- 1Posgrado en Ciencias Químico Biológicas, Facultad de Química, Universidad Autónoma de Querétaro, Santiago de Querétaro, Mexico
- 2Databiology Ltd., Oxford, United Kingdom
- 3Laboratorio de Investigación Química y Farmacológica de Productos Naturales, Facultad de Química, Universidad Autónoma de Querétaro, Santiago de Querétaro, Mexico
Introduction
Hydrocorals from the genus Millepora (Phylum Cnidaria, Class Hydrozoa) are conspicuous organisms commonly found in shallow waters of tropical regions. These cnidarians, which are considered the second reef builders in the world (Rojas-Molina et al., 2012), are vital components of marine ecosystems and provide habitat for many coral reef-dependent communities (Lewis, 2006).
The genus Millepora includes calcareous hydroids that are not classified in the group of true stony corals (Radwan and Aboul-Dahab, 2004). Milleporids are commonly known as “fire corals” due to their ability to inflict painful wounds in human epithelial tissues through the secretion of toxins (Radwan, 2002), which are stored in the nematocysts (Shiomi et al., 1989; David et al., 2008).
Millepora complanata is a common resident of the Mesoamerican Reef System in the Mexican Caribbean (Ibarra-Alvarado et al., 2007). This organism inhabits shallow water and the reef crest (Stromgren, 1976) where it forms plate-like colonies composed of multiple clones (Lewis, 1991). Like many scleractinian corals and other cnidarians, M. complanata lives in mutualistic symbiosis with dinoflagellate algae of the family Symbiodiniaceae (commonly known as zooxanthellae) (Davy et al., 2012).
Dinoflagellates reside in the symbiosomes, which are phagosome-derived vacuoles within cnidarian gastrodermal cells (Lehnert et al., 2014). Symbiodiniaceae algae provide photosynthetic fixed carbon to their host, in exchange, cnidarians supply their symbionts with nitrogenous compounds (Sproles et al., 2018). Within host tissues, coral-associated symbionts may reach population densities of several millions per square centimeter and the holobiont may contain more than one Symbiodiniaceae genus (LaJeunesse, 2002). Diverse studies have analyzed the symbiotic relationship between stony corals (class Anthozoa) and their symbionts (Furla et al., 2005; Davy et al., 2012; Gusmão et al., 2020; Tivey et al., 2020; Xiang et al., 2020). However, hydrocoral-Symbiodiniaceae symbiosis is poorly understood. Investigations on the mutualistic relationship between reef-building cnidarians and Symbiodiniaceae algae have increased in the “omics age” (Meyer and Weis, 2012; Bi et al., 2019; Simona, 2019) and the sea anemone Exaiptasia diaphana, formerly known as Aiptasia pallida, has been widely employed as a model to study cnidarian endosymbiosis (Lehnert et al., 2012; Tortorelli et al., 2020). A gene expression analysis of cnidarian-algae association in Anthopleura elegantissima evidenced a complex modulation of host gene expression related to oxidative stress, apoptosis, cell proliferation, cell adhesion, and lipid metabolism (Rodriguez-Lanetty et al., 2006).
It has been demonstrated that reef-building cnidarians are also associated with a diverse community of bacteria and other microorganisms (Tandon et al., 2020). These microorganisms are key components of the coral holobiont and play a major role in nutrient cycling, which is fundamental for reef development (Rajasabapathy et al., 2020).
Environmental stressors, such as UV radiation and high temperature can lead to “bleaching,” the disruption of the symbiosis between cnidarians and Symbiodiniaceae (Hughes et al., 2017). Unfortunately, the frequency and seriousness with which bleaching occurs have increased in recent years, seriously compromising coral populations around the world (Sully et al., 2019). Considering that perturbations induced by climate change have threatened the integrity and resilience of marine ecosystems, several investigations have analyzed the bleaching phenomenon, using transcriptomic approaches to understand the molecular mechanisms underlying the responses of cnidarians and symbionts to heat stress (Mayfield et al., 2011, 2014; Pinzón et al., 2015).
Investigations aimed at characterizing the transcriptomes of reef-building cnidarians have focused on species of the class Anthozoa (Kitchen et al., 2015; Kenkel and Bay, 2017; Pootakham et al., 2018; Veglia et al., 2018; Rivera-García et al., 2019; Ryu et al., 2019; Chiu et al., 2020). Regarding organisms of the class Hydrozoa, analysis of the transcriptomes from Hydractinia echinata and Hydra vulgaris showed the presence of evolutionary-conserved proteins and hitherto undescribed sequences in metazoans (Soza-Ried et al., 2010; Wenger and Galliot, 2013). However, with respect to de novo transcriptome assemblies of cnidarians of the genus Millepora, only one study has been reported on the branching hydrocoral M. alcicornis from the Caribbean (Ortiz-González et al., 2017).
RNA-seq-based transcriptomic approaches have become powerful tools directed toward recognizing all the expressed genes codified by a genome under certain conditions. Additionally, generation of new gene catalogs enables identification of ortholog sequences across related taxa. In this study, we used a metatranscriptomic sequencing approach to describe, for first time, the metatranscriptome of M. complanata and its associate symbionts in order to generate a new reference metatranscriptome of reef-building cnidarians of the class Hydrozoa.
Data Description
Sample Collection
Fragments of M. complanata colonies (~10 cm) were collected by SCUBA (Self-Contained Underwater Breathing Apparatus) diving at the Parque Nacional Arrecife located in Puerto Morelos, Quintana Roo, México (21°00′00′′ and 20°48′33′′ North latitude and 86°53′14.40′′ and 86°46′38.94′′ West longitude), during November 2016 (Permit No PFP/DGOPA-139/15). Samples were immediately frozen in liquid nitrogen and transported to the “Laboratorio de Investigación Química y Farmacológica de Productos Naturales in the Universidad Autónoma de Querétaro,” Queretaro, Mexico for RNA isolation.
RNA Extraction
Total RNA from three biologically independent M. complanata colonies was extracted according to Valderrama-Cháirez et al. (2002) with some modifications. Briefly, 0.1 g of hydrocoral tissue (previously ground with mortar and pestle in liquid nitrogen) was mixed with 1 mL of extraction buffer (150 mM Tris base, 2% SDS, 1% 2-mercaptoethanol, and 100 mM EDTA adjusted to pH 7.5 with saturated boric acid). Afterward, 100 μL of potassium acetate 5 M, 250 μL of absolute ethanol, and 500 μL of chloroform-isoamyl alcohol (25:1) were added and mixed by vortex for 1 min. Samples were centrifuged at 12.0E3 rpm for 10 min, the top layer was removed and 500 μL of lithium chloride 8 M were added. Samples were stored overnight. Extracted RNA was precipitated by centrifugation at 12.0E3 rpm for 10 min, washed with 250 μL of ethanol 70% and re-suspended in sterile water. Isolated RNA was resolved with a 4% agarose electrophoresis gel (run conditions: 75 V during 60 min) and the RNA integrity number was measured with an Agilent™ Eukaryote Total RNA Nano chip. Run results generated by the Nexseq500 sequencing system yielded 39.18 Gbp with high quality reads (78.43% ≥ Q30) in 2 × 150 medium performance plate.
cDNA Libraries Preparation, Sequencing, and Metatranscriptome Assembly
RNA samples were sent to the “Laboratorio de Servicios Genómicos,” LANGEBIO CINVESTAV-Campus Irapuato for cDNA libraries preparation with Truseq RNA sample prep v2 of Illumina, according to the manufacturer's instructions. Subsequently, cDNA libraries were sequenced on the Illumina NextSeq500™ platform (2x150) generating 35 GB and 120 million reads. Quality control of raw reads was assessed using FASTQC (Brown et al., 2017), none of the libraries showed any issue. Each library was independently assembled with rnaSPAdes (Bankevich et al., 2012). To improve the accuracy of isoform reconstruction after initial assembly, all sample contigs were merged to reduce sequence redundancy using CD-HIT-EST (Li and Godzik, 2006). A unique identifier was assigned to each FASTA sequence of the assembled transcriptome. Raw data obtained from the sequencing was submitted to the NCBI Sequence Read Archive (SRA) database (Bioproject accession: PRJNA524427; Biosample accession: SAMN11026413). Pair-end reads yield displayed as a maximum 16,431,435 reads. FASTQC analysis of all samples showed a GC content of 40.3%. Assembled transcripts resulted in an N50 value of 510 and an average contig length of 480, whereas statistics of transcriptome assemblies previously obtained from M. alcicornis showed an N50 value of 749 and an average contig length of 550 (Ortiz-González et al., 2017). Metatranscriptomic contigs were annotated in order to separate cnidarian and symbiont transcripts.
Splitting of the Transcripts and Transcriptome Completeness Estimation
Sequences from M. complanata and Symbiodiniaceae were classified by comparing two custom databases. A cnidarian database was constructed using the transcriptomes from Millepora alcicornis (ID GFAS01, BIOPROJECT PRJNA344421), Hydra vulgaris (ID GGKF01, BIOPROJECT PRJNA399692), Millepora squarrosa (ID GFGU01, BIOPROJECT PRJNA350711), and Nematostella vectensis (ID HADP0, BIOPROJECT PRJEB13676). On the other hand, a database containing the following Symbiodiniaceae transcriptomes was created: Symbiodinium sp. (clade A1, ID GAKY01, BIOPROJECT PRJNA206215), Symbiodinium sp. (clade A2, ID GBGW01, BIOPROJECT PRJNA237994), Breviolum sp. (clade B2, ID GBRZ01, BIOPROJECT PRJNA260330), Cladocopium sp. (clade C, ID GBSC01, PRJNA260340), Cladocopium sp. (clade C, ID GAFO01, PRJNA175601), uncultured Cladocopium sp. (clade C, ID IADM01, BIOPROJECT PRJDB4715), Durisdinium sp. (clade D, ID GAFP01, BIOPROJECT PRJNA175600), Durisdinium sp. (clade D, ID GBRR01, BIOPROJECT PRJNA260333), uncultured Durisdinium sp. (clade D, ID IADN01, BIOPROJECT PRJDB4715), and Symbiodinium muscatinei (ID GFDR03, BIOPROJECT PRJNA359147). M. complanata metatranscriptome was compared against the customized cnidarian and Symbiodiniaceae databases using Blastn. Python scripts were used for splitting and retrieving confidently identified sequences (e-value threshold of 1.0E-20) from M. complanata and Symbiodiniaceae. This procedure enabled assigment of 60, 907 (42,6%) sequences to M. complanata, and 61, 139 (42.8%) sequences to Symbiodiniaceae algae. Completeness of the separated transcriptome sequences was assessed with BUSCO using the Eukaryota database (Simão et al., 2015). Sequences assigned to Cnidaria possessed 75.3% of orthologs from Eukaryota core, whereas Symbiodiniaceae sequences displayed 51.8%. Figure 1 shows the relative contribution of M. complanata and Symbiodiniaceae algae to the assembly. Statistics of split sequences showed that M. complanata sequences possessed 36.8% GC content and a maximum contig length of 5, 954 bp, whereas symbionts' transcripts exhibited 50.9% GC content and a maximum contig length of 6,744 bp (Figure 1A). GC content distribution and the length of split sequences are shown in Figure 1B. The assembled transcriptome was submitted to the Transcriptome Shotgun Assembly (TSA) project of NCBI. This TSA has been deposited in DDBJ/EMBL/GenBank under the accession GIXC00000000. The version described in this paper (GIXC01000000) is the first one.
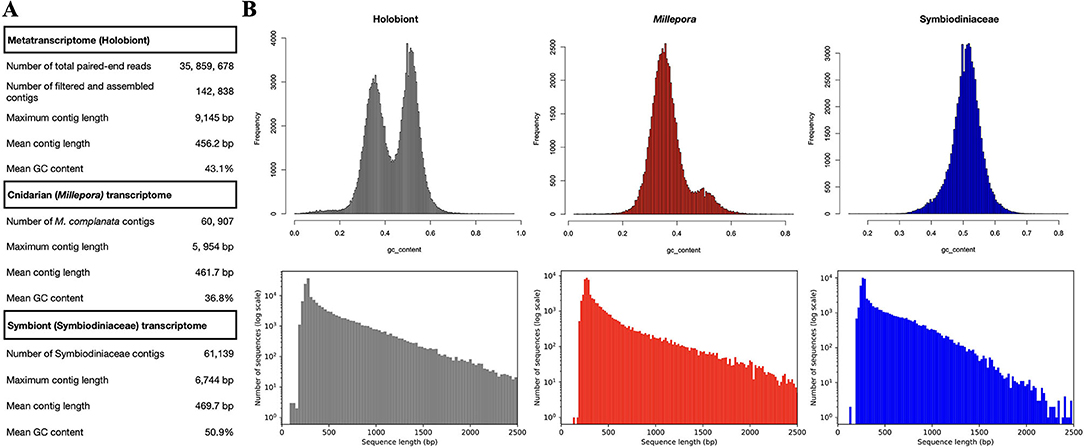
Figure 1. (A) M. complanata's metatranscriptome assembly statistics. (B) Relative contribution of M. complanata and Symbiodiniaceae algae to the assembly (differences in GC content and sequence length from split sequences).
Functional Annotation and Gene Ontology
All bioinformatic analyses were performed in the Databiology Lab platform (https://www.lab.databiology.net/). Sequences were annotated by sequence homology against the NCBI non-redundant database using Diamond (Buchfink et al., 2015) (https://www.ncbi.nlm.nih.gov/) (e-value threshold of 1.0E-6). The annotation of transcripts for M. complanata and Symbiodiniacea subsets are found in Supplementary Material (M_complanata_annotations.xlsx and Symbiont_annotations.xlsx). Gene Ontology (GO) terms were retrieved from Blastx hits with the Blast2GO software v. 5.2. Sequences were analyzed with “Mapping and Annotation.” GO were grouped into Biological Process (BP), Molecular Function (MF), and Cellular Component (CC) domains. GO annotations across sub-ontologies were visualized with WEGO 2.0 (https://wego.genomics.cn). The most over-represented GO terms across sub-ontologies for M. complanata were “cell” with 53.2% (CC), “catalytic activity” with 47% (MF), and “cellular process” with 58% (BP). Whereas, the most prevalent GO terms for Symbiodiniacea were “membrane” with 48.7% (CC), “catalytic activity” with 58.4% (MF), and “metabolic process” with 49.2% (BP) (Figure 2).
Taxonomic Analysis
The assembled metatranscriptome was compared against the NCBI non-redundant database (https://www.ncbi.nlm.nih.gov/) using Diamond (Buchfink et al., 2015) (e-value threshold of 1.0E-6) and MEGAN v. 6.19.8 was employed to perform the taxonomic analysis (Huson et al., 2016). Taxonomy assignments resulted in 61, 604 hits, most of them were assigned to Dinophyceae (41.2%), Cnidaria (16.8%), and Proteobacteria (3.8%), the remaining percentage (37.2%) corresponded to non-dominant phyla such as Bacteroidetes, Actinobacteria, Firmicutes, Haptista, Ascomycota, Microsporidia, Chordata, Nematoda, Arthropoda, Mollusca, Placozoa, Ciliophora, Perkinsozoa, Bacillariophyta, Oomycota, Chlorophyta, and Streptophyta.
Novelty of Presented Data
We describe, for the first time, the metatranscriptome of the M. complanata holobiont. After assignment of transcripts, cnidarian and symbiont transcriptomes were classified either as Millepora (42.6%) or Symbiodiniaceae (42.8%). Over-represented Dinophyceae hits imply a dominance of symbiotic algae sequences, which indicates that Symbiodiniaceae algae represent a common characteristic of hydrocorals' holobionts. The information obtained from M. complanata metatranscriptome will be a key piece for understanding the cellular survival processes, phylogeny, and physiology of milleporids from the class Hydrozoa.
Data Availability Statement
The datasets presented in this study can be found in online repositories. The names of the repository/repositories and accession number(s) can be found at: https://www.ncbi.nlm.nih.gov/, PRJNA524427 and https://www.ncbi.nlm.nih.gov/genbank/, GIXC00000000.
Author Contributions
VH-E: conceptualization, investigation, formal analysis, and writing-original draft preparation. NO-L and RH-M: investigation. JC-P: supervision, formal analysis, review, and editing. CI-A: supervision, review, and editing. AR-M: conceptualization, project administration, funding acquisition, review, and editing. All authors contributed to the article and approved the submitted version.
Conflict of Interest
JC-P was employed by the company Databiology Ltd.
The remaining authors declare that the research was conducted in the absence of any commercial or financial relationships that could be construed as a potential conflict of interest.
Acknowledgments
VH-E acknowledges Consejo Nacional de Ciencia y Tecnología (CONACYT) for his graduate grant (CVU: 709502). The authors acknowledge computer facilities provided by Databiology Ltd. to perform all bioinformatic analyses.
Supplementary Material
The Supplementary Material for this article can be found online at: https://www.frontiersin.org/articles/10.3389/fmars.2021.566913/full#supplementary-material
References
Bankevich, A., Nurk, S., Antipov, D., Gurevich, A. A., Dvorkin, M., Kulikov, A. S., et al. (2012). SPAdes: a new genome assembly algorithm and its applications to single-cell sequencing. J. Comput. Biol. 19, 455–477. doi: 10.1089/cmb.2012.0021
Bi, Y., Wang, F., and Zhang, W. (2019). Omics analysis for dinoflagellates biology research. Microorganisms 7:288. doi: 10.3390/microorganisms7090288
Brown, J., Pirrung, M., and McCue, L. A. (2017). FQC Dashboard: integrates FastQC results into a web-based, interactive, and extensible FASTQ quality control tool. Bioinformatics 33, 3137–3139. doi: 10.1093/bioinformatics/btx373
Buchfink, B., Xie, C., and Huson, D. H. (2015). Fast and sensitive protein alignment using DIAMOND. Nat. Methods 12, 59–60. doi: 10.1038/nmeth.3176
Chiu, Y.-L., Shikina, S., Yoshioka, Y., Shinzato, C., and Chang, C.-F. (2020). The first de novo transcriptome assembly from the gonads of a scleractinian coral Euphyllia ancora: molecular mechanisms underlying scleractinian Gametogenesis. 21:732 doi: 10.21203/rs.3.rs-33092/v1
David, C. N., Özbek, S., Adamczyk, P., Meier, S., Pauly, B., Chapman, J., et al. (2008). Evolution of complex structures: minicollagens shape the cnidarian nematocyst. Trends Genet. 24, 431–438. doi: 10.1016/j.tig.2008.07.001
Davy, S. K., Allemand, D., and Weis, V. M. (2012). Cell biology of cnidarian-dinoflagellate symbiosis. Microbiol. Mol. Biol. Rev. 76, 229–261. doi: 10.1128/MMBR.05014-11
Furla, P., Allemand, D., Shick, J. M., Ferrier-Pagès, C., Richier, S., Plantivaux, A., et al. (2005). The symbiotic anthozoan: a physiological chimera between alga and animal. Integr. Comp. Biol. 45, 595–604. doi: 10.1093/icb/45.4.595
Gusmão, L. C., Van Deusen, V., Daly, M., and Rodríguez, E. (2020). Origin and evolution of the symbiosis between sea anemones (Cnidaria, Anthozoa, Actiniaria) and hermit crabs, with additional notes on anemone-gastropod associations. Mol. Phylogenet. Evol. 148:106805. doi: 10.1016/j.ympev.2020.106805
Hughes, T. P., Kerry, J. T., Álvarez-Noriega, M., Álvarez-Romero, J. G., Anderson, K. D., Baird, A. H., et al. (2017). Global warming and recurrent mass bleaching of corals. Nature 543, 373–377. doi: 10.1038/nature21707
Huson, D. H., Beier, S., Flade, I., Górska, A., El-Hadidi, M., Mitra, S., et al. (2016). MEGAN community edition-interactive exploration and analysis of large-scale microbiome sequencing data. PLoS Comput. Biol. 12:e1004957. doi: 10.1371/journal.pcbi.1004957
Ibarra-Alvarado, C., García, J. A., Aguilar, M. B., Rojas, A., Falcón, A., and de la Cotera, E. P. H. (2007). Biochemical and pharmacological characterization of toxins obtained from the fire coral Millepora complanata. Comp. Biochem. Physiol. Part C Toxicol. Pharmacol. 146, 511–518. doi: 10.1016/j.cbpc.2007.06.002
Kenkel, C. D., and Bay, L. K. (2017). Novel transcriptome resources for three scleractinian coral species from the Indo-Pacific. GigaScience 6:gix074. doi: 10.1093/gigascience/gix074
Kitchen, S. A., Crowder, C. M., Poole, A. Z., Weis, V. M., and Meyer, E. (2015). De novo assembly and characterization of four anthozoan (phylum Cnidaria) transcriptomes. G3 Genes Genomes Genet. 5, 2441–2452. doi: 10.1534/g3.115.020164
LaJeunesse, T. (2002). Diversity and community structure of symbiotic dinoflagellates from Caribbean coral reefs. Mar. Biol. 141, 387–400. doi: 10.1007/s00227-002-0829-2
Lehnert, E. M., Burriesci, M. S., and Pringle, J. R. (2012). Developing the anemone Aiptasia as a tractable model for cnidarian-dinoflagellate symbiosis: the transcriptome of aposymbiotic A. pallida. BMC Genomics 13:271. doi: 10.1186/1471-2164-13-271
Lehnert, E. M., Mouchka, M. E., Burriesci, M. S., Gallo, N. D., Schwarz, J. A., and Pringle, J. R. (2014). Extensive differences in gene expression between symbiotic and aposymbiotic cnidarians. Genes Genomes Genet. 4, 277–295. doi: 10.1534/g3.113.009084
Lewis, J. (1991). Testing the coral fragment size-dependent survivorship hypothesis for the calcareous hydrozoan Millepora complanata. Mar. Ecol. Prog. Ser. 70, 101–104. doi: 10.3354/meps070101
Lewis, J. B. (2006). Biology and ecology of the hydrocoral Millepora on coral reefs. Adv. Mar. Biol. 50, 1–55. doi: 10.1016/S0065-2881(05)50001-4
Li, W., and Godzik, A. (2006). Cd-hit: a fast program for clustering and comparing large sets of protein or nucleotide sequences. Bioinformatics 22, 1658–1659. doi: 10.1093/bioinformatics/btl158
Mayfield, A. B., Wang, L.-H., Tang, P.-C., Fan, T.-Y., Hsiao, Y.-Y., Tsai, C.-L., et al. (2011). Assessing the impacts of experimentally elevated temperature on the biological composition and molecular chaperone gene expression of a reef coral. PLoS ONE 6:e26529. doi: 10.1371/journal.pone.0026529
Mayfield, A. B., Wang, Y.-B., Chen, C.-S., Lin, C.-Y., and Chen, S.-H. (2014). Compartment-specific transcriptomics in a reef-building coral exposed to elevated temperatures. Mol. Ecol. 23, 5816–5830. doi: 10.1111/mec.12982
Meyer, E., and Weis, V. M. (2012). Study of cnidarian-algal symbiosis in the “omics” age. Biol. Bull. 223, 44–65. doi: 10.1086/BBLv223n1p44
Ortiz-González, I. C., Rivera-Vicéns, R. E., and Schizas, N. V. (2017). De novo transcriptome assembly of the hydrocoral Millepora alcicornis (branching fire coral) from the Caribbean. Mar. Genomics 32, 27–30. doi: 10.1016/j.margen.2016.11.005
Pinzón, J. H., Kamel, B., Burge, C. A., Harvell, C. D., Medina, M., Weil, E., et al. (2015). Whole transcriptome analysis reveals changes in expression of immune-related genes during and after bleaching in a reef-building coral. R. Soc. Open Sci. 2:140214. doi: 10.1098/rsos.140214
Pootakham, W., Naktang, C., Sonthirod, C., Yoocha, T., Sangsrakru, D., Jomchai, N., et al. (2018). Development of a novel reference transcriptome for scleractinian coral Porites lutea using single-molecule long-read isoform sequencing (Iso-Seq). Front. Mar. Sci. 5:122. doi: 10.3389/fmars.2018.00122
Radwan, F. F. (2002). Comparative toxinological and immunological studies on the nematocyst venoms of the Red Sea fire corals Millepora dichotoma and M. platyphylla. Comp. Biochem. Physiol. Part C Toxicol. Pharmacol. 131, 323–334. doi: 10.1016/S1532-0456(02)00017-0
Radwan, F. F., and Aboul-Dahab, H. M. (2004). Milleporin-1, a new phospholipase A 2 active protein from the fire coral Millepora platyphylla nematocysts. Comp. Biochem. Physiol. Part C Toxicol. Pharmacol. 139, 267–272. doi: 10.1016/j.cca.2004.12.002
Rajasabapathy, R., Ramasamy, K. P., Manikandan, B., Mohandass, C., and Arthur James, R. (2020). Bacterial communities associated with healthy and diseased (skeletal growth anomaly) reef coral Acropora cytherea from Palk Bay, India. Front. Mar. Sci. 7:92. doi: 10.3389/fmars.2020.00092
Rivera-García, L., Rivera-Vicéns, R. E., Veglia, A. J., and Schizas, N. V. (2019). De novo transcriptome assembly of the digitate morphotype of Briareum asbestinum (Octocorallia: Alcyonacea) from the southwest shelf of Puerto Rico. Mar. Genomics 47:100676. doi: 10.1016/j.margen.2019.04.001
Rodriguez-Lanetty, M., Phillips, W. S., and Weis, V. M. (2006). Transcriptome analysis of a cnidarian – dinoflagellate mutualism reveals complex modulation of host gene expression. BMC Genomics 7:23. doi: 10.1186/1471-2164-7-23
Rojas-Molina, A., García-Arredondo, A., Ibarra-Alvarado, C., and Bah, M. (2012). Millepora (“fire corals “) species: toxinological studies until 2011. Adv. Environ. Res. 26, 133–148.
Ryu, T., Cho, W., Yum, S., and Woo, S. (2019). Holobiont transcriptome of colonial scleractinian coral Alveopora japonica. Mar. Genomics 43, 68–71. doi: 10.1016/j.margen.2018.07.003
Shiomi, K., Hosaka, M., Yanaike, N., Yamanaka, H., and Kikuchi, T. (1989). Partial characterization of venoms from two species of fire corals Millepora platyphylla and Millepora dichotoma. Nippon Suisan Gakkaishi 55, 357–362. doi: 10.2331/suisan.55.357
Simão, F. A., Waterhouse, R. M., Ioannidis, P., Kriventseva, E. V., and Zdobnov, E. M. (2015). BUSCO: assessing genome assembly and annotation completeness with single-copy orthologs. Bioinformatics 31, 3210–3212. doi: 10.1093/bioinformatics/btv351
Simona, F. (2019). Application of Quantitative Phosphoproteomics to the Study of Cnidarian-Dinoflagellate Symbiosis. Thuwal: KAUST Research Repository. doi: 10.25781/KAUST-7MZ7C
Soza-Ried, J., Hotz-Wagenblatt, A., Glatting, K.-H., del Val, C., Fellenberg, K., Bode, H. R., et al. (2010). The transcriptome of the colonial marine hydroid Hydractinia echinata. FEBS J. 277, 197–209. doi: 10.1111/j.1742-4658.2009.07474.x
Sproles, A. E., Kirk, N. L., Kitchen, S. A., Oakley, C. A., Grossman, A. R., Weis, V. M., et al. (2018). Phylogenetic characterization of transporter proteins in the cnidarian-dinoflagellate symbiosis. Mol. Phylogenet. Evol. 120, 307–320. doi: 10.1016/j.ympev.2017.12.007
Stromgren, T. (1976). Skeleton growth of the hydrocoral Millepora complanata Lamarck in relation to light. Limnol. Oceanogr. 21, 156–160. doi: 10.4319/lo.1976.21.1.0156
Sully, S., Burkepile, D. E., Donovan, M. K., Hodgson, G., and van Woesik, R. (2019). A global analysis of coral bleaching over the past two decades. Nat. Commun. 10:1264. doi: 10.1038/s41467-019-09238-2
Tandon, K., Lu, C.-Y., Chiang, P.-W., Wada, N., Yang, S.-H., Chan, Y.-F., et al. (2020). Comparative genomics: dominant coral-bacterium Endozoicomonas acroporae metabolizes dimethylsulfoniopropionate (DMSP). ISME J. 14, 1290–1303. doi: 10.1038/s41396-020-0610-x
Tivey, T. R., Parkinson, J. E., and Weis, V. M. (2020). Host and symbiont cell cycle coordination is mediated by symbiotic state, nutrition, and partner identity in a model cnidarian-dinoflagellate symbiosis. Mbio 11:e02626-19. doi: 10.1128/mBio.02626-19
Tortorelli, G., Belderok, R., Davy, S. K., McFadden, G. I., and van Oppen, M. J. (2020). Host genotypic effect on algal symbiosis establishment in the coral model, the anemone Exaiptasia diaphana, from the great barrier reef. Front. Mar. Sci. 6:833. doi: 10.3389/fmars.2019.00833
Valderrama-Cháirez, M. L., Cruz-Hernández, A., and Paredes-López, O. (2002). Isolation of functional RNA from cactus fruit. Plant Mol. Biol. Report. 20, 279–286. doi: 10.1007/BF02782463
Veglia, A. J., Hammerman, N. M., Rivera-Vicéns, R. E., and Schizas, N. V. (2018). De novo transcriptome assembly of the coral Agaricia lamarcki (Lamarck's sheet coral) from mesophotic depth in southwest Puerto Rico. Mar. Genomics 41, 6–11. doi: 10.1016/j.margen.2018.08.003
Wenger, Y., and Galliot, B. (2013). Punctuated emergences of genetic and phenotypic innovations in eumetazoan, bilaterian, euteleostome, and hominidae ancestors. Genome Biol. Evol. 5, 1949–1968. doi: 10.1093/gbe/evt142
Keywords: Millepora complanata, Symbiodiniaceae, metatranscriptomic analysis, RNAseq, reef-building cnidarians
Citation: Hernández-Elizárraga VH, Olguín-López N, Hernández-Matehuala R, Caballero-Pérez J, Ibarra-Alvarado C and Rojas-Molina A (2021) Comprehensive Metatranscriptome Analysis of the Reef-Building Holobiont Millepora complanata. Front. Mar. Sci. 8:566913. doi: 10.3389/fmars.2021.566913
Received: 29 May 2020; Accepted: 04 February 2021;
Published: 26 February 2021.
Edited by:
Carla Zilberberg, Federal University of Rio de Janeiro, BrazilReviewed by:
Jian-Wen Qiu, Hong Kong Baptist University, Hong KongKonstantin Khalturin, Okinawa Institute of Science and Technology Graduate University, Japan
Copyright © 2021 Hernández-Elizárraga, Olguín-López, Hernández-Matehuala, Caballero-Pérez, Ibarra-Alvarado and Rojas-Molina. This is an open-access article distributed under the terms of the Creative Commons Attribution License (CC BY). The use, distribution or reproduction in other forums is permitted, provided the original author(s) and the copyright owner(s) are credited and that the original publication in this journal is cited, in accordance with accepted academic practice. No use, distribution or reproduction is permitted which does not comply with these terms.
*Correspondence: Alejandra Rojas-Molina, rojasa@uaq.mx