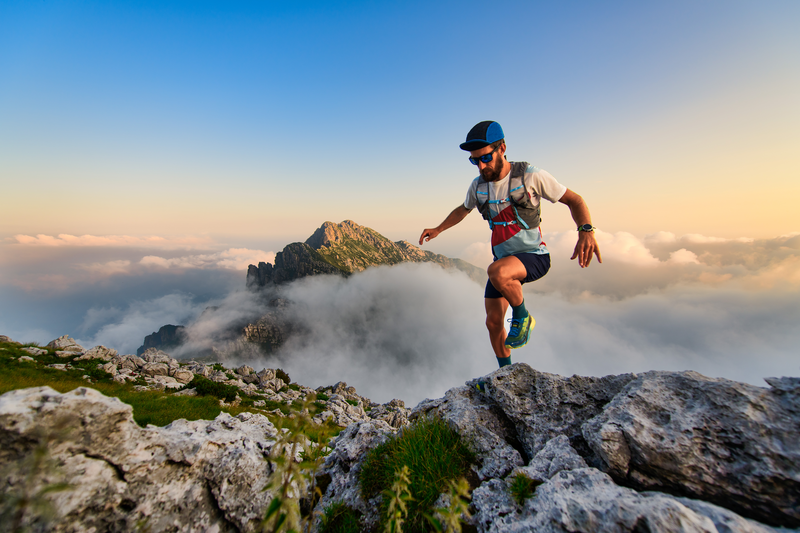
94% of researchers rate our articles as excellent or good
Learn more about the work of our research integrity team to safeguard the quality of each article we publish.
Find out more
ORIGINAL RESEARCH article
Front. Mar. Sci. , 16 December 2020
Sec. Marine Megafauna
Volume 7 - 2020 | https://doi.org/10.3389/fmars.2020.596619
This article is part of the Research Topic Movement and Connectivity of Large Pelagic Sharks View all 22 articles
Silky sharks (Carcharhinus falciformis) represent a major component of global shark catch, both directly and as bycatch, and populations are declining as a result. An improved understanding of their movement ecology is needed to support conservation efforts. We deployed satellite and acoustic tags (2013–2018) and analyzed historical fisheries records (1997–2009), to investigate the spatial ecology of silky sharks in the central Indian Ocean and a large Marine Protected Area (MPA; 640,000 km2) around the Chagos Archipelago. We observed high fidelity to the MPA, and a sustained diurnal association with a seamount complex, with individuals moving off at night and returning at sunrise. Yet, we also observed large-scale divergent movements in two satellite tagged individuals and documented the furthest recorded displacement distance for a satellite tagged silky shark to date, with one individual moving from the MPA to the Kenyan coast—a displacement distance of 3,549 km (track distance ∼4,782 km). Silky sharks undertook diel vertical migrations and oscillatory diving behavior, spending > 99% of their time in the top 100 m, and diving to depths of greater than 300 m, overlapping directly with typical deployments of purse seine and longline sets in the Indian Ocean. One individual was recorded to a depth of 1,112 m, the deepest recorded silky shark dive to date. Individuals spent 96% of their time at liberty within water temperatures between 24 and 30°C. Historic fisheries data revealed that silky sharks were a major component of the shark community around the archipelago, representing 13.69% of all sharks caught by longlines before the fishery closed in 2010. Over half (55.88%) of all individuals caught by longlines and purse seiners were juveniles. The large proportion of juveniles, coupled with the high site fidelity and residence observed in some individuals, suggests that the MPA could provide considerable conservation benefits for silky sharks, particularly during early life-history stages. However, their high mobility potential necessitates that large MPAs need to be considered in conjunction with fisheries regulations and conservation measures in adjacent EEZs and in areas beyond national jurisdiction.
Significant historical declines have been observed in pelagic shark populations (Baum et al., 2003; Ferretti et al., 2010; Dulvy et al., 2014). This has largely been driven by high spatial overlap with industrial fishing activities (Queiroz et al., 2016, 2019), where pelagic sharks account for over half of shark catch globally (Worm et al., 2013). Central to developing the effective conservation and management strategies urgently needed for these species is a firm understanding of their spatial ecology (Boerder et al., 2019).
Pelagic sharks spend considerable periods of time on the high seas, in areas beyond national jurisdiction (Queiroz et al., 2019), where there are limited legal frameworks to protect them (Wright et al., 2019). However, electronic tagging indicates some pelagic shark species exploit their environment in predictable ways (Block et al., 2011; Lea et al., 2015; Doherty et al., 2017) or show residency to certain sites or features (e.g., Jorgensen et al., 2010; Queiroz et al., 2016; Carlisle et al., 2019; Aldana-Moreno et al., 2020) providing target areas for effective management. Yet, existing studies into pelagic shark spatial ecology are taxonomically and geographically biased. In a recent analysis of pelagic shark tagging data, nearly 77% of pelagic sharks tagged were from just six species (blue shark Prionace glauca, shortfin mako Isurus oxyrinchus, tiger shark Galeocerdo cuvier, salmon shark Lamna ditropis, whale shark Rhincodon typus, and white shark Carcharodon carcharias) and highlighted that the Indian Ocean was generally data-poor compared to the Pacific and Atlantic Oceans (Queiroz et al., 2019). As such, there is a pressing need to broaden our understanding of pelagic shark ecology to understudied species and underrepresented regions.
Silky sharks (Carcharhinus falciformis) are important, yet relatively understudied, pelagic predators that inhabit continental shelves, slopes, and offshore waters from the surface down to 500 m (Compagno, 1984; Bonfil, 2008). Adult silky sharks are primarily piscivorous (Compagno, 1984), with juveniles also feeding on pelagic crustaceans (Filmalter et al., 2017), and they form large feeding aggregations when food is plentiful (Bonfil, 2008). In the Gulf of Mexico, neonates and young juveniles have been shown to live a more coastal, demersal lifestyle than adults (Branstetter, 1987; Bonfil, 1997). However, elsewhere, juveniles have been regularly reported in open ocean environments (e.g., Filmalter et al., 2015; Hutchinson et al., 2019; Bonnin et al., 2020), often joining schools of large pelagic fishes, such as tuna (Camhi, 2009; Sánchez-De Ita et al., 2011). Silky sharks grow up to 330 cm in length (Camhi, 2009), and can be highly mobile, with maximum recorded displacement distances of over 3,000 km (Schaefer et al., 2019). Biotelemetry devices have been deployed in the West Indian Ocean and Pacific Ocean to explore the movement and mortality rates of these sharks in response to fisheries’ bycatch (Musyl et al., 2003, 2011a; Filmalter et al., 2011; Poisson et al., 2011, 2014; Hutchinson et al., 2015; Musyl and Gilman, 2018), and to quantify associations with fishing gear, such as fish aggregation devices (FADs) (Filmalter et al., 2010, 2015; Muir et al., 2012). A few tagging studies have also evaluated key behaviors such as residency patterns or habitat utilization, but these have been focused in the Pacific Ocean (Musyl et al., 2011b; Hutchinson et al., 2019) and the Red Sea (Clarke et al., 2011a), covering just a fraction of their circumtropical range.
Silky sharks are one of the most heavily exploited elasmobranch species, targeted by both artisanal and industrial longline shark fisheries (Hazin et al., 2007; Bonfil, 2008; Henderson et al., 2009). Their tendency to associate with schools of tuna also results in them representing a major component of bycatch in tropical longline and purse seine fisheries (Román-Verdesoto and Orozco-Zöller, 2005; Bonfil, 2008; Watson et al., 2009; Clarke et al., 2011b; Gilman, 2011). Furthermore, their propensity to associate with floating objects, especially as juveniles (Romanov, 2002; Amandè et al., 2008), makes them particularly vulnerable to FAD fishing (Filmalter et al., 2011), where they can comprise up to 95% of the total elasmobranch bycatch (Román-Verdesoto and Orozco-Zöller, 2005; Gilman, 2011; Lawson, 2011), with a high proportion being juveniles (Amandè et al., 2008, 2010). Mortality rates of sharks caught by purse seiners is also high, with less than 20% of released individuals thought to survive (Poisson et al., 2014; Hutchinson et al., 2015). As a result, the silky shark population in the Atlantic Ocean is declining (Rigby et al., 2017) and stocks in the Western and Central Pacific (WCPFC, 2018) and Indian Ocean are “subject to overfishing” (Urbina et al., 2018). A number of conservation and management actions have been initiated to address perceived declines, such as the listing of silky sharks on1 of the Convention on the Conservation of Migratory Species of Wild Animals (CMS) and see footnote1 of the Convention on International Trade in Endangered Species of Wild Fauna & Flora (CITES), or the prohibition of all vessels from retaining or landing any silky shark in the Western and Central Pacific Region (CMM 2013–08). Yet, these measures alone have not reversed population declines, and the status of silky sharks globally was recently uplisted from “Near Threatened” to “Vulnerable” on The IUCN Red List of Threatened Species (Rigby et al., 2017).
No-take marine protected areas (MPAs), also known as marine reserves, have been proposed as effective shark conservation strategies within exclusive economic zones (EEZ) (Baum et al., 2003; Robbins et al., 2006; Watson et al., 2009). However, the success of small-scale no-take MPAs in protecting sharks can be limited (Robbins et al., 2006), as even reef sharks can range beyond the limits of small MPAs (Chapman et al., 2005). Large no-take MPAs that encompass vast swathes of open ocean could be part of the solution for the conservation of sharks (Wood et al., 2008; Game et al., 2009; Koldewey et al., 2010). Yet there is limited evidence that large no-take MPAs can protect mobile pelagic species such as sharks or tunas, with protected areas potentially displacing fishing effort to other areas (De Santo et al., 2011; Kaplan et al., 2014; Curnick et al., 2020). Therefore, the efficacy of MPAs for pelagic sharks will be dependent on the spatial relationship between the habitats of sharks and protected area coverage.
In this study, we investigate movement behaviors of silky sharks in the central Indian Ocean and consider the role of the Chagos Archipelago, and the British Indian Ocean Territory (BIOT) MPA that surrounds it, in their spatial ecology. It has been previously hypothesized that this MPA’s large size and habitat heterogeneity could provide conservation benefits for pelagic sharks (Koldewey et al., 2010). However, important knowledge of the spatial ecology and habitat use of pelagic sharks within the central Indian Ocean is sparse. Using a combination of biotelemetry tags (both satellite and acoustic) and historical fisheries data, we aimed to (i) understand the horizontal movement patterns, habitat use and site fidelity of silky sharks within the MPA and how they are connected to the wider Indian Ocean, (ii) quantify the vertical and thermal habitat use by silky sharks, and (iii) assess the relative abundance and population demographics of silky sharks around the Chagos Archipelago. This study contributes to the understanding of silky shark ecology with several novel insights gained into their movement behavior.
The Chagos Archipelago is situated 500 km south of the Maldives and is a group of 55 islands, submerged banks (Sheppard et al., 2012), and 73 seamounts (Yesson et al., 2020). Extending out to the full EEZ, the BIOT MPA covers 640,000 km2 of ocean, with the vast majority being deep oceanic water with maximum depths of over 5,000 m. The BIOT MPA was declared a no-take protected area in 2010 with the exclusion of all industrial fishing practices. Prior to the establishment of the MPA, the main licensed commercial fishery was for tunas and billfishes, using both longline and purse seine methods (Dunn and Curnick, 2019; Curnick et al., 2020), with considerable shark bycatch (Koldewey et al., 2010).
Silky sharks were caught around the Chagos Archipelago in February and March of 2013 and 2018 using baited handlines with barbless circle hooks. Once hooked, sharks were brought alongside the tagging platform, tail-roped and inverted to initiate tonic immobility (Kessel and Hussey, 2015). Once secured, total length, sex, tagging location (latitude and longitude) and date were recorded for each individual (except for one shark where sex was not recorded). Silky sharks were tagged with either pop-up archival transmitting satellite tags (MiniPATs models 247 and 386; Wildlife Computers, Redmond, WA, United States), Smart Position or Temperature Transmitting tags (SPOT5; Wildlife Computers, Redmond, WA, United States), acoustic tags (V16, 69 kHz, transmission interval 30–90 s or 125–175 s; Vemco, Halifax, Nova Scotia, Canada), or ‘double tagged’ with a combination of satellite and acoustic tags (Table 1).
Table 1. Tag deployments on silky sharks (Carcharhinus falciformis) around the Chagos Archipelago between 2013 and 2018.
MiniPATs (n = 6) were leadered with 15 cm of 180 kg monofilament (Moimoi, Kobe, Japan), covered with a single layer of aramide braided cord, two layers of shrink wrap (thermoplastic), and attached to a custom titanium dart (Wilson et al., 2015). MiniPATs were embedded with a stainless-steel tip into the muscle tissue at the base of the dorsal fin through a small incision made with a sterile scalpel blade. Tags were set to activate upon entering the water and record ambient light, temperature and depth and were programmed to detach from tagged sharks after 100–300 days (see Table 1). Depth and temperature data were summarized into 24 h bins, and for two individuals, transmitted time series at 2.5- or 10-min intervals when available. At the end of the deployment period, or after a premature release, MiniPATs surfaced and relayed the data via the Argos satellite system2. Data messages were then viewed, processed and downloaded through the Wildlife Computers Data Portal3. A premature release procedure, whereby the tag would detach from the tether if the tag recorded a constant depth (±3 m) for a period greater than 3 days, was programmed to ensure tag retrieval in the case of shark mortality.
SPOT tags (n = 2) were attached to the top of the first dorsal fin of two sharks using a two-bolt attachment following Weng et al. (2005). SPOT tags were cleaned with alcohol prior to attachment and had a thin rubber pad to limit abrasion with the shark fin. Tags transmitted location data to Argos satellites whenever the shark’s fin broke the surface of the water long enough for the tag to send a message (∼15–30 s). The accuracy of position estimates, referred to as location class (LC), was variable and depended on the number and time between transmissions received during a satellite pass. Position accuracy records are classified as either LC 3 (<250 m), 2 (250–500 m), 1 (500–1,500 m), 0 (>1,500 m), A or B (no estimate of spatial accuracy), or Z (invalid) as determined by the Argos system (see Witt et al., 2010)2. Only Argos-derived locations with LC 1, 2, or 3 were used in this study.
To explore potential residency to geographic features within the MPA, four sharks were tagged at the Sandes-Swart seamount complex in the south of the archipelago with long-term (VEMCO V16) acoustic transmitters in March 2018. Acoustic tags were soaked in betadine and implanted intraperitoneally through a small incision created with a sterile blade (∼2–3 cm) just off the midline of their abdomen. A single suture (Ethilon, United States) was then used to close the incision. Acoustic tags transmit a unique identification code at regular intervals with a nominal delay of either 30–90 (Sharks 4 and 5) or 125–175 s (Sharks 6 and 7), for the duration of their battery life (up to 10 years). This code is detected and recorded by a receiver when the shark swims within ∼500 m (Jacoby et al., 2020). Sharks were acoustically tracked as part of a broader tagging program (including both reef-associated and pelagic species) between March 2013 and March 2020 around the Chagos Archipelago (see Carlisle et al., 2019; Andrzejaczek et al., 2020; Jacoby et al., 2020). In March 2019, data (corresponding to March 2018 to March 2019) were downloaded from 47 acoustic receivers [VR2Ws, VR2W-ARs (AR—Acoustic Release), VR2W-UWMs, and VR4Gs; Vemco, Nova Scotia, Canada] located across Peros Banhos atoll (n = 25), Salomon Atoll (n = 7), Great Chagos Bank (n = 3), Nelson Island (n = 1), Egmont Atoll (n = 4), Speakers Bank (n = 3), Benares Shoal (n = 2), Blenheim Reef (n = 1), and Victory Bank (n = 1) (see Carlisle et al., 2019; Jacoby et al., 2020; Figure 1). Receivers were deployed on or adjacent to reefs on the ocean-side of geographic features (atoll, shore, or bank) and at depths between 20 and 30 m (Figure 1). Across the same monitoring period, three VR2W-ARs were deployed linearly east-to-west on the Sandes-Swart seamount complex (SS01 72.225, −7.146; SS03 72.192, −7.137; and SS04 72.133, −7.149). These summits each reach a depth of ∼70m and rise from depths of more than 600m (for full description, see Hosegood et al., 2019). Receivers were deployed at depths between ∼95 and 350 m (Figure 1) and the distances between SS01 and SS03 was ∼3.8 km and between SS03 and SS04 was ∼6.6 km.
Figure 1. The location of Vemco acoustic receivers across the Chagos Archipelago between March 2018 and March 2019. Colors represent receiver type. Labels depict the location of major island groups, atolls and submerged banks referred to in this study.
All procedures were approved by the Stanford University Administrative Panel on Laboratory Animal Care (APLAC) under permit APLAC-10765 and by the Zoological Society of London’s Ethics Committee under permit 186-BME-0652.
For individuals tagged with MiniPATs, location estimates were processed using the manufacturer’s software, Geolocation Processing Estimator 3 (GPE3; Wildlife Computers Inc., Redmond, WA, United States), which has been used widely to process elasmobranch tracking data (e.g., Skomal et al., 2017; Hutchinson et al., 2019; Peel et al., 2020). GPE3 generates two maximum likelihood position estimates per day using a hidden Markov model (Patterson et al., 2009) with a 0.25° × 0.25° grid spacing, and position estimates (two per day) interpolated and smoothed with a cubic spline. Program parameters were defined for silky sharks to generate maximum likelihood position estimates and maximum likelihood tracks (MLT). These included animal speed (representing the standard deviation of a normal distribution of the diffusion rate for the animal), model domain (using only the marine domain to exclude points on land), sea surface temperature (referencing position estimates against known environmental conditions; NOAA High Resolution SST data provided by the NOAA/OAR/ESRL PSD, Boulder, Colorado, United States, from their website at http://www.esrl.noaa.gov/psd/); underlying bathymetry (referencing position estimates against known depths; Amante and Eakins, 2009); and by comparing observed twilight light readings with expected twilight data. GPE3 model runs were further constrained by the inclusion of known positions from SPOT fixes (using only positions of LC 1, 2, and 3), and acoustic tag detections (limited to two locations per 24 h, with the middle detection of the sequence taken on occasions that sharks were detected multiple times on the same receiver on the same day) during the period that the animal was tagged with a MiniPAT. Input data to the model also included the deployment location recorded with GPS and the endpoint tag pop-up location from Argos. Speed filters were applied iteratively to the longest track by distance (Shark 4), with GPE3 model runs examined every 0.25 m/s, from 0.5 to 3.0 m/s. Outputs were compared using the GPE3 quality score provided, with 1.5 m/s producing the most likely track (Supplementary Table 1). For consistency, this speed filter was then applied to the tracks of the other three sharks. MLTs were plotted in R (R Development Core Team, 2018) using the “ggplot2” and “marmap” packages over a bathymetry layer obtained from the ETOPO1 database hosted on the NOAA website at 4 min resolution through the “marmap” package in R. As Shark 3’s MiniPAT did not report, its track was generated only using SPOT fixes of LC 1, 2, and 3. Total track length (km) and displacement distance (kilometers between tagging location and pop-off location, km) were calculated within the “move” package (Kranstauber and Smolla, 2015). We then averaged the GPE3 generated 12-h probability density surfaces for each tag and resampled the 0.25° GPE3 grid at a resolution of 0.0125° using bilinear interpolation using the R package “raster” (sensu Stewart et al., 2016). From these, we calculated the 50, 75, and 95% polygons as a metric for each animal’s activity space from the merged probability surfaces and estimated their size (km2) and overlap (proportion of full probability surface) with the BIOT MPA.
For those individuals carrying acoustic tags, we first defined the “monitoring period” as the number of days between the tagging date (17/03/2018, the same for all tagged individuals) and when the receivers were downloaded at the end of the study (23/03/2019). Second, we defined ‘detection period’ as the number of days between the tagging date and the last detection by an individual on any one of the acoustic receivers around the archipelago. False detections caused by tag clashes or ambient noise (69 kHz) were removed (as per Jacoby et al., 2020). Two measures of residency were then calculated as per Cochran et al. (2019). First, we calculated the minimum residency index (Rmin) as the proportion of unique days detected across the monitoring period for each shark. However, Rmin is conservative and is sensitive to fieldwork schedules and assumes the animal is alive and the tag is functioning across the full monitoring period, potentially underrepresenting true residency. We therefore also calculated the maximum residency index (Rmax) as the proportion of unique days detected across the detection period. In conjunction, these metrics represent the upper and lower bounds for each animal’s true residency behavior (Cochran et al., 2019). Visit characteristics (duration and frequency of visits to receiver locations) were calculated for each individual following Meyer et al. (2009, 2018). A visit started and ended when either the location changed, or the transmitter was not detected for 30 min. Visits consisting of single transmitter detections were considered to last 5.9 min (equivalent to the transmitter pulse train duration of 3.6 s, preceded and followed by listening periods equivalent to the maximum nominal delay of 175 s) (Supplementary Table 3). We examined temporal overlap of individuals visiting the same receiver station. Detection time was converted to local time (Indian Chagos Time, UTC+6 h) and rounded to the nearest hour to explore diel patterns in occurrence at the seamounts. We then calculated the average hourly presence rates for each individual on any of the receivers from the hour of their first detection through to the hour of their last detection. For example, if shark “x” was detected at 06:00:00 14 times across a full 50-day detection period, the presence rate at 06:00:00 would be 0.28.
Depth and temperature data from MiniPATs were either summarized into daily (24 h) bins (Sharks 2 and 4) or transmitted as time series at 150 s (Shark 6) or 600 s (Shark 1) intervals (Table 1). All values are expressed as the daily mean ± standard deviation. For the summarized data, the temperature bins were: < 5°C; 5 ≤ 10°C; 10 ≤ 14°C; 14 ≤ 18°C; 18 ≤ 20°C; 20 ≤ 22°C; 22 ≤ 24°C; 24 ≤ 26°C; 26 ≤ 28°C; 28 ≤ 30°C; 30 ≤ 32°C and > 32°C. For depth, these bins were: < 5 m; 5 ≤ 10 m; 10 ≤ 25 m; 25 ≤ 50 m; 50 ≤ 75 m; 75 ≤ 100 m; 100 ≤ 150 m; 150 ≤ 200 m; 200 ≤ 250 m; 250 ≤ 300 m; 300 ≤ 600 m and > 600 m. Time series data were split into diel phases using the R package “suncalc” (Thieurmel and Elmarhraoui, 2019) in combination with estimated daily locations, and mean depths occupied during the day and night were compared using a Wilcoxon signed rank test for each individual. Dives beyond 140 m depths were quantified, with dive duration estimated as the period of time between leaving and returning to the top 100 m. To minimize the influence of the capture process on shark behavior, we removed the first 24 h post-tagging from our analyses (Cliff and Thurman, 1984).
We obtained fisheries observer data from around the Chagos Archipelago from the Marine Resources Assessment Group (MRAG) who managed fishing licences in the area prior to the MPA’s establishment (Mees et al., 2009).
To assess the relative abundance of silky sharks around the Chagos Archipelago, we calculated mean nominal catch per unit effort (CPUE—number of sharks caught divided by the number of hooks deployed, multiplied by 1,000) for silky sharks from longline hook survey data (n = 41 sets) between November 2000–January 2003 (equating to ∼1.3% of all longlines set during this period). Equivalent data for purse seine fishing were not available. To quantify the relative contribution of silky sharks to the total catch and total shark catch, the CPUEs of all other major species caught were also calculated.
To characterize silky shark population demographics within the BIOT, we analyzed the fishery observer reports from 116 fishing events between November 1997 to November 2009 (equating to ∼0.7% of all logged longline and purse seine events during this period). For each observed fishing event, data on the sex, weight and fork lengths (FL) of individuals caught were recorded, along with the gear used (“Longline,” “Purse Seine,” or “Unknown”). In order to easily compare these data with published length-at-maturity studies, we converted FL to total length (TL), using the relationship published by Joung et al. (2008) (TL = 1.21 ∗ FL + 2.36). Following a Shapiro-Wilk test for normality, a two-sample t-test was used to compare the sizes of sharks caught by longline and purse seine vessels. Silky sharks were deemed as sexually mature if their TL exceeded 210.0 cm for females and 212.5 cm for males (Joung et al., 2008). A two-proportions Z-test was then carried out to assess whether there was a significant difference in the proportion of immature individuals caught by each gear type (longline versus purse seine).
Six silky sharks (four female, one male and one of unknown sex) were satellite tagged with MiniPATs in the BIOTMPA in February and March 2013 and March 2018 (Table 1). All individuals were immature, except for Shark 3. Four sharks were successfully tracked for durations that ranged from 15 to 270 days with a mean duration of 154 days. These silky sharks showed high variability in individual movement behaviors (Table 2 and Figure 2). We estimate that 77% of Shark 1’s 50% polygon (70,540 km2) and 37% of its 95% polygon (265,391 km2) overlapped with the BIOT MPA. By comparison, 29% of Shark 4’s 50% polygon (155,082 km2) and 12% of its 95% polygon (416,723 km2) overlapped with the BIOT MPA (Supplementary Table 2). Shark 1 headed predominately due east, with the tag popping off in the high-seas, 1,150 km away from the tagging location (Figure 2A). Shark 4 headed predominantly due west, with the tag popping off along the coast of Kenya, 3,549 km away from the tagging location, and having crossed four different EEZs (BIOT, Mauritius, Seychelles and Kenya) (Figure 2A). Conversely, Shark 2 was tracked for 270 days, had an estimated track length of over 3,000 km, yet its total 95% probability surface (53,082 km2) was entirely within the MPA boundary (Table 2, Figure 2B, and Supplementary Table 2). The 95% probability surfaces of Shark 3 and 6 were also entirely within the MPA boundary, but with considerably shorter track durations (Table 2, Figure 2C, and Supplementary Table 2).
Table 2. Satellite tag (MiniPATs, Wildlife Computers) deployments on silky sharks (Carcharhinus falciformis) around the Chagos Archipelago between 2013 and 2018.
Figure 2. Tracks of five silky sharks (Carcharhinus falciformis) tagged around the Chagos Archipelago between 2013 and 2018. Sharks 1, 2, 4, and 6 were tagged with MiniPATs and MLTs were derived using manufacturers geolocation software (GPE3; Wildlife Computers Inc., Redmond, WA, United States), with position estimates interpolated to a 0.025° × 0.025° grid spacing and smoothed with a cubic spline. Speed filters were set to 1.5 m/s for all MLTs. As Shark 3’s MiniPAT did not report, its track was reconstructed from SPOT fixes only. MLTs are those of Shark 1 (Orange) and Shark 4 (Blue) (both A), Shark 2 (Green, B), Shark 3 (Red), and Shark 6 (Purple) (both C). Tagging location is indicated with a white circle, tag pop-up position with a white triangle. Shark 5 (satellite tag did not report) and Shark 7 (acoustic tag only) were tagged at the same location as Sharks 4 and 6. Track color lightens as the track progresses. Polygons represent the merged probability density surfaces for each MiniPAT, with light to dark shades representing 95, 75, and 50% probability contours. The boundary of the British Indian Ocean Territory (BIOT) Marine Protected Area (MPA) is shown with the solid black line. The gray outline depicts islands, atolls and submerged reefs. Underlying bathymetry was obtained from the ETOPO1 database hosted on the NOAA website at 4 min resolution through the ‘marmap’ package in R (R Core Team, 2020).
Acoustically tagged silky sharks were only detected on the three receivers deployed on the Sandes-Swart seamount complex (SS01, SS03 and SS04). All sharks were detected on all three seamount receivers, although principally on receivers SS03 and SS04, that were furthest west (Figures 3A,B). Sharks 4, 5, and 6 were not detected on any receiver after 29, 11, and 23 days, respectively, with Rmin ranging from 0.01 to 0.07 and Rmax ranging from 0.17 to 0.91 (Table 3 and Figure 3A). Shark 7 was at liberty for 326 days, with a Rmin of 0.57 and a Rmax of 0.65 (Table 3). Across the four individuals, average presence rates on any of the seamount receivers peaked at dawn (0.36, 06:30–07:30) and was lowest around midnight (0.002, Supplementary Figure 1). Diel detections varied by receiver. Detections on the receivers SS04 and SS03 predominately occurred during daylight hours, with a drop-off in detections after 19:00 and increasing again after 06:00. Detections on SS01 primarily occurred at night, between 19:00 and 07:00 (Figure 3B). Shark 7 was detected at SS03 on 130 unique days—equivalent to this individual visiting at least once every 2–3 days—suggesting a core use area. The total number of visits to each receiver by each individual ranged from 1 (Shark 6 at SS04) to 270 (Shark 7 at SS03), with a maximum of eight visits to a receiver by an individual within a single day (Supplementary Table 3). With the exception of Shark 6 (lowest residency), the mean time elapsed between consecutive visits (i.e., absence period) by each individual to their most frequented receiver station (SS03) was relatively brief (Shark 4: 0.55 days; Shark 5: 0.28 days; Shark 7: 1.17 days). Mean visit durations at each receiver station were typically brief (overall mean of 16.5 min), and varied by individual, ranging from 5.89 min (Shark 6 on receivers SS03 and SS04) to 30.17 min (Shark 5 on SS04) (Supplementary Table 3). Maximum visit duration was 148.67 min (Shark 4 on receiver SS04); however, visits exceeding 1 h at any receiver station were relatively uncommon, accounting for only 3.90% of all visits. The maximum number of individuals simultaneously visiting the same receiver station was two, and only occurred on six and five occasions at SS03 and SS04, respectively, and lasted between 1.69 to 48.35 min (overall mean 10.49 min).
Figure 3. Acoustic detections of four silky sharks (Carcharhinus falciformis) at the Sandes-Swart seamount complex in the Chagos Archipelago in March 2018. (A) Detections over time for all four individuals. (B) Diel detections for individuals (circle = Shark 4, square = Shark 5, diamond = Shark 6, and triangles = Shark 7) across the full time series. Dark gray shaded areas represent night-time (19:30–06:30), and lighter gray shaded areas represent dawn (06:30–07:30) and dusk (18:30–19:30). Colors represent the receivers the shark was detected on with blue = SS01; red = SS03; and yellow = SS04.
Table 3. Acoustic tag deployments on silky sharks (Carcharhinus falciformis) around the Chagos Archipelago between 2013 and 2018.
Depth and temperature data were recorded by MiniPATs deployed on four sharks (Sharks 1, 2, 4, and 6; Table 1). Tagged individuals spent 99.41 ± 0.20% of their time in the top 100 m of the water column, with the largest proportion of time in depths of 25–50 m (31.13 ± 8.57%), followed by depths of 10–25 m (30.55 ± 5.47%; Figure 4A). All sharks dived to depths greater than 300 m, with a maximum recorded depth of 1112 m reported from Shark 2. Tagged sharks occupied temperatures of 24–30°C for 96.11 ± 1.52% of the deployment period (Figure 4B).
Figure 4. Summarized depth and temperature data recorded by MiniPAT tags deployed on four silky sharks (Carcharhinus falciformis) tagged around the Chagos Archipelago. Histograms represent mean percent time spent at (A) depth and (B) temperature. Error bars indicate ± one standard error.
Time-series data collected at 10- and 2.5-min intervals from Sharks 1 (n = 8 days) and 6 (n = 16 days), respectively, revealed patterns of diel vertical movement and oscillatory diving (Figure 5). Mean depths were significantly deeper in the day than at night (Table 2; Wilcoxon signed rank tests: Shark 1: V = 35, p = 0.02; Shark 6: V = 133, p ≤ 0.01). These two sharks continued to oscillate through the water column between diel phases, spending considerably less time in the surface 5 m during the day and shifting to a shallower distribution at night (Figure 5 and Table 2). We observed seven dives beyond 140 m by Shark 6, with five occurring within an hour of sunrise (∼07:00) or sunset (∼19:00), one at 04:00 and another at 11:00 local time. The median dive duration was 7.5 min. Shark 1 did not undertake any dives beyond 140 m throughout its time at liberty.
Figure 5. MiniPAT depth (blue) and temperature (red) time-series data from tags deployed on two silky sharks (Carcharhinus falciformis) tagged around the Chagos Archipelago. Gray shaded areas represent night-time (19:00–07:00) and white daytime (07:00–19:00). (A) Representative diel vertical movements of shark 1 over 2 days. (B) The entire transmitted depth and temperature series from shark 6.
In the months of November to January between 2000 and 2003, the catch of longliners operating around the Chagos Archipelago was dominated by yellowfin Thunnus albacares (28.33% of catch by number) and bigeye tuna Thunnus obesus (25.91%). Sharks contributed 4.18% of the total catch by number. Furthermore, blue sharks (0.66), pelagic thresher sharks Alopias pelagicus (0.44), and silky sharks (0.34) were the most frequently caught per set. Silky sharks accounted for 14.84% of all sharks identified to species level and 13.69% of all sharks caught (Table 4). Silky sharks were caught at an average catch rate of 0.20 individuals per thousand hooks set (for comparison, the target species of tuna were caught at roughly 10 individuals per thousand hooks). Pelagic thresher sharks were caught at a rate of 0.25 per thousand hooks and blue sharks at a rate of 0.68 per thousand hooks (Table 4).
Table 4. Summary table of the frequency (f), CPUE (Number caught per thousand hooks), standard errors (s.e.), and percentage contributions to catches for tunas and sharks from 41 longline sets surveyed as part of an observer run hook survey between November and February in 2000 to 2003 around the Chagos Archipelago.
Of the 677 sharks reported by fisheries observers between November 1997 and November 2009, 69 were identified as silky sharks and reported as being caught within BIOT. Of all the silky sharks reported, 23 were from longlines (15 female, 7 male and 1 unsexed), 22 from purse seines (12 female and 10 male) and 24 from unrecorded fishing methods (11 female, 8 male and 5 unsexed) (Figure 6). The average length of silky sharks caught around the Chagos Archipelago was 211.12 cm (± 8.01 s.e.), with no statistical difference between sex (females 204.49 ± 10.58 cm; males 205.83 ± 12.82 cm), and 55.88% of individuals being immature juveniles. The average length of silky sharks caught by longlines (211.46 ± 8.01 cm) was significantly larger than individuals caught by purse seines (148.55 ± 6.10 cm) (two sample t-test: t = 5.31, df = 33.96, p < 0.01) and purse seines (100%) caught a significantly higher proportion of juveniles than longlines (54.55%) (Chi-squared = 10.48, df = 1, p < 0.01). Only 16.67% of sharks from unrecorded fishing methods were immature, and all were female (Figure 6).
Figure 6. The size distribution of silky sharks (Carcharhinus falciformis) caught around the Chagos Archipelago between November 1997 and November 2009 by purse seine, longline and unknown gear (left) and of female, male and individuals of an unknown sex caught by all gear types (right). Vertical dashed lines represent the mean lengths recorded by each respective group. Vertical dotted lines represent the estimated total length of maturity of female (210 cm) and male (212 cm) silky sharks (Joung et al., 2008).
Understanding the movement patterns, depth and thermal preferences of vulnerable pelagic sharks can inform efforts to minimize negative fisheries interactions (Queiroz et al., 2016; Hazen et al., 2018). Through analyses of biotelemetry and fisheries data in the central Indian Ocean, we demonstrate the high variability in horizontal movement behavior of silky sharks in the region and consider the ecological significance of the Chagos Archipelago and the MPA that surrounds it. This study advances our understanding of this relatively understudied species in a region where many fish stocks are already heavily depleted and pressure is expected to increase considerably, with nearly half the world’s human population expected to live around the Indian Ocean by 2050 (Doyle, 2018). Our findings contribute toward the understanding and the evaluation of the role that large-scale spatial management strategies, like MPAs, may have in the conservation of mobile pelagic species.
Silky sharks are known to be highly mobile, with previous studies reporting maximum displacement distances of 1,010 km (Hutchinson et al., 2019), 1,339 km (Kohler et al., 1998), 2,200 km (Lara-Lizardi et al., 2020), and even 3,195 km (Schaefer et al., 2019). Two of the sharks tagged within this study exhibited large ranging movements, with Shark 4 (displacement distance 3,549 km, total track distance of 4,782 km) considerably exceeding the published maximum displacement distance for the species. Although both sharks spent considerable periods of time within the BIOT MPA before exiting, such wide-ranging movements suggest large distribution ranges, spanning multiple EEZs and the areas beyond national jurisdiction. This emphasizes the importance of implementing effective fishery management and developing multi-national conservation measures, especially for purse seiners where mortality rates are high, even if sharks are released (Poisson et al., 2014; Hutchinson et al., 2015; Eddy et al., 2016). For example, the use of non-entangling FADs (Franco et al., 2012; Lopez et al., 2017) can reduce the potential of ghost-fishing events (Filmalter et al., 2013) and the introduction of best practice handling and release procedures can reduce post-release mortality (Poisson et al., 2016). Yet, spatial protection can be effective for wide-ranging marine predators even if they do not encapsulate the species’ entire home range, if they contain core habitats supporting key life-history stages, such as breeding, feeding or gestation (Hooker et al., 2011).
The distribution and movement of pelagic predators has been shown to correlate with environmental conditions, such as thermal fronts, chlorophyll-a concentrations and upwelling events (Block et al., 2011; Lezama-Ochoa et al., 2016; Braun et al., 2019; Lopez et al., 2020). The two silky sharks with the largest displacement distances within this study displayed divergent movement trajectories, with one heading east and the other west. It is important to note that these two individuals were tagged at a similar time of the year, although 5 years apart. As such, inter-annual fluctuations of climate and prevailing oceanic conditions, such as the Indian Ocean Dipole (IOD) (Saji et al., 1999), may have played a factor in the direction and nature of their respective movements. When the IOD is positive, upwelling occurs along coasts of Sumatra and Java, increasing primary productivity in the east and decreasing productivity in the west (Marsac, 2008). Interestingly, Shark 1 headed east during a negative IOD phase and Shark 4 headed west during a positive IOD phase, contrary to our expectation that silky sharks would migrate toward more productive areas. Importantly, given our small sample size, differences observed here could be simply attributable to individual variation, especially as divergent longitudinal movements of silky sharks have been observed in individuals tagged at several other locations (Musyl et al., 2011b; Filmalter et al., 2015; Hutchinson et al., 2019). Alternatively, movement behaviors of individuals may have been artificially impacted by association with drifting FADs (Hutchinson et al., 2019), although a recent study demonstrated that at least 30% of juvenile silky shark movements were not associated with surface currents (Bonnin et al., 2020).
Whilst the aforementioned individuals demonstrate the high mobility potential of silky sharks, the majority of the tagging data available for the species indicate far shorter displacement distances and stronger residency behavior (Kohler et al., 1998; Clarke et al., 2011a; Hutchinson et al., 2019). Shark 2’s 270-day maximum likelihood track and 95% probability polygon was entirely within the MPA boundary and around the geographical features of the Chagos Archipelago. Shark 7 also exhibited prolonged residency at the Sandes-Swart seamount complex. The Chagos Archipelago has been previously described as harboring reef fish biomass up to six times higher than elsewhere in the Indian Ocean (Graham et al., 2013) and having high levels of primary production (Hosegood et al., 2019) compared to the surrounding largely oligotrophic ocean (Morel et al., 2010). The archipelago also contains ∼73 seamounts (Yesson et al., 2020) that are hotspots of pelagic biodiversity (Morato et al., 2010; Letessier et al., 2019), and silky sharks are known to frequently use shallow areas adjacent to deep water (Compagno, 1984; Clarke et al., 2011a). As only one adult silky shark was tagged for a limited duration, we cannot rule out that adult sharks may be less resident than the juveniles tracked for longer here. However, the rich resources and varied habitats of the Chagos Archipelago may be providing all the resources required, reducing the need for wide-ranging foraging behavior, especially for juveniles and early life stages. As reducing juvenile mortality has profound positive effects on future population growth (Cortés, 2002; Beerkircher and Shivji, 2003), the exclusion of industrial fisheries, the biggest threat to juvenile silky sharks (Lawson, 2011; Clarke et al., 2018), from the BIOT MPA could be providing considerable benefits for the species. However, this is dependent on effective enforcement and management. The BIOT MPA is threatened by targeted illegal, unreported and unregulated fishing (IUU) vessels, principally from Sri Lanka and India (Clark et al., 2015; Ferretti et al., 2018; Tickler et al., 2019; Jacoby et al., 2020) and FADs still drift through, potentially aggregating biomass and exporting it outside and into fishable waters.
Notwithstanding some spatial overlap, individuals were temporally separated and visits to receiver stations were typically brief and relatively frequent. We observed considerable differences in detection patterns between individuals, ranging from prolonged occupancy across ∼11 months to repeated detections across a shorter period, 11–29 days. Large variation in residency behavior, including prolonged occupancies, have previously been documented amongst a silky shark population in the Red Sea (Clarke et al., 2011a). Shark 7 remained associated with the seamount for nearly an entire year, suggesting a high degree of fidelity or philopatry to this feature. Seamounts have been previously demonstrated as important sites for pelagic sharks (Morato et al., 2010, 2016; Letessier et al., 2019) and oceanographic observations of the Sandes-Swart seamount complex have attributed the aggregations of predators at its summit to the accumulation of biomass induced by the presence and formation of internal lee waves (Hosegood et al., 2019). Conversely, the relatively high Rmax and low Rmin indices in Sharks 4 and 5, suggest intense use of these features during short time periods. Sharks may therefore be utilizing the seamounts as meeting or feeding points intermittently during larger movements. Such association behavior is also consistent with silky shark interactions with FADs, which they typically associate with for between 15 and 30 days (Filmalter et al., 2015; Hutchinson et al., 2019). Importantly, we recognize that tag failure or mortality can also cause the subsequent lack of detections and is a limitation of acoustic data. We also note that, despite acoustic receivers being located throughout the archipelago (Carlisle et al., 2019), silky sharks were only detected on the receivers located on Sandes-Swart seamount complex, close to where they were tagged. However, as the next nearest receivers were located at Egmont Atoll, over 90 km to the north west, we cannot rule out that silky sharks were using other shallow features nearer by, such as Diego Garcia, the Great Chagos Bank or Pitt Bank, that were not monitored by acoustic receivers during this study.
There were clear diel variations in the detections of silky sharks at the seamount. Consistent detections on the seamount during the day and absences at night are in parallel to silky shark behavior observed around FADs, where night-time absences were attributed to feeding excursions (Filmalter et al., 2015). Juvenile silky sharks may therefore be behaving as central place foragers around this feature, as has been demonstrated in reef sharks around Palmyra Atoll in the Pacific (Papastamatiou et al., 2018), during the duration of their association.
We report that tagged silky sharks displayed patterns of diel vertical migration, oscillatory diving behavior and spent > 99% of their time in the top 100 m of the water column, while also diving to depths of greater than 300 m. Our findings are therefore broadly consistent with observations of silky sharks in the Pacific (Musyl et al., 2011b; Hutchinson et al., 2019). Yet, we also observed much deeper dives, with one individual recorded to a depth of 1,112 m, the deepest recorded dive for the species (previously reported at 640 m; Hueter et al., 2018). The vertical distribution and diving behavior of pelagic predatory fishes is typically driven by the need to optimize prey encounter rates and energy expenditure, while remaining within preferred environmental conditions (i.e., temperature, oxygen) (Carey et al., 1990; Klimley et al., 2002; Meyer et al., 2009; Andrzejaczek et al., 2019). Consistent with studies in the Pacific (Hutchinson et al., 2019), Atlantic (Hueter et al., 2018), and western Indian Ocean (Filmalter et al., 2015), silky shark diving depths were, on average, shallower at night than during the day, but deeper dives were generally associated with local dawn and dusk. We interpret this to be foraging behavior, as mesopelagic species are a regular occurrence in the diet of Indian Ocean silky sharks (Filmalter et al., 2017). Overall, the vertical niche occupied by silky sharks in this study overlaps directly with the hanging depth of purse seine nets (up to 200 m) in the Indian Ocean (Kaplan et al., 2014), putting them at high risk of capture should they leave the protection of the MPA. However, typical depths of longline hooks set in the Indian Ocean for yellowfin or bigeye are deeper, between 100–250 m and 100–400 m, respectively (Nishida et al., 2003). As such, there is less direct vertical overlap with longlines, although sharks may undertake deeper vertical movements if attracted to baited hooks, as they are frequently caught by longliners in the Indian Ocean (Huang and Liu, 2010).
It has been hypothesized that pelagic shark species display thermal (Musyl et al., 2011b) and vertical niche partitioning (Choy et al., 2009). Previous studies have shown silky sharks residing in waters within 2°C of the surface water temperature in the Red Sea (Clarke et al., 2011a), but being limited to water temperatures above 23°C (Musyl et al., 2011b) and occupying water temperatures between 24 and 29°C in the Pacific (Hutchinson et al., 2019). Similarly, individuals tagged around the Chagos Archipelago occupied the 24–30°C thermal band for 96% of their time at liberty. Thermal niche is coupled with horizontal and vertical distributions and is temporally and spatially variable (Musyl et al., 2011a). The interannual variability in sea surface temperature driven by climatic oscillations, such as the IOD (Saji et al., 1999), could therefore drive temporal variation in vertical habitat use. This may explain the variation in mean diel depths between the two sharks reporting time-series data tagged in similar months, though 5 years apart, although more tagging data is needed in this area to investigate this hypothesis further.
Analysis of the historical fisheries data indicated that silky sharks were a significant component of the pelagic shark community around the Chagos Archipelago between November 2000 and February 2003, prior to the MPA’s establishment. Furthermore, the vast majority of individuals caught were juvenile, indicating that the MPA may be an important area for silky sharks during early life stages. Silky sharks contributed 0.6% of total longline catch. This is broadly consistent with those observed across the Indian Ocean by longlines targeting bigeye tuna, although considerably lower than those targeting yellowfin tuna (7.5%) (Huang and Liu, 2010). This is primarily driven by variations in fishing method, such as hook depth, which is increased when targeting bigeye tuna and can lower silky shark catch by a factor of 6.4 (Gilman et al., 2008). Unfortunately, no data were available on hook depth, nor other fishing method variations, such as bait selection, soak time or deployment time, which are also known to influence catch rates and composition (Romanov, 2002). However, as yellowfin tuna were the dominant catch, we can assume some lines were targeted at this species and therefore silky shark catches were lower than expected. These are likely attributable to the ban on wire leaders in BIOT in 1999 (Dunne et al., 2014), a measure previously shown to reduce the silky shark catch rates by ∼40% (Ward et al., 2008), or the prohibition of fishing within 12 nautical miles of the islands, atolls and reefs of the archipelago (Dunne et al., 2014), as shallow coastal habitats can be an important habitat for juvenile silky sharks (Branstetter, 1987; Bonfil, 1997).
The fisheries observer data represent the best available information on historic pelagic shark communities for the territory, yet there are important considerations when making inferences given their limited coverage. For example, we cannot rule out seasonal variation in silky shark catch rates. The longline hook survey only spanned three years and all sets surveyed were carried out between November and January, the traditional peak fishing season around the Chagos Archipelago (Dunn and Curnick, 2019; Curnick et al., 2020), when migrating tuna are thought to pass through the area (Pearce, 1996). This may in turn lead to increased catch rates of pelagic predators during this time, especially silky sharks who associate with these target species. Species specific shark catches, however, were not routinely recorded in logbooks, despite the IOTC requesting their inclusion in 2005 due to limited shark-specific data (Molina et al., 2005). As such, it is difficult to ascertain whether the patterns seen in the observer data, given the relatively low number of lines surveyed and uneven sampling, were truly representative of catches by the fishery. Further, no quantitative survey data were available for purse seiners in BIOT, where silky shark bycatch is higher (Molina et al., 2005; González et al., 2007), especially for those using FADs (Amandè et al., 2008; Watson et al., 2009; Gilman, 2011).
Within a relatively small sample size, we observed very distinct spatial tracks with a large degree of variation between individuals. Such intra-species variation has been previously noted in silky sharks (Filmalter et al., 2015) and other elasmobranch species, such as the reef manta ray (Mobula alfredi) (Andrzejaczek et al., 2020) and Galapagos shark (Carcharhinus galapagensis) (Lowe et al., 2006). We advocate that an extensive tagging program with deployments across multiple sexes, life stages, regions and seasons is urgently needed to confirm whether behaviors observed here are consistent through time and ontogeny and are representative of the Indian Ocean population. Such data are crucial to inform future stock assessments for the species that are currently uncertain and data-poor, resulting in management advice being unclear (IOTC, 2017). Further, such data could support the development of adaptive spatial management or dynamic ocean management (Lopez et al., 2020), which are seen as promising tools to improve the conservation and management of these species, and are being considered by several fisheries management organizations (Hobday et al., 2013; Maxwell et al., 2015; Hazen et al., 2018; Welch et al., 2019).
We provide intriguing insights into the behavior of silky sharks in the central Indian Ocean, documenting the furthest displacement distance and deepest dive for the species, yet also demonstrating high site fidelity and prolonged residency. However, given the small number of animals tracked and high variability observed, further investigations are required to confirm how silky sharks found in the MPA are connected to the wider Indian Ocean, whether their behaviors are consistent through time and ontogeny, and what environmental conditions drive these behaviors. We also highlight the value of combining telemetry studies with historical fisheries analyses, and the importance of broadening elasmobranch research to understudied species in data-poor regions. High site fidelity to geographic features and predictable utilization of them by juveniles, as observed here, suggests that well enforced protection in key areas could be an effective conservation strategy for the species. We conclude that spatial protection should be a component of a holistic management strategy for the species, alongside fisheries regulations and conservation measures in adjacent EEZs and in areas beyond national jurisdiction.
The datasets presented in this study can be found in online repositories. The names of the repository/repositories and accession number(s) can be found below: https://www.gtopp.org/ or requested from the corresponding author.
This animal study was reviewed and approved by the Stanford University Administrative Panel on Laboratory Animal Care (APLAC) under permit APLAC-10765 and the Zoological Society of London’s ethics committee under permit 186-BME-0652.
DJC, DMPJ, BAB, HJK, and BC secured the funding for the program. DJC and BC conceived the manuscript. DJC, DMPJ, ABC, TKC, FF, RJS, and TW collected the field data. DJC, SA, DMPJ, and DMC analyzed the data and drafted the manuscript, with input from all other co-authors. All authors, except for BC who sadly passed away during the preparation of the manuscript, read and approved the final manuscript.
This work was funded by the Bertarelli Foundation through the Bertarelli Programme in Marine Science, the Zoological Society of London, and a University College London Impact Award. Additional funding for tags was provided by the JSF Pollitzer Charitable Trust, The Rufford Foundation and the Ernest Kleinwort Charitable Trust through the Chagos Conservation Trust.
The authors declare that the research was conducted in the absence of any commercial or financial relationships that could be construed as a potential conflict of interest.
This manuscript is dedicated to co-author, friend, and mentor Dr. Ben Collen. We would like to thank the MRAG for the provision of the fisheries data used within the study. We would also like to extend our thanks to the various ship captains and crews who supported the deployment and retrieval of our equipment, often in challenging circumstances. We would also like to thank the three reviewers for their thorough and constructive reviews.
The Supplementary Material for this article can be found online at: https://www.frontiersin.org/articles/10.3389/fmars.2020.596619/full#supplementary-material
Aldana-Moreno, A., Hoyos-Padilla, E. M., González-Armas, R., Galván-Magaña, F., Hearn, A., Klimley, A. P., et al. (2020). Residency and diel movement patterns of the endangered scalloped hammerhead Sphyrna lewini in the Revillagigedo National Park. J. Fish Biol. 96, 543–548. doi: 10.1111/jfb.14239
Amandè, M. J., Ariz, J., Chassot, E., de Molina, A. D., Gaertner, D., Murua, H., et al. (2010). Bycatch of the European purse seine tuna fishery in the Atlantic Ocean for the 2003–2007 period. Aquat. Living Resour. 23, 353–362. doi: 10.1051/alr/2011003
Amandè, M. J., Chassot, E., Chavance, P., and Pianet, R. (2008). Silky Shark (Carcharhinus falciformis) Bycatch in the French Tuna Purse-Seine Fishery of the Indian Ocean (Seychelles: IOTC), 22p.
Amante, C., and Eakins, B. W. (2009). ETOPO1 1 Arc-Minute Global Relief Model: Procedures, Data Sources and Analysis. NOAA Technical Memorandum NESDIS NGDC-24. Washington, DC: NOAA, doi: 10.1594/PANGAEA.769615
Andrzejaczek, S., Chapple, T., Curnick, D., Carlisle, A., Castleton, M., Jacoby, D., et al. (2020). Individual variation in residency and regional movements of reef manta rays Mobula alfredi in a large marine protected area. Mar. Ecol. Prog. Ser. 639, 137–153. doi: 10.3354/meps13270
Andrzejaczek, S., Gleiss, A. C., Pattiaratchi, C. B., and Meekan, M. G. (2019). Patterns and drivers of vertical movements of the large fishes of the epipelagic. Rev. Fish Biol. Fish. 29, 335–354. doi: 10.1007/s11160-019-09555-1
Baum, J. K., Myers, R. A., Kehler, D. G., Worm, B., Harley, S. J., and Doherty, P. A. (2003). Collapse and conservation of shark populations in the Northwest Atlantic. Science 299, 389–392. doi: 10.1126/science.1079777
Beerkircher, L., and Shivji, M. S. (2003). A Monte Carlo demographic analysis of the silky shark (Carcharhinus falciformis): implications of gear selectivity. Fish. Bull. 101:168.
Block, B., Jonsen, I., Jorgensen, S., Winship, A., Shaffer, S., Bograd, S. J., et al. (2011). Tracking apex marine predator movements in a dynamic ocean. Nature 475, 86–90. doi: 10.1038/nature10082
Boerder, K., Schiller, L., and Worm, B. (2019). Not all who wander are lost: improving spatial protection for large pelagic fishes. Mar. Policy 105, 80–90. doi: 10.1016/j.marpol.2019.04.013
Bonfil, R. (1997). Status of shark resources in the Southern Gulf of Mexico and Caribbean: implications for management. Fish. Res. 29, 101–117. doi: 10.1016/S0165-7836(96)00536-X
Bonfil, R. (2008). “The biology and ecology of the silky shark, Carcharhinus falciformis,” in Sharks of the Open Ocean: Biology, Fisheries and Conservation, eds M. D. Camhi, E. K. Pikitch, and E. A. Babcock (Hoboken, NJ: Wiey), 114–127. doi: 10.1002/9781444302516.ch10
Bonnin, L., Lett, C., Dagorn, L., Filmalter, J. D., Forget, F., Verley, P., et al. (2020). Can drifting objects drive the movements of a vulnerable pelagic shark? Aquat. Conserv. Mar. Freshw. Ecosyst. doi: 10.1002/aqc.3420 [Epub ahead of print].
Branstetter, S. (1987). Age, growth and reproductive biology of the silky shark, Carcharhinus falciformis, and the scalloped hammerhead, Sphyrna lewini, from the northwest Gulf of Mexico. Environ. Biol. Fishes 19, 161–173. doi: 10.1007/BF00005346
Braun, C. D., Gaube, P., Sinclair-Taylor, T. H., Skomal, G. B., and Thorrold, S. R. (2019). Mesoscale eddies release pelagic sharks from thermal constraints to foraging in the ocean twilight zone. Proc. Natl. Acad. Sci. U.S.A. 116, 17187. doi: 10.1073/pnas.1903067116
Camhi, M. (2009). The Conservation Status of Pelagic Sharks and Rays: Report of the IUCN Shark Specialist Group Pelagic Shark Red List Workshop. Oxford: University of Oxford.
Carey, F. G., Scharold, J. V., and Kalmijn, A. J. (1990). Movements of blue sharks (Prionace glauca) in depth and course. Mar. Biol. 106, 329–342. doi: 10.1007/BF01344309
Carlisle, A. B., Tickler, D., Dale, J. J., Ferretti, F., Curnick, D. J., Chapple, T. K., et al. (2019). Estimating space use of mobile fishes in a large marine protected area with methodological considerations in acoustic array design. Front. Mar. Sci. 6:256. doi: 10.3389/fmars.2019.00256
Chapman, D. D., Pikitch, E. K., Babcock, E., and Shivji, M. S. (2005). marine reserve design and evaluation using automated acoustic telemetry: a case-study involving coral reef-associated sharks in the mesoamerican caribbean. Mar. Technol. Soc. J. 39, 42–55. doi: 10.4031/002533205787521640
Choy, C. A., Popp, B. N., Kaneko, J. J., and Drazen, J. C. (2009). The influence of depth on mercury levels in pelagic fishes and their prey. Proc. Natl. Acad. Sci. U.S.A. 106, 13865–13869. doi: 10.1073/pnas.0900711106
Clark, M. J., Duffy, H., Pearce, J., and Mees, C. (2015). Update on the Catch and Bycatch Composition of Illegal Fishing in the British Indian Ocean Territory (BIOT) and A Summary of Abandoned and Lost Fishing. London: MRAG Ltd.
Clarke, C., Lea, J. S. E., and Ormond, R. F. G. (2011a). Reef-use and residency patterns of a baited population of silky sharks, Carcharhinus falciformis, in the Red Sea. Mar. Freshw. Res. 62, 668–675. doi: 10.1071/MF10171
Clarke, S., Harley, S., Hoyle, S., and Rice, J. (2011b). An Indicator-Based Analysis of Key Shark Species Based on Data Held by SPC-OFP. Kolonia: Western Central Pacific Fisheries Commission.
Clarke, S., Langley, A., Lennert-Cody, C. E., Aires-da-Silva, A., and Maunder, M. N. (2018). Pacific-wide Silky Shark (Carcharhinus falciformis) Stock Status Assessment. Available online at: https://www.wcpfc.int/file/216441/download?token=wBsGzwyd (accessed July 15, 2020).
Cliff, G., and Thurman, G. D. (1984). Pathological and physiological effects of stress during capture and transport in the juvenile dusky shark, Carcharhinus obscurus. Comp. Biochem. Physiol. Part A Physiol. 78, 167–173. doi: 10.1016/0300-9629(84)90111-7
Cochran, J. E. M., Braun, C. D., Fernando Cagua, E., Campbell, M. F., Hardenstine, R. S., Kattan, A., et al. (2019). Multi-method assessment of whale shark (Rhincodon typus) residency, distribution, and dispersal behavior at an aggregation site in the Red Sea. PLoS One 14:e0222285. doi: 10.1371/journal.pone.0222285
Compagno, L. J. (1984). Sharks of the world. FAO Species Cat. Fish. Purp. 4, 251–255. doi: 10.1016/j.neuropharm.2006.07.020
Cortés, E. (2002). Incorporating uncertainty into demographic modeling: application to shark populations and their conservation. Conserv. Biol. 16, 1048–1062. doi: 10.1046/j.1523-1739.2002.00423.x
Curnick, D. J., Collen, B., Koldewey, H. J., Jones, K. E., Kemp, K. M., and Ferretti, F. (2020). Interactions between a large Marine Protected Area, pelagic tuna and associated fisheries. Front. Mar. Sci. 7:318. doi: 10.3389/fmars.2020.00318
De Santo, E. M., Jones, P. J. S., and Miller, A. M. M. (2011). Fortress conservation at sea: a commentary on the Chagos marine protected area. Mar. Policy 35, 258–260. doi: 10.1016/j.marpol.2010.09.004
Doherty, P. D., Baxter, J. M., Gell, F. R., Godley, B. J., Graham, R. T., Hall, G., et al. (2017). Long-term satellite tracking reveals variable seasonal migration strategies of basking sharks in the north-east Atlantic. Sci. Rep. 7:42837. doi: 10.1038/srep42837
Doyle, T. (2018). Blue economy and the Indian Ocean Rim. J. Indian Ocean Reg. 14, 1–6. doi: 10.1080/19480881.2018.1421450
Dulvy, N. K., Fowler, S. L., Musick, J. A., Cavanagh, R. D., Kyne, P. M., Harrison, L. R., et al. (2014). Extinction risk and conservation of the world’s sharks and rays. eLife 3:e00590. doi: 10.7554/eLife.00590
Dunn, N., and Curnick, D. (2019). Using historical fisheries data to predict tuna distribution within the British Indian Ocean Territory Marine Protected Area, and implications for its management. Aquat. Conserv. Mar. Freshw. Ecosyst. 29, 2057–2070. doi: 10.1002/aqc.3204
Dunne, R. P., Polunin, N. V. C., Sand, P. H., and Johnson, M. L. (2014). The creation of the Chagos marine protected area: a fisheries perspective. Adv. Mar. Biol. 69, 79–127. doi: 10.1016/B978-0-12-800214-8.00003-7
Eddy, C., Brill, R., and Bernal, D. (2016). Rates of at-vessel mortality and post-release survival of pelagic sharks captured with tuna purse seines around drifting fish aggregating devices (FADs) in the equatorial eastern Pacific Ocean. Fish. Res. 174, 109–117. doi: 10.1016/j.fishres.2015.09.008
Ferretti, F., Curnick, D., Liu, K., Romanov, E. V., and Block, B. A. (2018). Shark baselines and the conservation role of remote coral reef ecosystems. Sci. Adv. 4:eaaq0333. doi: 10.1126/sciadv.aaq0333
Ferretti, F., Worm, B., Britten, G. L., Heithaus, M. R., and Lotze, H. K. (2010). Patterns and ecosystem consequences of shark declines in the ocean. Ecol. Lett. 13, 1055–1071. doi: 10.1111/j.1461-0248.2010.01489.x
Filmalter, J., Cowley, P., Forget, F., and Dagorn, L. (2015). Fine-scale 3-dimensional movement behaviour of silky sharks Carcharhinus falciformis associated with fish aggregating devices (FADs). Mar. Ecol. Prog. Ser. 539, 207–223. doi: 10.3354/meps11514
Filmalter, J. D., Capello, M., Deneubourg, J. L., Cowley, P. D., and Dagorn, L. (2013). Looking behind the curtain: quantifying massive shark mortality in fish aggregating devices. Front. Ecol. Environ. 11:291–296. doi: 10.1890/130045
Filmalter, J. D., Cowley, P. D., Potier, M., Ménard, F., Smale, M. J., Cherel, Y., et al. (2017). Feeding ecology of silky sharks Carcharhinus falciformis associated with floating objects in the western Indian Ocean. J. Fish Biol. 90, 1321–1337. doi: 10.1111/jfb.13241
Filmalter, J. D., Dagorn, L., Cowley, P. D., and Taquet, M. (2011). First descriptions of the behavior of silky sharks, Carcharhinus falciformis, around drifting fish aggregating devices in the Indian Ocean. Bull. Mar. Sci. 87, 325–337. doi: 10.5343/bms.2010.1057
Filmalter, J. D., Dagorn, L., and Soria, M. (2010). Double Tagging Juvenile Silky Sharks to Improve Our Understanding of the Behavioural Ecology: Preliminary Results. Seychelles: Indian Ocean Tuna Commission.
Franco, J., Moreno, G., López, J., and Sancristobal, I. (2012). Testing new designs of drifting fish aggregating device (DFAD) in the Eastern Atlantic to reduce turtle and shark mortality. Collect. Vol. Sci. Pap. ICCAT 68, 1754–1762.
Game, E. T., Grantham, H. S., Hobday, A. J., Pressey, R. L., Lombard, A. T., Beckley, L. E., et al. (2009). Pelagic protected areas: the missing dimension in ocean conservation. Trends Ecol. Evol. 24, 360–369. doi: 10.1016/j.tree.2009.01.011
Gilman, E., Clarke, S., Brothers, N., Alfaro-Shigueto, J., Mandelman, J., Mangel, J., et al. (2008). Shark interactions in pelagic longline fisheries. Mar. Policy 32, 1–18. doi: 10.1016/j.marpol.2007.05.001
Gilman, E. L. (2011). Bycatch governance and best practice mitigation technology in global tuna fisheries. Mar. Policy 35, 590–609. doi: 10.1016/j.marpol.2011.01.021
González, I., Ruiz, J., Moreno, G., Murua, H., and Artetxe, I. (2007). AZTI Discard sampling programme in the spanish purse-seiner fleet in the Western Indian Ocean (2003–2006). Seychelles: Indian Ocean Tuna Commission.
Graham, N. A. J., Pratchett, M. S., McClanahan, T. R., and Wilson, S. K. (2013). “The status of coral reef fish assemblages in the chagos archipelago, with implications for protected area management and climate change,” in Coral Reefs of the United Kingdom Overseas Territories, ed. C. Sheppard (Berlin: Springer), 253–270. doi: 10.1007/978-94-007-5965-7_19
Hazen, E. L., Scales, K. L., Maxwell, S. M., Briscoe, D. K., Welch, H., Bograd, S. J., et al. (2018). A dynamic ocean management tool to reduce bycatch and support sustainable fisheries. Sci. Adv. 4, eaar3001. doi: 10.1126/sciadv.aar3001
Hazin, F., Oliveira, P., and Macena, B. (2007). Aspects of the Reproductive Biology of the Silky Shark, Carcharhinus falciformis (Nardo, 1827), in the vicinity of Archipelago of Saint Peter and Saint Paul, in the equatorial Atlantic Ocean. Available online at: http://www.iccat.int/documents/cvsp/cv060_2007/no_2%5CCV060020648.pdf (accessed January 11, 2016).
Henderson, A. C., McIlwain, J. L., Al-Oufi, H. S., Al-Sheile, S., and Al-Abri, N. (2009). Size distributions and sex ratios of sharks caught by Oman’s artisanal fishery. Afr. J. Mar. Sci. 31, 233–239. doi: 10.2989/AJMS.2009.31.2.11.883
Hobday, A. J., Young, J. W., Abe, O., Costa, D. P., Cowen, R. K., Evans, K., et al. (2013). Climate impacts and oceanic top predators: moving from impacts to adaptation in oceanic systems. Rev. Fish Biol. Fish. 23, 537–546. doi: 10.1007/s11160-013-9311-0
Hooker, S. K., Cañadas, A., Hyrenbach, K. D., Corrigan, C., Polovina, J. J., and Reeves, R. R. (2011). Making protected area networks effective for marine top predators. Endanger. Species Res. 13, 203–218. doi: 10.3354/esr00322
Hosegood, P. J., Nimmo-Smith, W. A. M., Proud, R., Adams, K., and Brierley, A. S. (2019). Internal lee waves and baroclinic bores over a tropical seamount shark ‘hot-spot.’. Prog. Oceanogr. 172, 34–50. doi: 10.1016/j.pocean.2019.01.010
Huang, H. W., and Liu, K. M. (2010). Bycatch and discards by Taiwanese large-scale tuna longline fleets in the Indian Ocean. Fish. Res. 106, 261–270. doi: 10.1016/j.fishres.2010.08.005
Hueter, R. E., Tyminski, J. P., Pina-Amargós, F., Morris, J. J., Abierno, A. R., Valdés, J. A. A., et al. (2018). Movements of three female silky sharks (Carcharhinus falciformis) as tracked by satellite-linked tags off the Caribbean coast of Cuba. Bull. Mar. Sci. 94, 345–358. doi: 10.5343/bms.2017.1162
Hutchinson, M., Coffey, D. M., Holland, K., Itano, D., Leroy, B., Kohin, S., et al. (2019). Movements and habitat use of juvenile silky sharks in the Pacific Ocean inform conservation strategies. Fish. Res. 210, 131–142. doi: 10.1016/j.fishres.2018.10.016
Hutchinson, M., Itano, D., Muir, J., and Holland, K. (2015). Post-release survival of juvenile silky sharks captured in a tropical tuna purse seine fishery. Mar. Ecol. Prog. Ser. 521, 143–154. doi: 10.3354/meps11073
IOTC (2017). Status of the Indian Ocean silky shark (FAL: Carcharhinus falciformis). Victoria: IOTC, doi: 10.5281/zenodo.3263244
Jacoby, D. M. P., Ferretti, F., Freeman, R., Carlisle, A. B., Chapple, T. K., Curnick, D. J., et al. (2020). Shark movement strategies influence poaching risk and can guide enforcement decisions in a large, remote marine protected area. J. Appl. Ecol. 57, 1782–1792. doi: 10.1111/1365-2664.13654
Jorgensen, S. J., Reeb, C. A., Chapple, T. K., Anderson, S., Perle, C., Van Sommeran, S. R., et al. (2010). Philopatry and migration of Pacific white sharks. Proc. Biol. Sci. 277, 679–688. doi: 10.1098/rspb.2009.1155
Joung, S. J., Chen, C. T., Lee, H. H., and Liu, K. M. (2008). Age, growth, and reproduction of silky sharks, Carcharhinus falciformis, in northeastern Taiwan waters. Fish. Res. 90, 78–85. doi: 10.1016/j.fishres.2007.09.025
Kaplan, D. M., Chassot, E., Amandé, J. M., Dueri, S., Demarcq, H., Dagorn, L., et al. (2014). Spatial management of Indian Ocean tropical tuna fisheries: potential and perspectives. ICES J. Mar. Sci. 71, 1728–1749. doi: 10.1093/icesjms/fst233
Kessel, S. T., and Hussey, N. E. (2015). Tonic immobility as an anaesthetic for elasmobranchs during surgical implantation procedures. Can. J. Fish. Aquat. Sci. 72, 1287–1291. doi: 10.1139/cjfas-2015-0136
Klimley, P. A., Beavers, S. C., Curtis, T. H., and Jorgensen, S. J. (2002). Movements and swimming behavior of three species of sharks in La Jolla Canyon, California. Environ. Biol. Fishes 63, 117–135. doi: 10.1023/A:1014200301213
Kohler, N. E., Casey, J. G., and Turner, P. A. (1998). NMFS cooperative shark tagging program, 1962-93: an atlas of shark tag and recapture data. Mar. Fish. Rev. 60, 1–87. doi: 10.7755/mfr.81.2.1
Koldewey, H. J., Curnick, D., Harding, S., Harrison, L. R., and Gollock, M. (2010). Potential benefits to fisheries and biodiversity of the Chagos Archipelago/British Indian Ocean Territory as a no-take marine reserve. Mar. Pollut. Bull. 60, 1906–1915. doi: 10.1016/j.marpolbul.2010.10.002
Kranstauber, B., and Smolla, M. (2015). move: Visualizing and Analyzing Animal Track Data. Available online at: http://cran.r-project.org/package=move (accessed January 11, 2016).
Lara-Lizardi, F., Hoyos-Padilla, M., Hearn, A., Klimley, A. P., Galván-Magaña, F., Arauz, R., et al. (2020). Shark movements in the Revillagigedo Archipelago and connectivity with the Eastern Tropical Pacific. bioRxiv[Preprint] doi: 10.1101/2020.03.02.972844
Lawson, T. (2011). Estimation of Catch Rates and Catches of Key Shark Species in Tuna Fisheries of the Western and Central Pacific Ocean Using Observer Data. Western and Central Pacific Fisheries Commission (WCPFC). Available online at: http://www.wcpfc.int/system/files/EB-IP-02 [Estimation of Catch Rates and Catches of Key Shark Species].pdf (accessed January 11, 2016).
Lea, J. S. E., Wetherbee, B. M., Queiroz, N., Burnie, N., Aming, C., Sousa, L. L., et al. (2015). Repeated, long-distance migrations by a philopatric predator targeting highly contrasting ecosystems. Sci. Rep. 5:11202. doi: 10.1038/srep11202
Letessier, T. B., Mouillot, D., Bouchet, P. J., Vigliola, L., Fernandes, M. C., Thompson, C., et al. (2019). Remote reefs and seamounts are the last refuges for marine predators across the IndoPacific. PLoS Biol. 17:e3000366. doi: 10.1371/journal.pbio.3000366
Lezama-Ochoa, N., Murua, H., Chust, G., Van Loon, E., Ruiz, J., Hall, M., et al. (2016). Present and future potential habitat distribution of Carcharhinus falciformis and Canthidermis maculata by-catch species in the tropical tuna purse-seine fishery under climate change. Front. Mar. Sci. 3:34. doi: 10.3389/fmars.2016.00034
Lopez, J., Alvarez-Berastegui, D., Soto, M., and Murua, H. (2020). Using fisheries data to model the oceanic habitats of juvenile silky shark (Carcharhinus falciformis) in the tropical eastern Atlantic Ocean. Biodivers. Conserv. 29, 2377–2397. doi: 10.1007/s10531-020-01979-7
Lopez, J., Goñi, N., Arregi, I., Ruiz, J., Krug, I., Murua, H., et al. (2017). Main Results of the Spanish Best Practices Program: Evolution of the Use of Non-Entangling FADs, Interaction with Entangled Animals, and Fauna Release Operations. IOTC–2017–WGFAD01–11. Seychelles: IOTC.
Lowe, C. G., Wetherbee, B. M., and Meyer, C. G. (2006). Using acoustic telemetry monitoring techniques to quantity movement patterns and site fidelity of sharks and giant trevally around French frigate shoals and midway atoll. Atoll Res. Bull. 543, 281–303.
Marsac, F. (2008). Outlook of Ocean Climate Variability in the West tropical Indian Ocean, 1997–2008. Seychelles: Indian Ocean Tuna Commission.
Maxwell, S. M., Hazen, E. L., Lewison, R. L., Dunn, D. C., Bailey, H., Bograd, S. J., et al. (2015). Dynamic ocean management: defining and conceptualizing real-time management of the ocean. Mar. Policy 58, 42–50. doi: 10.1016/j.marpol.2015.03.014
Mees, C., Pearce, J., Clarke, J., and Wilson, O. (2009). UK (Chagos/BIOT) National Report. IOTC Twelfth Session of the Scientific Committee Mahé. Seychelles: IOTC.
Meyer, C. G., Anderson, J. M., Coffey, D. M., Hutchinson, M. R., Royer, M. A., and Holland, K. N. (2018). Habitat geography around Hawaii’s oceanic islands influences tiger shark (Galeocerdo cuvier) spatial behaviour and shark bite risk at ocean recreation sites. Sci. Rep. 8, 1–18. doi: 10.1038/s41598-018-23006-0
Meyer, C. G., Clark, T. B., Papastamatiou, Y. P., Whitney, N. M., and Holland, K. N. (2009). Long-term movement patterns of tiger sharks Galeocerdo cuvier in Hawaii. Mar. Ecol. Prog. Ser. 381, 223–235. doi: 10.3354/meps07951
Molina, A. D. D., Ariz, J., Sarralde, R., de Molina, A. D., Pallarés, P., and Santana, J. C. (2005). Activity of the Spanish Purse Seine Fleet in the Indian Ocean and by-Catch Data Obtained from Observer Programmes Conducted in 2003 and 2004. Seychelles: IOTC.
Morato, T., Hoyle, S. D., Allain, V., and Nicol, S. J. (2010). Seamounts are hotspots of pelagic biodiversity in the open ocean. Proc. Natl. Acad. Sci. U.S.A. 107, 9707–9711. doi: 10.1073/pnas.0910290107
Morato, T., Miller, P. I., Dunn, D. C., Nicol, S. J., Bowcott, J., and Halpin, P. N. (2016). A perspective on the importance of oceanic fronts in promoting aggregation of visitors to seamounts. Fish Fish. 17, 1227–1233. doi: 10.1111/faf.12126
Morel, A., Claustre, H., and Gentili, B. (2010). The most oligotrophic subtropical zones of the global ocean: similarities and differences in terms of chlorophyll and yellow substance. Biogeosciences 7:3139. doi: 10.5194/bg-7-3139-2010
Muir, J., Itano, D., Hutchinson, M., Leroy, B., and Holland, K. (2012). Behavior of Target and Non-Target Species on Drifting FADs and When Encircled by Purse Seine Gear. Busan: Western and Central Pacific Fisheries Commission-Scientific Committee.
Musyl, M. K., Brill, R. W., Boggs, C. H., Curran, D. S., Kazama, T. K., and Seki, M. P. (2003). Vertical movements of bigeye tuna (Thunnus obesus) associated with islands, buoys, and seamounts near the main Hawaiian Islands from archival tagging data. Fish. Oceanogr. 12, 152–169. doi: 10.1046/j.1365-2419.2003.00229.x
Musyl, M. K., Brill, R. W., Curran, D. S., Fragoso, N. M., McNaughton, L. M., Nielsen, A., et al. (2011a). Postrelease survival, vertical and horizontal movements, and thermal habitats of five species of pelagic sharks in the central Pacific Ocean. Fish. Bull. 109, 341–368. doi: 10.1186/1471-2199-9-107
Musyl, M. K., Domeier, M. L., Nasby-Lucas, N., Brill, R. W., McNaughton, L. M., Swimmer, J. Y., et al. (2011b). Performance of pop-up satellite archival tags. Mar. Ecol. Prog. Ser. 433, 1–28. doi: 10.3354/meps09202
Musyl, M. K., and Gilman, E. L. (2018). Post-release fishing mortality of blue (Prionace glauca) and silky shark (Carcharhinus falciformis) from a Palauan-based commercial longline fishery. Rev. Fish Biol. Fish. 28, 567–586. doi: 10.1007/s11160-018-9517-2
Nishida, T., Bigelow, K., Mohri, M., and Marsac, F. (2003). Comparative Study on Japanese Tuna Longline Cpue Standardization of Yellowfin Tuna (Thunnus Albacares) in the Indian Ocean Based on Two Methods: - General Linear Model (Glm) and Habitat-Based Model (Hbm)/Glm Combined (Seychelles: IOTC), 6, 48–69.
Papastamatiou, Y. P., Watanabe, Y. Y., Demšar, U., Leos-Barajas, V., Bradley, D., Langrock, R., et al. (2018). Activity seascapes highlight central place foraging strategies in marine predators that never stop swimming. Mov. Ecol. 6, 9. doi: 10.1186/s40462-018-0127-3
Patterson, T. A., Basson, M., Bravington, M. V., and Gunn, J. S. (2009). Classifying movement behaviour in relation to environmental conditions using hidden Markov models. J. Anim. Ecol. 78, 1113–1123. doi: 10.1111/j.1365-2656.2009.01583.x
Pearce, J. (1996). A review of the British Indian Ocean Territory fisheries conservation and management zone tuna fishery, 1991 – 1995. Available online at: http://www.oceandocs.org/bitstream/handle/1834/497/EC601-17.pdf?sequence=1 (accessed January 11, 2016).
Peel, L. R., Stevens, G. M. W., Daly, R., Daly, C. A. K., Collin, S. P., Nogués, J., et al. (2020). Regional movements of reef manta rays (Mobula alfredi) in Seychelles Waters. Front. Mar. Sci. 7:558. doi: 10.3389/fmars.2020.00558
Poisson, F., Crespo, F. A., Ellis, J. R., Chavance, P., Pascal, B., Santos, M. N., et al. (2016). Technical mitigation measures for sharks and rays in fisheries for tuna and tuna-like species: turning possibility into reality. Aquat. Living Resour. 29:402. doi: 10.1051/alr/2016030
Poisson, F., Filmalter, J. D., Vernet, A., and Dagorn, L. (2014). Mortality rate of silky sharks (Carcharhinus falciformis) caught in the tropical tuna purse seine fishery in the Indian Ocean. Can. J. Fish. Aquat. Sci. 71, 795–798. doi: 10.1139/cjfas-2013-0561
Poisson, F., Vernet, A. L., Filmalter, J. D., Goujon, M., and Dagorn, L. (2011). Survival Rate of Silky Sharks (Carcharhinus falciformis) Caught Incidentally Onboard French Tropical Purse Seiners (Seychelles: IOTC), 6.
Queiroz, N., Humphries, N. E., Couto, A., Vedor, M., da Costa, I., Sequeira, A. M. M., et al. (2019). Global spatial risk assessment of sharks under the footprint of fisheries. Nature 572, 461–466. doi: 10.1038/s41586-019-1444-4
Queiroz, N., Humphries, N. E., Mucientes, G., Hammerschlag, N., Lima, F. P., Scales, K. L., et al. (2016). Ocean-wide tracking of pelagic sharks reveals extent of overlap with longline fishing hotspots. Proc. Natl. Acad. Sci. U.S.A. 113, 1582–1587. doi: 10.1073/pnas.1510090113
R Development Core Team (2018). R: A Language and Environment for Statistical Computing. Vienna: R Foundation Statistical Computing.
Rigby, C. L., Sherman, C. S., Chin, A., and Simpfendorfer, C. (2017). Carcharhinus falciformis. The IUCN Red List of Threatened Species, e.T39370A117721799. doi: 10.2305/IUCN.UK.2017-3.RLTS.T39370A117721799.en
Robbins, W. D., Hisano, M., Connolly, S. R., and Choat, J. H. (2006). Ongoing collapse of coral-reef shark populations. Curr. Biol. 16, 2314–2319. doi: 10.1016/j.cub.2006.09.044
Romanov, E. V. (2002). Bycatch in the tuna purse-seine fisheries of the western Indian Ocean. Fish. Bull. 100, 90–105.
Román-Verdesoto, M., and Orozco-Zöller, M. (2005). Bycatches of Sharks in the Tuna Purse-Seine Fishery of the Eastern Pacific Ocean Reported by Observers of the Inter-American Tropical Tuna Commission, 1993–2004 (San Diego, CA: IATTC).
Saji, N. H., Goswami, B. N., Vinayachandran, P. N., and Yamagata, T. (1999). A dipole mode in the tropical Indian Ocean. Nature 401, 360–363. doi: 10.1038/43854
Sánchez-De Ita, J. A., Quiñónez-Velázquez, C., Galván-magaña, F., Bocanegra-Castillo, N., and Félix-Uraga, R. (2011). Age and growth of the silky shark Carcharhinus falciformis from the west coast of Baja California Sur, Mexico. J. Appl. Ichthyol. 27, 20–24. doi: 10.1111/j.1439-0426.2010.01569.x
Schaefer, K. M., Fuller, D. W., Aires-Da-Silva, A., Carvajal, J. M., Martínez-Ortiz, J., and Hutchinson, M. R. (2019). Postrelease survival of silky sharks (Carcharhinus falciformis) following capture by longline fishing vessels in the equatorial eastern Pacific Ocean. Bull. Mar. Sci. 95, 355–369. doi: 10.5343/bms.2018.0052
Sheppard, C. R. C., Ateweberhan, M., Bowen, B. W., Carr, P., Chen, C. A., Clubbe, C., et al. (2012). Reefs and islands of the Chagos Archipelago, Indian Ocean: why it is the world’s largest no-take marine protected area. Aquat. Conserv. Mar. Freshw. Ecosyst. 22, 232–261. doi: 10.1002/aqc.1248
Skomal, G. B., Braun, C. D., Chisholm, J. H., and Thorrold, S. R. (2017). Movements of the white shark Carcharodon carcharias in the North Atlantic Ocean. Mar. Ecol. Prog. Ser. 580, 1–16. doi: 10.3354/meps12306
Stewart, J. D., Beale, C. S., Fernando, D., Sianipar, A. B., Burton, R. S., Semmens, B. X., et al. (2016). Spatial ecology and conservation of Manta birostris in the Indo-Pacific. Biol. Conserv. 200, 178–183. doi: 10.1016/j.biocon.2016.05.016
Thieurmel, B., and Elmarhraoui, A. (2019). suncalc: Compute Sun Position, Sunlight Phases, Moon Position and Lunar Phase. Available online at: https://CRAN.R-project.org/package=suncalc (accessed October 1, 2020).
Tickler, D. M., Carlisle, A. B., Chapple, T. K., Curnick, D. J., Dale, J. J., Schallert, R. J., et al. (2019). Potential detection of illegal fishing by passive acoustic telemetry. Anim. Biotelemetry 7:1. doi: 10.1186/s40317-019-0163-9
Urbina, J. O. D., Brunel, T., Coelho, R., Merino, G., Daniela, R., Santos, C., et al. (2018). A Preliminary Stock Assessment for the Silky Shark in the Indian Ocean Using a Data-Limited Approach. Seychelles: IOTC.
Ward, P., Lawrence, E., Darbyshire, R., and Hindmarsh, S. (2008). Large-scale experiment shows that nylon leaders reduce shark bycatch and benefit pelagic longline fishers. Fish. Res. 90, 100–108. doi: 10.1016/j.fishres.2007.09.034
Watson, J. T., Essington, T. E., Lennert-Cody, C. E., and Hall, M. A. (2009). Trade-offs in the design of fishery closures: management of silky shark bycatch in the Eastern Pacific Ocean Tuna fishery. Conserv. Biol. 23, 626–635. doi: 10.1111/j.1523-1739.2008.01121.x
Welch, H., Hazen, E. L., Bograd, S. J., Jacox, M. G., Brodie, S., Robinson, D., et al. (2019). Practical considerations for operationalizing dynamic management tools. J. Appl. Ecol. 56, 459–469. doi: 10.1111/1365-2664.13281
Weng, K. C., Castilho, P. C., Morrissette, J. M., Landeira-Fernandez, A. M., Holts, D. B., Schallert, R. J., et al. (2005). Satellite tagging and cardiac physiology reveal niche expansion in salmon sharks. Science 310, 104–106. doi: 10.1126/science.1114616
Wilson, S. G., Jonsen, I. D., Schallert, R. J., Ganong, J. E., Castleton, M. R., Spares, A. D., et al. (2015). Tracking the fidelity of Atlantic bluefin tuna released in Canadian waters to the Gulf of Mexico spawning grounds. Can. J. Fish. Aquat. Sci. 72, 1700–1717. doi: 10.1139/cjfas-2015-0110
Witt, M. J., Åkesson, S., Broderick, A. C., Coyne, M. S., Ellick, J., Formia, A., et al. (2010). Assessing accuracy and utility of satellite-tracking data using Argos-linked Fastloc-GPS. Anim. Behav. 80, 571–581. doi: 10.1016/j.anbehav.2010.05.022
Wood, L. J., Fish, L., Laughren, J., and Pauly, D. (2008). Assessing progress towards global marine protection targets: shortfalls in information and action. Oryx 42, 340–351. doi: 10.1017/S003060530800046X
Worm, B., Davis, B., Kettemer, L., Ward-Paige, C. A., Chapman, D., Heithaus, M. R., et al. (2013). Global catches, exploitation rates, and rebuilding options for sharks. Mar. Policy 40, 194–204. doi: 10.1016/j.marpol.2012.12.034
Wright, G., Gjerde, K. M., Johnson, D. E., Finkelstein, A., Ferreira, M. A., Dunn, D. C., et al. (2019). Marine spatial planning in areas beyond national jurisdiction. Mar. Policy doi: 10.1016/j.marpol.2018.12.003 [Epub ahead of print].
Keywords: fisheries, seamount, Carcharhinus falciformis, habitat use, biotelemetry, residency, migration, marine protected area
Citation: Curnick DJ, Andrzejaczek S, Jacoby DMP, Coffey DM, Carlisle AB, Chapple TK, Ferretti F, Schallert RJ, White T, Block BA, Koldewey HJ and Collen B (2020) Behavior and Ecology of Silky Sharks Around the Chagos Archipelago and Evidence of Indian Ocean Wide Movement. Front. Mar. Sci. 7:596619. doi: 10.3389/fmars.2020.596619
Received: 19 August 2020; Accepted: 10 November 2020;
Published: 16 December 2020.
Edited by:
Mourier Johann, Institut de Recherche Pour le Développement (IRD), FranceReviewed by:
John David Filmalter, South African Institute for Aquatic Biodiversity, South AfricaCopyright © 2020 Curnick, Andrzejaczek, Jacoby, Coffey, Carlisle, Chapple, Ferretti, Schallert, White, Block, Koldewey and Collen. This is an open-access article distributed under the terms of the Creative Commons Attribution License (CC BY). The use, distribution or reproduction in other forums is permitted, provided the original author(s) and the copyright owner(s) are credited and that the original publication in this journal is cited, in accordance with accepted academic practice. No use, distribution or reproduction is permitted which does not comply with these terms.
*Correspondence: David J. Curnick, ZGF2aWQuY3Vybmlja0B6c2wub3Jn
†Deceased
Disclaimer: All claims expressed in this article are solely those of the authors and do not necessarily represent those of their affiliated organizations, or those of the publisher, the editors and the reviewers. Any product that may be evaluated in this article or claim that may be made by its manufacturer is not guaranteed or endorsed by the publisher.
Research integrity at Frontiers
Learn more about the work of our research integrity team to safeguard the quality of each article we publish.