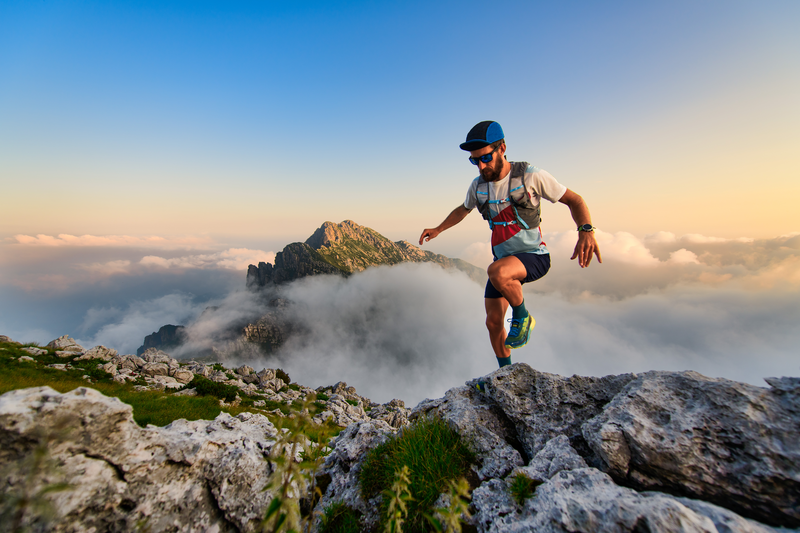
95% of researchers rate our articles as excellent or good
Learn more about the work of our research integrity team to safeguard the quality of each article we publish.
Find out more
ORIGINAL RESEARCH article
Front. Mar. Sci. , 23 February 2021
Sec. Marine Ecosystem Ecology
Volume 8 - 2021 | https://doi.org/10.3389/fmars.2021.612195
This article is part of the Research Topic Oceanography and Benthic Ecology of Patagonian fjords - 500 years from the Discovery of the Strait Magellan View all 16 articles
The Chilean fjord region includes many remote and poorly known areas where management plans for the marine living resources and conservation strategies are urgently needed. Few data are available about the spatial distribution of its marine invertebrate fauna, prevalently influenced by complex interactions between biotic and abiotic factors, animal behavior and human activities. Patagonian fjords are a hotspot for finfish aquaculture, elevating Chile to the world’s second producer of farmed salmon, after Norway, a condition that emphasizes the necessity to develop strategies for a sustainable aquaculture management. The present study focuses on the emblematic cold-water coral Desmophyllum dianthus, dwelling the Comau Fjord from shallow to deep waters, with the aim to illustrate population structure, demography and adaptation of the species and its potential use for the development of a sustainable conservation and management plan for human activities. The analyses of microsatellite loci of D. dianthus individuals from four sampling localities along horizontal and vertical gradients of Comau Fjord, lead to identify them as a panmictic population. The results also contributed to consider a careful examination of the synchrony between the temporal and spatial variations of environmental factors and the biological cycle of the species as key role player in the inference of autecology of the species. The discussion stresses the importance of molecular analyses as extremely helpful tools for studies focusing on remote areas and non-model organisms, where logistic difficulties and limited scientific knowledge hamper a better management and conservation of marine resources, and in particular the relevance of multidisciplinary approaches to reduce the extensive knowledge gap on the remote fjord ecosystems of Patagonia. This study also highlights the importance of oceanographic information in the entire process of the analyses and interpretation of genetic results.
Marine biodiversity and unsustainable maritime activities cannot stand up together. Efficient management plans are urgently needed. Patagonian fjords are not only a hotspot of marine biodiversity, but also for finfish aquaculture, elevating Chile to the world’s second producer of farmed salmon, after Norway (Bjørndal, 2002; FAO, 2020), a further condition that emphasizes the necessity to develop strategies for a sustainable aquaculture management. Due to its remoteness and enormous coastline, the Chilean fjord region includes many poorly known areas. Only few data are available about the spatial distribution of its marine invertebrate fauna, prevalently influenced by complex interactions between biotic and abiotic factors (Häussermann and Försterra, 2009).
Comau Fjord, located in the northern Patagonia of Chile, is one the most exploited fjords along the Patagonian coast in term of maritime activities, which are mostly characterized by artisanal fishing (18%) and aquaculture (60%), which is a large industry in Chile (Fillinger and Richter, 2013; Soto et al., 2019). An unexpected deep-water coral diversity and abundance was also described from off the Chilean coast and Central Chilean Patagonia (Bravo et al., 2005; Häussermann and Försterra, 2007, 2014; Cañete and Häussermann, 2012). Many large and dense assemblages of Cold-Water Coral (CWC) communities were recorded in shallow water of Patagonian fjords (Försterra and Häussermann, 2003; Försterra et al., 2005; Häussermann and Försterra, 2007, 2014). Among them there is the emblematic cold-water coral Desmophyllum dianthus, dwelling Chilean Patagonian coast and specifically the Comau Fjord from shallow to deep waters. With aquaculture and artisanal fisheries growing exponentially (see Soto et al., 2019), the Comau Fjord changed from near pristine in 2003 to heavily impacted in 2013, where abundances of benthic communities were reduced down to 25%. Similarly, several species have declined from common to very rare within the fjord (Häussermann et al., 2013). The productivity was reported to have doubled in 20 years (Mayr et al., 2014). In 2012, 99% of the D. dianthus specimens died along at least 15 km of the coast, probably after a large algal bloom in combination with exceptionally high efflux of harmful substances (methane and sulfide) enriched water from the cold vents due to increased salmon farming activity and volcanic activities, respectively (Försterra et al., 2014).
Strategies of marine conservation have been (and still are) based on qualitative and quantitative data describing the status of marine ecosystems, marine biodiversity, pressures and impacts of anthropogenic activities. Among all this environmental indicators, there was no room for genetic data, which on the contrary have been mostly used for taxonomic, phylogenetic and evolutionary studies in the lasts decades. However, molecular tools can be extremely helpful to determine structure, demography and environmental adaptation of marine organisms, also providing evidence especially in studies focused on remote areas and non-model organisms, where logistic difficulties and limited scientific knowledge hamper a better management and conservation of marine resources. This type of knowledge represents the baseline information necessary for the development of an effective marine conservation strategy and management plan for sustainable maritime activities.
Since not many data on the population structure of marine organisms on the Chilean fjords are available whether along horizontal or vertical distribution (see Fillinger and Richter, 2013) we hypothesize that, not excluding a horizontal gradient along the fjord (head-mouth), the steep oceanographic gradients (salinity and temperature) might have shaped the structure of its benthic communities, generating a benthic vertical zonation within the fjord.
The present study aims to assess the structure of Desmophyllum dianthus populations of the Comau Fjord, analyzing the genetic profile with microsatellite loci and the environmental condition of the fjord. The application of a multidisciplinary approach highlights the importance of oceanographic information in the entire process of the analyses and interpretation of genetic results, reducing the knowledge gap on the marine ecosystems in Patagonian fjords.
Comau Fjord is located in Northern Patagonia, Chile (42°15′ S, 72°29′ W). It extends approx. 35 km along a north-south axis and possesses two lateral fjords, Quintupeu and Cahuelmó. At Lilihuapi Island, the Comau mouth faces west toward the Interior Sea of Chiloé. The fjord presents no sill and is characterized by a typical “U”-shape with very steep slopes, reaching a maximum depth of 497 m close to its mouth. The main freshwater inputs occur at the fjord head, where two rivers bring in average ∼6,000 mm per year of rainwater (Hromic, 2009) and tides reach up to 7.5 m (Försterra, 2009; Sobarzo, 2009). The hydrogeomorphologic features of the fjord shape an accentuated vertical variability (Figure 1B).
Figure 1. Location and information on coral samples collected in Comau Fjord (A): (1) Lilihuapi Island (ILC, 42°09′ S 72°35′ W), −20 m; (2) Punta Huinay (PHC, 42°22′ S 72°25′ W), −20 m; (3) Cross Huinay (XHC, 42°23′ 55″ S, 72°27′ 18″W), −20 m; (4) Punta Gruesa (PGC_D, 42°24′ S 72°25′ W), −250 m, D, Deep indicated in red. Simplified scheme of water layers and movement of the Comau Fjord (B). A, surface freshwater layer; B, brackish water; and C, deep saline water; N, north S, south (Google Earth, Image © 2021 CNES/Airbus, Landsat/Copernicus, Maxar Technologies).
There are three main layers within Comau Fjord according to the vertical oceanographic gradients: (a) surface freshwater (0–10 m depth) that is under the direct influence of climatic events (e.g., rain and wind), and presents a strong seasonality with salinity (2-20 PSU, Practical Salinity Unit) and temperature (5–20°C), varying largely during the year; (b) a layer of brackish water (20–30 PSU) between 5 and 20 m depth that presents a sharp pycnocline mainly caused by salinity changes (halocline), except during summer months when temperature changes (from 18 to 12°C), also contributing to the stratification of the water column; (c) deep saline water (below 20 m depth), with salinity >32 (PSU) and a constant temperature (∼11°C) (Sobarzo, 2009; Sánchez et al., 2011; Fillinger and Richter, 2013). The freshwater inputs enhance the stratification of the water column within Comau, which creates a layer system with a different horizontal advection for each layer (Silva et al., 1997; Zhou et al., 2005), known as estuarine circulation. Similarly, water mass exchange with the Interior Sea of Chiloé generates a horizontal gradient across the head-mouth axis of the fjord (Figure 1B; Häussermann and Försterra, 2009). These changes are most likely related to the phase of the tide. The temperature also varies along the year and moreover toward the head of the fjord, where it is increasing during some months (e.g., 0.5°C in September), decreasing in others (e.g., 0.7°C in May), or remaining stable in other months (e.g., 0.1°C in December). Important seasonal changes in salinity and temperature also occur vertically in the first 50 m of the water column, while below 50–60 m the changes are negligible (Sánchez et al., 2011; Fillinger and Richter, 2013; Höfer et al., unpublished data). Mixing water events are relevant as well. Their effects depend on wind intensity, direction, and duration of blowing under specific conditions. In the case of Huinay (i.e., sampling location no. 2, Figure 1A), due to the configuration of the fjord, and the presence of huge tides that produce strong currents throughout the entire water column, winds from the N or NE in combination with strong tide currents can mix the waters down to the first 100 m of the water column, introducing and removing a large amount of water from the fjord and making sessile organisms pass from one layer to another depending on the high or low tide.
Due to the large abundance of living coral aggregations below 20 m water depths (Försterra et al., 2005), the sampling was planned to encompass the main oceanographic gradients present in the Comau Fjord: salinity (halocline, vertical, and horizontal gradient), temperature (thermocline, vertical gradient) as well as water mass exchange along a head-mouth axis. Four sampling localities across the mouth-head axis of the fjord were selected and samples were collected at different depths: Isla Lilihuapi (ILC, 42°09′ S 72°35′ W), −20 m, 25 PSU, 18°C–36 individuals; Punta Huinay (PHC, 42°22′ S 72°25′ W), −20 m, 25 PSU, 12°C–29 individuals; Cross Huinay (XHC, 42°23′ 55″ S, 72°27′ 18″W), −20 m, 25 PSU, 12°C–23 individuals; Punta Gruesa (PGC_D, 42°24′ S 72°25′ W), −250 m–37 individuals (Figure 1).
Coral tissue from 125 specimens taxonomically identified as D. dianthus was sampled and preserved in absolute ethanol. Additional samples of D. dianthus from the Mediterranean Sea (Adriatic Sea, 41°30’ N 17°17’ E, 26 specimens) were incorporated to the Comau dataset as a sort of outgroup to support the informative capacity of microsatellites analysis.
Following the procedure described in Addamo et al. (2020), total genomic DNA was extracted from the mesenteric tissue using the QIAGEN BioSprint 15 DNA Blood Kit (Qiagen Iberia S.L., Madrid), with slight modifications, including the optional RNase treatment and an extended period of proteinase K lysis (overnight incubation at 55°C). DNA concentration was quantified using the Qubit 2.0 Fluorometer, and diluted to a final concentration of 2 ng/μl. Thirty-one microsatellite loci developed for D. dianthus (25 markers from Addamo et al., 2015a; six markers from Miller and Gunasekera, 2017) were organized in 1 tetraplex, 7 triplex, and 3 duplex by Multiplex Manager 1.0 (Holloley and Geerts, 2009) and analyzed in each sample. Multiplex PCRs were performed using 1X Qiagen Multiplex PCR Master Mix (Qiagen, Hilden, Germany), and following the PCR conditions described in Addamo et al. (2015a). Fluorescently labeled PCR products were run on an ABI PRISM 3730 DNA Sequencer (Applied Biosystems), scored using the GeneScan-500 (LIZ) size standard, and analyzed with the GeneMapper software (Applied Biosystems). Estimates of null allele frequency, error scoring, and large allele dropout were calculated with the Brookfield-1 method (Brookfield, 1996) using Micro-Checker (Van Oosterhout et al., 2004) and FreeNa (Chapuis and Estoup, 2007). Due to possible asexual reproduction of colonial and free-living corals (e.g., via budding, transverse division) (Tokuda et al., 2017), which includes D. dianthus (Miller and Gunasekera, 2017), individuals with identical multilocus genotype were identified using the index of probability of identity (PI the probability of two individuals sharing the same genotype) calculated using GenAlEx 6.5 (Peakall and Smouse, 2012). Departures from Hardy-Weinberg Equilibrium (HWE) and genotypic linkage disequilibrium (LD) were tested using Genepop on the web version 4.7 (Raymond and Rousset, 1995; Rousset, 2008) and GenAlEx 6.5 (Peakall and Smouse, 2012). Sequential Holm-Bonferroni correction (Holm, 1979) was applied to the multiple tests. Basic information on genetic variability and diversity within and among sampling localities was estimated as allele frequency and richness, heterozygosity (Ho, He) and fixation index (FIS) (Table 1). Computations were made using GenAlEx 6.5 and Genepop on the web version 4.7.
Table 1. Genetic variability and diversity by sampling locations of Desmophyllum dianthus in Comau Fjord.
To investigate population structure, the number of genetic clusters (K) from multilocus genotype data was inferred with a Bayesian model-based approach implemented in Structure v2.3.4 (Falush et al., 2003). Bayesian analyses of genetic admixture model, including the information of sampling localities (LOCPRIOR) were run with settings including 50,000 MCMC interactions after a burn-in of 10,000 iterations. Ten independent chains were run to test each value of K from 1 to 5. The results from Structure were then processed in Structure Harvester (Earl and vonHoldt, 2012), Structure Selector (Li and Liu, 2018), and CLUMPAK (Kopelman et al., 2015) to detect the best-fit number of genetic clusters representing the genetic discontinuity of the data. The highest mean lnPr(X| K) (Pritchard et al., 2000), the ΔK (Evanno et al., 2005), and MedMeaK, MaxMeaK, MedMedK, MaxMedK (Puechmaille, 2016) were all considered to identify and evaluate the optimum value of K. Each cluster identified in the initial Structure run was analyzed separately using the same settings to identify potential within-cluster structure (Evanno et al., 2005). Individual/population assignment and genetic differentiation among clusters suggested by Structure, were calculated using analyses of molecular variance (AMOVA) implemented in GeneClass2 (Piry et al., 2004), Arlequin and GenAlEx 6.5, respectively. Samples were subjected to spatial genetic analysis using principal coordinate analysis (PCoA) and Isolation-by-distance Mantel test implemented in GenAlEx 6.5 and Genepop on the web version 4.7. The regression of linearized FST [i.e., FST/(1- FST)] vs. marine geographic distance (km) was performed to assess the correlation between genetic and geographic distances. Marine geographic distances between localities were calculated using Google Earth (Google Inc, 2009) and considering the most direct marine route.
Population demography analysis was computed using Bottleneck (Cornuet and Luikart, 1997) to test a recent effective population size reduction from allele data frequencies. Detection of first-generation migrants was determined with GeneClass2 (Piry et al., 2004), setting the frequency-based method with Monte-Carlo resampling, minimum number of 10,000 simulated individuals, and 0.01 for Type I error (alpha) value.
After initial data check (i.e., detection and elimination of loci/sample with null allele and with 10% of missing data), the final genotype dataset includes 23 microsatellites and 118 individuals [including Mediterranean population (A)], or 99 individuals [excl. Mediterranean population (B)] (Addamo et al., 2021). Further analyses with Micro-Checker, FreeNa and GenePop confirm the absence of null alleles and linkage disequilibrium between loci in the reduced dataset (Supplementary Table 1). All loci were polymorphic and by locality, their allelic richness was maximum up to 34 (e.g., locus C6 in PGC_D) alleles, while the observed heterozygosity (Ho) was lowest in PGC_D (0.56 ± 0.05) and largest in PHC (0.60 ± 0.06) (Table 1). Analyses of populations structure indicated two main genetic clusters of D. dianthus (K = 2), corresponding to population from in the Mediterranean Sea, on one side, and the four Chilean sampling localities, on the other (Figure 2A). Instead, further genetic structuring was not detected between Chilean localities, which were clearly identified in a unique genetic cluster (Figure 2B). The optimal values of genetic clusters K representing the genetic discontinuity among D. dianthus individuals from the Mediterranean Sea and Chile, and the clear genetic homogeneity of the four Chilean localities in the Comau Fjord were identified by the three approaches: highest mean lnPr(X| K) (Pritchard et al., 2000), ΔK (Evanno et al., 2005), and MedMeaK, MaxMeaK, MedMedK, MaxMedK (Puechmaille, 2016; Supplementary Figure 1).
Figure 2. Proportional membership of Desmophyllum dianthus individuals from sequential cluster analyses using STRUCTURE SELECTOR and CLUMPAK, including (A) or excluding (B) individuals from Mediterranean Sea (MED). The clusters (K = 1–5) are shown with the vertical bars representing each individual broken into colored segments based on the proportion of the genome estimated to have originated from each cluster. For complete locality names (see Figure 1). MED stands for Mediterranean Sea, and C_ for Chile.
In concordance with the results on population differentiation, the genetic distance computed through the analysis of principal coordinates (PCoA) and the population assignment test among individuals estimated full self-population attribution (100%) for individuals from Mediterranean Sea, while most of the individuals from Chilean sites were assigned to sampling localities from Comau Fjord (84%) and only minor percentage (16%) to self-sampling localities attribution (Figure 3). The molecular variance (AMOVA) also indicated appreciable differences only among Mediterranean and Chilean populations group pairs (total FST = 0.23, p-value = 0.000), supporting the hypothesis of two different regions with an observed variation of 24% between both regions (Supplementary Figure 2).
Figure 3. Population assignment (A,B) and genetic distance (C,D) among D. dianthus individuals from each sampling locality, including (A,C) or excluding (B,D) individuals from Mediterranean Sea (MED). For complete locality names (see Figure 1). MED, Mediterranean.
Positive and highly significant genetic-spatial correlation was also detected from the Mantel test between Mediterranean Sea and Chile (p-value = 9.26E–15). Indeed, pairwise genetic distances between original localities increased significantly with geographic distances, showing a pattern of isolation-by-distance (IBD) on the large spatial scale (Supplementary Figure 3A), as also indicated by a previous study (Addamo et al., 2020). Localities appeared clustered in two major genetic divergence groups: one cluster includes specimens from the Mediterranean Sea (FST ≈ 0.19, marine distance 20,000 km), a second cluster that represents individuals from Chile (FST ≈ 0.01, marine distance range 2–20 km). In contrast, no significant genetic-distance correlation was detected from the Mantel test for localities within Comau Fjord (p-value = 0.826) (Supplementary Figure 3B). The mean number of migrants (Nm) varied considerably whether including Mediterranean population (A) (3.3 ± 0.48) or not (B) (17.6 ± 1.35) (Table 1). Further tests on first generation migrant detection (short-term) revealed no migrants between Mediterranean Sea and Chile, and a significant (p = value < 0.01) exchanging volume of individuals among localities within Comau Fjord. All these results furtherly confirmed cluster K = 1 as the most probable population structure for individuals from Comau Fjord. Demographically, all populations presented a normal L-shape distribution of alleles frequency, having not experienced any bottleneck event (i.e., severe reduction in population size) in recent times.
This study aimed to identify the genetic structure of D. dianthus populations in Comau Fjord, evaluating if the currents, water column stratification and the layer system characterizing the waters of the fjord hinder the gene flow along the fjord (head-mouth) or its vertical axis (shallow-deep). As mentioned earlier in the manuscript, the study included samples from the Mediterranean Sea into the Comau dataset as a sort of outgroup to support the informative capacity of microsatellites analysis. The genetic difference between individuals from Mediterranean Sea and Chile and the potential species misclassification have been discussed in previous studies, demonstrating the that they belong to the same species D. dianthus either by genetic, morphological or histological characters (Addamo et al., 2015b, 2016, 2020), confirming the widely spread distribution that denotes the species.
Although previous studies showed vertical and horizontal zonation for foraminifera communities (Hromic, 2009) within Comau Fjord, our results provide evidence of a panmictic population of D. dianthus within it, both along a horizontal (head-mouth) and a vertical (shallow-deep) axis. Such panmixia may be the consequence of temporal or spatial changes in the physical structure of the water column. For example, in this region the freshwater inputs toward the sea peak from June to August and then steadily decrease until March, displaying a seasonality that is going to increase due to climate change (Aguayo et al., 2019). Similarly, along the fjord the stratification caused by salinity is more intense near its head or the Cahuelmó lateral fjord (Schwabe et al., 2006), where most of the freshwater inputs arrive to the sea. Finally, surface winds and massive tides, up to 7.5 m (Försterra, 2009; Sobarzo, 2009), are able to mix the waters within Comau Fjord, weakening the water column stratification and even temporally breaking up its layer system. The strong currents throughout the entire column of water, produced by huge tides, surely favors the exchange with the Chiloe Sea and the movement of larvae and gametes along the fjord. Indeed, a combination of all these sources of spatial and temporal homogeneity in the physical structure may promote gene flow in Comau, maintaining a panmictic population spread across different locations and depths within the fjord.
The strong seasonality of the fjord system influences the highly seasonal reproduction of D. dianthus, which exhibits broadcast spawning around August, with the gamete production beginning in September (Feehan et al., 2019), when the freshwater inputs start declining and the spring tides are higher due to the proximity to the spring equinox. This situation enhances water column mixing around D. dianthus reproductive season, which in turn would increase the exchange of propagules and larvae across the fjord as supported by genetic results. All the physical processes aforementioned may be enough to promote the genetic exchange, suggesting that haloclines may not be such a strong barrier for the dispersal of D. dianthus propagules.
The fact that the population of D. dianthus in Comau Fjord extents along steep oceanographic gradients, both horizontally and with depth, resulting tolerant to a wide range of oceanographic conditions, suggests that larval dispersal along these axes is fast enough to maintain the population connected. However, it remains unknown if there is reproduction success along the entire distribution range or if we are dealing with a source/sink situation. If the latter is the case, it is important to assess which portion of the population represents the source and which the sink. In 2012 a mayor mortality of D. dianthus occurred in a larger portion of the Comau Fjord with large areas showing a mortality rate close to 100% (Försterra et al., 2014). The recovery and recruitment patterns observed thereafter may help to reveal the sources and sinks of larvae, and thus mapping the gene flow within the fjord. Considering the increase in farming activities also planned in other areas of Chilean Patagonia, and their potential impact on marine biodiversity, further studies would need to reveal if for example the corals from other biogeographic areas, such as Central Patagonia, belong to the same population. This information is pivotal for managing efficiently the maritime activities and using sustainably the marine resources. The 2012 mortality event (Försterra et al., 2014) shows that despite the resilience to fluctuations in certain oceanographic conditions D. dianthus may be sensitive to other key parameters or that the fluctuations may occasionally exceed the ecophysiological limits of the species. This and gene flow direction are key questions that need to be addressed to install efficient conservation measures for these unique cold water coral populations. The genetic results rejected the initial hypotheses of a clear populations′ structure within the fjord, which is characterized by the vertical stratification of its water column. A carefully analysis of the biology of the species combined with the environmental and oceanographic condition, including seasonality and occasional variations that occur specifically in Comau Fjord, would help to understand why the genetic structure of D. dianthus population differs from other organisms, e.g., foraminifera communities (Hromic, 2009). These results highlight the importance of including detailed oceanographic information (and data based on real-time observations, when available) in the entire process of the analyses and interpretation of genetic results as extremely helpful tools for studies focusing on remote areas and non-model organisms, where logistic difficulties and limited scientific knowledge hamper a better management and conservation of marine resources.
The present study stresses the importance of multidisciplinary approach to reduce the extensive knowledge gap on the marine ecosystems in Patagonian fjords. The multidisciplinary approach, including a detailed analyses of the oceanographic characteristics of the area, molecular studies and analyses of the reproduction of the species, allowed a better understanding on the biophysical processes driving the genetic diversity of the coral population in the Comau Fjord. Although the present study establishes a useful knowledge baseline to provide guidance for ecosystem-based management and conservation, the ecological analyses and conservation implication in the Chilean Patagonia still suffer from important knowledge gaps and limitations of interdisciplinary and methodological approaches. Indeed, further studies should be carried out including other areas where smaller populations of corals have been described (Försterra et al., 2017): e.g., Reñihue Fjord, Pitipalena Fjord or the area of the mouth of the Messier Channel (48°S). In addition, other marine organisms and real-time oceanographic observations should be included to better understand the biological and ecological characteristic of marine ecosystems in Patagonia.
The original contributions presented in the study are included in the article/Supplementary Material and Zenodo 10.5281/zenodo.4435966, further inquiries can be directed to the corresponding author/s.
This work was realized under the collaboration of all authors. AA, GF, and AM collected the samples. AA and RG-J carried out the molecular analyses and post-processing of the genotyping. AA and SZ performed the population genetic analyses. AA, SZ, GF, JH, RG-J, GC, and AM participated in the discussion of the results and in the writing of the manuscript. All authors approved the final version. The views expressed are purely those of the writers and may not in any circumstance be regarded as stating an official position of the European Commission.
This work was funded by the Spanish Ministry of Science and Innovation (CGL2011−23306), Fondecyt (Projects 1150843 and 1201717), and the Fundación San Ignacio del Huinay-Endesa & CSIC Projects 2011 and 2013.
The authors declare that the research was conducted in the absence of any commercial or financial relationships that could be construed as a potential conflict of interest.
We would like to thank the Huinay staff for the logistical support, Jurgen Laudien (AWI) for coral samples from Lilihuapi Island, V. López Márquez (MNCN) for the collaboration in the laboratory. This was publication number 184 of Huinay Scientific Field Station. This manuscript has been finalized at the time of the COVID-19 pandemic.
The Supplementary Material for this article can be found online at: https://www.frontiersin.org/articles/10.3389/fmars.2021.612195/full#supplementary-material
Addamo, A. M., Zaccara, S., Försterra, G., Höfer, J., García-Jiménez, R., Crosa, G., et al. (2021). Data from: Microsatellites of Desmophyllum dianthus. Comau Fjord: Zenodo. doi: 10.5281/zenodo.4435966
Addamo, A. M., García-Jiménez, R., Taviani, M., and Machordom, A. (2015a). Development of microsatellite markers in the deep-sea cup coral Desmophyllum dianthus by 454 sequencing and cross-species amplifications in Scleractinia order. J. Hered. 106, 322–330. doi: 10.1093/jhered/esv010
Addamo, A. M., Martínez-Baraldés, I., Vertino, A., López-González, P. J., Taviani, M., and Machordom, A. (2015b). Morphological polymorphism of Desmophyllum dianthus (Anthozoa: Hexacorallia) over a wide ecological and biogeographic range: stability in deep habitats? Zool. Anz. 259, 113–130. doi: 10.1016/j.jcz.2015.10.004
Addamo, A. M., Miller, K. J., Häussermann, V., Taviani, M., and Machordom, A. (2020). Global-scale genetic structure of a cosmopolitan cold-water coral species. Mar. Freshwater Ecosyst. 31, 1–14. doi: 10.1002/aqc.3421
Addamo, A. M., Vertino, A., Stolarski, J., García-Jiménez, R., Taviani, M., and Machordom, A. (2016). Merging scleractinian genera: the overwhelming genetic similarity between solitary Desmophyllum and colonial Lophelia. BMC Evol. Biol. 16:108. doi: 10.1186/s12862-016-0654-8
Aguayo, R., León-Muñoz, J., Vargas-Baecheler, J., Montecinos, A., Garreaud, R., Urbina, M., et al. (2019). The glass half-empty: climate change drives lower freshwater input in the coastal system of the Chilean Northern Patagonia. Clim. Change 155, 417–435. doi: 10.1007/s10584-019-02495-6
Bjørndal, T. (2002). The competitiveness of the Chilean salmon aquaculture industry. Aquac. Econ. Manag. 6, 97–116. doi: 10.1080/13657300209380306
Bravo, A., Försterra, G., and Häussermann, V. (2005). “Fishing in troubled waters – evidence for higher diversity and high abundance of cold water corals along the Chilean coast,” in Proceedings of the 3rd International Symposium on Deep-Sea Corals Science and Management, eds R. Brock and R. Y. George (Miami, FL: Rosenstiel School of Marine and Atmospheric Science), 234.
Brookfield, J. F. Y. (1996). A simple new method for estimating null allele frequency from heterozygote deficiency. Mol. Ecol. 5, 453–455. doi: 10.1046/j.1365-294X.1996.00098.x
Cañete, J. I., and Häussermann, V. (2012). Colonial life under the humboldt current system: deep-sea corals from O’Higgins I seamount. Lat. Am. J. Aquat. Res. 40, 467–472.
Chapuis, M.-P., and Estoup, A. (2007). Microsatellite null alleles and estimation of population differentiation. Mol. Biol. Evol. 24, 621–631. doi: 10.1093/molbev/msl191
Cornuet, J. M., and Luikart, G. (1997). Description and power analysis of two tests for detecting recent population bottlenecks from allele frequency data. Genetics 144, 2001–2014. doi: 10.1093/genetics/144.4.2001
Earl, D. A., and vonHoldt, B. M. (2012). STRUCTURE HARVESTER: a website and program for visualizing STRUCTURE output and implementing the Evanno method. Conserv. Genet. Resour. 4, 359–361. doi: 10.1007/s12686-011-9548-7
Evanno, G., Regnaut, S., and Goudet, J. (2005). Detecting the number of clusters of individuals using the software STRUCTURE: a simulation study. Mol. Ecol. 14, 2611–2620. doi: 10.1111/j.1365-294X.2005.02553.x
Falush, D., Stephens, M., and Pritchard, J. K. (2003). Inference of population structure using multilocus genotype data: linked loci and correlated allele frequencies. Genetics 164, 1567–1587. doi: 10.1111/j.1471-8286.2007.01758.x
FAO (2020). GLOBEFISH Highlights April 2020 Issue, with Annual 2019 Statistics – A Quarterly Update on World Seafood Markets. Globefish Highlights No. 2–2020. Rome: FAO. doi: 10.4060/ca9528en
Feehan, K. A., Waller, R. G., and Häussermann, V. (2019). Highly seasonal reproduction in deep-water emergent Desmophyllum dianthus (Scleractinia: Caryophylliidae) from the Northern Patagonian Fjords. Mar. Biol. 166:52. doi: 10.1007/s00227-019-3495-3
Fillinger, L., and Richter, C. (2013). Vertical and horizontal distribution of Desmophyllum dianthus in Comau Fjord, Chile: a cold-water coral thriving at low pH. PeerJ 1:e194. doi: 10.7717/peerj.194
Försterra, G. (2009). “Ecological and biogeographical aspects of the Chilean Fjord region,” in Marine Benthic Fauna of Chilean Patagonia, eds V. Häussermann and G. Försterra (Santiago: Nature in Focus), 61–76.
Försterra, G., Beuck, L., Häussermann, V., and Freiwald, A. (2005). “Shallow water Desmophyllum dianthus (Scleractinia) from Chile: characteristics of the biocenoses, the bioeroding community, heterotrophic interactions and (palaeo)-bathymetrical implications,” in Cold-Water Corals and Ecosystems, eds A. Freiwald and J. M. Roberts (Berlin: Springer-Verlag), 937–977. doi: 10.1007/3-540-27673-4_48
Försterra, G., and Häussermann, V. (2003). First report on large scleractinian (Cnidaria: Anthozoa) accumulations in cold-temperate shallow water of south Chilean fjords. Zool. Verh. 345, 117–128.
Försterra, G., Häussermann, V., and Laudien, J. (2017). “Animal forests in the Chilean fiord region: discoveries and perspectives in shallow and deep waters,” in Marine Animal Forests, eds S. Rossi, L. Bramanti, A. Gori, and C. Orejas Saco del Valle (Cham: Springer), 1–35. doi: 10.1007/978-3-319-17001-5_3-1
Försterra, G., Häussermann, V., Laudien, J., Jantzen, C., Sellanes, J., and Muñoz, P. (2014). Mass die off of the cold-water coral Desmophyllum dianthus in the Chilean Patagonian Fjord Region. Bull. Mar. Sci. 90, 895–899. doi: 10.5343/bms.2013.1064
Google Inc (2009). Google Earth. Available online at: https://www.google.com/earth/versions/#download-pro (accessed December 1, 2019).
eds V. Häussermann and G. Försterra (2009). Marine Benthic Fauna of Chilean Patagonia. Santiago: Nature in Focus.
Häussermann, V., and Försterra, G. (2007). Extraordinary abundance of hydrocorals (Cnidaria, Hydrozoa, Stylasteridae) in shallow water of the Patagonian fjord region. Polar Biol. 30, 487–492. doi: 10.1007/s00300-006-0207-5
Häussermann, V., and Försterra, G. (2014). Vast reef-like accumulation of the hydrocoral Errina antarctica (Cnidaria, Hydrozoa) wiped out in Central Patagonia. Coral Reefs 33:29. doi: 10.1007/s00338-013-1088-z
Häussermann, V., Försterra, G., Melzer, R. R., and Meyer, R. (2013). Gradual changes of benthic biodiversity in Comau Fjord, Chilean Patagonia – lateral observations over a decade of taxonomic research. Spixiana 36, 161–171.
Holloley, C. E., and Geerts, P. G. (2009). Multiplex Manager 1.0: a cross-platform computer program that plans and optimizes multiplex PCR. BioTechniques 46, 511–517. doi: 10.2144/000113156
Hromic, T. (2009). Estructura comunitaria del taxa Foraminiferida (Protozoa), según profundidad, en el fiordo Comau, Chiloé, Chile. An. Inst. Patagon. (Chile) 37, 39–51. doi: 10.4067/S0718-686X2009000100004
Kopelman, N. M., Mayzel, J., Jakobsson, M., Rosenberg, N. A., and Mayrose, I. (2015). CLUMPAK: a program for identifying clustering modes and packaging population structure inferences across K. Mol. Ecol. Resour. 15, 1179–1191. doi: 10.1111/1755-0998.12387
Li, Y.-L., and Liu, J.-X. (2018). StructureSelector: a web based software to select and visualize the optimal number of clusters using multiple methods. Mol. Ecol. Resour. 18, 176–177. doi: 10.1111/1755-0998.12719
Mayr, C., Rebolledo, L., Schulte, K., Schuster, A., Zolitschka, B., Försterra, G., et al. (2014). Responses of nitrogen and carbon deposition rates in Comau Fjord (42°S, Southern Chile) to natural and anthropogenic impacts during the last century. Cont. Shelf Res. 78, 29–38. doi: 10.1016/j.csr.2014.02.004
Miller, K. J., and Gunasekera, R. M. (2017). A comparison of genetic connectivity in two deep sea corals to examine whether seamounts are isolated islands or stepping stones for dispersal. Sci. Rep. 7:46103. doi: 10.1038/srep46103
Peakall, R., and Smouse, P. E. (2012). GenAlEx 6.5: genetic analysis in excel. population genetic software for teaching and research—an update. Bioinformatics 28, 2537–2539. doi: 10.1093/bioinformatics/bts460
Piry, S., Alapetite, A., Cornuet, J.-M., Paetkau, D., Baudouin, L., and Estoup, A. (2004). GeneClass2: a software for genetic assignment and first-generation migrant detection. J. Hered. 95, 536–539. doi: 10.1093/jhered/esh074
Pritchard, J. K., Stephens, M., and Donnelly, P. (2000). Inference of population structure using multilocus genotype data. Genetics 155, 945–959.
Puechmaille, S. J. (2016). The program structure does not reliably recover the correct population structure when sampling is uneven: subsampling and new estimators alleviate the problem. Mol. Ecol. Resour. 16, 608–627. doi: 10.1111/1755-0998
Raymond, M., and Rousset, F. (1995). GENEPOP version1.2: population genetics software for exact tests and ecumenicism. J. Hered. 86, 248–249. doi: 10.1093/oxfordjournals.jhered.a111573
Rousset, F. (2008). Genepop’007: a complete re-implementation of the genepop software for windows and linux. Mol. Ecol. Resour. 8, 103–106. doi: 10.1111/j.1471-8286.2007.01931.x
Sánchez, N., González, H. E., and Iriarte, J. L. (2011). Trophic interactions of pelagic crustaceans in Comau Fjord (Chile): their role in the food web structure. J. Plankton Res. 33, 1212–1229. doi: 10.1093/plankt/fbr022
Schwabe, E., Försterra, G., Häussermann, V., Melzer, R., and Schrödl, M. (2006). Chitons (Mollusca: Polyplacophora) from the southern Chilean Comau Fjord, with reinstatement of Tonicia calbucensis plate, 1897. Zootaxa 1341, 1–27. doi: 10.11646/zootaxa.1341.1.1
Silva, N., Calvete, C., and Sievers, H. (1997). Características oceanográficas físicas y químicas de canales australes chilenos entre Puerto Montt y Laguna San Rafael (Crucero Cimar-Fiordo 1). Cienc. Tecnol. Mar. 20, 23–106.
Sobarzo, M. (2009). “The fjord region of southern chile: oceanographic aspects,” in Marine Benthic Fauna of Chilean Patagonia, eds V. Häussermann and G. Försterra (Santiago: Nature in Focus), 53–60.
Soto, M. V., Arratia, P., Cabello, M., Moreno, R., and Whyndam, K. (2019). Natural hazards and exposure of strategic connectivity in extreme territories. Comau Fjord, North Patagonia, Chile. Rev. Geogr. Norte Gd. 73, 57–75.
Tokuda, Y., Haraguchi, H., and Ezaki, Y. (2017). First real-time observation of transverse division in azooxanthellate scleractinian corals. Sci. Rep. 7:41762. doi: 10.1038/srep41762
Van Oosterhout, C., Hutchinson, W. F., Wills, D. P. M., and Shipley, P. (2004). Micro-Checker: software for identifying and correcting genotyping errors in microsatellite data. Mol. Ecol. Notes 4, 535–538. doi: 10.1111/j.1471-8286.2004.00684.x
Keywords: cold water corals, genetic structure, oceanographic conditions, conservation, aquaculture sustainability, Patagonian fjord region
Citation: Addamo AM, Zaccara S, Försterra G, Höfer J, García-Jiménez R, Crosa G and Machordom A (2021) Genetic Conservation Management of Marine Resources and Ecosystems of Patagonian Fjords. Front. Mar. Sci. 8:612195. doi: 10.3389/fmars.2021.612195
Received: 30 September 2020; Accepted: 26 January 2021;
Published: 23 February 2021.
Edited by:
Giorgio Bavestrello, University of Genoa, ItalyReviewed by:
Leocadio Blanco-Bercial, Bermuda Institute of Ocean Sciences, BermudaCopyright © 2021 Addamo, Zaccara, Försterra, Höfer, García-Jiménez, Crosa and Machordom. This is an open-access article distributed under the terms of the Creative Commons Attribution License (CC BY). The use, distribution or reproduction in other forums is permitted, provided the original author(s) and the copyright owner(s) are credited and that the original publication in this journal is cited, in accordance with accepted academic practice. No use, distribution or reproduction is permitted which does not comply with these terms.
*Correspondence: Anna Maria Addamo, YW0uYWRkYW1vQGdtYWlsLmNvbQ==; YW5uYS5hZGRhbW9AZWMuZXVyb3BhLmV1
Disclaimer: All claims expressed in this article are solely those of the authors and do not necessarily represent those of their affiliated organizations, or those of the publisher, the editors and the reviewers. Any product that may be evaluated in this article or claim that may be made by its manufacturer is not guaranteed or endorsed by the publisher.
Research integrity at Frontiers
Learn more about the work of our research integrity team to safeguard the quality of each article we publish.