- 1U.S. Geological Survey, Wetland and Aquatic Research Center, Gainesville, FL, United States
- 2Cherokee Nations Systems Solutions, Contracted to U.S. Geological Survey, Wetland and Aquatic Research Center, Lafayette, LA, United States
The neritic environment is rich in resources and as such plays a crucial role as foraging habitat for multi-species marine assemblages, including sea turtles. However, this habitat also experiences a wide array of anthropogenic threats. To prioritize conservation funds, targeting areas that support multi-species assemblages is ideal. This is particularly important in the Gulf of Mexico where restoration actions are currently ongoing following the Deepwater Horizon oil spill. To better understand these areas in the Gulf of Mexico, we characterized two multi-species aggregations of sea turtles captured in different neritic habitats. We described species composition and size classes of turtles, and calculated body condition index for 642 individuals of three species captured from 2011 to 2019: 13.6% loggerheads (Caretta caretta), 44.9% Kemp’s ridleys (Lepidochelys kempii) and 41.4% green turtles (Chelonia mydas). Species composition differed between the two sites with more loggerheads captured in seagrass and a greater proportion of green turtles captured in sand bottom. Turtles in sand bottom were smaller and weighed less than those captured in seagrass. Although small and large turtles were captured at both sites, the proportions differed between sites. Body condition index of green turtles was lower in sand habitat than seagrass habitat; there was no difference for Kemp’s ridleys or loggerheads. In general, smaller green turtles had a higher body condition index than larger green turtles. We have identified another habitat type used by juvenile sea turtle species in the northern Gulf of Mexico. In addition, we highlight the importance of habitat selection by immature turtles recruiting from the oceanic to the neritic environment, particularly for green turtles.
Introduction
Habitat loss in marine environments is occurring at an alarming rate and many of these habitats are found in neritic waters (Millennium Ecosystem Assessment, 2013; Babcock et al., 2019). Mangroves have declined 35%, coral reefs have been reduced 20% with an additional 20% identified as degraded, and seagrasses have been disappearing at a rate of 110 km2 year–1 since 1980 (Waycott et al., 2009; Millennium Ecosystem Assessment, 2013). These losses have necessitated conservation actions for many marine species that rely on neritic habitats such as sea turtles (Sellas et al., 2005; Wakefield et al., 2011; Hart et al., 2018a). Neritic habitat plays a consistent and important role for foraging hard-shelled sea turtles (Hays et al., 2004) throughout their entire lives (Bolten, 2003; Lamont et al., 2015). Juvenile sea turtles forage in neritic habitat for a decade or longer, after undertaking an ontogenetic habitat shift from the oceanic environment (Bolten, 2003). However, different turtle species shift into different habitats. For example, hawksbill turtles typically use coral reefs whereas green turtles migrate into seagrass beds. Identifying habitats used by juvenile turtles is crucial because this life-stage is the most important to protect when trying to recover populations (Crouse et al., 1987). Managers cannot ensure that a necessary habitat is available for turtles if managers are unaware that turtles rely on that habitat. In addition, as adults, sea turtles maintain foraging home ranges that are often located in neritic waters (Hart et al., 2014; Braun McNeill et al., 2020; Gredzens and Shaver, 2020), although variation in habitat use occurs among individuals (Hawkes et al., 2007; Hatase et al., 2013; Cameron et al., 2019).
Neritic habitat is also where most anthropogenic threats occur in the oceans. The majority of oil and gas platforms are in water depths < 300 m (Muehlenbachs et al., 2013), commercial shrimping activity occurs primarily in neritic waters (McDaniel et al., 2000), vessel strikes appear to have higher mortality rates in nearshore than offshore waters (Foley et al., 2019), and because of its proximity to land, neritic waters suffer from increased levels of pollution (Fang et al., 2017). Although these activities result in broadscale impacts to habitats that most likely affect organisms on the community-level, many studies focus only on single-species (Hart et al., 2014; Eguchi et al., 2020; Ramirez et al., 2020). However, individual species respond to environmental stressors in different ways; for example, species at a higher trophic level appear to be disproportionally impacted by habitat fragmentation (Didham, 1998; Hovel and Lipcius, 2001; Layman et al., 2007; Roslin et al., 2014; Rielly-Carroll and Freestone, 2017). To identify areas that support multi-species assemblages and, as such. represent high conservation priority (Brodie et al., 2015; Rich et al., 2016), more information on habitats used by these assemblages is needed, particularly those that rely on neritic waters for survival (Easter et al., 2019).
Multi-species assemblages of marine animals have been studied, however, most research has focused on invertebrates or fish (Barnes, 2019; Moyes and Magurran, 2019; Palumbi et al., 2019). Large marine vertebrates also maintain multi-species groups (Augé et al., 2018; Drymon et al., 2020; Sutton et al., 2020) and because these species typically inhabit a higher trophic level, changes in habitat may have serious consequences. Sea turtle foraging assemblages provide the ideal opportunity to examine multi-species groups of marine vertebrates in neritic habitats (Hart et al., 2018a; Lamont and Iverson, 2018; Wildermann et al., 2019). In the Gulf of Mexico (GoM), sea turtles forage in groups that include herbivores such as green turtles (Chelonia mydas) and carnivores such as loggerheads (Caretta caretta) and Kemp’s ridleys (Lepidochelys kempii; Lamont and Iverson, 2018; Wildermann et al., 2019). This provides us with a unique opportunity to collect information across species that may differ in their vulnerability to human disturbances, environmental change, and habitat fragmentation (Easter et al., 2019). Characterizing multi-species assemblages of sea turtles in neritic habitats can help maximize conservation dollars and prioritize restoration efforts, particularly in the GoM where restoration actions following the Deepwater Horizon oil spill are currently being conducted.
Juvenile turtles throughout the world have been shown to exhibit multi-year fidelity to foraging areas (González Carman et al., 2016; Metz et al., 2020; Siegwalt et al., 2020), which further highlights their importance in the conservation and recovery of these species. In Australia, green turtles and loggerheads remained in foraging habitats for 17 and 23 years, respectively. The size of these home ranges varies greatly among species and studies, most likely a factor of study methods used, duration of tracking periods, and environmental conditions (Metz et al., 2020). For example, mean size of core use areas for green turtles in Northeast Florida was 4.4 ± 1.3 km2 (Wildermann et al., 2019) while along the Texas coast, the mean size for green turtles in summer was much larger (125.4 ± 47.5 km2) and when examining only winter core use areas, the mean size in Texas was even greater (543.7 ± 230.6 km2). This variability illustrates the importance of understanding habitat use by turtles under wide array of conditions and habitat types.
In this study, our goal was to characterize immature sea turtles of three species that were captured at two sites in the GoM: a sand bottom habitat in nearshore GoM waters and seagrass-dominated habitat in a coastal bay. We compared species composition and size classes of individuals captured at both locations. In addition, we calculated a body condition index (BCI) for captured turtles as a proxy for fitness to assess whether resource quality between sites affected turtle fitness (Bjorndal and Bolten, 2010; Peig and Green, 2010).
Materials and Methods
St. Joseph Bay (SJB), located in northwest Florida (Figure 1) in the northern GoM, covers approximately 26,000 ha. It has a mean depth of 7 m, the greatest depth being 13.3 m in the northern end and the shallowest being <1 m in the southern end (Florida Department of Environmental Protection, 2008). Seagrass beds cover approximately 16% of the bay (4,000 ha) and are most abundant in the shallow southern end (Florida Department of Environmental Protection, 2008). The most abundant seagrass species is Thalassia testudinum. The sediments in St. Joseph Bay are predominantly sand, sand-silt-clay, sandy clay, and silty clay (Florida Department of Environmental Protection, 2008). The bay is considered one of the most pristine coastal bays in all of Florida. The high salinity and clear water found immediately nearshore in a shallow, low-energy environment in the northern Gulf of Mexico is unique and provides for a diverse ecosystem (Florida Department of Environmental Protection, 2008). St. Joseph Bay offers some of the world’s best fishing grounds for a variety of species including bay scallops (Argopecten irradians) and these activities serve as the foundation for the economy of this area (Florida Department of Environmental Protection, 2008).
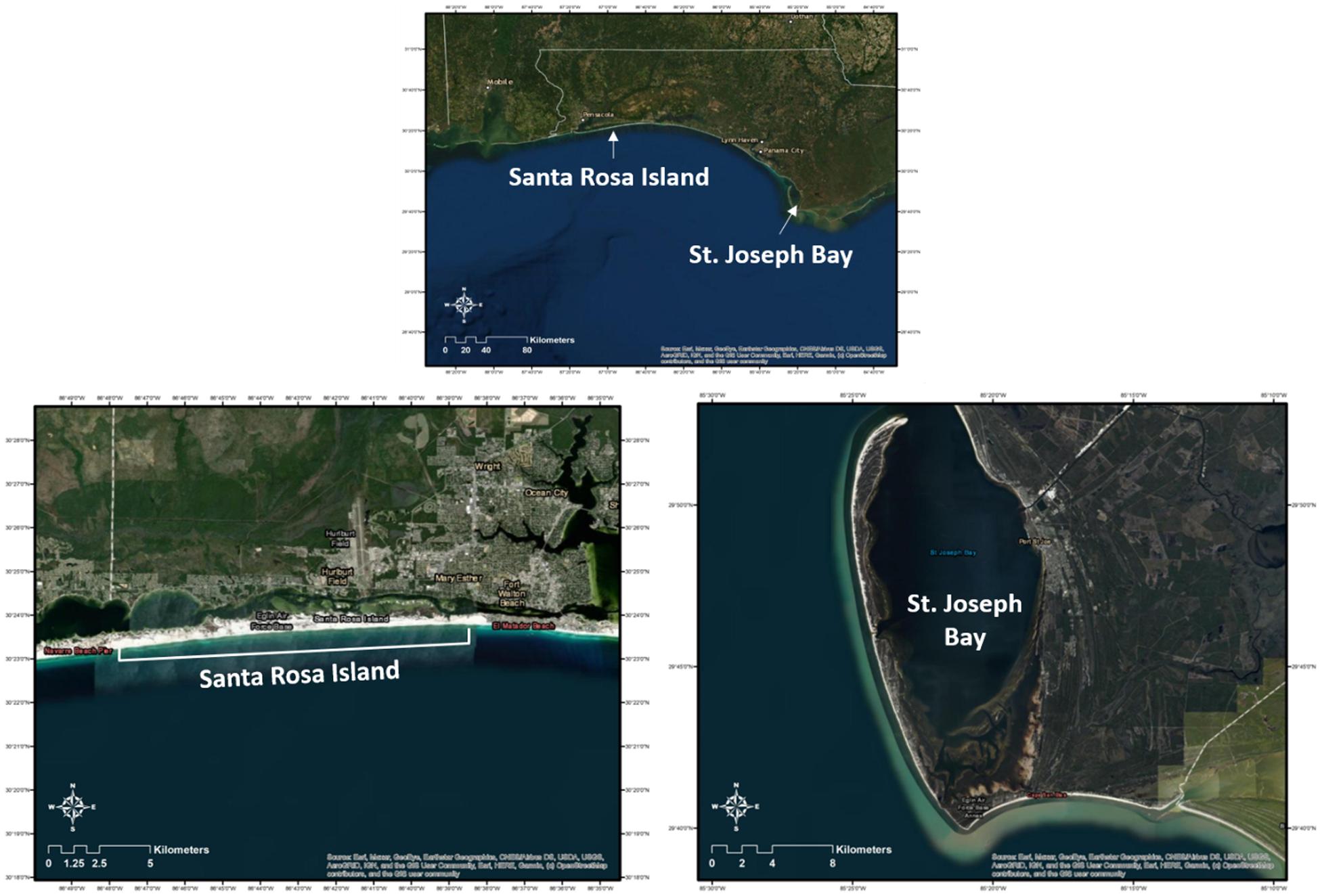
Figure 1. Location of Santa Rosa Island (SRI) and St. Joseph Bay (SJB) in Northwest Florida where immature sea turtles were captured from 2011 to 2019 (SJB) and 2014–2019 (SRI). Also included are individual images of each study site. These maps (with self-created symbols/shapes/text) were created using ESRI (Environmental Systems Resource Institute; http://www.esri.com/software/arcgis) ArcMap software, version 10.7.1. Basemap sources for all Figures, except c (terrapin), include: Esri, Maxar, GeoEye, Earthstar Geographics, CNES/Airbus DS, USDA, USGS, AeroGRID, IGN, and the GIS User Community.
The Santa Rosa Island (SRI) site encompasses approximately 21 km of GoM coastline that is owned by Eglin Air Force Base. The nearshore sediments in this area are predominately fine silica sand (Williams et al., 2012). The study site is bordered by Okaloosa Island to the east, with the Okaloosa pier located approximately 4 km east of the study site boundary and the Destin pass approximately 8 km beyond the pier. The Destin pass serves as the entrance to Choctawhatchee Bay, which supports approximately 2,300 ha (7%) of seagrass habitat. The western end of the study site is bounded by Navarre Beach. The Navarre Beach pier is located approximately 1.4 km from the study site boundary. In addition, about 0.5 km west of the study site is the Navarre Beach Marine Sanctuary, an artificial reef that consists of 78 structures constructed of piling-mounted concrete disks located 340 feet south of the mean high tide line.
All turtles were captured between March and November 2011–2019 (SJB) and 2014–2019 (SRI). In SJB, turtles were surveyed for and captured from a boat using a set net, dip net, cast net or by hand. At SRI, turtles were surveyed for from all-terrain vehicles (ATVs) ridden on the beach. Once observed, turtles were captured using a modified set net technique. In this method, turtles were observed in nearshore waters typically less than 2 m deep and within 100 m of shore. When a turtle was observed, biologists deployed a short (approximately 20 m) set net about 100 m in front of the turtle while additional personnel entered the water behind the turtle. As the turtle swam forward to avoid the personnel approaching from its rear, it became tangled in the net. All captured turtles at both locations were individually marked with a metal Inconel tag placed along the trailing edge of each front flipper and a passive integrated transponder (PIT) tag placed subcutaneously in the left shoulder. Turtles were measured using two methodologies: (1) straight carapace length (SCL) and width (SCW) using calipers and (2) curved carapace length (CCL) and width (CCW) using a cloth tape measure. Weight (Wgt; in kilograms) was determined by placing the turtle in a harness and hanging the harness from a hand-held Pesola spring scale.
Straight carapace lengths were used in all analyses. If SCL was not gathered for an individual, CCL was converted to SCL using the following regression equations from Teas (1993):
SCL = −1.442 + (0.948 × CCL) for loggerheads
SCL = 0.013 + (0.945 × CCL) for Kemp’s ridleys
SCL = 0.294 + (0.937 × CCL) for green turtles.
Body condition was calculated as Fulton’s K (BCI = body mass/SCL3 × 104; Bjorndal et al., 2000).
A generalized linear model (GLM) was used to evaluate relationships among years, species, sites, size classes and seasons. To account for differences in life-stages within species, we placed individuals into two groups: small juveniles and large juvenile/adults. For Kemp’s, small juveniles were <45 cm SCL and for greens they were <60 cm SCL. Because loggerheads were all large juveniles/adults, we did not divide them into groups. The response variables were SCL, BCI, and Wgt. We ran three univariate models on SCL, BCI, and Wgt and calculated the residuals. Using Pearson’s correlation test on the residuals, we found that BCI and Wgt were not correlated (p > 0.5), yet SCL and Wgt were correlated for both sites (p < 0.0001). Hence, a 2-way MANOVA was run on the correlated responses of SCL and Wgt and an ANOVA was run on BCI. Loggerheads were dropped from the analyses because of a lack of samples at SRI (n = 1). We used the Wilks’ Lambda statistic for the MANOVA tests. PROC GLM in SAS 9.4 was used to run the analyses and an alpha = 0.05 was established for all analyses. Models were checked for homogeneity and normality of residuals. Mean differences for main effects were compared using Tukey’s test and significant interactions were compared using a Bonferroni correction test. Finally, a Log Likelihood model comparison test was used to identify the best final model for BCI.
Results
From 2011 to 2019 (SJB) and 2014–2019 (SRI), 642 turtles were captured at both sites (Table 1). Mean water depth at capture in SJB was 1.32 ± 0.45 m (range 0.18–3.26 m) and at SRI was 1.13 ± 0.61 (range 0.60–3.66). The majority (78.0%) of turtles were captured in SJB. Captured turtles included 88 (13.7%) loggerheads, 288 (44.9%) Kemp’s ridleys, and 266 (41.4%) green turtles. Of all turtles captured, we had SCL and weight for 535 (83.3%) individuals, which allowed for calculation of BCI (Table 1). The majority of turtles captured at both sites were juveniles. Using 87.0 cm SCL as size at sexual maturity for loggerheads (NMFS and USFWS, 2008) only 5.7% of loggerheads captured in SJB were adults. Using the minimum size of nesting Kemp’s of 57.2 cm (Shaver et al., 2016) and size at reproduction for greens of 83.2 cm SCL (Goshe et al., 2010), none of the Kemp’s ridleys we captured at either site were adults. We captured two adult male green turtles at SRI but all other green turtles captured at both sites were juveniles.
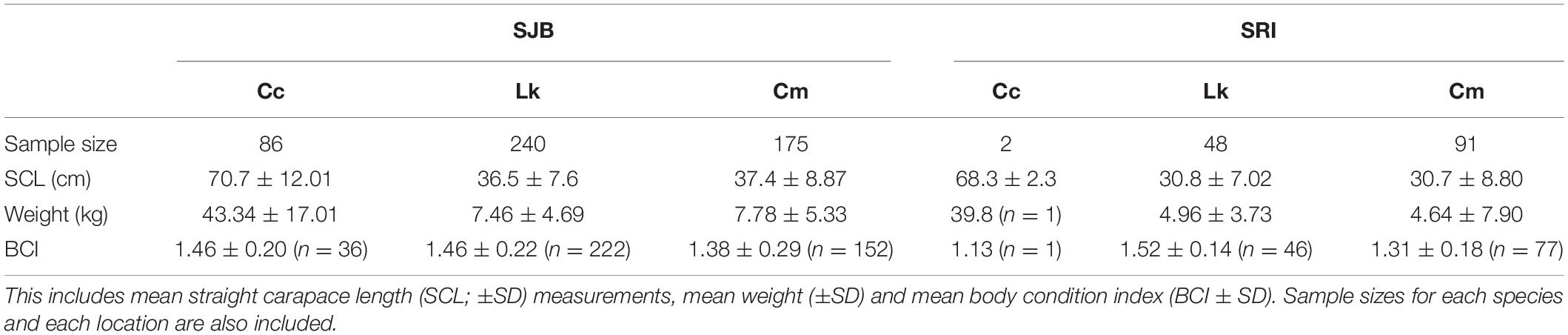
Table 1. Characteristics of loggerhead (Cc), Kemp’s ridley (Lk) and green (Cm) turtles captured in St. Joseph Bay (SJB) and off Santa Rosa Island (SRI), Florida from 2011 to 2019 (SJB) and 2014–2019 (SRI).
St. Joseph Bay
From 2011 to 2019, 501 turtles of all three species were caught in SJB (Table 1). Most (54.7%) of turtles were captured by hand, while 27.8% were captured in a set net, 17.0% in a dip net, and 0.4% in a cast net. Turtles were captured in every month of the year with most captured in July (17.4%) and October (18%). Mean SCL and weight for all turtles captured in SJB are presented in Table 1 and proportion per size class in Figure 2. Mean recapture rate for all turtles in SJB was 10.2% and was lowest for loggerheads (5.8%) and highest for Kemp’s ridleys (11.7%). Recapture rate for green turtles was 9.1%. Mean recapture interval for all turtles in SJB was 399 days (n = 29; SD 411.3; range 6–1,649).
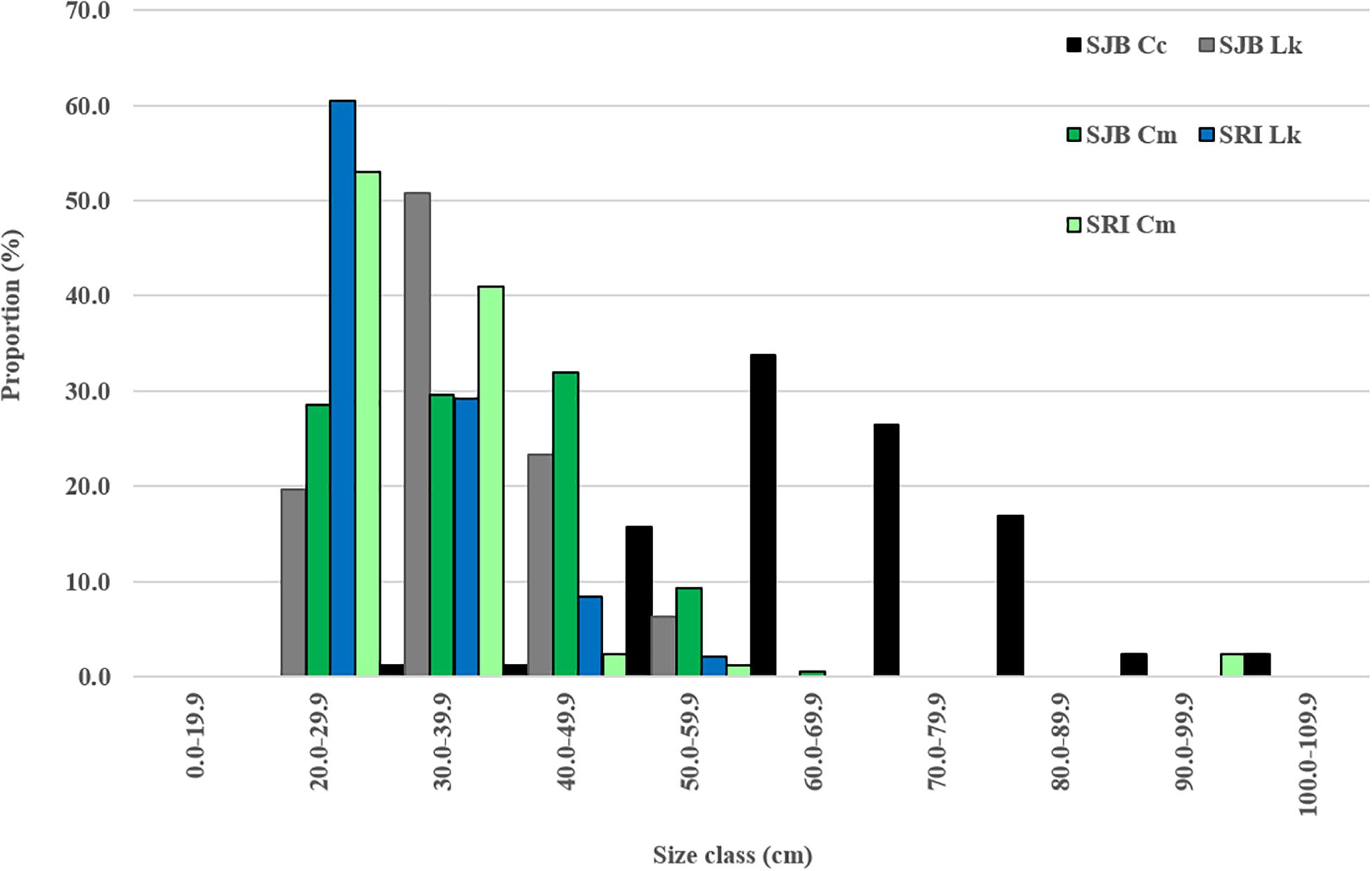
Figure 2. Proportion of all captures that were loggerheads (Cc), Kemp’s ridleys (Lk) and green turtles (Cm) per size class (straight carapace length in cm) at two sites in Northwest Florida: off Santa Rosa Island (SRI) from 2014 to 2019 and in St. Joseph Bay (SJB) from 2011 to 2019. Dashed vertical lines illustrate minimum size at maturity for all three species.
Mean BCI for loggerheads in SJB was 1.46 (SD 0.20, range 0.90–1.87). Mean BCI for Kemp’s ridleys in SJB was 1.46 (SD 0.22, range 0.47–3.17). Mean BCI for green turtles was 1.38 (SD 0.29, range 0.87–4.13). Mean BCI per size class for each species is presented in Table 2.
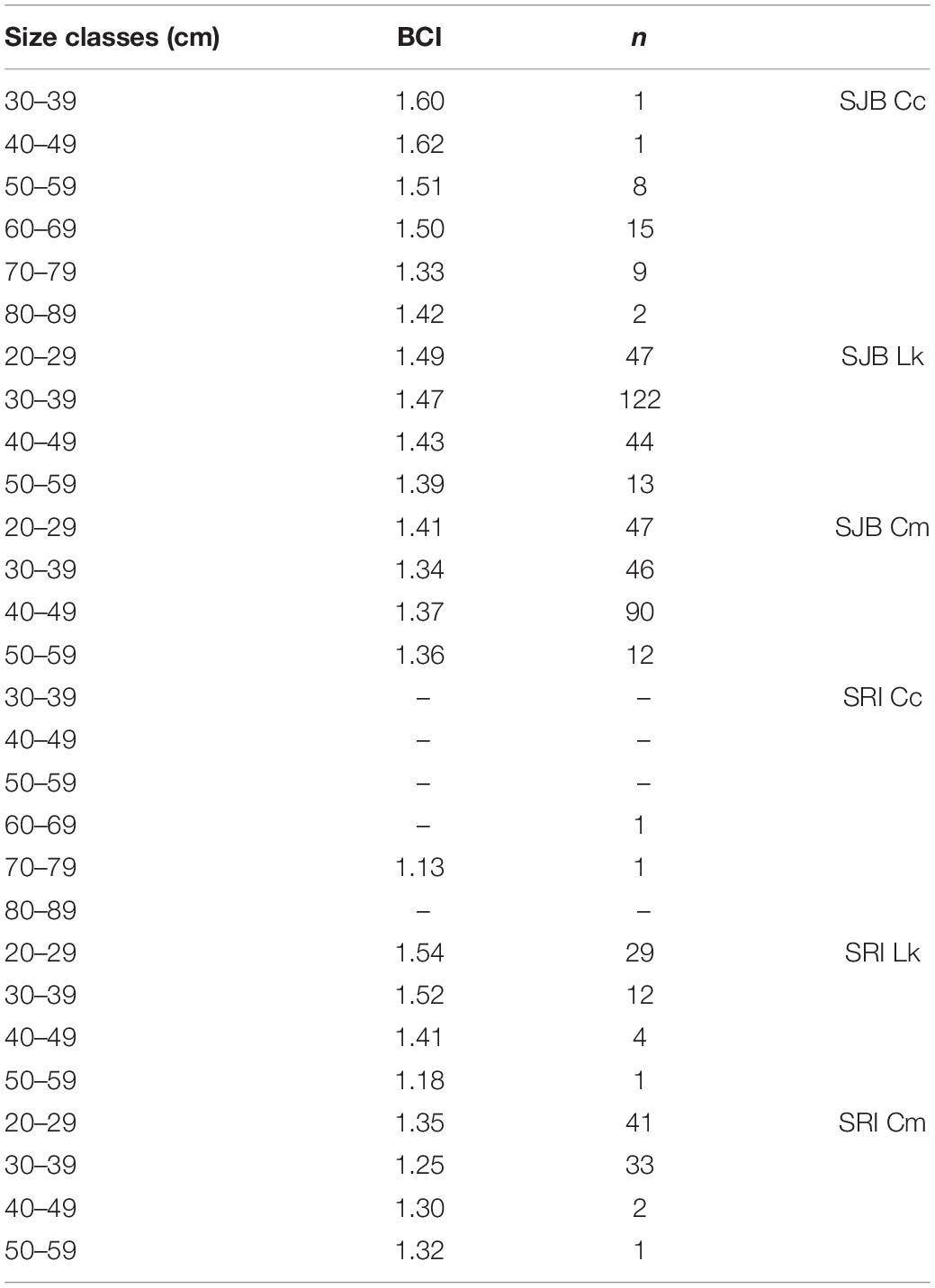
Table 2. Body condition index (BCI), calculated as body mass/SCL3 × 104, per size class (straight carapace length, SCL, in cm) for Kemp’s ridleys (Lk) and green turtles (Cm) captured off Santa Rosa Island (SRI) from 2014 to 2019 and in St. Joseph Bay (SJB), Florida from 2011 to 2019, and for loggerheads (Cc) captured in SJB from 2011 to 2019. Only two loggerheads were captured off SRI.
Santa Rosa Island
From 2014 to 2019, 141 turtles were captured off SRI (Table 1). All turtles (except one which was captured by hand) were captured using the modified set net technique. Turtles were captured May through October with most captures occurring in September (26.2%) and October (44.7%). Mean SCL and weight for all turtles captured in SJB are presented in Table 1 and proportion per size class in Figure 2. Two of the green turtles captured at SRI were adult males; with those two individuals excluded the mean size of remaining green turtles was 29.9 cm (SD 4.86, range 22.0–55.0 cm) and weight was 3.79 Kg (SD 2.50, range 1.5–17.0 kg). Mean recapture rate for all turtles was 28.4% and was highest for green turtles (30.8%) and Kemp’s ridleys (25.0%) and lowest for loggerheads (0%). Mean recapture interval was 133 days (n = 27; SD 176.2; range 1–724).
Of the 141 turtles captured at SRI, we had SCL and weight on 123 turtles allowing for calculation of BCI (Table 1). We were unable to record weight for one of the loggerheads captured at SRI therefore we were unable to include this individual in BCI calculations. As such, we also dropped the one remaining loggerhead from statistical comparisons. The 123 turtles used in statistical analyses included 46 Kemp’s ridleys and 77 green turtles. Mean size of all turtles was 30.23 cm (SD 5.78, range 22.0–52.7 cm). Mean BCI per size class for Kemp’s ridleys and green turtles is presented in Table 2.
Data Analyses
In the MANOVA, the only significant parameters were site (p = 0.0001), year (p = 0.0003), and group (p ≤ 0.0001; Supplementary Table 1A). The overall model for BCI was significant (p < 0.0001). Of the independent variables (species, site, size class and season), the highest order significant interactions (Supplementary Table 1B) were species ∗ site (p = 0.0084) and species ∗ size (p = 0.0164). All additional comparisons were not significant. Means comparisons using Tukey’s test revealed that SCL and weight were smaller at SRI than SJB. All other comparisons were not significant. Because the model suggested year had no influence on BCI, we did not adjust for year in the analyses. However, because year and size (i.e., group) was shown to influence SCL, we adjusted for those two variables in the model and found differences in SCL between sites was still significantly different.
Green turtles off SRI had lower BCI than green turtles in SJB (p = 0.0115). At SRI, BCI of greens turtles was lower than BCI of Kemp’s (p < 0.0001). Among all size classes of green turtles at both sites, BCI of 20.0–29.9 and 30.0–39.9 cm SCL turtles was lower than BCI of similarly sized Kemp’s ridleys (there was no difference among larger turtles). Within all green turtles (combined between sites), BCI of individuals 30.0–39.9 cm SCL was lower than BCI of individuals 20.0–29.9 cm SCL. All other comparisons were not significant.
Discussion
The role neritic habitat plays in the development of immature sea turtles has been broadly acknowledged (Musick and Limpus, 1997; Bolten, 2003; Broadbent et al., 2020). However, our results highlight variability in composition and habitat use of multi-species assemblages within this zone and suggest sea turtles in the northern GoM recruit into at least two different neritic habitats: seagrass meadows in coastal bays and nearshore sand bottom habitat. This variation in habitat selection may have consequences to turtles in the form of lower fitness (Diez and Van Dam, 2002). In addition, results of this study may contribute to restoration activities currently underway in the GoM in response to the Deepwater Horizon oil spill. Many of those activities are occurring in nearshore waters; a better understanding of what habitats are important to juvenile Kemp’s ridleys and green turtles can help optimize these restoration projects for juvenile sea turtles. This is particularly important considering the juvenile life-stage is the most responsive to recovery actions (Crouse et al., 1987). Although new recruits to the neritic environment must move through nearshore waters on their way to coastal bays, the long-term and consistent presence of individuals off SRI supports the idea that this area may also serve as developmental habitat for immature turtles until they reach reproductive maturity.
Immature sea turtles exhibit variability in foraging habitat selection (McClellan and Read, 2010; Seney and Landry, 2011; Lamont and Iverson, 2018; Schmid and Tucker, 2018). For example, green turtles forage in habitats ranging from tidal creeks in the Florida Everglades (Hart and Fujisaki, 2010) to pristine seagrass beds (Lamont and Iverson, 2018) to human-made and/or altered environments (Kubis et al., 2009; Eguchi et al., 2020). As such, it was not unusual for us to document immature turtles of all three species in two different habitat types in the northern GoM. Green turtles and Kemp’s ridleys represented the majority of captures at both sites (82.8% in SJB; 98.6% off SRI) but loggerheads represented a larger proportion of captures in SJB (17.2%) than off SRI (1.4%). The difference in proportion of loggerheads captured may reflect dispersal patterns by hatchling loggerheads (Putman et al., 2020) or differences in recruitment by immature loggerheads (Bolten, 2003), however, it may also simply reflect variation in capture methods between the two sites. In SJB, we used a boat to survey for and then hand capture loggerheads, whereas at SRI, we conducted surveys from land. These land-based surveys limit the distance from shore at which we are able to capture individuals. Loggerheads may not travel as close to the coast as green turtles and Kemp’s ridleys (Hart et al., 2018b) thereby making them less available for capture at SRI than in SJB.
The proximity of SRI to fishing piers may contribute to differential habitat use by species between the two sites, particularly for Kemp’s ridleys and green turtles. Both of those species are captured frequently in recreational fishing activities from piers (Coleman et al., 2016; Cook et al., 2020) even though the prey available at fishing piers (i.e. bait) are not typical foraging items for these species (Williams et al., 2013; Ramirez et al., 2020). If juvenile Kemp’s ridleys and green turtles are attracted to fishing piers as foraging locations, the presence of those structures in nearshore sandy-bottom habitats across the GoM may provide foraging sites for multi-species assemblages. The quality of that habitat may not be ideal, however, and the lower BCI we documented for juvenile green turtles at SRI versus in SJB support that theory. Further studies examining turtle use of fishing piers are needed to better understand the impacts of these structures on turtle populations.
In addition to species composition, we documented variation in size classes of turtles captured at the two sites. Green turtles and Kemp’s ridleys at SRI were smaller than those in SJB. Although small individuals (<25 cm SCL) of both species were captured at SJB and SRI, a larger proportion of small individuals were captured at SRI (Figure 2). As with loggerheads, differences in size classes captured at both sites could reflect capture methods. Larger green turtles and Kemp’s ridleys might remain in deeper waters more often than smaller individuals making them less available for capture, however, this would seemingly affect turtles in SJB also since we are typically unable to hand capture turtles in deep (>4 m) waters in SJB due to turbidity. Therefore, we suspect this difference reflects ontogenetic habitat shifts by immature turtles in the neritic environment. The smallest turtles captured at both sites are most likely new recruits to neritic habitat. Analysis of cloacal microbiome in green turtles captured at SJB and SRI showed bacterial communities of turtles at SRI were more similar to turtles captured in the oceanic environment than to those captured in seagrass habitat in SJB (Price et al., 2017), which suggests these small individuals may have recently recruited from the oceanic zone.
However, long-term recaptures (>365 days) of green turtles and Kemp’s ridleys at SRI demonstrates multi-year fidelity by some individuals to that site. The longest recapture at SRI for green turtles was 388 days and for Kemp’s was 724 days. Without movement data (i.e., satellite or acoustic tracking), it is not possible to say for certain that turtles did not move from SRI into seagrass habitat and back again during these time periods. Tracking studies in SJB are limited but show that distance moved from core use areas differed among species (Lamont and Iverson, 2018). Loggerheads moved a mean distance of 59.6 km from the core use areas whereas greens moved a mean distance of 14.6 km and Kemp’s ridleys traveled 28.8 km. If turtles at SRI moved similar distances, they would have access to seagrass habitat in Choctawhatchee Bay (located approximately 13 km from the eastern boundary of our study site). Additional diet studies, including gut bacteria and stable isotopes (Burgett et al., 2018; Campos et al., 2019), could help clarify whether SRI serves only as a stop-over area for new recruits moving into the neritic zone or also as a long-term developmental habitat for immature turtles.
If SRI is a developmental habitat for immature turtles, it appears to provide sub-optimal foraging resources for green turtles. The BCI for green turtles was lower off SRI than in SJB, even though SRI green turtles were on average smaller than SJB green turtles, and mean BCI of smaller (20.0–29.9 cm) green turtles was greater than mean BCI of larger (30.0–39.9 cm) green turtles (see Table 2). Body condition index is a rough proxy for nutritional status and health (Diez and Van Dam, 2002) and our two sites differ in availability of one major source of nutrition for green turtles: seagrass. SJB supports more than 4,000 ha of seagrass while SRI has none. Diet of green turtles at SRI is unknown, however, we have observed turtles foraging on algal-covered structures and analyses of cloacal bacteria of green turtles at SRI suggested an algal diet (Price et al., 2017). Although it is not uncommon for immature green turtles to forage on algae (Bjorndal, 1980; Williams et al., 2013), growth rates of individuals in seagrass beds along Florida’s east coast was higher than that of turtles using algal habitat (Kubis et al., 2009). The lack of difference in BCI for Kemp’s ridleys between the two sites support this idea, as Kemp’s ridleys forage on benthic invertebrates, particularly crabs (Shaver, 1991; Witzell and Schmid, 2004) that are common at both sites.
Foraging in atypical habitat does not always result in negative consequences for sea turtles. For example, hawksbills in the Bahamas foraged in seagrass beds rather than on coral reefs, however, growth rates and BCI of individuals did not differ between the two habitats (Bjorndal and Bolten, 2010). Although there is seagrass habitat in coastal bays near SRI (e.g., Choctawhatchee Bay and Santa Rosa Sound), it appears from recaptures that at least some green turtles remain off SRI rather than moving into the nearby bays. Why they choose to remain in seemingly suboptimal habitat is unknown. Off the Cape Verde Islands, Africa most adult loggerheads forage in oceanic waters even though neritic foragers were larger and laid bigger clutches (Eder et al., 2012). Eder et al. (2012) suggest these loggerheads forage in oceanic waters as juveniles and as such select that habitat as adults (Hays et al., 2010). As they mature, however, some individuals may become aware of the productive neritic habitat and move into those waters. This may be the case with immature green turtles; as they recruit from oceanic to neritic habitat, some may encounter algae resources and remain to forage whereas others do not and continue into seagrass meadows. Examination of movement patterns and diet of green turtles in these two habitats is needed to address that question.
Mean BCI for all species was within the range reported at other sites and we found no differences in BCI among species (Table 3; Labrada-Martagón et al., 2010; Bjorndal et al., 2014). However, differences in morphology among sea turtle species limit among-species comparisons of Fulton’s BCI (i.e., a lower BCI for green turtles as compared to Kemp’s ridleys does not suggest green turtles have lower fitness than Kemp’s ridleys). Fulton’s BCI is based on the relationship between mass and body length. Sea turtle species differ in size and shape; for example, of the hard-shelled sea turtles, green turtles are the largest whereas Kemp’s ridleys are the smallest (Wyneken, 2001). Peig and Green (2010) found Fulton’s BCI produced CI’s that decreased with size therefore we would expect variation in BCI among larger and smaller turtle species. Although Fulton’s index has received some criticism (see Stevenson and Woods, 2017), it performed well in a comparison of different CI methods (Peig and Green, 2010). In general, body condition has been shown to be closely related to an animal’s health (Peig and Green, 2009) and has been widely considered an important indication of fitness (Peig and Green, 2010). This is exemplified in the loggerhead we captured off SRI. This individual was lethargic and sent to a rehabilitation center after capture. The BCI for this individual was 1.13 compared to the mean BCI of 1.46 for loggerheads captured in SJB. The low BCI for the SRI loggerhead highlights the functionality of Fulton’s BCI for this species. However, among species comparisons must consider morphological differences among those the species being examined (Peig and Green, 2010).
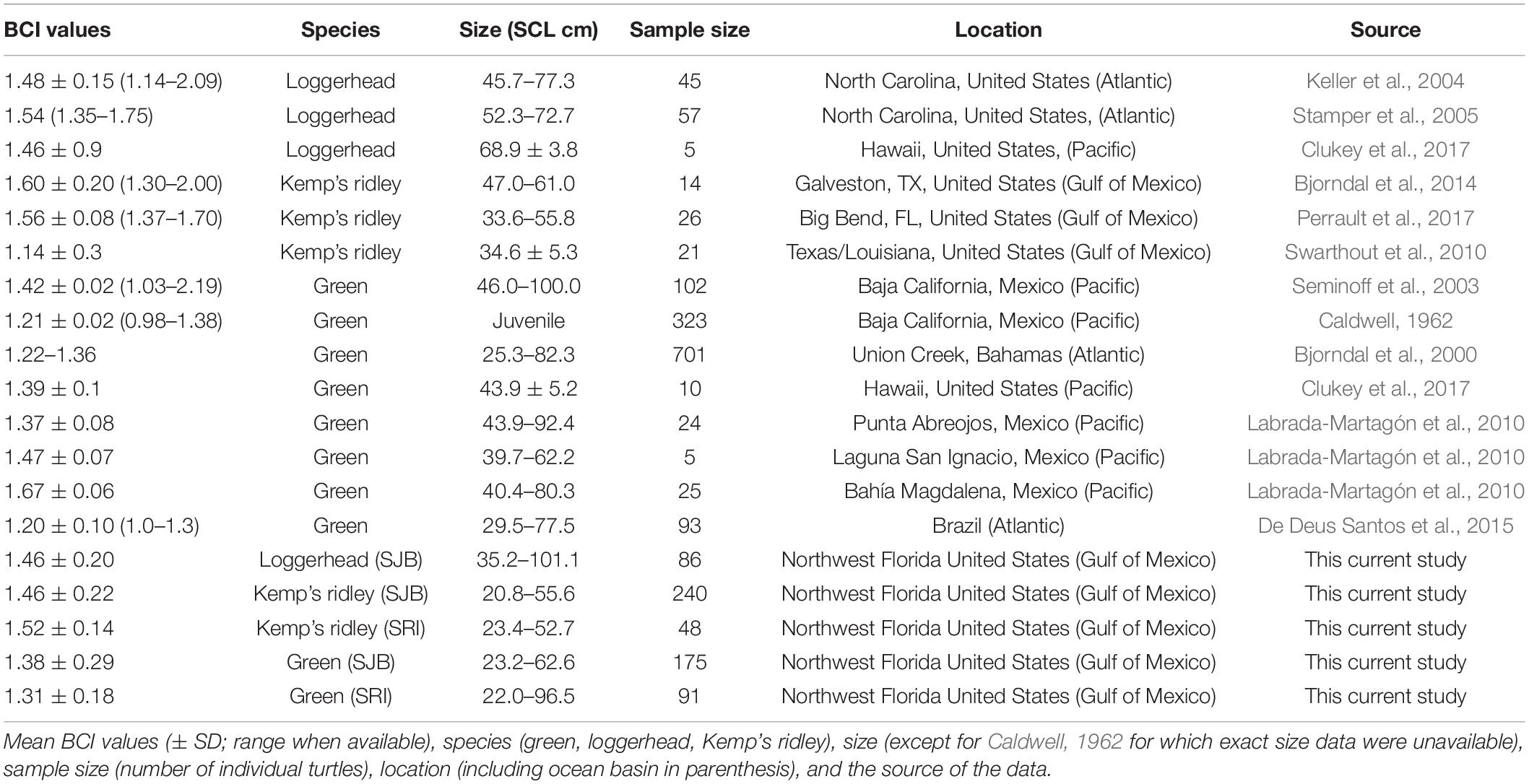
Table 3. Body condition index (BCI) values for green, Kemp’s ridley or loggerhead sea turtles reported from sites throughout the world.
Body condition index for green turtles has also been linked to population density and growth rates (Bjorndal et al., 2000). As population densities increase, growth rates and BCI decrease (Bjorndal et al., 2000; Seminoff et al., 2003; Labrada-Martagón et al., 2010). It has been suggested that green turtles in SJB are reaching carrying capacity and may be a threat to the bay’s seagrass beds through overgrazing (Rodriguez and Heck, 2020). However, high population densities should result in lower growth rates and BCIs (Bjorndal et al., 2000), and in our study, the BCIs we calculated for green turtles from 2011 to 2019 were similar to or higher than those reported for green turtles captured in SJB from 2001 to 2004 by McMichael et al. (2008), and for green turtles that stranded in SJB in 2010 by Avens et al. (2012; Supplementary Table 2). Growth rates of green turtles captured during a mass stranding event in SJB in 2010 fell within the range of growth rates reported from elsewhere in the world (Avens et al., 2012) and did not appear to decrease over time when compared to rates reported by McMichael et al. (2008). An updated analysis of growth rates for green turtles in SJB is warranted to address this question, however, the data that are currently available on BCI (this study) and growth rates (McMichael et al., 2008; Avens et al., 2012) do not support the theory that the green turtle population in SJB has reached carrying capacity.
Our characterization of these multi-species sea turtle assemblages that utilize two different neritic habitats identifies high value areas that may be targeted for conservation actions and raises questions about habitat selection by new recruits to the neritic environment, particularly for green turtles. Additional studies across the Gulf of Mexico, and other ocean basins, would increase sample sizes and clarify habitat needs for neritic turtles. Gulf-wide studies are particularly needed in light of the intense habitat restoration occurring in neritic waters. Nearshore sand bottom habitat appears optimal for immature Kemp’s ridleys but less ideal for green turtles. This habitat selection may therefore have serious consequences to the fitness of immature turtle populations. This study also highlights the need for more research on diet and fine-scale habitat use to further explore these questions.
Data Availability Statement
The datasets generated for this study will not be made publicly available. Restrictions apply to the datasets. Raw data is exempt from publication due to the sensitivity of endangered species information. Requests to access the datasets should be directed to the corresponding author. All other data used for analyses are presented in the manuscript.
Ethics Statement
The animal study was reviewed and approved by the USGS IACUC committee.
Author Contributions
ML conceived of and designed the study. DJ performed statistical analyses. ML and DJ created figures. Both authors contributed to writing the manuscript, read, and approved the submitted version.
Funding
Funding for this work was provided by Eglin Air Force Base (AFB) Division of Natural Resources.
Conflict of Interest
DJ was employed by the company Cherokee Nations Systems Solutions.
The remaining authors declare that the research was conducted in the absence of any commercial or financial relationships that could be construed as a potential conflict of interest.
Acknowledgments
We are grateful to Kathleen Gault at Eglin Air Force Base for continued collaboration. We thank B. Hagedorn and J. Johnson with Eglin AFB for their constant support, and E. Laine, B. Stephens, D. Catizone, C. Gindl, D. Seay, J. Alday, C. Arends and multiple technicians for field assistance.
Supplementary Material
The Supplementary Material for this article can be found online at: https://www.frontiersin.org/articles/10.3389/fmars.2020.608740/full#supplementary-material
References
Augé, A. A., Dias, M. P., Lascelles, B., Baylis, A. M. M., Black, A., Boersma, P. D., et al. (2018). Framework for mapping key areas for marine megafauna to inform Marine Spatial Planning: The Falkland Islands case study. Mar. Policy 92, 61–72. doi: 10.1016/j.marpol.2018.02.017
Avens, L., Goshe, L. R., Harms, C. A., Anderson, E. T., Hall, A. G., Cluse, W. M., et al. (2012). Population characteristics, age structure, and growth dynamics of neritic juvenile green turtles in the northeastern Gulf of Mexico. Mar. Ecol. Prog. Ser. 458, 213–229. doi: 10.3354/meps09720
Babcock, R. C., Bustamante, R. H., Fulton, E. A., Fulton, D. J., Haywood, M. D. E., Hobday, A. J., et al. (2019). Severe continental-scale impacts of climate change are happening now: Extreme climate events impact marine habitat forming communities along 45% of Australia’s coast. Front. Mar. Sci. 6:1–14. doi: 10.3389/fmars.2019.00411
Barnes, R. S. K. (2019). Spatial structure of a multi-species guild: the dominant biofilm-grazing microgastropods of seagrass. Hydrobiologia 827, 293–307. doi: 10.1007/s10750-018-3781-y
Bjorndal, K. A. (1980). Nutrition and grazing behavior of the green turtle Chelonia mydas. Mar. Biol. 56, 147–154. doi: 10.1007/BF00397131
Bjorndal, K. A., and Bolten, A. B. (2010). Hawksbill sea turtles in seagrass pastures: Success in a peripheral habitat. Mar. Biol. 157, 135–145. doi: 10.1007/s00227-009-1304-0
Bjorndal, K. A., Bolten, A. B., and Chaloupka, M. Y. (2000). Green turtle somatic growth model: evidence for density dependence. Ecol. Appl. 10:269. doi: 10.2307/2641001
Bjorndal, K. A., Parsons, J., Mustin, W., and Bolten, A. B. (2014). Variation in age and size at sexual maturity in Kemp’s ridley sea turtles. Endanger. Species Res. 25, 57–67. doi: 10.3354/esr00608
Bolten, A. B. (2003). “Active swimmers - passive drifters: the oceanic juvenile stage of loggerheads in the Atlantic system,” in Loggerhead Sea Turtles, eds A. B. Bolten and B. E. Witherington (Washington, D.C: Smithsonian Institution Press), 63–78.
Braun McNeill, J., Avens, L., Goodman Hall, A., Fujisaki, I., and Iverson, A. (2020). Foraging and overwintering behavior of loggerhead sea turtles Caretta caretta in the western North Atlantic. Mar. Ecol. Prog. Ser. 641, 209–225. doi: 10.3354/meps13296
Broadbent, H. A., Grasty, S. E., Hardy, R. F., Lamont, M. M., Hart, K. M., Lembke, C., et al. (2020). West Florida shelf pipeline serves as sea turtle benthic habitat based on in situ towed camera observations. Aquat. Biol. 29, 17–31. doi: 10.3354/ab00722
Brodie, J. F., Giordano, A. J., Dickson, B., Hebblewhite, M., Bernard, H., Mohd-Azlan, J., et al. (2015). Evaluating multispecies landscape connectivity in a threatened tropical mammal community. Conserv. Biol. 29, 122–132. doi: 10.1111/cobi.12337
Burgett, C. M., Burkholder, D. A., Coates, K. A., Fourqurean, V. L., Kenworthy, W. J., Manuel, S. A., et al. (2018). Ontogenetic diet shifts of green sea turtles (Chelonia mydas) in a mid-ocean developmental habitat. Mar. Biol. 165, 1–12. doi: 10.1007/s00227-018-3290-6
Caldwell, D. K. (1962). Carapace length–body weight relationship and size and sex ratio of the northeastern Pacific green turtle, Chelonia mydas carrinegra. Contrib. Sci. 62, 3–10.
Cameron, S. J. K., Baltazar-Soares, M., Stiebens, V. A., Reischig, T., Correia, S. M., Harrod, C., et al. (2019). Diversity of feeding strategies in loggerhead sea turtles from the Cape Verde archipelago. Mar. Biol. 166, 1–12. doi: 10.1007/s00227-019-3571-8
Campos, P., Guivernau, M., Prenafeta-Boldú, F. X., and Cardona, L. (2019). Fast acquisition of a polysaccharide fermenting gut microbiome by juvenile green turtles Chelonia mydas after settlement in coastal habitats. Microbiome 6, 1–11. doi: 10.1186/s40168-018-0454-z
Clukey, K. E., Lepczyk, C. A., Balazs, G. H., Work, T. M., and Lynch, J. M. (2017). Investigation of plastic debris ingestion by four species of sea turtles collected as bycatch in pelagic Pacific longline fisheries. Mar. Pollut. Bull. 120, 117–125. doi: 10.1016/j.marpolbul.2017.04.064
Coleman, A. T., Pulis, E. E., Pitchford, J. L., Crocker, K., Heaton, A. J., Carron, A. M., et al. (2016). Population ecology and rehabilitation of incidentally captured kemp’s ridley sea turtles (Lepidochelys kempii) in the Mississippi sound. USA. Herpetol. Conserv. Biol. 11, 253–264.
Cook, M., Dunch, V. S., Coleman, A. T., Shaver, D. J., and Maxwell, S. M. (2020). An Interview-Based Approach to Assess Angler Practices and Sea Turtle Captures on Mississippi Fishing Piers. Front. Mar. 7:1–9. doi: 10.3389/fmars.2020.00655
Crouse, D. T., Crowder, L. B., and Caswell, H. (1987). A stage-based population model for loggerhead sea turtles and implications for conservation. Ecology 68, 1–17.
De Deus Santos, M. R., Martins, A. S., Baptistotte, C., and Work, T. M. (2015). Health condition of juvenile Chelonia mydas related to fibropapillomatosis in southeast Brazil. Dis. Aquat. Organ. 115, 193–201. doi: 10.3354/dao02883
Didham, R. K. (1998). Trophic structure stability and extinction dynamics of beetles (Coleoptera) in tropical forest fragments. Philos. Trans. R. Soc. B Biol. Sci. 353, 437–451. doi: 10.1098/rstb.1998.0221
Diez, C. E., and Van Dam, R. P. (2002). Habitat effect on hawksbill turtle growth rates on feeding grounds at Mona and Monito Islands. Puerto Rico. Mar. Ecol. Prog. Ser. 234, 301–309. doi: 10.3354/meps234301
Drymon, J. M., Dedman, S., Froeschke, J. T., Seubert, E. A., Jefferson, A. E., Kroetz, A. M., et al. (2020). Defining Sex-Specific Habitat Suitability for a Northern Gulf of Mexico Shark Assemblage. Front. Mar. Sci. 7:1–18. doi: 10.3389/fmars.2020.00035
Easter, T., Bouley, P., and Carter, N. (2019). Opportunities for biodiversity conservation outside of Gorongosa National Park. Mozambique: A multispecies approach. Biol. Conserv. 232, 217–227. doi: 10.1016/j.biocon.2019.02.007
Eder, E., Ceballos, A., Martins, S., Pérez-García, H., Marín, I., Marco, A., et al. (2012). Foraging dichotomy in loggerhead sea turtles Caretta caretta off northwestern Africa. Mar. Ecol. Prog. Ser. 470, 113–122. doi: 10.3354/meps10018
Eguchi, T., Bredvik, J., Graham, S., LeRoux, R., Saunders, B., and Seminoff, J. A. (2020). Effects of a power plant closure on home ranges of green turtles in an urban foraging area. Endanger. Species Res. 41, 265–277. doi: 10.3354/ESR01027
Fang, X., Hou, X., Li, X., Hou, W., Nakaoka, M., and Yu, X. (2017). Ecological connectivity between land and sea: a review. Ecol. Res. 33, 51–61. doi: 10.1007/s11284-017-1549-x
Florida Department of Environmental Protection. (2008). St. Joseph Bay Aquatic Preserve Management Plan. Tallahassee, FL: Florida Department of Environmental Protection.
Foley, A. M., Stacy, B. A., Hardy, R. F., Shea, C. P., Minch, K. E., and Schroeder, B. A. (2019). Characterizing watercraft-related mortality of sea turtles in florida. J. Wildl. Manage. 83, 1057–1072. doi: 10.1002/jwmg.21665
González Carman, V., Bruno, I., Maxwell, S., Álvarez, K., Albareda, D., Acha, E. M., et al. (2016). Habitat use, site fidelity and conservation opportunities for juvenile loggerhead sea turtles in the Río de la Plata. Argentina. Mar. Biol. 163, 1–13. doi: 10.1007/s00227-015-2795-5
Goshe, L. R., Avens, L., Scharf, F. S., and Southwood, A. L. (2010). Estimation of age at maturation and growth of Atlantic green turtles (Chelonia mydas) using skeletochronology. Mar. Biol. 157, 1725–1740. doi: 10.1007/s00227-010-1446-0
Gredzens, C., and Shaver, D. J. (2020). Satellite Tracking Can Inform Population-Level Dispersal to Foraging Grounds of Post-nesting Kemp’s Ridley Sea Turtles. Front. Mar. Sci. 7:1–15. doi: 10.3389/fmars.2020.00559
Hart, K., and Fujisaki, I. (2010). Satellite tracking reveals habitat use by juvenile green sea turtles Chelonia mydas in the Everglades. Florida, USA. Endanger. Species Res. 11, 221–232. doi: 10.3354/esr00284
Hart, K. M., Iverson, A. R., Fujisaki, I., Lamont, M. M., Bucklin, D., and Shaver, D. J. (2018a). Marine threats overlap key foraging habitat for two imperiled sea turtle species in the Gulf of Mexico. Front. Mar. Sci. 5:1–9. doi: 10.3389/fmars.2018.00336
Hart, K. M., Iverson, A. R., Fujisaki, I., Lamont, M. M., Bucklin, D., and Shaver, D. J. (2018b). Sympatry or syntopy? Investigating drivers of distribution and co-occurrence for two imperiled sea turtle species in Gulf of Mexico neritic waters. Ecol. Evol. 8, 12656–12669. doi: 10.1002/ece3.4691
Hart, K. M., Lamont, M. M., Sartain, A. R., and Fujisaki, I. (2014). Migration, foraging, and residency patterns for northern gulf loggerheads: Implications of local threats and international movements. PLoS One 9:103453. doi: 10.1371/journal.pone.0103453
Hatase, H., Omuta, K., and Tsukamoto, K. (2013). A mechanism that maintains alternative life histories in a loggerhead sea turtle population. Ecology 94, 2583–2594. doi: 10.1890/12-1588.1
Hawkes, L. A., Broderick, A. C., Coyne, M. S., Godfrey, M. H., and Godley, B. J. (2007). Only some like it hot - Quantifying the environmental niche of the loggerhead sea turtle. Divers. Distrib. 13, 447–457. doi: 10.1111/j.1472-4642.2007.00354.x
Hays, G. C., Fossette, S., Katselidis, K. A., Mariani, P., and Schofield, G. (2010). Ontogenetic development of migration: Lagrangian drift trajectories suggest a new paradigm for sea turtles. J. R. Soc. Interface 7, 1319–1327. doi: 10.1098/rsif.2010.0009
Hays, G. C., Houghton, J. D. R., and Myers, A. E. (2004). Pan-Atlantic leatherback turtle movements. Nature 429, 522. doi: 10.1038/429522a
Hovel, K. A., and Lipcius, R. N. (2001). Habitat fragmentation in a seagrass landscape: Patch size and complexity control blue crab survival. Ecology 82, 1814–1829. doi: 10.1890/0012-96582001082[1814:HFIASL]2.0.CO;2
Keller, J. M., Kucklick, J. R., Stamper, M. A., Harms, C. A., and McClellan-Green, P. D. (2004). Associations between organochlorine contaminant concentrations and clinical health parameters in loggerhead sea turtles from North Carolina, USA. Environ. Health Perspect. 112, 1074–1079. doi: 10.1289/ehp.6923
Kubis, S., Chaloupka, M., Ehrhart, L., and Bresette, M. (2009). Growth rates of juvenile green turtles Chelonia mydas from three ecologically distinct foraging habitats along the east central coast of Florida. USA. Mar. Ecol. Prog. Ser. 389, 257–269. doi: 10.3354/meps08206
Labrada-Martagón, V., Méndez-Rodríguez, L. C., Gardner, S. C., Cruz-Escalona, V. H., and Zenteno-Saívn, T. (2010). Health indices of the green turtle (Chelonia mydas) along the pacific Coast of baja California Sur, Mexico. II. body condition index. Chelonian Conserv. Biol. 9, 173–183. doi: 10.2744/CCB-0807.1
Lamont, M. M., and Iverson, A. R. (2018). Shared habitat use by juveniles of three sea turtle species. Mar. Ecol. Prog. Ser. 606, 187–200. doi: 10.3354/meps12748
Lamont, M. M., Putman, N. F., Fujisaki, I., and Hart, K. M. (2015). Spatial requirements of different life-stages of the loggerhead turtle (Caretta caretta) from a distinct population segment in the northern Gulf of Mexico. Herpetol. Conserv. Biol. 10, 26–43.
Layman, C. A., Quattrochi, J. P., Peyer, C. M., and Allgeier, J. E. (2007). Niche width collapse in a resilient top predator following ecosystem fragmentation. Ecol. Lett. 10, 937–944. doi: 10.1111/j.1461-0248.2007.01087.x
McClellan, C. M., and Read, A. J. (2010). Confronting the gauntlet: Understanding incidental capture of green turtles through fine-scale movement studies. Endanger. Species Res. 10, 165–179. doi: 10.3354/esr00199
McDaniel, C. J., Crowder, L. B., and Priddy, J. A. (2000). Spatial dynamics of sea turtle abundance and shrimping intensity in the U.S. Gulf of Mexico. Ecol. Soc. 4, 1–16. doi: 10.5751/es-00181-040115
McMichael, E., Seminoff, J., and Carthy, R. (2008). Growth rates of wild green turtles, Chelonia mydas, at a temperate foraging habitat in the northern Gulf of Mexico: Assessing short-term effects of cold-stunning on growth. J. Nat. Hist. 42, 2793–2807. doi: 10.1080/00222930802357335
Metz, T. L., Gordon, M., Mokrech, M., and Guillen, G. (2020). Movements of Juvenile Green Turtles (Chelonia mydas) in the Nearshore Waters of the Northwestern Gulf of Mexico. Front. Mar. Sci. 7:1–17. doi: 10.3389/fmars.2020.00647
Millennium Ecosystem Assessment. (2013). Summary for decision makers. Washington, D.C: Island Press, doi: 10.5822/978-1-61091-484-0_1
Moyes, F., and Magurran, A. E. (2019). Change in the dominance structure of two marine-fish assemblages over three decades. J. Fish Biol. 94, 96–102. doi: 10.1111/jfb.13868
Muehlenbachs, L., Cohen, M. A., and Gerarden, T. (2013). The impact of water depth on safety and environmental performance in offshore oil and gas production. Energy Policy 55, 699–705. doi: 10.1016/j.enpol.2012.12.074
NMFS, and USFWS (2008). Recovery Plan for the Northwest Atlantic Population of the Loggerhead Sea Turtle (Second Revision). Silver Spring, MD: National marine fisheries service.
Palumbi, S. R., Evans, T. G., Pespeni, M. H., and Somero, G. N. (2019). Present and future adapation of marine species assemblages. Oceanography 32, 82–93.
Peig, J., and Green, A. J. (2009). New perspectives for estimating body condition from mass/length data: The scaled mass index as an alternative method. Oikos 118, 1883–1891. doi: 10.1111/j.1600-0706.2009.17643.x
Peig, J., and Green, A. J. (2010). The paradigm of body condition: A critical reappraisal of current methods based on mass and length. Funct. Ecol. 24, 1323–1332. doi: 10.1111/j.1365-2435.2010.01751.x
Perrault, J. R., Stacy, N. I., Lehner, A. F., Mott, C. R., Hirsch, S., Gorham, J. C., et al. (2017). Potential effects of brevetoxins and toxic elements on various health variables in Kemp’s ridley (Lepidochelys kempii) and green (Chelonia mydas) sea turtles after a red tide bloom event. Sci. Total Environ. 605–606, 967–979. doi: 10.1016/j.scitotenv.2017.06.149
Price, J. T., Paladino, F. V., Lamont, M. M., Witherington, B. E., Bates, S. T., and Soule, T. (2017). Characterization of the juvenile green turtle (Chelonia mydas) microbiome throughout an ontogenetic shift from pelagic to neritic habitats. PLoS One 12:1–13. doi: 10.1371/journal.pone.0177642
Putman, N. F., Seney, E. E., Verley, P., Shaver, D. J., López-Castro, M. C., Cook, M., et al. (2020). Predicted distributions and abundances of the sea turtle ‘lost years’ in the western North Atlantic Ocean. Ecography (Cop.). 43, 506–517. doi: 10.1111/ecog.04929
Ramirez, M. D., Avens, L., Goshe, L. R., Snover, M. L., Cook, M., Haas, H. L., et al. (2020). Regional environmental drivers of Kemp’s ridley sea turtle somatic growth variation. Mar. Biol. 167, 1–20. doi: 10.1007/s00227-020-03754-2
Rich, L. N., Miller, D. A. W., Robinson, H. S., McNutt, J. W., and Kelly, M. J. (2016). Using camera trapping and hierarchical occupancy modelling to evaluate the spatial ecology of an African mammal community. J. Appl. Ecol. 53, 1225–1235. doi: 10.1111/1365-2664.12650
Rielly-Carroll, E., and Freestone, A. L. (2017). Habitat fragmentation differentially affects trophic levels and alters behavior in a multi-trophic marine system. Oecologia 183, 899–908. doi: 10.1007/s00442-016-3791-2
Rodriguez, A., and Heck, K. L. (2020). Green turtle herbivory and its effects on the warm, temperate seagrass meadows of St. Joseph Bay, Florida (USA). Mar. Ecol. Prog. Ser. 639, 37–51. doi: 10.3354/meps13285
Roslin, T., Várkonyi, G., Koponen, M., Vikberg, V., and Nieminen, M. (2014). Species-area relationships across four trophic levels - decreasing island size truncates food chains. Ecography 37, 443–453. doi: 10.1111/j.1600-0587.2013.00218.x
Schmid, J. R., and Tucker, A. D. (2018). Comparing Diets of Kemp’s Ridley Sea Turtles (Lepidochelys kempii) in Mangrove Estuaries of Southwest Florida. J. Herpetol. 52, 252–258. doi: 10.1670/16-164
Sellas, A. B., Wells, R. S., and Rosel, P. E. (2005). Mitochondrial and nuclear DNA analyses reveal fine scale geographic structure in bottlenose dolphins (Tursiops truncatus) in the Gulf of Mexico. Conserv. Genet. 6, 715–728. doi: 10.1007/s10592-005-9031-7
Seminoff, J. A., Todd Jones, T., Resendiz, A., Nichols, W. J., and Chaloupka, M. Y. (2003). Monitoring green turtles (Chelonia mydas) at a coastal foraging area in Baja California, Mexico: Multiple indices to describe population status. J. Mar. Biol. Assoc. U K 83, 1355–1362. doi: 10.1017/S0025315403008816
Seney, E. E., and Landry, A. M. (2011). Movement patterns of immature and adult female kemp’s ridley sea turtles in the northwestern gulf of mexico. Mar. Ecol. Prog. Ser. 440, 241–254. doi: 10.3354/meps09380
Shaver, D. J. (1991). Feeding Ecology of Wild and Head-Started Kemp’s Ridley Sea Turtles in South Texas Waters. J. Herpetol. 25, 327–334.
Shaver, D. J., Hart, K. M., Fujisaki, I., Rubio, C., Sartain-Iverson, A. R., Peña, J., et al. (2016). Migratory corridors of adult female Kemp’s ridley turtles in the Gulf of Mexico. Biol. Conserv. 194, 158–167. doi: 10.1016/j.biocon.2015.12.014
Siegwalt, F., Benhamou, S., Girondot, M., Jeantet, L., Martin, J., Bonola, M., et al. (2020). High fidelity of sea turtles to their foraging grounds revealed by satellite tracking and capture-mark-recapture: New insights for the establishment of key marine conservation areas. Biol. Conserv. 250:108742. doi: 10.1016/j.biocon.2020.108742
Stamper, M. A., Harms, C., Epperly, S. P., Braun-McNeill, J., Avens, L., and Stoskopf, M. K. (2005). Relationship between barnacle epibiotic load and hematologic parameters in loggerhead sea turtles (Caretta caretta), a comparison between migratory and residential animals in Pamlico Sound, North Carolina. J. Zoo Wildl. Med. 36, 635–641. doi: 10.1638/04-074.1
Stevenson, R. D., and Woods, W. A. (2017). Condition Indices for Conservation: New Uses for Evolving Tools. Oxford J. 46, 1169–1190. doi: 10.1093/icb/ic1052
Sutton, G., Pichegru, L., Botha, J. A., Kouzani, A. Z., Adams, S., Bost, C. A., et al. (2020). Multi-predator assemblages, dive type, bathymetry and sex influence foraging success and efficiency in African penguins. PeerJ. 2020, 1–26. doi: 10.7717/peerj.9380
Swarthout, R. F., Keller, J. M., Peden-Adams, M., Landry, A. M., Fair, P. A., and Kucklick, J. R. (2010). Organohalogen contaminants in blood of Kemp’s ridley (Lepidochelys kempii) and green sea turtles (Chelonia mydas) from the Gulf of Mexico. Chemosphere 78, 731–741. doi: 10.1016/j.chemosphere.2009.10.059
Wakefield, E. D., Phillips, R. A., Trathan, P. N., Arata, J., Gales, R., Huin, N., et al. (2011). Habitat preference, accessibility, and competition limit the global distribution of breeding Black-browed Albatrosses. Ecol. Monogr. 81, 141–167. doi: 10.1890/09-0763.1
Waycott, M., Duarte, C. M., Carruthers, T. J. B., Orth, R. J., Dennison, W. C., Olyarnik, S., et al. (2009). Accelerating loss of seagrasses across the globe threatens coastal ecosystems. Proc. Natl. Acad. Sci. U. S. A. 106, 12377–12381. doi: 10.1073/pnas.0905620106
Wildermann, N. E., Sasso, C. R., Stokes, L. W., Snodgrass, D., and Fuentes, M. M. P. B. (2019). Habitat use and behavior of multiple species of marine turtles at a foraging area in the Northeastern Gulf of Mexico. Front. Mar. Sci. 6:1–13. doi: 10.3389/fmars.2019.00155
Williams, N. C., Bjorndal, K. A., Lamont, M. M., and Carthy, R. R. (2013). Winter Diets of Immature Green Turtles (Chelonia mydas) on a Northern Feeding Ground: Integrating Stomach Contents and Stable Isotope Analyses. Estuar. Coasts 37, 986–994. doi: 10.1007/s12237-013-9741-x
Williams, S. J., Flocks, J., Jenkins, C., Khalil, S., and Moya, J. (2012). Offshore sediment character and sand resource assessment of the Northern Gulf of Mexico, Florida to Texas. J. Coast. Res. 60, 30–44. doi: 10.2112/SI
Witzell, W. N., and Schmid, J. R. (2004). Immature sea turtles in Gullivan Bay, Ten Thousand Islands, southwest Florida. Gulf Mex. Sci. 22, 54–61. doi: 10.18785/goms.2201.05
Keywords: multi-species, neritic, Gulf of Mexico, Kemp’s ridley, body condition index, sea turtle, fitness
Citation: Lamont MM and Johnson D (2021) Variation in Species Composition, Size and Fitness of Two Multi-Species Sea Turtle Assemblages Using Different Neritic Habitats. Front. Mar. Sci. 7:608740. doi: 10.3389/fmars.2020.608740
Received: 21 September 2020; Accepted: 10 December 2020;
Published: 08 January 2021.
Edited by:
Nathan Freeman Putman, LGL, United StatesReviewed by:
Morgan Jaime DuBois, University of West Florida, United StatesAllen Foley, Florida Fish and Wildlife Research Institute, United States
Michael Arendt, South Carolina Department of Natural Resources, United States
Copyright © 2021 Lamont and Johnson. This is an open-access article distributed under the terms of the Creative Commons Attribution License (CC BY). The use, distribution or reproduction in other forums is permitted, provided the original author(s) and the copyright owner(s) are credited and that the original publication in this journal is cited, in accordance with accepted academic practice. No use, distribution or reproduction is permitted which does not comply with these terms.
*Correspondence: Margaret M. Lamont, mlamont@usgs.gov
†ORCID: Margaret M. Lamont, orcid.org/0000-0001-7520-6669; Darren Johnson, orcid.org/0000-0002-0502-6045