- 1Mediterranean Institute of Oceanography, IRD, CNRS, Aix-Marseille University, Marseille, France
- 2Institut de Recherchen pour le Développement (IRD), Noumea, New Caledonia
- 3Department of Ocean Sciences, University of California, Santa Cruz, Santa Cruz, CA, United States
- 4Ecosystèmes Insulaires Ocèaniens (EIO), IRD, UPF, Ifremer, ILM, Tahiti, French Polynesia
- 5Marine Biological Section, Department of Biology, University of Copenhagen, Helsingør, Denmark
Coastal and open ocean regions of the Western Tropical South Pacific ocean have been identified as a hotspot of N2 fixation. However, the environmental factors driving the temporal variability of abundance, composition, and activity of diazotrophs are still poorly understood, especially during the winter season. To address this, we quantified N2 fixation rates and the abundance of seven diazotroph phylotypes (UCYN-A1 symbiosis, UCYN-B, UCYN-C, Trichodesmium, Het-1, Het-2, and Het-3) on a monthly basis during two full years (2012 to 2014) at four stations along a coast to open ocean transect in the New Caledonian lagoon. The total nifH gene concentration (sum of all nifH gene copies) clearly decreased from the barrier reef to the shore. Apart from UCYN-B, which peaked at very high abundances (106–108 nifH gene copies L–1) at two occasions at the coastal station, the UCYN-A1 symbiosis was the most abundant group at all stations, accounting for 79% of the total nifH gene copy counts along the transect (average abundance 4.2 ± 10.3 × 104 nifH gene copies L–1). The next most abundant groups were in order Trichodesmium (accounting for 14% of the total nifH gene copies), Het-groups (6% of the total) and UCYN-C (1% of the total). Statistical analyses reveal that the UCYN-A1 symbiosis and Het groups were associated with cold (<25°C) waters, high NOx and PO43–, weak winds from the south (occasionally southwest), while Trichodesmium and UCYN-C were associated with warmer (>25°C) waters, low NOx and PO43– concentrations, strong and (mostly) easterly winds. Average N2 fixation rates over the survey were 6.5 ± 6.7 nmol N L–1 d–1 and did not differ significantly among seasons. The year to year variability was more pronounced with average integrated rates significantly higher in the second year of the survey (162 ± 122 μmol N m–2 d–1) than the first year (66 ± 91 μmol N m–2 d–1). This dataset suggests that seasonality is less pronounced than previously thought, and that relatively high N2 fixation rates are maintained in the New Caledonian lagoon all year long, despite seasonal changes in the diazotroph community composition.
Introduction
Biological dinitrogen (N2) fixation is a main source of fixed N to the oceans and is performed by a suite of specialized cyanobacteria, bacteria and archaea called “diazotrophs” (Zehr, 2011). Fixed N input fluxes on a global basis vary between 106–120 Tg N year–1 (Galloway et al., 2004; Gruber, 2008; Jickells et al., 2017). The accuracy of those estimates is inevitably affected by the temporal and spatial resolution of measurements. Only few studies have addressed how the paucity of data on spatial and temporal distribution of N2 fixation may affect global fixed N input estimates. For instance, the global database compiled in Luo et al. (2012) contains 2480 data points of volumetric seawater N2 fixation rates from the northern hemisphere, while samples from the southern hemisphere only amount to 502. The temporal distribution of these data points also suffers from a strong seasonal bias as >65% of the N2 fixation measurements were made during the spring-summer season. Hence, most of our recapitulative knowledge of marine N2 fixation stems from the northern hemisphere in spring-summer conditions, largely from the sunlit layer of open ocean regions of the North Atlantic and North Pacific Oceans (Benavides and Voss, 2015; Böttjer et al., 2017).
Although the number of N2 fixation studies has increased in recent years, temporal and spatial resolution remains coarse, as oceanographic cruises commonly sample transects with stations separated by tens of nautical miles, and typically at monthly or seasonal time scales. For instance, N2 fixation rates varied four- to nine-fold between summer and winter at Station ALOHA in the Pacific Ocean between 2005 and 2013 (Böttjer et al., 2017). If higher diazotrophic activity during summer prevails among the available datasets it could cause an overestimation of basin-wide estimates, which illustrates the need for conducting sampling programs at finer temporal scales and throughout all seasons of the year.
Recent intensive sampling of the western tropical South Pacific (WTSP) Ocean revealed this region as a hotspot of N2 fixation with some of the highest rates ever recorded (Messer et al., 2015; Berthelot et al., 2017; Bonnet et al., 2017). However, most of our understanding of diazotrophic activity and diversity in the WTSP derives from open ocean cruises, the majority of which took place in austral summer. Little sampling effort has been devoted to coastal environments, despite their central role in the global cycling of nutrients (Bauer et al., 2013) and increasing evidence of their role as hotspots of diazotrophic activity (Mulholland et al., 2012, 2019; Tang and Cassar, 2019). In the Australian Great Barrier Reef, Hewson et al. (2007) reported nifH gene sequences corresponding to usual open-ocean diazotroph phylotypes such as the UCYN-A symbiosis, UCYN-B and the diatom-diazotroph association (DDA) Het-1 (Richelia associated with Rhizosolenia). Also in the Great Barrier Reef, Messer et al. (2017) found Trichodesmium erythraeum to be the dominant diazotroph in their nifH gene libraries and measured N2 fixation rates from 3 to 68 nmol N L–1 d–1.
The only other coastal N2 fixation studies in the WTSP are those from the reef systems of New Caledonia, the second largest in the world after the Great Barrier Reef, enclosing a 24,000 km2 lagoon. The New Caledonian lagoon waters are shallow (∼20 m on average) and mostly oligotrophic, although inorganic nutrients are detectable year-round in the waters affected by the river and anthropogenic discharges near Noumea city (Neveux et al., 2009; Ouillon et al., 2010). Previous studies have documented high N2 fixation rates (1.9 to 29.3 nmol N L–1 d–1, Garcia et al., 2007; Bonnet et al., 2016), occurrence of large Trichodesmium blooms (Rodier and Le Borgne, 2008; Tenório et al., 2018) and co-occurrence with other common oceanic diazotrophs such as DDAs, UCYN-A/haptophyte symbioses, Crocosphaera (UCYN-B; Biegala and Raimbault, 2008; Turk-Kubo et al., 2015; Henke et al., 2018), and UCYN-C (Turk-Kubo et al., 2015). Most of these studies were performed during austral summer conditions when diazotrophs are considered to be most abundant and N2 fixation rates maximal. Yet the effect of seasonal variability and nutrient inputs from the coast on the distribution and activity of these diazotrophic groups is lacking. Here we examine N2 fixation rates and diazotroph phylotype abundances at four stations along a coast to open-ocean transect in the New Caledonian lagoon during two full years (2012 to 2014) on a monthly basis in the framework of the “Grand Observatoire de l’environnement et de la biodiversité terrestre et marine du Pacifique Sud” (GOPS). Henke et al. (2018) examined the seasonal variability and diversity of UCYN-A/haptophyte symbioses relative to environmental conditions. In the current study we sought to expand our knowledge on the broader diazotroph community and on diazotrophic activity.
Materials and Methods
Sampling, Hydrographic and Meteorological Parameters
Between July 2012 and April 2014, seawater samples were collected monthly in the southwestern New Caledonian lagoon along a transect of four stations (L2, M09, M99, and D39; Figure 1) spanning from the reef barrier (Dumbea Pass) to the shore (Dumbea Bay), into which the Dumbea river flows. The sampling always started just before high tide to ensure that results would be comparable. Seawater was collected from three depths spanning the water column of the lagoon (1, 8, and 16 m at station L2, M09, D39, and 1, 3, and 6 m at station M99) using a trace metal clean Teflon pump connected to polyethylene tubing. Vertical hydrographic profiles were obtained at each station by casting a Seabird SBE19 CTD equipped with additional turbidity (Seapoint optical back scatter) and in situ fluorescence (Wet Lab Wetstar) sensors. Daily values of rainfall, wind direction and velocity were obtained from the Météo-France station at Faubourg Blanchot (Noumea, 22°16.30’ S–166°27.06’ E). Here we used averages of the data from the week preceding sampling in order to integrate meteorological variations.
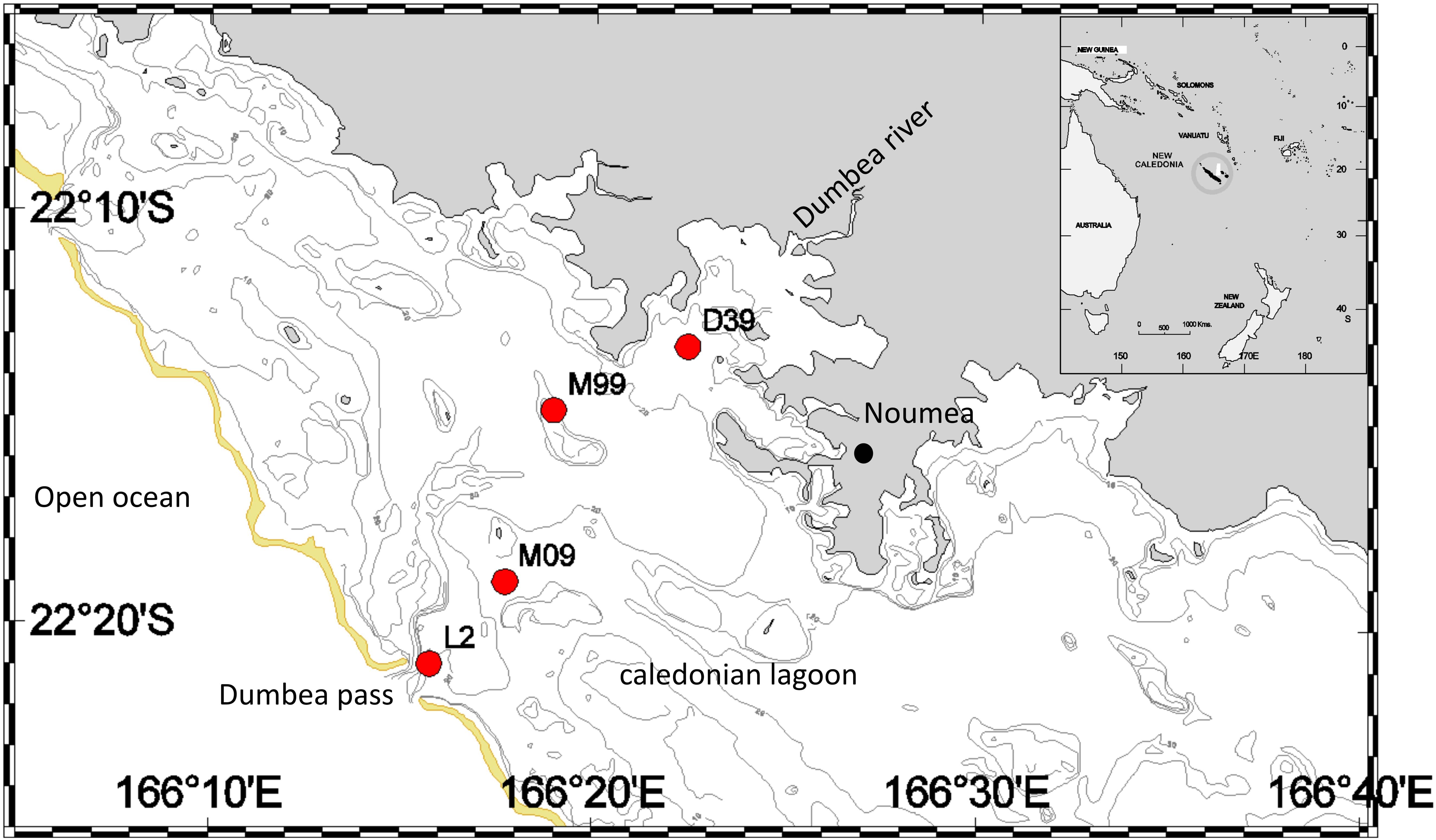
Figure 1. Map of the study site in the New Caledonian lagoon (Western Tropical South Pacific), including stations L2, M09, M99, and D39.
Nutrient and Chlorophyll a Analyses
Seawater samples for nutrient analyses were collected at each depth in acid-washed scintillation vials, poisoned with HgCl2 (10 μg L–1 final concentration) and maintained in the dark at 4°C until analysis. Soluble reactive phosphorus (PO43–; detection limit = 0.05 μmol L–1) and NOx (nitrite (NO2–) + nitrate (NO3–); detection limit = 0.01 μmol L–1) concentrations were determined according to Aminot and Kérouel (2007). Silicate concentrations were measured according to Mullin and Riley (1955) as modified by Fanning and Pilson (1973; detection limit = 0.05 μmol L–1). All assays were performed with a Bran + Luebbe AA3 autoanalyzer. Chlorophyll a (Chl a) concentrations were determined fluorometrically (Lorenzen, 1966) using a Turner Designs fluorometer (Trilogy module Chl a Acid–040) calibrated with pure Chl a standards (Sigma-Aldrich).
N2 Fixation Rates
Seawater samples were collected at each depth in transparent 4.5 L-polycarbonate bottles closed gas-tight with septum screw caps (Nalgene). Bottles were filled to overflow and amended with 5 mL of 98.9 atom% 15N2 (Cambridge isotopes) either by directly injecting the tracer as a bubble (Montoya et al., 1996) or as dissolved in a subset of seawater previously N2 degassed (Mohr et al., 2010) according to the methodology fully described in Berthelot et al. (2015). A comparison between the two methods did not show any significant difference (p > 0.05) between N2 fixation estimates (Supplementary Figure 1). To ensure accurate rate calculations, the 15N/14N ratio of the dissolved N2 pool in the incubation bottles was measured regularly whatever the method used. For this purpose, after 24 h of incubation with the 15N2 tracer, 12 mL subsamples of each bottle were moved into Exetainers® fixed with HgCl2 (final concentration 10 μg L–1), which were stored upside down in the dark at 4°C until analyzed by membrane inlet mass spectrometry (MIMS) according to Kana et al. (1994). Lastly, time zero (not 15N-enriched) samples were collected at each monthly survey and station to determine the natural N isotopic signature (EA-IRMS) of ambient particulate N (PN). The 15N2 gas batches used did not contain significant concentrations of other nitrogenous compounds labeled with 15N, as previously measured (Dabundo et al., 2014; Benavides et al., 2015). The minimum quantifiable rates (quantification limit, QL) were 0.035 nmol N L–1 d–1. Integrated rates over the water column were calculated by using the trapezoidal method.
DNA Extraction and Quantitative PCR
For DNA analyses, seawater samples were collected only from the surface (1 m) in 2.3 L polycarbonate bottles and 930–2,300 mL were filtered within 1 h through 0.2 μm Supor filters (Pall Gelman), that were stored at −80°C in bead beater tubes containing a mixture of 0.1-mm and 0.5-mm diameter glass beads (BioSpec Products, Bartlesville, OK, United States). DNA was extracted using the Qiagen Plant kit with additional steps for enhanced DNA recovery from cyanobacteria (Moisander et al., 2008) before automated on-column purification and elution using a Qiacube (Qiagen). All DNA samples were quantified by NanoDrop ND-1000 Spectrophotometry (Thermo Scientific).
The abundance of diazotrophs was determined using TaqMan® qPCR assays on either an ABI 7500 or StepOnePlus Real-Time PCR System (Applied Biosystems), with primer-probe sets for Trichodesmium (Church et al., 2005), UCYN-B (Moisander et al., 2010), UCYN-C (Church et al., 2005), Het-1 (Richelia in Rhizosolenia), Het-2 (Richelia in Hemiaulus), and Het-3 (Calothrix in Chaetoceros; Foster et al., 2007), and UCYN-A1 and UCYN-A2 (Henke et al., 2018). For the purpose of this study, UCYN-A1 and UCYN-A2 have been summed, yielding to total UCYN-A abundances. Standard dilutions (107–101) were run in duplicate, and samples and no-template controls (NTCs) were run in triplicate. NTCs were undetectable. The efficiency of the primer-probe sets was 82–102%. Inhibition tests were carried out on all samples and each primer-probe set by adding 2 μL of 105 standard to each sample. No inhibition was detected. The limit of detection (LOD) and detected but not quantifiable (DNQ) limits used were 1 and 8 gene copies per reaction, respectively, (Goebel et al., 2010). Samples that were below LOD were designated a value of 0 in the data set, whereas gene copies higher than LOD but less than DNQ were designated a conservative value of 1 nifH gene copy L–1. Note that abundances are reported as nifH gene copies L–1; extrapolating cell numbers from nifH gene copies can be difficult due to the possibility that some taxa have multiple genome copies (polyploidy; Sargent et al., 2016; White et al., 2018).
Statistical Analyses
Normal distribution tests (Shapiro–Wilk) by descriptive statistics were performed on the following parameters: temperature, salinity, Si(OH)4, NOx, PO43–, PON, N2 fixation, rainfall, wind speed, wind direction and nifH gene copies per liter seawater for UCYN-A symbiosis, UCYN-B, UCYN-C, Trichodesmium, Het-1, Het-2, and Het-3. All targets (UCYN-A symbiosis, UCYN-B, UCYN-C, Trichodesmium, Het-1, Het-2, and Het-3) and eight environmental parameters (PO43–, Chl a, silicates, rainfall, wind direction, NOx, salinity, and N2 fixation) were not normally distributed (Shapiro–Wilk, p < 0.05). To investigate the relationship between diazotroph community structure and environmental variables, a redundancy analysis (RDA) was conducted using XLSTAT, version 2020.1.1. To test if the abundance of diazotrophs was different between stations and seasons, two non-parametric tests were used: the Kruskal–Wallis test and the Mann–Whitney test with 72 observations (18 per station).
Results
Environmental Conditions
Seawater temperature ranged from 21.7°C to 28.1°C over the course of the survey, and was relatively homogeneous throughout the water column (Figure 2A). Maximum temperatures were measured between December and February, while minima were recorded between August and September, corresponding to the New Caledonian warm and cool seasons. The stations close to the coast (D39, M99) were generally warmer than those located close to the reef barrier (M09, L2; Figure 2A). Salinity ranged from 34.29 to 35.72, showing three sharp decreases in August 2012, May 2013 and January 2014 (Figure 2B), consistent with intense rainfall (Supplementary Table 1). This freshening was particularly pronounced at coastal stations D39 and M99 located close to the Dumbea river (Figure 2B).
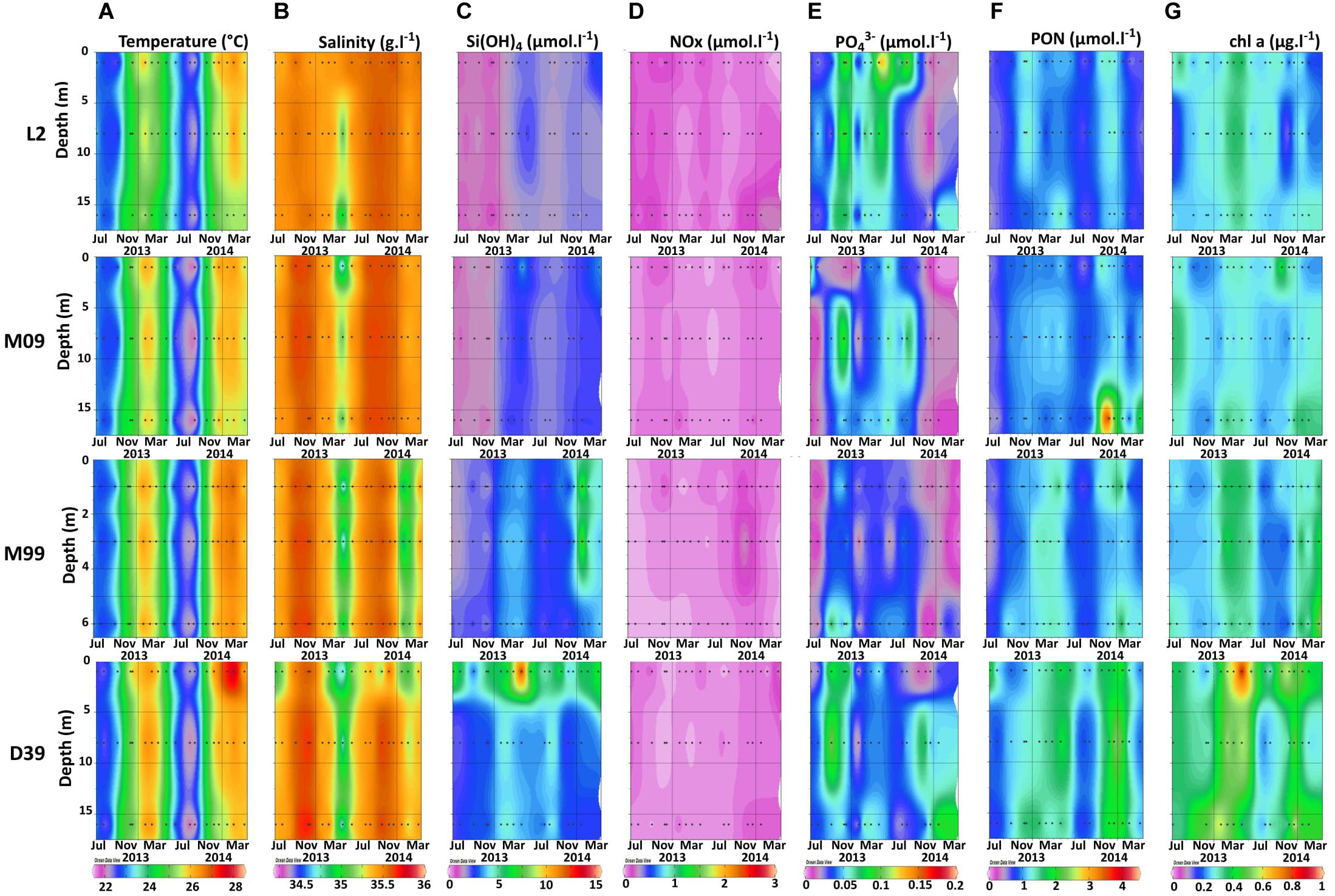
Figure 2. Vertical distributions of seawater temperature (A), salinity (B), Si(OH)4 concentrations (C), NOx concentrations (D), PO43– concentrations (E), PON concentrations (F), and chlorophyll a concentrations (G) over the 2-year survey at the stations L2, M09, M99, and D39.
NOx and PO43– concentrations (Figures 2D,E) ranged from undetectable to 0.31 μmol L–1 and to 0.16 μmol L–1, respectively, (Figure 2), with no clear seasonal trend. The NOx:PO43– was on average 3:1 over the study period, indicative of PO43– excess relative to NOx. The Si(OH)4 concentrations (range 0.8–15.0 μmol L–1) gradually increased from the reef (0.84–2.92 μmol L–1) to the coast (2.0–15.0 μmol L–1), where they were the highest at the surface (Figure 2C). The Si(OH)4:NOx ratio was on average 45 over the study period, indicative of Si(OH)4 excess relative to NOx. The biomass indicators (Chl a and PON concentrations) were higher at coastal stations (D39 and M99) compared to reef stations (M09 and L2), and generally higher during the warm season (November-April) compared to the cold one (May-October; Figures 2F,G). The average weekly wind direction indicated E-SE settled trade winds (6–10 m s–1) most of the year, with some sporadic S-SW episodes recorded in late winter-early summer 2012 and 2013 (Supplementary Table 1).
Rates of N2 Fixation in New Caledonian Waters
N2 fixation rates ranged from not quantifiable to 51.9 nmol N L–1 d–1 (mean over the survey: 6.5 ± 6.7 nmol N L–1 d–1). Consistent with hydrographic parameters, they were relatively homogeneous over the well-mixed water column and displayed similar temporal patterns among stations, although less variability was observed at station M99 compared to the three others (note that this station is shallower than the three others; Figure 3A). Generally, rates integrated over the water column (Figure 3B) were highest during austral summer months (October-March), but not significantly different (p > 0.05), and highly variable from one monthly survey to another. The year to year variability was more pronounced than the seasonal one: the average annual rates were significantly (p < 0.05) lower during the first year of the survey (July 2012–May 2013, 66 ± 91 μmol N m–2 d–1) than during the second year (June 2013–April 2014, 162 ± 122 μmol N m–2 d–1). When averaged over the whole survey, rates were the highest at station M09 (168 ± 153 μmol N m–2 d–1), followed by stations D39 (168 ± 122 μmol N m–2 d–1), L2 (117 ± 110 μmol N m–2 d–1), and M99 (48 ± 36 μmol N m–2 d–1, note that the depth of integration is 8 m instead of 16 m for the others).
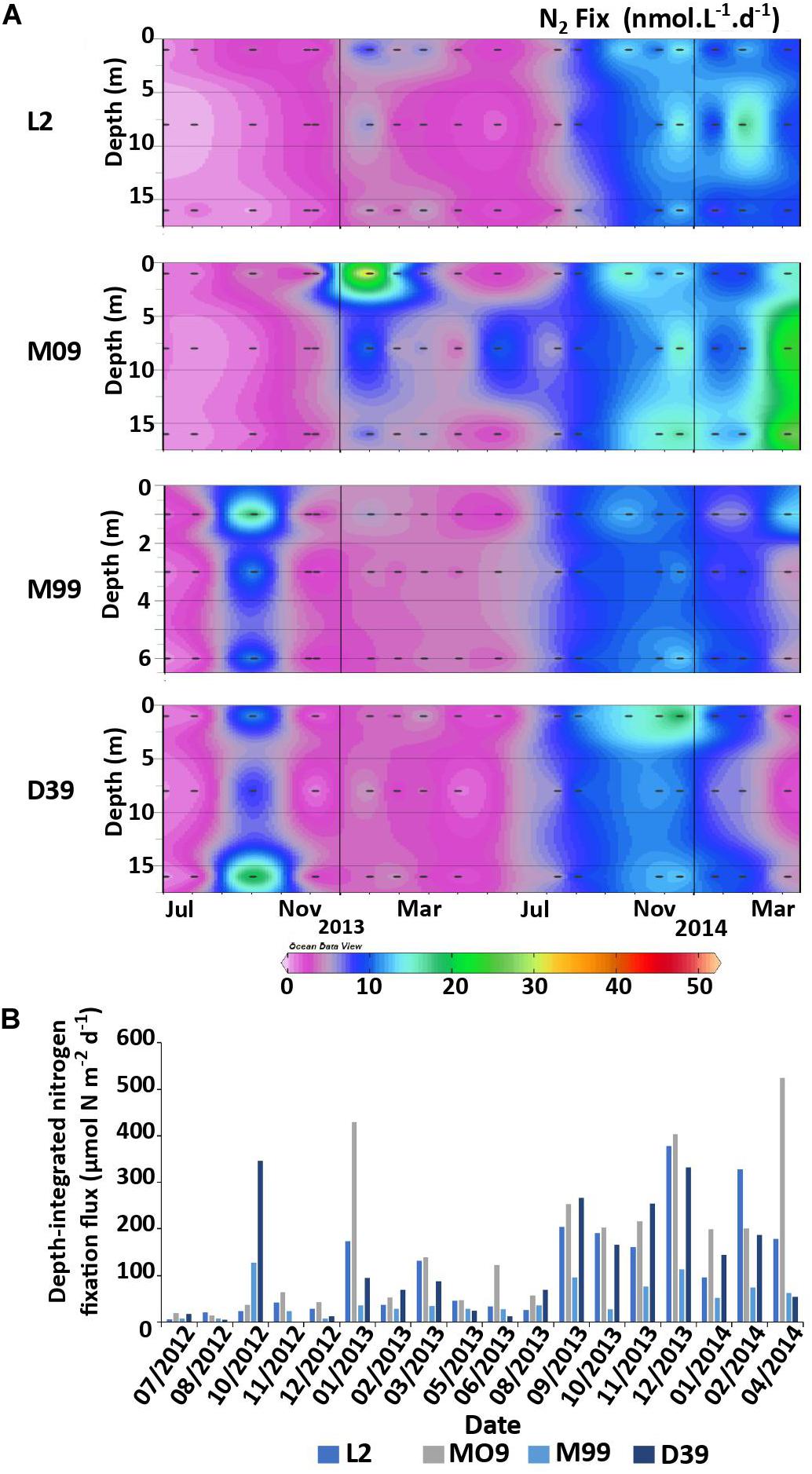
Figure 3. Vertical distributions of N2 fixation rates (nmol N L–1 d–1) over the 2-year survey at the stations L2, M09, M99, and D39 (A). Temporal dynamics of integrated N2 fixation rates at the four stations studied (B; the error bars correspond to the error propagation from the IRM measurements).
Spatiotemporal Variability of Diazotroph Abundances
Total nifH counts L–1 (sum of all nifH gene copies L–1 of the five phylotypes targeted by qPCR assays) showed limited seasonal variability and were on average 2.9 ± 23 × 106 nifH gene copies L–1 along the entire survey (Figure 4A). UCYN-B was the most abundant group, accounting for >99% of the total nifH gene copy counts on average over the survey all stations combined. This was due to very high abundances on two occasions at the coastal site D39 (8.4 × 106 and 2.0 × 108 nifH gene copies L–1 on Feb. 28 and May 2 2013; Figure 4B), but abundances were otherwise sporadic (below the limit of detection in 57 out of 71 samples). Not taking into account those two peaks, the total nifH clearly decreased along the transect from the barrier reef (Dumbea pass) to the shore (Dumbea river; Figure 4C). The UCYN-A symbiosis was the most abundant group, accounting for 79% of the total nifH gene copy counts along the transect (average abundance 4.2 ± 10.3 × 104 nifH gene copies L–1). The next most abundant groups were Trichodesmium (7.7 ± 28.2 × 103 nifH gene copies L–1, accounting for 14% of the total nifH gene copies), Het-groups (3.0 ± 7.9 × 103 nifH gene copies L–1, 6% of the total; Het-1 accounted for 96% of the Het gene copies), UCYN-C (6.8 ± 9.7 × 102 nifH gene copies L–1, 1% of the total nifH gene copies), and finally UCYN-B (1.5 ± 4.8 × 102 nifH gene copies L–1, 0.3% of the total nifH gene copies; Figure 4A). The average abundance of Trichodesmium and UCYN-A increased significantly (p < 0.05) from the coast (station D39; 6.7 × 102 and 4.2 × 103 nifH gene copies L–1, respectively) to the reef (station L2; 2.2 × 104 and 1.1 × 105 nifH gene copies L–1, respectively). On the contrary, Het- groups were significantly (p < 0.05) more abundant at the two inner stations M99 and D39 compared to the reef stations.
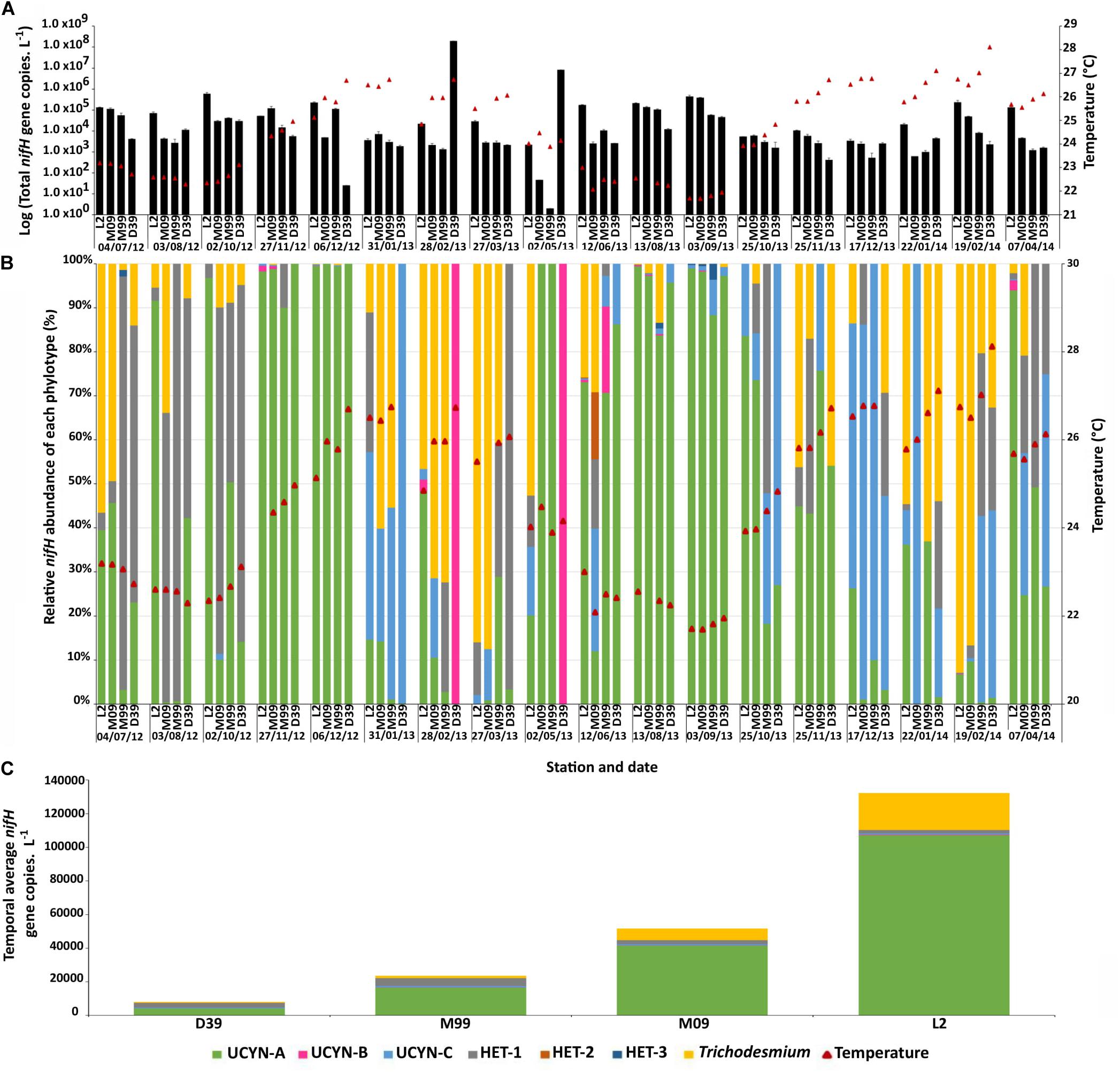
Figure 4. Temporal dynamics of total nifH gene counts L–1 over the survey in surface waters (A; the errors bars correspond to the errors propagation from triplicate analyses), relative abundance of each diazotroph phylotype targeted in this study and sea surface temperature (SST, red triangles; B), and average nifH counts L–1 over the survey per target group and per station (C).
While the total nifH gene count did not differ between seasons, the nifH gene abundance per target group showed temporal variability (Figure 4B). Using temperature categories (< or >25°C; Moisander et al., 2010; Bonnet et al., 2015), the UCYN-A symbiosis and Het groups were 4.8 and 4.6 times more abundant in <25°C waters compared to >25°C. The opposite trend was observed for Trichodesmium and UCYN-C, which were 1.6 and 1.2 more abundant in >25°C waters.
The redundancy analysis (RDA) shows that the correlation between environmental factors and diazotroph phylotypes is mainly explained by the first two axes (75% of the total variance; Figure 5). The factorial axis 1 separates two phylotypes: (i) Trichodesmium and UCYN-C, associated with low NOx and PO43– concentrations, warm waters, strong and (mostly) wind direction (dominant easterly winds), and high N2 fixation rates, (ii) UCYN-A symbiosis and Het groups, associated with higher NOx and PO43–, colder waters, weaker winds from the south (occasionally southwest), and lower N2 fixation rates. The factorial axis 2 separates UCYN-B from all other nifH phylotypes. UCYN-B are mainly associated with rainfall, desalted and warm waters characterized by high biomass (POC, PON, and Chl a).
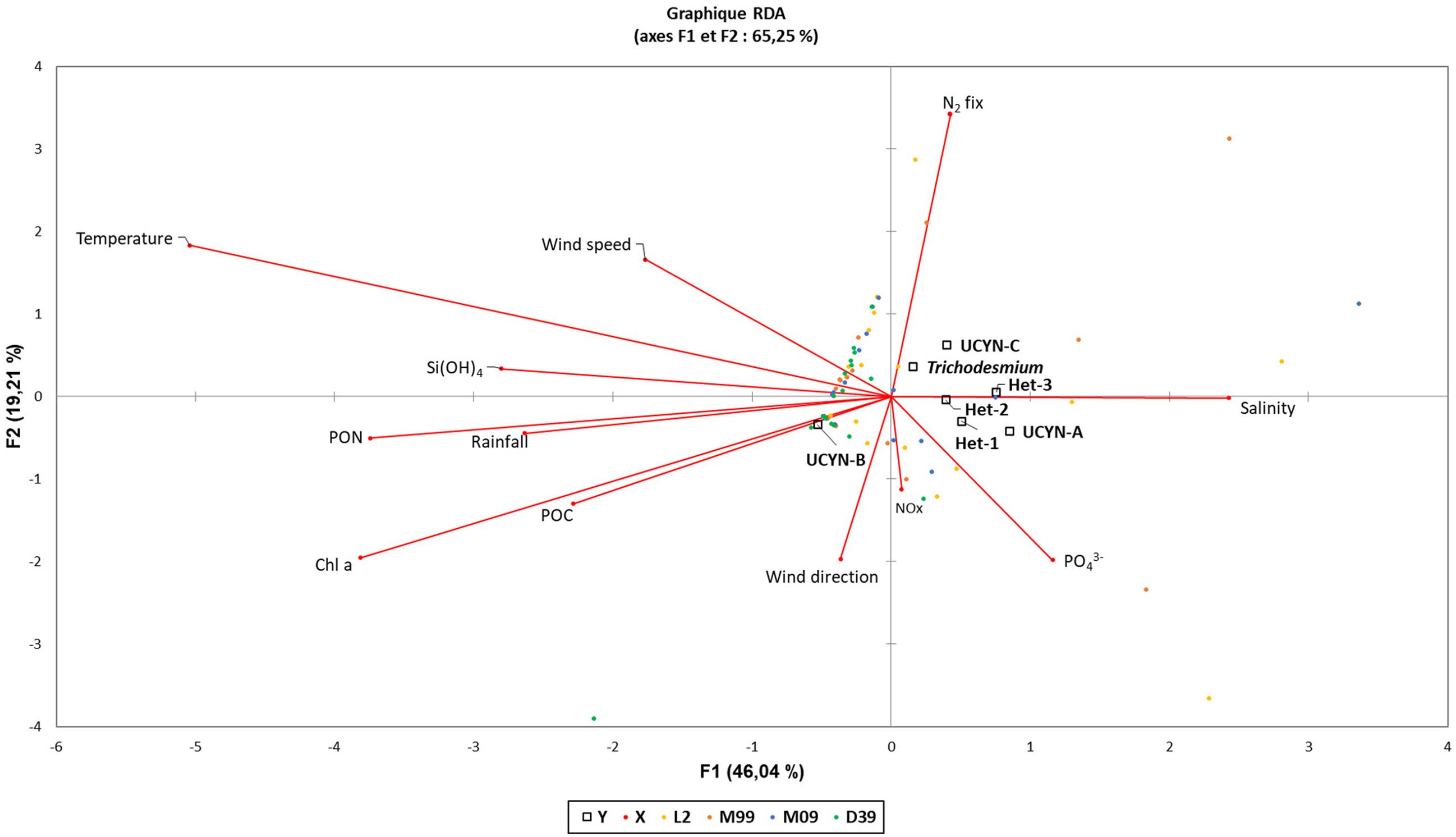
Figure 5. Redundancy analysis (RDA) biplot of abundance of each diazotroph phylotype targeted in this study (UCYN-A, UCYN-B, UCYN-C, Trichodesmium, Het-1, Het-2, and Het-3) and environmental parameters [sea surface temperature, Si(OH)4, PON, POC, rainfall, Chl a, wind direction, wind speed, NOx, PO43–, Salinity, and N2 fixation rates]. Points represents one of all diazotrophic phylotypes targeted at a specific time-point (n = 71), each diazotrophic phylotype are represented by squares and environmental parameters are indicated by lines with arrows.
Discussion
Here we present a spatiotemporal study of N2 fixation rates and diazotrophic community composition over 2 years in the New Caledonian lagoon. N2 fixation was detected and diazotrophs were present throughout the year with generally high total mean abundances (106 nifH gene copies L–1). Inorganic nutrients are supplied to the lagoon by the Dumbea river, however, bioavailable forms are generally low (∼0.05 μmol L–1) in most of the lagoon waters, and NOx are depleted relative to PO43–, making these waters favorable for N2 fixation. The total number of diazotrophs detected by qPCR increased toward the more oligotrophic reef stations (Figure 4C). This pattern was mainly influenced by the UCYN-A symbiosis, which were more than two orders of magnitude more abundant at offshore stations receiving open-ocean influences (Fichez et al., 2010) compared to inner stations. This is in accordance with previous studies reporting surface UCYN-A symbiosis abundances of 103–106 nifH gene copies L–1 in the Coral Sea off New Caledonia (Moisander et al., 2010; Bonnet et al., 2015; Messer et al., 2015), and at the entrance of the Noumea lagoon (Turk-Kubo et al., 2015). This is also consistent with earlier reports of significant N2 fixation and diazotroph abundances in the <3 μm size-fraction in New Caledonian waters (Garcia et al., 2007; Biegala and Raimbault, 2008). However, our results contrast with data from open ocean waters around Melanesian archipelagos, where Trichodesmium and UCYN-B outnumber other groups and the UCYN-A symbiosis is almost absent in surface waters and only develop deeper in the water column (Berthelot et al., 2017; Stenegren et al., 2018), at least during austral summer conditions. However, Moisander et al. (2010) found high abundances of the UCYN-A symbiosis close to New Caledonia (>106 nifH gene copies L–1), coinciding with a local upwelling system characterized by colder temperatures (∼25°C) compared with surrounding waters at the same latitude (27–30°C). Bonnet et al. (2015) also reported high UCYN-A symbiosis abundances in the Coral Sea off New Caledonia but during austral winter conditions [Surface Sea Temperature (SST) ∼22°C]. These studies support a niche partitioning between surface warm (>25°C) waters where Trichodesmium and UCYN-B thrive, and cooler (<25°C) waters preferred by the UCYN-A symbiosis. We observed the same pattern in the inner lagoon waters, where the UCYN-A symbiosis was ∼5 times more abundant during the winter season (SST < 25°C), but to a lesser extent compared to open ocean waters. Indeed, the UCYN-A symbiosis still accounted for ∼60% of the diazotroph community during the summer season (SST > 25°C), which is not the case in the nearby open ocean waters (Moisander et al., 2010; Stenegren et al., 2018). The dominance of the UCYN-A symbiosis in the New Caledonian lagoon also contrasts with recent reports of Trichodesmium dominance and low abundances of the UCYN-A symbiosis at Great Barrier Reef and in the adjacent Coral Sea waters off Australia, especially during the winter season (Messer et al., 2015, 2017).
The UCYN-A symbiosis dominated the diazotrophic community at the four stations over both the austral summer and winter seasons (Figure 4), suggesting the lagoon waters provide favorable conditions for their growth. Henke et al. (2018) found that SST and Chl a concentrations were negative predictors of UCYN-A symbiosis abundance, and NOx concentration was a positive predictor. This may be explained by the SE winds triggering an upwelling off the southwestern coast of New Caledonia (north of the Dumbea pass) that may decrease seawater temperature by 5°C in summer and 1°C in winter (Hénin and Cresswell, 2005; Ganachaud et al., 2010), and affects the lagoon SST by incoming tides. SE winds also drive cool, oligotrophic South Equatorial Current waters through the lagoon, arguing for a possible open ocean origin of the UCYN-A symbiosis detected in lagoon waters (Henke et al., 2018). The positive correlation between the UCYN-A symbiosis and S and SE winds (Henke et al., 2018) supports this hypothesis. NOx concentrations are an order of magnitude higher in the lagoon than in open-ocean waters (Moutin et al., 2018) and Great Barrier Reef (Messer et al., 2017), which may also explain why UCYN-A thrive in the New Caledonian lagoon compared to other more oligotrophic areas of the WTSP (open-ocean waters of the Melanesian archipelagos; Bonnet et al., 2015; Stenegren et al., 2018). This is also in accordance with Mills et al. (2020) who recently reported an enhanced activity of the UCYN-A symbiosis after NO3- additions in N-limited environments. Finally, due to the inability of UCYN-A to fix inorganic carbon (Tripp et al., 2010), it is likely that the high abundance of the symbiosis in lagoon waters is sustained by photosynthates of the haptophyte host and/or labile dissolved organic matter input from the Dumbea River. Indeed, a recent study reported an increase in N2 fixation rates and UCYN-A nifH gene expression in the mouth of the Dumbea River compared to waters located close to the reef (Benavides et al., 2018).
Surprisingly, Trichodesmium abundances were lower than those of the UCYN-A symbiosis (Figure 4). Over the past 20 years, Trichodesmium has been routinely studied in the New Caledonian lagoon and surrounding waters during summer conditions using satellite observations (Dupouy et al., 2000, 2011; Dupouy et al., 2018a,b) and direct field measurements (Rodier and Le Borgne, 2008; Turk-Kubo et al., 2015). These studies have revealed higher abundances in summer (∼0–250 trichomes L–1 and ∼104 nifH genes copies L–1, respectively) than during our surveys (7.7 × 103 nifH genes copies L–1; note that microscope cell counts do not equal nifH counts; White et al., 2018). Trichodesmium abundances gradually increased from the coast to the open-ocean (D39 = 6.66 × 102; M99 = 1.4 × 103; M09 = 6.89 × 103; and L2 = 2.19 × 104 nifH gene copies L–1), suggesting that they could have an open-ocean origin and could be advected toward the coast with rising tide currents that flow over the reef (Jarrige et al., 1975; Rougerie, 1986). The abundance of Trichodesmium was higher during the summer and SST clustered positively with the abundance of Trichodesmium, in agreement with earlier findings (Tenório et al., 2018). This is consistent with previous studies showing that Trichodesmium is constrained by SST (20–30°C; Capone et al., 1997) and thrives at temperatures of 25°C or warmer (e.g., Breitbarth et al., 2007; Fu et al., 2014). The distribution pattern of Trichodesmium determined in this study is opposite to that of the UCYN-A symbiosis, whose abundances peaked during the winter. This points toward an ecological niche partitioning between the two phylotypes, a temporal segregation that mirrors the spatial segregation observed in open-ocean waters (Moisander et al., 2010; Bonnet et al., 2015; Stenegren et al., 2018).
Heterocystous cyanobacterial symbionts (Het- groups) were present almost throughout the survey (mean 3.0 ± 7.9 × 103 nifH gene copies L–1; Figure 4), and were clearly dominated by Het-1 targeting Richelia, in line with previous lagoon (Turk-Kubo et al., 2015) and regional open-ocean studies (Stenegren et al., 2018). The abundance of Het- groups was maximum at the two inner stations (close to the Dumbea river mouth) impacted by nutrient input, and mostly in waters <25°C, consistent with previous studies reporting high Het- abundances in the vicinity of the Amazon and Mekong river plumes (e.g., Carpenter et al., 1999; Subramaniam et al., 2008; Bombar et al., 2011).
Apart from the two peaks of UCYN-B at the coastal station (see below), the unicellular groups B and C were the least abundant in lagoon waters over the 2-years survey. They did not show any clear spatial or seasonal pattern, but were present at low abundances (102 to 103 nifH gene copies L–1) over space and time, which is consistent with our observations during a 3-week survey performed close to the reef (Turk-Kubo et al., 2015). UCYN-B peaked on two occasions (8.4 × 106 and 2.0 × 108 nifH gene copies L–1 on February 28 and May 2 2013) coinciding with high rainfall and a peak of Si(OH)4 on May, but not in February, making a linkage questionable.
N2 fixation rates (unquantifiable to 51.9 nmol N L–1 d–1; mean over the survey: 6.5 ± 6.7 nmol N L–1 d–1) are in line with those measured previously in the lagoon (8.0–27.7 nmol N L–1 d–1; Biegala and Raimbault, 2008; Berthelot et al., 2015; Bonnet et al., 2016) and open-ocean mixed layer waters around the Melanesian archipelagos (unquantifiable to 48 nmol N L–1 d–1, mean 8.9 ± 10.0 nmol N L–1 d–1, Bonnet et al., 2018), but lower than those off the tropical north-northeast coast of Australia (3 to 68 nmol N L–1 d–1, mean 32.0 ± 24.0 nmol N L–1 d–1; Messer et al., 2017). When compared with Pacific surface water rates at similar latitudes, including the Eastern tropical South Pacific (∼0.9 nmol N L–1 d–1; Dekaezemacker et al., 2013) and the tropical South Pacific Gyre (∼0.5 nmol N L–1 d–1; Raimbault and Garcia, 2008; Bonnet et al., 2018), the rates reported here are high. Indeed, our observations, along with those previously reported in the WTSP (Montoya et al., 2004; Bonnet et al., 2009, 2015, 2018; Messer et al., 2015, 2017; Berthelot et al., 2017), reinforce the view of the WTSP region as a hotspot of diazotroph activity within the southern hemisphere (Bonnet et al., 2017).
N2 fixation rates were higher (although not statistically significant) during summer, but the year to year variability was more pronounced than the seasonal variability with significantly higher N2 fixation rates in the second year of the survey (2013; Figure 3), consistent with low PO43– concentrations. This pattern was mostly dictated by offshore stations and are synchronous with higher Trichodesmium and UCYN-C abundances (by a factor of 2.5) at station L2 in the second year. The explanation for such an increase is unclear and may be due to a combination of: (i) higher seawater temperatures (25.44°C) in austral summer 2013 compared to 2012 (24.67°C), which may have stimulated diazotroph activity (Stal, 2009), (ii) higher occurrence of S-SW winds in 2013 that may have advected more Trichodesmium from the open-ocean. Notably, the ENSO index and rainfall did not differ significantly between the 2 years and are, therefore, unlikely to explain the observed differences.
With a few exceptions (Garcia et al., 2007; Bonnet et al., 2015), previous N2 fixation studies from the New Caledonian lagoon and the WTSP were conducted over the austral summer, which is considered the main N2 fixation season in the region (Garcia et al., 2007). Our data suggest that seasonality in the New Caledonian lagoon is less pronounced than previously thought, and that relatively high N2 fixation rates are maintained all year long, despite seasonal changes in the dominant diazotrophs. During the 2 years of study in the New Caledonian lagoon, the composition of the diazotrophic community was variable, but provided a constant N input.
Assuming that diazotrophs fix on average 115 ± 47 μmol N m–2 d–1 throughout the year (the average of the four stations) and considering a southwestern lagoon area of 2,066 km2, planktonic N2 fixation would introduce 0.6–1.7 × 109 g N year–1 to the lagoon. For comparison, the inorganic N inputs from the three major rivers flowing into the southwestern lagoon (Dumbea, Pirogue and La Coulée rivers) calculated from mean annual river flows from Direction of Food and Rural Veterinary Affairs (Direction des Affaires Vétérinaires Alimentaires et Rurales, DAVAR, New Caledonia) and dissolved NOx concentrations data would introduce 0.03 × 109 g N year–1, i.e., 22–56 times less than N2 fixation. Consequently, planktonic N2 fixation represents a significant source of N for biological communities of the southwestern lagoon. In comparison, Charpy et al. (2007) reported mean benthic N2 fixation rates for the southwestern lagoon of 293 ± 96 μmol N m–2 d–1, i.e., three times higher than planktonic N2 fixation. Collectively, these results suggest that N2 fixation plays a critical role in sustaining the productivity of the New Caledonian waters, which features an exceptional diversity and is listed as a UNESCO World Heritage Site.
Conclusion
Several studies have pinpointed the importance of N2 fixation for sustaining both planktonic (Berthelot et al., 2015) and benthic (Benavides et al., 2016) productivity during the summer season in the New Caledonian lagoon, but here we document that high fixed N input takes place also during the cold season. Our analyses suggest that the meteorological and physicochemical characteristics of New Caledonian lagoon waters lead to the heterogeneity of diazotrophic populations both at seasonal and annual timescales. The UCYN-A symbiosis dominate the diazotrophic community, even during warmer, summer months, and likely provide a constant background of fixed N to surrounding waters, together with other UCYN groups (UCYN-B and UCYN-C). Trichodesmium is second most abundant group, peaking preferentially during summer months, and adding additional fixed N during the summer. However, the year to year variability was more pronounced, highlighting the need for longer time-series studies with fine temporal and geographical resolution to refine our understanding of the environmental factors regulating diazotrophs.
Data Availability Statement
The raw data supporting the conclusions of this article will be made available by the authors, without undue reservation.
Author Contributions
SB, MR, and CD conceived the study. SB, AD, and CD acquired samples and data. ES, MB, KT-K, HC, BH, SB, MR, OG, and CD analyzed samples and data. ES, MB, KT-K, BH, LR, and SB interpreted the data. ES, SB, and MB wrote the manuscript. All authors revised the manuscript.
Conflict of Interest
The authors declare that the research was conducted in the absence of any commercial or financial relationships that could be construed as a potential conflict of interest.
Funding
This research is a contribution of the GOPS project funded by the Institut de Recherche pour le Développement (IRD). SB, ES, and MB are supported by IRD. KT-K, BH, and HC were supported by a grant from the Simons Foundation (SCOPE Award ID 329108, to Jonathan Zehr) and MB and LR were supported by grant 6108–00013 from the Danish Council for independent research. The lagoon Figure 1 was realized with the MapCamelia software developed by P. Douillet, Mediterranean Institute of Oceanography (MIO).
Acknowledgments
The authors thank the crew of the R/V Coris, as well as the Laboratoire des Moyens Analytiques (IMAGO, Noumea) for nutrient analyses and in situ support during surveys.
Supplementary Material
The Supplementary Material for this article can be found online at: https://www.frontiersin.org/articles/10.3389/fmars.2020.581755/full#supplementary-material
References
Aminot, A., and Kérouel, R. (2007). “Dosage automatique des nutriments dans les eaux marines: Méthodes en flux continu,” in Méthodes d’analyse en Milieu Marin, ed. Ifremer 188.
Bauer, J. E., Cai, W. J., Raymond, P. A., Bianchi, T. S., Hopkinson, C. S., and Regnier, P. A. G. (2013). The changing carbon cycle of the coastal ocean. Nature 504, 61–70. doi: 10.1038/nature12857
Benavides, M., Houlbreque, F., Camps, M., Lorrain, A., Grosso, O., and Bonnet, S. (2016). Diazotrophs: A non-negligible source of nitrogen for the tropical coral Stylophora pistillata. J. Exp. Biol. 219, 2608–2612. doi: 10.1242/jeb.139451
Benavides, M., Martias, C., Elifantz, H., Berman-Frank, I., Dupouy, C., and Bonnet, S. (2018). Dissolved organic matter influences N2 fixation in the New Caledonian lagoon (Western Tropical South Pacific). Front. Mar. Sci. 5:89. doi: 10.3389/fmars.2018.00089
Benavides, M., Moisander, P., Berthelot, H., Dittmar, T., Grosso, O., and Bonnet, S. (2015). Mesopelagic N2 fixation related to organic matter composition in the Solomon and Bismarck Seas (Southwest Pacific). PLoS One 10:e0143775. doi: 10.1371/journal.pone.0143775
Benavides, M., and Voss, M. (2015). Five decades of N2 fixation research in the North Atlantic Ocean. Front. Mar. Sci. 2:40. doi: 10.3389/fmars.2015.00040
Berthelot, H., Benavides, M., Moisander, P. H., Grosso, O., and Bonnet, S. (2017). High-nitrogen fixation rates in the particulate and dissolved pools in the Western Tropical Pacific (Solomon and Bismarck Seas). Geophys. Res. Lett. 44, 8414–8423. doi: 10.1002/2017GL073856
Berthelot, H., Moutin, T., L’Helguen, S., Leblanc, K., Hélias, S., Grosso, O., et al. (2015). Dinitrogen fixation and dissolved organic nitrogen fueled primary production and particulate export during the VAHINE mesocosm experiment (New Caledonia lagoon). Biogeosciences 12, 4099–4112. doi: 10.5194/bg-12-4099-2015
Biegala, I. C., and Raimbault, P. (2008). High abundance of diazotrophic picocyanobacteria (<3 μm) in a Southwest Pacific coral lagoon. Aquat. Microb. Ecol. 51, 45–53. doi: 10.3354/ame01185
Bombar, D., Moisander, P. H., Dippner, J. W., and Foster, R. A. (2011). Distribution of diazotrophic microorganisms and nifH gene expression in the Mekong River plume during intermonsoon. Mar. Ecol. Prog. Ser. 424, 39–52. doi: 10.3354/meps08976
Bonnet, S., Berthelot, H., Turk-Kubo, K., Cornet-Barthaux, V., Fawcett, S., Berman-Frank, I., et al. (2016). Diazotroph derived nitrogen supports diatom growth in the South West Pacific: a quantitative study using nanoSIMS. Limnol. Oceanogr. 61, 1549–1562. doi: 10.1002/lno.10300
Bonnet, S., Biegala, I. C., Dutrieux, P., Slemons, L. O., and Capone, D. G. (2009). Nitrogen fixation in the western equatorial Pacific: rates, diazotrophic cyanobacterial size class distribution, and biogeochemical significance. Glob. Biogeochem. Cycles 23:GB3012. doi: 10.1029/2008GB003439
Bonnet, S., Caffin, M., Berthelot, H., Grosso, O., Benavides, M., Helias-Nunige, S., et al. (2018) In depth characterization of diazotroph activity across the Western Tropical South Pacific hot spot of N2 fixation. Biogeosciences 15, 4215–4232. doi: 10.5194/bg-15-4215-2018
Bonnet, S., Caffin, M., Berthelot, H., and Moutin, T. (2017). Hot spot of N2 fixation in the western tropical South Pacific pleads for a spatial decoupling between N2 fixation and denitrification. Proc. Natl. Acad. Sci. U.S.A. 114, E2800–E2801. doi: 10.1073/pnas.1619514114
Bonnet, S., Rodier, M., Turk-Kubo, K. A., Germineaud, C., Menkes, C., Ganachaud, A., et al. (2015). Contrasted geographical distribution of N2 fixation rates and nifH phylotypes in the Coral and Solomon Seas (southwestern Pacific) during austral winter conditions. Glob. Biogeochem. Cycles 29, 1874–1892. doi: 10.1002/2015gb005117
Böttjer, D., Dore, J. E., Karl, D. M., Letelier, R. M., Mahaffey, C., Wilson, S. T., et al. (2017). Temporal variability of nitrogen fixation and particulate nitrogen export at Station ALOHA. Limnol. Oceanogr. 62, 200–216. doi: 10.1002/lno.10386
Breitbarth, E., Oschlies, A., and Laroche, J. (2007). Physiological constraints on the global distribution of Trichodesmium - effect of temperature on diazotrophy. Biogeosciences 4, 53–61. doi: 10.5194/bg-4-53-2007
Capone, D. G., Zehr, J. P., Paerl, H. W., Bergman, B., and Carpenter, E. J. (1997). Trichodesmium, a globally significant marine cyanobacterium. Science 276, 1221–1229. doi: 10.1126/science.276.5316.1221
Carpenter, E. J., Montoya, J. P., Burns, J., Mulholland, M. R., Subramaniam, A., and Capone, D. G. (1999). Extensive bloom of a N2-fixing diatom/cyanobacterial association in the tropical Atlantic Ocean. Mar. Ecol. Prog. Ser. 185, 273–283. doi: 10.3354/meps185273
Charpy, L., Alliod, R., Rodier, M., and Golubic, S. (2007). Benthic nitrogen fixation in the SW New Caledonia lagoon. Aquat. Microb. Ecol. 47, 73–81. doi: 10.3354/ame047073
Church, M. J., Jenkins, B. D., Karl, D. M., and Zehr, J. P. (2005). Vertical distributions of nitrogen-fixing phylotypes at Stn ALOHA in the oligotrophic North Pacific Ocean. Aquat. Microb. Ecol. 38, 3–14. doi: 10.3354/ame038003
Dabundo, R., Lehmann, M. F., Treibergs, L., Tobias, C. R., Altabet, M. A., Moisander, P. H., et al. (2014). The contamination of commercial 15N2 gas stocks with 15N-labeled nitrate and ammonium and consequences for nitrogen fixation measurements. PLoS One 9:e110335. doi: 10.1371/journal.pone.0110335
Dekaezemacker, J., Bonnet, S., Grosso, O., Moutin, T., Bressac, M., and Capone, D. G. (2013). Evidence of active dinitrogen fixation in surface waters of the eastern tropical South Pacific during El Niño and la Niña events and evaluation of its potential nutrient controls. Glob. Biogeochem. Cycles 27, 768–779. doi: 10.1002/gbc.20063
Dupouy, C., Benielli-Gary, D., Neveux, J., Dandonneau, Y., and Westberry, T. K. (2011). An algorithm for detecting Trichodesmium surface blooms in the South Western Tropical Pacific. Biogeosciences 8, 3631–3647. doi: 10.5194/bg-8-3631-2011
Dupouy, C., Frouin, R., Tedetti, M., Maillard, M., Rodier, M., Duhamel, S., et al. (2018a). Diazotrophic Trichodesmium impact on UV VIS radiance and pigment composition in the South West Tropical Pacific. Biogeosciences 15, 5249–5269. doi: 10.5194/bg-15-5249-2018
Dupouy, C., Lefèvre, J., Wattelez, G., Martias, C., Andreoli, R., and Lille, D. (2018b). “Suivi par satellite de l’environnement des lagons et récifs,” in Nouvelle-Calédonie : Archipel de Corail, Marseille (FRA), ed. P. Claude and M. Jean-Paul (pref.) (Noumea: IRD), 33–38. doi: 10.4000/books.irdeditions.27857
Dupouy, C., Neveux, J., Subramaniam, A., Mulholland, M. R., Montoya, J. P., Campbell, L., et al. (2000). Satellite captures Trichodesmium blooms in the southwestern tropical Pacific. Eos 81, 13–16. doi: 10.1029/00eo00008
Fanning, K. A., and Pilson, M. E. Q. (1973). On the spectrophotometric determination of dissolved silica in natural waters. Anal. Chem. 45, 136–140. doi: 10.1021/ac60323a021
Fichez, R., Chifflet, S., Douillet, P., Gérard, P., Gutierrez, F., Jouon, A., et al. (2010). Biogeochemical typology and temporal variability of lagoon waters in a coral reef ecosystem subject to terrigenous and anthropogenic inputs (New Caledonia). Mar. Pollut. Bull. 61, 309–322. doi: 10.1016/j.marpolbul.2010.06.021
Foster, R. A., Subramaniam, A., Mahaffey, C., Carpenter, E. J., Capone, D. G., and Zehr, J. P. (2007). Influence of the Amazon River plume on distributions of free-living and symbiotic cyanobacteria in the western tropical north Atlantic Ocean. Limnol. Oceanogr. 52, 517–532. doi: 10.4319/lo.2007.52.2.0517
Fu, F., Yu, E., Garcia, N., Gale, J., Luo, Y., Webb, E., et al. (2014). Differing responses of marine N2 fixers to warming and consequences for future diazotroph community structure. Aquat. Microb. Ecol. 72, 33–46. doi: 10.3354/ame01683
Galloway, J. N., Dentener, F. J., Capone, D. G., Boyer, E. W., Howarth, R. W., Seitzinger, S. P., et al. (2004). Nitrogen cycles: past, present, and future. Biogeochemistry 70, 153–226. doi: 10.1007/s10533-004-0370-0
Ganachaud, A., Vega, A., Rodier, M., Dupouy, C., Maes, C., Marchesiello, P., et al. (2010). Observed impact of upwelling events on water properties and biological activity off the southwest coast of New Caledonia. Mar. Pollut. Bull. 61, 449–464. doi: 10.1016/j.marpolbul.2010.06.042
Garcia, N., Raimbault, P., and Sandroni, V. (2007). Seasonal nitrogen fixation and primary production in the Southwest Pacific: Nanoplankton diazotrophy and transfer of nitrogen to picoplankton organisms. Mar. Ecol. Prog. Ser. 343, 25–33. doi: 10.3354/meps06882
Goebel, N. L., Turk, K. A., Achilles, K. M., Montoya, J. P., Edwards, C. A., and Zehr, J. P. (2010). Abundance and distribution of major groups of diazotrophic cyanobacteria and their potential contribution to N2 fixation in the tropical Atlantic ocean. Environ. Microbiol. 12, 3272–3289. doi: 10.1111/j.1462-2920.2010.02303.x
Hénin, C., and Cresswell, G. R. (2005). Upwelling along the western barrier reef of New Caledonia. Mar. Freshw. Res. 56, 1005–1010. doi: 10.1071/MF04266
Henke, B. A., Turk-Kubo, K. A., Bonnet, S., and Zehr, J. P. (2018). Distributions and abundances of sublineages of the N2-fixing cyanobacterium Candidatus Atelocyanobacterium thalassa (UCYN-A) in the New Caledonian coral lagoon. Front. Microbiol. 9:554. doi: 10.3389/fmicb.2018.00554
Hewson, I., Moisander, P. H., Morrison, A. E., and Zehr, J. P. (2007). Diazotrophic bacterioplankton in a coral reef lagoon: phylogeny, diel nitrogenase expression and response to phosphate enrichment. ISME J. 1, 78–91. doi: 10.1038/ismej.2007.5
Jarrige, F., Radok, R., Krause, G., and Rual, P. (1975). Courants dans le lagon de Noumea, Nouvelle Calédonie. Rapp. ORSTOM (Noumea). Adelaide: FUA Publication.
Jickells, T. D., Buitenhuis, E., Altieri, K., Baker, A. R., Capone, D., Duce, R. A., et al. (2017). A reevaluation of the magnitude and impacts of anthropogenic atmospheric nitrogen inputs on the ocean. Glob. Biogeochem. Cycles 31, 289–305. doi: 10.1002/2016GB005586
Kana, T. M., Darkangelo, C., Hunt, M. D., Oldham, J. B., Bennett, G. E., and Cornwell, J. C. (1994). Membrane Inlet mass spectrometer for rapid high-precision determination of N2, O2, and Ar in environmental water samples. Anal. Chem. 66, 4166–4170. doi: 10.1021/ac00095a009
Lorenzen, C. J. (1966). A method for the continuous measurement of in vivo chlorophyll concentration. Deep Sea Res. 13, 223–227. doi: 10.1016/0011-7471(66)91102-8
Luo, Y.-W., Doney, S. C., Anderson, L. A., Benavides, M., Berman-Frank, I., Bode, A., et al. (2012). Database of diazotrophs in global ocean: abundance, biomass and nitrogen fixation rates. Earth Syst. Sci. Data 4, 47–73. doi: 10.5194/essd-4-47-2012
Messer, L. F., Brown, M. V., Furnas, M. J., Carney, R. L., McKinnon, A. D., and Seymour, J. R. (2017). Diversity and activity of diazotrophs in great barrier reef surface waters. Front. Microbiol. 8:967. doi: 10.3389/fmicb.2017.00967
Messer, L. F., Mahaffey, C., Robinson, C. M., Jeffries, T. C., Baker, K. G., Isaksson, J. B., et al. (2015). High levels of heterogeneity in diazotroph diversity and activity within a putative hotspot for marine nitrogen fixation. ISME J. 10, 1499–1513. doi: 10.1038/ismej.2015.205
Mills, M. M., Turk-Kubo, K. A., van Dijken, G. L., Henke, B. A., Harding, K., Wilson, S. T., et al. (2020). Unusual marine cyanobacteria/haptophyte symbiosis relies on N2 fixation even in N-rich environments. ISME J. 14, 2395–2406. doi: 10.1038/s41396-020-0691-6
Mohr, W., Großkopf, T., Wallace, D. W. R., and LaRoche, J. (2010). Methodological underestimation of oceanic nitrogen fixation rates. PLoS One 5:e12583. doi: 10.1371/journal.pone.0012583
Moisander, P. H., Beinart, R. A., Hewson, I., White, A. E., Johnson, K. S., and Carlson, C. A. (2010). Unicellular cyanobacterial distributions broaden the oceanic N2 fixation domain. Science 327, 1512–1514. doi: 10.1126/science.1185468
Moisander, P. H., Beinart, R. A., Voss, M., and Zehr, J. P. (2008). Diversity and abundance of diazotrophic microorganisms in the South China Sea during intermonsoon. ISME J. 2, 954–967.
Montoya, J. P., Holl, C., Zehr, J. P., Hansen, A., Villareal, T., and Capone, D. G. (2004). High rates of N2 fixation by unicellular diazotrophs in the oligotrophic Pacific Ocean. Nature 430, 1027–1032. doi: 10.1038/nature02744.1
Montoya, J. P., Voss, M., Kahler, P., and Capone, D. G. (1996). A Simple, High-precision, high-sensitivity tracer assay for N2 fixation. Appl. Environ. Microbiol. 62, 986–993. doi: 10.1128/aem.62.3.986-993.1996
Moutin, T., Wagener, T., Caffin, M., Fumenia, A., Gimenez, A., Baklouti, M., et al. (2018). Nutrient availability and the ultimate control of the biological carbon pump in the western tropical South Pacific Ocean. Biogeosciences 15, 2961–2989. doi: 10.5194/bg-15-2961-2018
Mulholland, M. R., Bernhardt, P. W., Blanco-Garcia, J. L., Mannino, A., Hyde, K., Mondragon, E., et al. (2012). Rates of dinitrogen fixation and the abundance of diazotrophs in North American coastal waters between Cape Hatteras and Georges Bank. Limnol. Oceanogr. 57, 1067–1083. doi: 10.4319/lo.2012.57.4.1067
Mulholland, M. R., Bernhardt, P. W., Widner, B. N., Selden, C. R., Chappell, P. D., Clayton, S., et al. (2019). High rates of N2 fixation in temperate, Western North Atlantic coastal waters expand the realm of marine Diazotrophy. Glob. Biogeochem. Cycles 33, 826–840. doi: 10.1029/2018GB006130
Mullin, J. B., and Riley, J. P. (1955). The colorimetric determination of silicate with special reference to sea and natural waters. Anal. Chim. Acta 12, 162–176. doi: 10.1016/S0003-2670(00)87825-3
Neveux, J., Tenório, M. M. B., Jacquet, S., Torréton, J.-P., Douillet, P., Ouillon, S., et al. (2009). Chlorophylls and phycoerythrins as markers of environmental forcings including Cyclone Erica Effect (March 2003) on phytoplankton in the southwest lagoon of New Caledonia and oceanic adjacent area. Int. J. Oceanogr. 2009:232513. doi: 10.1155/2009/232513
Ouillon, S., Douillet, P., Lefebvre, J. P., Le Gendre, R., Jouon, A., Bonneton, P., et al. (2010). Circulation and suspended sediment transport in a coral reef lagoon: the south-west lagoon of New Caledonia. Mar. Pollut. Bull. 61, 269–296. doi: 10.1016/j.marpolbul.2010.06.023
Raimbault, P., and Garcia, N. (2008). Evidence for efficient regenerated production and dinitrogen fixation in nitrogen-deficient waters of the South Pacific Ocean: impact on new and export production estimates. Biogeosciences 5, 323–338. doi: 10.5194/bg-5-323-2008
Rodier, M., and Le Borgne, R. (2008). Population dynamics and environmental conditions affecting Trichodesmium spp. (filamentous cyanobacteria) blooms in the south – west lagoon of New Caledonia. J. Exp. Mar. Biol. Ecol. 358, 20–32. doi: 10.1016/j.jembe.2008.01.016
Rougerie, F. (1986). Le Lagon Sud-Ouest de la Nouvelle-Calédonie : Spécificité Hydrologique et Productivité. Nouméa: ORSTOM, 231.
Sargent, E. C., Hitchcock, A., Johansson, S. A., Langlois, R., Moore, C. M., LaRoche, J., et al. (2016). Evidence for polyploidy in the globally important diazotroph Trichodesmium. FEMS Microbiol. Lett. 363:fnw244. doi: 10.1093/femsle/fnw244
Stal, L. J. (2009). Is the distribution of nitrogen-fixing cyanobacteria in the oceans related to temperature? Environ. Microbiol. 11, 1632–1645. doi: 10.1111/j.1758-2229.2009.00016.x
Stenegren, M., Caputo, A., Berg, C., Bonnet, S., and Foster, R. A. (2018). Distribution and drivers of symbiotic and free-living diazotrophic cyanobacteria in the western tropical South Pacific. Biogeosciences 15, 1559–1578. doi: 10.5194/bg-15-1559-2018
Subramaniam, A., Yager, P. L., Carpenter, E. J., Mahaffey, C., Bjorkman, K., Cooley, S., et al. (2008). Amazon River enhances diazotrophy and carbon sequestration in the tropical North Atlantic Ocean. Proc. Natl. Acad. Sci. U.S.A. 105, 1460–1465. doi: 10.1029/2006GB002751
Tang, W., and Cassar, N. (2019). Data-driven modeling of the distribution of diazotrophs in the global ocean. Geophys. Res. Lett. 46, 12258–12269. doi: 10.1029/2019GL084376
Tenório, M., Dupouy, C., Rodier, M., and Neveux, J. (2018). Trichodesmium and other filamentous Cyanobacteria in New Caledonian waters (South West Tropical Pacific) during an El Niño Episode. Aquat. Microb. Ecol. 81, 219–241. doi: 10.3354/ame01873
Tripp, H. J., Bench, S. R., Turk, K. A., Foster, R. A., Desany, B. A., Niazi, F., et al. (2010). Metabolic streamlining in an open-ocean nitrogen-fixing cyanobacterium. Nature 464, 90–94. doi: 10.1038/nature08786
Turk-Kubo, K. A., Frank, I. E., Hogan, M. E., Desnues, A., Bonnet, S., and Zehr, J. P. (2015). Diazotroph community succession during the VAHINE mesocosm experiment (New Caledonia lagoon). Biogeosciences 12, 7435–7452. doi: 10.5194/bg-12-7435-2015
White, A. E., Watkins-Brandt, K. S., and Church, M. J. (2018). Temporal Variability of Trichodesmium spp. and Diatom-Diazotroph Assemblages in the North Pacific Subtropical Gyre. Front. Mar. Sci. 5:27. doi: 10.3389/fmars.2018.00027
Keywords: N2 fixation, diazotrophs, nifH, abundance, New Caledonia
Citation: Saulia E, Benavides M, Henke B, Turk-Kubo K, Cooperguard H, Grosso O, Desnues A, Rodier M, Dupouy C, Riemann L and Bonnet S (2020) Seasonal Shifts in Diazotrophs Players: Patterns Observed Over a Two-Year Time Series in the New Caledonian Lagoon (Western Tropical South Pacific Ocean). Front. Mar. Sci. 7:581755. doi: 10.3389/fmars.2020.581755
Received: 09 July 2020; Accepted: 08 October 2020;
Published: 06 November 2020.
Edited by:
Toshi Nagata, The University of Tokyo, JapanReviewed by:
Arvind Singh, Physical Research Laboratory, IndiaCamila Fernandez, UMR 7621 Laboratoire D’océanographie Microbienne (LOMIC), France
Copyright © 2020 Saulia, Benavides, Henke, Turk-Kubo, Cooperguard, Grosso, Desnues, Rodier, Dupouy, Riemann and Bonnet. This is an open-access article distributed under the terms of the Creative Commons Attribution License (CC BY). The use, distribution or reproduction in other forums is permitted, provided the original author(s) and the copyright owner(s) are credited and that the original publication in this journal is cited, in accordance with accepted academic practice. No use, distribution or reproduction is permitted which does not comply with these terms.
*Correspondence: Sophie Bonnet, c29waGllLmJvbm5ldEBtaW8ub3N1cHl0aGVhcy5mcg==