- 1Texas Marine Mammal Stranding Network, Galveston, TX, United States
- 2Clymene Enterprises, Lakeside, CA, United States
- 3Department of Marine Biology, Texas A&M University at Galveston, Galveston, TX, United States
Construction-related loss of habitat, degradation of existing habitat, noise pollution, and vessel activity are growing issues for Indo-Pacific humpback dolphins (Sousa chinensis) that occur in the shallow, near-shore, highly industrialized waters off Lantau Island, Hong Kong. We studied the occurrence of dolphins in discrete locations, fine-scale movement patterns, and dolphin behavioral activity states. Potential explanatory variables varied and included year, season, time of day, dolphin group size and behavioral activity state, proximity to construction activity, and vessel type and number. Land-based observations and theodolite tracking of dolphins and vessels were conducted from seven locations to the north of Lantau Island, Hong Kong, and marine construction activities near survey sites were identified. A total of 636 groups of dolphins were recorded, totaling 150.91 h of tracking, from 405 days of observation effort. Hurdle models were used to analyze dolphin occurrence, multivariate generalized additive models were used to analyze fine-scale movement patterns, and log-likelihood ratio and binomial z score post hoc tests were used to analyze behavioral activity states. Dolphin occurrence was lower in historically important areas near long-term, low-intensity construction activity, and dolphin swimming speed was higher in response to vessel presence. Overall, foraging and traveling were the most frequently observed behavioral activity states and resting behavior was observed off only one location that was not in proximity to construction activities. Temporal overlap in adjacent marine construction areas may displace animals for extended periods and nearby ecologically similar habitats should be identified and designated as marine protected areas to mitigate effects of such disturbance.
Introduction
Ecosystem disturbance may be problematic for small populations of cetaceans (whales, dolphins, and porpoises) that show high site fidelity to areas used for foraging, mating, resting, or calf rearing, particularly where multiple industrial projects overlap (Würsig, 1989; Bejder et al., 2009). Construction-related noise (e.g., pile driving), direct loss of habitat (e.g., artificial land formation, port development), degradation of existing habitat (e.g., dredging), and an increase in construction-related vessel activity alter natural seascapes, which can influence the distribution and behavior of marine mammals (Brandt et al., 2011; Pirotta et al., 2013; Thompson et al., 2013). Coastal development is a growing issue for humpback dolphins (genus Sousa) that typically occur in shallow (<20 m deep), near-shore, brackish waters associated with estuarine systems. These waters are among the most productive aquatic systems for primary and secondary production (Beck et al., 2001). However, near-shore distribution exposes Sousa to recreational and commercial maritime activities, particularly for those living near densely populated urban areas and industrialized coastal areas.
Hong Kong is one of the most densely urbanized coastal regions in the world (Shelton et al., 2013), and ongoing development contributes to anthropogenic noise and activity in the habitats of the Indo-Pacific humpback dolphin (S. chinensis; herein “humpback dolphins” or “dolphins”; Jefferson et al., 2009; Karczmarski et al., 2016). Humpback dolphins display limited habitat flexibility relative to water depths and distances to shore (Parra and Ross, 2009; Jefferson and Curry, 2015; Jefferson and Smith, 2016). The distribution of dolphins off Hong Kong is influenced by freshwater flowing from the Pearl River, where dolphins occur in higher densities in areas with steeper benthic slopes, often along natural island shores and rocky coastlines (Hung, 2008; Jefferson and Smith, 2016). Research concentrating on the Pearl River Estuary (PRE) population of dolphins began in the early 1990s (Parsons, 1997; Jefferson, 2000), and data show that the waters around Lantau Island (Figure 1) have historically been an important habitat for dolphins (Jefferson and Hung, 2004; Marcotte et al., 2015). In the PRE, prey availability is one of the most important factors for predicting dolphin density (Hung, 2008), and foraging opportunities may be impacted if foraging grounds of prey species are disrupted.
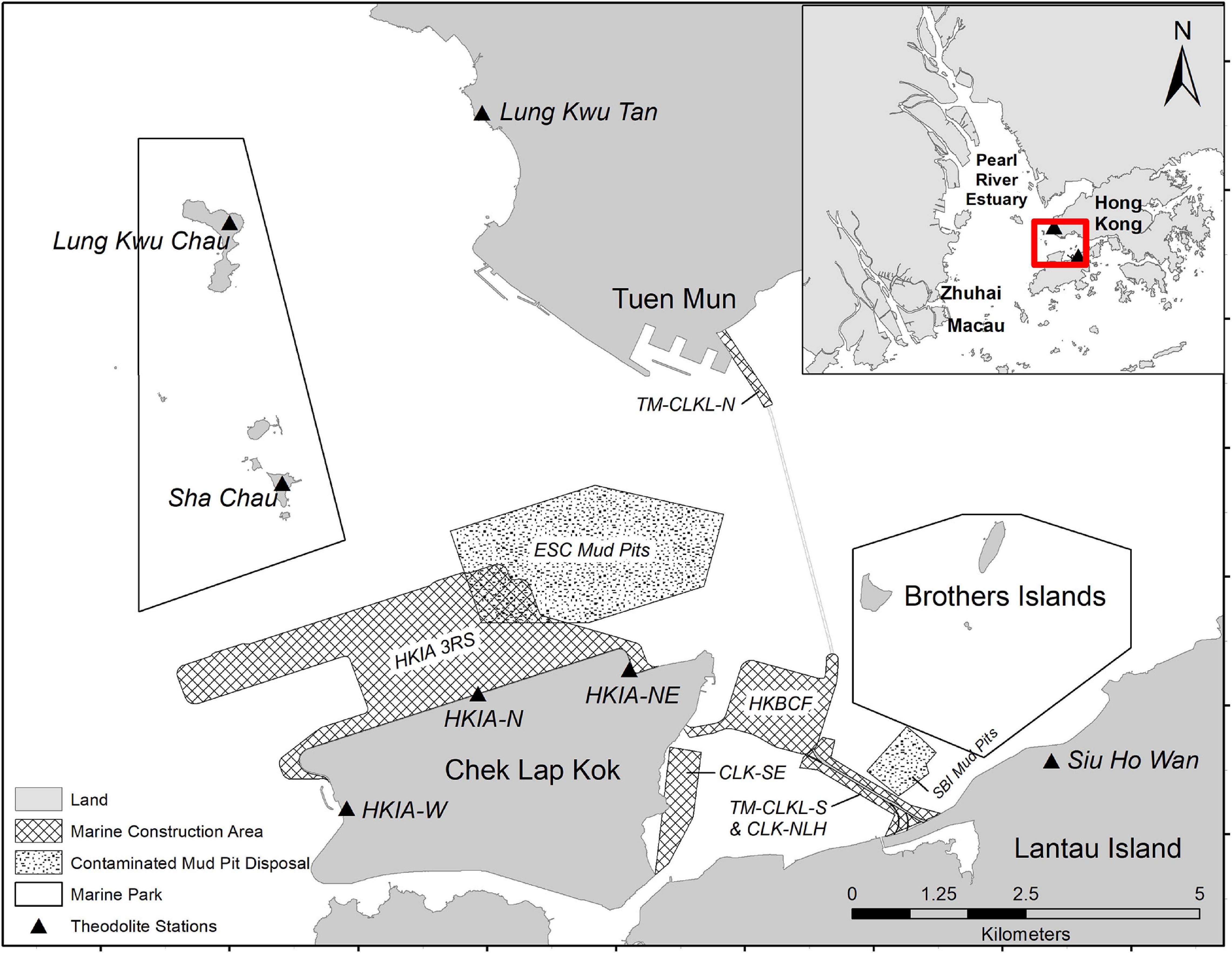
Figure 1. Study area off Lantau Island, Hong Kong. Map shows land-based theodolite observation locations, marine construction and mud pit disposal areas, and marine park boundaries. Map created using ArcGIS (Version 10.2.2, http://www.esri.com/software/arcgis).
Although the PRE population of Sousa appears to be somewhat resilient to habitat alterations (Jefferson and Rosenbaum, 2014), dolphin abundance has significantly declined in the waters around Lantau Island over the past decade (Hung, 2017; Jefferson, 2018). The International Union for Conservation of Nature (IUCN) Red List of Threatened Species designated S. chinensis as Near Threatened through 2016 (Reeves et al., 2008; Jefferson and Smith, 2016). However, this designation did not reflect the widely recognized taxonomic reclassification, which separated humpback dolphins into four species: S. chinensis, S. plumbea, S. sahulensis, and S. teuszii (Jefferson and Rosenbaum, 2014). Under this new taxonomic restructuring, S. chinensis meets the IUCN Red List requirements for Vulnerable status (Jefferson and Smith, 2016; Jefferson et al., 2017), one of the threatened categories. Populations of Sousa spp. are regionally endemic with small geographic ranges, some of which show evidence of restricted gene flow (Mendez et al., 2011). Thus, research, conservation, and management efforts should focus not only on distinct species, but on separate populations (Reeves, 2009).
Several large-scale development projects are recently completed, currently underway, or planned for the near future in the waters north of and adjacent to Lantau Island, Hong Kong, with unknown consequences to humpback dolphins (Figure 1). From 2009 to 2018, a large bridge and tunnel system was constructed to connect Hong Kong to Macau and Zhuhai (the Hong Kong/Zhuhai/Macau Bridge, HZMB). The bridge extends directly through dolphin habitat from Macau through mainland China waters and to the west of Chek Lap Kok Island (Chen et al., 2010). One hundred and fifty hectares of artificial land was created to the east of Chek Lap Kok to support border crossing facilities (HKBCF) associated with the HZMB, and the southern landfall associated with the Tuen Mun-Chek Lap Kok Link (TM-CLKL). Artificial land (16.5 ha) for the northern landfall for TM-CLKL was also formed just off Tuen Mun, which connects with the southern landfall via a subsea tunnel. In addition to these projects, the Hong Kong International Airport (HKIA) is constructing a third runway to meet the needs of future air traffic growth. Construction for the third runway system officially commenced in August 2016, adding 640 ha of artificial land north of Chek Lap Kok. In addition to ocean noise created by marine construction, commercial passenger airplanes contribute underwater noise in audible ranges of a variety of dolphins (Erbe et al., 2018). With increased flight capacity provided by the HKIA expansion, noise pollution from airplanes is also of concern. Furthermore, marine vessel traffic used to transport people, equipment, and supplies has increased to facilitate construction projects. Hong Kong waters are also used by many other types of vessels including ferries, high speed hydrofoils and catamarans, commercial fishing vessels, tankers and container ships, dolphin tour vessels, government vessels, and private recreational vessels. Escalating marine development and associated vessel traffic in Hong Kong has the potential to further alter dolphin occurrence and behavior, with unknown long-term consequences (Ng and Leung, 2003; Jefferson et al., 2009; Marcotte et al., 2015).
The effects of persistent marine construction and associated vessels on small odontocetes (toothed whales) have previously been described, but are confounded when multiple industrial activities co-occur with varying methods and intensity (Todd et al., 2014). Several studies have shown significant variation in odontocete behavior and habitat-use relative to construction. For example, construction of a gas pipeline reduced harbor porpoise (Phocoena phocoena) presence in a historically important area off Ireland (Culloch et al., 2016). In another study off Europe, common bottlenose dolphins (Tursiops truncatus) spent significantly less time in a known foraging area when local dredging activity intensified (Pirotta et al., 2013). In Chinese waters, dolphin swimming speed increased significantly during industrial pile-driving and overall presence declined directly following pile-driving activity (Würsig et al., 2000). Bottlenose dolphin (T. aduncus) occurrence near Perth, Australia, was negatively affected by vessel density in one discrete location, but not in an adjacent site within the same estuary. However, no dolphins were observed on days when marine dredgers were in operation (Marley et al., 2016). In contrast, Australian humpback dolphins (S. sahulensis) in Moreton Bay, Australia, are abundant and appear to co-exist with extensive coastal development. However, a proactive management approach has been recommended in Moreton Bay to provide protection to the endemic species that occur there (Chilvers et al., 2005). Based on these examples, the effects of construction activity can be measured by assessing shifts in animal behavioral activity states, and spatio-temporal presence and distribution patterns proximate to construction and related vessel activity.
In this study, we analyzed S. chinensis occurrence in proximity to maritime construction activities, movement patterns in the presence and absence of vessels, and behavioral activity states in discrete locations in the waters north of Lantau Island, Hong Kong. Specific objectives were to: (1) determine how dolphin track duration (i.e., amount of time dolphins spent in each location) varies with natural and anthropogenic factors to better understand when and where dolphins are more likely to be sighted, (2) quantify dolphin movement patterns to assess dolphin behavioral responses to vessels, (3) analyze behavioral activity states in discrete near-shore locations, and (4) provide recommendations for behavior-based management to contribute to conservation efforts. Findings from this study can be broadly informative to areas where small cetaceans and human activity overlap, and more specifically, where dolphins with small near-shore ranges overlap with heavily populated urbanized coasts where development is diverse and growing.
Materials and Methods
Sampling Methods
Data collection was part of large-scale assessments of two coastal urban development projects in the waters to the north of Lantau Island, Hong Kong including: (1) the HKIA third runway system (3RS) coordinated by the Hong Kong Airport Authority in partnership with Mott MacDonald, Ltd., and (2) a land reclamation feasibility study coordinated by the Hong Kong Civil Engineering and Development Department (CEDD) in partnership with Ove Arup, Ltd. Land-based observations and theodolite tracking were conducted from seven locations to the north of Lantau Island (22° N, 113° E; Table 1 and Figure 1). Passive acoustic monitoring (PAM) and boat-based surveys were conducted separately as part of the broader Environmental Monitoring and Audit works, in addition to the theodolite research described herein. Five of the theodolite sites were established for the 3RS study, and two were established for the CEDD land reclamation feasibility study (Table 1). Station platforms included natural hilltops and building rooftops, based on availability of elevated positions at each location, which varied in height (Table 1). Theodolite tracking was conducted discontinuously between October 2012 and December 2016, excluding most of 2015, with varying effort at individual sites.
Each daily survey comprised approximately 6 h of effort unless inclement weather delayed or prematurely ended a session. Data collection involved a theodolite operator, an observer, and a data-entry computer operator. Observers systematically scanned for dolphins once per hour using handheld binoculars (7 × 50 magnification) followed by continuous non-systematic searching by unaided eye and binoculars until the next hourly systematic scan. Theodolite tracking sessions were initiated when an individual dolphin or a group of dolphins was located. Digital theodolites (Sokkia/Sokkisha Model DT5) with 30-power magnification and ± 5-s precision were used to obtain the vertical and horizontal angle of each dolphin or dolphin group (Würsig et al., 1991). Data were recorded and angles were converted to geographic coordinates (latitude and longitude) using Pythagoras software, Version 1.2 (Gailey and Ortega-Ortiz, 2002).
When possible, a distinguishable individual dolphin, based on body coloration, was selected for a focal follow session. Humpback dolphins range from gray to white with varying degrees of spotting, based on age, which can help distinguish individuals in the field (Jefferson, 2000; Jefferson et al., 2012). The focal individual (Altmann, 1974; Mann, 1999) was continuously tracked via theodolite with a position recorded (when possible) each time the dolphin surfaced. If an individual could not be positively distinguished from other members, the group was tracked by recording a position based on a central point within the group whenever the animals surfaced (Bejder, 2005; Martinez, 2010). If dolphins within a group surfaced asynchronously, the theodolite operator would pause to determine where all dolphins in the group were prior to recording the central position. Dolphins were considered part of a group if they were (1) within 10 m of each other (Smolker et al., 1992), regardless of individual behavior, or (2) within 100 m of each other and engaged in the same behavioral activity state (Mann et al., 2000). Tracking continued until animals were lost from view, moved beyond the range of reliable visibility, or environmental conditions obstructed visibility (e.g., intense haze, Beaufort sea state > 3). Dolphin data were recorded continuously in the field and later subsampled and interpolated at 60-s intervals. For each positional fix of dolphins, data collection included geographic position, group size, calf presence/absence, and predominant group (≥50% of individuals) behavioral activity state. Calves were distinguished by their smaller size and primarily gray skin pigmentation (Jefferson, 2000; Dungan et al., 2012). Surface behavioral activity states were broadly based on definitions from Karczmarski and Cockcroft (1999; Table 2).
All vessels that moved within approximately 1 km of the focal individual/group were also tracked via theodolite, including cargo and container ships, construction transport vessels, transportation ferries, commercial fishing vessels, dolphin tour vessels, government vessels, and private recreational vessels. Vessel data included geographic position, vessel type, and vessel activity (e.g., traveling, stationary, following dolphins).
Over the course of this research, eight major marine construction areas (and associated activities) were identified in the waters north of Lantau Island with varying proximity to dolphin research locations. Data on proximate marine construction projects underway during the sampling periods were obtained from the Government of the Hong Kong Special Administrative Region, Marine Department (GHKSAR, 2021). Activities included dredging, backfilling, capping of contaminated mud pits, non-dredge land reclamation, seawall construction, and stone column installation. Information on geographic coordinates of working boundaries and start/end dates of individual projects are included in Appendix Table 1. General details were available, but fine-scale information on daily construction activities was not obtained. It was necessary to establish a distance threshold to classify the presence or absence of construction activity in a given habitat. One study predicted that dredging noise could be detected by unspecified “marine fauna” up to 6 km from the sound source, depending on local conditions (Thomsen et al., 2009). A study in the PRE estimated that sounds produced by the OCTA-KONG, the world’s largest vibration hammer, may be detectable by dolphins up to 3.5 km from the sound source (Wang et al., 2014). Another study described behavioral changes by harbor porpoises up to 15 km from sound-intensive pile-driving activity (Madsen et al., 2006). In the absence of data clearly relating the effects of chronic low-intensity marine construction activity on dolphin responses based on distance, a 5 km diameter threshold was selected as a moderate metric. Construction boundaries were plotted in ArcMap (Version 10.2.2), and distances from each of the seven theodolite locations were calculated.
Statistical Analysis
Dolphin Track Duration
Dolphin track duration was based on the total duration of dolphin tracking sessions (hh:mm:ss) at each location standardized by survey time on-effort. Potential explanatory variables included year, solar season (spring, summer, autumn, or winter), oceanographic season (wet or dry), time of day, location (seven discrete areas associated with theodolite tracking stations), and construction activity (presence/absence of proximate construction activity as defined in sampling methods; Appendix Table 2). The potential explanatory variables of location and year were dropped from the fully saturated model due to output errors associated with confounding factors. Solar season included spring (March-May), summer (June-August), autumn (September-November), and winter (December-February). Oceanographic season was based on total monthly rainfall (wet season defined as a month with average rainfall ≥60 mm, generally late spring through early autumn; dry season defined as a month with average rainfall <60 mm, generally late autumn through early spring). Sunrise and sunset times fluctuated throughout the study period. Therefore, a time of day index was calculated to standardize the percentile of daylight hours where sunrise = 0 and sunset = 1.
Variation in track duration was assessed using regression models with time converted to count data (i.e., each second equates to an integer of 1). Theodolite observation sessions with no dolphins present were treated as zero counts, leading to zero-inflated count data. Negative binomial hurdle models were fit using survey effort as the offset and model selection was based on the Akaike Information Criterion with correction (AICc; Hurvich and Tsai, 1989; Burnham and Anderson, 2004). Hurdle models use a two-stage approach in which one model predicts the probability of an observation to be zero or not, and one model predicts amounts for positive counts (Hu et al., 2011). The political science computational laboratory package in program R was used to run hurdle models (Jackman, 2020).
Fine-Scale Movement Patterns
For movement pattern analysis, data were filtered to omit dolphin tracks with only 1 positional fix, <10 min in total track time, and/or potentially erroneous positions (e.g., positional fixes on land). To standardize across observations that varied in duration, individual tracking sessions were separated into 10-min segments (Bejder et al., 1999; Lundquist et al., 2012), comprising 11 interpolated data records per segment, based on 60 s intervals. The selected time interval reduces issues associated with non-linear travel observed by dolphins. Temporal autocorrelation was assessed to identify potential pseudo-replication (Dray et al., 2010) for groups with multiple segments. Significant autocorrelation was not detected in the residuals and all segments were retained for analyses. From the 636 dolphin groups tracked, 417 10-min segments were appropriate for movement pattern analyses. Collinearity among potential explanatory variables was evaluated using augmented pairs plots (i.e., correlation coefficients > 0.50 indicated potential masking effects) and Variance Inflation Factor values (i.e., values > 5 indicated potential masking effects). If collinearity was expressed among potential explanatory variables, the most interpretable variable was retained, and the masking variable was dropped.
Dolphin response variables calculated for every 10-min segment were mean swimming speed, reorientation rate, and linearity. Swimming speed (km/h) was calculated by dividing the distance traveled by the duration between two consecutive positions (Gailey et al., 2007). Reorientation rate is the degrees per minute of change in direction of a tracked individual or group of dolphins. Reorientation rate was calculated by dividing the sum of the absolute values of bearing changes within a segment by the total duration of that segment (Smultea and Würsig, 1995). Linearity is an index of net movement represented by a range from 0 to 1, where 0 represents no net movement by dolphins (i.e., a circular path) and 1 represents movement in a straight line. Linearity was calculated by dividing the net distance (i.e., distance between the first and last position of a segment) by the sum of all distances traveled between each of the 11 interpolated positional fixes within each segment (Lundquist et al., 2013).
Dolphin movement was evaluated relative to vessel presence (when vessels were within 100 m of dolphins)/absence (when vessels were farther than 100 m from dolphins). Several vessel distance thresholds (i.e., distance in which vessels were considered “present”) were evaluated during the exploratory analysis phase, including 500, 300, and 100 m, to select an appropriate threshold. Variation emerged for the 100 m threshold only, and this distance has been established in other odontocete literature involving effects of human activity (Constantine, 2001; Williams and Ashe, 2007; Weir et al., 2010). The “no vessel” control category included segments in which no moving vessels were within 100 m during, and for at least 10 min prior to, the dolphin tracking session. To calculate distances, vessel positions with two or more positional fixes were interpolated post hoc relative to dolphin locations.
Multivariate generalized additive models (GAMs), appropriate for evaluating complex data that are multivariate and non-linear (Hastie and Tibshirani, 1986), were used to analyze fine-scale movement patterns in response to natural and anthropogenic candidate explanatory variables (Appendix Table 2). Models were run using the multiple generalized cross-validation (mgcv) package in program R (Wood, 2006). These models evaluate variables simultaneously, reducing problems associated with many step-wise techniques. The mgcv package uses thin-plate regression splines to fit smooth terms for potential explanatory variables, allowing smoothing of multiple terms at once. Knots are used to model the smoothed relationship for each term, set at a default of 10. Knots were reduced for the smoothed terms, time and group size, that had insufficient unique values to support the default. Cubic regression splines, defined by knots spread evenly through the covariate values (Wood, 2006), were selected for the smoothed terms. Response variables were transformed (Log10 for swimming speed, Square Root for reorientation rate, and Empirical Logit for linearity) to approximate normal distribution and were modeled using the quasi-likelihood family with an identity link.
The fully saturated GAM included the fixed effects of time of day, solar season, dolphin group size, behavioral activity state, and vessel number. The candidate explanatory variable “vessel type” was dropped from the fully saturated model due to issues with multicollinearity with “vessel number.” The procedure for dropping model terms was based on protocol outlined by Wood (2001). Smooth terms were dropped from the model if the following three conditions were met: (1) the estimated degrees of freedom was close to 1, (2) the 95% confidence interval for the term included 0 everywhere, and (3) the generalized cross-validation (GCV) score dropped and deviance explained increased when the term was removed. If only the first condition was met, the smooth term was replaced with a linear term. Linear terms were dropped from the model if the parameter coefficient was close to 0, the significance of the term was near to 1, and the GCV score dropped and the deviance explained increased when the term was removed (Wood, 2001). If these conditions were not met, the terms were retained in the model.
Computational analyses of swimming speeds, reorientation rates, and linearity were calculated in Microsoft Excel 2013. R statistical software (Version 3.2.2) was used to perform exploratory work and GAM analyses. Linear mixed-effect modeling was run to assess autocorrelation, using the lme function (package nlme) in R.
Behavioral Activity States
The 10-min segments described in section “Fine-scale Movement Patterns” were also used to analyze behavioral activity state data. However, each of the 11 data points within segments were included in analysis due to the potential for behavioral activity state to fluctuate over the course of 10 min. Unknown behavioral activity states were excluded from behavioral activity state analysis. Univariate log-likelihood ratio and binomial z score post hoc tests were calculated in Microsoft Excel 2013 and used to assess behavioral activity states based on discrete locations (Appendix Table 2). The binomial z score decision rule was based on the critical value 1.96. Computational analyses and statistical tests were calculated in Microsoft Excel 2013.
Results
A total of 405 observation days, including 2301.55 h, of survey effort were conducted. During this time, 636 groups of dolphins were recorded totaling 150.91 h of dolphin tracks (Figure 2). Only 5% (n = 32) of groups contained calves. Vessels were recorded in proximity to 13% (n = 54) of dolphin groups. Dolphin track duration varied among study locations. The highest percentage of dolphin tracks was collected from LKC, twice the amount of any other station (Table 3).
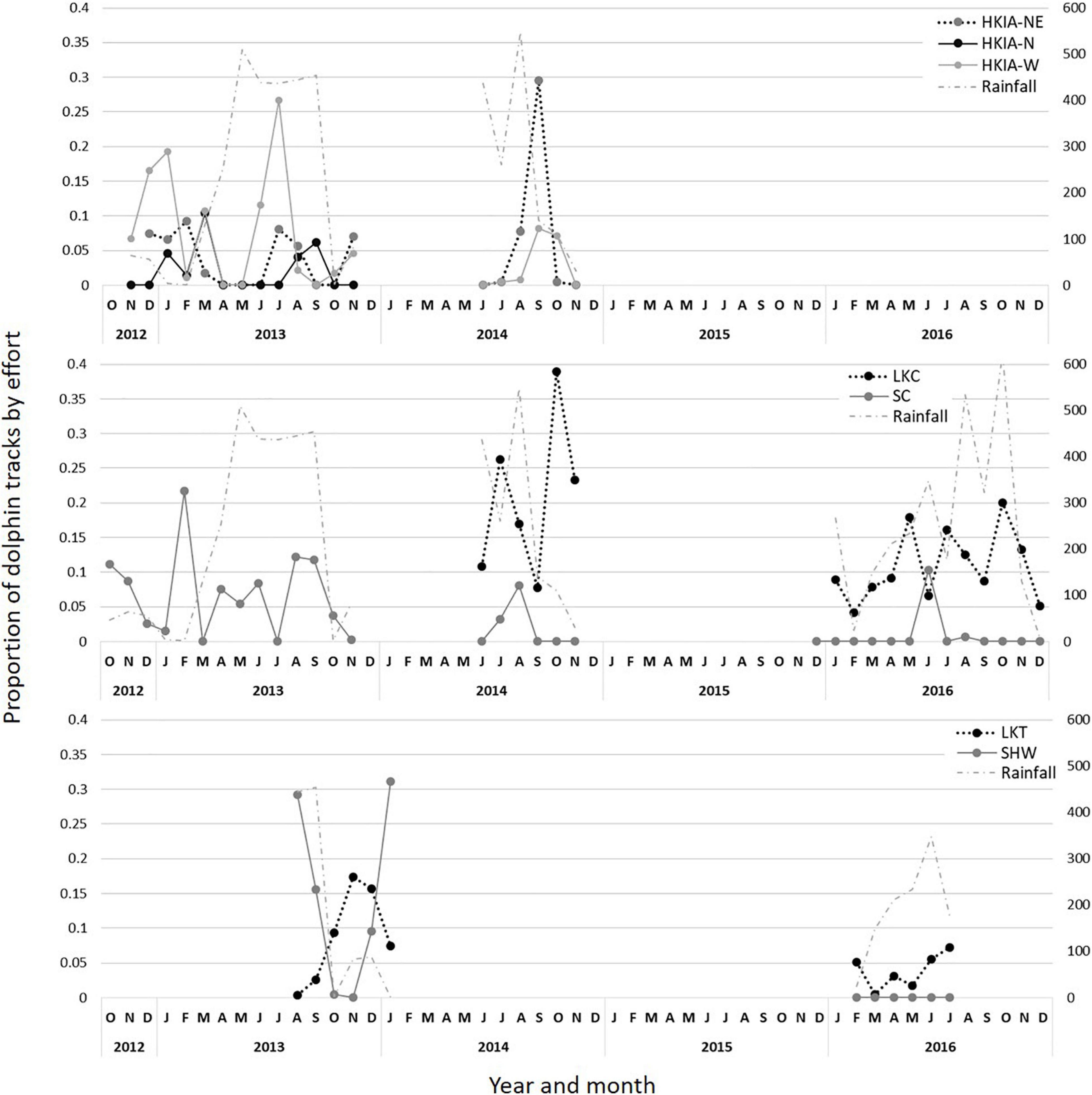
Figure 2. Proportion of Indo-Pacific humpback dolphin track duration standardized by on-effort survey time. Figure includes year, month, and rainfall for each land-based location. Months with no points indicate zero effort.
Dolphin Track Duration
The negative binomial hurdle model described solar season, time of day, and construction activity as important factors for predicting presence/absence of dolphins. The selected hurdle model fit the data well, provided plausible estimates, and included four potential explanatory variables and an offset variable for on-effort search time:
Dolphin track duration was significantly lower during the spring than any other season, and significantly lower in areas within 5 km of chronic construction activity (θ = 1.19, p < 0.001; Table 4). Variation was also expressed based on time of day, with higher overall track duration during mid-morning hours between 9:00 AM and 12:00 PM (Figure 3). However, variation also exists among stations.
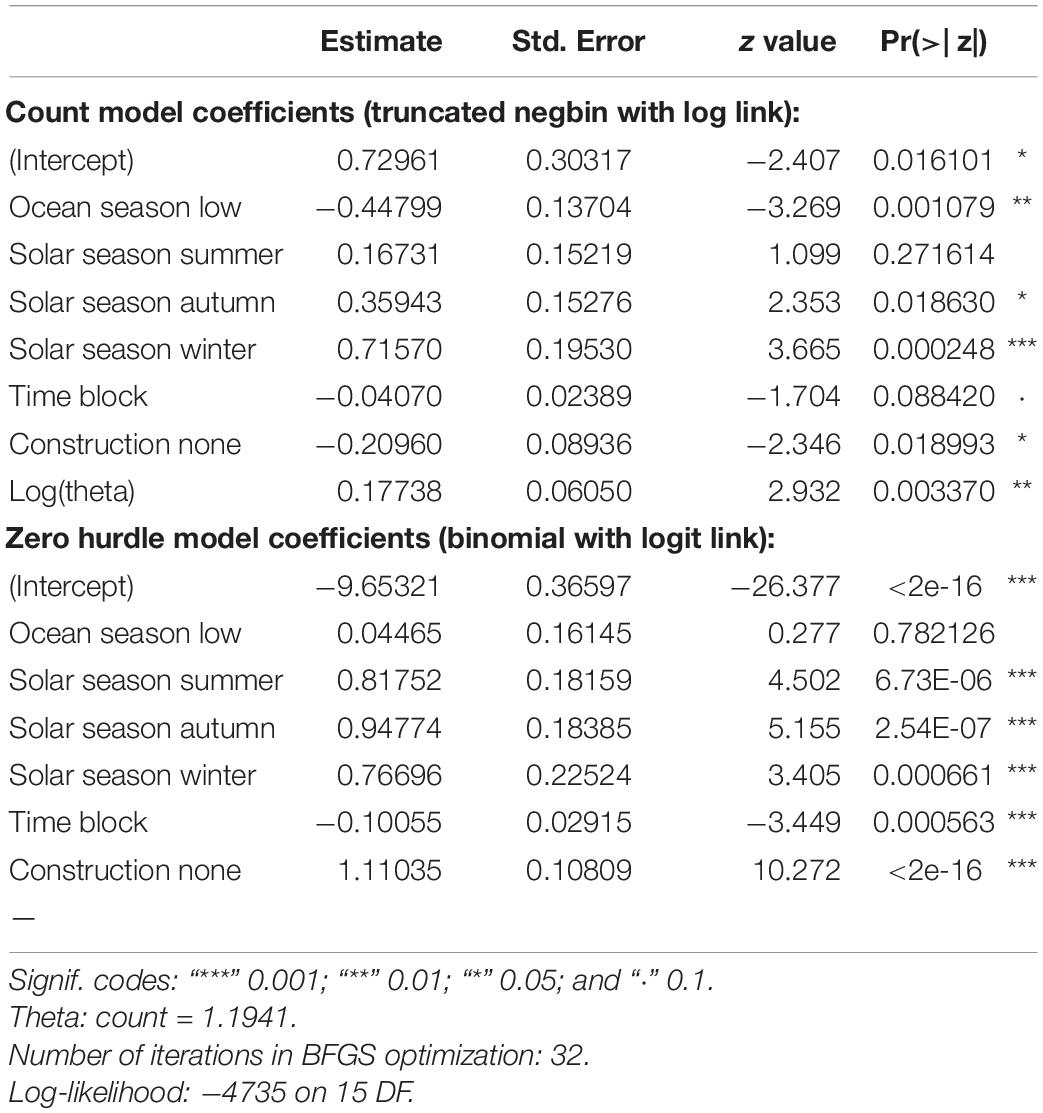
Table 4. Summary of output for the plausible negative binomial hurdle model for Indo-Pacific humpback dolphin occurrence.
Fine-Scale Movement Patterns
Swimming Speed
The GAM described variation in swimming speed, at the 0.05 alpha level, explaining 14% of the deviance (adj-R2 = 0.098, GCV = 0.061, and n = 417). The best fitting model included all five candidate explanatory variables, with a smooth term for both time of day and dolphin group size, and linear terms for predominant group behavior, solar season, and vessel number present:
Traveling behavior was associated with higher swimming speed along the horizontal plane than foraging and milling. Swimming speed was higher in the spring than in the autumn and winter. Swimming speed increased as vessel number increased. Time of day and dolphin group size had no significant effect on swimming speed (Table 5 and Figure 4).
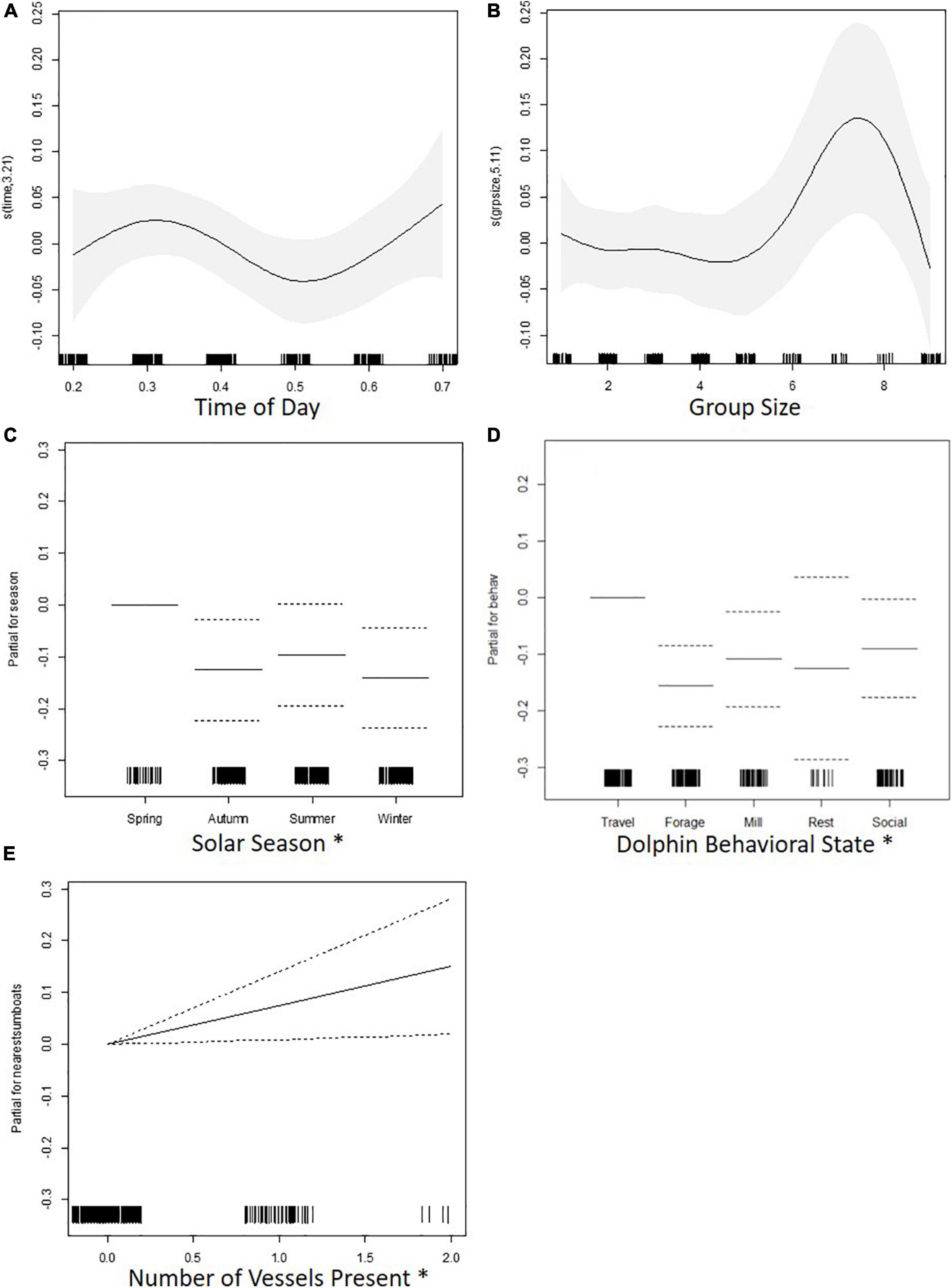
Figure 4. Charts for the partial contribution of individual explanatory variables in the fitted GAM for swimming speed. Charts include: (A) time of day, (B) dolphin group size, (C) solar season, (D) dolphin behavioral state, and (E) number of vessels present. The rugplot along the x-axis indicates the density of observations for each factor. On the y-axis, values > 0 indicate a positive correlation with swimming speed, values < 0 indicate a negative correlation, and a value of 0 indicates no effect. Signif. codes: “*” 0.05; “⋅” 0.1.
Reorientation Rate
The GAM described a small amount of variation in reorientation rate, at the 0.05 alpha level, explaining 8% of the deviance (adj-R2 = 0.054, GCV = 3.482, and n = 417). The best fitting model included all five candidate explanatory variables, with a smooth term for both time of day and dolphin group size, and linear terms for predominant group behavior, solar season, and vessel number present:
Traveling behavior was associated with lower reorientation rate along the horizontal plane than foraging, milling, and socializing. Time of day, dolphin group size, season, and number of vessels had no significant effect on reorientation rate (Table 6 and Figure 5).
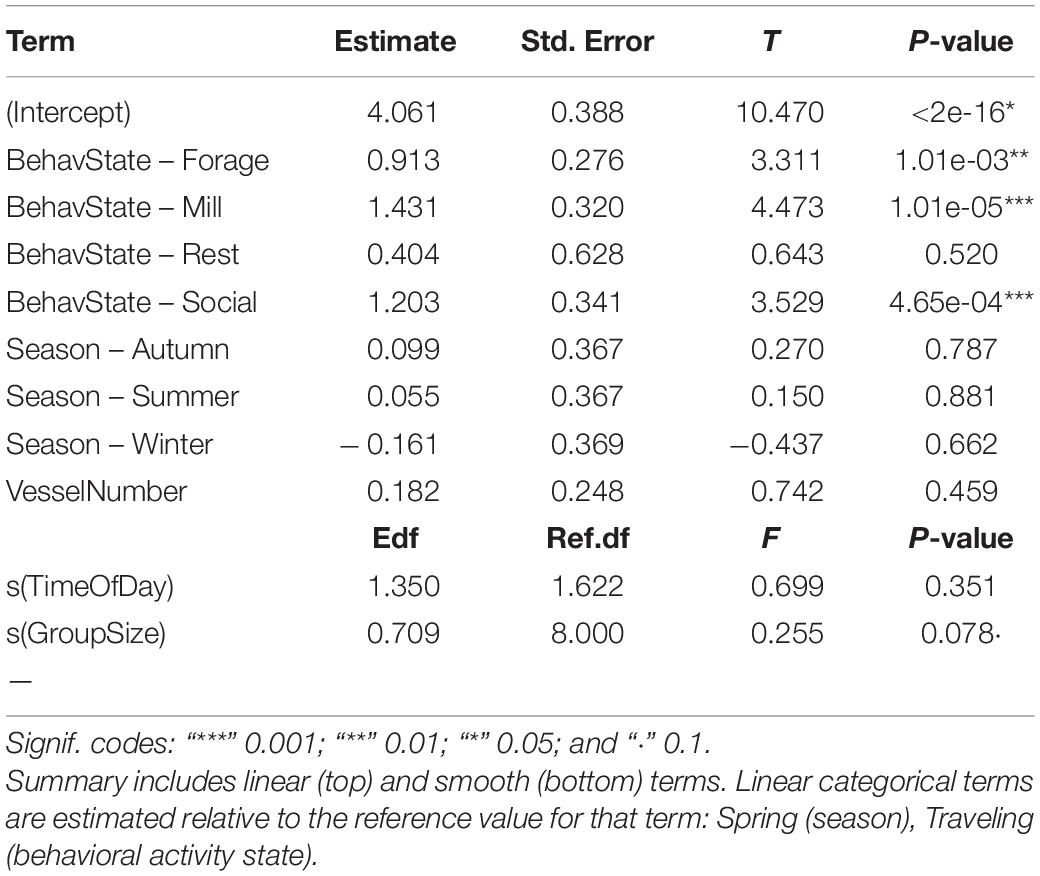
Table 6. Summary of output for best fitting model for Indo-Pacific humpback dolphin reorientation rate.
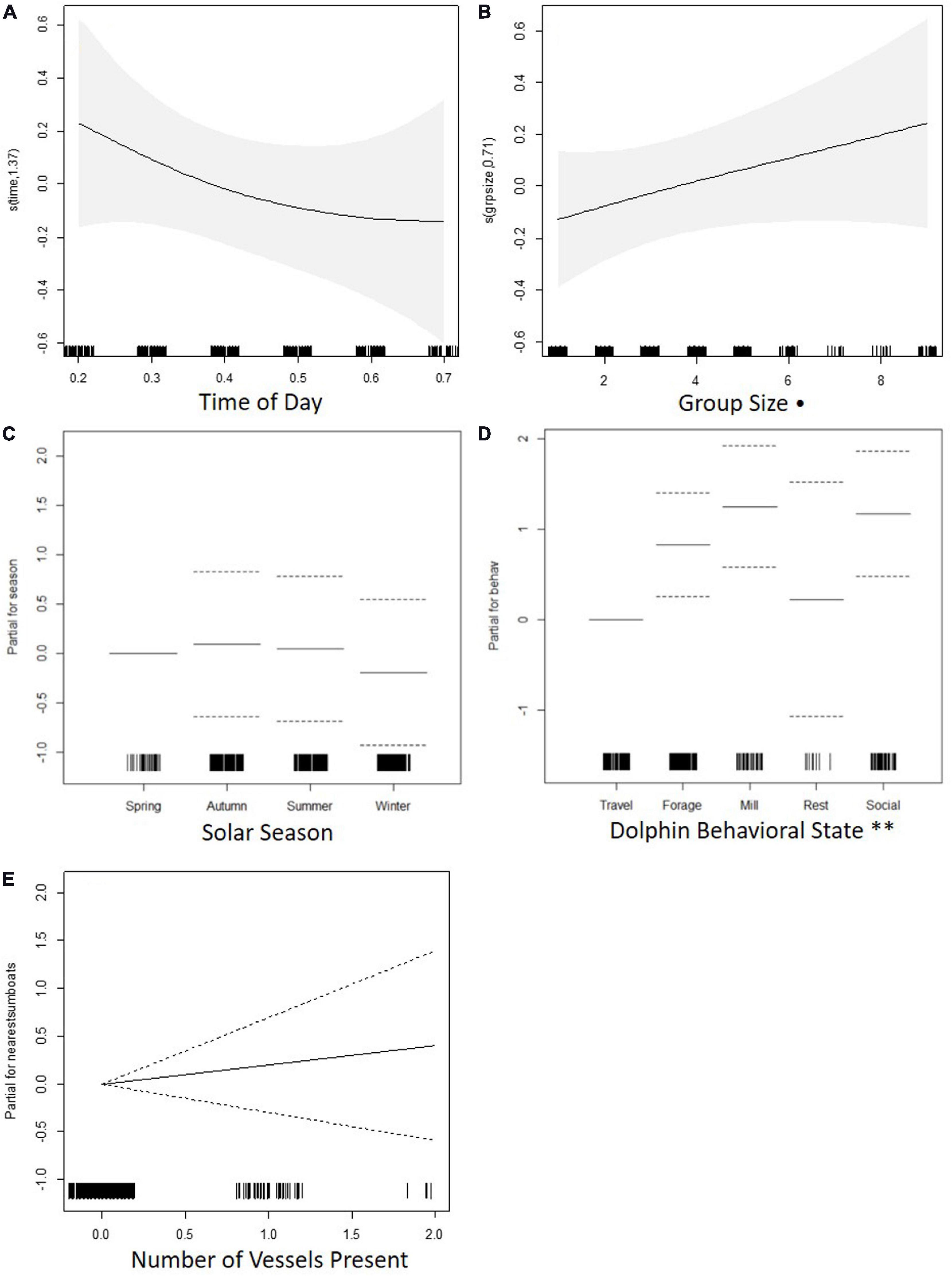
Figure 5. Charts for the partial contribution of individual explanatory variables in the fitted GAM for reorientation rate. Charts include: (A) time of day, (B) dolphin group size, (C) solar season, (D) dolphin behavioral state, and (E) number of vessels present. The rugplot along the x-axis indicates the density of observations for each factor. On the y-axis, values > 0 indicate a positive correlation with reorientation rate, values < 0 indicate a negative correlation, and a value of 0 indicates no effect. Signif. codes: “**” 0.01; “⋅” 0.1.
Linearity
The GAM described a small amount of variation in linearity, at the 0.05 alpha level, explaining 4% of the deviance (adj-R2 = 0.014, GCV = 3.292, and n = 417). The best fitting model included all five candidate explanatory variables, with a smooth term for both time of day and dolphin group size, and linear terms for predominant group behavior, solar season, and vessel number present:
Traveling behavior was associated with higher linearity along the horizontal plane than foraging and milling. Time of day, dolphin group size, season, and number of vessels had no significant effect on linearity (Table 7 and Figure 6).
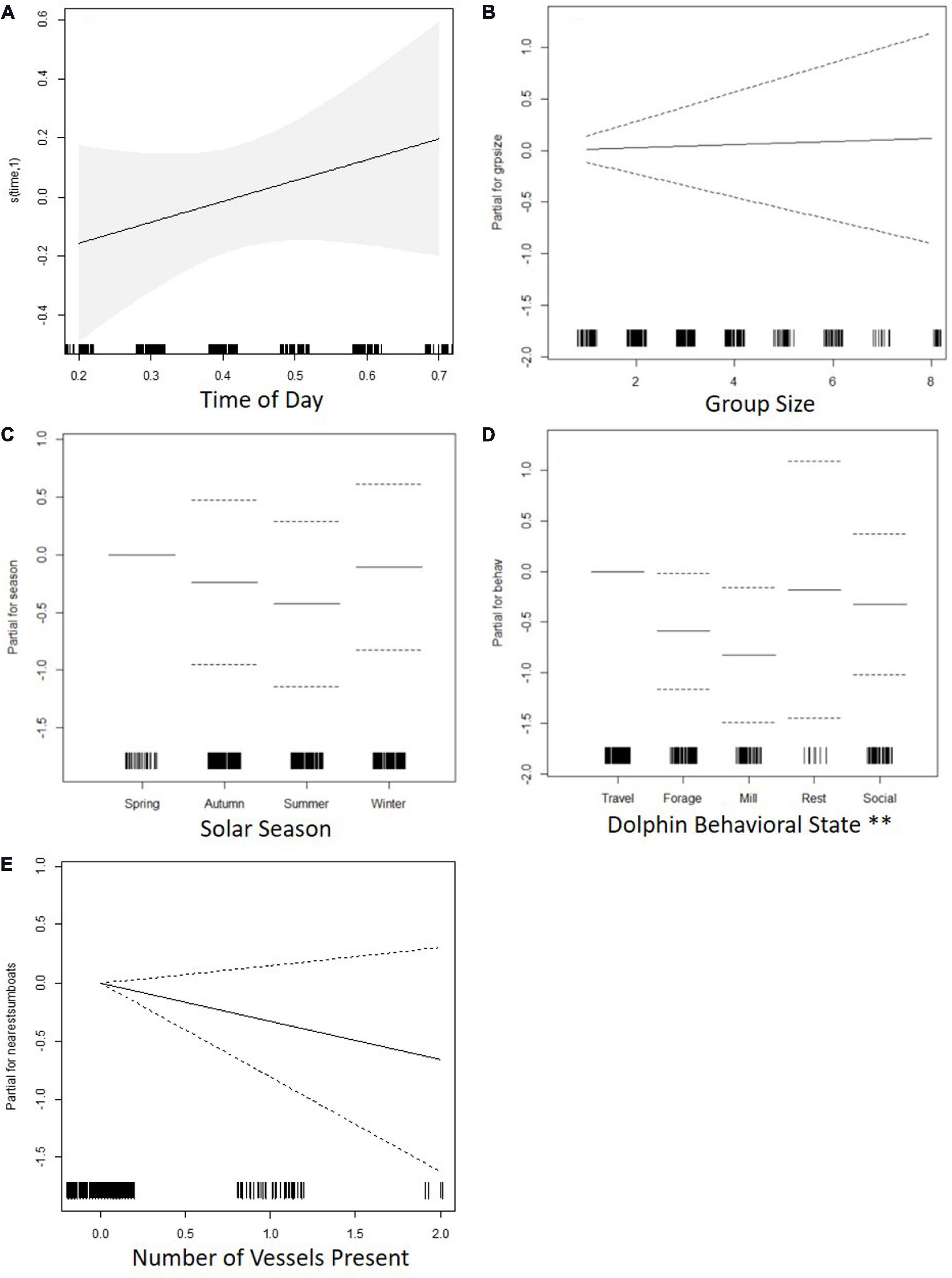
Figure 6. Charts for the partial contribution of individual explanatory variables in the fitted GAM for linearity. Charts include: (A) time of day, (B) dolphin group size, (C) solar season, (D) dolphin behavioral state, and (E) number of vessels present. The rugplot along the x-axis indicates the density of observations for each factor. On the y-axis, values > 0 indicate a positive correlation with linearity, values < 0 indicate a negative correlation, and a value of 0 indicates no effect. Signif. codes: “**” 0.01.
Behavioral Activity States
Behavioral data totaled 4,587 records (i.e., 11 records per 417 filtered segments). Unknown behavioral activity states (n = 1,854) were excluded from behavioral activity state analysis. Overall, foraging (32%, n = 881), and traveling (32%, n = 877) were the most frequently observed behavioral activity states, followed by milling (22%, n = 597), socializing (11%, n = 287), and resting (3%, n = 80). Resting was only observed off LKC and was excluded from univariate analysis due to an inadequate minimum expected value. Behavioral activity states varied significantly based on discrete location (log-likelihood ratio test, G2 = 458.98, n = 2,642, df = 24, and P < 0.001; Figure 7). Post hoc tests showed that dolphins were more likely to be foraging off HKIA-NE (z = 9.14) and LKC (z = 6.10), and less likely to be foraging off HKIA-N/W (z =−6.43/−3.76), LKT (z =−1.98) and SHW (z =−3.11). Dolphins were more likely to be traveling off LKT (z = 2.29) and SHW (z = 4.46), and less likely to be traveling off HKIA-NE (z =−3.39) and SC (z =−3.93). Dolphins were more likely to be milling off HKIA-W (z = 4.57), LKT (z = 4.98), and SHW (z = 2.10), and less likely to be milling off HKIA-N (z =−5.19) and LKC (z =−4.94). Dolphins were more likely to be socializing off HKIA-N (z = 10.91) and SC (z = 4.29), and less likely to be socializing off HKIA-NE (z =−5.11), LKT (z =−5.15), and SHW (z =−3.41).
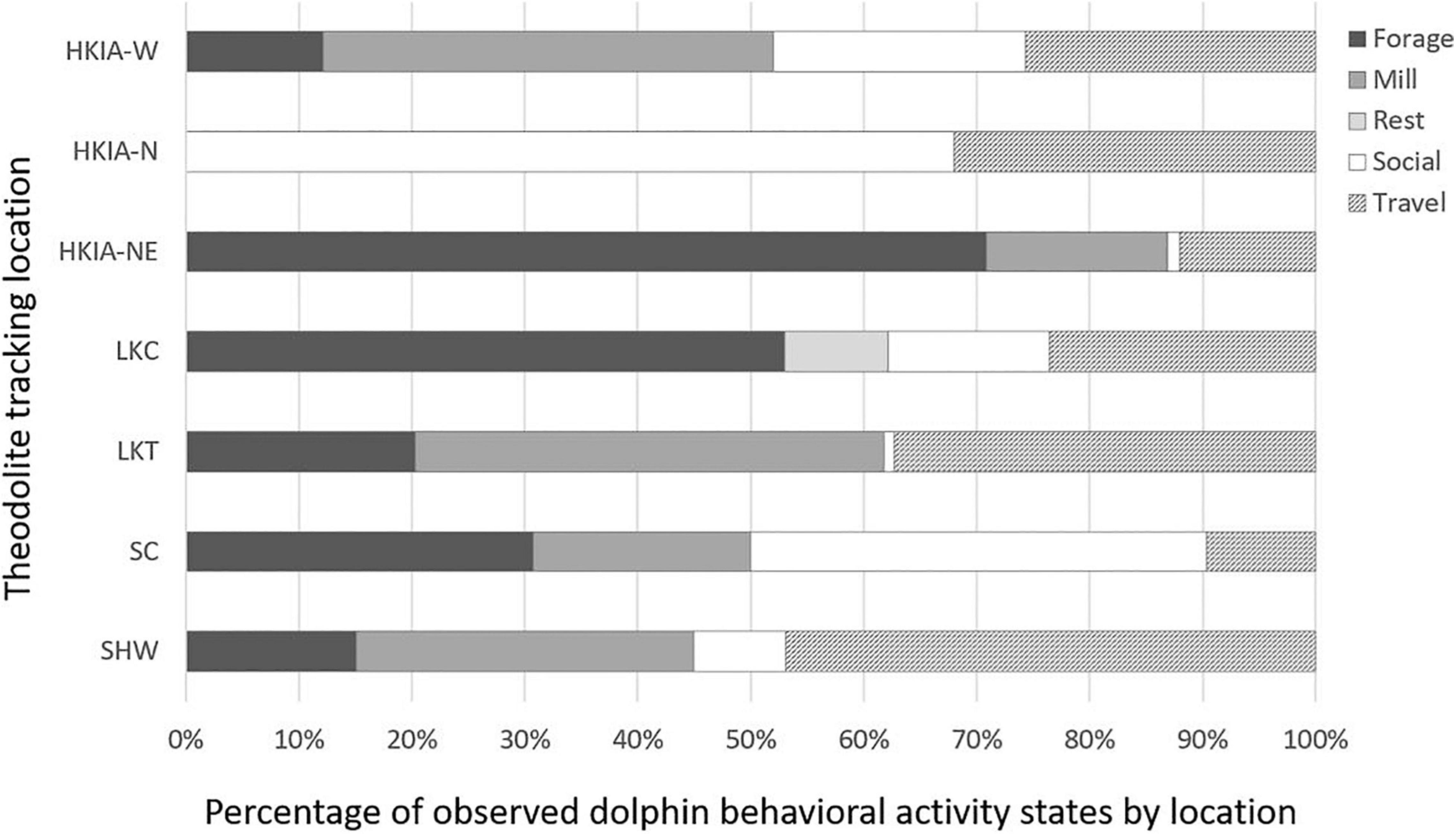
Figure 7. Stacked bar graph showing behavioral activity state percentages of Indo-Pacific humpback dolphins off Hong Kong, based on theodolite tracking locations.
Discussion
In this study, the overall dolphin day-time track duration was lower in discrete areas near construction activity, lower during the spring season, and lower during early morning and afternoon hours. Dolphin movement patterns varied based on natural and anthropogenic factors. Swimming speed increased in the presence of vessels, was higher in spring than in autumn and winter, and was higher during traveling behavior than during foraging and milling. Reorientation rate was lower during traveling than during socializing. Overall, foraging and traveling were the most frequently observed behavioral activity states. Resting was observed the least, and only off LKC, one of two study areas that were not in close proximity to construction activity.
Theodolite tracking is an excellent tool for recording precise coordinates of dolphins and vessels, and collecting behavioral activity states without influencing dolphin behavior. However, there are inherent limitations involved with elevated land-based research, including limited viewing range, distance to focal animals, and the inability to identify individual dolphins (Piwetz et al., 2018). For example, it is difficult to observe discrete behaviors (e.g., pectoral flipper rubbing, object carrying) or examine individual heterogeneity in movement patterns. For this study, there were also limitations relative to the statistical analyses. Some of the potential explanatory variables in the GAMs include categories with small sample size, indicated by a sparse rugplot and wide confidence interval (e.g., number of vessels). Furthermore, the relatively weak explanatory power (i.e., deviance explained) by the GAM models, particularly linearity, indicate that there are additional factors influencing dolphin movement patterns in the waters off Lantau Island, Hong Kong, that were not considered here. Therefore, the GAM output results should be interpreted with caution, due to low sample size and deviance explained, and additional data are needed to detect statistical differences among factors.
Dolphin track duration was significantly lower in areas proximate to long-term, low-intensity construction activity, indicating that dolphins spent less time in areas near construction work. One trend that was not captured in the analysis is that dolphin track duration off SHW, which was relatively high in 2013–2014, dropped to zero in 2016 concurrent with an increase in the number of proximate construction projects. The area off SHW is historically important for dolphin foraging and socializing activity (Hung, 2008), making the total lack of dolphins as seen from visual surveys during 2016 concerning. Acoustic detections of vocalizing dolphins collected during the same time period continued to be made in the area, mainly at night (see Munger et al., 2016 and Munger et al., 2018 for details). Likewise, track duration declined to zero off SC in all but 2 months of 2016, also concurrent with an increase in the number of proximate construction projects.
Dolphin track duration varied seasonally, with significantly lower duration during the spring season than any other season, which is congruent with PAM findings (Munger et al., 2016). Dolphins occur south of Lantau Island, particularly during the wet season (Hung, 2008), which may reflect an overall shift southward during the late spring. Shifts in distribution off Hong Kong may potentially relate to calving season that peaks in the spring and early summer (Jefferson et al., 2012), or more likely to seasonal changes in prey distribution. Seasonal shifts in distribution patterns have also been described for Sousa off South Africa where dolphins inhabit Plettenberg Bay for significantly shorter periods in spring than in winter (Parsons, 2004).
Variation in track duration was also identified based on time of day, with generally higher duration between mid-morning and mid-day and lower duration during early morning and afternoon hours. However, temporal patterns also varied based on location. PAM described significantly higher dolphin click detection rates at night than during daylight hours. Dolphins may be foraging more at night and/or using the monitored sites less during the day, possibly due to heightened vessel traffic and construction activity during daylight hours (Munger et al., 2016).
An increase in swimming speed was the only movement pattern that varied based on vessel presence. Two previous studies on Sousa behavior and vessel traffic show no reactions by dolphins to slow-moving vessels. Dolphins off Hong Kong and Indian Ocean humpback dolphins (S. plumbea) in the Indus Delta Region, Pakistan, displayed no observable behavioral changes when vessels operated in the vicinity at slow speeds (Pilleri and Gihr, 1974; Ng and Leung, 2003). However, dolphin responses off Hong Kong (in the previous study) were nominal (e.g., categorized as positive, negative, and neutral) and vessel type and vessel distance (estimated by visual observation) were tested separately (Ng and Leung, 2003). Currently, high speed ferries off Hong Kong are of great concern in terms of potential vessel collision and acoustic disturbance (Hung, 2015; Marcotte et al., 2015). Considering the heavy traffic off Hong Kong and the increased swimming speeds of dolphins in the vicinity of vessels, the low sample size of dolphin groups in proximity to vessels likely reflects avoidance of vessels in general. In December 2015, speed control mitigation measures were placed on high speed ferries rerouted to travel around the northern portion of the SCLKC Marine Park off LKC, where dolphin presence is historically high (Jefferson, 2018). Vessels in this context may be more predictable, easier to move away from, and therefore perceived as less threatening.
In addition to human activity, dolphin movement patterns also varied based on natural factors. Swimming speed was higher during the spring season than in the autumn and winter. Humpback dolphin mating season in the South China Sea is around April to June (Wang and Sun, 1982; Jefferson, 2000; Jefferson et al., 2012), during which high speed mating chases (i.e., males chasing females) may be more common. Traveling behavior was associated with higher swimming speed, lower reorientation rate, and increased linearity than foraging and milling behavior. Reorientation rate was also lower during traveling than during socializing. This is not surprising as foraging, milling, and socializing behaviors include asynchronous movements in various directions, in which dolphins change bearing more frequently.
Hearing is considered the most important sensory modality for odontocetes (Thewissen, 2009), necessary for foraging, navigating the environment, and maintaining social contact with conspecifics. Odontocetes possess good functional hearing generally ranging from 200 Hz to 100,000 Hz and above, depending on species (Ketten, 2004). However, there are insufficient data on species-specific hearing ranges to establish exposure guidelines. The lower hearing sensitivity of S. chinensis is not known, with hearing only recently tested and with no useful data at low frequencies (<5,600 Hz), where much of the noise from industrial and vessel activities occurs (Li et al., 2012). Odontocete species that have been tested at low frequencies can hear down to 40–75 Hz (Richardson and Würsig, 1997), well within the range of many chronic industrial activities. Noise emitted during construction activities off Hong Kong during this study, including drilling and dredging, were likely persistent at lower frequencies than noise produced by acute high-intensity sound energy with higher peak frequency levels, such as pile driving. Dredging can be a source of continuous noise in nearshore regions, strongest at low frequencies (<1,000 Hz) but can exceed 6,000 Hz (Richardson et al., 1995; Todd et al., 2014). Large vessels, such as cargo and tanker ships, emit primarily low frequency sounds (10–500 Hz) and general vessel sounds examined off Hong Kong in 2010–2011 ranged from 315–45,000 Hz, well within the audible range of dolphins (Sims et al., 2012). These chronic, low-intensity sounds may affect dolphins directly (e.g., masking communication), where noise reflected off the seabed and steep benthic slopes in shallow systems can result in multiple transmission paths (Reine et al., 2014), or indirectly (e.g., shift in prey distribution). Although high-frequency echolocation clicks are not likely masked by most chronic industrial noise, S. chinensis may use passive listening to detect soniferous prey species such as croakers (Family Sciaenidae), the most numerically important prey found in S. chinensis stomach contents (Barros and Cockcroft, 1999; Barros et al., 2004; Parsons, 2004). Sciaenids produce sounds at dominant frequencies between 100–1,000 Hz (Ramcharitar et al., 2006), and low frequency anthropogenic noise may mask sounds that they produce. The ability to detect prey via passive sonar tracking may be particularly important for dolphins off Hong Kong that live in a naturally turbid, estuarine environment, further agitated by construction activity in which visibility is limited.
Marine mammals may compensate for small–scale changes in prey distribution by moving to alternate foraging areas, switching prey species, or devoting more time to foraging. The differences in dolphin track duration at different locations and high overall percentage of foraging behavior when present may reflect such compensation, but more research is needed to explore this potential. The consequences of displacement are poorly understood, but may include increased stress responses and reduced foraging opportunities. Long term impacts of habitat displacement are dependent on the duration of the disturbance, the quality and proximity of alternate available habitat, and the potential for the historically used habitat to recover.
Prior research in other areas has shown optimistic trends of marine mammals returning to previously abandoned areas. For example, harbor porpoises returned to Puget Sound and San Francisco Bay after decades of being absent from both of these areas (Jefferson et al., 2016). Causes for the initial decline are not fully understood, but potentially relate to large-scale human-induced changes (e.g., pollution, fisheries bycatch, habitat loss, and degradation; Jefferson, 2018). A similar situation for harbor porpoises in the southern North Sea was reported, wherein porpoises have returned after a significant decline in the mid-twentieth century (Thomsen et al., 2006). Likewise, gray whales (Eschrichtius robustus) were displaced from a breeding area for more than 5 years in response to industrial sounds, returning years after activities ceased (Jones et al., 1994). We have seen the same behavior in humpback dolphins that returned to areas off Chek Lap Kok once intensive development activities stopped. These are encouraging outcomes, but habitats must recover and be protected for re-establishment of dolphins to be possible.
Alternatively, the outcome can be devastating for populations in areas that continue to degrade and where research and mitigation measures are absent or insufficient. An extreme example of a habitat alteration impact on a marine mammal species is the baiji (Lipotes vexillifer) that was recently declared extinct due to intensive habitat degradation and bycatch in fishing gear (Turvey et al., 2007). Populations with small home ranges and strong site fidelity are especially vulnerable to habitat displacement in highly disturbed areas because they may have few suitable alternatives (Todd et al., 2014; Forney et al., 2017). Indo-Pacific humpback dolphins in Hong Kong waters are part of a larger population (>2,000; Jefferson, 2018) that occurs in the PRE, and demographic modeling indicates a rate of decline around 2.46% per annum (Huang et al., 2012). However, the “subpopulation” within Hong Kong’s boundaries has declined by about 50% over the past 15 or so years, likely due in part to range shifts (Hung, 2014; Jefferson et al., 2017; Jefferson, 2018). In addition to habitat loss and degradation, dolphins in Hong Kong waters experience a number of anthropogenic threats. For example, environmental contaminants, such as organochlorines, have been detected in high concentrations in dolphin blubber. However, it is unclear how the overall population is affected by these pollutants (Jefferson et al., 2006). Bycatch in trawler gear and vessel collisions appear to be among the greatest causes of human-caused mortality, with growing evidence that they contribute directly to the declining numbers of dolphins in the PRE (Jefferson et al., 2017). Dolphins may also be particularly vulnerable to climate change, given their restricted geographical distribution with limited opportunity to expand their range.
One of the biggest challenges to Sousa conservation is protecting and maintaining suitable high-quality habitat (Parra and Ross, 2009), especially considering the cumulative nature of human activities in developing densely populated regions. An important management tool for protecting portions of S. chinensis habitat is proper designation and enforcement of Marine Protected Areas (MPAs) in known dolphin “hotspots” that include areas used for life-history processes (e.g., foraging; Hoyt, 2011). Marine Parks in Hong Kong are protected areas that prohibit some fishing, collection of animals or plants, operating vessels at greater than 10 knots, and damaging shoreline features, among other restrictions. If previously disturbed areas become designated MPAs once construction activity ceases, dolphins may return. Lung Kwu Chau, an important foraging area, lies within the Sha Chau and Lung Kwu Chau (SCLKC) Marine Park. The area off HKIA-NE, which is also a historically important foraging area for dolphins, currently includes a marine park around the Brothers Islands, designated in December 2016, but was not protected during this research. Waters off SC and HKIA-N, which are in close proximity to one another, appear to be important for social behavior. Although SC lies within the bounds of the SCLKC Marine Park, both SC and HKIA-N overlapped directly with construction activity. Traveling was observed more than any other behavior off SHW (near construction and vessels), a historically important area for dolphin foraging and social activity.
Marine Protected Area boundaries should be developed and managed with knowledge of humpback dolphin habitat use, movement patterns, and behavior. For example, dolphin habitat currently or historically used for biological processes (e.g., foraging, calf-rearing, and resting) are good candidates for protection. We also suggest a much larger matrix of MPAs, with travel corridors between areas, be considered for a more comprehensive coverage. Currently, MPAs make up only 2.45% (4,050 ha) of the Hong Kong Special Administrative Region (165,000 ha), and only 1.71% (2,820) were designated with dolphins in mind (AFCD, 2020). Most recently, 650 ha around the southwestern coastal waters of Lantau Island have been designated as a marine park to help conserve dolphins. As part of the mitigation/compensation measures for the new third runway expansion of the HKIA, a large marine park encompassing much of the currently unprotected sections of habitat north of Lantau Island are slated to be established, once the third runway construction is complete (Jefferson, 2018). These parks are expected to be helpful to dolphin conservation efforts in Hong Kong, although critical habitat, especially along the west coast of Lantau Island, still remains largely unprotected (Karczmarski et al., 2017; Jefferson, 2018). These waters have been identified as important foraging, calving, and nursery areas (Jefferson, 2018). A proposal has also been made for a large matrix of MPAs in western Hong Kong, which would provide important conservation benefits to the dolphins, though so far there has been little to no movement by the government to make it happen (Karczmarski et al., 2016).
We recommend continued monitoring during intensive construction activity using a diverse methodological approach, including boat-based surveys, theodolite tracking, and PAM. This approach yields information on dolphin abundance, distribution, and site fidelity from boat-based surveys, occurrence, fine-scale movement patterns, and behavior in discrete locations from theodolite tracking, and presence when visual observations are not possible (e.g., night time and inclement weather) from acoustic research. Continued monitoring is also important during post-construction phases to document potential shifts in habitat, including re-establishing previously used areas, which can be used to inform management decisions. Off Hong Kong, a multi-organizational approach is also encouraged to better understand cumulative impacts from the considerable number of overlapping and logistically complex projects that affect the same population of dolphins.
Data Availability Statement
The datasets presented in this article are not readily available to the public because they are bound by confidentiality agreements. Requests to access the datasets should be directed to SP, spiwetz@tmmsn.org.
Ethics Statement
Ethical review and approval was not required for this animal study. Research was conducted from elevated, shore-based locations and no approaches to or harassment of animals were made. Permits were not required for this fully non-invasive, observational method.
Author Contributions
SP contributed to the conceptualization, methodology, formal analysis, writing of the original draft, and visualization. TJ contributed to the conceptualization, review and editing of drafts, visualization, and supervision of the project. BW contributed to the conceptualization, methodology, review and editing of drafts, visualization, and supervision of the project. All authors contributed to the article and approved the submitted version.
Funding
The work presented here was funded largely by the Hong Kong Airport Authority and Hong Kong Civil Engineering and Development Department.
Conflict of Interest
TJ was associated with the company Clymene Enterprises (sole proprietorship).
The remaining authors declare that the research was conducted in the absence of any commercial or financial relationships that could be construed as a potential conflict of interest.
The reviewer HP declared a past co-authorship with one of the authors, SP, to the handling editor.
Acknowledgments
We thank Mott McDonald, Inc., Hong Kong for field logistics and data collection. We thank Dr. Blair Sterba-Boatwright for advice on statistical analyses. We also thank the Hong Kong Airport Authority, Ove Arup & Partners Hong Kong Limited, and the Hong Kong Civil Engineering and Development Department for access to data collected under contract. Portions of this work have previously appeared in a dissertation chapter (Piwetz, 2018).
References
AFCD (2020). Designated Marine Parks and Marine Reserve. Agriculture, Fisheries and Conservation Department of Hong Kong Special Administrative Region Government. Available online at: https://www.afcd.gov.hk/english/country/cou_vis/cou_vis_mar/cou_vis_mar.html (accessed April 19, 2020).
Altmann, J. (1974). Observational study of behavior: sampling methods. Behaviour 49, 227–266. doi: 10.1163/156853974X00534
Barros, N., and Cockcroft, V. (1999). “Prey resource partitioning between Indo-Pacific humpback dolphins (Sousa chinensis) and bottlenose dolphins (Tursiops truncatus) off South Africa: competitive exclusion or mutual tolerance,” in Proceedings of the 13th Biennial Conference on the Biology of Marine Mammals, Wailea, MI.
Barros, N. B., Jefferson, T. A., and Parsons, E. (2004). Feeding habits of Indo-Pacific humpback dolphins (Sousa chinensis) stranded in Hong Kong. Aquat. Mamm. 30, 179–188. doi: 10.1578/AM.30.1.2004.179
Beck, M., Heck, K. Jr., Able, K., Childers, D., Eggleston, D., Gillanders, B., et al. (2001). The identification, conservation, and management of estuarine and marine nurseries for fish and invertebrates: a better understanding of the habitats that serve as nurseries for marine species and the factors that create site-specific variability in nursery quality will improve conservation and management of these areas. Bioscience 51, 633–641. doi: 10.1641/0006-3568(2001)051[0633:TICAMO]2.0.CO;2
Bejder, L. (2005). Linking Short and Long-Term Effects of Nature-Based Tourism on Cetaceans. Doctor of Philosophy thesis, Dalhousie University, Halifax, NS, 173.
Bejder, L., Dawson, S., and Harraway, J. (1999). Responses by Hector’s dolphins to boats and swimmers in Porpoise Bay, New Zealand. Mar. Mamm. Sci. 15, 738–750. doi: 10.1111/j.1748-7692.1999.tb00840.x
Bejder, L., Samuels, A., Whitehead, H., Finn, H., and Allen, S. (2009). Impact assessment research: use and misuse of habituation, sensitisation and tolerance in describing wildlife responses to anthropogenic stimuli. Mar. Ecol. Prog. Ser. 395, 177–185. doi: 10.3354/meps07979
Brandt, M., Diederichs, A., Betke, K., and Nehls, G. (2011). Responses of harbour porpoises to pile driving at the Horns Rev II offshore wind farm in the Danish North Sea. Mar. Ecol. Prog. Ser. 421, 205–216. doi: 10.3354/meps08888
Burnham, K., and Anderson, D. (2004). Multimodel inference understanding AIC and BIC in model selection. Sociol. Methods Res. 33, 261–304. doi: 10.1177/0049124104268644
Chen, T., Hung, S., Qiu, Y., Jia, X., and Jefferson, T. (2010). Distribution, abundance, and individual movements of Indo-Pacific humpback dolphins (Sousa chinensis) in the Pearl River Estuary, China. Mammalia 74, 117–125. doi: 10.1515/mamm.2010.024
Chilvers, B., Lawler, I., Macknight, F., Marsh, H., Noad, M., and Paterson, R. (2005). Moreton Bay, Queensland, Australia: an example of the co-existence of significant marine mammal populations and large-scale coastal development. Biol. Conserv. 122, 559–571. doi: 10.1016/j.biocon.2004.08.013
Constantine, R. (2001). Increased avoidance of swimmers by wild bottlenose dolphins (Tursiops truncatus) due to long-term exposure to swim-with-dolphin tourism. Mar. Mamm. Sci. 17, 689–702. doi: 10.1111/j.1748-7692.2001.tb01293.x
Culloch, R., Anderwald, P., Brandecker, A., Haberlin, D., McGovern, B., Pinfield, R., et al. (2016). Effect of construction-related activities and vessel traffic on marine mammals. Mar. Ecol. Prog. Ser. 549, 231–242. doi: 10.3354/meps11686
Dray, S., Royer-Carenzi, M., and Calenge, C. (2010). The exploratory analysis of autocorrelation in animal-movement studies. Ecol. Res. 25, 673–681. doi: 10.1007/s11284-010-0701-7
Dungan, S., Hung, S., Wang, J., and White, B. (2012). Two social communities in the Pearl River Estuary population of Indo-Pacific humpback dolphins (Sousa chinensis). Can. J. Zool. 90, 1031–1043. doi: 10.1139/z2012-071
Erbe, C., Williams, R., Parsons, M., Parsons, S., Hendrawan, I., and Dewantama, I. (2018). Underwater noise from airplanes: an overlooked source of ocean noise. Mar. Pollut. Bull. 137, 656–661. doi: 10.1016/j.marpolbul.2018.10.064
Forney, K., Southall, B., Slooten, E., Dawson, S., Read, A., Baird, R., et al. (2017). Nowhere to go: noise impact assessments for marine mammal populations with high site fidelity. Endanger. Spec. Res. 32, 391–413. doi: 10.3354/esr00820
Gailey, G., and Ortega-Ortiz, J. (2002). A note on a computer-based system for theodolite tracking of cetaceans. J. Cetacean Res. Manag. 4, 213–218. doi: 10.1007/s10661-007-9812-1
Gailey, G., Würsig, B., and McDonald, T. (2007). Abundance, behavior, and movement patterns of western gray whales in relation to a 3-D seismic survey, Northeast Sakhalin Island, Russia. Environ. Monit. Assess. 134, 75–91.
GHKSAR (2021). List of marine works in progress in Hong Kong, in The Government of the Hong Kong Special Administrative Region, Marine Department. Available online at: https://www.mardep.gov.hk/en/pub_services/worklist.html (accessed January 17, 2021).
Hastie, T., and Tibshirani, R. (1986). Generalized additive models. Stat. Sci. 1, 297–310. doi: 10.1214/ss/1177013604
Hoyt, E. (2011). Marine Protected Areas for Whales Dolphins and Porpoises: a World Handbook for Cetacean Habitat Conservation, 2nd Edn, Abingdon, OX: Earthscan. doi: 10.4324/9781849775557
Hu, M., Pavlicova, M., and Nunes, E. (2011). Zero-inflated and hurdle models of count data with extra zeros: examples from an HIV-risk reduction intervention trial. Am. J. Drug Alcohol Abuse 37, 367–375. doi: 10.3109/00952990.2011.597280
Huang, S.-L., Karczmarski, L., Chen, J., Zhou, R., Lin, W., Zhang, H., et al. (2012). Demography and population trends of the largest population of Indo-Pacific humpback dolphins. Biol. Conserv. 147, 234–242. doi: 10.1016/j.biocon.2012.01.004
Hung, S. (2008). Habitat Use of Indo-Pacific Humpback Dolphins (Sousa chinensis) in Hong Kong. Doctor of Philosophy thesis, The University of Hong Kong, Pok Fu Lam, 253.
Hung, S. (2014). Monitoring of Marine Mammals in Hong Kong Waters (2013-14). in the Final Report to the Agriculture, Fisheries and Conservation Department of Hong Kong SAR Government, Hong Kong, 1-198.
Hung, S. (2015). Monitoring of marine mammals in Hong Kong Waters (2014-15). in: Final Report to the Agriculture, Fisheries and Conservation Department of Hong Kong SAR Government. Hong Kong. p 1-151.
Hung, S. (2017). Monitoring of Marine Mammals in Hong Kong Waters (2016-17). In: Final Report to the Agriculture, Fisheries and Conservation Department of Hong Kong SAR Government. Hong Kong. p 1-162.
Hurvich, C., and Tsai, C. (1989). Regression and time series model selection in small samples. Biometrika 76, 297–307. doi: 10.1093/biomet/76.2.297
Jackman, S. (2020). pscl: Classes and Methods for R Developed in the Political Science Computational Laboratory. New South Wales: United States Studies Centre, University of Sydney.
Jefferson, T. (2000). Population biology of the Indo-Pacific hump-backed dolphin in Hong Kong waters. Wildl. Monogr. 144, 1–65.
Jefferson, T. (2018). Hong Kong’s Indo-Pacific humpback dolphins (Sousa chinensis): assessing past and future anthropogenic impacts and working toward sustainability. Aquat. Mamm. 44, 711–728. doi: 10.1578/AM.44.6.2018.711
Jefferson, T., and Curry, B. (2015). “Humpback dolphins: a Brief Introduction to the Genus Sousa,” in Advances in Marine Biology, eds T. Jefferson and B. Curry (Oxford: Academic Press), 1–16. doi: 10.1016/bs.amb.2015.04.001
Jefferson, T., and Hung, S. (2004). A review of the status of the Indo-Pacific humpback dolphin (Sousa chinensis) in Chinese waters. Aquat. Mamm. 30, 149–158. doi: 10.1578/AM.30.1.2004.149
Jefferson, T., Hung, S., and Lam, P. (2006). Strandings, mortality and morbidity of Indo-Pacific humpback dolphins in Hong Kong, with emphasis on the role of organochlorine contaminants. J. Cetacean Res. Manag. 8, 181–193.
Jefferson, T., Hung, S., Robertson, K., and Archer, F. (2012). Life history of the Indo-Pacific humpback dolphin in the Pearl River Estuary, southern China. Mar. Mamm. Sci. 28, 84–104. doi: 10.1111/j.1748-7692.2010.00462.x
Jefferson, T., Hung, S., and Würsig, B. (2009). Protecting small cetaceans from coastal development: impact assessment and mitigation experience in Hong Kong. Mar. Policy 33, 305–311. doi: 10.1016/j.marpol.2008.07.011
Jefferson, T., and Rosenbaum, H. (2014). Taxonomic revision of the humpback dolphins (Sousa spp.), and description of a new species from Australia. Mar. Mamm. Sci. 30, 1494–1541. doi: 10.1111/mms.12152
Jefferson, T., and Smith, B. (2016). “Re-assessment of the conservation status of the Indo-Pacific humpback dolphin (Sousa chinensis) using the IUCN Red List Criteria,” in Advances in Marine Biology, eds T. A. Jefferson and B. E. Curry (Oxford: Academic Press), 1–26. doi: 10.1016/bs.amb.2015.04.002
Jefferson, T., Smith, B., Braulik, G., and Perrin, W. (2017). Sousa chinensis (errata version published in 2018). IUCN Red List Threat. Spec. 2017:e.T82031425A123794774. doi: 10.2305/IUCN.UK.2017-3.RLTS.T82031425A50372332.en
Jefferson, T., Smultea, M., Courbis, S., and Campbell, G. (2016). Harbor porpoise (Phocoena phocoena) recovery in the inland waters of Washington: estimates of density and abundance from aerial surveys, 2013-2015. Can. J. Zool. 94, 505–515. doi: 10.1139/cjz-2015-0236
Jones, M., Swartz, S., and Dahlheim, M. (1994). Census of Gray Whale Abundance in San Ignacio Lagoon: a Follow-Up Study in Response to Low Whale Counts Recorded During an Acoustic Playback Study of Noise Effects on Gray Whales. Rep No NTIS PB94195062. Washington, DC: US Marine Mammal Commission.
Karczmarski, L., and Cockcroft, V. (1999). Daylight behaviour of humpback dolphins Sousa chinensis in Algoa Bay, South Africa. Zeitschrift Säugetierkunde 64, 19–29.
Karczmarski, L., Huang, S.-L., and Chan, S. (2017). Threshold of long-term survival of a coastal delphinid in anthropogenically degraded environment: Indo-Pacific humpback dolphins in Pearl River Delta. Sci. Rep. 7:42900. doi: 10.1038/srep42900
Karczmarski, L., Huang, S.-L., Or, C., Gui, D., Chan, S., Lin, W., et al. (2016). “Humpback dolphins in Hong Kong and the Pearl River Delta: status, threats, and conservation challenges,” in Humpback Dolphins (Sousa spp.): Current Status and Conservation, Part 2: Advances in Marine Biology, eds T. A. Jefferson and B. E. Curry (Amsterdam: Elsevier), 27–64.
Ketten, D. (2004). Marine mammal auditory systems: a summary of audiometric and anatomical data and implications for underwater acoustic impacts. Polarforschung 72, 79–92.
Li, S., Wang, D., Wang, K., Taylor, E., Cros, E., Shi, W., et al. (2012). Evoked-potential audiogram of an Indo-Pacific humpback dolphin (Sousa chinensis). J. Exper. Biol. 215, 3055–3063. doi: 10.1242/jeb.070904
Lundquist, D., Gemmell, N., and Würsig, B. (2012). Behavioural responses of dusky dolphin groups (Lagenorhynchus obscurus) to tour vessels off Kaikoura, New Zealand. PLoS One 7:e41969. doi: 10.1371/journal.pone.0041969
Lundquist, D., Gemmell, N., Würsig, B., and Markowitz, T. (2013). Dusky dolphin movement patterns: short-term effects of tourism. N. Z. J. Mar. Freshwat. Res. 47, 430–449. doi: 10.1080/00288330.2013.778301
Madsen, P., Wahlberg, M., Tougaard, J., Lucke, K., and Tyack, P. (2006). Wind turbine underwater noise and marine mammals: implications of current knowledge and data needs. Mar. Ecol. Prog. Ser. 309, 279–295. doi: 10.3354/meps309279
Mann, J. (1999). Behavioral sampling methods for cetaceans: a review and critique. Mar. Mamm. Sci. 15, 102–122. doi: 10.1111/j.1748-7692.1999.tb00784.x
Mann, J., Connor, R., Barre, L., and Heithaus, M. (2000). Female reproductive success in bottlenose dolphins (Tursiops sp.): life history, habitat, provisioning, and group-size effects. Behav. Ecol. 11, 210–219. doi: 10.1093/beheco/11.2.210
Marcotte, D., Hung, S., and Caquard, S. (2015). Mapping cumulative impacts on Hong Kong’s pink dolphin population. Ocean Coast Manag. 109, 51–63. doi: 10.1016/j.ocecoaman.2015.02.002
Marley, S., Salgado Kent, C., and Erbe, C. (2016). Occupancy of bottlenose dolphins (Tursiops aduncus) in relation to vessel traffic, dredging, and environmental variables within a highly urbanised estuary. Hydrobiologia 792, 243–263. doi: 10.1007/s10750-016-3061-7
Martinez, E. (2010). Responses of South Island Hector’s Dolphins (Cephalorhynchus hectori hectori) to Vessel Activity (including Tourism Operations) in Akaroa Harbour, Banks Peninsula, New Zealand. Doctor of Philosophy thesis, Massey University, Auckland, 415.
Mendez, M., Subramaniam, A., Collins, T., Minton, G., Baldwin, R., Berggren, P., et al. (2011). Molecular ecology meets remote sensing: environmental drivers to population structure of humpback dolphins in the Western Indian Ocean. Heredity 107, 349–361. doi: 10.1038/hdy.2011.21
Munger, L., Lammers, M., Cifuentes, M., Würsig, B., Jefferson, T., and Hung, S. (2016). Indo-Pacific humpback dolphin occurrence north of Lantau Island, Hong Kong, based on year-round passive acoustic monitoring. J. Acoust. Soc. Am. 140, 2754–2765. doi: 10.1121/1.4963874
Munger, L., Piwetz, S., Lammers, M. O., and Wursig, B. (2018). Passive acoustic monitoring and concurrent theodolite observations of Indo-Pacific humpback dolphins (Sousa chinensis) in Hong Kong: a case study. Aquat. Mamm. 44, 729–736. doi: 10.1578/AM.44.6.2018.729
Ng, S., and Leung, S. (2003). Behavioral response of Indo-Pacific humpback dolphin (Sousa chinensis) to vessel traffic. Mar. Environ. Res. 56, 555–567. doi: 10.1016/S0141-1136(03)00041-2
Parra, G., and Ross, G. (2009). “Humpback dolphins,” in The Encyclopedia of Marine Mammals, 2nd Edn, eds W. Perrin, B. Würsig, and J. Thewissen (San Francisco, CA: Elsevier), 576–582. doi: 10.1016/B978-0-12-373553-9.00134-6
Parsons, E. (1997). Hong Kong’s Cetaceans: The Biology, Socioecology and Behaviour of Sousa chinensis and Neophocaena phocaenoides. Doctor of Philosophy thesis, The University of Hong Kong, Pok Fu Lam, 271.
Parsons, E. (2004). The behavior and ecology of the Indo-Pacific humpback dolphin (Sousa chinensis). Aquat. Mamm. 30, 38–55. doi: 10.1578/AM.30.1.2004.38
Pilleri, G., and Gihr, M. (1974). Contribution to the knowledge of the cetaceans of southwest and monsoon Asia (Persian Gulf, Indus Delta, Malabar, Andaman Sea and Gulf of Siam). Investig. Cetacea 5, 95–149.
Pirotta, E., Laesser, B., Hardaker, A., Riddoch, N., Marcoux, M., and Lusseau, D. (2013). Dredging displaces bottlenose dolphins from an urbanised foraging patch. Mar. Pollut. Bull. 74, 396–402. doi: 10.1016/j.marpolbul.2013.06.020
Piwetz, S. (2018). Effects of Human Activities on Coastal Dolphin Behavior. Department of Marine Biology thesis, Texas A&M University at Galveston, Galveston, 160.
Piwetz, S., Gailey, G., Munger, L., Lammers, M., Jefferson, T., and Würsig, B. (2018). Theodolite tracking in marine mammal research: from Roger Payne to the present. Aquat. Mamm. 44:683. doi: 10.1578/AM.44.6.2018.683
Ramcharitar, J., Gannon, D., and Popper, A. (2006). Bioacoustics of fishes of the family Sciaenidae (croakers and drums). Trans. Am. Fish. Soc. 135, 1409–1431. doi: 10.1577/T05-207.1
Reeves, R. (2009). “Conservation efforts,” in The Encyclopedia of Marine Mammals, 2nd Edn, eds W. F. Perrin, B. Würsig, and J. G. M. Thewissen (San Francisco, CA: Elsevier), 275–289. doi: 10.1016/B978-0-12-373553-9.00066-3
Reeves, R., Dalebout, M., Jefferson, T., Karczmarski, L., Laidre, K. L., O’Corry-Crowe, G., et al. (2008). Sousa chinensis. IUCN Red List Threat. Spec. doi: 10.2305/IUCN.UK.2008.RLTS.T20424A9197694.en
Reine, K., Clarke, D., and Dickerson, C. (2014). Characterization of underwater sounds produced by hydraulic and mechanical dredging operations. J. Acoust. Soc. Am. 135, 3280–3294. doi: 10.1121/1.4875712
Richardson, W., Greene, C. Jr., Malme, C., and Thomson, D. (1995). Marine Mammals and Noise. San Diego: Academic Press, 576. doi: 10.1016/B978-0-08-057303-8.50011-2
Richardson, W. J., and Würsig, B. (1997). Influences of man-made noise and other human actions on cetacean behaviour. Mar. Freshw. Behav. Physiol. 29, 183–209. doi: 10.1080/10236249709379006
Shelton, B., Karakiewicz, J., and Kvan, T. (2013). The Making of Hong Kong: from Vertical to Volumetric. New York, NY: Routledge, 183.
Sims, P., Hung, S., and Würsig, B. (2012). High-speed vessel noises in West Hong Kong waters and their contributions relative to Indo-Pacific humpback dolphins (Sousa chinensis). J. Mar. Biolo. 2012, 1–11. doi: 10.1155/2012/169103
Smolker, R., Richards, A., Conner, R., and Pepper, J. (1992). Sex differences in patterns of association among Indian Ocean bottlenose dolphins. Behaviour 123, 38–69. doi: 10.1163/156853992X00101
Smultea, M., and Würsig, B. (1995). Behavioral reactions of bottlenose dolphins to the Mega Borg oil spill, Gulf of Mexico 1990. Aquat. Mamm. 21, 171–181.
Thewissen, J. (2009). “Sensory biology: overview,” in The Encyclopedia of Marine Mammals, 2nd Edn, eds W. Perrin, B. Würsig, and J. Thewissen (San Francisco, CA: Elsevier), 1003–1005. doi: 10.1016/B978-0-12-373553-9.00232-7
Thompson, P., Brookes, K., Graham, I., Barton, T., Needham, K., Bradbury, G., et al. (2013). Short-term disturbance by a commercial two-dimensional seismic survey does not lead to long-term displacement of harbour porpoises. Proc. R. Soc. B Biol. Sci. 280:20132001. doi: 10.1098/rspb.2013.2001
Thomsen, F., Laczny, M., and Piper, W. (2006). A recovery of harbour porpoises (Phocoena phocoena) in the southern North Sea? A case study off Eastern Frisia, Germany. Helgol. Mar. Res. 60, 189–195. doi: 10.1007/s10152-006-0021-z
Thomsen, F., McCully, S., Wood, D., Pace, F., and White, P. (2009). A Generic Investigation into Noise Profiles of Marine Dredging in Relation to the Acoustic Sensitivity of the Marine Fauna in UK Waters with Particular Emphasis on Aggregate Dredging: PHASE 1 Scoping and Review of Key Issues. Suffolk: Centre for Environment, Fisheries & Aquaculture Science, 61.
Todd, V., Todd, I., Gardiner, J., Morrin, E., MacPherson, N., DiMarzio, N., et al. (2014). A review of impacts of marine dredging activities on marine mammals. ICES J. Mar. Sci. 72, 328–340. doi: 10.1093/icesjms/fsu187
Turvey, S., Pitman, R., Taylor, B., Barlow, J., Akamatsu, T., Barrett, L., et al. (2007). First human-caused extinction of a cetacean species? Biol. Lett. 3, 537–540. doi: 10.1098/rsbl.2007.0292
Wang, P., and Sun, J. (1982). Studies on the Zhonghua white dolphin, Sousa chinensis from the South China Sea. Trans. Liaoning Zool. Soc. 3, 67–74.
Wang, Z., Wu, Y., Duan, G., Cao, H., Liu, J., Wang, K., et al. (2014). Assessing the underwater acoustics of the world’s largest vibration hammer (OCTA-KONG) and its potential effects on the Indo-Pacific humpbacked dolphin (Sousa chinensis). PLoS One 9:e110590. doi: 10.1371/journal.pone.0110590
Weir, C., Van Waerebeek, K., Jefferson, T., and Collins, T. (2010). “Challenges and priorities for the conservation of the Vulnerable Atlantic humpback dolphin (Sousa teuszii), with a case study from Namibe Province, Angola,” in Proceedings of the IWC Scientific Committee Document SC/62/SMx, Agadir, MR, 1–17.
Williams, R., and Ashe, E. (2007). Killer whale evasive tactics vary with boat number. J. Zool. 272, 390–397. doi: 10.1111/j.1469-7998.2006.00280.x
Wood, S. (2006). Generalized Additive Models: An Introduction with R. Boca Raton, FL: CRC Press. doi: 10.1201/9781420010404
Würsig, B., Cipriano, F., and Würsig, M. (1991). “Dolphin movement patterns: information from radio and theodolite tracking studies,” in Dolphin Societies: Discoveries and Puzzles, eds K. Pryor and K. S. Norris (Berkeley: University of California Press), 79–111.
Appendix
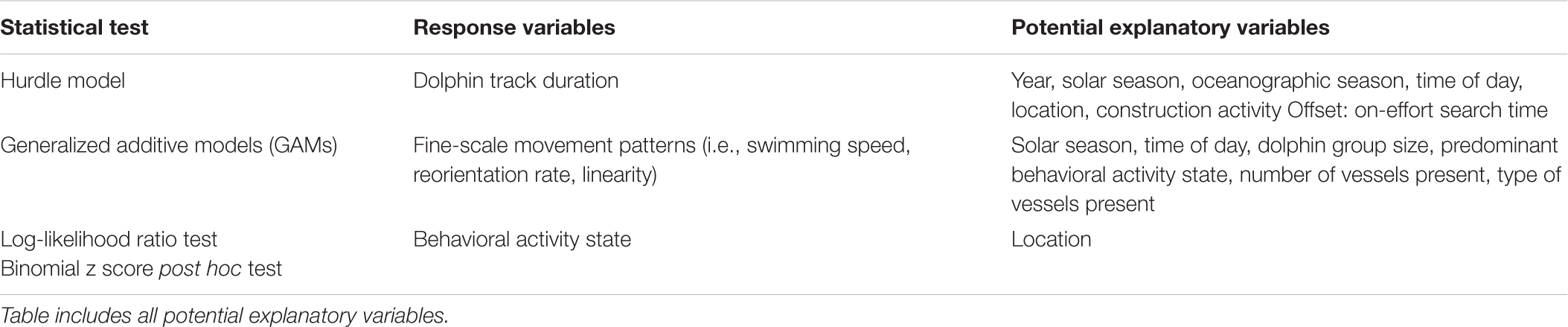
Appendix Table 2 Summary of statistical tests used to analyze Indo-Pacific humpback dolphin track duration, fine-scale movement patterns, and behavioral activity state.
Keywords: Sousa chinensis, habitat-use, movement patterns, behavior, construction, vessel activity, Hong Kong
Citation: Piwetz S, Jefferson TA and Würsig B (2021) Effects of Coastal Construction on Indo-Pacific Humpback Dolphin (Sousa chinensis) Behavior and Habitat-Use Off Hong Kong. Front. Mar. Sci. 8:572535. doi: 10.3389/fmars.2021.572535
Received: 14 June 2020; Accepted: 15 February 2021;
Published: 04 March 2021.
Edited by:
Karen A. Stockin, Massey University, New ZealandReviewed by:
Phil J. Bouchet, University of St Andrews, United KingdomHeidi Christine Pearson, University of Alaska Southeast, United States
Krista Hupman, National Institute of Water and Atmospheric Research (NIWA), New Zealand
Copyright © 2021 Piwetz, Jefferson and Würsig. This is an open-access article distributed under the terms of the Creative Commons Attribution License (CC BY). The use, distribution or reproduction in other forums is permitted, provided the original author(s) and the copyright owner(s) are credited and that the original publication in this journal is cited, in accordance with accepted academic practice. No use, distribution or reproduction is permitted which does not comply with these terms.
*Correspondence: Sarah Piwetz, spiwetz@tmmsn.org; sarahpiwetz@gmail.com