- 1Department of Biology, “Tor Vergata” University of Rome, Rome, Italy
- 2Department of Integrative Marine Ecology, Anton Dohrn Zoological Station, Naples, Italy
- 3Department of Biology, University of Bari “Aldo Moro,” Bari, Italy
- 4National Inter-University Consortium for Marine Sciences (CoNISMa), Rome, Italy
- 5Institute for Environmental Protection and Research (ISPRA), Rome, Italy
Bryozoan assemblages of three mesophotic habitats, recently found in the Adriatic and Ionian Seas, were investigated in terms of species composition and diversity, colonial growth forms, and species ecological affinity, with the purposes to analyze variations and similarities between the different bioconstructions and to compare the mesophotic assemblages with those of other benthic habitats. Bryozoans came from three sites off the Apulian coast: Monopoli, 30–55-m depth, where scleractinians are dominant; Otranto, 45–64-m depth; and Santa Maria di Leuca, 45–70-m depth, where the bivalve Neopycnodonte cochlear is the main bioconstructor. A total number of 50 species of bryozoans were recorded, accounting for about 10% of the total number of Mediterranean species. Only few species were shared between the three sites, whereas a considerable pool of species was characteristic of each site. The β-diversity and Sörensen similarity analyses highlighted the highest similarity between the two southern sites, Otranto and Santa Maria di Leuca, with respect to the northern site of Monopoli, in agreement with the hydrological pattern of the area. The encrusting zoarial type was the most abundant in each site, and few species with erect, celleporiform and petraliform colonies were found. The Sörensen similarity, based on data of zoarial forms composition, revealed values considerably higher than those based on species composition, thus highlighting the similar ecological role played by the bryozoans in all the sites. Moreover, significant differences between the mesophotic bryozoan assemblages and those of other benthic Mediterranean habitats were highlighted, showing higher affinity with the coralligenous and detritic sciaphilic habitats. The relevant ecological role of bryozoans as binders in the bioconstructions of the Mediterranean mesophotic zone has been exhibited.
Introduction
Exploration of marine biotopes of deep-sea dark and mesophotic zones recently has been made possible, thanks to advancement in marine technologies that allow observations on so far poorly studied benthic ecosystems (Hinderstein et al., 2010; Kahng et al., 2017; Chimienti et al., 2018a). In the Mediterranean Sea, particular attention is currently being paid to aphotic deep-sea habitats, such as seamounts, cold-water coral habitats, and submarine canyons (Mastrototaro et al., 2010; Bo et al., 2011a, 2012a,b, 2015; Cau et al., 2015; D’Onghia et al., 2015; Fanelli et al., 2017; Taviani et al., 2017, 2019; Chimienti et al., 2018b, 2019; Cardone et al., 2019). On the other hand, the mesophotic zone is located in the deeper photic zone, twilight zone, at a depth ranging from 30 to 40 m, which corresponds to the limit of conventional SCUBA diving, to >150 m, where there is sufficient sunlight penetration to support photosynthesis and living zooxanthellate corals (Kahng et al., 2014; Cerrano et al., 2019). In tropical and subtropical regions, mesophotic ecosystems are receiving scientific attention as they may offer refuge to shallow species due to their less altered environmental conditions (e.g., Baldwin et al., 2018; Bo et al., 2019; Sánchez et al., 2019). In the Mediterranean Sea, diversified habitats are hosted in the mesophotic zone. The bioconstructions mainly built by calcareous algae, i.e., coralligenous, have been studied for a long time (Ballesteros, 2006); conversely, knowledge on other mesophotic habitats, where animals are the main bioconstructors, is very scarce. Currently, these latter are attracting increasing attention of scientists since they support high habitat complexity and recruitment of shallow-water and deepwater species and thus enhance local biodiversity (Cerrano et al., 2010; Bianchelli et al., 2013; Cánovas Molina et al., 2016; Idan et al., 2018; Bo et al., 2019; Enrichetti et al., 2019). Most of the studies on the mesophotic habitats off the Italian coast deal with the Ligurian and Tyrrhenian Seas (Bo et al., 2009, 2011a,b; Cerrano et al., 2010) and Southern Adriatic Sea (Corriero et al., 2019; Cardone et al., 2020; Chimienti et al., 2020).
Bryozoans are a major component of marine benthic communities from shallow-water ecosystems to the deep sea and significantly contribute to structure benthic habitats, also in the Mediterranean Sea (Rosso and Di Martino, 2016). They are important bioengineers, thanks to their capability to create three-dimensional structures, which largely increase habitat heterogeneity and enhance biodiversity of both invertebrates and fishes, thus providing diverse ecosystem services (Cocito, 2004). Being modular organisms, bryozoans form colonies with a very large spectrum of morphologies. According to the construction mode, the colony can be rigid as in the case of contiguous frameworks or flexible when articulated junctions or feebly calcified connections exist. The encrusting colonies can cover the substrate with unilaminar or multilaminar layers; in this latter case, the superimposed layers, folding on themselves and incorporating debris, can create massive structures; other colony morphologies may be pedunculate or fungiform (Cocito, 2009). Bryozoans play a great role in bioconstructions where they can act as constructor and binders, particularly in the Mediterranean Sea where they are relevant structuring organisms (Lombardi et al., 2014). Bryozoans are the main former of the coralligenous biogenic structure, where they are present with high abundance and species diversity in association with other bioconstructors, such as coralline algae, scleractinians, sponge (Cocito et al., 2002; Ballesteros, 2006; Rosso and Sanfilippo, 2009; Casoli et al., 2017; Harmelin, 2017). They also are the principal frame builders in other circalittoral priority habitats, such as in the coastal detritic bottom, where they form the facies with luxuriant assemblages of erect and calcified large bryozoans (Bianchi, 2009). They also act as frame builders of conspicuous monospecific bioconstructions, as in the case of the Pentapora fascialis reefs (Ferdeghini and Cocito, 1999; Novosel et al., 2004a; Lombardi et al., 2008) and the Schizoporella errata (Waters, 1878) buildups (Cocito et al., 2000), and as habitat formers in the case of the Cellaria salicornioides meadows (McKinney and Jaklin, 2000). Bryozoans contribute to creating three-dimensional biogenic structures also in deepwater habitats, such as in the cold-water coral communities (Zabala et al., 1993; Mastrototaro et al., 2010; D’Onghia et al., 2015). They are dominant components of the sessile fauna in the semi-dark and dark submarine caves (Rosso et al., 2013a,b, 2018, 2019a; Sanfilippo et al., 2015). However, bryozoans are mainly reported in association with other organisms as secondary frame builders, hidden encrusters, cavity dwellers, and cavity fillers (Cuffey, 1977, 2006; Ferdeghini et al., 2001; Cocito, 2004; Cocito and Lombardi, 2007).
Though many species of bryozoans thrive in dim light conditions, this is the first study on bryozoans of animal-structured mesophotic assemblages. Here, we analyze the bryozoan assemblages found in three bioconstructions located along the Apulian coast in the Southern Adriatic Sea and Ionian Sea to contribute to filling the knowledge gap on the bryozoan fauna in the mesophotic zone. Our aims were: (i) to analyze differences and similarities in terms of species composition among sites dominated by different building organisms; (ii) to analyze the growth form morphologies of the colonies and their distribution in the three sites; (iii) to compare the observed assemblages with those of other Mediterranean habitats; (iv) to investigate the role played by bryozoans in the mesophotic bioconstructions.
Materials and Methods
Study Areas
The three study areas are located along the Southern Apulian coast, at a distance of approximately 78 nautical miles, between Monopoli (MON) and Otranto (OTR), and 23 nautical miles, between OTR and Santa Maria di Leuca (SML). MON study area is located along the southern Adriatic coast, ca 1.5 nautical miles off the city of MON (Figure 1). In this area, the bioconstruction is mainly formed by two non-symbiotic scleractinian species, Phyllangia americana mouchezii (Lacaze Duthiers, 1897) and Polycyathus muellerae (Abel, 1959) (Figure 2A). The bioconstruction develops discontinuously along the coastline for 2.09 km, between 30 and 55 m, reaching up to 2 m in thickness and resulting from superimposed colonies. The structural complexity of the bioconstruction provided a high heterogeneity of microhabitats where a diversified associated fauna occurred. Besides bryozoans, more than 200 species, mostly ascribed to porifera, mollusks, and polychaetes, have been reported (Corriero et al., 2019).
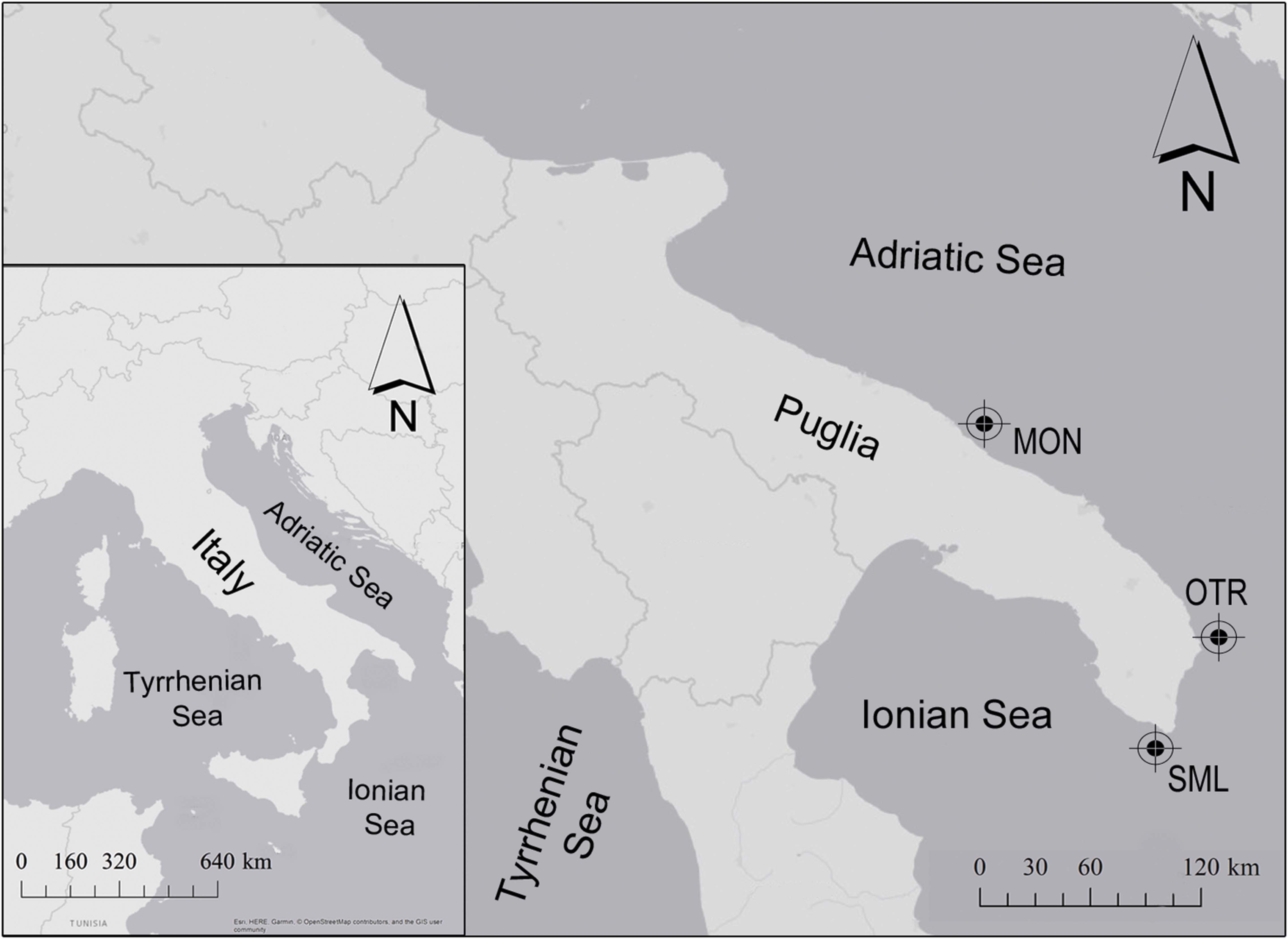
Figure 1. Map of the geographic location of the three mesophotic investigated habitats: Monopoli (MON), Otranto (OTR), and Santa Maria di Leuca (SML).
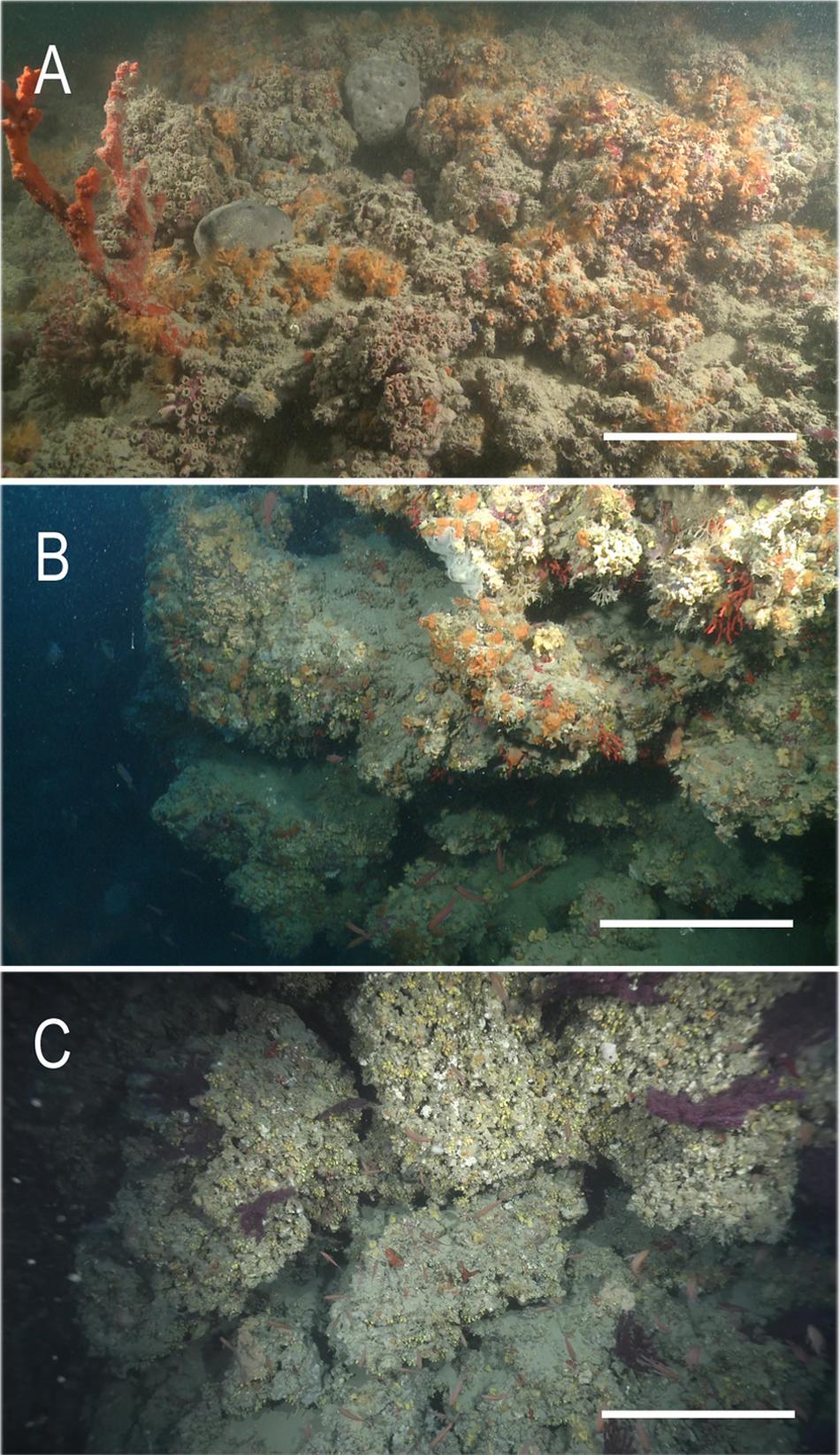
Figure 2. The mesophotic biocostructions of the Apulian coast (Italy): (A) Monopoli, 43 m depth; (B) Otranto, 52 m depth, (C) Santa Maria di Leuca, 60 m depth. Scale bars: (A) 10 cm; (B) 30 cm; (C) 60 cm.
The OTR and SML study areas are located, respectively, off Otranto city in the Southern Adriatic Sea and Santa Maria di Leuca city in the Ionian Sea (Figure 1). Here, the bioconstructions are mainly built by the bivalve Neopycnodonte cochlear (Poli, 1795) (Cardone et al., 2020) and discontinuously develop along 600 m of the coastline within a bathymetric range of 45–64 m at OTR (Figure 2B) and approximately along 450 m of the coastline within a bathymetric range of 45–70 m at SML (Figure 2C). The biogenic formations are mainly formed by aggregations of the Neopycnodonte shells developing in pinnacles and globose structures with thickness of 50 cm at OTR and more than 1.5 m at SML. They are associated with complex and diverse communities accounting for 110 and 136 taxa, respectively, at OTR and at SML (Cardone et al., 2020).
Sampling Methods and Taxonomical Analysis
For the characterization of the bryozoan assemblages associated with the investigated mesophotic bioconstructions, at each study area, three samples were collected by technical divers at a distance of approximately 2 m from each other at a bathymetric range of 45–50 m. Each sample of approximately 3 L in volume was scraped from the bioconstruction using a spatula.
Taxonomic identification of bryozoans and updated nomenclature were carried out according to Zabala and Maluquer (1988); Chimenz Gusso et al. (2014), Rosso and Di Martino (2016), and WORMS1. The surface covered by abundant colonies was measured considering their projection on the sampled substratum area; this latter was photographed underwater with a high-resolution digital camera equipped with laser beams. Cover values were expressed as percentages. Areal cover of small and intertwined colonies was visually evaluated. We assessed the colony abundance for all species and grouped data in three percentage classes of abundance: <10%, 11–50%, >51%.
Colony Morphology and Habitat
The colony morphology of each species was detected with the purpose of investigating the colonization patterns of bryozoans. The colonial shapes (zoarial growth forms) are very different, ranging from flat encrusting to erect rigid with foliaceous and arborescent shape to erect flexible with jointed stems, and they reflect the bryozoan adaptation to diverse substrates and varying environmental conditions. Thus, the zoarial growth form (zoarial type) is informative of the environmental characteristics and of the strategy fulfilled by each species. In this study, the growth form showed by the colonies was observed, and the zoarial types were identified for each species. The frequency of the zoarial types is also computed as the sum of occurrence of all species belonging to each type.
For comparison between the analyzed bryozoan assemblages and those of other benthic habitats, a list was made out. The list combined all the species found in our study with the species reported in Ballesteros (2006); Rosso and Sanfilippo (2009), Harmelin (2017) (coralligenous), Rosso (1996); Madurell et al. (2013), Rosso et al. (2014) (detritic, shelf sciaphilic habitats), Di Martino and Taylor (2014) (Posidonia meadow), Novosel et al. (2004b); Catra et al. (2019), Rosso et al. (2019b) (shallow photophilic shelf habitats), Rosso et al. (2013a, b), Rosso et al. (2019a) (semi-dark submarine caves), Mastrototaro et al. (2010), and D’Onghia et al. (2015) (cold-water coral habitats, outer shelf–upper slope deep habitats), most of which were extracted from the revision by Rosso and Di Martino (2016) on Mediterranean bryozoan diversity. The selected species accounted for 45–65% of the total number of species of each habitat according to Rosso and Di Martino (2016). Thus, the species subsets represented a substantial portion of bryozoan assemblage of each habitat, which is helpful as sound information source and for reducing redundancy. The whole dataset, including 222 species, was provided in Supplementary Table 1. The ecological affinity was estimated for each species combining personal information and available literature data from Rosso (1996), Novosel et al. (2004b), Rosso and Sanfilippo (2009), Mastrototaro et al. (2010), Rosso et al. (2013a,b), Di Martino and Taylor (2014), D’Onghia et al. (2015), Rosso and Di Martino (2016), Harmelin (2017), Rosso et al. (2018), Catra et al. (2019), and Rosso et al. (2019a,b).
Data Analysis
The number of species for each study area was computed as a measure of α-diversity; in addition, the β-diversity was calculated as a measure of species turnover along the geographical North–South gradient using the Whittaker Index βw = (S/) – 1, S being the total number of species that results from merging the number of all sites and the average number of species per individual sample (Whittaker, 1972; Koleff et al., 2003). Such measures were computed to evaluate the proportion by which the three study areas differ from each other in species composition. Bryozoan data were analyzed by means of ordination technique non-metric multidimensional scaling (nMDS) on the basis of Bray–Curtis dis/similarity measure. The hierarchical clustering routine for Q-mode based on the unweighted pair-group average algorithm (Clarke and Warwick, 2001) was also used to find groupings of sites/habitats on the basis of their similarity in species composition. Significance non-parametric test for differences between sites/habitats was performed by means of analysis of similarities (ANOSIM) based on Bray–Curtis similarity measure (Clarke, 1993). The Similarity Percentage (SIMPER) test (Clarke and Warwick, 2001) was performed in order to evaluate which species are primarily responsible for differences between groups of sites/habitats. All the data analyses were carried out by means of the software Past3.
Results
Species and Zoarial Types Composition
A total of 50 species of Bryozoa were found and included in Table 1, along with their zoarial forms, ecological affinity, and percentage of cover. Most species (n = 46) belong to Gymnolaemata Cheilostomatida, and only four species account for Stenolaemata Cyclostomatida. The heterogeneity among sampling sites was verified by ANOSIM test that showed significant differences between the three mesophotic bryozoan assemblages in terms of species composition, with the global R statistic = 1, the mean rank within groups = 5, and the mean rank between groups = 23 (p same = 0.003).
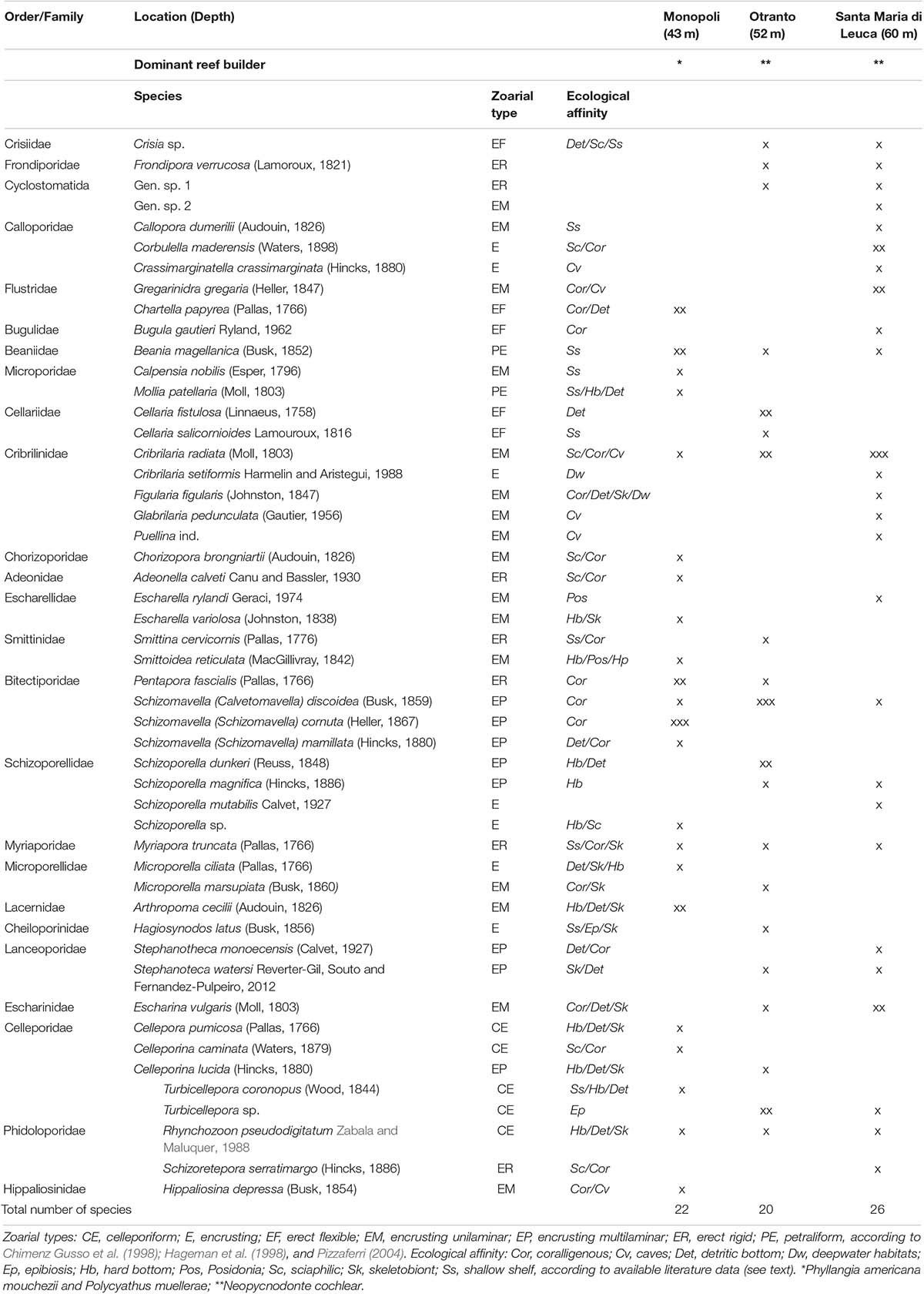
Table 1. List of the bryozoan species and their percentage coverage (x = < 10%, xx = 10%–50%, xxx = > 50%) in each site.
At MON, 22 species were identified that belong to the order Cheilostomatida. Most of them have been found to form encrusting unilaminar colonies, e.g., Arthropoma cecilii, Calpensia nobilis, Hippaliosina depressa, and Schizoporella mamillata, and the most abundant species, Schizomavella spp. and Schizomavella cornuta, showed multilaminar colonies. Only few species with erect colonies occurred, such as Chartella papyrea, Bugula gautieri (flexible) and Myriapora truncata, P. fascialis (rigid), this latter being abundant along the outer edge particularly at a shallower depth. Few petraliform, e.g., Beania magellanica and Mollia patellaria, and celleporiform species, e.g., Cellepora pumicosa and Rhynchozoon pseudodigitatum, were also found particularly in the central area of the entire surface covered with bioconstructions, together with other small-sized thin encrusting colonies that mostly colonized fissures and interstices.
At OTR, 17 Cheilostomatid and three Cyclostomatid species occurred (Table 1). Most taxa exhibited encrusting multilayered colonies, e.g., Schizomavella discoidea, Schizoporella dunkeri, and S. magnifica, and thin colonies, e.g., Escharina vulgaris, Microporella marsupiata, and Cribrilaria radiata; other species showed erect forms of growth, such as the most abundant flexible Cellaria fistulosa and the rigid Smittina cervicornis, M. truncata, and P. fascialis. The unilaminar encrusting large-sized colonies of Cribrilaria showed a high percentage of cover on the surface of Neopycnodonte valvae and hosted as epibionts many specimens of tube-dwelling spirorbid polychaetes and other small-sized colonies of bryozoans; also, the multilayered colonies of S. discoidea formed a thick layer near the edges of the oysters. In this site, few petraliform, B. magellanica, and celleporiform, Celleporina lucida, R. pseudodigitatum, and Turbicellepora sp., species occurred. Colonies of such species extensively encrusted the Neopycnodonte valvae and also partly filled spaces between specimens, while the erect rigid bryozoans largely occurred along the outer edge of the bioconstruction.
Twenty-two Cheilostomatida and four Cyclostomatida occurred in SML (Table 1). Most species, such as Cellepora dumerilii, Cribrilaria setiformis, Schizoporella mutabilis, and C. radiata, had encrusting colonies. Particularly, this latter species, together with E. vulgaris, adhered on the Neopycnodonte valvae, reaching a high percent cover. Such encrusting species hosted other bryozoan colonies and spirorbid polychaetes in epibiosis. Few species were erect (Schizoretepora serratimargo, M. truncata, rigid, and B. gautieri, flexible) and celleporiform (R. pseudodigitatum and Turbicellepora sp.).
The total number of species for each zoarial type in the three sampling sites is shown in Figure 3. The encrusting type is the most abundant in each site, but different encrusting species accounted for the assemblages of the three sites: only two species, C. radiata and S. discoidea, commonly occurred in the three sites. Further two species, E. vulgaris and S. magnifica, are shared by OTR and SML. Few species with erect-rigid colonies, such as M. truncata and P. fascialis, occurred in two–three sites, contrarily to the erect-flexible bryozoans, which characterized a single site: e.g., C. papyrea in MON, Cellaria fistulosa and C. salicornioides in OTR, and B. gautieri in SML. Only the celleporiform R. pseudodigitatum and the petraliform B. magellanica were found in all three sites.
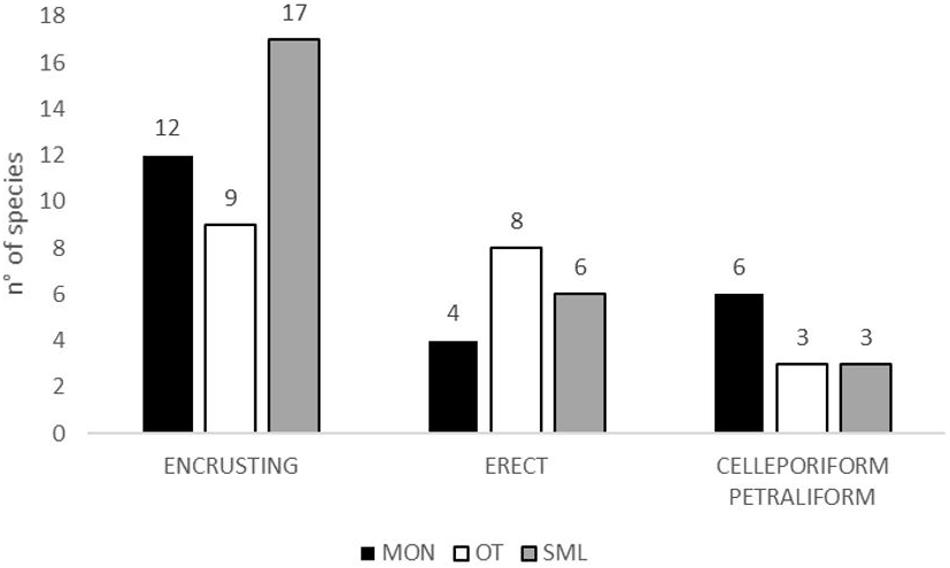
Figure 3. Number of species for zoarial types for each site; Monopoli = black; Otranto = white; Santa Maria di Leuca = gray.
The bryozoan α-diversity was similar in the three examined sites and ranged from 20 (OTR) to 26 taxa (SML) (Table 1). Conversely, the β-diversity varied from the highest values, 0.71 and 0.79, computed between MON–OTR and MON–SML, respectively, to the lowest value, 0.48, found between OTR and SML (Table 2).
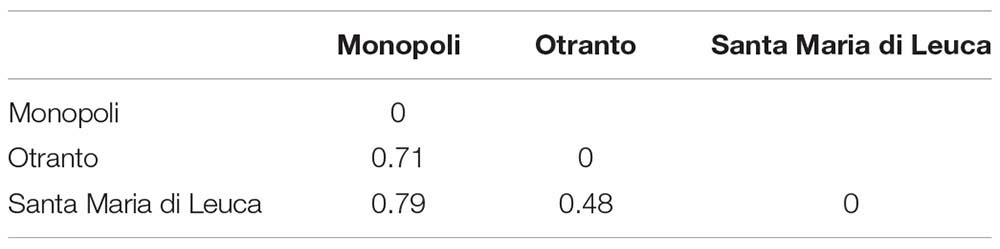
Table 2. Paired values of β-diversity determined using Whittaker’s βw measure at the three mesophotic sites investigated.
Bryozoan Assemblages From Mesophotic and Other Benthic Habitats
The bryozoan assemblages of the three investigated areas partially differed in species composition (Table 1). OTR and SML accounted for the highest Sörensen similarity (0.32), while MON–OTR showed the lowest similarity levels (0.18) and MON–SML intermediate values (0.22) (Table 3).
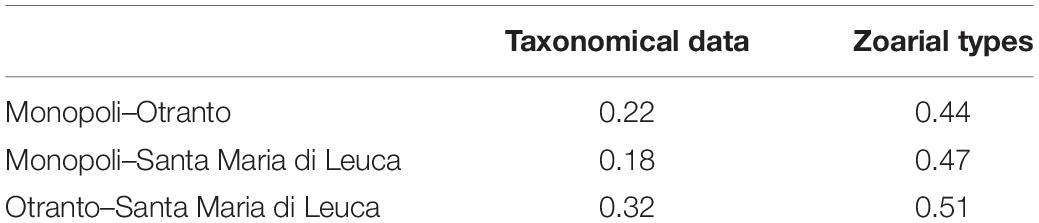
Table 3. Pairwise similarity calculated using Sörensen index based on the presence/absence of taxonomical data and zoarial types data for each pair of sites.
The results of the pairwise similarity regarding the zoarial types (Table 3) showed higher levels in comparison with those computed on taxonomical data: OTR and SML showed the highest similarity (0.51), MON–OTR the lowest (0.44), and MON–SML intermediate values (0.47).
The MDS plot (Figure 4A) revealed the bryozoan assemblages from MON, OTR, and SML to be clearly separated from those of other Mediterranean habitats; the shallowest assemblages, such as those of Posidonia and the shallow shelf, and the circalittoral assemblages, e.g., coralligenous and detritic bottom, were respectively grouped in two clusters; the assemblages of the white corals and semi-dark caves were separated points. The cluster analysis (Figure 4B) results were coherent with those of the nMDS ordination, showing four main clusters, embracing respectively the mesophotic investigated sites (group 1), the circalittoral habitats (group 2), and the shallowest habitats (group 3), while the other habitats (group 4) were clustered at low levels of similarity. The ANOSIM test confirmed significant differences between the mesophotic groups and the other Mediterranean habitats revealed by the nMDS ordination and the cluster analysis, being the global R statistic = 0.47, the mean rank within groups = 20.83, and the mean rank between groups = 36.31 (p < 0.001).
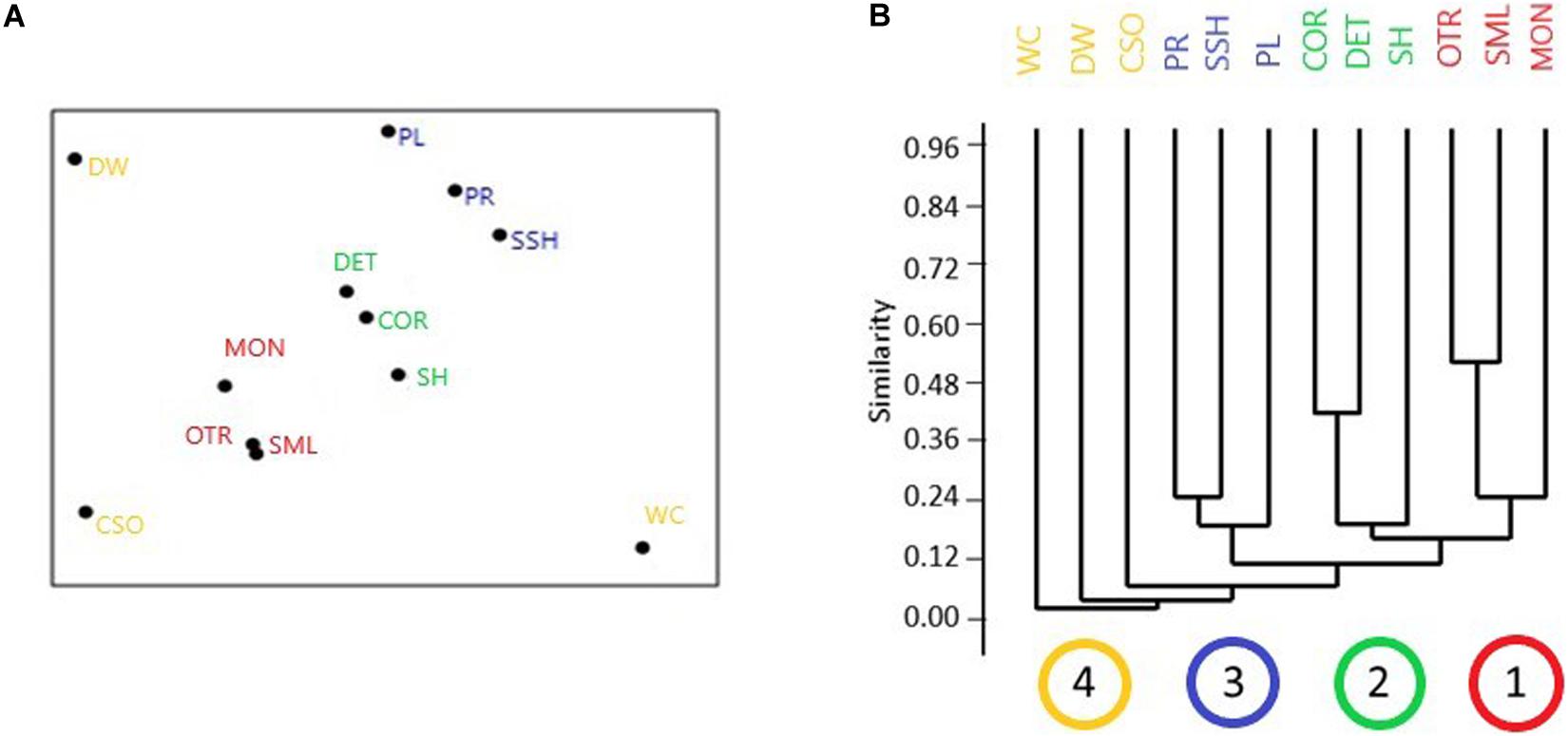
Figure 4. Graphical results of non-metric multidimensional scaling (nMDS) (A) and cluster (B) analysis for the comparison between bryozoan assemblages of the mesophotic sites and those of other benthic habitats. Mesophotic sites: MON, Monopoli; OTR, Otranto; SML, Santa Maria di Leuca. Other benthic habitats considered: COR, coralligenous; CSO, semi-dark submarine caves; DET, detritic bottoms; DW, outer shelf–upper slope deep habitats; PL, Posidonia meadow leaves level; PR, Posidonia meadow rhyzomes level; SH, shelf sciaphilic habitats; SSH, shallow shelf photophilic habitats; WC, white coral banks. In the abscissa axis of panel (B), the four main clusters are shown: brown = the mesophotic investigated sites (MON, OTR, SML); green = the circalittoral and sciaphilic habitats (DET, COR, SH); blue = the shallowest and photophilic habitats (PL, PR, SSH); yellow = other habitats (CSO, DW, WC) (nMDS stress 0.11).
The SIMPER analysis identified a number of species ranging from 82 (group 1 vs. 2) to 64 (group 1 vs. 3) and to 47 (group 1 vs. 4) out of a total of 222 taxa considered for the comparison between mesophotic and the other habitats; such numbers of species were responsible for the 70% cumulative contribution of the distinction between the habitats (Table 4). Fourteen species discriminating group 1 vs. 2 accounted for 1% average contribution and principally included R. pseudodigitatum, S. discoidea, Aetea truncata, and Scrupocellaria delilii; the top discriminating species between group 1 vs. 3 on a total of 28 species that accounted for 1% average contribution were M. truncata, B. magellanica, Scupocellaria bertolleti, C. radiata, R. pseudodigitatum, and S. discoidea; finally, the discrimination of group 1 vs. 4 mostly depended on this latter species together with P. fascialis.
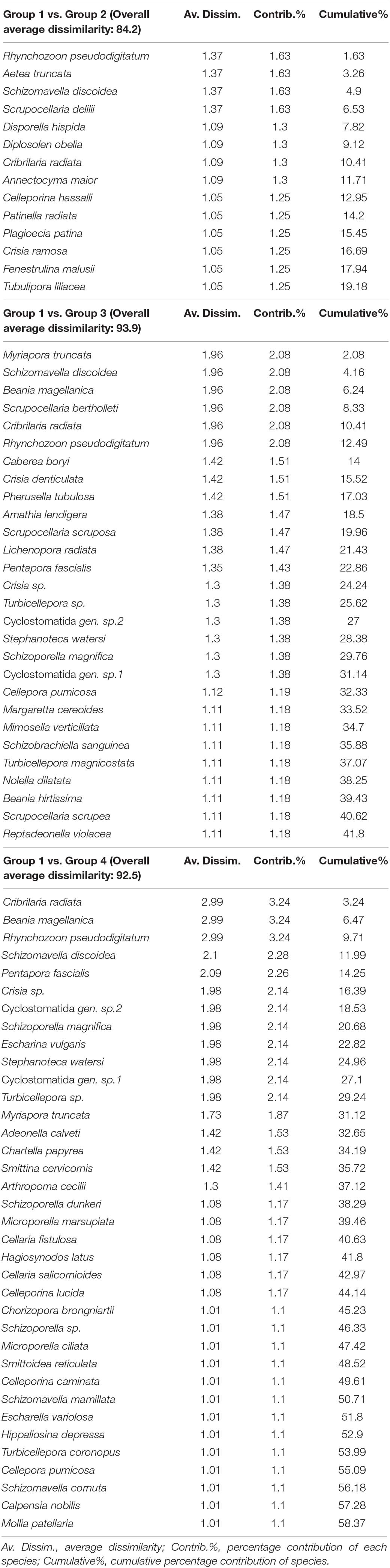
Table 4. List of the top discriminating species with contribution of more than 1% by SIMPER analysis for the four main clusters extrapolated by the cluster analysis and confirmed by the analysis of similarities (ANOSIM) test.
Discussion
This study supports the hypothesis that the mesophotic habitats investigated off the Apulian coast represent suitable environments for bryozoans, since they revealed a high level of α-diversity accounting to approximately 10% of the total number of species of bryozoans reported for the Mediterranean Sea (Rosso and Di Martino, 2016). The taxonomical analysis of the bryozoan fauna highlighted a considerable pool of species, which was characteristic for each site. Most of them are encrusting, such as A. cecilii, Microporella ciliata, and S. cornuta at MON, C. lucida, S. dunkeri, and Hagyosynodus latus at OTR, and C. dumerilii, Cribrilaria setiformis, and Stephanoteca monoecensis at SML. Our results shed light on the distinct contribution of bryozoans to the taxonomical diversity and the ecological role in the mesophotic assemblages investigated. Indeed, they showed a high rate of exclusivity among sites in terms of species composition; on the contrary, the colony morphologies were only partially responsible for the differentiation among the bryozoan assemblages. The Sörensen similarity among sites based on zoarial forms was considerably higher than the similarity computed on species composition. Therefore, we argued that in such mesophotic bioconstructions, the bryozoans play a similar role, since their encrusting colonies mainly play the role of binders, rather than the role of primary engineers. In other bioconstructions, in fact, the bryozoans are reported to form thin and thick encrusting zoaria, which largely coat the bioconstruction surfaces, forming bridge structures and offering their own colonies to host other organisms in epibiosis (Rosso and Sanfilippo, 2009; Wood et al., 2012; Lombardi et al., 2014). Such issues explain the rich and complex colonization pattern of the investigated bioconstructions and are in agreement with other studies that highlighted the similar capabilities of bryozoans (Cocito, 2009; Rosso and Sanfilippo, 2009).
Our analysis on mesophotic bryozoan assemblages of the Apulian coast also reflected the notable species turnover rate between sites, which varied along the North–South geographical gradient: the Whittaker’s β-diversity varied among the mesophotic sites where the highest values were found between the northern site, MON, and the southern sites, SML and OTR (0.79 and 0.71 respectively), whereas the lowest value (0.48) was computed between the two southern sites, OTR and SML. We attributed the observed differences to the substitution of species, as, e.g., it occurred within the genera Schizoporella and Schizomavella. In agreement with such results, the highest Sörensen similarity (0.32) came out between the two southern sites with respect to the similarity measured between the northern and the two southern sites (0.22 and 0.18, respectively, for OTR and SML).
Our results pinpoint the marked heterogeneity of the bryozoan assemblages that emerged as peculiarity of the investigated mesophotic bioconstructions. Concerning the causes of the observed patterns, we hypothesize that distinctions in bryozoan biodiversity are mainly related to stochastic and biological factors; they both were notable in driving the colonization patterns of benthic assemblages. The first factors were principally interrelated to the hydrographic characteristics of the area, where the surface currents flow southeastward along the Adriatic Italian coast according the hydrological cyclonic pattern (Damiani et al., 1988; Russo and Artegiani, 1996; Poulain, 2001; Zavatarelli et al., 2002; Zavatarelli and Pinardi, 2003). So, such currents can transport larvae and propagules, responsible for different connectivity degrees among sites. Indeed, the bryozoan assemblages of the southern close sites, OTR and SML, resulted in more connections to each other in terms of species composition with respect to the assemblage of the northern site of MON, which showed clear taxonomic differences. Relevant aspects of the population connectivity, due to processes occurring in the water column, in the studies of benthic community dynamics were stressed by Giangrande et al. (2017), and coherently with our results, similar conclusions have been recently highlighted for other Mediterranean habitats, such as coralligenous, hydrothermal areas, and brackish waters (Cardone et al., 2014; Longo et al., 2018; Donnarumma et al., 2019; Gravina et al., 2020). On the other hand, we are aware of the well-known role of biological factors in benthic community dynamics, which are mainly linked to the species dispersal capability and their life history traits; but here, we mainly intend to emphasize the role of the different primary bioconstructor species. In the different sites, they created heterogeneous substrates, which were colonized by different species. At MON, the bioconstruction was edified by scleractinians Phallangia americana mouchezii and Polycyathus muellerae, whose corallites were covered by the large encrusting colonies of S. cornuta and S. mamillata. Here, at the basal portion of the bioconstruction, large erected colonies of P. fascialis, up to 40 cm in height, replace the scleractinians and act as additional bioconstructors (Corriero et al., 2019). Differently to MON, at OTR and SML, the valvae of the engineer species N. cochlear were extensively colonized by numerous exclusive species, e.g., H. latus, M. marsupiata, Figularia figularis, and Glabrilaria pedunculata, whose encrusting colonies covered the basal substratum of the bioconstruction and became epibionts on Serpulids tubes and other bryozoan colonies. In light of the above, the bryozoan assemblages were far to be monotonous, even though they occurred within a restricted geographical area.
The comparison with other Mediterranean habitats highlighted the strong affinity between the mesophotic bryozoan fauna and that of the sciaphilic hard and sedimentary substrata. Indeed, the bioconstructions here investigated are in poor light availability conditions, which are shared with the coralligenous and coastal detritic. Therefore, contrary to differences in the primary bioconstructors (calcareous algae vs. animals), both coralligenous and mesophotic habitats share a similar complex substrata, where the presence of heterogeneous surfaces rich in interstices and crevices offer a wide range of microhabitats suitable for the bryozoans (Harmelin, 1985; Ferdeghini et al., 2001; Ballesteros, 2006; Casas Güell et al., 2016). In particular, in the investigated mesophotic habitats, the highest number of bryozoan species is shared with the coralligenous (40% of species) and the detritic bottoms (30% of species); intermediate numbers of species are shared with hard bottoms and both shallow shelf and sciaphilic habitats (25–12% of species); finally, a small number of species (<10%) shows ecological affinity for other habitats, such as Posidonia meadow and deepwater coral banks. This is in accordance with the distribution of bryozoan biodiversity among the bryozoan-rich habitats at largest geographical scale reported by Rosso and Di Martino (2016) for the Mediterranean Sea and by Novosel (2005) for the Adriatic Sea. These authors highlighted the rough and uneven hard surfaces and the coarse biogenic detritic substrata to be particularly suitable for bryozoans due to availability of a wide range of microhabitats. The analysis of SIMPER confirmed the pattern described, exhibiting the species that mostly contributed to the similarity between mesophotic and coralligenous and detritic habitats: they were S. discoidea, R. pseudodigitatum, S. delilii, and A. truncata, frequently recorded in coralligenous and detritic bottoms (Rosso and Sanfilippo, 2005; Cocito et al., 2012; Rosso et al., 2013a,b; Harmelin, 2017). Other sciaphilous species usually associated with coralligenous communities, and sciaphilic shelf habitats mostly discriminated the mesophotic assemblages from shallow shelf habitats and Posidonia, such as M. truncata, S. discoidea, B. magellanica, C. radiata, and R. pseudodigitatum. Moreover, the same species listed above mostly contributed to dissimilarity between the mesophotic and deep habitats.
In conclusion, our study highlighted for the first time similarities and distinctions between three mesophotic bryozoan assemblages and their comparison with further benthic habitats. The twofold approach of our analysis, based on taxonomy and colony morphologies, consistently contributed in shedding light on the biodiversity of bryozoans, showing their relevant ecological role mainly as binders in different bioconstuctions of the Mediterranean mesophotic zone.
Data Availability Statement
The raw data supporting the conclusions of this article will be made available by the authors, without undue reservation.
Author Contributions
JG: conceptualization, data curation, investigation, taxononomical and formal analysis, methodology, writing – original draft, and writing – review and editing. FC: conceptualization, data curation, investigation, field methodology, formal analysis, and writing – review and editing. GC: investigation and writing – review and editing. MG: conceptualization, data curation, investigation, formal analysis, and writing – review and editing. LN: supervision, conceptualization, data curation, investigation, taxonomical and formal analysis, writing – original draft, and writing – review and editing. All authors contributed to the article and approved the submitted version.
Funding
This study was partially funded by CoNISMa (National Inter-University Consortium for Marine Sciences) under the monitoring program for the implementation of the Marine Strategy Framework Directive (art. 11 D. Lgs. 190/2010), Modulo 7 – Habitat Coralligenous, according to the convention between Italian Ministry of Environment, Land and Sea Protection (MATTM) and the Environmental Protection Agency of Apulia (ARPA Puglia) with CoNISMa.
Conflict of Interest
The authors declare that the research was conducted in the absence of any commercial or financial relationships that could be construed as a potential conflict of interest.
Supplementary Material
The Supplementary Material for this article can be found online at: https://www.frontiersin.org/articles/10.3389/fmars.2020.581292/full#supplementary-material
Footnotes
References
Baldwin, C. C., Tornabene, L., and Robertson, D. R. (2018). Below the mesophotic. Sci. Rep. 8:4920. doi: 10.1038/s41598-018-23067-1
Ballesteros, E. (2006). Mediterranean coralligenous assemblages: a synthesis of present knowledge. Oceanogr. Mar. Biol. Ann. Rev. 44, 123–195. doi: 10.1201/9781420006391.ch4
Bianchelli, S., Pusceddu, A., Canese, S., Greco, S., and Danovaro, R. (2013). High meiofaunal and nematodes diversity around mesophotic coral oases in the Mediterranean Sea. PLoS One 8:e66553. doi: 10.1371/journal.pone.0066553
Bianchi, C. N. (2009). “Facies a grandi Briozoi,” in Gli Habitat Prioritari Del Protocollo SPA/BIO (Convenzione di Barcellona) Presenti in Italia, eds G. Relini and G. Giaccone (Genova, IT: Erredi Grafiche Editoriali), 209–212.
Bo, M., Bavestrello, G., Angiolillo, M., Calcagnile, L., Canese, S., Cannas, R., et al. (2015). Persistence of pristine deep-sea coral gardens in the Mediterranean Sea (SW Sardinia). PLoS One 10:e0119393. doi: 10.1371/journal.pone.0119393
Bo, M., Bavestrello, G., Canese, S., Giusti, M., Angiolillo, M., Cerrano, C., et al. (2011a). Coral assemblage off the calabrian coast (South Italy) with new observations on living colonies of Antipathes dichotoma. It. J. Zool. 78, 231–242. doi: 10.1080/11250001003652619
Bo, M., Bertolino, M., Borghini, M., Castellano, M., Covazzi Harriague, A., Di Camillo, C. G., et al. (2011b). Characteristics of the mesophotic megabenthic assemblages of the vercelli seamount (North Tyrrhenian Sea). PLoS One 6:e16357. doi: 10.1371/journal.pone.0016357
Bo, M., Bavestrello, G., Canese, S., Giusti, M., Salvati, E., Angiolillo, M., et al. (2009). Characteristics of a black coral meadow in the twilight zone of the central Mediterranean Sea. Mar. Ecol. Prog. Ser. 397, 53–61. doi: 10.3354/meps08185
Bo, M., Bertolino, M., Bavestrello, G., Canese, S., Giusti, M., Angiolillo, M., et al. (2012a). Role of deep sponge ground. Hydrobiologia 687, 163–177.
Bo, M., Canese, S., Spaggiari, C., Pusceddu, A., Bertolino, M., Angiolillo, M., et al. (2012b). Deep coral oases in the South Tyrrhenian Sea. PLoS One 7:e49870. doi: 10.1371/journal.pone.0049870
Bo, M., Montgomery, A. D., Opresko, D. M., Wagner, D., and Bavestrello, G. (2019). “Antipatharians of the mesophotic zone: four case studies,” in Mesophotic Coral Ecosystems, eds Y. Loya, K. A. Puglise, and T. C. L. Bridge (New York, NY: Springer International Publishing), 683–708. doi: 10.1007/978-3-319-92735-0_37
Cánovas Molina, A., Montefalcone, M., Bavestrello, G., Cau, A., Bianchi, C. N., Morri, C., et al. (2016). A new ecological index for the status of mesophotic megabenthic assemblages in the Mediterranean based on ROV photography and video footage. Cont. Shelf Res. 121, 13–20. doi: 10.1016/j.csr.2016.01.008
Cardone, F., Corriero, G., Fianchini, A., Gravina, M. F., and Nonnis Marzano, C. (2014). Biodiversity of transitional waters: species composition and comparative analysis of hard bottom communities from the south-eastern Italian coast. J. Mar. Biol. Assoc. 94, 25–34. doi: 10.1017/s0025315413001306
Cardone, F., Corriero, G., Longo, C., Mercurio, M., Tarantini, S. O., Gravina, M. F., et al. (2020). Massive bioconstructions built by neopycnodonte cochlear (Mollusca, Bivalvia) in a mesophotic environment in the central Mediterranean Sea. Sci. Rep. 10:6337.
Cardone, F., Pansini, M., Corriero, G., and Bertolino, M. (2019). Two new species of deep-sea sponges (Porifera) from submarine canyons of the sardinian channel (Central Mediterranean Sea). Zootaxa 4688, 407–419. doi: 10.11646/zootaxa.4688.3.7
Casas Güell, E., Cebrian, E., Garrabou, J., Ledoux, J. B., Linares, C., and Teixidó, N. (2016). Structure and biodiversity of coralligenous assemblages dominated by the precious red coral corallium rubrum over broad spatial scales. Sci. Rep. 6:36535.
Casoli, E., Ventura, D., Cutroneo, L., Capello, M., Jona Lasinio, G., Rinaldi, R., et al. (2017). Assessment of the impact of salvaging the costa concordia wreck on the deep coralligenous habitats. Ecol. Indic. 80, 124–134. doi: 10.1016/j.ecolind.2017.04.058
Catra, M., Alongi, G., Leonardi, R., Negri, M. P., Sanfilippo, R., Sciuto, F., et al. (2019). Degradation of a photophilic algal community and its associated fauna from eastern Sicily (Mediterranean Sea). Mediterr. Mar. Sci. 20, 74–89.
Cau, A., Follesa, M. C., Moccia, D., Alvito, A., Bo, M., Angiolillo, M., et al. (2015). Deepwater corals biodiversity along roche du large ecosystems with different habitat complexity along the south Sardinia continental margin (CW Mediterranean Sea). Mar. Biol. 162, 1865–1878. doi: 10.1007/s00227-015-2718-5
Cerrano, C., Bastari, A., Calcinai, B., Di Camillo, C., Pica, D., Puce, S., et al. (2019). Temperate mesophotic ecosystems: gaps and perspectives of an emerging conservation challenge for the Mediterranean Sea. Eur. Zool. J. 86, 370–388. doi: 10.1080/24750263.2019.1677790
Cerrano, C., Danovaro, R., Gambi, C., Pusceddu, A., Riva, A., and Schiaparelli, S. (2010). Gold coral (Savalia savaglia) and gorgonian forests enhance benthic biodiversity and ecosystem functioning in the mesophotic zone. Biodivers. Conserv. 19, 153–167. doi: 10.1007/s10531-009-9712-5
Chimenz Gusso, C., Gravina, M. F., and Nicoletti, L. (1998). Diversità delle strategie vitali dei briozoi infralitorali mediterranei. Biol. Mar. Mediterr. 5, 205–213.
Chimenz Gusso, C., Nicoletti, L., and Bondanese, C. (2014). Briozoi. Biol. Mar. Mediterr. 21, 1–336.
Chimienti, G., Angeletti, L., Rizzo, L., Tursi, A., and Mastrototaro, F. (2018a). ROV vs trawling approaches in the study of benthic communities: the case of pennatula rubra (Cnidaria: Pennatulacea). J. Mar. Biol. Assoc. 98, 1859–1869. doi: 10.1017/s0025315418000851
Chimienti, G., Bo, M., and Mastrototaro, F. (2018b). Know the distribution to assess the changes: mediterranean cold-water coral bioconstructions. Rend. Lincei sci. Fis. Nat. 29, 583–588. doi: 10.1007/s12210-018-0718-3
Chimienti, G., Bo, M., Taviani, M., and Mastrototaro, F. (2019). “Occurrence and biogeography of mediterranean cold-water corals,” in Mediterranean Cold-Water Corals: Past, Present and Future, eds C. Orejas and C. Jiménez (Berlin, DE: Springer International Publishing), 213–243. doi: 10.1007/978-3-319-91608-8_19
Chimienti, G., De Padova, D., Mossa, M., and Mastrototaro, F. (2020). A mesophotic black coral forest in the Adriatic Sea. Sci. Rep. 10:8504.
Clarke, K. R. (1993). Non-parametric multivariate analyses of changes in community structure. Austral Ecol. 18, 117–143. doi: 10.1111/j.1442-9993.1993.tb00438.x
Clarke, K. R., and Warwick, R. M. (2001). A further biodiversity index applicable to species lists: variation in taxonomic distinctness. Mar. Ecol. Prog. Ser. 216, 265–278. doi: 10.3354/meps216265
Cocito, S. (2004). Bioconstruction and biodiversity: their mutual influence. Sci. Mar. 68, 137–144. doi: 10.3989/scimar.2004.68s1137
Cocito, S., Bedulli, D., and Sgorbini, S. (2002). Distribution patterns of the sublittoral epibenthic assemblages on a rocky shoal in the Ligurian Sea (NW Mediterranean). Sci. Mar. 66, 175–118. doi: 10.3989/scimar.2002.66n2175
Cocito, S., Ferdeghini, F., Morri, C., and Bianchi, C. N. (2000). Patterns of bioconstruction in the cheilostome bryozoan Schizoporella errata: the influence of hydrodynamics and associated biota. Mar. Ecol. Prog. Ser. 192, 153–161. doi: 10.3354/meps192153
Cocito, S., and Lombardi, C. (2007). Competitive interactions in the coralligenous assemblages of SM Leuca (Ionian Sea). Biol. Mar. Mediterr. 14, 176–177.
Cocito, S., Lombardi, C., Ciuffardi, F., and Gambi, M. C. (2012). Colonization of Bryozoa on seagrass Posidonia oceanica “mimics”: biodiversity and recruitment pattern over time. Mar. Biodivers. 42, 189–201. doi: 10.1007/s12526-011-0104-1
Corriero, G., Pierri, C., Mercurio, M., Nonnis Marzano, C., Tarantini, S. O., Gravina, M. F., et al. (2019). A Mediterranean mesophotic coral reef built by non-symbiotic scleractinians. Sci. Rep. 9:3601.
Cuffey, R. J. (1977). Bryozoan contributions to reefs and bioherms through geologic time. AAPG stud. Geol. 4, 181–194. doi: 10.1306/st4393c14
Cuffey, R. J. (2006). Bryozoan-built reef mounds - the overview from integrating recent studies with previous Investigation. Cour. Forschungsinst. Senckenberg 257, 35–48.
Damiani, V., Bianchi, C. N., Ferretti, O., Bedulli, D., Morri, C., Viel, M., et al. (1988). Risultati di una ricerca ecologica sul sistema marino costiero pugliese. Thalassia salent. 18, 153–169.
Di Martino, E., and Taylor, P. D. (2014). A brief review of seagrass-associated bryozoans, recent and fossil. Sud. Trent. Sci. Nat. 94, 79–94.
D’Onghia, G., Capezzuto, F., Cardone, F., Carlucci, R., Carluccio, A., Chimienti, G., et al. (2015). Macro-and megafauna recorded in the submarine bari canyon (southern Adriatic, Mediterranean Sea) using different tools. Mediterr. Mar. Sci. 16, 180–196. doi: 10.12681/mms.1082
Donnarumma, L., Appolloni, L., Chianese, E., Bruno, R., Baldrighi, E., Guglielmo, R., et al. (2019). Environmental and benthic community patterns of the shallow hydrothermal area of Secca delle Fumose (Baia, Naples, Italy). Front. Mar. Sci. 6:685.
Enrichetti, F., Bo, M., Morri, C., Montefalcone, M., Toma, M., Bavestrello, G., et al. (2019). Assessing the environmental status of temperate mesophotic reefs: a new, integrated methodological approach. Ecol. Indic. 102, 218–229. doi: 10.1016/j.ecolind.2019.02.028
Fanelli, E., Delbono, I., Ivaldi, R., Pratellesi, M., Cocito, S., and Peirano, A. (2017). Cold-water coral Madrepora oculata in the eastern Ligurian Sea (NW Mediterranean): historical and recent findings. Aquat. Conserv. 27, 965–975. doi: 10.1002/aqc.2751
Ferdeghini, F., and Cocito, S. (1999). Biologically generated diversity in two bryozoan buildups. Biol. Mar. Mediterr. 6, 191–197. doi: 10.1127/zdgg/2016/0071
Ferdeghini, F., Cocito, S., Azzaro, L., Sgorbini, S., and Cinelli, F. (2001). Bryozoan biocostructions in the coralligenous formations of SM Leuca (Apulia, Italy). Biol. Mar. Mediterr. 8, 238–245.
Giangrande, A., Gambi, M. C., and Gravina, M. F. (2017). Paradigm shifts in community ecology: open versus closed units, challenges and limits of connectivity studies. Mar. Ecol. 38:e12480. doi: 10.1111/maec.12480
Gravina, M. F., Cabiddu, S., Como, S., Floris, A., Padedda, B. M., Pusceddu, A., et al. (2020). Disentangling heterogeneity and commonalities in nanotidal Mediterranean lagoons through environmental features and macrozoobenthic assemblages. Estuar. Coast. Mar. Sci. 237:106688. doi: 10.1016/j.ecss.2020.106688
Hageman, S. J., Bock, P. E., Bone, Y., and McGowran, B. (1998). Bryozoan growth habits: classification and analysis. J. Paleontol. 72, 418–436. doi: 10.1017/s0022336000024161
Harmelin, J. G. (1985). “Bryozoan dominated assemblages in Mediterranean cryptic environments,” in Bryozoa: Ordovician to Recent, eds C. Nielsen and G. P. Larwood (Fredensborg, DK: Olsen & Olsen), 135–143.
Harmelin, J. G. (2017). Bryozoan facies in the coralligenous community: two assemblages with contrasting features at port-cros archipelago (Port-Cros National Park, France, Mediterranean). Sci. Rep. Port Cros Natl. Park 31, 105–123.
Hinderstein, L. M., Marr, J. C. A., Martinez, F. A., Dowgiallo, M. J., Puglise, K. A., Pyle, R. L., et al. (2010). Theme section on “Mesophotic coral ecosystems: characterization, ecology, and management”. Coral Reefs 29, 247–251. doi: 10.1007/s00338-010-0614-5
Idan, T., Shefer, S., Feldstein, T., Yahel, R., Huchon, D., and Ilan, M. (2018). Shedding light on an East-Mediterranean mesophotic sponge ground community and the regional sponge fauna. Mediterr. Mar. Sci. 19, 84–106. doi: 10.12681/mms.13853
Kahng, S., Copus, J. M., and Wagner, D. (2017). “Mesophotic coral ecosystems,” in Marine Animal Forests, eds S. Rossi, L. Bramanti, A. Gori, and C. Orejas (New York, NY: Springer International Publishing), 855–886.
Kahng, S. E., Copus, J. M., and Wagner, D. (2014). Recent advances in the ecology of mesophotic coral ecosystems (MCEs). Curr. Opin. Env. Sust. 7, 72–81. doi: 10.1016/j.cosust.2013.11.019
Koleff, P., Gaston, K. J., and Lennon, J. J. (2003). Measuring beta diversity for presence-absence data. J. Anim. Ecol. 72, 367–382. doi: 10.1046/j.1365-2656.2003.00710.x
Lombardi, C., Cocito, S., Occhipinti Ambrogi, A., and Porter, J. S. (2008). Distribution and morphological variation of colonies of the bryozoan Pentapora fascialis (Bryozoa: Cheilostomata) along the western coast of Italy. J. Mar. Biol. Assoc. 88, 711–717. doi: 10.1017/s0025315408001525
Lombardi, C., Taylor, P. D., and Cocito, S. (2014). “Bryozoan constructions in a changing Mediterranean Sea,” in The Mediterranean Sea, eds S. Goffredo and Z. Dubinsky (Dordrecht, NL: Springer International Publishing), 373–384. doi: 10.1007/978-94-007-6704-1_21
Longo, C., Cardone, F., Pierri, C., Mercurio, M., Mucciolo, S., Marzano, C. N., et al. (2018). Sponges associated with coralligenous formations along the Apulian coasts. Mar. Biodivers. 48, 2151–2163. doi: 10.1007/s12526-017-0744-x
Madurell, T., Zabala, M., Dominguez-Carrió, C., and Gili, J. M. (2013). Bryozoan faunal composition and community structure from the continental shelf off Cap de Creus (Northwestern Mediterranean). J. Sea Res. 83, 123–136. doi: 10.1016/j.seares.2013.04.013
Mastrototaro, F., D’Onghia, G., Corriero, G., Matarrese, A., Maiorano, P., Panetta, P., et al. (2010). Biodiversity of the white coral bank off cape santa maria di leuca (Mediterranean Sea): an update. Deep-Sea Res. Part II 57, 412–430. doi: 10.1016/j.dsr2.2009.08.021
McKinney, F. K., and Jaklin, A. (2000). Spatial niche partitioning in the cellaria meadow epibiont association, northern Adriatic Sea. Cah. Biol. Mar. 41, 1–18.
Novosel, M., Olujic, G., Cocito, S., and Pozar Domac, A. (2004a). “Submarine freshwater springs in the Adriatic Sea: a unique habitat for the bryozoan Pentapora fascialis,” in Bryozoan Studies 2004, eds H. Moyano, J. Cancino, and P. Wise Jackson (Lisse, NL: A. A. Balkema Publishers), 215–222. doi: 10.1201/9780203970799.ch21
Novosel, M., Požar-Domac, A., and Pasarić, M. (2004b). Diversity and distribution of the Bryozoa along underwater cliffs in the Adriatic Sea with special reference to thermal regime. Mar. Ecol. 25, 155–170. doi: 10.1111/j.1439-0485.2004.00022.x
Pizzaferri, C. (2004). Briozoi ciclostomi e cheilostomi. forme zoariali e loro abitudini ambientali. Parva Naturalia 6, 3–69.
Poulain, P. M. (2001). Adriatic Sea surface circulation as derived from drifter data between 1990 and 1999. J. Marine Syst. 29, 3–32. doi: 10.1016/s0924-7963(01)00007-0
Rosso, A. (1996). Valutazione della biodiversità in mediterraneo: l’esempio dei popolamenti a briozoi della biocenosi del detritico costiero. Biol. Mar. Mediterr. 3, 58–65.
Rosso, A., and Di Martino, E. (2016). Bryozoan diversity in the Mediterranean Sea: an update. Mediterr. Mar. Sci. 17, 567–607. doi: 10.12681/mms.1706
Rosso, A., Di Martino, E., Pica, D., Galanti, L., Cerrano, C., and Novosel, M. (2018). Non-indigenous bryozoan species from natural and artificial substrata of Mediterranean submarine caves. Mar. Biodivers. 48, 1345–1355. doi: 10.1007/s12526-016-0602-2
Rosso, A., Di Martino, E., Sanfilippo, R., and Di Martino, V. (2013a). “Bryozoan communities and thanatocoenoses from submarine caves in the Plemmirio marine protected area (SE Sicily),” in Bryozoan Studies 2010, eds A. Ernst, P. Schäfer, and J. Scholz (Berlin: Springer International Publishing), 251–269. doi: 10.1007/978-3-642-16411-8_17
Rosso, A., Sanfilippo, R., Taddei Ruggieri, E., and Di Martino, E. (2013b). Faunas and ecological groups of serpuloidea, bryozoa and brachiopoda from submarine caves in sicily (Mediterranean Sea). Boll. Soc. Paleontol. Ital. 52, 167–176.
Rosso, A., Gerovasileiou, V., Sanfilippo, R., and Guido, A. (2019a). Bryozoans assemblages from two submarine caves in the Aegean Sea (Eastern Mediterranean). Mar. Biodivers. 49, 707–726. doi: 10.1007/s12526-018-0846-0
Rosso, A., Sanfilippo, R., Sciuto, F., Serio, D., Catra, M., Alongi, G., et al. (2019b). Preliminary information on bryozoans associated with selected infralittoral algae communities from eastern Sicily (Mediterranean). Australas. Palaeontol. Mem. 52:115.
Rosso, A., and Sanfilippo, R. (2005). Bryozoans and serpuloideans in skeletobiont communities from the Pleistocene of Sicily: spatial utilization and competitive interactions. Sezione di Museologia Scientifica e Naturalistica 1, 115–124.
Rosso, A., and Sanfilippo, R. (2009). “The contribution of Bryozoans and serpuloideans to coralligenous concretions from SE sicily,” in Proceedings of the 1st Mediterranean Symposium on the Conservation of the Coralligenous and other Calcareous Bio-Concretions, eds C. Pergent Martini and M. Brichet (Tabarka: RAC/SPA publ.), 123–128.
Rosso, A., Sanfilippo, R., and Sciuto, F. (2014). Open-shelf, soft-bottom bryozoans from the Ciclopi Marine protected area (E Sicily, Mediterranean). Stud. Trent. Sci. Nat. 94, 199–211.
Sánchez, J. A., Gonzalez Zapata, F. L., Dueñas, L. F., Andrade, J., Pico Vargas, A. L., Sarmiento, A., et al. (2019). Corals in the mesophotic zone (40-115 m) at the barrier reef complex from San Andrés Island (Southwestern Caribbean). Front. Mar. Sci. 6:536. doi: 10.3389/fmars.2019.00536
Sanfilippo, R., Rosso, A., Mastrandrea, A., Russo, F., Riding, R., and Taddei Ruggiero, E. (2015). Metazoan/microbial biostalactites from present-day submarine caves in the Mediterranean Sea. Mar. Ecol. 36, 1277–1293. doi: 10.1111/maec.12229
Taviani, M., Angeletti, L., Canese, S., Cannas, R., Cardone, F., Cau, A., et al. (2017). The “sardinian cold-water coral province” in the economy of Mediterranean coral ecosystems. Deep-Sea Research Part II. 145, 61–78. doi: 10.1016/j.dsr2.2015.12.008
Taviani, M., Angeletti, L., Cardone, F., Montagna, P., and Danovaro, R. (2019). A unique and threatened deep water coral-bivalve biotope new to the Mediterranean Sea offshore the Naples megalopolis. Sci. Rep. 9:3411.
Whittaker, R. H. (1972). Evolution and measurement of species diversity. Taxon 21, 213–251. doi: 10.2307/1218190
Wood, A. L., Probert, P. K., Rowden, A. A., and Smith, A. M. (2012). Complex habitat generated by marine bryozoans: a review of its distribution, structure, diversity, threats and conservation. Aquat. Conserv. 22, 547–563. doi: 10.1002/aqc.2236
Zabala, M., and Maluquer, P. (1988). Illustrated keys for the classification of Mediterranean Bryozoa. Ajuntament de Barcellona. Treb. Mus. Zool. 4, 1–294. doi: 10.2989/ajms.2007.29.1.1.66
Zabala, M., Maluquer, P., and Harmelin, J. G. (1993). Epibiotic bryozoans on deep-water seleractinian corals from the Catalonia slope (western Mediterranean, Spain, France). Sci. Mar. 57, 65–78.
Zavatarelli, M., and Pinardi, N. (2003). The Adriatic Sea modelling system: a nested approach. Ann. Geophys. 21, 345–364. doi: 10.5194/angeo-21-345-2003
Keywords: biogenic structures, distribution, colony growth forms, benthic habitats, Apulia, Southern Adriatic Sea, Ionian Sea
Citation: Giampaoletti J, Cardone F, Corriero G, Gravina MF and Nicoletti L (2020) Sharing and Distinction in Biodiversity and Ecological Role of Bryozoans in Mediterranean Mesophotic Bioconstructions. Front. Mar. Sci. 7:581292. doi: 10.3389/fmars.2020.581292
Received: 08 July 2020; Accepted: 09 November 2020;
Published: 08 December 2020.
Edited by:
Stelios Katsanevakis, University of the Aegean, GreeceReviewed by:
Jasmine Ferrario, University of Pavia, ItalyChiara Lombardi, Italian National Agency for New Technologies, Energy and Sustainable Economic Development (ENEA), Italy
Antonietta Rosso, University of Catania, Italy
Copyright © 2020 Giampaoletti, Cardone, Corriero, Gravina and Nicoletti. This is an open-access article distributed under the terms of the Creative Commons Attribution License (CC BY). The use, distribution or reproduction in other forums is permitted, provided the original author(s) and the copyright owner(s) are credited and that the original publication in this journal is cited, in accordance with accepted academic practice. No use, distribution or reproduction is permitted which does not comply with these terms.
*Correspondence: Jacopo Giampaoletti, jacopo.giampaoletti@gmail.com