- 1Institute for the Study of Anthropogenic Impacts and Sustainability in the Marine Environment, National Research Council, Trapani, Italy
- 2Department of Life Sciences and Systems Biology, University of Torino, Torino, Italy
- 3Jonian Dolphin Conservation, Taranto, Italy
- 4Department of Biology, University of Bari, Bari, Italy
- 5National Research Council, Bari, Italy
- 6Consorzio Nazionale Interuniversitarioper le Scienze del Mare (CoNISMa), Roma, Italy
Many gregarious species require complex patterns of communication for maintaining coordinated behaviors, articulated social structure and group cohesion. In mammal species, social complexity has been considered the driving force for the development of advanced acoustic communication systems. Striped dolphins are highly social, showing large group size with females maintaining strong bonds with kin. Here, we tested the hypothesis that more complex acoustic pattern plays a key role in social activity in the striped dolphins. The production rate of clicks, whistles and burst pulses, and the acoustic features of whistles have been related to the activity context (feeding, traveling, resting, and socializing). Furthermore, complex calls, consisting of a combination of frequency-modulated, and/or pulsed components were detected. Higher whistles and burst pulses production rates were recorded during socializing. Also, the social activity can be discriminated basing on the modulation of the whistle contour. Biphonic calls were especially recorded during social interaction events, suggesting that these phenomena can encode information about individual or group identity to conspecifics. Outcomes indicate the pivotal role of vocal complexity during social context and elicit further investigations of the communication system of small odontocetes from local to wider spatial scale.
Introduction
Group living and the relationships between individuals imply the need to recognize each other and to strengthen bonds (Kappeler, 2019). Many gregarious species require complex patterns of communication for maintaining coordinated behaviors, articulated social structure, and group cohesion (Brudzynski, 2014). Indeed, communication is pivotal in regulating dynamics within a social group (Fichtel and Manser, 2010). Social complexity has been considered the driving force for the development of advanced acoustic communication systems (Blumstein and Armitage, 1997; Freeberg et al., 2012a; Van Cise et al., 2018; Gustison et al., 2019). The “social complexity hypothesis for communicative complexity (SCHCC)” posits that variations in communication systems develop due to social structuring and that more complex social structures can lead to more complex communication systems (Freeberg et al., 2012a; Bouchet et al., 2013). This approach bases on comparative research among species with different levels of social complexity. For instance, analyses on the vocalizations of several non-human primate species revealed a positive correlation between the acoustic repertoire size, group size, and time spent grooming, suggesting that vocal communication system may shape increases in the levels of social relationships (McComb and Semple, 2005). Also, social rodents showed a higher number of different call types compared to the solitary species (Vanden Hole et al., 2014).
However, estimating social and communicative complexity faces a rising definition issue across taxa. A system is generally considered more complex if it is made up of more parts, has more connections between parts and vary greatly (Peckre et al., 2019). A first approximation to estimating complexity has been based on the group size, on the number of units and their connections (Peckre et al., 2019), on the counting of discrete behaviors and how they are arranged (Blumstein et al., 1997), or on other social features, such as the type of relationships among organisms (Kappeler, 2019). Proxies of communicative complexity were identified especially in the vocal repertoire size, in the number of signaling units, in the call rate and its variability, in the combination of the call (simultaneous or in sequence), in their stereotypy or flexibility, and the tonal sound modulation (Peckre et al., 2019).
However, since signaling is likely the result of a combination of several selective pressures, among which ecology, morphology and phylogenetic (Freeberg et al., 2012b; Ord and Garcia-Porta, 2012; Ramsier et al., 2012; Manser et al., 2014), how social factors directly affects signal variation may be poorly understood. The study of communication signals during different social activities can reveal specific links and dynamic changes in signal structure across contexts (Wilkins et al., 2015; Papale et al., 2017). Signals can connect several specimens within a group that may communicate simultaneously. Therefore, isolating individuals to study the acoustic repertoire might prevent uncover communication interactions, especially in species living in large groups.
This is true for dolphin species that show extensive range in social group size and aggregations, in which individuals maintain dynamic relations and affiliations (Gazda et al., 2015). Vocalizations are a pervasive feature of odontocete social life and vocal learning has also been recorded (Deecke et al., 2000; Filatova et al., 2010; Musser et al., 2014). They use acoustic signals to interact with conspecifics and produce a variety of sounds, categorized as clicks, whistles, and burst pulses (Janik, 2009; Jones et al., 2020). However, not all odontocetes’ vocal events fit into this classification. Bottlenose dolphins, for example, produce a variety of multi-unit signals, called bray series (dos Santos et al., 1995; Luís et al., 2019; Jones et al., 2020). Killer and false killer whales emit complex calls as a continuum from whistles to pulses or vice-versa (Murray et al., 1998; Wellard et al., 2015, 2020), by changing frequency modulation and inter-pulse intervals. Furthermore, two sounds can be produced simultaneously (also in a stereotyped manner) in the form of two overlapped tonal signals, or combined whistle-burst pulses, or whistle-click train (Wellard et al., 2020; Jones et al., 2020). Biphonation is a phenomenon-occurring in the repertoire of several mammalian species. Among the odontocetes, it was identified for Tursiops truncatus (Reiss, 1988; Bojanowski et al., 2000; Kriesell et al., 2014; Papale et al., 2015), Orcinus orca (Filatova et al., 2009), Stenella frontalis (Herzing, 1996; Evans-Wilent and Dudzinski, 2013; Kaplan et al., 2018), Grampus griseus (Corkeron and Van Parijs, 2001), and Inia araguaiaensis (Melo-Santos et al., 2019).
The tonal sounds, like whistles, are the signals more studied as implicated in communication efforts, and their modulation complexity has been related to the evolutionary level of social structure (May-Collado et al., 2007). Furthermore, signature whistles, individually distinctive stereotyped tonal signals, have been found in some delphinid species (Caldwell and Caldwell, 1965; Janik and Slater, 1998; van Parijs and Corkeron, 2001) often produced in sequences with 1–10 s intervals between them (Janik et al., 2013).
Some species (such as G. griseus, Cephalorhynchus hectorii, S. frontalis, T. truncatus), use also, or almost exclusively, burst pulses (signals made up of a series of clicks) to coordinate during some behaviors and to support social interactions (Dawson, 1991; Herzing, 1996; Thomas et al., 2002; Lammers et al., 2003; La Manna et al., 2013; Papale et al., 2017). Moreover, biphonic events have been suggested to provide directionality and identity cues (Filatova et al., 2013; Papale et al., 2015; Kaplan et al., 2018), which are crucial during social interactions in large groups.
From Norris et al. (1994), a relation was observed among the level of social activity and the complexity of the vocal pattern in terms of calling rate (dos Santos et al., 2005), whistle modulation (May-Collado et al., 2007) and presence and number of complex signaling (Nemiroff, 2009; Wellard et al., 2020), defined as the combination of more than one signal or component, often stereotypically repeated and overlapped (Hebets and Papaj, 2005). In particular, a higher repetition rate and markedly modulated tonal signals were recorded at increasing social interactions for Beluga whale (Panova et al., 2012), T. truncatus (Hawkins, 2010; López, 2011), Globicephala macrorhynchus (Sayigh et al., 2013; Zwamborn and Whitehead, 2017), Sotalia guianensis (May-Collado, 2013), Sousa chinensis (van Parijs and Corkeron, 2001), and S. frontalis (Herzing, 1996; Dudzinski, 1998; Papale et al., 2017; Kaplan et al., 2018). This increased complexity has been observed not only during the so-called socializing behavior (i.e., when animals are engaged in direct physical contact, mating, chasing, body inspections toward each other), but also during cooperative interactions functional to feeding, or coordination during movements (King and Janik, 2015; Eskelinen et al., 2016).
Even if the striped dolphin S. coeruleoalba is a worldwide species, living in large social groups, there is a distinct paucity of studies showing its vocal complexity. The species is highly social, showing large group sizes (in the Mediterranean from less than 20 to more than 200 animals) and females maintaining stronger bonds with female kin than with males (Gaspari et al., 2007). For this species, the study of acoustic repertoire, in terms of patterns and structural features of sounds in relation to behaviors, can shed light on the role of social communication in the group dynamics and can represent a crucial step for effective Passive Acoustic Monitoring programs. However, although few studies described striped dolphin’s sounds (Kastelein et al., 2003; Oswald et al., 2003, 2007; Gannier et al., 2010; Papale et al., 2013a, 2015; Azzolin et al., 2014), currently, no data link the behavior to the acoustic complexity. To that regard, this study aims to evaluate the hypothesis that striped dolphin’s vocal patterns can function as a cue to acoustically distinguish social and other behavioral activity. Therefore, we focused on the calling rate of clicks, whistles and burst pulses, on whistle’s modulation, and the type and occurrence of complex calls, in particular biphonating events.
Materials and Methods
Study Area
The Gulf of Taranto (Northern Ionian Sea, Central-Eastern Mediterranean Sea) covers an area of approximately 14,000 km2 from Santa Maria di Leuca to Punta Alice (Figure 1). A narrow continental shelf with a steep slope and several channels characterizes the western sector, while the eastern sector shows descending terraces toward the “Taranto Valley,” a NW–SE submarine canyon with no clear bathymetric connection to a major river system (Capezzuto et al., 2010; Russo et al., 2017; Carlucci et al., 2018a). The striped dolphin regularly occurs in the northernmost portion of the Taranto Valley (Azzolin et al., 2020), most likely attracted by the high productivity of the sub-marine canyon system and its upwelling currents (Carlucci et al., 2018c).
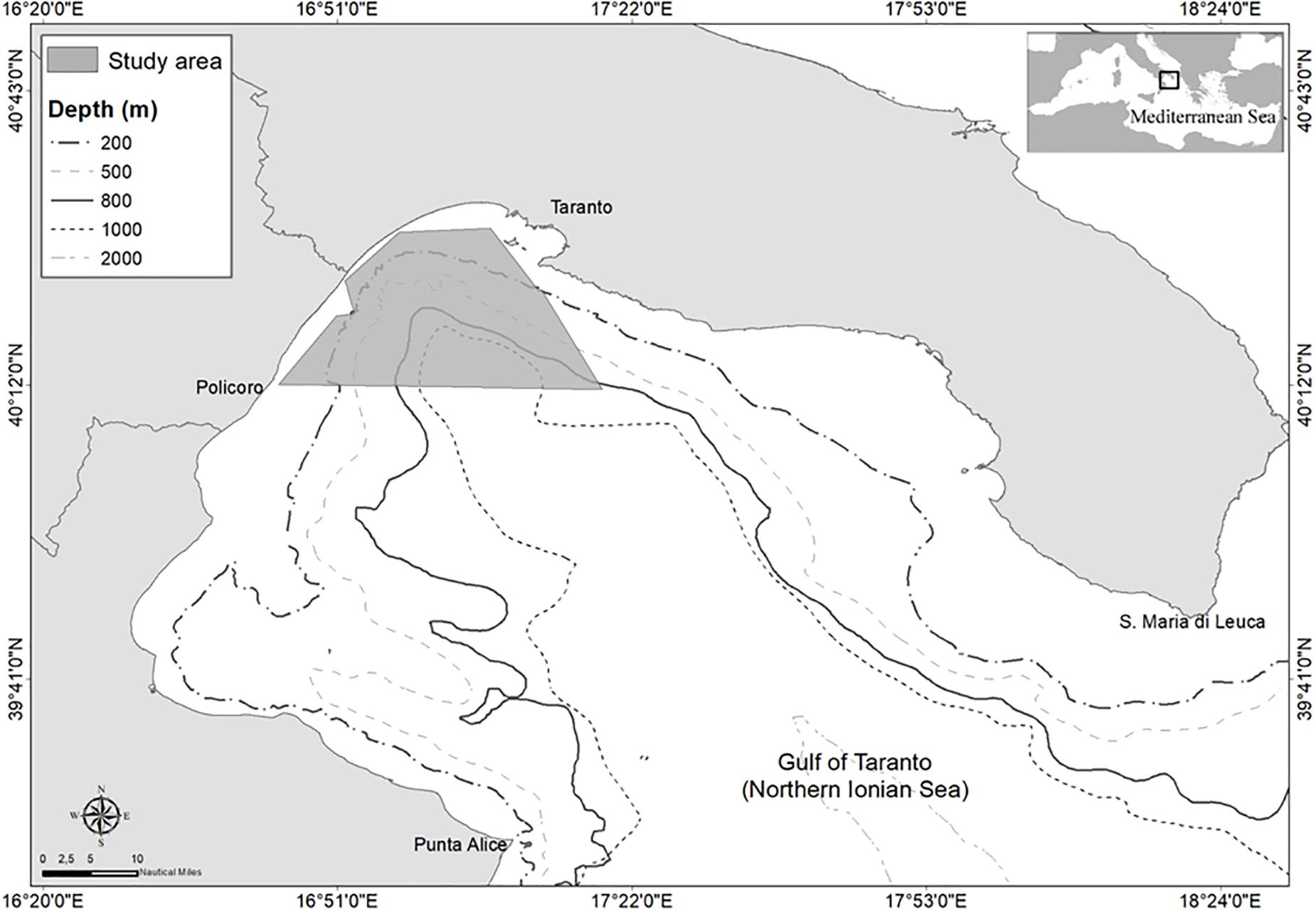
Figure 1. Map of the study area. In the square, the survey area investigated in 2016–2017 in the Gulf of Taranto (Central-Eastern Mediterranean Sea).
Data Collection
Acoustic recordings and behavioral data were collected during standardized vessel-based surveys carried out in summers 2016, 2017, and 2019, investigating an area of about 960 km2. The sampling effort was set at about 5 h/day along 35 nautical miles. Speed was maintained between 7 and 8 knots and trips occurred only in favorable sea-weather conditions (Douglas scale ≤ 3 and Beaufort scale ≤ 4). On board of a 12 m catamaran research vessel equipped with two 75 hp inboard engines, three experienced observers monitored the sea surface with the naked eye or 7 × 50 binoculars. One observer searched for targets around 180° and counted dolphins during each sighting, while the others supported the activities of the former, searching in a sector from the track-line to 90° on the starboard and port sides, respectively. When a group of dolphins was encountered, it was followed switching to off-effort (Irvine et al., 1981; Buckland et al., 2004). Date, sea-weather condition, geographic coordinates, depth (m), time of first contact, and group size (number of individuals) were recorded. A group was defined as dolphins within approximately 100 m radius of each other (Irvine et al., 1981) that were observed in apparent association, moving in the same direction and often, but not always, engaged in the same activity (Shane, 1990). Acoustic data and behavioral pattern were recorded with the engine switched off to exclude the disturbance in recordings and minimize the impact on dolphin’s activities.
The sampling of activity state of the focal group was carried out by scanning instantaneously the focal group and then, assigning the predominant group activity state. This step was repeated every 3 min for at least 5 times (for a total session of 15 min during which acoustic data were simultaneously recorded). According to Altmann (1974), it should be considered that in an ideal instantaneous sampling, the state of every individual in the group would be noted at the same moment. In practice, however, the observation, classification, and recording of a state takes time (from few seconds to minutes) especially when a group was composed of many individuals. After recording the predominant activity state for each instantaneous scan (Table 1 according to Carlucci et al., 2018b), the prevalent behavioral category during the entire session was considered as the activity state of the focal group during the encounter. Thus, for example if we have recorded 3 out of 5 occasions the socializing category, the prevalent behavior in that encounter was defined as socializing. Sightings were excluded when the predominant behavior was not undoubted defined. However, in this study only in 2 out of 32 sightings, we have recorded a change in the behavioral state.
The focal group was defined as aggregation of dolphins engaged in the same activity within 100 m from the boat. This prevent possible bias in the identification of behavioral activity during sightings with a group size greater than 100 dolphins. We limited the focal group to reduce the error that can deriving in observing a large group (that can extend well beyond 100 m from the boat) and in associating to them the vocalizations recorded from the boat.
Since the group size estimation ranged from 10 to 80 individuals, three classes were considered as: group 1 = × < 20 individuals, group 2 = 20 ≤ × < 35, group 3 ≥ 35. Basing on data, this classification allowed to lump data in well separated groups, preventing close boundaries among them.
Acoustic data were collected simultaneously to behavioral data, by using a mobile recording system made up of a pre-amplified omnidirectional hydrophone (ColmarGP0190) with a sensitivity of –175 ± 5 dB re 1 V/μPa among 5 and 170 kHz, a 20 m cable, and an onboard junction box with a USB cable connected to a laptop for data recording and preliminary analysis through its monitoring software. The software allowed a real time monitoring via time domain waveform, audio listening and the spectrogram of the signal. Also, an autonomous recorder RASP12 (NautaTM) was deployed from the boat during the sightings, for a set of data during summer 2017, by using the same data collection protocol. It was made up of a pre-amplified omnidirectional hydrophone (Sensor Technology SQ26-05), with a sensitivity of −168.8 ± 5 dB re 1 V/μPa among 100 Hz and 50kHz. Recordings were digitized at a sampling rate of 500 kHz with the first instruments, and of 96 kHz with the second.
Data Analysis
All the recordings were down sampled at 96 kHz to homogenize the dataset. Recordings were first manually analyzed using the spectrogram (time vs. frequency graph) view in iZotopeRX3 (iZotope, Inc., Cambridge, MA, United States) (1024 Fast Fourier Transform size and a Blackmann-Harris window), and only data coupled with behavioral data were selected.
For the single clicks and the train of clicks identification and count, a high pass filter of 5 kHz was applied. Clicks were identified through the Pulse Train Analysis in Avisoft SAS-Lab Pro (Avisoft Bioacoustics, Germany), using the methods of envelope modification “RMS+decimation” and of pulse detection “Peak search with Hysteresis,” setting the hysteresis at 12 dB, and the time constant at 0.3 ms. Due to the different sensitivities of the hydrophones used, two different energy thresholds were selected: 0.001 V for the first one and 0.03 V for the second. All the impulsive signals that overpassed this threshold were considered as possible clicks and labeled by the software. In order to count only single clicks or clicks emitted in train, excluding possible false positive errors due to noise presence or clicks emitted in burst pulses, an expert operator visually checked all the recordings, manually correcting the possible errors.
Whistles and burst pulses with a good signal-to-noise ratio [i.e., signals were considered only if they were well defined and prominent in intensity of at least 20 dB over the background noise (following Bonato et al., 2015)] were detected and counted through visual inspection of the recordings by two expert operators in iZotopeRX3. Signals too faint compared to the background noise or signals which complete contour could not be recognized were discarded (Papale et al., 2013a). Following this selection we could reasonably detect the acoustic emission of the focal group within 100 m from the boat (Papale et al., 2019).
Biphonating calls in the form of overlapped multiple frequency-modulated and or pulsed components were detected. Since group was often numerous, and signals can randomly overlap because of simultaneous emissions by more individuals, especially in the case of increasing arousal, we decided to be conservative and considered the call only if it was clearly independent by other signal emissions or if it was stereotypically repeated. The overlap of the components could not necessarily start and end together but were never recorded separately.
The calling rates of clicks, whistles, burst pulses and complex calls were calculated for each sighting as:
In order to measure the whistle parameters to describe signal complexity, signals not overlapping with others and completely recognizable in their time-frequency contour were further selected. Whistles considered with similar contours by two experienced observers were measured only once. Agreement between observers was evaluated and resulted 78%. The percent of agreement was defined as the number of agreements between observers in assigning contour similarity divided by the sum of both agreements and disagreements, and then multiplied by 100.
Ten parameters [time duration (in seconds), beginning frequency, end frequency, maximum, and minimum frequency of the contour (in Hz), frequency range (as the difference among maximum and minimum frequency), number of inflection points (defined as the point where a slope change occurred), number of steps (a discontinuity in frequency), number of maxima, and minima in the contour (local maxima and minima within the contour)] were manually measured by visual inspection of the spectrogram.
Kruskall Wallis tests were applied to point out any variations between behavioral categories in the whistle rate, burst pulses rate, biphonic signals rate, and in the parameters of whistles. The Tamhane post-hoc test was chosen for comparing data with unequal variance and unequal sample size. Since the number of clicks was automatically calculated by using two different thresholds in relation to the hydrophones and thus could influence the results, their calling rate under different behavioral contexts was examined by using Generalized Linear Mixed Models (GLMMs) with a Penalized-Quasi Likelihood (PQL) with glmmPQL package in R (R core Team). In this case, the variable hydrophone, was considered as a random effect of the model, and the behavior as fixed effect.
Moreover, a Stepwise Cross-Validated Discrimination Function Analysis was performed in order to investigate the possibility of distinguishing behavioral activities classes through the parameters of whistles. We considered each whistle within a recording in order to have the sizes of the dependent variables not grossly different and more than five times the number of independent variables. Furthermore, a large sample allow data distribution to be approximated to normality. The leave-one-out procedure (Lachenbruch and Mickey, 1968) was then used for cross validation.
Finally, Linear Models (LMs) were used to evaluate the single and combined effect of the activity classes and of the group size on the features of whistles. Parameters values were chosen as the response variable and tested as a function of the predictors “activity class” (discrete variable ranging from 1 to 4), and “group size” (discrete variable ranging from 1 to 3). A Gaussian distribution and identity link function were chosen. The model assumptions were checked by examining the observed versus predicted values plot (for linearity), the residuals versus predicted values plot (for homoscedasticity), and the normal Q–Q plot (for normality). The best fitting model was chosen basing on AICc criterion. Where ΔAICc < 2 between the best models, we used model averaging approach on the top set of models (Grueber et al., 2011) to obtain robust predictions. All the analyses were performed in R (R Core Team).
Results
Overall Results
A total of 6 h and 47 min of acoustic recordings of S. coeruleoalba were collected during 32 encounters. Selected periods of undoubted activity with simultaneous acoustic data were classified as follow: 5 during resting [20% group size 1 (<20 individuals), 60% group size 2 (20 ≤ × < 35 individuals), 20% group size 3 (>35 individuals)], 13 during socializing (15.5% group size 1, 30.7% group size 2, 53.8% group size 3), 10 during traveling (10% group size 1, 40% group size 2, 50% group size 3), and 4 during feeding (75% group size 1, 25% group size 2). The presence and number of vocalizations were summarized in Table 2, reporting the mean calling rate for each activity class. Only 422 whistles were selected for analyzing acoustic parameters. In details, 107 were collected during traveling, 37 during resting, 75 during feeding and 203 during socializing.

Table 2. Details of the number of encounters, group size and vocalization (mean (SD) calling rate (calls/[minutes*group size], and number of calls) per activity classes.
Biphonic calls recorded during the study were represented by signals often stereotyped, made up of a combination of whistle with burst pulsed, whistle with click train, or two tonal fundamental frequencies (Figure 2). As noted before, we univocally identified these signals only when clearly independent from other sounds and if they were repeated at least once. Even if this can lead to a lower count of these signals we preferred to be conservative in identifying the biphonation and not a random overlap of clicks and whistles as they both can frequently occur and coming from different specimens. All the group size classes were involved but an age discrimination cannot be performed due to the simultaneous presence of calves, juveniles and adults in all the observed groups. They prevalently occurred during socializing activity (Table 2). Furthermore, a chorusing event was recorded during traveling. In this case, the focal group size was 30 individuals. The event was represented by a stereotyped sequence of two overlapped complex calls, both combination of whistle and click train (Figure 3). These two signals were recorded independently before and after the chorus.
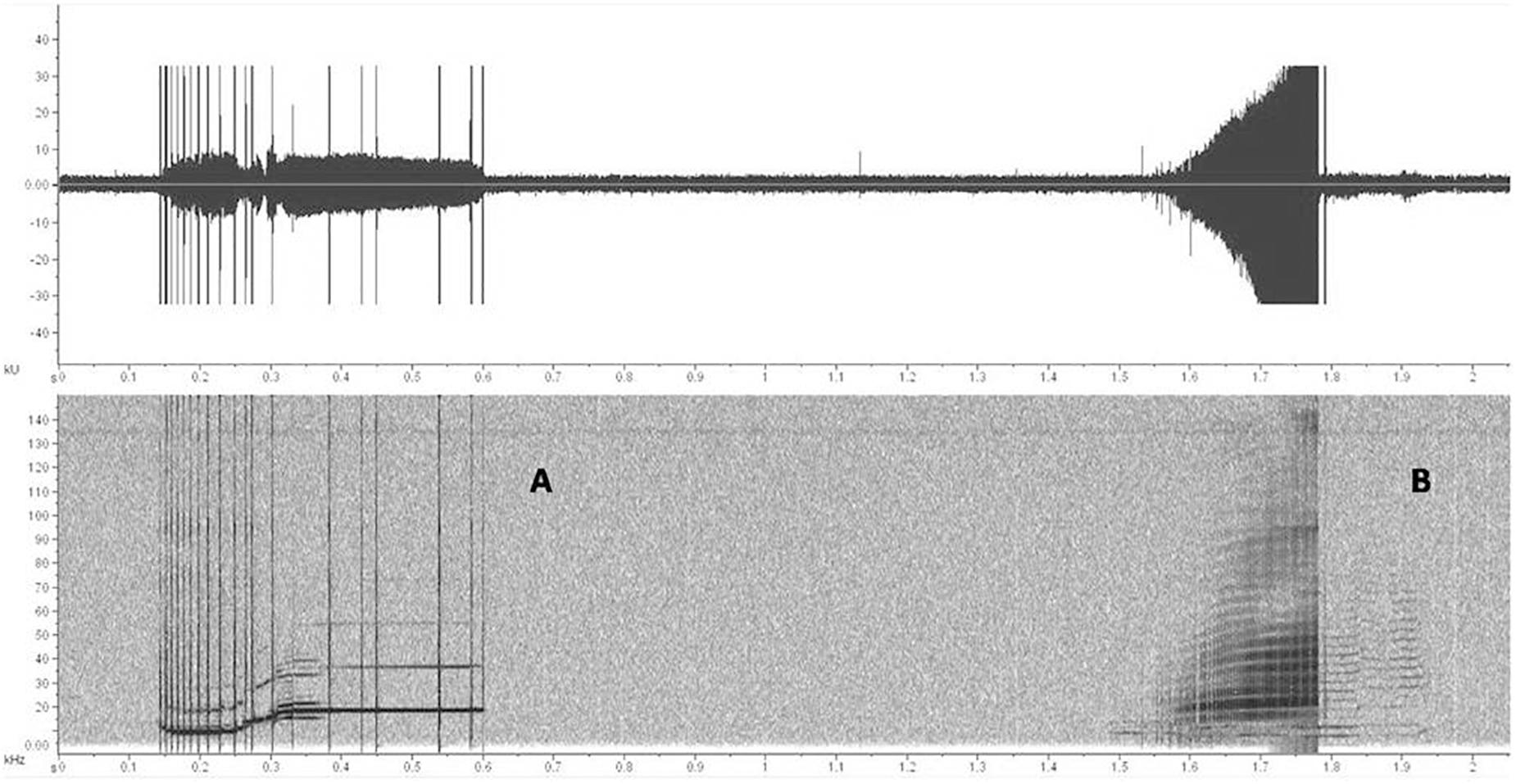
Figure 2. Spectrogram (1024 Fast Fourier Transform size and a Blackmann-Harris window) and waveform (graph energy vs. time) of two complex calls: (A) a combined whistle-click train signal; (B) overlap of a tonal and a burst pulsed component.
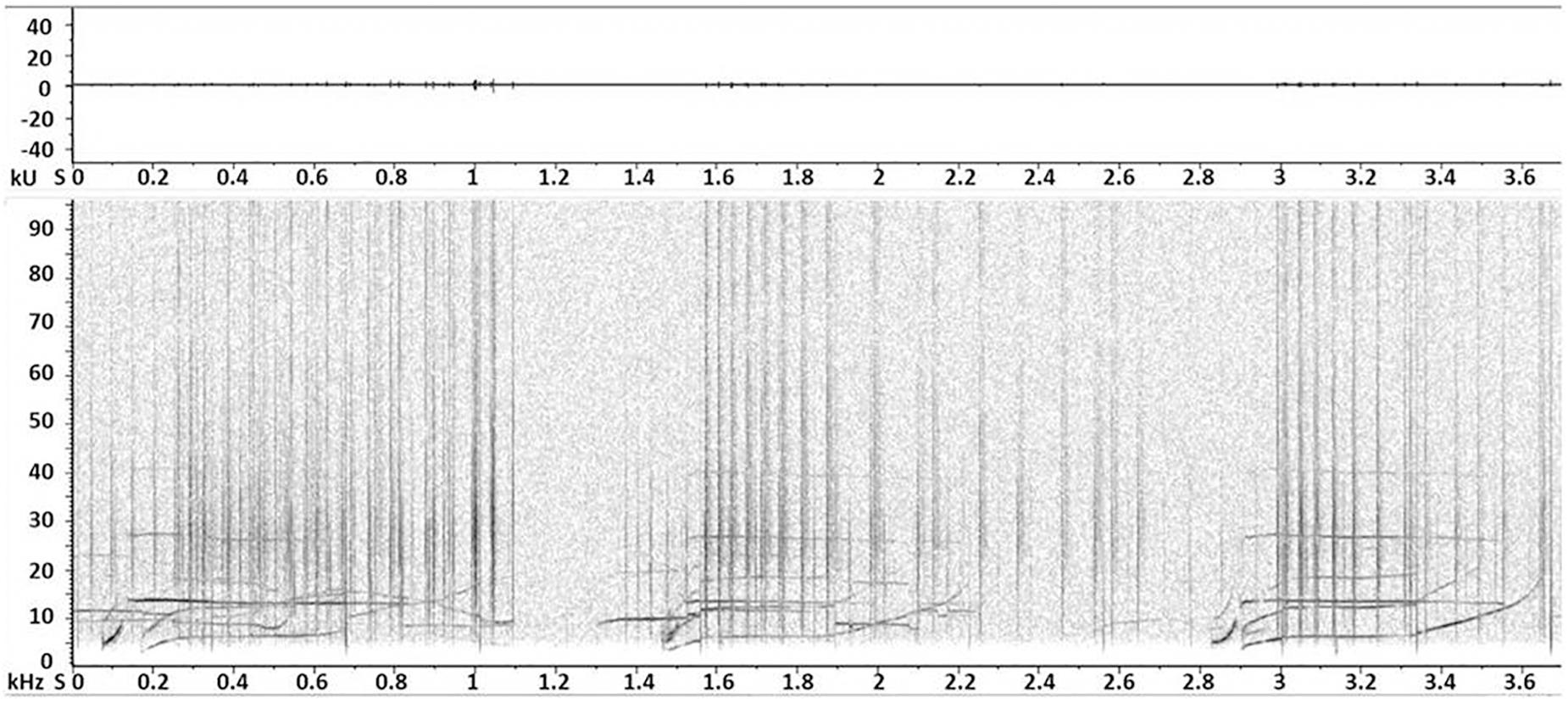
Figure 3. Spectrogram (resolution of 1024 Fast Fourier Transform size and a Blackmann-Harris window) and waveform (graph energy vs. time) of a chorusing event made up of two overlapped complex calls, both combination of whistle and click train. Both signals were also independently detected during the recording.
The calling rates of clicks did not show any variations during the different activity classes (GLMM estimate among −0.37 and 0.31, t-value among −0.62 and 0.58, p > 0.5), while the calling rates of whistles and burst pulses were significantly higher during encounters when socializing was the dominant behavior (Kruskall Wallis test p < 0.001, p = 0.03, respectively, Table 2 and Figure 4). However, the Multiple Comparison test revealed a significant increase in the calling rate of whistles during socializing behavior compared to traveling and resting (Tamhane test: p < 0.05), while in the calling rate of burst pulses during socializing behavior only compared to resting (Tamhane test: p < 0.05). Also complex calls were recorded significantly more during socializing (Kruskall Wallis test p < 0.001) compared to feeding and resting (Tamhane test: p < 0.05).
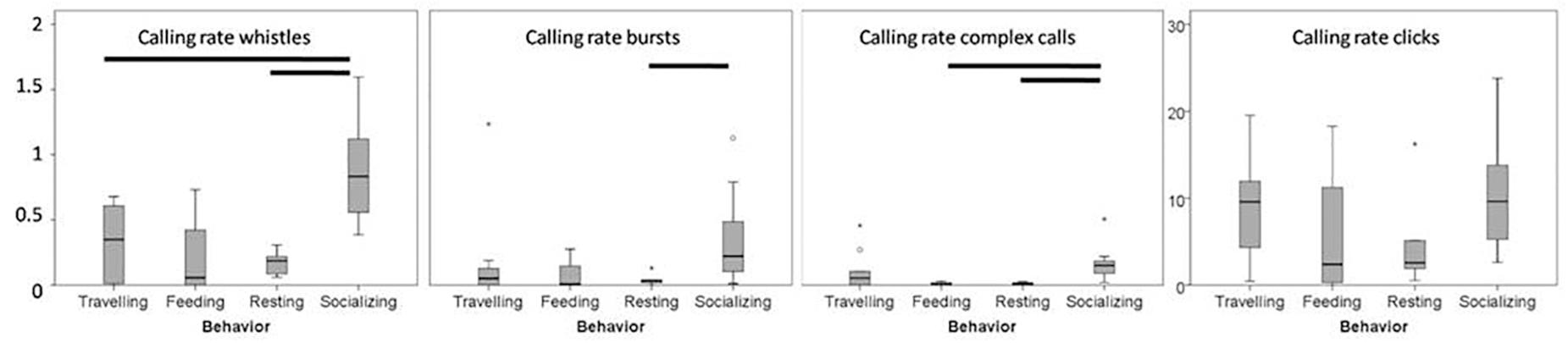
Figure 4. Box plots of the values of the calling rate of the vocalizations in relation to the different activity classes. Black bars represent significant variations between behaviors. The y scale of the whistles calling rate is the same for whistles, bursts and complex calls.
Whistles Parameters and Behavioral Context
Whistle modulation (the number of inflection points, the number of steps and the number of minima and maxima) supported activity class discrimination (total discrimination percentage: 42.0%, Standardized Coefficients: number of inflection points = −0.950; number of steps = –0.712; number of minima = 0.862; number of maxima = 0.911, p < 0.001). Feeding was the best classified activity with a correct percentage of 48.0%, followed by socializing and resting (43.8 and 40.6%, respectively).
Whistles emitted during socializing were characterized by a more complex modulation compared to signals emitted during feeding (Tamhane test: number of minima and of maxima p < 0.001) and during traveling contexts (Tamhane test: number of maxima p = 0.006) (Figure 5).
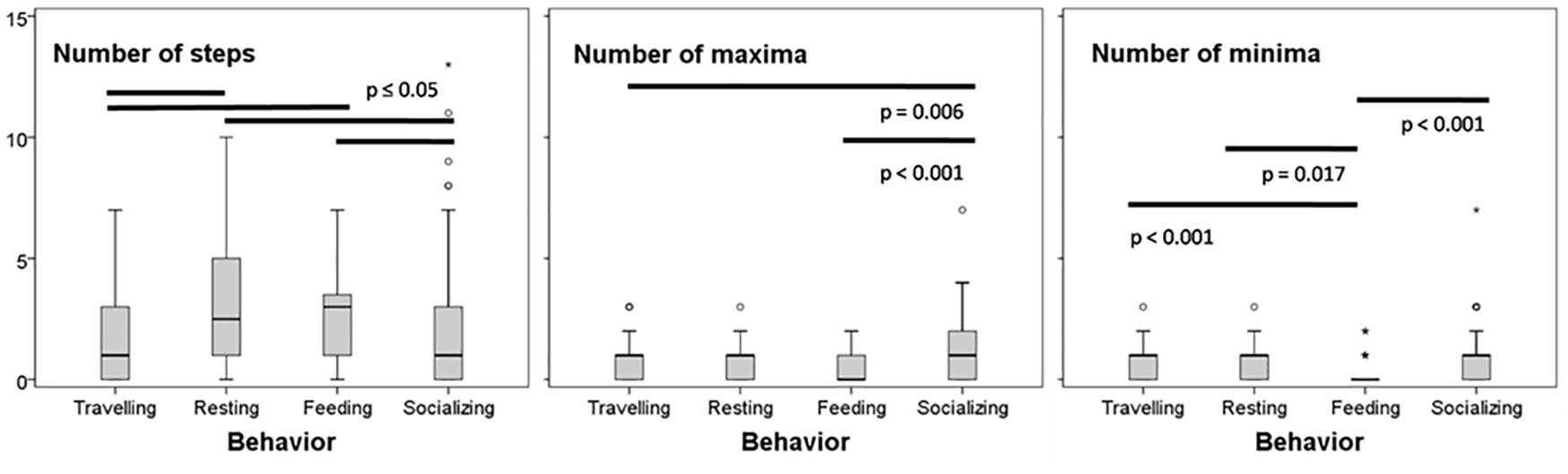
Figure 5. Box plots of the whistle parameters significantly varying among activity class. Black bars represent significant variations between behaviors.
The lower number of minima characterized whistles recorded during feeding also compared to all the other activity classes (Tamhane test: p = 0.017 for resting behavior, p < 0.001 for traveling), while a higher number of steps were measured during both feeding and resting (Tamhane test for feeding: vs. traveling < 0.001, vs. socializing = 0.002; and for resting: vs. traveling p = 0.03, vs. socializing p = 0.05). Mean values of the parameters measured are summarized in Table 3.
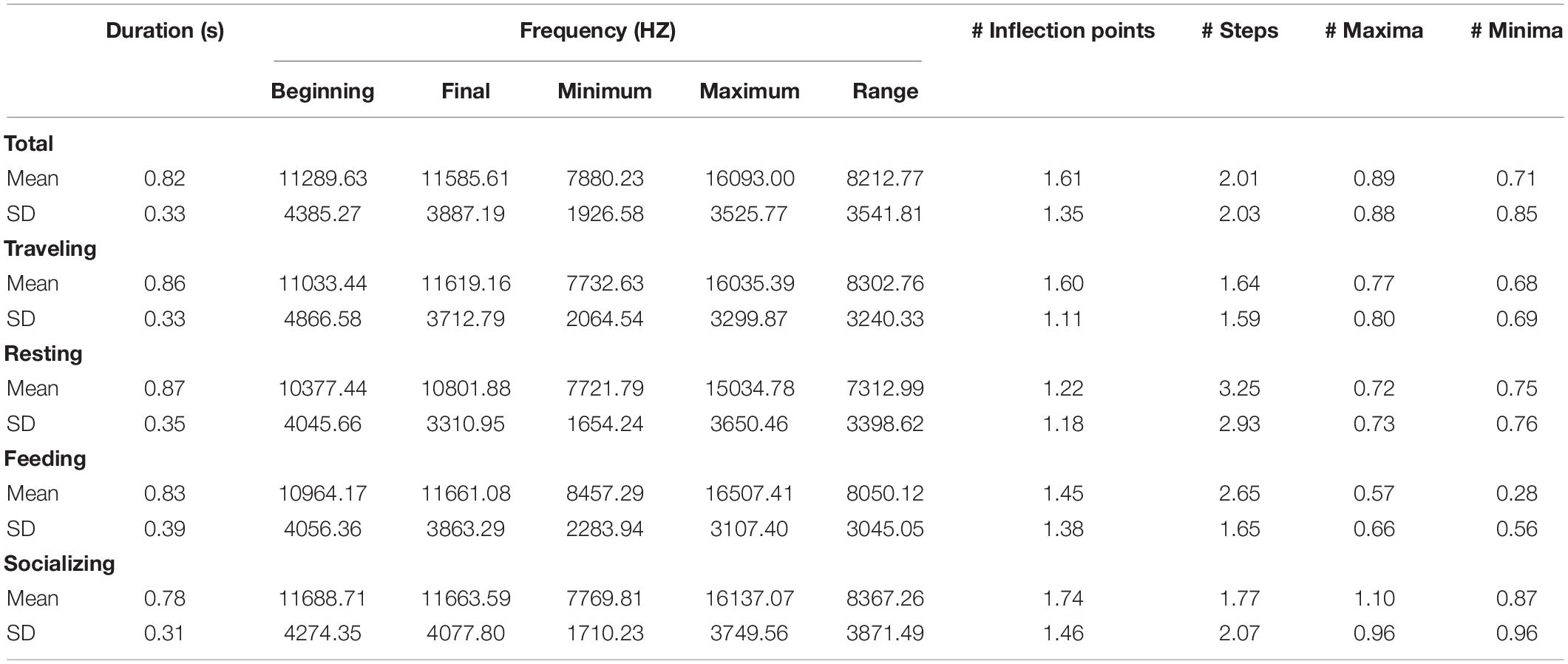
Table 3. Mean values (and Standard deviation) of the whistle parameters as a whole and in relation to behaviors.
Only the number of maxima were significantly affected by the activity classes (LM: Conditional model Estimate = 0.285, p < 0.001), while the duration and the number of minima were influenced by the combined effect of both activity and group size (LM: Conditional model Estimate = –0.153, p = 0.008; Conditional model Estimate = –0.448, p = 0.002) (Supplementary Material).
Discussion
Although S. coeruleoalba is worldwide distributed, its vocal emissions are almost unknown. Except for Anichini (2011) that associated the click and whistle rate to its behavioral states, no other comparable study is available for the species at the Mediterranean and global scale. This study specifically assesses the pivotal role of vocal complexity during social context. In addition, these outcomes support the chance to detect and remotely investigate through passive acoustic monitoring, periods and areas potentially sensitive for social interactions, crucial for the survival of the species.
Results demonstrated that the calling rate of clicks did not show any variations among the investigated activity states. This outcome was partially expected because of the biosonar function of clicks. An increase of clicks calling rate is possible during navigation and searching for prey especially in groups relatively limited (Janik, 2009), but this result did not emerge during this study. Instead, whistle and burst pulses rates resulted higher during socializing. The observed differences in the calling rate were in the range of what reported for the congeneric S. frontalis, as well as T. truncatus and Delphinus delphis, for which especially a higher number of whistles and burst pulses is usually recorded during high-intensity social activity (Jacobs et al., 1993; Jones and Sayigh, 2002; Cook et al., 2004; dos Santos et al., 2005; Gridley et al., 2016). These vocalizations are commonly used as communication signals to provide conspecifics with social information regarding identity, conflicting and sexual interactions (Nakahara, 2002; Janik, 2009). They are also used to keep contact, and coordinate the movements (Janik, 2009).
Furthermore, complex signaling, never reported before for the species, was collected. They seem to be probably more present and flexibly used than previously thought, and therefore worthy of a more detailed description among different populations. The identification of biphonating events is still a challenge, and questions remain regarding the message encoded (Fitch et al., 2002). They were recorded especially when the striped dolphins were engaged in socializing activity, but also other behavioral classes such as during traveling when the requirement of maintaining contact among individuals increased. These signals have been suggested to serve as the way to increase the potential for identity recognition in mammal species (Volodina et al., 2006; Papale et al., 2015; Kaplan et al., 2018). Signal redundancy has been suggested to increase the chance to detect the signaler in a noisy environment (Brumm and Slater, 2006), mostly in the chatty context of a large dolphin social group. As for terrestrial mammals, biphonic calls could compensate for the difficulty of perceiving visual stimuli, providing cues to individuality within large socializing groups. Therefore, an increase in this kind of signals could be predictable when individuals should communicate to keep in contact, cohesion or coordination during social interactions. Also, the chorusing event recorded should require a high level of coordination. As for the choruses of songbird species, it may play a cooperative role allowing groups to coordinate activities (Bradley and Mennill, 2009; Farina and Ceraulo, 2017). This hypothesis agrees with what reported for S. frontalis with similar events recorded during feeding activity (Papale et al., 2013b).
As already observed for S. frontalis and T. truncatus (López and Shirai, 2009; Azevedo et al., 2010; Papale et al., 2017), whistles were more modulated when dolphins were engaged in social activity. Similarly, we observed that striped dolphins emitted a higher number of modulated whistles when the social contexts predominated, and when the number of individuals increased. Results demonstrated that the number of maxima in the contour of the sound was strongly related to the social activity state, while other modulation and duration parameters showed changes because of both activity and group size.
For the bottlenose dolphin, changes in frequency modulation of whistles have been suggested to indicate the transmission of motivational information and individual differentiation within a socio-behavioral context (Janik et al., 1994 or in Esch et al., 2009; López, 2011; Heiler et al., 2016). This is especially linked to signature whistle use (Cook et al., 2004). However, even if stereotyped whistles have been recorded for striped dolphins during this study, the certain use of signatures could not be assessed because the methods used did not allow to assign calls to individuals. The increasing in whistle modulation during social events, as also the presence of stereotypically repeated biphonic signals suggest the possibility that animals use more complex calls to communicate signaler’s position, identity or group belonging.
During this study, the striped dolphins showed different group sizes in feeding and resting compared to what occurred when in traveling and socializing. As reported by Carlucci et al. (2018c), they aggregate in the area in larger groups when socializing. Even if a relation among the group size and the activity is not clear, the number of individuals influenced the signal modulation. Large groups engaged in other activity classes than socializing, imply anyway a level of interactions among the individuals. Therefore, it is likely that social interaction functional to different behaviors, could be the driving force of the acoustic complexity more than the pure socializing activity.
Therefore, results suggest that complex vocal patterns, in the form of high calling rate, signal modulation and biphonic signaling, can mediate social interactions in S. coeruleoalba. These outcomes match studies on other dolphins species, such as the spotted and bottlenose dolphins. Dolphin species can represent a useful model for studying social communication, since they exhibit high variation in their social structure: group composition and cohesion change frequently within populations (Connor et al., 2000), and they can show among populations differences in sex and age segregation (with strong associations between or within sexes, aggregations of nursery females, or small groups of juveniles) (Wells et al., 1980; Wells et al., 1987; Lusseau et al., 2003). In addition, the mixing of individuals, genetically related or not, can also result in structured dolphin sub-communities (Lusseau and Newman, 2004). Hence, the evolution of the social communication system should be further investigated looking to the small odontocetes from local to wider spatial scale.
Data Availability Statement
The raw data supporting the conclusions of this article will be made available by the authors, without undue reservation.
Ethics Statement
Ethical review and approval was not required for this study because only Passive Acoustic Monitoring was carried out and no animal manipulation or invasive technique were performed.
Author Contributions
EP, CF, GB, RM, and RCa conceived the study and coordinated the data collection, provided ideas, materials and analysis tools. EP, MC, and MG performed the data analyses. GC and RCr contributed to data collection and analyses. RG, VR, PR, and FS contributed to data collection. All authors contributed to writing the manuscript.
Funding
Funding for data collection was provided by the Jonian Dolphin Conservation.
Conflict of Interest
The authors declare that the research was conducted in the absence of any commercial or financial relationships that could be construed as a potential conflict of interest.
Acknowledgments
We are grateful to Dr. Daniela Silvia Pace for the invaluable support and the fine revision of the paper and to Valeria Sini and Cristina Turco for their contribution in data selection.
Supplementary Material
The Supplementary Material for this article can be found online at: https://www.frontiersin.org/articles/10.3389/fmars.2020.584301/full#supplementary-material
References
Altmann, J. (1974). Observational study of behavior: sampling methods. Behaviour 49, 227–266. doi: 10.1163/156853974x00534
Anichini, M. (2011). Relationship Between Vocal and Behaviour Activities in Striped dolphin 366 (Stenella coeruleoalba). Master degree thesis, University of Turin, Italy.
Azevedo, A. F., Flach, L., Bisi, T. L., Andrade, L. G., Dorneles, P. R., and Lailson-Brito, J. (2010). Whistles emitted by Atlantic spotted dolphins (Stenella frontalis) in southeastern Brazil. J. Acoust. Soc. Am. 127, 2646–2651. doi: 10.1121/1.3308469
Azzolin, M., Arcangeli, A., Cipriano, G., Crosti, R., Maglietta, R., Pietroluongo, G., et al. (2020). Spatial distribution modelling of striped dolphin (Stenellacoeruleoalba) at different geographical scales within the EU Adriatic and Ionian Sea Region, central-eastern Mediterranean Sea. Aquat. Conserv. Mar. Freshw. Ecosyst. 30, 1194–1207. doi: 10.1002/aqc.3314
Azzolin, M., Gannier, A., Lammers, M. O., Oswald, J. N., Papale, E., Buscaino, G., et al. (2014). Combining whistle acoustic parameters to discriminate Mediterranean odontocetes during passive acoustic monitoring. J. Acoust. Soc. Am. 135, 502–512. doi: 10.1121/1.4845275
Blumstein, D. T., and Armitage, K. B. (1997). Does Sociality Drive the Evolution of Communicative Complexity? A Comparative Test with Ground-Dwelling Sciurid Alarm Calls. Am. Nat. 150, 179–200. doi: 10.1086/286062
Blumstein, D. T., Steinmetz, J., Armitage, K. B., and Daniel, J. C. (1997). Alarm calling in yellow-bellied marmots: II. The importance of direct fitness. Anim. Behav. 53, 173–184. doi: 10.1006/anbe.1996.0286
Bojanowski, E., Veit, F., and Todt, D. (2000). The development of a bivocal signature whistle in a bottlenose dolphin calf. Eur. Res. Cetaceans 14, 70–74.
Bonato, M., Papale, E., Pingitore, G., Ricca, S., Attoumane, A., Ouledi, A., et al. (2015). Whistle characteristics of the spinner dolphin population in the Comoros Archipelago. J. Acoust. Soc. Am. 138, 3262–3271. doi: 10.1121/1.4935518
Bouchet, H., Blois-Heulin, C., and Lemasson, A. (2013). Social complexity parallels vocal complexity: a comparison of three non-human primate species. Front. Psychol. 4:390. doi: 10.3389/fpsyg.2013.00390
Bradley, D. W., and Mennill, D. J. (2009). Solos, duets and choruses: vocal behaviour of the Rufous-naped Wren (Campylorhynchusrufinucha), a cooperatively breeding neotropical songbird. J. Ornithol. 150, 743–753. doi: 10.1007/s10336-009-0393-3
Brudzynski, S. M. (2014). “Social origin of vocal communication in rodents,” in Biocommunication of Animals, Ed. G. Witzany (Dordrecht: Springer), 63–79. doi: 10.1007/978-94-007-7414-8_5
Brumm, H., and Slater, P. J. B. (2006). Ambient noise, motor fatigue, and serial redundancy in chaffinch song. Behav. Ecol. Sociobiol. 60, 475–481. doi: 10.1007/s00265-006-0188-y
Buckland, S. T., Anderson, D. R., Burnham, K. P., Laake, J. L., Borchers, D. L., and Thomas, L. (2004). Advanced Distance Sampling. Oxford: Oxford University Press.
Caldwell, M. C., and Caldwell, D. K. (1965). Individualized whistle contours in bottle-nosed dolphins (Tursiops truncatus). Nature 207, 434–435. doi: 10.1038/207434a0
Capezzuto, F., Carlucci, R., Maiorano, P., Sion, L., Battista, D., Giove, A., et al. (2010). The bathyal benthopelagic fauna in the north-western Ionian Sea: structure, patterns and interactions. Chem. Ecol. 26, 199–217. doi: 10.1080/02757541003639188
Carlucci, R., Bandelj, V., Ricci, P., Capezzuto, F., Sion, L., Maiorano, P., et al. (2018a). Exploring spatio-temporal changes in the demersal and benthopelagic assemblages of the north-western Ionian Sea (central Mediterranean Sea). Mar. Ecol. Prog. Ser. 598, 1–19. doi: 10.3354/meps12613
Carlucci, R., Cipriano, G., Paoli, C., Ricci, P., Fanizza, C., Capezzuto, F., et al. (2018b). Random Forest population modelling of striped and common-bottlenose dolphins in the Gulf of Taranto (Northern Ionian Sea, Central-eastern Mediterranean Sea). Estuar. Coast. Shelf Sci. 204, 177–192. doi: 10.1016/j.ecss.2018.02.034
Carlucci, R., Ricci, P., Cipriano, G., and Fanizza, C. (2018c). Abundance, activity and critical habitat of the striped dolphin Stenellacoeruleoalba in the Gulf of Taranto (northern Ionian Sea, central Mediterranean Sea). Aquat. Conserv. Mar. Freshw. Ecosyst. 28, 324–336. doi: 10.1002/aqc.2867
Cook, M. L., Sayigh, L. S., Blum, J. E., and Wells, R. S. (2004). Signature–whistle production in undisturbed free–ranging bottlenose dolphins (Tursiops truncatus). Proc. R. Soc. Lond. B Biol. Sci. 271, 1043–1049. doi: 10.1098/rspb.2003.2610
Corkeron, P. J., and Van Parijs, S. M. (2001). Vocalizations of eastern Australian Risso’s dolphins. Grampus griseus. Can. J. Zool. 79, 160–164. doi: 10.1139/z00-180
Connor, R. C., Wells, R. S., Mann, J., and Read, A. J. (2000). “The bottlenose dolphin: social relationships in a fission-fusion society,” in Cetacean Societies: Field Studies of Dolphins and Whales, eds J. Mann, R. Connor, P. Tyack, and H. Whitehead (Chicago: The University of Chicago Press), 91–126.
Dawson, S. M. (1991). Clicks and communication: the behavioural and social contexts of Hector’s dolphin vocalizations. Ethology 88, 265–276. doi: 10.1111/j.1439-0310.1991.tb00281.x
Deecke, V. B., Ford, J. K., and Spong, P. (2000). Dialect change in resident killer whales: implications for vocal learning and cultural transmission. Anim. Behav. 60, 629–638. doi: 10.1006/anbe.2000.1454
dos Santos, M. E., Ferreira, A. J., and Harzen, S. (1995). “Rhythmic sound sequences emitted by aroused 246 bottlenose dolphins in the Sado estuary, Portugal,” in Sensory Systems of Aquatic Mammals, eds R. A. Kastelein, J. A. Thomas, and P. E. Nachtigall (Woerden: De Spil Publishers), 325–334.
dos Santos, M. E., Louro, S., Couchinho, M. N., and Brito, C. M. (2005). Whistles of bottlenose dolphins (Tursiops truncatus) in the Sado Estuary, Portugal: characteristics, production rates, and long-term contour stability. Aquat. Mamm. 31, 453–462. doi: 10.1578/am.31.4.2005.453
Dudzinski, K. M. (1998). Contact behavior and signal exchange in Atlantic spotted dolphins (Stenella frontalis). Aquat. Mamm. 24, 129–142.
Esch, H. C., Sayigh, L. S., and Wells, R. S. (2009). Quantifying parameters of bottlenose dolphin signature whistles. Mar. Mammal Sci. 25, 976–986. doi: 10.1111/j.1748-7692.2009.00289.x
Eskelinen, H. C., Winship, K. A., Jones, B. L., Ames, A. E. M., and Kuczaj, S. A. (2016). Acoustic behavior associated with cooperative task success in bottlenose dolphins (Tursiops truncatus). Anim. Cogn. 19, 789–797. doi: 10.1007/s10071-016-0978-971
Evans-Wilent, J., and Dudzinski, K. M. (2013). Vocalizations associated with pectoral fin contact in bottlenose dolphins (Tursiops truncatus). Behav. Process. 100, 74–81. doi: 10.1016/j.beproc.2013.07.025
Farina, A., and Ceraulo, M. (2017). “The acoustic chorus and its ecological significance,” in Ecoacoustics: The Ecological Role of Sounds, eds A. Farina and S. H. Gage (Hoboken, NJ: John Wiley & Sons).
Fichtel, C., and Manser, M. (2010). “Vocal communication in social groups,” in Animal Behaviour: Evolution and Mechanisms, (Berlin: Springer), 29–54. doi: 10.1007/978-3-642-02624-9_2
Filatova, O. A., Burdin, A. M., and Hoyt, E. (2010). Horizontal transmission of vocal traditions in killer whale (Orcinus orca) dialects. Biol. Bull. 37, 965–971. doi: 10.1134/S1062359010090104
Filatova, O. A., Fedutin, I. D., Nagaylik, M. M., Burdin, A. M., and Hoyt, E. (2009). Usage of monophonic and biphonic calls by free-ranging resident killer whales (Orcinus orca) in Kamchatka, Russian Far East. Acta Ethol. 12, 37–44. doi: 10.1007/s10211-009-0056-57
Filatova, O. A., Guzeev, M. A., Fedutin, I. D., Burdin, A. M., and Hoyt, E. (2013). Dependence of killer whale (Orcinus orca) acoustic signals on the type of activity and social context. Biol. Bull. 40, 790–796. doi: 10.1134/S1062359013090045
Fitch, W. T., Neubauer, J., and Herzel, H. (2002). Calls out of chaos: the adaptive significance of nonlinear phenomena in mammalian vocal production. Anim. Behav. 63, 407–418. doi: 10.1006/anbe.2001.1912
Freeberg, T. M., Dunbar, R. I., and Ord, T. J. (2012a). Social Complexity as a Proximate and Ultimate Factor in Communicative Complexity. London: The Royal Society.
Freeberg, T. M., Ord, T. J., and Dunbar, R. I. M. (2012b). The social network and communicative complexity: preface to theme issue. Philos. Trans. R. Soc. B Biol. Sci. 367, 1782–1784. doi: 10.1098/rstb.2011.0294
Gannier, A., Fuchs, S., Quèbre, P., and Oswald, J. N. (2010). Performance of a contour-based classification method for whistles of Mediterranean delphinids. Appl. Acoust. 71, 1063–1069. doi: 10.1016/j.apacoust.2010.05.019
Gaspari, S., Azzellino, A., Airoldi, S., and Hoelzel, A. R. (2007). Social kin associations and genetic structuring of striped dolphin populations (Stenellacoeruleoalba) in the Mediterranean Sea. Mol. Ecol. 16, 2922–2933. doi: 10.1111/j.1365-294x.2007.03295.x
Gazda, S., Iyer, S., Killingback, T., Connor, R., and Brault, S. (2015). The importance of delineating networks by activity type in bottlenose dolphins (Tursiops truncatus) in Cedar Key. Florida. R Soc Open Sci. 2:140263. doi: 10.1098/rsos.140263
Gridley, T., Elwen, S. H., Rashley, G., Badenas Krakauer, A., and Heiler, J. (2016). Bottlenose dolphins change their whistling characteristics in relation to vessel presence, surface behavior and group composition. Proc. Meet. Acoust. 27:010030. doi: 10.1121/2.0000312
Grueber, C. E., Nakagawa, S., Laws, R. J., and And Jamieson, I. G. (2011). Multimodel inference in ecology and evolution: challenges and solutions. J. Evol. Biol. 24, 699–711. doi: 10.1111/j.1420-9101.2010.02210.x
Gustison, M. L., Johnson, E. T., Beehner, J. C., and Bergman, T. J. (2019). The social functions of complex vocal sequences in wild geladas. Behav. Ecol. Sociobiol. 73, 1–12. doi: 10.1007/s00265-018-2612-2615
Hawkins, E. R. (2010). Geographic variations in the whistles of bottlenose dolphins (Tursiops aduncus) along the east and west coasts of Australia. J. Acoust. Soc. Am. 128, 924–935. doi: 10.1121/1.3459837
Hebets, E. A., and Papaj, D. R. (2005). Complex signal function: developing a framework of testable hypotheses. Behav. Ecol. Sociobiol. 57, 197–214. doi: 10.1007/s00265-004-0865-867
Heiler, J., Elwen, S. H., Kriesell, H. J., and Gridley, T. (2016). Changes in bottlenose dolphin whistle parameters related to vessel presence, surface behaviour and group composition. Anim. Behav. 117, 167–177. doi: 10.1016/j.anbehav.2016.04.014
Herzing, D. L. (1996). Vocalizations and associated underwater behavior of free-ranging Atlantic spotted dolphins, Stenella frontalis and bottlenose dolphins, Tursiops truncatus. Aquat. Mamm. 22, 61–79.
Irvine, A. B., Scott, M. D., Wells, R. S., and Kaufmann, J. H. (1981). Movements and activities of the Atlantic bottlenose dolphin, Tursiops truncatus, near Sarasota, Florida. Fish. Bull. 79, 671–688.
Jacobs, M., Nowacek, D. P., Gerhart, D. J., Cannon, G., Nowicki, S., and Forward, R. B. (1993). Seasonal changes in vocalization during behavior of the Atlantic bottlenose dolphin. Estuaries 16, 241–246. doi: 10.2307/1352496
Janik, V. M. (2009). Acoustic communication in delphinids. Adv. Study Behav. 40, 123–157. doi: 10.1016/s0065-3454(09)40004-4
Janik, V. M., King, S. L., Sayigh, L. S., and Wells, R. S. (2013). Identifying signature whistles from recordings of groups of unrestrained bottlenose dolphins (Tursiops truncatus). Mar. Mammal Sci. 29, 109–122. doi: 10.1111/j.1748-7692.2011.00549.x
Janik, V. M., and Slater, P. J. (1998). Context-specific use suggests that bottlenose dolphin signature whistles are cohesion calls. Anim. Behav. 56, 829–838. doi: 10.1006/anbe.1998.0881
Janik, V. M., Todt, D., and Dehnhardt, G. (1994). Signature whistle variations in a bottlenosed dolphin. Tursiops truncatus. Behav. Ecol. Sociobiol. 35, 243–248. doi: 10.1007/bf00170704
Jones, B., Zapetis, M., Samuelson, M. M., and Ridgway, S. (2020). Sounds produced by bottlenose dolphins (Tursiops): a review of the defining characteristics and acoustic criteria of the dolphin vocal repertoire. Bioacoustics 29, 399–440.
Jones, G. J., and Sayigh, L. S. (2002). Geographic variation in rates of vocal production of free-ranging bottlenose dolphins. Mar. Mammal Sci. 18, 374–393. doi: 10.1111/j.1748-7692.2002.tb01044.x
Kaplan, J. D., Melillo-Sweeting, K., and Reiss, D. (2018). Biphonal calls in Atlantic spotted dolphins (Stenella frontalis): bitonal and burst-pulse whistles. Bioacoustics 27, 145–164. doi: 10.1080/09524622.2017.1300105
Kappeler, P. M. (2019). A framework for studying social complexity. Behav. Ecol. Sociobiol. 73:13. doi: 10.1007/s00265-018-2601-2608
Kastelein, R. A., Hagedoorn, M., Au, W. W., and de Haan, D. (2003). Audiogram of a striped dolphin (Stenellacoeruleoalba). J. Acoust. Soc. Am. 113, 1130–1137. doi: 10.1121/1.1532310
King, S. L., and Janik, V. M. (2015). Come dine with me: food-associated social signalling in wild bottlenose dolphins (Tursiops truncatus). Anim. Cogn. 18, 969–974. doi: 10.1007/s10071-015-0851-857
Kriesell, H. J., Elwen, S. H., Nastasi, A., and Gridley, T. (2014). Identification and characteristics of signature whistles in wild bottlenose dolphins (Tursiops truncatus) from Namibia. PLoS One 9:e106317. doi: 10.1371/journal.pone.0106317
La Manna, G., Manghi, M., Pavan, G., Lo Mascolo, F., and Sarà, G. (2013). Behavioural strategy of common bottlenose dolphins (Tursiops truncatus) in response to different kinds of boats in the waters of Lampedusa Island (Italy). Aquat. Conserv. Mar. Freshw. Ecosyst. 23, 745–757.
Lachenbruch, P. A., and Mickey, M. R. (1968). Estimation of Error Rates in Discriminant Analysis. Technometrics 10, 1–11. doi: 10.2307/1266219
Lammers, M. O., Au, W. W., and Herzing, D. L. (2003). The broadband social acoustic signaling behavior of spinner and spotted dolphins. J. Acoust. Soc. Am. 114, 1629–1639. doi: 10.1121/1.1596173
López, B. D. (2011). Whistle characteristics in free-ranging bottlenose dolphins (Tursiops truncatus) in the Mediterranean Sea: Influence of behaviour. Mamm. Biol. 76, 180–189. doi: 10.1016/j.mambio.2010.06.006
López, B. D., and Shirai, J. A. B. (2009). “Mediterranean common bottlenose dolphin’s repertoire and communication use,” in Dolphins: Anatomy, Behavior and Threats, eds L. M. Correa, and A. G. Pearce 129–148.
Luís, A. R., Alves, I. S., Sobreira, F. V., Couchinho, M. N., and Dos Santos, M. E. (2019). Brays and bits: information theory applied to acoustic communication sequences of bottlenose dolphins. Bioacoustics 28, 286–296. doi: 10.1080/09524622.2018.1443285
Lusseau, D., and Newman, M. E. (2004). Identifying the role that animals play in their social networks. Proc. R. Soc. Lond. B Biol. Sci. 271, S477–S481.
Lusseau, D., Schneider, K., Boisseau, O. J., Haase, P., Slooten, E., and Dawson, S. M. (2003). The bottlenose dolphin community of Doubtful Sound features a large proportion of long-lasting associations. Behav. Ecol. Sociobiol. 54, 396–405. doi: 10.1007/s00265-003-0651-y
Manser, M. B., Jansen, D. A., Graw, B., Hollén, L. I., Bousquet, C. A., Furrer, R. D., et al. (2014). “Vocal complexity in meerkats and other mongoose species,” in Advances in the Study of Behavior, eds J. S. Rosenblatt, R. A. Hinde, C. Beer, and M.-C. Busnel (Amsterdam: Elsevier), 281–310. doi: 10.1016/b978-0-12-800286-5.00006-7
May-Collado, L. J. (2013). Guyana dolphins (Sotaliaguianensis) from Costa Rica emit whistles that vary with surface behaviors. J. Acoust. Soc. Am. 134, EL359–EL365.
May-Collado, L. J., Agnarsson, I., and Wartzok, D. (2007). Phylogenetic review of tonal sound production in whales in relation to sociality. BMC Evol. Biol. 7:136. doi: 10.1186/1471-2148-7-136
McComb, K., and Semple, S. (2005). Coevolution of vocal communication and sociality in primates. Biol. Lett. 1, 381–385. doi: 10.1098/rsbl.2005.0366
Melo-Santos, G., Rodrigues, A. L. F., Tardin, R. H., Maciel, I. D. S., Marmontel, M., Silva, M. L. D., et al. (2019). The newly described Araguaian river dolphins, Inia araguaiaensis (Cetartiodactyla, Iniidae), produce a diverse repertoire of acoustic signals. PeerJ 7:e6670. doi: 10.7717/peerj.6670
Murray, S. O., Mercado, E., and Roitblat, H. L. (1998). Characterizing the graded structure of false killer whale (Pseudorca crassidens) vocalizations. J. Acoust. Soc. Am. 104, 1679–1688. doi: 10.1121/1.424380
Musser, W. B., Bowles, A. E., Grebner, D. M., and Crance, J. L. (2014). Differences in acoustic features of vocalizations produced by killer whales cross-socialized with bottlenose dolphins. J. Acoust. Soc. Am. 136, 1990–2002. doi: 10.1121/1.4893906
Nakahara, F. (2002). Social functions of cetacean acoustic communication. Fish. Sci. 68, 298–301. doi: 10.2331/fishsci.68.sup1_298
Nemiroff, L. (2009). Structural Variation and Communicative Functions of Long-finned Pilot Whale (Globicephala Melas) Pulsed Calls and Comple Whistles. Available online at: http://whitelab.biology.dal.ca/ln/PDFs/Nemiroff_MSc_thesis_2009.pdf (accessed September 16, 2018).
Neumann, D. R. (2001). Activity budget of free-ranging common dolphins (Delphinus delphis) in the northwestern Bay of Plenty. New Zealand. Aquat. Mamm. 27, 121–136.
Norris, K. S., Wursig, B., Wells, R. S., and Wursig, M. (1994). The Hawaiian Spinner Dolphin. Berkeley, CA: University of California Press.
Ord, T. J., and Garcia-Porta, J. (2012). Is sociality required for the evolution of communicative complexity? Evidence weighed against alternative hypotheses in diverse taxonomic groups. Philos. Trans. R. Soc. B Biol. Sci. 367, 1811–1828. doi: 10.1098/rstb.2011.0215
Oswald, J. N., Barlow, J., and Norris, T. F. (2003). Acoustic identification of nine delphinid species in the eastern tropical Pacific Ocean. Mar. Mammal Sci. 19, 20–037. doi: 10.1111/j.1748-7692.2003.tb01090.x
Oswald, J. N., Rankin, S., Barlow, J., and Lammers, M. O. (2007). A tool for real-time acoustic species identification of delphinid whistles. J. Acoust. Soc. Am. 122, 587–595. doi: 10.1121/1.2743157
Panova, E. M., Belikov, R. A., Agafonov, A. V., and Bel’Kovich, V. M. (2012). The relationship between the behavioral activity and the underwater vocalization of the beluga whale (Delphinapterus leucas). Oceanology 52, 79–87. doi: 10.1134/s000143701201016x
Papale, E., Alonge, G., Caruso, F., Grammauta, R., Mazzola, S., Mussi, B., et al. (2019). The higher, the closer, the better? Influence of sampling frequency and distance on the acoustic properties of short-beaked common dolphins burst pulses in the Mediterranean Sea. Aquat. Conserv. Mar. Freshw. Ecosyst. 2019, 1–10. doi: 10.1002/aqc.3158
Papale, E., Azzolin, M., Cascao, I., Gannier, A., Lammers, M. O., Martin, V. M., et al. (2013a). Geographic variability in the acoustic parameters of striped dolphin’s (Stenellacoeruleoalba) whistles. J. Acoust. Soc. Am. 133, 1126–1134. doi: 10.1121/1.4774274
Papale, E., Perez Gil, M., Castrillon, J. P., Perez Gil, E., Ruiz, L., Tejedor, M., et al. (2013b). “Chorusing underwater: Atlantic spotted dolphin’s acoustic activity during feeding,” in Proceedings of the 27th CONFERENCE OF THE (Escola de Mar), Spain, 210–210.
Papale, E., Gamba, M., Perez-Gil, M., Martin, V. M., and Giacoma, C. (2015). Dolphins adjust species-specific frequency parameters to compensate for increasing background noise. PLoS One 10:e0121711. doi: 10.1371/journal.pone.0121711
Papale, E., Perez-Gil, M., Castrillon, J., Perez-Gil, E., Ruiz, L., Servidio, A., et al. (2017). Context specificity of Atlantic spotted dolphin acoustic signals in the Canary Islands. Ethol. Ecol. Evol. 29, 311–329. doi: 10.1080/03949370.2016.1171256
Peckre, L., Kappeler, P. M., and Fichtel, C. (2019). Clarifying and expanding the social complexity hypothesis for communicative complexity. Behav. Ecol. Sociobiol. 73:11. doi: 10.1007/s00265-018-2605-2604
Ramsier, M. A., Cunningham, A. J., Finneran, J. J., and Dominy, N. J. (2012). Social drive and the evolution of primate hearing. Philos. Trans. R. Soc. B 367, 1860–1868. doi: 10.1098/rstb.2011.0219
Reiss, D. (1988). “Observations on the development of echolocation in young bottlenose dolphins,” in Animal Sonar, eds R.-G. Busnel, and J. F. Fish (Berlin: Springer), 121–127. doi: 10.1007/978-1-4684-7493-0_14
Russo, T., Bitetto, I., Carbonara, P., Carlucci, R., D’Andrea, L., Facchini, M. T., et al. (2017). A holistic approach to fishery management: evidence and insights from a central mediterranean case study (Western Ionian Sea). Front. Mar. Sci. 4:193.
Sayigh, L., Quick, N., Hastie, G., and Tyack, P. (2013). Repeated call types in short-finned pilot whales, Globicephala macrorhynchus. Mar. Mammal Sci. 29, 312–324. doi: 10.1111/j.1748-7692.2012.00577.x
Shane, S. H. (1990). Behavior and ecology of the bottlenose dolphin at Sanibel Island, Florida. Bottlenose Dolphin 1990, 245–265. doi: 10.1016/b978-0-12-440280-5.50016-0
Thomas, R. E., Fristrup, K. M., and Tyack, P. L. (2002). Linking the sounds of dolphins to their locations and behavior using video and multichannel acoustic recordings. J. Acoust. Soc. Am. 112, 1692–1701. doi: 10.1121/1.1494805
Van Cise, A. M., Mahaffy, S. D., Baird, R. W., Mooney, T. A., and Barlow, J. (2018). Song of my people: dialect differences among sympatric social groups of short-finned pilot whales in Hawai’i. Behav. Ecol. Sociobiol. 72:193.
van Parijs, S. M., and Corkeron, P. J. (2001). Evidence for signature whistle production by a Pacific humpback dolphin. Sousa chinensis. Mar. Mammal Sci. 17, 944–949. doi: 10.1111/j.1748-7692.2001.tb01308.x
Vanden Hole, C., Van Daele, P. A., Desmet, N., Devos, P., and Adriaens, D. (2014). Does sociality imply a complex vocal communication system? A case study for Fukomysmicklemi (Bathyergidae, Rodentia). Bioacoustics 23, 143–160. doi: 10.1080/09524622.2013.841085
Volodina, E. V., Volodin, I. A., Isaeva, I. V., and Unck, C. (2006). Biphonation may function to enhance individual recognition in the dhole, Cuonalpinus. Ethology 112, 815–825. doi: 10.1111/j.1439-0310.2006.01231.x
Wellard, R., Erbe, C., Fouda, L., and Blewitt, M. (2015). Vocalisations of killer whales (Orcinus orca) in the Bremer Canyon, Western Australia. PLoS One 10:e0136535. doi: 10.1371/journal.pone.0136535
Wellard, R., Pitman, R. L., Durban, J., and Erbe, C. (2020). Cold call: the acoustic repertoire of Ross Sea killer whales (Orcinus orca, Type C) in McMurdo Sound, Antarctica. R. Soc. Open Sci. 7:191228. doi: 10.1098/rsos.191228
Wells, R. S., Irvine, A. B., and Scott, M. D. (1980). “The social ecology of inshore odontocetes,” in Cetacean Behavior: Mechanisms and Functions, ed. L. M. Herman (New York, NY: J.Wiley & Sons), 263–317.
Wells, R. S., Scott, M. D., and Irvine, A. B. (1987). “The social structure of free-ranging bottlenose dolphins,” in Current Mammalogy, Vol. 1, ed. H. Genoways (New York, NY: Plenum Press), 247–305. doi: 10.1007/978-1-4757-9909-5_7
Wilkins, M. R., Shizuka, D., Joseph, M. B., Hubbard, J. K., and Safran, R. J. (2015). Multimodal signalling in the North American barn swallow: a phenotype network approach. Proc. R. Soc. B 282, 325–334. doi: 10.1098/rspb.2015.1574
Keywords: acoustic behavior, dolphin, Mediterranean Sea, complex calls, social interactions, Ionian Sea
Citation: Papale E, Fanizza C, Buscaino G, Ceraulo M, Cipriano G, Crugliano R, Grammauta R, Gregorietti M, Renò V, Ricci P, Santacesaria FC, Maglietta R and Carlucci R (2020) The Social Role of Vocal Complexity in Striped Dolphins. Front. Mar. Sci. 7:584301. doi: 10.3389/fmars.2020.584301
Received: 16 July 2020; Accepted: 12 October 2020;
Published: 09 November 2020.
Edited by:
Rebecca Dunlop, The University of Queensland, AustraliaReviewed by:
Stephanie Laura King, University of Bristol, United KingdomSimon Elwen, Stellenbosch University, South Africa
Copyright © 2020 Papale, Fanizza, Buscaino, Ceraulo, Cipriano, Crugliano, Grammauta, Gregorietti, Renò, Ricci, Santacesaria, Maglietta and Carlucci. This is an open-access article distributed under the terms of the Creative Commons Attribution License (CC BY). The use, distribution or reproduction in other forums is permitted, provided the original author(s) and the copyright owner(s) are credited and that the original publication in this journal is cited, in accordance with accepted academic practice. No use, distribution or reproduction is permitted which does not comply with these terms.
*Correspondence: Elena Papale, ZWxlbmFiaWFuY2FwYXBhbGVAZ21haWwuY29t; Pasquale Ricci, cGFzcXVhbGUucmljY2lAdW5pYmEuaXQ=