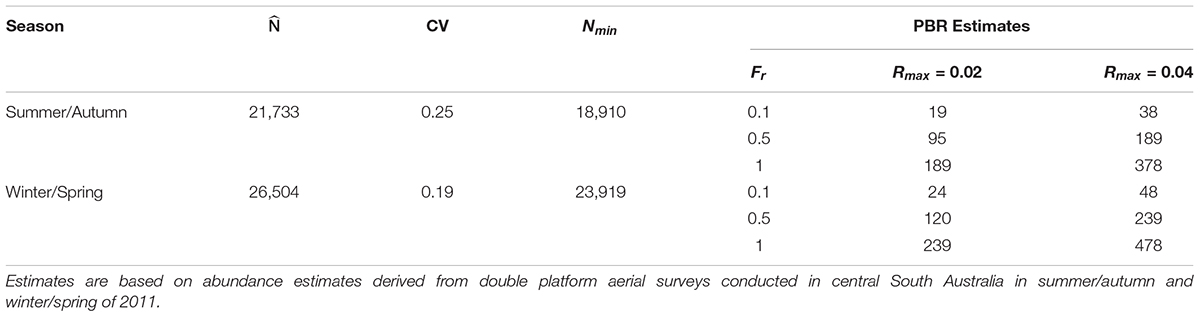
Table 4. Estimates of abundance , coefficient of variation (CV), 20th Percentile of abundance (Nmin) and the maximum number of common dolphins (Delphinus delphis) that may be removed sustainably (Potential Biological Removal, PBR) from central South Australia under different recovery factors (Fr) and maximum population growth rates (Rmax).
Discussion
Common dolphins in South Australian waters are subject to incidental bycatch in the purse seine and gillnet fisheries. Within this region, most of the dolphin entanglements and mortalities occur in the SASF core fishing area in central South Australian waters including Spencer Gulf, Gulf St. Vincent, Investigator Strait, and adjacent central shelf waters (Ward et al., 2018). The impact and sustainability of these and other fisheries-related mortalities on common dolphins in this region is uncertain without an estimate of common dolphin abundance. This study shows that common dolphins are a relatively abundant species in central South Australian waters, with estimates ranging from 21,733 (CV = 0.25) to 26,504 (CV = 0.19) individuals in summer/autumn and in winter/spring, respectively. Annual PBR estimates varied from tens of individuals considering a conservative Rmax of 0.02 and Fr of 0.1 for species at risk or in decline, to hundreds of animals if we assume an Rmax of 0.04 and an Fr of 0.5 or 1 for species of unknown conservation status or not at risk, respectively.
Abundance estimates from visual aerial surveys of cetaceans are typically negatively biased due to availability and perception bias, and unmodelled heterogeneity in detection probability (Marsh and Sinclair, 1989; Buckland et al., 2015). Additionally, measurement errors (e.g., species misidentification, or mis-measurement of declination angles and school sizes), and animal movement in response to the observer, or independently of the observer, can cause biases in abundance estimates (Borchers et al., 2010; Glennie et al., 2015). Using aerial-line transect surveys with a double-observer platform and mark-recapture distance sampling analyses, we were able to derive relatively precise estimates with CVs close to ≤ 20% (Buckland et al., 2001), while correcting for availability and perception bias. Furthermore, we incorporated covariates (Supplementary Table 1) other than distance from the line into the detection function models, which are known to have a significant effect on detectability of cetaceans at the surface, and result in unmodelled heterogeneity in detection probability (Holt and Cologne, 1987; Barlow, 2015). Aerial surveys generally do not cause dolphins to move toward or away from the transect line given the high flight altitude, and dolphin travel speed being slower than the survey platform, thus independent animal movement and responsive movement are unlikely to have caused bias to our abundance estimates (Dawson et al., 2008; Buckland et al., 2015). Double counting of dolphin schools due to movement of animals through the study area across the survey period is possible, but only has a minimal effect on abundance estimates (Buckland et al., 2001).
Erroneous estimation of dolphin school sizes during surveys can lead to biased estimation of abundance (Hamilton et al., 2018; Gerrodette et al., 2019). During our aerial surveys, whenever school size was uncertain while on transect, a circle-back procedure was followed to obtain more accurate school size estimates. In addition, sightings with uncertain or missing school sizes were not considered for analyses. The schools of common dolphins we encountered were generally small (summer/autumn: mean ± SD = 17.4 ± 19.9, winter/spring: mean ± SD = 7.6 ± 9.8), thus facilitating school size estimation. Therefore, we consider that the error in observer estimates of school size was likely to be negligible and thus caused little bias in abundance estimation. Lastly, we trained field personnel to collect and record high quality data and minimize measurement error. Therefore, we believe our abundance estimates are robust and represent a solid baseline of the number of common dolphins within the study area at the time the surveys were undertaken.
Abundance and density estimates for common dolphins indicate that this species is the most abundant delphinid species in central South Australia. The abundance and density of the other delphinid sighted regularly in the study area, the bottlenose dolphin (Tursiops sp.), was estimated at 3,493 (CV = 0.21; 95% CI = 2,327–5,244, density = 0.08 dolphins/km2) in summer/autumn, and 3,213 (CV = 0.20; 95% CI = 2,151–4,801, density = 0.08 dolphins/km2) in winter/spring of 2011 (Bilgmann et al., 2019). The only other estimate of abundance of common dolphins available at a large scale (over 10,000 km2) in Australia is for waters off the western Eyre Peninsula (29,822 km2), located to the west and adjacent to our study area (Bilgmann et al., 2018). Using a single platform aerial survey and distance sampling methodology (without a mark-recapture component), the abundance of common dolphins off the western Eyre Peninsula was estimated to be 20,214 individuals (CV = 0.31, density = 0.67 dolphins/km2, using multiple covariate distance sampling) and 21,884 individuals (CV = 0.28, density = 0.73 dolphins/km2, using conventional distance sampling) (Bilgmann et al., 2018). Together these estimates indicate that waters over the South Australian continental shelf up to 100 m depth represent an important habitat for common dolphins, and that these dolphins are likely one of the most abundant large mesopredator species in this ecosystem.
Globally, common dolphins are an abundant species that feed on a variety of fish and cephalopods in coastal and oceanic areas. Estimates of abundance in the order of millions have been reported for the Eastern Tropical Pacific (19.6 million km2) in 2006 (3,127,203, CV = 0.26, density = 0.15 dolphins/km2) (Gerrodette et al., 2008), and the California Current Ecosystem (1.14 million km2) along the United States West Coast in 2014 (1,427,576, CV = 0.25, density = 1.25 dolphins/km2) (Barlow, 2016), and tens of thousands for the United States Atlantic coast (463,000 km2) in 2011 (N = 67,191, CV = 0.29, density = 0.14 dolphins/km2) (Palka, 2012), European Atlantic shelf waters (1.37 million km2) in 2005 (N = 56,221, CV = 0.23, density = 0.041 dolphins/km2) (Hammond et al., 2013), and the southwestern Mediterranean sea (19,189 km2) between 2000 and 2004 (N = 19,428, CV = 0.18, density = 1.01 dolphins/km2) (Cañadas and Hammond, 2008). Across these areas, concentrations of common dolphins are often found in association with highly productive upwelling regions where prey is abundant (Ballance et al., 2006; Jefferson et al., 2009; Giralt Paradell et al., 2019).
The southern continental shelves of Australia host one of the largest seasonal coastal upwelling systems on earth referred to as the Great South Australian Coastal Upwelling System (Kämpf et al., 2004; Kämpf, 2015). This upwelling system occurs during the austral summer/autumn months (December to April) and consists of four upwelling centers (off southern Eyre Peninsula, off southwestern Kangaroo Island, along the Bonney Coast, and off the western Tasmanian shelf) spanning ∼1,500 km (∼ from 133 to 146°E longitude) along South Australia, Victoria and Tasmania (Kämpf et al., 2004; Kämpf, 2015). These upwelling centers are hotspots of primary productivity and support a large biomass of sardines (Sardinops sagax) and anchovies (Engraulis australis) (Dimmlich et al., 2004; Ward et al., 2006), which are known prey of common dolphins in South Australia (Gibbs, 2011) and elsewhere (Meynier et al., 2008; Marçalo et al., 2018). Thus, as in other areas of the Atlantic and Pacific Oceans, the high abundance and density of common dolphins in South Australia is likely driven by local oceanographic currents and upwelling events that allow for seasonal high densities of small pelagic fish.
Estimates of common dolphin abundance and density in central South Australian waters tended to be higher in winter/spring than in summer/autumn, but the confidence intervals overlapped broadly making it difficult to assess if this seasonal difference was of biological significance or not. It is possible that differences in seasonal abundance estimates reflect seasonal movements or immigration influxes of individuals from neighboring areas adjacent to our study site. Moderate migration rates (3–11%) have been detected between different subpopulations across southern Australia (Bilgmann et al., 2014; Barceló et al., 2021) and at least on one occasion, individuals from the Australian east coast (Pacific Ocean) stock were detected in the study region (Bilgmann et al., 2014). Seasonal fluctuations in abundance and movement of common dolphins have been reported for the Western and Eastern North Atlantic (Gowans and Whitehead, 1995; Luca et al., 2009), the Eastern Tropical Pacific (Kerri and Susan, 2006), off the south-east coast of southern Africa (Cockcroft and Peddemors, 1990), and the north-western Bay of Plenty, New Zealand (Neumann, 2001). These changes in abundance and movements appear to be associated with fluctuations in sea-surface temperature, upwellings, and ultimately prey availability. Productivity in the study region in central South Australian waters tends to be low during winter, but then increases during the austral summer–autumn season due to coastal upwelling at several locations along the coast (Kämpf et al., 2004; Kämpf, 2015). Further systematic surveys within and beyond our study area are needed across seasons to establish if there is truly a significant seasonal shift in abundance as a result of immigration or movements of common dolphins. Regardless, the high number of common dolphins present in the area across seasons likely reflects the high abundance of prey present year-round. Although catch rates of sardines peak in March to June (∼ 23,000 tons in 2011) in South Australia, sardines are still caught in relatively large amounts in winter/spring (August to September: ∼1,000 tons in 2011) (Ward et al., 2017).
The only currently existing abundance estimate for common dolphins over the core fishing area of the SASF in central South Australian waters is the one provided here for 2011. If common dolphin abundance in 2011 was similar to 2004/5, when dolphin bycatch was the highest recorded in the SASF (423 dolphin mortalities), all PBR estimates, with the exception of those assuming a maximum population growth rate of Rmax = 0.04 and a recovery factor of Fr = 0.1 for species not at risk, suggested that common dolphin mortality in this fishery alone was likely unsustainable. However, if reported common dolphin mortalities in the SASF fishery after 2004/05 (0–113 dolphin mortalities) are a true account of the total numbers of dolphins incidentally killed, and no residual dolphin mortality exists from encirclements, the industry Code of Practice (CoP) appears to be effective at reducing dolphin mortality. More recent bycatch levels from the SASF alone fall below most of the PBR estimates based on the 2011 estimated abundance. Reported common dolphin mortalities in the GHAT (0–26) and the SPF (0–9 dolphin mortalities) fisheries since 2012 indicate that the cumulative incidental common dolphin mortality across the fisheries affecting southern Australia would also be below (the 2011 abundance-based) PBR estimates.
Despite the apparent effectiveness of the range of measures that have been introduced in the SASF, GHAT and SPF fisheries across the years to minimize dolphin interactions, uneven observer coverage among vessels, months and regions, and most importantly, underreporting of dolphin mortalities by fishers in the absence of an observer, remain key challenges to fully understanding the magnitude and effects of fisheries bycatch. For example, the reporting of dolphin mortality rates in the SASF in 2018–2019 (observer coverage = 12.4%), was 99 times higher for net-sets with observers compared to those without (Goldsworthy et al., 2019), an extremely high discrepancy that highlights the importance of observer programs and increased coverage levels. Underreporting of dolphin interactions by fishers in logbook records is a common problem across most impacted dolphin populations and regions (Lewison et al., 2004; Read et al., 2006; Allen et al., 2014). While well designed observer programs may provide the most robust measure of marine mammal bycatch, they may also underestimate bycatch, as many small entangled marine mammals such as dolphins may drop out of fishing gear (e.g., gillnets) or go undetected by fishers or observers while fishing gear is being retrieved (Lewison et al., 2004; Read et al., 2006). This is particularly a problem for electronic monitoring systems, but can also occur when a human observer is on board. Currently, a minimum of 10% of the SASF, GHAT, and SPF fishing activities, respectively, are independently reviewed or observed, either by using electronic monitoring or an observer on board (Ward et al., 2018; AFMA, 2019a,b). This relatively low coverage, combined with the ongoing underreporting of dolphin interactions by fishers, means that estimates of dolphin bycatch rates are not robust. Thus, it is imperative that the SASF, GHAT and SPF observer programs continue to operate while reassessing the observer coverage needed (including the percentages viewed from electronic monitoring) to ensure accurate reporting of dolphin mortalities, help reduce future interactions, and aid management and the future conservation status of this species in the region.
It is important to note that the PBR estimates provided here refer to a segment of this regional population from which common dolphins are mainly taken, and do not apply to its entire range which extends across the southern Australian coast (Barceló et al., 2021). If anthropogenic mortality mainly occurs in a portion of a population or stock’s geographic range, as is the case in our study, the advice is to avoid extrapolating abundance estimates from the surveyed area to unsurveyed areas to estimate range-wide PBRs, and base PBR calculations on abundance estimates corresponding to the area where human caused mortality is concentrated (Moore and Merrick, 2011). Extrapolation of density estimates into unsurveyed areas can lead to highly biased abundance estimates (and thus PBR estimates), because relationships between species densities and covariates are mostly unknown in extrapolated areas (Mannocci et al., 2017). Future surveys covering the full geographic extent of the southern coast of Australia regional population are needed to resolve uncertainty in abundance and obtain range wide PBR estimates.
Understanding the effects of cumulative human impacts is key to assess risk to wildlife populations and guide effective protection and management measures. Small cetaceans are vulnerable to a range of human-induced mortality and have a limited capacity for population recovery due to their slow life histories and reproduction (late sexual maturity, long interbirth intervals, extensive maternal care and high longevity). Comparing PBR numbers with those of fisheries bycatch alone gives a false sense of security that these numbers can safely be removed without potentially leading to population declines. Thus, when comparing PBR numbers to those of fisheries bycatch mortality, it is important to also consider other sources of anthropogenic mortality including those that are non-fishery related as cumulative threats can lead to reduced fitness and population declines.
Apart from fisheries interactions, anthropogenic threats to common dolphins in South Australian waters span a wide range of stressors, including over-exploitation of prey resources, water pollution, diseases, urban and industrial habitat degradation and climate change (Kemper et al., 2016; Robbins et al., 2017). For example, modeling has shown that extreme epizootic events and climate change disturbance scenarios with high frequency and intensity have the biggest influence on population trends of bottlenose dolphins in Spencer Gulf and Gulf St. Vincent (Reed et al., 2020), and these are likely to also pose a threat to common dolphins. Furthermore, there has been no assessment of the potential long-term impacts (e.g., enhanced stress levels, mother-calf separations) that fisheries interactions (e.g., encirclement and release operations in purse seine nets) may have on dolphins, and how this may impact reproductive success and survival rates in the affected populations (Archer et al., 2004; Edwards, 2006; Wade et al., 2007; Cramer et al., 2008). Annual dolphin mortalities due to these stressors are unknown, but if these mortalities are of a large magnitude, they could, in conjunction with incidental mortality in fisheries, reach or overpass PBR levels presented here. Given the common dolphin’s high abundance in central South Australia and upper trophic level, they have the potential to affect the structure and function of local marine ecosystems through direct predation, predation risks and behaviorally mediated indirect interactions (Kiszka et al., 2015), and their decline could lead to a trophic cascade (Goldsworthy et al., 2013). Therefore, knowledge of the effects of cumulative stressors on their distribution and population dynamics are required to aid the future conservation status of these species in the region. The abundance and potential biological removal estimates presented here offer a starting point for fisheries authorities to develop precautionary measures to manage bycatch impacts on common dolphins based on local abundance estimates (e.g., by setting local limits on bycatch that allow for incremental progress toward limiting mortality for the total population, Curtis et al., 2015). Further research on the range-wide abundance and cumulative human-caused mortality of common dolphins in southern Australia should facilitate wider application of findings across the species range.
Data Availability Statement
The raw data supporting the conclusions of this article will be made available by the authors, without undue reservation.
Ethics Statement
The animal study was reviewed and approved by Flinders University and Southern Adelaide Health Service Animal Welfare Committee, permit number E326. All data were collected under a research permit of the Department of Environment and Natural Resources, Government of South Australia (E25889-1/2).
Author Contributions
GJP, KB, and LM planned and designed the project. GJP, KB, LM, and KP collected and edited the data. GJP wrote the manuscript and analyzed the data. All authors reviewed and edited the manuscript.
Funding
This study was funded by the Australian Marine Mammal Centre (AMMC) Grants Program, Australian Antarctic Division (AAD) (Project Number 2010/33).
Conflict of Interest
The authors declare that the research was conducted in the absence of any commercial or financial relationships that could be construed as a potential conflict of interest.
Publisher’s Note
All claims expressed in this article are solely those of the authors and do not necessarily represent those of their affiliated organizations, or those of the publisher, the editors and the reviewers. Any product that may be evaluated in this article, or claim that may be made by its manufacturer, is not guaranteed or endorsed by the publisher.
Acknowledgments
We are thankful to our pilot Brad Welch and business partner Alexey Suarez Gonzalez, Observair Ptd. Lty., who greatly contributed to the success of this study. We are grateful to Prof. Philip Hammond and Dr. Ana Cañadas for their advice on the aerial survey design, and to Dr. Amanda Hodgson for the initial aerial survey training. We would like to extend our thanks to our aerial observer team: Lauren Holmes, Dr. Matthias Schmidt, Juliet Shrimpton, Rebecca Tite, Dr. Nikki Zanardo, and Dr. Jimena Rodriguez-Zarate.
Supplementary Material
The Supplementary Material for this article can be found online at: https://www.frontiersin.org/articles/10.3389/fmars.2021.617075/full#supplementary-material
Footnotes
References
AFMA (2011). Regulation Impact Statement: Managing Interactions with Dolphins in the Gillnet Hook and Trap Sector of the Southern and Eastern Scalefish and Shark Fishery. Canberra ACT: Australian Fisheries Management Authorithy.
AFMA (2012). Regulation Impact Statement: Managing Interactions with Dolphins in the Gillnet Hook and Trap Sector of the Southern and Eastern Scalefish and Shark Fishery. Canberra: Australia: Australian Fisheries Management Authority.
AFMA (2013). Regulation Impact Statement: Managing Interactions with Dolphins in the Gillnet Hook and Trap Sector of the Southern and Eastern Scalefish and Shark Fishery. Canberra: Australia: Australian Fisheries Management Authority.
AFMA (2014). Dolphin Strategy: Minimising Gillnet Bycatch. Canberra: Australia: Australian Fisheries Management Authority.
AFMA (2019a). Gillnet Dolphin Mitigation Strategy: Minimising Dolphin Interactions with Gillnets in the Southern and Eastern Scalefish and Shark Fishery. Canberra: Australia: Australian Fisheries Management Authority.
AFMA (2019b). Small Pelagic Fishery Dolphin Strategy: Minimising Dolphin Interactions in the Small Pelagic Fishery. Canberra: Australia: Australian Fisheries Management Authority.
AFMA (2020). Protected Species Interactions Quarterly Reports: 2012-2019. Canberra: Australia: Australian Fisheries Management Authority.
Allen, S. J., Tyne, J. A., Kobryn, H. T., Bejder, L., Pollock, K. H., and Loneragan, N. R. (2014). Patterns of dolphin bycatch in a north-western australian trawl fishery. PLoS One 9:e93178. doi: 10.1371/journal.pone.0093178
Archer, F., Gerrodette, T., Chivers, S., and Jackson, A. (2004). Annual estimates of the unobserved incidental kill of pantropical spotted dolphin (Stenella attenuata attenuata) calves in the tuna purse-seine fishery of the eastern tropical Pacific. Fish. Bull. 102, 233–244.
Archer, F., Gerrodette, T., Dizon, A., Abella, K., and Southern, S. (2001). Unobserved kill of nursing dolphin calves in a tuna purse-seine fishery. Mar. Mamm. Sci. 17, 540–554. doi: 10.1111/j.1748-7692.2001.tb01003.x
Ballance, L. T., Pitman, R. L., and Fiedler, P. C. (2006). Oceanographic influences on seabirds and cetaceans of the eastern tropical Pacific: a review. Prog. Oceanogr. 69, 360–390. doi: 10.1016/j.pocean.2006.03.013
Barceló, A., Sandoval-Castillo, J., Stockin, K. A., Bilgmann, K., Attard, C. M., Zanardo, N., et al. (2021). A matter of scale: population genomic structure and connectivity of fisheries at-risk common dolphins (Delphinus delphis) from Australasia. Front. Mar. Sci. 8:616673. doi: 10.3389/fmars.2021.616673
Barlow, J. (1988). Harbor porpoise, Phocoena phocoena, abundance estimation for California, Oregon, and Washington: 1. Ship surveys. Fish. Bull. 86, 417–432.
Barlow, J. (2015). Inferring trackline detection probabilities, g(0), for cetaceans from apparent densities in different survey conditions. Mar. Mamm. Sci. 31, 923–943. doi: 10.1111/mms.12205
Barlow, J. (2016). Cetacean Abundance in the California Current Estimated From Ship-Based Line-Transect Surveys in 1991-2014. NOAA Southwest Fisheries Science Center, Administrative Report, LJ-2016-01. Washington, D.C: NOAA, 63.
Bilgmann, K., Möller, L. M., Harcourt, R. G., Gales, R., and Beheregaray, L. B. (2008). Common dolphins subject to fisheries impacts in Southern Australia are genetically differentiated: implications for conservation. Anim. Conserv. 11, 518–528. doi: 10.1111/j.1469-1795.2008.00213.x
Bilgmann, K., Möller, L. M., Harcourt, R. G., Gales, R., and Beheregaray, L. B. (2009). Reply to ‘Clarifying the interpretation of Hamer et al. (2008) by Bilgmann et al. (2008)’. Animal Conservation 12, 289–290. doi: 10.1111/j.1469-1795.2009.00270.x
Bilgmann, K., Parra, G. J., Holmes, L., Peters, K. J., Jonsen, I. D., and Möller, L. M. (2019). Abundance estimates and habitat preferences of bottlenose dolphins reveal the importance of two gulfs in South Australia. Sci. Rep. 9:8044. doi: 10.1038/s41598-019-44310-3
Bilgmann, K., Parra, G. J., and Möller, L. M. (2018). Occurrence, distribution and abundance of cetaceans off the western Eyre Peninsula in the Great Australian Bight. Deep Sea Res. Part II: Top. Stud. Oceanogr. 157-158, 134–145. doi: 10.1016/j.dsr2.2017.11.006
Bilgmann, K., Parra, G. J., Zanardo, N., Beheregaray, L. B., and Möller, L. M. (2014). Multiple management units of short-beaked common dolphins subject to fisheries bycatch off southern and southeastern Australia. Mar. Ecol. Prog. Ser. 500, 265–279. doi: 10.3354/meps10649
Borchers, D., Marques, T., Gunnlaugsson, T., and Jupp, P. (2010). Estimating distance sampling detection functions when distances are measured with errors. J. Agric. Biol. Environ. Stat. 15, 346–361. doi: 10.1007/s13253-010-0021-y
Borchers, D. L., Laake, J. L., Southwell, C., and Paxton, C. G. M. (2006). Accommodating unmodeled heterogeneity in double-observer distance sampling surveys. Biometrics 62, 372–378. doi: 10.1111/j.1541-0420.2005.00493.x
Brownell, R. L. Jr., Reeves, R. R., Read, A. J., Smith, B. D., Thomas, P. O., Ralls, K., et al. (2019). Bycatch in gillnet fisheries threatens critically endangered small cetaceans and other aquatic megafauna. Endanger. Species Res. 40, 285–296. doi: 10.3354/esr00994
Buckland, S. T., Anderson, D. R., Burnham, K. P., Laake, J. L., Borchers, D. L., and Thomas, L. (2001). Introduction to Distance Sampling: Estimating Abundance of Biological Populations. Oxford: Oxford University Press.
Buckland, S. T., Rexstad, E. A., Marques, T. A., and Oedekoven, C. (2015). Distance Sampling: Methods and Applications. Berlin: Springer.
Burt, M. L., Borchers, D. L., Jenkins, K. J., and Marques, T. A. (2014). Using mark–recapture distance sampling methods on line transect surveys. Methods Ecol. Evol. 5, 1180–1191. doi: 10.1111/2041-210X.12294
Cagnazzi, D., Parra, G. J., Westley, S., and Harrison, P. L. (2013). At the heart of the industrial boom: australian snubfin dolphins in the capricorn coast, queensland, need urgent conservation action. PLoS One 8:e56729. doi: 10.1371/journal.pone.0056729
Cañadas, A., and Hammond, P. S. (2008). Abundance and habitat preferences of the short-beaked common dolphin Delphinus delphis in the southwestern Mediterranean: implications for conservation. Endanger. Species Res. 4, 309–331. doi: 10.3354/esr00073
Cockcroft, V. G., and Peddemors, V. M. (1990). Seasonal distribution and density of common dolphins Delphinus delphis off the south-east coast of southern Africa. South Afr. J. Mar. Sci. 9, 371–377. doi: 10.2989/025776190784378853
Cramer, K. L., Perryman, W. L., and Gerrodette, T. (2008). Declines in reproductive output in two dolphin populations depleted by the yellowfin tuna purse-seine fishery. Mar. Ecol. Progr. Ser. 369, 273–285. doi: 10.3354/meps07606
Curtis, K. A., Moore, J. E., Boyd, C., Dillingham, P. W., Lewison, R. L., Taylor, B. L., et al. (2015). Managing catch of marine megafauna: guidelines for setting limit reference points. Mar. Policy 61, 249–263. doi: 10.1016/j.marpol.2015.07.002
Dawson, S., Wade, P., Slooten, E., and Barlow, J. A. Y. (2008). Design and field methods for sighting surveys of cetaceans in coastal and riverine habitats. Mamm. Rev. 38, 19–49. doi: 10.1111/j.1365-2907.2008.00119.x
DEE (2018). Assessment of the Commonwealth Small Pelagic Fishery October 2018. Canberra: Australian Government Department of the Environment and Energy.
DEH (2006). Assessment of the Small Pelagic Fishery. Canberra: Australian Government Department of the Environment and Energy.
Dimmlich, W. F., Breed, W. G., Geddes, M., and Ward, T. M. (2004). Relative importance of gulf and shelf waters for spawning and recruitment of Australian anchovy, Engraulis australis, in South Australia. Fish. Oceanogr. 13, 310–323. doi: 10.1111/j.1365-2419.2004.00296.x
Edwards, E. F. (2006). Duration of unassisted swimming activity for spotted dolphin (Stenella attenuata) calves: implications for mother-calf separation during tuna purse-seine sets. Fish. Bull. 104, 125–135.
Gerrodette, T., Perryman, W. L., and Oedekoven, C. S. (2019). Accuracy and precision of dolphin group size estimates. Mar. Mamm. Sci. 35, 22–39. doi: 10.1111/mms.12506
Gerrodette, T., Watters, G., Perryman, W., and Balance, L. (2008). Estimates of 2006 Dolphin Abundance in The Eastern Tropical Pacific, With Revised Estimates From 1986-2003.NOAA-TMNMFS-SWFSC-422. Washington, D.C: NOAA.
Gibbs, S. E. (2011). Multi-Species Niche Partitioning in High Trophic Level Marine Predators in South Australia. Ph.D. Thesis. Sydney: Macquarie University.
Giralt Paradell, O., Díaz López, B., and Methion, S. (2019). Modelling common dolphin (Delphinus delphis) coastal distribution and habitat use: insights for conservation. Ocean Coast. Manag. 179:104836. doi: 10.1016/j.ocecoaman.2019.104836
Glennie, R., Buckland, S. T., and Thomas, L. (2015). The effect of animal movement on line transect estimates of abundance. PLoS One 10:e0121333. doi: 10.1371/journal.pone.0121333
Goldsworthy, S. D. (2018). Effectiveness of an Industry Code of Practice in Mitigating the Operational Interactions of the South Australian Sardine Fishery with Short-Beaked Common Dolphins and the South Australian Sardine Fishery: 2017-18. Report to PIRSA Fisheriesand Aquaculture. South Australian Research and Development Institute (Aquatic Sciences), Adelaide. SARDI Publication No. F2010/000726-9. SARDI Research Report Series No. 1000. Urrbrae: South Australian Research and Development Institute.
Goldsworthy, S. D., Ferguson, G. J., and Ward, T. M. (2019). Assesment of Dolphin Interactions, Effectiveness of Code of Practice and Fishing Behaviour in the South Australian Sardine Fishery: 2018-19. Report to PIRSA Fisheries and Aquaculture. South Australian Research and Development Institute (Aquatic Sciences), Adelaide. F2010/000726-10. SARDI Research Report Series No. 1041. Urrbrae: South Australian Research and Development Institute, 36.
Goldsworthy, S. D., Page, B., Rogers, P. J., Bulman, C., Wiebkin, A., McLeay, L. J., et al. (2013). Trophodynamics of the eastern Great Australian Bight ecosystem: ecological change associated with the growth of Australia’s largest fishery. Ecol. Model. 255, 38–57. doi: 10.1016/j.ecolmodel.2013.01.006
Gowans, S., and Whitehead, H. (1995). Distribution and habitat partitioning by small odontocetes in the Gully, a submarine canyon on the Scotian Shelf. Can. J. Zool. 73, 1599–1608. doi: 10.1139/z95-190
Hamer, D. J., Ward, T. M., and McGarvey, R. (2008). Measurement, management and mitigation of operational interactions between the South Australian Sardine Fishery and short-beaked common dolphins (Delphinus delphis). Biolo. Conserv. 141, 2865–2878. doi: 10.1016/j.biocon.2008.08.024
Hamilton, O. N. P., Kincaid, S. E., Constantine, R., Kozmian-Ledward, L., Walker, C. G., and Fewster, R. M. (2018). Accounting for uncertainty in duplicate identification and group size judgements in mark–recapture distance sampling. Methods Ecol. Evol. 9, 354–362. doi: 10.1111/2041-210x.12895
Hammond, P. S., Bearzi, G., Bjørge, A., Forney, K., Karczmarski, L., Kasuya, T., et al. (2008). Delphinus delphis. The IUCN Red List of Threatened Species 2008: e.T6336A12649851. Available online at: https://dx.doi.org/10.2305/IUCN.UK.2008.RLTS.T6336A12649851.en. (accesses April 06, 2020).
Hammond, P. S., Macleod, K., Berggren, P., Borchers, D. L., Burt, L., Cañadas, A., et al. (2013). Cetacean abundance and distribution in European Atlantic shelf waters to inform conservation and management. Biol. Conserv. 164, 107–122. doi: 10.1016/j.biocon.2013.04.010
Harwood, J. (1999). A risk assessment framework for the reduction of cetacean by-catches. Aquat. Conserv. Mar. Freshw. Ecosyst. 9, 593–599. doi: 10.1002/(sici)1099-0755(199911/12)9:6<593::aid-aqc388>3.0.co;2-1
Harwood, M. B., and Hembree, D. (1987). Incidental Catch of Small Cetaceans in the Offshore Gillnet Fishery in Northern Australian Waters: 1981-1985. Reports of the International Whaling Commission, Vol. 37. Cambridge: International Whaling Commission, 363–367.
Heide-Jørgensen, M. P., Laidre, K. L., Burt, M. L., Borchers, D. L., Marques, T. A., Hansen, R. G., et al. (2010). Abundance of narwhals (Monodon monoceros) on the hunting grounds in Greenland. J. Mamm. 91, 1135–1151. doi: 10.1644/09-mamm-a-198.1
Heppell, S. S., Heppell, S. A., Read, A. J., and Crowder, L. B. (2005). Effects of Fishing on Long-Lived Marine Organisms. Washington, DC: Island Press, 211–231.
Holt, R. S., and Cologne, J. (1987). Factors affecting line transect estimates of dolphin school density. J. Wildlife Manag. 51, 836–843. doi: 10.2307/3801749
Innes, S., Heide-Jørgensen, M. P., Laake, J. L., Laidre, K. L., Cleator, H. J., Richard, P., et al. (2002). Surveys of belugas and narwals in the Canadian High Arctic in 1996. NAMMCO Sci. Publications 4, 169–190. doi: 10.7557/3.2843
Jaramillo-Legorreta, A. M., Cardenas-Hinojosa, G., Nieto-Garcia, E., Rojas-Bracho, L., Thomas, L., Hoef, J. M. V., et al. (2019). Decline towards extinction of Mexico’s vaquita porpoise (Phocoena sinus). R. Soc. Open Sci. 6:190598. doi: 10.1098/rsos.190598
Jefferson, T. A., Fertl, D., Bolaños-Jiménez, J., and Zerbini, A. N. (2009). Distribution of common dolphins (Delphinus spp.) in the western Atlantic Ocean: a critical re-examination. Mar. Biol. 156, 1109–1124. doi: 10.1007/s00227-009-1152-y
Kämpf, J. (2015). Phytoplankton blooms on the western shelf of Tasmania: evidence of a highly productive ecosystem. Ocean Sci. 11, 1–11. doi: 10.5194/os-11-1-2015
Kämpf, J., Doubell, M., Griffin, D., Matthews, R. L., and Ward, T. M. (2004). Evidence of a large seasonal coastal upwelling system along the southern shelf of Australia. Geophys. Res. Lett. 31:L09310. doi: 10.1029/2003gl019221
Kemper, C. M., and Gibbs, S. E. (2001). Dolphin interactions with tuna feedlots at Port Lincoln, South Australia and recommendations for minimising entanglements. J. Cetacean Res. Manag. 3, 283–292.
Kemper, C. M., Tomo, I., Bingham, J., Bastianello, S. S., Wang, J., Gibbs, S. E., et al. (2016). Morbillivirus-associated unusual mortality event in South Australian bottlenose dolphins is largest reported for the Southern Hemisphere. R. Soc. Open Sci. 3:160838–160838. doi: 10.1098/rsos.160838
Kerri, D., and Susan, J. C. (2006). Habitat-based spatial and temporal variability in life history characteristics of female common dolphins Delphinus delphis in the eastern tropical Pacific. Mar. Ecol. Prog. Ser. 318, 277–286. doi: 10.3354/meps318277
Kiszka, J., Heithaus, M., and Wirsing, A. (2015). Behavioural drivers of the ecological roles and importance of marine mammals. Mar. Ecol. Prog. Ser. 523, 267–281. doi: 10.3354/meps11180
Laake, J. L., and Borchers, D. L. (2004). “Methods for incomplete detection at distance zero,” in Advanced Distance Sampling, eds S. T. Buckland, D. R. Anderson, K. P. Burnham, J. L. Laake, D. L. Borchers, and L. Thomas (Oxford: Oxford University Press), 108–189.
Laake, J. L., Calambokidis, J., Osmek, S. D., and Rugh, D. J. (1997). Probability of detecting harbor porpoise from aerial surveys: estimating g(0). J. Wildlife Manag. 61, 63–75. doi: 10.2307/3802415
Lerczak, J. A., and Hobbs, R. C. (1998). Calculating sighting distances from angular readings during shipboard, aerial, and shore-based marine mammal surveys. Mar. Mamm. Sci. 14, 590–598. doi: 10.1111/j.1748-7692.1998.tb00745.x
Lewison, R. L., Crowder, L. B., Read, A. J., and Freeman, S. A. (2004). Understanding impacts of fisheries bycatch on marine megafauna. Trends Ecol. Evol. 19, 598–604. doi: 10.1016/j.tree.2004.09.004
Lewison, R. L., Crowder, L. B., Wallace, B. P., Moore, J. E., Cox, T., Zydelis, R., et al. (2014). Global patterns of marine mammal, seabird, and sea turtle bycatch reveal taxa-specific and cumulative megafauna hotspots. Proc. Natl. Acad. Sci. U.S.A. 111, 5271–5276. doi: 10.1073/pnas.1318960111
López, A., Pierce, G. J., Santos, M. B., Gracia, J., and Guerra, A. (2003). Fishery by-catches of marine mammals in Galician waters: results from on-board observations and an interview survey of fishermen. Biol. Conserv. 111, 25–40. doi: 10.1016/S0006-3207(02)00244-6
Luca, M., Andrew, W., Emer, R., Patricia, R., Andrew, R., Jamie, C., et al. (2009). Population structure of short-beaked common dolphins (Delphinus delphis) in the North Atlantic Ocean as revealed by mitochondrial and nuclear genetic markers. Mar. Biol. 156, 821–834. doi: 10.1007/s00227-008-1120-y
Mackay, A. I., and Goldsworthy, S. D. (2016). Mitigating Operational Interactions with Short-Beaked Common Dolphin (Delphinus delphis): Application of the South Australian Sardine Fishery industry Code of Practice 2016-17. Report to PIRSA Fisheries and Aquaculture. South Australian Research and Development Institute (Aquatic Sciences), Adelaide. SARDI Publication No. F2010/000726-7. SARDI Research Report Series No. 934. Adelaide: South Australian Research and Development Institute.
Mackay, A. I., and Goldsworthy, S. D. (2017). Mitigating Operational Interactions with Short-Beaked Common Dolphin (Delphinus delphis): Application of the South Australian Sardine Fishery industry Code of Practice 2016-17. Report to PIRSA Fisheries and Aquaculture. South Australian Research and Development Institute (Aquatic Sciences), Adelaide. SARDI Publication No. F2010/000726-8. SARDI Research Report Series No. 970. Adelaide: South Australian Research and Development Institute.
Mackay, A. I., Goldsworthy, S. D., and Harrison, P. (2016). Critical Knowledge Gaps: Estimating Potential Maximum Cumulative Anthropogenic Mortality limits of Key Marine Mammal Species to Inform Management. Fisheries Research and Development Corporation and South Australian Research and Development Institute (Aquatic Sciences). Adelaide: South Australian Research and Development Institute.
Mannocci, L., Dabin, W., Augeraud-Véron, E., Dupuy, J.-F., and Ridoux, V. (2012). Assessing the impact of bycatch on dolphin populations: the case of the common dolphin in the eastern north atlantic. PLoS one 7:e32615. doi: 10.1371/journal.pone.0032615
Mannocci, L., Roberts, J. J., Miller, D. L., and Halpin, P. N. (2017). Extrapolating cetacean densities to quantitatively assess human impacts on populations in the high seas. Conserv. Biol. 31, 601–614. doi: 10.1111/cobi.12856
Marçalo, A., Nicolau, L., Giménez, J., Ferreira, M., Santos, J., Araújo, H., et al. (2018). Feeding ecology of the common dolphin (Delphinus delphis) in Western Iberian waters: has the decline in sardine (Sardina pilchardus) affected dolphin diet? Mar. Biol. 165:44. doi: 10.1007/s00227-018-3285-3
Marsh, H., Lawler, I. R., Kwan, D., Delean, S., Pollock, K., and Alldredge, M. (2004). Aerial surveys and the potential biological removal technique indicate that the Torres Strait dugong fishery is unsustainable. Anim. Conserv. 7, 435–443. doi: 10.1017/s1367943004001635
Marsh, H., and Sinclair, D. F. (1989). Correcting for visibility bias in striptransect aerial surveys of aquatic fauna. J. Wildlife Manag. 53, 1017–1024. doi: 10.2307/3809604
Meynier, L., Pusineri, C., Spitz, J., Santos, M. B., Pierce, G. J., and Ridoux, V. (2008). Intraspecific dietary variation in the short-beaked common dolphin Delphinus delphis in the Bay of Biscay: importance of fat fish. Mar. Ecol. Prog. Ser. 354, 277–287. doi: 10.3354/meps07246
Milner-Gulland, E. J., and Akçakaya, H. R. (2001). Sustainability indices for exploited populations. Trends Ecol. Evol. 16, 686–692. doi: 10.1016/S0169-5347(01)02278-9
Moore, J. E., and Merrick, R. (eds) (2011). Guidelines for Assessing Marine Mammal Stocks: Report of the GAMMS III Workshop, February 15 – 18, 2011, La Jolla, California. Dept. of Commerce, NOAA Technical Memorandum NMFS-OPR-47. Washington DC: NOAA.
Neumann, D. R. (2001). Seasonal movements of short-beaked common dolphins (Delphinus delphis) in the north-western Bay of Plenty, New Zealand: Influence of sea surface temperature and El Niño/La Niña. N. Z. J. Mar. Freshw. Res. 35, 371–374. doi: 10.1080/00288330.2001.9517007
Palka, D. L. (2012). Cetacean Abundance Estimates in US Northwestern Atlantic Ocean Waters From Summer 2011 Line Transect Survey US Dept Commer, Northeast Fish Sci Cent Ref Doc. 12–29. Washington DC: NOAA.
Panigada, S., Lauriano, G., Burt, L., Pierantonio, N., and Donovan, G. (2011). Monitoring winter and summer abundance of cetaceans in the pelagos sanctuary (Northwestern Mediterranean Sea) through aerial surveys. PLoS One 6:e22878. doi: 10.1371/journal.pone.0022878
Panigada, S., Lauriano, G., Donovan, G., Pierantonio, N., Cañadas, A., Vázquez, J. A., et al. (2017). Estimating cetacean density and abundance in the Central and Western Mediterranean Sea through aerial surveys: Implications for management. Deep Sea Res. Part II: Top. Stud. Oceanogr. 141(Supplement C), 41–58. doi: 10.1016/j.dsr2.2017.04.018
Parra, G. J., and Cagnazzi, D. (2016). Chapter seven – conservation status of the australian humpback dolphin (Sousa sahulensis) using the IUCN red list criteria. Adv. Mar. Biol. 73, 157–192. doi: 10.1016/bs.amb.2015.07.006
Perrin, W. F., and Reilly, S. (1984). Reproductive parameters of dolphins and small whales of the family Delphinidae. Rep. Int. Whaling Commission 6, 97–133.
Pichler, F. B., and Baker, C. S. (2000). Loss of genetic diversity in the endemic Hector’s dolphin due to fisheries-related mortality. Proc. R. Soc. Lond. Ser. B Biol. Sci. 267, 97–102. doi: 10.1098/rspb.2000.0972
Read, A. J., Drinker, P., and Northridge, S. (2006). Bycatch of marine mammals in U.S. and global fisheries. Conserv. Biol. 20, 163–169. doi: 10.1111/j.1523-1739.2006.00338.x
Reed, J., Harcourt, R., New, L., and Bilgmann, K. (2020). Extreme effects of extreme disturbances: a simulation approach to assess population specific responses. Front. Mar. Sci. 7:519845. doi: 10.3389/fmars.2020.519845
Reeves, R. R., McClellan, K., and Werner, T. B. (2013). Marine mammal bycatch in gillnet and other entangling net fisheries, 1990 to 2011. Endanger. Species Res. 20, 71–97. doi: 10.3354/esr00481
Robbins, W. D., Huveneers, C., Parra, G. J., Möller, L., and Gillanders, B. M. (2017). Anthropogenic threat assessment of marine-associated fauna in Spencer Gulf, South Australia. Mar. Policy 81, 392–400. doi: 10.1016/j.marpol.2017.03.036
Salgado Kent, C., Jenner, K., Jenner, M., Bouchet, P., and Rexstad, E. (2012). Southern hemisphere breeding stock ‘D’ humpback whale population estimates from North West Cape, Western Australia. J. Cetacean Res. Manag. 12, 29–38.
SEWPaC. (2012). Assessment of The Commonwealth Small Pelagic Fishery”. Australian Government Department of Sustainability, Environment, Water, Population and Communities. Canberra: Australian Government Department of Sustainability.
Shaughnessy, P., Kirkwood, R., Cawthorn, M., Kemper, C., and Pemberton, D. (2003). “Pinnipeds, cetaceans and fisheries in Australia: a review of operational interactions,” in Marine Mammals: Fisheries, Tourism and Management Issues, eds N. J. Gales, M. A. Hindell, and R. Kirkwood (Melbourne: CSIRO Publishing), 136–152.
Slooten, E., and Dawson, S. (2008). Sustainable levels of human impact for Hector’s Dolphin. Open Conserv. Biol. J. 2, 37–43. doi: 10.2174/1874839200802010037
Slooten, E., Dawson, S. M., and Rayment, W. J. (2004). Aerial surveys for coastal dolphins: abundance of Hector’s dolphins off the South Island west coast. N. Z. Mar. Mamm. Sci. 20, 477–490. doi: 10.1111/j.1748-7692.2004.tb01173.x
Strindberg, S., and Buckland, S. T. (2004). “Design of distance sampling surveys and geographic information systems,” in Advanced Distance Sampling: Estimating Abundance of Biological Populations, eds S. T. Buckland, D. R. Anderson, K. P. Burnham, J. L. Laake, D. L. Borchers, and L. Thomas (New York NY: Oxford University Press), 190–228.
Sucunza, F., Danilewicz, D., Andriolo, A., Azevedo, A. F., Secchi, E. R., and Zerbini, A. N. (2020). Distribution, habitat use, and abundance of the endangered franciscana in southeastern and southern Brazil. Mar. Mammal Sci. 36, 421–435. doi: 10.1111/mms.12650
Taylor, B. L., Martinez, M., Gerrodette, T., Barlow, J., and Hrovat, Y. N. (2007). Lessons from monitoring trends in abundance of marine mammals. Mar. Mammal Sci. 23, 157–175. doi: 10.1111/j.1748-7692.2006.00092.x
Taylor, B. L., Rojas-Bracho, L., Moore, J., Jaramillo-Legorreta, A., Ver Hoef, J. M., Cardenas-Hinojosa, G., et al. (2017). Extinction is Imminent for Mexico’s endemic porpoise unless Fishery Bycatch is Eliminated. Conserv. Lett. 10, 588–595. doi: 10.1111/conl.12331
Taylor, B. L., Wade, P. R., De Master, D. P., and Barlow, J. (2000). Incorporating uncertainty into management models for marine mammals. Conserv. Biol. 14, 1243–1252. doi: 10.1046/j.1523-1739.2000.99409.x
Thomas, L., Buckland, S. T., Rexstad, E. A., Laake, J. L., Strindberg, S., Hedley, S. L., et al. (2010). Distance software: design and analysis of distance sampling surveys for estimating population size. J. Appl. Ecol. 47, 5–14. doi: 10.1111/j.1365-2664.2009.01737.x
Thomas, L., Laake, J. L., Rexstad, E., Strindberg, S., Marques, F. F. C., Buckland, S. T., et al. (2009). Distance 6.0. Release 2. Research Unit for Wildlife Population Assessment. Scotland: University of St. Andrews.
Tulloch, V., Pirotta, V., Grech, A., Crocetti, S., Double, M., How, J., et al. (2020). Long-term trends and a risk analysis of cetacean entanglements and bycatch in fisheries gear in Australian waters. Biodiversity Conserv. 29, 251–282. doi: 10.1007/s10531-019-01881-x
Turvey, S. T., Pitman, R. L., Taylor, B. L., Barlow, J., Akamatsu, T., Barrett, L. A., et al. (2007). First human-caused extinction of a cetacean species? Biol. Lett. 3, 537–540. doi: 10.1098/rsbl.2007.0292
Wade, P. R. (1998). Calculating limits to the allowable human-caused mortality of cetaceans and pinnipeds. Mar. Mamm. Sci. 14, 1–37. doi: 10.1111/j.1748-7692.1998.tb00688.x
Wade, P. R., Watters, G. M., Gerrodette, T., and Reilly, S. B. (2007). Depletion of spotted and spinner dolphins in the eastern tropical Pacific: modeling hypotheses for their lack of recovery. Mar. Ecol. Prog. Ser. 343, 1–14. doi: 10.3354/meps07069
Ward, T. M., Ivey, A., and Burch, P. (2011). Effectiveness of an Industry Code of Practice in Mitigating the Operational Interactions of the South Australian Sardine Fishery With Short-Beaked Common Dolphin (Delphinus delphis). Report to PIRSA Fisheriesand Aquaculture. South Australian Research and Development Institute (Aquatic Sciences), Adelaide. SARDI Publication No. F2010/000726-2. SARDI Research Report Series No. 583. Adelaide: South Australian Research and Development Institute.
Ward, T. M., Ivey, A., and Burch, P. (2012). Effectiveness of an Industry Code of Practice in Mitigating the Operational Interactions of the South Australian Sardine Fishery With Short-Beaked Common Dolphin (Delphinus delphis). Report to PIRSA Fisheriesand Aquaculture. South Australian Research and Development Institute (Aquatic Sciences), Adelaide. SARDI Publication No. F2010/000726-3. SARDI Research Report Series No. 666. Adelaide: South Australian Research and Development Institute, 27.
Ward, T. M., Ivey, A., and Burch, P. (2013). Effectiveness of an Industry Code of Practice in Mitigating the Operational Interactions of the South Australian Sardine Fishery with Short-Beaked Common Dolphin (Delphinus delphis). Report to PIRSA Fisheriesand Aquaculture. South Australian Research and Development Institute (Aquatic Sciences), Adelaide. SARDI Publication No. F2010/000726-4. SARDI Research Report Series No. 743. Adelaide: South Australian Research and Development Institute, 32.
Ward, T. M., Ivey, A., and Carroll, J. (2018). Code of practice for reducing accidental mortality of dolphins in purse-seine fisheries. Mar. Policy 87, 203–211. doi: 10.1016/j.marpol.2017.10.032
Ward, T. M., Ivey, A., Hamer, D. J., and Burch, P. (2010). Effectiveness of an Industry Code of Practice in Mitigating the Operational Interactions of the South Australian Sardine Fishery With Short-Beaked Common Dolphin (Delphinus delphis). Report to PIRSA Fisheriesand Aquaculture. South Australian Research and Development Institute (Aquatic Sciences), Adelaide. SARDI Publication No. F2010/000726-1. SARDI Research Report Series No. 493. Adelaide: South Australian Research and Development Institute.
Ward, T. M., Ivey, A. O. B., and Carroll, J. K. (2015a). Effectiveness of the Industry Code of Practice in Mitigating Operational Interactions of the South Australian Sardine Fishery with the Short-Beaked Common Dolphin (Delphinus delphis). Report to PIRSA Fisheries and Aquaculture. South Australian Research and Development Institute (Aquatic Sciences), Adelaide. SARDI Publication No. F2010/000726-5. SARDI Research Report Series No. 821. Adelaide: South Australian Research and Development Institute.
Ward, T. M., Ivey, A. O. B., and Carroll, J. K. (2015b). Effectiveness of the Industry Code of Practice in Mitigating Operational Interactions of the South Australian Sardine Fishery with the Short-Beaked Common Dolphin (Delphinus delphis). Report to PIRSA Fisheries and Aquaculture. South Australian Research and Development Institute (Aquatic Sciences), Adelaide. SARDI Publication No. F2010/000726-6. SARDI Research Report Series No. 876. Adelaide: South Australian Research and Development Institute.
Ward, T. M., Mcleay, L. J., Dimmlich, W. F., Rogers, P. J., Mcclatchie, S., Matthews, R., et al. (2006). Pelagic ecology of a northern boundary current system: effects of upwelling on the production and distribution of sardine (Sardinops sagax), anchovy (Engraulis australis) and southern bluefin tuna (Thunnus maccoyii) in the Great Australian Bight. Fish. Oceanogr. 15, 191–207. doi: 10.1111/j.1365-2419.2006.00353.x
Ward, T. M., Smart, J., and Ivey, A. (2017). Stock assessment of Australian Sardine (Sardinops sagax) off South Australia 2017. Report to PIRSA Fisheries and Aquaculture. South Australian Research and Development Institute (Aquatic Sciences), Adelaide. SARDI Publication No. F2007/000765-6. SARDI Research Report Series No. 971. Adelaide: South Australian Research and Development Institute, 107.
Keywords: dolphins, Delphinus delphis, aerial survey, distance sampling, fishery interactions, bycatch, potential biological removal, conservation
Citation: Parra GJ, Bilgmann K, Peters KJ and Möller LM (2021) Abundance and Potential Biological Removal of Common Dolphins Subject to Fishery Impacts in South Australian Waters. Front. Mar. Sci. 8:617075. doi: 10.3389/fmars.2021.617075
Received: 13 October 2020; Accepted: 10 August 2021;
Published: 09 September 2021.
Edited by:
Diego Horacio Rodriguez, Consejo Nacional de Investigaciones Científicas y Técnicas (CONICET), ArgentinaReviewed by:
Elisabeth Slooten, University of Otago, New ZealandPhil J. Bouchet, University of St Andrews, United Kingdom
Copyright © 2021 Parra, Bilgmann, Peters and Möller. This is an open-access article distributed under the terms of the Creative Commons Attribution License (CC BY). The use, distribution or reproduction in other forums is permitted, provided the original author(s) and the copyright owner(s) are credited and that the original publication in this journal is cited, in accordance with accepted academic practice. No use, distribution or reproduction is permitted which does not comply with these terms.
*Correspondence: Guido J. Parra, guido.parra@flinders.edu.au