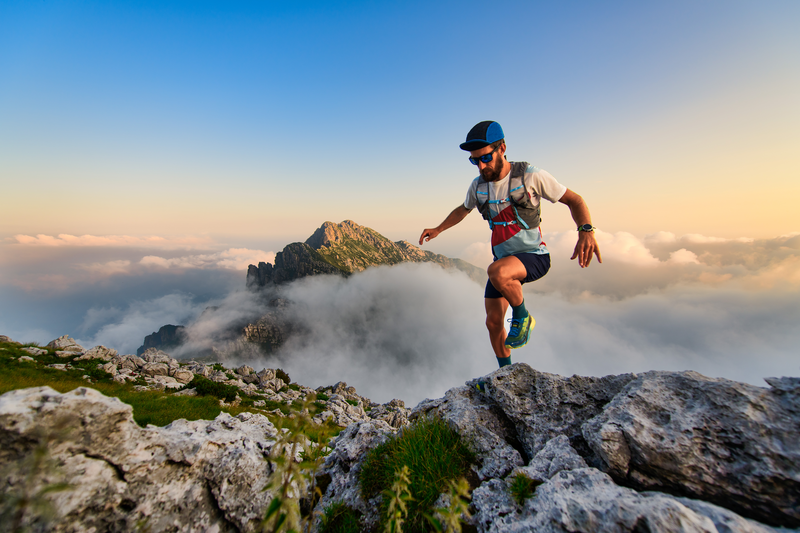
94% of researchers rate our articles as excellent or good
Learn more about the work of our research integrity team to safeguard the quality of each article we publish.
Find out more
REVIEW article
Front. Mar. Sci. , 04 November 2020
Sec. Marine Conservation and Sustainability
Volume 7 - 2020 | https://doi.org/10.3389/fmars.2020.544105
This article is part of the Research Topic Marine Ecosystem Restoration (MER) – Challenges and New Horizons View all 23 articles
Coastal marine ecosystems provide critical goods and services to humanity but many are experiencing rapid degradation. The need for effective restoration tools capable of promoting large-scale recovery of coastal ecosystems in the face of intensifying climatic stress has never been greater. We identify four major challenges for more effective implementation of coastal marine ecosystem restoration (MER): (1) development of effective, scalable restoration methods, (2) incorporation of innovative tools that promote climate adaptation, (3) integration of social and ecological restoration priorities, and (4) promotion of the perception and use of coastal MER as a scientifically credible management approach. Tackling these challenges should improve restoration success rates, heighten their recognition, and accelerate investment in and promotion of coastal MER. To reverse the accelerating decline of marine ecosystems, we discuss potential directions for meeting these challenges by applying coastal MER tools that are science-based and actionable. For coastal restoration to have a global impact, it must incorporate social science, technological and conceptual advances, and plan for future climate scenarios.
Humanity is facing serious environmental challenges at the onset of the Anthropocene (Crutzen, 2002; Kareiva et al., 2011; He and Silliman, 2019). The swift decay of natural ecosystems, their biodiversity, and services to humans presents a global challenge (Dobson et al., 2006; Dirzo et al., 2014; Hautier et al., 2015). Coastal marine ecosystems are immensely important for human well-being (Barbier, 2012; Duarte et al., 2013), and they are among those facing the most rapid ecological degradation (Lotze et al., 2006; Duke et al., 2007; Waycott et al., 2009; Beck et al., 2011; Burke et al., 2011; Bugnot et al., 2020), resulting in declines in the goods and services that they provide to society (Cesar, 2000; Barbier, 2012; Costanza et al., 2014).
The decline of many coastal ecosystems and current lack of effective solutions for reversing this trend have triggered growing interest in developing tools for the restoration of degraded marine environments (Edwards, 1999; Elliott et al., 2007; Borja, 2014; Possingham et al., 2015; Kienker et al., 2018; Airoldi et al., 2020). For example, recovering ecosystem structure and function through restoration has recently been identified as one of eight “grand challenges” in marine ecosystems ecology (Borja, 2014). Although significant progress has been made in some coastal systems, notably mangroves, kelp forests, wetlands, seagrass meadows, oyster reefs, and to some extent, coral reefs (Hashim et al., 2010; Beck et al., 2011; Roman and Burdick, 2012; Campbell et al., 2014; van Katwijk et al., 2016; Boström-Einarsson et al., 2020; Eger et al., 2020), restoration science of coastal marine ecosystems lags behind terrestrial and freshwater counterparts (Craig, 2002; Suding, 2011).
Restoration has been defined in multiple ways (Elliott et al., 2007). Here, we use the common definition, “the process of assisting the recovery of damaged, degraded, or destroyed ecosystems” (Hobbs et al., 2004; SER, 2004), which views restoration as a broad term that spans from preventative management aimed at stress relief to full habitat reconstruction. We consider restoration to be an integral part of conservation management (Abelson et al., 2015; Possingham et al., 2015), but the full recognition of ecological restoration as an essential element of coastal marine management (Murcia et al., 2014) will require well-defined and achievable objectives, and reliable cost-effective restoration tools (Bayraktarov et al., 2016). While we acknowledge that progress has been made in developing novel tools for marine ecosystem restoration (MER; e.g., eco-engineering or nature-based solutions; Morris et al., 2019), the increasing rate of degradation of coastal environments emphasizes the need for rapid development of integrative approaches to science-based restoration of marine ecosystems (e.g., Elliott et al., 2007; Abelson et al., 2015; Possingham et al., 2015; Airoldi et al., 2020). An important first step in this process is to identify major scientific, societal and operational gaps in coastal MER, which should help to accelerate the development of more effective, scalable tools and practical approaches for coastal MER. Overall, our goal is to build an effective framework for enhancing the multidisciplinary science of coastal MER via the following objectives: (1) development of cost-effective, scalable restoration tools, (2) use of these tools to promote adaptation of coastal marine ecosystems to cope with climate change and global stressors, (3) integration of social and ecological restoration priorities, and (4) fostering the acceptance and routine consideration of coastal MER as a scientifically credible management tool (Figure 1).
Figure 1. Conceptual framework of the four major coastal Marine Ecosystem Restoration (MER) challenges (i.e., development of tools, adaptation to global stressors, social-ecological integration, and promotion of MER perception; blue circles), and their governing and interacting factors (red rectangles), all of which influence the state of coastal MER (i.e., the success and progress of MER projects; hexagon). Solid arrows indicate flow of knowledge, labor and other monetary values. Broken arrows indicate interactions among the coastal MER positive-feedback loop (“stagnation loop”).
Many current coastal MER tools (techniques and methodologies) have been criticized for high costs that exceed perceived benefits, often with superficial treatment of symptoms rather than the causes of degradation (Elliott et al., 2007; Mumby and Steneck, 2008; van Katwijk et al., 2016; but see Lefcheck et al., 2018; Reguero et al., 2018). Four common and potentially inter-related methodological problems that can result in coastal MER failure are: (1) lack of clear criteria for success, (2) challenging site selection, (3) inadequate or inappropriate tool selection/availability including scalability commensurate with the scale of degraded habitats, and (4) poorly designed assessment protocols (Suding, 2011; Abelson et al., 2015; Bayraktarov et al., 2016).
The implementation of clear, measurable restoration goals requires quantifiable benchmarks for determining whether or not the stated restoration goals are achieved (SER, 2004; Suding, 2011). Specific criteria used to measure success (such as resilience indicators; Maynard et al., 2015) will by necessity vary depending on project goals and stakeholder interests, and should be specified at the outset. The inclusion of key stakeholders and the institutions they represent is crucial in the framing of restoration strategies and related expectations of the outcomes of the MER effort. Projects are likely to gain wider acceptance if their goals are broadened to include ecosystem services such as coastal protection and job creation (Temmerman et al., 2013; Kittinger et al., 2016; World Bank, 2016) that benefit a variety of aware and connected stakeholders (Coen and Luckenbach, 2000; Abelson et al., 2015; Strain et al., 2019).
Appropriate site selection, especially complicated in increasingly urbanized and fragmented systems, is a major determinant of restoration success (Suding, 2011; Bayraktarov et al., 2016). The selection of sites to be restored should be done carefully with consideration of both ecological (e.g., connectivity among populations) and social (e.g., business plan for long-term stewardship) objectives that can reduce the risk of restoration failures (Abelson et al., 2015; Bayraktarov et al., 2016). Also, restoration should be prioritized in areas where the local stressors responsible for the initial degradation of the site are known and can be reduced to levels compatible with the long-term sustainability of the intervention. In cases of non-manageable stressors, e.g., climate-change effects or heavy boat traffic, a different restoration approach should be applied, which promotes adaptation to cope with climate-change conditions (see section “Promoted adaptation”), or eco-engineering techniques, such as living breakwaters, to insulate against stressors (see New tools, approaches, and conceptual framework, below). If multiple candidate sites are available, then these should be compared by relating past, present and predicted future community states using information on environmental conditions, ambient stressors, risks, biodiversity values, and ecosystem services (e.g., Game et al., 2008; Abelson et al., 2015, 2016a).
Inadequate funding for well-designed monitoring aimed at evaluating the success of a project in meeting its objectives is another major drawback of restoration efforts in general (Palmer and Filoso, 2009; Suding, 2011), and in coastal marine ecosystems in particular (Bayraktarov et al., 2016). Even in cases where monitoring and evaluation is planned, it is often funded for a short period of time, not allowing for proper assessment of the outcome of the project over time (Statton et al., 2012, 2018). In other cases, monitoring is overlooked and considered to be an unnecessary additional cost of restoration (Bayraktarov et al., 2016). However, information gained from monitoring (ecological and social parameters) is not only necessary for determining whether the restoration goals are being met, but is essential in determining the reasons for failures, which are critically important for informing future restoration and conservation efforts. Such information is also essential for evaluating the long-term resilience of MER interventions in the face of changing climatic and societal pressures such as land use that results in continued degradation of water quality and habitat destruction (Bouma et al., 2014). The length of monitoring will depend on the stated restoration goals and performance criteria, and on the ecology of the system being restored, which influences rates of recovery. Additionally, the timescales of recovery periods may be related to the life-history characteristics of the key species targeted for restoration (e.g., ecosystem engineer species, Montero-Serra et al., 2018). Therefore, the design of monitoring programs should include relevant ecological (e.g., demographic knowledge) and social performance metrics and governance indicators (e.g., fish functional diversity, fish catch yields, coastal erosion rates, level of conflict among stakeholders), with the cost of developing and implementing a monitoring plan included as a prerequisite for all restoration projects.
Advancements in restoration tools and approaches that optimize success and cost-effectiveness of coastal MER may take several directions. First, indirect restoration tools can revitalize damaged ecosystems by alleviating physical stressors or improving local conditions (also termed passive restoration; Perrow and Davy, 2002). For example, improving the quality of coastal waters by restoring terrestrial ecosystems within the relevant watershed area (e.g., by re-forestation, retention ponds and constructed wetlands; Bartley et al., 2014; Abelson et al., 2016a; Roque et al., 2016; Lefcheck et al., 2018). The restoration of the hydrological conditions in mangrove rehabilitation areas provides another example, including dismantling weirs and removing dikes and dams to reduce the duration of inundation with polluted water. This in turn may enhance the dispersal and successful colonization of propagules, and promote the chances of natural regeneration (Van Loon et al., 2016). The implementation of indirect tools that have the potential to accelerate recovery and enhance resilience of restored systems should be considered in combination with direct approaches (e.g., planting and seeding) to achieve restoration goals (e.g., Lefcheck et al., 2018).
Second, technological advances can lead to efficiencies of scale and drastic reductions in cost. For instance, restoring corals through large-scale capture and release of coral larvae on decayed reefs is predicted to be much cheaper than restoring the same amount of area with garden-grown adult corals (Doropoulos et al., 2019). Likewise, restoring marsh grasses and seagrasses is sometimes more successful when they are outplanted with biodegradable structures that protect them from wave action and sediment erosion (Temmink et al., 2020, but see Orth et al., 1999; Statton et al., 2018). Another potential direction for optimizing restoration success is the development of relatively low-cost restoration tools that can be effectively scaled to different sized projects (Spurgeon, 1999; Spurgeon and Lindahl, 2000; Bayraktarov et al., 2016). An example of one such restoration approach involves restocking of key consumers (also termed “biomanipulation;” Lindegren et al., 2010). For instance, depleted herbivorous fish populations on degraded coral reefs can lead to undesirable algal-dominated phase shifts following natural disturbance events (e.g., Bellwood et al., 2006). However, in many cases the recovery of fish populations under strict fishery management and fishing bans may take many years (up to several decades; e.g., MacNeil et al., 2015). Therefore, restocking of herbivorous fish populations (accepting the prerequisite of protection in the restored site) may prevent the excessive proliferation of macroalgae, or accelerate their eradication and aid in the recovery of degraded reefs that have undergone a phase shift to an undesirable macroalgal-dominated state (Abelson et al., 2016b; Obolski et al., 2016). Under certain circumstances, eradication or culling of, for example, herbivores may be included in the restoration, mainly in temperate ecosystems (Piazzi and Ceccherelli, 2019; Guarnieri et al., 2020; Medrano et al., 2020).
Third, to improve outplanting yields, the paradigm in restoration ecology can be expanded from one frameworks that systematically identifies and reduces physical stressors, to one that also systematically harnesses positive species interactions at all levels of biological organization. This paradigm change was first proposed by Halpern et al. (2007) and Gedan and Silliman (2009) and received the first experimental support by Silliman et al. (2015), who found that planting marsh plants in clumps rather than in dispersed patterns as the paradigm called for resulted in a 100–200% increase in plant yields at no extra cost. Importantly, this study did not add extra resources to the restoration project; instead a simple design change in planting arrangement allowed for naturally occurring positive interactions to occur, as plants in clumps interacted to resist erosion and oxygen stress in the soil (Silliman et al., 2015).
Recent conceptual papers highlight that inserting positive species interactions into restoration of corals, seagrasses and mangroves, as well as into eco-engineered structures, can have beneficial outcomes and need not be limited to just intraspecific facilitation (Shaver and Silliman, 2017; Bulleri et al., 2018; Renzi et al., 2019; Smith et al., 2020; Valdez et al., 2020). Interspecific facilitation and mutualism could be equally or more important. For example, manipulation of the bacterial community is likely to enhance settlement and establishment of foundation species (e.g., corals, seaweeds and mangroves; Holguin et al., 2001; Kelly et al., 2014; Qiu et al., 2019); waterborne chemicals from various species could be mimicked at scale to induce coral settlement and fish grazing behavior (Dixson and Hay, 2012; Dixson et al., 2014); key autogenic ecosystem engineer species can enhance stress tolerance for associated organisms (i.e., “human-assisted evolution;” sensu Palumbi et al., 2014; van Oppen et al., 2015; see: section “Promoted adaptation”); predators can facilitate regrowth of seagrass systems and increase their tolerance to nutrient stress by promoting populations of algal grazing sea slugs (Hughes, 2014); and positive landscape-scale interactions involving fluxes of energy, materials and organisms among ecosystems can facilitate the establishment and persistence of foundation species (Gillis et al., 2014; van de Koppel et al., 2015). While incorporating positive species interactions into restoration designs holds great promise, a recent review unfortunately found that only 3% of over 600 studies investigating coastal restoration actually tested for the effects of inserting facilitation by design (Zhang et al., 2018).
Finally, management concepts should be implemented that combine restoration efforts with protection. Currently, protection and restoration are rarely integrated into management programs. Protection from anthropogenic stressors is generally not a prerequisite for MER projects, and restoration is often disregarded as a tool in MPA (marine protected area) management plans (Abelson et al., 2016a). We believe that including protection (MPAs) and stress relief in restoration projects as part of ecosystem-based management may be highly effective in conservation and the recovery of coastal marine ecosystems, and therefore, should be a normative baseline.
At present, coastal MER tools rarely enhance ecosystem resistance to climate-change related stressors such as ocean warming, sea-level rise and acidification (but see Shaver et al., 2018; He and Silliman, 2019). However, restoration of coastal vegetation-based ecosystems, which are major carbon sinks (i.e., saltmarshes, mangrove forests and seagrass meadows) can help mitigate climate change over large scales (Gattuso et al., 2018). When combined with other local-management actions, they can also help buffer global climatic impacts and compensate for critical ecosystem services that are impaired (Duarte et al., 2013; Possingham et al., 2015; Abelson et al., 2016a; Anthony et al., 2017; Darling and Côté, 2018; He and Silliman, 2019). Nevertheless, as climate-change mitigation (reduction of greenhouse gases emission) can take at least decades to affect the Earth’s climate (Solomon et al., 2009), there is a growing recognition of the need to identify practical tools to promote adaptation to climate change, so that coastal marine ecosystems can continue to function and provide ecosystem services under a range of future environmental conditions (Webster et al., 2017; Darling and Côté, 2018; Abelson, 2020). We suggest that beyond fostering the services and ecosystem health of degraded coastal marine ecosystems, restoration tools be used to promote adaptation management to cope with future climate-change conditions. We further argue that under the reality of climate-change conditions, practices that promote adaptation should be included in coastal MER projects to improve their long-term success.
Promoted adaptation can be implemented via two potential directions: “Predict-and-Prescribe” approaches (e.g., “assisted evolution” and “designer reefs;” Mascarelli, 2014; Webster et al., 2017; Darling and Côté, 2018), which attempt to foresee future conditions; and the “Portfolio” approach, which considers the range of uncertainty of future conditions (Schindler et al., 2015; Webster et al., 2017; Figure 2). Although the two strategies are distinct, they may serve as complementary tools. That is, even though their applications depend on specific circumstances, both strategies can be simultaneously applied to increase the likelihood of recovery as well as helping to cope with future unpredictable conditions.
Figure 2. Applying coastal Marine Ecosystem Restoration (MER) to build adaptation via two directions: “Predict and Prescribe” and “Portfolio management” approaches. Restoration tools can serve as implementation vectors of promoted adaptation. Alternatively, adaptation concepts can serve as considerations in planning sound restoration interventions.
Predict and prescribe approaches are based on the notion that future environmental conditions can be predicted to some extent. Promoting adaptation of coastal marine ecosystems to predicted plausible climate change scenarios can be achieved by increasing either the intrinsic or extrinsic resistance of a system (Darling and Côté, 2018). Adaptation, in the context of “intrinsic resistance,” often involves manipulating species or genotypes of ecosystem engineers (e.g., coral, mangrove, and seagrass species) to make the system better equipped to contend with changing conditions (e.g., elevated temperature and acidification), and to better resist climate change and other global stressors. Restoration employing “intrinsic resistance” approaches involves identifying or developing resistant genotypes or species, stockpiling them in sufficient quantity (e.g., via culture), and transplanting, re-introducing, or restocking them in areas most influenced by changing conditions – a process termed “assisted colonization” or “assisted migration” (Hoegh-Guldberg et al., 2008; Palumbi et al., 2014; van Oppen et al., 2015; Darling and Côté, 2018; Coleman and Goold, 2019).
Restoration employing “extrinsic resistance” approaches involves identifying and ensuring spatial refuge sites (i.e., “Resistance and Refuge;” Darling and Côté, 2018). Existing “no-take” MPAs tend to support high fish biomass, but typically provide little resistance to large-scale disturbances (Bates et al., 2019; but see Bates et al., 2014), which suggests a need for management to identify and protect regional refugia (Graham et al., 2008). Suitable refugia may include locations that are less vulnerable to climate disturbances (e.g., cool currents and deeper sites; Darling and Côté, 2018), or stressful or frequently disturbed habitats (e.g., high sedimentation, elevated temperature, acidified waters) whose constituent species are locally adapted to tolerate exposure to chronic stressors (Fabricius, 2005; Palumbi et al., 2014; Shamberger et al., 2014; Webster et al., 2017). Such habitats could serve as potential refugia due to their future resistance potential (e.g., Palumbi et al., 2014). Local refugia have the potential to drive cascading processes of large-scale recovery (“robust source sites;” Hock et al., 2017) by possessing high connectivity with the wider ecosystem network, and a low risk of exposure or sensitivity to disturbances. They serve as a source of replenishment when other sites are depleted, and promote the recovery of desirable species (Hock et al., 2017). Sites identified as potential local refugia need to be protected and the recovery of degraded sites of potentially high extrinsic resistance (“potential refugia”) should be promoted by relevant restoration interventions. That is, sites can play a role as potential refugia thanks to favorable environmental conditions dictated by their location. However, if these sites are in a degraded state due to local anthropogenic stressors, they cannot serve as effective refugia, unless those local stressors have been eliminated or reduced and these systems have recovered. Also, for effective restoration and the selection of potential refugia, empirical genetic information is required to assess diversity and the potential adaptive capacity to cope with future conditions (Coleman et al., 2020). This is particularly pertinent for species that exhibit limited dispersal and are therefore susceptible to reduced gene flow (e.g., Buonomo et al., 2017).
The “Portfolio” approach is a risk management tool adopted from financial portfolio theory, which exploits information about spatial covariances in future ecological conditions and applies that tool to spatial targeting of conservation and restoration investments (Schindler et al., 2015; Webster et al., 2017). Recent research in fisheries and terrestrial ecosystems suggests that the portfolio theory can be applied as a potential approach to promote adaptation, while taking into account our inability to fully understand or predict the impacts of large-scale stressors (Crowe and Parker, 2008; Ando and Mallory, 2012; Schindler et al., 2015; Webster et al., 2017). The portfolio approach can be applied in coastal marine ecosystem management via two operational routes: portfolio of sites (adaptation networks of management units; Webster et al., 2017), and portfolio of genotypes and species (optimal sets of propagules; Crowe and Parker, 2008).
This approach is applied via adaptation networks, which are regional systems of managed areas (i.e., “management units”) with attributes that promote adaptation (i.e., managed areas of high diversity, connectivity, and spatial risk mitigation; Webster et al., 2017). The management units should comprise sites of different states, depths and locations, and under diverse environmental conditions, but which are connected physically (horizontally and/or vertically) or demographically (via passive dispersal or active movement) to form networks. To maximize the ecological outcomes of each “management unit,” adequate investment in protection features (planning and maintenance), notably staff capacity, fishery governance, effective enforcement, and MPA area size, has to be ensured (Edgar et al., 2014; Cinner et al., 2016; Gill et al., 2017). However, as most coastal marine ecosystems experience some extent of degradation, protection alone is insufficient and should therefore be integrated with restoration (Possingham et al., 2015; Abelson et al., 2016a). This requires investment in the exploration, examination, and development of restoration tools (e.g., Rogers et al., 2015; Abelson et al., 2016a; Anthony et al., 2017), the aim of which is to improve the recovery of each management unit.
Even if a minimum viable fraction of a given ecosystem can be protected, isolated sites may substantially weaken connectivity among the management units within the potential adaptation network (Green et al., 2015), which may in turn compromise ecosystem functioning and neutralize the effectiveness of the network (Gaines et al., 2010; Berglund et al., 2012; Green et al., 2015). Thus, the restoration of degraded coastal marine ecosystems can promote the recovery of otherwise low-quality management units and subsequently improve the connectivity (e.g., Abelson et al., 2016a; Bayraktarov et al., 2016) and the effectiveness of the “adaptation networks.”
Another application of portfolio theory is to select an optimal set of propagule sources (“propagule portfolio;” i.e., larvae, seeds, seedlings, and fragments) to be used to restore sites in environments of multiple plausible future climates, based on the results of a climate change impact model (e.g., Crowe and Parker, 2008). This approach combines the “intrinsic resistance” and the portfolio approaches, by applying the restoration tools required for the former with the concept of the portfolio of genotypes and species, which expands the set of propagules by a wide range of source sites under diverse environmental conditions.
To apply the “propagule portfolio,” consideration should be given to selecting and culturing propagules comprising an optimal set of genotypes (i.e., a set that minimizes risk of maladaptation across a variety of future plausible climates, while meeting targets on mean adaptive suitability; Crowe and Parker, 2008), collected from populations that experience different environmental conditions, to use in the restoration of a target site via transplantation or restocking. This approach requires two data sources: (1) provenance trial data derived from multiple common culture trials of multiple propagule sources collected from populations located at various environmental conditions (“geographic points”) within a region (e.g., genotypes adapted to pollution; Whitehead et al., 2017); and (2) environmental data for those geographic points (Crowe and Parker, 2008).
The portfolio approach is still largely theoretical with regard to the marine realm (but see Beyer et al., 2018). However, there is a growing array of models and proposed implementation methods that support its high potential as a management approach to cope with climate change and other unpredictable effects (e.g., Aplet and McKinley, 2017; Holsman et al., 2019; Walsworth et al., 2019). Moreover, some studies, from terrestrial and aquatic ecosystems, provide encouraging support for its applicability (e.g., Crowe and Parker, 2008; Penaluna et al., 2018; Eaton et al., 2019).
A major question related to ecosystem restoration in the Anthropocene is whether we can devise and implement restoration practices that service both the needs of society and promote sustained ecological functions and values (i.e., social-ecological restoration). The concept of “social-ecological restoration” extends beyond the usual scientific scope of “ecological restoration,” to include reciprocal relationships between ecosystems and humans (Geist and Galatowitsch, 1999). We give this concept particular attention as restoration is a fundamentally human endeavor and social processes have been historically understudied (Wortley et al., 2013), despite the fact that they can be integral to project success (Bernhardt et al., 2007; Druschke and Hychka, 2015). Social-ecological restoration is not meant to replace ecological restoration and the consideration of natural heritage or biodiversity values, but rather to complement, as they are both nested subsets within the overall definition of restoration. Here, we highlight a few key ways that MER may benefit from the inclusion of social priorities as restoration goals and via the broadened participation of society.
The adoption of a social-ecological approach to restoration can help delineate clearer goals and aid in evaluating project achievements through performance criteria that go beyond just habitat creation (e.g., Palmer and Filoso, 2009) and contribute to the “blue economy” (World Bank United Nations Department of Economic and Social Affairs, 2017). Practically, this can be implemented by prioritizing targeted ecological and social restoration goals (e.g., conservation value, job creation, flood risk reduction; Abelson et al., 2015) that are valued by relevant stakeholders. For example, Stone et al. (2008) found that different resource user-groups were willing to contribute time and money to mangrove restoration in India, but the motivations and level of support were not consistent across groups and related to different perceived ecosystem services (i.e., fisherman supported restoration because they believed mangroves were good fish nurseries whereas rice farmers believed mangroves would control erosion). Accordingly, understanding local motivations for restoration and using that information to set and communicate clear and relevant restoration goals may enhance community buy-in and ongoing support for restoration initiatives. Furthermore, increasing societal understanding of and connection to restoration projects may facilitate more widespread support of ecological restoration as an effective management tool (Challenge 3; e.g., Edwards et al., 2013; NOAA SAB, 2014; World Bank, 2016; Strain et al., 2019).
With emerging threats from climate change and coastal urbanization, we can expect heightened conflict between MER, the propagation of new development and infrastructure, and shifting ecosystems that may impede MER efforts (e.g., mussel restocking in the wake of ocean acidification). Rising to this challenge, the field of eco-engineering has emerged with the goal of restoring ecosystems in a way that maximizes services that are desired by humans (e.g., coastal protection, waste-water treatment), rather than restoring to a previous state. These “designer ecosystems” are unlikely to deliver on all restoration goals (e.g., maximizing the restoration of biodiversity), but they are nevertheless likely to become a vital component of future coastal conservation plans for several reasons (Airoldi et al., 2020). First, eco-engineering projects that combine habitat restoration with infrastructure may be applicable in highly urbanized marine environments where large-scale restoration projects are infeasible or undesirable (Sutton-Grier et al., 2015; Morris et al., 2019). Recent research suggests that perceptions about what is desirable and acceptable in the marine environment seem to be normalizing toward degraded and artificial states (Strain et al., 2019); in these cases, eco-engineering projects can act as demonstration sites exhibiting some of the benefits of restoration within communities that are otherwise disconnected from nature. Second, eco-engineering projects may be able to provide a direct substitute for gray infrastructure that individuals and municipalities are already accustomed to paying for, and thus we may be able to redirect funding that has typically been spent to build and repair expensive gray infrastructure toward restoration (McCreless and Beck, 2016; Sutton-Grier et al., 2018; Airoldi et al., 2020). Finally, by diversifying the goals and motivations behind coastal MER projects, away from purely ecological priorities, it is likely that a larger sector of society will be engaged, restoration will be possible in a greater variety of environments, and highly urbanized areas will be able to contribute toward global restoration goals (e.g., The Bonn Challenge).
Societal involvement in the planning, implementation, and monitoring of restoration projects can play an important role in restoration success. Past experience suggests that integrated coastal MER projects that include consensus among different stakeholder groups are likely to be the most successful and cost-effective, especially in developing countries (Bayraktarov et al., 2016). Moreover, awareness of and connectedness to the marine environment can strongly predict social support for projects aimed at coastal rehabilitation (Strain et al., 2019). Therefore, the early and continuous engagement of key stakeholders (on multiple levels) should be integrated into restoration plans (Figure 1; Abelson et al., 2015; Zhang et al., 2018; Gann et al., 2019). Potential applications for such integration include “Marine Spatial Planning” (MSP; Tallis et al., 2012), marine protected area planning (Giakoumi et al., 2018) and other quantitative frameworks (Samhouri et al., 2012; Tallis et al., 2012). Furthermore, it has become increasingly popular to involve volunteers and citizen scientists in restoration practice and monitoring (Huddart et al., 2016), which can lower project costs (Bayraktarov et al., 2016), confer benefits to the participants including greater life satisfaction (Miles et al., 1998), and foster a stronger environmental ethos (Leigh, 2005). This in turn could help to raise support for other restoration initiatives that volunteers are not directly involved with, and potentially increase the social acceptability of projects. This mirrors the common notion that local communities are responsible for granting (or withholding) social license for a restoration effort, as these will be felt locally. Yet in practice, the dynamics of social acceptance frequently extend beyond local regions and can include stakeholders that are based far from the site in question. As Moffat et al. (2016) argue, restricting social license to local communities “neglects the organizational reality in a modern globalized world”; social license cannot therefore be restricted to “the exclusive domain of fence-line community members and operational managers.” Nevertheless, volunteer efforts may not be feasible or cost-effective in certain contexts or at large scales, in which case it may be more efficient to employ local professionals.
Currently, in many conservation and restoration projects, high paying jobs and management positions go to outside professional experts and significant benefits do not reach local communities (e.g., Blue economy; World Bank United Nations Department of Economic and Social Affairs, 2017). Training and incorporating community-based professionals (e.g., Australia’s Vocational Education Training programs in “Natural Area Restoration” and “Marine Habitats Conservation and Restoration”) as active participants in all project stages will increase societal benefits as well as reduce potential tensions.
The value of implementing a social-ecological restoration approach in management frameworks is gaining traction. This is partly due to the ongoing degradation of coastal marine ecosystem services and the failure of traditional management practices to halt this decline (Possingham et al., 2015; Golden et al., 2016). Incorporating a social-ecological restoration component that focuses on ecosystem service outcomes, rather than exclusively relying on outcomes like biodiversity, may help compensate for decreasing ecosystem services, which now lie well below historical levels in many regions due to misuse, over-exploitation and the emerging threats of climate change (e.g., Golden et al., 2016 and citations therein). Expanding coastal MER to an integrated social-ecological system will increase the scope and complexity of restoration science and governance, and therefore demands expanded investments in development, implementation and maintenance.
The end of the 20th and early years of the 21st century yielded several key studies that raised the scientific background and awareness of ecological restoration, including coastal MER (e.g., Dobson et al., 1997; Edwards, 1999; Jaap, 2000; Young, 2000; Palmer et al., 2004).
In a literature search (Google Scholar) of the terms (restor∗ or rehabilitat∗) and (marine ecosystem∗, coral, mangrove, oyster, saltmarsh, kelp, or seagrass) in the title, we found relatively few restoration papers published prior to 2000 (Figure 3). The trend changed significantly circa 2000 with an order of magnitude increase in the number of restoration studies in six major coastal ecosystems (Figure 3). However, the total number of coastal MER studies remains negligible relative to restoration studies in terrestrial (e.g., forests) and freshwater (e.g., rivers and lakes) ecosystems. We recognize that our figures may be underestimates of the actual numbers of restoration studies. However, figures obtained by our search should provide a reasonable indication of the general trend of restoration ecology as a field of science, and the relative fraction of each sub-field for different ecosystems.
Figure 3. The number of coastal Marine Ecosystem Restoration (MER) papers: the general term “marine ecosystems” and each of the six major coastal ecosystems: coral reefs, mangrove forests, oyster reefs, salt marshes, kelp forests and seagrass meadows, pre- and post-2000.
If the number of peer-reviewed publications serves as a proxy of investment in science (Ebadi and Schiffauerova, 2016), then it can be argued that, despite the growing research in coastal MER, investment is still relatively low, lagging behind restoration research of non-marine environments. A possible reason for this lagging behind of restoration of marine ecosystems is that their restoration projects are still undervalued (Gordon et al., 2020), mainly due to criticism about their limited spatial scale and high costs, which are too expensive to combat the extent of anthropogenic threats driving habitat loss (Gordon et al., 2020). The consequence is that major gaps remain in the applicability (e.g., cost-effectiveness) and relevance (i.e., goals detached from the definition of ecological restoration) of many coastal MER projects and practices, which may explain the current poor perception of coastal MER among many marine scientists (e.g., Adger et al., 2005; Mumby and Steneck, 2008: Bayraktarov et al., 2020). Although large-scale successful and relatively low-cost projects exist (notably large-scale mangrove forest, oyster reef and salt-marsh restoration projects; e.g., Beck et al., 2011; Bayraktarov et al., 2016; Friess et al., 2016; Duarte et al., 2020) many restoration projects are costly, conducted at small scales, and with narrow goals that do not benefit a diverse stakeholder group (including the majority of coral reef restoration projects; Bayraktarov et al., 2016). At present, a widespread goal of coastal MER projects is to achieve “item-based success” (i.e., survival of planted transplants, seedlings, or spat; sensu Bayraktarov et al., 2016), which in part reflects a common expectation for quick, measurable results, and a general assumption that associated ecosystem services will follow. The consequence is that basic science and “non-simplistic” applied research projects are missing, but are needed to promote tools, practices and scaling up of coastal MER (Bayraktarov et al., 2020). Moreover, MER is seen as a “risky choice” for resource managers and science policy-makers. Basic science is an important source of new ideas that figure prominently into developing solutions for many of society’s needs (Remedios, 2000). Therefore, support for basic long-term research is crucial for the development and implementation of coastal MER. However, at present the development and implementation of most coastal MER sectors suffer from the effects of a “performance-perception-funding” cycle (“stagnation loop;” Figure 1), in which poorly performing restoration projects lead to poor images of coastal MER, and therefore hinder adequate investment in development of coastal MER science and practice despite general recognition of ecosystem decline. Breaking out of this “stagnation loop” requires major achievements by restoration projects in the relevant ecosystems.
Potential advantages of coastal MER compared with conservation-based management approaches reliant on area protection are best highlighted by successful restoration projects involving mangrove, oyster reefs (Beck et al., 2011; Bayraktarov et al., 2016; Friess et al., 2016) and seagrass meadows (Orth et al., 2012). However, although the list of successful large-scale MER projects continues to increase over time, modeling studies that compare the expected ecological and socio-economic benefits of different management approaches through time should be encouraged to demonstrate the economic benefits of restoration. Results from such studies done to date suggest that restoration-based conservation programs in coral reefs and large-scale efforts in seagrass-based restoration, despite the costly investment, may prove to be worthwhile due to the faster recovery and enhanced ecosystem services (Obolski et al., 2016; van Katwijk et al., 2016).
Targeted restoration projects with realistic ecological and socio-economic goals should help identify important knowledge gaps in coastal MER (i.e., SER, 2004 definition). Such goals include ecosystem-level parameters (e.g., fish species diversity and biomass) and upgraded ecosystem services, rather than “item-based success” indicators (e.g., survival of planted ecosystem engineer species). Likewise, coastal MER projects should be scaled up, beyond the usual but limited experimental scales, provided that the stressors that led to the degradation have been eliminated or minimized, or new tools, which help overcome the still existing stressors, are applied. The current proliferation of small-scale, item-based, trial projects, with no stakeholder involvement (Bayraktarov et al., 2016), is unlikely to fill the gaps and needs of realistic coastal MER. Hence, a shift toward realistic coastal MER interventions (i.e., feasible interventions of ecological and socio-economic benefits) is critically needed for coastal MER to gain wider acceptance. We believe that combining coastal MER and coastal ecosystem conservation into a single social-ecological framework (Possingham et al., 2015) has great potential to provide significant socially relevant gains in conserving and restoring highly valued coastal ecosystems. Such integration may further help to increase the traction of coastal MER and improve its perception and acceptance as an effective management strategy.
In view of the ongoing degradation of coastal marine ecosystems, restoration is an inevitable component of conservation management. Successful coastal MER offers great promise for accelerating the recovery of collapsed populations (including globally threatened species), destroyed habitats, and impaired ecosystem services, which may otherwise take much longer to recover (years to decades), if at all. To this end, effective implementation of coastal MER will benefit from incorporation of socio-economic elements, a wider portfolio of methodological tools, more focused post-restoration assessment, climate-change considerations, and wider stakeholder acceptance and engagement. We note that policy and legislation to enable this approach is critical, and notable efforts are being made, including, for example, the United Nations Decade of Ocean Science for Sustainable Development (2021–2030), the United Nations Decade of Ecosystem Restoration (2021–2030), and the European Green Deal, which makes restoration one of the key objectives. We encourage the development of specific recommendations in this field to further support restoration as a fundamental strategy in the race to reverse the decline of coastal marine ecosystems.
– Indirect tools that remove or modulate stressors, accelerate recovery and enhance the resilience of restored systems should be used in combination with direct approaches (e.g., planting and seeding) to achieve restoration goals. Basic scientific research will contribute to identification of such indirect tools.
– The growing need for large-scale restoration interventions, notably projects that combine remediation of degraded ecosystems due to past impacts and adaptation to cope with future threats, requires refinement of existing methods scaled to address the extent of degraded habitat, and support for multidisciplinary research that explores and identifies new tools and approaches. Such research requires adequate funding and a substantial breadth of skills; however, inadequacies in both have hampered the advancement of coastal MER. Therefore, concept promotion and education by ecological restoration proponents is essential for fundamental breakthroughs and coastal MER progress.
– Improved identification and understanding of social processes, drivers and priorities is needed to ensure broad public support and the long-term success of restoration efforts. Ideally, restoration and conservation approaches should be integrated with marine and coastal management. Under this umbrella, engaging local communities in the planning and monitoring of MER projects and designing projects with them to deliver specific socio-economic benefits will greatly enhance the long-term success of both conservation and restoration activities.
– Beyond fostering the ecosystem health and services of degraded coastal marine ecosystems, restoration tools can be used to promote adaptation to cope with
climate-change. Promoted adaptation can be implemented via two potential directions: the “Predict-and-Prescribe” approaches (e.g., “assisted evolution” and “designer reefs”), which attempt to foresee future conditions; and the “Portfolio” approach, which considers the range in uncertainty of future conditions. We argue that MER-based practices that can promote adaptation should be included in coastal zone management plans to improve their long-term success.
All authors have conceived the study. AA led the writing of the manuscript and project coordination. All co-authors contributed to the draft and gave final approval for publication.
LA was supported by projects TETRIS (PRIN 2011, Italian Ministry of Education, University and Research) and CASCADE (INTERREG V A Italy-Croatia CBC Program), and DR by the United States National Science Foundation’s Long Term Ecological Research program. BS was supported by the Lenfest Ocean Program. GK travel to the workshop was funded through an Australian Research Council Linkage grant (LP130100155). The Israel’s Ministry of Environmental Protection and the Israel Society of Ecology and Environmental Sciences.
The authors declare that the research was conducted in the absence of any commercial or financial relationships that could be construed as a potential conflict of interest.
This study was conducted as a part of the “Marine Ecological Restoration Working Group” (MER-WG) workshop held at the Inter University Institute for Marine Science, Eilat (IUI). Thanks are due to an anonymous foundation, the Israel’s Ministry of Environmental Protection and the Israel Society of Ecology and Environmental Sciences, the support of which enabled the workshop. We wish to thank the Dolphin Reef, Eilat, for their help and support, Dr. Sinaia Netanyahu for her support, and the directors and staff of the IUI for their help and hospitality. This is Contribution No. 3887 of the Virginia Institute of Marine Science, College of William & Mary.
Abelson, A. (2020). Are we sacrificing the future of coral reefs on the altar of the “climate change” narrative? ICES J. Mar. Sci. 77, 40–45. doi: 10.1093/icesjms/fsz226
Abelson, A., Halpern, B. S., Reed, D. C., Orth, R. J., Kendrick, G. A., Beck, M. W., et al. (2015). Upgrading marine ecosystem restoration using ecological–social concepts. Bioscience 66, 156–163. doi: 10.1093/biosci/biv171
Abelson, A., Nelson, P., Edgar, G., Shashar, N., Reed, D., Belmaker, J., et al. (2016a). Expanding marine protected areas to include degraded coral reefs. Conserv. Biol. 30, 1182–1191. doi: 10.1111/cobi.12722
Abelson, A., Obolski, U., Regoniel, P., and Hadany, L. (2016b). Restocking herbivorous fish populations as a social-ecological restoration tool in coral reefs. Front. Mar. Sci. 3:138. doi: 10.3389/fmars.2016.00138
Adger, W. N., Hughes, T. P., Folke, C., Carpenter, S. R., and Rockstrom, J. (2005). Social-ecological resilience to coastal disasters. Science 309, 1036–1039. doi: 10.1126/science.1112122
Airoldi, L., Beck, M. W., Firth, L. B., Bugnot, A. B., Steinberg, P. D., and Dafforn, K. A. (2020). Emerging solutions to return nature to the urban ocean. Ann. Rev. Mar. Sci. doi: 10.1146/annurev-marine-032020-020015 [Epub ahead of print].
Ando, A. W., and Mallory, M. L. (2012). Optimal portfolio design to reduce climate-related conservation uncertainty in the Prairie Pothole Region. Proc. Natl. Acad. Sci. U.S.A. 109, 6484–6489. doi: 10.1073/pnas.1114653109
Anthony, K., Bay, L. K., Costanza, R., Firn, J., Gunn, J., Harrison, P., et al. (2017). New interventions are needed to save coral reefs. Nat. Ecol. Evol. 1, 1420–1422.
Aplet, G. H., and McKinley, P. S. (2017). A portfolio approach to managing ecological risks of global change. Ecosyst. Health Sustainabil. 3:e01261. doi: 10.1002/ehs2.1261
Barbier, E. B. (2012). Progress and challenges in valuing coastal and marine ecosystem services. Rev. Environ. Econ. Policy 6, 1–19. doi: 10.1093/reep/rer017
Bartley, R., Bainbridge, Z. T., Lewis, S. E., Kroon, F. J., Wilkinson, S. N., Brodie, J. E., et al. (2014). Relating sediment impacts on coral reefs to watershed sources, processes and management: a review. Sci. Total Environ. 468, 1138–1153. doi: 10.1016/j.scitotenv.2013.09.030
Bates, A. E., Barrett, N. S., Stuart-Smith, R. D., Holbrook, N. J., Thompson, P. A., and Edgar, G. J. (2014). Resilience and signatures of tropicalization in protected reef fish communities. Nat. Clim. Change 4, 62–67. doi: 10.1038/nclimate2062
Bates, A. E., Cooke, R. S. C., Duncan, M. I., Edgar, G. J., Bruno, J. F., Benedetti-Cecchi, L., et al. (2019). Climate resilience in marine protected areas and the ‘Protection Paradox’. Biol. Conserv. 236, 305–314. doi: 10.1016/j.biocon.2019.05.005
Bayraktarov, E., Brisbane, S., Hagger, V., Smith, C. S., Wilson, K. A., Lovelock, C. E., et al. (2020). Priorities and motivations of marine coastal restoration research. Front. Mar. Sci. 7:484. doi: 10.3389/fmars.2020.00484
Bayraktarov, E., Saunders, M. I., Abdullah, S., Mills, M., Beher, J., Possingham, H. P., et al. (2016). The cost and feasibility of marine coastal restoration. Ecol. Appl. 26, 1055–1074. doi: 10.1890/15-1077
Beck, M. W., Brumbaugh, R. D., Airoldi, L., Carranza, A., Coen, L. D., Crawford, C., et al. (2011). Oyster reefs at risk and recommendations for conservation, restoration, and management. Bioscience 61, 107–116. doi: 10.1525/bio.2011.61.2.5
Bellwood, D. R., Hoey, A. S., Ackerman, J. L., and Depczynski, M. (2006). Coral bleaching, reef fish community phase shifts and the resilience of coral reefs. Glob. Change Biol. 12, 1587–1594. doi: 10.1111/j.1365-2486.2006.01204.x
Berglund, M., Jacobi, M. N., and Jonsson, P. R. (2012). Optimal selection of marine protected areas based on connectivity and habitat quality. Ecol. Modell. 240, 105–112. doi: 10.1016/j.ecolmodel.2012.04.011
Bernhardt, E. S., Sudduth, E. B., Palmer, M. A., Allan, J. D., Meyer, J. L., Alexander, G., et al. (2007). Restoring rivers one reach at a time: results from a survey of U.S. river restoration practitioners. Restor. Ecol. 15, 482–493. doi: 10.1111/j.1526-100X.2007.00244.x
Beyer, H. L., Kennedy, E. V., Beger, M., Chen, C. A., Cinner, J. E., Darling, E. S., et al. (2018). Risk-sensitive planning for conserving coral reefs under rapid climate change. Conserv. Lett. 11:e12587. doi: 10.1111/conl.12587
Borja, A. (2014). Grand challenges in marine ecosystems ecology. Front. Mar. Sci. 1:1. doi: 10.3389/fmars.2014.00001
Boström-Einarsson, L., Babcock, R. C., Bayraktarov, E., Ceccarelli, D., Cook, N., Ferse, S. C. A., et al. (2020). Coral restoration – A systematic review of current methods, successes, failures and future directions. PLoS One 15:e0226631. doi: 10.1371/journal.pone.0226631
Bouma, T. J., van Belzen, J., Balke, T., Zhu, Z., Airoldi, L., Blight, A. J., et al. (2014). Identifying knowledge gaps hampering application of intertidal habitats in coastal protection: opportunities & steps to take. Coast. Eng. J. 87, 147–157. doi: 10.1016/j.coastaleng.2013.11.014
Bugnot, A. B., Mayer-Pinto, M., Airoldi, L., Heery, E. C., Johnston, E. L., Critchley, L. P., et al. (2020). Current and projected global extent of marine infrastructure. Nat. Sustainabil. doi: 10.1038/s41893-020-00595-1
Bulleri, F., Eriksson, B. K., Queirós, A., Airoldi, L., Arenas, F., Arvanitidis, C., et al. (2018). Harnessing positive species interactions as a tool against climate-driven loss of coastal biodiversity. PLoS Biol. 16:e2006852. doi: 10.1371/journal.pbio.2006852
Buonomo, R., Assis, J., Fernandes, F., Engelen, A. H., Airoldi, L., and Serrão, E. A. (2017). Habitat continuity and stepping-stone oceanographic distances explain population genetic connectivity of the brown alga Cystoseira amentacea. Mol. Ecol. 26, 766–780. doi: 10.1111/mec.13960
Burke, L. M., Reytar, K., Spalding, M., and Perry, A. (2011). Reefs at Risk Revisited. Washington, DC: World Resources Institute.
Campbell, A. H., Marzinelli, E. M., Vergés, A., Coleman, M. A., and Steinberg, P. D. (2014). Towards restoration of missing underwater forests. PLoS One 9:e84106. doi: 10.1371/journal.pone.0084106
Cesar, H. S. J. (2000). “Coral reefs: their functions, threats and economic value” in Collected Essays on the Economics of Coral Reefs, ed. H. S. J. Cesar (Kalmar: University of Kalmar), 14–39.
Cinner, J. E., Huchery, C., MacNeil, M. A., Graham, N. A., McClanahan, T. R., Maina, J., et al. (2016). Bright spots among the world’s coral reefs. Nature 535, 416–419.
Coen, L. D., and Luckenbach, M. W. (2000). Developing success criteria and goals for evaluating oyster reef restoration: ecological function or resource exploitation? Ecol. Eng. 15, 323–343. doi: 10.1016/S0925-8574(00)00084-7
Coleman, M. A., and Goold, H. (2019). Harnessing synthetic biology for kelp forest conservation. J. Phycol. 55, 745–751. doi: 10.1111/jpy.12888
Coleman, M. A., Wood, G., Filbee-Dexter, K., Minne, A. J., Goold, H. D., Vergés, A., et al. (2020). Restore or redefine: future trajectories for restoration. Front. Mar. Sci. 7:237. doi: 10.3389/fmars.2020.00237
Costanza, R., de Groot, R., Sutton, P., van der Ploeg, S., Anderson, S. J., Kubiszewski, I., et al. (2014). Changes in the global value of ecosystem services. Glob. Environ. Change 26, 152–158.
Craig, R. K. (2002). Taking the long view of ocean ecosystems: historical science, marine restoration, and the oceans act of 2000. Ecol. Law Q. 29:649.
Crowe, K. A., and Parker, W. H. (2008). Using portfolio theory to guide reforestation and restoration under climate change scenarios. Clim. Change 89, 355–370. doi: 10.1007/s10584-007-9373-x
Darling, E. S., and Côté, I. M. (2018). Seeking resilience in marine ecosystems. Science 359, 986–987. doi: 10.1126/science.aas9852
Dirzo, R., Young, H. S., Galetti, M., Ceballos, G., Isaac, N. J., and Collen, B. (2014). Defaunation in the anthropocene. Science 345, 401–406. doi: 10.1126/science.1251817
Dixson, D. L., Abrego, D., and Hay, M. E. (2014). Chemically mediated behavior of recruiting corals and fishes: a tipping point that may limit reef recovery. Science 345, 892–897. doi: 10.1126/science.1255057
Dixson, D. L., and Hay, M. E. (2012). Corals chemically cue mutualistic fishes to remove competing seaweeds. Science 338, 804–807. doi: 10.1126/science.1225748
Dobson, A., Lodge, D., Alder, J., Cumming, G. S., Keymer, J., McGlade, J., et al. (2006). Habitat loss, trophic collapse, and the decline of ecosystem services. Ecology 87, 1915–1924. doi: 10.1890/0012-9658(2006)87[1915:hltcat]2.0.co;2
Dobson, A. P., Bradshaw, A. D., and Baker, A. J. M. (1997). Hopes for the future: restoration ecology and conservation biology. Science 277, 515–522. doi: 10.1126/science.277.5325.515
Doropoulos, D., Elzinga, J., ter Hofstede, R., van Koningsveld, M., and Babcock, R. C. (2019). Optimizing industrial scale coral reef restoration: comparing harvesting wild coral spawn slicks and transplanting gravid adult colonies. Restor. Ecol. 27, 758–767. doi: 10.1111/rec.12918
Druschke, C. G., and Hychka, K. C. (2015). Manager perspectives on communication and public engagement in ecological restoration project success. Ecol. Soc. 20:58. doi: 10.5751/ES-07451-200158
Duarte, C. M., Agusti, S., Barbier, E., Britten, G. L., Castilla, J. C., Gattuso, J. P., et al. (2020). Rebuilding marine life. Nature 580, 39–51.
Duarte, C. M., Losada, I. J., Hendriks, I. E., Mazarrasa, I., and Marbà, N. (2013). The role of coastal plant communities for climate change mitigation and adaptation. Nat. Clim. Change 3, 961–968. doi: 10.1038/nclimate1970
Duke, N. C., Meynecke, J. O., Dittmann, S., Ellison, A. M., Anger, K., Berger, U., et al. (2007). A world without mangroves? Science 317, 41–42.
Eaton, M. J., Yurek, S., Haider, Z., Martin, J., Johnson, F. A., Udell, B. J., et al. (2019). Spatial conservation planning under uncertainty: adapting to climate change risks using modern portfolio theory. Ecol. Appl. 29:e01962.
Ebadi, A., and Schiffauerova, A. (2016). How to boost scientific production? A statistical analysis of research funding and other influencing factors. Scientometrics 106, 1093–1116. doi: 10.1007/s11192-015-1825-x
Edgar, G. J., Stuart-Smith, R. D., Willis, T. J., Kininmonth, S., Baker, S. C., Banks, S., et al. (2014). Global conservation outcomes depend on marine protected areas with five key features. Nature 506, 216–220.
Edwards, A. (1999). Rehabilitation of coastal ecosystems. Mar. Pollut. Bull. 37, 371–372. doi: 10.1016/s0025-326x(99)00149-6
Edwards, P. E. T., Sutton-Grier, A. E., and Coyle, G. E. (2013). Investing in nature: restoring coastal habitat blue infrastructure and green job creation. Mar. Policy 38, 65–71. doi: 10.1016/j.marpol.2012.05.020
Eger, A. M., Marzinelli, E., Gribben, P., Johnson, C. R., Layton, C., Steinberg, P. D., et al. (2020). Playing to the positives: using synergies to enhance kelp forest restoration. Front. Mar. Sci. 7:544. doi: 10.3389/fmars.2020.00544
Elliott, M., Burdon, D., Hemingway, K. L., and Apitz, S. E. (2007). Estuarine, coastal and marine ecosystem restoration: confusing management and science - a revision of concepts. Estuar. Coast. Shelf Sci. 74, 349–366. doi: 10.1016/j.ecss.2007.05.034
Fabricius, K. E. (2005). Effects of terrestrial runoff on the ecology of corals and coral reefs: review and synthesis. Mar. Pollut. Bull. 50, 125–146. doi: 10.1016/j.marpolbul.2004.11.028
Friess, D. A., Lee, S. Y., and Primavera, J. H. (2016). Turning the tide on mangrove loss. Mar. Pollut. Bull. 2, 673–675. doi: 10.1016/j.marpolbul.2016.06.085
Gaines, S. D., White, C., Carr, M. H., and Palumbi, S. R. (2010). Designing marine reserve networks for both conservation and fisheries management. Proc. Natl. Acad. Sci. U.S.A. 107, 18286–18293. doi: 10.1073/pnas.0906473107
Game, E. T., McDonald-Madden, E., Puotinen, M. L., and Possingham, H. P. (2008). Should we protect the strong or the weak? Risk, resilience, and the selection of marine protected areas. Conserv. Biol. 22, 1619–1629. doi: 10.1111/j.1523-1739.2008.01037.x
Gann, G. D., McDonald, T., Walder, B., Aronson, J., Nelson, C. R., Jonson, J., et al. (2019). International principles and standards for the practice of ecological restoration. Second edition. Restor. Ecol. 27, S1–S46.
Gattuso, J. P., Magnan, A. K., Bopp, L., Cheung, W. W., Duarte, C. M., Hinkel, J., et al. (2018). Ocean solutions to address climate change and its effects on marine ecosystems. Front. Mar. Sci. 5:337. doi: 10.3389/fmars.2018.00337
Gedan, K. B., and Silliman, B. R. (2009). Using facilitation theory to enhance mangrove restoration. Ambio 38:109. doi: 10.1579/0044-7447-38.2.109
Geist, C., and Galatowitsch, S. M. (1999). Reciprocal model for meeting ecological and human needs in restoration projects. Conserv. Biol. 13, 970–979. doi: 10.1046/j.1523-1739.1999.98074.x
Giakoumi, S., McGowan, J., Mills, M., Beger, M., Bustamante, R., Charles, A., et al. (2018). Revisiting “success” and “failure” of marine protected areas: a conservation scientist perspective. Front. Mar. Sci. 5:223. doi: 10.3389/fmars.2018.00223
Gill, D. A., Mascia, M. B., Ahmadia, G. N., Glew, L., Lester, S. E., Barnes, M., et al. (2017). Capacity shortfalls hinder the performance of marine protected areas globally. Nature 543, 665–669. doi: 10.1038/nature21708
Gillis, L., Bouma, T., Jones, C., van Katwijk, M., Nagelkerken, I., Jeuken, C., et al. (2014). Potential for landscape-scale positive interactions among tropical marine ecosystems. Mar. Ecol. Prog. Ser. 503, 289–303. doi: 10.3354/meps10716
Golden, C. D., Allison, E. H., Cheung, W. W. L., Dey, M. M., Halpern, B. S., McCauley, D. J., et al. (2016). Nutrition: fall in fish catch threatens human health. Nature 534, 317–320. doi: 10.1038/534317a
Gordon, T. A., Radford, A. N., Simpson, S. D., and Meekan, M. G. (2020). Marine restoration projects are undervalued. Science 367, 635–636.
Graham, N. A., McClanahan, T. R., MacNeil, M. A., Wilson, S. K., Polunin, N. V., Jennings, S., et al. (2008). Climate warming, marine protected areas and the ocean-scale integrity of coral reef ecosystems. PLoS One 3:e3039. doi: 10.1371/journal.pone.0003039
Green, A. L., Maypa, A. P., Almany, G. R., Rhodes, K. L., Weeks, R., Abesamis, R. A., et al. (2015). Larval dispersal and movement patterns of coral reef fishes, and implications for marine reserve network design. Biol. Rev. 90, 1215–1247. doi: 10.1111/brv.12155
Guarnieri, G., Bevilacqua, S., Figueras, N., Tamburello, L., and Fraschetti, S. (2020). Large-scale sea urchin culling drives the reduction of subtidal barren grounds in the Mediterranean Sea. Front. Mar. Sci. 7:519. doi: 10.3389/fmars.2020.00519
Halpern, B. S., Silliman, B. R., Olden, J. D., Bruno, J. P., and Bertness, M. D. (2007). Incorporating positive interactions in aquatic restoration and conservation. Front. Ecol. Environ. 5, 153–160. doi: 10.1890/1540-9295(2007)5[153:ipiiar]2.0.co;2
Hashim, R., Kamali, B., Tamin, N. M., and Zakaria, R. (2010). An integrated approach to coastal rehabilitation: mangrove restoration in Sungai Haji Dorani, Malaysia. Estuar. Coast. Shelf Sci. 86, 118–124. doi: 10.1016/j.ecss.2009.10.021
Hautier, Y., Tilman, D., Isbell, F., Seabloom, E. W., Borer, E. T., and Reich, P. B. (2015). Anthropogenic environmental changes affect ecosystem stability via biodiversity. Science 348, 336–340. doi: 10.1126/science.aaa1788
He, Q., and Silliman, B. R. (2019). Climate change, human impacts, and coastal ecosystems in the Anthropocene. Curr. Biol. 29, R1021–R1035.
Hobbs, R. J., Davis, M. A., Slobodkin, L. B., Lackey, R. T., Halvorson, W., and Throop, W. (2004). Restoration ecology: the challenge of social values and expectations. Front. Ecol. Environ. 2, 43–48. doi: 10.1890/1540-9295(2004)002[0043:retcos]2.0.co;2
Hock, K., Wolff, N. H., Ortiz, J. C., Condie, S. A., Anthony, K. R., Blackwell, P. G., et al. (2017). Connectivity and systemic resilience of the great barrier reef. PLoS Biol. 15:e2003355. doi: 10.1371/journal.pbio.2003355
Hoegh-Guldberg, O., Hughes, L., McIntyre, S., Lindenmayer, D. B., Parmesan, C., Possingham, H. P., et al. (2008). Assisted colonization and rapid climate change. Science 321, 345–346. doi: 10.1126/science.1157897
Holguin, G., Vazquez, P., and Bashan, Y. (2001). The role of sediment microorganisms in the productivity, conservation, and rehabilitation of mangrove ecosystems: an overview. Biol. Fertil. Soils 33, 265–278. doi: 10.1007/s003740000319
Holsman, K. K., Hazen, E. L., Haynie, A., Gourguet, S., Hollowed, A., Bograd, S. J., et al. (2019). Towards climate resiliency in fisheries management. ICES J. Mar. Sci. 76, 1368–1378.
Huddart, J. E., Thompson, M. S., Woodward, G., and Brooks, S. J. (2016). Citizen science: from detecting pollution to evaluating ecological restoration. Wiley Interdiscip. Rev. Water 3, 287–300. doi: 10.1002/wat2.1138
Hughes, B. B. (2014). Food Webs, Resilience, and Functioning of an Estuary Under Multiple Threats: Lessons Learned from Elkhorn Slough. Doctoral dissertation, University of California, Santa Cruz, CA.
Kareiva, P., Lalasz, R., and Marvier, M. (2011). Conservation in the Anthropocene. Breakthrough J. 2, 26–36.
Kelly, L. W., Williams, G. J., Barott, K. L., Carlson, C. A., Dinsdale, E. A., Edwards, R. A., et al. (2014). Local genomic adaptation of coral reef-associated microbiomes to gradients of natural variability and anthropogenic stressors. Proc. Natl. Acad. Sci. U.S.A. 111, 10227–10232. doi: 10.1073/pnas.1403319111
Kienker, S. E., Coleman, R. A., Morris, R. L., Steinberg, P., Bollard, B., Jarvis, R., et al. (2018). Bringing harbours alive: assessing the importance of eco-engineered coastal infrastructure for different stakeholders and cities. Mar. Policy 94, 238–246. doi: 10.1016/j.marpol.2018.04.028
Kittinger, J. N., Bambico, T. M., Minton, D., Miller, A., Mejia, M., Kalei, N., et al. (2016). Restoring ecosystems, restoring community: socioeconomic and cultural dimensions of a community-based coral reef restoration project. Reg. Environ. Change 16, 301–313. doi: 10.1007/s10113-013-0572-x
Lefcheck, J. S., Orth, R. J., Dennison, W. C., Wilcox, D. J., Murphy, R. R., Keisman, J., et al. (2018). Long-term nutrient reductions lead to the unprecedented recovery of a temperate coastal region. Proc. Natl. Acad. Sci. U.S.A. 115:201715798. doi: 10.1073/pnas.1715798115
Leigh, P. (2005). The ecological crisis, the human condition, and community-based restoration as an instrument for its cure. Ethics Sci. Environ. Polit. 5, 3–15. doi: 10.3354/esep005003
Lindegren, M., Möllmann, C., and Hansson, L. A. (2010). Biomanipulation: a tool in marine ecosystem management and restoration? Ecol. Appl. 20, 2237–2248.
Lotze, H. K., Lenihan, H. S., Bourque, B. J., Bradbury, R. H., Cooke, R. G., Kay, M. C., et al. (2006). Depletion, degradation, and recovery potential of estuaries and coastal seas. Science 312, 1806–1809. doi: 10.1126/science.1128035
MacNeil, M. A., Graham, N. A., Cinner, J. E., Wilson, S. K., Williams, I. D., Maina, J., et al. (2015). Recovery potential of the world’s coral reef fishes. Nature 520, 341–344.
Mascarelli, A. (2014). Designer reefs: biologists are directing the evolution of corals to prepare them to fight climate change. Nature 508, 444–447. doi: 10.1038/508444a
Maynard, J. A., McKagan, S., Raymundo, L., Johnson, S., Ahmadia, G. N., Johnston, L., et al. (2015). Assessing relative resilience potential of coral reefs to inform management. Biol. Conserv. 192, 109–119. doi: 10.1016/j.biocon.2015.09.001
McCreless, E., and Beck, M. W. (2016). Rethinking our global coastal investment portfolio. J. Ocean Coastal. Econ. 3:6.
Medrano, A., Hereu, B., Cleminson, M., Pagès-Escolà, M., Rovira, G., Solà, J., et al. (2020). From marine deserts to algal beds: treptacantha elegans revegetation to reverse stable degraded ecosystems inside and outside a No-Take marine reserve. Restor. Ecol. 28, 632–644. doi: 10.1111/rec.13123
Miles, I., Sullivan, W. C., and Kuo, F. E. (1998). Ecological restoration volunteers: the benefits of participation. Urban Ecosyst. 2, 27–41.
Moffat, K., Lacey, J., Zhang, A., and Leipold, S. (2016). The social licence to operate: a critical review. Forestry 89, 477–488. doi: 10.1093/forestry/cpv044
Montero-Serra, I., Garrabou, J., Doak, D. F., Figuerola, L., Hereu, B., Ledoux, J. B., et al. (2018). Accounting for life-history strategies and timescales in marine restoration. Conserv. Lett. 11:e12341. doi: 10.1111/conl.12341
Morris, R. L., Heery, E. C., Loke, L. H. L., Lau, E., Strain, E. M. A., Airoldi, L., et al. (2019). Design options, implementation issues and evaluating success of ecologically engineered shorelines. Oceanogr. Mar. Biol. 57, 169–228. doi: 10.1201/9780429026379-4
Mumby, P. J., and Steneck, R. S. (2008). Coral reef management and conservation in light of rapidly evolving ecological paradigms. Trends Ecol. Evol. 23, 555–563. doi: 10.1016/j.tree.2008.06.011
Murcia, C., Aronson, J., Kattan, G. H., Moreno-Mateos, D., Dixon, K., and Simberloff, D. (2014). A critique of the ‘novel ecosystem’ concept. Trends Ecol. Evol. 29, 548–553. doi: 10.1016/j.tree.2014.07.006
NOAA SAB (2014). Restoration of Coastal Habitats: An Evaluation of NOAA’s Current and Potential Role. Ecosystem Science and Management Working Group. NOAA Science Advisory Board. Available online at: ftp://ftp.oar.noaa.gov/SAB/sab//Meetings/2014/april/NOAA%20Restoration%20Review%20Findings% 20%20Recommendations%20Final%20041714.pdf (accessed March 15, 2020).
Obolski, U., Hadany, L., and Abelson, A. (2016). Potential contribution of fish restocking to the recovery of deteriorated coral reefs: an alternative restoration method? PeerJ 4:e1732. doi: 10.7717/peerj.1732
Orth, R., Moore, K., Luckenbach, M., Bonniwell, S., Curry, A.Jr., Fate, S., et al. (2012). Task 10 Eelgrass and Bay Scallop Restoration in the Seaside Bays of Virginia. (April 1, 2011, to Nov. 30, 2012). Gloucester Point, VA: Virginia Institute of Marine Science.
Orth, R. J., Harwell, M. C., and Fishman, J. R. (1999). A rapid and simple method for transplanting eelgrass using single, unanchored shoots. Aquat. Bot. 64, 77–85. doi: 10.1016/s0304-3770(99)00007-8
Palmer, M., Bernhardt, E., Chornesky, E., Collins, S., Dobson, A., Duke, C., et al. (2004). Ecology for a crowded planet. Science 304, 1251–1252. doi: 10.1126/science.1095780
Palmer, M. A., and Filoso, S. (2009). Restoration of ecosystem services for environmental markets. Science 325, 575–576. doi: 10.1126/science.1172976
Palumbi, S. R., Barshis, D. J., Traylor-Knowles, N., and Bay, R. A. (2014). Mechanisms of reef coral resistance to future climate change. Science 344, 895–898. doi: 10.1126/science.1251336
Penaluna, B. E., Reeves, G. H., Barnett, Z. C., Bisson, P. A., Buffington, J. M., Dolloff, C. A., et al. (2018). Using natural disturbance and portfolio concepts to guide aquatic–riparian ecosystem management. Fisheries 43, 406–422. doi: 10.1002/fsh.10097
Perrow, M. R., and Davy, A. J. (2002). Handbook of Ecological Restoration: Restoration in Practice, Vol. 2. Cambridge: Cambridge University Press.
Piazzi, L., and Ceccherelli, G. (2019). Effect of sea urchin human harvest in promoting canopy forming algae restoration. Estuar. Coast. Shelf Sci. 219, 273–277. doi: 10.1016/j.ecss.2019.02.028
Possingham, H. P., Bode, M., and Klein, C. J. (2015). Optimal conservation outcomes require both restoration and protection. PLoS Biol. 13:e1002052. doi: 10.1371/journal.pbio.1002052
Qiu, Z., Coleman, M. A., Provost, E., Campbell, A. H., Kelaher, B. P., Dalton, S. J., et al. (2019). Future climate change is predicted to affect the microbiome and condition of habitat-forming kelp. Proc. R. Soc. B Biol. Sci. 286:20181887. doi: 10.1098/rspb.2018.1887
Reguero, B. G., Beck, M. W., Bresch, D. N., Calil, J., and Meliane, I. (2018). Comparing the cost effectiveness of nature-based and coastal adaptation: a case study from the gulf coast of the United States. PLoS One 13:e0192132. doi: 10.1371/journal.pone.0192132
Remedios, C. D. (2000). The Value of Fundamental Research. IUPAB. Available online at: http://iupab.org/publications/value-of-fundamental-research/ (accessed March 15, 2020).
Renzi, J., He, Q., and Silliman, B. R. (2019). Harnessing positive species interactions to enhance coastal wetland restoration. Front. Ecol. Evol. 7:131. doi: 10.3389/fevo.2019.00131
Rogers, A., Harborne, A. R., Brown, C. J., Bozec, Y. M., Castro, C., Chollett, I., et al. (2015). Anticipative management for coral reef ecosystem services in the 21st century. Glob. Change Biol. 21, 504–514.
Roman, C. T., and Burdick, D. M. (2012). Tidal Marsh Restoration: a Synthesis of Science and Management. Washington, DC: Island Press.
Roque, F. O., Ochoa-Quintero, J., Ribeiro, D. B., Sugai, L. S., Costa-Pereira, R., Lourival, R., et al. (2016). Upland habitat loss as a threat to Pantanal wetlands. Biol. Conserv. 30, 1131–1134. doi: 10.1111/cobi.12713
Samhouri, J. F., Lester, S. E., Selig, E. R., Halpern, B. S., Fogarty, M. J., Longo, C., et al. (2012). Sea sick? Setting targets to assess ocean health and ecosystem services. Ecosphere 3, 1–18. doi: 10.1890/ES11-00366.1
Schindler, D. E., Armstrong, J. B., and Reed, T. E. (2015). The portfolio concept in ecology and evolution. Front. Ecol. Environ. 13, 257–263. doi: 10.1890/140275
SER (2004). Society for Ecological Restoration International Science & Policy Working Group. The SER International Primer on Ecological Restoration. Tucson, AZ: Society for Ecological Restoration International.
Shamberger, K. E., Cohen, A. L., Golbuu, Y., McCorkle, D. C., Lentz, S. J., and Barkley, H. C. (2014). Diverse coral communities in naturally acidified waters of a Western Pacific reef. Geophys. Res. Lett. 41, 499–504. doi: 10.1002/2013gl058489
Shaver, E. C., Burkepile, D. E., and Silliman, B. R. (2018). Local management actions can increase coral resilience to thermally-induced bleaching. Nat. Ecol. Evol. 2, 1075–1079. doi: 10.1038/s41559-018-0589-0
Shaver, E. C., and Silliman, B. R. (2017). Time to cash in on positive interactions for coral restoration. PeerJ 5:e3499. doi: 10.7717/peerj.3499
Silliman, B. R., Schrack, E., He, Q., Cope, R., Santoni, A., Van Der Heide, T., et al. (2015). Facilitation shifts paradigms and can amplify coastal restoration efforts. Proc. Natl. Acad. Sci. U.S.A. 112, 14295–14300. doi: 10.1073/pnas.1515297112
Smith, C. S., Rudd, M., Gittman, R., Melvin, E., Patterson, V., Renzi, J., et al. (2020). Coming to terms with living shorelines: a scoping review of novel restoration strategies for shoreline protection. Front. Mar. Sci. 7:434. doi: 10.3389/fmars.2020.00434
Solomon, S., Plattner, G., Knutti, R., and Friedlingstein, P. (2009). Irreversible climate change due to carbon dioxide emissions. Proc. Natl. Acad. Sci. U.S.A. 106, 1704–1709. doi: 10.1073/pnas.0812721106
Spurgeon, J. (1999). The socio-economic costs and benefits of coastal habitat rehabilitation and creation. Mar. Pollut. Bull. 37, 373–382. doi: 10.1016/s0025-326x(99)00074-0
Spurgeon, J. P. G., and Lindahl, U. (2000). “Economics of coral reef restoration” in Collected Essays on the Economics of Coral Reefs, ed. H. S. J. Cesar (Kalmar: University of Kalmar), 125–136.
Statton, J., Dixon, K. W., Hovey, R. K., and Kendrick, G. A. (2012). A comparative assessment of approaches and outcomes for seagrass revegetation in Shark Bay and Florida Bay. Mar. Freshw. Res. 63, 984–993. doi: 10.1071/mf12032
Statton, J., Dixon, K. W., Irving, A. D., Jackson, E. L., Kendrick, G. A., Orth, R. J., et al. (2018). “Decline and restoration ecology of Australian seagrasses” in Seagrasses of Australia, eds A. Larkum, G. Kendrick, and P. Ralph (Cham: Springer), 665–704. doi: 10.1007/978-3-319-71354-0_20
Stone, K., Bhat, M., Bhatta, R., and Mathews, A. (2008). Factors influencing community participation in mangroves restoration: a contingent valuation analysis. Ocean Coast. Manag. 51, 476–484. doi: 10.1016/j.ocecoaman.2008.02.001
Strain, E. M. A., Alexander, K. A., Kienker, S., Morris, R., Jarvis, R., Coleman, R., et al. (2019). Urban blue: a global analysis of the factors shaping people’s perceptions of the marine environment and ecological engineering in harbours. Sci. Total Environ. 658, 1293–1305. doi: 10.1016/j.scitotenv.2018.12.285
Suding, K. N. (2011). Toward an era of restoration in ecology: successes, failures, and opportunities ahead. Annu. Rev. Ecol. Evol. Syst. 42, 465–487. doi: 10.1146/annurev-ecolsys-102710-145115
Sutton-Grier, A. E., Gittman, R. K., Arkema, K. K., Bennett, R. O., Benoit, J., Blitch, S., et al. (2018). Investing in natural and nature-based infrastructure: building better along our coasts. Sustainability 10:523. doi: 10.3390/su10020523
Sutton-Grier, A. E., Wowk, K., and Bamford, H. (2015). Future of our coasts: the potential for natural and hybrid infrastructure to enhance the resilience of our coastal communities, economies and ecosystems. Environ. Sci. Policy 51, 137–148. doi: 10.1016/j.envsci.2015.04.006
Tallis, H., Lester, S. E., Ruckelshaus, M., Plummer, M., McLeod, K., Guerry, A., et al. (2012). New metrics for managing and sustaining the ocean’s bounty. Mar. Policy 36, 303–306. doi: 10.1016/j.marpol.2011.03.013
Temmerman, S., Meire, P., Bouma, T. J., Herman, P. M. J., Ysebaert, T., and De Vriend, H. J. (2013). Ecosystem-based coastal defence in the face of global change. Nature 504, 79–83. doi: 10.1038/nature12859
Temmink, R. J. M., Christianen, M. J. A., Fivash, G. S., Angelini, C., Boström, C., Didderen, K., et al. (2020). Mimicry of emergent traits amplifies coastal restoration success. Nat. Commun. 11, 3668. doi: 10.1038/s41467-020-17438-4
Valdez, S. R., Zhang, Y. S., van der Heide, T., Vanderklift, M. A., Tarquinio, F., Orth, R. J., et al. (2020). Positive ecological interactions and the success of seagrass restoration. Front. Mar. Sci. 7:91. doi: 10.3389/fmars.2020.00091
van de Koppel, J., van der Heide, T., Altieri, A. H., Eriksson, B. K., Bouma, T. J., Olff, H., et al. (2015). Long-distance interactions regulate the structure and resilience of coastal ecosystems. Ann. Rev. Mar. Sci. 7, 139–158. doi: 10.1146/annurev-marine-010814-015805
van Katwijk, M. M., Thorhaug, A., Marbà, N., Orth, R. J., Duarte, C. M., Kendrick, G. A., et al. (2016). Global review of seagrass restoration and the importance of large-scale planting. J. Appl. Ecol. 53, 567–578.
Van Loon, A. F., Te Brake, B., Van Huijgevoort, M. H. J., and Dijksma, R. (2016). Hydrological classification, a practical tool for mangrove restoration. PLoS One 11:e0150302. doi: 10.1371/journal.pone.0150302
van Oppen, M. J., Oliver, J. K., Putnam, H. M., and Gates, R. D. (2015). Building coral reef resilience through assisted evolution. Proc. Natl. Acad. Sci. U.S.A. 112, 2307–2313. doi: 10.1073/pnas.1422301112
Walsworth, T. E., Schindler, D. E., Colton, M. A., Webster, M. S., Palumbi, S. R., Mumby, P. J., et al. (2019). Management for network diversity speeds evolutionary adaptation to climate change. Nat. Clim. Change 9, 632–636. doi: 10.1038/s41558-019-0518-5
Waycott, M., Duarte, C. M., Carruthers, T. J., Orth, R. J., Dennison, W. C., Olyarnik, S., et al. (2009). Accelerating loss of seagrasses across the globe threatens coastal ecosystems. Proc. Natl. Acad. Sci. U.S.A. 106, 12377–12381. doi: 10.1073/pnas.0905620106
Webster, M. S., Colton, M. A., Darling, E. S., Armstrong, J., Pinsky, M. L., Knowlton, N., et al. (2017). Who should pick the winners of climate change? Trends Ecol. Evol. 32, 167–173. doi: 10.1016/j.tree.2016.12.007
Whitehead, A., Clark, B. W., Reid, N. M., Hahn, M. E., and Nacci, D. (2017). When evolution is the solution to pollution: key principles, and lessons from rapid repeated adaptation of killifish (Fundulus heteroclitus) populations. Evol. Appl. 10, 762–783. doi: 10.1111/eva.12470
World Bank (2016). Managing Coasts with Natural Solutions: Guidelines for Measuring and Valuing the Coastal Protection Services of Mangroves and Coral Reefs, eds M. W. Beck and G. M. Lange (Washington, DC: World Bank).
World Bank United Nations Department of Economic and Social Affairs (2017). The Potential of the Blue Economy: Increasing Long-Term Benefits of the Sustainable Use of Marine Resources for Small Island Developing States and Coastal Least Developed Countries. Washington, DC: World Bank.
Wortley, L., Hero, J., and Howes, M. (2013). Evaluating ecological restoration success: a review of the literature. Restor. Ecol. 21, 537–543. doi: 10.1111/rec.12028
Young, T. P. (2000). Restoration ecology and conservation biology. Biol. Conserv. 92, 73–83. doi: 10.1016/s0006-3207(99)00057-9
Keywords: coastal marine ecosystems, social-ecological restoration, coral reefs, seagrass, mangrove, oyster reefs, kelp, saltmarshes
Citation: Abelson A, Reed DC, Edgar GJ, Smith CS, Kendrick GA, Orth RJ, Airoldi L, Silliman B, Beck MW, Krause G, Shashar N, Stambler N and Nelson P (2020) Challenges for Restoration of Coastal Marine Ecosystems in the Anthropocene. Front. Mar. Sci. 7:544105. doi: 10.3389/fmars.2020.544105
Received: 19 March 2020; Accepted: 30 September 2020;
Published: 04 November 2020.
Edited by:
Antonio Di Franco, Stazione Zoologica Anton Dohrn, ItalyReviewed by:
Cristina Linares, University of Barcelona, SpainCopyright © 2020 Abelson, Reed, Edgar, Smith, Kendrick, Orth, Airoldi, Silliman, Beck, Krause, Shashar, Stambler and Nelson. This is an open-access article distributed under the terms of the Creative Commons Attribution License (CC BY). The use, distribution or reproduction in other forums is permitted, provided the original author(s) and the copyright owner(s) are credited and that the original publication in this journal is cited, in accordance with accepted academic practice. No use, distribution or reproduction is permitted which does not comply with these terms.
*Correspondence: Avigdor Abelson, YXZpZ2RvckB0YXVleC50YXUuYWMuaWw=
Disclaimer: All claims expressed in this article are solely those of the authors and do not necessarily represent those of their affiliated organizations, or those of the publisher, the editors and the reviewers. Any product that may be evaluated in this article or claim that may be made by its manufacturer is not guaranteed or endorsed by the publisher.
Research integrity at Frontiers
Learn more about the work of our research integrity team to safeguard the quality of each article we publish.