- 1Faculty of Maritime Engineering and Marine Sciences, Escuela Superior Politécnica del Litoral, ESPOL Polytechnic University, Guayaquil, Ecuador
- 2Faculty of Life Sciences, Escuela Superior Politécnica del Litoral, ESPOL Polytechnic University, Guayaquil, Ecuador
- 3Biotechnology Research Center, Escuela Superior Politécnica del Litoral, ESPOL Polytechnic University, Guayaquil, Ecuador
The pathogen Vibrio vulnificus has been associated with the majority of clinical cases of septicemia and deaths attributed to shellfish consumption. However, reports on biocontrol agents against this pathogen are scarce. In this study, the strain A5 of Bacillus amyloliquefaciens (A5) was evaluated against V. vulnificus. The sensitivity of V. vulnificus to A5 was first assessed in vitro using selected solid media as well as autoclaved oysters (Crassostrea gigas). Then, the ability of A5 to colonize live oysters was evaluated, and the biocontrol efficacy was investigated in vivo using oysters inoculated with V. vulnificus before or after inoculation with A5. The survival of the pathogen in oysters was evaluated after 2, 3, 4, and 6 days of exposure to A5 in all the experiments. In vitro, A5 showed inhibition halos of 18 mm against V. vulnificus. In autoclaved oysters, A5 caused a significant reduction in the levels of V. vulnificus on day 2 at 5.14 log CFU/g, but the pathogen’s counts were restored after day 3. In vivo, A5 was able to survive in live oysters and prevented the colonization of V. vulnificus only when the biocontrol agent was inoculated before the pathogen. Results show the potential of A5 to prevent V. vulnificus uptake by oysters when administered prior to the pathogen.
Introduction
Vibrio vulnificus are Gram-negative, opportunistic, and highly adaptable halophilic bacteria that are part of the natural flora of marine coastal environments around the world (Kelly, 1982; Lee et al., 2014), including seawater (Baffone et al., 2006), sediments, and marine species of human consumption such as shrimp, fish, oysters, and clams (DePaola et al., 1998; Baffone et al., 2006; Mahmud et al., 2008; Huehn et al., 2014). V. vulnificus has been responsible for the majority of deaths associated with the consumption of raw seafood and has shown the highest death rate and per-case economic burden of all the foodborne pathogens in the United States (Jones and Oliver, 2009; Hoffmann et al., 2015; Oliver, 2015; Pu et al., 2018). If ingested, V. vulnificus can cause vomiting, diarrhea, abdominal pain, and fever starting 24–48 h after consuming contaminated oysters (Chen et al., 2002; Haq and Dayal, 2005). Severe cases produce primary sepsis and gastrointestinal infections with mortality rates that can exceed 50% of the incidences (Cazorla et al., 2011; Horseman and Surani, 2011). Another kind of V. vulnificus infection occurs in wounds exposed to seawater contaminated with the pathogen. In this case, the infection can progress to necrotizing fasciitis with reddening of the skin and pigmented blood blisters with estimated lethality rates of 25% (Bross et al., 2007; Dechet et al., 2008; Zetti et al., 2009). The risk of V. vulnificus infection is higher in individuals with a compromised immune system, such as people with chronic liver disease, alcoholism, diabetes, and hemochromatosis (Bross et al., 2007; Barton and Acton, 2009; Yun and Kim, 2018). The Centers for Disease Control and Prevention of the United States (CDC) estimates that around 80,000 people get vibrio infections every year, including 500 hospitalizations and 100 deaths, with V. vulnificus as the most prevalent causal agent (Araujo et al., 2007; Scallan et al., 2011; Oliver, 2015; CDC, 2018).
Oysters can filter large amounts of seawater during their feeding activities, thus concentrating marine bacteria such as V. vulnificus in the mollusk’s body. About 74% of the retail oysters in the United States can contain V. vulnificus (DePaola et al., 2010), and the presence of the pathogen has been observed in other regions, such as the coasts of South America, with clinical cases reported in Uruguay, Ecuador, and Peru (Raszl et al., 2016).
Oyster species, including Crassostrea gigas—commonly grown in the Pacific coast—are mostly consumed raw or slightly processed (Baker, 2016), and the washing, roughing, plus other standard depuration methods have not been effective in reducing the pathogen’s levels from the mollusk (Froelich and Noble, 2014). Various alternatives have been tested to control V. vulnificus in live oysters, such as the use of antibiotics (Wong et al., 2015), sodium hypochlorite and gamma-ray irradiation (Park et al., 2018), bacteriophages (Pelon et al., 2005; Lee et al., 2012), seaweed (Genovese et al., 2012), and curcumin (Na et al., 2011) extracts, among others. However, these methods did not effectively reduce the vibrio levels, increased the production costs (Kim et al., 2011), and caused modifications in the taste or appearance of the oysters (Cruz-Romero et al., 2007).
Biological control using antagonistic microorganisms offers new opportunities to reduce the levels of pathogenic bacteria, improving food safety and quality (Gálvez et al., 2010). Microbial biocontrol agents can inhibit or eliminate foodborne pathogens through nutrient competition and production of antimicrobial metabolites, among other mechanisms. Biocontrol agents such as lactic acid bacteria and bacteriophages have been effective against Listeria monocytogenes in ready-to-eat meat products (Castellano et al., 2017) and Campylobacter jejuni in broiler chickens (Richards et al., 2019), respectively. Antagonistic microorganisms have also been tested against V. vulnificus. Phaeobacter inhibens DSM 17395, which produces the antibacterial compound tropodithietic acid, inhibited V. vulnificus in autoclaved oyster juice, but no significant reduction in the pathogen’s levels was observed in live oysters (Porsby and Gram, 2016).
Bacillus spp. are some of the most effective biocontrol agents, and bacillus-based formulations account for approximately half of the commercially available bacterial biocontrol products (Fravel, 2005). Metabolites from Bacillus amyloliquefaciens have shown antilisterial activity and have been suggested as a food preservative (Halimi et al., 2010; Hu et al., 2010). Other human pathogens such as Candida albicans (Song et al., 2013) and Vibrio spp. (Xu et al., 2014) have also shown high sensitivity to B. amyloliquefaciens. Lipopeptides produced by B. amyloliquefaciens M1 isolated from mangroves showed a high in vitro antibacterial activity against Vibrio pathogens including Vibrio harveyi, Vibrio anguillarum, Vibrio parahaemolyticus, V. vulnificus, Vibrio salmonicida, Vibrio fischeri, Vibrio splendidus, Vibrio septicus, and Vibrio ichthyoenteri (Xu et al., 2014). Similarly, the strain A5 of B. amyloliquefaciens stood out among other antagonistic bacteria capable of controlling V. vulnificus in several in vitro tests (Serrano, 2014). However, applications of B. amyloliquefaciens in live oysters have not been reported. The purpose of this research was to evaluate B. amyloliquefaciens as a biocontrol agent against V. vulnificus in vitro and in oyster (C. gigas) models.
Materials and Methods
Strains and Culture Conditions
Bacillus amyloliquefaciens A5 was isolated from organic soil amendments (Serrano, 2014), and V. vulnificus V4 (GenBank accession MN712474) was donated by the Pathology Laboratory at ESPOL University in Ecuador. Both strains were kept frozen at −80°C in potato dextrose broth (PDB; BD Difco, Sparks, MD, United States) and Luria–Bertani (LB; Invitrogen, Waltham, MA, United States), respectively, with 15% glycerol and 2% NaCl (Merck, Darmstadt, Germany). For the experiments, both bacterial strains were reactivated in tryptic soy agar (TSA; BD Difco, Sparks, MD, United States) with 2% NaCl (Merck, Darmstadt, Germany). The strain A5 was incubated at 30°C and V. vulnificus at 35°C for 24 h (Wang et al., 2010a; Ramos et al., 2012).
The identity confirmation of both bacteria was by DNA extraction and sequencing, as suggested elsewhere (Wang et al., 2010a, b). Briefly, a colony of each bacterium was suspended in conical microtubes (Axygen, IL, United States) containing 200 μl of lysis buffer [0.05 N NaOH, 0.025% sodium dodecyl sulfate (SDS), and ultrapure water] and heated for 10 min at 95°C in a water bath (Grant W28, Cambridge, United Kingdom). The tubes were chilled on ice for 2 min and then centrifuged at 10,000 × g for 10 min. A volume of 150 μl of the supernatants was transferred to 1.5-ml microtubes containing 300 μl of 95% frozen ethanol and centrifuged at 10,000 × g for 5 min. The supernatants were discarded, and the pellet was resuspended in 100 μl of 70% frozen ethanol. The tubes were then centrifuged at 10,000 × g for 5 min, and the supernatants were discarded. Finally, the pellet with the extracted DNA was suspended in 50 μl of ultrapure water (Invitrogen, Waltham, MA, United States). PCR amplification of strains was carried out in a 25-μl volume using GoTaq® DNA Polymerase (Promega, Madison, WI, United States). For A5, a 466-bp region from the 16S rRNA gene was amplified with the universal primers 341Fw (CCTACGGGAGGCAGCAG) and 806Rv (GGACTACHVGGGTWTCTAAT) (Yu et al., 2005), while for V. vulnificus V4, the 277-bp region of the virulence-correlated gene (vcg) was amplified with the primers P2 (5′-CTCAATTGACAATGATCT-3′) and P3 (5′-CGCTTAGGATGATCGGTG-3′) (Rosche et al., 2005). The amplicons obtained were sequenced in a service lab (Macrogen, Seoul, South Korea), and the data were analyzed using the Basic Local Alignment Search Tool (BLAST) algorithm from NCBI.
In vitro Antagonistic Evaluation
The antagonistic effect of A5 and its metabolites against V. vulnificus was assessed in vitro using three recovery liquid media including PDB, tryptic soy broth (TSB; Neogen, Lansing, MI, United States), or Mueller Hinton Broth (MHB; Sigma Aldrich, St. Louis, MO, United States) supplemented with 2% NaCl each, followed by plating onto four solid media including Mueller Hinton Agar (MHA; BD Difco, Sparks, MD, United States) (Hewitt and Vincent, 1989), TSA (BD Difco, Sparks, MD, United States), potato dextrose agar (PDA; Sigma Aldrich, St. Louis, MO, United States), or blood agar (BA; Medibac Lab, Ecuador) supplemented with 2% NaCl each.
One colony of a freshly grown A5 was suspended in 10 ml of each of the three recovery liquid media. The suspensions were then incubated at 30°C for 5 days (Serrano, 2014) in an orbital shaker (Thermo ScientificTM MaxQTM 4000, Waltham, MA, United States) at 110 rpm.
In vitro sensitivity tests were carried out through diffusion tests in wells as described in previous reports (López et al., 2005; Serrano, 2014). Briefly, Petri dishes with each of the solid media were spread-inoculated with V. vulnificus using sterile swabs. Three wells of 6-mm diameter were made on the inoculated media using a sterile punch. A total of 50 μl of a 5-day-old A5 culture grown in the different recovery liquid media, 50 μl of the same A5 culture previously filter-sterilized using 0.22-μm syringe filters, or 50 μl of the filter-sterilized A5 culture autoclaved at 121°C for 15 min were placed in the agar wells. The plates were incubated at 30°C for 24 to 48 h, and inhibition halos were measured around each well. All experiments were run in triplicate.
Oyster Acclimatization
Oysters (C. gigas) of 8 cm of anterior–posterior length were obtained from the beach El Palmar–Santa Elena in the coast of Ecuador. After harvest, the bivalves were submerged in microfiltered and ultraviolet light-sterilized seawater, kept at ambient temperature for 72 h, and transported to the laboratory.
The acclimatization of the oysters to laboratory conditions was carried out as described in previous studies (Wang et al., 2010a, b; Porsby and Gram, 2016). Briefly, the oysters were washed with autoclaved saline solution and placed in containers with autoclaved ionized seawater with 33.0 ± 1.0 ppt salinity at a rate of 500 ml per oyster. The average temperature was 23°C ± 2°C, and constant aeration of 3 L/min (Wang et al., 2010a, b; Ramos et al., 2012) was maintained during the 4 days of acclimatization. There was no mortality during acclimatization of oysters.
Conditions of Artificial Inoculation of V. vulnificus and A5
The inoculation method was modified from Wang et al. (2010a). A colony of V. vulnificus was inoculated in 100 ml of LB (Invitrogen, MA, United States) with 2% NaCl and incubated at 35°C for 24 h at 110 rpm in an orbital shaker (Thermo ScientificTM MaxQTM 4000, Waltham, MA, United States). The bacterial suspension was then centrifuged at 4,500 × g (Sorvall ST16, Thermo Fisher Scientific, Osterode, Germany) for 5 min, and the pellet was resuspended in an autoclaved 2% NaCl solution.
For the A5 strain, four bacterial colonies were individually inoculated in separate bottles containing 100 ml of PDB with 2% NaCl and incubated at 30°C for 24 h at 110 rpm in an orbital shaker. To obtain a higher concentration of A5, the bacterial cultures were centrifuged at 5,000 × g for 7 min, and the supernatants were discarded. The resulting pellets were resuspended in 300 ml of PDB with 2% NaCl and incubated again at 30°C in an orbital shaker at 110 rpm for 24 h. The cultures were then centrifuged at 5,000 × g for 7 min, and the supernatants were discarded. The four pellets were then put together in a single falcon tube and resuspended in 50 ml of an autoclaved 2% NaCl solution.
The bacterial concentration of both A5 and V. vulnificus was determined by measuring the OD600nm with a BioPhotometer 6131 (Eppendorf, Hamburg, Germany) spectrophotometer. For this, each bacterial suspension was 10-fold serial diluted with autoclaved 2% NaCl to obtain bacterial concentrations in the range of 102–109 CFU/ml. Each dilution (100 μl) was spread-plated onto TSA with 2% NaCl and incubated at 35°C for V. vulnificus or at 30°C for A5 during 24 h. The number of colony-forming units (CFUs) was counted and correlated to the OD600nm values in a standard curve. The inoculation volume of the pure culture (Vi) required to achieve a desired final calculated bacterial concentration (FCBC) in an oyster’s container was estimated using the equation:
where Vt is the total volume of the autoclaved seawater in the inoculation tank with oysters, and BCi is the bacterial concentration of the pure culture estimated by the OD600nm.
Levels of FCBC between 106 and 107 for both bacteria were initially tested in oysters, and the best FCBC levels were selected for all experiments.
Antagonism in Autoclaved Oysters
Autoclaved oysters were used to assess the ability of A5 to grow in oyster meat and validate the antagonistic effect of A5 against V. vulnificus in an oyster matrix. Forty-five oysters were individually wrapped in aluminum foil, placed in a 2-L cylindrical vessel, and autoclaved (Sterilizer SM510, Yamato Scientific, Japan) at 121°C for 15 min. Three plastic containers of 41 cm × 30 cm × 28 cm (PYCCA, Guayaquil, Ecuador) were disinfected with boiling water followed by a 70% ethanol rinse. Then, 15 autoclaved oysters along with autoclaved seawater at a rate of 500 ml per oyster were placed in each container. In the first container, the oysters were inoculated with A5 to an FCBC of 1.0 × 106 CFU/ml and with V. vulnificus to an FCBC of 1.0 × 107 CFU/ml (A5 + Vv treatment). The oysters from the second container were only inoculated with A5 to an FCBC of 1.0 × 106 CFU/ml (A5 treatment), while the oysters from the third container were inoculated with V. vulnificus to an FCBC of 1.0 × 107 CFU/ml (Vv treatment). All inoculations were performed in the oyster–seawater mix, and bacterial levels in oysters and in the water from each container were assessed immediately after autoclaving (before inoculation) and on days 0, 2, 3, 4, and 6 after inoculation exactly as described in the Microbiological Analysis of Live Oysters section below. The measurements were done in triplicate.
Antagonism in Live Oysters
Three bioassays were performed to determine the colonization capacity of all strains and to assess the biocontrol effect of A5 in live oysters inoculated with V. vulnificus before or after inoculation with the biocontrol agent, respectively. Table 1 shows a summary of the bioassays executed. The oysters were kept at 23°C ± 2°C with constant aeration of 3 L/min during the whole experiment in the three bioassays (Wang et al., 2010a, b; Ramos et al., 2012).
To determine if A5 and V. vulnificus were capable of colonizing the tissues of live oysters (bioassay 1), three plastic containers of 41 cm × 30 cm × 28 cm (PYCCA, Guayaquil, Ecuador) with 15 oysters plus 7.5 L (500 ml/oyster) of autoclaved seawater at 33 ± 1.0 ppt salinity each were used (Porsby and Gram, 2016). Oysters from the first container were inoculated with V. vulnificus to an FCBC of 1.0 × 107 CFU/ml and reinoculated with the same dose of the vibrio 24 h after the first inoculation. Similarly, oysters from the second container were inoculated with A5 to an FCBC of 1.0 × 106 CFU/ml and reinoculated with the same dose 24 h after the first inoculation. Oysters from the third container were kept in the autoclaved seawater as a control (Tirapé, 2007; Wang et al., 2010a, b). Bacteria counts in oysters and water from each container were measured on TSA and thiosulfate-citrate-bile salt-sucrose (TCBS) on days 0, 2, 3, 4, and 6 of the bioassays exactly as described in the Microbiological Analysis of Live Oysters section below. The measurements were done in triplicate.
The antagonist effect of A5 was assessed in oysters previously colonized by V. vulnificus (bioassay 2) as well as oysters colonized with A5 prior to the inoculation with the vibrio (bioassay 3). For both bioassays, three containers with 18 oysters and autoclaved seawater at a rate of 500 ml/oyster in each container were used. Container characteristics and seawater conditions were identical to those of bioassay 1. For bioassay 2, containers were labeled as Vv (V. vulnificus only), Vv + A5 (inoculation with V. vulnificus followed by A5), and control (control oysters with no inoculation), respectively. Oysters from the Vv and the Vv + A5 containers were inoculated on day 0 with V. vulnificus to an FCBC of 2.0 × 107 CFU/ml. On day 1, the water of the three containers were replaced with an identical volume of freshly autoclaved seawater to remove the excess of non-colonizing V. vulnificus as described in previous studies (Porsby and Gram, 2016). The Vv + A5 container was inoculated with A5 to an FCBC of 1.0 × 106 CFU/ml immediately after water replacement and reinoculated with the same dose of A5 on day 2. Oysters from the control container were kept in the autoclaved seawater as a control (Tirapé, 2007; Wang et al., 2010a, b). The bacteria counts in oysters as well as in the water of each container were measured on days 0, 1, 2, 3, 4, and 6 of the bioassay using TSA and TCBS, as described in the Microbiological Analysis of Live Oysters section below.
For bioassay 3, the three oyster containers were labeled as Vv (V. vulnificus only), A5 + Vv (inoculation with A5 followed by V. vulnificus), and control (control oysters with no inoculation), respectively. The A5 + Vv container was inoculated with A5 to an FCBC of 3.0 × 106 CFU/ml on day 0. On day 1, the water from the three containers was replaced with freshly autoclaved seawater maintaining the ratio of 500 ml/oyster. Then, both the Vv and A5 + Vv containers were inoculated with V. vulnificus to an FCBC of 1.0 × 107 CFU/ml on days 1 and 2. Oysters from the control container were kept in the autoclaved seawater as a control (Tirapé, 2007; Wang et al., 2010a, b). The bacteria counts in oysters and in the water were assessed on days 0, 1, 2, 3, 4, and 6 of the bioassays using TSA and TCBS, as described in the Microbiological Analysis of Live Oysters section. Bioassay 3 was fully repeated 6 months later using fresh oysters to check the reproducibility of the biocontrol effect.
Microbiological Analysis of Live Oysters
Microbiological analyses were carried out according to U.S. Food and Drug Administration (FDA)’s Bacteriological Analytical Manual (Kaysner and DePaola, 2004). Briefly, oysters were randomly picked for analysis at each selected time. Each oyster was shucked with a sterile knife and separated from the shell (Phuvasate et al., 2012). Then, 0.1 g of shucked oysters was homogenized with 0.9 ml of 2% autoclaved NaCl solution at high speed for 1 min using a three-speed blender (Oster Blender 4655, United States). Homogenized oysters were serial diluted using a 2% NaCl solution and plated onto TCBS and TSA + 2% NaCl. Plates were then incubated at 35°C and 30°C during 24 h for the growth of V. vulnificus and A5, respectively. Colonies on TCBS were considered as total vibrio, whereas green colonies were counted as V. vulnificus (West et al., 1982; Cerdà-Cuéllar et al., 2000). Colonies in TSA were considered as total aerobic bacteria.
At the end of the incubation time, the bacterial count was carried out on Petri dishes containing less than 300 colonies. To obtain the number of CFU/ml or CFU/g, the following formula was applied:
CFU/ml or CFU/g = Number of colonies per plate × The dilution factor/Volume (ml) of the inoculated sample.
Therefore, the limits of detection (LODs) were 2 log CFU/g for oyster tissue and 1 log CFU/ml for seawater (Kaysner and DePaola, 2004). The reported values were an average and standard deviation of the three replicates.
Statistical Analysis
The data obtained from the in vitro assays were submitted to an analysis of variance (ANOVA) to compare bacterial levels among treatments, and significance was reported at p < 0.05. Bacterial counts from the experiments with live and autoclaved oysters were Log-transformed prior to the ANOVAs. All statistical tests were carried out in Microsoft Excel.
Results
Strains ID Confirmation
The DNA sequence from the A5 strain shared 99% similarity with B. amyloliquefaciens Access Number KJ603234.1, while the sequence of the vibrio strain shared 98% similarity with V. vulnificus Access Number AY626584.1 from the GenBank database.
In vitro Biocontrol Tests
The strain A5 was able to reduce the growth of V. vulnificus in vitro, with inhibition halos between 5 and 18 mm. The highest inhibition (p < 0.05) was observed when using PDB + 2% NaCl or MHB + 2% NaCl as a recovery liquid medium in MHA + 2% NaCl as a solid medium, yielding average inhibition halos of 18 and 17 mm, respectively (Figure 1). However, no inhibition was observed on PDA + 2% NaCl, TSA + 2% NaCl, and BA + 2% NaCl when MHB + 2% NaCl was used as a recovery liquid medium. Similarly, plates with PDA + 2% NaCl did not support the growth of V. vulnificus. The results of the antagonism tests were measured after 24 and 48 h, but no significant differences were observed between the two incubation periods. No inhibition halos were observed when testing the filter-sterilized or autoclaved supernatants.
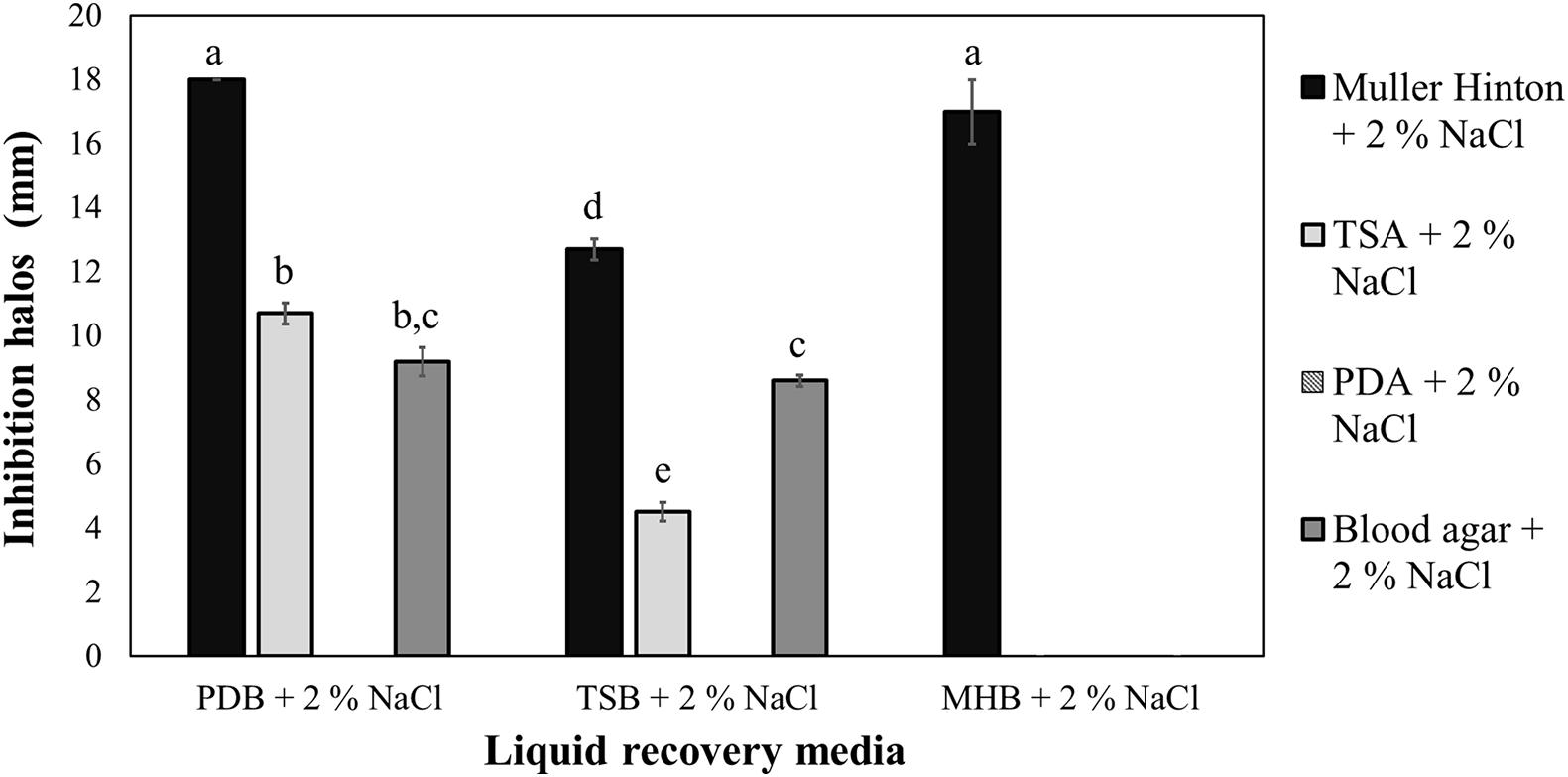
Figure 1. In vitro sensitivity of Vibrio vulnificus to the strain A5 of Bacillus amyloliquefaciens (A5) recovered in three liquid media and assessed on four solid media. Equal letters indicate no significant differences (p > 0.05). The absence of bars represents no observed inhibition halos.
Biocontrol Tests in Autoclaved Oysters
The preliminary experiments showed that the recovery of V. vulnificus and A5 was negligible in oysters inoculated to an FCBC below 107 and 106 CFU/ml, respectively. Additionally, when using A5, the maximum FCBC achieved was 106 CFU/ml (data not shown). Therefore, FCBCs of 106 and 107 CFU/ml were used for the inoculation of A5 and V. vulnificus, respectively, in all experiments.
Both A5 and V. vulnificus were able to persist in autoclaved oysters (Figure 2) and seawater (Figure 3). On day 0—before sample inoculation—no CFUs were detected in both sample types. The total aerobic bacteria (Figures 2A, 3A) and total vibrio counts (Figures 2B, 3B) increased significantly after the inoculations, reaching values above 5 log CFU/g in oysters and 4 log CFU/ml in water samples from all treatments. Similarly, the levels of V. vulnificus in oyster (Figure 2C) and water samples (Figure 3C) from the A5 + Vv and the Vv treatments increased significantly after the inoculations, while no V. vulnificus was detected in the A5 treatment throughout the experiment. On day 2, the total vibrio (Figure 2B) and V. vulnificus (Figure 2C) counts were significantly higher (p < 0.05) in oysters from the Vv treatment when compared to the A5 or the A5 + Vv treatments, but no significant differences were observed between treatments from day 3 onward. Similarly, no significant differences were detected in the levels of total aerobic bacteria among oysters from the different treatments at any time point.
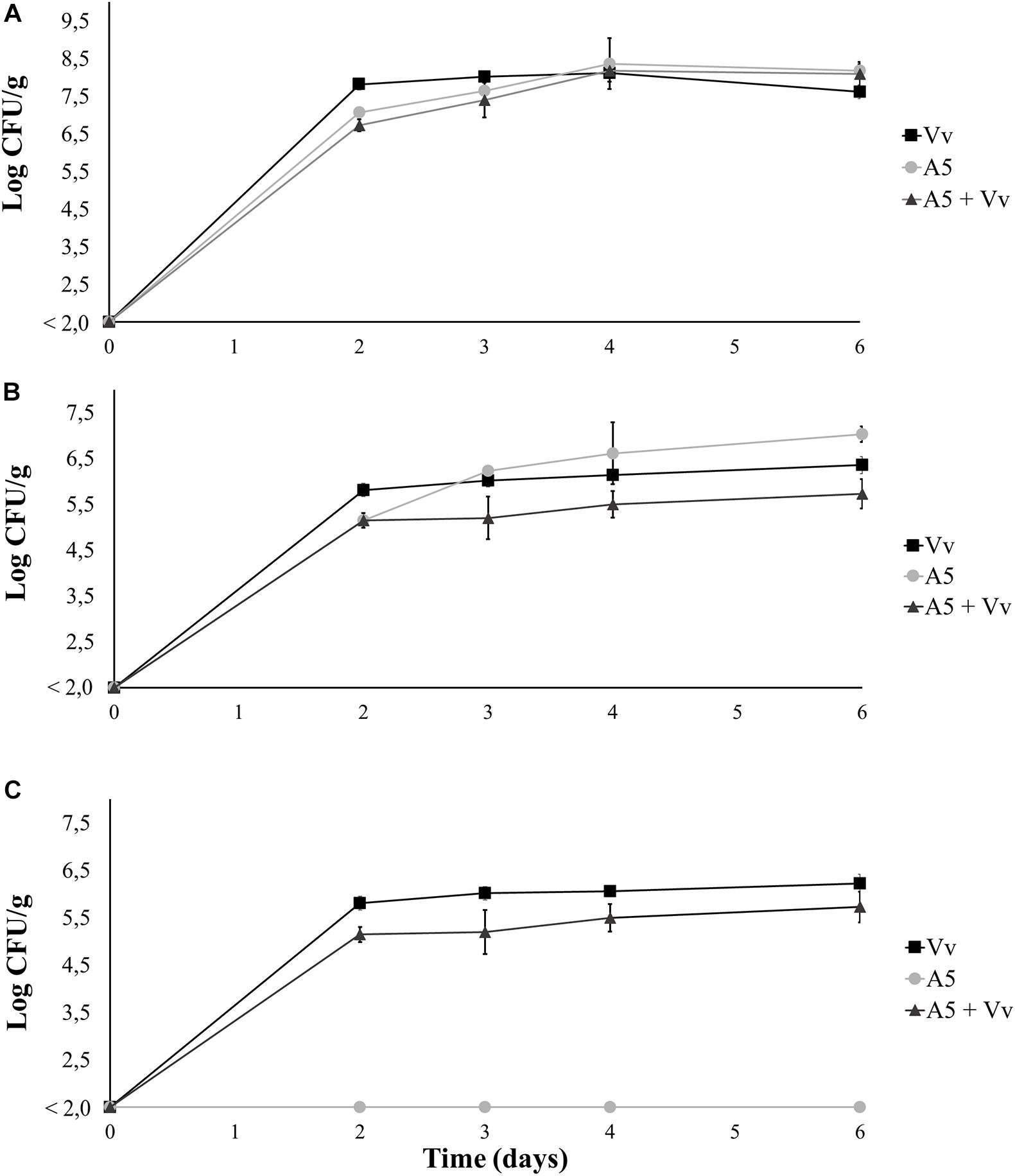
Figure 2. Total aerobic bacteria (A), total vibrio (B), and Vibrio vulnificus (C) levels in coculture experiments in autoclaved oysters. Oysters from the Vv treatment were inoculated with V. vulnificus only (■), oysters from the A5 treatment were inoculated with A5 only (•), oysters from the A5 + Vv treatment were co-inoculated with A5 and V. vulnificus (▲). The absence of colonies is represented as below the limit of detection (LOD) of 2.0 log CFU/g.
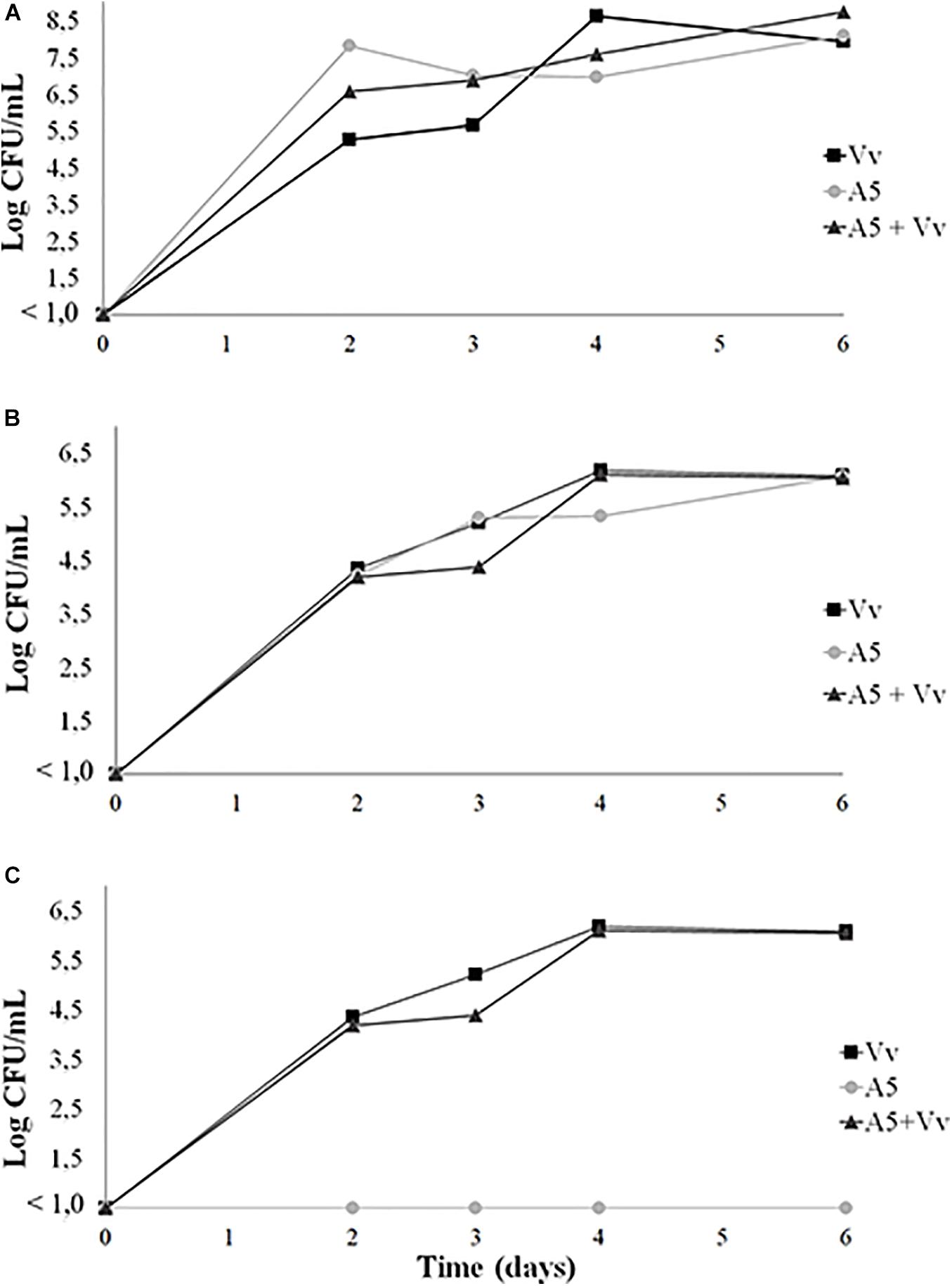
Figure 3. Total aerobic bacteria (A), total vibrio (B), and Vibrio vulnificus (C) levels in coculture experiments in autoclaved seawater. Seawater from the Vv treatment was inoculated with V. vulnificus only (■), seawater from the A5 treatment was inoculated with A5 only (•), seawater from the A5 + Vv treatment was co-inoculated with A5 and V. vulnificus (▲). The absence of colonies is represented as below the limit of detection (LOD) (<1.0 log CFU/g).
Biocontrol Tests in Live Oysters
Results from bioassay 1 showed that inoculation with A5 did not cause significant changes in the total aerobic counts of live oysters, as the bacterial levels were similar to those from control oysters during the whole experiment. However, the addition of V. vulnificus to live oysters yielded significantly lower total aerobic counts when compared to oysters from A5 or control treatments from day 4 onward (Figure 4A). Oysters inoculated with A5 showed significantly higher levels of total vibrio when compared to uninoculated oysters in day 3, but no significant differences were observed after this sampling time. Similarly, oysters inoculated with V. vulnificus showed the highest total vibrio levels on day 2 only (Figure 4B). From day 2 onward, the total vibrio levels in oysters from the Vv treatment consisted of green colonies only, as no yellow colonies were observed in the samples. Uninoculated oysters from the control treatment as well as oysters from the A5 treatment showed undetectable levels of V. vulnificus during the whole experiment (Figure 4C). In water samples, A5 caused a significant increase in the total aerobic counts on days 2 and 3, but no significant differences among treatments was observed at the other sampling times (Figure 5A). Similarly, A5 caused a significant increase in the levels of total vibrio in water samples on days 2 and 3, but V. vulnificus only increased the total vibrio levels at day 2 (Figure 5B). In the Vv treatment, total vibrio consisted of green colonies only. Similarly, no V. vulnificus was detected in water samples from the A5 or control treatments (Figure 5C).
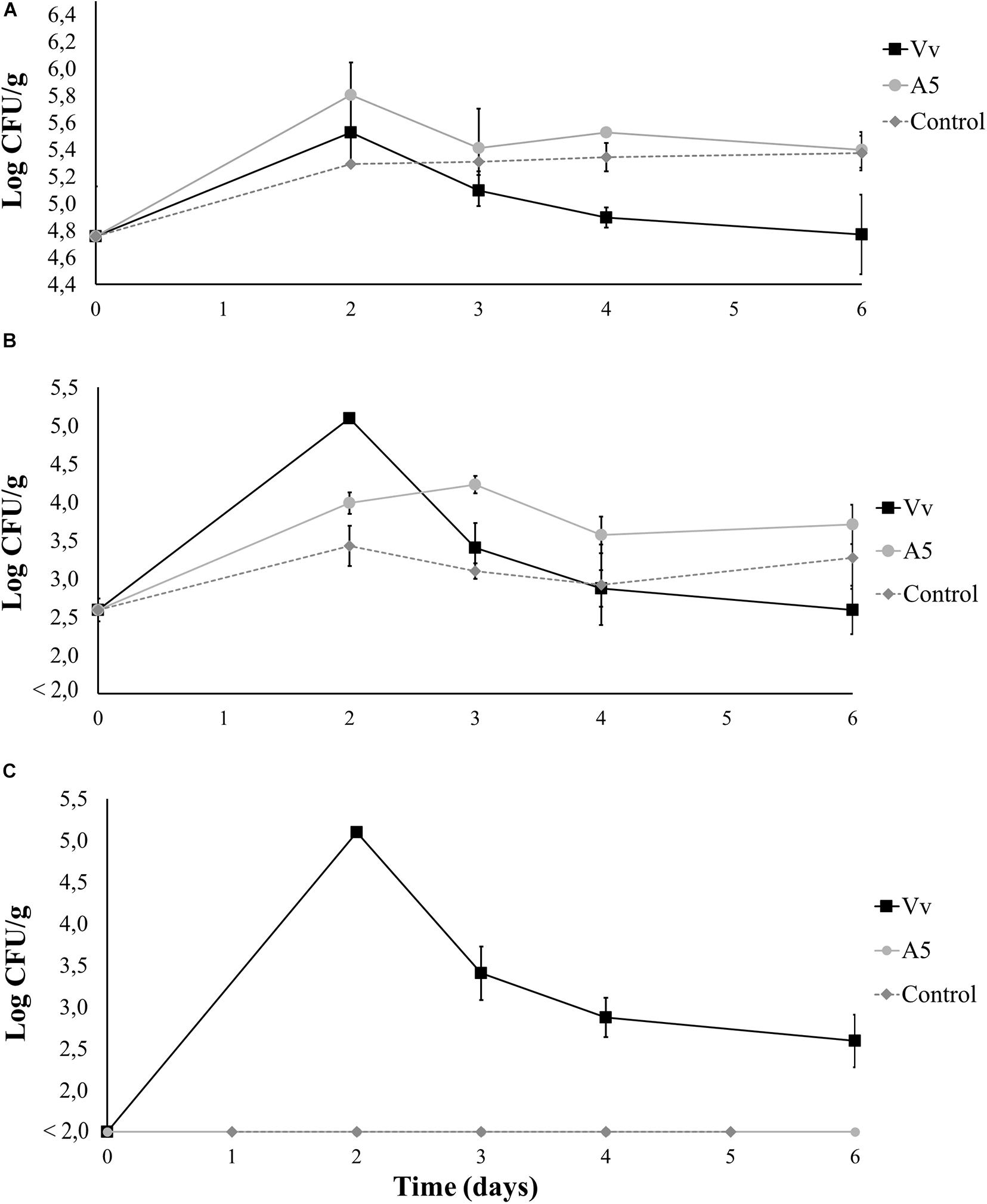
Figure 4. Total aerobic bacteria (A), total vibrio (B), and Vibrio vulnificus (C) levels in oysters from bioassay 1 (evaluation of colonization potential). Three treatments are shown: Vv inoculated with V. vulnificus only (■), A5 inoculated with A5 only (•), and control (◆ dotted line). The absence of colonies is represented as below the limit of detection (LOD) (<2.0 log CFU/g).
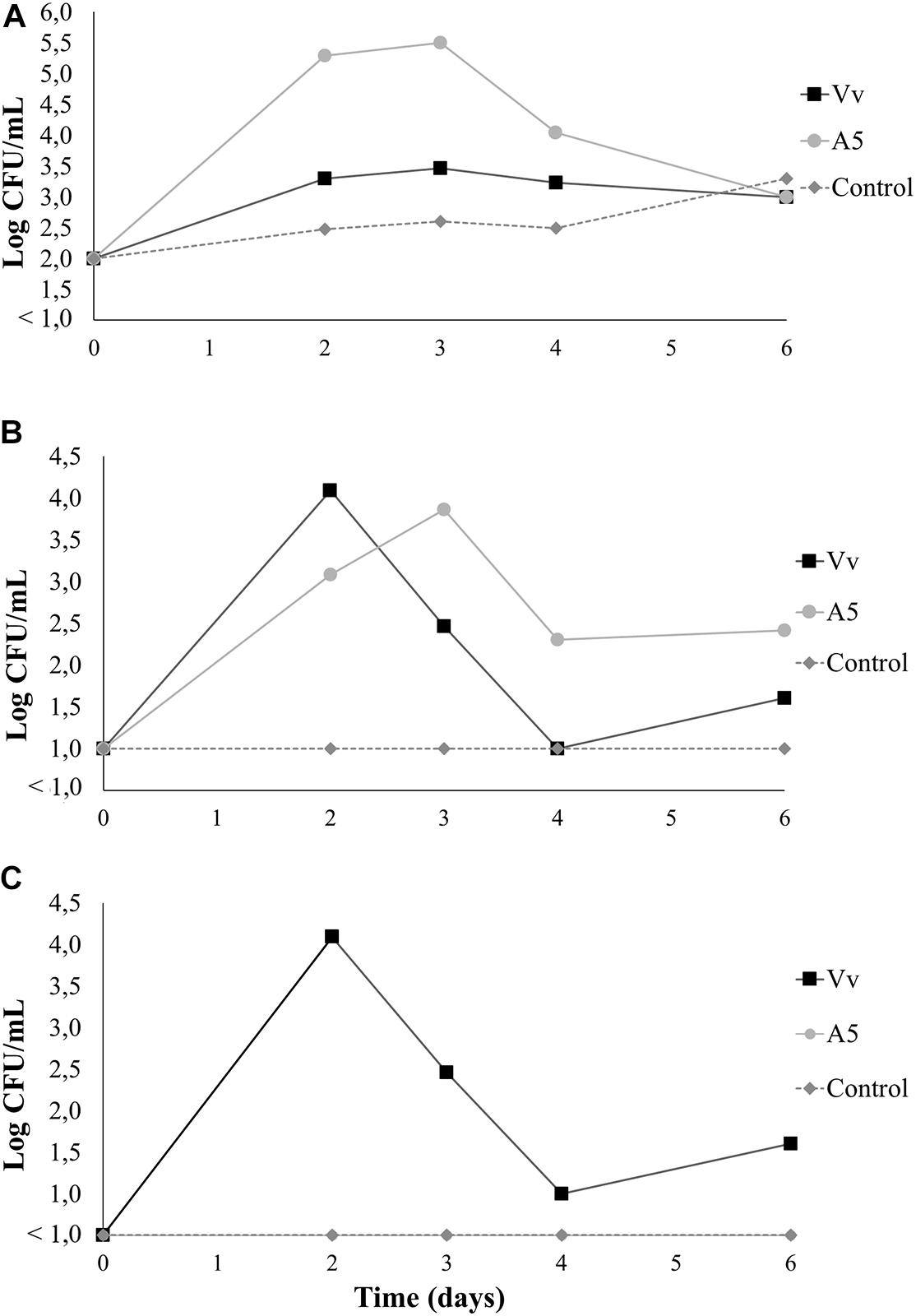
Figure 5. Total aerobic bacteria (A), total vibrio (B), and Vibrio vulnificus (C) levels in seawater from bioassay 1 (evaluation of colonization potential). Three treatments are shown: Vv inoculated with V. vulnificus only (■), A5 inoculated with A5 only (•), and control (◆ dotted line). The absence of colonies is represented as below the limit of detection (LOD) (<1.0 log CFU/g).
In the control treatment from bioassays 2 and 3, the total aerobic counts of oysters stayed in the range between 3.4 and 6.3 log CFU/g for all experiments (Figures 4A, 6A, 8A), whereas the total vibrio counts were between 2.0 and 3.4 log CFU/g (Figures 4B, 6B, 8B). In the water samples from the control treatment, the total aerobic counts were between 2.0 and 3.6 log CFU/ml (Figures 5A, 7A, 9A), while the total vibrio counts remained between 1.0 and 2.2 log CFU/ml in all the experiments (Figures 5B, 7B, 9B). Total vibrio counts in both oysters and water samples from the control treatment consisted of yellow colonies only, as green colonies (V. vulnificus) were not detected during the whole experiment (Figures 4C, 5C, 6C, 7C, 8C, 9C).
Results from bioassay 2 showed that the total aerobic counts in oysters from the Vv + A5 treatment significantly increased from 3.9 to 5.5 log CFU/g during the first 2 days of the experiment but decreased thereafter to final levels of 4.8 log CFU/g. However, oysters from the Vv treatment maintained total aerobic counts of around 4.5 log CFU/g during the whole experiment. The total aerobic counts were significantly higher in oysters from the Vv + A5 treatment when compared to those from the Vv treatment on days 2, 3, and 4, but no significant differences were observed at the other sampling times (Figure 6A). The total vibrio counts in oysters from all treatments were 2.3 log CFU/g on day 0 (before inoculations), while on day 1 (after inoculation with V. vulnificus), vibrio counts increased to 4.4 and 3.9 log CFU/g in oysters from the Vv and Vv + A5 treatments, respectively, with only green colonies detected. From day 3 onward, yellow colonies were also detected, and oysters from the Vv + A5 treatment showed significantly higher levels (p < 0.05) of total vibrio than that of the Vv treatment (Figure 6B). Similarly, the levels of V. vulnificus in the Vv and Vv + A5 treatments increased from 0 to average values of 4.4 and 3.9 log CFU/g in oysters from the Vv and Vv + A5 treatments, respectively, on day 1 but decreased thereafter, with no significant differences observed between treatments (Figure 6C). In water samples, the behavior of total aerobic counts was similar to that observed in oysters. Water samples from the Vv + A5 treatment maintained total aerobic counts of around 4.7 log CFU/ml from day 2 onward, while samples from the Vv treatment showed lower total aerobic counts at around 2.0 log CFU/ml at the same sampling times (Figure 7A). The initial total vibrio counts in the water were 1.0 log CFU/ml, increasing in samples from the Vv and Vv + A5 treatments to 3.2 and 2.9 log CFU/ml, respectively, on day 1. However, total vibrio levels remained nearly unchanged in the water from the Vv + A5 treatment while decreasing in the Vv treatment from day 2 onward (Figure 7B). No V. vulnificus was detected at time 0 (before inoculations) in the water samples from all treatments, but the inoculations caused an increase in the V. vulnificus levels at day 1 in both Vv and Vv + A5 treatments. However, from day 2 onward, the levels of V. vulnificus decreased in both treatments, but counts were higher in the Vv + A5 treatment when compared to the Vv treatment from days 2 to 4 (Figure 7C).
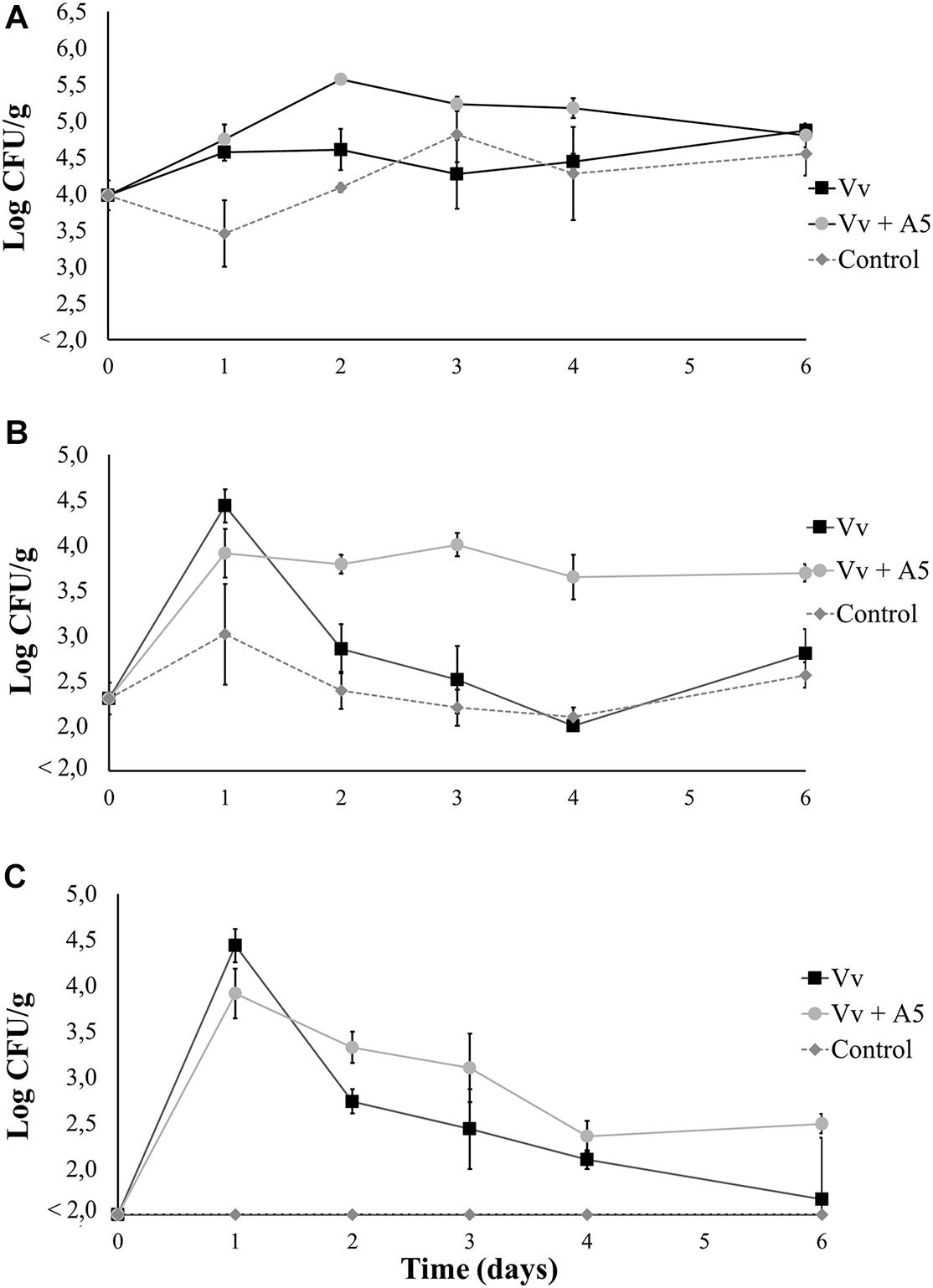
Figure 6. Total aerobic bacteria (A), total vibrio (B), and Vibrio vulnificus (C) levels in oysters from bioassay 2 (inoculation with V. vulnificus prior to inoculation with A5). Three treatments are shown: Vv inoculated with V. vulnificus only (■), Vv + A5 first inoculated with V. vulnificus and then with A5 (•), and control (◆ dotted line). The absence of colonies is represented as below the limit of detection (LOD) (<2.0 log CFU/g).
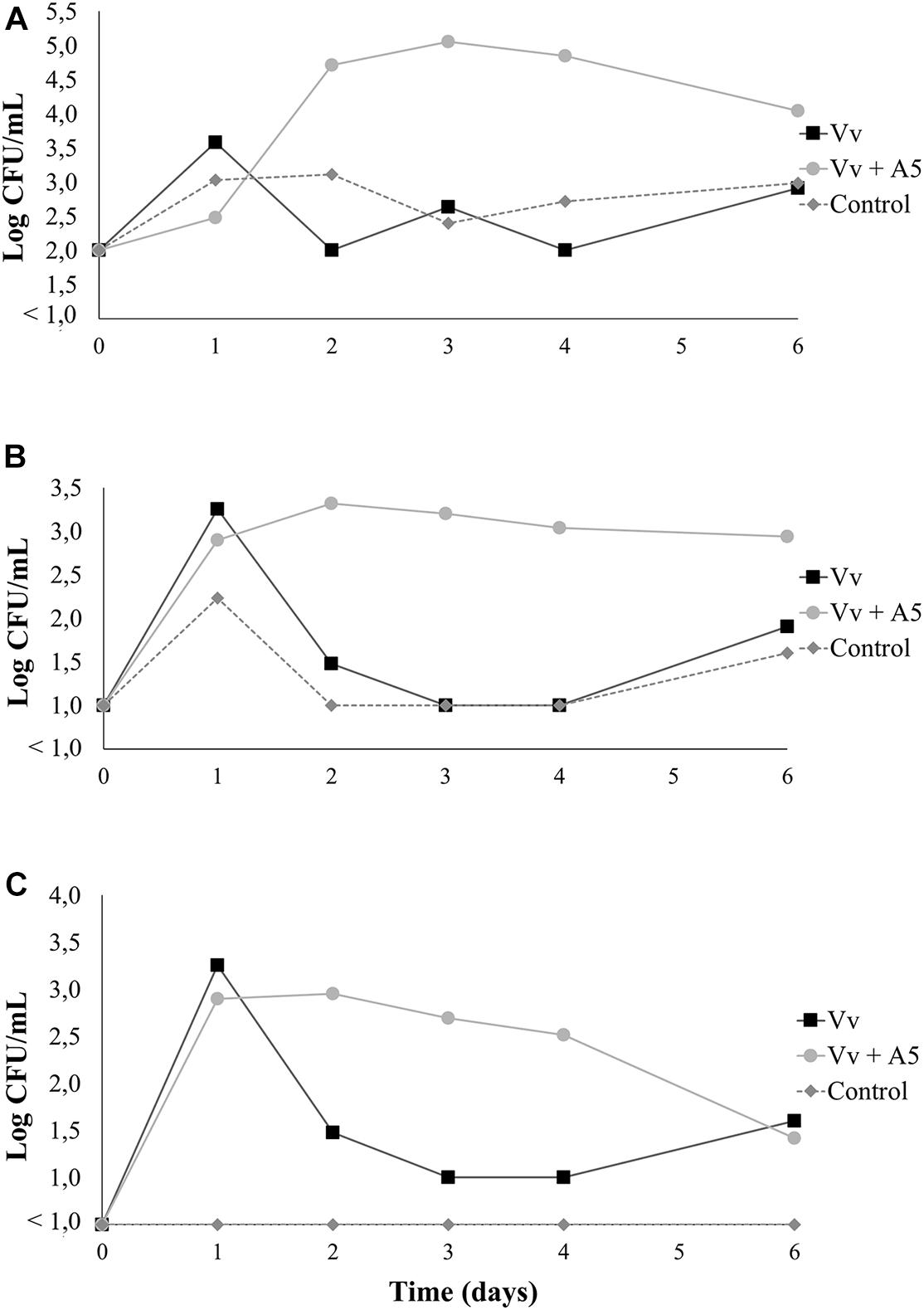
Figure 7. Total aerobic bacteria (A), total vibrio (B), and Vibrio vulnificus (C) levels in seawater from bioassay 2 (inoculation with V. vulnificus prior to inoculation with A5). Three treatments are shown: Vv inoculated with V. vulnificus only (■), Vv + A5 first inoculated with V. vulnificus and then with A5 (•), and control (◆ dotted line). The absence of colonies is represented as below the limit of detection (LOD) (<1.0 log CFU/g).
Results from bioassay 3 showed that the total aerobic counts in oysters (Figure 8A) and water (Figure 9A) from the A5 + Vv treatment were significantly higher than those from the Vv treatment during the whole experiment. Similarly, the total vibrio counts in oysters (Figure 8B) and water (Figure 9B) from the A5 + Vv treatment were significantly higher than those from the Vv treatment at days 1, 4, and 6 of the experiment, but no significant differences were observed at the other time points. However, the V. vulnificus levels in oysters from the A5 + Vv treatment were significantly lower than those from the Vv treatment during the whole experiment, and V. vulnificus was not detected on day 6 in the oysters from the A5 + Vv treatment, but the pathogen’s counts were above 2.5 log CFU/g in the Vv treatment (Figure 8C). Similarly, no V. vulnificus was detected in water samples from the A5 + Vv treatment throughout the experiment, but the pathogen’s levels in the Vv treatment were above 1 log CFU/ml (Figure 9C).
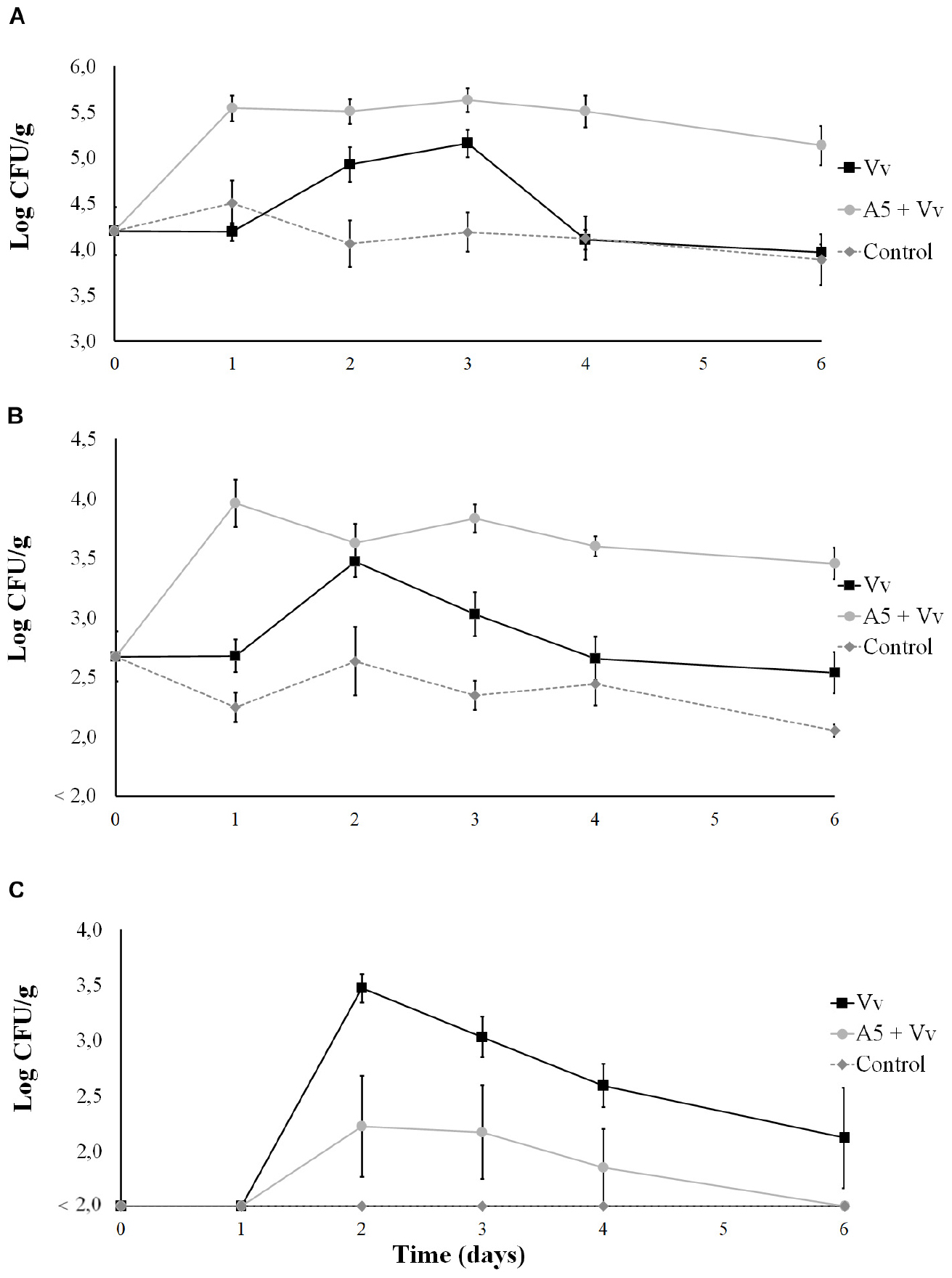
Figure 8. Total aerobic bacteria (A), total vibrio (B), and Vibrio vulnificus (C) levels in oysters from bioassay 3 (inoculation with A5 prior to inoculation with V. vulnificus). Three treatments are shown: Vv inoculated with V. vulnificus only (■), A5 + Vv first inoculated with A5 and then with V. vulnificus (•), and control (◆ dotted line). The absence of colonies is represented as below the limit of detection (LOD) (<2.0 log CFU/g).
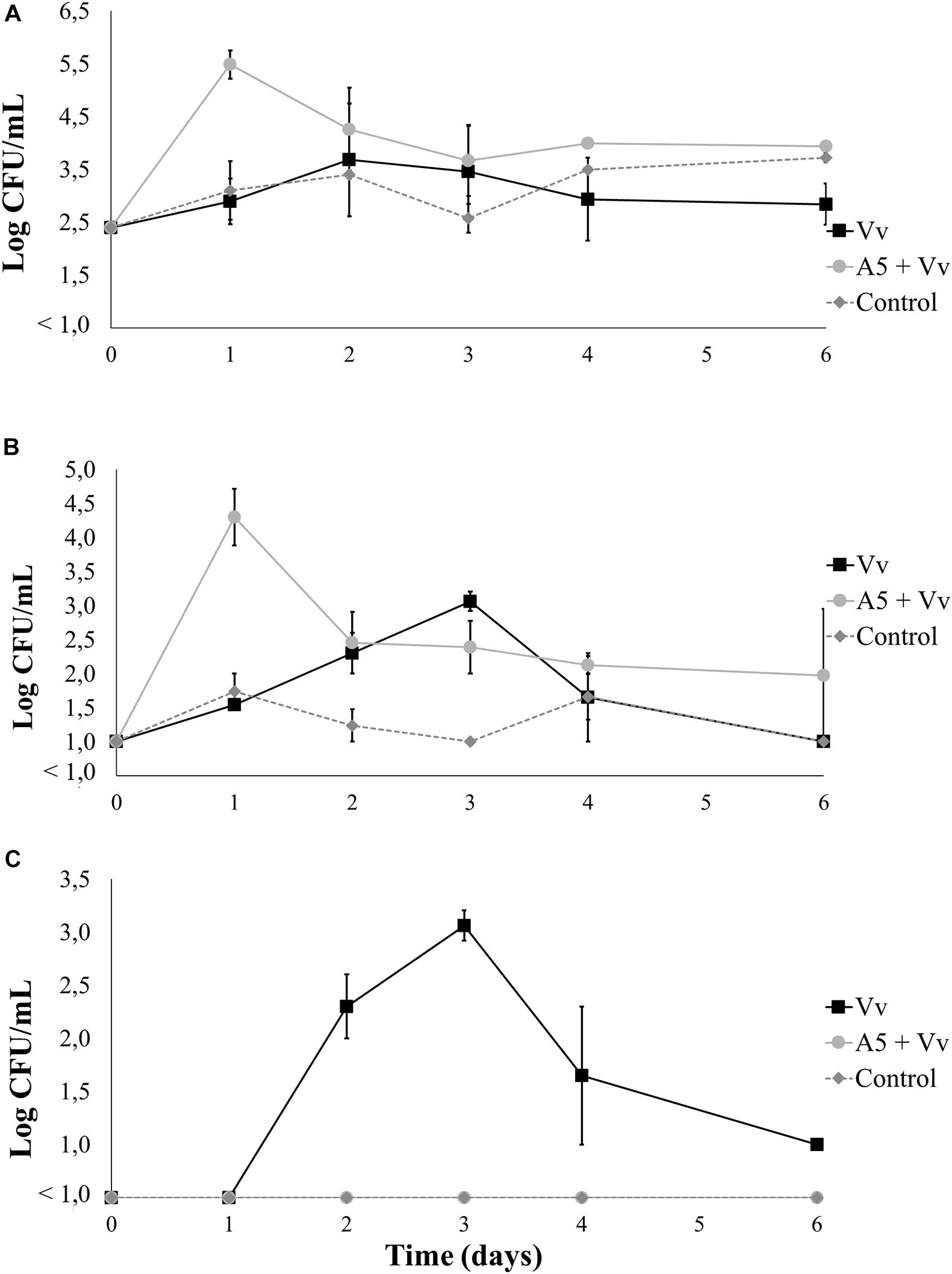
Figure 9. Total aerobic bacteria (A), total vibrio (B), and Vibrio vulnificus (C) levels in seawater from bioassay 3 (inoculation with A5 prior to inoculation with V. vulnificus). Three treatments are shown: Vv inoculated with V. vulnificus only (■), A5 + Vv first inoculated with A5 and then with V. vulnificus (•), and control (◆ dotted line). The absence of colonies is represented as below the limit of detection (LOD) (<1.0 log CFU/g).
Discussion
The majority of studies oriented to the control of bacterial pathogens in live seafood have focused on the use of immunostimulants, antibiotics, and other chemicals (Elston and Ford, 2011) that can cause bacterial resistance and environmental pollution. Therefore, the use of innocuous biocontrol agents stands out as an environment-friendly strategy against pathogens (Verschuere et al., 2000). In this study, we evaluate the use of the B. amyloliquefaciens A5 as an alternative to control V. vulnificus in oysters. Total aerobic counts in autoclaved and live oysters were used as an indicator of the A5 levels during the study. Total vibrio levels were also analyzed to assess the impact of A5 in this bacteria group, as various Vibrio spp. are of high importance to the oyster industry.
In vitro Biocontrol Tests
In culture media, A5 was able to inhibit the growth of V. vulnificus, but cell-free supernatants from A5 cultures did not cause inhibition. Results suggest that the potential inhibitors were only produced in agar or lost during the cell removal procedures, as well as indicated the need for viable A5 cells to produce the inhibition. Results are similar to those reported in previous studies showing that live lactic acid bacteria but not their metabolites were able to control the growth of pathogens such as V. parahaemolyticus (Hwanhlem et al., 2010).
Biocontrol Tests in Autoclaved Oysters
Autoclaved oysters were used as a follow-up study of the in vitro tests in order to assess the inhibition of A5 co-inoculated with V. vulnificus in oyster meat. The order of bacterial colonization was not assessed in autoclaved oysters, as only live individuals are able to filter seawater and concentrate bacteria in their bodies. When using autoclaved oysters and seawater, high levels of yellow colonies were consistently recovered on TCBS after treatment with A5. This was an unexpected result, as uninoculated-autoclaved oysters showed no bacterial growth and A5 was not able to grow on TCBS. Results suggest that the natural vibrio flora was not eliminated by autoclaving, and surviving cells remained below the LOD in the oysters. Pathogens can survive heat treatments—thanks to the protection from the food matrix, and populations of Vibrio spp. were probably preserved by the protein and lipid chains in the oysters (Pelon et al., 2005; Seo et al., 2005, 2010, 2011). After sterilization at 121°C for 15 min, the oyster’s tissue turned into a paste-like suspension. Higher sterilization temperatures and times may be needed to completely eliminate the vibrio load from the oysters but were not tested in this study to prevent additional alterations in the composition of the mollusk (Shim et al., 2015).
Despite that interfering microorganisms were not fully eliminated, the strain A5 produced a significant reduction of V. vulnificus in autoclaved oysters on day 2, but no reduction was observed from day 3 onward. The V. vulnificus inhibition by A5 in autoclaved oysters was probably affected by the surviving bacteria from the mollusk. Results are in agreement with previous studies showing the loss of the inhibition effect from biocontrol agents over time, suggesting the need of continuous reinoculations of the beneficial bacteria (Hong et al., 2005; Lalloo et al., 2007; Karim et al., 2013; Nemutanzhela et al., 2014).
Biocontrol Tests in Live Oysters
The levels of FCBC below 107 CFU/ml of V. vulnificus yielded negligible recovery counts of the vibrio from oysters. High levels of bacteria have been needed for the inoculation of bivalves in previous studies (Herrfurth et al., 2013), as inoculum levels are usually affected by the oyster immune system (Kaysner et al., 1989) and the changes in the physiological state of the inoculated V. vulnificus (Quevedo et al., 2005).
The data from the three assays carried out using live oysters suggest that A5 was harmless to the mollusk, as a 100% survival rate was observed among the individuals inoculated with a high dose (∼6 log CFU/g) of the biocontrol agent. This is consistent with previous reports, proposing the use of Bacillus spp. as probiotics in aquaculture and regarding B. amyloliquefaciens as a non-pathogenic bacterium (Lončar et al., 2014). The first bioassay showed that the total aerobic levels of oysters from the A5 treatment increased at day 2 but decreased thereafter (Figure 4A), suggesting the need of reinoculations with A5 for commercial uses. Continuous reinoculation of biocontrol agents including the application of Bacillus-based products has been described as a common practice in aquaculture (Hong et al., 2005; Lalloo et al., 2007; Karim et al., 2013; Nemutanzhela et al., 2014). Similarly, the levels of V. vulnificus in oysters decreased slowly after inoculation (Figure 4C), suggesting the activation of the mollusk’s defense mechanisms such as the hemocyte-mediated phagocytosis against V. vulnificus (Genthner et al., 1999; Canesi et al., 2002; Fuhrmann et al., 2018). However, the inability of some hemocytes to kill V. vulnificus has been observed, and the pathogen population may not be completely eliminated (Froelich and Oliver, 2013). Despite the slow decrease in the levels of the inoculated bacteria, the data suggest that both A5 and V. vulnificus can colonize the oyster’s tissues. Results are in agreement with those of previous studies showing that oysters can concentrate bacteria from the surrounding water in a matter of hours (Froelich and Oliver, 2013) and that probiotic microorganisms have the ability to adhere to the mucous epithelium of the host’s gastrointestinal tract (Chi et al., 2014). Further research is needed to assess the adherence of A5 to oysters’ gastrointestinal tracts.
Results from bioassay 2 showed that A5 did not exert a biocontrol effect in oysters previously inoculated with V. vulnificus (Figure 6C). Vibrio populations previously adapted to oyster tissues have shown the ability to prevent the colonization of beneficial bacteria (Verschuere et al., 2000; Froelich and Oliver, 2013), and V. vulnificus probably prevented A5 to grow to high enough concentrations to produce the antagonizing effect. Probiotic bacteria usually require pre-colonization of surfaces or tissues of the animal to inhibit pathogen colonization and growth (Karim et al., 2013; Zhao et al., 2016). Rather than a biocontrol effect, A5 significantly stimulated the growth of TCBS’s yellow colonies and total aerobic bacteria in both oysters and water on days 3 and 4, respectively. Results are similar to those from a previous study showing that probiotics can increase Vibrio diversity in aquaculture (Stevick et al., 2019). Further research is needed to determine the effect of A5 in vibrio populations.
The data from bioassay 3 showed that V. vulnificus was not able to colonize the oyster’s tissue treated with A5 (Figure 8C). Results suggest competitive inhibition against V. vulnificus or an increased immune response in the oysters promoted by A5 (Vanbelle et al., 1990). The A5 strain probably competed for the adhesion sites in the intestinal tissues or other oyster surfaces, preventing the colonization by V. vulnificus (Gismondo et al., 1999; Verschuere et al., 2000). In contrast, when V. vulnificus was already in the tissue—as in bioassay 2—A5 probably failed to compete for the space that the vibrio has already colonized (Olsson et al., 1992; Verschuere et al., 2000). Additionally, the inoculation of Bacillus sp. in live seafood has been shown to improve the health of the host by stimulating its immune response (Rengpipat et al., 2000).
Strains of B. amyloliquefaciens are frequently commercialized as bioactive compound producers, and several patents involving food-safety applications of this species have been approved (Hsieh and Kao, 2010; Yaowei et al., 2011; Rasimus-Sahari et al., 2015). The safety of B. amyloliquefaciens applications in food products can be inferred, as pathogenicity of this bacterium has not been reported (Rasimus-Sahari et al., 2015), and the FDA has listed B. amyloliquefaciens as a food additive-producer with a “generally recognized as safe” (GRAS) status (Health Center for Devices and Radiological, 2018). Similarly, B. amyloliquefaciens has been regarded as non-pathogenic/non-toxigenic toward humans and proposed for the “qualified presumption of safety” (QPS) status by the European Food Safety Authority (EFSA) (EFSA Panel on Biological Hazards, 2013). An advantage of using Bacillus spp. as a biocontrol agent is that they are not generally involved in horizontal gene transfer processes with other organisms such as Vibrio spp. and are unlikely to acquire genes for antibiotic resistance or virulence from pathogens (Moriarty, 1999). However, further research is needed to assess the safety of the A5 strain of B. amyloliquefaciens.
Conclusion
Live cells of B. amyloliquefaciens A5 inhibited the growth of V. vulnificus in vitro, but no inhibition was observed when the metabolites from A5 were used. The strain A5 was able to colonize live oysters and prevented the colonization of V. vulnificus. However, A5 was not able to reduce the levels of the already colonizing cells of V. vulnificus in oysters, suggesting the need of inoculating the biocontrol agent at the early production stages. The levels of total vibrio (yellow colonies) increased significantly in oysters and seawater after inoculation with A5. Further research is needed to determine the pathogenicity of the Vibrio spp. favored by A5. Results are a preliminary indication of the potential of A5 to control V. vulnificus, but additional work needs to be done to determine the feasibility of this approach against multiple strains of the pathogen and in real production environments.
Data Availability Statement
The raw data supporting the conclusions of this article will be made available by the authors, without undue reservation.
Author Contributions
AF-P contributed to the experimental operation and analysis and interpretation of data and drafted the manuscript. JC-C contributed to the conception and design of the study. JC-C, AT, and JL-Z contributed to the guidance on experimental design and review and editing of the manuscript. All authors read and approved the final manuscript.
Funding
This research was partially supported by the Corporación Ecuatoriana para el Desarrollo de la Investigación y la Academia (Cuenca, Ecuador), grant number CEPRA XIII-2019-04, and by the VLIR–UOS grant “VLIR Network Ecuador.”
Conflict of Interest
The authors declare that the research was conducted in the absence of any commercial or financial relationships that could be construed as a potential conflict of interest.
Acknowledgments
This study was carried out within the framework of the joint postgraduate program VLIR Network Ecuador. The authors are thankful to the National Center for Aquaculture and Marine Research (CENAIM) for their valuable assistance in providing the oysters and seawater for the experiments. In addition, the authors thank the staff of the Biomedical Research Laboratory, the Pathology Laboratory, and the Laboratory of Ecotoxicology and Environmental Health of ESPOL for their valuable collaboration in lending their facilities and equipment for the preparation of bacterial samples.
References
Araujo, M. R., Aquino, C., Scaramal, E., Ciola, C., Schettino, G., and Machado, M. (2007). Vibrio vulnificus infection in São Paulo, Brazil: case report and literature review. Braz. J. Infect. Dis. 11, 302–305. doi: 10.1590/S1413-86702007000200029
Baffone, W., Tarsi, R., Pane, L., Campana, R., Repetto, B., Mariottini, G. L., et al. (2006). Detection of free-living and plankton-bound vibrios in coastal waters of the Adriatic sea (Italy) and study of their pathogenicity-associated properties. Environ. Microbiol. 8, 1299–1305. doi: 10.1111/j.1462-2920.2006.01011.x
Baker, G. L. (2016). Food safety impacts from post-harvest processing procedures of molluscan shellfish. Foods 5:29. doi: 10.3390/foods5020029
Barton, J. C., and Acton, R. T. (2009). Hemochromatosis and Vibrio vulnificus wound infections. J. Clin. Gastroenterol. 43, 890–893. doi: 10.1097/MCG.0b013e31819069c1
Bross, M. H., Soch, K., Morales, R., and Mitchell, R. B. (2007). Vibrio vulnificus infection: diagnosis and treatment. Am. Fam. Phys. 76, 539–544.
Canesi, L., Gallo, G., Gavioli, M., and Pruzzo, C. (2002). Bacteria–hemocyte interactions and phagocytosis in marine bivalves. Microsc. Res. Tech. 57, 469–476. doi: 10.1002/jemt.10100
Castellano, P., Pérez, M., Blanco, M., Fontana, C., and Vignolo, G. (2017). Strategies for pathogen biocontrol using lactic acid bacteria and their metabolites: a focus on meat ecosystems and industrial environments. Microorganisms 5:38. doi: 10.3390/microorganisms5030038
Cazorla, C., Guigon, A., Noel, M., Quilici, M. L., and Lacassin, F. (2011). Fatal Vibrio vulnificus infection associated with eating raw oysters, New Caledonia. Emerg. Infect. Dis. 17, 136–137. doi: 10.3201/eid1701.100603
CDC, (2018). Burden of Foodborne Illness: Findings | Estimates of Foodborne Illness | CDC. Available at: https://www.cdc.gov/foodborneburden/2011-foodborne-estimates.html (accessed November 19, 2018).
Cerdà-Cuéllar, M., Jofre, J., and Blanch, A. (2000). A selective medium and a specific probe for detection of Vibrio vulnificus. Appl. Environ. Microbiol. 66, 855–859. doi: 10.1128/aem.66.2.855-859.2000
Chen, Y., Satoh, T., and Tokunaga, O. (2002). Vibrio vulnificus infection in patients with liver disease: report of five autopsy cases. Virchows Arch. 441, 88–92. doi: 10.1007/s00428-002-0613-1
Chi, C., Jiang, B., Yu, X., Liu, T., Xia, L., and Wang, G. (2014). Effects of three strains of intestinal autochthonous bacteria and their extracellular products on the immune response and disease resistance of common carp, Cyprinus carpio. Fish Shellfish Immunol. 36, 9–18. doi: 10.1016/j.fsi.2013.10.003
Cruz-Romero, M., Kelly, A. L., and Kerry, J. P. (2007). Effects of high-pressure and heat treatments on physical and biochemical characteristics of oysters (Crassostrea gigas). Innov. Food Sci. Emerg. Technol. 8, 30–38. doi: 10.1016/j.ifset.2006.05.002
Dechet, A. M., Yu, P. A., Koram, N., and Painter, J. (2008). Nonfoodborne Vibrio infections: an important cause of morbidity and mortality in the United States, 1997-2006. Clin. Infect. Dis. 46, 970–976. doi: 10.1086/529148
DePaola, A., Jones, J. L., Woods, J., Burkhardt, W., Calci, K. R., Krantz, J. A., et al. (2010). Bacterial and viral pathogens in live oysters: 2007 United States market survey. Appl. Environ. Microbiol. 76, 2754–2768. doi: 10.1128/aem.02590-09
DePaola, A., Motes, M. L., Chan, A. M., and Suttle, C. A. (1998). Phages infecting Vibrio vulnificus are abundant and diverse in oysters (Crassostrea virginica) collected from the Gulf of Mexico. Appl. Environ. Microbiol. 64, 346–351. doi: 10.1128/aem.64.1.346-351.1998
EFSA Panel on Biological Hazards, (2013). Scientific opinion on the maintenance of the list of QPS biological agents intentionally added to food and feed (2013 update). EFSA J. 11:3449. doi: 10.2903/j.efsa.2013.3449
Elston, R. A., and Ford, S. E. (2011). “Shellfish diseases and health management,” in Shellfish Aquaculture and the Environment, ed. S. E. Shumway, (Hoboken, NJ: John Wiley & Sons), 359–394. doi: 10.1002/9780470960967.ch13
Fravel, D. R. (2005). Commercialization and implementation of biocontrol. Annu. Rev. Phytopathol. 43, 337–359. doi: 10.1146/annurev.phyto.43.032904.092924
Froelich, B., and Oliver, J. D. (2013). The interactions of Vibrio vulnificus and the oyster Crassostrea virginica. Microb. Ecol. 65, 807–816. doi: 10.1007/s00248-012-0162-3
Froelich, B. A., and Noble, R. T. (2014). Factors affecting the uptake and retention of Vibrio vulnificus in oysters. Appl. Environ. Microbiol. 80, 7454–7459. doi: 10.1128/aem.02042-14
Fuhrmann, M., Delisle, L., Petton, B., Corporeau, C., and Pernet, F. (2018). Metabolism of the pacific oyster, Crassostrea gigas, is influenced by salinity and modulates survival to the ostreid herpesvirus OsHV-1. Biol. Open 7:bio028134. doi: 10.1242/bio.028134
Gálvez, A., Abriouel, H., Benomar, N., and Lucas, R. (2010). Microbial antagonists to food-borne pathogens and biocontrol. Curr. Opin. Biotechnol. Food Biotechnol. Plant Biotechnol. 21, 142–148. doi: 10.1016/j.copbio.2010.01.005
Genovese, G., Faggio, C., Gugliandolo, C., Torre, A., Spanò, A., Morabito, M., et al. (2012). In vitro evaluation of antibacterial activity of Asparagopsis taxiformis from the straits of Messina against pathogens relevant in aquaculture. Mar. Environ. Res. 73, 1–6. doi: 10.1016/j.marenvres.2011.10.002
Genthner, F. J., Volety, A. K., Oliver, L. M., and Fisher, W. S. (1999). Factors influencing in vitro killing of bacteria by hemocytes of the eastern oyster (Crassostrea virginica). Appl. Environ. Microbiol. 65, 3015–3020. doi: 10.1128/aem.65.7.3015-3020.1999
Gismondo, M., Drago, R. L., and Lombardi, A. (1999). Review of probiotics available to modify gastrointestinal flora. Int. J. Antimicrob. Agents 12, 287–292. doi: 10.1016/s0924-8579(99)00050-3
Halimi, B., Dortu, C., Arguelles-Arias, A., Thonart, P., Joris, B., and Fickers, P. (2010). Antilisterial activity on poultry meat of amylolysin, a bacteriocin from Bacillus amyloliquefaciens GA1. Probiotics Antimicrob. Proteins 2, 120–125. doi: 10.1007/s12602-010-9040-9
Haq, S. M., and Dayal, H. (2005). Chronic liver disease and consumption of raw oysters: a potentially lethal combination–a review of Vibrio vulnificus septicemia. Am. J. Gastroenterol. 100, 1195–1199. doi: 10.1111/j.1572-0241.2005.40814.x
Health Center for Devices and Radiological, (2018). Code of Federal Regulations - Title 21 - Food and Drugs. Silver Spring, MD: FDA.
Herrfurth, D., Oeleker, K., Pund, R. P., Strauch, E., Schwartz, K., Kleer, J., et al. (2013). Uptake and localization of Vibrio cholerae, Vibrio parahaemolyticus, and Vibrio vulnificus in blue mussels (Mytilus edulis) of the Baltic Sea. J. Shellfish Res. 32, 855–859. doi: 10.2983/035.032.0329
Hewitt, W., and Vincent, S. (1989). Theory and Application of Microbiological Assay. Cambridge, MA: Academic Press.
Hoffmann, S. A., Maculloch, B., and Batz, M. B. (2015). Economic Burden of Major Foodborne Illnesses Acquired in the United States. Economic Information Bulletin No. 140. Scotts Valley, CA: CreateSpace.
Hong, H. A., Duc, L. H., and Cutting, S. M. (2005). The use of bacterial spore formers as probiotics. FEMS Microbiol. Rev. 29, 813–835. doi: 10.1016/j.femsre.2004.12.001
Horseman, M. A., and Surani, S. (2011). A comprehensive review of Vibrio vulnificus: an important cause of severe sepsis and skin and soft-tissue infection. Int. J. Infect. Dis. 15, e157–e166. doi: 10.1016/j.ijid.2010.11.003
Hsieh, F. C., and Kao, S. (2010). Novel strain of Bacillus amyloliquefaciens and its use. U.S. Patent No 20,100,143,316. Washington, DC: U.S. Patent and Trademark Office.
Hu, H. Q., Li, X. S., and He, H. (2010). Characterization of an antimicrobial material from a newly isolated Bacillus amyloliquefaciens from mangrove for biocontrol of capsicum bacterial wilt. Biol. Control 54, 359–365. doi: 10.1016/j.biocontrol.2010.06.015
Huehn, S., Eichhorn, C., Urmersbach, S., Breidenbach, J., Bechlars, S., Bier, N., et al. (2014). Pathogenic vibrios in environmental, seafood and clinical sources in Germany. Int. J. Med. Microbiol. 304, 843–850. doi: 10.1016/j.ijmm.2014.07.010
Hwanhlem, N., Watthanasakphuban, N., Riebroy, S., Benjakul, S., Kittikun, A., and Maneerat, S. (2010). Probiotic lactic acid bacteria from kung-som: isolation, screening, inhibition of pathogenic bacteria. Int. J. Food Sci. Technol. 45, 594–601. doi: 10.1111/j.1365-2621.2010.02172.x
Jones, M. K., and Oliver, J. D. (2009). Vibrio vulnificus: disease and pathogenesis. Infect. Immun. 77, 1723–1733. doi: 10.1128/iai.01046-08
Karim, M., Zhao, W., Rowley, D., Nelson, D., and Gomez-Chiarri, M. (2013). Probiotic strains for shellfish aquaculture: protection of eastern oyster, Crassostrea virginica, larvae and juveniles againsl bacterial challenge. J. Shellfish Res. 32, 401–408. doi: 10.2983/035.032.0220
Kaysner, C. A., and DePaola, A. (2004). Bacteriological Analytical Manual Chapter 9 Vibrio. Silver Spring, MD: U.S. Food and Drug Administration.
Kaysner, C. A., Tamplin, M. L., Wekell, M., Stott, R. F., and Colburn, K. G. (1989). Survival of Vibrio vulnificus in shellstock and shucked oysters (Crassostrea gigas and Crassostrea virginica) and effects of isolation medium on recovery. Appl. Environ. Microbiol. 55, 3072–3079. doi: 10.1128/aem.55.12.3072-3079.1989
Kelly, M. T. (1982). Effect of temperature and salinity on Vibrio (Beneckea) vulnificus occurrence in a gulf coast environment. Appl. Environ. Microbiol. 44, 820–824. doi: 10.1128/aem.44.4.820-824.1982
Kim, J. H., Choresca, C. H. Jr., Shin, S. P., Han, J., Jun, J. W., and Park, S. (2011). Occurrence and antibiotic resistance of Vibrio vulnificus in seafood and environmental waters in Korea. J. Food Saf. 31, 518–524. doi: 10.1111/j.1745-4565.2011.00329.x
Lalloo, R., Ramchuran, S., Ramduth, D., Görgens, J., and Gardiner, N. (2007). Isolation and selection of Bacillus Spp. as potential biological agents for enhancement of water quality in culture of ornamental fish. J. Appl. Microbiol. 103, 1471–1479. doi: 10.1111/j.1365-2672.2007.03360.x
Lee, H. S., Choi, S., and Choi, S. H. (2012). Complete genome sequence of Vibrio vulnificus bacteriophage SSP002. J. Virol. 86:7711. doi: 10.1128/jvi.00972-12
Lee, H. S., Choi, S., Shin, H., Lee, J., and Choi, S. H. (2014). Vibrio vulnificus bacteriophage SSP002 as a possible biocontrol agent. Appl. Environ. Microbiol. 80, 515–524. doi: 10.1128/aem.02675-13
Lončar, N., Gligorijević, N., Božić, N., and Vujčić, Z. (2014). Congo red degrading laccases from Bacillus amyloliquefaciens strains isolated from salt spring in Serbia. Int. Biodeterior. Biodegradation 91, 18–23. doi: 10.1016/j.ibiod.2014.03.008
López, A. J., García, A. M., and Rojas, J. (eds). (2005). “Evaluación de dos metodologías para determinar la actividad antimicrobiana de plantas medicinales,” in Boletín Latinoamericano y Del Caribe de Plantas Medicinales y Aromáticas, (Santiago: Universidad de Santiago de Chile).
Mahmud, Z. H., Neogi, S. B., Kassu, A., Huong, B., Jahid, I., Islam, M., et al. (2008). Occurrence, seasonality and genetic diversity of Vibrio vulnificus in coastal seaweeds and water along the Kii Channel, Japan. FEMS Microbiol. Ecol. 64, 209–218. doi: 10.1111/j.1574-6941.2008.00460.x
Moriarty, D. (1999). “Disease control in shrimp aquaculture with probiotic bacteria,” in Proceedings of the 8th International Symposium on Microbial Ecology. Atlantic Canada Society for Microbial Ecology Halifax, Canada, 237–243.
Na, H. S., Cha, M. H., Oh, D. R., Cho, C., Rhee, J. H., and Kim, Y. R. (2011). Protective mechanism of curcumin against Vibrio vulnificus infection. FEMS Immunol. Med. Microbiol. 63, 355–362. doi: 10.1111/j.1574-695X.2011.00855.x
Nemutanzhela, M. E., Roets, Y., Gardiner, N., and Lalloo, R. (2014). “The use and benefits of Bacillus based biological agents in aquaculture,” in Sustainable Aquaculture Techniques, ed. M. Hernandez-Vergara (London, UK: IntechOpen), 1–33. doi: 10.5772/57375
Olsson, J. C., Westerdahl, A., Conway, P. L., and Kjelleberg, S. (1992). Intestinal colonization potential of turbot (Scophthalmus maximus)- and dab (Limanda limanda)-associated bacteria with inhibitory effects against Vibrio anguillarum. Appl. Environ. Microbiol. 58, 551–556. doi: 10.1128/aem.58.2.551-556.1992
Park, S. Y., Chung, M. S., and Ha, S. (2018). Combined effect of sodium hypochlorite and gamma-irradiation for the control of Vibrio vulnificus in fresh oyster and clam. LWT 91, 568–572. doi: 10.1016/j.lwt.2018.01.087
Pelon, W., Luftig, R. B., and Johnston, K. H. (2005). Vibrio vulnificus load reduction in oysters after combined exposure to Vibrio vulnificus–specific bacteriophage and to an oyster extract component. J. Food Prot. 68, 1188–1191. doi: 10.4315/0362-028x-68.6.1188
Phuvasate, S., Chen, M. H., and Su, Y. (2012). Reductions of Vibrio parahaemolyticus in pacific oysters (Crassostrea gigas) by depuration at various temperatures. Food Microbiol. 31, 51–56. doi: 10.1016/j.fm.2012.02.004
Porsby, C. H., and Gram, L. (2016). Phaeobacter inhibens as biocontrol agent against Vibrio vulnificus in oyster models. Food Microbiol. 57, 63–70. doi: 10.1016/j.fm.2016.01.005
Pu, M., Duriez, P., Arazi, M., and Rowe-Magnus, D. (2018). A conserved tad pilus promotes Vibrio vulnificus oyster colonization. Environ. Microbiol. 20, 828–841. doi: 10.1111/1462-2920.14025
Quevedo, A. C., Smith, J. G., Rodrick, G. E., and Wright, A. C. (2005). Ice immersion as a postharvest treatment of oysters for the reduction of Vibrio vulnificus. J. Food Prot. 68, 1192–1197. doi: 10.4315/0362-028x-68.6.1192
Ramos, R. J., Miotto, M., Lagreze, F., Cirolini, A., Ferreira, J. F., and Werneck, C. (2012). Depuration of oysters (Crassostrea gigas) contaminated with Vibrio parahaemolyticus and Vibrio vulnificus with UV light and chlorinated seawater. J. Food Prot. 75, 1501–1506. doi: 10.4315/0362-028x.jfp-11-467
Rasimus-Sahari, S., Teplova, V., Andersson, M. A., Mikkola, R., Kankkunen, P., Matikainen, S., et al. (2015). The peptide toxin amylosin of Bacillus Amyloliquefaciens from moisture-damaged buildings is immunotoxic, induces potassium efflux from mammalian cells, and has antimicrobial activity. Appl. Environ. Microbiol. 81, 2939–2949. doi: 10.1128/aem.03430-14
Raszl, S. M., Froelich, B. A., Vieira, C. R., Blackwood, A. D., and Noble, R. T. (2016). Vibrio parahaemolyticus and Vibrio vulnificus in South America: water, seafood and human infections. J. Appl. Microbiol. 121, 1201–1222. doi: 10.1111/jam.13246
Rengpipat, S., Rukpratanporn, S., Piyatiratitivorakul, S., and Menasaveta, P. (2000). Immunity enhancement in black tiger shrimp (Penaeus monodon) by a probiont bacterium (Bacillus S11). Aquaculture 191, 271–288. doi: 10.1016/s0044-8486(00)00440-3
Richards, P. J., Connerton, P. L., and Connerton, I. F. (2019). Phage biocontrol of Campylobacter jejuni in chickens does not produce collateral effects on the gut microbiota. Front. Microbiol. 10:476. doi: 10.3389/fmicb.2019.00476
Rosche, T. M., Yano, Y., and Oliver, J. D. (2005). A rapid and simple PCR analysis indicates there are two subgroups of Vibrio vulnificus which correlate with clinical or environmental isolation. Microbiol. Immunol. 49, 381–389. doi: 10.1111/j.1348-0421.2005.tb03731.x
Scallan, E., Hoekstra, R. M., Angulo, F. J., Tauxe, R. V., Widdowson, M., Roy, S. L., et al. (2011). Foodborne illness acquired in the United States–major pathogens. Emerg. Infect. Dis. 17, 7–15. doi: 10.3201/eid1701.091101p1
Seo, J. K., Crawford, J. M., Stone, K. L., and Noga, E. J. (2005). Purification of a novel arthropod defensin from the American oyster, Crassostrea virginica. Biochem. Biophys. Res. Commun. 338, 1998–2004. doi: 10.1016/j.bbrc.2005.11.013
Seo, J. K., Stephenson, J., Crawford, J. M., Stone, K. L., and Noga, E. J. (2010). American oyster, Crassostrea virginica, expresses a potent antibacterial histone H2B protein. Mar. Biotechnol. 12, 543–551. doi: 10.1007/s10126-009-9240-z
Seo, J. K., Stephenson, J., and Noga, E. J. (2011). Multiple antibacterial histone H2B proteins are expressed in tissues of American oyster. Comp. Biochem. Physiol. B Biochem. Mol. Biol. 158, 223–229. doi: 10.1016/j.cbpb.2010.11.011
Serrano, L. (2014). Control Biológico de Patógenos de Camarón Mediante El Uso de Microorganismos Aislados de Muestras de Biol y Suelo de La Antártida, February. Available at: http://www.dspace.espol.edu.ec/handle/123456789/25086 (accessed February 13, 2014).
Shim, K. B., Kim, M., Park, K., Son, K., and Lim, C. (2015). Effect of heat treatment on biochemical characteristics of pacific oyster Crassostrea gigas. Korean J. Fish. Aquat. Sci. 48, 876–882. doi: 10.5657/KFAS.2015.0876
Song, B., Rong, Y., Zhao, M., and Chi, Z. (2013). Antifungal activity of the lipopeptides produced by Bacillus amyloliquefaciens anti-CA against Candida albicans isolated from clinic. Appl. Microbiol. Biotechnol. 97, 7141–7150. doi: 10.1007/s00253-013-5000-0
Stevick, R. J., Sohn, S., Modak, T. H., Nelson, D. R., Rowley, D. C., Tammi, K., et al. (2019). Bacterial community dynamics in an oyster hatchery in response to probiotic treatment. Front. Microbiol. 10:1060. doi: 10.3389/fmicb.2019.01060
Tirapé, A. (2007). Contribution à L’étude de L’ontogenčse Du Systčme Immunitaire Chez L’huître Creuse Crassostrea gigas. Ph.D. thesis, Montpellier 2 University, Montpellier.
Vanbelle, M., Teller, E., and Focant, M. (1990). Probiotics in animal nutrition: a review. Arch. Tierernahr. 40, 543–567. doi: 10.1080/17450399009428406
Verschuere, L., Rombaut, G., Sorgeloos, P., and Verstraete, W. (2000). Probiotic bacteria as biological control agents in aquaculture. Microbiol. Mol. Biol. Rev. 64, 655–671. doi: 10.1128/mmbr.64.4.655-671.2000
Wang, D., Yu, S., Chen, W., Zhang, D., and Shi, X. (2010a). Enumeration of Vibrio parahaemolyticus in oyster tissues following artificial contamination and depuration. Lett. Appl. Microbiol. 51, 104–108. doi: 10.1111/j.1472-765X.2010.02865.x
Wang, D., Zhang, D., Chen, W., Yu, S., and Shi, X. (2010b). Retention of Vibrio parahaemolyticus in oyster tissues after chlorine dioxide treatment. Int. J. Food Microbiol. 137, 76–80. doi: 10.1016/j.ijfoodmicro.2009.10.022
West, P. A., Russek, E., Brayton, P. R., and Colwell, R. (1982). Statistical evaluation of a quality control method for isolation of pathogenic Vibrio species on selected thiosulfate-citrate-bile salts-sucrose agars. J. Clin. Microbiol. 16, 1110–1116. doi: 10.1128/jcm.16.6.1110-1116.1982
Wong, K. C., Brown, A. M., Luscombe, G. M., Wong, S. J., and Mendis, K. (2015). Antibiotic use for Vibrio infections: important insights from surveillance data. BMC Infect. Dis. 15:226. doi: 10.1186/s12879-015-0959-z
Xu, H., Rong, Y., Zhao, M., Song, B., and Chi, Z. (2014). Antibacterial activity of the lipopeptides produced by Bacillus amyloliquefaciens M1 against multidrug-resistant Vibrio Spp. isolated from diseased marine animals. Appl. Microbiol. Biotechnol. 98, 127–136. doi: 10.1007/s00253-013-5291-1
Yaowei, K., Shawn, S., Jessica, S., and Michael, F. (2011). Bacillus amyloliquefaciens Strain. WO 2011/140051 A1. Available at: https://www.lens.org/lens/patent/WO_2011_140051_A1 (accessed May 3, 2011).
Yu, Y., Lee, C., Kim, J., and Hwang, S. (2005). Group-specific primer and probe sets to detect methanogenic communities using quantitative real-time polymerase chain reaction. Biotechnol. Bioeng. 89, 670–679. doi: 10.1002/bit.20347
Yun, N. R., and Kim, D. M. (2018). Vibrio vulnificus infection: a persistent threat to public health. Korean J. Intern. Med. 33, 1070–1078. doi: 10.3904/kjim.2018.159
Zetti, Z. R., Norazlah, B., and Raha, A. R. (2009). A fatal case of Vibrio vulnificus cellulitis with septicaemia. Med. J. Malaysia 64, 246–247.
Keywords: foodborne disease, vibriosis, shellfish, biocontrol, raw food
Citation: Freire-Peñaherrera AM, Tirapé A, Landívar-Zambrano J and Cevallos-Cevallos JM (2020) A Strain of Bacillus amyloliquefaciens Can Prevent Vibrio vulnificus Colonization in Crassostrea gigas Oysters. Front. Mar. Sci. 7:596343. doi: 10.3389/fmars.2020.596343
Received: 19 August 2020; Accepted: 27 October 2020;
Published: 26 November 2020.
Edited by:
Johannes F. Imhoff, GEOMAR Helmholtz Centre for Ocean Research Kiel, GermanyReviewed by:
Jessica L. Jones, United States Food and Drug Administration, United StatesDavid R. Nelson, University of Rhode Island, United States
Copyright © 2020 Freire-Peñaherrera, Tirapé, Landívar-Zambrano and Cevallos-Cevallos. This is an open-access article distributed under the terms of the Creative Commons Attribution License (CC BY). The use, distribution or reproduction in other forums is permitted, provided the original author(s) and the copyright owner(s) are credited and that the original publication in this journal is cited, in accordance with accepted academic practice. No use, distribution or reproduction is permitted which does not comply with these terms.
*Correspondence: Juan Manuel Cevallos-Cevallos, am1jZXZhQGVzcG9sLmVkdS5lYw==