- 1Instituto de Ingeniería, Universidad Nacional Autónoma de México, Mexico City, Mexico
- 2Leichtweiß-Institute for Hydraulic Engineering and Water Resources, TU Braunschweig, Braunschweig, Germany
- 3Red de Ecología Funcional, Instituto de Ecología, Xalapa, Mexico
- 4Red de Ambiente y Sustentabilidad, Instituto de Ecología, Xalapa, Mexico
- 5Unidad Académica Sistemas Arrecifales, Instituto de Ciencias del Mar y Limnología, Universidad Nacional Autónoma de México, Mexico City, Mexico
- 6Utrecht Centre for Water, Oceans and Sustainability Law, School of Law, Utrecht University, Utrecht, Netherlands
- 7Department of Estuarine and Delta Systems, Royal Netherlands Institute for Sea Research, Utrecht University, Yerseke, Netherlands
- 8Department of Physical Geography, Faculty of Geosciences, Utrecht University, Utrecht, Netherlands
- 9Building With Nature Group, HZ University of Applied Sciences, Vlissingen, Netherlands
Sandy coasts represent about one-third of the global coastline and are among the most valuable and most vulnerable areas for humans and many other species. Socio-economic development and climate change impacts, together with traditional engineering for shore protection, have pervasively resulted in coastal squeeze, thereby threatening coastal life and economic activities, and the very survival of coastal ecosystems. In the past, the responses to problems such as land loss, coastal erosion and flooding were primarily reactive, through gray engineering solutions, with little interest shown in the ecosystem processes impacted by coastal armoring. In recent decades, coastal management strategies have become more diverse, embracing traditional engineering solutions alongside ecosystem-based measures. Even so, many of these new strategies still fail to meet sustainability criteria. Inspired by Per Bruun’s “The Ten Demands for Coastal Protection” from 1972, this article attempts to consider these changes and knowledge acquired since the 1970s, in order to tentatively formulate “Ten Commandments” for the sustainability of sandy coasts in face of climate change and socio-economic development. As such, the paper offers a new vision and briefly summarizes good practices for the management of sandy coasts, particularly useful for those who, at whatever level of influence, could contribute to the long-term realization of this new vision.
Introduction
Trends in Coastline Development
Low elevation coastal zones (LECZ, i.e., regions up to 10 m above sea level) cover only 2% of the surface of our planet (McGranahan et al., 2007), but are highly prized, both ecologically and economically. They include many different landforms, such as sandy beaches (with adjacent coastal dunes), barrier islands, tidal flats, estuaries, and river deltas. The ecosystems in the LECZ are extremely valuable (Costanza et al., 1997), estimated to be around $125 trillion/year and up to 77% of the value of all ecosystems worldwide, in terms of productivity, coastal protection and commerce, as well as for their strategic locations (Martínez et al., 2007; Costanza et al., 2014). As a result, our coasts have become increasingly developed and densely populated and around 10% of the world’s population, and 13% of the world’s urban population, now live in LECZ (McGranahan et al., 2007). Fifteen of the world’s 20 megacities are in coastal areas (Luijendijk et al., 2018). Coastal population growth has increased demands on the limited space, while urbanization has transformed many coastal zones into built-up areas protected by artificial defenses, resulting in massive losses of ecosystems and keystone species with consequential large-scale and cumulative effects (Lai et al., 2015; Heery et al., 2017).
Risks related to climate change exacerbate the loss of space for coastal ecosystems (Webster et al., 2005). For example, (Mentaschi et al., 2018) found that between 1984 and 2015 worldwide coastal erosion was approximately 28,000 km2. The area covered by tidal flats has fallen 16% in the last 30 years (Murray et al., 2019) and 24% of the world’s sandy beaches are eroding due to human activities (Luijendijk et al., 2018). These trends are likely to continue, and (Vousdoukas et al., 2020b) found that ∼50% of the world’s sandy beaches could be severely eroded by the end of the century, resulting in the destruction and loss of more than 130,000 km of coastline. However, in many locations the erosive trends are caused by human activities, and hence can be managed. The latter requires informed and effective adaptive measures that will sustainably manage our coasts and related sediment fluxes.
Coastal Management in a Changing World
The fundamental challenge for coastal managers facing global change is to balance the preservation of coastal ecosystems with ever-growing socio-economic pressures (Oumeraci, 2000). This is increasingly complex, because it is very difficult to predict the impacts of socio-economic development and climate change on our coasts (Wright and Nichols, 2019). Monitoring and research can reduce, though not eliminate, uncertainties; the risks remain. Moreover, coastal managers often do not have time to wait for research outcomes; they must act rapidly, continuously adapting management plans. Strategies for adapting to climate change impacts have been extensively reviewed for muddy coasts, particularly the widespread use of wetlands (mainly mangroves and salt marshes) for flood defense (Temmerman et al., 2013), and important lessons can be obtained from analyzing historical floods (Zhu et al., 2020). In contrast, flood defense strategies on sandy coasts are few, or at local scale, such as the Sand-engine in Netherlands (Stive et al., 2013). It is therefore necessary to have robust practical guidelines that can be easily adapted to meet changing scenarios and fit into sustainability frameworks for all types of coasts.
Conventional solutions to protect coastal infrastructure from erosion and flooding include seawalls, jetties, dikes (Pranzini, 2018), and beach nourishment actions (Hinkel et al., 2013). Depending on the local conditions, such solutions might be effective, but they often have unforeseen side-effects, such as transferring erosion to downdrift shorelines (Bruun, 1995; Mentaschi et al., 2018) or/and affecting coastal ecosystems (Peterson and Bishop, 2005; Peterson et al., 2006; Parkinson and Ogurcak, 2018). In recent years, rather than converting our coastlines into artificially built environments, a broad variety of Nature-Based/Green Solutions have been proposed and have been adopted by various authors and agencies to preserve and use coastal ecosystems (Temmerman et al., 2013; Nesshöver et al., 2017; Silva et al., 2017).
The aim of this work is to summarize good practices in beach use and management, and highlight key points that still need consideration. By revising some relevant examples, we illustrate these practices for those people who, at whatever level of influence, can contribute to the proper use and development of sandy coasts.
Toward a New Vision for Sandy Coasts
The challenges described above have been discussed for decades. For example, (Bruun et al., 1957) analyzed the causes of extensive coastal erosion in Florida and later theorized that sea level rise was a cause of coastal erosion worldwide (Bruun, 1962). Subsequently, (Bruun, 1972) analyzed the effects of the most common coastal structures on the physical environment, highlighting the lessons learned in his “Ten Demands for Coastal Protection” (Table 1). New information generated since the 70s demonstrates that some of these precepts must be updated. For example, the limitations of certain inappropriately used design tools of that time were soon recognized (Bruun, 1988), while the effects of extreme events and human activities are now better understood (Oppenheimer et al., 2019). Effects of climate variability, such as the El Niño oscillation (Odériz et al., 2020), have only recently been better understood, and were not considered by Bruun (1972).
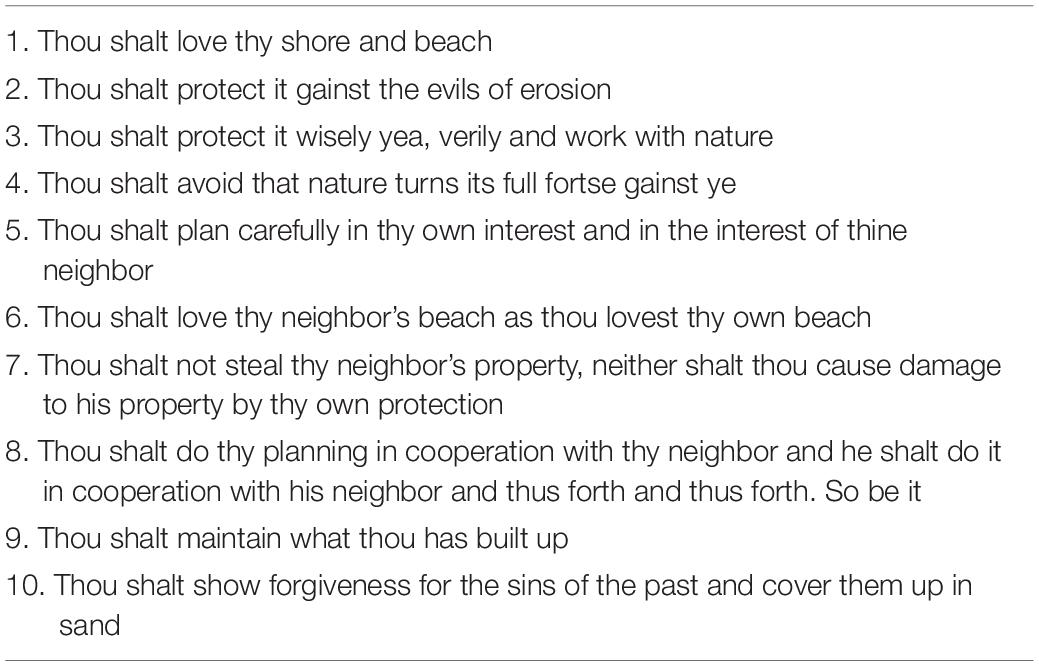
Table 1. The ten demands for coastal protection (Bruun, 1972).
Bruun’s Demands reflected the increasing environmental awareness of 1972. At that time, conservation efforts concentrated on decreasing degradation, restoring habitats and protecting representative species by excluding humans from specific areas. The crucial role of connectivity among ecosystems (Gillis et al., 2014; Magris et al., 2016), different actors and policy domains were not contemplated. Nevertheless, in spite of all the shortcomings of Bruun’s demands, if they had been more widely included in decision-making processes since 1972, the condition of the coastal environment today would be considerably different.
Indeed, the cumulative damage to the coastal environment by the inappropriate infrastructure built in the last five decades is incalculable. This might be due to the fact that coastal protection design and construction were merely performed from an anthropic perspective (i.e., solely driven by the safety of human lives and properties) and generally considered on a case by case basis, i.e., ignoring integrative approaches and with insufficient consideration of cumulative effects (Cooper et al., 2020). Moreover, the performance and impacts of these schemes were not properly monitored, and were rarely well-documented, by current standards. For instance, many of the projects that have been successful are reported very poorly; as in many areas of knowledge, when a solution works, it is taken for granted. Similarly, a lack of monitoring actions has prevented us from determining exactly the negative consequences of wrongly placed infrastructure. Furthermore, decisions on project implementation are often governed by investment costs, by a rapid economic or political return, or by having some visible results quickly.
Certain processes, such as beach erosion and periodic flooding, are often seen as a risk for humans. Nevertheless, these are natural processes and, in fact, coastal ecosystems depend on them, as pointed out by e.g., Cooper et al. (2020). In this case, dune erosion provides sediment for beaches and the nearshore zone, thus allowing the coastline to adjust to changing conditions and preventing coastal squeeze. In a similar vein, flooding provides wetlands with fresh sediment and nutrients. Strategies that only focus on preventing erosion and flooding, using hard infrastructure, could in the long-term damage coastal ecosystems by disrupting the dynamics, which are natural in many soft-sediment habitats. The adverse effects of protecting the coast through gray infrastructure include the alteration of habitats, and interruption the natural sedimentary dynamics (Airolbdi and Beck, 2007; Dugan et al., 2011; Dugan et al., 2018), preventing energy attenuation, inducing coastal squeeze. But letting human-induced erosion/accretion and flooding processes evolve freely, without proper management, system knowledge and long-term monitoring, may have even more devastating consequences on ecosystems and their services. Thus, achieving long-term sustainable solutions is inherently complex and requires intelligent use of state-of-the-art interdisciplinary knowledge, as reflected in the proposed ten commandments.
Ten Commandments for Sustainable Coasts
Since Bruun’s Demands were published in 1972, blinkered coastal management decisions have often been unsuccessful; environmental degradation continues, and new threats have arisen. New strategies for coastal protection have shifted toward Ecosystem-based Management (Arkema et al., 2006; Levin and Lubchenco, 2008; Granek et al., 2010; Harris et al., 2015; Fernandino et al., 2018; Silva et al., 2019; O’Higgins et al., 2020), which recognizes the intra- and inter-connectivity among ecosystems, (e.g., Gillis et al., 2014, 2017), cross-scale processes, (e.g., Lithgow et al., 2019), trans-discipline cooperation, (e.g., Alexander et al., 2019), science-based decisions, (e.g., Piet et al., 2019) and community participation, (e.g., Stori et al., 2019). Bruun’s Demands were visionary, in emphasizing both the dynamics of the shores and their physical inter-connectivity. Moreover, the concept of working with nature was set in Bruun’s Demands, although the main objective was still to defend the coast against the forces of nature, rather than to make use of them.
Since 1972, the knowledge available to decision makers has greatly evolved, it must now include changes in socio-economic and climatic systems. This prompted us to revisit the “Ten Demands,” complementing and updating them to become “Ten Commandments for Sustainable, Safe and W/Healthier Sandy Coasts Facing Global Change” (Figure 1). The most important changes require (i) working in transdisciplinary teams, (ii) accepting uncertainty and (iii) developing legislation and governance arrangements that facilitate adaptive management and increase social-ecological resilience as well as social justice.
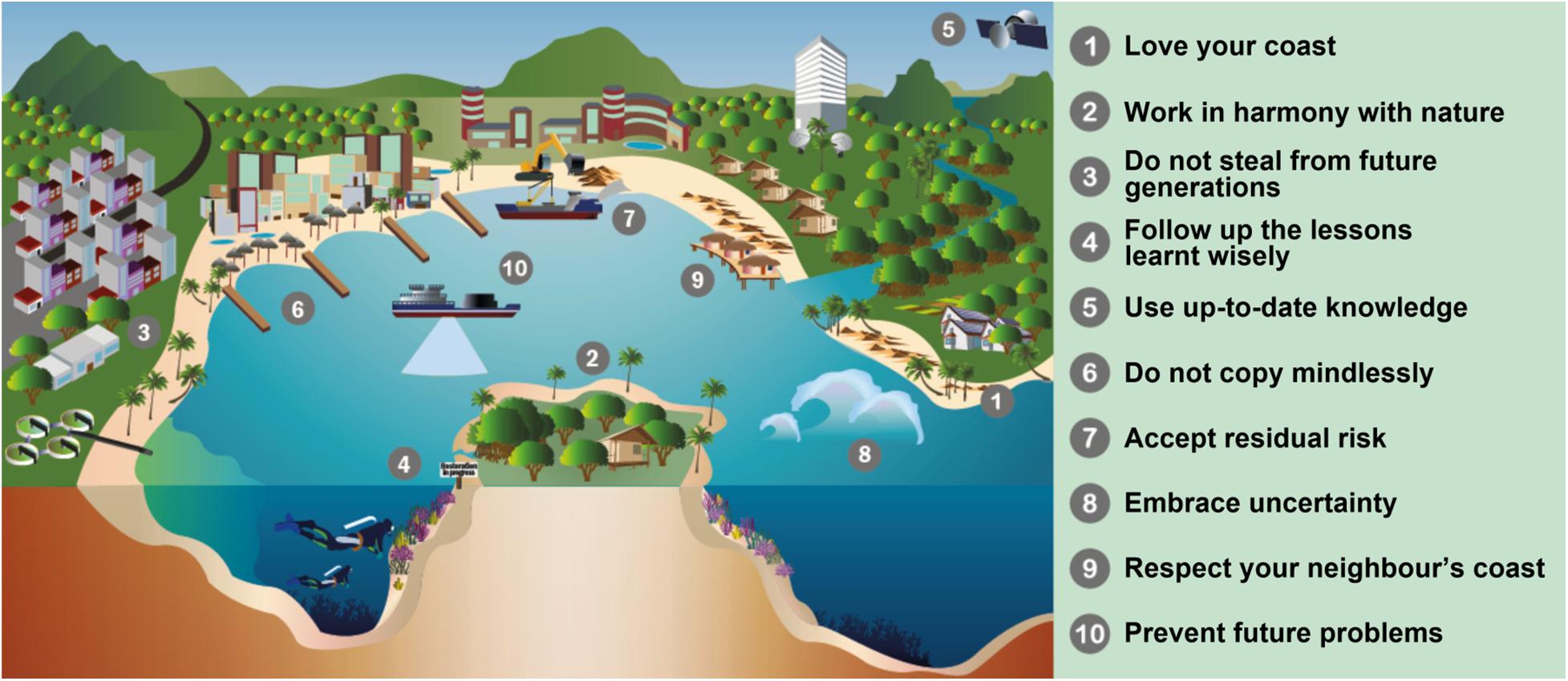
Figure 1. Ten commandments for sustainable, safe, and W/Healthier sandy coasts facing global change.
Love Your Coast – You Shall Make Your Coast a Sustainable Place to Live
Working in transdisciplinary schemes is essential to achieve sustainable coasts (Arkema et al., 2017; Schoonees et al., 2019). The results should integrate ecosystem functioning and connectivity with socio-economic needs to face current and future demands on coastal and marine resources (Borgström et al., 2015; Silva et al., 2019).
Whereas this is widely recognized, and lip-service is paid to it, the main challenge for the coming 50 years is the genuine implementation of sustainable solutions that produce healthy and wealthy coastal environments.
Work in Harmony With Nature – You Shall Further Your Understanding of the Relationships Between Natural Resources and Human Welfare
Ecosystems provide services with provisioning, regulating, supporting and cultural benefits (Costanza et al., 2017). Mangrove forests, salt marshes, seagrass meadows, coral reefs, and coastal dunes attenuate the impact of waves, prevent erosion and provide coastal protection (Koch et al., 2009; Barbier, 2017). This has major management implications. For example, preserving seagrass and algae foreshores can in some cases be a viable alternative to repeated (costly) sand nourishment employed to maintain tropical beaches (James et al., 2019). To maximize benefits, in addition to preserving, we also need to find ways of restoring these ecosystems and their services where they are needed (Bouma et al., 2014). Fortunately, insights in successful restoration are rapidly progressing (Schotanus et al., 2020; Temmink et al., 2020).
Many green engineering projects (Silva et al., 2017) mimic natural functions that provide an ecosystem service. Unlike traditional gray infrastructure, which considers only socio-economic and political factors, green infrastructure is multiscale and based on the concepts of connectivity, multi-functionality and integration (Davies and Lafortezza, 2017). Also, while gray actions, such as groins, present a finished “product,” a green action continues evolving, adapting to changing conditions, perhaps unforeseen. The feasibility of implementing a green project depends on the site characteristics (Ruckelshaus et al., 2016), but there are general trends in the requirements for each type of green infrastructure (Figure 2).
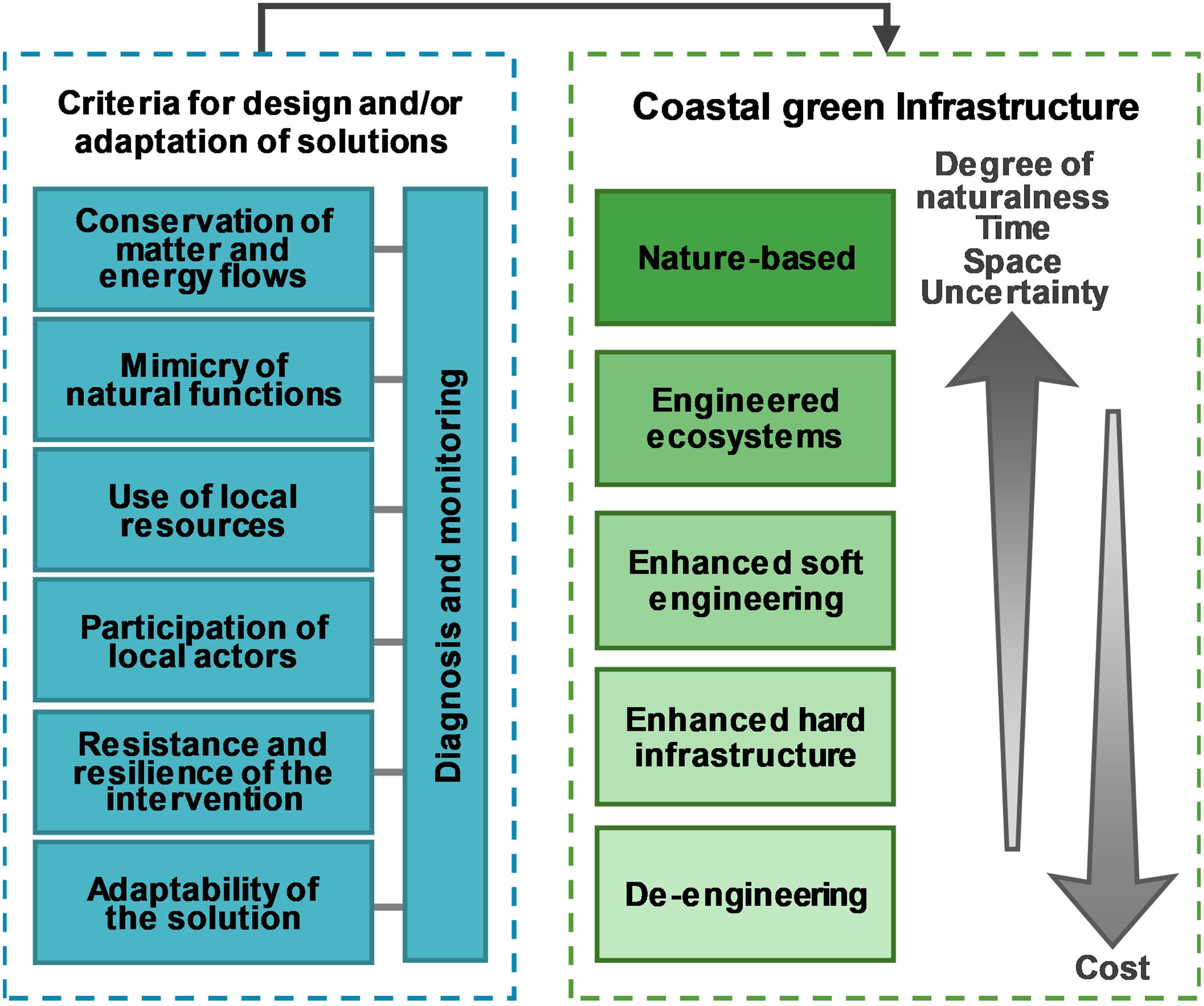
Figure 2. Interaction of the different elements. De-engineering (relocating landward, or removing, infrastructure) is the only alternative if space is insufficient to allow for ecosystem processes, the available time for ecosystem responses is insufficient (too short) and the degree of uncertainty of the solution must be low.
An important consideration is that while an option with a greater degree of naturalness requires more time and space, it is usually cheaper. In critical situations, where time to act and space available are limited, a less natural alternative may provide a bridging solution that will need to be transformed over time. Currently, the success of projects with a greater degree of naturalness tends to have higher levels of uncertainty. Therefore, more space or more flexible and adaptive legislation and governance arrangements, focusing on sustainability goals, may be required (Garmestani et al., 2019b). Reducing, or dealing with this uncertainty is the aim of trans-disciplinary research.
Do Not Steal From Future Generations – You Shall Preserve Coastal Ecosystems and Ecosystem Services by Maintaining Ecosystem Functioning
Coastal ecosystems provide numerous services to many millions of people, and failure to maintain them will deprive future generations of these services. As we cannot know the needs of future generations, we cannot assume that the engineering solutions we implement that cause the degradation of natural ecosystems, will be beneficial to them.
Preserving ecosystem services requires a holistic approach (Silva et al., 2019). Ensuring a balance in the fluxes of energy, matter and organisms is needed to safeguard the health of coastal ecosystems. These fluxes are often interconnected (Gillis et al., 2014, 2017; Graham et al., 2018). Trophic cascades across ecosystems may bring unexpected outcomes. For example (Graham et al., 2018) demonstrated how seabirds enhanced coral productivity when invasive rats were absent from the Chagos Archipelago. Certainly, we are only beginning to understand the implications of altering the interconnectivity across ecosystems, which requires a transdisciplinary focus. Silva et al., 2020 showed how changes in the health of any of coastal ecosystems (mangroves, dunes, beaches, seagrass beds, and corals) generate undesirable consequences in the adjacent ecosystems by inducing coastal squeeze. These undesirable changes can have local, regional, or global origin. The authors also underline that, unlike anthropic change, extreme events and periods of calm are both important for the renewal and existence of these ecosystems.
We need to account for evolving socioeconomic demands and the impacts of human interventions, under the possibility of sudden dramatic changes induced by extreme events, as well as for slow changes over a long period (Oumeraci, 2000).
Follow Up the Lessons Learnt Wisely – You Shall Not Simply Repeat Defense Schemes From the Past but Build on Recent Experience
When designing coastal defense strategies, it is crucial to start afresh, but also to take into account previous knowledge. This implies the possibility of adapting earlier measures to the unique characteristics of a new case. For example, the Hondsbossche sea dike on the northwest coast of the Netherlands successfully protected reclaimed land from 1880. However, new insights led not to its reinforcement, but to the building of a 5.5 km long dune barrier in front of the old dike (2014–2015). This provided flood protection as well as new recreational space for the community, and connectivity between two ecologically valuable and biodiverse back-dune ecosystems (Wittebrood et al., 2018).
Scientists should search for adaptable, timely solutions that improve on the tried-and-tested, giving better efficiency. This may involve coupled phases, where a short-term solution is chosen as an immediate response to decrease risk, followed by medium- and long-term solutions that enhance overall coastal resilience in terms of safety, socio-economic and ecological needs. To do so, fundamental knowledge of the physical, ecological and socio-economic governance and legal systems is required (Garmestani et al., 2019a).
Use Up-to-Date Knowledge – You Shall Protect Your Coast Against Degradation Using the Latest Advances in Science and Technology
An ideal coastal diagnosis is based on data of sufficient quality and quantity. For this, skills, time, and money are required, meaning that such data are often unavailable and so decisions are made with the information and knowledge available at that time (Silva et al., 2019). However, it is vital to invest in extending the frontiers of our knowledge.
(Jackson et al., 2017) provide an example of how the identification of problems and dealing with them, has changed over time at the mouth of the river Tweed, in Australia. In the 1960s, coastal researchers only collected data for the target beaches and nearby seabed. A long-term coastal management plan gradually evolved as decision-makers gained new understanding of the system. Firstly, they set up gray measures; from the early 1970s until 2010, erosion and flooding problems were managed by building more structures and borrowing offshore sand, and sediments were transferred to the neighboring beaches. Meanwhile, monitoring was showing that the problems of the beaches for surfers and some subaquatic ecosystems, were, in fact, induced by previously built coastal infrastructure. Subsequently, they implemented a ground-breaking sand bypass solution, based on ecosystem management, which has been very successful, as they have been able to mimic the natural longshore transport of sediments. This evolving accumulation of knowledge was the foundation for a new, science-based, solution to an old problem.
As new methodologies and tools emerge, they can help us move to a more sustainable use of our coastal areas. Without investing in science, this solution would not have been conceived and the required professional specialists would not have emerged. As a scientific community, we must highlight this kind of successful examples to coastal managers.
Do Not Copy Mindlessly – You Shall Assess Your Diagnosis and Choose the Best Management and Protection Practices Based on Local Information
A prompt, accurate diagnosis for a particular locality and situation is vital for coastal problems. One of the peculiarities of coastal zones is their high dynamics; their variability in space and time, making local data collection essential. Using non-local data can lead to diagnostic errors and cause irreversible loss of ecosystem resilience and services, even triggering socio-environmental damage. As shown in Figure 2, the diagnosis must be carried out in a way that provides sufficient information to successfully meet the design criteria.
An example of poorly selected coastal protection schemes is found on the Caribbean coast of Colombia. By 2016, almost 1,500 hard structures had been installed along this coastline (Rangel-Buitrago et al., 2018). With no accurate diagnosis of the erosion problems, the authorities opted to build groins, based on successful practice elsewhere. However, almost 90% of the groins did not provide erosion protection, and simply aggravated the situation, often inducing more complex problems as the coastline was hardened. With rigid coastal structures, ecosystems do not have space to evolve. A wider initial search for other solutions, such as restoring or mimicking sand fluxes, may have led to a better alternative being implemented.
As scientists, we need to publicize the many examples of this type of coastal disaster, so that decision makers are not pushed into erroneous strategies by ignorance.
Accept Residual Risk – You Shall Not Mislead Yourself and Others; A Coastal Defense Strategy With “Zero Risk” Does Not Exist, and Never Will
Any coastal defense scheme must be sufficiently resilient to not place human lives, economic or cultural activities at risk. It must guarantee resilience over both long periods of calm conditions, and when faced with rare, extreme events. Oumeraci et al., 2015 showed that uncertainties associated with lack of information bring higher risks and therefore add to the cost of coastal works. Whenever explicit knowledge and quantification of uncertainties from each risk source are unavailable, overly expensive solutions are needed to safeguard against residual risks. In the face of climate change, this problem will increase.
As scientists, we should offer coastal management strategies that are adaptive, based on the availability of money, space and time. When the interrelated dynamics of a site are better known, it is more likely that an adequate diagnosis will be made; less uncertainty will then follow, enabling more reliable, less expensive solutions to problems. Social approval must be won by explaining the risks and uncertainties through various types of community participation.
Embrace Uncertainty – You Shall Learn From Unpredictable Events in the Past so as to Be Prepared for the Unpredictable
The effects induced by previously occurring unpredictable phenomena, such as hurricanes, tsunamis and landslides, should be documented as well and as accurately as possible (Silva et al., 2014, 2016). For example, the 2011 tsunami was generated by the greatest earthquake ever recorded in Japan. Until then, the chain of events which followed were unpredictable, causing infrastructure failures, socioenvironmental and economic problems (Suppasri et al., 2013). The emergency solutions developed immediately afterward can be built upon for future events. Conversely, the reanalysis of the historic dike breaches of 1717 and 1953 in NW Europe inspired novel and improved flood protection schemes based on natural coastal defense functions (Zhu et al., 2020).
Human interference in the natural flows of the planet can also create unforeseen events. For example, the massive arrival of pelagic sargassum onto the coasts of Africa and the Greater Caribbean from 2011 onward, has brought unforeseen, far-reaching negative consequences on the environment (e.g., death of seagrass, mangroves and corals, loss of beach sediments), as well as socio-economic problems (Johnson et al., 2013; van Tussenbroek et al., 2017; Wang et al., 2019; Chávez et al., 2020). Whenever an unpredictable event occurs, it should be seen as an opportunity for learning; to prepare for what may come in the future.
Respect Your Neighbor’s Coast – You Shall Consider Interactions Beyond the Local Scale, so as Not to Harm Other Areas by Your Actions
There are numerous cases of coastal structures which induce erosion problems on adjacent beaches (Silva et al., 2014). In essence all protective structures transfer the erosion along the shoreline. The extent of the downdrift erosion depends on the amount of sediment retained and/or diverted offshore by the structure, which in turn depends on the type, size and protrusion of that structure, and also on the length of the shoreline it stabilizes. Because of this, some form of regulation must be in place to holistically protect all coastal areas and the people who live there.
This connectivity is seen when we look back at the protection works around the mouth of the river Tweed, Australia. The 19th century seawalls, built to aid navigation, altered sediment dynamics and erosion processes began to be observed on downdrift beaches. Extreme storms in 1967 produced serious damage to infrastructure on those beaches, devastating the tourist economy. The sand bypass scheme subsequently implemented keeps the river mouth clear of sand and allows longshore sediment transport to continue to feed the neighboring beaches (Jackson et al., 2017).
Coastal plans need to acknowledge the wider implications of local actions; how local socio-economic conditions could be affected, whether legislation could avoid shifting the problems elsewhere, and whether appropriate governance conditions are in place. Some academics argue that projects are often designed mainly to increase property values and that they displace already marginalized inhabitants, see Liao (2019). It is generally true that the increasing number and severity of natural events in coastal regions disproportionately affect the most impoverished people (Chaplin-Kramer et al., 2019). In this context, green infrastructure projects can promote social justice and equity by protecting communities located in high-risk areas (Ruckelshaus et al., 2020) and recovering the hydrosedimentary fluxes that may have been interrupted to protect a specific “valuable” area, and that are causing erosion and subsidence to downdrift communities.
Given the still-open fundamental questions concerning the Human-Nature relationship, the controversial notions of justice, in general, and environmental justice, in particular, remain crucial for any development aiming for safe, wealthy and healthy coasts. As environmental justice is not limited to any one disciplinary tradition, a discursive forum is needed for broader, more targeted debates, involving contributors from natural sciences, social sciences, humanities, public administration, industry, and other stakeholders. Such debate might include reformulating the perceptions and principles of justice for coastal communities, and elaborating guidance for environmentally just, safe, wealthy and healthy coasts facing global change. Some of the open issues awaiting clarification in such debate are:
(i) Differences in the perception of the value of biophysical ecosystems, and particularly of the less tangible cultural ecosystem services (Lau et al., 2019; Smart et al., 2021);
(ii) The dynamic relationship between “safe” and “just” based on the three dimensions of justice: Distributional, procedural, and recognitional (Lau et al., 2021);
(iii) The boundaries of socio-economic and ecological sustainability;
(iv) Justice criteria in the local context and justice metrics for comparative studies at a community level.
Frameworks for such debates might be provided by the various environmental governance theories reviewed and analyzed by Partelow et al. (2020) or the visual framework for “safe and just spaces” provided by Raworth (2012).
Prevent Future Problems – You Shall Monitor the Performance of Your Defense Schemes, Because Coastal Protection Is a Perpetual Process
Infrastructure designed for coastal protection should only be built when local diagnosis demonstrates that it is necessary. Before and after the installation of any coastal work, monitoring is essential, using the best techniques available. These should include emerging technologies, such as autonomous operated vehicles, satellite sensing, drones, inexpensive sensors and mobile-phone technologies.
Erosion on the long sandy beach at Newbiggin-by-the-Sea, England, was reduced by a substantial seawall, built in 1877, and subsequently enlarged and renovated. In 2002, monitoring showed that the toe of the seawall had become exposed and it was being undermined; the beach was again at risk. In 2007, a costly sand nourishment project took place, complemented by the construction of an offshore breakwater in the center of the bay. In 2008, a build-up of sand was recorded in the lee of the breakwater, as a tombolo. Monitoring showed that windblown sand was causing problems; the lifeboat station and fishing port had difficulty launching vessels, recreational infrastructure was being buried and a rocky shore platform, previously used for feeding by migrating birds, was now smothered by sand. In 2011, remedial action was implemented; sand from the areas of accretion was used to nourish areas of erosion (Cooper et al., 2019).
To generate flexible plans, ongoing monitoring and updating are crucial, biophysical, economic and social components. An appropriate response to accurate monitoring determines the success, or failure, of any coastal project. It would therefore be prudent to compel coastal managers to continue monitoring after the installation of any project, since changes in conditions are inherent to all coastal areas.
With reliable information and widespread continuous monitoring, local actions may contribute to mitigating processes associated with climate change. In light of new data, adapted infrastructures must be congruent with natural cycles.
Concluding Comments
Although the unique biodiversity and ecological functions of beaches are increasingly acknowledged, recognition of their relevance still lags far behind that of other coastal habitats, such as reefs and wetlands. The connectivity between sandy shores and other marine and terrestrial ecosystems is largely missing from coastal management actions (James et al., 2019 #67). This is particularly important with regard to terrigenous and biogenic sand supply, but also applies to organic matter inputs and other fluxes. In this sense, coastal management should consider structures in rivers, streams and coastal areas, which affect how much sediment reaches the coast.
In the face of the unprecedented challenges associated with global climate change, the need to make sandy coasts more sustainable, safer and wealthier is growing. Reducing the detrimental effects of the unsustainable use of coastal zones is vital to avoid disrupting ecosystem connectivity and prevent reaching points of no return in their functioning. The ever growing, cumulative impacts on the natural environment of anthropic interventions, such as “coastal armoring,” are the main drivers of these imbalances.
More adaptive solutions are required to counter the huge uncertainties in predicting climate change consequences and the evolution of the socio-economic conditions in coastal zones. The need of adaptive solutions coincides with recent diverse advances in (i) green based approaches, which focus on continuous assessment and adaptation rather than on fixed solutions and end–state blueprints; (ii) ongoing systematic monitoring, databases, analysis and updating strategies and tools, and; (iii) integrated socioecological analyses and modeling.
Despite the widespread, increasing perception of the relevance of nature-based/green solutions resulting from scientific publications, government programs and the media, the use of conventional protective structures (e.g., seadikes, seawalls, revetments, groins, and detached breakwaters) around the world is, in fact, expected to increase. While these “hard” solutions are seen as being coastal armoring, with adverse effects on sediment transport and coastal morphology as well as on cultural and other types of ecosystem services, they are undeniably the most efficient against severe damage. They are also the strategy offering the “most predictable levels of safety against coastal extremes” (Vousdoukas et al., 2020a). This consideration is a particularly determinant factor in urban areas where softer alternative solutions for managing coastal erosion and floods are not feasible. In such cases, the negative ecological impacts may be reduced by implementing hybrid approaches that combine innovative hard structures (existing and/or new) with biotic components to enhance them ecologically.
There are still various challenges that need to be met for the practical, widespread implementation of sustainable infrastructure on the coast: improvements in biophysical knowledge, to achieve robust schemes, concepts, tools and techniques; consistent frameworks and guidance for sustainable and just coastal environments; detailed guidelines and standards for the implementation of solutions and; effective institutional bases for legislation and regulation. The biggest challenge, however, is to ensure that all those involved in decision making processes affecting the coastal zone are aware of the importance of the ecosystems there for human beings.
The ten Commandments proposed are considered a modest contribution in this direction. Table 2 presents the updated version of Bruun’s “Ten Demands for Coastal Protection.” With this agenda it is hoped that researchers will be inspired to produce more and better information that will help promote a more responsible use of coastal environments in the face of global changes in the next 50 years, with a growing number of people settling on the coasts, and an ever-changing climate.
Data Availability Statement
The original contributions presented in the study are included in the article, further inquiries can be directed to the corresponding author.
Author Contributions
RS suggested the idea, coordinated the contributions, and wrote the final version of the manuscript. HO provided the initial idea for the manuscript. VC and DL drafted the examples and figure content and corrected the manuscript. All authors were involved in the discussion of the manuscript.
Funding
The authors are grateful to the Centro Mexicano de Innovación en Energía del Océano (CEMIE-Océano) funded by the CONACYT-SENER Sustentabilidad Energética project: FSE-2014-06-249795 and the German Academic Exchange Service (DAAD), Excellence Center for Development Cooperation – Sustainable Water Management (EXCEED/SWINDON) for their financial and technical support.
Conflict of Interest
The authors declare that the research was conducted in the absence of any commercial or financial relationships that could be construed as a potential conflict of interest.
References
Airoldi, L., and Beck, M. (2007). Loss, status and trends for coastal marine habitats of Europe. Oceanogr. Mar. Biol. 45, 345–405. doi: 10.1201/9781420050943.ch7
Alexander, K. A., Hobday, A. J., Cvitanovic, C., Ogier, E., Nash, K. L., Cottrell, R. S., et al. (2019). Progress in integrating natural and social science in marine ecosystem-based management research. Mar. Freshw. Res. 70, 71–83. doi: 10.1071/mf17248
Arkema, K. K., Abramson, S. C., and Dewsbury, B. M. (2006). Marine ecosystem-based management: from characterization to implementation. Front. Ecol. Environ. 4, 525–532. doi: 10.1890/1540-929520064[525:MEMFCT]2.0.CO;2
Arkema, K. K., Griffin, R., Maldonado, S., Silver, J., Suckale, J., and Guerry, A. D. (2017). Linking social, ecological, and physical science to advance natural and nature-based protection for coastal communities. Ann. N. Y. Acad. Sci. 1399, 5–26. doi: 10.1111/nyas.13322
Borgström, S., Bodin, Ö, Sandström, A., and Crona, B. (2015). Developing an analytical framework for assessing progress toward ecosystem-based management. Ambio 44 Suppl 3, 357–369. doi: 10.1007/s13280-015-0655-7
Bouma, T. J., Van Belzen, J., Balke, T., Zhu, Z., Airoldi, L., Blight, A. J., et al. (2014). Identifying knowledge gaps hampering application of intertidal habitats in coastal protection: opportunities & steps to take. Coast. Eng. 87, 147–157. doi: 10.1016/j.coastaleng.2013.11.014
Bruun, P. (1962). Sea-level rise as a cause of shore erosion. J. Waterways and Harbors Div. 88, 117–132.
Bruun, P. (1972). “The history and philosophy of coastal protection,” in Proceedings of the 13th International Conference on Coastal Engineering Proceedings (Reston, VA: ASCE), 33–74.
Bruun, P. (1988). The Bruun rule of erosion by sea-level rise: a discussion on large-scale two- and three-dimensional usages. J. Coast. Res. 4, 627–648.
Bruun, P., Gerritsen, F., and Morgan, W. H. (1957). Florida coastal problems. Coast. Eng. 1, 463–509.
Chaplin-Kramer, R., Sharp, R. P., Weil, C., Bennett, E. M., Pascual, U., Arkema, K. K., et al. (2019). Global modeling of nature’s contributions to people. Science 366:255–258.
Chávez, V., Uribe-Martínez, A., Cuevas, E., Rodríguez-Martínez, R. E., Van Tussenbroek, B. I., Francisco, V., et al. (2020). Massive influx of pelagic sargassum spp. on the coasts of the Mexican Caribbean 2014–2020: challenges and opportunities. Water 12, 1–24.
Cooper, J. A. G., O’connor, M. C., and Mcivor, S. (2020). Coastal defences versus coastal ecosystems: a regional appraisal. Mar. Policy 111:102332. doi: 10.1016/j.marpol.2016.02.021
Cooper, N., Siddle, R., and Rowe, S. (2019). “The value of monitoring data to sustainable coastal management in Northeast England,” in Proceedings of the 2019 Conference on Coastal Management, 185–199.
Costanza, R., D’arge, R., De Groot, R., Farber, S., Grasso, M., Hannon, B., et al. (1997). The value of the world’s ecosystem services and natural capital. Nature 387, 253–260.
Costanza, R., De Groot, R., Braat, L., Kubiszewski, I., Fioramonti, L., Sutton, P., et al. (2017). Twenty years of ecosystem services: how far have we come and how far do we still need to go? Ecosyst. Serv. 28, 1–16. doi: 10.1016/j.ecoser.2017.09.008
Costanza, R., De Groot, R., Sutton, P., Van Der Ploeg, S., Anderson, S. J., Kubiszewski, I., et al. (2014). Changes in the global value of ecosystem services. Glob. Environ. Change 26, 152–158.
Davies, C., and Lafortezza, R. (2017). Urban green infrastructure in Europe: is greenspace planning and policy compliant? Land Use Policy 69, 93–101. doi: 10.1016/j.landusepol.2017.08.018
Dugan, J., Airoldi, L., Chapman, M., Walker, S., Schlacher, T., Wolanski, E., et al. (2011). “8.02-Estuarine and coastal structures: environmental effects, a focus on shore and nearshore structures,” in Proceedings of the Treatise on Estuarine and Coastal Science, eds E. Wolanski and D. McLusky (London: Academic Press), 8, 17–41.
Dugan, J. E., Emery, K. A., Alber, M., Alexander, C. R., Byers, J. E., Gehman, A. M., et al. (2018). Generalizing ecological effects of shoreline armoring across soft sediment environments. Estuaries Coast. 41, 180–196. doi: 10.1007/s12237-017-0254-x
Fernandino, G., Elliff, C. I., and Silva, I. R. (2018). Ecosystem-based management of coastal zones in face of climate change impacts: challenges and inequalities. J. Environ. Manage. 215, 32–39. doi: 10.1016/j.jenvman.2018.03.034
Garmestani, A., Craig, R. K., Gilissen, H. K., Mcdonald, J., Soininen, N., Van Doorn-Hoekveld, W. J., et al. (2019a). The role of social-ecological resilience in coastal zone management: a comparative law approach to three Coastal Nations. Front. Ecol. Evol. 7:410. doi: 10.3389/fevo.2019.00410
Garmestani, A., Ruhl, J. B., Chaffin, B. C., Craig, R. K., Van Rijswick, H. F. M. W., Angeler, D. G., et al. (2019b). Untapped capacity for resilience in environmental law. Proc. Natl. Acad. Sci. U.S.A. 116:19899. doi: 10.1073/pnas.1906247116
Gillis, L. G., Bouma, T. J., Jones, C. G., Van Katwijk, M. M., Nagelkerken, I., Jeuken, C. J. L., et al. (2014). Potential for landscape-scale positive interactions among tropical marine ecosystems. Mar. Ecol. Prog. Ser. 503, 289–303. doi: 10.3354/meps10716
Gillis, L. G., Jones, C. G., Ziegler, A. D., Van Der Wal, D., Breckwoldt, A., and Bouma, T. J. (2017). Opportunities for protecting and restoring tropical coastal ecosystems by utilizing a physical connectivity approach. Front. Mar. Sci. 4:374. doi: 10.3389/fmars.2017.00374
Graham, N. A. J., Wilson, S. K., Carr, P., Hoey, A. S., Jennings, S., and Macneil, M. A. (2018). Seabirds enhance coral reef productivity and functioning in the absence of invasive rats. Nature 559, 250–253. doi: 10.1038/s41586-018-0202-3
Granek, E. F., Polasky, S., Kappel, C. V., Reed, D. J., Stoms, D. M., Koch, E. W., et al. (2010). Ecosystem services as a common language for coastal ecosystem-based management. Conserv. Biol. 24, 207–216.
Harris, L., Nel, R., Holness, S., and Schoeman, D. (2015). Quantifying cumulative threats to sandy beach ecosystems: a tool to guide ecosystem-based management beyond coastal reserves. Ocean Coast. Manag. 110, 12–24. doi: 10.1016/j.ocecoaman.2015.03.003
Heery, E. C., Bishop, M. J., Critchley, L. P., Bugnot, A. B., Airoldi, L., Mayer-Pinto, M., et al. (2017). Identifying the consequences of ocean sprawl for sedimentary habitats. J. Exp. Mar. Biol. Ecol. 492, 31–48. doi: 10.1016/j.jembe.2017.01.020
Hinkel, J., Nicholls, R. J., Tol, R. S. J., Wang, Z. B., Hamilton, J. M., Boot, G., et al. (2013). A global analysis of erosion of sandy beaches and sea-level rise: an application of DIVA. Glob. Planet. Change 111, 150–158. doi: 10.1016/j.gloplacha.2013.09.002
Jackson, L. A., Tomlinson, R. J. A. C., and Nature, P. W. W. (2017). “50 years of seawall and nourishment strategy evolution on the gold coast,” in Australasian Coasts & Ports 2017: Working with Nature, eds National Committee for Coastal and Ocean Engineering, PIANC Australia, Institution of Professional Engineers New Zealand, Engineers Australia (Gold Coast: Engineers Australia).
James, R. K., Silva, R., Van Tussenbroek, B. I., Escudero-Castillo, M., Mariño-Tapia, I., Dijkstra, H. A., et al. (2019). Maintaining tropical beaches with Seagrass and Algae: a promising alternative to engineering solutions. BioScience 69, 136–142. doi: 10.1093/biosci/biy154
Johnson, D. R., Ko, D. S., Franks, J. S., Moreno, P., and Sanchez-Rubio, G. (2013). “The sargassum invasion of the eastern caribbean and dynamics of the equatorial north atlantic,” in Proceedings of the 65th Gulf and Caribbean Fisheries Institute (Santa Marta), 102–103.
Koch, E. W., Barbier, E. B., Silliman, B. R., Reed, D. J., Perillo, G. M., Hacker, S. D., et al. (2009). Non-linearity in ecosystem services: temporal and spatial variability in coastal protection. Front. Ecol. Environ. 7, 29–37. doi: 10.1890/080126
Lai, S., Loke, L. H. L., Hilton, M. J., Bouma, T. J., and Todd, P. A. (2015). The effects of urbanisation on coastal habitats and the potential for ecological engineering: a Singapore case study. Ocean Coast. Manag. 103, 78–85. doi: 10.1016/j.ocecoaman.2014.11.006
Lau, J. D., Gurney, G. G., and Cinner, J. (2021). Environmental justice in coastal systems: perspectives from communities confronting change. Glob. Environ. Change 66:102208. doi: 10.1016/j.gloenvcha.2020.102208
Lau, J. D., Hicks, C. C., Gurney, G. G., and Cinner, J. E. (2019). What matters to whom and why? Understanding the importance of coastal ecosystem services in developing coastal communities. Ecosyst. Serv. 35, 219–230. doi: 10.1016/j.ecoser.2018.12.012
Levin, S. A., and Lubchenco, J. (2008). Resilience, robustness, and marine ecosystem-based management. BioScience 58, 27–32. doi: 10.1641/b580107
Liao, K.-H. (2019). The socio-ecological practice of building blue-green infrastructure in high-density cities: what does the ABC waters program in Singapore tell us? Socio Eco. Prac. Res. 1, 67–81. doi: 10.1007/s42532-019-00009-3
Lithgow, D., De La Lanza, G., and Silva, R. (2019). Ecosystem-based management strategies to improve aquaculture in developing countries: case study of Marismas Nacionales. Ecol. Eng. 130, 296–305. doi: 10.1016/j.ecoleng.2017.06.039
Luijendijk, A., Hagenaars, G., Ranasinghe, R., Baart, F., Donchyts, G., and Aarninkhof, S. (2018). The state of the world’s beaches. Sci. Rep. 8:6641.
Magris, R. A., Treml, E. A., Pressey, R. L., and Weeks, R. (2016). Integrating multiple species connectivity and habitat quality into conservation planning for coral reefs. Ecography 39, 649–664. doi: 10.1111/ecog.01507
Martínez, M. L., Intralawan, A., Vázquez, G., Pérez-Maqueo, O., Sutton, P., and Landgrave, R. (2007). The coasts of our world: ecological, economic and social importance. Ecol. Econ. 63, 254–272. doi: 10.1016/j.ecolecon.2006.10.022
McGranahan, G., Balk, D., and Anderson, B. (2007). The rising tide: assessing the risks of climate change and human settlements in low elevation coastal zones. Environ. Urban. 19, 17–37. doi: 10.1177/0956247807076960
Mentaschi, L., Vousdoukas, M. I., Pekel, J.-F., Voukouvalas, E., and Feyen, L. (2018). Global long-term observations of coastal erosion and accretion. Sci. Rep. 8:12876.
Murray, N. J., Phinn, S. R., Dewitt, M., Ferrari, R., Johnston, R., Lyons, M. B., et al. (2019). The global distribution and trajectory of tidal flats. Nature 565, 222–225. doi: 10.1038/s41586-018-0805-8
Nesshöver, C., Assmuth, T., Irvine, K. N., Rusch, G. M., Waylen, K. A., Delbaere, B., et al. (2017). The science, policy and practice of nature-based solutions: an interdisciplinary perspective. Sci. Total Environ. 579, 1215–1227. doi: 10.1016/j.scitotenv.2016.11.106
Odériz, I., Silva, R., Mortlock, T. R., and Mori, N. (2020). El Niño-southern oscillation impacts on global wave climate and potential coastal hazards. J. Geophys. Res. Oceans 125:e2020JC016464.
O’Higgins, T. G., Lago, M., and Dewitt, T. H. (2020). Ecosystem-Based Management, Ecosystem Services and Aquatic Biodiversity: Theory, Tools and Applications. Berlin: Springer Nature.
Oppenheimer, M., Glavovic, B. C., Hinkel, J., Van De Wal, R., Magnan, A. K., Abd-Elgawad, A., et al. (2019). “Sea level rise and implications for low-lying islands, coasts and communities,” in IPCC Special Report on the Ocean and Cryosphere in a Changing Climate, eds H.-O. Pörtner, D. C. Roberts, V. Masson-Delmotte, P. Zhai, M. Tignor, E. Poloczanska, et al. Geneva: IPCC.
Oumeraci, H. (2000). “The sustainability challenge in coastal engineering,” in Keynote Lecture Proceedings of the International Conference on Hydrodynamics ICHD, (Yokohama).
Oumeraci, H., Kortenhaus, A., Burzel, A., Naulin, M., Dassanayake, D. R., Jensen, J., et al. (2015). XtremRisK — integrated flood risk analysis for extreme storm surges at open coasts and in estuaries: methodology, key results and lessons learned. Coastal Eng. J. 57, 1–23.
Parkinson, R. W., and Ogurcak, D. E. (2018). Beach nourishment is not a sustainable strategy to mitigate climate change. Estuar. Coast. Shelf Sci. 212, 203–209. doi: 10.1016/j.ecss.2018.07.011
Partelow, S., Schlüter, A., Armitage, D., Bavinck, M., Carlisle, K., Gruby, R. L., et al. (2020). Environmental governance theories: a review and application to coastal systems. Ecol. Soc. 25, 1–21. doi: 10.4337/9781782548508.00008
Peterson, C. H., and Bishop, M. J. (2005). Assessing the environmental impacts of beach nourishment. BioScience 55, 887–896. doi: 10.1641/0006-3568(2005)055[0887:ateiob]2.0.co;2
Peterson, C. H., Bishop, M. J., Johnson, G. A., D’anna, L. M., and Manning, L. M. (2006). Exploiting beach filling as an unaffordable experiment: benthic intertidal impacts propagating upwards to shorebirds. J. Exp. Mar. Biol. Ecol. 338, 205–221. doi: 10.1016/j.jembe.2006.06.021
Piet, G., Culhane, F., Jongbloed, R., Robinson, L., Rumes, B., and Tamis, J. (2019). An integrated risk-based assessment of the North Sea to guide ecosystem-based management. Sci. Total Environ. 654, 694–704. doi: 10.1016/j.scitotenv.2018.11.001
Pranzini, E. (2018). Coastal erosion and shore protection: a brief historical analysis. J. Coast. Conserv. 22, 827–830. doi: 10.1007/s11852-017-0521-9
Rangel-Buitrago, N., Williams, A. T., and Anfuso, G. (2018). Hard protection structures as a principal coastal erosion management strategy along the Caribbean coast of Colombia. A chronicle of pitfalls. Ocean Coast. Manag. 156, 58–75. doi: 10.1016/j.ocecoaman.2017.04.006
Ruckelshaus, M., Reguero, B. G., Arkema, K., Compeán, R. G., Weekes, K., Bailey, A., et al. (2020). Harnessing new data technologies for nature-based solutions in assessing and managing risk in coastal zones. Int. J. Disaster Risk Reduct. 51:101795. doi: 10.1016/j.ijdrr.2020.101795
Ruckelshaus, M. H., Guannel, G., Arkema, K., Verutes, G., Griffin, R., Guerry, A., et al. (2016). Evaluating the benefits of green infrastructure for coastal areas: location, location, location. Coast. Manag. 44, 504–516. doi: 10.1080/08920753.2016.1208882
Schoonees, T., Gijón Mancheño, A., Scheres, B., Bouma, T. J., Silva, R., Schlurmann, T., et al. (2019). Hard structures for coastal protection, towards greener designs. Estuaries Coast. 42, 1709–1729. doi: 10.1007/s12237-019-00551-z
Schotanus, J., Walles, B., Capelle, J. J., Van Belzen, J., Van De Koppel, J., and Bouma, T. J. (2020). Promoting self-facilitating feedback processes in coastal ecosystem engineers to increase restoration success: testing engineering measures. J. Appl. Ecol. 57, 1958–1968. doi: 10.1111/1365-2664.13709
Silva, R., Chávez, V., Bouma, T. J., Van Tussenbroek, B. I., Arkema, K. K., Martínez, M. L., et al. (2019). The incorporation of biophysical and social components in coastal management. Estuaries Coast. 42, 1695–1708. doi: 10.1007/s12237-019-00559-5
Silva, R., Lithgow, D., Esteves, L. S., Martínez, M. L., Moreno-Casasola, P., Martell, R., et al. (2017). Coastal risk mitigation by green infrastructure in Latin America. Proc. Inst. Civ. Eng. Marit. Eng. 170, 39–54. doi: 10.1680/jmaen.2016.13
Silva, R., Martínez, M. L., Hesp, P. A., Catalan, P., Osorio, A. F., Martell, R., et al. (2014). Present and future challenges of coastal erosion in Latin America. J. Coast. Res. 71, 1–16. doi: 10.2112/si71-001.1
Silva, R., Martínez, M. L., Van Tussenbroek, B. I., Guzmán-Rodríguez, L. O., Mendoza, E., and López-Portillo, J. (2020). A Framework to manage coastal squeeze. Sustainability 12:10610. doi: 10.3390/su122410610
Silva, R., Mendoza, E., Mariño-Tapia, I., Martínez, M. L., and Escalante, E. (2016). An artificial reef improves coastal protection and provides a base for coral recovery. J. Coast. Res. 75, 467–471. doi: 10.2112/si75-094.1
Smart, L. S., Vukomanovic, J., Sills, E. O., and Sanchez, G. (2021). Cultural ecosystem services caught in a ‘coastal squeeze’ between sea level rise and urban expansion. Glob. Environ. Change 66:102209. doi: 10.1016/j.gloenvcha.2020.102209
Stive, M. J. F., De Schipper, M. A., Luijendijk, A. P., Aarninkhof, S. G. J., Van Gelder-Maas, C., Van Thiel De Vries, J. S. M., et al. (2013). A new alternative to saving our beaches from sea-level rise: the sand engine. J. Coast. Res. 29, 1001–1008. doi: 10.2112/jcoastres-d-13-00070.1
Stori, F. T., Peres, C. M., Turra, A., and Pressey, R. L. (2019). Traditional ecological knowledge supports ecosystem-based management in disturbed coastal marine social-ecological systems. Front. Mar. Sci. 6:571. doi: 10.3389/fmars.2019.00571
Suppasri, A., Shuto, N., Imamura, F., Koshimura, S., Mas, E., and Yalciner, A. C. (2013). Lessons learned from the 2011 Great East Japan tsunami: performance of tsunami countermeasures, coastal buildings, and tsunami evacuation in Japan. Pure Appl. Geophys. 170, 993–1018. doi: 10.1007/s00024-012-0511-7
Temmerman, S., Meire, P., Bouma, T. J., Herman, P. M. J., Ysebaert, T., and De Vriend, H. J. (2013). Ecosystem-based coastal defence in the face of global change. Nature 504, 79–83. doi: 10.1038/nature12859
Temmink, R. J. M., Christianen, M. J. A., Fivash, G. S., Angelini, C., Boström, C., Didderen, K., et al. (2020). Mimicry of emergent traits amplifies coastal restoration success. Nat. Commun. 11:3668.
van Tussenbroek, B. I., Hernández Arana, H. A., Rodríguez-Martínez, R. E., Espinoza-Avalos, J., Canizales-Flores, H. M., González-Godoy, C. E., et al. (2017). Severe impacts of brown tides caused by Sargassum spp. on near-shore Caribbean seagrass communities. Mar. Pollut. Bull. 122, 272–281. doi: 10.1016/j.marpolbul.2017.06.057
Vousdoukas, M. I., Mentaschi, L., Hinkel, J., Ward, P. J., Mongelli, I., Ciscar, J.-C., et al. (2020a). Economic motivation for raising coastal flood defenses in Europe. Nat. Commun. 11:2119.
Vousdoukas, M. I., Ranasinghe, R., Mentaschi, L., Plomaritis, T. A., Athanasiou, P., Luijendijk, A., et al. (2020b). Sandy coastlines under threat of erosion. Nat. Clim. Chang. 10, 260–263. doi: 10.1038/s41558-020-0697-0
Wang, M., Hu, C., Barnes, B. B., Mitchum, G., Lapointe, B., and Montoya, J. P. (2019). The great atlantic Sargassum belt. Science 365:83. doi: 10.1126/science.aaw7912
Webster, P. J., Holland, G. J., Curry, J. A., and Chang, H. R. (2005). Changes in tropical cyclone number, duration, and intensity in a warming environment. Science 309:1844. doi: 10.1126/science.1116448
Wittebrood, M., De Vries, S., Goessen, P., and Aarninkhof, S. (2018). Aeolian sediment transport at a man-made dune system; building with nature at the Hondsbossche dunes. Coast. Eng. Proc. 1:83. doi: 10.9753/icce.v36.papers.83
Wright, L. D., and Nichols, C. R. (2019). Tomorrow’s Coasts: Complex and Impermanent. Berlin:Springer.
Keywords: climate change, coastal sustainability, risk reduction, coastal management, coastal resilience, coastal protection
Citation: Silva R, Oumeraci H, Martínez ML, Chávez V, Lithgow D, van Tussenbroek BI, van Rijswick HFMW and Bouma TJ (2021) Ten Commandments for Sustainable, Safe, and W/Healthy Sandy Coasts Facing Global Change. Front. Mar. Sci. 8:616321. doi: 10.3389/fmars.2021.616321
Received: 12 October 2020; Accepted: 01 February 2021;
Published: 23 February 2021.
Edited by:
Wen-Cheng Wang, National Taiwan Normal University, TaiwanReviewed by:
Jenifer Dugan, University of California, Santa Barbara, United StatesGoneri Le Cozannet, Bureau de Recherches Géologiques et Minières, France
Copyright © 2021 Silva, Oumeraci, Martínez, Chávez, Lithgow, van Tussenbroek, van Rijswick and Bouma. This is an open-access article distributed under the terms of the Creative Commons Attribution License (CC BY). The use, distribution or reproduction in other forums is permitted, provided the original author(s) and the copyright owner(s) are credited and that the original publication in this journal is cited, in accordance with accepted academic practice. No use, distribution or reproduction is permitted which does not comply with these terms.
*Correspondence: Rodolfo Silva, UlNpbHZhQ0BpaW5nZW4udW5hbS5teA==; M. Luisa Martínez, bWFyaXNhLm1hcnRpbmV6QGluZWNvbC5teA==; Valeria Chávez, VkNoYXZlekNAaWluZ2VuLnVuYW0ubXg=