- 1Area Sorveglianza Epidemiologica, Istituto Zooprofilattico Sperimentale della Sicilia (IZSSi), Palermo, Italy
- 2Dipartimento Alimenti, Area Chimica e Tecnologie Alimentari, Istituto Zooprofilattico Sperimentale della Sicilia (IZSSi), Palermo, Italy
- 3Dipartimento per le Attività Sanitarie e Osservatorio Epidemiologico, Assessorato Regionale della Salute Regione Siciliana, Palermo, Italy
- 4Laboratory of Blue Biotechnology & Aquatic Bioproducts, Institut National des Sciences et Technologies de la mer, (INSTM), Tunis, Tunisia
- 5Direction Générale des Services Vétérinaires (DGSV), Tunis, Tunisia
A risk assessment, related to the consumption of farmed sea bass, was carried out by meta-analysis, taking into account the concentration of trace metals (Cd, Pb, Hg) reported in the literature, the estimated weekly intake (EWI), the provisional tolerable weekly intake (PTWI), and the target hazard quotient (THQ). The concentrations of Cd, Pb, and Hg in farmed sea bass marketed in Sicily (Southern Italy) were also assessed by inductively coupled plasma mass spectrometry (ICP-MS) and atomic absorption spectroscopy (AAS) to have screening data for the comparison with literature results. In any case, the results obtained by meta-analysis and by screening were lower than the pre-established legal limits for each metal. The meta-analysis results showed very low levels of Cd (0.031 μg g–1 w.w.), Pb (0.110 μg g–1 w.w.), and Hg (0.023 μg g–1 w.w.). The EWI estimation confirmed safety limits for human health (0.004, 0.015, and 0.003 μg kg–1 for Cd, Pb, and Hg, respectively). Even the THQ demonstrated that farmed sea bass represent a secure food for humans (0.0006, 0.0005, and 0.0048 for Cd, Pb, and Hg, respectively). The comparison with our screening data showed a significant difference only for the Pb levels (p < 0.05). The results obtained were compared with the same indexes relative to other food types (meat, cereals, vegetables, etc.). The EWI and THQ calculated were always lower than same indexes relative to other food types considered.
Introduction
In recent decades, the demand for fish by consumers has increased significantly with the awareness of the positive effects on human health arising from the consumption of aquatic products (Food and Agriculture Organization, 2020a, b).
To overcome this increasing demand, intensive aquaculture systems of different fish species were carried out. The contribution of aquaculture to world food supply of aquatic products has been increasing, in comparison to the decline in fish resources (Christensen et al., 2014). At present, approximately 44% of the fish consumed by humans comes from aquaculture, and this percentage is predicted to reach 52% by 2025 (Food and Agriculture Organization, 2020a).
In the recent past, the Mediterranean aquaculture sector has steadily increased, becoming economically significant for the region (Squadrone et al., 2016), where seabream (Sparus aurata) and sea bass (Dicentrarchus labrax) are the fish species most produced and commercialized (Ferreira et al., 2010; Arechavala-Lopez et al., 2013; Traina et al., 2015; Bella et al., 2018).
In 2017, the total consumption of fresh seafood products mainly marketed in Italy was 336,799 tons; 18,243 tons concerns European sea bass (European Market Observatory for Fisheries and Aquaculture Products, 2018). In the same year, 7,000 tons of farmed sea bass was produced in Italy (European Market Observatory for Fisheries and Aquaculture Products, 2018), and 237 tons of European sea bass was captured in Italy (Food and Agriculture Organization, 2017). Therefore, the percentage of farmed sea bass consumed in Italy was equal to 5.3% of the total fish products consumed, and locally raised sea bass was 38.9% of those consumed.
Fish products represent a major source of polyunsaturated fatty acids (omega 3, omega 6), as well as other essential nutritional factors (proteins, minerals, vitamins) to human health, reducing cholesterol levels and the incidence of heart disease, stroke, and preterm delivery (Graham and Wiener, 1997; Daviglus et al., 2002; Patterson, 2002; Olmedo et al., 2013; Domingo, 2016).
However, there is still apprehension of the consumption of these products because of the high ability of several marine organisms to accumulate contaminants [trace metals, polycyclic aromatic hydrocarbons (PAHs), polychlorinated biphenyls (PCBs), dioxins, etc.], making them one of the most responsible of contaminants intake (Llobet et al., 2003; Usero et al., 2004; Carubelli et al., 2007; Lo Turco et al., 2007; Medeiros et al., 2012). In addition, contaminants such as trace metals could bioaccumulate and biomagnify along the marine trophic chain, reaching the highest levels in predatory fish (Marcotrigiano and Storelli, 2003; Cretì et al., 2010; Storelli and Barone, 2013; Bonsignore et al., 2018). Different studies have shown that the exposure to high levels of these toxic substances may cause immunotoxicity, reproductive deficits, teratogenicity, endocrine toxicity, and carcinogenetic effects (Tchounwou et al., 2012; Bosch et al., 2016).
Farmed fish have the advantage of being reared and harvested under controlled conditions, so that hazards associated with fish consumption can be more easily controlled (Fuentes et al., 2010). Contrasting results have been reported within the reviewed literature: wild sea bass contain a higher proportion of trace metals, such as Hg and Pb, probably as a consequence of pollution of the seawater by industrial discharges (Monti et al., 2005). Wild specimens have lower levels of total PCBs and DDTs, both in the liver and muscle, compared with cultured sea bass (Antunes and Gil, 2004; Carubelli et al., 2007; Fernandes et al., 2007; Lo Turco et al., 2007). Higher exposure to PAHs was also observed in wild sea bass (Fernandes et al., 2007). These differences have been attributed to the level of contaminants present in the diet and different feeding characteristics due to the intensive culture in the farms (Antunes and Gil, 2004). Therefore, this technique is likely to be more related to the feeding history of the fish and/or to the level of contamination of the site where the fish was farmed or caught (Arechavala-Lopez et al., 2013).
Human dietary exposure to trace metals derives, in general, from population dietary habits. For this reason, it is very important to deepen the consequences of contaminants presence in different food types (Marti-Cid et al., 2008; Tsatsakis et al., 2016, 2017; Esposito et al., 2018; Gupta et al., 2019).
The European Food Safety Authority (EFSA) provided updated information on the levels of trace metals found in a range of foods on the European market and estimated exposure using individual data from the Comprehensive European Food Consumption Database (European Food Safety Authority, 2012a, b, c). EFSA also encourage other studies on the identification of the major dietary sources of trace metals, including fish products. Actually, specific data on the possible risk of trace metals exposure related to the consumption of farmed fish products are still lacking.
The present work aimed at assessing the risk of trace metals exposure related to the consumption of farmed sea bass consumed in Italy, by meta-analytic techniques and screening data from farmed sea bass samples marketed in Sicily.
Materials and Methods
Data Screening and Sample Collection
A total of 16 farmed sea bass samples marketed in Sicily (Southern Italy) were collected during March–April 2015 as part of the SecurAqua Project to obtain a screening dataset for the risk assessment. Sea bass were bought not alive by randomly selecting some supermarkets in Sicily. All the samples were analyzed at the residues laboratory of the Istituto Zooprofilattico Sperimentale della Sicilia (IZSSi). Furthermore, these data were compared with the data obtained by meta-analysis based on what was stated on the label. All the 16 farmed sea bass samples came from farms located in Greece [n = 10, Food and Agriculture Organization (FAO) 37.3] and Malta (n = 6, FAO 37.2). The fish samples revealed a mean weight of 390.6 ± 16.2 g. The screening data were expressed in middle bound (MB), lower bound (LB), and upper bound (UB).
Reagents and Standards
All solutions were prepared with analytical ultrapure grade reagents. Water for chromatography LiChrosolv® was purchased from Merck KgaA (Darmstadt, Germany). Ultrapure nitric acid 60% was purchased from Merck KgaA (Darmstadt, Germany). The standard solution was as follows: the multielement calibration solutions were prepared at different concentration levels (0.001–50 μg/L) from 1,000 mg/L single element inductively coupled plasma mass spectrometry (ICP-MS) grade standard from VWR International LTD (Randon, Pennsylvania, United States). For Hg determination, the standards for the instrument calibration were prepared on the basis of monoelement-certified reference solution (VWR, Milan, Italy). A tuning solution for ICP-MS, capable of covering a wide range of masses (Ce, Co, Li, Mg, Tl, and Y, 1 μg/L) was purchased from Agilent Technologies (Santa Monica, CA, United States) to optimize the performance of ICP-MS before use. For the internal standard solution, 100 mg/L standard stock solution of scandium (Sc), yttrium (Y), indium (In), terbium (Tb), rhodium (Rh), lutetium (Lu), Lithium 6 (Li), indium (In), germanium (Ge), and bismuth (Bi) was purchased from Agilent Technologies (Santa Monica, CA, United States). Ultrapure grade carrier gas (Ar, 99.9995% pure) was purchased from SOL S.p.a. (Monza, Mi, Italy). Ultrapure grade dilution gas (He, 99.9995% pure) was purchased from SOL S.p.a. (Monza, Mi, Italy). Ultrapure grade dilution gas (H2 99.9995% pure) was purchased from SOL S.p.a. (Monza, Mi, Italy).
ICP-MS Analysis
Cd and Pb determination was carried out according to Lo Dico et al. (2015). All samples were digested fresh. About 1 g of the samples was transferred into previously decontaminated poly-tetrafluoroethylene-tetrafluoroethylene (PTFE) vessels with 3 ml of 60% ultrapure nitric acid (V/V) and 5 ml of water. The samples digestion was carried out using a microwave digester Multiwave 3000 (Anton-Paar, Graz, Austria) with the digestion conditions listed below:
A first step at 600 W with a ramp for 10 min and a hold stage of 40 min.
A second step at 0 W with and hold time of 15 min.
The extracts were made up to 50 ml of volume with ultrapure water, filtered, and analyzed by 7700× series ICP-MS (Agilent Technologies, Santa Monica CA, United States). The samples extracted were pumped by a peristaltic pump from tubes arranged on an autosampler ASX-500 Series (Agilent Technologies, Santa Monica (CA), United States), combined with a quartz cyclonic spray chamber.
A calibration curve of eight standard points (BlankCal: 0.01–0.05–0.1–0.5–1–5–10–50 μg/L) was made to evaluate the linearity. A pool of digested samples was used for this test. The linearity of the calibration curve was considered acceptable for r2 > 0.999. The instrumental/method limits of detection and quantification (LOD and LOQ) were calculated by the 3σ and 10σ approach. The trueness of the method was assessed using the recovery of three concentration levels (50–100–250 μg/kg, Supplementary Tables 1–3). An acceptance limit between 90 and 110% was chosen.
All the results under the LOQ of the method were considered for the statistical analysis as half of the LOQ values, according to Helsel (2005).
Hg Assessment
The Hg levels were detected according to the protocols of Cammilleri et al. (2018). Each sample (0.09 ± 0.01 g) (w/w) was added into nickel boats and introduced in a DMA-80® direct analyzer (Milestone, Bergamo, Italy). The DMA-80 is based on the principles of sample thermal decomposition, Hg amalgamation, and atomic absorption detection. The instrumental conditions were as follows: combustion temperature, 650°C; catalyst temperature, 565°C; cuvette temperature, 125°C; decomposition temperature, 600°C; start max temperature, 250°C; purge time, 60 s; and time for signal registration, 30 s.
The amount of Hg present in the samples was detected and quantified according to calibration curves of five concentration points (0.050–2 mg kg–1). Hg was quantitatively measured by atomic absorption at 253.65 nm. The method was validated for repeatability and expanded measurement uncertainty according to ISO 17025:2018. LOD and LOQ of the method were also assessed. The validation procedure showed satisfactory results with repeatability values between 0.021 and 0.826 mg/kg for the five concentration points considered. The expanded measurement uncertainty showed a range of values between 0.020 and 0.710. The LOD and LOQ found were 0.041 and 0.050 mg/kg, respectively.
Meta-analysis of Trace Metals in Farmed Sea Bass
Meta-analysis refers to the statistical analysis of the data from independent primary studies focused on the same question, which aims to generate a quantitative estimate of the studied phenomenon (Gopalakrishnan and Ganeshkumar, 2013).
The aims of a meta-analysis are the following:
• increase power of the study by combining information from multiple studies;
• estimate an average effect (a single synthetic effect measure instead of N distinct effect measures);
• identify subsets of studies showing similar results; and
• estimate which features of the studies may have led to differences in the results.
We calculated the concentrations of trace metals in the muscle of farmed sea bass by meta-analytic techniques according to Borenstein et al. (2009) by consulting the government reports and routine reports using MEDLINE, SCOPUS, and the reference of articles. The study included sea bass farmed in Mediterranean countries (Italy, Portugal, Spain, Greece, Turkey, and Malta). The analysis was conducted in accordance with Preferred Reporting Items for Systematic Reviews and Meta-Analyses (PRISMA) (Moher et al., 2009; Figure 1). Studies were selected using the keywords: sea bass and cadmium; sea bass and lead; and sea bass and mercury. The search was limited to studies published in English.
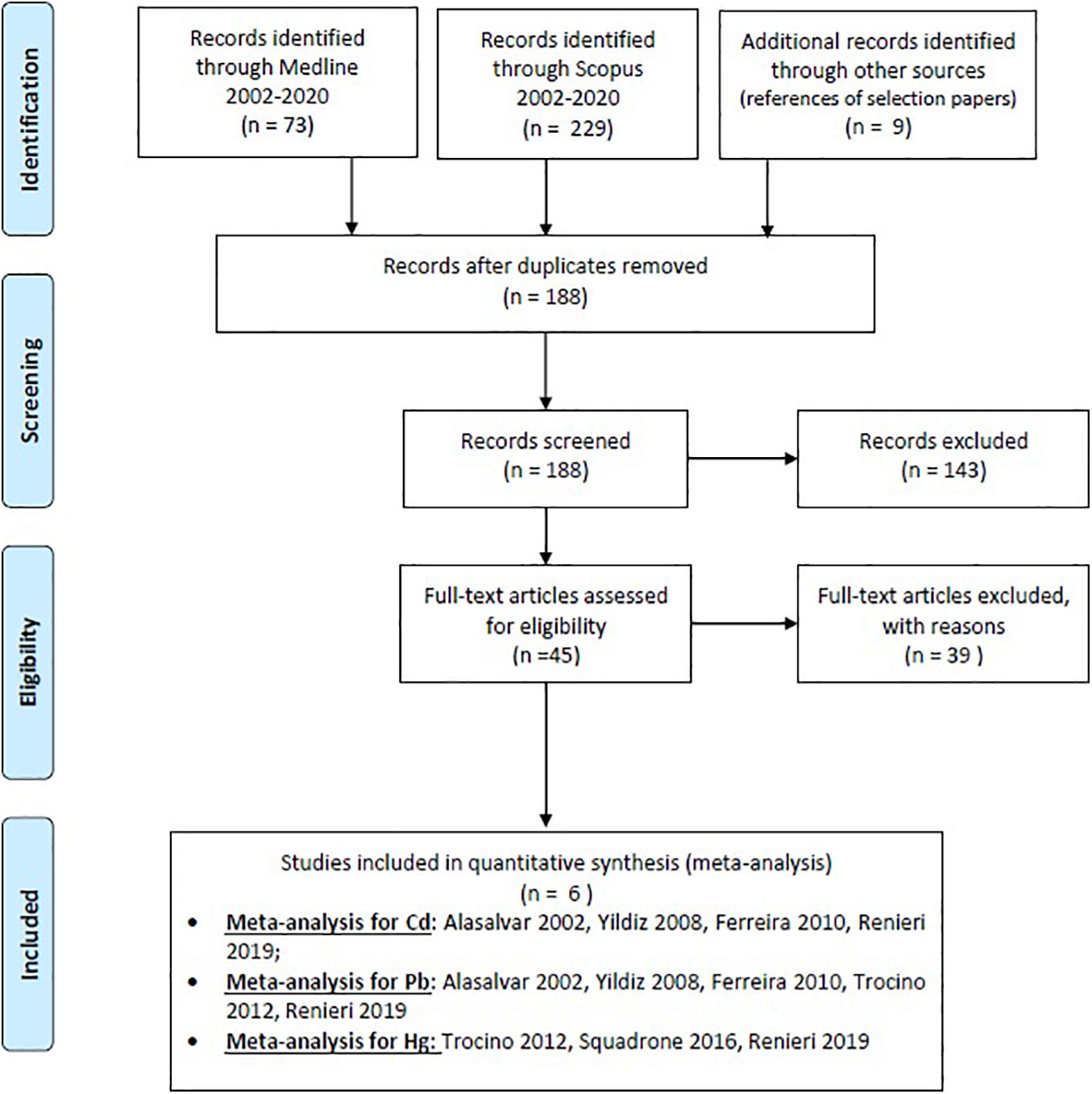
Figure 1. Preferred Reporting Items for Systematic Reviews and Meta-Analyses (PRISMA)—flow diagram for study selection.
We examined 311 articles with the inclusion criteria described below:
• studies on the concentration of trace metals in sea bass (commercial size > 200 g) farmed in the Mediterranean countries published from 2002 to 2020; and
• studies with quantitative estimates of the concentrations of trace metals in the muscle of farmed sea bass (mean, variability, and sample size).
We excluded from meta-analysis the studies presented only in abstract form and not focused on Mediterranean countries. Of the 311 studies examined, only 6 possessed the criteria for inclusion described above and then considered for the meta-analysis (Table 1).
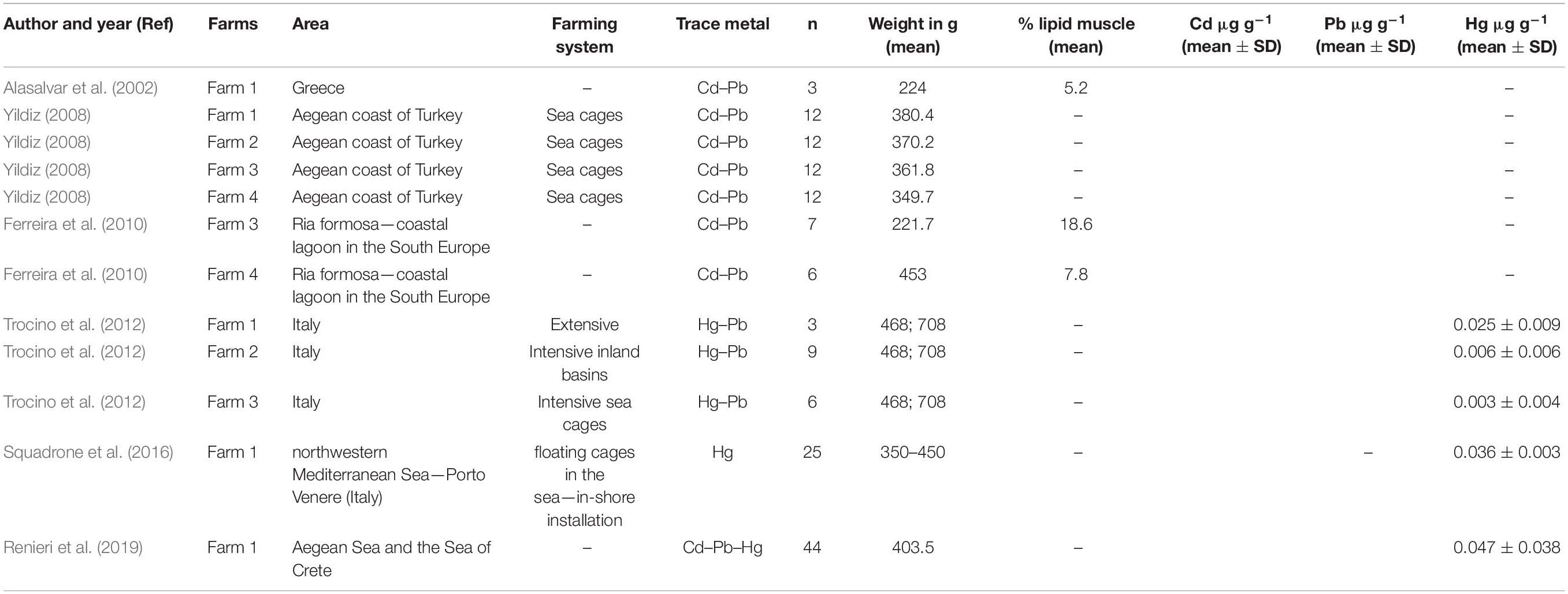
Table 1. Characteristics of the studies included in the meta-analysis for estimate of the concentration of cadmium, lead, and mercury.
A dataset for each contaminant was constructed giving the following variables:
• identification number of the study (doi, PubMed code, etc.);
• author and year of publication; and
• year of the study;
for each study, relative to the farmed sea bass:
• country where the sea bass is farmed;
• detail of farming area;
• type of farming;
• weight of fish (mean and standard deviation or standard error);
• percentage lipid in the muscle (mean and standard deviation or standard error);
• number of samples evaluated; and
• the contaminant concentration (mean and standard deviation or standard error) into the muscle.
All selected studies have outcome with comparable measures and, for this reason, we used the “mean” of concentrations.
In relation to our paper, where we wanted to calculate the overall mean concentration and standard deviation of concentration observed in all of the studies together, we performed that written by Lipsey and Wilson (2001). In fact, these authors showed an example about the effect sizes of arithmetic means (with no control or comparison groups) and how meta-analysis can help to synthesize arithmetic means.
The literature research was conducted by two investigators (RA and AC) independently. Two authors (RA and AC) selected potentially eligible studies for inclusion independently. Disagreements between reviewers were resolved by consensus; when no agreement was reached, a third author (CDB) was selected for the final decision.
Six studies reported the concentration of Cd and/or Pb and/or Hg in farmed sea bass: four of these allowed a meta-analytical assessment for Cd (Alasalvar et al., 2002; Yildiz, 2008; Ferreira et al., 2010; Renieri et al., 2019), five of these allowed a meta-analytical assessment for Pb (Alasalvar et al., 2002; Yildiz, 2008; Ferreira et al., 2010; Trocino et al., 2012; Renieri et al., 2019), and finally, three allowed to observe the Hg concentrations (Trocino et al., 2012; Squadrone et al., 2016; Renieri et al., 2019; Table 1).
Only total mercury (T-Hg) was determined, and Hg levels were expressed as wet weight. The Cd and Pb levels expressed as dry weight (d.w.) were converted to wet weight (w.w.), using the formula reported by Maffucci et al. (2005), cited by Jerez et al. (2010). In particular, we took into account the mean moisture contents reported by Türkka et al. (2008), having weight and length very close to those examined in this study. We used this conversion in order to have a larger number of works for comparison and meta-analysis as possible.
A random-effects model was used to estimate the summarized effect size, assuming heterogeneity always exists. We used a test for heterogeneity to examine the null hypothesis that all studies are evaluating the same effect. The heterogeneity may be attributed to several factors: characteristics of the population (clinical heterogeneity), study design (type of design, selection procedures, data collection methods) (methodological heterogeneity), and different statistical methods (statistical heterogeneity). In the presence of heterogeneity it may not be appropriate to combine results. To verify the presence of heterogeneity, Cochran’s Q test is usually used. In this test, the null hypothesis is that all studies share a common effect size. Under the null hypothesis, Q will follow a central chi-squared distribution with degrees of freedom equal to k - 1, so we can report a p-value for any observed value of Q (Borenstein et al., 2009). A significant Q value indicates that studies show greater variability than can be attributed to a sampling error. In this work, the power of the test could be low for the small number of studies in meta-analysis, so we used I2 statistic to study the heterogeneity of studies, too.
I2 = 100% × (Q - df)/Q, where Q is Cochran’s heterogeneity statistic and df the degrees of freedom.
I2 is expressed as a ratio with a range of 0–100%; it is not directly affected by the number of studies in the analysis, it is a measure of the degree of inconsistency in the studies’ results, and it reflects the percentage of total variation across studies due to heterogeneity (Higgins et al., 2003). Higgins et al. (2003) suggest that values on the order of 25, 50, and 75% might be considered as low, moderate, and high, respectively.
In the random effect model, the differences observed in the studies are attributed both to random and differences between the populations studied or related to the characteristics of the individual studies (study variability and variability between studies). Under the random-effects model, we allow that the true effect could vary from study to study. In a random-effects meta-analysis, we usually assume that the true effects (θi) are normally distributed. To compute a study’s variance under the random-effects model, we need to know both the within-study variance and τ2 (tau-squared), where τ2 is the between-studies variance (the variance of the effect size parameters across the population of studies) (Moher et al., 2009):
where is the within-study variance for study (i), and T2 is a sample estimate of between-studies variance (τ2).
If yi observed effects, then the weighted mean, M∗, is then computed as
that is, the sum of the products (effect size multiplied by weight) divided by the sum of the weights.
The observational nature of the studies used to the meta-analysis did not permit any method of the methodological quality assessment (i.e., about the risk of bias in individual studies or by studies or other quality elements).
A comparative analysis between the concentration of trace metal in meta-analysis and IZSSi’s screening was conducted with Student’s t-test (two tailed).
Statistical analysis was performed using the software SAS 9.4 (Statistical Analysis Software 9.4, SAS Institute Inc., Cary, North Carolina, United States) and R Statistical Software (version 4.0.3; R Foundation for Statistical Computing).
Method for Risk Evaluation
The risk evaluation regarding the presence of trace metals in fish products was carried out on the basis of EFSA’s indexes and the US Environmental Protection Agency (US EPA) method [target hazard quotient (THQ)]. In particular, we used:
(I) EFSA’s indexes:
• Provisional tolerable weekly intake (PTWI).
PTWI estimates the maximum amount per unit body weight of a potentially harmful substance or contaminant in food or water that can be ingested weekly without risk of adverse health effects. The PTWI for each trace metal was fixed following a joint study between FAO/WHO Expert Committee on Food Additives (World Health Organization and Food and Agriculture Organization, 2011). In particular:
• PTWI Cd: 2.5 μg/kg—body weight (neurotoxicity, nephrotoxicity, carcinogenic, etc.);
• PTWI Pb: 25 μg/kg—body weight (neurotoxicity, nephrotoxicity, etc.);
• PTWI Hg: 4 μg/kg—body weight (neurotoxicity, nephrotoxicity, carcinogenic, teratogenic, etc.).
The assessment of exposure to trace metals (Cd, Pb, and Hg) is calculated using the estimated weekly intake (EWI) (Storelli and Barone, 2013; Barone et al., 2015) with the following formula:
where:
Cm = trace metal concentration;
WFC = weekly food consumed;
BW = average body weight (70 kg).
The EWI calculation was carried out considering the Italian per capita weekly consumption (WFC = 9.83 g/week) (European Market Observatory for Fisheries and Aquaculture Products, 2019) and the Cd, Pb, and Hg concentration derived from the meta-analysis of data obtained from the scientific literature and the screening data.
The EWI was also compared with the PTWI of each metal and expressed as a percentage (Storelli and Barone, 2013).
(II) US EPA index:
THQ, calculated by the formula:
where EF is exposure frequency (365 days/year); ED is the exposure duration (80 years), equivalent to the average lifetime; FIR is the per capita weekly consumption of farmed sea bass for Italian population (1.40 g/day person) (European Market Observatory for Fisheries and Aquaculture Products, 2019); C is the metal concentration in farmed sea bass (μg g–1); RfD is the reference oral dose, in μg/kg BW/day (0.1 for Hg, 1 Cd, and 3.57 for Pb) (United States Environmental Protection Agency, 1989, 2000, 2004); BW is the average body weight (70 kg); and AT is the averaging exposure time for non-carcinogens (365 days/year ∗ ED). A safety level is considered below 1.
Results
Estimate of Trace Metals by Meta-analysis and Screening
At first, 311 potentially eligible articles were retrieved (73 in Medline, 229 in Scopus, and 9 in other source, Figure 1). We excluded 123 articles because they were duplicates; 143 articles were removed because they were not pertinent with the aim of meta-analysis (studies presented only in abstract form or not focused on Mediterranean countries or not focused on Cd, Pb, and Hg). Thirty-nine pertinent studies were removed because they did not respect all inclusion criteria. Finally, only six studies were used for meta-analysis:
• Meta-analysis for Cd (Alasalvar et al., 2002; Yildiz, 2008; Ferreira et al., 2010; Renieri et al., 2019);
• Meta-analysis for Pb (Alasalvar et al., 2002; Yildiz, 2008; Ferreira et al., 2010; Trocino et al., 2012; Renieri et al., 2019); and
• Meta-analysis for Hg (Trocino et al., 2012; Squadrone et al., 2016; Renieri et al., 2019).
The main objectives of the selected studies were different from our aim: Alasalvar et al. (2002); Yildiz (2008), Ferreira et al. (2010), and Renieri et al. (2019) investigated the differences between cultured and wild sea bass in relation to concentration of trace metals and other elements. In these cases, we only used the information from farmed fish in relation to the concentration of Cd, Pb, and Hg. Ferreira et al. (2010) investigated the differences between cultured and wild sea bass at different growth stages in the aquaculture production, and for this reason, we used only the values of concentration of trace metal in farmed sea bass with commercial size (>200 g). Yildiz (2008) evaluated the differences between farmed sea bass (cultured in the sea cages) and wild sea bass in four farms that differ by feed type and feeding level. Moreover, starting with differences between cultured and wild sea bass, Renieri et al. (2019) estimated the potential health risk for consumers. Trocino et al. (2012) and Squadrone et al. (2016) investigated farmed sea bass cultured in Italy. Trocino et al. (2012) evaluated metal and other contamination in European sea bass from farms characterized by different rearing systems (extensive lagoons, intensive inland basins, intensive sea cages).
In Alasalvar et al. (2002), there were no significant differences between cultured and wild sea bass in relation to concentrations of Cd and Pb in the muscle, and the concentrations in farmed sea bass were 0.27 ± 0.07 μg g–1 (data were expressed as mean ± SD on a dry weight basis) and 1.03 ± 0.20 μg g–1, respectively for Cd and Pb.
In Yildiz (2008), there were significant differences observed between cultured and wild sea bass in any farm (Farms 1, 2, 3, and 4) in relation to concentration of Cd and Pb in the muscle, and the concentrations of Cd in farmed sea bass were 0.3 ± 0.03 (Farm 1), 0.4 ± 0.02 (Farm 2), 0.1 ± 0.01 (Farm 3), and 0.2 ± 0.01 (Farm 4). Data were expressed in mg kg–1 as mean ± SD on a dry weight basis. Instead, the concentration of Pb in farmed sea bass were 0.9 ± 0.05 (Farm 1), 1.2 ± 0.13 (Farm 2), 0.8 ± 0.03 (Farm 3), and 0.7 ± 0.01 (Farm 4); data were expressed in mg kg–1 as mean ± SD on a dry weight basis.
In Ferreira et al. (2010), significant differences were observed between cultured and wild sea bass only in sea bass with no commercial size that were excluded by meta-analysis group, and the concentrations of Cd in muscle of farmed sea bass were 0.0037 ± 0.0002 (Farm III), 0.0024 ± 0.0010 (Farm IV); values were presented in μg g–1 as mean ± SE on a dry weight basis.
In Renieri et al. (2019), wild sea bass presented significantly higher mean values of Pb than farmed. This study has a higher samples size. The concentration of Cd in the muscle of farmed sea bass was 0.001 ± 0.022 (data was expressed in ng mg–1 as mean ± SD on wet weight basis), the concentration of Pb was 0.056 ± 0.094 (data was expressed in ng mg–1 as mean ± SD on wet weight basis), and the concentration of Hg was 0.047 ± 0.038 (data was expressed in ng mg–1 as mean ± SD on wet weight basis).
In Trocino et al. (2012), Hg levels were significantly (p < 0.001) higher in the sea bass caught in extensive systems (0.025 ± 0.009 mg kg–1 wet weight, Farm F1) compared with fish reared in intensive inland (0.006 ± 0.006 mg kg–1 wet weight, Farm F2) and sea cages systems (0.003 ± 0.004 mg kg–1 wet weight, Farm F3). However, Hg concentration was below the maximum regulatory level for food products (0.5 mg kg–1 w.w.) (European Commission, 2006). Pb concentrations in the muscle of farmed sea bass were not significantly different in the three different farm: 0.065 ± 0.078 (Farm F1, extensive lagoons), 0.110 ± 0.103 (Farm F2, intensive inland basins), and 0.022 ± 0.049 (Farm F3, intensive sea cages), data were expressed in mg kg–1 as mean ± SD on wet weight basis. These values were far below the maximum admitted level of 0.3 mg/kg w.w. (European Commission, 2006).
In Squadrone et al. (2016), toxic metals such as Cd, Pb, and Hg were found at levels below the current maximum limits set by the European Community for sea bass. Only the concentration of Hg was evaluated in our meta-analysis because the concentrations of Cd and Pb in the muscle of sea bass that were reported in paper were not useful. Hg concentrations in fish muscle were 0.036 ± 0.003 mg kg–1 wet weight.
Table 1 shows the characteristics of selected studies and the concentrations of Cd, Pb, and Hg used in the meta-analysis after carrying out the necessary conversions (all data were expressed as mean ± SD in μg g–1 on wet weight basis).
A total of 108 sea bass were involved in meta-analysis of the concentration of Cd, 126 in meta-analysis of the concentration of Pb, and 87 in meta-analysis of the concentration of Hg.
A random-effects model was used to estimate the summarized effect size, assuming heterogeneity always exists. Random-effects model considers both within- and between-study variations for the observed heterogeneity between studies (Borenstein et al., 2009). The meta-analysis of Cd, Pb, and Hg levels showed a significant Q values [respectively, QCd = 11,579.09, df(Q) = 7, p < 0.00001; QPb = 23,004.37, df(Q) = 10, p < 0.00001; QHg = 526.89, df(Q) = 4, p < 0.00001] and = 99.9%, = 99.9% and = 99.2% that indicated a high inconsistency in the studies’ results. The forest plots in Figures 2A–C show the results of meta-analysis, respectively, of concentration of Cd, Pb, and Hg in the muscle of sea bass.
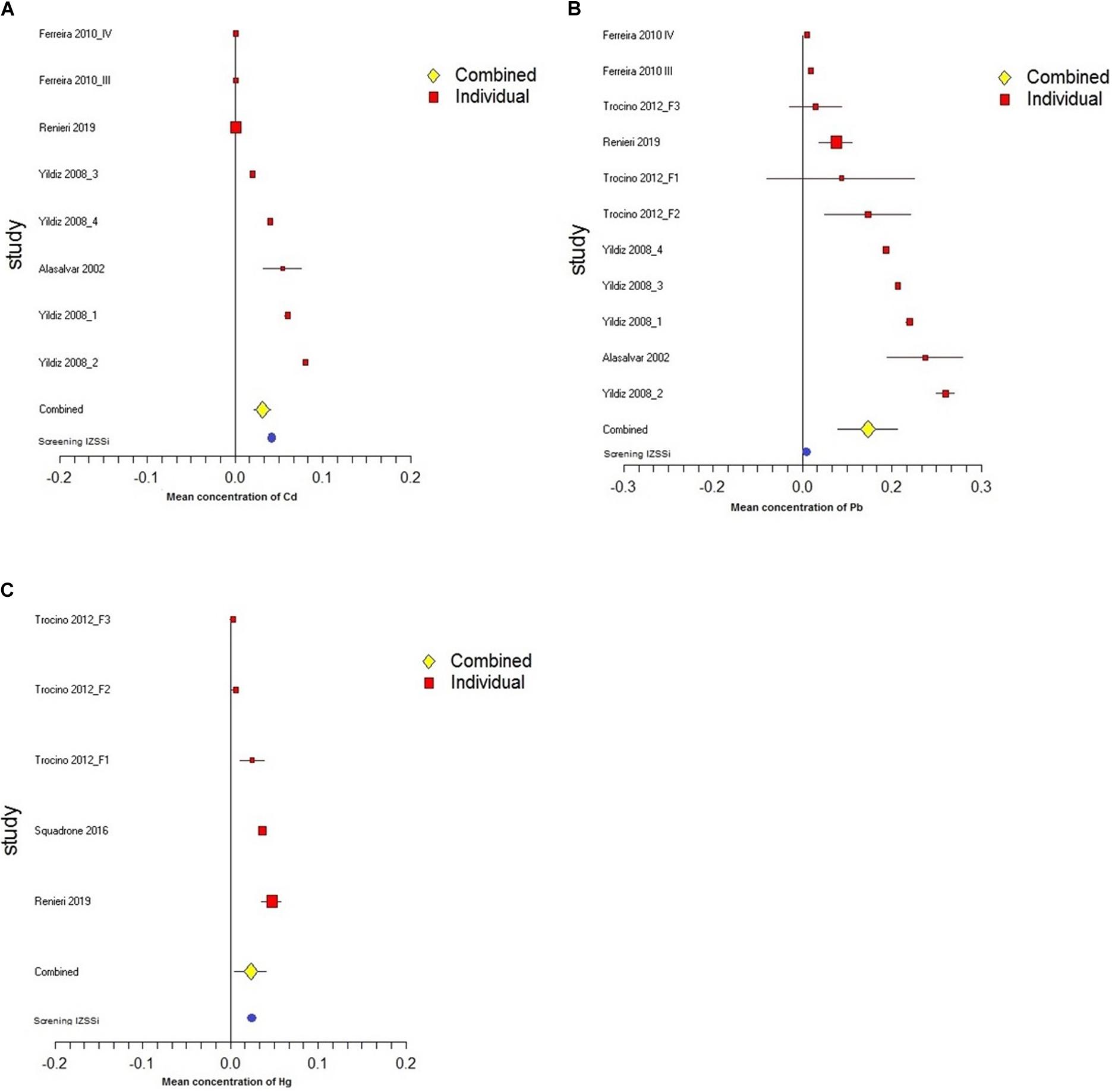
Figure 2. Forest plot of meta-analysis of concentration of (A) Cd, (B) Pb, and (C) Hg. Solid diamonds and horizontal lines indicate the study-specific mean concentrations (μg g– 1 w.w.) and 95% CIs. The size of the area reflects the study-specific statistical weight. The vertical solid line shows the mean effect of 0.
The meta-analysis data, representative of various regions in the Mediterranean Sea, have demonstrated the poor contamination by trace metals of sea bass farmed in the Mediterranean area.
In IZSSi’s screening, a total of 16 fish samples of farmed sea bass were bought not alive by randomly selecting some supermarkets in Sicily: 10 sea bass samples came from farms located in Greece (FAO 37.3), and 6 sea bass came from farms located in Malta (FAO 37.2). The mean weight ± SD of farmed sea bass was 390.6 ± 16.2 g.
Cd was detected (>LOD) in three samples (18.8%), Pb in six (37.5%), while Hg was detected < LOD in all samples. With respect to Cd, mean measured concentration (>LOD) was 0.22 ± 0.028 μg g–1, while with respect to Pb, mean measured concentration (>LOD) was 0.014 ± 0.007 μg g–1. For the undetected metals in fish samples, LOD/2 was imputed for purposes of the statistical analysis. The small sample size did not allow the comparison between sea bass samples that came from farms located in Greece and sea bass samples that came from farms located in Malta.
The IZSSi’s screening results showed that the estimates of the concentrations of Cd and Pb were in agreement with the meta-analysis data. To be more specific, the estimate of Cd by meta-analysis was lower than screening (0.031 μg g–1 w.w. for meta-analysis and 0.042 μg g–1 w.w. for screening), but the difference was not statistically significant (p = 0.2, t-test); the Hg results, obtained in both cases, were quite similar (0.024 μg g–1 w.w. for meta-analysis and 0.025 μg g–1 w.w. for screening, p = 0.4). Instead, there was a significant difference about the estimate of Pb (p < 0.0001, t-test), between meta-analysis data and screening data: 0.11 μg g–1 w.w. for meta-analysis and 0.007 μg g–1 w.w. for screening.
In any case, the results obtained by meta-analysis and by screening were lower than the pre-established legal limits for each metal (Table 2).
The results shown in Table 2 were used, with the data of per capita consumption of sea bass farming, to calculate the indexes of risk evaluation for each metal (EWI and THQ, Table 3).
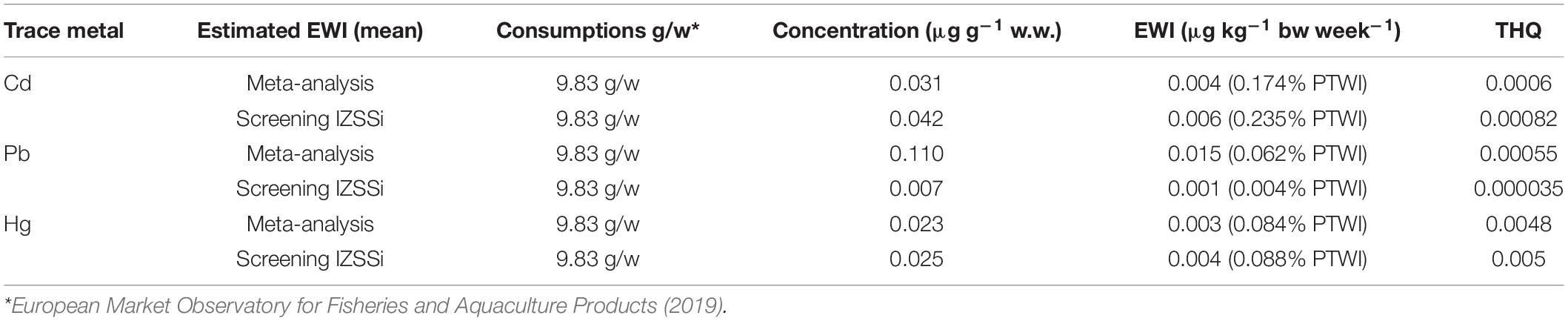
Table 3. Estimated weekly intakes (EWIs and EWIs compared to PTWIs) and target hazard quotients (THQs) for individual metals (Cd, Pb, and Hg) by consumption and concentrations observed in meta-analysis and screening of farmed sea bass.
EWI and THQ Assessment
Table 3 shows the results related to the EWI and THQ calculation for Cd, Pb, and Hg, considering the concentrations obtained from the meta-analysis and screening. The EWI was also compared with the specific PTWI of each metal and expressed as a percentage. This percentage allowed us to determine the impact that the consumption of a given food has on PTWI (Storelli and Barone, 2013; Renieri et al., 2019).
Similar values were found in both the results obtained by meta-analysis and screening, especially for Hg (0.084 and 0.88% of PTWI). Also for Cd, the difference were minimal (0.174 and 0.235% of PTWI), whereas the differences for Pb were marked (0.062 and 0.004% of PTWI). However, the weekly intake of each trace metal was minimal compared with the PTWI.
The results of this work showed a negligible health risk for consumers related to the consumption of farmed sea bass. The comparison with PTWI showed values largely below critical levels, almost insignificant. Even the calculation of THQ revealed very low values, giving a range of 0.000035 and 0.005, up to 28,000 times lower than the values considered safe by the US EPA (0.1). As confirmed by Kalogeropoulos et al. (2012), THQ values between 0.1 and 1.0 prove a low hazard, indicating that the level of daily exposure is unlikely to cause any adverse effects during a person’s lifetime (Chien et al., 2002; Jović and Stanković, 2014; Marengo et al., 2018). In fact, THQ values higher than 1.0 are considered potentially hazardous to human health.
Dietary Exposure to Trace Metals in Various Food
Figure 3 shows the EWI and the THQ about different foods. We calculated the EWI and the THQ for different kinds of food using EFSA data of consumption. In particular, we considered the main foodstuffs in Mediterranean diet: cereals and cereal products, meat and meat products, and vegetables.
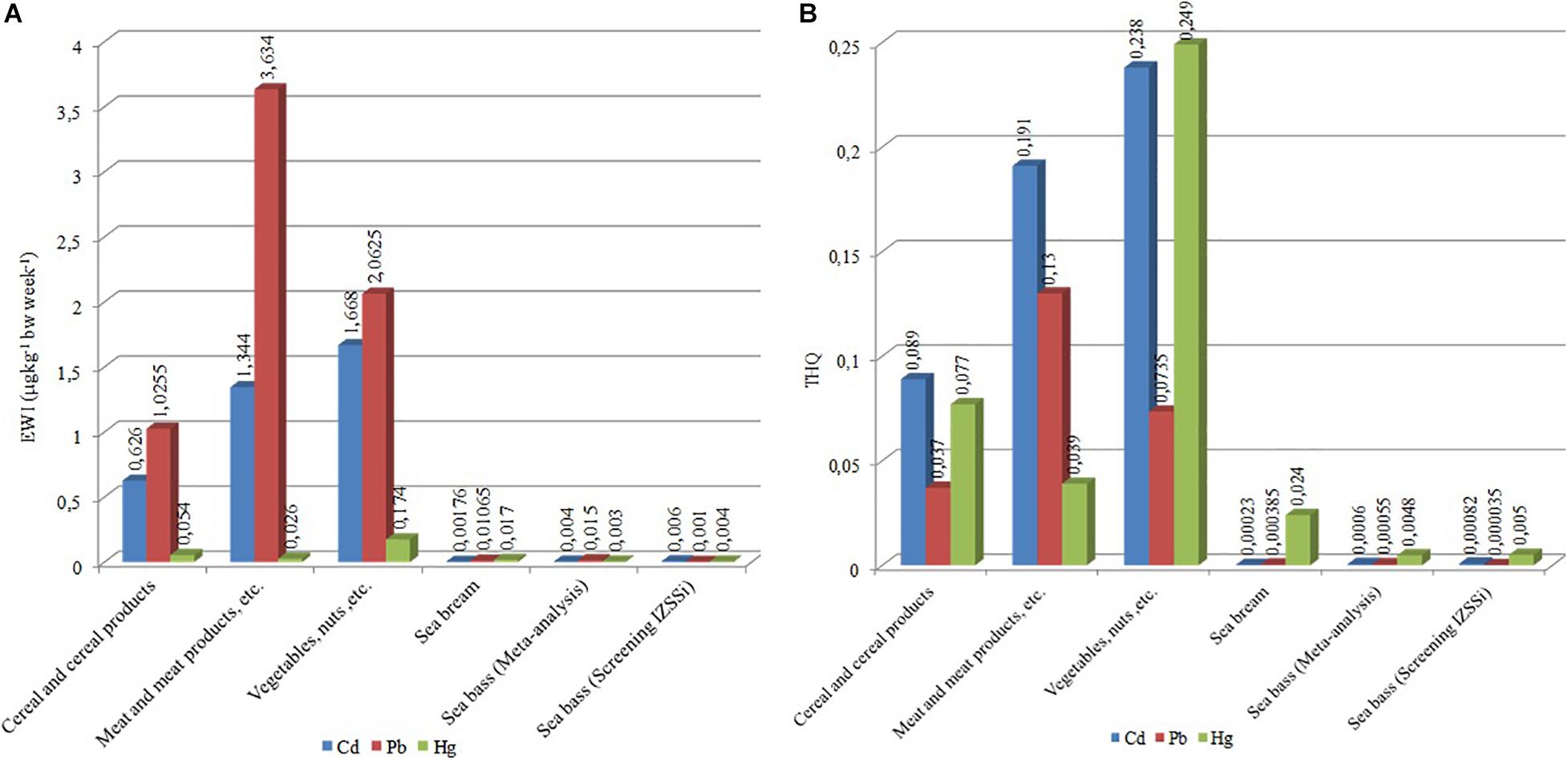
Figure 3. (A) Estimated weekly intakes (EWIs) (μg kg–1 bw week–1) and (B) target hazard quotients (THQs) for Cd, Pb, and Hg in different foods.
The results showed that the intake of Cd is particularly high in vegetables (EWI = 1.67 μg kg–1 bw week–1, Figure 3A and Table 4), representing 66.7% of PTWI. In other words, the weekly Cd intake related to the vegetable consumption represents 66.7% of the safety limit (PTWI). High values of Cd are obtained also in meat, EWI = 1.34 μg kg–1 bw week–1 (53.8% of PTWI), and in cereals, EWI = 1.34 μg kg–1 bw week–1 (25% of PTWI). Even the comparison for Pb produced values higher than what was found for sea bass results (Figure 3A). Differently from Cd and Pb, the differences are less evident for Hg results; the values of EWI always remain low (cereal, 0.054 μg kg–1 bw week–1; meat, 0.026 μg kg–1 bw week–1; vegetables, 0.174 μg kg–1 bw week–1, Table 4). It is well known that, in aquatic ecosystems, inorganic Hg (II) is microbiologically converted in the readily bioaccumulated toxic organometallic species methyl Hg (CH3Hg+). Unlike inorganic Hg (II), CH3Hg+ undergoes biomagnification up the aquatic food chain; hence, most of the Hg in fish consists of CH3Hg+, although inorganic Hg predominates in associated water. Elevated CH3Hg+ concentrations in fish are found not only in Hg-contaminated habitats but also in virtually unpolluted ones where conditions favoring CH3Hg+ production occur (Jackson, 1998). Finally, we calculated the THQ in different food types (Table 4); the values obtained are higher than THQ values obtained for farmed sea bass (Figure 3B). However, these values remain below the hazard levels for human health (<1).
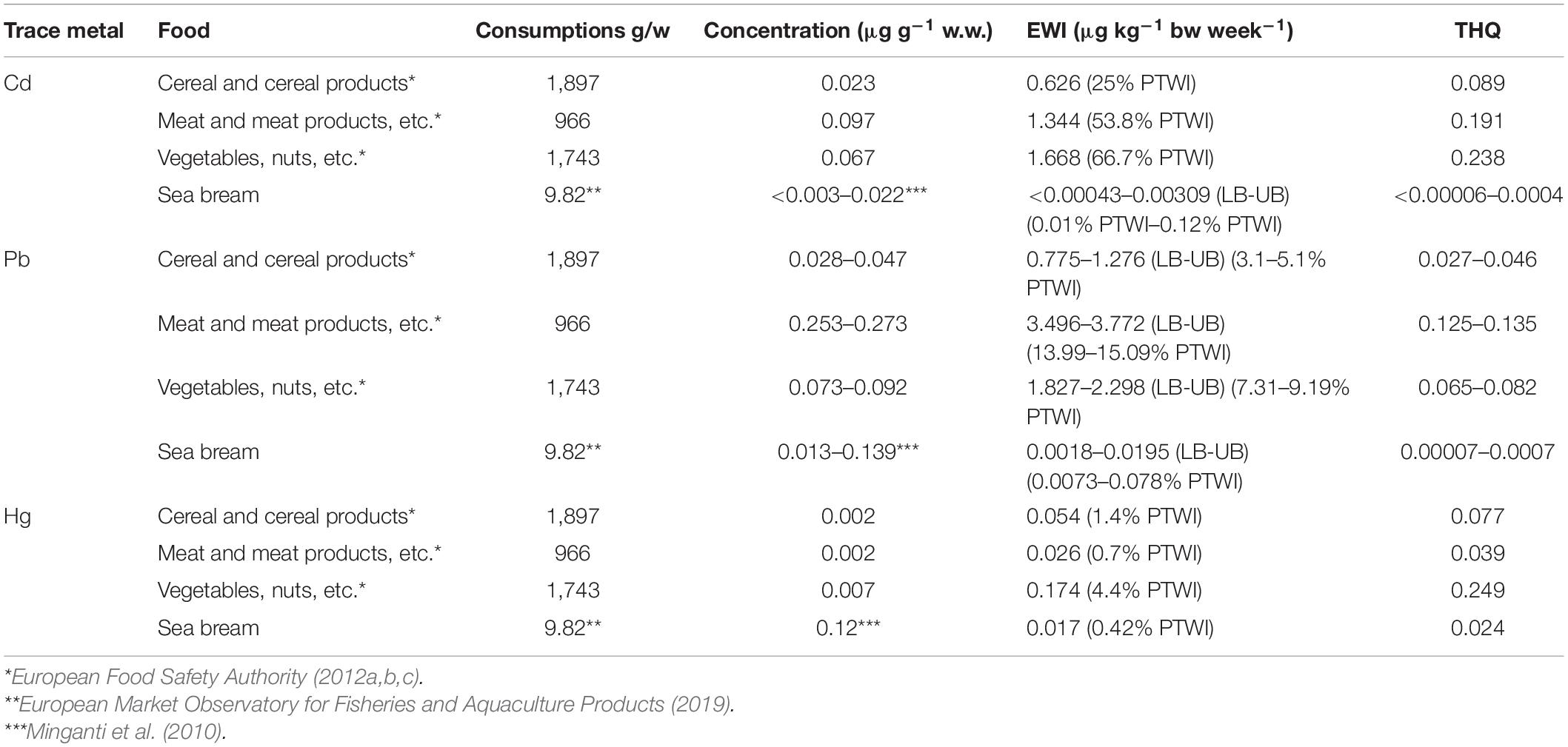
Table 4. Estimated weekly intakes (EWIs and EWIs compared to PTWIs) and target hazard quotients (THQs) for trace metals (Cd, Pb, and Hg) by consumptions and concentrations for different foods.
Discussion
The aim of this study was the assessment of the risk of trace metals exposure related to the consumption of farmed sea bass consumed in Italy focusing on three trace metals (Cd, Pb, and Hg). This assessment was performed with a systematic review of the scientific literature concerned sea bass farmed in Mediterranean countries, a meta-analysis of data of the selected literature, screening data from farmed sea bass samples marketed in Sicily, and finally a risk evaluation on the basis of EFSA’s indexes and US EPA method (THQ).
To the best of our knowledge, this was the first study based on meta-analytic techniques for the risk assessment of trace metals related to the consumption of farmed sea bass in Italy. It is important to highlight the small number of studies selected for meta-analysis, and this fact implies the need to treat the subject in a more exhaustive way with new studies that could strengthen the conclusions observed so far. On the other hand, despite the small number of studies, often characterized by a very small sample size (only the most recent studies have a larger sample size), and despite the heterogeneity of the objectives of the selected studies, the results of this work are not discordant. This enables to consider the information of the selected studies very reliable, and consequently, the results of meta-analysis can be a good synthesis of values of concentration of Cd, Pb, and Hg in Mediterranean farmed sea bass.
Several studies indicated that the concentration of trace metals in fish was influenced by various factors such as seasonal and biological differences (species, size, dark/white muscle, age, sex, and sexual maturity), food sources, and environment (water chemistry, salinity, temperature, contaminants, etc.) (Bodsha and Sainsbyry, 1978; Farmer et al., 1979; Maage et al., 1991; Lal, 1995; Berntssen et al., 1999; Hossain and Furuichi, 2000; Storebakken et al., 2000; Alasalvar et al., 2002; Apines et al., 2003; Vangen and Hemre, 2003; Helland et al., 2005; Roy and Lall, 2006; Ye et al., 2006; Storelli, 2008; Yildiz, 2008; Martignago et al., 2009; Ferreira et al., 2010; Medeiros et al., 2012; Trocino et al., 2012; Renieri et al., 2014, 2019; Rodríguez-Hernández et al., 2017; Copat et al., 2018; Nasyitah Sobihah et al., 2018). The differences observed in concentrations of Cd, Pb, and Hg of the selected studies for meta-analysis and IZSSi’s screening could be directly related to different feeds, different rearing systems, and different environments (salinity, temperature, pollutions, etc.) where the fish are farmed. These aspects need to be further developed with larger studies. The study design of Trocino et al. (2012) is very interesting to investigate about factors that can influence the concentration of trace metal in farmed sea bass, but a larger sample size would make the results more reliable. Trocino et al. (2012) observed that the concentrations of metals in sea bass filets did not change with fish size, and they were poorly correlated (0.10 < r < 0.30) with the concentrations of metals in commercial feeds. Moreover, in this study, cadmium was not detectable in the sea bass filets, and mercury concentrations significantly differed between extensively and intensively reared fish. Unfortunately, in our study, we could not observe the environmental aspect, the diet of the fish, and the type of farming: in meta-analysis, the small number of selected studies did not allow further investigation while screening regarded farmed sea bass marketed in Sicily, and we do not have reliable data about feed, rearing systems, and environment.
Our results observed in the screening data are in agreement or comparable with the recent literature on Cd, Hg, and Pb concentrations in the muscle tissues of farmed sea bass, collected from various regions in the Mediterranean Sea (Alasalvar et al., 2002; Yildiz, 2008; Ferreira et al., 2010; Trocino et al., 2012; Iamiceli et al., 2015; Squadrone et al., 2016; Renieri et al., 2019). The concentrations of Cd and Hg observed in farmed sea bass collected for screening were not significantly different with observed summary values in meta-analysis, while the concentration of Pb was considerably lower; a significant difference (p < 0.0001) was observed between the concentration of Pb in sea bass of the screening and summary values in meta-analysis.
This work allowed us to make some observations on the comparison between farmed and wild bass. The Cd levels observed in the screening data of this work are surely lower than what was found by Türkmen et al. (2010, 2011) in wild sea bass from Paradeniz and Yelkoma lagoons in Turkey (0.1 ± 0.01 μg g–1 and 0.67 ± 0.01 μg g–1) but comparable to those reported by Yildiz (2008) in wild sea bass collected from Aegean coasts (0.04 μg g–1), revealing no significant differences between wild and farmed sea bass. On the contrary, the screening data obtained for Hg and Pb are much lower than what was reported in the literature (Abreu et al., 2000; Orban et al., 2002; Türkmen et al., 2010, 2011; Schnitzler et al., 2011; Miniero et al., 2013), confirming the potential variation in Hg and Pb load of fish muscle between farmed and wild fish in Mediterranean Sea assumed by Ferreira et al. (2010). Besides the environment, food is also a pathway to route accumulation for toxic metals. Adult wild sea bass are top predators, capturing shrimps, crabs, squids, and small fish, with higher metal concentrations than aquaculture feedstuffs (Abreu et al., 2000). Furthermore, it has been demonstrated that the difference in trace metals accumulation does not apparently depend on fish size, emphasizing the significance of feeding patterns in trace metals accumulation (Miniero et al., 2013). However, it should be considered that fishmeal is increasingly substituted in farmed fish diets with ingredients of terrestrial origin, which may affect the mineral content (Domínguez et al., 2019).
The 2020 edition of The State of World Fisheries and Aquaculture continues to demonstrate the significant and growing role of fisheries and aquaculture in providing food, nutrition, and employment (Food and Agriculture Organization, 2020b).
The traditional fish supplies have plateaued, and as such, modern aquaculture has become the most plausible means of meeting the gap in food fish supplies for the growing population (De Silva, 2012).
While captured fisheries will remain relevant, aquaculture has already demonstrated its crucial role in global food security, with its production growing at 7.5% per year since 1970 (Food and Agriculture Organization, 2020b). In agreement with the recent literature, our result showed that the consumption of farmed sea bass marketed in Sicily was safe from the point of view of health. Instead, consumers often consider the aquaculture product to be an industrial, standardized, and artificial product, and they mistakenly perceive it as less healthy than the wild product. For this reasons, the consumers are still cautionary with farmed fish consumption and consider that many factors influence the consumption of fish, and one of these factors is information on the product (Verbeke et al., 2008; Hallstein and Villas-Boas, 2013). This study could be useful to increase consumer confidence in the consumption of farmed sea bass.
Moreover, people are focused on the risk of exposure to trace metals through fish consumption, while the risk inherent to other food items is real and more relevant. Indeed, considering our results regarding dietary exposure to trace metals in various food, it is evident that the consumption of products of the Mediterranean diet (cereals and cereal products, meat and meat products, and vegetables) has a greater impact (in terms of EWI, % of PTWI, and THQ) than the consumption of farmed sea bass, taking into consideration the concentration of trace metals and the quantity of product consumed weekly.
Fish and seafood products contain nutrients beneficial for human health, such as omega-3 fatty acids, vitamins, and minerals (Altekruse et al., 1995; Trondsen et al., 2004; Smith and Sahyoun, 2005; Verbeke and Vackier, 2005; Castro-González and Méndez-Armenta, 2008; Pieniak et al., 2009; Food and Agriculture Organization, 2017), and their consumption has been recommended to prevent cardiovascular and other diseases (Cahu et al., 2004; Simopoulos, 2008; Innes and Calder, 2020). For this reason, World Health Organization (2017) recommends eating one to two portions of seafood per week, and the American Heart Association (2017) recommends at least two portions per week, but European consumers do not follow the dietary recommendations of eating two portions of fish per week, of which one should be fatty fish (Kris-Etherton et al., 2003; Pieniak et al., 2008).
The results of the meta-analysis as well as the results of the selected literature and the results of screening and results with regard to dietary exposure (in term of EWI, % of PTWI, and THQ) suggest that a possible increase in per capita consumption of farmed sea bass would not lead to an increase in risk. Dietary exposure to trace metal cannot be attributed only to fish but is a result of consumption of various food (Kostoff et al., 2018). Considering the consumption data and the concentration of trace metals found in farmed sea bass, it represents a secure food for Italian consumers.
By contrast to other studies based on fish consumption, this work took into account exclusively the sea bass Italian consumption rate (European Market Observatory for Fisheries and Aquaculture Products, 2019). This work wants to define the impact of farmed sea bass consumption to consumer’s health regarding the presence of toxic metals, and it also wants to represent a starting point for a total risk assessment in order to have a constant monitoring of trace metals presence in the food. Farmed fish have the advantage of being reared and harvested under controlled conditions, so that hazards associated with fish consumption can be more easily controlled.
It is also clear that there is in the literature a non-conformity of the data, in particular, in relation to the characteristics of the sample and in relation to the methods used for the determination of trace metals. In this regard, we used a conversion (Maffucci et al., 2005; Türkka et al., 2008; Jerez et al., 2010) in order to have comparable values with what was reported in the literature.
The results obtained make it necessary to start epidemiological studies on wild and farmed fish to assess the health risks associated with these chemical and biological contaminants. Indeed, it is very important to reliably estimate the risk through fish consumption considering all factors that influence the concentration of trace metals in fish (seasonal and biological differences, food sources, and environment), although this would involve a complex study design with sample sizes much larger than those present in the scientific literature today. Risk evaluation for consumers performed by Renieri et al. (2019) is a good starting point. In addition, the calculation of total target hazard quotient (TTHQ) is important because the exposure to two or more pollutants may result in additive and/or interactive effects (Bonsignore et al., 2018; Renieri et al., 2019). In this work, the calculation of the TTHQ (Hallenbeck, 1993) suggested the absence of health risks considering the sum of the THQ of each trace metal considered.
Data Availability Statement
The raw data supporting the conclusions of this article will be made available by the authors, without undue reservation, to any qualified researcher.
Ethics Statement
Ethical review and approval was not required for the animal study because this study involving farmed sea bass marketed in Sicily. Sea bass were bought not alive by randomly selecting some supermarkets in Sicily.
Author Contributions
CD, AC, and RA made substantial contributions to the conception and design of the study and conducted the specific bibliographic research for meta-analysis. CD, AC, RA, and GC drafted and critically revised the manuscript for its intellectual content, gave final approval of the version to be published, and agreed to be accountable for all aspects of the work. AC, GC, DL, VC, LB, GB, VF, and GL made substantial contributions to the fishes chemical analyses and data acquisition. CD, AC, PS, GB, SS, IT, and RA worked to preliminary bibliographic research. RA was responsible for statistical analysis. CD coordinated and supervised all the phases of the study and the drafting of the article. All authors read and approved the final version of the manuscript, contributed to the article, and approved the submitted version.
Funding
This work was supported by the Programme de Coopération Transfrontalière Italie- Tunisie 2007–2013, Project “SECURAQUA - Sécurité et Qualité des Produits Aquacoles: le Développement d’une Voie Commune Tuniso-Sicilienne (Projet n°2AS1.3/020) – CUP H78B13000010006.
Conflict of Interest
The authors declare that the research was conducted in the absence of any commercial or financial relationships that could be construed as a potential conflict of interest.
Acknowledgments
We wish to thank the colleagues involved in the scientific collaboration among the Istituto Zooprofilattico Sperimentale della Sicilia (IZSSi), the Institut National des Sciences et Technologies de la mer-Tunisia (INSTM), and the Direction Générale des Services Vétérinaires-Tunisia.
Supplementary Material
The Supplementary Material for this article can be found online at: https://www.frontiersin.org/articles/10.3389/fmars.2021.616488/full#supplementary-material
References
Abreu, S., Pereira, E., Vale, C., and Duarte, A. (2000). Accumulation of mercury in sea bass from a contaminated lagoon (Ria de Aveiro, Portugal). Mar. Pollut. Bull. 40, 293–297. doi: 10.1016/s0025-326x(99)00187-3
Alasalvar, C., Taylor, K. D. A., Zubcov, E., Shahidi, F., and Alexis, M. (2002). Differentiation of cultured and wild sea bass (Dicentrarchus labrax): total lipid content fatty acid and trace mineral composition. Food Chem. 79, 145–150. doi: 10.1016/S0308-8146(02)00122-X
Altekruse, S. F., Timbo, B. B., Headrick, M. L., and Klontz, K. C. (1995). Associations between diet and health behavior: Results from the 1992 Rhode Island behavior risk factor survey. J. Behav. Med. 18, 225–232. doi: 10.1007/BF01857870
American Heart Association (2017). Fish and Omega-3 Fatty Acids. Available online at: http://www.heart.org/HEARTORG/HealthyLiving/HealthyEating/HealthyDietGoals/Fish-and-Omega-3-Fatty-Acids_UCM_303248_Article.jsp#.WWzL_WcUnyA (accessed December 1, 2020)
Antunes, P., and Gil, O. (2004). PCB and DDT contamination in cultivated and wild sea bass from Ria de Aveiro, Portugal. Chemosphere 54, 1503–1507. doi: 10.1016/j.chemosphere.2003.08.029
Apines, M. J. S., Satoh, S., Kiron, V., Watanabe, T., and Aoki, T. (2003). Availability of supplemental amino acid-chelated trace elements in diets containing tricalcium phosphate and phytate to rainbow trout, Oncorhynchus mykiss. Aquaculture 225, 431–444. doi: 10.1016/s0044-8486(03)00307-7
Arechavala−Lopez, P., Fernandez-Jover, D., Black, K. D., Ladoukakis, E., Bayle−Sempere, J. T., Sanchez-Jerez, P., et al. (2013). Differentiating the wild or farmed origin of Mediterranean fish: a review of tools for sea bream and sea bass. Rev. Aquac. 5, 137–157. doi: 10.1111/raq.12006
Barone, G., Storelli, A., Garofalo, R., Busco, V. P., Quaglia, N. C., Centrone, G., et al. (2015). Assessment of mercury and cadmium via seafood consumption in Italy: estimated dietary intake (EWI) and target hazard quotient (THQ). Food. Addit. Contam. A 32, 1277–1286. doi: 10.1080/19440049.2015.1055594
Bella, G. D., Russo, E., Potortì, A. G., Lo Turco, V., Saija, E., Mansour, H. B., et al. (2018). Persistent organic pollutants in farmed European sea bass (Dicentrarchus labrax, Linnaeus, 1758) from Sicily (Italy). Food Addit. Contam. A 35, 282–291. doi: 10.1080/19440049.2017.1372642
Berntssen, M. H. G., Lundebye, A. K., and Maage, A. (1999). Effects of elevated dietary copper concentrations on growth, feed utilisation and nutritional status of Atlantic salmon Salmo salar L. fry. Aquaculture 174, 164–181. doi: 10.1016/s0044-8486(99)00015-0
Bodsha, K. S., and Sainsbyry, M. (1978). Aspects of the biology and heavy metal accumulation of Ciliata mustela. J. Fish. Biol. 12, 213–220. doi: 10.1111/j.1095-8649.1978.tb04167.x
Bonsignore, M., Salvagio Manta, D., Mirto, S., Quinci, E. M., Ape, F., Montalto, V., et al. (2018). Bioaccumulation of heavy metals in fish, crustaceans, molluscs and echinoderms from the Tuscany coast. Ecotoxicol. Environ. Saf. 162, 554–562. doi: 10.1016/j.ecoenv.2018.07.044
Borenstein, M., Hedges, L. V., Higgins, J. P. T., and Rothstein, H. R. (2009). Introduction to Meta-Analysis. Chichester: John Wiley & Sons, Ltd.
Bosch, A. C., O’Neill, B., Sigge, G. O., Kerwath, S. E., and Hoffman, L. C. (2016). Heavy metals in marine fish meat and consumer health: a review. J. Sci. Food Agric. 96, 32–48. doi: 10.1002/jsfa.7360
Cahu, C., Salen, P., and De Lorgeril, M. (2004). Farmed and wild fish in the prevention of cardiovascular diseases: assessing possible differences in lipid nutritional values. Nutr. Metab. Cardiovasc. Dis. 14, 34–41. doi: 10.1016/s0939-4753(04)80045-0
Cammilleri, G., Vazzana, M., Arizza, V., Giunta, F., Vella, A., Lo Dico, G., et al. (2018). Mercury in fish products: what’s the best for consumers between bluefin tuna and yellowfin tuna? Nat. Prod. Res. 32, 457–462. doi: 10.1080/14786419.2017.1309538
Carubelli, G., Fanelli, R., Mariano, G., Nichetti, S., Crosa, G., Calamari, D., et al. (2007). PCB contamination in farmed and wild sea bass (Dicentrarchus labrax L.) from a coastal wetland area in central Italy. Chemosphere 68, 1630–1635. doi: 10.1016/j.chemosphere.2007.04.004
Castro-González, M. I., and Méndez-Armenta, M. (2008). Heavy metals: implications associated to fish consumption. Environ. Toxicol. Pharmacol. 26, 263–271. doi: 10.1016/j.etap.2008.06.001
Chien, L. C., Hung, T. C., Choang, K. Y., Yeh, C. Y., Meng, P. J., Shieh, M. J., et al. (2002). Daily intake of TBT, Cu, Zn, Cd and As for fishermen in Taiwan. Sci. Total Environ. 285, 177–185. doi: 10.1016/S0048-9697(01)00916-0
Christensen, V., Coll, M., Piroddi, C., Steenbeek, J., Buszowski, J., and Pauly, D. (2014). A century of fish biomass decline in the ocean. Mar. Ecol. Prog. Ser. 512, 155–166. doi: 10.3354/meps10946
Copat, C., Grasso, A., Fiore, M., Cristaldi, A., Zuccarello, P., Signorelli, S., et al. (2018). Trace elements in seafood from the Mediterranean sea: an exposure risk assessment. Food Chem. Toxicol. 115, 13–19. doi: 10.1016/j.fct.2018.03.001
Cretì, P., Trinchella, F., and Scudiero, R. (2010). Heavy metal bioaccumulation and metallothionein content in tissues of the sea bream Sparus aurata from three different fish farming systems. Environ. Monit. Assess. 165, 321–329. doi: 10.1007/s10661-009-0948-z
Daviglus, M., Sheeshka, J., and Murkin, E. (2002). Health benefits from eating fish. Comments Toxicol. 8, 345–374. doi: 10.1080/08865140215064
De Silva, S. (2012). Aquaculture – a newly emergent food production sector – and perspectives of its impacts on biodiversity and conservation. Biodivers. Conserv. 21, 3187–3220. doi: 10.1007/s10531-012-0360-9
Domingo, J. L. (2016). Nutrients and chemical pollutants in fish and shellfish. Balancing health benefits and risks of regular fish consumption. Crit. Rev. Food Sci. Nutr. 56, 979–988. doi: 10.1080/10408398.2012.742985
Domínguez, D., Sarmiento, P., Sehnine, Z., Castro, P., Robaina, L., Fontanillas, R., et al. (2019). Effects of copper levels in diets high in vegetable ingredients on gilthead sea bream (Sparus aurata) fingerlings. Aquaculture 507, 466–474. doi: 10.1016/j.aquaculture.2019.04.044
Esposito, F., Nardone, A., Fasano, E., Scognamiglio, G., Esposito, D., Agrelli, D., et al. (2018). A systematic risk characterization related to the dietary exposure of the population to potentially toxic elements through the ingestion of fruit and vegetables from a potentially contaminated area. A case study: the issue of the “Land of Fires” area in Campania region, Italy. Environ. Pollut. 243, 1781–1790. doi: 10.1016/j.envpol.2018.09.058
European Commission (2006). Commission Regulation (EC) No 1881/2006 of 19 December 2006 Setting Maximum Levels for Certain Contaminants in Foodstuffs (Text with EEA relevance). Available online at: https://eur-lex.europa.eu/LexUriServ/LexUriServ.do?uri=OJ:L:2006:364:0005:0024:EN:PDF (accessed September 6, 2020).
European Commission (2014). Commission Regulation (EU) No 488/2014 of 12 May 2014 amending Regulation (EC) No 1881/2006 as Regards Maximum Levels of Cadmium in Foodstuffs Text with EEA Relevance. 32014R0488OJ L. Available online at: https://eur-lex.europa.eu/legal-content/EN/TXT/PDF/?uri=CELEX:32014R0488&from=ET (accessed September 6, 2020).
European Food Safety Authority (2012a). Cadmium dietary exposure in the European population. EFSA J. 10, 2551. doi: 10.2903/j.efsa.2012.2551
European Food Safety Authority (2012b). Lead dietary exposure in the European population. EFSA J. 10, 2831. doi: 10.2903/j.efsa.2012.2831
European Food Safety Authority (2012c). Scientific Opinion on the risk for public health related to the presence of mercury and methylmercury in food. EFSA J. 10:2985. doi: 10.2903/j.efsa.2012.2985
European Market Observatory for Fisheries and Aquaculture Products (2018). Household Consumption of Fresh Products/Main Commercial Species by Country. Available online at: https://www.eumofa.eu/consumption (accessed November 23, 2020)
European Market Observatory for Fisheries and Aquaculture Products (2019). EUMOFA 2019. Available online at: https://www.eumofa.eu/documents/20178/121372/PTAT+Case+Study+-+Seabass+in+the+EU.pdf (accessed September 5, 2020)
European Union (2006). Commission Regulation (EU) 2015/1005 of 25 June 2015 Amending Regulation (EC) No 1881/2006 as Regards Maximum Levels of Lead in Certain Foodstuffs (Text with EEA relevance). Available online at: https://eur-lex.europa.eu/legal-content/EN/TXT/PDF/?uri=CELEX:32015R1005 (accessed September 6, 2020).
Farmer, G. J., Ashfield, D., and Samant, H. S. (1979). Effects of zinc on juvenile Atlantic salmon (Salmo salar): acute toxicity, food intake, growth and bioaccumulation. Environ. Pollut. 19, 103–117. doi: 10.1016/0013-9327(79)90142-3
Fernandes, D., Porte, C., and Bebianno, M. J. (2007). Chemical residues and biochemical responses in wild and cultured European sea bass (Dicentrarchus labrax L.). Environ. Res. 103, 247–256. doi: 10.1016/j.envres.2006.05.015
Ferreira, M., Caetano, M., Antunes, P., Costa, J., Gil, O., Bandarra, N., et al. (2010). Assessment of contaminants and biomarkers of exposure in wild and farmed sea bass. Ecotoxicol. Environ. Saf. 73, 579–588. doi: 10.1016/j.ecoenv.2010.01.019
Food and Agriculture Organization (2003). Heavy Metal Regulations – Faolex. Legal Notice no. 66/2003. Available online at: http://faolex.fao.org/docs/pdf/eri42405.pdf (accessed December 01 2020)
Food and Agriculture Organization (2017). Fisheries and Aquaculture Information and Statistics Branch. Available online at: http://www.fao.org/figis/servlet/SQServlet?file=/usr/local/tomcat/8.5.16/figis/webapps/figis/temp/hqp_6902054250267509720.xml&outtype=html (accessed November 23, 2020)
Food and Agriculture Organization (2020a). FAO Fisheries & Aquaculture - Statistics – Introduction. Available online at: http://www.fao.org/fishery/statistics/en (accessed September 5, 2020)
Food and Agriculture Organization (2020b). The State of World Fisheries and Aquaculture 2020. Quebec City, QC: Food and Agriculture Organization, doi: 10.4060/ca9229en
Fuentes, A., Fernández-Segovia, I., Serra, J. A., and Barat, J. M. (2010). Comparison of wild and cultured sea bass (Dicentrarchus labrax) quality. Food Chem. 119, 1514–1518. doi: 10.1016/j.foodchem.2009.09.036
Gopalakrishnan, S., and Ganeshkumar, P. (2013). Systematic reviews and meta-analysis: understanding the best evidence in primary healthcare. J. Fam. Med. Prim. Care 2, 9–14. doi: 10.4103/2249-4863.109934
Graham, J. D., and Wiener, J. B. (1997). Risk Vs. Risk. Cambridge, MA: Harvard University Press, 352.
Gupta, N., Yadav, K. K., Kumar, V., Kumar, S., Chadd, R. P., and Kumar, A. (2019). Trace elements in soil-vegetables interface: translocation, bioaccumulation, toxicity and amelioration – a review. Sci. Total Environ. 651, 2927–2942. doi: 10.1016/j.scitotenv.2018.10.047
Hallenbeck, W. H. (1993). Quantitative Risk Assessment for Environmental and Occupational Health. Boca Raton, FL: Taylor & Francis.
Hallstein, E., and Villas-Boas, S. B. (2013). Can household consumers save the wild fish? Lessons from a sustainable seafood advisory. J. Environ. Econ. Manag. 66, 52–71. doi: 10.1016/j.jeem.2013.01.003
Helland, S., Refstie, S., Espmark, A., Hjelde, K., and Baeverfjord, G. (2005). Mineral balance and bone formation in fast-growing Atlantic salmon parr (Salmo salar) in response to dissolved metabolic carbon dioxide and restricted dietary phosphorus supply. Aquaculture 250, 364–376. doi: 10.1016/j.aquaculture.2005.03.032
Helsel, D. R. (2005). Nondetects and Data Analysis. Statistics for Censored Environmental Data. Hoboken, NJ: John Wiley & Sons Inc.
Higgins, J. P. T., Thompson, S. G., Deeks, J. J., and Altman, D. G. (2003). Measuring inconsistency in meta-analyses. BMJ 327, 557–560. doi: 10.1136/bmj.327.7414.557
Hossain, M. A., and Furuichi, M. (2000). Essentiality of dietary calcium supplement in fingerling scorpion fish Sebastiscus marmoratus. Aquaculture 189, 155–163. doi: 10.1016/S0044-8486(00)00366-5
Iamiceli, A. L., Ubaldi, A., Lucchetti, D., Brambilla, G., Abate, V., De Felip, E., et al. (2015). Metals in Mediterranean aquatic species. Mar. Pollut. Bull. 94, 278–283. doi: 10.1016/j.marpolbul.2015.02.034
Innes, J. K., and Calder, P. C. (2020). Marine Omega-3 (N-3) fatty acids for cardiovascular health: an update for 2020. Int. J. Mol. Sci. 21:1362. doi: 10.3390/ijms21041362
Jackson, T. A. (1998). “Mercury in aquatic ecosystems,” in Metal Metabolism in Aquatic Environments, eds W. J. Langston and M. J. Bebianno (Boston, MA: Springer), 77–158.
Jerez, S., Motas, M., Cánovas, R. A., Talavera, J., Almela, R. M., and Del Río, A. B. (2010). Accumulation and tissue distribution of heavy metals and essential elements in loggerhead turtles (Caretta caretta) from Spanish Mediterranean coastline of Murcia. Chemosphere 78, 256–264. doi: 10.1016/j.chemosphere.2009.10.062
Jović, M., and Stanković, S. (2014). Human exposure to trace metals and possible public health risks via consumption of mussels Mytilus galloprovincialis from the Adriatic coastal area. Food Chem. Toxicol. 70, 241–251. doi: 10.1016/j.fct.2014.05.012
Kalogeropoulos, N., Karavoltsos, S., Sakellari, A., Avramidou, S., Dassenakis, M., and Scoullos, M. (2012). Heavy metals in raw, fried and grilled Mediterranean finfish and shellfish. Food Chem. Toxicol. 50, 3702–3708. doi: 10.1016/j.fct.2012.07.012
Kostoff, R. N., Goumenou, M., and Tsatsakis, A. (2018). The role of toxic stimuli combinations in determining safe exposure limits. Toxicol. Rep. 5, 1169–1172. doi: 10.1016/j.toxrep.2018.10.010
Kris-Etherton, P. M., Harris, W. S., Appel, L. J., and Comm, A. N. (2003). Omega-3 fatty acids and cardiovascular disease – new recommendations from the American Heart Association. Arterioscler. Thromb. Vasc. Biol. 23, 151–152. doi: 10.1161/01.atv.0000057393.97337.ae
Lal, S. P. (1995). “Macro and trace elements in fish and shellfish,” in Fish and Fishery Products: Composition, Nutritive Properties and Stability, ed. A. Ruiter (Wallingford: CAB International), 187–214.
Llobet, J. M., Falcò, G., Casas, C., Teixidò, A., and Domingo, J. L. (2003). Concentrations of arsenic, cadmium, mercury and lead in common foods and estimated daily intake by children, adolescents, adults and seniors of Catalonia, Spain. J. Agric. Food. Chem. 51, 838–842. doi: 10.1021/jf020734q
Lo Dico, G. M., Cammilleri, G., Macaluso, A., Vella, A., Giangrosso, G., Vazzana, M., et al. (2015). Simultaneous determination of As, Cu, Cr, Se, Sn, Cd, Sb and Pb levels in infant formulas by ICP-MS after microwave-assisted digestion: method validation. J. Environ. Anal. Toxicol. 5:328. doi: 10.4172/2161-0525.1000328
Lo Turco, V., Di Bella, G., La Pera, L., Conte, F., Macro, B., and Dugo, G. (2007). Organochlorine pesticides and polychlorinated biphenyl residues in reared and wild Dicentrarchus labrax from the Mediterranean Sea (Sicily, Italy). Environ. Monit. Assess. 132, 411–417. doi: 10.1007/s10661-006-9543-8
Maage, A., Julshamm, K., and Ulgenes, Y. (1991). A comparison of tissue levels for trace elements in wild and farmed Atlantic salmon (Salmo salar). Fisk. Dir. Skr. Ser Ernaer. 4, 111–116.
Maffucci, F., Caurant, F., Bustamante, P., and Bentivegna, F. (2005). Trace elements (Cd, Cu, Hg, Se, Zn) accumulation and tissue distribution in loggerhead turtles (Caretta caretta) from Western Mediterranean Sea (southern Italy). Chemosphere 58, 535–542. doi: 10.1016/j.chemosphere.2004.09.032
Marcotrigiano, G. O., and Storelli, M. M. (2003). Heavy metal, polychlorinated biphenyl and organochlorine pesticide residues in marine organisms: risk evaluation for consumers. Vet. Res. Commun. 27, 183–195. doi: 10.1023/B:VERC.0000014137.02422.f4
Marengo, M., Durieux, E. D. H., Ternengo, S., Lejeune, P., Degrange, E., Pasqualini, V., et al. (2018). Comparison of elemental composition in two wild and cultured marine fish and potential risks to human health. Ecotoxicol. Environ. Saf. 158, 204–212. doi: 10.1016/j.ecoenv.2018.04.034
Marti-Cid, R., Llobet, J. M., Castell, V., and Domingo, J. L. (2008). Dietary intake of arsenic, cadmium, mercury, and lead by the population of catalonia, Spain. Biol Trace Elem. Res. 125, 120–132. doi: 10.1007/s12011-008-8162-3
Martignago, R., Trinchella, F., Scudiero, R., and Cretì, P. (2009). Cadmium, lead and metallothionein contents in tissues of the sea bream Sparus aurata from three different fish farming systems. Comp. Biochem. Physiol. Mol. Integr. Physiol. 154:S21. doi: 10.1016/J.CBPA.2009.05.076
Medeiros, R. J., Dos Santos, L. M. G., Freire, A. S., Santelli, R. E., Braga, A. M. C., Krauss, T. M., et al. (2012). Determination of inorganic trace elements in edible marine fish from Rio de Janeiro State, Brazil. Food Control 23, 535–541. doi: 10.1016/j.foodcont.2011.08.027
Minganti, V., Drava, G., Pellegrini, R., and De Siccardi, C. (2010). Trace elements in farmed and wild gilthead seabream, Sparus aurata. Mar. Pollut. Bull. 60, 2022–2025. doi: 10.1016/j.marpolbul.2010.07.023
Miniero, R., Beccaloni, E., Carere, M., Ubaldi, A., Mancini, L., Marchegiani, S., et al. (2013). Mercury (Hg) and methyl mercury (MeHg) concentrations in fish from the coastal lagoon of Orbetello, central Italy. Mar. Pollut. Bull. 76, 365–369. doi: 10.1016/j.marpolbul.2013.08.012
Moher, D., Liberati, A., Tetzlaff, J., and Altman, D. G. (2009). PRISMA Group. Preferred reporting items for systematic reviews and meta-analyses: the PRISMA statement. BMJ 339:b2535. doi: 10.1136/bmj.b2535
Monti, G., De Napoli, L., Mainolfi, P., Barone, R., Guida, M., Marino, G., et al. (2005). Monitoring food quality by microfluidic electrophoresis, gas chromatography, and mass spectrometry techniques: effects of aquaculture on the sea bass (Dicentrarchus labrax). Anal. Chem. 77, 2587–2594. doi: 10.1021/ac048337x
Nasyitah Sobihah, N., Ahmad Zaharin, A., Khairul Nizam, M., Ley Juen, L., and Kyoung-Woong, K. (2018). Bioaccumulation of heavy metals in maricultured fish, Lates calcarifer (Barramudi), Lutjanus campechanus (red snapper) and Lutjanus griseus (grey snapper). Chemosphere 197, 318–324. doi: 10.1016/j.chemosphere.2017.12.187
Olmedo, P., Pla, A., Hernándeza, A. F., Barbier, F., Ayouni, L., and Gil, F. (2013). Determination of toxic elements (mercury, cadmium, lead, tin and arsenic) in fish and shellfish samples. Risk assessment for the consumers. Environ. Int. 59, 63–72. doi: 10.1016/j.envint.2013.05.005
Orban, E., Di Lena, G., Nevigato, T., Casini, I., Santaroni, G., Marzetti, A., et al. (2002). Quality characteristics of sea bass intensively reared and from lagoon as affected by growth conditions and the aquatic environment. J. Food Sci. 67, 542–546. doi: 10.1111/j.1365-2621.2002.tb10635.x
Patterson, J. (2002). Introduction–comparative dietary risk: balance the risk and benefits of fish consumption. Comments Toxicol. 8, 337–343. doi: 10.1080/08865140215062
Pieniak, Z., Verbeke, W., Perez-Cueto, F., Brunsø, K., and De Henauw, S. (2008). Fish consumption and its motives in households with versus without medical history of CVD: a consumer survey from five European countries. BMC Public Health 8:306. doi: 10.1186/1471-2458-8-306
Pieniak, Z., Verbeke, W., and Vackier Scholderer, J. (2009). Health-related beliefs and consumer knowledge as determinants of fish consumption. J. Hum. Nutr. Diet. 23, 480–488. doi: 10.1111/j.1365-277X.2010.01045.x
Renieri, E., Alegakis, A., Kiriakakis, M., Vinceti, M., Ozcagli, E., Wilks, M., et al. (2014). Cd, Pb and Hg biomonitoring in fish of the mediterranean region and risk estimations on fish consumption. Toxics 2, 417–442. doi: 10.3390/toxics2030417
Renieri, E. A., Safenkova, I. V., Alegakis, A. K, Slutskaya, E. S., Kokaraki, V., Kentouri, M., et al. (2019). Cadmium, lead and mercury in muscle tissue of gilthead seabream and seabass: Risk evaluation for consumers. Food Chem. Toxicol. 124, 439–449. doi: 10.1016/j.fct.2018.12.020
Rodríguez-Hernández, Á, Camacho, M., Henríquez-Hernández, L. A., Boada, L. D., Valerón, P. F., Zaccaroni, A., et al. (2017). Comparative study of the intake of toxic persistent and semi persistent pollutants through the consumption of fish and seafood from two modes of production (wild-caught and farmed). Sci. Total Environ. 575, 919–931. doi: 10.1016/j.scitotenv.2016.09.142
Roy, P. K., and Lall, S. P. (2006). Mineral nutrition of haddock Melanogrammus aeglefinus (L.): a comparison of wild and cultured stock. J. Fish. Biol. 68, 1460–1472. doi: 10.1111/j.0022-1112.2006.001031.x
Schnitzler, J. G., Thomé, J. P., Lepage, M., and Das, K. (2011). Organochlorine pesticides, polychlorinated biphenyls and trace elements in wild European sea bass (Dicentrarchus labrax) off European estuaries. Sci. Total. Environ. 409, 3680–3686. doi: 10.1016/j.scitotenv.2011.06.018
Simopoulos, A. P. (2008). The importance of the omega-6/omega-3 fatty acid ratio in cardiovascular disease and other chronic diseases. Exp. Biol. Med. 233, 674–688. doi: 10.3181/0711-MR-311
Smith, K. M., and Sahyoun, N. R. (2005). Fish consumption: recommendations versus advisories, can they be reconciled? Nutr. Rev. 63, 39–46. doi: 10.1111/j.1753-4887.2005.tb00120.x
Squadrone, S., Brizio, P., Stella, C., Prearo, M., Pastorino, P., Serracca, L., et al. (2016). Presence of trace metals in aquaculture marine ecosystems of the northwestern Mediterranean Sea (Italy). Environ. Pollut. 215, 77–83. doi: 10.1016/j.envpol.2016.04.096
Storebakken, T., Shearer, K. D., Baeverfjord, G., Nielsen, B. G., Asgard, T., Scott, T., et al. (2000). Digestibility of macronutrients, energy and amino acids, absorption of elements and absence of intestinal enteritis in Atlantic salmon, Salmo salar, fed diets with wheat gluten. Aquaculture 184, 115–132.
Storelli, M., and Barone, G. (2013). Toxic metals (Hg, Pb, and Cd) in commercially important demersal fish from Mediterranean sea: contamination levels and dietary exposure assessment. J. Food Sci. 78, 362–366. doi: 10.1111/j.1750-3841.2012.02976.x
Storelli, M. M. (2008). Potential human health risks from metals (Hg, Cd, and Pb) and polychlorinated biphenyls (PCBs) via seafood consumption: estimation of target hazard quotients (THQs) and toxic equivalents (TEQs). Food Chem. Toxicol. 46, 2782–2788. doi: 10.1016/J.FCT.2008.05.011
Tchounwou, P. B., Yedjou, C. G., Patlolla, A. K., and Sutton, D. J. (2012). Heavy metal toxicity and the environment. Exp. Suppl. 101, 133–164. doi: 10.1007/978-3-7643-8340-4_6
Traina, A., Oliveri, E., Salvagio Manta, D., Barra, M., Mazzola, S., and Cuttitta, A. (2015). Metals content in otoliths of Dicentrarchus labrax from two fish farms of Sicily. Environ. Monit. Assess. 187:360. doi: 10.1007/s10661-015-4434-5
Trocino, A., Xiccato, G., Majolini, D., Tazzoli, M., Tulli, F., Tibaldi, E., et al. (2012). Levels of dioxin-like polychlorinated biphenyls (DL-PCBs) and metals in European sea bass from fish farms in Italy. Food Chem. 134, 333–338. doi: 10.1016/j.foodchem.2012.02.153
Trondsen, T., Braaten, T., Lund, E., and Eggen, A. E. (2004). Health and fish consumption patterns among women 45–69 years. A Norwegian Fish Consumption Study 1996. Food Qual. Prefer. 15, 117–128. doi: 10.1016/S0950-3293(03)00038-7
Tsatsakis, A. M., Docea, A. O., and Tsitsimpikou, C. (2016). New challenges in risk assessment of chemicals when simulating real exposure scenarios; simultaneous multi-chemicals’ low dose exposure. Food Chem. Toxicol. 96, 174–176. doi: 10.1016/j.fct.2016.08.011
Tsatsakis, A. M., Kouretas, D., Tzatzarakis, M. N., Stivaktakis, P., Tsarouhas, K., Golokhvast, K. S., et al. (2017). Simulating real-life exposures to uncover possible risks to human health: a proposed consensus for a novel methodological approach. Hum. Exp. Toxicol. 36, 554–564. doi: 10.1177/096032711668165
Türkka, A. U., Cakli, S., and Kilinc, B. (2008). Effects of cooking methods on the proximate composition and fatty acid composition of seabass (Dicentrarchus labrax, Linnaeus, 1758). Food Bioprod. Process 86, 163–166. doi: 10.1016/j.fbp.2007.10.004
Türkmen, A., Türkmen, M., Tepe, Y., and Çekiç, M. (2010). Metals in tissues of fish from Yelkoma Lagoon, northeastern Mediterranean. Environ. Monit. Assess. 168, 223–230. doi: 10.1007/s10661-009-1106-3
Türkmen, M., Türkmen, A., and Tepe, Y. (2011). Comparison of metals in tissues of fish from paradeniz lagoon in the coastal area of Northern East Mediterranean. Bull. Environ. Contam. Toxicol. 87:381. doi: 10.1007/s00128-011-0381-1
United States Environmental Protection Agency (1989). US EPA. Available online at: https://cfpub.epa.gov/ncea/iris/iris_documents/documents/subst/0141_summary.pdf (accessed September 5, 2020).
United States Environmental Protection Agency (2000). US EPA 2000. Available online at: https://cfpub.epa.gov/ncea/iris/iris_documents/documents/subst/0073_summary.pdf (accessed September 5, 2020).
United States Environmental Protection Agency (2004). US EPA 2004. Available online at: https://cfpub.epa.gov/ncea/iris/iris_documents/documents/subst/0277_summary.pdf (accessed September 5, 2020).
Usero, J., Izquierdo, C., Morillo, J., and Gracia, I. (2004). Heavy metals in fish (Solea vulgaris, Anguilla anguilla and Liza aurata) from salt marshes on the southern Atlantic coast of Spain. Environ. Int. 29, 949–956. doi: 10.1016/S0160-4120(03)00061-8
Vangen, B., and Hemre, G. I. (2003). Dietary carbohydrate, iron and zinc interactions in Atlantic salmon (Salmo salar). Aquaculture 219, 597–611. doi: 10.1016/S0044-8486(02)00206-5
Verbeke, W., and Vackier, I. (2005). Individual determinants of fish consumption: application of the theory of planned behaviour. Appetite 44, 67–82. doi: 10.1016/j.appet.2004.08.006
Verbeke, W., Vanhonacker, F., Frewer, L. J., Sioen, I., De Henauw, S., and Van Camp, J. (2008). Communicating risks and benefits from fish consumption: impact on Belgian consumers’ perception and intention to eat fish. Risk Anal. 28, 951–967. doi: 10.1111/j.1539-6924.2008.01075.x
World Health Organization (2017). 5. Population nutrient intake goals for preventing dietrelated chronic diseases. Available online at: http://www.who.int/nutrition/topics/5_population_nutrient/en/index13.html (accessed December 1, 2020)
World Health Organization, and Food and Agriculture Organization (2011). Evaluation of Certain Food Additives and Contaminants: Seventy-Fourth [74th] Report of the Joint FAO/WHO Expert Committee on Food Additives. Available online at: https://apps.who.int/iris/handle/10665/44788 (accessed September 5, 2020)
Ye, C. X., Liu, Y. J., Tian, L. X., Mai, K. S., Zhen-Yu Du, Z. Y., Hui-Jun Yang, H. J., et al. (2006). Effect of dietary calcium and phosphorus on growth, feed efficiency, mineral content and body composition of juvenile grouper, Epinephelus coioides. Aquaculture 255, 263–271. doi: 10.1016/j.aquaculture.2005.12.028
Keywords: farmed sea bass, trace metals, meta-analysis, estimated weekly intake, provisional tolerable weekly intake, target hazard quotient
Citation: Di Bella C, Calagna A, Cammilleri G, Schembri P, Lo Monaco D, Ciprì V, Battaglia L, Barbera G, Ferrantelli V, Sadok S, Tliba I, Lo Dico GM and Allegro R (2021) Risk Assessment of Cadmium, Lead, and Mercury on Human Health in Relation to the Consumption of Farmed Sea Bass in Italy: A Meta-Analytical Approach. Front. Mar. Sci. 8:616488. doi: 10.3389/fmars.2021.616488
Received: 15 October 2020; Accepted: 12 January 2021;
Published: 22 February 2021.
Edited by:
Lotfi Rabaoui, King Fahd University of Petroleum and Minerals, Saudi ArabiaReviewed by:
Periyadan K. Krishnakumar, King Fahd University of Petroleum and Minerals, Saudi ArabiaRadhouan El Zrelli, Independent Researcher, Aspach-Le-Bas, France
Copyright © 2021 Di Bella, Calagna, Cammilleri, Schembri, Lo Monaco, Ciprì, Battaglia, Barbera, Ferrantelli, Sadok, Tliba, Lo Dico and Allegro. This is an open-access article distributed under the terms of the Creative Commons Attribution License (CC BY). The use, distribution or reproduction in other forums is permitted, provided the original author(s) and the copyright owner(s) are credited and that the original publication in this journal is cited, in accordance with accepted academic practice. No use, distribution or reproduction is permitted which does not comply with these terms.
*Correspondence: Rosalinda Allegro, cm9zYWxpbmRhLmFsbGVncm9AZ21haWwuY29t