- 1Departamento de Biología Marina, Facultad de Ciencias del Mar, Universidad Católica del Norte, Coquimbo, Chile
- 2ANID – Millennium Science Initiative Program – Millenium Nucleus for Ecology and Sustainable Management of Oceanic Islands (ESMOI), Departamento de Biología Marina, Universidad Católica del Norte, Coquimbo, Chile
- 3Departamento de Ecología y Biodiversidad, Facultad de Ciencias de la Vida, Universidad Andres Bello, Santiago, Chile
Heterogeneous environmental conditions along the Humboldt Current System (HCS) influence the life-history strategy of a variety of species in different ways. There is limited information on latitudinal traits of coastal cephalopods as part of the interacting species in pelagic and benthic environments. The present study used the loliginid squid Doryteuthis gahi as a model organism to: (1) evaluate latitudinal traits on egg laying patterns, (2) characterize a particular spawning ground, (3) quantify the isotopic variation from different tissues, (4) evaluate potential trophic ontogenetic changes, (5) determinate trophic position, and (6) isotopically estimate the relative importance of putative preys in the squid’s assimilated diet. Results evidenced that egg-masses collected between 2014 and 2020 presented similar patterns along northern-central Chile (27–36°S), with females attaching small egg-capsules (10–50 mm length) through the year and over a variety of anthropogenic and natural substrates. At a small scale (Coquimbo; 29°S), early life history traits showed distinct patterns depending on SST, with warmer collection periods (∼18°C) evidencing larger capsules and smaller embryos, although small paralarvae were obtained over the 4-year sampling period. In this site, sampling of older ontogenetic stages supported the constant presence of small-sized squids (19–77 mm ML). Males had larger mean sizes compared to females and undetermined specimens, with a high proportion of mature stages. Observations in captivity were extended for up to 110 d, validating that small females (45–64 mm ML) spawn the small egg-capsules typically observed in the field. Differences in stable isotope composition between beaks and soft tissues of adult squids were lower for δ13C values (1.1 vs. 1.4‰, respectively) and higher for δ15N values (5.3 vs. 4.7‰, respectively). Isotopic composition through ontogeny found similar δ13C and δ15N values, suggesting that carbon sources (pelagic origin) and trophic position did not change significantly, with copepods, euphausiids and nereid polychaetes being the most important preys. This study unveils the permanent occurrence of a small reproductive morphotype of D. gahi in shallow coastal habitats of northern-central Chile (i.e., center of the distribution range), providing the first insights for understanding the species’ potential adaptations to heterogeneous conditions in the HCS and the unexplored distribution gap between the two centers of abundance (Peru and the Falkland/Malvinas islands).
Introduction
The effects of environmental changes, either natural or anthropogenic, usually result in direct consequences for reproductive traits, including age at first reproduction, fecundity, parental investment, and spawning habitats (Byrne, 2011). In species with complex life-history strategies, like most marine species, development in a multi-stressor world can change at any stage; therefore, understanding the onset of environmental fluctuations on planktonic (e.g., embryos, larvae) and benthic/pelagic (e.g., juvenile, adults) life-history stages is crucial to identify their ontogenetic-associated vulnerabilities (Byrne, 2011). Consequently, evaluating species’ latitudinal and/or longitudinal distributions, and their associated reproductive traits, may provide important information on how different species (and so populations) will respond to environmental gradients (Fernández et al., 2007).
Among the diversity of marine species dealing with environmental variability, cephalopods are thought to be especially sensitive as they have a unique set of life-history characteristics such as rapid and labile growth, short lifespans, and strong life-history plasticity; that allow them to quickly adapt to changing environmental conditions (Pierce et al., 2008). The extreme flexibility exhibited through their life-cycles, the increased population abundances (Doubleday et al., 2016), and the rapid ways of turnover at the population level (e.g., invasive range expansion of the Humboldt squid Dosidicus gigas; Zeidberg and Robison, 2007), suggest that cephalopods’ responses to changing environmental conditions are likely to be much more complex than in other co-occurring taxa, as fish species, especially during larval and juvenile phases (Pecl and Jackson, 2008; Pierce et al., 2008; Pimentel et al., 2012).
In this context, the Southeast Pacific provide an interesting scenario to evaluate differential effects of environmental variability at latitudinal and longitudinal gradients. Along the Chilean coast (∼3000 km of coastline), nutrient-rich, cold waters characterize the environmental conditions in the Humboldt Current System (HCS; Thiel et al., 2007), providing an appropriate environment for ∼86 species of cephalopods between 18° and 56°S (Ibáñez et al., 2009). However, most information on this group has been focused only on commercial species, including three octopuses (Octopus mimus, Enteroctopus megalocyathus, and Robsonella fontaniana), and two squids (Dosidicus gigas, Doryteuthis gahi) (see Rocha and Vega, 2003; Ibáñez et al., 2010). Among coastal species, the loliginid squid D. gahi, is the only one inhabiting through the entire southeastern Pacific, from Northern Peru to Southern Chile. The distribution of this small species [130–170 mm dorsal mantle length (ML)] has been proposed from southern Peru and Chile in the Pacific Ocean, to southern Argentina and the Falklands/Malvinas Islands in the Atlantic Ocean (Jereb and Roper, 2010), with two population units being detected, the Peruvian group and the Chilean – Falklands/Malvinas group (Ibáñez and Poulin, 2014; McKeown et al., 2019). In fact, it has been assumed that populations off Peru and Chile are connected by squids that occur in northern Chile (Jereb and Roper, 2010; Arkhipkin et al., 2015b); however, there are only few records of D. gahi occurrence in the region between 20°S and 36°S (Ibáñez et al., 2012; Carrasco and Pérez-Matus, 2016).
Members of the family Loliginidae, such as the Patagonian long-finned squid D. gahi, have a sessile benthic egg stage and tend to be neritic in habit, with seasonal distributions that shift between shallow coastal waters and deeper offshore waters along continental shelves (Boyle and Rodhouse, 2005; Arkhipkin et al., 2013). Consequently, loliginid populations are physically structured by shallow water topography and seasonal variations in water temperature, affecting embryonic development, growth rates, and so the timing at which early life stages would be subject to intense predation (Arkhipkin et al., 2013). In the case of D. gahi, the biology and ecology of the species is mostly known from the Falkland/Malvinas population (Arkhipkin et al., 2013), with few studies describing life-history traits along its Pacific distribution (Guerra et al., 2001; Villegas, 2001; Cardoso et al., 2005; Ibáñez et al., 2005; Carrasco et al., 2016).
Through the Southeast Pacific distribution range, D. gahi has been recognized as a prey item for a variety of coastal fishes (González and Chong, 1997; Cubillos et al., 2003; González and Oyarzún, 2003; Chong et al., 2006) and one mammal (Sielfeld et al., 2018); nonetheless, its role as an opportunistic and efficient predator has been scarcely assessed through pelagic food webs in the HCS (Guerra et al., 1991). Information regarding the species diet has been mostly obtained from the South Atlantic region, evidencing that planktonic crustaceans (e.g., euphausiids, amphipods, and copepods) are the most important components, whereas fish may have a minor contribution as a prey (Guerra et al., 1991; Arkhipkin et al., 2013). However, in the Eastern Pacific (Peru), the fish Odontesthes regia and nereid polychaetes (Pseudonereis gallapagensis and Nereis callaona) were identified as important components of stomach contents (Cardoso et al., 1998). In Chilean waters, the trophic role of D. gahi in coastal food webs is still unknown, being practically absent from ecological studies regarding pelagic and/or benthic communities from the HCS (see Thiel et al., 2007; Espinoza et al., 2017; Pérez-Matus et al., 2017; Pizarro et al., 2019).
Considering the overlooked ecological interactions involving the squid D. gahi in coastal environments of the HCS, and especially in the distribution gap between 12 and 34°S, this study aimed to: (1) provide latitudinal observations on spawning habitats and egg laying patterns along the Chilean coast, (2) characterize a particular spawning ground based on different ontogenetic stages collected from the field, (3) quantify and determine variability of isotopic composition among beaks and soft tissues (arms and mantle), (4) evaluate potential trophic ontogenetic changes, (5) determinate the trophic position of this coastal squid in relation to other pelagic consumers, and (6) isotopically estimate the relative importance of putative preys on the squid’s assimilated diet.
According to the latest information available for D. gahi regarding spawning habitats in central Chile (Carrasco and Pérez-Matus, 2016) and population genetic studies through most of the distribution range (McKeown et al., 2019), we hypothesized that: (1) The existing distribution gap from southern Peru to central Chile will be characterized by the occurrence of small (= low fecundity) squids, which is evidenced in the small size of the egg capsules (with few encapsulated embryos) permanently observed through the northern-central Chilean coast (i.e., “abundant edge distribution”), and (2) other than evident differences in size (and so reproductive traits), the ecological and/or functional role of the small Humboldt specimens (e.g., diet, trophic position) will be comparable to that in larger specimens occurring in the main abundance centers.
Materials and Methods
Latitudinal Traits on Spawning Habitats
In addition to previous published records, updated information on sporadic collections of egg masses of D. gahi along the Chilean coast from 2014 to 2020 [i.e., ∼3,500 km of coast; from Caldera (27°S) to Punta Arenas (53°S); Figure 1a] was summarized and typified using actual photographic records from the field and morphometric approaches. Whenever possible, the collected egg masses were evaluated in laboratory including the following standard measurements: capsule length (mm), number of encapsulated embryos, egg size (mm), and paralarvae size (mantle length; ML, mm) (for details on methods see Carrasco and Pérez-Matus, 2016).
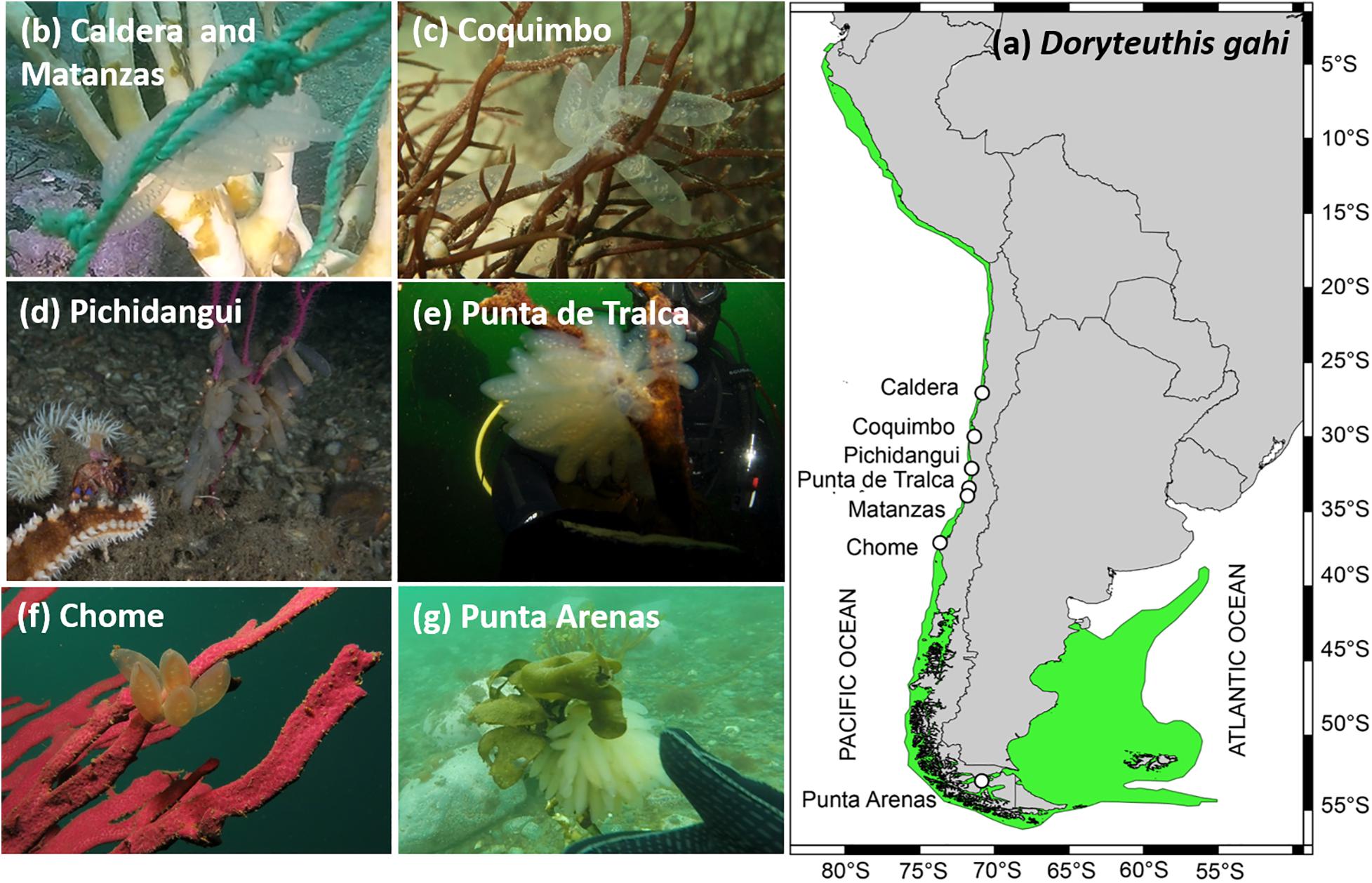
Figure 1. Schematic representation of spawning sites and substrates recorded from 2014 to 2020 along the Chilean Coast, where: (a) geographic distribution of Doryteuthis gahi in green; (b) Caldera and Matanzas: rope mooring; (c) Coquimbo: Sarcodiotheca gaudichaudii; (d) Pichidangui: Gorgoniidae; (e) Punta de Tralca: defoliated Lessonia trabeculata; (f) Chome: Gorgoniidae; (g) Punta Arenas: Lessonia flavicans. Photo credits: (b,c) Sergio Carrasco, (d) Alejandro Soza, (e) Alejandro Pérez-Matus, (f) Iván Hinojosa, and (g) César Cárdenas.
Characterization of a Spawning Ground
Early Life Stages
From all locations presented above, and due to its proximity to the laboratory, a permanent sampling site was selected at La Herradura Bay, Coquimbo, northern-central Chile (29°S; Figure 1a). In this site, fifteen subtidal surveys were carried out from May 2016 to March 2020, and whenever possible, egg masses were hand-collected by SCUBA diving at depths between 6 and 30 m. Samples were transported in seawater to the laboratory and each egg mass placed in a 20 L plastic container with constantly flowing fresh seawater at ambient temperature (range: 12–15°C in winter, and 16–20°C in summer). For each sampling date, sea surface temperature (SST, °C) was also obtained from the Hydrographic and Oceanographic Service of the Chilean Navy1 databases, allowing further contrasts with the early life stages analyzed. Morphometric traits were evaluated in fresh samples, including number of capsules per egg mass, capsule size (mm), number of encapsulated embryos, egg size (mm), and paralarvae size (mm). Only eggs in early developmental stages (i.e., stage 16–17; see Guerra et al., 2001) and recently hatched paralarvae [i.e., 48 h post-hatch; mantle length (ML, mm)] were considered for morphometry (see Carrasco and Pérez-Matus, 2016). All measurements were performed by using a manual caliper and a dissecting scope at 20X magnification (Zeiss Stemi 2000-C) at Sala de Colecciones Biológicas, Universidad Católica del Norte (SCBUCN). At this time, egg capsules and paralarvae were frozen at −20°C for further stable isotope analyses (see below sections). In order to assess differences in early life-stages (i.e., capsules, eggs, and paralarvae size) among sampling temperatures (SST), independent Generalized Least Square Models (GLS) were performed for the evaluated traits. In each case, normality and homoscedasticity were previously checked by using Shapiro–Wilk and Levene’s tests, respectively.
Juvenile and Adult Stages
Collections of juvenile and adult stages were conducted during four nocturnal surveys (20:30 PM to 01:30 AM) carried out between October 2018 and March 2020 in different points of the same bay (La Herradura). Squids were attracted to the boat by light fishing, carefully collected with a hand net, and individualized into 10 or 20 L plastic containers depending on the specimens’ size. In the case of collecting a considerable fraction of the school (i.e., up to 60 individuals), specimens were maintained at a maximum density of 3–4 individuals per 20 L container, and carefully transported to the laboratory located nearby within the same bay. In general, squids from low density collections remained individualized into the same 20 L containers with running seawater at ambient temperature (16.05 ± 1.4°C; mean ± SD). However, at high density collections, individuals were separated into two size classes (20–40 and 50–70 mm ML) and placed in two 3,000 L flow-through tanks, allowing them to maintain schools of around 30 individuals each. In all cases, squids were fed at a daily basis with amphipods, decapod megalopae, shrimps, and fish (depending on availability). Survival was also evaluated at that time, with any dead individual being removed from the tank to be sexed, measured with a manual caliper to the nearest millimeter (ML, mm), and weighed (g) using a digital balance (Radwag WTC 2000). All specimens were frozen at −20°C. Stomach content was also visually inspected by ventrally dissecting six adult specimens (i.e., 1–2 survival days in laboratory); however, considering that all samples evidenced highly digested food with no recognizable prey remains, no further analyses were carried out. Cannibalized squids from the laboratory (n = 20) were excluded from the analysis as only the mantles (i.e., no head or arms/tentacles) were recovered. With this information, a length-weight relationship was calculated using most specimens collected (46 out of 76) regardless the survival time in captivity (from 1 to 110 days). A power function model was used to evaluate the relationship between ML and weight. Additionally, ML of males, females, and undetermined juveniles, was contrasted with an ANOVA test after normality and homoscedasticity were evaluated using Shapiro–Wilk and Levene’s tests, respectively. Frequency distributions of macroscopic maturity stages (immature, maturing, and mature) between male and females were analyzed using a 2-sample test for equality of proportions with Yate’s correction (Proportion test). Accordingly, the maturing stage was characterized by the absence of spermatophores in males and the development of the nidamental glands in females.
Stable Isotope Analyses
Sample Collection and Preparation
As described above, benthic egg capsules of D. gahi were collected during subtidal surveys, whereas juvenile/adult squids, small juvenile fishes, zooplankton (e.g., copepods, euphausiids, brachyuran zoeae), and emergent benthic fauna (e.g., amphipods, nereid polychaetes) were collected during nocturnal surveys. For squids’ egg capsules (post-spawning capsules without embryos) and newly hatched paralarvae, complete individuals were considered in the analyses. In the case of juvenile and adult squids, mantle, arms, and lower beaks were dissected to compare potential differences in stable isotopes values. Samplings from lower beaks were collected as suggested for several species of squids (Cherel and Hobson, 2005; Cherel et al., 2009a, b, 2019; Xavier et al., 2015) and benthic octopuses (Guerreiro et al., 2015; Matias et al., 2019). For juvenile fish, muscle pieces were sampled, whereas for euphausiids, amphipods and polychaetes, complete individuals were used. Due to their small sizes and weights, samples of copepods and brachyuran zoeae were obtained by pooling ∼ 10 individuals to complete the necessary mass. In all cases, around 10 mg of wet tissue (in order to get ∼1 mg dry mass) was obtained, washed with Milli-Q water, placed in pre-combusted vials, and dried in an oven (60°C) for 48 h to be grounded into a fine powder with an agate mortar. Small amounts (∼0.5 mg) were placed in pre-weighed tin capsules and stored in a desiccator until the shipment for stable isotope analyses. When possible, voucher specimens were preserved in 95% ethanol and deposited at Sala de Colecciones Biológicas Universidad Católica del Norte (SCBUCN) for further taxonomic corroboration and genetic analyses. These ethanol-preserved specimens are also suitable for future isotopic analyses (see Pauli et al., 2017).
Carbon (δ13C) and Nitrogen (δ15N) Stable Isotope Ratios
Analyses of carbon (δ13C) and nitrogen (δ15N) stable isotope ratios were conducted at UC Davis Stable Isotope Facility, using a PDZ Europa ANCA-GSL elemental analyzer interfaced to a PDZ Europa 20-20 isotope ratio mass spectrometer (IRMS, Sercon Ltd., Cheshire, United Kingdom). Samples were combusted at 1000 °C in a reactor packed with chromium oxide and silvered copper oxide. Following combustion, oxides were removed in a reduction reactor (reduced copper at 650°C). The helium carrier then flowed through a water trap (magnesium perchlorate and phosphorous pentoxide). N2 and CO2 were separated on a Carbosieve GC column (65°C, 65 mL/min) before entering the IRMS. Stable isotope ratios were reported in the δ notation as the deviation relative to international standards (Vienna Pee Dee Belemnite for δ13C and atmospheric N2 for δ15N), so δ13C or δ15N = [(R sample /R standard) – 1], where R is 13C/12C or 15N/14N, respectively. Typical precision of the analyses was ±0.2‰ for δ13C and ±0.3‰ for δ15N.
Lipid Normalization
Since lipids are 13C-depleted relative to protein (DeNiro and Epstein, 1977), lipid normalization was applied for invertebrate and fish using C:N ratios (molar) [Eq. (3) in Post et al., 2007]. This correction was applied to samples with C:N values > 3.5 (>5% lipid content) according to the following equation:
Stable Isotope Composition on Squids’ Tissues and Ontogenetic Variability
In order to determine differences in mean values of δ13C and δ15N among different squid’s body parts (beaks, mantle, and arms), stable isotope values were independently compared through analyses of variance (ANOVA). Previous to the analyses, normality and homoscedasticity were tested with Shapiro-Wilk and Levene’s tests, respectively. Parametric post hoc Tukey’s tests were used to determine statistical differences in isotopic values among tissues.
To detect potential changes in prey selection and trophic position of D. gahi through ontogeny, Pearson’s correlation coefficient was used to determine the relationship between mantle length (i.e., 2 to 70 mm ML) with δ13C and δ15N values from mantle tissues, respectively. Mantle tissue was selected because it is more stable through ontogeny than arm tissue, which could be damaged and regenerated due agonistic and/or predatory-prey interactions. Data normality was tested through the Shapiro-Wilk test.
Estimating Trophic Position
To assess the status of D. gahi within the pelagic food web, we estimated the trophic position for different consumers (e.g., squids, invertebrates, and fish) using the “oneBaseline” model, incorporating uncertainty in the trophic discrimination factor (TDF) for muscle nitrogen (mean ± SD Δ15N = 3.4 ± 0.1‰) (Post, 2002), as well as baseline and consumer δ15N values through Bayesian inference with tRophicPosition package (Quezada-Romegialli et al., 2018). Assuming that pelagic species of squids, invertebrates, and fish, could be supported mainly by pelagic carbon and nitrogen (Thiel et al., 2007), copepods were used as baseline with a mean trophic position of 2.2 (see Chen et al., 2018). Models were run with 2 chains, 20,000 adapting samplings, and 20,000 iterations.
Contribution of Putative Preys to Squid’s Assimilated Diet
To evaluate the relative contribution of six potential preys (n ≥ 3 individuals), including four invertebrates (i.e., Euphausia mucronata, Heterophoxus oculatus, Pseudonereis gallapagensis, and cyclopoida copepods) and two fishes (Odontesthes regia and Hypsoblennius sordidus) to the assimilated diet of D. gahi population (estimated for both mantle and arm tissue), Bayesian stable isotope mixing models were used (MixSiar; Stock et al., 2018) employing δ13C and δ15N values as tracers and elemental concentration from preys (concentration-dependence model). The TDF values for muscle (see trophic position methods above) were taken from a global meta-analyses (Post, 2002). The error term “Process∗ Residual” was selected for the mixture (i.e., consumers). The residual error incorporates the potential variation associated with consumers (e.g., different metabolic rate, assimilation efficiency or digestibility), whereas the process error incorporates the variation associated to sampling process and consumer specialization (Stock and Semmens, 2016). Uninformative priors (all values between 0 and 1 are likely equally) were used in the models. MixSiar were run using a “normal” chain length through Markov Chain Monte Carlo (MCMC) and assessed through Gelman-Rubin and Geweke diagnostic test (Stock and Semmens, 2016).
All statistical analyses were performed in RStudio (R Studio Team, 2020).
Results
Latitudinal Traits on Spawning Habitats
Field samplings and observations over the last 6 years (2014-2020) evidenced that egg masses of D. gahi presented similar laying patterns along the Chilean coast (27°S to 53°S), with females attaching the translucent and gelatinous egg capsules through the year and over a variety of structures, including anthropogenic substrates such as rope mooring in Caldera and Matanzas (Figure 1b), and natural substrates such as the red algae Sarcodiotheca gaudichaudii in Coquimbo (Figure 1c), cold-water corals Family Gorgoniidae in Pichidangui and Chome (Figures 1d,f), heavily grazed stipes of kelp Lessonia trabeculata in Punta de Tralca (Figure 1e), and solitary stipes of kelp Lessonia flavicans in Punta Arenas (Figure 1g) (see details in Table 1). In general, morphological traits of egg capsules and early stages of development from northern-central Chile evidenced similar patterns, with typical egg capsules ranging from 10 to 40 mm length (Table 1). The presence of larger capsules seems to be consistent in the coldest and southernmost site (Punta Arenas; 53°S), although one egg mass recorded in northern Chile (Coquimbo; 29°S) also contained large capsules (n = 10 capsules; range: 72–96 mm; Table 2). Accordingly, the number of encapsulated embryos and paralarvae size (ML, mm) from Punta Arenas were 3-fold and 1.1-fold larger than the bigger counterparts from central Chile (Valparaíso; 32°S) (i.e., 76 vs. 25; and 3.2 vs. 2.8 mm, respectively) (Table 1).
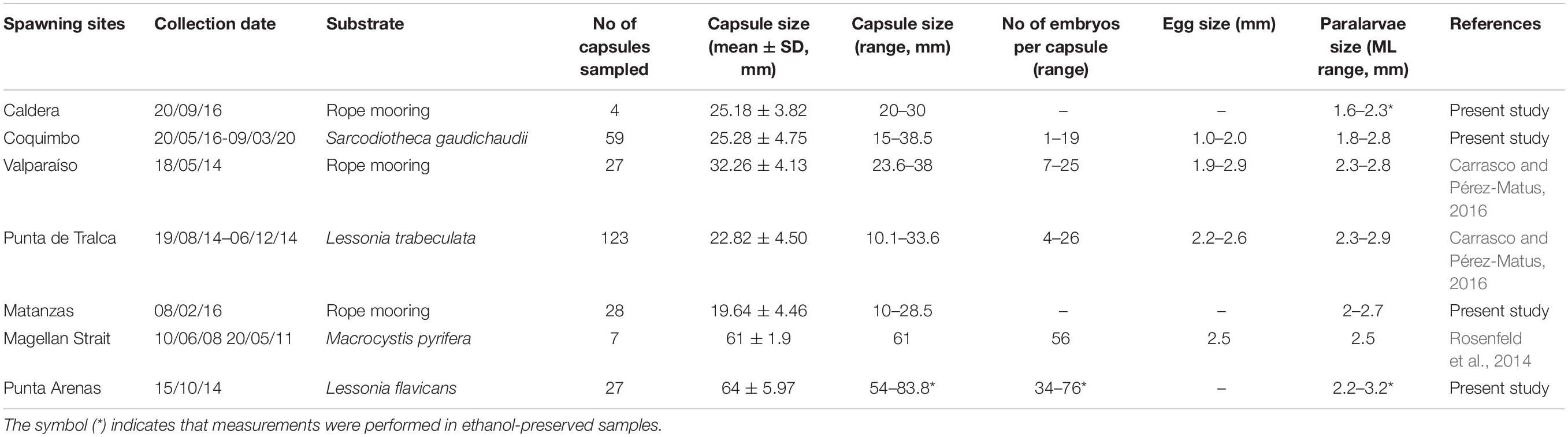
Table 1. Morphometric traits on egg capsules and early developmental stages (i.e., eggs and paralarvae) of Doryteuthis gahi recorded in different spawning sites and substrates along the Chilean Coast from 2014 to 2020 (27° to 53°S; Figure 1).
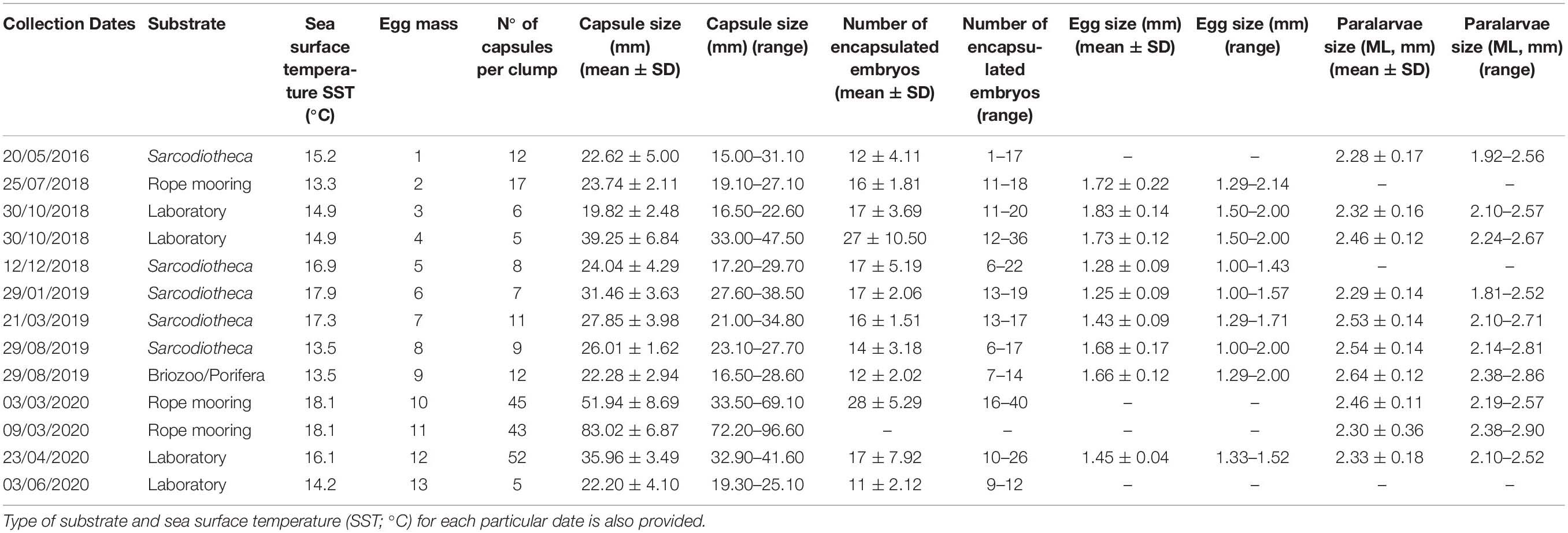
Table 2. Morphometric traits of egg capsules and early developmental stages (i.e., eggs and paralarvae) of Doryteuthis gahi collected from field and laboratory spawnings between 2016 and 2020 (Coquimbo; 29° 58’ S; Figure 1).
Characterization of a Spawning Ground
Early Life Stages
As in latitudinal observations above, when considering a particular spawning site (i.e., La Herradura bay, Coquimbo), egg capsules were obtained from field and laboratory conditions during different months of the 4-year observation period. Overall, successful collections were recorded in January, March, April, May, June, July, August, October, and December (Table 2), with early life history traits (i.e., capsule, eggs, and paralarvae) evidencing distinct patterns depending on SST at the specific collection date. Egg capsules showed a significant positive association with sampling temperatures (β = 6.51; t = 11.41; p < 0.001), with larger capsules being recorded during warmer periods of around 18°C experienced during 2020 (range: 19–96 mm; n = 62; Table 2; and Figure 2a), compared with smaller capsules recorded during colder periods of around ∼15.5 during years 2016, 2018, and 2019 (range: 15–38 mm; n = 87; Table 2; and Figure 2a). Conversely, egg size evidenced a significant negative association with sampling temperatures (β = −0.10; t = −34.20; p < 0.001), with smaller eggs being observed during warmer periods of 2020 (range: 1.33–1.52; n = 87), in comparison with egg sizes observed during the colder years 2018 (range: 1.00–2.14 mm; n = 509) and 2019 (range: 1.00–2.00 mm; n = 559; Table 2; and Figure 2b). In the other hand, paralarvae size showed a weak but significant negative association with sampling temperatures (β = −0.032; t = −3.60; p < 0.001), although small individuals (<2 mm ML) were spread over the 4-year sampling period (n = 172; Table 2; and Figure 2c).
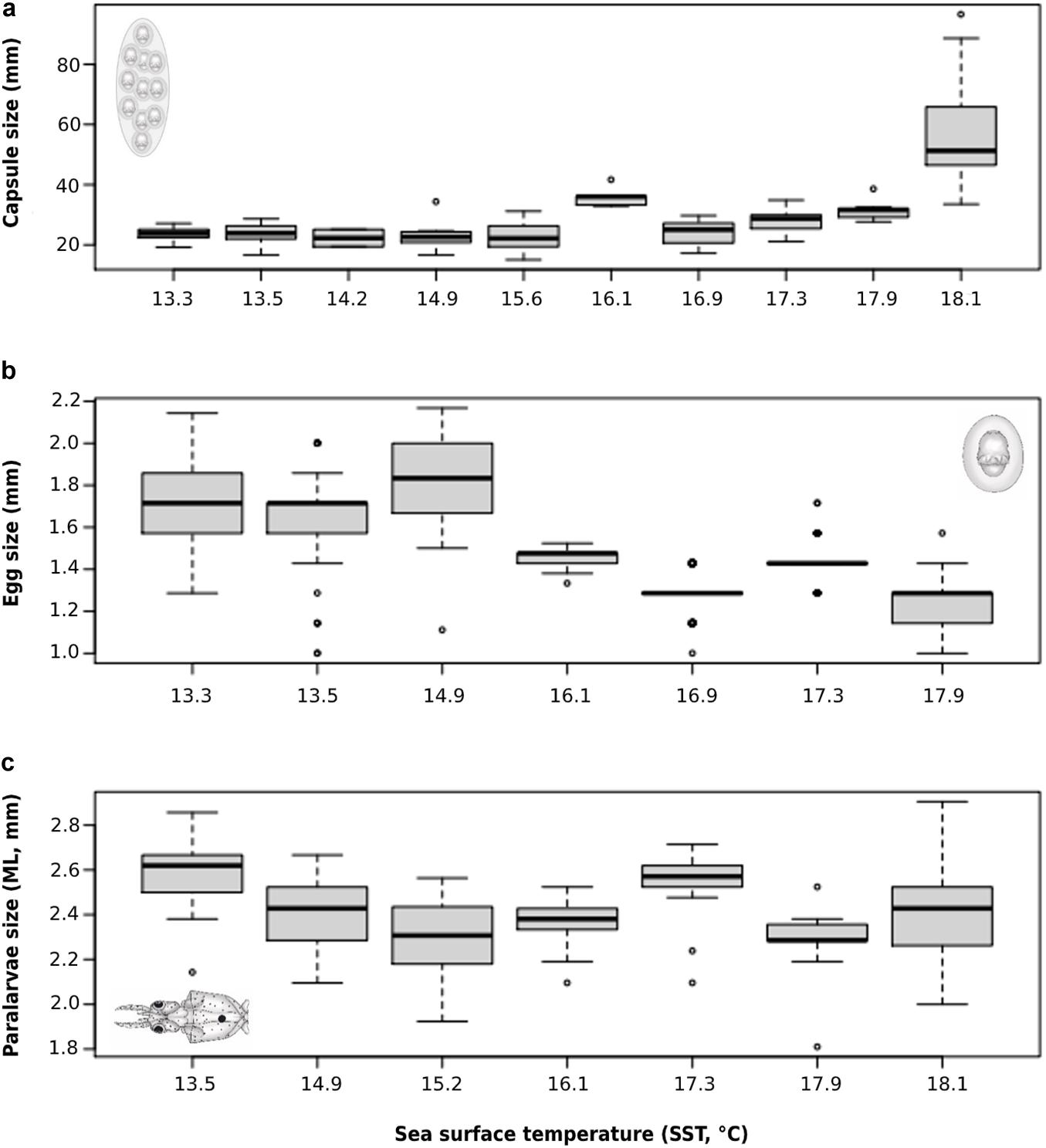
Figure 2. Early life-history traits of D. gahi collected at Herradura Bay, Coquimbo, between 2016 and 2020 under different sea surface temperature (SST) conditions, where: (a) capsule size (mm), (b) egg size (mm), and (c) paralarvae size (ML, mm). Central horizontal lines indicate median.
In general, egg capsules were attached to a variety of available substrata, including the typical rope moorings (from 6 to 20 m depth), the red alga Sarcodiotheca gaudichaudii in shallow waters up to 10 m, and a mix of unidentified porifera and erect bryozoans in a deep collection of around 30 m (Table 2). Egg capsules (range: 15–96 mm) and paralarvae (range: 1.8–2.9 mm ML) presented similar morphological traits compared to previous collections from central Chile (Table 1), suggesting that similar adult sizes would be responsible for reproductive interactions and egg laying in these coastal environments.
Juvenile and Adult Stages
Samplings of older ontogenetic stages evidenced the constant presence of juvenile/adult squids through the year. In sum, 76 squids were collected with sizes ranging from around 19 to 77 mm ML and total wet weights from 0.3 to 15 g (Table 3). When combining all specimens collected (regardless survival time in laboratory), the power function model evidenced a strong positive relationship between squids’ mantle length (ML, mm) and weight (gr) (TW = 0.0014 ML2.642; t1,44 = 19.256, p < 0.001; Figure 3a).

Table 3. Morphometric and gravimetric traits of juvenile and adult Doryteuthis gahi collected between 2018 and 2020 at a single spawning site, La Herradura bay, Coquimbo (29° 58’ S).
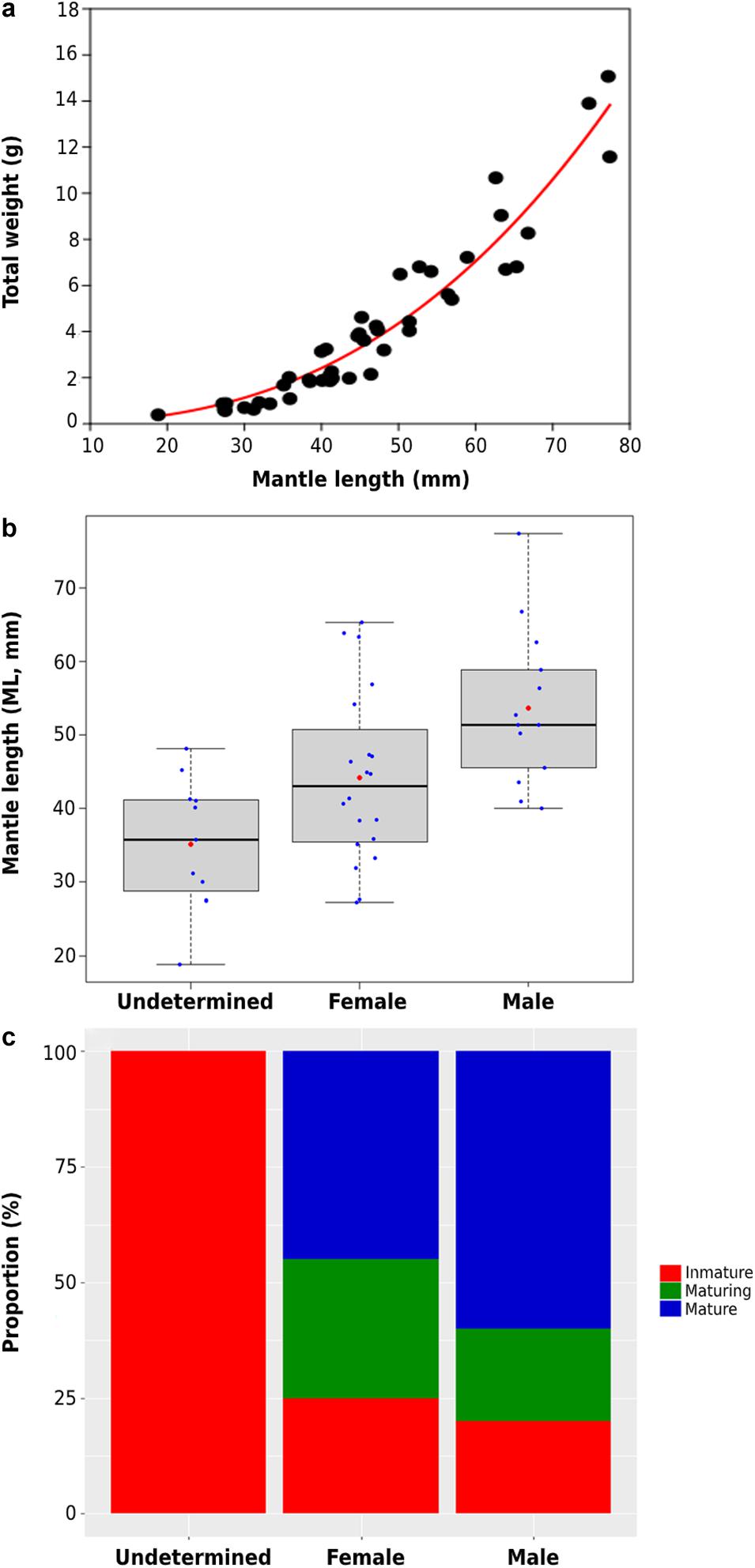
Figure 3. Juvenile and adult traits of D. gahi collected at La Herradura Bay, Coquimbo, between 2018 and 2020, where: (a) length-weight relationship, (b) size (ML, mm) for males, females, and undetermined specimens, and (c) maturity stage frequency for males and females.
From the forty-six specimens evaluated, 43% corresponded to females (n = 20), 33% to males (n = 15), and 24% to small juveniles of undetermined sex (n = 11). Mantle lengths (mean ± SD) evidenced significant differences (ANOVA, F2,41 = 8.912; p < 0.001), with males showing slightly larger sizes (54 ± 11 mm ML) compared to females (44 ± 11 ML mm ML; Tukey’s test: p = 0.045) and undetermined specimens (35 ± 9 mm) (Tukey’s test: p < 0.001) (Figure 3b). Frequency distribution of maturity stages evidenced that a high proportion of squids (i.e., 60% males and 45% females) corresponded to “mature” individuals, with sex evidencing no significant differences (Proportion test, chi2 = 0.288, df = 1, p = 0.59; Figure 3c). Similar results were observed between “maturing” (20% males and 30% females; Proportion test, chi2 = 0.077, df = 1, p = 0.78), and “immature” stages (20% males and 25% females; Proportion test, chi2 < 0.001, df = 1, p = 1) (Figure 3c).
In captivity, individualized squids from first collections (2018–2019) did not survive longer than 10 days (Table 3); however, two females of different size (45 and 64 mm ML) laid eggs in the tank’s walls and in the artificial structures provided. Morphometrics on those egg masses and paralarvae have been provided in Table 2 (see egg masses 3 and 4, respectively). In the other hand, squids maintained in schools (20–40 and 50–70 mm ML) survived for up to 110 days (Table 3), which to our knowledge correspond to the longest captivity time recorded for D. gahi to date. Females of both size classes laid eggs between 2 and 3 months under these controlled conditions, although larger specimens produced more and larger egg capsules (and so more eggs per capsule) compared with smaller squids (see egg masses 12 and 13, respectively; Table 2). Within each of the two size classes, nearly 20% of the mortality was due to cannibalism over smallest sizes, suggesting that similar behaviors (reproductive and trophic) could be structuring schools in the wild.
Stable Isotopes
Isotopic Composition of Beaks and Soft Tissues
The mean (±SD) δ13C values evidenced significant differences among the analyzed structures (ANOVA, F2,33 = 4.708, p = 0.0159), with beaks being significantly more 13C-enriched than arms (−16.1 ± 1.2‰ vs. −17.6 ± 1.2‰; Tukey’s test, p = 0.019). Mantle tissue had intermediate values (−17.4 ± 1.5‰), but did not show significant differences with arms or beaks (Tukey’s test, p > 0.05 in both cases; Figure 4a). The δ15N evidenced significant differences in the mean values (± SD) of the structures analyzed (ANOVA, F2,33 = 186.9, p < 0.001), with beaks being significantly lower (12.2 ± 0.7‰) in contrast to arms (16.9 ± 0.8‰) and mantle (17.5 ± 0.6‰) (Tukey’s test, p < 0.001 in both cases; Figure 4b). Intra-individual differences of δ13C and δ15N values between lower beak and soft tissues (i.e., mantle, arms) (correction factor) evidenced low differences for mean δ13C values (1.1 ± 0.6‰ vs. 1.4 ± 0.7‰, respectively; Table 4) and higher for δ15N values (5.3 ± 0.5‰ vs. 4.7 ± 0.4‰, respectively; Table 4).
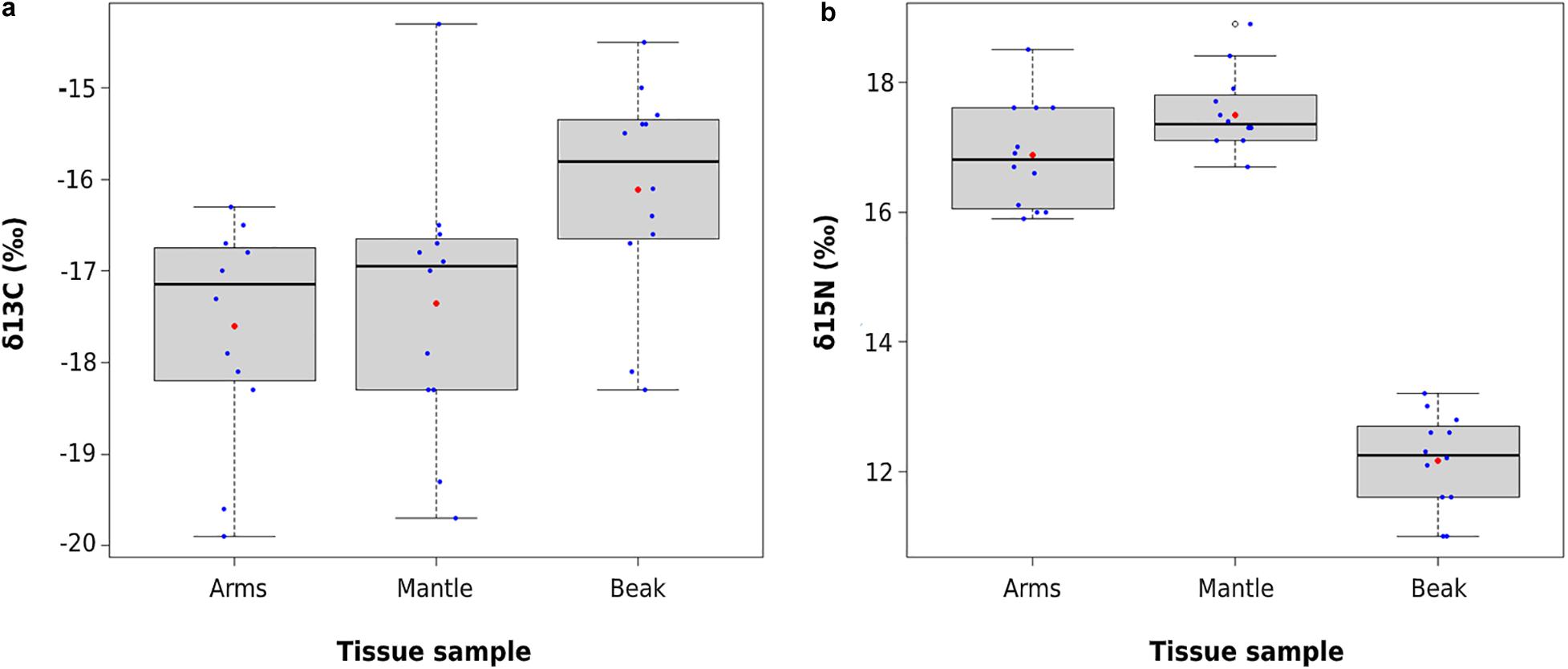
Figure 4. Boxplot of isotopic composition of D. gahi, where: (a) δ13C and (b) δ15N values for different tissues (arms, mantle, and beak). Red circles indicate mean value and blue circles indicate individual data points. Central horizontal lines indicate median.
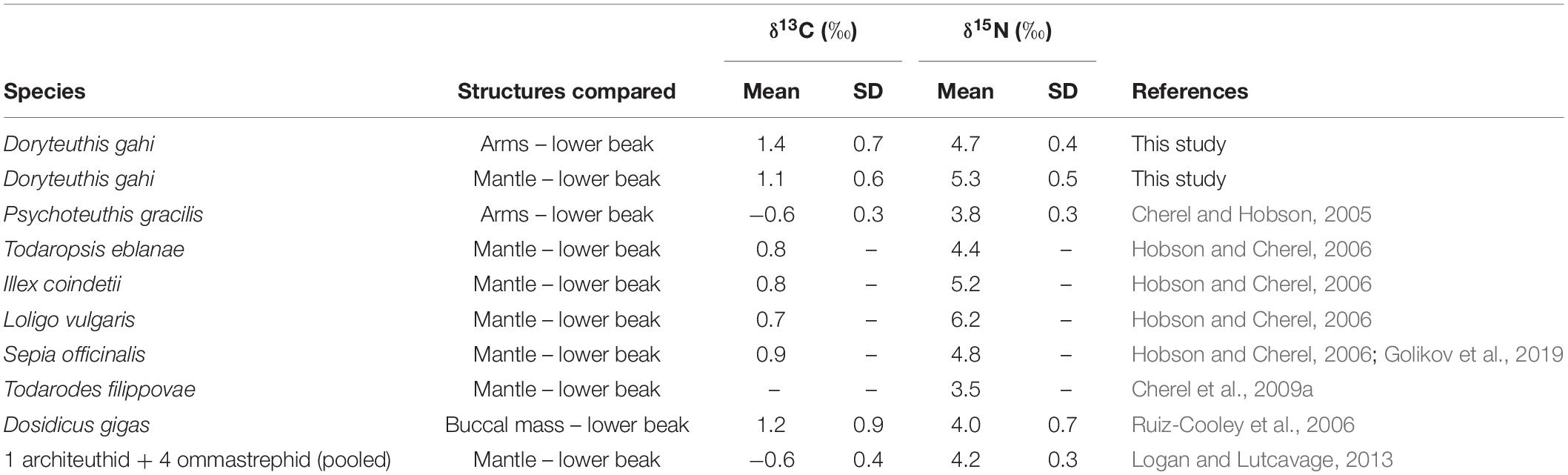
Table 4. Summary of estimated differences of δ13C and δ15N values (correction factor) between hard (beak) and soft tissues (e.g., mantle, arms and buccal mass) from Doryteuthis gahi and other cephalopods obtained from the literature.
Ontogenetic Changes on Squids’ Isotopic Composition
Pearson’s regressions evidenced a positive but weak relationship between squids’ mantle length (ML, mm) and both δ13C (y = 0.013x–18.27; r2 = 0.029, df = 12, p = 0.55; Figure 5a) and δ15N values (y = 0.0197x+16.382; r2 = 0.22, df = 12, p = 0.09; Figure 5b). These findings suggest that through ontogeny (e.g., 2, 40, and 60 mm ML; see Figures 5c–e, respectively), D. gahi may present similar carbon sources (i.e., pelagic origin) and only slight increments in their trophic position.
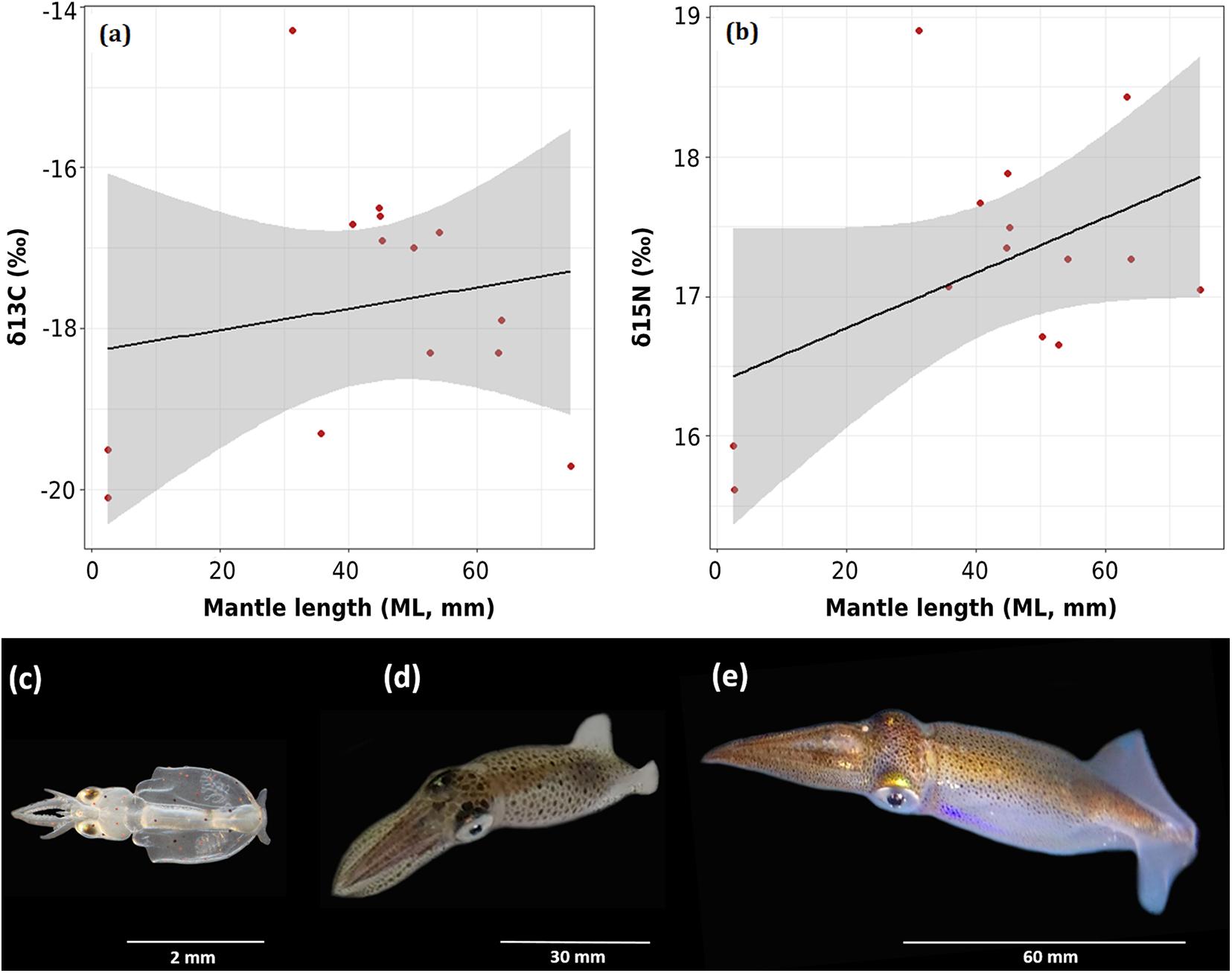
Figure 5. Ontogenetic changes in D. gahi, where: (a,b) Pearson’s regression between mantle length (ML, mm) and δ13C and δ15N values from mantle tissue, respectively. Gray region indicates the 95% confidence interval limits, (c) newly hatched paralarva of 2 mm ML, (d) juvenile ranging 20–40 mm ML, and (e) adult ranging 50–70 mm ML. Photo credits: Sergio Carrasco.
Trophic Position of D. gahi Within the Pelagic Community
Nocturnal pelagic species evidenced a wide variation of δ13C (7.3‰) and δ15N values (6.4‰), with the most 13C-depleted values (mean ± SD) reported in the blenniid Hypsoblennius sordidus (−21.7 ± 0.7‰) and the common krill Euphausia mucronata (−20.3 ± 0.7‰), whereas the most 13C-enriched values were detected in the polychaete Pseudonereis gallapagensis (−15.5 ± 3.9‰) and the amphipod Heterophoxus oculatus (−14.5 ± 0.3‰) (Figure 6 and Table 5). The δ15N values were 15N-depleted in E. mucronata (12.2 ± 1.3‰) and brachyuran zoeae (12.5‰), and 15N-enriched in P. gallapagensis (16.5 ± 1.0‰) and H. oculatus (18.6 ± 0.4‰) (Figure 6 and Table 5).
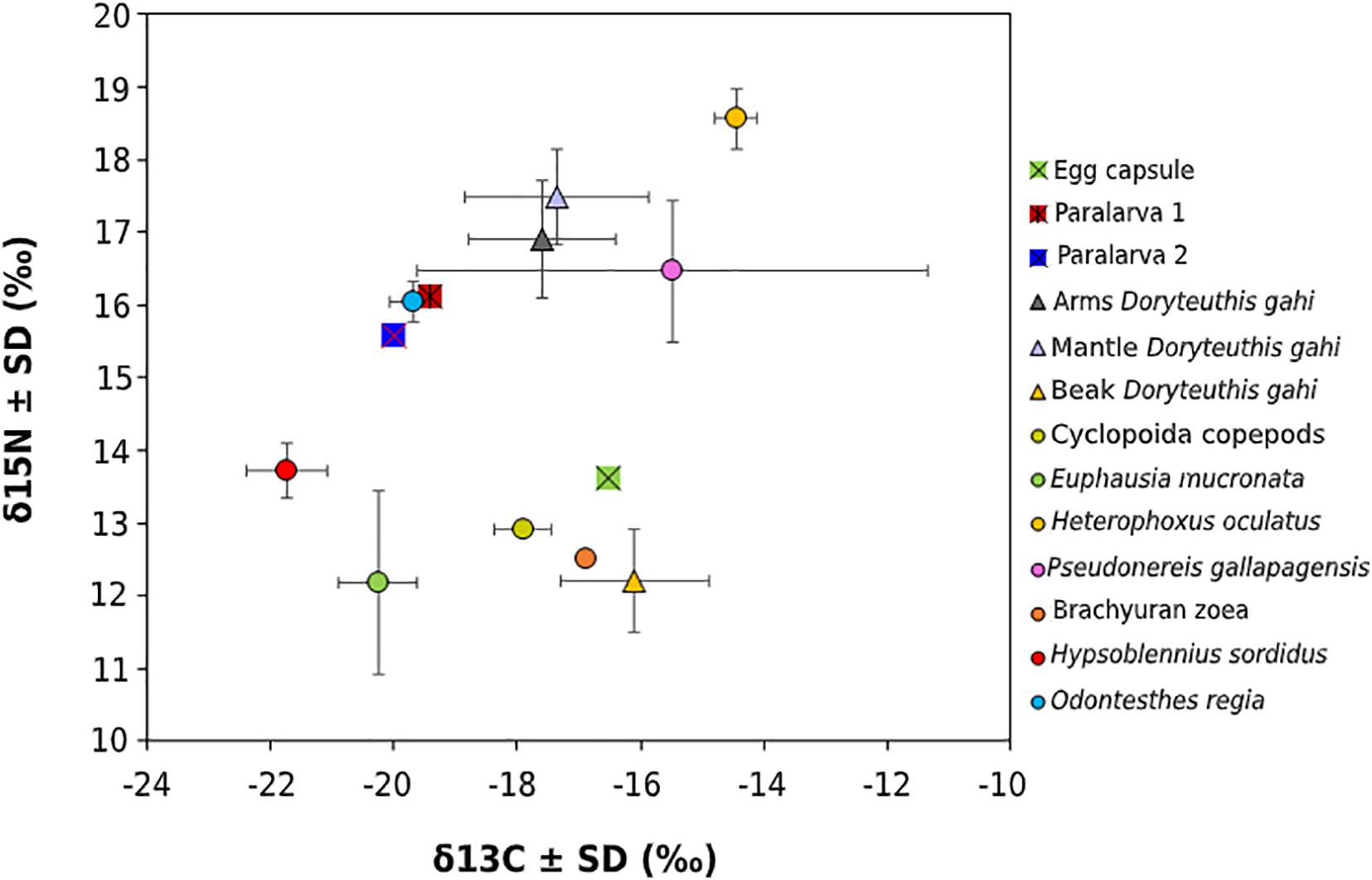
Figure 6. Biplot of δ13C and δ15N stable isotope composition of D. gahi (empty egg capsules, paralarvae, beak, and soft tissues) within a fraction of the nocturnal pelagic assemblage at La Herradura Bay, Coquimbo.
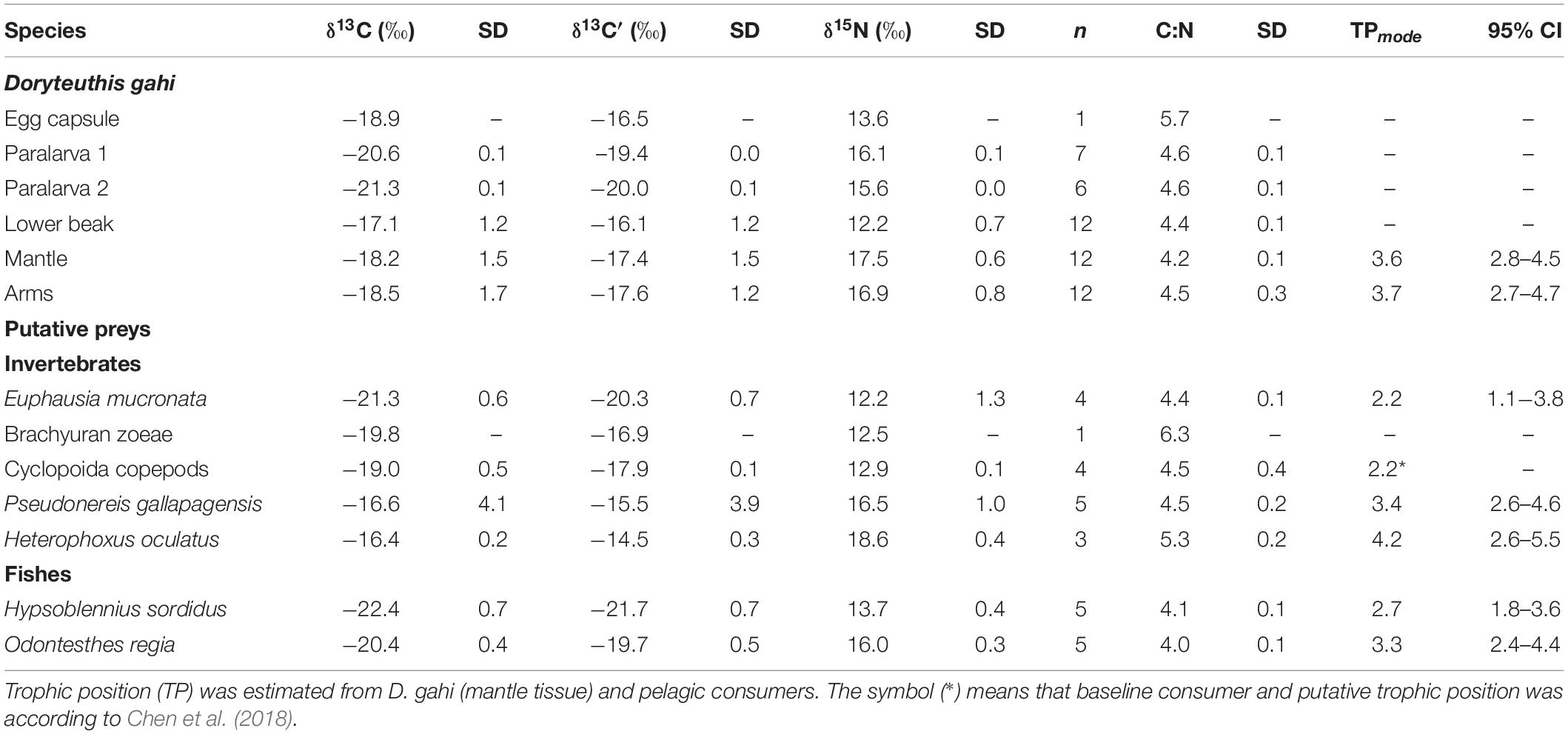
Table 5. Summary of δ13C, δ13C’ (lipid-corrected values), δ15N isotopes composition, and C:N ratio for the squid Doryteuthis gahi [i.e., egg capsules, paralarvae, soft tissues (mantle and arms), and lower beaks] and nocturnal pelagic species.
Estimations of trophic position indicated that primary consumers included E. mucronata (TPmode = 2.2; 95% credible interval [CI] = 1.1-3.8), and H. sordidus (TPmode = 2.7; 95% CI = 1.8-3.6; Table 5), followed by secondary consumers that included O. regia juveniles (TPmode = 3.3; 95% CI = 2.4-4.4), P. gallapagensis (TPmode = 3.4; 95% CI = 2.6-4.6) and D. gahi (mantle: TPmode = 3.6; 95% CI = 2.8-4.5, arms: TPmode = 3.7; 95% CI = 2.7-4.7; Table 5), and tertiary consumers such as H. oculatus (TPmode = 4.2; 95% CI = 2.6-5.5; Table 5).
Bayesian Mixing Models Outputs
The Bayesian mixing models indicated that among the putative preys included in this study, Cyclopoida copepods could be the most important prey item assimilated in the diet of D. gahi, with a median estimate of 37.9% in the mantle (95% CI = 5.5–62.4; Figure 7a; Table 6), and 42.5% in the arm tissue (95% CI = 5.8-73.3%; Figure 7b; Table 6). The euphausiid E. mucronata was also relatively important in the mantle (median = 16.8%; 95% CI = 1.0–46.3) and arm tissue (median = 22.2; 95% CI = 1.7–53). For both tissues (mantle and arms), the contribution of pelagic fishes was in general low, with O. regia showing a 7.9% and 5.4%, respectively (Table 6), followed by H. sordidus with 6.7 and 5.0%, respectively (Table 6).
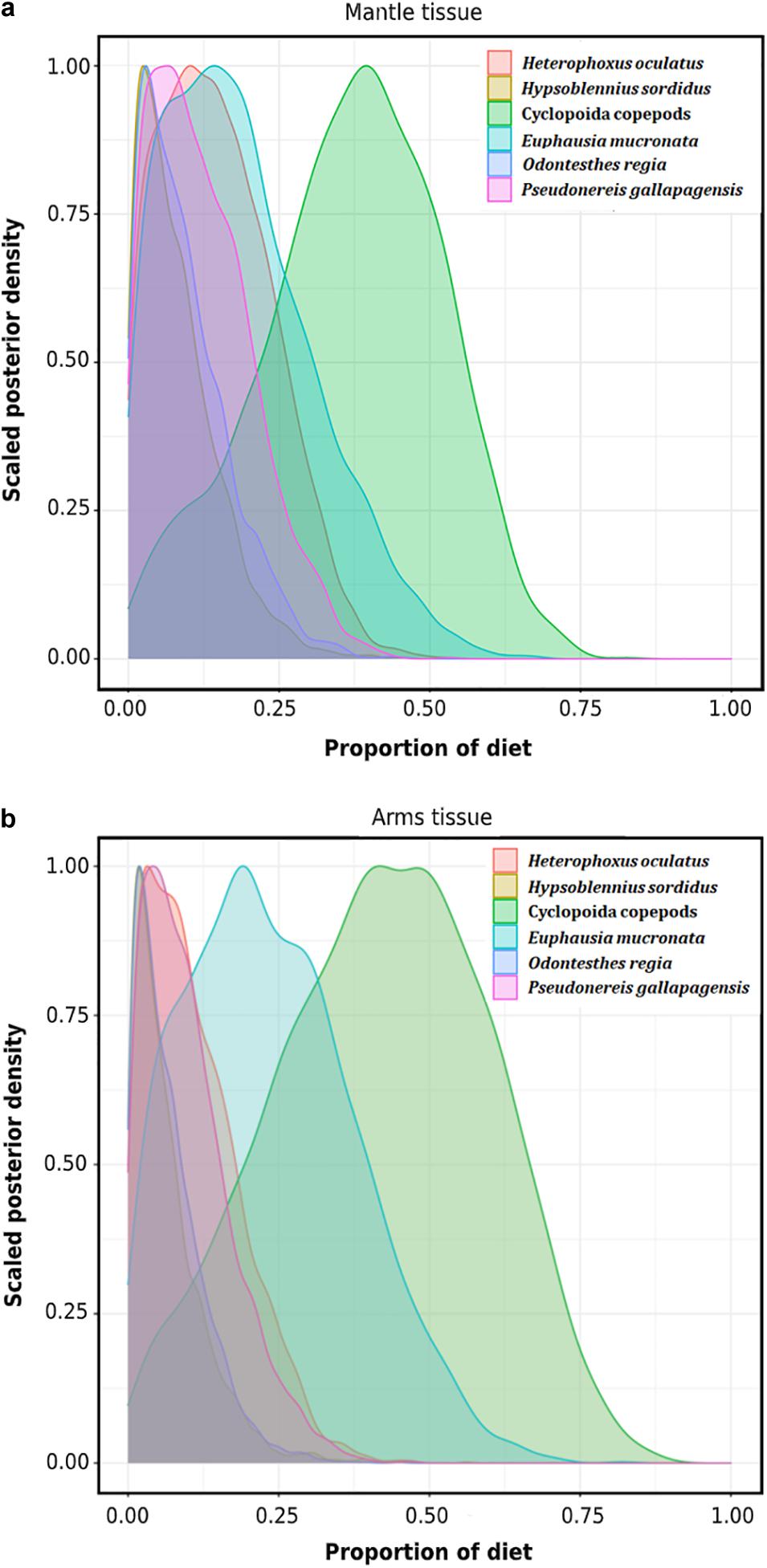
Figure 7. Density plots of potential contribution of six putative preys (n ≥ 3; invertebrates and fishes) to the assimilated diet of D. gahi inferred from (a) mantle and (b) arm tissues.
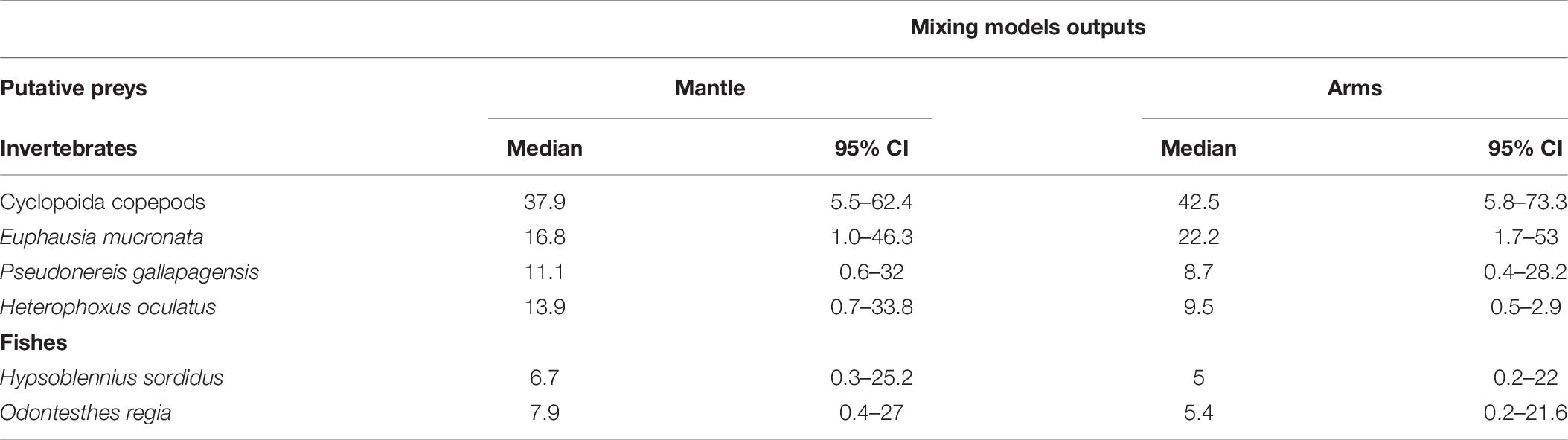
Table 6. Statistical summary of concentration dependence Bayesian mixing models (median and 95% credibility intervals) for contribution of five putative preys to the assimilated diet of Doryteuthis gahi inferred from soft tissues (mantle and arms).
Discussion
Latitudinal Traits on Spawning Habitats
Observations carried out during the preset surveys allowed identifying the use of different areas along the Chilean coast by females D. gahi to spawn their egg-capsules, suggesting that plasticity in the substrate selected for egg-capsule deposition may play an important role allowing the occurrence of these discrete spawning areas in sites with contrasting structural properties (e.g., erected kelp forests or cold-water corals, branched bottom algae, anthropogenically disturbed sites). Specific traits of egg-capsules associated to different spawning habitats will still require larger datasets at different scales (and specially in northern and southern Chile) to fully understand latitudinal and/or selection patterns influencing females’ reproductive outputs.
Nonetheless, latitudinal patterns of egg-laying were similar to those previously described in central Chile (Carrasco and Pérez-Matus, 2016), corresponding to small egg-capsules (10–50 mm length) deposited at depths between 6 and 30 m. Larger capsules (up to 90 mm length) were evidenced only in one occasion during the 4-year observation period (March 2020; see Table 2), being considered uncommon. Unlike this pattern, records from the northernmost geographic distribution of the species (Peru) have evidenced the presence of at least 2-fold larger egg capsules attached on coarse sand (up to 169 and 220 mm length; Cardoso et al., 2005 and Argüelles et al., 2008a, respectively), suggesting that larger females (i.e., 100–400 mm ML; Villegas, 2001) could be responsible for spawning these type of egg-capsules. Similarly, at the southernmost distribution sites, larger egg-capsules have also been consistently recorded in the Magellanic channels and Fjords, Chile (up to 60 and 90 mm length; Rosenfeld et al., 2014 and present study, respectively), the Argentinean Patagonia (based on the number of embryos per capsule; average 69, and up to 154; Barón, 2001), and the Falkland/Malvinas Islands (up to 86 mm length; Arkhipkin et al., 2000). As in northern sites, larger egg capsules could therefore be attributable to larger (and so high fecundity) mature females (80–300 mm ML) (Laptikhovsky et al., 2002).
Although information on changes in life history traits owing latitudinal (and so temperature) differences have been described for several species through the HCS (e.g., Fernández et al., 2007; Rivadeneira et al., 2010; Haye et al., 2019), large-scale variation in life-history traits of coastal cephalopods along the SE Pacific coast has not been previously evaluated. In this context, D. gahi has been described as the only loliginid squid adapted to reproduce in cold-water environments (ca. 6–9°C; see Arkhipkin et al., 2000; Arkhipkin and Middleton, 2003); however, current observations suggest that higher temperatures experienced in the northernmost distribution limit (i.e., Northern Peru; 17–24°C)2 might not be generating marked differences in some life-history traits (e.g., adult size, egg-capsule size, number of encapsulated embryos) when compared with specimens from the Falklands/Malvinas islands, which correspond to a different genetic population unit (McKeown et al., 2019).
Evaluating inshore spawning aggregations from a latitudinal perspective has filled important gaps in the life-history of D. gahi along SE Pacific waters, agreeing with our assumptions and suggesting that groups inhabiting the northern and southern distribution ends are characterized by larger adult sizes that are able to lay larger egg capsules with more encapsulated embryos, fitting the “abundant edge distribution” pattern described for several marine invertebrate species (Sagarin and Gaines, 2002). These findings are also consistent with the higher abundances and larger sizes necessaries to maintain the important fishery developed in both geographic regions since early 80’s (Arkhipkin et al., 2015b). Potential mechanisms driving the observed differences in life-history traits of D. gahi between both extremes and the center of the distribution (e.g., variable plastic responses, inadequate levels of successful reproduction, offspring survival; see Sagarin et al., 2006; Gaston, 2009), as well as the persistence of these traits along the HCS, is a work in progress.
Characterization of a Spawning Ground
The permanent evaluation of a single spawning site evidenced similar patterns as in latitudinal observations described above, with the typical small capsules (developmental stages 16-17; Guerra et al., 2001) being selectively attached to a variety of natural and anthropogenic substrates (i.e., rope mooring, red algae, bryozoans) devoid of structures that could produce mechanical disturbance to the gelatinous egg masses (see Carrasco and Pérez-Matus, 2016). Similar to what has been described for D. gahi in some areas around the Falkland/Malvinas islands (Arkhipkin et al., 2000), the occurrence of egg masses in the present study site was patchy and variable through the sampling period, with several negative dives (not reported in this manuscript) evidencing the sporadic nature of these findings. An increase of underwater sampling hours would probably help improving the reduced data set.
The presence of two egg masses containing more capsules than usual (43 and 45) is not rare in field collections, as up to 57 capsules per clump have been previously recorded (Carrasco and Pérez-Matus, 2016). However, the large capsule sizes observed in those egg masses (up to 69 and 96 mm length, respectively) is considered uncommon for these latitudes. Although there are no other field observations to compare these findings, two possible scenarios could be expected: first, one or multiple large-sized females (>70 mm ML) would be responsible for egg capsule deposition, and second, the small reproductive females commonly observed in the wild (∼50 mm ML) would be able to invest, at least under some circumstances, a higher reproductive effort in generating these larger capsules deposited communally. Despite evident differences in capsule size, eggs and paralarvae morphometrics seems not to differ from previous collections. In fact, after embryonic development occurred in the laboratory (from 10 to 28 days), newly hatched paralarvae evidenced similar sizes (1.8–2.9 mm ML) compared with those recorded in Peru (1.9–2.8 mm ML; Cardoso et al., 2005), central Chile (2.3–2.9 mm ML; Carrasco and Pérez-Matus, 2016), and Magellanic channels (2.5 mm ML; Rosenfeld et al., 2014), but smaller than those from the Falkland/Malvinas islands (3.1–3.4 mm ML; Arkhipkin et al., 2000).
Although the variable nature of field collections may limit the ecological interpretation of these local spawning events (29°S), it is interesting to point out that larger capsules were recorded in warmer conditions experienced during 2020 (SST: ∼18°C), contrasting with the generally lower temperatures during previous sampling years (2016, 2018, and 2019; SST: ∼15.5°C). Considering that larger egg capsules tend to have more encapsulated embryos (Arkhipkin et al., 2000; Carrasco and Pérez-Matus, 2016), and eggs seems to be larger in colder conditions (Arkhipkin et al., 2000; Laptikhovsky, 2006; present study), it would be plausible to expect that the relationship capsule size/embryo size is operating similarly in different sections of the distribution range of the species regardless obvious differences in temperature between both locations (i.e., Falkland/Malvinas islands and northern-central Chile, respectively). In this context, and as described for several cephalopod species (Boyle and Rodhouse, 2005; Pierce et al., 2008; Pimentel et al., 2012; Rosa et al., 2012) including D. gahi (Cinti et al., 2004; Cardoso et al., 2005), the temperature experienced during these early developmental stages would directly influence different paralarval traits, including hatchling size and performance. In fact, previous laboratory manipulation of thermal conditions during the early development of D. gahi (i.e., 9, 12, and 16°C) agree with those observations, evidencing that embryos incubated at lower temperatures had longer developmental times, larger hatching sizes, and longer survival times than siblings incubated at higher temperatures (Carrasco et al., 2016). Therefore, it would be possible to hypothesize that paralarvae D. gahi should be present in the coastal habitats of northern-central Chile through the year, experiencing and surviving different temperature conditions ranging from 9 to 17°C, corresponding to common mean daily SST recorded at these latitudes (see Wieters, 2005; Aravena et al., 2014). However, other than the present results, there is no field information to validate this assumption, evidencing that the entire life-cycle of the species (from their encapsulated embryos to adult reproductive stages) has been completely overlooked as a component of coastal pelagic communities along the SE Pacific.
The possibility to collect and maintain juvenile and adult stages of D. gahi in controlled laboratory conditions for up to 110 days, allowed demonstrating that mature stages can be found at small sizes of around 40 mm ML in both sexes, being corroborated by the presence of well-developed spermatophores and eggs in males and females, respectively. Accordingly, laboratory spawns allowed confirming that females as small as 45 mm ML are fully mature and fertilized, depositing egg capsules that developed normally until hatching and that resemble the small-sized capsules typically described in northern-central Chile (Carrasco and Pérez-Matus, 2016). Considering that the minimum size recorded for mature females is about 90 mm ML in Concepción, southern-central Chile (Ibáñez et al., 2005) and 130 mm ML in Falkland/Malvinas islands (Arkhipkin et al., 2013), the present study describes the smallest egg-laying female D. gahi (45 mm ML) recorded through the complete distributional range of the species. Since a length-weight power function on adult specimens revealed a coefficient <3, these findings also support the dwarf morphotype hypothesis, suggesting that a small reproductive morphotype is consistently occurring along the HCS between the two genetically different centers of abundance, the Chilean – Falklands/Malvinas and the Peruvian groups (Ibáñez and Poulin, 2014; McKeown et al., 2019). Similar morphological changes owing environmental variability have also been described in males D. gahi from the Patagonian shelf population (Jones et al., 2019) and also in the jumbo squid D. gigas from Peruvian waters (Argüelles et al., 2008b; Keyl et al., 2011; Arkhipkin et al., 2015a), enhancing the role of cephalopod plasticity when facing variable environmental conditions. Ongoing work on this Humboldt morphotype of D. gahi will complement population genetic studies as well as behavioral components associated to reproductive and trophic interactions in captive conditions.
Trophic Ecology of D. gahi in Northern-Central Chile
There was no evidence of a marked relationship between ML and δ13C and δ15N values, suggesting that carbon sources and trophic position, respectively, may not change significantly through the size range analyzed (2–70 mm ML). In fact, δ15N values from hatched paralarvae were slightly 15N-depleted than arms and mantle tissue from larger squids, reflecting a relatively constant diet through the ontogenetic stages evaluated. Similar to these findings, recent research exploring trophic allometries in cephalopods have also suggested that there are some species or Families (i.e., Cranchiidae) that do not exhibit ontogenetic trophic position shifts across different size classes, differing from the general description of cephalopod as voracious predators. These low activity level cephalopods were characterized by having low trophic positions, feeding largely upon herbivorous zooplankton and do not shifting to higher levels of piscivory until larger sizes (see Murphy et al., 2020).
Accordingly, previous studies have suggested similar changes in the diet of D. gahi, shifting from crustaceans in small-sized individuals (5.1-15 cm ML) to fish in larger sizes (15.1-25 cm ML) (Rosas-Luis et al., 2014). Changes in δ13C and δ15N values through the gladius sections have also supported dietary observations, suggesting important trophic changes through ontogeny (Rosas-Luis et al., 2017). Therefore, our findings are consistent with both observations, demonstrating a common source of food and trophic position in small and large-sized squids (via stable isotope analyses), and directly corroborating in laboratory that larger specimens D. gahi are able to prey upon different fish species, as well as cannibalize other squids, if predator and prey sizes are appropriate (i.e., ∼50% of squid’s ML; SA. Carrasco, unpublished data).
The trophic position (TP) estimated here for D. gahi (TP = 3.6 to 3.7) corroborate the trophic role of the species as a zooplanktivore (secondary consumer) in pelagic food webs. When contrasting TP values with other pelagic species occurring in the Humboldt Current system (all through C and N stable isotopes), these values were comparable to other pelagic species such as the mackerel Trachurus murphyi (TP = 3.8; Pizarro et al., 2019), the anchovy Engraulis ringens (TP = 3.7), and the jumbo squid Dosidicus gigas (TP = 3.9) (Espinoza et al., 2017). These estimations were comparable among studies given the use of the same trophic discrimination factor (TDF: ± 3.4‰; Post, 2002), except the trophic position of copepods (2.2) that was slightly lower than the value 2.5 used by Espinoza et al. (2017). In the other hand, TP estimated for D. gahi through different sections of the gladius evidenced higher values for juveniles (TP = 4.2) and adult stages (TP = 4.3) (Rosas-Luis et al., 2017); however, these values matched the error measurements from the TP models of mantle and arms.
Despite stomach content in the six specimens analyzed here only evidenced a liquefied content where no further taxonomic identifications of the dietary items were possible, the importance of planktonic crustaceans in the diet of D. gahi has been confirmed through stomach content in other areas of the distribution range, including Peru (Cardoso et al., 1998), Falkland/Malvinas Islands (Guerra et al., 1991), and South West Atlantic (Arkhipkin et al., 2013; Rosas-Luis et al., 2014). Bayesian mixing models were consistent with those observations and agreed with preliminary expectations, suggesting that copepods and euphausiids would be the most important putative preys, followed in a minor proportion by amphipods and polychaetes. In this context, the Coquimbo Bay system is located within an important upwelling center (Moraga et al., 2001; Thiel et al., 2007), with copepods dominating the zooplankton community structure, while euphausiids are frequently observed in lower abundances (Mattos and Mujica, 2012; Torreblanca et al., 2016) as response to extensive diel vertical migration into the oxygen minimum zone (Thiel et al., 2007). The presence of amphipods and swimming nereid polychaetes in nocturnal pelagic assemblages is not rare, and has been associated to the incidence of artificial lights in shallow depths (<30 m) in the former (Carrasco et al., 2017), or to the presence of reproductive stages (i.e., epitokes) attracted by lunar phases in the latter (Fong, 1993). Consistently with the present records, the presence of the polychaete P. gallapagensis has been reported in stomach content of individuals D. gahi from Peru (Cardoso et al., 1998) as well as in other members of the Family Loliginidae (Rocha et al., 1994), whereas amphipods have been recorded in the Atlantic Ocean (Arkhipkin et al., 2013). Therefore, the potential contribution of polychaetes and amphipods in the diet of D. gahi may provide temporal trophic subsidize to juvenile and adult squids in these coastal waters via emergent macrobenthic fauna (see Carrasco et al., 2017).
In the other hand, the trophic role of cephalopods as preys has been better understood given the presence of chitinous beaks within the stomach contents of upper consumers, including fishes, birds, mammals and other squids (reviewed in Arkhipkin et al., 2013). Differences in stable isotope composition between beaks and soft tissues (mantle and arms) observed in the present study show the same pattern reported in other cephalopods, with beaks tending to be slightly 13C-enriched and significantly 15N-depleted compared with soft tissues (Cherel and Hobson, 2005; Hobson and Cherel, 2006). The lower δ15N values in beaks compared to soft tissues could be explained by the presence of 15N-depleted chitin molecules (Cherel et al., 2009a). Therefore, differences in the present values for D. gahi could be useful for obtaining corrections factors for beaks found in different predators’ stomach contents, improving our understanding on the trophic role of the species along the HCS.
Overall, the present study unveils the permanent occurrence of a dwarf reproductive morphotype of D. gahi in shallow coastal habitats along the Humboldt Current System, providing the first insights for understanding potential adaptations to heterogeneous conditions in different sections of the distribution range (e.g., temperature, food availability), but also through the life cycle of the species (e.g., egg-capsules, paralarvae, adults). The combination of direct underwater surveys, isotopic composition, and behavioral components of alive organisms in laboratory, provided a strong approach for teasing out some of the ecological mechanisms underlying spatial connectivity patterns and the species’ role in a crucial (but unexplored) section of its geographic range, which connect the two genetically distinct centers of abundance, the Chilean – Falklands/Malvinas and the Peruvian. The incorporation of pelagic/benthic nutrients from small invertebrates (e.g., copepods, amphipods, euphausiids, and nereid polychaetes) and its transmission to upper trophic levels (fish, mammals, seabirds, and humans), enhance the role of D. gahi as an important nutritional link in these coastal environments and potentially through the complete distributional range.
Data Availability Statement
The raw data supporting the conclusions of this article are available from the corresponding author (SC), upon reasonable request.
Ethics Statement
The use of animals during this study was reviewed and approved by the Comité Ético Científico de la Facultad de Medicina de la Universidad Católica del Norte, Coquimbo, Chile (CECFAMED – UCN N° 18/2020).
Author Contributions
SC conceived the idea, designed the study, and led the writing of the manuscript. SC and GZ-H performed samplings. GZ-H, SC, and CI analyzed the data. MB reviewed samples, generated databases, and assisted in specimens’ maintenance in laboratory. All authors have read and commented on the manuscript.
Funding
Funding for this research has been provided by a FONDECYT research grant (#11170617) awarded to SC.
Conflict of Interest
The authors declare that the research was conducted in the absence of any commercial or financial relationships that could be construed as a potential conflict of interest.
Acknowledgments
We would like to thank people who provided important assistance during the study, especially to colleague divers for their photographic records or samples from in situ observations, including: Carlos Oroza (Estudios del mar y su gente), Ignacio Borgeaud (BajoMar EcoDive), Alejandro Pérez-Matus (PUC), Alejandro Soza (ODC Dive Center), Iván Hinojosa (UCSC), and César Cárdenas (INACH). The assistance of Helmo Pérez (HP Mar) during field surveys at La Herradura Bay is greatly appreciated. The comments of two reviewers greatly improved a preliminary version of the manuscript.
Footnotes
References
Aravena, G., Broitman, B., and Stenseth, N. C. (2014). Twelve years of change in coastal upwelling along the Central-Northern coast of Chile: spatially heterogeneous responses to climatic variability. PLoS One 9:e90276. doi: 10.1371/journal.pone.0090276
Argüelles, J., Taipe, A., and Aguilar, S. (2008a). “Number of eggs per capsule of Loligo gahi (Cephalopoda: Myopsida) off Callao – Peru, during 2007–2008,” in Proceeding of the 4th International Symposium of Pacific Squid and 3rd International Workshop on Squid, (Coquimbo: Universidad Católica del Norte), 32.
Argüelles, J., Tafur, R., Taipe, A., Villegas, P., Keyl, F., Dominguez, N., et al. (2008b). Size increment of jumbo flying squid Dosidicus gigas mature females in Peruvian waters, 1989–2004. Progr. Oceanogr. 79, 308–312. doi: 10.1016/j.pocean.2008.10.003
Arkhipkin, A. I., Laptikhovsky, V. V., and Middleton, D. A. J. (2000). Adaptations for cold water spawning in loliginid squid: Loligo gahi in Falkland waters. J. Molluscan Stud. 66, 551–564. doi: 10.1093/mollus/66.4.551
Arkhipkin, A. I., and Middleton, D. A. J. (2003). In-situ monitoring of the duration of embryonic development in the squid Loligo gahi (Cephalopoda: Loliginidae) on the Falkland shelf. J. Molluscan Stud. 69, 123–133. doi: 10.1093/mollus/69.2.123
Arkhipkin, A. I., Hatfield, E. M., and Rodhouse, P. G. K. (2013). “Doryteuthis gahi, Patagonian long-finned squid,” in Advances in Squid Biology, Ecology and Fisheries. Part I – Myopsid Squids, eds R. Rosa, R. O’Dor, and G. J. Pierce (Hauppauge, NY: Nova Science Publishers), 123–158.
Arkhipkin, A., Argüelles, J., Shcherbich, Z., and Yamashiro, C. (2015a). Ambient temperature influences adult size and life span in jumbo squid (Dosidicus gigas). Can. J. Fish. Aquat. Sci. 72, 400–409. doi: 10.1139/cjfas-2014-0386
Arkhipkin, A. I., Rodhouse, P. G. K., Pierce, G. J., Sauer, W., Sakai, M., Allcock, L. A., et al. (2015b). World squid fisheries. Rev. Fish. Sci. Aquac. 23, 92–252.
Barón, P. J. (2001). First description and survey of the egg masses of Loligo gahi and Loligo sanpaulensis from the Patagonian coastal waters. J. Shellfish Res. 20, 289–295.
Boyle, P., and Rodhouse, P. G. K. (2005). Cephalopods. Ecology and fisheries. 1st edition. Oxford: Blackwell Publishing, doi: 10.1002/9780470995310
Byrne, M. (2011). “Impact of ocean warming and ocean acidification on marine invertebrate life history stages: vulnerabilities and potential for persistence in a changing ocean,” in Oceanography and Marine Biology: An Annual Review, 49, ed. J. D. M. Gordon (Boca Raton, FL: CRC Press), 1–42.
Cardoso, F., Tarazona, J., and Paredes, C. (1998). Aspectos biológicos del calamar patagónico Loligo gahi (Cephalopoda: Loliginidae) en Huarmey. Perú. Rev. peru. biol. 5, 9–14.
Cardoso, F., Baltazar, P., and Bautista, J. (2005). The early development of the Patagonian squid Loligo gahi D’Orbigny, 1835 in Peruvian waters (Cephalopoda: Loliginidae). Rev. peru. biol. 12, 369–376.
Carrasco, S. A., and Pérez-Matus, A. (2016). Inshore spawning grounds of the squid Doryteuthis gahi suggest the consistent use of defoliated kelp Lessonia trabeculata in central Chilean waters. Mar. Biol. Res. 12, 323–328. doi: 10.1080/17451000.2015.1136064
Carrasco, S. A., Blanco, M., Ospina-Álvarez, A., Pérez-Matus, A., Muñóz, R., Dijkstra, M., et al. (2016). “Temperature-mediated development and post-hatching performance in three coastal species with contrasting life-history patterns,” in Proceeding of the 11th International Temperate Reef Symposium, (Italy: Pisa), 48.
Carrasco, S. A., Vandecasteele, L., Rivadeneira, M. M., Fernández, M., and Pérez-Matus, A. (2017). Spatial and short-term variability of larval, post-larval and macrobenthic assemblages associated with subtidal kelp forest ecosystems in Central Chile. Mar. Biol. Res. 13, 1041–1058. doi: 10.1080/17451000.2017.1322704
Chen, M., Dongyoung, K., Liu, H., and Kang, C.-H. (2018). Variability in copepod trophic levels and feeding selectivity based on stable isotope analysis in Gwangyang Bay of the southern coast of the Korean Peninsula. Biogeosciences 15, 2055–2073. doi: 10.5194/bg-15-2055-2018
Cherel, Y., and Hobson, K. A. (2005). Stable isotopes, beaks and predators: a new tool to study the trophic ecology of cephalopods, including giant and colossal squids. Proc. R. Soc. B. 272, 1601–1607. doi: 10.1098/rspb.2005.3115
Cherel, Y., Fontaine, C., Jackson, G. D., Jackson, C. H., and Richard, P. (2009a). Tissue, ontogenic and sex-related differences in δ13C and δ15N values of the oceanic squid Todarodes filippovae (Cephalopoda: Ommastrephidae). Mar. Biol. 156, 699–708. doi: 10.1007/s00227-008-1121-x
Cherel, Y., Ridoux, V., Spitz, J., and Richard, P. (2009b). Stable isotopes document the trophic structure of a deep-sea cephalopod assemblage including giant octopod and giant squid. Biol. Lett. 5, 364–367. doi: 10.1098/rsbl.2009.0024
Cherel, Y., Bustamante, P., and Richard, P. (2019). Amino acid d13C and d15N from sclerotized beaks: a new tool to investigate the foraging ecology of cephalopods, including giant and colossal squids. Mar. Ecol. Prog. Ser. 624, 89–102. doi: 10.3354/meps13002
Chong, J., Sepúlveda, K., and Ibáñez, C. M. (2006). Variación temporal en la dieta del congrio colorado, Genypterus chilensis (Guichenot, 1881) frente al litoral de Talcahuano, Chile (36°32′S - 36°45′S). Rev. Biol. Mar. Oceanogr. 41, 195–202.
Cinti, A., Barón, P. J., and Rivas, A. L. (2004). The effects of environmental factors on the embryonic survival of the Patagonian squid Loligo gahi. J. Exp. Mar. Bio. Ecol. 313, 225–240. doi: 10.1016/j.jembe.2004.05.017
Cubillos, L. A., Rebolledo, H. P., and Hernández, A. F. (2003). Prey composition and estimation of Q/B for the Chilean hake, Merluccius gayi (Gadiformes–Merluccidae), in the central-south area off Chile (34° – 40° S). Arch. Fish. Mar. Res 50, 271–286.
DeNiro, M., and Epstein, S. (1977). Mechanism of carbon isotope fractionation associated with lipid synthesis. Science 197, 261–263. doi: 10.1126/science.327543
Doubleday, Z. A., Prowse, T. A. A., Arkhipkin, A., Pierce, G. J., Semmens, J., Steer, M., et al. (2016). Global proliferation of cephalopods. Curr. Biol. 26, 406–407. doi: 10.1016/j.cub.2016.04.002
Espinoza, P., Lorrain, A., Ménard, F., Cherel, Y., Tremblay-Boyer, L., Argüelles, J., et al. (2017). Trophic structure in the northern Humboldt Current system: new perspectives from stable isotope analysis. Mar. Biol. 164, 86. doi: 10.1007/s00227-017-3119-8
Fernández, M., Calderón, R., Cancino, J. M., and Jeno, K. (2007). The effect of temperature on the development of encapsulated embryos of Concholepas concholepas along a latitudinal cline. Mar. Ecol. Prog. Ser. 348, 229–237. doi: 10.3354/meps07032
Fong, P. P. (1993). Lunar control of epitokal swarming in the polychaete Platynereis bicanaliculata (Baird) from central California. Bull. Mar. Sci. 52, 911–924.
Gaston, K. J. (2009). Geographic range limits: achieving synthesis. Proc. R. Soc. B. 276, 1395–1406. doi: 10.1098/rspb.2008.1480
Golikov, A. V., Ceia, F. R., Sabirov, R. M., Ablett, J. D., Gleadall, I. G., Gudmundsson, G., et al. (2019). The first global deep-sea stable isotope assessment reveals the unique trophic ecology of vampire squid Vampyroteuthis infernalis (Cephalopoda). Sci. Rep. 9:19099. doi: 10.1038/s41598-019-55719-1
González, P., and Chong, J. (1997). Alimentación del lenguado de ojos chicos Paralichthys microps (Günther, 1881) (Pleuronectiformes: Paralichthyidae) en Bahía de Concepción (VIII Región, Chile). Gayana Zool. 61, 7–13.
González, P., and Oyarzún, C. (2003). Diet of the Chilean sandperch, Pinguipes chilensis (Perciformes, Pinguipedidae) in southern Chile. J. Appl. Ichthyol. 19, 371–375. doi: 10.1111/j.1439-0426.2003.00444.x
Guerra, A., Castro, B. G., and Nixon, M. (1991). Preliminary study on the feeding by Loligo gahi (Cephalopoda: Loliginidae). Bull. Mar. Sci. 49, 309–311.
Guerra, A., Rocha, F. J., González, A. F., and Buckle, L. F. (2001). Embryonic stages of the patagonian squid Loligo gahi (Mollusca: Cephalopoda). Veliger 44, 109–115.
Guerreiro, M., Phillips, R. A., Cherel, Y., Ceia, F. R., Alvito, P., Rosa, R., et al. (2015). Habitat and trophic ecology of Southern Ocean cephalopods from stable isotope analyses. Mar. Ecol. Prog. Ser. 530, 119–134. doi: 10.3354/meps11266
Haye, P. A., Segovia, N. I., Varela, A. I., Rojas, R., Rivadeneira, M. M., and Thiel, M. (2019). Genetic and morphological divergence at a biogeographic break in the beach-dwelling brooder Excirolana hirsuticauda Menzies (Crustacea, Peracarida). BMC Evol. Biol. 19:118. doi: 10.1186/s12862-019-1442-z
Hobson, K., and Cherel, Y. (2006). Isotopic reconstruction of marine food webs using cephalopod beaks: new insight from captively raised Sepia officinalis. Can. J. Zool. 84, 766–770. doi: 10.1139/Z06-049
Ibáñez, C. M., Chong, J., and Pardo-Gandarillas, M. C. (2005). Relaciones somatométricas y reproductivas del calamar Loligo gahi Orbigny, 1835 en bahía Concepción, Chile. Invest. Mar. Valparaíso 33, 211–215. doi: 10.4067/S0717-71782005000200009
Ibáñez, C. M., Camus, P. A., and Rocha, F. J. (2009). Diversity and distribution of cephalopod species off the coast of Chile. Mar. Biol. Res. 5, 374–384. doi: 10.1080/17451000802534873
Ibáñez, C. M., Vega, M. A., and Rocha, F. (2010). Historia de las investigaciones en sistemática de cefalópodos en Chile. Amici Molluscarum 18, 7–11.
Ibáñez, C. M., Argüelles, J., Yamashiro, C., Adasme, L., Céspedes, R., and Poulin, E. (2012). Spatial genetic structure and demographic inference of the Patagonian squid Doryteuthis gahi in the south-eastern Pacific Ocean. J. Mar. Biolog. Assoc. UK. 92, 197–203. doi: 10.1017/S0025315411000440
Ibáñez, C. M., and Poulin, E. (2014). Genetic structure and diversity of squids with contrasting life histories in the Humboldt Current System. Hidrobiológica 24, 1–10.
Jereb, P., and Roper, C. (2010). Cephalopods of the World. An Annotated And Illustrated Catalogue of Species Known to Date. Myopsid and Oegopsid Squids, Vol. 2. Rome: FAO Species Catalogue for Fishery Purposes, 649.
Jones, J. B., Pierce, G. J., Brickle, P., Shcherbich, Z. N., and Arkhipkin, A. I. (2019). ‘Superbull’ males: what role do they play and what drives their appearance within the Doryteuthis gahi Patagonian Shelf population? Mar. Freshw. Res. 70, 1805–1817. doi: 10.1071/MF18285
Keyl, F., Argüelles, J., and Tafur, R. (2011). Interannual variability in size structure, age, and growth of jumbo squid (Dosidicus gigas) assessed by modal progression analysis. ICES J. Mar. Sci. 68, 507–518. doi: 10.1093/icesjms/fsq167
Laptikhovsky, V. (2006). Latitudinal and bathymetric trends in egg size variation: a new look at Thorson’s and Rass’s rules. Mar. Ecol. 27, 7–14. doi: 10.1111/j.1439-0485.2006.00077.x
Laptikhovsky, V. V., Arkhipkin, A. I., Middleton, D. A. J., and Butcher, L. R. (2002). Ovary maturation and fecundity of the squid Loligo gahi on the southeast shelf of the Falkland Islands. Bull. Mar. Sci. 71, 449–464.
Logan, J. M., and Lutcavage, E. M. (2013). Assessment of trophic dynamics of cephalopods and large pelagic fishes in the central North Atlantic Ocean using stable isotope analysis. Deep Sea Res. II. 95, 63–73. doi: 10.1016/j.dsr2.2012.07.013
Matias, R. S., Gregory, S., Ceia, F. R., Baeta, A., Seco, J., Rocha, M. S., et al. (2019). Show your beaks and we tell you what you eat: Different ecology in sympatric Antarctic benthic octopods under a climate change context. Mar. Environ. Res. 150:104757. doi: 10.1016/j.marenvres.2019.104757
Mattos, H., and Mujica, A. (2012). Composición de zooplancton superficial en zonas lisas y rugosas en la bahía de Coquimbo (noviembre 2001). Lat. Am. J. Aquat. Res. 40, 453–461. doi: 10.3856/vol40-issue2-fi1lltext-21
McKeown, N. J., Arkhipkin, A. I., and Shaw, P. W. (2019). Genetic analysis reveals historical and contemporary population dynamics in the longfin squid Doryteuthis gahi: implications for cephalopod management and conservation. ICES J. Mar. Sci. 76, 1019–1027. doi: 10.1093/icesjms/fsz009
Moraga, J., Valdebenito, E., and Rutllant, J. (2001). Condiciones oceanográficas durante la fase de relajación de un evento de surgencia invernal frente a Punta Lengua de Vaca. Coquimbo. Invest. Mar. 29, 59–71. doi: 10.4067/S0717-71782001000100006
Murphy, K. J., Pecl, G. T., Richards, S. A., Semmens, J. M., Revill, A. T., Suthers, I. M., et al. (2020). Functional traits explain trophic allometries of cephalopods. J. Anim. Ecol. 89, 2692–2703. doi: 10.1111/1365-2656.13333
Pauli, J. N., Newsome, S. D., Cook, J. A., Harrod, C., Steffan, S. A., Baker, C. J. O., et al. (2017). Why we need a centralized repository for isotopic data. Proc. Natl. Acad. Sci. U.S.A. 114, 2997–3001. doi: 10.1073/pnas.1701742114
Pecl, G. T., and Jackson, G. D. (2008). The potential impacts of climate change on inshore squid: biology, ecology and fisheries. Rev. Fish. Biol. Fish. 18, 373–385. doi: 10.1007/s11160-007-9077-3
Pérez-Matus, A., Ospina-Álvarez, A., Camus, P. A., Carrasco, S. A., Fernandez, M., Gelcich, S., et al. (2017). Temperate rocky subtidal reef community reveals human impacts across the entire food web. Mar. Ecol. Prog. Ser. 567, 1–16. doi: 10.3354/meps12057
Pierce, G. J., Valavanis, V. D., Guerra, A., Jereb, P., Orsi-Relini, L., Bellido, J. M., et al. (2008). A review of cephalopod–environment interactions in European Seas. Hydrobiologia 612, 49–70. doi: 10.1007/978-1-4020-9141-4_5
Pimentel, M. S., Trübenbach, K., Faleiro, F., Boavida-Portugal, J., Repolho, T., and Rosa, R. (2012). Impact of ocean warming on the early ontogeny of cephalopods: a metabolic approach. Mar. Biol. 159, 2051–2059. doi: 10.1007/s00227-012-1991-9
Pizarro, J., Docmac, F., and Harrod, C. (2019). Clarifying a trophic black box: stable isotope analysis reveals unexpected dietary variation in the Peruvian anchovy Engraulis ringens. PeerJ 7:e6968. doi: 10.7717/peerj.6968
Post, D. (2002). Using stable isotopes to estimate trophic position: models, methods, and assumptions. Ecology 83, 703–718. doi: 10.2307/3071875
Post, D. M., Layman, C. A., Arrington, D. A., Takimoto, G., Quattrochi, J., and Montaña, C. (2007). Getting to the fat of the matter: models, methods and assumptions for dealing with lipids in stable isotope analyses. Oecologia 152, 179–189. doi: 10.1007/s00442-006-0630-x
Quezada-Romegialli, C., Jackson, A. L., Hayden, B., Kahilainen, K. K., Lopes, C., and Harrod, C. (2018). TrophicPosition, an R package for the Bayesian estimation of trophic position from consumer stable isotope ratios. Methods Ecol. Evol. 9, 1592–1599. doi: 10.1111/2041-210X.13009
Rivadeneira, M. M., Hernández, P., Baeza, J. A., Boltaña, S., Cifuentes, M., Correa, C., et al. (2010). Testing the abundant-centre hypothesis using intertidal porcelain crabs along the Chilean coast: linking abundance and life-history variation. J. Biogeogr. 37, 486–498. doi: 10.1111/j.1365-2699.2009.02224.x
Rocha, F., and Vega, M. A. (2003). Overview of cephalopod fisheries in Chilean waters. Fish. Res. 60, 151–159. doi: 10.1016/S0165-7836(02)00080-2
Rocha, F., Castro, B. G., Gil, M. S., and Guerra, A. (1994). The diets of Loligo vulgaris and L. forbesi (Cephalopoda: Loliginidae) in Northwestern Spanish Atlantic waters. Sarsia 73, 119–126. doi: 10.1080/00364827.1994.10413552
Rosas-Luis, R., Sánchez, P., Portela, J. M., and del Rio, J. L. (2014). Feeding habits and trophic interactions of Doryteuthis gahi, Illex argentinus and Onykia ingens in the marine ecosystem off the Patagonian shelf. Fish. Res. 152, 37–44. doi: 10.1016/j.fishres.2013.11.004
Rosas-Luis, R., Navarro, J., Martínez-Baena, F., and Sánchez, P. (2017). Differences in the trophic niche along the gladius of the squids Illex argentinus and Doryteuthis gahi based on their isotopic values. Reg. Stud. Mar. Sci. 11, 17–22. doi: 10.1016/j.rsma.2017.02.003
Rosa, R., Pimentel, M. S., Boavida-Portugal, J., Teixeira, T., Trübenbach, K., and Diniz, M. (2012). Ocean warming enhances malformations, premature hatching, metabolic suppression and oxidative stress in the early life stages of a keystone squid. PLoS One 7:e38282. doi: 10.1371/journal.pone.0038282
Rosenfeld, S., Ojeda, J., Hüne, M., and Mansilla, A. (2014). Egg masses of the Patagonian squid Doryteuthis (Amerigo) gahi attached to giant kelp (Macrocystis pyrifera) in the sub-Antarctic ecoregion. Polar Res. 33:21636. doi: 10.3402/polar.v33.21636
Ruiz-Cooley, R. I., Markaida, U., Gendron, D., and Aguiñiga, S. (2006). Stable isotopes in jumbo squid (Dosidicus gigas) beaks to estimate its trophic position: comparison between stomach contents and stable isotopes. J. Mar. Biol. Ass. UK. 86, 437–445. doi: 10.1017/S0025315406013324
Sagarin, R. D., and Gaines, S. D. (2002). Abundance distributions of coastal invertebrates: using one-dimensional ranges to test biogeographic hypotheses. J. Biogeogr. 29, 985–997. doi: 10.1046/j.1365-2699.2002.00705.x
Sagarin, R. D., Gaines, S. D., and Gaylord, B. (2006). Moving beyond assumptions to understand abundance distributions across the ranges of species. Trends Ecol. Evol. 21, 524–530. doi: 10.1016/j.tree.2006.06.008
Sielfeld, W., Barraza, J., and Amado, N. (2018). Patrones locales de alimentación del león marino sudamericano Otaria byronia: el caso de Punta Patache, Norte de Chile. Rev. Biol. Mar. Oceanogr. 53, 307–319. doi: 10.22370/rbmo.2018.53.3.1356
Stock, B. C., and Semmens, B. X. (2016). Unifying error structures in commonly used biotracer mixing models. Ecology 97, 2562–2569. doi: 10.1002/ecy.1517
Stock, B. C., Jackson, A. L., Ward, E. J., Parnell, A. C., Phillips, D. L., and Semmens, B. X. (2018). Analyzing mixing systems using a new generation of Bayesian tracer mixing models. PeerJ 6:e5096. doi: 10.7717/peerj.5096
Thiel, M., Macaya, E., Acuña, E., Arntz, W. E., Bastias, H., Brokordt, K., et al. (2007). The Humboldt Current System of Northern-Central Chile: oceanographic processes, ecological interactions. Oceanogr. Mar. Biol. Ann. Rev. 45, 195–344. doi: 10.1201/9781420050943.ch6
Torreblanca, M. L., Pérez-Santos, I., San Martin, B., Varas, E., Zilleruelo, R., Riquelme-Bugueño, R., et al. (2016). Seasonal dynamics of zooplankton in a northern Chile bay exposed to upwelling conditions. Rev. Biol. Mar. Oceanogr. 51, 273–291. doi: 10.4067/S0718-19572016000200006
Villegas, P. (2001). Growth, life cycle and fishery biology of Loligo gahi (d’Orbigny 1835) off the Peruvian coast. Fish. Res. 54, 123–131. doi: 10.1016/S0165-7836(01)00376-9
Wieters, E. A. (2005). Upwelling control of positive interactions over mesoscales: a new link between bottom-up and top-down processes on rocky shores. Mar. Ecol. Prog. Ser. 301, 43–54. doi: 10.3354/meps301043
Xavier, J. C., Allcock, A. L., Cherel, Y., Lipinski, M. R., Pierce, G. J., Rodhouse, P. G. K., et al. (2015). Future challenges in cephalopod research. J. Mar. Biol. Ass. UK. 95, 999–1015.
Keywords: egg capsules, egg size, paralarvae, stable isotopes, trophic ecology, Loliginidae
Citation: Carrasco SA, Bravo M, Ibáñez CM and Zapata-Hernández G (2021) Discrete Spawning Aggregations of the Loliginid Squid Doryteuthis gahi Reveal Life-History Interactions of a Dwarf Morphotype at the Center of Its Distribution Range. Front. Mar. Sci. 7:616116. doi: 10.3389/fmars.2020.616116
Received: 05 November 2020; Accepted: 28 December 2020;
Published: 18 January 2021.
Edited by:
Enric Gisbert, Institute of Agrifood Research and Technology (IRTA), SpainReviewed by:
Guiomar Rotllant, Instituto de Ciencias del Mar (CSIC), SpainDharmamony Vijai, National Centre for Biological Sciences, India
Copyright © 2021 Carrasco, Bravo, Ibáñez and Zapata-Hernández. This is an open-access article distributed under the terms of the Creative Commons Attribution License (CC BY). The use, distribution or reproduction in other forums is permitted, provided the original author(s) and the copyright owner(s) are credited and that the original publication in this journal is cited, in accordance with accepted academic practice. No use, distribution or reproduction is permitted which does not comply with these terms.
*Correspondence: Sergio A. Carrasco, c2NhcnJhc2NvLm9AZ21haWwuY29t