- 1Hotchkiss Brain Institute, Alberta Children’s Hospital Research Institute, Calgary, AB, Canada
- 2Department of Neuroscience, University of Calgary, Calgary, AB, Canada
- 3Department of Cell Biology and Anatomy, University of Calgary, Calgary, AB, Canada
The incidence of depression among humans is growing worldwide, and so is the use of selective serotonin reuptake inhibitors (SSRIs), such as sertraline hydrochloride. Our fundamental understanding regarding the mechanisms by which these antidepressants function and their off-target synaptic effects remain poorly defined, owing to the complexity of the mammalian brain. As all brain functions rely on proper synaptic connections between neurons, we examined the effect of sertraline on synaptic transmission, short-term potentiation underlying synaptic plasticity and synapse formation using identified neurons from the mollusk Lymnaea stagnalis. Through direct electrophysiological recordings, made from soma-soma paired neurons, we demonstrate that whereas sertraline does not affect short-term potentiation, it reduces the efficacy of synaptic transmission at both established and newly formed cholinergic synapses between identified neurons. Furthermore, Lymnaea neurons cultured in the presence of sertraline exhibited a decreased incidence of synaptogenesis. Our study provides the first direct functional evidence that sertraline exerts non-specific effects—outside of its SSRI role—when examined at the resolution of single pre- and post-synaptic neurons.
Introduction
Depression affects 1 in 10 people worldwide and is strongly associated with other disease states, such as suicidal ideation (WHO, 2001, 2015). Several hypotheses explaining the basis of depression have been proposed, but no cohesive theory has withstood the passage of time. One of the most influential among them is the monoamine hypothesis (aan het Rot et al., 2009). This theory posits that the concentration of serotonin (5-hydroxytryptamine; 5-HT) and other monoamines is likely reduced in the synaptic cleft due either to their deficiency resulting from the loss of aminergic neurons, the transmitter synthesis, loss of synapses or the release mechanisms. It should then be possible to restore pre-depressive function by increasing the concentration of serotonin, specifically in the cleft. Various drugs have thus been formulated to do just this; the most prominent among them are noradrenaline reuptake inhibitors (NRIs, NERIs), monoamine oxidase inhibitors (MAOIs), tricyclic antidepressants (TCAs), and selective serotonin reuptake inhibitors (SSRIs); the latter being the primary choice in the clinic (Lewis et al., 2019).
SSRI use has become prevalent among the adolescent and adult populations, with 12.7% of Americans over the age of 12 reporting usage of such medication between 2011 and 2014 (Pratt et al., 2017). This reflects a 65% increase on the equivalent numbers 15 years ago, a trend which is expected to increase because of the COVID-19 pandemic and the impact of ensuing social isolation. Notwithstanding their extensive usage, SSRIs may still be ineffective at inducing remission, as highlighted in the 2006 Sequenced Treatment Alternatives to Relieve Depression (STAR∗D) randomized control trial (Sinyor et al., 2010). This trial saw 2,876 unipolar major depressive disorder (MDD) patients treated with the SSRI citalopram for up to 14 weeks. Of this cohort, 1,893 (66%) patients required a second level of treatment—either by augmenting or switching their treatment paradigm. Remission rates were similarly not significantly higher in the second level (Sinyor et al., 2010), highlighting the challenges and difficulties in treating depression—even with a multi-pronged approach.
Sertraline hydrochloride is the most prescribed SSRI in the United States (Moore and Mattison, 2017). It is thought to block the pre-synaptic serotonin reuptake transporter SCL6A4, thereby raising the probability of serotonin availability at the synaptic cleft (aan het Rot et al., 2009; Sangkuhl et al., 2009). Elevated synaptic 5-HT concentration also initially upregulates pre-synaptic 5-HT1A auto-receptors thus reducing serotonin release pre-synaptically (Artigas et al., 1996; Romero et al., 1996). This would counteract the effects of an SSRI, resulting in a further reduction of serotonin release by the pre-synaptic neuron. These pre-synaptic auto-receptors desensitize over time due to prolonged exposure to elevated 5-HT concentrations at the terminal, thus raising the probability for successful treatment with SSRI (Hervás, 2001; El Mansari et al., 2005). As patients with MDD have increased 5-HT1A autoreceptor density (Stockmeier et al., 1998; Parsey et al., 2006; Boldrini et al., 2008), the desensitization of these receptors due to chronic treatment with SSRIs may explain their antidepressant properties. It thus stands to reason that SSRIs likely exert their antidepressant effects across the serotonin system by invoking both pre- and post-synaptic receptors (Artigas, 2013).
The effects of sertraline on the inputs to the serotonergic system remain poorly defined (Artigas, 2013). Moreover, while some side effects of sertraline have been established, such as fatigue, anxiety, and sexual dysfunction (Ferguson, 2001; US Food and Drug Administration, 2008), its impact on non-serotonergic systems, such as cholinergic transmission that underlies learning, memory and plasticity remains unknown. An important question to ask is thus: could the non-target effects of sertraline underlie the interpersonal variability of its clinical effects (Marken and Munro, 2000)?
An impediment that has proven difficult to overcome in the mammalian brain is its extremely complex neuronal connectivity, deterring one’s ability to measure direct effects of sertraline exposure on single pre- and post-synaptic neurons. The mollusk Lymnaea stagnalis on the other hand, offers a wonderful opportunity as large, individually identifiable and functionally characterized neurons can be studied at the synaptic level at a resolution not approachable in most mammalian models (Feng et al., 1997). Previous studies using this model have tested another SSRI, fluoxetine, which was found to cause significant reductions in neurite outgrowth of serotonergic neurons as well as synaptic connectivity, including transmission efficacy and synaptogenesis, at a cholinergic synapse (Xu et al., 2010; Getz et al., 2011). It remained unknown whether these findings were unique to fluoxetine or if they extend to other SSRIs. In the present study, we examined the effects of sertraline at the level of single pre-and post-synaptic neurons that were paired in the soma-soma configuration (Feng et al., 1997). We hypothesized that: (1) the amplitudes of excitatory post-synaptic potentials (EPSPs) between cholinergic neuron visceral dorsal 4 (VD4) and its serotonergic post-synaptic partner left pedal dorsal 1 (LPeD1) would change when sertraline hydrochloride was added to neuronal cultures in both established and developed networks; (2) the post-tetanic potentiation (PTP), which forms the basis for short-term memory (Naruo et al., 2005; Luk et al., 2011), would be altered in the presence of sertraline; and (3) sertraline would affect synaptogenesis between the paired cells. This study provides the first direct evidence that, similar to the effects of another SSRI fluoxetine (Xu et al., 2010; Getz et al., 2011), sertraline hydrochloride exerts detrimental effects on both established and developing synapses. In contrast, we found that sertraline does not affect PTP at this synapse.
Materials and Methods
Animal Care
Lymnaea stagnalis freshwater snails were housed at room temperature (maintained at 18–20°C) in well-aerated freshwater tanks and fed a diet of romaine lettuce twice per week.
Cell Culture
Neurons were isolated from 2 to 3-month-old snails with shell lengths of 10–15 mm. The procedures for cell isolation and culture have been described in detail previously (Syed et al., 1990; Ridgway et al., 1991; Woodin et al., 1999). In brief, snails were deshelled and anesthetized in normal saline (51.3 mM NaCl, 1.7 mM KCl, 4.1 mM CaCl2, 1.5 mM MgCl2, 10.0 mM HEPES; pH 7.9) containing 20% Listerine (Original; McNeil Consumer Healthcare, Fort Washington, PA, United States). The animals were then dissected to remove the central ring ganglia (CRG). The isolated ganglia were treated with 2 mg/mL trypsin (T9201; Sigma-Aldrich, St. Louis, MO, United States) dissolved in defined media (DM) (Leibovitz’s L-15 Media; Life Technologies, Gaithersburg, MD, United States; special order) for 18–20 min followed by 2 mg/mL trypsin inhibitor (T9003; Sigma-Aldrich) in DM for 15 min. The outer and inner sheaths surrounding the ganglia were then removed using fine forceps. Fire-polished pipettes (1B150-6; World Precision Instruments, Sarasota, FL, United States) treated with Sigmacote® (SL2; Sigma-Aldrich) with a tip diameter of 50–60 μm were used to isolate the VD4 and LPeD1 neurons through gentle suction. The cells were plated on poly-L-lysine (P1274; Sigma-Aldrich)-coated dishes containing Lymnaea brain conditioned media (CM) in a soma-soma configuration, where the cell bodies were juxtaposed against each other, to facilitate reliable synaptogenesis between the two neurons in the absence of neurite outgrowth (Feng et al., 1997). VD4 and LPeD1 neurons were chosen as they form a cholinergic synapse, which has been well-established and utilized in previous studies (Xu et al., 2009; Getz et al., 2011; Luk et al., 2015).
CM was prepared by removing the CRG from 3–6-month-old snails with shell lengths of 20–30 mm. The CRG were washed in antibiotic saline (gentamycin, 150 μg/mL) prior to incubation in a Sigmacote®-treated glass dish containing DM (12 ganglia per 6.5 mL DM). The media was maintained in a humified incubator for 3 days before the ganglia were removed to make 1× CM. The ganglia were then washed again in antibiotic saline prior to incubation in fresh DM for 4 days to make 2× CM. The CRG were removed once again and rewashed in antibiotic saline prior to incubation in fresh DM for 6 days to make 3× CM. Once the CRG were removed, all CM was stored at −20°C until use. All neurons in this study were cultured in either 2× CM or 3× CM.
Electrophysiology
Intracellular recordings of electrical activity from VD4-LPeD1 soma-soma paired neurons were made as detailed previously by Woodin et al. (1999). Filamented glass microelectrodes with an internal diameter of 1.5 μm (TW150F-6; World Precision Instruments) and a total resistance of 30–80 MΩ were filled with a saturated solution of K2SO4. Narashige micromanipulators (MM202 and MM204; Narashige, Tokyo, Japan) controlling the microelectrodes were used to impale VD4 and LPeD1 neurons under an inverted microscope (Axiovert 200M; Carl Zeiss AG, Oberkochen, Germany). Synaptic connections were tested via an amplified electrical signal using a NeuroData amplifier (IR283A; Cygnus Technology Inc., South Port, NC, United States) to inject current between 30 and 40 pA into the pre-synaptic VD4 neuron. The injected current triggered action potentials in the VD4 cell, resulting in EPSPs in the LPeD1 neuron at a 1:1 ratio. The electrical responses were recorded using a digitizer (Digidata 1322A; MDS Inc., Toronto, ON, Canada) and recording software Axoscope 10.3 (MDS Inc.). EPSP amplitudes were also measured using Axoscope 10.3.
Drug Concentrations
The sertraline concentrations tested were 1.65 and 2.475 μg/mL. These concentrations were chosen as the amounts found in human cerebrospinal fluid (CSF) that correspond to the therapeutic concentrations in blood plasma of 0.10 and 0.15 μg/mL, respectively; sertraline levels in CSF are at least 16.5 times higher than those found in plasma (Wille et al., 2008; Rhein et al., 2016). Stock aliquots of 0.1 mg/mL sertraline were prepared by dissolving sertraline hydrochloride (S6319; Sigma-Aldrich) in DM and 0.005% DMSO. The final %v/v DMSO concentration in culture was less than 0.0002%.
Synaptic Transmission Experiments at Established Synapses
VD4-LPeD1 soma-soma paired neurons were cultured overnight (17–20 h) in CM alone. Action potentials were elicited in the pre-synaptic VD4 neuron by injecting current of 30–40 pA, which resulted in 1:1 EPSPs in the post-synaptic LPeD1 neuron. The average of five EPSP amplitudes per VD4/LPeD1 pair was taken to measure the baseline strength of the synaptic connection. Then, 5 mL sertraline solution at concentrations of 1.65 or 2.475 μg/mL was perfused over the cultures at a rate of 2 mL/min using a peristaltic pump (Minipuls 2; Gilson, Middleton, WI, United States). After a 10-min waiting period to ensure thorough diffusion of the drug, the synaptic connection strength was measured following the above procedure. Following the recordings, sertraline was washed out through DM perfusion for 10 min at a rate of 2 mL/min using a peristaltic pump to determine whether the effects of sertraline on synaptic transmission were reversible. The synaptic connection strength was remeasured post-washout.
Synaptic Plasticity Experiments
VD4-LPeD1 soma-soma paired neurons were cultured overnight (17–20 h) in CM alone. Using electrophysiological techniques, an action potential elicited in the pre-synaptic VD4 neuron produced an EPSP in the post-synaptic LPeD1 neuron at a 1:1 ratio. A tetanic burst, consisting of high-frequency stimulations (generating 7–12 action potentials), was then induced though current injection for approximately 3 s. Following the tetanic stimulation, an induced action potential in the VD4 neuron elicited a post-tetanic EPSP (pEPSP) in the LPeD1 neuron with augmented EPSPs and compound PSPs. A post-tetanic action resulted in enhanced EPSPs and this increase in the ratio of pEPSP/EPSP is known as post-tetanic potentiation (PTP)—a form of synaptic plasticity underlying short-term potentiation (Getz et al., 2011). This procedure was repeated following the exposure to 1.65 or 2.475 μg/mL sertraline and after drug washout. Drug additions and washout were performed in the same manner as the synaptic transmission experiments at established synapses.
Synaptogenesis and Synaptic Transmission Experiments at Developing Synapses
VD4-LPeD1 soma-soma paired neurons were cultured overnight (17–20 h) in CM containing 1.65 or 2.475 μg/mL sertraline or in CM alone as a control. Action potentials were then elicited in the pre-synaptic VD4 neuron using techniques described above, producing EPSPs in the post-synaptic LPeD1 neuron at a 1:1 ratio. The average of five EPSP amplitudes per trace was taken to measure the synaptic connection strength. The incidence of synapse formation was determined by the presence of EPSPs in the post-synaptic recordings (Getz et al., 2011). To determine whether sertraline-induced effects were reversible, the drug was then washed out through DM perfusion for 10 min at a rate of 2 mL/min followed by CM perfusion at the same rate using a peristaltic pump. CM was required as the formation of appropriate excitatory synapses is contingent upon the availability of Lymnaea trophic factors that are present in the CM (Woodin et al., 1999). The synaptic strength was then measured using the aforementioned technique 5 h after the washout period. The timepoint of 5 h was chosen to ensure adequate time for synapses to form as synaptogenesis generally occurs within 4 h under these experimental conditions (Xu et al., 2009).
Statistical Analysis
Analyses for synaptic transmission at established synapses and synaptic plasticity experiments were performed using the REML mixed-effects model and Bonferroni correction. Analyses for the incidence of synapse formation utilized Fisher’s exact test. For experiments testing synaptic transmission at developing synapses, REML mixed-effects model and Bonferroni correction were employed to analyze comparisons between drug-exposed and post-washout conditions for the two sertraline concentrations, while Welch ANOVA and Dunnett’s T3 test were used to analyze comparisons between independent samples. Statistical analyses were performed and graphs generated using GraphPad Prism 8.0.1 (GraphPad Software Inc., San Diego, CA, United States). Test statistics were considered significant at a corresponding p-value less than the significance cut-off of p = 0.05. Values on the graphs represent the mean ± standard error of the mean (SEM). The n for all experiments refers to the number of biological replicates (VD4/LPeD1 pairs) under each condition.
Results
Sertraline Reduced the Efficacy of Synaptic Transmission at Established Synapses
VD4 and LPeD1, which form a well-characterized and one-way cholinergic synapse, were cultured in a soma-soma configuration (Figure 1A) overnight (17–20 h) in CM to facilitate reliable synapse formation (Xu et al., 2010). The average of five EPSPs, measured by sharp-electrode recordings in LPeD1, in response to current stimulation of VD4 was compared across three conditions: (1) prior to drug exposure; (2) following drug exposure; and (3) after drug washout (Figure 1B). Sertraline significantly reduced the amplitude of the induced EPSPs in LPeD1 [F(1.355,29.81) = 9.217; p = 0.002]. Specifically, after exposure of neurons to 1.65 μg/mL sertraline (n = 17), the amplitude of the observed EPSPs (4.31 ± 0.73 mV) was significantly lower than prior to drug exposure (n = 17; EPSPs = 7.91 ± 1.44 mV; t16 = 3.699; p = 0.006) (Figure 1C), indicating that the efficacy of transmission at established synapses was affected by 1.65 μg/mL sertraline. Similarly, exposure of neurons to 2.475 μg/mL sertraline (n = 12) resulted in EPSPs (3.81 ± 0.81 mV) with amplitudes that were significantly reduced compared to pre-exposure (n = 12; EPSPs = 6.45 ± 0.75 mV; t11 = 3.920; p = 0.007) (Figure 1C), demonstrating that 2.475 μg/mL sertraline treatment also impacted the efficacy of transmission at established synapses. These effects did not differ significantly between the tested sertraline concentrations [F(1,27) = 0.247; p = 0.623], demonstrating that both doses used depressed synaptic transmission independent of the concentrations used (Figures 1B,C). The EPSPs amplitudes after treatment with 1.65 μg/mL sertraline and washout (n = 10; EPSPs = 4.81 ± 0.74 mV) did not recovered fully to their pre-treatment levels (t9 = 2.359; p = 0.128) nor were they significantly different from those data obtained during the drug exposure (t9 = 0.640; p > 0.999) (Figure 1C). Thus, the effects of sertraline did not completely return to their pre-treatment levels upon washout within the prescribed time window. Similarly, EPSP amplitude following washout of 2.475 μg/mL sertraline (n = 9; EPSPs = 5.56 ± 1.38 mV) was not significantly different from those prior to drug exposure (t8 = 0.597; p > 0.999) nor from those following drug exposure (t8 = 1.249; p = 0.741) (Figure 1C), indicating some ambiguity regarding the reversibility of the effects of sertraline. Additionally, we noted that the pre-synaptic cell VD4 exhibited clamping of its action potentials in the presence of the drug showing a direct effect of sertraline on its intrinsic membrane properties (Figure 1D). Taken together, these data demonstrate that when used in a clinical range, sertraline significantly reduces the amplitude of synaptic transmission between cholinergic neurons VD4-LPeD1, and that even the lower doses exhibit a sealing effect. Moreover, we found that within a prescribed washout period, the synaptic transmission did not fully recover upon washout with DM.
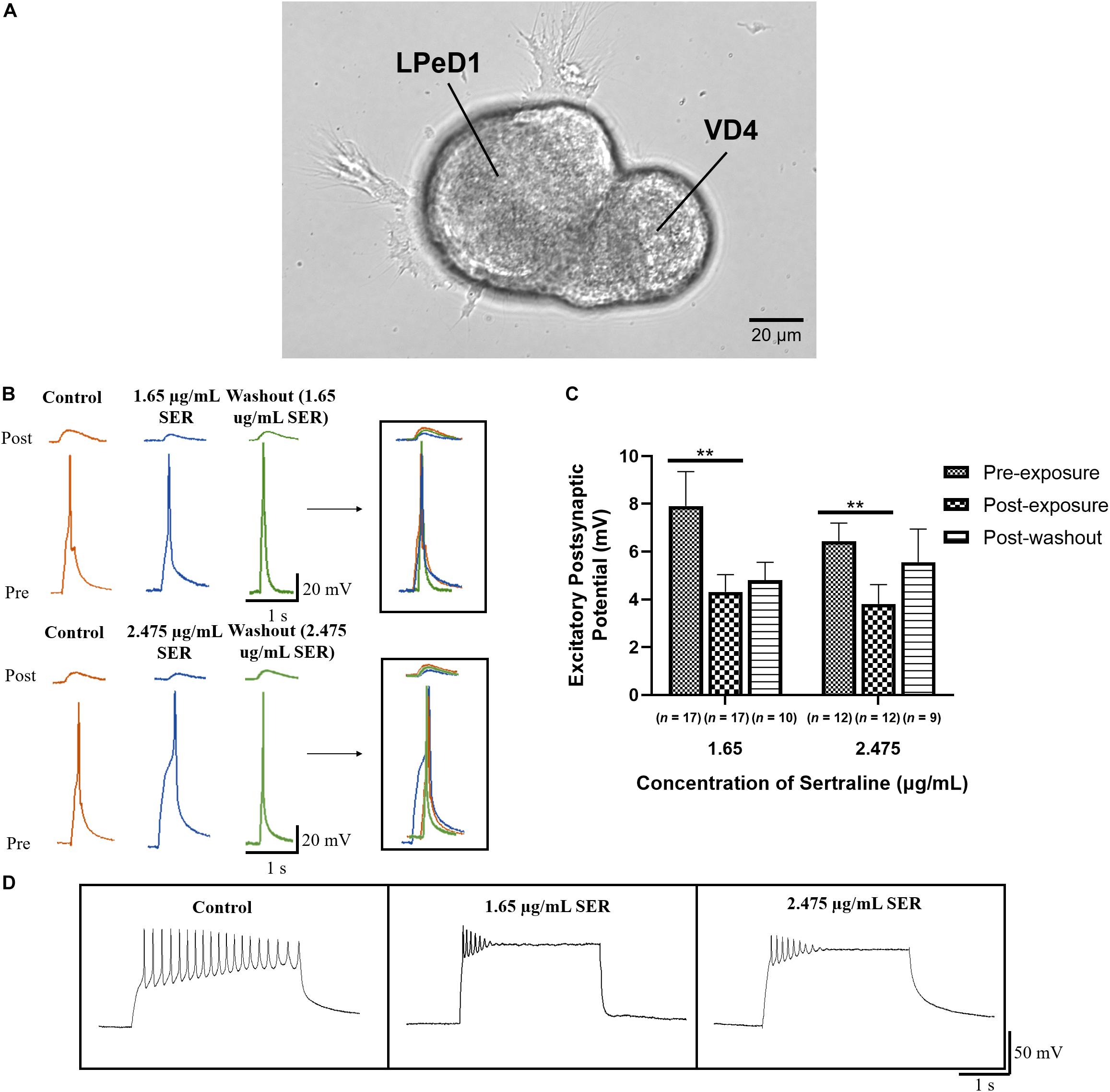
Figure 1. Sertraline reduced the efficacy of synaptic transmission at established cholinergic synapses. (A) Phase image of LPeD1 and VD4 neurons cultured in a soma-soma configuration. Scale bar = 20 μm. (B) Electrophysiological recordings of VD4 (pre) and LPeD1 (post) neurons of established synapses prior to drug exposure (control), following exposure to 1.65 or 2.475 μg/mL sertraline, and following drug washout. Induced action potentials in VD4 generated 1:1 EPSPs in LPeD1. (C) Both 1.65 and 2.475 μg/mL sertraline significantly reduced the amplitudes of the induced EPSP; the synaptic transmission did not return to its baseline levels upon washout. Error bars indicate SEM across at least nine biological replicates. Statistical significance was calculated using REML mixed-effects model and Bonferroni correction. **p < 0.01. (D) Both sertraline concentrations significantly affected pre-synaptic VD4 neuronal firing as demonstrated by the clamping of action potential during an induced burst. SER, sertraline.
Sertraline Did Not Affect Short-Term Synaptic Plasticity at Established Synapses
The increase in the ratio of pEPSP/EPSP known as PTP which is deemed a measure of short-term synaptic plasticity (Getz et al., 2011), was compared in the absence, presence, and following sertraline washout at concentrations of 1.65 or 2.475 μg/mL (Figure 2A). There was no significant difference in PTP after exposure to 1.65 μg/mL sertraline [n = 16; PTP = 2.03 ± 0.32; REML: F(1.266,27.23) = 2.901, p = 0.092; Bonferroni: t15 = 0.558, p > 0.999] nor following drug washout [n = 10; PTP = 1.90 ± 0.29; REML: F(1.266,27.23) = 2.901, p = 0.092; Bonferroni: t9 = 0.840, p > 0.999] compared to control (n = 17; PTP = 2.18 ± 0.22) (Figure 2B). Additionally, there was no significant difference compared to control (n = 12; PTP = 2.86 ± 0.40) after treatment with 2.475 μg/mL sertraline [n = 12; PTP = 2.29 ± 0.57; REML: F(1.266,27.23) = 2.901, p = 0.092; Bonferroni: t11 = 0.865, p > 0.999] nor after drug washout [n = 9; PTP = 1.89 ± 0.29; REML: F(1.266,27.23) = 2.901, p = 0.092; Bonferroni: t8 = 1.698, p = 0.384; Figure 2B]. The data suggest that exposure to sertraline did not affect short-term potentiation underlying synaptic plasticity at this established synapse.
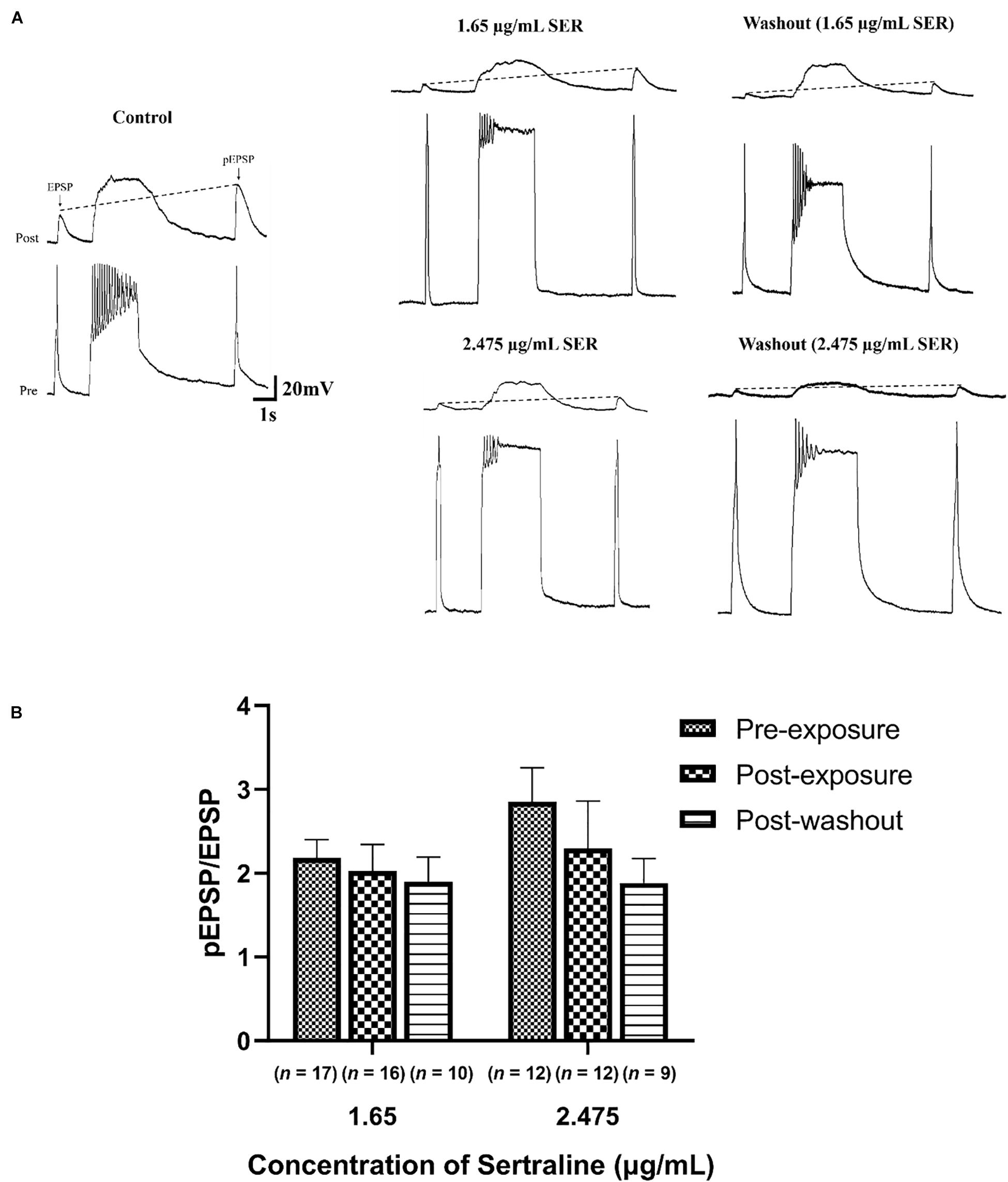
Figure 2. Sertraline did not impact short-term plasticity at established cholinergic synapses. (A) Representative traces of post-tetanic potentiation (PTP) under control conditions (CM only), following exposure to 1.65 or 2.475 μg/mL sertraline, and after drug washout. An action potential was elicited in VD4 neuron (pre), producing a 1:1 EPSP in the LPeD1 (post). The action potential induced following tetanic stimulation produced an EPSP with an increased amplitude (pEPSP), indicating potentiation of the signal. PTP was determined from an increase in the ratio of pEPSP/EPSP, represented by the dotted line. (B) Neither 1.65 nor 2.475 μg/mL significantly affected pEPSP/EPSP ratio following drug exposure or washout—notwithstanding the fact that both suppressed the pre-tetanic EPSP amplitude. Error bars indicate SEM across at least nine biological replicates. Statistical significance was calculated using REML mixed-effects model and Bonferroni correction.
Sertraline Exposure Reduced the Incidence of Synaptogenesis
Compared to control (n = 19), which had a 94.7% incidence of synapse formation, the incidence of synaptogenesis after exposure to 1.65 μg/mL sertraline (n = 17) was significantly lower at 52.9% (p = 0.006) (Figure 3A). Furthermore, the incidence of synapse formation after exposure to 2.475 μg/mL sertraline (n = 11) was also significantly reduced at 27.3% compared to control (p < 0.001) (Figure 3A). However, the incidence of synaptogenesis between sertraline concentrations was not significantly different (p = 0.253) (Figure 3A). Thus, exposure to sertraline decreased the incidence of synaptogenesis, but this reduction was dose independent. Following drug washout, the incidence of synapse formation for neurons exposed to both 1.65 μg/mL sertraline (n = 10; 80% formation) and 2.475 μg/mL sertraline (n = 8; 100% formation) increased to levels that were not significantly different from control (p = 0.267 and p > 0.999, respectively) (Figure 3B). These results indicate that, whereas sertraline blocked the formation of synapses between VD4 and LPeD1, these inhibitory effects were, however, reversible after the drug removal from the dishes.
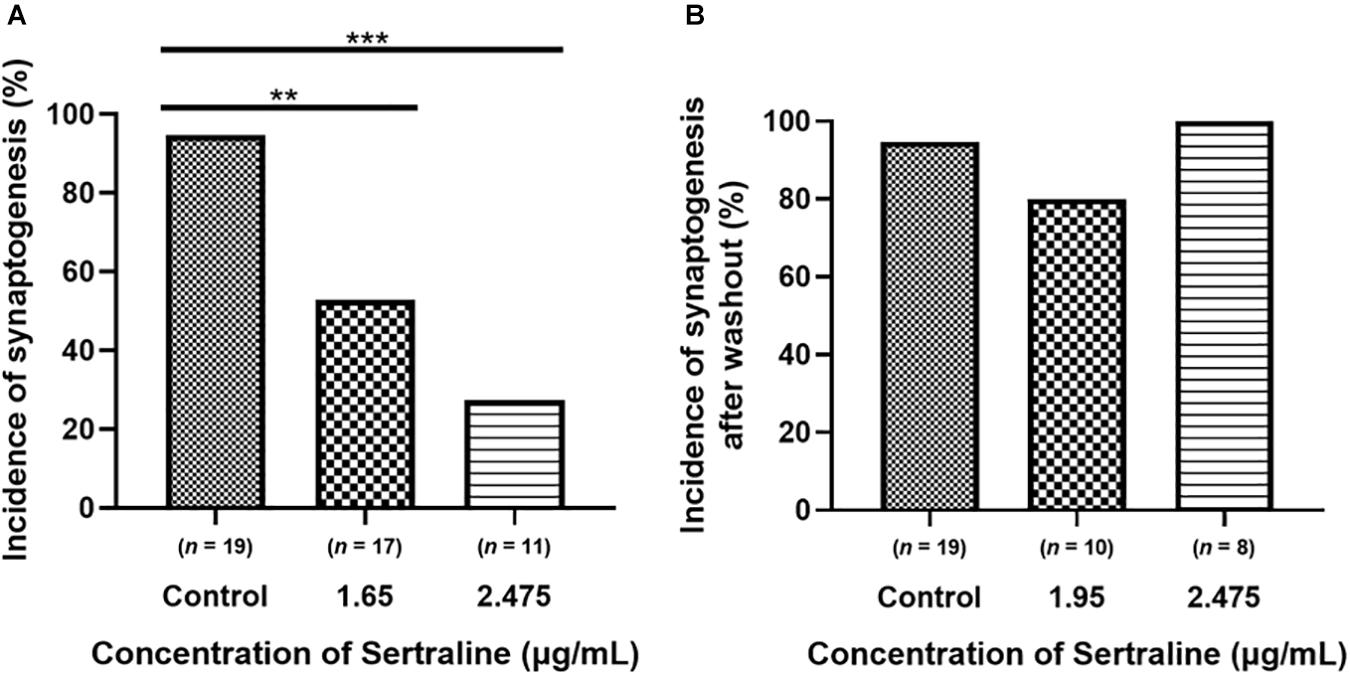
Figure 3. Sertraline significantly reduced the incidence of cholinergic synaptogenesis between VD4 and LPeD1. (A) Both 1.65 and 2.475 μg/mL sertraline significantly reduced the number of neurons establishing synapses across at least 11 biological replicates. (B) The incidence of synaptogenesis between cells exposed to 1.65 and 2.475 μg/mL sertraline returned to control levels 5 h following drug washout across at least eight biological replicates. Statistical significance was calculated using Fisher’s exact test. **p < 0.01, ***p < 0.001.
Sertraline Exposure Reduced the Efficacy of Transmission at Developing Synapses
Exposure to 1.65 μg/mL sertraline (n = 9) resulted in EPSPs (4.25 ± 0.98 mV) with significantly lower amplitudes than those measured in the control [n = 18; EPSPs = 8.18 ± 1.18 mV; Welch ANOVA: F(2,13.73) = 8.931, p = 0.003; Dunnett’s T3: t24.18 = 2.563, p = 0.033; Figure 4B]. These results demonstrate that 1.65 μg/mL sertraline treatment also reduced the efficacy of transmission at newly formed synapses. After drug washout, the amplitudes of EPSPs of neurons exposed to 1.65 μg/mL sertraline (n = 8; EPSPs = 6.00 ± 0.66 mV) were not significantly different from those found in the control [Welch ANOVA: F(2,19.26) = 4.321, p = 0.028; Dunnett’s T3: t23.67 = 1.614, p = 0.216] nor from those measured during sertraline exposure [REML: F(1,5) = 3.345, p = 0.127; Bonferroni: t5 = 1.749, p = 0.281; Figure 4B]; therefore, the reversibility of the effects of sertraline at this concentration were unclear. Exposure to 2.475 μg/mL (n = 3) significantly reduced the amplitudes of EPSPs (2.46 ± 0.62 mV) as well compared to control [Welch ANOVA: F(2,13.73) = 8.931, p = 0.003; Dunnett’s T3: t16.89 = 4.297, p = 0.001]. However, EPSP amplitudes post-washout (n = 8; EPSPs = 4.09 ± 0.77 mV) were significantly different from control [Welch ANOVA: F(2,19.26) = 4.321, p = 0.028; Dunnett’s T3: t23.99 = 2.899, p = 0.015] but not drug-exposed neurons [REML: F(1,5) = 3.345, p = 0.127; Bonferroni: t5 = 1.006, p = 0.722; Figure 4B], indicating that the reduction of transmission efficacy at this higher sertraline concentration may not either be reversible or will likely take much longer time in these newly formed synapses. There was however, no significant difference in the EPSP amplitudes between the two concentrations [REML: F(1,19) = 3.382, p = 0.082; Figure 4B], suggesting that the effects of sertraline on the efficacy of synaptic transmission itself were not dose dependent. Additionally, clamping of action potential bursts occurred in the pre-synaptic VD4 neuron at both concentrations, indicating perturbation of its intrinsic membrane properties (Figure 4C). Thus, the results suggest that sertraline decreased the efficacy of synaptic transmission at newly formed synapses and that they are more susceptible to drug washout—especially when the drug was used in the higher dose range.
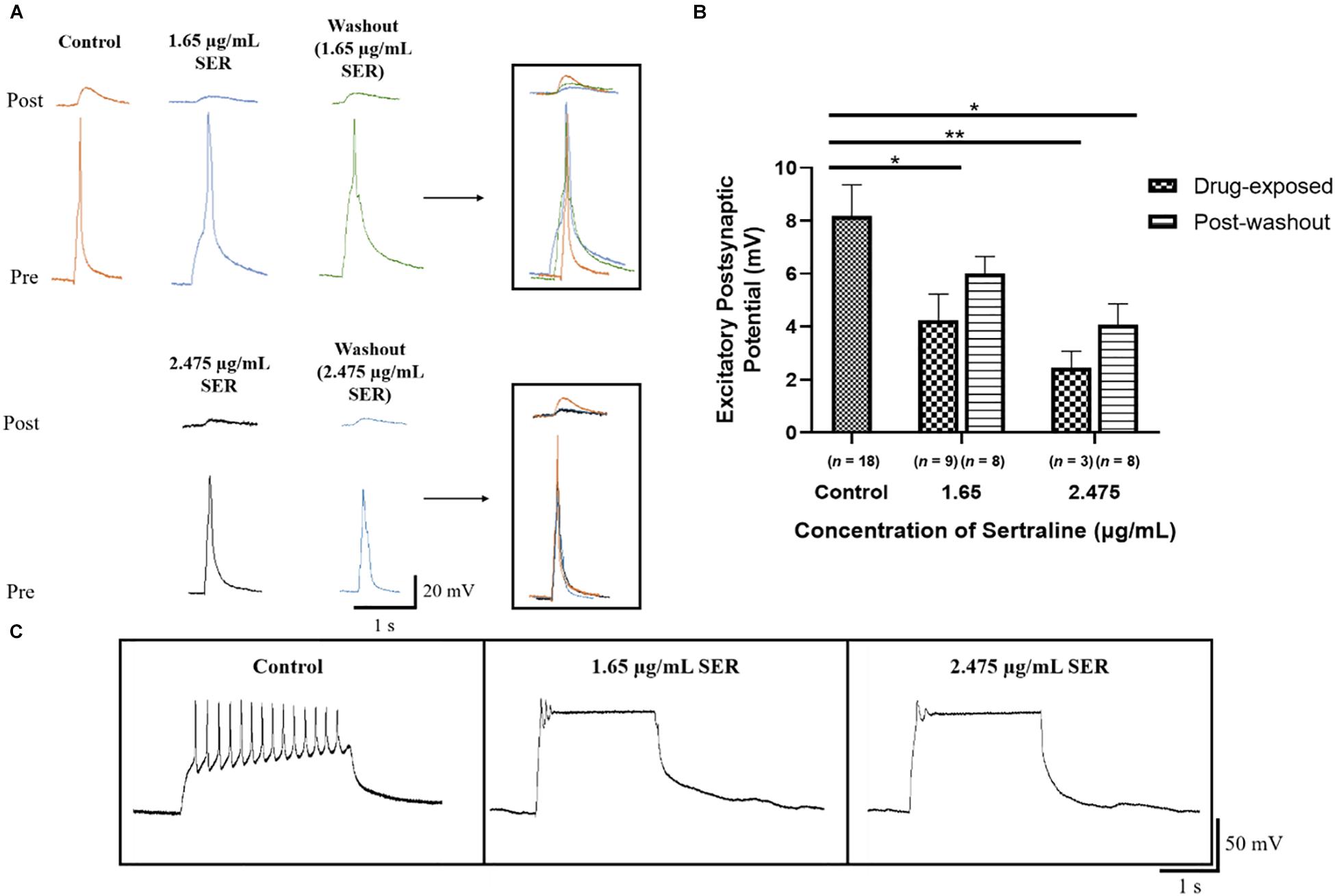
Figure 4. Sertraline affected the efficacy of synaptic transmission at developing cholinergic synapses. (A) Electrophysiological recordings of VD4 (pre) and LPeD1 (post) neurons of newly formed synapses cultured in CM only (control) or in CM containing 1.65 or 2.475 μg/mL sertraline and 5 h following the initial drug washout. Induced action potentials generated 1:1 EPSPs at the VD4-LPeD1 synapse that were simultaneously recorded intracellularly. (B) Both 1.65 and 2.475 μg/mL sertraline significantly reduced the EPSP amplitudes; greatest reduction seen in neurons exposed to 2.475 μg/mL sertraline which failed to recover upon washout. Error bars indicate SEM across at least three biological replicates. Statistical significance was calculated using Welch’s ANOVA and Dunnett’s T3 test for independent samples and REML mixed-effects model and Bonferroni correction for samples with repeated exposures. *p < 0.05, **p < 0.01. (C) Both sertraline concentrations visibly affected VD4 firing as demonstrated by clamping of action potential bursting during a burst induction. SER, sertraline.
Discussion
From fundamental ion channels underlying neuronal excitability to neurotransmitters, all modes of synaptic transmission are highly conserved in both vertebrate and invertebrate neurons, and it therefore stands to reason that the conclusions drawn from this study may also be applicable to most clinical situations. The rationale for using the soma-soma synapse model was that synapse formation—independent of the effects of sertraline on neurite outgrowth, could be investigated more precisely. It is important to note that both morphologically and electrophysiologically, the soma-soma synapses between VD4 and LPeD1 are similar to those seen both in vivo and in vitro (neurite-neurite) (Feng et al., 1997).
This study presented the first functional evidence that sertraline reduces the efficacy of synaptic transmission at both established (overnight cultures) and newly formed (5 h) synapses and decreases the incidence of synapse formation between a non-serotonergic neuron and a serotonergic target cell. These data thus demonstrate that sertraline-induced effects that were independent of its function as an SSRI at the synaptic level. Our data also demonstrates that sertraline reaches a ceiling effect at a lower concentration thus underscoring the importance that any higher doses in non-responsive patients may be futile as they may cause greater harm. Specifically, the data presented in Figure 4 shows that when newly formed synapses are exposed to a higher concentration of sertraline that the recovery of synaptic transmission either does not or only occurs partially.
On the brighter side, sertraline was found not to impact short-term potentiation underlying synaptic plasticity, which would suggest that this drug may not affect PTP underlying working memory. The auxiliary effects of sertraline on synaptic connectivity contrast those of the SSRIs fluoxetine and citalopram on Lymnaea neurons (Getz et al., 2011). Fluoxetine has been shown to diminish the efficacy of transmission at established and newly formed synapses, perturb short-term plasticity, and lower the incidence of synaptogenesis, while citalopram disrupted only synaptic transmission efficacy between established synapses. Thus, our results corroborate with the idea that each SSRI has unique non-specific effects on synaptic processes that are independent of their overarching effect on the serotonin system. We attribute these differential effects—even when the experiments were conducted on the same neurons and under identical experimental conditions—to their respective selectivity. Among these SSRI, citalopram is the most selective SSRI with the fewest effects on synaptic connectivity, followed by sertraline and then fluoxetine as the least selective and the most disruptive to synaptic processes (Hyttel, 1994; Getz et al., 2011). As such, we encourage exploration of other SSRIs in similar experiments.
Whereas the present study was designed to test the off-target effects of sertraline on a non-serotonergic system, one could argue that the suppression of synaptic transmission observed here in the presence of sertraline may still have involved LPeD1 which is known to be serotonergic. Specifically, we had previously demonstrated that when VD4 is paired concurrently (soma-soma-soma tripartite synapses) with its aminergic partners LPeD1 (serotonin) and RPeD1 (dopamine) that the stimulation of LPeD1 (or the exogenous application of serotonin) significantly depresses synaptic transmission between VD4 (cholinergic) and its dopaminergic partner. The induced release of serotonin from LPeD1via direct current injection—either prior to or during VD4 stimulation—was found to reduce inward calcium currents in VD4 through a G-protein, cAMP-dependent pathway (McCamphill et al., 2008). Reduction of inward calcium current by serotonin released non-synaptically, from LPeD1 at the VD4-LPeD1-RPeD1 contact sites was attributed to the suppression of acetylcholine release from VD4, thus resulting in synaptic depression. This possibility is, however, highly unlikely in the present study. Specifically, in this study we only used two neurons VD4-LPeD1 which do not form a reciprocal synapse; it is only a one-way cholinergic synapse from VD4 to LPeD1. Moreover, we ensured throughout the experiment that LPeD1 was prevented from triggering action potentials by keeping its membrane potential held below the spiking threshold via direct hyperpolarizing current injection, and as such, the EPSP amplitude also remained subthreshold. This approach ensured that no non-synaptic transmitter release occurred from LPeD1. Thus, our data rule out the possibility that the suppression of synaptic transmission between VD4 and LPeD1 may have still involved serotonin release from the post-synaptic cell—albeit non-synaptically (McCamphill et al., 2008). Our data thus strongly suggest that the observed effects of sertraline were indeed off-target (i.e., not the intended function) effects that involved cholinergic synaptic transmission between VD4 and LPeD1.
While our data clearly show that sertraline affects normal synaptic function, the potential site for this action was not identified in the present study. The clamping of the pre-synaptic burst in the presence of sertraline would, however, indicate a direct effect either on the pre-synaptic membrane conductance or its intrinsic membrane properties. In rats, sertraline has been shown to inhibit L-type calcium currents in myocytes and prevent Ca2+ levels from rising when induced by Ca2+ channel activator 4-aminopyridine in hippocampal nerve endings (Lee et al., 2012; Sitges et al., 2016). Clear evidence has been established regarding the crucial involvement of Ca2+ at both pre-synaptic and post-synaptic neurons for synaptogenesis to occur in Lymnaea (Feng et al., 2002; Spafford et al., 2003; Xu et al., 2009). At the pre-synaptic neuron, Feng et al. (2002) reported Ca2+ gradients that were both target cell and synaptic site specific. Furthermore, increases in Ca2+ influx were observed only at sites where synaptic contact had been established compared to non-contact sites, indicating that Ca2+ plays an important role in cell-cell recognition and synapse formation (Feng et al., 2002). Moreover, Xu et al. (2009) demonstrated that oscillating Ca2+ signals are necessary for post-synaptic nicotinic acetylcholine receptor (nAChR) expression required for cholinergic synaptogenesis and synaptic transmission. Thus, sertraline may either have blocked or perturbed Ca2+ channels at both or either the pre-synaptic or post-synaptic neurons, resulting in the reduced incidence of synaptogenesis and efficacy of transmission. The clamping of action potential bursts in the pre-synaptic VD4 neurons exposed to sertraline resembled those treated with nifedipine and tetraethylammonium ions, which are inhibitors of L-type Ca2+ channels and Ca2+-induced K+ channels, respectively (Spafford et al., 2006), suggesting that sertraline likely affected the pre-synaptic neuron.
Previous studies have shown that VD4 contains and releases more than one type of neurotransmitter. Specifically, it was found to contain FRMFamide-like peptide which is released at myriad of its targets (Saunders et al., 1992; Santama et al., 1995; Getz et al., 2020) where it acts through G-protein coupled receptors. It is important to note that in instances where VD4 invokes a peptide transmitter for its actions, it often requires a regenerative burst and that the post-synaptic response occurs after a considerable latency. In contrast, we have demonstrated that the excitatory synaptic transmission between VD4 and LPeD1 is purely cholinergic and completely blocked by curare and other acetylcholine receptor antagonists (Woodin et al., 2002). An in depth analysis of various transmitters released from VD4 and the underlying signaling that regulates its secretory machinery at both cholinergic and peptidergic synapses has recently been published by us in detail (Getz et al., 2020).
Sertraline has been shown previously to block pre-synaptic Na+ channels (Aldana and Sitges, 2012; Sitges et al., 2016) and several types of K+ channels (Ohno et al., 2007; Kobayashi et al., 2011; Lee et al., 2012, 2016), both of which are crucial in regulating depolarization and repolarization events underlying action potentials; this may be an alternative mechanism by which sertraline perturbs synaptic transmission. The present study does not, however, rule out the possibility that sertraline may have affected either pre-or post-synaptic currents, in addition to its effects on synaptic transmission; this would still need to be determined experimentally and may require patch clamp or Ca2+ imaging techniques.
While our study demonstrated reduced synaptogenesis as a result of sertraline exposure, others have suggested the SSRI may increase synapse formation—albeit demonstrated indirectly through elevated levels of synaptic proteins in its presence (Seo et al., 2014). Specifically, Seo et al. (2014) showed that sertraline increases the expression of the pre-synaptic protein synaptophysin and post-synaptic proteins post-synaptic density protein 95 (PSD-95) and promote the release of brain-derived neurotrophic factor (BDNF) in rat hippocampal neurons, all of which play important roles in synaptic structures and function. However, it is important to note that these increases were only significant in a toxic B27-deprived environment, which is required for optimal growth and survival of rat hippocampal neurons. The only significant increase reported in this study was of the BDNF levels in an optimal growth environment and that too occurred at the highest sertraline concentration (1 μM) tested. It is nevertheless possible that sertraline may influence synaptogenic program; in our study, however, we found it to be synapse suppressive. These observations highlight the complexity of SSRIs such as sertraline in both their intended and unintended effects. Although we found that sertraline-induced effects on synaptic transmission and synapse formation were transient, they were not, however, completely reversible in the time window that we examined. The elucidation of the auxiliary effects of sertraline and other SSRIs is imperative considering their substantial and continued rise in usage and variable efficacy (Sinyor et al., 2010; Moore and Mattison, 2017; Pratt et al., 2017).
The data presented in the present study highlights the importance of testing compounds in various simple animal models first where not only the specific neurons but also their synapses are accessible for direct electrophysiological analysis before proceeding to clinical trials. The mechanisms underlying antidepressant action could, if fully understood, minimize the plethora of SSRIs that most depressive patients must take for effective treatment, as reported in the STAR∗D study (Sinyor et al., 2010) and elsewhere (Getz et al., 2012). The studies, such as the present paper, exploiting a simple aquatic organism could provide significant benefit—not only to drug companies developing better antidepressants with least side effects but also to clinicians and their patients.
Data Availability Statement
The raw data supporting the conclusions of this article will be made available by the authors, without undue reservation, upon request by any qualified researcher.
Ethics Statement
Ethical review and approval was not required for the animal study because the research utilized the lower invertebrate Lymnaea stagnalis. Thus, the experiments were conducted in accordance with the guidelines set by the Canadian Council on Animal Care.
Author Contributions
JC and NS conceptualized and designed the study. NS secured funding and supervised the study. WZ performed the cell culture. JC performed the experiments, data collection, and wrote the first draft of the manuscript. JC, AT, and FI performed the statistical analyses. AT and FI wrote sections of the manuscript. All authors reviewed and contributed revisions to the final manuscript.
Funding
This research was supported by the Canadian Institutes of Health Research (CIHR; #10015088). AT was supported by Mitacs through the Mitacs Research Training Award.
Conflict of Interest
The authors declare that the research was conducted in the absence of any commercial or financial relationships that could be construed as a potential conflict of interest.
References
aan het Rot, M., Mathew, S. J., and Charney, D. S. (2009). Neurobiological mechanisms in major depressive disorder. CMAJ 180, 305–313. doi: 10.1503/cmaj.080697
Aldana, B. I., and Sitges, M. (2012). Sertraline inhibits pre-synaptic Na(+) channel-mediated responses in hippocampus-isolated nerve endings. J. Neurochem. 121, 197–205. doi: 10.1111/j.1471-4159.2012.07674.x
Artigas, F. (2013). Serotonin receptors involved in antidepressant effects. Pharmacol. Ther. 137, 119–131. doi: 10.1016/j.pharmthera.2012.09.006
Artigas, F., Romero, L., de Montigny, C., and Blier, P. (1996). Acceleration of the effect of selected antidepressant drugs in major depression by 5-HT1A antagonists. Trends Neurosci. 19, 378–383. doi: 10.1016/S0166-2236(96)10037-0
Boldrini, M., Underwood, M. D., Mann, J. J., and Arango, V. (2008). Serotonin-1A autoreceptor binding in the dorsal raphe nucleus of depressed suicides. J. Psychiatr. Res. 42, 433–442. doi: 10.1016/j.jpsychires.2007.05.004
El Mansari, M., Sanchez, C., Chouvet, G., Renaud, B., and Haddjeri, N. (2005). Effects of acute and long-term administration of escitalopram and citalopram on serotonin neurotransmission: an in vivo electrophysiological study in rat brain. Neuropsychopharmacology 30, 1269–1277. doi: 10.1038/sj.npp.1300686
Feng, Z. P., Grigoriev, N., Munno, D., Lukowiak, K., MacVicar, B. A., Goldberg, J. I., et al. (2002). Development of Ca2+ hotspots between Lymnaea neurons during synaptogenesis. J. Physiol. 539(Pt 1), 53–65. doi: 10.1113/jphysiol.2001.013125
Feng, Z. P., Klumperman, J., Lukowiak, K., and Syed, N. I. (1997). In vitro synaptogenesis between the somata of identified Lymnaea neurons requires protein synthesis but not extrinsic growth factors or substrate adhesion molecules. J. Neurosci. 17, 7839–7849. doi: 10.1523/jneurosci.17-20-07839.1997
Ferguson, J. M. (2001). SSRI antidepressant medications: adverse effects and tolerability. Prim Care Companion J. Clin. Psychiatry 3, 22–27. doi: 10.4088/pcc.v03n0105
Getz, A., Xu, F., and Syed, N. (2012). “Antidepressant pharmacotherapy – do the benefits outweigh the risks?,” in Mental Illnesses – Evaluation, Treatments and Implications, ed. L. L’Abate (London: IntechOpen), 331–354.
Getz, A., Xu, F., Zaidi, W., and Syed, N. I. (2011). The antidepressant fluoxetine but not citalopram suppresses synapse formation and synaptic transmission between Lymnaea neurons by perturbing presynaptic and postsynaptic machinery. Eur. J. Neurosci. 34, 221–234. doi: 10.1111/j.1460-9568.2011.07757.x
Getz, A. M., Janes, T. A., Visser, F., Zaidi, W., and Syed, N. I. (2020). Neurotrophic factors and target-specific retrograde signaling interactions define the specificity of classical and neuropeptide cotransmitter release at identified Lymnaea synapses. Sci. Rep. 10, 13526. doi: 10.1038/s41598-020-70322-5
Hervás, I. (2001). Desensitization of 5-HT1A autoreceptors by a low chronic fluoxetine dose effect of the concurrent administration of WAY-100635. Neuropsychopharmacology 24, 11–20. doi: 10.1016/s0893-133x(00)00175-5
Hyttel, J. (1994). Pharmacological characterization of selective serotonin reuptake inhibitors (SSRIs). Int. Clin. Psychopharmacol. 9(Suppl. 1), 19–26. doi: 10.1097/00004850-199403001-00004
Kobayashi, T., Washiyama, K., and Ikeda, K. (2011). Inhibition of G protein-activated inwardly rectifying K+ channels by different classes of antidepressants. PLoS One 6:e28208. doi: 10.1371/journal.pone.0028208
Lee, H. A., Kim, K. S., Hyun, S. A., Park, S. G., and Kim, S. J. (2012). Wide spectrum of inhibitory effects of sertraline on cardiac ion channels. Korean J. Physiol. Pharmacol. 16, 327–332. doi: 10.4196/kjpp.2012.16.5.327
Lee, H. M., Hahn, S. J., and Choi, B. H. (2016). Blockade of Kv1.5 channels by the antidepressant drug sertraline. Korean J. Physiol. Pharmacol. 20, 193–200. doi: 10.4196/kjpp.2016.20.2.193
Lewis, G., Duffy, L., Ades, A., Amos, R., Araya, R., Brabyn, S., et al. (2019). The clinical effectiveness of sertraline in primary care and the role of depression severity and duration (PANDA): a pragmatic, double-blind, placebo-controlled randomised trial. Lancet Psychiatry 6, 903–914. doi: 10.1016/S2215-0366(19)30366-9
Luk, C. C., Lee, A. J., Wijdenes, P., Zaidi, W., Leung, A., Wong, N. Y., et al. (2015). Trophic factor-induced activity ‘signature’ regulates the functional expression of postsynaptic excitatory acetylcholine receptors required for synaptogenesis. Sci. Rep. 5:9523. doi: 10.1038/srep09523
Luk, C. C., Naruo, H., Prince, D., Hassan, A., Doran, S. A., Goldberg, J. I., et al. (2011). A novel form of presynaptic CaMKII-dependent short-term potentiation between Lymnaea neurons. Eur. J. Neurosci. 34, 569–577. doi: 10.1111/j.1460-9568.2011.07784.x
Marken, P. A., and Munro, J. S. (2000). Selecting a selective serotonin reuptake inhibitor: clinically important distinguishing features. Prim Care Companion J. Clin. Psychiatry 2, 205–210. doi: 10.4088/pcc.v02n0602
McCamphill, P. K., Dunn, T. W., and Syed, N. I. (2008). Serotonin modulates transmitter release at central Lymnaea synapses through a G-protein-coupled and cAMP-mediated pathway. Eur. J. Neurosci. 27, 2033–2042. doi: 10.1111/j.1460-9568.2008.06180.x
Moore, T. J., and Mattison, D. R. (2017). Adult utilization of psychiatric drugs and differences by sex, age, and race. JAMA Intern. Med. 177, 274–275. doi: 10.1001/jamainternmed.2016.7507
Naruo, H., Onizuka, S., Prince, D., Takasaki, M., and Syed, N. I. (2005). Sevoflurane blocks cholinergic synaptic transmission postsynaptically but does not affect short-term potentiation. Anesthesiology 102, 920–928. doi: 10.1097/00000542-200505000-00010
Ohno, Y., Hibino, H., Lossin, C., Inanobe, A., and Kurachi, Y. (2007). Inhibition of astroglial Kir4.1 channels by selective serotonin reuptake inhibitors. Brain Res. 1178, 44–51. doi: 10.1016/j.brainres.2007.08.018
Parsey, R. V., Olvet, D. M., Oquendo, M. A., Huang, Y. Y., Ogden, R. T., and Mann, J. J. (2006). Higher 5-HT1A receptor binding potential during a major depressive episode predicts poor treatment response: preliminary data from a naturalistic study. Neuropsychopharmacology 31, 1745–1749. doi: 10.1038/sj.npp.1300992
Pratt, L. A., Brody, D. J., and Gu, Q. (2017). Antidepressant Use Among Persons Aged 12 and Over: United States, 2011-2014. Hyattsville, MD.
Rhein, J., Morawski, B. M., Hullsiek, K. H., Nabeta, H. W., Kiggundu, R., Tugume, L., et al. (2016). Efficacy of adjunctive sertraline for the treatment of HIV-associated cryptococcal meningitis: an open-label dose-ranging study. Lancet Infect. Dis. 16, 809–818. doi: 10.1016/S1473-3099(16)00074-8
Ridgway, R. L., Syed, N. I., Lukowiak, K., and Bulloch, A. G. (1991). Nerve growth factor (NGF) induces sprouting of specific neurons of the snail, Lymnaea stagnalis. J Neurobiol. 22, 377–390. doi: 10.1002/neu.480220406
Romero, L., Bel, N., Artigas, F., de Montigny, C., and Blier, P. (1996). Effect of pindolol on the function of pre- and postsynaptic 5-HT1A receptors: in vivo microdialysis and electrophysiological studies in the rat brain. Neuropsychopharmacology 15, 349–360. doi: 10.1016/0893-133X(95)00240-E
Sangkuhl, K., Klein, T. E., and Altman, R. B. (2009). Selective serotonin reuptake inhibitors pathway. Pharmacogenet. Genomics 19, 907–909. doi: 10.1097/FPC.0b013e32833132cb
Santama, N., Wheeler, C. H., Skingsley, D. R., Yeoman, M. S., Bright, K., Kaye, I., et al. (1995). Identification, distribution and physiological activity of three novel neuropeptides of Lymnaea: EFLRlamide and pQFYRlamide encoded by the FMRFamide gene, and a related peptide. Eur. J. Neurosci. 7, 234–246. doi: 10.1111/j.1460-9568.1995.tb01059.x
Saunders, S. E., Kellett, E., Bright, K., Benjamin, P. R., and Burke, J. F. (1992). Cell-specific alternative RNA splicing of an FMRFamide gene transcript in the brain. J. Neurosci. 12, 1033–1039. doi: 10.1523/JNEUROSCI.12-03-01033.1992
Seo, M. K., Lee, C. H., Cho, H. Y., Lee, J. G., Lee, B. J., Kim, J. E., et al. (2014). Effects of antidepressant drugs on synaptic protein levels and dendritic outgrowth in hippocampal neuronal cultures. Neuropharmacology 79, 222–233. doi: 10.1016/j.neuropharm.2013.11.019
Sinyor, M., Schaffer, A., and Levitt, A. (2010). The sequenced treatment alternatives to relieve depression (STAR∗D) trial: a review. Can. J. Psychiatry 55, 126–135. doi: 10.1177/070674371005500303
Sitges, M., Chiu, L. M., and Reed, R. C. (2016). effects of levetiracetam, carbamazepine, phenytoin, valproate, lamotrigine, oxcarbazepine, topiramate, vinpocetine and sertraline on presynaptic hippocampal Na(+) and Ca(2+) channels permeability. Neurochem. Res. 41, 758–769. doi: 10.1007/s11064-015-1749-0
Spafford, J. D., Dunn, T., Smit, A. B., Syed, N. I., and Zamponi, G. W. (2006). In vitro characterization of L-type calcium channels and their contribution to firing behavior in invertebrate respiratory neurons. J. Neurophysiol. 95, 42–52. doi: 10.1152/jn.00658.2005
Spafford, J. D., Munno, D. W., Van Nierop, P., Feng, Z. P., Jarvis, S. E., Gallin, W. J., et al. (2003). Calcium channel structural determinants of synaptic transmission between identified invertebrate neurons. J. Biol. Chem. 278, 4258–4267. doi: 10.1074/jbc.M211076200
Stockmeier, C. A., Shapiro, L. A., Dilley, G. E., Kolli, T. N., Friedman, L., and Rajkowska, G. (1998). Increase in serotonin-1A autoreceptors in the midbrain of suicide victims with major depression-postmortem evidence for decreased serotonin activity. J. Neurosci. 18, 7394–7401. doi: 10.1523/jneurosci.18-18-07394.1998
Syed, N. I., Bulloch, A. G., and Lukowiak, K. (1990). In vitro reconstruction of the respiratory central pattern generator of the mollusk Lymnaea. Science 250:282. doi: 10.1126/science.2218532
US Food and Drug Administration. (2008). Zoloft (Sertraline HCl) Tablets/Oral Concentrate Medication Guide. Silver Spring, MD: US Food and Drug Administration.
WHO (2001). The World Health Report 2001: Mental Health: New Understanding, New Hope. Geneva: World Health Organization.
WHO (2015). Health in 2015: from MDGs, Millennium Development Goals to SDGs, Sustainable Development Goals. Geneva: World Health Organization.
Wille, S. M., Cooreman, S. G., Neels, H. M., and Lambert, W. E. (2008). Relevant issues in the monitoring and the toxicology of antidepressants. Crit. Rev. Clin. Lab. Sci. 45, 25–89. doi: 10.1080/10408360701713112
Woodin, M. A., Hamakawa, T., Takasaki, M., Lukowiak, K., and Syed, N. I. (1999). Trophic factor-induced plasticity of synaptic connections between identified Lymnaea neurons. Learn. Mem. 6, 307–316.
Woodin, M. A., Munno, D. W., and Syed, N. I. (2002). Trophic factor-induced excitatory synaptogenesis involves postsynaptic modulation of nicotinic acetylcholine receptors. J. Neurosci. 22, 505–514. doi: 10.1523/jneurosci.22-02-00505.2002
Xu, F., Hennessy, D. A., Lee, T. K., and Syed, N. I. (2009). Trophic factor-induced intracellular calcium oscillations are required for the expression of postsynaptic acetylcholine receptors during synapse formation between Lymnaea neurons. J. Neurosci. 29, 2167–2176. doi: 10.1523/JNEUROSCI.4682-08.2009
Xu, F., Luk, C., Richard, M. P., Zaidi, W., Farkas, S., Getz, A., et al. (2010). Antidepressant fluoxetine suppresses neuronal growth from both vertebrate and invertebrate neurons and perturbs synapse formation between Lymnaea neurons. Eur. J. Neurosci. 31, 994–1005. doi: 10.1111/j.1460-9568.2010.07129.x
Keywords: SSRI, antidepressant, Lymnaea stagnalis, synapse, synaptic transmission, synaptic plasticity, synaptogenesis
Citation: Chow J, Thompson AJ, Iqbal F, Zaidi W and Syed NI (2020) The Antidepressant Sertraline Reduces Synaptic Transmission Efficacy and Synaptogenesis Between Identified Lymnaea Neurons. Front. Mar. Sci. 7:603789. doi: 10.3389/fmars.2020.603789
Received: 07 September 2020; Accepted: 02 December 2020;
Published: 21 December 2020.
Edited by:
Silvia Franzellitti, University of Bologna, ItalyReviewed by:
Varvara Dyakonova, Koltzov Institute of Developmental Biology (RAS), RussiaAlexander V. Sidorov, Belarusian State University, Belarus
Copyright © 2020 Chow, Thompson, Iqbal, Zaidi and Syed. This is an open-access article distributed under the terms of the Creative Commons Attribution License (CC BY). The use, distribution or reproduction in other forums is permitted, provided the original author(s) and the copyright owner(s) are credited and that the original publication in this journal is cited, in accordance with accepted academic practice. No use, distribution or reproduction is permitted which does not comply with these terms.
*Correspondence: Naweed I. Syed, nisyed@ucalgary.ca
†These authors have contributed equally to this work