- 1Monterey Bay Aquarium Research Institute, Moss Landing, CA, United States
- 2MIO UM 110, IRD, CNRS, Aix-Marseille University – Université de Toulon, Marseille, France
- 3Department of Biomolecular Engineering and Bioinformatics, University of California, Santa Cruz, Santa Cruz, CA, United States
One dominant ecological trait in the dimly-lit deep-sea is the ability of organisms to emit bioluminescence. Despite its many ecological roles in deep-sea ecosystems, the presence of inherent bioluminescence in marine sponges has been debated for more than a century. This work reports repeated observations of luminescence from six individuals of an undescribed carnivorous sponge species (Cladorhizidae) sampled near 4,000 m depth off Monterey Bay (CA, United States). These are the first fully documented records of bioluminescence in the phylum Porifera. Videos and photographs of the sponges’ bioluminescence were recorded on board after collection and in vitro bioluminescence assays indicate that the bioluminescence system is a coelenterazine-based luciferase. Coelenterazine luciferin is already described in various organisms such as cnidarians, chaetognaths, copepods, cephalopods, ctenophores, ostracods, and some mysid or decapod shrimps. Based on these observations we discuss new ecological hypotheses of functional traits such as bioluminescence and carnivory in deep sea organisms.
Introduction
The ability to emit light, bioluminescence, is common among organisms living in dark marine habitats. A broad range of organisms have been described as capable of emitting light, from bacteria to jellies and fish. Bioluminescence can be either directly produced by an individual using light-emitting molecules and enzymes or by a symbiont associated with the host. This production of light is a chemical reaction between an enzyme (called a “luciferase”) and a substrate (called a “luciferin”). As an example, the luciferase from the benthic anthozoan sea pansy Renilla (Pennatulacea) is commonly used in biochemical assays. This luciferase will initiate the reaction of coelenterazine (a luciferin) resulting in the emission of light at wavelengths close to blue-green for human eyes. Emission of light is an advantage in predator-prey relationships, and can facilitate mating, prey capture, and predator avoidance in the deep ocean (Haddock et al., 2010). However, its roles are still far from understood for most species, and it can have different functions for benthic or pelagic organisms. Bioluminescence is a major functional trait in the ocean, with three quarters of pelagic macro-organisms described as bioluminescent (Martini and Haddock, 2017), and about a third of epibenthic organisms known to have such capability (Martini et al., 2019). Among them, few clades, such as Porifera or Nemertea have no reliable descriptions of bioluminescent members yet. There are several challenges to assessing bioluminescence: it is challenging to collect organisms in condition to confidently assess luminescence capability; the scientific community working on benthic bioluminescence is small so the bioluminescence capabilities of many taxa remain undescribed; and there is still a lack of instrumentation, routinely deployed, to observe and document bioluminescence in situ.
Among Porifera, the skeleton of demosponges and hexactinellids is generally composed of elongated or intricately shaped spicules of silica. These structures have the properties of natural optical-fiber systems (Cattaneo-Vietti et al., 1996; Aizenberg et al., 2004), but the potential ecological roles of such light waveguides in sponges has been barely investigated. Several dubious accounts of sponge bioluminescence exist in the literature. The first record of autogenous sponge luminescence is a 19th-century mention of light-emitting sponge embryos (Pagenstecher, 1881). Other observations of luminous sponges over the next several decades were ultimately attributed to worms or other invertebrates living in the pores of the sponge tissue (Dahlgren, 1916; Okada, 1925). One record from the 20th century describes light being emitted from a sponge itself (Harvey, 1921), but this has also been considered doubtful by later authors (Herring, 1987). A recent publication claims the discovery of a luminous sponge (Demospongiae, Suberites domuncula) and a luciferase, however, the authors argue that this marine sponge uses a firefly luciferase homolog and firefly luciferin as its luminescence system (Wiens et al., 2010; Wang et al., 2012). Because sponges filter large volumes of water, it is challenging to distinguish between luminescence of the animal itself or light produced by other organisms concentrated within its tissues. Bioluminescence observations in sponges may have been induced by numerous bioluminescent symbiotic, entrained, or captured bacteria living in sponges (Hentschel et al., 2006), or other associated eukaryotes. Given the unclear records of luminescence in sponges, whether or not autogenically luminous sponges exist remains a mystery.
In this work, we aim at describing the first reliable and independent observations of bioluminescence in an undescribed deep-sea sponge. Based on morphological and molecular data we show that this sponge belongs to Cladorhizidae. The observation of bioluminescence emission after stimulation highlights new hypotheses about the feeding strategy of these organisms. This report aims at increasing interest of deep sea ecologists to integrate bioluminescence as a common functional trait and share their observations about bioluminescent benthic organisms.
Materials and Methods
Sample Collection
In three consecutive years, between June, 2017 and July, 2019, MBARI’s ROV Doc Ricketts, was used to collect six specimens of an undescribed poriferan (hereafter named individuals Clado1-Clado6), in the deep Northeast Pacific Ocean (Figure 1A) approximately two hundred kilometers offshore from Big Sur, CA, United States (Table 1 and Supplementary Table S1). All specimens were sampled on the seafloor composed of silt and clay. Other macro-organisms were observed in the surrounding area, including Holothuroidea (Scotoplanes sp.) and benthic ctenophores. These sponges were anchored to the substrate with rhizoids. The sponges were collected using the ROV’s robotic arm to clasp the stem and were dropped into a sampling container dedicated to biological collections. Once the specimens were retrieved from the ROV, they were kept in a dark 4°C room in seawater.
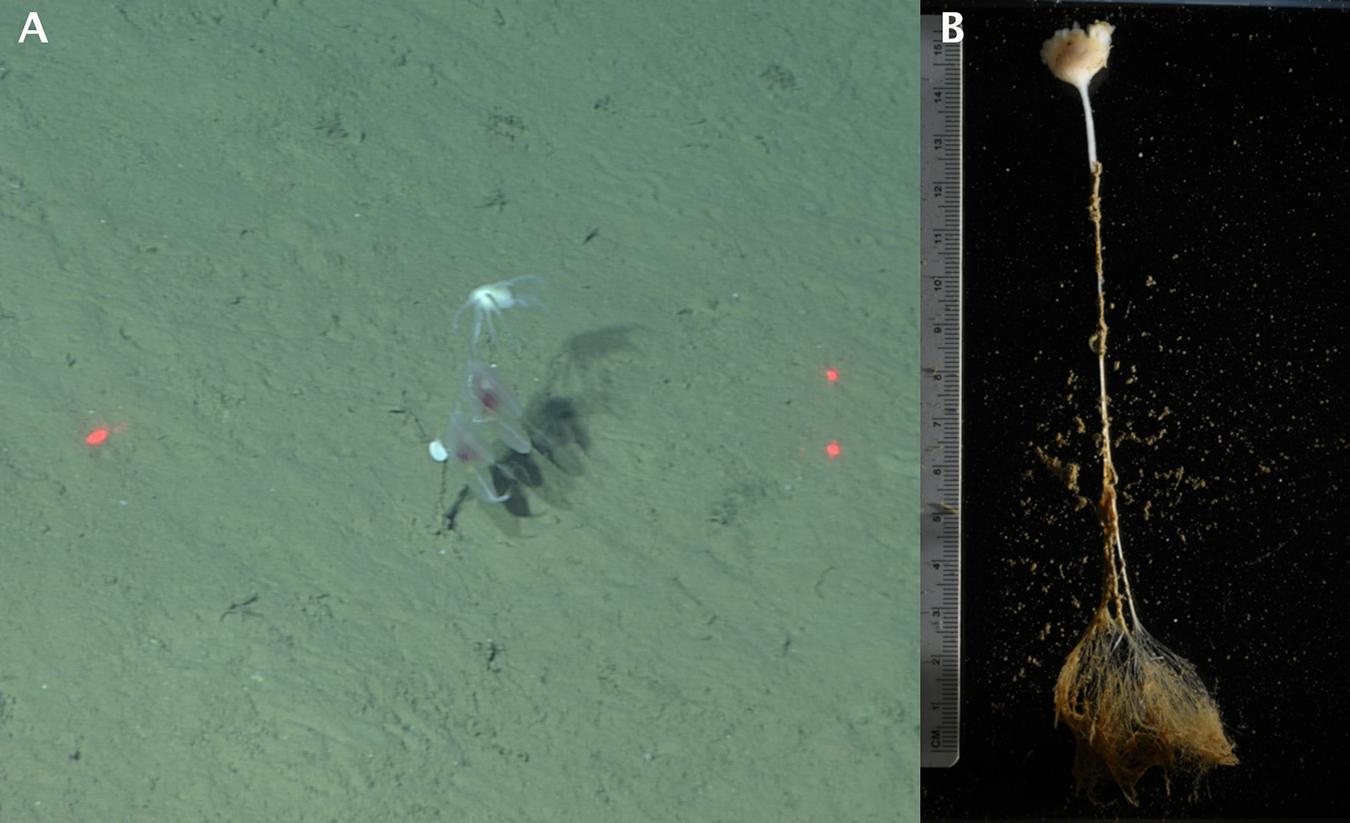
Figure 1. Sampling of the Cladorhizidae sponge and morphological observation. The horizontal distance between the red laser dots is 29 cm. (A) In situ observation of Clado6 by ROV, with two benthic ctenophores attached on the stalk. (B) In the lab observation of Clado6. Filaments are retracted.
Bioluminescence Observations
Remaining sediments were cleaned from the samples (Figure 1B), then the samples were transferred to fresh 4°C seawater and were left to rest for 10 min before being gently stimulated to determine whether they emitted light. To document light emission, images were taken using a Sony α7S camera.
In vitro Bioluminescence Assays
After bioluminescence had been mechanically stimulated, approximately 200 mg portions of Clado3 and Clado5 were cut from the sponge tissue to use for biochemical tests. Each sample was ground in a loose-fitting 1 mL Dounce homogenizer on ice in approximately 750 μL of 20 mM Tris–HCL 750 mM NaCl buffer equilibrated to pH 8.0 at 3°C. The homogenate was centrifuged at 14,000 rcf at 4°C for 10 min on the ship. The supernatant was removed and placed in a new tube. The pellet was resuspended in 500 μL of the Tris–HCl, NaCl buffer described above. Bioluminescence measurements were taken in a custom-built integrating sphere that enables using a micropipettor to inject samples into the measurement chamber while recording. Measurements were made at a sampling frequency of 40 ms (Haddock et al., 2001). The measurement protocol was as follows: (1) place 1–10 μL of buffer, coelenterazine, or Renilla luciferase solution in a clear 1.5 mL microcentrifuge tube inside the integrating sphere, (2) place a micropipettor with 90–190 μL of homogenate or other sample inside the tube in the integrating sphere, but not touching the 1–10 μL of liquid, (3) close the door to the integrating sphere and record the baseline luminescence of the unmixed analytes for 4 s, (4) inject the contents of the micropipettor into the tube to mix, without removing the micropipettor, (5) allow the assay to continue to completion at 20 s total.
Using Clado3, we conducted the following assays. (A) To test for coelenterazine-specific luciferase activity, we added 90 μL of homogenate to 10 μL of 1 μg/mL coelenterazine in Tris/NaCl buffer. We also added 90 μL of homogenate to 10 μL of 0.1 μg/mL coelenterazine. As a negative control we used 10 μL of Tris/NaCl buffer and 90 μL of homogenate. (B) To determine if the sponge homogenate contained coelenterazine we added 98 μL of homogenate to 2 μL of 1.4 μg/mL of Renilla luciferase (RLuc) in the Tris/NaCl buffer described above. We conducted this test twice. As a negative control we used 2 μL of Tris/NaCl buffer and 90 μL of homogenate. (C) We assayed the heat stability of the luciferase by measuring the baseline activity of 60 μL of homogenate and 10 μL of 1 μg/mL coelenterazine in Tris/NaCl buffer. Unused homogenate was incubated in a heat block at 96°C for 3 min, then placed on ice. 60 μL of heat-treated homogenate was mixed with 10 μL of 1 μg/mL coelenterazine in Tris/NaCl buffer. (D) Lastly, we mixed 90 μL of Tris/NaCl buffer with 10 μL of Tris/NaCl buffer as an absolute control, and conducted the same assay with a homogenate preparation from a non-luminous sponge from the genus Caulophacus.
Using Clado5, we prepared homogenate as described above, then performed additional sample clarification to ensure that the observed luminescence was from soluble proteins and not bacteria. The homogenate described above was passed through a 0.45 μm filter spin column (Millipore Sigma Ultrafree-MC) at 12,000 rcf for 10 min at 4°C, then that filtrate was passed through a 0.1 μm filter spin column under the same conditions. This material was used to conduct the following tests. 90 μL of homogenate was mixed with 10 μL of the following compounds to assay for cofactors triggering luminescence: (A) 3% H2O2, (B) 200 mM calcium acetate, (C) 1M CaCl2, (D) 2M KCl, and (E) 3M NaCl. We also used the clarified sample to conduct the following assays: (F) 90 μL homogenate and 10 μL of 1 μg/mL coelenterazine in Tris/NaCl buffer, (G) 90 μL homogenate and 10 μL of Tris/NaCl buffer, (H) 90 μL homogenate and 10 μL of 1.4 μg/mL of Renilla luciferase in Tris/NaCl buffer.
To determine the size of the protein or protein complex responsible for light emission, we concentrated 150 μL of the 0.45 μm- and 0.1 μm-filtered Clado5 homogenate on a 50 kDa spin column (Millipore Sigma Ultrafree-MC) at 12,000 rcf until the sample had 75 μL in the retentate and 75 μL in the filtrate. We assayed for luminescence by mixing (I) 60 μL of the retentate with 10 μL of 1 μg/mL coelenterazine solution, and (J) 60 μL of the filtrate with 10 μL of 1 μg/mL coelenterazine solution. The Clado5 0.45 and 0.1 μm filter-clarified homogenate still emitted light when it was mixed with coelenterazine. The Clado5 clarified homogenate’s light-emitting activity with coelenterazine was concentrated on a 50 kDa spin column, and the 50 kDa spin column filtrate had little light-emitting activity when mixed with coelenterazine.
Morphological Observation
To characterize Clado1, spicules from fragments of each body part were prepared for light microscopy by dissolving tissue in nitric acid rinsed, filtered, and mounted on glass microscope slides using the methods of Reiswig and Browman (1987). Spicules were then observed using a compound microscope fitted with a Lumera Infinity 3 digital camera. The length of microscleres helped diagnose the specimen to genus (Figure 2).
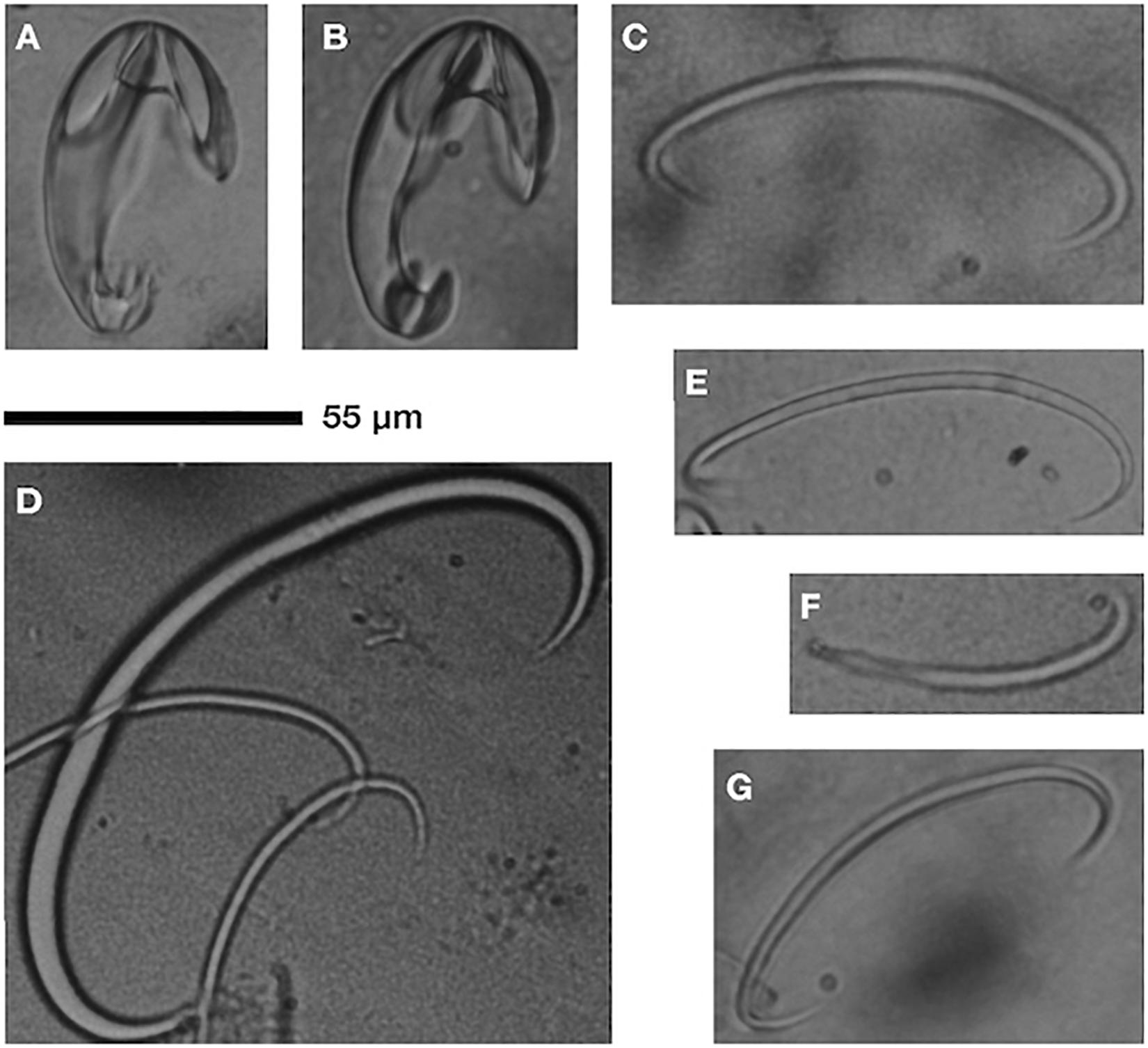
Figure 2. Cladorhiza sp. nov. (undescribed) spicules: unguiferate anisochelae (A,B), C-shaped sigmas (C–E,G), sigmancistra (F); scale is 55 μm. Observations from Clado1.
Sequencing and COI Assembly
The Clado1 sponge was rinsed in filtered seawater, then a subsample was frozen in liquid nitrogen. The sample was later pulverized with a blue plastic pestle in tissue lysis buffer and genomic DNA was isolated with an E.Z.N.A. Mollusc DNA kit (Omega Bio-tek). DNA was sheared with a Bioruptor sonicator and a whole-genome shotgun (WGS) sequencing library was prepared (Meyer and Kircher, 2010). This library, Clado1, was sequenced on a 2 × 75PE MiSeq run in the UC Santa Cruz Paleogenomics laboratory to a depth of approximately two million read pairs.
Reads were mapped to the mitochondrial genome of Negombata magnifica (Belinky et al., 2008) using bwa mem (Li, 2013), both SNPs and indels were corrected using pilon v1.22 (Walker et al., 2014). The corrected assembly was then used again for another round of mapping and correction. This process was iteratively repeated ten times to generate mitochondrial regions containing the sequence from Clado1. The corrected N. magnifica COX1 sequence had two regions to which Clado1 reads mapped. These two regions were used as seeds for two independent runs of MITObim v1.9.1 (Hahn et al., 2013). The sequences from the two MITObim assemblies were aligned into a single contig of approximately 3 kbp. Clado1 reads were aligned to the contig using bwa mem, then SNPs and indels were corrected with pilon. The assembly was verified by visualizing reads mapped to the contig (see Supplementary Figure 1) using IGV (Robinson, 2011) and by visually inspecting a COX1 protein alignment between Clado1 and closely related species (see Supplementary Figure 2). Geneious v11.1.5 was used to identify ORFs in the contig, and blastx was used to determine the identity of the ORFs (Altschul, 1997).
Molecular Identification and Phylogeny
To identify the most similar species to the sample, the complete COX1 ORF in the mitochondrial contig was used as a blastn query against all sponge nucleotide sequences in NCBI.
To generate a COX1 phylogeny we downloaded select Poecilosclerida (NCBI:txid27925) sponge sequences from NCBI, largely based on the literature (Hestetun et al., 2016). Sequences used are visible in Figure 3. The COX1 sequences were aligned using MUSCLE v3.8.425 (Edgar, 2004). A maximum likelihood phylogeny was generated with raxmlHPC-PTHREADS using model GTRGAMMA with the extended majority-rule consensus tree criterion (-# autoMRE) (Stamatakis, 2014) using Guitarra antarctica LN870510 as an outgroup. The analysis completed after 450 bootstrap replicates. A Bayesian phylogeny was generated using MrBayes v3.2.6 using a chain length of 60000, four heated chains, a heated chain temperature of 0.2, subsampling frequency of 200, a burn-in of 2500, a random seed of 2020, and a HKY85 model with gamma rate variation (Huelsenbeck and Ronquist, 2001; Ronquist and Huelsenbeck, 2003).
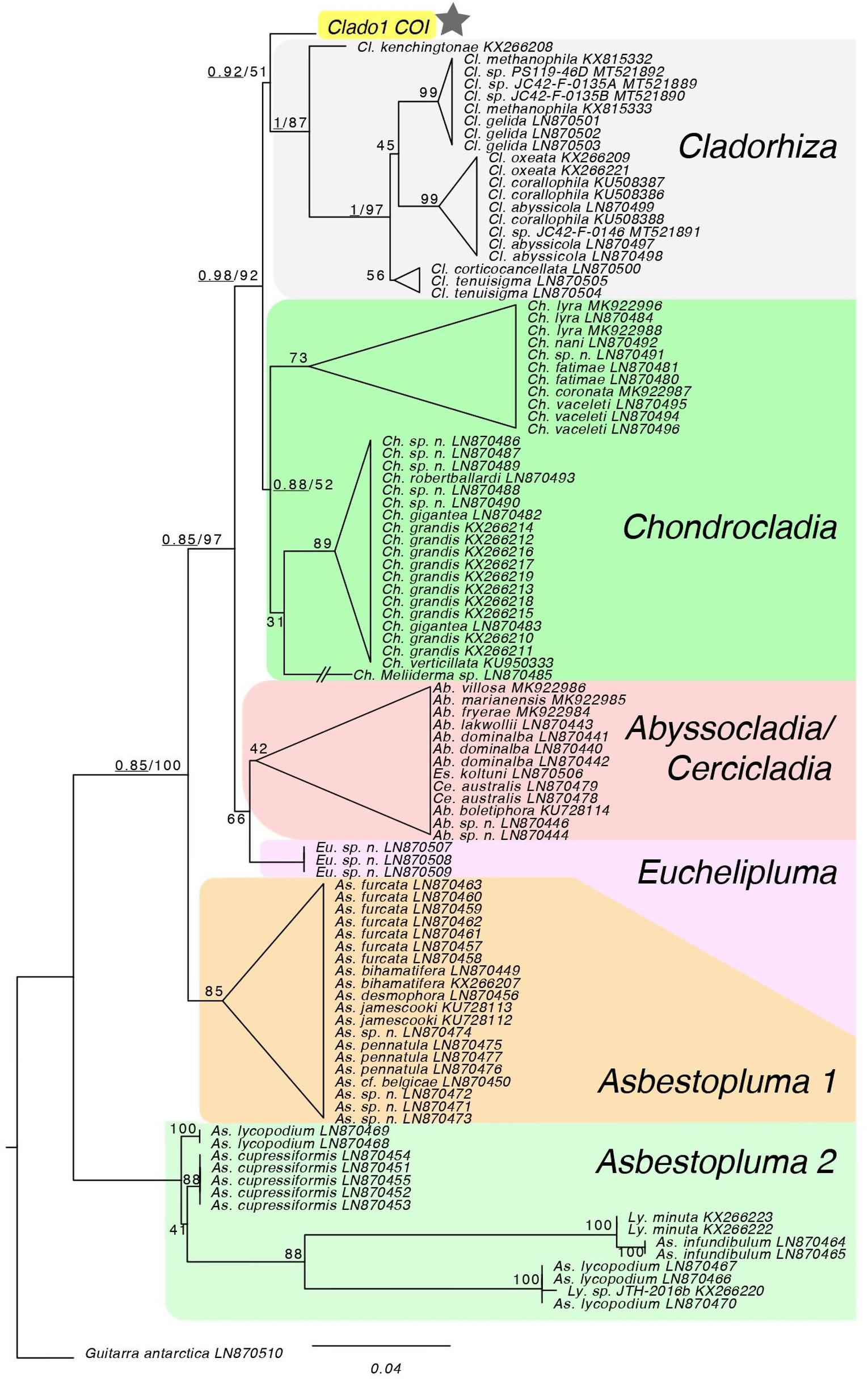
Figure 3. Maximum likelihood analysis of COI locus. The topology of the RAxML tree matches the genus-level topology of the 28S rDNA, COI and ALG11 tree from Hestetun et al. (2016). The COI sequence from Clado1 falls as sister to the Cladorhiza genus, but with low support so it effectively forms a polytomy with Chondrocladia. Bayesian support for major nodes are prepended, underlined, to bootstrap values. * Is the sample of interest.
Metagenomic Analysis
We used bbmerge.sh to merge the Clado1 read pairs (Bushnell et al., 2017). In order to have one query per pair of unmerged reads, the unmerged reads were concatenated together with 40 Ns, and the R2 read for each pair was in the reverse complement orientation. The 2,020,492 merged reads were queried against the NCBI nt database using blastn (Altschul, 1997). MEGAN v6.5.4 was used to perform metagenomic binning of the sequences (Huson et al., 2007). Clado1 reads were mapped against the lux operons of Vibrio, Photobacterium, Aliivibrio, Photorhabdus, and Shewanella using bwa mem. Reads were submitted to NCBI SRA with accession number PRJNA556048.
Results
Bioluminescence Observation
Bioluminescence was tested and observed in all of the six specimens sampled (Table 1). After gently touching each individual sponge with a gloved hand or round-pointed forceps, we observed blue-green bioluminescence localized around the point of mechanical depression (Figure 4, Supplementary Figures 3–6 and Supplementary Movie 1). Composite images of bioluminescence video frame grabs show that the luminescence in the animals occurs in various parts of the animal rather than in a single location (Figures 4B,D and Supplementary Figures 3–6). Local light responses were emitted from the globular mass, filamentous processes and the siliceous stalk, but not from the rhizoid structure. The light kinetics were bright and visible to the naked eye for 5–10 s. Repetitive stimulations were reproducible, and the light did not appreciably dim over time. We attempted to stimulate bioluminescence in Clado1 by flashing a white light at the organism, but it did not produce a visible bioluminescent response. Potassium chloride, calcium chloride, and freshwater did not cause the animal to emit light. Only mechanical stimulation caused the sponge to luminesce.
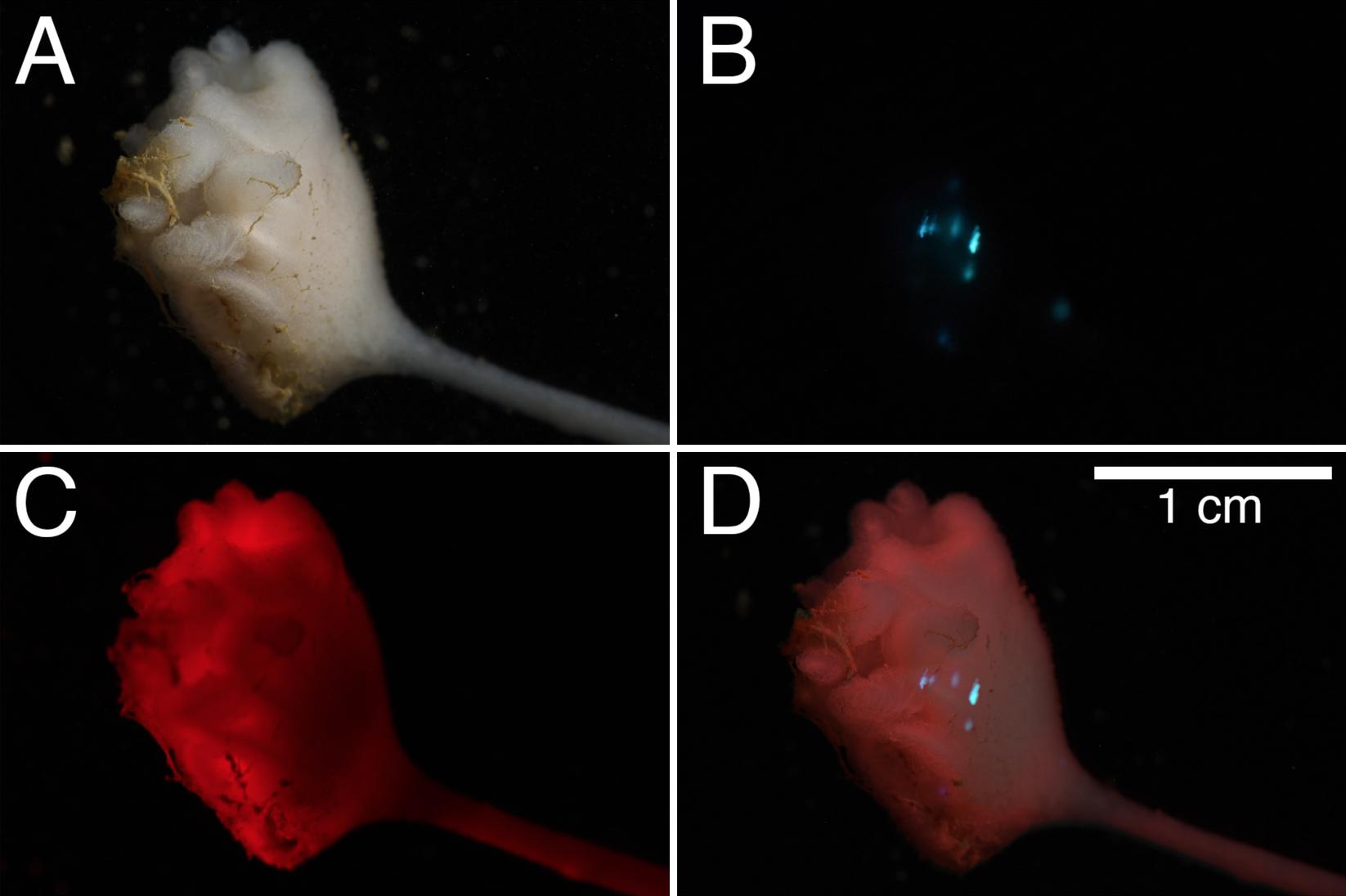
Figure 4. Composite observation of bioluminescence from Clado6. (A) observation of the sponge under white-light, (B) 2.5 s exposure of the bioluminescence after mechanical stimulation, (C) red light observation of the individual, and (D) composite of panels (A–C).
Furthermore, the luminescence did not correspond with patches of residual marine snow or detritus that remain on the animal even after cleaning. We found no visible animals living in or on the sponges that could be responsible for the luminescence.
In vitro Bioluminescence Assays
To verify the presence of a luciferin and luciferase reaction, biochemical tests were performed. The homogenate’s supernatant from both individuals Clado 3 and 5 glowed above background without the addition of any cofactors (Figure 5). Adding homogenate to a coelenterazine-containing solution caused a light-emitting reaction consistent with luciferase-luciferin type reactions from other species (Figures 5, 6). The intensity of the light-emitting reaction was proportional to the quantity of coelenterazine mixed with the Clado3 homogenate. Adding Clado3 and Clado5 homogenate to Renilla luciferase resulted in a light-emitting reaction (Figure 5A). The light-emitting reaction was not triggered in Clado5 homogenate by any of the following compounds: calcium chloride, calcium acetate, hydrogen peroxide, potassium chloride, sodium chloride (Figure 5B). In comparison, homogenized tissue from a non-luminous sponge species did not produce a light-emitting reaction when added to coelenterazine. Mixing non-luminous sponge homogenate and Renilla luciferase also did not produce light (Figure 5B).
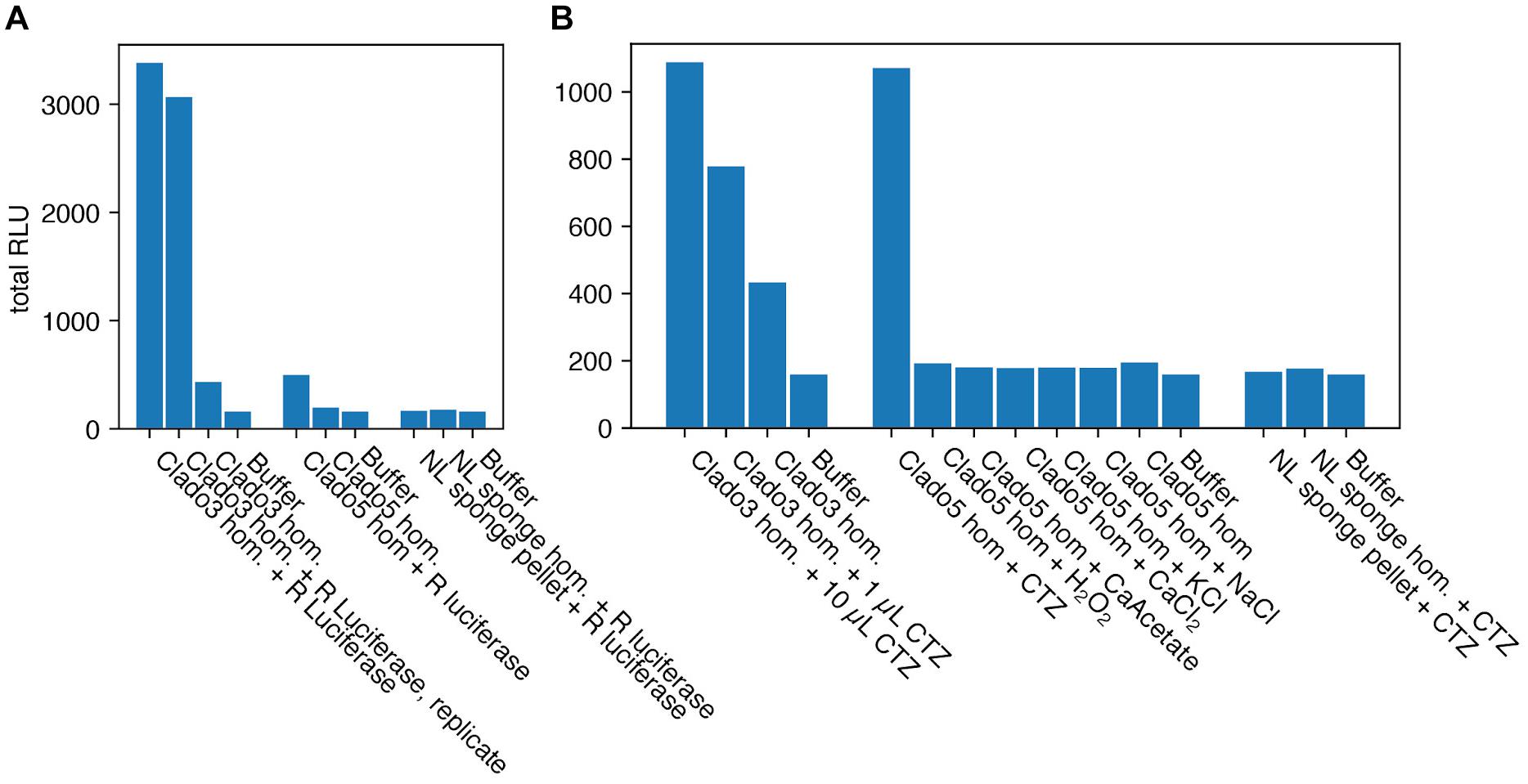
Figure 5. Bioluminescence assays for the Cladorhizid sponges. Relative Light Units (RLU), integrated for 14 s. (A) Renilla luciferase (R luciferase) added to Clado3 and Clado5 homogenate (hom.) resulted in a bioluminescent reaction. Renilla luciferase added to a non-luminous (NL) sponge did not result in a bioluminescent reaction. This suggests that Clado3 and Clado5 contain coelenterazine while the non-luminous sponge does not. (B) Coelenterazine (CTZ) added to Clado3 and Clado5 homogenate resulted in a bioluminescent reaction in a dose-dependent fashion (1 and 10 μL). Compounds that are possible triggers for bioluminescent reactions in photoproteins did not cause a bioluminescent reaction. Coelenterazine added to the non-luminous sponge homogenate did not produce a bioluminescent reaction.
Heating the Clado3 homogenate at 96°C for 3 min reduced the light-emitting activity of the solution by 93% (Figure 6).
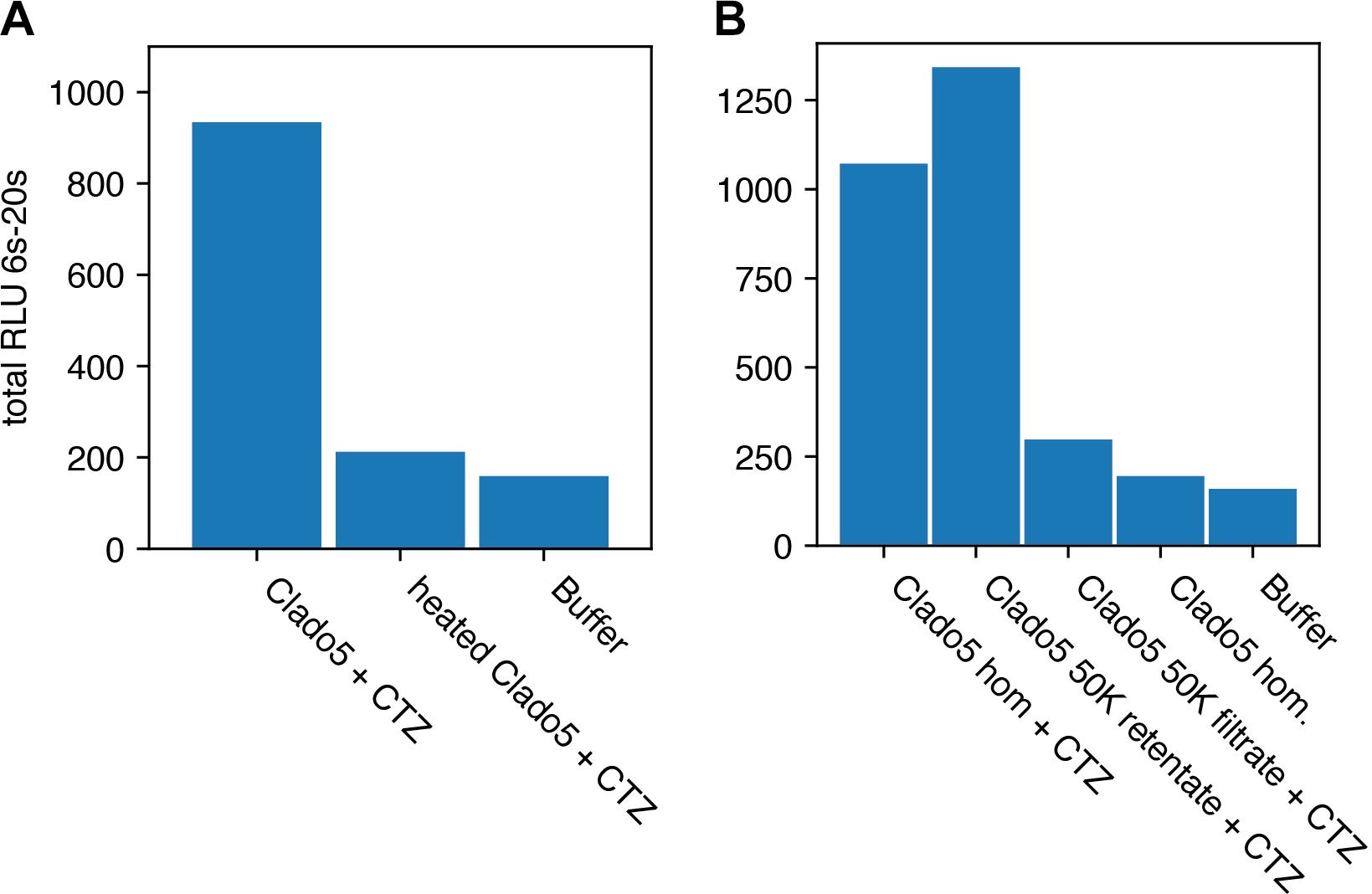
Figure 6. Heat deactivation and activity concentration on a 50K filter. Relative Light Units (RLU) is integrated for 14 s. (A) Shows that heat treating the homogenate (hom.) caused a reduction in bioluminescent activity when coelenterazine (CTZ) was added. (B) Clado5 homogenate bioluminescence activity passed through a 0.2 μm filter, but was concentrated on a 50KDa filter. These results together (Clado5 50K filtrate and retentate) imply that the bioluminescent system is protein-based and consists of a protein or protein complex larger than 50KDa. The Clado5 0.45 μm and 0.1 μm filter-clarified homogenate still emitted light when it was mixed with coelenterazine.
Morphological Description
Sponges had the bulk of their tissue in a globular mass (8–18 mm length, 5–14 mm width) with numerous (n = 12) filamentous processes (9–15 mm length), suspended upon short stalks (37 to 79 mm length, 0.44–0.65 mm width), with a rhizoid anchoring structure (41–80 mm in length). Spicule observations reveal unguiferous anisochelae in one size class, 55–59 μm (Figures 2A,B). Good separation between large alae (wing-shaped projections) of head, three prominent sharp teeth on foot. C-shaped sigmas of two size classes 77–107 μm (Figures 2C,E,G) and ∼170 μm (Figure 2D). Sigmancistra 47–55 μm (Figure 2G). Long, slender megascleres in two size classes (not imaged or measured). Full spicule description and diagnostic treatment awaiting species description. Preliminary analysis supports assignment of the specimen in the genus Cladorhiza but without a match to previously described species from the taxonomic literature.
Molecular Identification and Phylogeny
A contig containing COX1 was assembled using the whole-genome shotgun (WGS) library and validated using read coverage and AA similarity to closely related species (Supplementary Figures 1, 2).
The top two blastn hits for the complete COX1 nucleotide sequence were close to the COX1 sequences from Cladorhiza sp. (KX266208.1) and from Chondrocladia sp. (LN870486.1). Both blastn hits were 97.7% identical to the Clado1 query (Genbank accession number is MN418897). The Cladorhiza species and Chondrocladia species did not form distinct clades in Bayesian and maximum likelihood phylogenetic analyses, and the Clado1 COX1 sequence fell into a polytomy with these sequences (Figure 3).
Metagenomic Analysis
The analysis of 2,020,492 Illumina read pairs found 64,221 reads, or 3.17% of all read pairs, that were identifiable to a taxonomic unit. This low percent of identifiable reads is likely due to the fact that this sponge’s genome, the primary component of the sequencing library, is not present in any online database. One percent of the identifiable read pairs (20,322) were of bacterial origin, although very few read pairs were identifiable as belonging to the bioluminescent bacterial genera Vibrio (133 read pairs; 0.2% of 64,221), Photorhabdus (42 read pairs; 0.07% of 64,221), or Photobacterium (11 reads pairs; 0.02% of 64,221) (Supplementary Figure 7). The remaining largest contributors of identifiable reads were derived from Euteleost fish (8784 reads, 13% of 64,221). Most reads that map to fish were identifiable to the Cyprinus carpio genome, and were attributable to Illumina adapters erroneously included in the Cyprinus carpio genome and occurring at a low rate in the Illumina library. Other reads with a large number of identifiable reads were identifiable as coming from Poecilosclerida sponges (2786 reads, 4.3%), and protostomes (mostly nematodes, 1087 reads, 1.7%). We did not find any reads that were identifiable as ctenophore-origin, suggesting that the sponges may not consume the ctenophores that live on their silicious stalks. We also attempted to map the Clado1 reads against the complete bacterial luciferase operons from multiple bacterial species, but no reads mapped.
Discussion
MBARI’s Video Annotation Reference System (VARS, Schlining and Stout, 2006), a 30 + year record of deep-sea observations, contains many occurrences of Cladorhizidae species. They were observed from different locations where MBARI has conducted ROV research in the Canadian Arctic Ocean, the northeast Pacific, the Gulf of California, and Hawaii. Numerous morphologically similar individuals of our new deep-sea sponge were observed at these sampling locations. Further phylogenetic analyses using additional loci, as well as an extensive morphological characterization, will be necessary to determine this bioluminescent sponge to species level. However, it is clear that these bioluminescent sponges are within a clade containing Chondrocladia and Cladorhiza (Hestetun et al., 2016). This report aims at increasing the awareness and interest of deep-sea ecologists with the hope that they may integrate bioluminescence verification into their collection protocols. It has to be considered as a common functional trait when sharing and publishing their observations and descriptions about benthic and pelagic organisms. This is especially important for a better understanding of deep-sea ecology. This work also aims at highlighting that, even if technologies are now becoming capable of recording in situ video of bioluminescence (Bessho-Uehara et al., 2020), it is still necessary to perform laboratory observations to rule out contamination or commensals from in situ observations.
Doubtful observations of bioluminescence in sponges have been reported since the end of the 19th century (e.g., Pagenstecher, 1881; Okada, 1925). False observations may be induced by numerous bioluminescent symbiotic (Hentschel et al., 2006), entrained, or captured bacteria or animals (annelids, copepods) in sponges (Dahlgren, 1916; Okada, 1925). In our observations, light emitted upon mechanical stimulation was localized to the general area of stimulation, and only lasted for several seconds. The brief glow of several seconds observed in these sponges does not correspond to the continuous glow characteristic of bacterial bioluminescence. We showed that sponge homogenate cross reacts with the luciferin coelenterazine, a molecule not used in any known bioluminescence biochemical system in bacteria or annelids. Another potential source of misinterpretation is the presence of partially digested luminous planktonic organisms, since these are carnivorous sponges. However, the sponge was cleaned before stimulation and light emission was observed in various parts of the sponge mechanically stimulated and for each specimen.
The sponge homogenate released light when mixed with coelenterazine even when it had passed through a 0.1 μm filter, a pore size through which only the smallest bacteria are known to pass (Wang et al., 2008). Moreover, in metagenomic analyses only a very small proportion of the reads were related to bioluminescent bacteria. We also checked carefully for contamination from other small organisms such as copepods or annelids. While we cannot verify with certainty that our observations are autogenic luminescence, the observation of similar bioluminescence on various body parts of six different specimens, from different collection times and locations, with corresponding light kinetics, and an absence of data suggesting that the luminescence is caused by bacteria or other contaminating animals certainly warrants further investigation. A future study confirming that the coelenterazine-luciferase is encoded in the sponge genome will be necessary to validate that these cladorhizid sponges are autogenically luminous.
The observation of bioluminescence in cladorhizid sponges also raises questions regarding the link between the sponges’ bioluminescence capabilities and predatory behavior. In food-deprived environments such as the deep sea, maintaining a water flow through bodies to obtain food and oxygen and to remove wastes may not be metabolically cost-effective for some sessile organisms. Carnivory is a characteristic of Cladorhizidae (Vacelet and Boury-Esnault, 1995; Vacelet and Duport, 2004; Vacelet, 2006; Godefroy et al., 2019) and is known to be an adaptation to a food-poor environment where the metabolic cost of filter feeding would be too inefficient to survive (Lundsten et al., 2017). This sponge family of Cladorhizidae uses hook-shaped spicules to capture small crustacean prey such as copepods and amphipods (Vacelet and Boury-Esnault, 1995). Bioluminescence emissions with long-duration kinetics are known to have a role in prey attraction (Haddock et al., 2010). Thus, it is possible that bioluminescence emissions have the potential to attract or increase prey interest, such that the metabolic cost of producing bioluminescence provides a net gain in prey capture for the sponge. This association of bioluminescence with carnivorous feeding fuels interest in understanding the potential ecological roles of bioluminescence in complex deep-sea food webs. To pursue this unexpected discovery we encourage deep-sea ecologists to integrate bioluminescence as a functional trait of interest.
Lastly, these findings raise questions around the evolution of marine bioluminescence in animals. In our hypothesis, these sponges use coelenterazine as their light-emitting molecule. Coelenterazine is the only luciferin used in the luminescent systems of a few protists and the other non-bilaterian clades, the Cnidaria and the Ctenophora (Haddock et al., 2010). The ancestors of these three phyla likely diverged before the Cambrian Explosion over 600 million years ago (Müller et al., 2008; Dohrmann and Wörheide, 2017), and bioluminescence is thought to play a role in speciation (Ellis and Oakley, 2016). Our results spark interest in the numerous roles of bioluminescence and its involvement in the speciation and diversification of life in the ocean.
Data Availability Statement
The datasets presented in this study can be found in online repositories. The names of the repository/repositories and accession number(s) can be found in the article/Supplementary Material.
Author Contributions
SM first discovered the bioluminescence in this sponge species. SH, SM, and DS collected specimens with the help of the ROV Doc Ricketts pilots and R/V Western Flyer crew. LL performed the morphological identification and spicules images. DS, SM, and SH photographed and filmed the animals on board the ship. DS performed the DNA-based analyses, and the in vivo biochemical assays. SM and DS wrote the original draft. All authors reviewed the manuscript.
Funding
This work was supported by the David and Lucile Packard Foundation, and SM was funded, in part, by a grant from the Bettencourt-Schueller Foundation. DS was funded in part by GRFP DGE 1339067 and NSF DEB-1542679. Two reviewers contributed to improving this manuscript.
Conflict of Interest
The authors declare that the research was conducted in the absence of any commercial or financial relationships that could be construed as a potential conflict of interest.
Acknowledgments
We thank the pilots of the ROV Doc Ricketts and the crew of the R/V Western Flyer. Kyra Schlining provided expertise onboard during both of these expeditions. We also thank Lynne Christianson for tests in molecular biology.
Supplementary Material
The Supplementary Material for this article can be found online at: https://www.frontiersin.org/articles/10.3389/fmars.2020.576476/full#supplementary-material
References
Aizenberg, J., Sundar, V. C., Yablon, A. D., Weaver, J. C., and Chen, G. (2004). Biological glass fibers: correlation between optical and structural properties. Proc. Natl. Acad. Sci. 101, 3358–3363. doi: 10.1073/pnas.0307843101
Altschul, S. (1997). Gapped BLAST and PSI-BLAST: a new generation of protein database search programs. Nucleic Acids Res. 25, 3389–3402. doi: 10.1093/nar/25.17.3389
Belinky, F., Rot, C., Ilan, M., and Huchon, D. (2008). The complete mitochondrial genome of the demosponge Negombata magnifica (Poecilosclerida). Mol. Phylogenet. Evol. 47, 1238–1243. doi: 10.1016/j.ympev.2007.12.004
Bessho-Uehara, M., Francis, W. R., and Haddock, S. H. D. (2020). Biochemical characterization of diverse deep-sea anthozoan bioluminescence systems. Mar. Biol. 167:114. doi: 10.1007/s00227-020-03706-w
Bushnell, B., Rood, J., and Singer, E. (2017). BBMerge–accurate paired shotgun read merging via overlap. PloS One 12:e0185056. doi: 10.1371/journal.pone.0185056
Cattaneo-Vietti, R., Bavestrello, G., Cerrano, C., Sarà, M., Benatti, U., Giovine, M., et al. (1996). Optical fibres in an Antarctic sponge. Nature 383, 397–398. doi: 10.1038/383397b0
Dahlgren, U. (1916). The production of light by animals. J. Frankl. Inst. 181, 805–843. doi: 10.1016/S0016-0032(16)90153-4
Dohrmann, M., and Wörheide, G. (2017). Dating early animal evolution using phylogenomic data. Sci. Rep. 7, 1–6. doi: 10.1038/s41598-017-03791-w
Edgar, R. C. (2004). MUSCLE: multiple sequence alignment with high accuracy and high throughput. Nucleic Acids Res. 32, 1792–1797. doi: 10.1093/nar/gkh340
Ellis, E. A., and Oakley, T. H. (2016). High Rates of Species Accumulation in Animals with Bioluminescent Courtship Displays. Curr. Biol. 26, 1916–1921. doi: 10.1016/j.cub.2016.05.043
Godefroy, N., Le Goff, E., Martinand-Mari, C., Belkhir, K., Vacelet, J., and Baghdiguian, S. (2019). Sponge digestive system diversity and evolution: filter feeding to carnivory. Cell Tissue Res. 377, 341–351. doi: 10.1007/s00441-019-03032-8
Haddock, S. H. D., Moline, M. A., and Case, J. F. (2010). Bioluminescence in the Sea. Annu. Rev. Mar. Sci. 2, 443–493. doi: 10.1146/annurev-marine-120308-081028
Haddock, S. H. D., Rivers, T. J., and Robison, B. H. (2001). Can coelenterates make coelenterazine? Dietary requirement for luciferin in cnidarian bioluminescence. Proc. Natl. Acad. Sci. 98, 11148–11151. doi: 10.1073/pnas.201329798
Hahn, C., Bachmann, L., and Chevreux, B. (2013). Reconstructing mitochondrial genomes directly from genomic next-generation sequencing reads—a baiting and iterative mapping approach. Nucleic Acids Res. 41:e129. doi: 10.1093/nar/gkt371
Harvey, E. N. (1921). Studies on bioluminescence: XIII. Luminescence in the coelenterates. Biol. Bull. 41, 280–287. doi: 10.2307/1536528
Hentschel, U., Usher, K. M., and Taylor, M. W. (2006). Marine sponges as microbial fermenters. FEMS Microbiol. Ecol. 55, 167–177. doi: 10.1111/j.1574-6941.2005.00046.x
Herring, P. J. (1987). Systematic distribution of bioluminescence in living organisms. J. Biolumin. Chemilumin. 1, 147–163. doi: 10.1002/bio.1170010303
Hestetun, J. T., Vacelet, J., Boury-Esnault, N., Borchiellini, C., Kelly, M., Ríos, P., et al. (2016). The systematics of carnivorous sponges. Mol. Phylogenet. Evol. 94, 327–345. doi: 10.1016/j.ympev.2015.08.022
Huelsenbeck, J. P., and Ronquist, F. (2001). MRBAYES: Bayesian inference of phylogenetic trees. Bioinformatics 17, 754–755. doi: 10.1093/bioinformatics/17.8.754
Huson, D. H., Auch, A. F., Qi, J., and Schuster, S. C. (2007). MEGAN analysis of metagenomic data. Genome Res. 17, 377–386. doi: 10.1101/gr.5969107
Li, H. (2013). Aligning Sequence Reads, Clone Sequences and Assembly Contigs With BWA-MEM. ArXiv13033997 Q-Bio. Available online at: http://arxiv.org/abs/1303.3997 [accessed June 26, 2019]
Lundsten, L., Reiswig, H. M., and Austin, W. C. (2017). Three new species of Cladorhiza (Demospongiae, Poecilosclerida, Cladorhizidae) from the Northeast Pacific Ocean. Zootaxa 4317, 247–260. doi: 10.11646/zootaxa.4317.2.3
Martini, S., and Haddock, S. H. D. (2017). Quantification of bioluminescence from the surface to the deep sea demonstrates its predominance as an ecological trait. Sci. Rep. 7:45750. doi: 10.1038/srep45750
Martini, S., Kuhnz, L., Mallefet, J., and Haddock, S. H. D. (2019). Distribution and quantification of bioluminescence as an ecological trait in the deep sea benthos. Sci. Rep. 9, 1–11. doi: 10.1038/s41598-019-50961-z
Meyer, M., and Kircher, M. (2010). Illumina Sequencing Library Preparation for Highly Multiplexed Target Capture and Sequencing. Cold Spring Harb. Protoc. 2010:5448. doi: 10.1101/pdb.prot5448
Müller, W. E. G., Kasueske, M., Wang, X., Schröder, H. C., Wang, Y., Pisignano, D., et al. (2008). Luciferase a light source for the silica-based optical waveguides (spicules) in the demosponge Suberites domuncula. Cell. Mol. Life Sci. 66:537. doi: 10.1007/s00018-008-8492-5
Okada, Y. K. (1925). Luminescence in Sponges. Science 62, 566–567. doi: 10.1126/science.62.1616.566-a
Pagenstecher, H. A. (1881). Allgemeine Zoologie, Oder Grundgesetze des Thierischen Baus Und Lebens. Sydney: Wentworth Press.
Reiswig, H. M., and Browman, H. I. (1987). Use of Membrane Filters for Microscopic Preparations of Sponge Spicules. Trans. Am. Microsc. Soc. 106, 10–20. doi: 10.2307/3226280
Ronquist, F., and Huelsenbeck, J. P. (2003). MrBayes 3: Bayesian phylogenetic inference under mixed models. Bioinformatics 19, 1572–1574. doi: 10.1093/bioinformatics/btg180
Schlining, B. M., and Stout, N. J. (2006). MBARI’s Video Annotation and Reference System. Oceans 2006, 1–5. doi: 10.1109/OCEANS.2006.306879
Stamatakis, A. (2014). RAxML version 8: a tool for phylogenetic analysis and post-analysis of large phylogenies. Bioinformatics 30, 1312–1313. doi: 10.1093/bioinformatics/btu033
Vacelet, J. (2006). New carnivorous sponges (Porifera, Poecilosclerida) collected from manned submersibles in the deep Pacific. Zool. J. Linn. Soc. 148, 553–584. doi: 10.1111/j.1096-3642.2006.00234.x
Vacelet, J., and Boury-Esnault, N. (1995). Carnivorous sponges. Nature 373, 333–335. doi: 10.1038/373333a0
Vacelet, J., and Duport, E. (2004). Prey capture and digestion in the carnivorous sponge Asbestopluma hypogea (Porifera: Demospongiae). Zoomorphology 123, 179–190. doi: 10.1007/s00435-004-0100-0
Walker, B. J., Abeel, T., Shea, T., Priest, M., Abouelliel, A., Sakthikumar, S., et al. (2014). Pilon: An Integrated Tool for Comprehensive Microbial Variant Detection and Genome Assembly Improvement. PLoS One 9:e112963. doi: 10.1371/journal.pone.0112963
Wang, X., Fan, X., Schröder, H. C., and Müller, W. E. G. (2012). Flashing light in sponges through their siliceous fiber network: A new strategy of “neuronal transmission” in animals. Chin. Sci. Bull. 57, 3300–3311. doi: 10.1007/s11434-012-5241-9
Wang, Y., Hammes, F., Duggelin, M., and Egli, T. (2008). Influence of size, shape, and flexibility on bacterial passage through micropore membrane filters. Environ. Sci. Technol. 42, 6749–6754.
Keywords: bioluminescence, Porifera, deep-sea, benthos, ecology
Citation: Martini S, Schultz DT, Lundsten L and Haddock SHD (2020) Bioluminescence in an Undescribed Species of Carnivorous Sponge (Cladorhizidae) From the Deep Sea. Front. Mar. Sci. 7:576476. doi: 10.3389/fmars.2020.576476
Received: 26 June 2020; Accepted: 09 November 2020;
Published: 03 December 2020.
Edited by:
Daphne Cuvelier, Marine and Environmental Sciences Centre, PortugalReviewed by:
Joana R. Xavier, University of Porto, PortugalVacelet Jean, UMR 7263 Institut Méditerranéen de Biodiversité et d’Ecologie Marine et Continentale (IMBE), France
Copyright © 2020 Martini, Schultz, Lundsten and Haddock. This is an open-access article distributed under the terms of the Creative Commons Attribution License (CC BY). The use, distribution or reproduction in other forums is permitted, provided the original author(s) and the copyright owner(s) are credited and that the original publication in this journal is cited, in accordance with accepted academic practice. No use, distribution or reproduction is permitted which does not comply with these terms.
*Correspondence: Séverine Martini, c2V2ZXJpbmUubWFydGluaUBtaW8ub3N1cHl0aGVhcy5mcg==
†These authors have contributed equally to this work