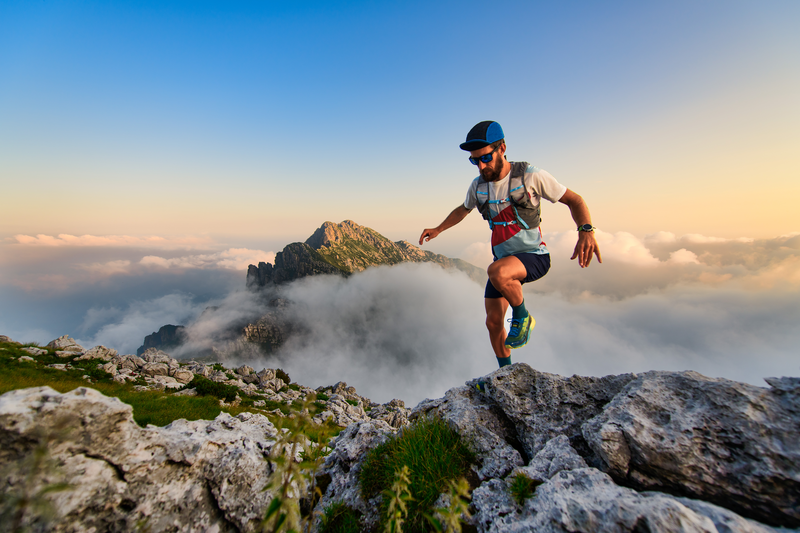
94% of researchers rate our articles as excellent or good
Learn more about the work of our research integrity team to safeguard the quality of each article we publish.
Find out more
ORIGINAL RESEARCH article
Front. Mar. Sci. , 13 November 2020
Sec. Marine Ecosystem Ecology
Volume 7 - 2020 | https://doi.org/10.3389/fmars.2020.603438
This article is part of the Research Topic Biogenic Reefs at Risk: Facing Globally Widespread Local Threats and Their Interaction with Climate Change View all 12 articles
Bottom-contact fisheries are unquestionably one of the main threats to the ecological integrity and functioning of deep-sea and circalittoral ecosystems, notably cold-water corals (CWC) and coral gardens. Lessons from the destructive impact of bottom trawling highlight the urgent need to understand how fisheries affect these vulnerable marine ecosystems. At the same time, the impact of other fishing gear and small-scale fisheries remains sparsely known despite anecdotal evidence suggesting their impact may be significant. This study aims to provide baseline information on coral bycatch by bottom-set gillnets used by artisanal fisheries in Sagres (Algarve, southwestern Portugal), thereby contributing to understand the impact of the activity but also the diversity and abundance of corals in this region. Coral bycatch frequency and species composition were quantified over two fishing seasons (summer-autumn and spring) for 42 days. The relationship with fishing effort was characterized according to métiers (n = 6). The results showed that 85% of the gillnet deployments caught corals. The maximum number of coral specimens per net was observed in a deployment targeting Lophius budegassa (n = 144). In total, 4,326 coral fragments and colonies of 22 different species were captured (fishing depth range of 57–510 m, mean 139 ± 8 m). The most affected species were Eunicella verrucosa (32%), Paramuricea grayi (29%), Dendrophyllia cornigera (12%), and Dendrophyllia ramea (6%). The variables found to significantly influence the amount of corals caught were the target species, net length, depth, and mesh size. The 22 species of corals caught as bycatch belong to Orders Alcyonacea (80%), Scleractinia (18%), Zoantharia (1%), and Antipatharia (1%), corresponding to around 13% of the coral species known for the Portuguese mainland coast. These results show that the impact of artisanal fisheries on circalittoral coral gardens and CWC is potentially greater than previously appreciated, which underscores the need for new conservation measures and alternative fishing practices. Measures such as closure of fishing areas, frequent monitoring onboard of fishing vessels, or the development of encounter protocols in national waters are a good course of action. This study highlights the rich coral gardens of Sagres and how artisanal fisheries can pose significant threat to corals habitats in certain areas.
The impact of human activities on marine life is a global crisis that has left virtually no area of the ocean unaffected, with benthic habitats like coral-dominated ecosystems among those most strongly impacted (Halpern et al., 2008). There are many stressors threatening the ecological integrity and functioning of coral ecosystems, including pollution (Ragnarsson et al., 2017; Consoli et al., 2020), overfishing (Hughes, 1994; Jackson, 2001), oil and gas extraction (Glover and Smith, 2003; Purser and Thomsen, 2012; Cordes et al., 2016), ocean acidification (Bramanti et al., 2013; Movilla et al., 2014; Albright et al., 2018), and global warming (Hughes et al., 2017, 2018). However, the direct impact of fisheries using bottom-contact gear remains the primary cause of habitat destruction and biomass removal (Hall–Spencer et al., 2002; Glover and Smith, 2003; Hourigan, 2009). This is of special concern for circalittoral and deep coral communities (i.e., those below 50 m depth, henceforth referred to as “deep”) such as coral gardens and cold-water corals (CWC), which have life-history traits (e.g., slow growth rates and late age at maturity) that make recovery from physical damage especially difficult, if even possible.
Coral gardens and CWC reefs are key ecosystems in the marine realm. The tridimensional complex species that build these habitats, known as engineers, create high structural complexity that provides shelter, feeding, and nursery grounds for many organisms, including many species of commercial value (Buhl-Mortensen et al., 2010; Ashford et al., 2019), supporting levels of biodiversity comparable to those found in tropical coral reefs and terrestrial forests (Rossi, 2013; Rossi et al., 2017). These habitats include coral species from several taxonomical groups (Orders Scleractinia, Zoantharia, Antipatharia, Corallimorpharia, Alcyonacea, and Pennatulacea), representing nearly 65% of all known coral species (Roberts, 2006; Cairns, 2007). In 2004, the United Nations General Assembly (UNGA) drew attention to the susceptibility of deep coral communities and other habitats to the impacts of deep-sea fisheries, designating them as vulnerable marine ecosystems (VMEs) that required urgent conservation and protection actions (UNGA, 2004; Fuller, 2008). As a result of several resolutions of the UNGA, Regional Fisheries Management Organizations (RFMO) and local governments adopted several measures to protect VMEs (UNGA, 2019), including the reduction of the frequency of significant adverse impacts by bottom-contact fisheries like trawling (e.g., encounter or “move-on” rule triggered by a bycatch threshold) (Parker et al., 2009; Aguilar et al., 2017; Davies et al., 2017) and the creation of Marine Protected Areas (MPA) in areas where VMEs occur (Armstrong and van den Hove, 2008; Huvenne et al., 2016).
Among the various types of gears used by deep-sea fisheries, bottom trawling is notorious for being the most destructive and has received increasing pressure for legislation banning its use worldwide. Indeed, in 2018 the European Parliament instituted a ban on trawling below 800 m depth in European waters (Clark et al., 2016; European Parliament and the Council of the European Union, 2016; Victorero et al., 2018). Other fishing techniques used in the deep-sea, such as longline, have been shown to have a much smaller impact on coral communities (Pham et al., 2015). However, some studies suggest that the extensive use and often considerable long configuration of this gear may also pose a threat to complex deep-sea benthic habitats, including coral communities. For instance, Mytilineou et al. (2014) have found that during experimental longline fishing in the Ionian Sea, 72% of the longline sets used in hake and blackspot seabream fisheries captured corals. In the Azores, Sampaio et al. (2012) reported that 15.2% (n = 45 out of 297) of the fishing trips of the longline fleet surveyed landed corals, with at least 205 specimens caught.
Although there are several studies on the impact of fishing gear on deep-sea ecosystems, most studies focused on large scale industrial fisheries, which represent a very small fraction of the fishing work force (Shester and Micheli, 2011). Worldwide, artisanal fisheries employ over 20 million workers, both directly and indirectly through processing, marketing, and distributing (McGoodwin, 2001; Teh and Sumaila, 2013). In the EU, artisanal fisheries represent 84% of the fishing fleet and employ around 100,000 workers (Garcia et al., 2008; Guyader et al., 2013; Lloret et al., 2018). Yet, studies documenting the impact of artisanal fisheries on deep coral communities and other benthic ecosystems are still scarce when compared to large-scale fisheries (Guyader et al., 2013; Lloret et al., 2018). Generally, artisanal fisheries are considered to have a lower impact on benthic communities. The actual effect, however, may be largely obscured and much greater than assumed due to the lack of reliable data for this sector and because some of the gears used are not selective (Lloret et al., 2018). For example, Shester and Micheli (2011) demonstrated experimentally that for bottom-set gillnets deployed over rocky reefs, ca. 77% of the interactions between nets and corals caused the removal or partial damage of the colonies. While that study focused on shallow-water communities, the results suggest that bottom-set gillnets represent a critical conservation concern that extends to deep coral communities as artisanal fisheries also operate over deep habitats.
This study investigates the impact of bottom-set gillnets used by artisanal fisheries on deep coral communities in Sagres, Algarve, southern Portugal. The aims of the study are to (1) better understand the biodiversity of corals in the area; (2) provide a baseline quantitative assessment of coral bycatch frequency and of the species affected by different types of bottom-set gillnets used in local fisheries; and (3) identify coral bycatch and diversity “hotspots” that could constitute priority management areas.
To assess the impact of bottom-set gillnets on circalittoral and deep coral habitats, the coral bycatch of a fishing vessel operating in Sagres, southern Portugal (Figure 1), was documented over 42 workdays during the summer-autumn of 2019 (1 September to 16 October) and spring of 2020 (11 May to 5 June). Coastal fisheries in Portugal are predominantly small-scale operations (∼91% of the fleet has <12 m hull length; DGRM, 2018) that can be categorized into different métiers, i.e., a group of fishing activities that targets a specific assemblage of species, using one kind of fishing gear, in a particular period of the year within the same area (EC, 2008; Deporte et al., 2012). In Sagres, the fishing fleet is mostly composed of small vessels (<12 m hull length) that operate locally and use multiple artisanal gear such as traps, pots, bottom longlines, trotlines, jigs, trammel nets, and small bottom-set gillnets. A few larger coastal multigear vessels (12–15 m hull length) use trammel nets and bottom-set gillnets to fish demersal and benthic species. We documented coral bycatch in a vessel belonging to the latter group, which mainly operates using bottom-set gillnets with different mesh sizes to fish several target species year-round (Table 1). The vessel mainly targets Black-bellied angler (Lophius budegassa) and John dory (Zeus faber). Several secondary species, including European spiny lobster (Palinurus elephas), pink spiny lobster (Palinurus mauritanicus), Atlantic wreckfish (Polyprion americanus), and blonde ray (Raja brachyura), are also targeted for their high commercial value. In this study, the métiers were defined according to the hierarchy presented in decision 2008/949/EC from the European Commission (EC, 2008), all of which are part of the category “set of gillnets,” differing at the levels of target assemblage (i.e., target species) and mesh size used (Table 1). For target species in which more than one mesh size was used (i.e., European spiny lobster and pink spiny lobster), we defined one métier per target species as few deployments used a smaller mesh size (one out of two in P. mauritanicus and three out of nine in P. elephas).
Figure 1. Map of the study area off the coast of Sagres (southern Portugal) showing the transects of bottom-set gillnets used to document coral bycatch in circalittoral and deep-sea habitats. (A) Summer-autumn sampling season (1 September to 31 October) with inset showing the location of the study area in Portugal. (B) Spring sampling season (11 May to 5 June) with insets zooming on nets deployed following a zigzag course. Sets of gillnets are shown by target species (colors). Bathymetric isobaths are as follows: 50 m, 100–500 m (increments of 100 m), and >500 m (increment of 200 m).
Table 1. Features of the target species métier (average ± standard error) of the bottom-set gillnets deployed during the documentation of coral bycatch in Sagres (southern Portugal) during the two seasons studied.
To simplify the results, our treatments were divided according to métier and the periods over which coral bycatch was monitored (i.e., “seasons”). These were chosen as a function of regulatory fishery closures for the target species and weather conditions, as some of the rocky-bottom-dwelling targeted species are not fished during winter to prevent damage or loss of the nets (Table 1). Coral bycatch and the amount of target species caught were quantified individually for each set of gillnets deployed. The geographic positions and depth at the start and endpoints of the nets, as well as the soaking time (in days) were also recorded. The average depth of each net set was calculated using the start and endpoint depths for deployments that followed a straight line, and the depth of each vertex point for deployments following a zigzag course (see Figure 1). Collected corals were preserved and identified to the maximum taxonomical level using available guides (e.g., Carpine and Grasshoff, 1975; Grasshoff, 1992; Cairns and Kitahara, 2012) and expert opinion. For the purpose of this study, the coral fauna assessed included members of the subclasses Octocorallia and Hexacorallia (orders Antipatharia, Zoantharia, and Scleractinia). For specimens in which species could not be identified based on visual inspection of colony alone, the morphology of skeletal sclerites (octocorals) and corallites (scleractinians) was analyzed. The maximum height and width of each specimen (orientation inferred from the presence of a holdfast or from the branching pattern characteristic to each species; Supplementary Figure S1) were measured in the lab. The specimens were classified as fragments or whole colony depending on the presence of holdfast (e.g., Octocorallia) or presence of substrate attached to the colony (Scleractinia). Additionally, the dry weight of Dendrophyllia spp. was also measured in order to estimate bycatch biomass.
In order to understand the relationship between target species landings and coral bycatch, the fishing and bycatch data were standardized as catch per unit of effort (CPUE). CPUE represents the number of specimens caught (N of fish or lobster vs. coral) as a function of the product of the soaking time (T in days) and the net length per 100 m deployed (L) (Equation 1). The analysis of the spatial distribution of the CPUEs did not include four of the 139 nets documented for which only one GPS coordinate was available, or the soaking time was not determined.
Equation 1. Fishing effort calculated as catch per unit of effort (CPUE) for each bottom-set gillnet in Sagres (Portugal) during the two sampling seasons documented. CPUE represents the number of specimens (fish/lobster or coral) caught (N) as a function of the product of the soaking time (T in days) and the net length per 100 m deployed (L).
The effect of different métiers on bycatch was tested with a general addictive model (GAM) using a Poisson distribution and a log-link function. We modeled the number of corals caught per net (response variable) as a function of target species, mesh size, depth, net length, and soaking time (fixed factors). Model selection was based on generalized cross-validation (GCV) criterion and adjusted R2. Because overdispersion was detected, the standard errors were corrected using a quasi-GAM model with the variance given by 2.06 × 1.04, where 2.06 represents the mean and 1.04 the dispersion parameter (ϕ). Backward selection and F-test were used to determine statistical significance of the variables and interaction terms. Model validation was performed through visual inspection of the residuals (quantile–quantile plot, histogram of residuals, residuals vs. predictors plot, and observed vs. fitted values plot) to detect any violation of the assumptions (Supplementary Figure S2). The analyses were performed using the MGCV package (Wood, 2017) in R version 3.6.2 (R Core Team, 2019).
The resemblance of the coral communities (species composition and abundance) caught by the different métiers was evaluated using principal coordinates analysis (PCoA). Because the GAM analysis did not show any relationship between coral bycatch and sampling season, the data were pooled. Coral species data for each net was used to calculate a dissimilarity matrix using Hellinger distance (Kindt and Coe, 2005). The resulting dissimilarity matrix was then used as input for the PCoA. Important contributions to the overall ordination along the first two PCoA axes were evaluated using Pearson correlation between the descriptors (coral species) and PCoA1 and PCoA2. To further analyze the results of the PCoA, the influence of depth on differences in species composition between métiers was evaluated with a distance-based redundancy analysis (db-RDA). In this technique, the ordination is constrained by the environmental variable. The species matrix was transformed using the Hellinger transformation (Kindt and Coe, 2005), which together with the environmental matrix (i.e., depth matrix) was used as input for the db-RDA. The significance of the constraint imposed by depth was tested with an ANOVA like permutation test (9999 permutations). Furthermore, scaling method 2 was used to represent db-RDA with the position of the species vectors representing the correlation between species. The PCoA and db-RDA analyses were performed using the BiodiversityR package (Kindt and Coe, 2005) in R version 3.6.2.
Coral bycatch was documented for a total of 139 net deployments: 78 in the summer-autumn and 61 in the spring sampling seasons. Coral specimens were caught in 118 of the nets (85%), covering a total length of 300.32 km. A total of 4326 specimens were collected (45% of which entire colonies) over the 42-day survey period: 2404 specimens over 22 days in the summer-autumn season and 1922 specimens over 20 days in the spring season. On average (±SE), we recovered 31.1 (±2.7) corals from each net, with a maximum of 144 corals caught in a single net (target species: Black-bellied angler). The maximum number of coral species found in a single net was 10 species, with an average (±SE) of 4.31 (±0.2) coral species per net.
The diversity of coral species captured as bycatch in the study area was high. A total of 22 different taxa were identified: 17 from the Order Alcyonacea (Acanthogorgia armata, Acanthogorgia hirsuta, Callogorgia verticillata, Corallium rubrum, Ellisella paraplexauroides, Eunicella verrucosa, Eunicella labiata, Eunicella gazella, Isidella elongata, Leptogorgia sarmentosa, Paramuricea clavata, Paramuricea grayi, Spinimuricea atlantica, Viminella flagellum, (Octocorallia) sp.1, (Octocorallia) sp.2, and (Octocorallia) sp.3), three from the Order Scleractinia (Dendrophyllia cornigera, Dendrophyllia Ramea, and Pourtalosmilia anthophyllites), one from the Order Zoantharia (Savalia savaglia), and 1 from the Order Antipatharia (Antipathella subpinnata). The gorgonians E. verrucosa (1380 specimens), P. grayi (1271 specimens), and C. verticillata (247), and the scleractinians D. cornigera (522 specimens) and D. ramea (249 specimens) were the most frequent species, making up 85% of the total amount of coral bycatch (Figure 2). It is worth noting that most C. verticillata specimens were caught in the spring sampling season in 12 net sets targeting Z. faber (four nets) and P. americanus (eight nets) deployed at 99–170 m depth. Overall, the diversity found in both sampling seasons was similar in terms of species composition and abundance. Exceptions include the species A. armata, I. elongata, (Octocorallia) sp.3, V. flagellum, and P. anthophyllites, which were only caught during the spring sampling season, and species (Octocorallia) sp.1 and (Octocorallia) sp.2 during the summer-autumn.
Figure 2. Species composition and total amount of the corals caught as bycatch in bottom-set gillnets during the two sampling seasons in Sagres (southern Portugal). In total, 4326 specimens (branch fragments or entire colonies) were collected from 118 gillnet deployments.
The size of the specimens collected varied considerably reflecting species-specific differences in growth form and size (Table 2). For instance, colonies of E. verrucosa had an average height and width of 22.9 cm (±0.3) and 15.5 cm (±0.2), respectively (Supplementary Figure S3A), whereas P. grayi colonies were on average 17.6 cm (±0.3) long and 11.9 cm (±0.2) wide (Supplementary Figure S3B). C. verticillata was the species with the largest fan area (Table 2). The giant gorgonian E. paraplexauroides with candelabrum-shaped colonies was the tallest coral species collected (Table 2). From the three scleractinian species caught as bycatch, D. ramea was the largest species (Table 2) with an average colony weight of 331.4 g (±95.1) and 38.1 (±8.9) polyps per colony.
Table 2. Species composition and description of the variability between colonies and fragments of the number of specimens (No) and sizes (height and width) of all specimens collected as bycatch in bottom-set gillnets during the two sampling seasons in Sagres (southern Portugal).
The majority of specimens and species were caught at locations shallower than 120 m depth (90 and 68%, respectively), where most fishing effort occurred (Figures 3, 4). Notable exceptions include the deep-water species I. elongata (296–510 m), A. subpinnata (85–510 m), and C. verticillata (99–293 m), which were caught at average depths of 417, 169, and 141 m, respectively. The octocorals P. grayi (85–97 m), L. sarmentosa (57–124 m), and C. rubrum (73–134 m) were the species collected at shallower areas, with average depths of 89, 92, and 97 m, respectively (Figure 3). Interestingly, several specimens of E. labiata and E. gazella were caught deeper than the bathymetric ranges reported in the literature (Figure 3).
Figure 3. Collection depth ranges of the coral species caught as coral bycatch by bottom-set gillnets in Sages (southern Portugal) during the two seasons studied. The bathymetric distribution recorded in the literature for each species is shown as gray band. The abundance of each species at each depth is also shown with a violin plot.
Figure 4. Spatial distribution of the total fishing effort for target species and coral bycatch in bottom-set gillnets off the coast of Sagres (southern Portugal). Fishing effort was calculated as the catch per unit effort (CPUE) and represents the number of specimens (fish/lobster or coral) as a function of the product of the net soaking time (days) and 100 m of net length deployed. (A) Summer-autumn sampling season (1 September to 16 October 2019). (B) Spring sampling season (11 May to 5 June 2020). CPUEs for the target species are displayed as heatmaps generated using triweight kernel density and corals displayed as bubbles. Bathymetric isobaths are as follows: 50 m, 100–500 m (increments of 100 m), and >500 m (increment of 200 m). Rocky substrate is represented as gray shadow.
Consistent with the expectations, total coral bycatch was generally higher when the nets were deployed on or nearby areas where rocky substrate is known to occur (Figure 4). When examining the CPUEs for the pooled dataset (i.e., irrespective of coral or target species), the mismatch between the amounts of coral and target species caught is evident, particularly in the summer-autumn for which the nets captured substantially more coral than fish or lobster (Figure 4A). For instance, six of the nets deployed in the summer-autumn sampling season had a CPUE for coral specimens higher than 3.40 (n/day.100 m; Figure 4A), which had an average net length of 1.99 km, thus corresponding to more than 60 corals per net. In contrast, for the spring survey season there is a better correspondence between the amount of coral and fish caught, with areas where coral bycatch was high, generally matching those with high fish or lobster catches (Figure 4B). Only three of the 61 nets deployed in the spring sampling season had a coral CPUE higher than 3.40 (n/day.100 m; Figure 4B).
The preferred Poisson GAM model (Supplementary Table S1) for the amount of coral caught as bycatch, supported by both the GCV and adjusted R2, included four significant factors: target species, depth, net length, and mesh size without any interaction term. The total deviance explained by the model was 40.3%. Overall, all variables have a strong effect on the amount of incidental coral catches (GCV = 21.53; R2 = 0.379): target species (df = 5, F = 6.049, p < 0.01), mesh size (df = 2, F = 4.910, p < 0.01), net length (df = 1, F = 15.820, p < 0.01), and depth (df = 1, F = 15.198, p < 0.01). The coral CPUE (n/day.100 m) was generally higher than that of the target species for the métiers documented, except for fishing activities targeting pink spiny lobster (P. mauritanicus) and blonde ray (R. brachyura; Supplementary Figure S4 and Figure 5). The spatial analysis of CPUEs by target species shows that the métiers targeting John dory and Atlantic wreckfish have the highest CPUEs, but also the highest removal rates of corals (Figure 5 and Supplementary Figure S4). In the case of the John dory fishery, the pattern is only evident at a few locations during the summer-autumn sampling season, with most net deployments capturing comparatively few fish (Figure 5C). Conversely, and despite being the dominant fishery of the vessel that we followed in this study, the métier used to fish black-bellied angler showed the lowest overall coral removal rates, with the exception of a single set that removed 144 coral specimens (Figures 5A,B).
Figure 5. Spatial distribution of the fishing effort for target species and coral bycatch in bottom-set gillnets off the coast of Sagres (Portugal) during the summer-autumn (left panels) and spring (right panels) sampling seasons. Fishing effort was calculated as the catch per unit of effort (CPUE) and represents the number of specimens (fish/lobster or coral) as a function of the product of the net soaking time (days) and 100 m of net length deployed. (A,B) Lophius budegassa; (C,D) Zeus faber; (E) Raja brachyura, Palinurus elephas, Palinurus mauritanicus pooled; and (F) Palinurus elephas and Polyprion americanus pooled. The maps in E and F show seasonally deployed nets for species that are targeted over specific periods of the year. CPUEs for the target species are displayed as heatmaps generated using triweight kernel density and corals displayed as bubbles. Bathymetric isobaths are as follows: 50 m, 100–500 m (increments of 100 m), and >500 m (increment of 200 m). Rocky substrate is represented as gray shadow.
For the five coral species most often caught as bycatch, the spatial segregation of fishing effort across the two sampling seasons is evident, with most incidental captures during the spring season occurring further offshore (Figure 6). Additionally, for P. grayi (Figure 6A), E. verrucosa (Figure 6B), and the two Dendrophyllia species (Figures 6C,D), more specimens were caught in the nets deployed in the summer-autumn sampling season (i.e., higher CPUEs). In contrast, C. verticillata (Figure 6E) was mainly caught during the spring sampling season with CPUE values being higher for this season.
Figure 6. Spatial distribution of the fishing effort for the five specimens of coral caught as bycatch in bottom-set-gillnets off the coast of Sagres (Portugal) during summer-autumn (blue color) and spring (red color) sampling seasons. Fishing effort was calculated as the catch per unit of effort (CPUE) and represents the number of specimens (coral) as a function of the product of the net soaking time (days) and 100 m of net length deployed. (A) Paramuricea grayi; (B) Eunicella verrucosa; (C) Dendrophyllia cornigera; (D) Dendrophyllia ramea; and (E) Callogorgia verticillata. CPUEs for the corals are displayed as bubbles. Bathymetric isobaths are as follows: 50 m, 100–500 m (increments of 100 m), and >500 m (increment of 200 m). Rocky substrate is represented as gray shadow.
The variation in coral community structure per gillnet set is illustrated in the PCoA analysis for the entire dataset, with the two axes capturing 43.59% of the variation in the ecological distances. The analysis shows weak separation in species composition and abundance between the majority of the métiers documented, with the exception of the métier for P. americanus, which is clearly segregated from the remaining métiers (Figure 7). This separation is strongly correlated with the coral species C. verticillata for which a high number of colonies was caught during the spring sampling season (the only season in which the métier was used; Figure 7).
Figure 7. Principal coordinate analysis (PCoA) showing the variation in the coral species community structure from bycatch using bottom-set gillnets during two sampling seasons off the coast of Sagres (Portugal). PCoA plot based on species composition per net deployed and métier. The species matrix describing the ecological resemblance per net was calculated using the Hellinger distance. The vectors display the Pearson correlations (p-value < 0.05) between the descriptors (i.e., coral species captures per net) and the PCoA1 and PCoA2. PCoA biplot of the métier documented in both sampling seasons. Significant groupings defined by métier are shown in color. For more information concerning the PCoA, refer to Section “Materials and Methods.”
As expected, the depth at which the nets were deployed was found to significantly affect coral bycatch species composition and abundance (df = 1, F = 11.861, p < 0.01). The variation in coral community structure per net set could be partially explained by differences in the depth of deployment, with the constrained ordination axis (i.e., that defined by depth) accounting for 9.42% of the total variation in the distance matrix. Overall, the constrained ordination axis and the first residual axis of the db-RDA contributed to explain 33.29% of the variation found in the distance matrix (Figure 8). The depth vector in the db-RDA indicates the direction in the graph for which net sets were deployed at deeper depths (right-hand side of Figure 8). This shows that deeper deployments contained more C. verticillata, I. elongata, A. subpinnata, and S. savaglia, whereas shallow deployments contained more P. grayi, E. verrucosa, D. ramea, D. cornigera, and L. sarmentosa (Figure 8). Additionally, the db-RDA analyses show that the species D. cornigera, D. ramea, and P. grayi are more correlated with each other, as their vector directions create small angles between them, implying that these species tend to appear in the same nets. The same pattern occurs for the pair of species C. verticillata with S. savaglia and I. elongata with A. subpinnata. Conversely, species like P. grayi and I. elongata or E. verrucosa and S. savaglia are negatively correlated (i.e., with opposite vector directions) and are not generally recovered in the same net.
Figure 8. Variation of the coral community according to the depth (db-RDA), from the assessment of coral bycatch using bottom-set gillnets during two sampling seasons off the coast of Sagres (Portugal). Species composition per net was converted to a resemblance matrix using Hellinger distance and the variable depth described as constrained ordination axis. The vectors display the Pearson correlations (p-value < 0.05) between the descriptors (i.e., coral species captures per net) and the dbRDA1 and PCoA1. Db-RDA biplot shows the variation of the coral community according to the depth at which nets were deployed. The depth vector indicates the trend from shallow (left) to deep (right). Significant groupings defined by métier are shown in color. For more information concerning the db-RDA refer to Section “Materials and Methods.”
The spatial abundance and alpha diversity found in each net allowed us to identify four main areas where the diversity and abundance are highest, which we classified as coral hotspots (Figure 9). In the hotspot areas, coral diversity was up to 22 species and 144 specimens. Other areas displayed lower but still relatively high diversity (11–16 species) and abundance (72–108 specimens) (Figure 9).
Figure 9. Spatial distribution of coral bycatch and biodiversity (no. of species) caught as incidental catches in bottom-set gillnets in Sagres (southern Portugal). Identification of possible hotspots of coral gardens that should be protected from significant adverse impacts. Hotspots coordinates as the center point of the hotspots in WGS84 (GPS). Hotspot 1: N37.0952 W-9.1058; Hotspot 2: N36.99565 W-9.06177; Hotspot 3: N36.98156 W-9.10291; Hotspot 4: N36.98032 W-9.04086. (A) Density map of corals showing the hotspots based on the number of corals collected in each net deployed. (B) Diversity map of corals showing hotspots based on the diversity of corals collected in each net deployed. Coral diversity and abundance are displayed as heatmaps generated using triangular kernel density. Bathymetric isobaths are as follows: 50 m, 100–500 m (increments of 100 m), and >500 m (increment of 200 m).
This study confirms anecdotal evidence suggesting that the impact of bottom-set gillnets on deep-sea coral communities in Portugal, and on marine animal forests in general, is greater than previously appreciated. The coral removal rates reported here, while far lower than those reported for bottom trawling (Clark et al., 2016; Victorero et al., 2018), are substantially higher than what has been described for other fishing gears such as longlines and traps (Mytilineou et al., 2014; Pham et al., 2015). Overall, our findings highlight the urgent need to better understand the large-scale impacts of artisanal and other coastal multifleet and multispecific fisheries, as well as the urgent need for appropriate management policies to conserve and protect existing coral gardens and CWCs.
Similar to previous studies conducted in other regions, bottom-set gillnets had a substantial impact on coral gardens and CWC reefs in Sagres with high levels of coral removal (Shester and Micheli, 2011). In total, 4326 coral specimens, a large proportion of which entire colonies (45%), were caught as bycatch in the 118 nets deployed over the 42-day period of our survey, corresponding to an average (±SE) of 31.1 (±2.7) corals per net. When considering each net’s length and soaking time, the removal rates become less pronounced (average coral CPUE of 0.92/day.100 m), although in some areas, particularly those for which the nets were deployed over rocky-bottom habitat, the CPUE was as high as 13.02/day.100 m (top 5% of 4.14–13.02/day.100 m). Based on the average of coral bycatch per net and daily number of nets recovered, a single fishing vessel using bottom-set gillnet can catch between 26,421–27,902 corals as bycatch per year (extrapolated to 214–226 fishing days to discount 27–39 days of bad weather). Such high levels of coral bycatch, although based on a different metric, are in line with the findings of Shester and Micheli (2011) for small-scale fisheries (SSF) in Baja California (Mexico), where gillnet sets had the highest removal rate (0.37 gorgonians per m2) when compared with fish and lobster traps. In that study, the authors report that only 21.7% of the gillnet sets interacted with gorgonians, which is much lower than what we observed here (85%), though it is possible this disparity reflects site-specific differences in coral density.
While not unexpected, our analysis indicates that the type of substrate over which the nets are deployed strongly influences coral bycatch, as the amount of coral caught in rocky-bottom areas was generally higher than in areas where hard seabed does not occur. Most coral species are found on hard substrate where they can form dense aggregations with complex architecture, which substantially increases the probability of corals becoming snagged or entangled in the nets, thus causing damage or detachment of the colonies. This association is well correlated with the ecology of the target species, which is particularly evident in the amount of coral caught when fisherman deploy sets for John Dory, a species that typically is associated with rocky habitats. In contrast, the fishery of Black-bellied angler, a species which lives on sandy or gravel-covered sea bottom (Maravelias and Papaconstantinou, 2003), had lower impact, except for deployments that crossed (or were very close to) rocky substrate. Unsurprisingly, our study also showed that coral community composition and species abundance vary significantly with depth. For instance, P. grayi and Dendrophyllia spp. are distributed in shallower habitats, while I. elongata, S. savaglia, C. verticillata, and A. subpinnata occur at greater depths. Different depths are normally associated with different environmental factors (i.e., sea bottom temperature, bottom current velocity, and chlorophyll-a concentration), which contribute to differences in community stratification as different species can have different optimal environments (Stone, 2006).
The magnitude of disturbance observed here is still considerably lower than that documented for trawlers. For example, in seamounts off the coast of Australia, it has been estimated that only 10 deep-sea trawlers passes would be required to completely decimate an area with 15–20% coral cover (Pitcher, 2000; Burridge et al., 2003; Clark et al., 2010). Although we did not attempt to quantify actual removal rates (i.e., amount removed according to the abundance in situ), our results suggest that it is likely that the community structure (i.e., size of colonies and species diversity) in the study area was different in the past. Deep-water coral species have slow growth rates and as such population recovery and reestablishment (Bavestrello et al., 1997) in the face of constant partial and total damage can be very slow (if possible at all), especially after decades of fishing. For instance, the recovery time of E. verrucosa has been estimated to range between 17 and 20 years, which can lead to the replacement of E. verrucosa colonies by shorter-lived species with quicker recovery rates (e.g., Alcyonium digitatum; Kaiser et al., 2018). These recovery times may be substantially longer for scleractinian and anthipatharian species that grow much slower.
Other fishing gears for which data are available like longlines and traps appear to have a much lower impact on coral communities compared to that caused by bottom-set gillnets. For instance, Pham et al. (2015) reported removal rates of 0.32 corals per 1000 hooks (1.15 corals per set) for deep-sea longline fishing in the Azores. For the vessel we followed in Sagres, the average coral CPUE for bottom-set gillnets was 0.92 per day.100 m (31.1 corals per set). These observations indicate that bottom-set gillnets have a higher removal rate, as we report 27 times the average coral removal per set of fishing gear. Additionally, Shester and Micheli (2011) did not report any coral bycatch from fish and lobster traps, suggesting that traps have the lowest overall impact on benthic communities.
In addition to complete removal of benthic habitat-formers, set gillnets can cause other types of physical damage, including abrasion, breakage, and partial mortality (Shester and Micheli, 2011; Bo et al., 2014). In the particular case of corals, the colonies are expected to survive and recover from partial mortality, as natural breakage is part of their population dynamics and evolutionary ecology (Hughes and Jackson, 1980; Hughes et al., 1992). However, partial colony mortality is known to have profound effects on fitness by reducing fecundity and resource availability (Wahle, 1985; Page and Lasker, 2012). Moreover, the damage caused by abrasion and breakage can promote the development of disease and necrosis points, which can further increase mortality (Bavestrello et al., 1997). While we did not evaluate the effect of these processes (beyond the biomass of fragments removed) on surviving colonies, it is expected that the extent of coral mortality caused by gillnets in Sagres, and globally, is an underestimation of the real impact (Sampaio et al., 2012). More broadly, decades of unchecked damage to these habitats, as is likely the case in Sagres, can result in long-term (potentially permanent) changes in community composition and structure, which can reduce local biodiversity and the associated fishing catches (Cryer et al., 2002; Clark and Rowden, 2009; Atkinson et al., 2011; Clark et al., 2016).
Overall, the gorgonians E. verrucosa (the pink sea fan) and P. grayi and the scleractinians Dendrophyllia spp. were the most severely impacted species, making up nearly 80% of the total bycatch. E. verrucosa, in particular, is listed as a species of principal importance in the United Kingdom and vulnerable in the IUCN Red List and may warrant protection (IUCN, 1996). For instance, our results indicate that the colonies of E. verrucosa caught as bycatch in Sagres were generally small (average height: 22.9 ± 0.3 cm; average width 15.5 ± 0.2 cm) considering the species can reach 25–50 cm in height and a similar width (Grasshoff, 1992). Similarly, the scleractinian D. cornigera (12% of total bycatch) can reach a height of 60 cm, yet the maximum height of the colonies collected in this study was 20 cm (Brito and Ocaña, 2004). These observations suggest that decades of accidental captures of these coral species by artisanal fisheries are taking a toll on the populations, as their recovery is too slow (Kaiser et al., 2018) to recover from such fishing pressures.
The diversity of coral species recovered as bycatch from bottom-set gillnets in Sagres was surprisingly high given the relatively small-scale and geographic coverage of our study. Previous assessments of deep-sea (<200 m depth) coral diversity for the Northeast Atlantic listed 173 species of corals, including antipatharians, gorgonians, and scleractinians (Hall-Spencer et al., 2007; Oliveira et al., 2015; Boavida et al., 2016a,b), with a total of 174 species known to occur in the exclusive economic zone (EEZ) of mainland Portugal (i.e., excluding Madeira and the Azores; Horton et al., 2020). We found a total of 22 species of corals belonging to the anthozoan Subclasses Octocorallia (n = 17) and Hexacorallia (n = 5), which corresponds to 13% of the species known to occur in mainland Portugal and more than previous recorded in the OCEANA/MeshAtlantic ROV campaign for circalittoral off Sagres (Monteiro et al., 2013; Nestorowicz, 2020). Despite the high number of species identified, this is likely an underestimation of the diversity of coral garden and CWC reef forming species in Sagres as our survey was limited to a 57–510 m depth range, and mostly to the upper 120 m (67% of the nets). Interestingly, the collection depths of the three species of Eunicella were higher than the bathymetric range documented in the literature.
Our analysis of the spatial distribution of coral bycatch alpha diversity and captures identified four main biodiversity hotspots in the study area with up to 22 species and 144 specimens. These findings are in accordance with recent recommendations put forward by OCEANA, which urged Portugal to expand the Natura 2000 Network to incorporate seamounts and other coral garden areas around Cape St. Vincent (Oceana., 2005, 2011). The unique richness of this area warrants a special status of protection, especially given the high direct impact of fisheries through coral removal (as documented here), as well as by lost and discarded fishing gear, a secondary effect of commercial fishing activities on benthic communities that has also been documented (Oliveira et al., 2015; Vieira et al., 2015).
This study shows that the impact of bottom-set gillnets on coral gardens and CWC reefs seems to be significant, underlining the conservation concern that fishing operations using this type of gear creates. Reducing the impact of gillnets on these habitats requires active measures that fall within one of several categories (not mutually exclusive), including measures of spatial management (i.e., MPAs), environmental legislation (i.e., list habitats as VMEs or Essential Fish Habitat-EFH), and fisheries management (i.e., temporary closures and other restrictions, and the use of alternative fishing gear). The creation of MPAs, eventually associated with VMEs and/or EFH, can be an effective measure to protect slow-growing benthic communities (and the biodiversity associated) such as coral gardens and CWCs. Only a few studies have attempted to assess the impact of deep water MPAs, as MPA placement in deep waters is still in its infancy (Markantonatou et al., 2014; Huvenne et al., 2016). Additionally, closure of certain areas to bottom trawling has been modeled and found to be potentially effective in the protection of coral gardens and CWCs, with negligible losses for bottom trawlers (Lagasse et al., 2015).
With regard to fisheries management, some of the strategies that have been adopted include frequent monitoring onboard of fishing vessels (i.e., in order to reinforce bycatch and landing laws), temporary closure of certain areas where the fishing effort is very low and coral bycatch very high (e.g., Hattonand Rockall Banks; Wright et al., 2015), and development of protocols for encounters such as move-on rules in national waters (UNGA, 2009). The move-on protocol, in particular, currently applies solely to areas beyond national jurisdiction and it mandates that when the catch of a fishing vessel (i.e., single trawl tow or set of static fishing gear) reaches a bycatch threshold of a VME indicator species, the vessel has to stop its fishing activity and move two nautical miles (NM) away from the site (Rogers and Gianni, 2010). In the Northwest Atlantic Fisheries Organization area, the thresholds were defined by weight and largely without scientific basis despite widespread advice of the scientific community for lower thresholds (Aguilar et al., 2017). In the North East Atlantic Fisheries Commission (NEAFC) area, the protocol defines that a temporary closure with 2NM on each side of the trawler track or a 2NM-radius from the most likely position of the encounters should be applied when encounters surpass a threshold of 30 kg of live coral or 400 kg of live sponges for trawler tows and other gear like gillnets, and 10 specimens per 1000 hooks or 1200 m of longline gear (FAO, 2016). If the NEAFC VME threshold for gillnets (30 kg of coral) was to be applied to the métiers studied, at least 10,345 colonies of E. verrucosa (average dry weight of colonies 2.901 ± 0.078 g, n = 988) or 350 colonies of Dendrophyllia spp. (average dry weight of colonies 85.49 ± 7.45 g, n = 819) would have to be caught in a single set to trigger the move-on rule, a value 67 and two times higher than the highest bycatch value documented in this study for a single set, respectively. Even though this estimates are based on weight data for entire colonies only (i.e., excluding fragments) and dry weight (as opposed to wet weight), a capture of more than 10,000 colonies to trigger the move-on rule would constitute a profound impact on the coral communities studied here.
Such protocols can be improved by lowering or adapting (i.e., account for the life-history traits of the dominant VME species) the thresholds based on bycatch data (as provided in this study), and by increasing the move-on and closure distances (Rogers and Gianni, 2010; Aguilar et al., 2017). These measures could also be adopted in waters of national jurisdiction, since many VME indicator species are found throughout these areas and have long been impacted by fisheries. For instance, in national waters, it may be advisable that each gear type have its own threshold and move-on distance. As an example for fishing activities using similar métiers to those documented in Sagres, the vessels could move at least 1.0NM from the middle point of the most likely position of encounters (e.g., biodiversity hotspots identified in Figure 9) because the average length of each net was 1.99 km for our study. Caution should be taken in setting distance that could be used as move-on as information about coral gardens distribution is scarce. In areas where gorgonians are common, fisheries regulators may consider instituting a threshold based on the number and size of specimens instead of weight, as it is easy to overlook the scale of the impact on the ecosystem when simply measuring the weight of a gorgonian colony (just a few grams for potentially quite old individuals). Counting specimens in these métiers is also simpler to implement.
Frequent monitoring onboard of fishing vessels, although expensive, can be a valuable management tool as well given that it can contribute to effectively implement and enforce fishing regulations (both proposed and existing), thereby reducing coral bycatch (Boenish et al., 2020). An alternative approach that has been used in a Portofino MPA (Ligurian Sea, Italy) and which could be adopted in other areas is to assess the spatial allocation of fisheries using bottom-contact gear to identify vulnerable areas (Markantonatou et al., 2014). Using different fishing gear that cause substantially lower impacts, while not a panacea, can also help reducing the impacts on coral gardens and CWC reefs. In that regard, a potential alternative is the use of bottom longlines or traps, as these gear cause substantially lower impacts to benthic ecosystems (Shester and Micheli, 2011).
This study is a pioneer assessment of the interaction between artisanal fisheries using bottom-set static gear and coral communities in mainland Portugal. Additional research will be required to fully understand the extent of the damage caused by these activities. SSF constitute more than 90% of the Portuguese fishing fleet and our findings may only show the “tip of the iceberg” of the potentially irreversible crippling of deep-sea coral habitats. Studies of this type provide essential contributions to the knowledge of the distribution and abundance of corals in southwestern Portugal, and worldwide in general. We have identified a number of important biodiversity hotspots for which habitat mapping using newer technologies like ROVs will prove essential to confirm the presence of VMEs and evaluate the scale of fishing impacts. Our findings also highlight the importance of stricter control measures onboard of fishing vessels and draws attention to the fact that artisanal fisheries as a whole pose a serious threat to the ecological functioning and integrity of coral gardens and CWC reefs in certain areas. Nevertheless, this study highlights the importance of collaborating with fishermen in order to better understand deep-sea coral biodiversity, as well as of how scientists and fishermen can work together to protect such vulnerable species. In that regard, recent efforts, including work led by our team (in prep), have shown that the tremendous amount of coral biomass generated in fishing vessels using bottom-contact gear constitutes a major resource for restoration ecology (Montseny et al., 2019, 2020).
The datasets presented in this study can be found in online repositories. The names of the repository/repositories and accession number(s) can be found below: https://doi.org/10.6084/m9.figshare.13142774.v2.
MC, JB, and ES conceived the study. VD conducted field work. VD, FO, and MC conducted data analyses and wrote the manuscript. JB, ES, and JG critically reviewed the manuscript. All authors gave the approval for the publication of the manuscript.
This research was funded by projects HABMAR (Grant No. MAR-01.04.02-FEAMP-0018) co-financed by the European Maritime and Fisheries Fund of the Operational Program MAR 2020 for Portugal (Portugal 2020), TECPESCA (Grant No. MAR2020 16-01-04-FMP-0010), and MARSW (Grant No. POSEUR/ICNF/LPN). Furthermore, this study received Portuguese national funds from FCT—Foundation for Science and Technology through project UIDB/04326/2020 and fellowship SFRH/BSAB/150485/2019. ES thanks a Pew Marine Fellowship.
The authors declare that the research was conducted in the absence of any commercial or financial relationships that could be construed as a potential conflict of interest.
We would like to thank DOCAPESCA Baleeira-Sagres for providing facilities to process the coral specimens. We are grateful to the captain Casimiro and his fishing crew for the collaboration and help onboard of the fishing vessel. In addition, we would like to thank the students Ana Carneiro, Sandra Costa, and Candice Parkes together with the volunteer Ana Gheorghiu for the help in measuring, tagging, and photographing the specimens collected.
The Supplementary Material for this article can be found online at: https://www.frontiersin.org/articles/10.3389/fmars.2020.603438/full#supplementary-material
Aguilar, R., Perry, A. L., and López, J. (2017). “Conservation and Management of Vulnerable Marine Benthic Ecosystems,” in Marine Animal Forests, eds S. Rossi, L. Bramanti, A. Gori, and C. Orejas (Cham: Springer International Publishing), 1–43. doi: 10.1007/978-3-319-17001-5_34-1
Albright, R., Takeshita, Y., Koweek, D. A., Ninokawa, A., Wolfe, K., Rivlin, T., et al. (2018). Carbon dioxide addition to coral reef waters suppresses net community calcification. Nature 555, 516–519. doi: 10.1038/nature25968
Armstrong, C. W., and van den Hove, S. (2008). The formation of policy for protection of cold-water coral off the coast of Norway. Mar. Policy 32, 66–73. doi: 10.1016/j.marpol.2007.04.007
Ashford, O. S., Kenny, A. J., Barrio Froján, C. R. S., Downie, A.-L., Horton, T., and Rogers, A. D. (2019). On the influence of vulnerable marine ecosystem habitats on peracarid crustacean assemblages in the northwest atlantic fisheries organization regulatory area. Front. Mar. Sci. 6:401. doi: 10.3389/fmars.2019.00401
Atkinson, L., Field, J., and Hutchings, L. (2011). Effects of demersal trawling along the west coast of southern Africa: multivariate analysis of benthic assemblages. Mar. Ecol. Prog. Ser. 430, 241–255. doi: 10.3354/meps08956
Bavestrello, G., Cerrano, C., Zanzi, D., and Cattaneo-Vietti, R. (1997). Damage by fishing activities to the Gorgonian coral Paramuricea clavata in the Ligurian Sea. Aquat. Conserv. 7, 253-262.
Bo, M., Bava, S., Canese, S., Angiolillo, M., Cattaneo-Vietti, R., and Bavestrello, G. (2014). Fishing impact on deep Mediterranean rocky habitats as revealed by ROV investigation. Biol. Conserv. 171, 167–176. doi: 10.1016/j.biocon.2014.01.011
Boavida, J., Assis, J., Reed, J., Serrão, E. A., and Gonçalves, J. M. S. (2016a). Comparison of small remotely operated vehicles and diver-operated video of circalittoral benthos. Hydrobiology 766, 247–260. doi: 10.1007/s10750-015-2459-y
Boavida, J., Paulo, D., Aurelle, D., Arnaud-Haond, S., Marschal, C., Reed, J., et al. (2016b). A well-kept treasure at depth: precious red coral rediscovered in atlantic deep coral gardens (SW Portugal) after 300 Years. PLoS One 11:e0147228. doi: 10.1371/journal.pone.0147228
Boenish, R., Willard, D., Kritzer, J. P., and Reardon, K. (2020). Fisheries monitoring: perspectives from the United States. Aquac. Fish. 5, 131–138. doi: 10.1016/j.aaf.2019.10.002
Bramanti, L., Movilla, J., Guron, M., Calvo, E., Gori, A., Dominguez-Carrió, C., et al. (2013). Detrimental effects of ocean acidification on the economically important Mediterranean red coral (Corallium rubrum). Glob. Change Biol. 19, 1897–1908. doi: 10.1111/gcb.12171
Brito, A., and Ocaña, O. (2004). Corals of the Canary Islands: skeleton anthozoa of the littoral and deep bottoms. La Laguna: Francisco Lemus.
Buhl-Mortensen, L., Vanreusel, A., Gooday, A. J., Levin, L. A., Priede, I. G., Buhl-Mortensen, P., et al. (2010). Biological structures as a source of habitat heterogeneity and biodiversity on the deep ocean margins: biological structures and biodiversity. Mar. Ecol 31, 21–50. doi: 10.1111/j.1439-0485.2010.00359.x
Burridge, C. Y., Pitcher, C., Wassenberg, T. J., Poiner, I., and Hill, B. J. (2003). Measurement of the rate of depletion of benthic fauna by prawn (shrimp) otter trawls: an experiment in the Great Barrier Reef. Australia. Fish. Res. 60, 237–253. doi: 10.1016/S0165-7836(02)00179-0
Cairns, S., and Kitahara, M. (2012). An illustrated key to the genera and subgenera of the Recent azooxanthellate Scleractinia (Cnidaria. Anthozoa), with an attached glossary. ZooKeys 227, 1–47. doi: 10.3897/zookeys.227.3612
Cairns, S. D. (2007). Deep-water corals: an overview with special reference to diversity and distribution of deep-water scleractinian corals. Bull. Mar. Sci. 81:12.
Carpine, C., and Grasshoff, M. (1975). Les Gorgonaires de la Méditerranée. Monaco: Institut océanographique.
Clark, M. R., Althaus, F., Schlacher, T. A., Williams, A., Bowden, D. A., and Rowden, A. A. (2016). The impacts of deep-sea fisheries on benthic communities: a review. ICES J. Mar. Sci. 73, i51–i69. doi: 10.1093/icesjms/fsv123
Clark, M. R., Bowden, D. A., Baird, S. J., and Stewart, R. (2010). Effects of fishing on the benthic biodiversity of seamounts of the “Graveyard” complex, northern Chatham Rise. N.Z. Aquat. Environ. Biodiv. Rep. 46, 1–40. doi: 10.1007/978-3-319-17001-5_37-1
Clark, M. R., and Rowden, A. A. (2009). Effect of deepwater trawling on the macro-invertebrate assemblages of seamounts on the Chatham Rise, New Zealand. Deep Sea Res. Part Oceanogr. Res. Pap. 56, 1540–1554. doi: 10.1016/j.dsr.2009.04.015
Consoli, P., Sinopoli, M., Deidun, A., Canese, S., Berti, C., Andaloro, F., et al. (2020). The impact of marine litter from fish aggregation devices on vulnerable marine benthic habitats of the central Mediterranean Sea. Mar. Pollut. Bull. 152:110928. doi: 10.1016/j.marpolbul.2020.110928
Cordes, E. E., Jones, D. O. B., Schlacher, T. A., Amon, D. J., Bernardino, A. F., Brooke, S., et al. (2016). Environmental impacts of the deep-water oil and gas industry: a review to guide management strategies. Front. Environ. Sci. 4:58. doi: 10.3389/fenvs.2016.00058
Cryer, M., Hartill, B., and O’Shea, S. (2002). Modification of marine benthos by trawling: toward a generalization for the deep ocean. Ecol. Appl. 12, 1824–1839. doi: 10.1890/1051-0761(2002)012[1824:mombbt]2.0.co;2
Davies, J. S., Guillaumont, B., Tempera, F., Vertino, A., Beuck, L., Ólafsdóttir, S. H., et al. (2017). A new classification scheme of European cold-water coral habitats: implications for ecosystem-based management of the deep sea. Deep Sea Res. Part II Top. Stud. Oceanogr. 145, 102–109. doi: 10.1016/j.dsr2.2017.04.014
Deporte, N., Ulrich, C., Mahévas, S., Demanèche, S., and Bastardie, F. (2012). Regional métier definition: a comparative investigation of statistical methods using a workflow applied to international otter trawl fisheries in the North Sea. ICES J. Mar. Sci. 69, 331–342. doi: 10.1093/icesjms/fsr197
DGRM (2018). RELATÓRIO ANUAL-FROTA DE PESCA PORTUGUESA -Ű 2018. Direção Geral de Recursos Naturais, Segurança e Serviços Marítimos. Available online at: https://www.ine.pt/xurl/pub/358627638 (accessed May 7, 2020).
EC (2008). Adopting a multiannual Community programme pursuant to Council Regulation (EC) No 199/2008 establishing a Community framework for the collection, management and use of data in the fisheries sector and support for scientific advice regarding the common fisheries policy. Off. J. Eur. Union L 346/37, 1–22.
European Parliament and the Council of the European Union (2016). REGULATION (EU) 2016/2336 of the European Parliament and of the Council - of 14 December 2016 - establishing specific conditions for fishing for deep-sea stocks in the north-east Atlantic and provisions for fishing in international waters of the north-east Atlantic and repealing Council Regulation (EC) No 2347 / 2002. Brussels: European Parliament and the Council of the European Union.
FAO (2016). Vulnerable marine ecosystems - Processes and practices in the high seas. FAO Fish. Aquac. Tech. Pap. 595, 1–30. doi: 10.1163/9789004248601_002
Fuller, S. D. (2008). Vulnerable Marine Ecosystems Dominated by Deep-Water Corals and Sponges in the NAFO Convention Area. Dartmouth: NAFO, 25.
Garcia, S. M., Allison, E. H., Andrew, N., Béné, C., Bianchi, G., de Graaf, G., et al. (2008). Towards Integrated Assessment and Advice in Small-Scale Fisheries: Principles and Processes. Rome: Food and Agriculture Organization of the United Nations.
Glover, A. G., and Smith, C. R. (2003). The deep-sea floor ecosystem: current status and prospects of anthropogenic change by the year 2025. Environ. Conserv. 30, 219–241. doi: 10.1017/S0376892903000225
Grasshoff, M. (1992). Die Flachwasser-Gorgonarien von Europa und Westafrika: Cnidaria, Anthozoa. Frankfurt am Main: Senckenbergische Naturforschende Ges.
Guyader, O., Berthou, P., Koutsikopoulos, C., Alban, F., Demanèche, S., Gaspar, M. B., et al. (2013). Small scale fisheries in Europe: a comparative analysis based on a selection of case studies. Fish. Res. 140, 1–13. doi: 10.1016/j.fishres.2012.11.008
Hall-Spencer, J., Rogers, A., Davies, J., and Foggo, A. (2007). Deep-sea coral distribution on seamounts, oceanic islands, and continental slopes in the Northeast Atlantic. Bull. Mar. Sci. 81, 135-146.
Hall–Spencer, J., Allain, V., and Fosså, J. H. (2002). Trawling damage to Northeast Atlantic ancient coral reefs. Proc. R. Soc. Lond. B Biol. Sci. 269, 507–511. doi: 10.1098/rspb.2001.1910
Halpern, B. S., Walbridge, S., Selkoe, K. A., Kappel, C. V., Micheli, F., D’Agrosa, C., et al. (2008). A global map of human impact on marine ecosystems. Science 319, 948–952. doi: 10.1126/science.1149345
Horton, T., Kroh, A., Ahyong, S., Bailly, N., Boyko, C. B., Brandão, S. N., et al. (2020). World Register of Marine Species (WoRMS). WoRMS Editorial Board. Available online at: https://www.marinespecies.org [accessed August 14, 2020).
Hourigan, T. (2009). Managing fishery impacts on deep-water coral ecosystems of the USA: emerging best practices. Mar. Ecol. Prog. Ser. 397, 333–340. doi: 10.3354/meps08278
Hughes, T. P. (1994). Catastrophes, phase shifts, and large-scale degradation of a caribbean coral reef. Science 265, 1547-1551.
Hughes, T. P., Ayre, D., and Connell, J. H. (1992). The evolutionary ecology of corals. Trends Ecol. Evol. 7, 292–295. doi: 10.1016/0169-5347(92)90225-Z
Hughes, T. P., and Jackson, J. B. C. (1980). Do corals lie about their age? some demographic consequences of partial mortality, fission, and fusion. Science 209:713. doi: 10.1126/science.209.4457.713
Hughes, T. P., Kerry, J. T., Álvarez-Noriega, M., Álvarez-Romero, J. G., Anderson, K. D., Baird, A. H., et al. (2017). Global warming and recurrent mass bleaching of corals. Nature 543, 373–377. doi: 10.1038/nature21707
Hughes, T. P., Kerry, J. T., Baird, A. H., Connolly, S. R., Dietzel, A., Eakin, C. M., et al. (2018). Global warming transforms coral reef assemblages. Nature 556, 492–496. doi: 10.1038/s41586-018-0041-2
Huvenne, V. A. I., Bett, B. J., Masson, D. G., Le Bas, T. P., and Wheeler, A. J. (2016). Effectiveness of a deep-sea cold-water coral marine protected area, following eight years of fisheries closure. Biol. Conserv. 200, 60–69. doi: 10.1016/j.biocon.2016.05.030
IUCN (1996). Eunicella verrucosa: World Conservation Monitoring Centre: The IUCN Red List of Threatened Species 1996: e.T8262A12903486. Gland: IUCN, doi: 10.2305/IUCN.UK.1996.RLTS.T8262A12903486.en
Jackson, J. B. C. (2001). Historical Overfishing and the Recent Collapse of Coastal Ecosystems. Science 293, 629–637. doi: 10.1126/science.1059199
Kaiser, M. J., Hormbrey, S., Booth, J. R., Hinz, H., and Hiddink, J. G. (2018). Recovery linked to life history of sessile epifauna following exclusion of towed mobile fishing gear. J. Appl. Ecol. 55, 1060–1070. doi: 10.1111/1365-2664.13087
Kindt, R., and Coe, R. (2005). Tree Diversity Analysis. A Manual and Software for Common Statistical Methods for Ecological and Biodiversity Studies. Nairobi: World Agroforestry Centre (ICRAF).
Lagasse, C., Knudby, A., Curtis, J., Finney, J., and Cox, S. (2015). Spatial analyses reveal conservation benefits for cold-water corals and sponges from small changes in a trawl fishery footprint. Mar. Ecol. Prog. Ser. 528, 161–172. doi: 10.3354/meps11271
Lloret, J., Cowx, I. G., Cabral, H., Castro, M., Font, T., Gonçalves, J. M. S., et al. (2018). Small-scale coastal fisheries in European Seas are not what they were: ecological, social and economic changes. Mar. Policy 98, 176–186. doi: 10.1016/j.marpol.2016.11.007
Maravelias, C., and Papaconstantinou, C. (2003). Size-related habitat use, aggregation patterns and abundance of angler fish (Lophius budegassa) in the Mediterranean Sea determined by generalized additive modelling. J. Mar. Biol. Assoc. 83, 1171-1178.
Markantonatou, V., Marconi, M., Cappanera, V., Campodonico, P., Bavestrello, A., Cattaneo-Vietti, R., et al. (2014). “Spatial Allocation of Fishing Activity on Coralligenous Habitats in Portofino MPA (Liguria, Italy),” in Proceedings of the 2nd Mediterranean Symposium on the Conservation of Coralligenous and Other Calcareous Bio-Concretions, Portoroz, 118–123.
McGoodwin, J. R. (2001). Understanding the Cultures of Fishing Communities: A Key to Fisheries Management and Food Security. Rome: FAO.
Monteiro, P., Bentes, L., Oliveira, F., Afonso, C., Rangel, M., Alonso, C., et al. (2013). Atlantic Area Eunis Habitats. Adding New Habitat Types From European Atlantic Coast to the EUNIS Habitat Classification. Faro: CCMAR-Universidade do Algarve.
Montseny, M., Linares, C., Viladrich, N., Capdevila, P., Ambroso, S., Díaz, D., et al. (2020). A new large-scale and cost-effective restoration method for cold-water coral gardens. Aquat. Conserv. Mar. Freshw. Ecosyst 30, 977-987. doi: 10.1002/aqc.3303
Montseny, M., Linares, C., Viladrich, N., Olariaga, A., Carreras, M., Palomeras, N., et al. (2019). First attempts towards the restoration of gorgonian populations on the Mediterranean continental shelf. Aquat. Conserv. Mar. Freshw. Ecosyst. 29, 1278–1284. doi: 10.1002/aqc.3118
Movilla, J., Orejas, C., Calvo, E., Gori, A., López-Sanz, À, Grinyó, J., et al. (2014). Differential response of two Mediterranean cold-water coral species to ocean acidification. Coral Reefs 33, 675–686. doi: 10.1007/s00338-014-1159-9
Mytilineou, C., Smith, C. J., Anastasopoulou, A., Papadopoulou, K. N., Christidis, G., Bekas, P., et al. (2014). New cold-water coral occurrences in the Eastern Ionian Sea: results from experimental long line finishing. Deep Sea Res. Part II Top. Stud. Oceanogr. 99, 146-157. doi: 10.1016/j.dsr2.2013.07.007
Nestorowicz, I.-M. (2020). Identifying Priority Habitats in the upper São Vicente Submarine Canyon (Portugal). Dissertation/master’s thesis. Belgium: University of Algarve/CCMAR/Ghent University.
Oceana. (2011). Workshop on the Improvement of the Definitions of Habitats on the OSPAR List. Bergen: OCEANA.
Oliveira, F., Monteiro, P., Bentes, L., Henriques, N. S., Aguilar, R., and Gonçalves, J. M. S. (2015). Marine litter in the upper São Vicente submarine canyon (SW Portugal): abundance, distribution, composition and fauna interactions. Mar. Pollut. Bull. 97, 401–407. doi: 10.1016/j.marpolbul.2015.05.060
Page, C. A., and Lasker, H. R. (2012). Effects of tissue loss, age and size on fecundity in the octocoral Pseudopterogorgia elisabethae. J. Exp. Mar. Biol. Ecol. 434, 47–52. doi: 10.1016/j.jembe.2012.07.022
Parker, S., Penney, A., and Clark, M. (2009). Detection criteria for managing trawl impacts on vulnerable marine ecosystems in high seas fisheries of the South Pacific Ocean. Mar. Ecol. Prog. Ser. 397, 309–317. doi: 10.3354/meps08115
Pham, C. K., Diogo, H., Menezes, G., Porteiro, F., Braga-Henriques, A., Vandeperre, F., et al. (2015). Deep-water longline fishing has reduced impact on Vulnerable Marine Ecosystems. Sci. Rep. 4:4837. doi: 10.1038/srep04837
Pitcher, C. (2000). Implications of the effects of trawling on sessile megazoobenthos on a tropical shelf in northeastern Australia. ICES J. Mar. Sci. 57, 1359–1368. doi: 10.1006/jmsc.2000.0911
Purser, A., and Thomsen, L. (2012). Monitoring strategies for drill cutting discharge in the vicinity of cold-water coral ecosystems. Mar. Pollut. Bull. 64, 2309–2316. doi: 10.1016/j.marpolbul.2012.08.003
R Core Team (2019). R: A Language and Environment for Statistical Computing. Vienna: R Foundation for Statistical Computing.
Ragnarsson, S. Á, Burgos, J. M., Kutti, T., van den Beld, I., Egilsdóttir, H., Arnaud-Haond, S., et al. (2017). “The Impact of Anthropogenic Activity on Cold-Water Corals,” in Marine Animal Forests, eds S. Rossi, L. Bramanti, A. Gori, and C. Orejas (Cham: Springer International Publishing), 989–1023. doi: 10.1007/978-3-319-21012-4_27
Roberts, J. M. (2006). Reefs of the deep: the biology and geology of cold-water coral ecosystems. Science 312, 543–547. doi: 10.1126/science.1119861
Rogers, D. A. D., and Gianni, M. (2010). The Implementation of UNGA Resolutions 61/105 and 64/72 in the Management of Deep-Sea Fisheries on the High Seas. London: International Programme on the State of the Ocean.
Rossi, S. (2013). The destruction of the ‘animal forests’ in the oceans: towards an over-simplification of the benthic ecosystems. Ocean Coast. Manag. 84, 77–85. doi: 10.1016/j.ocecoaman.2013.07.004
Rossi, S., Bramanti, L., Gori, A., and Orejas, C. (2017). “Animal Forests of the World: An Overview,” in Marine Animal Forests: The Ecology of Benthic Biodiversity Hotspots. Cham: Springer International Publishing, 3–23. doi: 10.1007/978-3-319-17001-5
Sampaio, Í, Braga-Henriques, A., Pham, C., Ocaña, O., de Matos, V., Morato, T., et al. (2012). Cold-water corals landed by bottom longline fisheries in the Azores (north-eastern Atlantic). J. Mar. Biol. Assoc. U.K. 92, 1547–1555. doi: 10.1017/S0025315412000045
Shester, G. G., and Micheli, F. (2011). Conservation challenges for small-scale fisheries: bycatch and habitat impacts of traps and gillnets. Biol. Conserv. 144, 1673–1681. doi: 10.1016/j.biocon.2011.02.023
Stone, R. P. (2006). Coral habitat in the Aleutian Islands of Alaska: depth distribution, fine-scale species associations, and fisheries interactions. Coral Reefs 25, 229–238. doi: 10.1007/s00338-006-0091-z
Teh, L. C. L., and Sumaila, U. R. (2013). Contribution of marine fisheries to worldwide employment: global marine fisheries employment. Fish Fish. 14, 77–88. doi: 10.1111/j.1467-2979.2011.00450.x
UNGA (2004). Resolution 59/25 Sustainable fisheries, including through the 1995 Agreement for the Implementation of the Provisions of the United Nations Convention on the Law of the Sea of 10 December 1982 relating to the Conservation and Management of Straddling Fish Stocks and Highly Migratory Fish Stocks, and related instruments. A/RES/59/25. New York, NY: UNGA.
UNGA (2009). Resolution 64/72 Sustainable fisheries, including through the 1995 Agreement for the Implementation of the Provisions of the United Nations Convention on the Law of the Sea of 10 December 1982 relating to the Conservation and Management of Straddling Fish Stocks and Highly Migratory Fish Stocks, and related instruments A/RES/64/72. New York, NY: UNGA.
UNGA (2019). 74/18. Sustainable fisheries, including through the 1995 Agreement for the Implementation of the Provisions of the United Nations Convention on the Law of the Sea of 10 December 1982 relating to the Conservation and Management of Straddling Fish Stocks and Highly Migratory Fish Stocks, and related instruments. A/RES/74/18. New York, NY: UNGA.
Victorero, L., Watling, L., Deng Palomares, M. L., and Nouvian, C. (2018). Out of sight. but within reach: a global history of bottom-trawled deep-sea fisheries from >400 m depth. Front. Mar. Sci. 5:98. doi: 10.3389/fmars.2018.00098
Vieira, R. P., Raposo, I. P., Sobral, P., Gonçalves, J. M. S., Bell, K. L. C., and Cunha, M. R. (2015). Lost fishing gear and litter at Gorringe Bank (NE Atlantic). J. Sea Res. 100, 91–98. doi: 10.1016/j.seares.2014.10.005
Wahle, C. M. (1985). Habitat-related patterns of injury and mortality among Jamaican gorgonians. Bull. Mar. Sci. 37, 23.
Wood, S. N. (2017). Generalized Additive Models: An Introduction with R, 2nd Edn. London: Chapman and Hall.
Keywords: coral gardens, marine animal forests, biodiversity conservation and management, vulnerable marine ecosystems, cold-water corals, benthic invertebrate bycatch, bottom fisheries impact
Citation: Dias V, Oliveira F, Boavida J, Serrão EA, Gonçalves JMS and Coelho MAG (2020) High Coral Bycatch in Bottom-Set Gillnet Coastal Fisheries Reveals Rich Coral Habitats in Southern Portugal. Front. Mar. Sci. 7:603438. doi: 10.3389/fmars.2020.603438
Received: 06 September 2020;
Accepted: 27 October 2020;
Published: 13 November 2020.
Edited by:
Carlo Cerrano, Marche Polytechnic University, ItalyReviewed by:
Alessandro Cau, University of Cagliari, ItalyCopyright © 2020 Dias, Oliveira, Boavida, Serrão, Gonçalves and Coelho. This is an open-access article distributed under the terms of the Creative Commons Attribution License (CC BY). The use, distribution or reproduction in other forums is permitted, provided the original author(s) and the copyright owner(s) are credited and that the original publication in this journal is cited, in accordance with accepted academic practice. No use, distribution or reproduction is permitted which does not comply with these terms.
*Correspondence: Márcio A. G. Coelho, bWFjb2VsaG9AdWFsZy5wdA==
Disclaimer: All claims expressed in this article are solely those of the authors and do not necessarily represent those of their affiliated organizations, or those of the publisher, the editors and the reviewers. Any product that may be evaluated in this article or claim that may be made by its manufacturer is not guaranteed or endorsed by the publisher.
Research integrity at Frontiers
Learn more about the work of our research integrity team to safeguard the quality of each article we publish.