- 1Commonwealth Scientific and Industrial Research Organisation (CSIRO) Oceans and Atmosphere, St. Lucia, QLD, Australia
- 2Commonwealth Scientific and Industrial Research Organisation (CSIRO) Oceans and Atmosphere, Crawley, WA, Australia
Knowledge of the movement patterns of the lemon shark Negaprion acutidens is poor in contrast to the allopatric N. brevirostis. Using acoustic telemetry, we investigated daily (diel and tidal) and seasonal patterns in residency, fidelity, home range, habitat preference, and migratory patterns along the Ningaloo coast, Australia. Thirty eight adult N. acutidens were monitored for up 6.1 years (mean 2.5 y) with 19 animals detected for more than 3 years and 5 for more than 5 y. Approximately 50% of the tagged animals remained within 10 km of their tagging location for more than 12 months (average core home range of 1.7 km2). Surprisingly, residency of adults was greater than juveniles in this known nursery area. Adults showed a strong preference for lagoon habitats and moved into shallow nearshore habitats at high tide and at night. During winter months, female sharks shifted their core home range 0.45 km further offshore into deeper lagoon areas, a shift which is likely due to behavioral thermoregulation. Space use by males and females within core areas was asynchronous indicating sexual segregation by resident sharks. Both resident and non-resident sharks were detected up to 140 km away. These highly directional (southerly) and rapid movements (140 km in 2 days) were largely correlated with the parturition and mating periods with males departing 1–2 months earlier than females. In females, periodicity of migrations was variable with evidence of annual and biannual patterns. Negaprion acutidens are highly susceptible to over-fishing and movement data are essential to quantify spatio-temporal overlap with fisheries and assist with developing spatially explicit stock assessment models.
Introduction
In order to mitigate anthropogenic impacts such as habitat modification or loss, fishing and long term changes to climate, we need to understand how animals use available space and how much space they require. Understanding the spatial and temporal patterns of animal movement is critical to understanding the mechanisms and ecological process that underpin space use. Key parameters of movement include when and for how long animals go to a particular place (residence), how much space they occupy (home range), repeated use (site fidelity/philopatry), and the timing, direction and distance of migrations (Bestley et al., 2013; Kessell et al., 2014; Heupel and Simpfendorfer, 2015).
Spatial management is used increasingly in conjunction with fisheries management to project populations of marine species (Babcock et al., 2010). Data on how environmental factors such as tide (Pillans et al., 2009; Lyon et al., 2017), temperature (Bestley et al., 2013; Kessell et al., 2014), salinity (Pillans et al., 2020) as well biological factors such as food availability (Sims et al., 2006) and mating and reproduction (Mourier et al., 2013; Chapman et al., 2015; Heupel and Simpfendorfer, 2015) are therefore required to understand how management can be tailored to maximize effectiveness.
It is well-established that the proportion of the population offered highest levels of protections is positively correlated with size of spatial closures and inversely correlated with species mobility (Kramer and Chapman, 1999; Gerber et al., 2002; Heupel and Simpfendorfer, 2005). Less is known about inter-individual variation, especially for species which exhibit multiple types of movement patterns such as partial migration (Jonsson and Jonsson, 1993) and residence versus nomadism which will influence how much time is spent outside of the core area or protected area (Martin et al., 2007; Reyier et al., 2014; Runge et al., 2014).
Elasmobranchs are more susceptible to overfishing than teleosts due to slow growth, late age at maturity and limited reproductive output (Stevens et al., 2000) with a recent assessment showing that more than one quarter of the world's elasmobranch species are threatened by overfishing (Dulvy et al., 2014). Large-bodied, coastal species are at the highest risk of extinction (Dulvy et al., 2014). The Lemon Shark, Negaprion acutidens is a coastal shark characterized by slow growth rates, large size at maturity and limited reproductive potential (Pillans, 2003). It is widely distributed in the Indian and western Central Pacific Oceans, extending from South Africa to French Polynesia (Last and Stevens, 2009). Within Australia, it is found from Moreton Bay (Queensland) along the northern coast to the Abrolhos Islands off western Australia (Last and Stevens, 2009). Limited movement (based on data from the allopatric N. brevirostus), combined with intensive coastal gillnet and line fisheries are thought to be responsible for significant declines of N. acutidens in parts of Southeast Asia. These population declines and possible localized extirpations (e.g., India, Indonesia, and Thailand) resulted in N. acutidens being assessed as Endangered in Southeast Asia and Vulnerable globally under the IUCN Red List (Pillans, 2003). Within Australia, small catches combined with limited overlap with the species extensive range in northern waters resulted in the species being assessed as Least Concern (Pillans, 2003).
In contrast to the allopatric N. brevirostrus, there are few data on the biology, movement and habitat use of the N. acutidens. The biology and ecology of juvenile N. brevirostrus is well-documented with research in the western Atlantic having been conducted over the past four decades (e.g., Gruber, 1982, Feldheim et al., 2001a, 2002; Kessell et al., 2014; Chapman et al., 2015). In contrast, ecological studies of adult N. brevirostris are relatively sparse (Feldheim et al., 2014; Kessell et al., 2014). In Australia and the Indo west Pacific, research on N. acutidens has been limited to juvenile movement (Speed et al., 2011, 2012, 2016; Oh et al., 2017), diet (White and Potter, 2004), and trophic ecology (Speed et al., 2012). Although sample size and study duration were limited, research in Australia and the Indian Ocean have demonstrated limited movement and high residence of juvenile animals in both oceanic and coastal systems (Stevens, 1984; Speed et al., 2011, 2016).
The Ningaloo Marine Park (NPM) encompasses the entire Ningaloo Reef covering 2,633 km2 and spans ~ 300 km of coastline and fringing reef (DEC, 2005) and is located within the Ningaloo Coast World Heritage Area. More than 34% of the park is zoned as “sanctuary zones” where all forms of fishing are prohibited with 18 sanctuary zones ranging in size from 0.008 to 44.7 km2. The NMP is also located within an area in which commercial shark fishing is prohibited; this are stretches from 26°30'S to 18°S. Ningaloo Reef is known for both high diversity and abundance of elasmobranchs including nursery areas for N. acutidens (Stevens et al., 2009; Speed et al., 2011; Vanderklift et al., 2014, 2020; Oh et al., 2017), and provides an ideal environment to investigate movement ecology in elasmobranch populations not adversely impacted by overfishing.
To better understand how adult N. acutidens move around and use coastal ecosystems and assess the implications of movement for spatial management, we used transmissions from 38 tagged individuals detected by an array of 77 acoustic receivers as well as another array of 44 receivers, and three sets of 7–14 receivers arranged in lines from the shoreline to 200 m depth spanning 150 km of coastline. We used these data to quantify residence, home range, and migration. The following research questions were articulated: (1) What proportion of the adult population is resident, how much space do individuals utilize and to what extent do animals roam within the ~28 km2 Mangrove Bay array? (2) Are there sex- and size-related patterns in residence, home range, extent of roaming? (3) To what extent does tide, time of day and season influence movement? (4) What are the preferred habitats of adult N. acutidens? And (5) Do adult N. acutidens undertake seasonal migrations and if so, is there an annual or biennial cycle to migration what are the potential drivers of movement (environmental or biological).
Methods
Study Area
The study was conducted within the Ningaloo Marine Park, which is in turn located within the Ningaloo Coast World Heritage Area. Ningaloo is a fringing reef that stretches ~300 km, with a shallow (usually <5 m deep) lagoon up to 6 km wide that is predominantly sand and low relief limestone. Seaward of the lagoon is a reef flat (usually < 150 m wide) punctuated by wide passages, and a reef slope to ~30–35 m depth. Mangrove Bay is a small, mostly intertidal (<1.5 m at Highest Astronomical Tide) embayment, surrounded by a lagoon that is up to 2 km wide. Sea surface temperatures are lowest in August-September, and highest in March-April (Vanderklift et al., 2020).
Acoustic Tagging
Lemon sharks (n = 41) were captured using single circle-hook lines from an anchored vessel between 2012 and 2017. Hooked sharks were brought alongside the vessel and maneuvered into a rubber sling attached to a davit. Sharks were then lifted above the water to facilitate measurements, sampling and tagging. Individuals were sexed by visual inspection of the presence or absence of claspers, measured (fork and total length, in centimeters) and females that were heavily pregnant, post-partum or had fresh mating scars were recorded. Animals chosen for acoustic tagging had a Vemco coded tag (V16–4H or V16–6H) surgically implanted into the peritoneal cavity. The nominal delay of tags was 120 s (range of 60–180 s) and battery life was either 7 or 10 y (V16–4H and V16–6H, respectively). Vemco VR2W receivers were downloaded every 12 months throughout the study and the batteries were changed when downloaded. All tagging was conducted under CSIRO animal ethics permit number: 15-03.
All sharks were tagged within the Mangrove Bay array (Figure 1). A small incision (15 mm long) was made with a scalpel in the ventral mid line between the pectoral and anal fins. A single acoustic tag (16 mm diameter 68 mm long) was then placed into the peritoneal cavity and the wound closed by 2–3 dissolving sutures. Prior to field work, internal tags were sterilized in a betadine bath for 30 min and held in a sterile autoclave paper bag. All surgical procedures were undertaken with disposable scalpels and suture packs. All other surgical equipment (forceps, needle holders, surgical scissors and scalpel holder) was stored in a waterproof container in a Hexawash solution (1% Hexacon, 10% Ethanol and 89% distilled water). This entire procedure from restraining to release normally took 4–5 min. The animals were immediately returned to the water and gently held until they swam off vigorously.
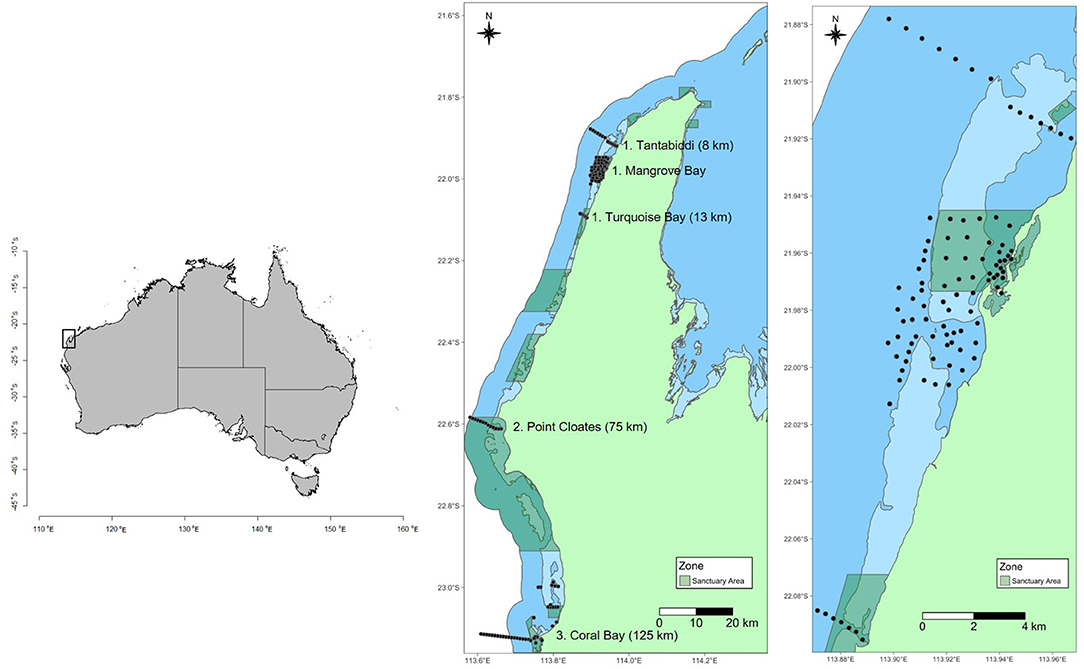
Figure 1. Map showing location of groups of receivers along the Ningaloo Reef. Dark blue shading is Ningaloo Marine Park boundary. Light blue shading shows intertidal areas (<2 m at Lowest Astronomical Tide –LAT). Green shading shows sanctuary zones (no fishing or other extractive activities allowed). Black circles show location of acoustic receivers from Tantabiddi to Coral Bay. Number in brackets next to site names is the distance in kilometers from the center of the Mangrove Bay array and the center of the site group.
Acoustic Array
Acoustic receivers spanned about 150 km of the coast and consisted of five discrete site groups. Receivers have been in place since 2012 with data from animals tagged in this study collected between 30 August 2012 and 14 October 2018. From north to south, these groups consisted of 14 (Tantabiddi), 77 (Mangrove Bay), seven (Turquoise Bay), 13 (Point Cloates), and 44 receivers (Coral Bay) (Figure 1). A dense array of acoustic receivers was located within and adjacent to the Mangrove Bay Sanctuary Zone. This array extended from <1 m water depth near the shoreline to >50 m water depth beyond the reef slope (Figure 1). Receivers were spaced 200–800 m apart and detection ranges generally did not overlap [for a detailed description, see Pillans et al. (2014)]. The array encompassed the following habitats: a mangrove-lined shallow embayment, shoreline reefs (consolidated pavement reef with some macroalgae), algae-dominated pavement reef (predominantly Sargassum spp. and other fucalean algae), extensive sandy areas within the lagoon, patch reefs that included large Porites spp. >8 m diameter and extensive coral reef flats <3 m dominated by Acropora spp., reef pass, shallow reef slope (<20 m and dominated by live coral and pavement), deep reef slope (>20 m deep and primarily sand). A near-continuous fringing reef creates a barrier to movement out of the lagoon at low tide and during times of high swell, but an adjacent reef pass provides direct access for animals to deeper reef slope waters. The reef slope consists of coral-dominated spur-and-groove habitat and limestone reef interspersed with sand. Beyond 35 m depth, the substratum is predominantly sandy sediment with occasional low relief limestone reef (Vanderklift et al., 2020).
Analysis of Acoustic Tag Detection Data
Analysis of acoustic data was approached in two ways. First, data from the array of receivers at Mangrove Bay (77 receivers covering about 28 km2) were used to investigate residence, roaming, home range area and habitat use and how they varied between males and females, day and night, high and low tide and seasons. Second, data from the five groups of receivers along the Ningaloo coastline (155 receivers) were used to investigate the spatial extent and timing of longer north-south movements among these site groups (using indices of movement range, utilization of multiple site groups and utilization of southern sites).
Detection Parameters and Home Range Estimates
The number of daily detections over time (using data from all 155 receivers) for each individual was plotted to provide an overview of detection span and detection frequency for animals tagged. Using detections from all receivers, the total number of detections and number of sites (acoustic receivers) at which individuals were detected were also calculated. The detection span of each individual was calculated as the number of days from the first detection to last detection, whereas days detected was the total number of days on which each individual was detected. The percentage Mangrove Bay array detections was the number of detections in Mangrove Bay (77 receivers) divided by total number of detections expressed as a percentage. The residence index (RI) was calculated as the total days detected divided by the number of days between tagging and the last detection. RI was calculated using all detections as well as just detections in Mangrove Bay. Roaming index for the Mangrove Bay array was the percentage of receiver sites in the array at which a tag was detected.
Roaming index is sensitive to detection span, which varied among individuals in our data, because it increases as an animal visits new sites (even briefly) over time. To reduce that effect, we calculated an “effective roaming index” using the methods that are used for species diversity indices. Effective roaming index for the Mangrove Bay array was the effective number of sites at which a tag was detected expressed as a percentage of the total number of receiver sites in the array. Effective number of sites was calculated as exp(H), where H is the Shannon diversity of visited sites:
Here, pi is the proportion of detections at site i and S is the number of sites at which the tag was detected. Effective number of sites was therefore equal to the effective number of species index that is used for species diversity (Jost, 2006), with sites analogous to species. For an array of ST sites (S ≤ ST), it varies from 1 (when the tag was detected at one site only) to ST (when the tag was detected at all sites and the same number of times at each site). Effective roaming index [exp(H) / ST × 100] can be interpreted as roaming index penalized by how unevenly the individual shares its visits among sites.
The use of space by the sharks in the Mangrove Bay array was estimated using the kernel utilization distribution (KUD), which is a probability distribution that provides the probability density of an individual's occurrence at each point in space (Worton, 1989). It is calculated from a set of locations at which the individual has been observed (in this case represented by the number of detections by each acoustic receiver). The distribution is the average of bivariate normal distributions centered on each location. The component distributions all share the same standard deviation (the KUD bandwidth). To account for simultaneous detections at multiple receivers, detections were binned on 20-min intervals, the location for each interval being the weighted average of the receiver latitudes and longitudes, with the weights being the number of detections by each receiver. The KUD bandwidth was set to 200 m based on range test data from stationary tags [see Pillans et al. (2017)]. The output grid resolution was 50 m. KUD area (50 and 95%) was calculated using the adehabitatHR package (Calenge, 2011) in R (version 3.6.1, R Foundation for Statistical Computing, Vienna, Austria). To provide a general index of the area used by an animal and how evenly it roamed over that area, we calculated effective KUD area from the KUD using the same formula as for effective number of sites (using grid cells in place of sites).
KUDs were calculated for 31 sharks detected on two or more receivers and for more than 30 days. For each individual, KUDs were calculated for the entire detection span of the animal using all detections, and separately for day and night and high/low tide. Day and night periods were calculated using the “maptools” package in R (version 3.6.1). Periods of high and low tide were defined as being within 1 h of high and low tide and when tide height was within the long-term upper (high tide) or lower (low tide) quartile (to exclude days of low amplitude). Hourly model predictions of tide height for Exmouth were obtained from the Western Australian Department of Transport.
Differences between the sexes, between day and night and between high and low tide in residence index, effective KUD area etc. were tested with Wilcoxon rank sum tests. Correlation with size (total length) was tested using Pearson correlation tests.
To examine regular intra-annual patterns in space utilization, monthly KUDs were also calculated for each animal. Monthly average distance from the coast was estimated as the weighted average distance from the coast of the KUD grid cell centers, with the weights being the KUD grid cell probabilities. Seasonal variation of residence index, effective KUD area and KUD distance from the coast was estimated with generalized additive models (GAMs) of the form:
where y is the variable of interest (e.g., KUD area), μ is the intercept, as is the fixed effect for sex s, f s(m) is a cyclic GAM spline term for month for sex s, ⋎t is the random effect for individual t and ε is the residual error (i.e., the month effect was tested separately for each sex). Tags were included if overall they had detections spanning at least 4 months of the year (to reduce misallocation of variance between the month and random effect terms). Additionally, for KUD area and distance from the coast, the monthly KUDs had to be based on at least 30 detections and 5 detection days. Models were fitted with the R mgcv package (Wood, 2017).
Temperature differences along a longitudinal gradient within the Mangrove Bay array were examined using data collected every 30 min on VEMCO Minilog temperature loggers attached to 10 acoustic receivers within the array from 2007 to 2009. Data from two – four loggers within the lagoon (water depth 0.5 – 5 m) were used to obtain average monthly temperatures at increasing distance from the shore (0.15 km, n = 2; 0.22 km, n = 2; 1.04 km, n – 4; 2.95 km, n = 2).
Habitat Use Within Mangrove Bay
To determine habitat use of N. acutidens, habitat types within the Mangrove Bay array were classified based on aerial imagery and a spatial habitat database collected over 10 years of extensive surveys (Pillans et al., 2017). Habitat polygons were generated manually in Google Earth. Habitat utilization was calculated as the proportion of the KUD within each habitat (i.e., the sum of the KUD grid cell probabilities within the polygons of the habitat). The total area of each habitat within the study area was calculated as the total area of the habitat within the extent of the acoustic array buffered by 700 m. Ivlev's electivity (E; Ivlev, 1961) was calculated as E = (ri – pi) / (ri + pi), where ri is the utilization of habitat i and pi is the proportion of the study area classified as that habitat. The electivities for the individual sharks were summarized as the average and bootstrap confidence interval for each habitat (confidence intervals were 95% BCa intervals calculated with the R boot package). The scale for electivity values is−1 to 1 with values around zero suggesting neither selection or avoidance. Decreasing negative values indicate avoidance and increasing positive values indicate increasing selection/preference for a habitat.
Seasonal Movements among Site Groups
Seasonal movements among and beyond the site groups (groups of receiver sites) were examined with monthly indices of movement range, roaming among site groups and southern utilization. Maximum linear distance was the maximum distance between detections in each month. Indices of site group utilization were based on the proportion of detections in each of three site groups, which were formed by combining the five site groups as follows: (1) Tantabiddi, Mangrove Bay and Turquoise Bay combined; (2) Point Cloates and (3) Coral Bay. To partially reduce bias toward site groups with more receivers (and therefore higher detection rates during periods of residence), the proportions were first calculated daily then averaged over the month. Roaming among site groups was indicated by the effective number of site groups (range 1–3), calculated using the above method based on Shannon diversity for effective number of sites (receivers). Southern utilization was indicated by the sum of the site group utilisations for the southern site groups (Point Cloates and Coral Bay).
For an alternative index of long distance roaming, we considered using roaming index (and effective roaming index) calculated as above for Mangrove Bay but using all receiver sites in all site groups. However, this index was not informative because it confounded roaming within and between site groups and was driven by roaming within the Mangrove Bay array (e.g., it was positively correlated with residency and roaming at Mangrove Bay and weakly negatively correlated with roaming among site groups).
Seasonal variation was estimated with GAMs of the form described above for Mangrove Bay. Again, tags were included if overall they had detections spanning at least 4 months of the year. Variation in site group utilization is only possible for individuals that move among site groups. Hence, for effective number of site groups, tags were included if their entire detection series included at least two site groups. Similarly, for southern utilization, tags were included if their entire detection series included at least one of the southern site groups.
Timing of Return Migrations
To examine differences in the timing of migration and return of resident animals we calculated departure days, days absent, and days resident. Resident animals were those that were detected in receivers within the Mangrove Bay array for more than 30 days. A departure was defined as an animal that was not detected for 14 days to ensure that brief movements outside of the array were not classified as a migration. Departure day was the number of days between an individual departing the Mangrove Bay Array since 1 January in the year of departure with the range of values between 0 (animal did not depart) or 365 (departed on 31 December). Days absent was the number of days between when an animal departed and then returned to the Mangrove Bay array. Return was classified as more than 14 days of detections on more than one receiver. Days resident was the number of days between returning and departing. These metrics were calculated for each individual in each year with days absent usually spanning 2 calendar years (i.e., animal departed in November and returned in June the following year).
Results
Detection Histories, Residence, and Migration
Forty-one N. acutidens (23 F, 18 M) were tagged within the Mangrove Bay Array and monitored by acoustic receivers between August 2012 and the October 2018 (Figure 2). The sex ratio of all animals captured (including those not tagged) was biased toward females (F:M = 1.7:1). The majority (n = 38) of tagged sharks were mature adults with only three sub-adult sharks tagged (Table 1). Three sub-adult sharks were excluded from spatial and temporal analysis. Adult male and female sharks were between 234 – 266 and 240 – 280 cm TL, respectively.
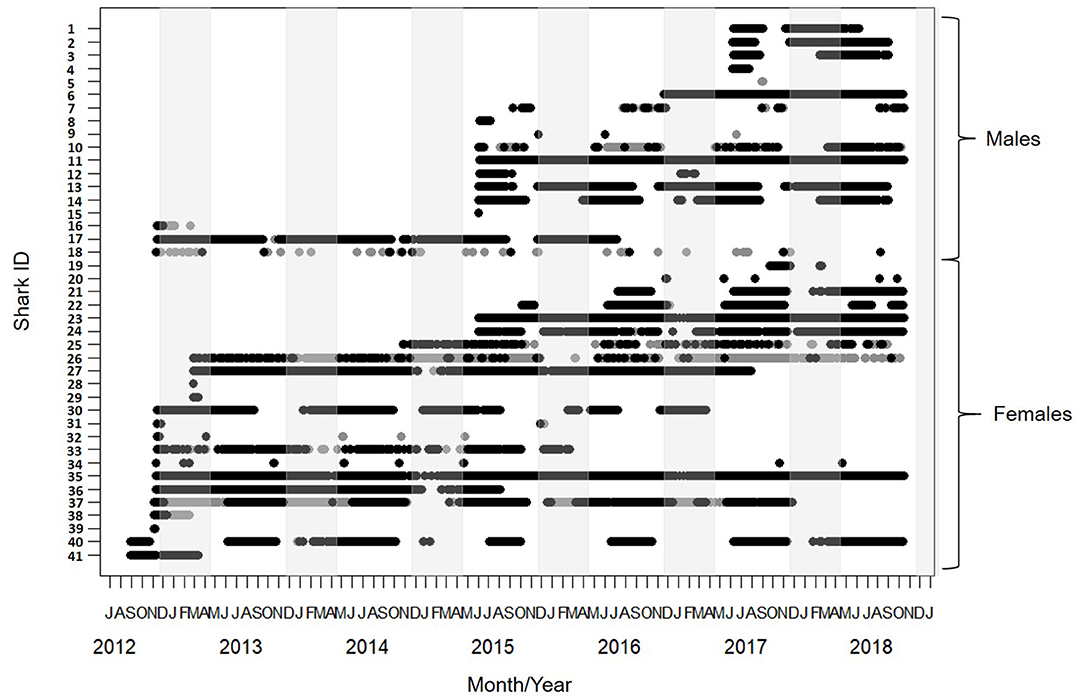
Figure 2. Detection span of 41 lemon sharks tagged between August 2012 and October 2018. Black circles show detections on receivers only within the Mangrove Bay array. Gray circles show detections on receivers outside the Mangrove Bay array. Males (Tag ID 1 - 18) and females (Tag ID 19 - 41) plotted separately. Light gray shading covers the period between November and April when parturition is thought to occur. Fresh mating scars were observed in November.
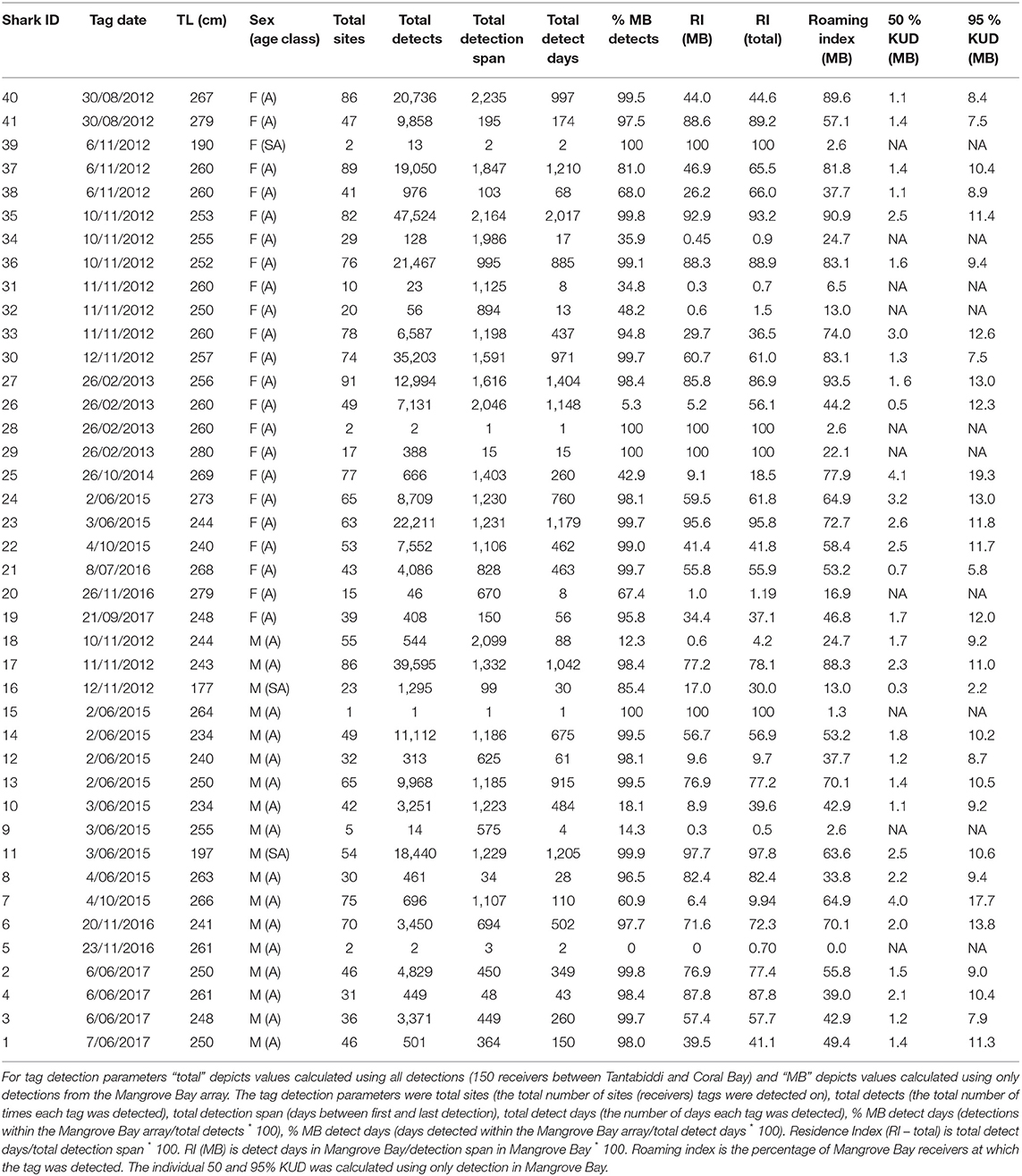
Table 1. Summary of individual tag identification (Tag ID), tag date, Total length [TL (cm)], sex and age class (A = adult, SA = sub-adult).
Tagged sharks were detected on 1–91 receivers with individuals detected 1–47,524 times (Table 1). The VEMCO acoustic tagging system can generate false detections, though only in specific circumstances and then rarely and randomly (Simpfendorfer et al., 2015). If the transmissions from two or more tags detected by a receiver overlap (transmission time is 3–5 s), then generally no detection will be recorded, but occasionally a detection for a semi-random incorrect tag ID will be recorded (Simpfendorfer et al., 2015). Such false detections are more likely when there are more tags within range of a receiver. They are problematic only when they match the IDs of tags deployed in the study, which is more likely when the deployed tags share similar IDs (as some of ours did). False detections would have been rare in our study given that distinct detections were mostly spaced beyond the transmission period of 3–5 s (e.g., 4.3% of detections were within 10 seconds of the previous detection of any tag by the same receiver). Most of our analyses (e.g., KUD analysis) were not sensitive to small numbers of false detections. The exception was the analysis of long-distance movements to the southern site groups (Point Cloates and Coral Bay); however, false detections would have been very rare at these locations, where 0.02 and 0.1% of detections were within 10 seconds of the previous detection respectively (presumably due to fewer resident tags, e.g., because our tags were all deployed at Mangrove Bay). Filtering out isolated detections in this case is likely to have removed more true detections than false detections and significantly reduced the information content of the data because about 20% of visits to site groups (e.g., transits through linear arrays) were detected by single detections. Further, false detections have less effect on comparisons between seasons or sexes, which were the focus of our analysis.
The detection span ranged between 1 and 2,235 days (mean = 911) with individuals detected for as many as 2017 days during the monitoring period (Table 1). The majority of detections were on receivers within the Mangrove Bay array (mean 79%, IQR 63–99%), however individuals were not always resident within the area encompassed by the array and there were periods of up to 1,096 days when individuals were not detected (Figures 2, 3). Four sharks (two males, two females) were detected continuously at Mangrove Bay for longer than 600 days and were classified as permanent residents (Figure 2). However, most (n = 37) sharks were not permanent residents within the array and were often not detected for periods up to several months before returning (Figure 2). Seven sharks were transients, that is they moved along the coast and were detected infrequently by receivers at annual or bi-annual intervals. These individuals [Shark ID 9 (M), 32 (M), 20 (F), 31 (F), 32 (F), 34 (F), and 18 (M)] were all tagged in October/November. One of these, a male (18), was mainly detected on receivers at Coral Bay and Point Cloates and infrequently at northern sites. Twenty one sharks (12 F, 9 M) showed both strong site fidelity (returning to similar location within the array following migration) and repeated seasonal migrations. These sharks were resident within Mangrove Bay or other groups of receivers for periods of time throughout the years they were detected but disappeared for several months before returning or disappearing permanently. Three animals (1 F, 2 M) were only detected for a few days after tagging and not detected again and three animals (1 F, 2 M) were detected for up to 6 months and not detected again (Table 1, Figure 2).
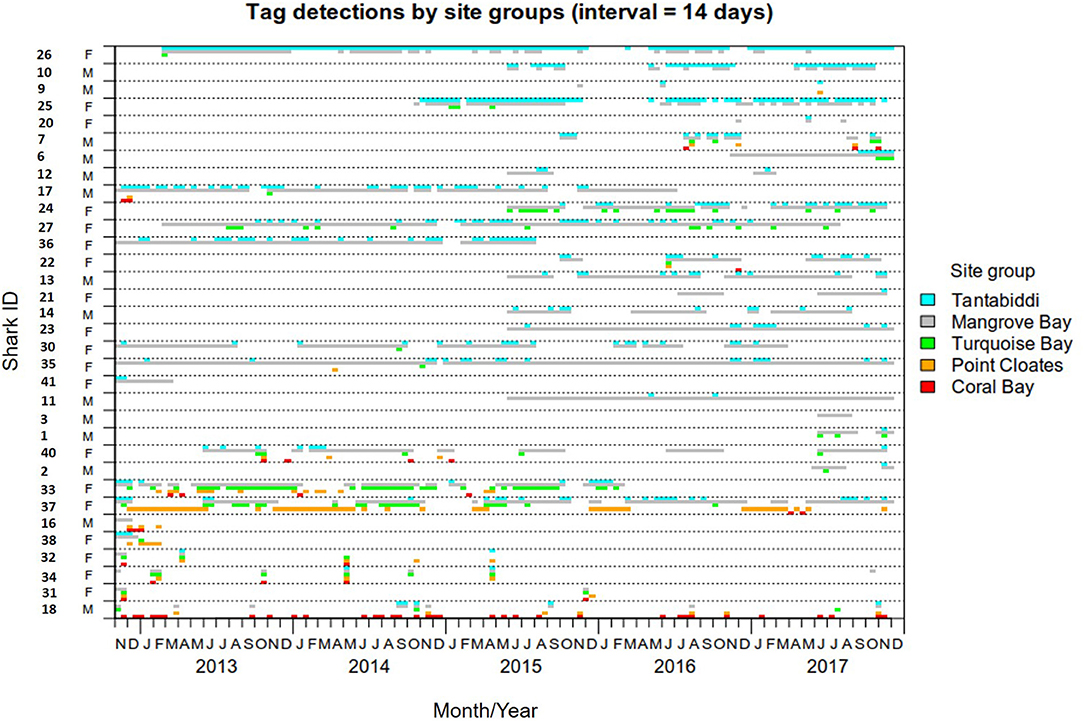
Figure 3. Detection span of 33 lemon sharks detected at more than one site group. Detections were binned into 14 days intervals and color coded by site group.
The mean residence index (RI) using only detections on the Mangrove Bay receivers was 45% (IQR 9–77%) and was slightly lower than RI using all receivers along the Ningaloo coast (mean 50%, IQR 28–74%) illustrating that on average individuals were not within range of receivers on about 50% of days. Mean roaming index for Mangrove Bay was 53% (IQR 38–73%) and not surprisingly increased with detection days (linear correlation r = 0.78). Effective roaming index averaged 24% (IQR 18–29%) and was less correlated with detection days (r = 0.41). Average 50 and 95% KUD areas were 1.7 km2 (range 0.3–4.1 km2) and 9.7 km2 (1.5–19.3 km2) respectively (Table 1). Effective KUD area was highly correlated with the 50 and 95% KUD areas (r = 0.98 and 0.91, respectively) and was between them in magnitude (generally about 80% of the 95% KUD area). Effective roaming index was also highly correlated with the three measures of KUD area (r = 0.88–0.96). RI, roaming, effective roaming and effective KUD area were not significantly different between sexes or correlated with size (for sex, Wilcoxon rank sum test, n = 34, p > 0.1 in all cases; for size, n = 34, |r| < 0.1, p > 0.1 in all cases).
The KUD areas indicated habitat use within Mangrove Bay only. Habitat use when animals where not detected cannot be estimated. Estimates of KUD within Mangrove Bay do however indicate that at least for part of the year, some animals were resident within Mangrove Bay for several months (Figure 3) with high site fidelity over multiple years. The overall KUD of all adults in the Mangrove Bay array is shown in Figure 4. The KUD of an adult female (Shark ID 37) and adult male (Shark ID 17) in July and June, respectively of 2013–2016 are shown in Figures 5A–H to illustrate site fidelity and stability in home range despite annual departure from the Mangrove Bay array.
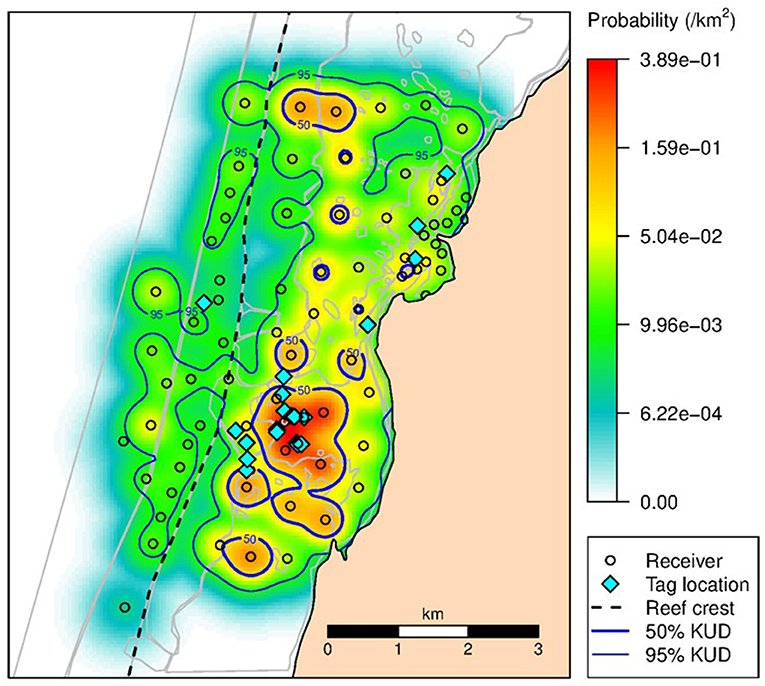
Figure 4. The 50 and 95% KUD contours for all adult N. acutidens. Shading reflects the conditional probability (per km2) of all tagged sharks occupying space within the array when it was within the array boundaries. Dashed line shows seaward edge of reef crest.
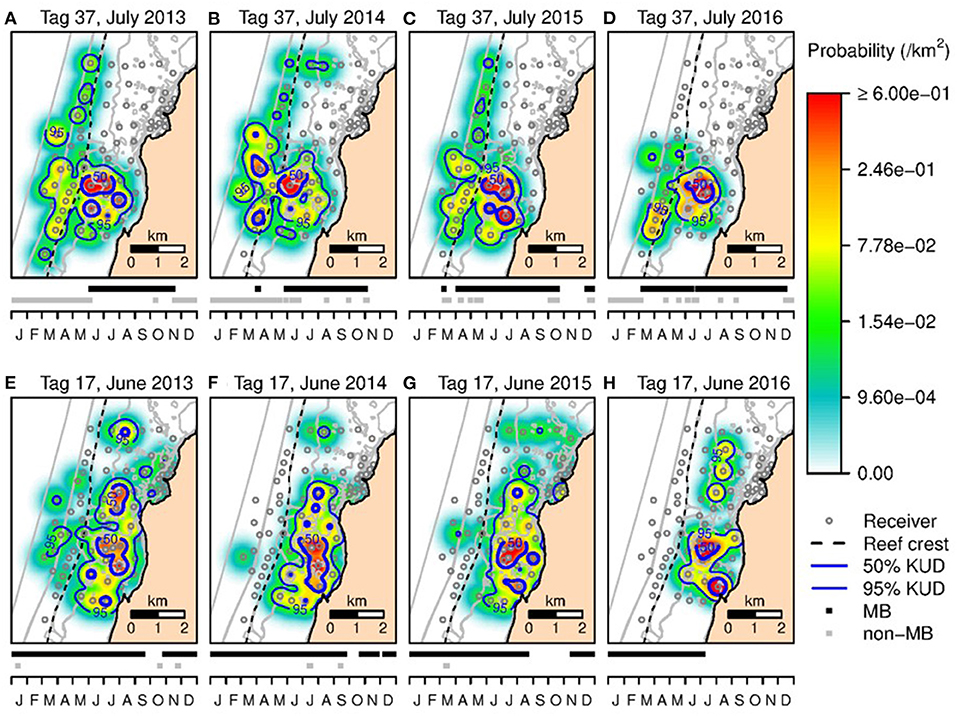
Figure 5. The 50 and 95% KUD contours for an adult female N. acutidens (shark ID = 37) in July 2013 (A), 2014 (B), 2015 (C), and 2016 (D). (E,F) The 50 and 95% KUD contours for an adult male N. acutidens (shark ID = 17) in June 2013 (E), 2014 (F), 2015 (G), and 2016 (H). Shading reflects the conditional probability (per km2) of a tagged shark occupying space within the array when it was within the array boundaries. Dashed line shows seaward edge of reef crest. The graph below each map shows the detection history in that year (with detections within the Mangrove Bay array and elsewhere).
Seasonal Changes in Residency and Home Range Within Mangrove Bay
Male residency in the Mangrove Bay array was highest in early (austral) winter and lowest in spring, ranging from 9% (IQR 0–8%) in October to 50% (IQR 0–100%) in July (Figure 6A, Table 2). Conversely, roaming within the Mangrove Bay array (as effective KUD area) was lowest in early winter and highest in spring (Figure 6B). This seasonality in roaming is not an artifact of the seasonality in residency because in that case roaming would have increased with residency. Smaller KUD areas in early winter were associated with a small estimated shift away from the coast of 0.2 km between spring and autumn (indicated by the KUD weighted average distance from the coast) (Figures 6B,C).
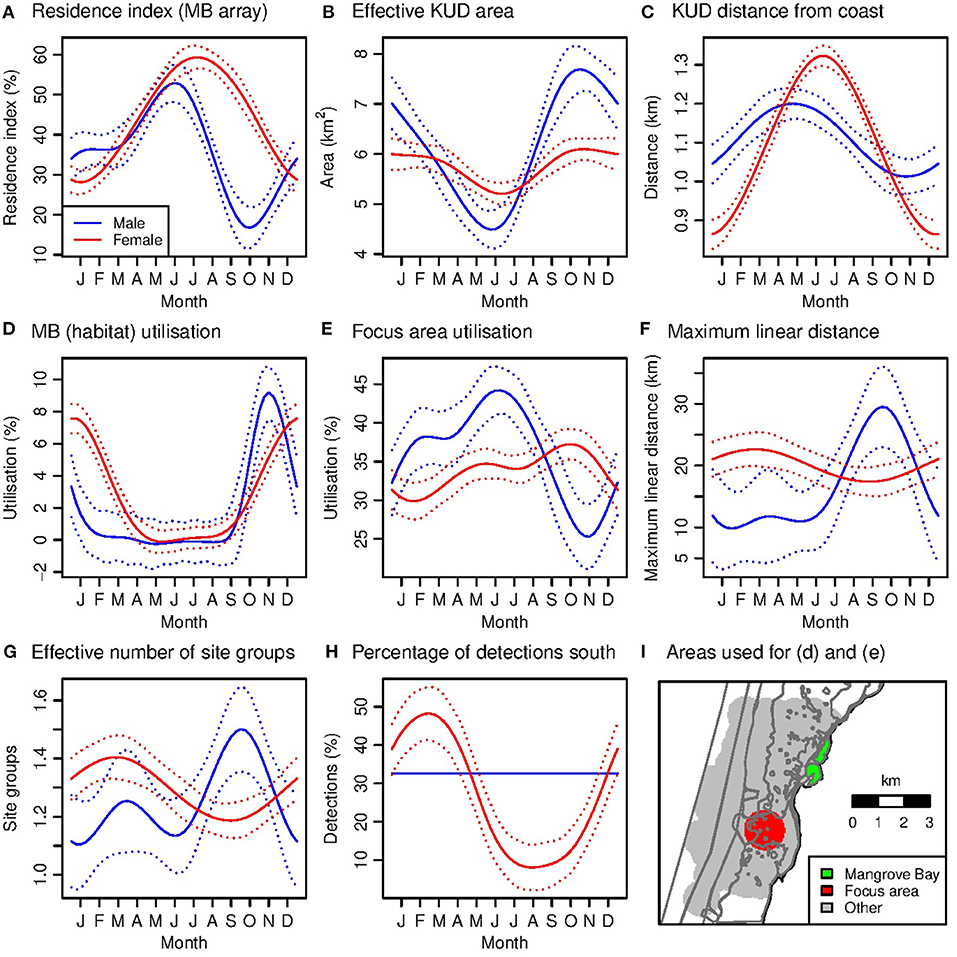
Figure 6. (A–I) Generalized additive model predictions of seasonal variation in habitat utilization within Mangrove Bay (A–F) and among site groups (G,H). The predictions are the average over tags. The dotted lines indicate the 95% confidence interval for the month term only, and therefore indicate the significance of seasonal variation but not differences between males and females in a given. The focal area used to calculate MB and focal area utilization is shown in (H).
Female residency in the Mangrove Bay array was highest in winter and lowest in summer, ranging from 27% (IQR 0–65%) in January to 61% (IQR 10–100%) in July (Figure 6A). Roaming within the Mangrove Bay array was less seasonal than that for males and was lowest in winter, when KUDs were estimated to be 0.45 km further offshore than in summer (Figures 6B,C).
The offshore shift in female home ranges between summer and winter was associated with temperature changes related to season and distance offshore. During winter, mean water temperature close to the shoreline (0.15 km) was around 2°C colder than 0.85 km further offshore (Figure 7). During winter, minimum temperatures within Mangrove Bay (0.15 km) were 5°C colder (15.9°C) compared to 0.85 km further offshore (21°C). During late spring and summer, temperatures closest to shore were 1 – 2°C warmer than sites further offshore (Figure 7).
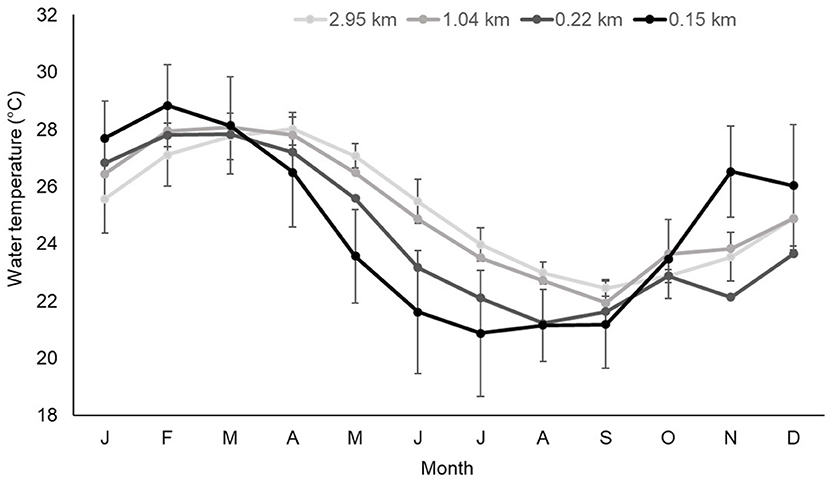
Figure 7. Mean (± Sdtev) monthly water temperature (°C) at receivers at increasing distance (kilometers) from the shoreline.
Habitat Use in Mangrove Bay
Habitat within the Mangrove Bay array is shown in Figure 8A. Only habitats within the lagoon were heavily or preferentially utilized by adult N. acutidens. The most heavily utilized habitats were algal pavement and sandy areas in the lagoon (Figure 8B), due in part to their large areas, but also due to selection (Figure 8C). Patch reefs also had positive electivity, but utilization was small due to their small total area. Because the patch reefs and shoreline reefs were small relative to receiver spacing and scattered over the algal pavement and sandy lagoon, their utilization was confounded with that of the enclosing habitats. Some individuals spent considerable time in Mangrove Bay (n = 9). The reef pass was neither avoided nor selected with electivity around zero (Figure 8C). Both the shallow (<20 m) coral and pavement dominated reef slope as well as the deep (>20 m) sand dominated reef slope were avoided by adult N. acutidens.
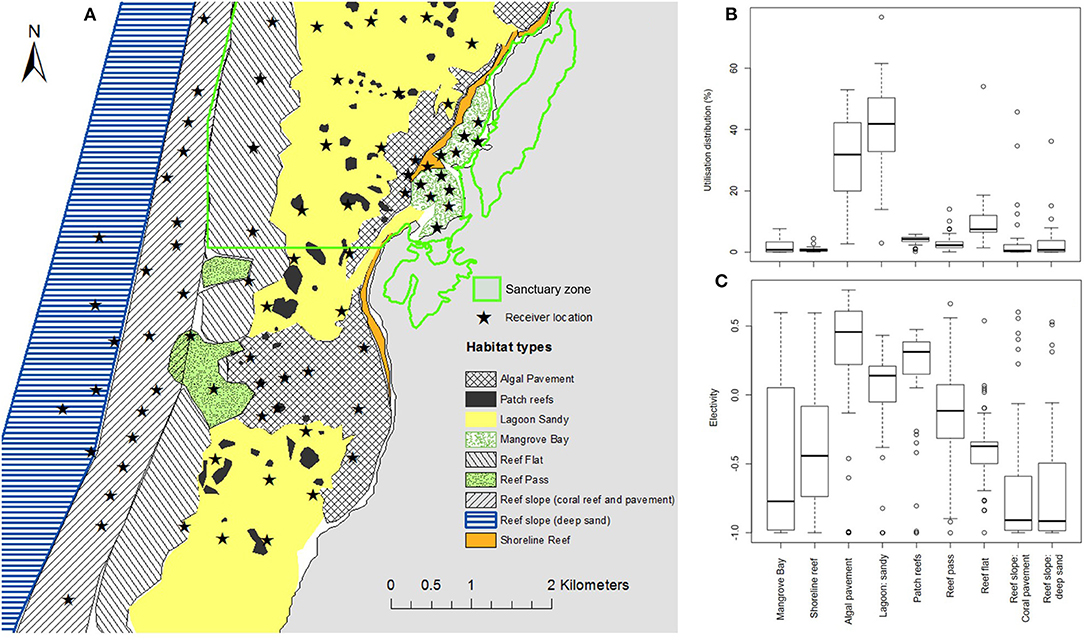
Figure 8. (A) Map of the Mangrove Bay array showing sanctuary zone boundary, receiver locations, and habitat polygons used to estimate electivity. (B) Box and whisker plot of the percentage of utilization distribution in each habitat type, (C) box and whisker plot of Ivlev's electivity index for each habitat. Individual electivity values from all individuals were sufficient data were available (n = 31) were used. Values above zero indicate increasing selection of that habitat, values of zero indicate neither avoidance or selection and values below zero indicate increasing avoidance of that habitat.
The sharks utilized the two shallow habitats (Mangrove Bay and reef flat) less at low tide than high tide. For the individuals that used Mangrove Bay (at least 1% utilization over the whole tide cycle), utilization was significantly lower at low tide (1.1%) than at high tide (7.1%) (Wilcoxon signed rank test, p = 0.004, n = 9). For reef flat, the difference was smaller (6.2 vs. 8.9%, p = 0.006, n = 20). Habitat use varied between the day and the night. In particular, for the two most heavily utilized habitats, differential diurnal utilization of algal pavement declined from 39% (day) to 25% (night) (Wilcoxon signed rank test, p < 0.001, n = 26) and inversely that of sandy lagoon increased from 38 to 48% (p < 0.001, n = 26).
Seasonal variation in habitat use varied between the sexes. For example, male preference for the Mangrove Bay habitat was highest in October–December (utilization average and IQR: males, 5.5%, 0.9–8.8%; females, 4.6%, 0.1–6.5%) (Figure 6D). Female preference for Mangrove Bay was highest a little later in December–February (males, 1.8%, 0.6–2.6%; females, 5.7%, 1.0–8.8%) (Figure 6D). For a ‘focus area’ in the core of the distribution (Figures 4, 6I), male preference was lowest in September–November, when male residency was also lowest and female preference was highest (utilization: males, 20%, 13–27%; females, 34%, 24–45%) (Figures 6A,E).
Seasonal Migrations
For males, consistent with the low spring residency at Mangrove Bay, roaming among site groups (indicated by MLD and effective number of site groups) was highest in spring (Figures 6F,G, Table 2). Average MLD (average MLD values, not GAM predictions) ranged from 6.5 km (IQR 5.7–6.7 km) in April to 41.2 km (IQR 10.1–59.7 km) in November. The percentage of detections in the southern site groups did not vary significantly among seasons (Figure 6H). For females, roaming among site groups was less seasonal than for males and lagged behind residency, being highest in early autumn and lowest in early spring (Figures 6F,G). Average MLD ranged from 8.9 km (IQR 6.0–11.6 km) in July to 22.8 km (IQR 5.9–17.2 km) in April. Utilization of southern sites was seasonal for females and followed a similar pattern to roaming, being highest in early autumn and lowest in late winter (Figure 6H). Although the GAMs estimated significant seasonal variation in average roaming, variation among individuals was high, and the average seasonal patterns were not evident in the data for every individual.
Timing of Repeat Migrations
Of the resident adult lemon sharks, 14 females and 11 males departed the array and then returned at least once with some individuals demonstrating both regular seasonal migration and site fidelity over 7 consecutive years. The majority of migrating males departed the array earlier than females and for a longer duration (~3 months) than females (~2 months) with most males departing before 27 October and most females by 27 November (Figure 9A). The period of absence away from Mangrove Bay also differed between the sexes with female absence spread evenly between 100 and 250 days (20–25%). Males were predominantly absent for either 100–150 (26%) or 200–250 days (29%) with all other time periods contributing <10% (Figure 9B).
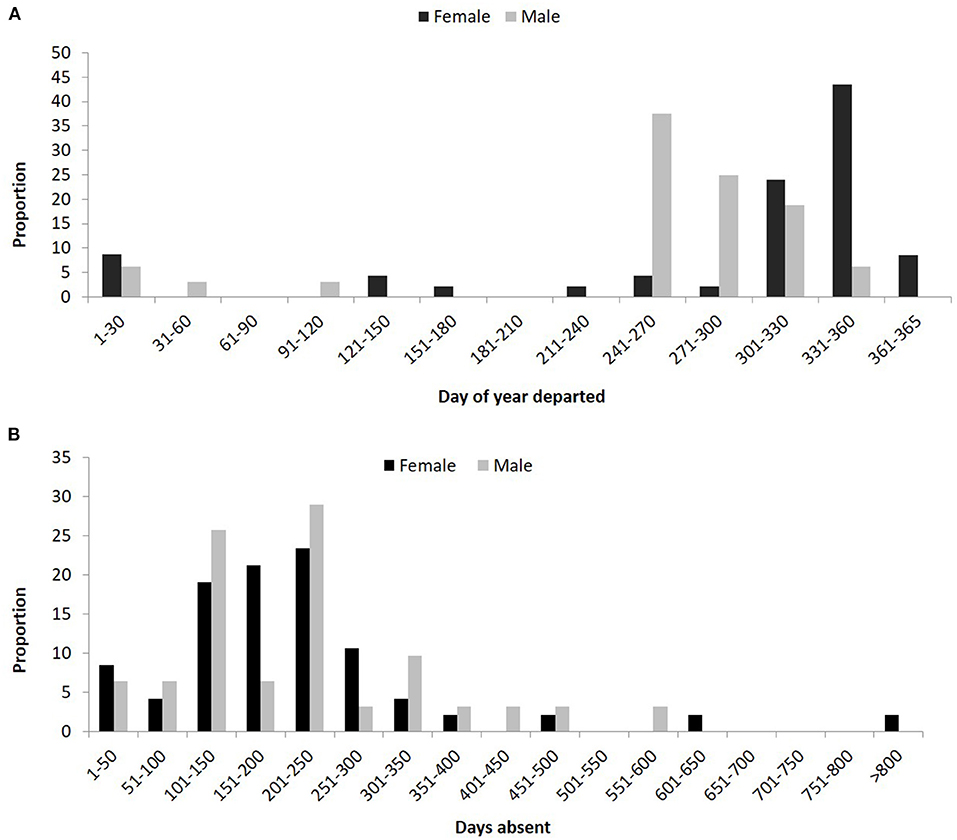
Figure 9. (A) Proportion of resident adult male and female lemon sharks that departed the Mangrove Bay array on day of year with 1 being January 1 and 365 December 31. (B) Proportion of resident adult male and female lemon sharks that were absent from the Mangrove Bay array for a certain number of days.
Of the resident lemon sharks, two females (Shark ID 23, 35) and one male (6) did not depart the Mangrove Bay array for more than a few days during their period of residency that spanned between 694 and 2,164 days. There were two females (27, 36) that only departed once during their monitoring period with both of these animals detected in Mangrove Bay for ~ 2 years before departing in October/November and subsequently returning. The timing of detections of non-resident sharks was largely opposite to resident sharks. Non-resident sharks were tagged in November and only detected by receivers in the Mangrove Bay array either between October–December or around March–June. There was some evidence of non-resident animals traveling to Mangrove Bay to mate and possibly give birth November with heavily pregnant (20, 31), post-partum (32) and females with bleeding mating scars (34) captured and tagged at Mangrove Bay. These animals were absent from the array for most of the year and only detected in the Mangrove Bay array in November–April.
Discussion
Individual adult N. acutidens demonstrated regular and predictable migrations, strong site fidelity over several years and outside of migrations had a small home range confined to the lagoon. Sharks exhibited long-term seasonal site fidelity (average of 5.7–7.4 months) interspersed with extended periods of absence (average of 4.8 −5.8 months) as well as continuous site fidelity spanning up to 6 years. Long-term (6 years) data from acoustic tagging revealed tidally influenced short term movements, regular seasonal shifts in the location of home ranges most likely related to temperature and regular seasonal patterns in long distance movements probably related to reproduction. Both male and females exhibited seasonal migrations, however there were distinct differences in patterns of residence, space use and the timing and magnitude of migrations.
Outside the reproductive season, home range size and habitat use were largely similar with both sexes using similar habitats and similar size core areas; however, use of the most selected habitat (algal pavement) by males and females varied seasonally, suggesting sexual segregation outside the breeding season. During the onset of the reproductive season (Sep–Oct) males moved larger distances and were detected moving between widely-separated groups of receivers more frequently than females. From December–April, this pattern was reversed, with females moving more. Both males and females undertook seasonal movements up to 130 km from their tagging location followed by a return after 3–6 months with high site fidelity that persisted for up to 6 years. Males and females spent similar times away from their home range with males returning over a 100–250 days period after departing while female returns were clustered between either 100–150 or 200–250 days after departing. The highly coordinated, annual migration of adults corresponds with the onset of the mating season, which was confirmed by observations of heavily pregnant females and signs of recent mating activity (such as bleeding bite marks on females) in October–November. Most females undertook annual movements during the reproductive season providing evidence of annual reproductive periodicity. Differences in the timing and distance moved by males may represent mate-searching behavior while movement patterns of females may be more influenced by habitat selection and re-location to areas favorable for gestation, parturition and mating. Space and habitat use of shark within Mangrove Bay array.
Four sharks (2M:2F) did not leave the 28 km2 Mangrove Bay array for more than a few days during the entire period spanning between 625 and 2,164 days; these individuals can be classified as residents. One female (Shark ID 35) was detected every week within the Mangrove Bay array for more than seven years, representing one of the longest documented residency periods of any shark species (Chapman et al., 2015). Twenty one sharks displayed high site fidelity and were resident within the Mangrove Bay array between March–December with a large degree of individual and sex-specific variability in departure and arrive dates. Adult lemon sharks within the Mangrove Bay array had restricted home ranges (average 95 % KUD = 9.7 km2) during their period of residence with individuals returning to the same location year after year. While there was considerable individual variation in habitat use, collectively, adults avoided the reef slope and reef flat habitats as well as shallow inshore habitats that encompassed Mangrove Bay. Adult N. acutidens tended to occupy lagoon habitats that included algal pavement and patch reefs interspersed with sandy areas. Their preference for these habitats is most likely due to their predominantly teleost based diet (Newman et al., 2012) with teleost density around patch reefs substantially higher than adjacent bare pavement and sand (Downie et al., 2013).
Adult female lemon sharks displayed an average 0.45 km seaward shift in home range during winter. For males, there was a distinct shift to the southwest (toward the focal area—Figure 6E). Since tidal amplitude at Ningaloo does not change seasonally, water temperature was the most plausible cause of this shift. However, seasonal changes in prey availability cannot be excluded. Mean water temperature closest to the shoreline was around 2°C colder and minimum temperatures were around 5°C colder than sites 0.85 km further west in the deeper areas of the lagoon. Lowest and most extreme winter temperatures were at the sites closest to the shoreline within Mangrove Bay (0.15 km) and at sites to the south (outside the confines of Mangrove Bay) despite similar distance from shore (0.22 km), winter temperatures were 1.5°C warmer and more stable. Sharks presumably moved slightly further west (for females) and southwest (males) to be in warmer water and escape extreme temperature fluctuations in shallow inshore waters. Movement into warmer environments at a fine scale has been demonstrated in blacktip and gray reef sharks at Ningaloo (Speed et al., 2012) showed that five adult female blacktip reef sharks had a body temperature being ~1°C warmer than mean water temperature, and suggested this was evidence of behavioral thermoregulation with gray and blacktip reef sharks detected more frequently in shallow inshore waters in the afternoon.
For animals whose home range encompassed the shallow mangrove lined bay (Mangrove Bay), there was a strong effect of tide on use of this habitat. These animals spent an average of 7 and 1% of their time in this habitat at high and low tide respectively (based on KUD overlap). Around 42% of the Mangrove Bay habitat is intertidal, suggesting a disproportionately high use of intertidal habitat by some individuals at high tide. Indeed, adults were observed actively hunting in shallow (<0.5 m) water adjacent to the shoreline in Mangrove Bay when the high tide coincided with late afternoon. Adults presumably access suitable foraging areas at high tide and are forced into deep water as the tide recedes, as opposed to juveniles where tide mediated selection of shallow habitats has been linked to both predator avoidance and improved foraging efficiency in juvenile N. acutidens (Oh et al., 2017) and N. brevirostris (Wetherbee et al., 2007; Guttridge et al., 2012). Filmalter et al. (2013) proposed a similar mechanism to explain increased detections of sub-adult lemon sharks at low tide with sharks moving into the lagoon as water depth on the surrounding flats became too shallow.
There was a diel shift in habitat use of the two most selected habitats (algal pavement and sandy areas of the lagoon). Sharks used algal pavement more during the day (39%) than the night (25%) and used sandy lagoon areas more at night (48%) than during the day (38%). Increased use of the sandy lagoon areas at night was attributed to shallow water depth in this habitat (0.5–2.0 m at LAT cf 2.0–5.0 m in algal pavement) with sharks potentially foraging in the shallow clear water at night and deeper water during the day. Movement into shallower habitat at night is well-established in elasmobranchs (Dawson and Starr, 2009; Vanderklift et al., 2014) with our results supporting the use of different areas during day and night (Hammerschlag et al., 2017).
There are few comparative studies on adult N. acutidens in the Indo-Pacific or N. brevirostris in the Atlantic. Using visual observations, Clua et al. (2010) demonstrated residence and site fidelity of some individual adult N. acutidens around a shark feeding site in Moorea with Mourier et al. (2013) providing additional support of high residency in the French Polynesia. Kessell et al. (2014) monitored movement of adult N. brevirostris with acoustic tags on the east coast of Florida and while estimates of home range were not made, they demonstrated high site fidelity of sharks to reefs off the Jupiter Inlet region. Individual sharks returned to the same area over multiple years with animals recorded up to 1,010 km away during the summer providing evidence of seasonal migrations and site fidelity.
Comparison Between Juveniles, Sub-Adults, and Adults
Our estimates of residence and days detected in adults demonstrated higher residency and more prolonged use of the Mangrove Bay array by adults than neonates. Oh et al. (2017) examined residence, home range and habitat use of 23 neonate N. acutidens within the Mangrove Bay array between 2013 and 2015 and demonstrated the neonates were not confined to the Mangrove Bay array with average RI, days detected and 50 % KUD of 0.42, 161, and 0.62 km2, respectively. While the core home range of adults was more than double the size of neonates (1.60 km2), adults had higher RI (0.49) and were detected for an average of 451 days suggesting higher site fidelity in adults. Based on electivity analysis of habitat preference, neonates were primarily confined to the shallow intertidal areas within Mangrove Bay. However, for adults the use of Mangrove Bay was highly variable with nine individuals utilizing this habitat frequently resulting in overlap with neonate space use. Overlapping space use in adults and juveniles has been observed in the gray reef shark Carcharhinus amblyrhynchos on the Great Barrier Reef (Heupel and Simpfendorfer, 2015) as well as Caribbean reef sharks (Bond et al., 2012). Filmalter et al. (2013) showed high site fidelity and residence in sub-adult N. acutidens monitored by acoustic telemetry at an isolated atoll in the Indian Ocean with residence index over 1 year around 0.79 and most sharks showing distinct habitat preferences within the lagoon. For the allopatric N. brevirostris, it has been suggested that as these sharks grow, individuals move into deeper reefs and increase their home range with the onset of sexual maturity (Gruber et al., 1988; Chapman et al., 2009). Our data show that while adults have a larger home range than neonates and juveniles in the same area, their core home range is remarkably small with adults largely confined to the lagoon with very few detections on the reef slope. Furthermore, adults maintain site fidelity to Mangrove Bay whereas all but two neonates that departed the array did not return (Oh et al., 2017). These differences suggest that juveniles at Ningaloo have less fidelity to specific nursery areas than N. brevirostris and are either more nomadic (whilst searching for suitable habitat) in their early years or that those animals tagged by Oh et al. (2017) moved to other suitable sites where they became resident.
Potential Influences of Migratory Behavior
Here we demonstrate a range of behaviors in adult lemon sharks including year-round residency, partial migration, seasonal residency, high site fidelity (returning to the same home range after migrations) as well as transient animals. The strong seasonal residency demonstrated by a large proportion of adults was most likely part of reproduction given the timing of these migrations, lack of significant latitudinal temperature changes and location of known nursery areas. Parturition and mating are thought to occur between October–March. Oh et al. (2017) recorded open umbilical scars on neonate N. acutidens within Mangrove Bay between November–March while we captured heavily pregnant females and females with bleeding mating scars in October/November. While telemetry data cannot verify whether animals were pregnant when they departed and/or gave birth prior to departing Mangrove Bay or once they arrive at an alternative pupping site, the timing of movements and behavior of sharks in other studies supports movements related to reproduction. Migration of adult female lemon sharks away from their home range at the onset of the pupping season is consistent with parturition site fidelity (Chapman et al., 2015), which has previously been documented in N. acutidens and N. brevirostris (Feldheim et al., 2004, 2014) with these studies providing evidence that females move outside of their home range to drop their pups before returning.
The movement of some adult females away from their area of residence during the reproductive season may be due to females returning to specific locations to pup. Similarly, movement of males away from resident areas has been documented in N. acutidens (Mourier et al., 2013) and other reef sharks (Heupel and Simpfendorfer, 2015) and is thought to be due to males searching for receptive females. At the onset of the reproductive season, some individual sharks (both males and females) were recorded moving repeatedly between groups of receivers at Mangrove Bay, Point Cloates and Coral Bay with animals moving several 100 km within a few days. These types of movement are potentially related to sharks searching for natal pupping grounds and or mating areas. Using microsatellite genotyping Feldheim et al. (2002) demonstrated that while adult female lemon sharks demonstrate strong philopatry to their nursery area (Bimini Lagoon), males were nomadic, only infrequently returning to the same mating group. Multiple paternity in N. brevirostris litters has been demonstrated by Feldheim et al. (2001b). Our data demonstrated that males moved greater distances and visited more sites during the reproductive season than females, potentially to increase the number of females they can mate with during this time. Feldheim et al. (2002) suggested that in N. brevirostris, nursery areas also serve as mating areas with complex reproductive processes resulting in multiple paternity as well as evidence that females mate with different males almost every breeding cycle. Our data showed that females generally departed and returned a month or two later than males and didn't move as much (smaller monthly maximum linear distance). In addition to sex-related difference in time of departure and return, we also demonstrate asynchronous timing of habitat use in Mangrove Bay which is a known pupping area and the only place where females were captured with still bleeding mating scars. Female use of Mangrove Bay peaked in Dec-Feb while use by males peaked in Oct-Nov. The mechanism behind segregation leading up to mating is likely due to avoidance of aggressive males and avoidance of mating attempts (Pratt and Carrier, 2001; Sims et al., 2001; Jacoby et al., 2010). In addition to asynchronous reproductive movements, we also demonstrated sex specific seasonal preferences of the most preferred habitat (algal pavement) with females using this habitat more than males between Jan-Sep and males more than females between Oct-Dec. Increased use of algal pavement by females for most of the year may have resulted in our catch rates of females being 25% greater than males. Sexual segregation in elasmobranchs is well established in both highly migratory species such as mako sharks, whale sharks, white sharks, and hammerhead sharks as well as coastal and reef associated species such as cat sharks, spot tail and gray reef sharks (McKibben and Nelson, 1986; Klimley, 1987; Stevens, 1990; Sims et al., 2001; Mucientes et al., 2009; Knip et al., 2012; Ketchum et al., 2013). Outside of mating season, reasons for segregation include preference for warmer water known to reduce gestation period (Wallman and Bennett, 2006; Hight and Lowe, 2007). Similarly, sex-specific preference for different prey items has been demonstrated in some species (McCord and Campana, 2003) and can lead to differences in habitat choice (Klimley, 1987).
Seasonal movement was not limited to resident animals, with strong evidence that non-resident sharks had similar seasonality in their movements. For non-residents, most detections occurred either around October–November (when resident sharks depart) or again in March–July (when residents were returning). The timing of these detections combined with the fact that most non-residents were tagged in November supports a theory that non-residents are moving to their pupping/mating sites at the same time residents depart Mangrove Bay with non-residents primarily detected moving past Mangrove Bay when residents depart and return. Two heavily pregnant (20, 31), one post-partum (32) and a female with bleeding mating scars (34) captured and tagged at Mangrove Bay in November were detected in subsequent years around the same time in the Mangrove Bay. Mangrove Bay has been identified as a nursery area for lemon sharks (Oh et al., 2017) with mating activity also occurring, so it is not surprising that our data suggest annual return movements as well year-round residence of adult females (who most likely pup at Mangrove Bay) and males. The disappearance of residents and appearance of non-residents coinciding with reproductive periods was also recorded by Mourier et al. (2013) who hypothesized that this behavior was a strategy to avoid inbreeding in this isolated and small population of N. acutidens.
The short detection span of six individuals tagged outside of the reproductive season are possibly due to a more transient proportion of the population (Pillans et al., 2014) or mortality (natural and fishing). While commercial fishing is not allowed within the Ningaloo Marine Park, at least two the sharks we tagged had previously been captured by recreational fishers targeting large sharks. One had been tagged with a dart tag and the other still had a large hook and steel trace in the mouth. Lemon sharks have been implicated in depredation of hooked fish at Ningaloo Reef (R.P. Pers. Obs) with increasing evidence that fishers are attempting to remove depredating sharks.
The periodicity of seasonal migrations may reveal information about reproductive periodicity with evidence that adult female sharks with biannual reproductive cycle typically return to seasonal sites on an alternate year cycles, e.g., white shark Carcharodon carcharias aggregations at Guadalupe (Domeier and Nasby-Lucas, 2007) and gray nurse shark Carcharias taurus in Australia (Bansemer and Bennett, 2009). Our data at Ningaloo Reef show that around 71% of resident adult females undertake annual migrations and 29% undertaking biannual migrations. If these migrations are strictly for reproduction it would appear that the population at Ningaloo comprises individuals that pup annually and biannually. While N. acutidens has been classified in the literature as having biannual pupping (Last and Stevens, 2009), Mourier et al. (2013) demonstrated both annual and biannual pupping in N. acutidens in Moorea with most sharks having a biannual cycle. Interestingly, Mourier et al. (2013) showed that while some females moved away from the resident area to mate, others (with recent mating scars) remained resident. More research on movements of pregnant females combined with visual observations are required to better understand reproductive periodicity and the extent, direction and destination of migrations during the reproductive season. Given frequent observations of adults in shallow water, dorsal fin mounted SPOT satellite tags may provide long term data when animals move outside the range of acoustic receivers.
The duration of reproductive migrations are largely unknown for most elasmobranchs with considerable variability in distance moved and absence from residence areas. In one study that monitored pregnant N. acutidens with visual observations at Moorea Island, heavily pregnant females only departed the study area (a shark feeding area in Moorea Island monitored by visual census over 4 years) for a few days before returning after giving birth with parturition occurring between July–November (Clua et al., 2010; Mourier et al., 2013). Pregnant and recently birthed females were easily identified by the shape of their belly (Clua et al., 2010). Resident males also disappeared for a few days–weeks during mating season (September–November) with non-resident males appearing at Moorea during the same time. These observations suggest females return to their area of residence soon after giving birth with evidence that mating occurs within the same month and up to 2 months after parturition. Given that the study area in Moorea was a shark feeding site and the authors demonstrated a significant effect of feeding on residence, it is uncertain whether this is normal behavior or whether female sharks returned rapidly due to provisioning. Few studies have monitored migratory patterns and return of pregnant female sharks. McMillan et al. (2019) tagged near term pregnant school sharks (Galeorhinus galeus) with pop-up satellite tags and demonstrated strong plasticity in movement away from the tagging sites. Animals tagged in The Great Australian Bight were more likely to be resident to that region (<15 km), whereas animals tagged around Kangaroo Island moved great distances (>3,000 km) with animals moving into Bass Strait and as far as New Zealand. The timing of return movements to over-wintering resident areas could not be determined in this study as satellite tags were programmed to release 6 months after tagging. Espinoza et al. (2016) demonstrated large scale individual variability in residence of bull sharks tagged on the Great Barrier Reef on the east coast of Australia where adult females undertook long distance movements (up to 1,400 km) during spring/summer before returning to reefs off Townsville within a few weeks–months. These movements were thought to be related to parturition with all but one female returning.
Strong seasonal site fidelity in carcharhinid sharks has mainly been demonstrated in lower latitudes, where cooling autumn/winter water temperature force animals to migrate to warmer environments (Conrath and Musick, 2010; Kessell et al., 2014; Reyier et al., 2014; Braccini et al., 2018) and in some cases where mating and gestation occurs in warmer waters up to 1,200 km from pupping areas (Bansemer and Bennett, 2009). Kessell et al. (2014) demonstrated long distance seasonal migrations of adult N. brevirostris on the east coast of the United States with individuals recorded over 1,500 km of coastline (where water temperatures ranged from 18–28°C) during the year and showing a distinct southward movement to warmer water in autumn with animals concentrated on offshore artificial reefs from November–April between Delray Beach and Port St. Lucie off the Florida coast over multiple years. Sharks showed a preference for 22–26°C and during the summer months, occupied a considerably larger latitudinal range.
While the driver for seasonal migrations in adult N. acutidens tagged around Mangrove Bay was most likely related to reproduction, temperature and other biological drivers cannot be excluded. Only five resident animals were detected as far south as Coral Bay suggesting that most resident animals did not move more than 135 km south of Mangrove Bay. The annual temperature range at Coral Bay and Tantabiddi were 22.0–28.4°C and 22.8–29.2°C (Fulton et al., 2014), respectively with winter and summer differences only 1–2°C. These temperatures are within the preferred range of the species and such small seasonal differences are unlikely to drive migrations with the 0.45 km shift in home range attributed to 2–3°C differences between shallow inshore waters and slightly deeper lagoon waters. If temperature was driving migration in adults, one might expect similar departure and arrival dates, however there were distinct differences in timing of departure and return of resident adult males and females.
For neonate N. acutidens tagged within Mangrove Bay, there was no strong evidence that temperature influenced residence (Oh et al., 2017). For females there was no monthly pattern in residency and in males, residence was slightly higher in winter and spring with only four out of 23 animals resident for more than 12 months. While seasonal differences in food availability can drive migrations (Sims et al., 2003), and there are latitudinal changes in species composition and abundance along the 300 km Ningaloo Reef (Babcock et al., 2008), there are no data to support seasonal changes in fish biomass that might explain migration of a portion of the adult lemon shark population adjacent to Mangrove Bay. Pillans et al. (2014, 2017) have previously demonstrated long term residence for different coral reef fish within the Mangrove Bay array, and while these species are likely preyed on by adult N. acutidens and have overlapping home range within the array, there was no seasonal pattern in the residence of prey that supports predator migration.
Movement in Relation to Spatial Management
Data on movement can inform spatial protection (Hunter et al., 2006; Knip et al., 2012), direct threats such as fishing (Vaudo et al., 2017; Braccini et al., 2018) and habitat modification as well as environmental changes (Lear et al., 2019; Pillans et al., 2020). While there are currently no direct threats to N. acutidens along the Ningaloo coast (due to absence of commercial fisheries), our ability to monitor large numbers of adults for up to 6 years provides important data relevant to the management of this species in areas where overfishing and habitat destruction have caused localized extinctions and populations declines (Pillans, 2003). Despite small and stable home range during periods of residence, the scale of latitudinal movements in adult N. acutidens along the Ningaloo Reef precludes small no-take MPA's from protecting animals year round with about 32% of tagged animals detected moving at least 130 km from their tagging site over the course of the study with an average monthly MLD of ~22 km. Static closures will therefore only protect individuals for part of the year with additional measures such as fishery closures along migration corridors that coincide with the timing of migrations/seasonal movements (e.g., Hunter et al., 2006; Dwyer et al., 2019), fishing that targets demographically more resilient life stages (i.e., juveniles—McAuley et al., 2007) and harvest controls (Butterworth and Punt, 1999) required to manage complex life history movements in this species.
Conclusion
As juveniles, N. acutidens are confined to shallow inshore areas with a strong preference for mangrove and seagrass (a habitat known to be threatened by coastal development and climate change). Sub-adults also appear to be spatially confined, albeit with data limited to an oceanic coral reef lagoon. Our data on N. acutidens at Ningaloo Reef demonstrated that adults have a strong preference for inshore areas within a few kilometers of the shoreline, had small and stable home range that persisted for at least 6 years in some individuals and had complex migrations, the timing and periodicity indicative of a reproductive driver. Despite patterns in some individuals there was a high degree of individual variability combined with sex-specific differences in both fine scale habitat use as well as the timing of departure and return from migratory movements. Our long-term data highlight the complexity of behaviors demonstrated by individuals within a population and underscore the need for long-term data to manage long-lived elasmobranchs that are particularly susceptible to anthropogenic impacts such as fishing and habitat destruction. Additional receiver coverage and or the use of archival pop-off tags are required to better understand the full extent of migrations. Genotyping (Feutry et al., 2020), close kin mark recapture (Hillary et al., 2018) as well as other parentage analysis (Mourier et al., 2013; Feldheim et al., 2014) would be the most effective means of addressing whether annual migration in females is for parturition.
Data Availability Statement
The original contributions presented in the study are included in the article/supplementary materials, further inquiries can be directed to the corresponding author/s.
Ethics Statement
The animal study was reviewed and approved by CSIRO animal ethics.
Author Contributions
RP, RB, and MV participated in the conception of ideas. RP and WR analyzing the data and producing the figures. All authors have agreed that the manuscript is ready for submission, the manuscript has not been published and is not under review at any other journal, contributed to the writing of the manuscript, with RP, MH, DT, RB, and MV conducting field work and collecting the data, and accept responsibility for the manuscript content.
Funding
This research was funded by a Caring for Country and Ningaloo Outlook (Ningaloo Outlook is a BHP -CSIRO Industry-Science Marine Research Partnership investing A$5.4 million over 5 years to gather new knowledge on the Ningaloo reef and its important ecological values).
Conflict of Interest
The authors declare that the research was conducted in the absence of any commercial or financial relationships that could be construed as a potential conflict of interest.
Acknowledgments
Detections of sharks outside of the CSIRO managed array were provided by the Animal tracking node of Australia's Integrated Marine Observing System (IMOS). IMOS is enabled by the National Collaborative Research Infrastructure Strategy (NCRIS). It is operated by a consortium of institutions as an unincorporated joint venture, with the University of Tasmania as Lead Agent.
References
Babcock, R., Haywood, M., Vanderklift, M., Clapin, G., Kleczkowski, M., Dennis, D., et al. (2008). Ecosystem Impacts of Human Usage and the Effectiveness of Zoning for Biodiversity Conservation: Broad-scale Fish Census. Final Analysis and Recommendations 2007. Hobart: CSIRO Marine and Atmospheric Research. Available online at: https://www.wamsi.org.au/sites/wamsi.org.au/files/Node%203.2.2a20Ecosystem%20impacts%20of%20human%20usage.pdf (accessed September 27, 2020).
Babcock, R. C., Shears, N. T., Alcala, A. C., Barrett, N. S., Edgar, G. J., Laffertye, K. D., et al. (2010). Decadal trends in marine reserves reveal differential rates of change in direct and indirect effects. Proc. Natl. Acad. Sci. U. S. A 107, 18256–18261. doi: 10.1073/pnas.0908012107
Bansemer, C. S., and Bennett, M. B. (2009). Reproductive periodicity, localised movements and behavioural segregation of pregnant Carcharias taurus at Wolf Rock, southeast Queensland, Australia. Mar. Ecol. Prog. Ser. 374, 215–227. doi: 10.3354/meps07741
Bestley, S., Jonsen, I. D., Hindell, M. A., Guinet, C., and Charrassin, J. B. (2013). Integrative modelling of animal movement: incorporating in situ habitat and behavioural information for a migratory marine predator. Proc. R. Soc. B 280:20122262. doi: 10.1098/rspb.2012.2262
Bond, M. E., Babcock, E. A., Pikitch, E. K., Abercrombie, D. L., Lamb, N. F., and Chapman, D. D. (2012). Reef sharks exhibit site fidelity and higher relative abundance in marine reserves on the Mesoamerican barrier reef. PLoS ONE 7:e32983. doi: 10.1371/journal.pone.0032983
Braccini, M., de Lestang, S., and McAuley, R. (2018). Dusky sharks (Carcharhinus obscurus) undertake large-scale migrations between tropical and temperate ecosystems. Can. J. Fish. Aquat. Sci. 75, 1525–1533. doi: 10.1139/cjfas-2017-0313
Butterworth, D. S., and Punt, A. E. (1999). Experiences in the evaluation and implementation of management procedures. ICES J. Mar. Sci. 56, 985–998. doi: 10.1006/jmsc.1999.0532
Calenge, C. (2011). Home Range Estimation in R: The adehabitatHR Package. Saint Benoist, Auffargis: Office national de la classe et de la faune sauvage.
Chapman, D. D., Babcock, E. A., Gruber, S. H., Dibattista, J. D., Franks, B. R., Kessel, S. A., et al. (2009). Long-term natal site-fidelity by immature lemon sharks (Negaprion brevirostris) at a subtropical island. Mol. Ecol. 18, 3500–3507. doi: 10.1111/j.1365-294X.2009.04289.x
Chapman, D. D., Feldheim, K. A., Papastamatiou, Y. P., and Hueter, R. E. (2015). There and back again: a review of residency and return migrations in sharks, with implications for population structure and management. Annu. Rev. Mar. Sci. 7, 547–570. doi: 10.1146/annurev-marine-010814-015730
Clua, E., Buray, N., Legendre, P., Mourier, J., and Planes, S. (2010). Behavioural response of sicklefin lemon sharks Negaprion acutidens to underwater feeding for ecotourism purposes. Mar. Ecol. Prog. Ser. 414, 257–266. doi: 10.3354/meps08746
Conrath, C. L., and Musick, J. A. (2010). Residency, space use and movement patterns of juvenile sandbar sharks (Carcharhinus plumbeus) within a Virginia summer nursery area. Mar. Fresh. Res. 61, 223–235. doi: 10.1071/MF09078
Dawson, C. L., and Starr, R. M. (2009). Movements of subadult prickly sharks Echinorhinus cookei in the Monterey Canyon. Mar. Ecol. Prog. Ser. 386, 253–262. doi: 10.3354/meps08067
DEC (2005). Management plan for the Ningaloo Marine Park and Murion Islands Marine Management Area 2005–2011. Perth, WA: DEC.
Domeier, M. L., and Nasby-Lucas, N. (2007). Annual re-sightings of photographically identified white sharks (Carcharodon carcharias) at an eastern Pacific aggregation site (Guadalupe Island, Mexico). Mar. Biol. 150, 977–984. doi: 10.1007/s00227-006-0380-7
Downie, R. A., Babcock, R. C., Thomson, D. P., and Vanderklift, M. A. (2013). Density of herbivorous fish and intensity of herbivory are influenced by proximity to coral reefs. Mar. Ecol. Prog. Ser. 482, 217–225. doi: 10.3354/meps10250
Dulvy, N. K., Fowler, S. L., Musick, J. A., Cavanagh, R. D., Kyne, P. M., Harrison, L. R., et al. (2014). Extinction risk and conservation of the world's sharks and rays. Elife 3:e00590. doi: 10.7554/eLife.00590
Dwyer, R. G., Campbell, H. A., Pillans, R. D., Watts, M. E., Lyon, B. J., Guru, S. M., et al. (2019). Using individual-based movement information to identify spatial conservation priorities for mobile species. Cons. Biol. 33, 1426–1437. doi: 10.1111/cobi.13328
Espinoza, M., Heupel, M. R., Tobin, A. J., and Simpfendorfer, C. A. (2016). Evidence of partial migration in a large coastal predator: opportunistic foraging and reproduction as key drivers? PLoS ONE 11:e0147608. doi: 10.1371/journal.pone.0147608
Feldheim, K. A., Gruber, S. H., and Ashley, M. V. (2001a). Population genetic structure of the lemon shark (Negaprion brevirostris) in the western Atlantic: DNA microsatellite variation. Mol. Ecol. 10, 295–303. doi: 10.1046/j.1365-294x.2001.01182.x
Feldheim, K. A., Gruber, S. H., and Ashley, M. V. (2002). The breeding biology of lemon sharks at a tropical nursery lagoon. Proc. Biol. Sci. 269, 1655–1661. doi: 10.1098/rspb.2002.2051
Feldheim, K. A., Gruber, S. H., and Ashley, M. V. (2004). Reconstruction of parental microsatellite genotypes reveals female polyandry and philopatry in the lemon shark, Negaprion brevirostris. Evol. 58, 2332–2342. doi: 10.1111/j.0014-3820.2004.tb01607.x
Feldheim, K. A., Gruber, S. H., Ashley, M. V., and McEachran, J. D. (2001b). Multiple paternity of a lemon shark litter (Chondrichthyes: Carcharhinidae). Copeia. 2001, 781–786. doi: 10.1643/0045-8511(2001)001[0781:MPOALS]2.0.CO;2
Feldheim, K. A., Gruber, S. H., DiBattista, J. D., Babcock, E. A., Kessel, S. T., Hendry, A. P., et al. (2014). Two decades of genetic profiling yields first evidence of natal philopatry and long-term fidelity to parturition sites in sharks. Mol. Ecol. 23, 110–117. doi: 10.1111/mec.12583
Feutry, P., Devloo-Delva, F., Tran Lu, Y. A., Mona, S., Gunasekera, R. M., Johnson, G., et al. (2020). One panel to rule them all: DArTcap genotyping for population structure, historical demography, and kinship analyses, and its application to a threatened shark. Mol. Ecol. Resour. 20, 1470–1485. doi: 10.1111/1755-0998.13204
Filmalter, J. D., Dagorn, L., and Cowley, P. D. (2013). Spatial behaviour and site fidelity of the sicklefin lemon shark Negaprion acutidens in a remote Indian Ocean atoll. Mar. Biol. 160, 2425–2436. doi: 10.1007/s00227-013-2237-1
Fulton, C. J., Depczynski, M., Holmes, T. H., Noble, M. M., Radford, B., Wernberg, T., et al. (2014). Sea temperature shapes seasonal fluctuations in seaweed biomass within the Ningaloo coral reef ecosystem. Limnol. Ocean. 59, 156–166. doi: 10.4319/lo.2014.59.1.0156
Gerber, L. R., Kareiva, P. M., and Bascompte, J. (2002). The interplay of life history attributes and fishing pressure in evaluating efficacy of marine reserves. Biol. Conserv. 106, 11–18. doi: 10.1016/S0006-3207(01)00224-5
Gruber, S. H. (1982). Role of the lemon shark, Negaprion brevirostris, as a predator in the tropical marine environment: a multidisciplinary study. Fla. Sci. 45, 46–75.
Gruber, S. H., Nelson, D. R., and Morrissey, J. F. (1988). Patterns of activity and space utilization of lemon sharks, Negaprion brevirostris, in a Shallow Bahamian Lagoon. Bull. Mar. Sci. 43, 61–67.
Guttridge, T. L., Gruber, S. H., Franks, B. R., Kessel, S. T., Gledhill, K. S., Uphill, J., et al. (2012). Deep danger: intra-specific predation risk influences habitat use and aggregation formation of juvenile lemon sharks Negaprion brevirostris. Mar. Ecol. Prog. Ser. 445, 279–291. doi: 10.3354/meps09423
Hammerschlag, N., Skubel, R. A., Calich, H., Nelson, E. R., Shiffman, D. S., Wester, J., et al. (2017). Nocturnal and crepuscular behavior in elasmobranchs: a review of movement, habitat use, foraging, and reproduction in the dark. Bull. Mar. Sci. 93, 355–374. doi: 10.5343/bms.2016.1046
Heupel, M. R., and Simpfendorfer, C. A. (2005). Using acoustic monitoring to evaluate MPAs for shark nursery areas: the importance of long-term data. Mar. Technol. Soc. J. 39, 10–18. doi: 10.4031/002533205787521749
Heupel, M. R., and Simpfendorfer, C. A. (2015). Long-term movement patterns of a coral reef predator. Coral Reefs 34, 679–691. doi: 10.1007/s00338-015-1272-4
Hight, B. V., and Lowe, C. G. (2007). Elevated body temperatures of adult female leopard sharks, Triakis semifasciata, while aggregating in shallow nearshore embayments: evidence for behavioural thermoregulation? J. Exp. Mar. Biol. Ecol. 352, 114–128. doi: 10.1016/j.jembe.2007.07.021
Hillary, R. M., Bravington, M. V., Patterson, T. A., Grewe, P., Bradford, R., Feutry, P., et al. (2018). Genetic relatedness reveals total population size of white sharks in eastern Australia and New Zealand. Sci. Rep. 8:2661. doi: 10.1038/s41598-018-20593-w
Hunter, E., Berry, F., Buckley, A., Stewart, C., and Metcalfe, J. (2006). Seasonal migration of thornback rays and implications for closure management. J. App. Ecol. 43, 710–720. doi: 10.1111/j.1365-2664.2006.01194.x
Ivlev, V. S. (1961). Experimental Ecology of the Feeding of Fishes. New Haven, CT: Yale University Press.
Jacoby, D. M. P., Busawon, D. S., and Sims, D. W. (2010). Sex and social networking: the influence of male presence on social structure of female shark groups. Behav. Ecol. 21, 808–818. doi: 10.1093/beheco/arq061
Jonsson, B., and Jonsson, N. (1993). Partial migration: niche shift versus sexual maturation in fishes. Rev. Fish Biol. Fish 3, 348–365. doi: 10.1007/BF00043384
Kessell, S. T., Chapman, D. D., Franks, B. R., Gedamke, T., Gruber, S. H., Newman, J. M., et al. (2014). Predictable temperature-regulated residency, movement and migration in a large, highly mobile marine predator (Negaprion brevirostris). Mar. Ecol. Prog. Ser. 514, 175–190. doi: 10.3354/meps10966
Ketchum, J. T., Galván-Magaña, F., and Klimley, A. P. (2013). Segregation and foraging ecology of whale sharks, Rhincodon typus, in the southwestern Gulf of California. Environ. Biol. Fish. 96, 779–795. doi: 10.1007/s10641-012-0071-9
Klimley, A. P. (1987). The determinants of sexual segregation in the scalloped hammerhead shark, Sphyrna lewini. Environ. Biol. Fish. 18, 27–40. doi: 10.1007/BF00002325
Knip, D. M., Heupel, M. R., and Simpfendorfer, C. A. (2012). Habitat use and spatial segregation of adult spottail sharks Carcharhinus sorrah in tropical nearshore waters. J. Fish. Biol. 80, 767–784. doi: 10.1111/j.1095-8649.2012.03223.x
Kramer, D. L., and Chapman, M. R. (1999). Implication of fish home range size and relocation of marine reserve function. Env. Biol. Fish. 55, 65–79. doi: 10.1023/A:1007481206399
Last, P. R., and Stevens, J. S. (2009). Sharks and Rays of Australia. Collingwood: CSIRO Publishing.
Lear, K. O., Gleiss, A. C., Whitty, J. M., Fazeldean, T., Alert, J. R., Green, N., et al. (2019). Recruitment of a critically endangered sawfish into a riverine nursery depends on natural flow regimes. Sci. Rep. 9:17071. doi: 10.1038/s41598-019-53511-9
Lyon, B. L., Dwyer, R. G., Pillans, R. D., Campbell, D., and Franklin, C. (2017). Distribution, seasonal movements and habitat utilisation of an endangered shark, Glyphis glyphis from northern Australia. Mar. Ecol. Prog. Ser. 573, 203–213. doi: 10.3354/meps12200
Martin, T. G., Chades, I., Arcese, P., Marra, P. P., Possingham, H. P., and Norris, D. R. (2007). Optimal conservation of migratory species. PLoS ONE 2:e751. doi: 10.1371/journal.pone.0000751
McAuley, R. B., Simpfendorfer, C. A., and Hall, N. G. (2007). A method for evaluating the impacts of fishing mortality and stochastic influences on the demography of two long-lived shark stocks. ICES J. Mar. Sci. 64, 1710–1722. doi: 10.1093/icesjms/fsm146
McCord, M. E., and Campana, S. E. (2003). A quantitative assessment of the diet of the blue shark (Prionace glauca) off Nova Scotia, Canada. J. Northwest Atl. Fish. Sci. 32, 57–63. doi: 10.2960/J.v32.a4
McKibben, J. N., and Nelson, D. R. (1986). Patterns of movement and grouping of gray reef sharks, Carcharhinus amblyrhynchos, at Enewetak, Marshall Islands. Bull. Mar. Sci. 38, 89–110.
McMillan, M. N., Huveneers, C., Semmens, J. M., and Gillanders, B. M. (2019). Partial female migration and cool-water migration pathways in an overfished shark. ICES J. Mar. Sci. 76, 1083–1093. doi: 10.1093/icesjms/fsy181
Mourier, J., Buray, N., Schultz, J. K., Clua, E., and Planes, S. (2013). Genetic network and breeding patterns of a sicklefin lemon shark (Negaprion acutidens) population in the Society Islands, French Polynesia. PLoS ONE 8:e73899. doi: 10.1371/journal.pone.0073899
Mucientes, G. R., Queiroz, N., Sousa, L. L., Tarroso, P., and Sims, D. W. (2009). Sexual segregation of pelagic sharks and the potential threat from fisheries. Biol. Lett. 5:156–159. doi: 10.1098/rsbl.2008.0761
Newman, S. P., Handy, R. D., and Gruber, S. H. (2012). Ontogenetic diet shifts and prey selection in nursery bound lemon sharks, Negaprion brevirostris, indicate a flexible foraging tactic. Environ. Biol. Fish. 95, 115–126. doi: 10.1007/s10641-011-9828-9
Oh, B. Z. L., Thums, M., Babcock, R. C., Meeuwig, J. J., Pillans, R. D., Speed, C., et al. (2017). Contrasting patterns of residency and space use of coastal sharks within a communal shark nursery. Mar. Fresh. Res. 68, 1501–1517. doi: 10.1071/MF16131
Pillans, R. (2003). Negaprion acutidens. The IUCN Red List of Threatened Species: e.T41836A10576957. Available online at: https://dx.doi.org/10.2305/IUCN.UK.2003.RLTS.T41836A10576957.en (accessed August 10, 2020).
Pillans, R. D., Babcock, R. C., Thomson, D. P., Haywood, M. D. E., Downie, R. A., Vanderklift, M. A., et al. (2017). Habitat effects on home range and schooling behaviour in a herbivorous fish (Kyphosus bigibbus) revealed by acoustic tracking. Mar. Fresh. Res. 68, 1454–1467. doi: 10.1071/MF16199
Pillans, R. D., Bearham, D., Boomer, A., Downie, R., Patterson, T. A., Thomson, D., et al. (2014). Multi year observations reveal variability in residence of a tropical demersal fish, Lethrinus nebulosus: implications for spatial management. PLoS ONE 10:e0118869. doi: 10.1371/journal.pone.0118869
Pillans, R. D., Fry, G. C., Steven, A. D. L., and Patterson, T. (2020). Environmental influences on long-term movement patterns of a euryhaline elasmobranch (Carcharhinus leucas) within a subtropical estuary. Estuar. Coast. 2020:8. doi: 10.1007/s12237-020-00755-8
Pillans, R. D., Stevens, J. D., Kyne, P. M., and Salini, J. (2009). Observations on the distribution, biology, short-term movements and habitat requirements of river sharks Glyphis spp. in northern Australia. End. Spec. Res. 10, 321–332. doi: 10.3354/esr00206
Pratt, H. L., and Carrier, J. C. (2001). A review of elasmobranch reproductive behavior with a case study on the nurse shark, Ginglymostoma cirratum. Env. Biol. Fish 60, 157–188. doi: 10.1023/A:1007656126281
Reyier, E. A., Franks, B. R., Chapman, D. D., Scheidt, D. M., Stolen, E. D., and Gruber, S. H. (2014). Regional-scale migrations and habitat use of juvenile lemon sharks (Negaprion brevirostris) in the US South Atlantic. PLoS ONE 9:e88470. doi: 10.1371/journal.pone.0088470
Runge, C. A., Martin, T. G., Possingham, H. P., Willis, S. G., and Fuller, R. A. (2014). Conserving mobile species. Front. Ecol. Environ. 12, 395–402. doi: 10.1890/130237
Simpfendorfer, C. A., Huveneers, C., Steckenreuter, A., Tattersall, A., Hoenner, X., Harcourt, R., et al. (2015). Ghosts in the data: false detections in VEMCO pulse position modulation acoustic telemetry monitoring equipment. Anim. Biotelemetry 3:55. doi: 10.1186/s40317-015-0094-z
Sims, D. W., Nash, J. P., and Morritt, D. (2001). Movements and activity of male and female dogfish in a tidal sea lough: alternative behavioural strategies and apparent sexual segregation. Mar. Biol. 139, 1165–1175. doi: 10.1007/s002270100666
Sims, D. W., Southall, E. J., Richardson, A. J., Reid, P. C., and Metcalfe, J. D. (2003). Seasonal movements and behaviour of basking sharks from archival tagging: no evidence of winter hibernation. Mar. Ecol. Prog. Ser. 248, 187–196. doi: 10.3354/meps248187
Sims, D. W., Witt, M. J., Richardson, A. J., Southall, E. J., and Metcalfe, J. D. (2006). Encounter success of free-ranging marine predator movements across a dynamic prey landscape. Proc. R. Soc. B 273, 1195–1201. doi: 10.1098/rspb.2005.3444
Speed, C. W., Meekan, M. G., Field, I. C., McMahon, C. R., Abrantes, K., and Bradshaw, C. J. A. (2012). Trophic ecology of reef sharks determined using stable isotopes and telemetry. Cor. Reef. 31, 357–367. doi: 10.1007/s00338-011-0850-3
Speed, C. W., Meekan, M. G., Field, I. C., McMahon, C. R., Harcourt, R. G., Stevens, J. D., et al. (2016). Reef shark movements relative to a coastal marine protected area. Reg. Stud. Mar. Sci. 3, 58–66. doi: 10.1016/j.rsma.2015.05.002
Speed, C. W., Meekan, M. G., Field, I. C., McMahon, C. R., Stevens, J. D., McGregor, F., et al. (2011). Spatial and temporal movement patterns of a multi-species coastal reef shark aggregation. Mar. Ecol. Prog. Ser. 429, 261–275. doi: 10.3354/meps09080
Stevens, J. D. (1984). Life-history and ecology of sharks at Aldabra Atoll, Indian Ocean. Proc. R. Soc. Lond. B Biol. Sci. 222, 79–106. doi: 10.1098/rspb.1984.0050
Stevens, J. D. (1990). Further results from a tagging study of pelagic sharks in the North-east Atlantic. J. Mar. Biol. Ass. UK 70, 707–772. doi: 10.1017/S0025315400058999
Stevens, J. D., Bonfil, R., Dulvy, N. K., and Walker, P. A. (2000). The effects of fishing on sharks, rays, and chimaeras (chondrichthyans), and the implications for marine ecosystems. ICES J. Mar. Sci. 57, 476–494. doi: 10.1006/jmsc.2000.0724
Stevens, J. D., Last, P. R., White, W. T., McAuley, R. B., and Meekan, M. G. (2009). Diversity, Abundance and Habitat Utilisation of Sharks and Rays: Final Report to West Australian Marine Science Institute. Hobart: CSIRO.
Vanderklift, M. A., Babcock, R. C., Barnes, P. B., Cresswell, A. C., Feng, M., Haywood, M. D. E., et al. (2020). The oceanography and marine ecology of Ningaloo, a world heritage area. Ocean. Mar. Biol. 58, 143–178. doi: 10.1201/9780429351495-4
Vanderklift, M. A., Boschetti, F., Roubertie, C., Pillans, R. D., Haywood, M. D. E., and Babcock, R. C. (2014). Density of reef sharks estimated by applying an agent-based model to video surveys. Mar. Ecol. Prog. Ser. 508, 201–209. doi: 10.3354/meps10813
Vaudo, J. J., Byrne, M. E., Wetherbee, B. M., Harvey, G. M., and Shivji, M. S. (2017). Long-term satellite tracking reveals region-specific movements of a large pelagic predator, the shortfin mako shark, in the western North Atlantic Ocean. J. Appl. Ecol. 54, 1765–1775. doi: 10.1111/1365-2664.12852
Wallman, H. L., and Bennett, W. A. (2006). Effects of parturition and feeding on thermal preference of Atlantic stingray, Dasyatis sabina (Lesueur). Env. Biol. Fish. 75, 259–267. doi: 10.1007/s10641-006-0025-1
Wetherbee, B. M., Gruber, S. H., and Rosa, R. S. (2007). Movement patterns of juvenile lemon sharks Negaprion brevirostris within Atol das Rocas, Brazil: a nursery characterized by tidal extremes. Mar. Ecol. Prog. Ser. 343, 283–293. doi: 10.3354/meps06920
White, W. T., and Potter, I. C. (2004). Habitat partitioning among four elasmobranch species in nearshore, shallow waters of a subtropical embayment in Western Australia. Mar. Biol. 145, 1023–1032. doi: 10.1007/s00227-004-1386-7
Wood, S. (2017). Generalized Additive Models: An Introduction with R. 2nd ed. London: Chapman and Hall CRC. doi: 10.1201/9781315370279
Keywords: movement, habitat use, migration, acoustic telemetry, reproduction, spatial management, lemon shark
Citation: Pillans RD, Rochester W, Babcock RC, Thomson DP, Haywood MDE and Vanderklift MA (2021) Long-Term Acoustic Monitoring Reveals Site Fidelity, Reproductive Migrations, and Sex Specific Differences in Habitat Use and Migratory Timing in a Large Coastal Shark (Negaprion acutidens). Front. Mar. Sci. 8:616633. doi: 10.3389/fmars.2021.616633
Received: 12 October 2020; Accepted: 11 January 2021;
Published: 05 February 2021.
Edited by:
Adrian C. Gleiss, Murdoch University, AustraliaReviewed by:
Matthew Ajemian, Florida Atlantic University, United StatesAaron Carlisle, University of Delaware, United States
Copyright © 2021 Pillans, Rochester, Babcock, Thomson, Haywood and Vanderklift. This is an open-access article distributed under the terms of the Creative Commons Attribution License (CC BY). The use, distribution or reproduction in other forums is permitted, provided the original author(s) and the copyright owner(s) are credited and that the original publication in this journal is cited, in accordance with accepted academic practice. No use, distribution or reproduction is permitted which does not comply with these terms.
*Correspondence: Richard D. Pillans, richard.pillans@csiro.au