- 1Centro de Investigación en Ecosistemas de la Patagonia (CIEP), Coyhaique, Chile
- 2Centro de Investigación Oceanográfica COPAS Sur-Austral, Universidad de Concepción, Concepción, Chile
- 3Dipartimento di Scienze della Vita e dell’Ambiente, Università Politecnica delle Marche, Ancona, Italy
- 4Dipartimento di Scienze della Terra, dell’Ambiente e della Vita, Università degli Studi di Genova, Genoa, Italy
Aulacomya atra is an active suspension feeder, spatially dominant in the shallow-water hard-bottom benthic communities of the Chilean Patagonia fjords. In this region, the vertical flux of autochthonous organic matter (OM) reaching the benthos is augmented by allochthonous OM both from a terrestrial origin and from intensive salmon farming. This mixed pool of OM represents a potential source of food for a variety of benthic consumers, but to date little is known about the degree of utilization of these materials by filter feeders organisms. In this context, feeding experiments on A. atra in Puyuhuapi Fjord, Chilean Patagonia, were conducted during summer and winter 2018–2019. These experiments were designed to determine ingestion rates (IR) of A. atra fed with autochthonous (bacterial and microplanktonic community) and allochthonous (salmon food pellet) OM. Additionally, samples of A. atra tissues and suspended particulate organic matter (SPOM) were taken from the study area for stable isotope analysis. Data from laboratory experiments indicated that A. atra can feed on both autochthonous and allochthonous OM, but higher IR were detected in individuals fed with salmon food pellets. Because the IR is sensitive to food particle density rather than specific type of food, diet preferences of A. atra in feeding experiments could not be determined. Stable isotope analyses indicate that A. atra in natural environment preferentially exploits food with an isotopic signal corresponding to autochthonous OM, highlighting the primary role of phytoplankton carbon in their diet. Extensive utilization of terrestrially derived OM is therefore unlikely, although utilization of OM derived from salmon farming is not precluded because of the overlap in isotopic signal between food pellets and marine plankton.
Introduction
In aquatic ecosystems, animals that feed on particles suspended in the water are collectively known as filter feeders (Jørgensen, 1990). Bivalves tend to be the dominant suspension feeders in benthic communities (Sebens et al., 2016; Filgueira et al., 2019), filtering large volumes of water whilst retaining a wide range of particle sizes (ca. 4–35 μm diameter) (Voudanta et al., 2016). By filtering water to satisfy their nutritional demands, these organisms remove substantial quantities of microscopic particles from seston, such as bacteria, phytoplankton, detritus, and suspended sediments (Wright et al., 1982; Langdon and Newell, 1996; Prins et al., 1998; Kreeger and Newell, 2000; Cranford et al., 2011). Therefore, these bottom dwelling organisms process large amounts of suspended organic matter (OM) and are often major agents of pelagic-benthic coupling and nutrient cycling (Norkko et al., 2001). The total amount of OM consumed by bivalves is largely determined by their ingestion rate (IR) (Riisgård, 1988) which is a parameter of great ecological importance, and critical to the understanding of their impact on particles fluxes in coastal environments (Winter, 1978). Filter feeding behavior in bivalves is known to be responsible for fluctuations in both the abundance and the composition of seston (Bayne, 1998; Prins et al., 1998). Several studies have suggested that bivalve grazing can control phytoplankton abundance (Cloern, 1982; Prins et al., 1998; Lonsdale et al., 2009; Lucas et al., 2016), favor small phytoplankton organisms (Greene et al., 2011; Jacobs et al., 2016; Cranford, 2019) and decrease phytoplankton production (Dolmer, 2000). Phytoplankton is clearly a major source of nutrition for these organisms (Vaughn and Hoellein, 2018), but bacteria and detritus from terrestrial origins have also been described as important food source for bivalves during periods of low phytoplankton abundance (Langdon and Newell, 1990; Kreeger and Newell, 1996). Mussels are also known to filter small particles of salmon feed (Reid et al., 2010; MacDonald et al., 2011) and fecal pellets from salmon farming areas (Reid et al., 2010), incorporating this waste material as a food source (Gao et al., 2006; Redmond et al., 2010; Handå et al., 2012a,b). Several studies have indicated that bivalves growing adjacent to salmon farming areas remove OM advected from cages by increasing their growth rates (Lander et al., 2004; Peharda et al., 2007; Sarà et al., 2009) and helping to reduce the negative ecological impacts of the salmon industry (Lefebvre et al., 2000; MacDonald et al., 2011) such as the increase in load of OM in the water column and seafloor (Quiñones et al., 2019).
In Chilean Patagonian fjords, salmon farming represents the principal aquaculture activity (Buschman et al., 2006). This industry releases large quantities of organic and inorganic wastes (uneaten feed, feces and excretory products) that modify the particulate and dissolved materials in the water column (Quiñones et al., 2019) and represent a permanent input of allochthonous OM into the local fjord ecosystem (Iriarte et al., 2014). This allochthonous OM, together with high levels of autochthonous OM produced by phytoplankton in Chilean fjords (Montero et al., 2011, 2017a,b), provides a heterogeneous pool of organic substrates available as food for benthic consumers.
Several studies have addressed trophic structure of benthic communities (Mayr et al., 2011; Zapata-Hernández et al., 2014; Andrade et al., 2016; Quiroga et al., 2016), but little information is available on the ecological role of bivalves species in the structure and functioning of Patagonian fjord ecosystems. One of the key suspension feeder in the Patagonian fjords is the ribbed mussel Aulacomya atra (Molina, 1782), which normally inhabits protected and semi-protected shallow rocky shores (Betti et al., 2017), and is often found in close vicinity to salmon farms. Despite its importance in bentho-pelagic carbon fluxes of the Patagonian fjords, little is known about the feeding behavior of A. atra, including its potential role in processing the uneaten salmon feed. The aim of the present study was to determine the capacity of A. atra to assimilate and ingest OM derived both from salmon feed (allochthonous), and from a phytoplankton and bacterial origin (autochthonous) by means of stable isotope analysis and experimental feeding studies conducted in Puyuhuapi Fjord, Chilean Patagonia, during summer and winter periods between 2018 and 2019.
Materials and Methods
Study Area and Sampling of Aulacomya atra
The study was conducted in Puyuhuapi Fjord which extends for about 90 km between 44°19′ S and 44°57′ S in northern Chilean Patagonia (Figure 1), and runs in a N–NE direction, connecting directly to the open sea via the Moraleda Channel at its mouth, and through the Jacaf Channel near the head (Schneider et al., 2014). The hydrography of this area is characterized by an estuarine type of circulation with a vertical two layer structure, comprised of a highly variable 5–10 m deep freshwater layer overlying a more uniform, saltier sub-pycnocline layer (Schneider et al., 2014 and references therein). The deeper saline water originates from Sub-Antarctic Surface Water (SAAW) characteristic of open ocean environments in these latitudes (Chaigneau and Pizarro, 2005). The freshwater upper layer is mainly supplied by the Cisnes River and by rain runoff (Schneider et al., 2014). The surface outflow of buoyant freshwater in the fjord carries high concentrations of silicic acid derived from rivers, while SAAW waters are typically enriched with nitrate and orthophosphate (Silva, 2008). Surface salinity is higher in the north than in the south, suggesting an intrusion of oceanic surface waters into the north of Puyuhuapi Fjord through the Jacaf Channel, forced by westerly winds (Schneider et al., 2014).
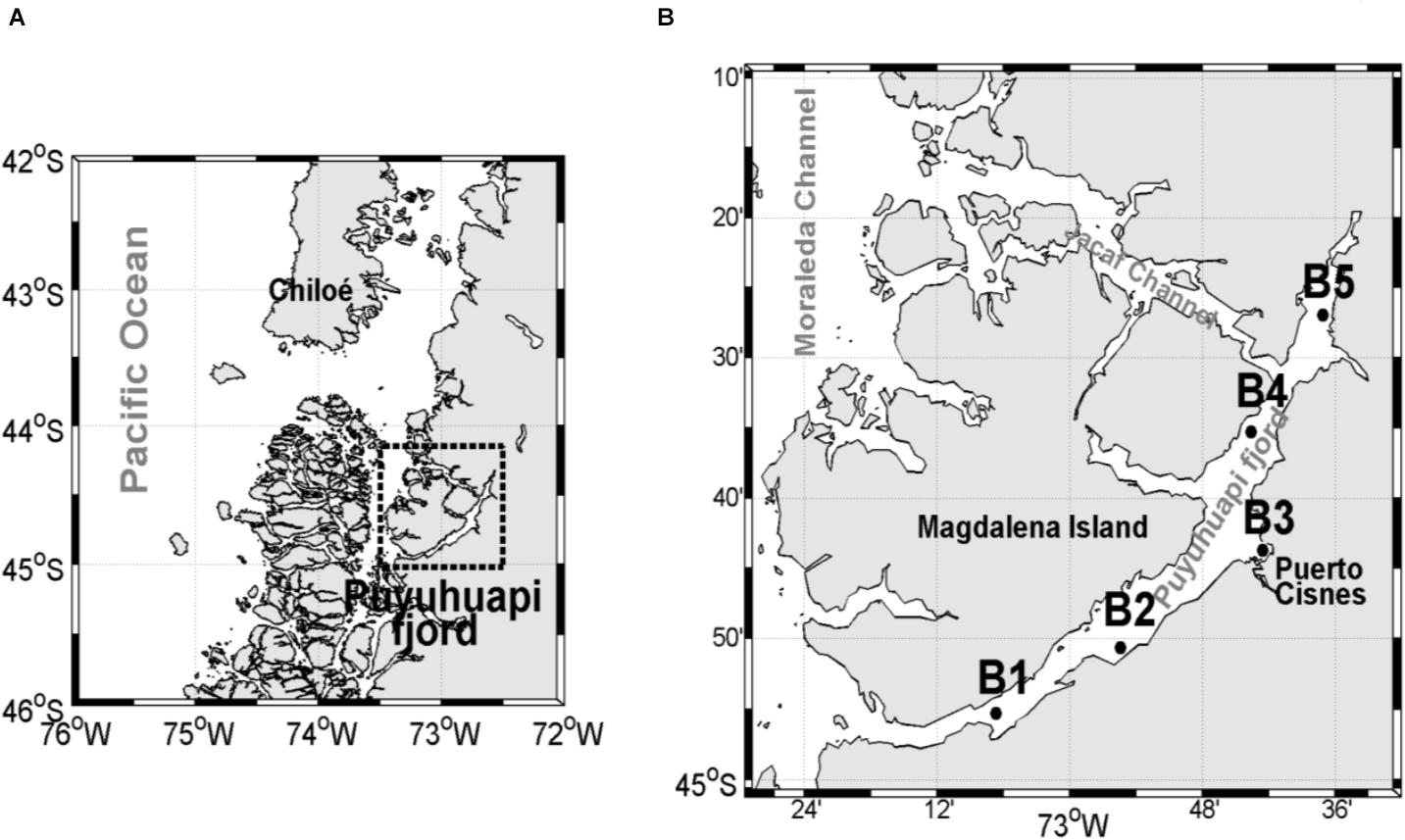
Figure 1. (A) Location of the study area in Chilean Patagonia, (B) close-up of the study area with the position of the sampling stations along the Puyuhuapi Fjord.
Aulacomya atra was collected in the Puyuhuapi Fjord during summer (February) and winter (July) in 2 years, 2018 and 2019 (Supplementary Table 1). Samples of A. atra for both feeding experiments and stable isotopes analysis were collected by scuba diving at depths ranging from 5 to 10 m at several sampling stations (Figure 1). After collection, mussels for the feeding experiment were transferred to the field laboratory, cleaned of epibionts and debris, and maintained in a 200-liters seawater tank with constant aeration and at ambient temperature prior to the experimental protocols (approximately 24 h after collection). Mussels for the stable isotopes analysis were treated as described in detail in section “Stable Isotopes.”
Feeding Experiments
The feeding experiments were performed using plastic aquaria, each of 6-liters capacity and provided with a pumped air supply (3 L/min). Three different type of food (treatments) were tested: bacterial community, planktonic community, and allochthonous OM derived from salmon food pellets.
The first treatment – referred here as the bacterial community treatment (BC_T) – was prepared by gravity filtering seawater onto 22-μm mesh to remove large zooplankton and microphytoplankton. A peristaltic pump was then used to gently filter this water through 0.8-μm pore size sterile membrane filters (Millipore) in order to separate bacteria from the rest of the planktonic community (including potential predators). The second treatment – referred here as planktonic community treatment (PC_T) – was prepared by gravity filtering seawater onto 150-μm mesh to remove larger plankton. The third treatment consisted of 0.2-μm filtered seawater amended with salmon food pellets (SFP_T). This treatment was prepared by gravity filtering seawater onto 22-μm mesh. Then, a peristaltic pump was used to gently filter this water through 0.2-μm sterile membrane filters (Millipore), in order to remove bacteria and other microorganisms. Finally, 1 g of salmon food pellet was finely ground into particles <1 mm using a mortar; this powder was then added to the filtered water. For each treatment, an experimental and a control aquaria were used. At the beginning of each experiment, both aquaria were filled with the filtered water (BC_T, PC_T, SFP_T water, depending on the treatment) and one specimen of A. atra was placed in each experimental aquarium, whilst the control ones remained without individuals. The experiments began as soon as A. atra valves opened. Triplicate water samples of 500 mL were taken from experimental and control aquaria at the initial time (t1), and again 1 h later (t2). Samples from t1 and t2 were processed for particulate organic carbon (POC) analyses. Total POC was measured by filtering 500 mL water samples on pre-combusted GF/F glass microfiber filters (4 h at 450°C) which were then frozen at –20°C until analysis. Prior to the analysis, filters were defrosted and acidified with HCL. After acidification, the HCL was removed and the filters were dried at 50°C for 24 h. Filters were analyzed at the Laboratory of Biogeochemistry and Applied Stable Isotopes (LABASI, PUC), Chile.
The rate of change in POC concentration from control (kc) and experimental (kg) aquaria was calculated according to Frost (1972) equation:
where C1 and C2 are POC concentrations (mg C L–1) at initial (t1) and final time (t2), respectively, and k (h–1) corresponds to the rate of change in POC concentration during the experiments. The grazing coefficient is g (h–1) and was calculated according to Ribes et al. (1998) equation:
where kc and kg are the rates of change in POC concentration from control and experimental aquaria, respectively.
The clearance rate, CR (volume swept clear per individual per time) was calculated according to Ribes et al. (1998) equation:
where V is the volume of the aquaria (L), n is the number of A. atra in the experimental aquaria and g is the grazing coefficient (h–1). For comparative purposes, clearance rates were also standardized (CRs) to constant biomass of 1 g A. atra dry flesh weight (Cranford et al., 2011). Finally, the ingestion rate, I (particles ingested per individual per time) was calculated as the product of CR (L individual–1 h–1) and the concentration of POC (mg L–1) using Ribes et al. (1998) formula:
where C is the initial POC concentration (C1) in the experimental aquaria, calculated according to the following equation (Frost, 1972; Saiz, 1993):
When the rate of change in POC concentrations was higher in experimental (kg) than in control (kc) aquaria, negative grazing coefficients (g) were obtained. Negative g are reported in Table 1; according to previous studies, negative values were set up to zero (Strom and Fredrickson, 2008; Stoecker et al., 2014, 2015; Anderson et al., 2018; Menden-Deuer et al., 2018; Anderson and Harvey, 2019), in order to calculate the mean and standard error of grazing in each treatment, and subsequently to estimate means and standard deviations in CR and IR. The significance of grazing (g values > 0) for each food type (treatments) was tested by comparing the rate of change in POC concentration from control (kc) and experimental (kg) aquaria using a two-tailed Wilcoxon test. Individual values of kc and kg for each experiment (Table 1) correspond to the average of three replicates obtained from control (Supplementary Table 1) and experimental (Supplementary Table 2) aquaria, respectively. Wilcoxon test was applied to seven experiments conducted under PC_T (autochthonous source), nine under BC_T (autochthonous source), and five under SFP_T (allochthonous source). In this last case, Wilcoxon test considered an alpha = 0.1 due the low number of sampling points.
To examine the relationship between CR and size/weight of the individuals under study, after each experiment, shell lengths and dry (24 h at 60°C) flesh weights were determined for each individual.
The non-parametric Kruskal–Wallis test (H) was used to examine differences in g, CR and IR between the different food sources (treatments). Kolmogorov-Smirnov test (K-S) was used to examine seasonal differences in CR and CRs.
Stable Isotopes
Three specimens of A. atra were collected from each sampling station for stable isotopes analysis in the study area (Figure 1). After collection, mussels were maintained in filtered seawater (0.2 μm) in a plastic container for 24 h and were then rinsed with distilled water and frozen at –20°C. Frozen samples were thawed and dissected. A. atra tissues were lyophilized and grounded prior to analysis.
In order to measure the stable isotope composition in suspended particulate organic matter (SPOM), samples were monthly collected from three depths (2, 10, and 20 m) from March to May 2018 and from July 2018 to February 2019 at sampling station B4. In addition, samples from 2 and 20 m depth were obtained from station B3 between March and May 2018 and in July 2018. Water samples (5 L) were filtered under gentle vacuum through GF/F filters (pre combusted for 4 h at 450°C) and refrigerated prior to analysis. The isotopic signal from salmon food pellets used in the feeding experiments of February/July 2018 and February 2019 was also measured. Tissues, filters and salmon food pellets samples were analyzed at the Laboratory of Biogeochemistry and Applied Stable Isotopes (LABASI, PUC, Chile) with an Isotope Ratio Mass Spectrometer (Thermo Fisher Scientific, Delta V Advantage IRMS) coupled with and Elemental Analyzer (Flash, EA 2000). Data are expressed in the standard ∂ unit notation:
where R represents the 13C/12C or 15N/14N ratio for carbon and nitrogen, respectively, reported relative to VPDB for carbon and to atmospheric N2 for nitrogen.
The non-parametric Kruskal–Wallis test (H) was used to examine seasonal differences in carbon (∂13C) and nitrogen (∂15N) signals from A. atra tissue and SPOM samples. In addition, isotopic signals (∂13C and ∂15N) from different sampling stations were also examined using test H.
To assess the potential contribution of the different carbon sources (allochthonous and autochthonous) in the diet of A. atra, and in the SPOM samples, an isotopic mixing model was used (Dauby, 1989; Fry, 2006). Allochthonous terrestrial OM (TOM) is isotopically lighter than its marine counterpart (Bianchi, 2007). In Chilean fjords TOM has an average δ13C isotopic signals of −29‰ while autochthonous marine OM (MOM) has an average δ13C isotopic signal of −1‰ (Vargas et al., 2011; Lafon et al., 2014; González et al., 2019). The isotopic mixing model considered three end members recorded in this study: (i) autochthonous OM from a marine source with a ∂13C of −18.3 (measured at station B4, 2 m depth), (ii) allochthonous OM from a terrestrial source with a ∂13C of −29.9 (measured at station B4, 20 m depth), and (iii) allochthonous OM from salmon food pellets used in feeding experiments with a ∂13C of −21.94.
Results and Discussion
Differences in rates of change of POC concentrations in control (Supplementary Table 2) and experimental (Supplementary Table 3) aquaria were used to calculate grazing by A. atra (Table 1). The highest average grazing values were recorded on bacterial community (0.20 h–1 ± SE 0.08) and salmon food pellet treatment (0.26 h–1 ± SE 0.12), while planktonic community treatment showed the lowest (0.05 h–1 ± SE 0.02). Grazing values greater than zero; obtained when the rate of change of POC concentration was significantly higher under control conditions than in experimental aquaria (Figure 2A), indicated that A. atra was able to feed on both of the autochthonous sources of OM (bacterial and total microplanktonic community; Wilcoxon test, p < 0.05) and on the allochthonous source (ground salmon food pellet; Wilcoxon test, p < 0.1). The present study therefore confirms observations that ribbed mussels are omnivores, feeding on a wide array of organic particles (Kreeger et al., 2018), and highlights the ability of A. atra to filter both small (bacterial community <0.8 μm) and large (ground salmon food pellet <1 mm) food particles. Bacteria are generally too small to be efficiently retained by the gills of most bivalves, however, the particular morphology of gills of ribbed mussels – such as A. atra and Geukensia demissa (Dillwyn, 1817) – makes these species very effective grazers of bacteria (Wright et al., 1982; Stuart and Klumpp, 1984; Langdon and Newell, 1990). The bacterial community therefore makes an appreciable contribution to the diet of these species (Stuart et al., 1982; Kreeger and Newell, 1996). Bacterial ingestion by A. atra may also represent an important step in the vertical transfer of food and energy in Patagonian fjord ecosystems, and could be a particularly important route for allochthonous OM (from terrestrial origin or from salmon farming waste) entering indirectly into the benthic food web as material assimilated by bacterial community (Zapata-Hernández et al., 2014).
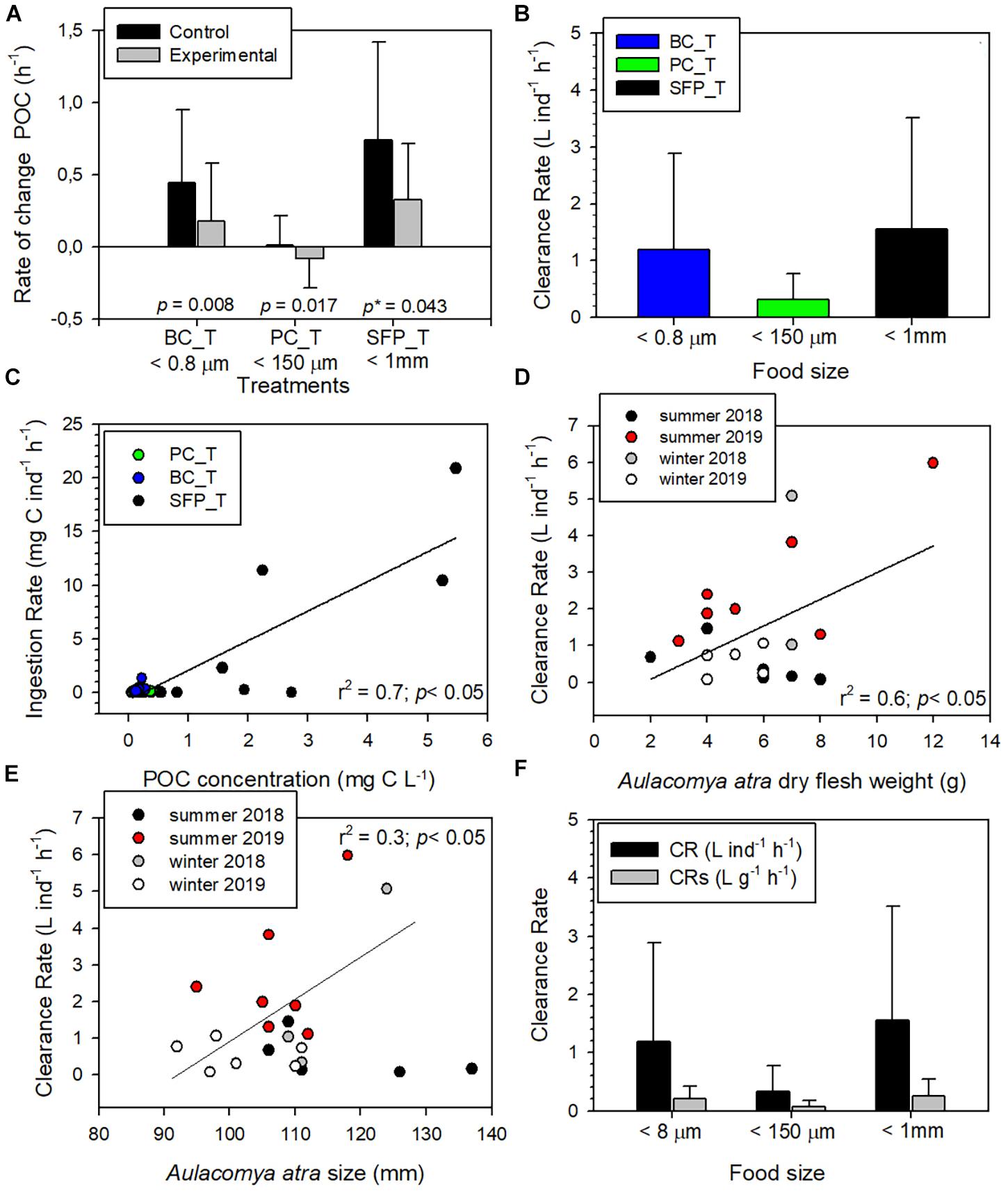
Figure 2. (A) Rate of change in POC concentrations (average ± SD) from feeding experiments in control and experimental aquaria under bacterial community (BC_T), planktonic community (PC_T) and salmon food pellet (SFP_T) treatments. The mean value in both control and experimental aquaria correspond to all experiments carried out under the same treatment with a grazing coefficient > 0 (Table 1), p: p-value with alpha = 0.05, p*: p-value with alpha = 0.1. (B) Aulacomya atra clearance rate (average ± SD) in each type of treatment. The mean value correspond to all experiments carried out under the same treatment. (C) Relation between POC concentration and ingestion rate (IR) of A. atra during feeding experiments. (D) Clearance rate as a function of dry flesh weight in A. atra during sampling campaigns. (E) Clearance rate as a function of A. atra size during sampling campaigns. (F) Comparison between clearance rates by individual (CR) and those standardized by weight (CRs) in each treatment.
Consumption of salmon waste particles (uneaten food and feces) by blue mussels (Mytilus edulis; Linnaeus, 1758) under laboratory conditions has been described in the literature (Reid et al., 2010; MacDonald et al., 2011; Handå et al., 2012b). These studies have shown that mussels not only have the ability to efficiently capture and ingest particulate organic material (POM) from salmon feed and feces, but in some cases, mussels can in fact select fish feed particles and utilize them more efficiently than feces particles (Handå et al., 2012b). POM derived from salmon cages has previously been shown to be a food source as important as microalgae for mussels (Reid et al., 2010). In feeding experiments, clearance rates (CR) measured in bacterial (0–5.98 L ind–1 h–1) and salmon food pellet (0–5.07 L ind–1 h–1) treatments were quite similar and showed a range of values greater than those measured in planktonic community treatment (0–1.30 L ind–1h–1) (Figure 2B). However, no significant differences were observed between the different food sources (Kruskal–Wallis test, p > 0.05). The highest ingestion rates (IR) (2.19 to 20.9 mg C ind–1 h–1) were obtained for salmon food pellet treatment (allochthonous OM) (Figure 2C) indicating that A. atra was able to capture and ingest salmon feed more efficiently than bacteria and microplanktonic organisms (Kruskal–Wallis test, p < 0.05). In contrast, highest IR values of between 0.17 and 0.31 mg C ind–1 h–1 recorded under autochthonous feeding conditions were significantly lower (Kruskal–Wallis test, p < 0.05) for the planktonic community treatment than the range of 0.28–1.31 mg C ind–1 h–1 recorded in bacterial treatment (Figure 2C and Table 1). These results do not necessarily represent a selection or preference of A. atra for a given food type since the diets provided in this study were not mixed. Highest IR values were associated with higher POC concentration (IR and POC concentration were positively correlated, Figure 2C, r2 = 0.73, p < 0.05). Overall, salmon food pellet treatment with POC concentration >2 mg C L–1 had a greater IR (>10 mg C ind–1 h–1) than those treatments with a lower POC concentration (Figure 2C).
Clearance rates (values > 0) was positively correlated to dry flesh weight of A. atra (more clearly observed during the 2019 summer campaign; r2 = 0.64, p < 0.05) and with A. atra size (more clearly observed during winter campaigns and summer 2019; r2 = 0.3, p < 0.05), suggesting that in most cases, an increase in weight and/or size of A. atra results in a higher CR (Figures 2D,E). The weight-standardized clearance rate (CRs) showed the same pattern as CR, although the values observed were lower (Figure 2F). Seasonally, CR and CRs were both significantly higher in summer than in winter (Kolmogorov-Smirnov test, p < 0.05), probably associated with the influence of water temperature on bivalve metabolic rates. Indeed, greater CR values are generally associated with high temperature (Haure et al., 1998; Sylvester et al., 2005; Kang et al., 2016). During summer, experiments were conducted within the 15–18°C temperature range whereas in the winter period the temperature range was lower (8–10°C). CR and IR values measured in this study for A. atra well agree with those reported in previous laboratory studies, both with A. atra (Griffiths and King, 1979) and with other species of mussels such as M. edulis (MacDonald et al., 2011; Handå et al., 2012b).
Stable isotopes show long-term nutrition, yielding accurate information about the characteristics of the assimilated food (Valiela, 1995), being among the most suitable tools to describe trophic relationships in coastal ecosystems (Wada et al., 1991). In this study carbon (∂13C) and nitrogen (∂15N) signatures from samples of A. atra tissue and SPOM from the Puyuhuapi Fjord were plotted together (Figure 3A). ∂13C and ∂15N values of A. atra tissues did not differ significantly (Kruskal–Wallis test, p > 0.05) among sampling station throughout the study period (B1: ∂13C −18.5 ± 0.9‰, ∂15N 9.5 ± 0.7‰; B2: ∂13C −19.5 ± 1.7‰, ∂15N 9.3 ± 0.7‰; ‰; B3: ∂13C −20.1 ± 2.2‰, ∂15N 9.1 ± 0.2‰; B4: ∂13C −19.9 ± 1.2‰, ∂15N 8.4 ± 0.4‰; B5: ∂13C −19.2 ± 1.9‰, ∂15N 9.2 ± 0.5‰) (Figure 3A and Supplementary Table 4). Likewise, the ∂15N of A. atra tissues was quite similar seasonally (February 2018: 8.9 ± 0.8‰, July 2018: 8.7 ± 0.2‰ and February 2019: 9.6 ± 0.7‰) and showed no significant differences (Kruskal–Wallis test, p > 0.05) (Figure 3A and Supplementary Table 4). On the contrary, ∂13C values of A. atra tissues were significantly different (Kruskal–Wallis test, p < 0.05) between February 2018 (−18.4 ± 0.8‰), July 2018 (−18.6 ± 0.7‰) and February 2019 (−20.9 ± 0.9‰) (Figure 3A and Supplementary Table 4). These seasonal differences observed in ∂13C values might be related to the different food source (i.e., autochthonous vs. allochthonous) that was available in the water column for consumption of A. atra. In fact, ∂13C values in SPOM samples – that include a wide range of potential food source for filter feeder organisms – between March and July 2018 at surface layer (2–10 m) ranged from −18.3 to −27.1‰, while between August 2018 and February 2019 ranged from −21.5 to −29.7‰ (Figure 3A). Different seasonal ranges observed in the isotopic signal of SPOM were possibly determined by the contribution of oceanic surface water and the increase in freshwater discharge, that usually occur in the Puyuhuapi Fjord during winter and spring months, respectively (Schneider et al., 2014). Oceanic waters are indicative of a strong marine component (∂13C average = −19‰), while freshwater discharge has a high terrestrial signature (∂13C average = −29‰) (Vargas et al., 2011; Lafon et al., 2014; González et al., 2019). In addition, depleted values of ∂15N with values low 4‰ (as those observed between August 2018 and February 2019 in the study area) are indicative of a high terrestrial signal (Sepúlveda et al., 2011). ∂13C and ∂15N values of SPOM from 2 and 20 m did not show significant differences between sampling station B3 and B4 (Kruskal–Wallis test, p < 0.05; Figure 3).
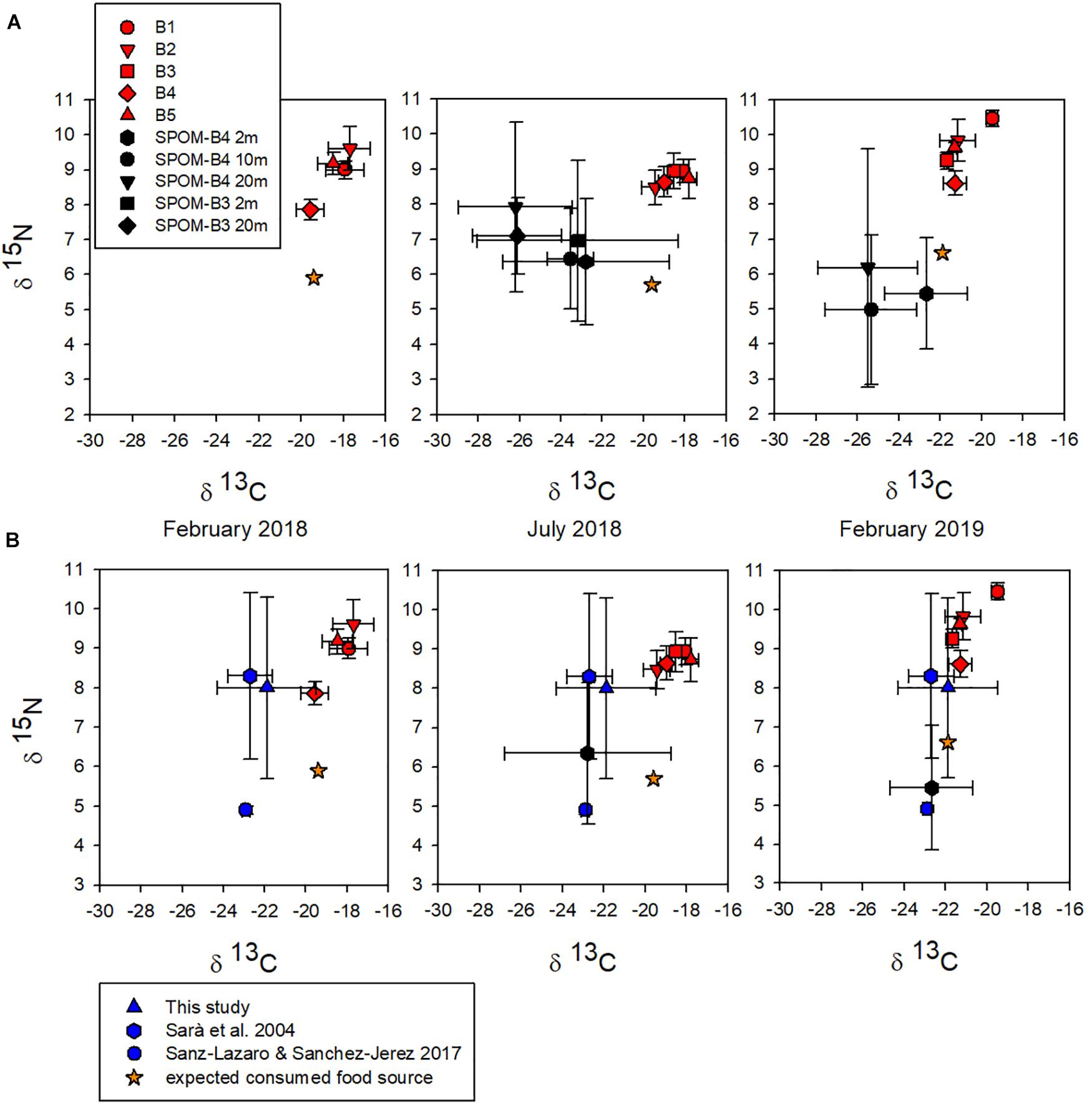
Figure 3. (A) ∂13C and ∂15N biplot showing stable isotopic composition of Aulacomya atra tissues (red shapes) and of suspended particulate organic matter (SPOM) (black shapes). Samples of A. atra were obtained from different sampling stations across the Puyuhuapi Fjord (from B1 to B5). Samples of SPOM were obtained from different sampling depths (2, 10, and 20 m) in B4 and B3 stations. The orange star indicates the expected isotopic signature of the preferred food source. (B) ∂13C and ∂15N biplot showing stable isotopic composition in A. atra tissues and in pellet salmon food from this study and others.
Average ∂13C values of SPOM (2–20 m) among March 2018 and July 2018 (−24.4 ± 1.7‰) and between August 2018 and February 2019 (−25.2 ± 0.4‰) were significantly more depleted (Kruskal–Wallis test, p > 0.05) than those measured in A. atra tissues in July 2018 (−18.6 ± 0.7‰) and February 2019 (−20.9 ± 0.9‰) (Figure 3A and Supplementary Table 4). Likewise, average ∂15N values of SPOM between March 2018 and July 2018 (6.9 ± 0.6‰) and between August 2018 and February 2019 (5.5 ± 0.6‰) were significantly lower (Kruskal–Wallis test, p > 0.05) than those measured in A. atra tissues in July 2018 (8.7 ± 0.2‰) and February 2019 (9.6 ± 0.7‰) (Figure 3A and Supplementary Table 4). These ∂13C and ∂15N signatures of A. atra tissues and SPOM samples well agree with previous reports in Chilean fjords (Mayr et al., 2011; Andrade et al., 2016) and with studies on bivalves in fish farming areas (Mazzola and Sarà, 2001; Gao et al., 2006; Redmond et al., 2010).
Since the potential food sources ingested by A. atra are those which are depleted by 1‰ in ∂13C and by ∼ 3‰ in ∂15N compared to the tissues of this consumer (Minagawa and Wada, 1984; Rau et al., 1990; Post, 2002), we might expect that the preferentially exploited food source shows values of −19.4‰∂13C and 5.9‰∂15N in February 2018, −19.6‰∂13C and 5.7‰∂15N in July 2018 and −21.9‰∂13C and 6.6‰∂15N in February 2019 (Figure 3). These isotopic ratios were close to the more enriched range of SPOM isotopic signal from 2m deep in the water column (Figure 3) that mainly correspond to autochthonous organic production by marine phytoplankton, with ranges of ∂13C between −18 and −22‰ (Goericke and Fry, 1994) and ∂15N between 6 and 7‰ (Wada et al., 1987). In this context, the results indicate that A. atra preferentially exploits food resources with signals consistent with autochthonous marine OM in Puyuhuapi Fjord. However, the average isotopic composition of the salmon food pellets used in feeding experiments from this study (∂13C −21.9 ± 2.4‰, ∂15N 8.0 ± 2.3‰) and in other studies (∂13C −22.7 ± 1.1‰, ∂15N 8.3 ± 2.1‰ from Sarà et al., 2004 and ∂13C −22.9 ± 0.2‰, ∂15N 4.9 ± 0.1‰ from Sanz-Lazaro and Sanchez-Jerez, 2017; Figure 3B) overlaps with marine phytoplankton signal in SPOM samples, mainly in February 2019 (Figure 3B). Estimating the contribution of this allochthonous salmon feed material to the diet of A. atra is therefore not so clear cut. The mixing equations clarify this point and indicate that both allochthonous material derived from uneaten salmon feed and autochthonous materials highly contribute to its diet (Supplementary Table 5). In February and July 2018, the greatest relative contributions (92–99%) came from autochthonous OM, while the terrestrial contribution to the diet of A. atra was minimal (1–23%) throughout the study (Supplementary Table 5). Regarding SPOM samples, the highest relative contributions were from autochthonous OM (40–60%) and allochthonous material from salmon pellet food (58–87%) in surface waters (2–10 m). In subsurface waters (20 m) the highest contributions (40–70%) were mainly from allochthonous terrestrial material (Supplementary Table 5).
The influence of allochthonous carbon sources on hard-bottom benthic communities in Chilean fjords has been poorly studied to date. Some authors have suggested that allochthonous material from a terrestrial origin plays a minor role as a food for suspension feeders (Mayr et al., 2011; Zapata-Hernández et al., 2014) and that autochthonous OM from phytoplankton production provides one of the principal food sources to these populations (Andrade et al., 2016). Our data confirm that phytoplankton carbon plays a primary role in the diet of A. atra and apparently excluding extensive utilization of terrestrially derived OM. However, the present study cannot preclude significant utilization of OM derived from salmon farming.
Data Availability Statement
The original contributions presented in the study are included in the article/Supplementary Material, further inquiries can be directed to the corresponding author.
Author Contributions
PM: study design, data collection, analysis of data, interpretation of results, and manuscript leader. MC: study design, data collection, analysis of data, and manuscript revision. FB: data collection and manuscript revision. GB: manuscript revision. GD: study design, critical revision, and edition of final version of the manuscript. All authors contributed to the writing of the manuscript.
Funding
This research was funded by FIC No 40000236-0 and (partially) by COPAS Sur-Austral ANID PIA APOYO CCTE AFB 170006 and Programa Regional CONICYT R17A10002, Laboratorio EcoClimático Región de Aysén.
Conflict of Interest
The authors declare that the research was conducted in the absence of any commercial or financial relationships that could be construed as a potential conflict of interest.
Publisher’s Note
All claims expressed in this article are solely those of the authors and do not necessarily represent those of their affiliated organizations, or those of the publisher, the editors and the reviewers. Any product that may be evaluated in this article, or claim that may be made by its manufacturer, is not guaranteed or endorsed by the publisher.
Acknowledgments
The authors thank the technical staff of Centro de Investigación en Ecosistemas de la Patagonia (CIEP) for support during field work.
Supplementary Material
The Supplementary Material for this article can be found online at: https://www.frontiersin.org/articles/10.3389/fmars.2021.612406/full#supplementary-material
Supplementary Table 1 | Date, season, and geographical coordinates of Aulacomya atra sampling sites in the Puyuhuapi Fjord.
Supplementary Table 2 | Initial (C1) and final (C2) POC concentration [mean ± standard deviation (SD)] in control aquaria from different treatments during feeding experiments. Kc corresponds to rate of change in POC concentration estimated in control aquaria during feeding experiments. PC_T, planktonic community treatment; BC_T, bacterial community treatment; and SFP_T, salmon food pellet treatment.
Supplementary Table 3 | Initial (C1) and final (C2) POC concentration [mean ± standard deviation (SD)] in experimental aquaria from different treatments during feeding experiments. Kg corresponds to rate of change in POC concentration estimated in experimental aquaria during feeding experiments. PC_T, planktonic community treatment; BC_T, bacterial community treatment; and SFP_T, salmon food pellet treatment.
Supplementary Table 4 | ∂13C and ∂15N values [mean ± standard deviation (SD)] measured in Aulacomya atra tissues and in suspended particulate organic matter (SPOM) in the Puyuhuapi Fjord during the study period. For the location of the sampling station in the Puyuhuapi Fjord, refer to Figure 1.
Supplementary Table 5 | Possible contributions (%) of the three main carbon sources to the carbon isotopic signal of Aulacomya atra and suspended particulate organic matter (SPOM) estimated using the mixing model (Dauby, 1989; Fry, 2006). Three δ13C end-member obtained in this study were used in the mixing model: autochthonous marine end-member δ13C (−18.3), allochthonous terrestrial end-member δ13C (−29.9) and allochthonous pellet end-member δ13C (−21.9).
References
Anderson, S. R., Diou-Cass, Q. P., and Harvey, E. L. (2018). Short-term estimates of phytoplankton growth and mortality in a tidal estuary. Limnol. Oceanogr. 63, 2411–2422. doi: 10.1002/lno.10948
Anderson, S. R., and Harvey, E. L. (2019). Seasonal variability and drivers of microzooplankton grazing and phytoplankton growth in a subtropical estuary. Front. Mar. Sci. 6:174. doi: 10.3389/fmars.2019.00174
Andrade, C., Ríos, C., Gerdes, D., and Brey, T. (2016). Trophis structure of shallow-water benthic communities in the sub-Antartic Strait of Magellan. Polar Biol. 39, 2281–2297. doi: 10.1007/s00300-016-1895-0
Bayne, B. L. (1998). The physiology of suspension feeding by bivalve molluscs: an introduction to the Plymouth “TROPHEE” workshop. J. Exp. Mar. Biol. Ecol. 219, 1–19. doi: 10.1016/s0022-0981(97)00172-x
Betti, F., Bavestrello, G., Bo, M., Enrichetti, F., Loi, A., Wabderlingh, A., et al. (2017). Benthic biodiversity and ecological gradients in the Seno Magdalena (Puyuhuapi Fjord. Chile). Estuar. Coast. Shelf Sci. 198, 269–278. doi: 10.1016/j.ecss.2017.09.018
Buschman, A. H., Riquelme, V., Hernández-González, M. C., Varela, D., Jiménez, J. E., Henríquez, L. A., et al. (2006). A review of the impacts of salmonid farming on marine coastal ecosystems in the southeast Pacific. ICES J. Mar. Sci. 63, 1338–1345. doi: 10.1016/j.icesjms.2006.04.021
Chaigneau, A., and Pizarro, O. (2005). Mean surface circulation and mesoscale turbulent flow characteristics in the eastern South Pacific from satellite tracked drifters. J. Geophys. Res. 110:C05014. doi: 10.1029/2004JC002628
Cloern, J. E. (1982). Does the benthos control phytoplankton biomass in south san francisco bay? Mar. Ecol. Prog. Ser. 9, 191–202. doi: 10.3354/meps009191
Cranford, P. J. (2019). “Magnitude and Extent of waterclarification services provided by bivalvesuspension feeding,” in Goods and Services of Marine Bivalves, eds A. C. Smaal, J. G. Ferreira, J. Grant, J. K. Petersen, and Ø Strand (Cham: Springer), doi: 10.1007/978-3-319-96776-9_8
Cranford, P. J., Ward, J. E., and Shumway, S. E. (2011). “Bivalve filter feeding: variability and limits of the aquaculture biofilter,” in Shellfish Aquaculture and the Environment, 1st Edn, ed. S. E. Shumway (Hoboken, NJ: Wiley-Blackwell), 81–124. doi: 10.1002/9780470960967.ch4
Dauby, P. (1989). The stable isotope ratios in benthic food webs of the Gulf of Calvi, Corsica. Cont. Shelf Res. 9, 181–195. doi: 10.1016/0278-4343(89)90091-5
Dillwyn, L. W. (1817). A Descriptive Catalogue of Recent Shells, Arranged According to the Linnean Method, Whit Particular Attention to the Synonymy, 1:1–580, Vol. 2. London: John and Arthur Arch, 581–1092.
Dolmer, P. (2000). Feeding activity of mussels Mytilus edulis related to near-bed currents and phytoplankton biomass. J. Sea Res. 44, 221–231. doi: 10.1016/S1385-1101(00)00052-6
Filgueira, R., Strohmeier, T., and Strand, Ø (2019). “Regulating services of bivalve molluscs in the context of the carbon cycle and implications for ecosystem valuation,” in Goods and Services of Marine Bivalves, eds A. C. Smaal, J. G. Ferreira, J. Grant, J. K. Petersen, and Ø Strand (Cham: Springer), doi: 10.1007/978-3-319-96776-9_12
Frost, B. W. (1972). Effects of size and concentration of food particles on the feeding behaviour of the marine planktonic copepod Calanus pacificus. Limnol. Oceanogr. 17, 805–815. doi: 10.4319/lo.1972.17.6.0805
Gao, Q. F., Shin, P. K. S., Lin, G. H., Chen, S. P., and Cheung, S. G. (2006). Stable isotope and fattyacid evidence for uptake of organic waste by green-lipped mussels Pernaviridis in a polyculture fish farm system. Mar. Ecol. Prog. Ser. 317, 273–283. doi: 10.3354/meps317273
Goericke, R., and Fry, B. (1994). Variations of marine plankton δ13C with latitude, temperature, and dissolved CO2 in the world ocean. Glob. Biogeochem. Cycles 8, 85–90. doi: 10.1029/93gb03272
González, H. E., Nimptsch, J., Giesecke, R., and Silva, N. (2019). Organic matter distribution, composition and its possible fate in the Chilean North-Patagonian estuarine system. Sci. Total Environ. 657, 1419–1431. doi: 10.1016/j.scitotenv.2018.11.445
Greene, V. E., Sullivan, L. J., Thompson, J. K., and Kimmerer, W. J. (2011). Grazing impact of the invasive clam Corbula amurensis on the microplankton assemblage of the northern San Francisco Estuary. Mar. Ecol. Prog. Ser. 431, 183–193. doi: 10.3354/meps09099
Griffiths, C. L., and King, J. A. (1979). Some relationships between size, food availability andenergy balance in the ribbed mussel Aulacomyaater. Mar. Biol. 51, 141–149. doi: 10.1007/bf00555193
Handå, A., Min, H., Wang, X., Broch, O. J., Reitan, K. I., Reinertsen, H., et al. (2012a). Incorporation of fish feed and growth of blue mussels (Mytilus edulis) in close proximity to salmon (Salmo salar) aquaculture: Implications for integrated multi-trophic aquaculture in Norwegian coastal waters. Aquaculture 356–357, 328–341. doi: 10.1016/j.aquaculture.2012.04.048
Handå, A., Ranheim, A., Olsen, A. J., Altin, D., Reitan, K. I., Olsen, Y., et al. (2012b). Incorporation of salmon fish feed and feces components in mussel (Mytilus edulis): implications for integrated multi-trophic aquaculture in cool-temperate North Atlantic waters. Aquaculture 370–371, 40–53. doi: 10.1016/j.aquaculture.2012.09.030
Haure, J., Penisson, C., Bougrier, S., and Baud, J. P. (1998). Influence of temperature on clearance and oxygen consumption rates of the flat oyster Ostrea edulis: determination of allometric coefficients. Aquaculture 169, 211–224. doi: 10.1016/s0044-8486(98)00383-4
Iriarte, J. L., Pantoja, S., and Daneri, G. (2014). Oceanographic processes in Chilean fjords of Patagonia: from small to large-scale studies. Prog. Oceanogr. 129, 1–7. doi: 10.1016/j.pocean.2014.10.004
Jacobs, P., Riegman, R., and van der Meers, J. (2016). Impact of introduced juvenile mussel cultures onthe pelagic ecosystem of the western Wadden Sea, The Netherlands. Aquac. Environ. Interact. 8, 553–566. doi: 10.3354/aei00196
Jørgensen, C. B. (1990). Bivalve Filter Feeding: Hydrodynamics, Bioenergetics, Physiology and Ecology. Fredensborg: Olsen & Olsen, 140.
Kang, C. K., Choy, E. J., Hur, Y. B., and Myeong, J. I. (2016). Isotopic evidence of particle size-dependent food partitioning in cocultured sea squirt Halocynthia roretzi and Pacific oyster Crassostrea gigas. Aquat. Biol. 6, 289–302. doi: 10.3354/ab00126
Kreeger, D. A., Gatenby, C. M., and Bergstrom, P. W. (2018). Restoration potential of several native species of bivalve molluscs for water quality improvement in mid-Atlantic watersheds. J. Shellfish Res. 37, 1121–1157. doi: 10.2983/035.037.0524
Kreeger, D. A., and Newell, R. I. E. (1996). Ingestion and assimilation of carbon from cellulotyc bacteria and heterotrophic flagellates by the mussels Geukensia demisa and Mytilus edulis (Bivalvia, Mollusca). Aquat. Microb. Ecol. 11, 205–214. doi: 10.3354/ame011205
Kreeger, D. A., and Newell, R. I. E. (2000). “Trophic complexity between primary producers and invertebrate consumers in salt marshes,” in Concepts and Controversies in Tidal Marsh Ecology, eds M. P. Weinstein and D. A. Kreeger (New York, NY: Kluwer Press), 187–220. doi: 10.1007/0-306-47534-0_10
Lafon, A., Silva, N., and Vargas, C. A. (2014). Contribution of allochthonous organic carbon across the Serrano River Basin and the adjacent fjord system in southern Chilean Patagonia: insights from the combined use of stable isotopes and fatty acid biomarkers. Prog. Oceanogr. 129, 98–113. doi: 10.1016/j.pocean.2014.03.004
Lander, T., Barrington, K., Robinson, S., MacDonald, B., and Martin, J. (2004). Dynamics of the blue mussel as an extractive organism in an integrated multi-trophic aquaculture system. Bull. Aquac. Assoc. Can. 104, 19–28. doi: 10.1016/j.aquaculture.2012.09.029
Langdon, C. J., and Newell, R. I. E. (1990). Utilization of detritus and bacteria as food sources by two bivalve suspension-feeders, the oyster Crassotrea virginica and the mussel Geukensia demisa. Mar. Ecol. Prog. Ser. 58, 299–310. doi: 10.3354/meps058299
Langdon, C. J., and Newell, R. I. E. (1996). “Digestion and nutrition of larvae and adults,” in The Eastern Oyster Crassostrea virginica, eds V. S. Kennedy, R. I. E. Newell, and A. F. Eble (College Park, MD: Maryland Sea Grant College), 231–270.
Lefebvre, S., Barillé, L., and Clerc, M. (2000). Pacific oyster (Crassostrea gigas) feeding responses to a fish-farm effluent. Aquaculture 187, 185–198. doi: 10.1016/s0044-8486(99)00390-7
Linnaeus, C. (1758). Systema Naturae per Regna Tria Naturae, Secundum Classes, Ordines, Genera, Species, Cum Characteribus, Differentiis, Synonymis, Locis. Editio Decimal, Reformarta, 10th revised Edn, Vol. 1. 824.
Lonsdale, D. J., Cerrato, R. M., Holland, R., Mass, A., Holt, L., Schaffner, R. A., et al. (2009). Influence of suspension-feeding bivalves on the pelagic food webs of shallow, coastal embayments. Aquat. Biol. 6, 263–279. doi: 10.3354/ab00130
Lucas, J., Koester, I., Wichels, A., Niggemann, J., Dittmar, T., Callies, U., et al. (2016). Short-term dynamics of North Sea bacterioplankton-dissolved organic matter coherence on molecular level. Front. Microbiol. 7:321. doi: 10.3389/fmicb.2016.00321
MacDonald, B. A., Robinson, S. M. C., and Barrington, K. A. (2011). Feeding activity of mussels (Mytilus edulis) held in the field at an integrated multi-trophic aquaculture (IMTA) site (Salmo salar) and exposed to fish food in the laboratory. Aquaculture 314, 244–251. doi: 10.1016/j.aquaculture.2011.01.045
Mayr, C., Försterra, G., Häussermann, V., Wunderlich, A., Grau, J., Zieringer, M., et al. (2011). Stable isotope variability in a Chilean fjord food web: implications for N- and C-cycles. Mar. Ecol. Prog. Ser. 428, 89–104. doi: 10.3354/meps09015
Mazzola, A., and Sarà, G. (2001). The effect of fish farming organic waste on food availability for bivalve molluscs (Gaeta Gulf, Central Tyrrhenian, MED): stable carbon isotopic analysis. Aquaculture 192, 361–379. doi: 10.1016/s0044-8486(00)00463-4
Menden-Deuer, S., Lawrence, C., and Franzè, G. (2018). Herbivorous protist growth and grazing rates at in situ and artificially elevated temperatures during an Artic phytoplankton spring bloom. PeerJ 6:e5264. doi: 10.7717/peerj.5264
Minagawa, M., and Wada, E. (1984). Stepwise enrichment of 15N along food chains: further evidence and the relation between δ15N and animal age. Geochem. Cosmochim. Acta 48, 1135–1140. doi: 10.1016/0016-7037(84)90204-7
Molina, J. I. (1782). Saggio Sulla Storia Natural del Chile, del Signor Abate Giovanni Ignazio Molina. Bologna: Nella Stamperia di S. Tommaso d’Aquino, 202.
Montero, P., Daneri, G., González, H. E., Iriarte, J. L., Tapia, F., Lizárraga, L., et al. (2011). Seasonal variability of primary production in a fjord ecosystem of the Chilean Patagonia: implications for the transfer of carbon within pelagic food webs. Cont. Shelf Res. 31, 202–215. doi: 10.1016/j.csr.2010.09.003
Montero, P., Daneri, G., Tapia, F., Iriarte, J. L., and Crawford, D. (2017a). Diatom blooms and primary production in a channel ecosystem of central Patagonia. Lat. Am. J. Aquat. Res. 45, 999–1016. doi: 10.3856/vol45-issue5-fulltext-16
Montero, P., Pérez-Santos, I., Daneri, G., Gutiérrez, M. H., Igor, G., Seguel, R., et al. (2017b). A winter dinoflagellate bloom drives high rates of primary production in a Patagonian fjord ecosystem. Estuar. Coast. Shelf Sci. 199, 105–116. doi: 10.1016/j.ecss.2017.09.027
Norkko, A., Hewitt, J. E., and Thrush, S. F. (2001). Benthic-pelagic coupling and suspension-feeding bivalves: linking site-specific sediment flux and biodeposition to benthic community structure. Limnol. Oceanogr. 56, 2067–2072. doi: 10.4319/lo.2001.46.8.2067
Peharda, M., Richardson, C. A., Mladineo, I., Sestanovic, S., Popovic, Z., Bolotin, J., et al. (2007). Age, growth and population structure of Modiolus barbatus from the Adriatic. Mar. Biol. 151, 629–638. doi: 10.1007/s00227-006-0501-3
Post, D. M. (2002). Using stable isotopes to estimate trophic position: models, method, and assumptions. Ecology 83, 703–718. doi: 10.1890/0012-9658(2002)083[0703:usitet]2.0.co;2
Prins, T. C., Smaal, A. D., and Dame, R. F. (1998). A review of the feedbacks between bivalve grazing and ecosystem processes. Aquat. Ecol. 31, 349–358.
Quiñones, R. A., Fuentes, M., Montes, R. M., Soto, D., and León-Muñoz, J. (2019). Environmental issues in Chilean salmon farming: a review. Rev. Aquac. 11, 375–402. doi: 10.1111/raq.12337
Quiroga, E., Ortíz, P., González-Saldías, R., Reid, B., Tapia, F. J., Pérez-Santos, I., et al. (2016). Seasonal benthic patterns in a glacial Patagonian fjord: the role of suspended sediment and terrestrial organic matter. Mar. Ecol. Prog. Ser. 561, 31–50. doi: 10.3354/meps11903
Rau, G. H., Teyssie, J. L., Rassoulzadegan, F., and Fowler, S. W. (1990). 13C/12C and 15N/14N variations among size-fractionated marine particles: implications for their origin and trophic relationships. Mar. Ecol. Prog. Ser. 59, 33–38. doi: 10.3354/meps059033
Redmond, K. J., Magnesen, T., Hansen, P. K., Strand, Ø, and Meier, S. (2010). Stable isotopes and fatty acids as tracers of the assimilation of salmon fish feed inblue mussels (Mytilus edulis). Aquaculture 298, 202–210. doi: 10.1016/j.aquaculture.2009.10.002
Reid, G. K., Liutkus, M., Bennett, A., Robinson, S. M. C., MacDonald, B., and Page, F. (2010). Absorption efficiency of blue mussels (Mytilus edulis and M. trossulus) feeding on Atlantic salmon (Salmo salar) feed and fecal particulates: implications for integrated multi-trophic aquaculture. Aquaculture 299, 165–169. doi: 10.1016/j.aquaculture.2009.12.002
Ribes, M., Coma, R., and Gili, J. M. (1998). Seasonal variations of in situ feeding rates by the temperate ascidian Halocynthia papillosa. Mar. Ecol. Progr. Ser. 175, 201–213.
Riisgård, H. U. (1988). Efficiency of particle retention and filtration rate in 6 species of northeast American bivalves. Mar. Ecol. Prog. Ser. 45, 217–223. doi: 10.3354/meps045217
Saiz, E. (1993). Sources of variability in zooplankton feeding experiments: the importance of accurate determination of algal growth rates. Sci. Mar. 57, 23–29.
Sanz-Lazaro, C., and Sanchez-Jerez, P. (2017). Mussels do not directly assimilate fish farm wastes: shifting the rationales of integrated multitrophic aquaculture to a broader scale. J. Environ. Manag. 201, 82–88. doi: 10.1016/j.jenvman.2017.06.029
Sarà, G., Scilipoti, D., Mazzola, A., and Modica, A. (2004). Effects of fish farming waste to sedimentary and particulate organic matter in a southern Mediterranean area (Gulf of Castellammare, Sicily): a multiple stable isotope study (d13C and d15N). Aquaculture 234, 199–213. doi: 10.1016/j.aquaculture.2003.11.020
Sarà, G., Zenone, A., and Tomasello, A. (2009). Growth of Mytilus galloprovincialis (mollusca, bivalvia) close to fish farms: a case of integrated multi-trophic aquaculture within the Tyrrhenian Sea. Hydrobiologia 636, 129–136. doi: 10.1007/s10750-009-9942-2
Schneider, W., Pérez-Santos, I., Ross, L., Bravo, L., Seguel, R., and Hernández, F. (2014). On the hydrography of Puyuhuapi Channel (Chilean Patagonia). Prog. Oceanogr. 129, 8–18. doi: 10.1016/j.pocean.2014.03.007
Sebens, K., Sarà, G., and Nishizaki, M. (2016). “Energetics, particle capture, and growth dynamics of benthic suspension feeders,” in Marine Animal Forest, ed. S. Rossi (Cham: Springer), doi: 10.1007/978-3-319-17001-5_17-1
Sepúlveda, J., Pantoja, S., and Hughen, K. A. (2011). Sources and distribution of organic matter in northern Patagonia fjords, Chile (∼44–47°S): a multi-tracer approach for carbon cycling assessment. Continent. Shelf Res. 31, 315–329. doi: 10.1016/j.csr.2010.05.013
Silva, N. (2008). “Dissolved oxygen, pH, and nutrients in the austral Chilean channels and fjords,” in Progress in the Oceanographic Knowledge of Chilean Interior Waters, from Puerto Montt to Cape Horn, eds N. Silva and S. Palma (Valparaíso: Pontificia Universidad Católica de Valparaíso), 37–43.
Stoecker, D. K., Nejstgaard, J. C., Madhusoodhanan, R., Pohnert, G., Wolfram, S., Jakobsen, H. H., et al. (2015). Understimation of microzooplankton grazing in dilution experiments due to inhibition of phytoplankton growth. Limnol. Oceanogr. 60, 1426–1438. doi: 10.1002/lno.10106
Stoecker, D. K., Weigel, A., and Goes, J. I. (2014). Microzooplankton grazing in the Eastern Bering in summer. Deep Sea Res. II 109, 145–156. doi: 10.1016/j.dsr2.2013.09.017
Strom, S. L., and Fredrickson, K. A. (2008). Intense stratification leads to phytoplankton nutrient limitation and reduced microzooplankton grazing in the southeastern Bering Sea. Deep Sea Res. II 55, 1761–1774. doi: 10.1016/j.dsr2.2008.04.008
Stuart, V., Field, J. G., and Newell, R. C. (1982). Evidence for absorption of kelp detritus by the ribbed mussel Aulacomya ater using a new 51Cr-labelled microsphere technique. Mar. Ecol. Prog. Ser. 9, 263–271. doi: 10.3354/meps009263
Stuart, V., and Klumpp, D. W. (1984). Evidence for food-resource partitioning by kelp-bed filter feeders. Mar. Ecol. Prog. Ser. 16, 27–37. doi: 10.3354/meps016027
Sylvester, F., Dorado, J., Boltovskoy, D., Juarez, A., and Cataldo, D. (2005). Filtration rates of the invasive pest bivalve Limnoperna fortunei as a function of size and temperature. Hydrobiologia 534, 71–80. doi: 10.1007/s10750-004-1322-3
Valiela, I. (1995). Marine Ecological Processes, 2nd Edn. New York, NY: Springer-Verlag, 696. doi: 10.1007/978-1-4757-4125-4
Vargas, C. A., Martínez, R. A., San Martin, V., Aguayo, M., Silva, N., and Torres, T. (2011). Allochthonous subsidies of organic matter across a lake–river–fjord landscape in the Chilean Patagonia: Implications for marine zooplankton in inner fjord areas. Cont. Shelf Res. 31, 187–201. doi: 10.1016/j.csr.2010.06.016
Vaughn, C. C., and Hoellein, T. J. (2018). Bivalve impacts in freshwater and marine ecosystems. Ann. Rev. Ecol. Evol. Syst. 49, 183–208. doi: 10.1146/annurev-ecolsys-110617-062703
Voudanta, E., Kormas, K. A., Monchy, S., Delegrange, A., Vincent, D., Genitsaris, S., et al. (2016). Mussel biofiltration effects on attached bacteria and unicellular eukaryotes in fish-rearing seawater. PeerJ 4:e1829. doi: 10.7717/peerj.1829
Wada, E., Mizutani, H., and Minagawa, M. (1991). The use of stable isotopes for food web analysis. Crit. Rev. Food Sci. Nutr. 30, 361–371. doi: 10.1080/10408399109527547
Wada, E., Minagawa, M., Mizutani, H., Tsuji, T., Imaizum, R., and Karasawa, K. (1987). Biogeochemical studies on the transport of organic matter along the Otsuchi River watershed, Japan. doi: 10.1016/0272-7714(87)90075-8
Winter, J. E. (1978). A review on the knowledge of suspension-feeding in lamellibranchiate bivalves, with special reference to artificial aquaculture systems. Aquaculture 13, 1–33. doi: 10.1016/0044-8486(78)90124-2
Wright, R. T., Coffin, R. B., Ersing, C. P., and Pearson, D. (1982). Field and laboratory measurements of bivalve filtration of natural marine bacterioplankton. Limnol. Oceanogr. 27, 91–98. doi: 10.4319/lo.1982.27.1.0091
Keywords: Aulacomya atra, ingestion rate, stable isotopes, autochthonous and allochthonous food, Puyuhuapi Fjord
Citation: Montero P, Coppari M, Betti F, Bavestrello G and Daneri G (2021) Feeding of Aulacomya atra Under Different Organic Matter Sources (Autochthonous and Allochthonous) in a Chilean Patagonia Fjord Ecosystem. Front. Mar. Sci. 8:612406. doi: 10.3389/fmars.2021.612406
Received: 30 September 2020; Accepted: 30 June 2021;
Published: 29 July 2021.
Edited by:
Angel Borja, Technological Center Expert in Marine and Food Innovation (AZTI), SpainReviewed by:
José Lino Vieira De Oliveira Costa, University of Lisbon, PortugalUrsula Felicitas Marianne Witte, University of Aberdeen, United Kingdom
Copyright © 2021 Montero, Coppari, Betti, Bavestrello and Daneri. This is an open-access article distributed under the terms of the Creative Commons Attribution License (CC BY). The use, distribution or reproduction in other forums is permitted, provided the original author(s) and the copyright owner(s) are credited and that the original publication in this journal is cited, in accordance with accepted academic practice. No use, distribution or reproduction is permitted which does not comply with these terms.
*Correspondence: Giovanni Daneri, gdaneri@ciep.cl