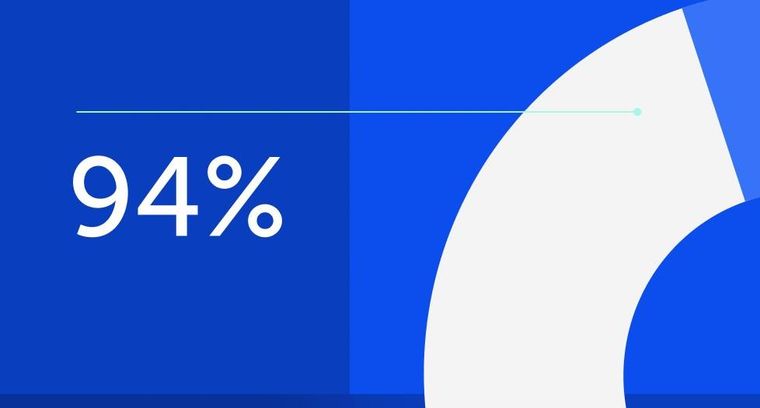
94% of researchers rate our articles as excellent or good
Learn more about the work of our research integrity team to safeguard the quality of each article we publish.
Find out more
ORIGINAL RESEARCH article
Front. Mar. Sci., 18 January 2021
Sec. Marine Megafauna
Volume 7 - 2020 | https://doi.org/10.3389/fmars.2020.624729
This article is part of the Research TopicSmall Cetacean Conservation: Current Challenges and OpportunitiesView all 51 articles
Small cetaceans face persistent threats from fisheries interactions, making effective mitigation a priority for conservation. In southwest Florida, interactions come primarily from small-scale recreational hook and line and trap/pot fisheries, with regional stranding network partners working with federal agency managers to assess and intervene as possible in cases of live animal entanglement. Evaluating success of intervention cases is difficult due to financial and logistical constraints which may preclude detailed follow-up monitoring. Survival over the initial 6 weeks post-release has been used as a marker of short-term success for small-cetacean rescue and/or rehabilitation cases. Early intervention prior to stranding, especially via remote disentanglement or rescue and immediate re-release onsite, can save entangled free-ranging dolphins facing life-threatening anthropogenic injuries. However, given the costs associated with interventions, it is important to understand the benefits of these endeavors not only to save individuals, but also to establish if and how saved individuals contribute to social functioning, survival and reproduction within small, resident populations facing multiple concurrent threats. Here we provide evidence from 27 well-documented common bottlenose dolphin (Tursiops truncatus) intervention cases during 1985–2019 where follow-up monitoring over multiple years sheds light on the longer-term success of these efforts and potential benefits to local populations. Nearly all rescued individuals (92%) survived longer than 6 weeks post-release (mean minimum survival period = 5 years, range 0–35 years), with 13 still observed frequently within their prior resident communities, in good physical health, and engaging in normal behavior. Survivorship rates did not decline substantially between 1 and 5 years post-rescue, meaning survival beyond 1 year may be a useful benchmark of long-term success. Rescued females that reached reproductive maturity (n = 4) have produced 12 post-intervention offspring to date. Social network analysis and demographic modeling applied to cases from the long-term resident community in Sarasota Bay confirmed that animals maintain social connections post-intervention and that interventions result in higher population growth rates. While not every intervention succeeds, this study demonstrates the conservation value of pre-stranding interventions which allow individuals that otherwise would be lost to remain viable and productive members of local populations when prevention of anthropogenic injury is not possible.
Small cetaceans around the world, particularly those found inshore and along the coast, are subject to increasing levels of human impact within their habitats (Notarbartolo di Sciara et al., 2016; de Vere et al., 2018). Animals face risks of injury from both direct interactions with potentially dangerous human activities, such as boating and fishing, as well as indirect interactions with discarded fishing gear and other types of marine debris (see for example, Laist, 1997; Read et al., 2006; Baulch and Perry, 2014; de Vere et al., 2018; Nichol et al., 2020). Despite efforts to minimize interactions and mitigate impacts, entanglement in fishing gear remains a major threat to many species, often resulting in unsustainable levels of mortality within localized populations (Read, 2008; Reeves et al., 2013; Hamilton and Baker, 2019). When possible, intervention efforts, including rescues and rehabilitation, may be undertaken to help individuals survive human-related injuries to which they may otherwise succumb. However, rescues are costly, logistically complicated, and have no guarantee of success (Wells et al., 2013). Rehabilitation is extremely expensive, time consuming, and fraught with complications due to the potential need to transport animals long distances or treat and care for injured individuals over long time periods before they can be released back into their original habitat (if their original habitat is known) (Moore et al., 2007). Additionally, there is the possibility that rehabilitated animals may become habituated to humans or they can develop secondary health issues related to long-term care, which can impact releasability (Moore et al., 2007; Wells et al., 2013). The logistical challenges and uncertainties associated with these efforts, coupled with limited financial and human resources available to devote to conservation (Parsons et al., 2015), raise a big question in terms of taking action to mitigate anthropogenic injuries – Are interventions really worth the trouble?
To answer this question, it is useful to step back to consider that the ultimate goal of intervention is for animals to remain in or return to wild populations to survive and reproduce. This goal has two complementary objectives. First, from an animal welfare perspective, interventions should help individuals survive and heal from life-threatening human-caused injuries. Second, from a conservation or population management perspective, animals that otherwise may have been lost should instead remain functioning members of local populations, contributing to population stability in the face of many concurrent and cumulative threats.
With this goal and objectives in mind, evaluating whether intervention efforts are ultimately successful is particularly important to better understand long-term outcomes. These data are critical to help managers and conservation practitioners make decisions and prioritize effective actions for populations at risk. For marine mammals in the United States, the risks and benefits of rehabilitation have been discussed at length in order to facilitate more effective decision-making toward multiple, sometimes conflicting, management needs (e.g., Moore et al., 2007). Unfortunately, for small cetaceans, follow-up monitoring after rescue, rehabilitation, and release is typically limited to a short time period during which either direct observations or remote tracking data are available (Wells et al., 2013). Therefore, the long-term outcomes of most cases remain unknown. However, evaluation of post-intervention survival for 10 species of odontocete cetaceans indicated that surviving more than 6 weeks post-release could be a useful benchmark for success, because survival rates did not decline substantially after this period (Wells et al., 2013). Additional indicators, such as animals exhibiting typical ranging patterns, habitat use, and social interactions for their species, without signs of abnormal behavior, provide a more comprehensive suite of success criteria to assess outcomes of different intervention strategies for individuals (Wells et al., 2013).
For a few marine mammal species in the United States, retrospective analyses of long-term intervention efforts have provided insight into their effectiveness as tools to help conserve endangered populations, as well as help individuals in immediate danger. For the Hawaiian monk seal (Neomonachus schauinslandi), a variety of opportunistic interventions aimed at reducing mortality risks for hundreds of seals over three decades saved individuals and provided substantial cumulative benefits to the population (Harting et al., 2014). Survival and reproduction of monk seals saved via intervention contributed to an estimated 17–32% of the population as of 2012 (Harting et al., 2014). Florida manatees (Trichechus manatus latirostris) have also benefited from more than four decades of directed rescue, rehabilitation, and release efforts aimed at assisting manatees impacted by entanglements, watercraft-related injuries, and other natural and anthropogenic threats (see for example, Reinert et al., 2017). Evaluation of post-release outcomes has helped to refine best practices and identify predictors of success to improve the ability of the overall manatee rehabilitation program to support wild population growth (Adimey et al., 2016). After near extirpation along the United States west coast, sea otter (Enhydra lutris) population recovery has been enhanced via a number of different conservation intervention tools, with rescue and rehabilitation primarily used to care for oiled animals or abandoned newborn pups (Estes and Tinker, 2018). Rehabilitation efforts following the Exxon Valdez oil spill were extremely expensive and ultimately not considered particularly effective from a conservation perspective (Estes, 1991; Williams and Williams, 1996; Estes and Tinker, 2018). In contrast, as protocols have improved over time, rescue and rehabilitation efforts for abandoned pups have shown much more promise to support local populations, with likelihood of survival and reproduction post-release now mirroring wild pups, and reductions in abnormal behavior following the implementation of a surrogacy program in California (Johnson and Mayer, 2015; Mayer et al., 2019). Furthermore, while the population-level benefits of rehabilitated pups depend on local growth rates and density dependence (Estes and Tinker, 2018), population models showed that 55% of observed population growth over recent decades at a restoration site was attributable to surrogate-reared otters and their progeny (Mayer et al., 2019) and that these additions have had a strong positive effect on population growth rates at the site (Estes and Tinker, 2018). These efforts have helped refine and improve the conservation impact of interventions for sea otter populations by strategically targeting areas to maximize impact (Estes and Tinker, 2018). Lastly, for North Atlantic right whales (Eubalaena glacialis), early detection and successful disentanglement of high-risk cases significantly improved survival outcomes of individuals in the first year post-entanglement, with rescued adults ultimately approaching the survival rates of non-entangled whales in subsequent years (Robbins et al., 2015). However, disentanglements were successfully performed for only 40% of North Atlantic right whale entanglement cases (Robbins et al., 2015), meaning that other strategies to facilitate disentanglement success (such as at-sea sedation, Moore et al., 2010) or to prevent entanglements from occurring in the first place (such as ropeless fishing, Myers et al., 2019) are necessary for conservation efforts to be effective for this species (Kraus et al., 2016).
Common bottlenose dolphins (Tursiops truncatus) in southwest Florida face increasing impacts from fisheries interactions, primarily due to recreational hook and line and trap/pot fisheries (Adimey et al., 2014). Most bay, sound, and estuary stocks in southwest Florida are categorized by National Oceanic and Atmospheric Administration’s (NOAA) National Marine Fisheries Service (NMFS) as “strategic,” meaning that human-caused mortality is likely to be significant relative to stock size (Hays et al., 2020). Dolphin-fishery interactions in this region are common and have resulted in many documented injuries, with entanglement, hooking, and/or ingestion of fishing gear often leading to lethal outcomes unless interventions are activated (e.g., Wells et al., 1998, 2008; Powell and Wells, 2011; Christiansen et al., 2016). Trend analysis from Florida stranding data showed significant increases in bottlenose dolphin entanglements during 1997–2009, both in the number of reported gear interaction cases and the proportion of gear interactions relative to total strandings, primarily due to increases on the Gulf coast (Adimey et al., 2014). Thus, regional stranding network partners in this area work closely with federal agency managers to assess and intervene as possible in cases of live animal entanglement.
Building from the post-intervention analyses in Wells et al. (2013), our objective here is to evaluate the longer-term success of rescue efforts for small cetaceans, over multiple years, focusing on well-documented inshore bottlenose dolphin cases from southwest Florida. Specifically, we assess long-term outcomes of the types of cases often viewed as both “most needed” (i.e., life threatening human-related injuries from entanglement) and “most likely to succeed” (i.e., pre-stranding interventions, primarily without long periods of rehabilitation). Many research and stranding network organizations operate in southwest Florida, centered on the intensively studied resident bottlenose dolphin community in Sarasota Bay, giving us a unique ability to track individuals long-term to determine outcomes and assess the ultimate likelihood of rescued animals “contributing” to the local populations from which they originated. This assessment provides insight into the potential broader conservation value of interventions to prevent population decline and mitigate human impacts in vulnerable populations.
Our study area included both inshore and nearshore waters along most of the central and southwest Florida coast in the Gulf of Mexico, stretching approximately 250 km from Clearwater to Marco Island (Figure 1). This geographic area has well-established research programs and consistent stranding network coverage over decades, and includes the intensively studied resident bottlenose dolphin community in Sarasota Bay, which has been monitored since 1970 and where many of the intervention cases originated. Within this region, we worked with stranding network partners and others doing regular photographic identification research to identify all confirmed common bottlenose dolphin live injury cases from the beginning of each collaborator’s historical records. Selected cases met the following criteria: (1) individuals were free-swimming when injuries were reported, (2) cases were confirmed anthropogenic injuries that could potentially be treated via rescue operations (primarily fishing gear entanglements), (3) injuries were deemed serious enough (i.e., life threatening) that pre-stranding interventions were authorized by NMFS, and (4) cases were documented with identification photographs from direct observations to facilitate follow-up monitoring beyond the 6 week “initial success” criterion from Wells et al. (2013). Interventions did not need to be successful for inclusion in the overall dataset, but individuals did need to be observed post-intervention for inclusion in any follow-up analyses. Most animals had both well-documented case histories prior to intervention and some follow-up observations where their identities were confirmed via photographic identification post-rescue. Necropsy reports were reviewed for all cases where individuals were confirmed dead post-rescue to determine whether the initial injury ultimately contributed to the animal’s death. Injuries severe enough to warrant intervention typically included one or more of the following: (1) fluke involvement or fluke insertion wrapped with trailing line, (2) multiple appendages wrapped with line, multiple line entanglements, or body wrapped, causing constriction, (3) mouth or jaw involvement with potential gear ingestion, and (4) additional associated injuries or complications (e.g., embedded line or muscle injuries, animals anchored in place or movement restricted, severe lacerations, entanglements associated with shark bites, propeller wounds, lacaziosis lesions, or signs of infection).
Figure 1. Map of the overall study area showing rescue locations for pre-stranding intervention cases in Southwest Florida.
Our final collaborative regional case list included 27 dolphins whose interventions took place during 1985–2019, with all but two meeting the 6-week criterion for short-term success (92.6%). Rescue operations were of three basic varieties: (1) remote disentanglements of free-swimming or anchored animals (n = 10), (2) temporary capture-release operations with treatment and immediate release onsite (n = 14), and (3) rescue captures followed by a period of human care and treatment in rehabilitation and later release (n = 3). In three cases, individuals were eventually rescued via capture-release operations after initial remote disentanglement efforts were unable to clear the animals of all life-threatening fishing gear. During our case review, we also identified an additional 23 potentially relevant pre-stranding entanglement or intervention cases within the region. However, these cases were excluded from analysis because animals either shed gear prior to authorized interventions (n = 4), only had satellite-linked tag data available post-rescue (n = 1), lacked clear dorsal fin photos necessary for follow-up identification (n = 9, includes several citizen disentanglement cases with unclear outcomes), or were being monitored for confirmed anthropogenic injuries where interventions were not authorized (due to injuries being deemed non-life-threatening or animals disappearing, n = 9).
We evaluated outcomes for each case based on several basic criteria indicative of success. For all cases, we assessed the minimum survival period post-intervention by calculating the duration between the intervention date and the most recent observation from either official permitted research and stranding network operations or opportunistic citizen reports confirmed via photographs (through August 2020). For all cases where the most recent observation was more than 1 year old and the individual had not already been confirmed dead, we cross-checked their dorsal fin identification photographs in the region-wide Gulf of Mexico Dolphin Identification System (GoMDIS1) for any evidence of animals leaving one area and going to another or carcasses being recovered by network partners who may have been unfamiliar with their case history.
We further calculated the proportion of cases meeting short-term (i.e., 6 weeks) and longer-term survival benchmarks (post-rescue survival durations greater than 1, 2, 5, and 10 years, respectively). Calculation of survival rates greater than 1 year post-rescue was complicated by the fact that interventions took place as recently as 2019, so only some cases could have reached the longer benchmarks of 2, 5, and 10 years post-rescue. Given the truncated nature of these estimates, multi-year survival estimates were calculated as the proportion of those cases observed post-rescue whose rescues took place greater than X years prior to August 2020 and had known fates as of X years post-rescue.
For all individuals observed post-rescue (n = 25), we examined whether the animals exhibited “typical” ranging behavior by assessing whether they remained in the general dolphin community range from which they were rescued. Inshore dolphins in southwest Florida are typically year-round residents to specific bay and estuary systems. While we ideally would have liked to assess the fine-scale ranging patterns of each individual based on multiple sightings over time, many of the cases outside of Sarasota Bay did not meet minimum sighting criteria for robust calculation of home range sizes, so we adopted a simplified range calculation based on data available across the region. This ranging metric was defined as the distance in kilometers between an individual’s rescue location and their most recent observation.
Individual observation records were examined to determine whether there was any evidence of “abnormal” behavior by dolphins post-rescue. Behaviors of particular interest to this assessment included any observations of dolphins seeking anthropogenic sources of food, such as begging or accepting food provisioning from humans, as well as patrolling, scavenging, or depredating from active fishing gear, since these unnatural behaviors make it more likely for individuals to suffer subsequent anthropogenic injuries (Christiansen et al., 2016).
Lastly, for those females who were either adults at the time of intervention or who reached sexual maturity post-intervention (n = 4) we reviewed their observation records to determine if they had reproduced, and if so, how many calves they produced post-rescue.
For dolphins within the well-studied Sarasota Bay community, we assessed whether key aspects of their social behavior and connectivity were altered post-rescue. Group size and association data were collected during boat-based photographic identification population monitoring surveys, where individuals were identified via photographs of unique dorsal fin characteristics. A group was defined as all individuals within a radius of ∼100 m, moving in the same direction and generally engaged in the same activity (Wells et al., 1987). Any individual with at least five sightings in the year prior to injury was included in these analyses (n = 12) and all but one were also observed frequently during the year post-intervention.
Average group size was compared across the year prior to injury, during injury, and the year post-rescue using a one-way repeated measures ANOVA in SigmaStat 3.5 (Systat Software, Inc., Chicago, IL, United States). Differences in number of associates and social network metrics were compared in the year prior to injury versus 1 and 2 years post-intervention following Greenfield et al. (2020). Two-year comparisons were only made if significant differences were found 1 year post-rescue. Association analyses were conducted in SOCPROG 2.8 (Whitehead, 2009) using the half-weight index (HWI) with the sampling period set to 1 day. The overall mean HWI from all possible interactions, including cases where individuals did not associate, was 0.03, and the mean HWI of all non-zero associations was 0.07. These values were used to generate filtered social networks of preferred associates at two levels of association strength (Whitehead, 2008; Titcomb et al., 2015): HWI greater than twice the overall mean (HWI > 0.06) and HWI greater than twice the non-zero mean (HWI > 0.14). Filtered networks were used to examine changes in social connectivity among closer associates in addition to social integration within the overall resident dolphin community.
Individual social network metrics, including strength, eigenvector centrality, clustering coefficient, and number of triangles, were calculated using SOCPROG 2.8 (Whitehead, 2009) and Gephi 0.9.2 (Bastian et al., 2009). These metrics evaluate several different aspects of direct and indirect connectivity within the overall social network.
For social network analyses, each injured individual was matched with a control animal from the same community and of the same age-sex class at the time of injury, and changes in network metrics were compared against patterns observed by control animals to assess whether injuries disrupted social associations (Greenfield et al., 2020). Because these data were non-normally distributed, median differences before and after injury were assessed using paired one-sample Wilcoxon signed rank tests for injured and control animals separately in JMP Pro 12 (Version 12; SAS Institute Inc., Cary, NC, United States).
To assess the population benefit of interventions, the Vortex population model (Lacy, 2000; Lacy and Pollak, 2020) was used to examine what would have been the impact on the Sarasota population of bottlenose dolphins if interventions had not occurred. The approach of modeling the population impacts, in addition to simply summing up the number of dolphins saved, accounts for the expected longevity and fecundity of rescued dolphins to yield the long-term population-level consequences of intervention.
Vortex is an individual-based simulation that projects population trajectories from the aggregate fates of individuals in the population. It can include uncertainty in the population mean demographic rates, fluctuations in demographic rates over time (“environmental variation”), and individual variation in probabilities of breeding or dying. Random variation in the fates of individuals (“demographic stochasticity”) is inherent in the Vortex model, emerging from the binomial process simulated as each individual experiences demographic events with the specified probabilities.
Our population model started with demographic rates that have been estimated from the intensive and consistent monitoring of the Sarasota population from 1993 through 2019, as described in detail in Wells et al. (in preparation) and Lacy et al. (in preparation). For modeling the population trajectory with the interventions that occurred, we used the model from Lacy et al. (in preparation) that assumed that 33% of deaths were documented from recovered carcasses (based on carcass recovery rates in Wells et al., 2015), while the small excess in disappearances above the number of documented deaths divided by 0.33 was assumed to have been emigrants. Those emigrants and the observed immigrants (mean 1.93/year) were not included in the model of intervention consequences, so the analyses represent the predicted population growth due to birth and death processes in the local population. Uncertainty in demographic parameters was included in the model by sampling for each independent iteration of each rate from a normal distribution with the mean and standard error of the mean estimates. Annual demographic rates in the population model were sampled from distributions that represent the environmental variation in each rate. Thus, the range of population trajectories produced by the simulation includes the uncertainty in parameter estimates, the annual environmental variation, and the inherent demographic stochasticity of demographic events (Lacy et al., in preparation).
We started the population projection with 91 dolphins, the population size estimated in 1985 (Wells and Scott, 1990). No upper limit (carrying capacity) was placed on the population; thus, the total population sizes generated by the model include any excess production that might lead to a net outflow of animals to surrounding areas.
We projected the population first from 1985 to 2020 and then for 50 years subsequently, with 10,000 iterations of the simulation for each scenario. The model generated a mean predicted population size of 156 in 2020. This compares to the observed population growth from N = 91 in 1985 to N = 160 as of January 1, 2020, validating that the initial model represents well the population demography.
The impact of interventions was assessed by removing from the modeled population those dolphins that would have died, but were rescued by an intervention. This assumes dolphins would have died without intervention, which could overestimate the impact of interventions conducted for non-life-threatening injuries. Only dolphins that survived more than 1 year after the intervention were considered to have been rescued. Of those that did survive more than 1 year, their survival rates were comparable to dolphins that never required intervention.
The predicted population trajectory if interventions had not occurred was modeled in two stages. First, dolphins of specified ages and sexes were removed from the simulated population in the years at which dolphins in the Sarasota population were rescued by successful interventions since 1985. This allowed for precise estimation of the impact to date of these specific interventions. Next, the simulation was continued for another 50 years, while modeling the effect of continued interventions at the same mean rate of successful interventions as occurred from 2003 through 2019. Over that time span, intervention was successful for a mean of 0.625 dolphins/year in a population of average size N = 147, equivalent to a 0.425% probability of a successful intervention for any given dolphin each year. In calculating this mean rate of intervention, we omitted the early years with the first three interventions (in 1985, 1992, and 1996) – before interventions became more frequent starting in 2003. In the model of no interventions, therefore, each dolphin (subsequent to 2020) was given a 0.425% probability of being removed from the population, to account for the losses that would be predicted to occur if interventions were stopped.
Projection of population-level consequences of interventions depends on both the survival of rescued dolphins and their further contribution to population growth through reproduction. In the model with interventions, the survival rates estimated for the population overall were applied to successfully rescued dolphins as well. Several dolphins that were rescued by an intervention have subsequently produced offspring, and there is no indication that they have lower fecundity than other dolphins. Therefore, we assumed that interventions, when they succeed in keeping the dolphin alive for at least 1 year, have no negative effect on subsequent reproductive capability. Conversely, removal from the population of dolphins that could otherwise have been rescued results in the immediate loss of those dolphins and also the loss of their potential offspring and later descendants.
The Vortex input file (DolphinInterventions.xml) used to generate the population-level consequences of deaths averted by interventions is available in the zenodo.orgrepository at http://doi.org/10.5281/zenodo.4081894.
Twenty-seven intervention cases met the criteria for inclusion (Table 1). These individuals represented all age-sex classes, but were skewed toward dependent calves (n = 14) and juveniles (n = 5), which together accounted for 70.4% of cases. All individuals were injured due to entanglements, primarily from monofilament or other fishing line (n = 20), with fewer animals entangled in crab trap float lines (n = 3), nets (n = 1), or other types of debris (n = 3). Minimum duration of injury (measured as number of days between the initial observation and intervention) varied widely, from less than a week (n = 8) to more than 9 months, averaging 41.1 ± 62 days (range 0–288 days).
Outcomes also varied, with 25 of the 27 (92.6%) surviving more than 6 weeks post-intervention and thus being considered preliminary successes, and 20 (74.1%) individuals surviving more than 1 year post-rescue. Likelihood of surviving more than 1 year post-rescue improved somewhat with age at intervention, with 64.3% of dependent calves, 80% of juveniles, and 85.7% of adults reaching this longer-term benchmark.
Two individuals were not observed post-rescue, and both were dependent calves less than 1 year old at the time of injury. Animals this young are not normally the subjects of capture-release operations due to the inherent risks involved, but for the serious entanglements in these cases the risks of capture-release were deemed acceptable to deal with their life threatening injuries. In total, four young calves (<1 year old) had interventions, two of which survived for >5 years post-rescue (50% success rate). For the two unsuccessful young calf cases, one died during capture-release rescue operations after remote disentanglement efforts had failed (CMA1923), and the other was in extremely poor condition by the time remote disentanglement tools were able to facilitate gear removal alongside a boat after multiple unsuccessful previous rescue attempts had been made over a 3 month period (C797). In this second case, remote disentanglement efforts were not able to remove fishing line during initial attempts while the animal was free-swimming alongside its mother, and during capture-release rescue attempts on two occasions, the dolphins were not in locations or group sizes allowing for safe net deployments.
As of August 2020, 13 individuals were still alive, 10 have been confirmed or are presumed dead due to a sudden cessation of previously consistent observations (four of which died or went missing within 6 months of intervention), and four have unknown fates, primarily because their ranges were centered on portions of the overall study area without regular observation coverage.
In the two cases described above, young calves died during or shortly after rescue operations, indicating that long-term survival is not a guaranteed outcome of intervention action. For the remaining 25 cases where individuals were observed post-rescue (including animals disentangled as recently as April 2019 – see Table 1), the mean minimum survival period was 5.4 ± 7.2 years post-intervention, ranging from 3 months to 35 years. All individuals who were observed post-rescue reached the short-term survival benchmark of 6 weeks, and 80% survived for more than 1 year post-rescue. Minimum survival duration did not differ based on sex, age class, intervention type or duration of injury (all p > 0.05).
Multiyear survival rates calculated for cases where rescues took place more than 2, 5, and 10 years ago indicated that most of the animals that reached the 1-year benchmark survived well beyond it. Sixteen of twenty (76.2%) cases survived more than 2 years post-rescue, dropping only slightly to 73.3% (11 of 15) surviving more than 5 years post-rescue. Only a handful of cases from Sarasota Bay had known fates more than 10 years post-rescue, with 50% (3 of 6) meeting this longest-term survival benchmark, although one animal that died during 5–10 years post-rescue was an older adult male unlikely to have survived longer (FB28: 42 years old at time of rescue).
Nearly all individuals remained within the general resident dolphin community range from which they were originally rescued (Figure 2). Average distance between an individual’s rescue location and their most recent observation was 9.68 ± 11.98 km (range 1–54 km; median 5; IQR 2–12.25 km; N = 25). How far away individuals were later observed from their rescue site did not differ based on sex [t(19) = −0.227, p = 0.823, males: 8.18 ± 6.19 km, females: 9.00 ± 10.07 km) or time span since rescue (Pearson correlation coefficient R = 0.309, p = 0.133, N = 25; Spearman correlation coefficient rho = 0.128, p = 0.539, N = 25). The two individuals who ranged farthest from their original rescue locations (54 and 35 km) were a male with the shortest (3 months) and female with the longest (35 years) post-rescue observation durations, respectively. In three cases, individuals had moved between communities prior to rescue, either while injured (F201, F301) or prior to injury (Cockatoo), but these individuals then appear to have remained in the locations where they were rescued (i.e., no confirmed observations or carcass recoveries elsewhere in the region post-rescue).
Figure 2. Map of rescue locations versus most recent sighting location for intervention cases observed post-rescue. Nearly all individuals remained within the dolphin community from which they were rescued.
Our ability to track specific behaviors exhibited by individuals outside of the core areas for photo-identification or directed follow-up monitoring was somewhat limited. However, abnormal behaviors were observed in several cases, including evidence of a reliance on human provisioning in one case and potential chronic fishing interactions in six other cases. Abnormal behaviors were first observed before injury in only one case, during injury in four cases (14.8% of total intervention cases) and post-rescue or rehabilitation in nine cases (33.3%), but only two of these began shortly after intervention while the others were first observed occasionally engaging in unnatural foraging behaviors years later. Unnatural foraging behaviors were observed at some point by each of the three individuals who spent periods of time in rehabilitation, with one individual (F300) later being observed repeatedly being provisioned and lingering near a commercial fish processing dock.
Four females in Sarasota Bay either were adults at time of injury or reached reproductive age post-intervention. Together, they have produced 12 post-rescue calves so far. FB11, the earliest intervention case in the group, was rescued as a young calf in 1985 and has subsequently produced five calves (all of which have survived), with three grandcalves born to her daughter as well. FB03, rescued as a juvenile, gave birth to her first calf 3 years later, but she then went missing shortly after that calf reached independence. F113, rescued as an adult, was the only female known to be sexually mature at the time of rescue and was caring for her third calf at the time of injury. That calf survived, and she produced four subsequent calves post-intervention. Finally, F221, rescued as a calf in 2010, had her own second calf in 2020. Outside of Sarasota Bay, one animal rescued in 2019 was observed in 2020 with a likely post-rescue calf in Charlotte Harbor (FMMSN1914). While sample sizes are too small for robust comparison of age-specific reproductive rates of intervention animals to the population at large, there is no indication that these individuals have reduced fecundity, and all rescued females that have reached sexual maturity have been able to reproduce successfully post-intervention.
Social behavior was only analyzed for cases within the well-studied Sarasota Bay dolphin community. A repeated measures ANOVA showed that group size differed by time period [F(2,21) = 9.04, p = 0.001]. However, a post hoc Tukey’s multiple comparison test revealed that there were no differences in group size from the year prior to injury (mean = 5.80 ± 3.10) versus the year post-intervention (mean = 4.87 ± 2.47; p = 0.478). Instead, the difference in group size by time was driven entirely by individuals being observed in significantly smaller groups during injury than either the time period before or after (Figure 3; mean = 2.62 ± 1.53; before versus during p = 0.001, during versus after p = 0.024). Similarly, number of associates also did not differ between the year prior to injury versus post-rescue (median + IQR: Pre = 40.5 + 40.25; Post = 31.0 + 37; paired Wilcoxon signed rank test T(10) = −7.00, p = 0.281, median difference between periods = −1.0).
Figure 3. Group size. Average group size for Sarasota Bay cases before injury, during injury, and post-rescue (n = 12). Group size while injured was significantly smaller than either the year before injury or after intervention, which did not differ from each other.
Social network analyses revealed no significant differences in any network metrics (strength, eigenvector centrality, clustering coefficient, or number of triangles), when assessed for the full unfiltered community networks or for networks based on stronger connections filtered to twice the average association strength across the community (Tables 2A,B). However, when networks were filtered to include only the strongest associates (2× average non-zero HWI), injured animals were found to have reduced strength, eigenvector centrality, and number of triangles post-intervention, indicating some loss in social connectivity that was not fully rectified even 2 years post-injury.
Figure 4 shows the mean population trajectory from Vortex models with interventions (projecting from the demographic rates that have been observed from 1993 to 2020) versus without interventions (i.e., removing the dolphins that had, or are predicted to have in the future, successful interventions). Mean population sizes and population growth rates for 1985–2020 (the recent years over which interventions occurred) as well as projected 50 years into the future indicated larger final population sizes and faster growth rates with interventions as compared to scenarios that removed rescued dolphins from the population (Table 3). There was large range of trajectories and overlap between the models with and without interventions (Figure 4), due to the multiple sources of stochasticity and uncertainty. The trajectory of any one simulated population or any one actual population is highly uncertain. Parameter error and environmental variation, the primary sources of uncertainty in the population trajectory (see Lacy et al., in preparation), would impact the population equally whether interventions occurred or not. For example, if mean survival or breeding rates were underestimated, the population trajectory would be represented by the more optimistic iterations for both the model with intervention and the model without intervention. Therefore, assessment of the population-level benefit of intervention requires comparing mean trajectories for the two models, rather than comparing high trajectories for one against low trajectories for the other.
Figure 4. Population projections. Mean population trajectory for Sarasota Bay from the Vortex population model with interventions (black line) versus without interventions (gray line). Dotted lines show the mean trajectories +1 SD and –1 SD.
Many of the final projected population sizes in 2070 are likely unrealistically high for the habitat in Sarasota because no carrying capacity was imposed in the model (see Lacy et al., in preparation for Sarasota Bay projections with appropriate constraints and sources of population regulation). The long-term projections, therefore, reflect the total potential future descendants of the population, some of which might have emigrated from the area.
Overall, the 2020 population is predicted to have about 16 more dolphins as a result of the interventions (the animals saved and their subsequent reproduction). A similar conclusion could be reached by tallying the number of rescued dolphins that are still alive, plus their living descendants, but the population model provides a means to project the effect of ongoing interventions on the future potential population growth. If intervention at the same rate continues into the future, the benefit to the population is compounded, resulting in an estimated 40% more dolphins 50 years in the future. For Sarasota Bay dolphins, the difference in population growth with versus without the intervention did not make a difference between a growing versus declining population, but did result in faster population growth, demonstrating that interventions enhance the demographic potential of the population. This benefit arises from even a relatively small number of interventions, even through preventing the death of fewer than one dolphin per year.
Our retrospective analysis of outcomes from well-documented bottlenose dolphin intervention cases over a 35-year period demonstrates the potential for achieving long-term success at the primary goal of wildlife rescues – helping animals remain in or return to wild populations to survive and reproduce. Approximately 75% of rescued dolphins survived over multiple years, all living animals remained in their local communities, and all sexually mature females have successfully reproduced in those communities. Together these findings strongly support the idea that interventions to save animals with life-threatening anthropogenic injuries provide benefits not only to the welfare of those individuals, but also to the stability and growth potential of their local populations. Social network and demographic modeling focused on cases from within the natural laboratory in Sarasota Bay confirmed that rescued animals maintain most of their social connections and that the survival of these individuals supports greater population sizes and growth rates than would have occurred without interventions taking place. While the apparent success of bottlenose dolphin intervention efforts in southwest Florida may not directly translate to other species or populations facing a different suite of threats, it does provide strong evidence that rescuing individuals can provide measurable population benefits, even when conducted at a low rate relative to overall population size (on average less than one individual rescued per year).
We recognize that southwest Florida may not be directly comparable to other sites in terms of rescue logistics or capacity to conduct interventions, so the types of early interventions (prior to stranding) that were our focus simply may not be possible everywhere without enhanced and/or sustained support. Within southwest Florida, we are fortunate to have a large, well-trained, multi-agency team capable of identifying and responding to free-swimming animals with human-related injuries. For example, we work closely with some of the same team members responsible for the successful Florida manatee rescue and rehabilitation program described in Adimey et al. (2016). We also have decades of experience safely conducting collaborative capture-release health assessments on resident dolphins in Sarasota Bay (Wells, 2009; Barratclough et al., 2019), so the necessary equipment and expertise are readily available to respond when entanglements are reported. In addition, many of these rescued animals were long-term resident dolphins that spend substantial amounts of time in shallow, inshore waters, which allowed for relatively easy case monitoring, facilitating sometimes multiple remote disentanglement attempts or capture-release rescue operations per individual, which increased our likelihood of success (Wells et al., 1998, 2008, 2013). Indeed, we chose this region to evaluate long-term intervention outcomes for precisely these reasons as it provided a unique opportunity to assess the fates of well-known individuals after many years in an area with extremely good photo-identification and stranding network coverage.
Developing this regional capacity has taken decades of financial support and dedicated training to increase our ability to respond to and treat injured animals and then conduct robust follow-up monitoring of cases to assess outcomes. Thus, while we would certainly recommend that managers consider developing similar capacity to conduct rescues of dolphins in life-threatening situations prior to stranding in other areas, we recognize that it is a time- and resource-intensive endeavor. Despite the logistical complications, for live animals facing life-threatening human-related injuries, these efforts would likely be warranted, particularly within small threatened or endangered populations where the survival of every individual is critical. These early disentanglement operations have perhaps the best chance of succeeding, as compared to attempting rehabilitation of already stranded animals whose condition is likely less recoverable. In addition, taking advantage of opportunities to save individuals that might otherwise be lost from the community, when feasible, could also help to partially offset population level impacts of other human activities that are not possible to directly mitigate (such as impacts from pollution or habitat destruction).
Where direct follow-up observations on individuals post-release are unlikely, we strongly recommend satellite-linked tracking of released animals, which provides short to medium-term information on survival, movements, and behavior allowing for likely outcomes to be identified, and facilitating further interventions if warranted. Although tagging and tracking can be another added expense to already tight budgets, the information gained is invaluable at helping to understand whether, and which, interventions are successful to help prioritize when to take future action to maximize the benefits to vulnerable populations.
One potential concern is the frequency of abnormal behaviors observed by injured animals. Within our case list, individuals who spent periods of time in rehabilitation were particularly likely to exhibit abnormal behavior, including some reliance on human provisioning, similar to rehabilitation-associated problems others have highlighted in the past (e.g., Moore et al., 2007; Wells et al., 2013). However, a few other animals that never spent time in human care also showed some evidence of repeated interactions with fisheries, either getting re-entangled or being observed foraging in close proximity to anglers. Future analyses should assess rates of abnormal behavior among entangled dolphins that received intervention, those that did not receive intervention, and non-entangled dolphins to determine whether those requiring intervention engage in risky human-related foraging behaviors at greater rates. If so, they could be more likely to become re-injured or to pass abnormal behaviors on to their close associates. Previous studies have confirmed the role of social learning in the adoption and spread of unnatural foraging behaviors, including patrolling, scavenging, or depredating from fishing gear, within resident dolphin communities (e.g., Donaldson et al., 2012; Christiansen et al., 2016; Wells, 2019). Individuals exhibiting such behaviors also face a higher likelihood of suffering anthropogenic injuries over their lifetimes (Christiansen et al., 2016). Thus, for extreme cases where injured animals are fully habituated to seeking human sources of food, there may be the potential to do more harm than good by returning them into local communities where they could influence the behavior of others in ways that might increase future risk of injury. Fortunately, within the cases we evaluated, we found no evidence of intervention animals clearly becoming sources of abnormal behavior. In particular, none of the reproductive females in Sarasota Bay have been documented passing human-related foraging behaviors on to their calves.
Another concern is the disproportionate impact of these injuries on young animals that also appear less likely to survive over multiple years post-rescue. Wells and Scott (1994) first noted the disproportionately high number of subadult dolphins involved in entanglement in Sarasota Bay, and this study confirmed these earlier findings within a larger timeframe and geographic area. In particular, calves less than 1 year old appear especially vulnerable to succumbing to injury. Only 50% survived post-intervention, highlighting the heightened risk to these individuals. One calf that did not survive post-rescue had become extremely emaciated after 3 months of unsuccessful rescue attempts of various kinds. Thus, prolonged serious injuries to dependent calves may interfere with maternal care, including nursing and development of foraging behavior, making early intervention especially important for these cases. Fortunately, when young calves are able to be successfully rescued, the benefits compound over time. Two individuals rescued at 1 year old or less are among those now successfully reproducing in the Sarasota Bay community, so the potential benefits of rescuing these animals are high given their lifetime reproductive potential.
One final issue requiring further exploration is to what extent injured animals truly re-integrate into their local social community. While overall gregariousness and social connectivity appear unaffected, social network analyses revealed potential lingering impacts to injured animals’ closest social associations, consistent with what was found for a broader group of individuals suffering from anthropogenic injuries in Sarasota Bay (Greenfield et al., 2020). Whether or not the subtle observed changes in strength of close associations and network centrality within these animals’ social “neighborhoods” are biologically meaningful remains to be seen, and their underlying mechanisms are not well understood. However, enough rescued females have reached sexual maturity to give us confidence that they remain sufficiently socially integrated to facilitate successful reproduction within their local communities. However, future analyses should assess maturing male social relationships to confirm that they develop and maintain strong alliances as would be expected. As of August 2020, there are only two cases in Sarasota Bay where males rescued as juveniles have continued to regularly be observed after reaching sexual maturity – one (F248) is now 22 years old and has formed a strong alliance with another resident male of similar age, while the other (F262) is now 14 years old but has not yet formed a stable alliance. So, while indications are that these types of injuries and interventions do not significantly impede male social behavior over the long term, our evidence is at this point anecdotal.
A major takeaway of this assessment is that even the low frequency of successful interventions observed in Sarasota Bay has the potential to contribute to population stability over long time periods. While the assumption that all rescued individuals would have died without interventions could overestimate effectiveness if used in situations where injuries were non-life-threatening, demographic modeling from Sarasota Bay indicated substantially higher population growth rates and final population sizes for scenarios incorporating interventions. Future work with larger sample sizes could estimate and compare age-specific survival and reproductive rates of entangled individuals that received intervention, did not receive intervention, and were not entangled to further develop the use of demographic models to assess the effectiveness of interventions over the long-term. Similar modeling approaches could also be used to estimate the cumulative benefit to a dolphin population – in terms of population growth rate, consequent population size, or number of dolphin-years gained – from any planned program of intervention. Thus these methods could be used to assess the likelihood of conservation benefits of successful interventions in other local populations, either as a mechanism to mitigate human impacts or weighed against other potential conservation actions.
Importantly, not all interventions are ultimately successful, so development of proactive strategies to reduce and prevent injuries from occurring must also be front and center in any mitigation strategies. Even rescues that appear to be short-term successes can result in unexpected failure years later if animals face lingering impacts from their injuries (e.g., Marks et al., 2020). While only two of our cases were apparent failures prior to this analysis, an additional five individuals failed to survive a full year post-intervention bringing the overall long-term survival success rate to 74%. Thus, while these long-term survivors provide clear evidence that interventions can successfully save individuals and support local populations, we recommend comprehensive management strategies which focus first and foremost on reducing the need for interventions in the first place. Overall, interventions are a beneficial tool allowing individuals that otherwise would be lost to remain viable and productive members of local populations when prevention of anthropogenic injury is not possible. However, rescuing animals with human-related injuries should be a useful last-resort option within a more comprehensive suite of conservation actions focused on preventing situations that may lead to a need for intervention.
The raw data supporting the conclusions of this article will be made available by the authors, without undue reservation.
The animal study was reviewed and approved by the Mote Marine Laboratory Institutional Animal Care and Use Committee, as well as National Marine Fisheries Service authorization conducted under the Marine Mammal Protection Act.
KAM and RW conceived and designed the overall analysis. RW further oversaw the long-term research program in Sarasota Bay and acquired funding for population monitoring efforts, as well as for rescues and follow-up monitoring for many of the intervention cases. KAM participated in field efforts, collected and curated regional case data, performed the basic analysis, and wrote the manuscript. DR contributed to design, analysis, and interpretation of social network analysis. MG performed the social network analysis and contributed to the corresponding sections of the manuscript. RL performed the population modeling and wrote the corresponding sections of the manuscript. JA participated in field efforts and was responsible for data curation, investigation, long-term sighting database administration and validation for CZS-SDRP records. AB and RW coordinated field efforts for rescues and follow-up monitoring led by CZS-SDRP. AB coordinated fieldwork for long-term population monitoring and led remote disentanglement efforts for several cases. KB-H participated in field efforts, contributed data, and performed the literature review. CC curated contributor data and performed regional searches in GoMDIS. GL, DF, DB, AP, AW, AS, LO, and KM participated in field efforts, and collected, contributed, and validated regional case data, including injury and necropsy reports as well as pre- and post-rescue sighting records. DB led remote disentanglement efforts and coordinated initial case evaluation and rescues for the Florida Fish and Wildlife Conservation Commission Southwest Field Lab (FMMSN) cases. GL, DF, and AS acquired funding, coordinated initial case evaluation and rescue efforts for Mote Marine Laboratory (MML) and Clearwater Marine Aquarium (CMA) cases. BM oversaw regional stranding network operations/initial case evaluation and authorized rescue operations. All authors reviewed and edited the final manuscript.
AW was employed by the company Good-Natured Statistics Consulting.
The remaining authors declare that the research was conducted in the absence of any commercial or financial relationships that could be construed as a potential conflict of interest.
This work was funded by a series of grants from the Disney Conservation Fund and NOAA’s John H. Prescott Marine Mammal Rescue Assistance Grant Program, as well as support from the Charles and Margery Barancik Foundation, the Batchelor Foundation, the National Fish and Wildlife Foundation, Save the Manatee Trust Fund, and institutional support for the members of the stranding network involved in the interventions, rehabilitation, and post-release follow-up monitoring, including the Chicago Zoological Society, Mote Marine Laboratory, Florida Fish and Wildlife Conservation Commission, Clearwater Marine Aquarium, NOAA’s NMFS, and the University of Florida College of Veterinary Medicine. The Vortex population modeling software is made available by the partners of the Species Conservation Toolkit Initiative (scti.tools).
We sincerely thank the many volunteers, program staff, state and federal agency employees, veterinarians, stranding network partners, and members of the public who reported observations of injured animals, participated in rescue or rehabilitation operations and follow-up monitoring efforts, and without whom none of this work would have been possible. In particular, we are indebted to Larry Fulford whose capture expertise facilitated our rescue operations throughout the region. We also thank Erin Fougères for her input on case inclusion criteria and reviewing the manuscript prior to submission, Krystan Wilkinson for map preparation, and Nathan Crum for helpful comments on the initial draft. Dolphin interventions were authorized by NMFS and conducted under the Marine Mammal Protection Act (MMPA) through 112c agreements or 109h authority, or under NMFS MMPA/Endangered Species Act Permits. The scientific results and conclusions, as well as any views or opinions expressed herein, are those of the authors and do not necessarily reflect the views of NOAA.
Adimey, N. M., Hudak, C. A., Powell, J. R., Bassos-Hull, K., Foley, A., Farmer, N. A., et al. (2014). Fishery gear interactions from stranded bottlenose dolphins, Florida manatees and sea turtles in Florida, U.S.A. Mar. Pollut. Bull. 81, 103–115. doi: 10.1016/j.marpolbul.2014.02.008
Adimey, N. M., Ross, M., Hall, M., Reid, J. P., Barlas, M. E., Keith Diagne, L. W., et al. (2016). Twenty-six years of post-release monitoring of Florida manatees (Trichechus manatus latirostris): evaluation of a cooperative rehabilitation program. Aquat. Mamm. 42, 376–391. doi: 10.1578/AM.42.3.2016.376
Barratclough, A., Wells, R. S., Schwacke, L. H., Rowles, T. K., Gomez, F. M., Fauquier, D. A., et al. (2019). Health assessments of common bottlenose dolphins (Tursiops truncatus): Past, present, and potential conservation applications. Front. Vet. Sci. 6:444. doi: 10.3389/fvets.2019.00444
Bastian, M., Heymann, S., and Jacomy, M. (2009). Gephi: an open source software for exploring and manipulating networks. ICWSM 8, 361–362.
Baulch, S., and Perry, C. (2014). Evaluating the impacts of marine debris on cetaceans. Mar. Pollut. Bull. 80, 210–221. doi: 10.1016/j.marpolbul.2013.12.050
Christiansen, F., McHugh, K. A., Bejder, L., Siegal, E. M., Lusseau, D., McCabe, E. B., et al. (2016). Food provisioning increases the risk of injury in a long-lived marine top predator. R. Soc. Open Sci. 3:160560. doi: 10.1098/rsos.160560
de Vere, A. J., Lilley, M. K., and Frick, E. E. (2018). Anthropogenic impacts on the welfare of wild marine mammals. Aquat. Mamm. 44, 150–180. doi: 10.1578/AM.44.2.2018.150
Donaldson, R., Finn, H., Bejder, L., Lusseau, D., and Calver, M. (2012). The social side of human-wildlife interaction: wildlife can learn harmful behaviors from each other. Anim. Conserv. 15, 427–435. doi: 10.1111/j.1469-1795.2012.00548.x
Estes, J. A. (1991). Catastrophes and conservation: lessons from sea otters and the Exxon Valdez. Science 254:1596. doi: 10.1126/science.254.5038.1596
Estes, J. A., and Tinker, M. T. (2018). “Rehabilitating sea otters: feeling good versus being effective,” in Effective Conservation Science: Data Not Dogma, eds P. Kareiva, M. Marvier, and B. Silliman (Oxford: Oxford University Press), 128–134. doi: 10.1093/oso/9780198808978.003.0020
Greenfield, M. R., McHugh, K. A., Wells, R. S., and Rubenstein, D. I. (2020). Anthropogenic injuries disrupt social associations of common bottlenose dolphins (Tursiops truncatus) in Sarasota Bay, Florida. Mar. Mamm. Sci. 37, 1–16. doi: 10.1111/mms.12729
Hamilton, S., and Baker, G. B. (2019). Technical mitigation to reduce marine mammal bycatch and entanglement in commercial fishing gear: lessons learnt and future directions. Rev. Fish Biol. Fish. 29, 223–247. doi: 10.1007/s11160-019-09550-6
Harting, A. L., Johanos, T. C., and Littnan, C. L. (2014). Benefits derived from opportunistic survival-enhancing interventions for the Hawaiian monk seal: the silver BB paradigm. Endangered Species Res. 25, 89–96. doi: 10.3354/esr00612
Hays, S. A., Josephson, E., Maze-Foley, K., and Rosel, P. E. (eds) (2020). U.S. Atlantic and Gulf of Mexico Marine Mammal Stock Assessments – 2019. NOAA Technical Memorandum NMFS-NE-264. https://www.fisheries.noaa.gov/new-england-mid-atlantic/northeast-fisheries-science-center-publications (accessed December 17, 2020).
Johnson, A., and Mayer, K. (2015). “The value of rescuing, treating, and releasing live-stranded sea otters,” in Sea Otter Conservation, eds S. Larson, J. Bodkin, and G. VanBlaricom (Amsterdam: Elsevier Science & Technology), 235–255. doi: 10.1016/B978-0-12-801402-8.00009-3
Kraus, S. D., Kenney, R. D., Mayo, C. A., McLellan, W. A., Moore, M. J., and Nowacek, D. P. (2016). Recent scientific publications cast doubt on North Atlantic right whale future. Front. Mar. Sci. 3:137. doi: 10.3389/fmars.2016.00137
Lacy, R. C. (2000). Structure of the VORTEX simulation model for population viability analysis. Ecol. Bull. 48, 191–203.
Lacy, R. C., and Pollak, J. P. (2020). VORTEX: A Stochastic Simulation of the Extinction Process. Version 10.5.0. Brookfield, IL: Chicago Zoological Society. Software available at www.scti.tools/vortex
Lacy, R. C., Wells, R. S., Scott, M. D., Allen, J. B., Barleycorn, A. A., Urian, K. W., et al. (in preparation). Assessing the viability of the Sarasota Bay population of bottlenose dolphins. Front. Mar. Sci.
Laist, D. W. (1997). “Impacts of marine debris: entanglement of marine life in marine debris including a comprehensive list of species with entanglement and ingestion records,” in Marine Debris. Springer Series on Environmental Management, eds J. M. Coe and D. B. Rogers (New York, NY: Springer), doi: 10.1007/978-1-4613-8486-1_10
Marks, W., Burton, S., Stratton, E., Zolman, E., Biedenbach, G., and Page-Karjian, A. (2020). A case study of monofilament line entanglement in a common bottlenose dolphin (Tursiops truncatus): entanglement, disentanglement, and subsequent death. BMC Vet. Res. 16:223. doi: 10.1186/s12917-020-02436-x
Mayer, K. A., Tinker, M. T., Nicholson, T. E., Murray, M. J., Johnson, A. B., Staedler, M. M., et al. (2019). Surrogate rearing a keystone species to enhance population and ecosystem restoration. Oryx 1–11. doi: 10.1017/S0030605319000346
Moore, M., Early, G., Touhey, L., Barco, S., Gulland, F., and Wells, R. (2007). Rehabilitation and release of marine mammals in the United States: risks and benefits. Mar. Mamm. Sci. 23, 731–750. doi: 10.1111/j.1748-7692.2007.00146.x
Moore, M., Walsh, M., Bailey, J., Brunson, D., Gulland, F., Landry, S., et al. (2010). Sedation at sea of entangled North Atlantic right whales (Eubalaena glacialis) to enhance disentanglement. PLoS One 5:e9597. doi: 10.1371/journal.pone.0009597
Myers, H. J., Moore, M. M., Baumgartner, M. F., Brillant, S. W., Katona, S. K., Knowlton, A. R., et al. (2019). Ropeless fishing to prevent large whale entanglements: ropeless Consortium report. Mar. Policy 107:103587. doi: 10.1016/j.marpol.2019.103587
Nichol, C., Bejder, L., Green, L., Johnson, C., Keeling, L., Noren, D., et al. (2020). Anthropogenic threats to wild cetacean welfare and a tool to inform policy in this area. Front. Vet. Sci. 7:57. doi: 10.3389/fvets.2020.00057
Notarbartolo di Sciara, G., Hoyt, E., Reeves, R. R., Ardron, J., Marsh, H., Vongraven, D., et al. (2016). Place-based approaches to marine mammal conservation. Aquat. Conserv. 26, 85–100. doi: 10.1002/aqc.2642
Parsons, E. C. M., Baulch, S., Bechshoft, T., Bellazzi, G., Bouchet, P., Cosentino, A. M., et al. (2015). Key research questions of global importance for cetacean conservation. Endanger. Species Res. 27, 113–118. doi: 10.3354/esr00655
Powell, J. R., and Wells, R. S. (2011). Recreational fishing depredation and associated behaviors involving common bottlenose dolphins (Tursiops truncatus) in Sarasota Bay, Florida. Mar. Mamm. Sci. 27, 111–129. doi: 10.1111/j.1748-7692.2010.00401.x
Read, A. J. (2008). The looming crisis: interactions between marine mammals and fisheries. J. Mammal. 89, 541–548. doi: 10.1644/07-mamm-s-315r1.1
Read, A. J., Drinker, P., and Northridge, S. (2006). Bycatch of marine mammals in U.S. and global fisheries. Conserv. Biol. 20, 163–169. doi: 10.1111/j.1523-1739.2006.00338.x
Reeves, R. R., McClellan, K., and Werner, T. B. (2013). Marine mammal bycatch in gillnet and other entangling fisheries, 1990 to 2011. Endanger. Species Res. 20, 71–91. doi: 10.3354/esr00481
Reinert, T. R., Spellman, A. C., and Bassett, B. L. (2017). Entanglement in and ingestion of fishing gear and other marine debris by Florida manatees, 1993 to 2012. Endanger. Species Res. 32, 415–427. doi: 10.3354/esr00816
Robbins, J., Knowlton, A. R., and Landry, S. (2015). Apparent survival of North Atlantic right whales after entanglement in fishing gear. Biol. Conserv. 191, 421–427. doi: 10.1016/j.biocon.2015.07.023
Titcomb, E. M., O’Corry-Crowe, G., Hartel, E. F., and Mazzoil, M. S. (2015). Social communities and spatiotemporal dynamics of association patterns in estuarine bottlenose dolphins. Mar. Mamm. Sci. 31, 1314–1337. doi: 10.1111/mms.12222
Wells, R. S. (2009). Learning from nature: bottlenose dolphin care and husbandry. Zoo Biol. 28, 1–17.
Wells, R. S. (2019). “Common bottlenose dolphin foraging: behavioral solutions that incorporate habitat features and social associates,” in Ethology and Behavioral Ecology of Odontocetes, Ethology and Behavioral Ecology of Marine Mammals, ed. B. Würsig (Cham: Springer Nature Switzerland), 331–344. doi: 10.1007/978-3-030-16663-2_15
Wells, R. S., Allen, J. B., Hofmann, S., Bassos-Hull, K., Fauquier, D. A., Barros, N. B., et al. (2008). Consequences of injuries on survival and reproduction of common bottlenose dolphins (Tursiops truncatus) along the west coast of Florida. Mar. Mamm. Sci. 24, 774–794.
Wells, R. S., Allen, J. B., Lovewell, G., Gorzelany, J., Delynn, R. E., Fauquier, D. A., et al. (2015). Carcass recovery rates for resident bottlenose dolphins in Sarasota Bay, Florida. Mar. Mamm. Sci. 31, 355–368. doi: 10.1111/mms.12142
Wells, R. S., and Scott, M. D. (1990). “Estimating bottlenose dolphin population parameters from individual identification and capture-release techniques,” in Individual Recognition of Cetaceans: Use of Photo-Identification and Other Techniques to Estimate Population Parameters. Report of the International Whaling Commission, Special Issue 12, eds P. S. Hammond, S. A. Mizroch, and G. P. Donovan (Cambridge: International Whaling Commission), 407–415.
Wells, R. S., and Scott, M. D. (1994). “Incidence of gear entanglement for resident inshore bottlenose dolphins near Sarasota, Florida,” in Gillnets and Cetaceans, Report of the International Whaling Commission, Special Issue 15, eds W. F. Perrin, G. P. Donovan, and J. Barlow (Cambridge: International Whaling Commission), 629.
Wells, R. S., Fauquier, D. A., Gulland, F. M., Townsend, F. I., and DiGiovanni, R. A. Jr. (2013). Evaluating post-intervention survival of free ranging odontocete cetaceans. Mar. Mamm. Sci. 29, 463–483.
Wells, R. S., Hofmann, S., and Moors, T. L. (1998). Entanglement and mortality of bottlenose dolphins, Tursiops truncatus, in recreational fishing gear in Florida. Fish. Bull. 96, 647–650.
Wells, R. S., Scott, M. D., and Irvine, A. B. (1987). “The social structure of free-ranging bottlenose dolphins,” in Current Mammalogy, Vol. 1, ed. H. Genoways (New York, NY: Plenum Press), 247–305. doi: 10.1007/978-1-4757-9909-5_7
Wells, R. S., Scott, M. D., Hohn, A. A., Sweeney, J. C., Townsend, F. I., Allen, J. B., et al. (in preparation). Life history and demographic parameters for common bottlenose dolphins (Tursiops truncatus) in Sarasota Bay, FL. Front. Mar. Sci.
Whitehead, H. (2008). Analyzing Animal Societies: Quantitative Methods for Vertebrate Social Analysis. Chicago, IL: University of Chicago Press.
Whitehead, H. (2009). SOCPROG programs: analysing animal social structures. Behav. Ecol. Sociobiol. 63, 765–778. doi: 10.1007/s00265-008-0697-y
Keywords: entanglement, rescue, rehabilitation, bottlenose dolphin, population modeling, case evaluation, anthropogenic injury, Tursiops
Citation: McHugh KA, Barleycorn AA, Allen JB, Bassos-Hull K, Lovewell G, Boyd D, Panike A, Cush C, Fauquier D, Mase B, Lacy RC, Greenfield MR, Rubenstein DI, Weaver A, Stone A, Oliver L, Morse K and Wells RS (2021) Staying Alive: Long-Term Success of Bottlenose Dolphin Interventions in Southwest Florida. Front. Mar. Sci. 7:624729. doi: 10.3389/fmars.2020.624729
Received: 01 November 2020; Accepted: 28 December 2020;
Published: 18 January 2021.
Edited by:
Lars Bejder, University of Hawai‘i at Mānoa, United StatesReviewed by:
Albert Harting, Harting Biological Consulting, United StatesCopyright © 2021 McHugh, Barleycorn, Allen, Bassos-Hull, Lovewell, Boyd, Panike, Cush, Fauquier, Mase, Lacy, Greenfield, Rubenstein, Weaver, Stone, Oliver, Morse and Wells. This is an open-access article distributed under the terms of the Creative Commons Attribution License (CC BY). The use, distribution or reproduction in other forums is permitted, provided the original author(s) and the copyright owner(s) are credited and that the original publication in this journal is cited, in accordance with accepted academic practice. No use, distribution or reproduction is permitted which does not comply with these terms.
*Correspondence: Katherine A. McHugh, a21jaHVnaEBtb3RlLm9yZw==
Disclaimer: All claims expressed in this article are solely those of the authors and do not necessarily represent those of their affiliated organizations, or those of the publisher, the editors and the reviewers. Any product that may be evaluated in this article or claim that may be made by its manufacturer is not guaranteed or endorsed by the publisher.
Research integrity at Frontiers
Learn more about the work of our research integrity team to safeguard the quality of each article we publish.