- 1School of Biological Sciences, The University of Queensland, Saint Lucia, QLD, Australia
- 2TropWATER, Centre for Tropical Water and Aquatic Ecosystem Research, College of Science and Engineering, James Cook University, Townsville, QLD, Australia
- 3The Nature Conservancy, Carlton, VIC, Australia
- 4College of Science and Engineering, Flinders University, Bedford Park, SA, Australia
- 5School of Biological Sciences and Oceans Institute, University of Western Australia, Crawley, WA, Australia
- 6Research Institute for Environment and Livelihoods, Charles Darwin University, Darwin, NT, Australia
Marine coastal (or “blue”) ecosystems provide valuable services to humanity and the environment, but global loss and degradation of blue ecosystems necessitates ecological restoration. However, blue restoration is an emerging field and is still relatively experimental and small-scale. Identification of the key barriers to scaling-up blue restoration will enable targeted problem solving and increase the likelihood of success. Here we describe the environmental, technical, social, economic, and political barriers to restoration of blue ecosystems, including saltmarsh, mangroves, seagrass, shellfish reefs, coral reefs, and kelp forests. We provide managers, practitioners, and decision-makers with solutions to construct barrier-informed blue restoration plans and illustrate these solutions through the use of case studies where barriers were overcome. We offer a way forward to build confidence in blue restoration for society, government, and restoration practitioners at larger and more ambitious scales.
In a nutshell
• Marine and coastal, or “blue” restoration is increasing in relevance and need due to degradation and loss of habitat, combined with increasing need for ecosystem-based climate change mitigation and adaptation.
• Restoration of blue ecosystems can be challenging due to environmental, technical, social, economic, and political barriers.
• Case studies provide insights of how to overcome barriers to restoration.
• Identifying barriers and evaluating potential pathways to achieve success is essential to enable blue restoration to play a major role in biodiversity conservation, and climate change mitigation and adaptation.
Introduction
Marine and coastal, or “blue” ecosystems, such as saltmarsh, mangroves, seagrass, shellfish reefs, coral reefs, tidal flats, and kelp forests and other seaweed beds, provide significant ecosystem services that have intrinsic value to environmental, social, and economic sustainability (MEA, 2005). These ecosystem services include regulating and maintenance services (e.g., coastal protection, climate regulation), provisioning (e.g., food, nutrient cycling, water quality), and cultural benefits (e.g., recreation, tourism) (Barbier et al., 2011). However, blue ecosystems are also some of the most heavily modified systems on Earth. Large scale global losses in habitat have been reported for mangroves and saltmarshes (Valiela et al., 2001; Gedan et al., 2009), kelp forests (Krumhansl et al., 2016), coral reefs (Hughes et al., 2017), shellfish reefs (Beck et al., 2011), seagrass (Waycott et al., 2009; Arias-Ortiz et al., 2018), and tidal flats (Murray et al., 2019). Drivers of loss vary and include pollution, invasive or pest species, disease, overexploitation or destructive fishing methods, land conversion, and the threats associated with climate change and associated extreme weather events (Valiela et al., 2001; Waycott et al., 2009; Beck et al., 2011). Ecological restoration is a priority where the natural recovery of degraded habitat(s) is slow, absent, or hindered by physical or biological factors (Perrow and Davy, 2002; SER, 2004).
Restoration describes interventions to assist in the recovery of an ecosystem, while “blue restoration” refers to interventions focused on the recovery of marine and coastal ecosystems. The importance of restoration of natural systems is recognized by numerous global accords and conventions, all aimed at accelerating ecological restoration. These include the Millennium Ecosystem Assessment (MEA, 2005), the Convention on Biological Diversity (CBD, 2010), Bonn Challenge (restoration of 350 million hectares by 2030), the United Nations Sustainable Development Goals (SDGs) in particular SDG14 “Life Below Water,” and the recent United Nations (UN) declarations for “Decade for Ecosystem Restoration 2021–2030” (Salvador, 2018; Waltham et al., 2020) and “Decade of Ocean Science for Sustainable Development 2021–2030” (UN, 2019).
Until recently, the focus of restoration has been largely terrestrial; with blue restoration still mostly small-scale, costly, and with limited long-term success (Bayraktarov et al., 2016). Meeting the ambitious global targets will require successful implementation of blue restoration at scales not yet seen. Successful blue restoration must overcome environmental, technical, social, economic, and political barriers to implementation. The identification and assessment of common barriers will allow managers to target, prioritize, and potentially eliminate barriers during the planning phase, increasing the likelihood of project success. Here we describe common barriers to blue restoration projects, evaluate the level of threat they pose to success for each ecosystem type, detail the relationship between barriers and restoration success, and provide real-world examples where barriers have been overcome. Now is the time to build confidence in society, government, and amongst restoration practitioners through resolving how to overcome multiple barriers to enable scaling-up blue restoration to ecologically-relevant scales.
Barriers to Blue Restoration
A series of workshop discussions among the authors drew on our knowledge of the restoration literature to identify common barriers to successful blue restoration at the scale required to meet future targets (MEA, 2005; CBD, 2010; Salvador, 2018; GMA, 2019). We categorized these into five broad classes: environmental, technical, social, economic, and political (Figure 1). To supplement the knowledge of the authors, we conducted a targeted search of the Web of Science databases and Google Scholar. The resulting literature (WebTable 1) was used to (1) describe how environmental, technical, social, economic, and political barriers can hinder success of blue restoration projects, and (2) give solutions to these barriers based on our literature search (Table 1). We further illustrate these solutions utilizing case studies including:
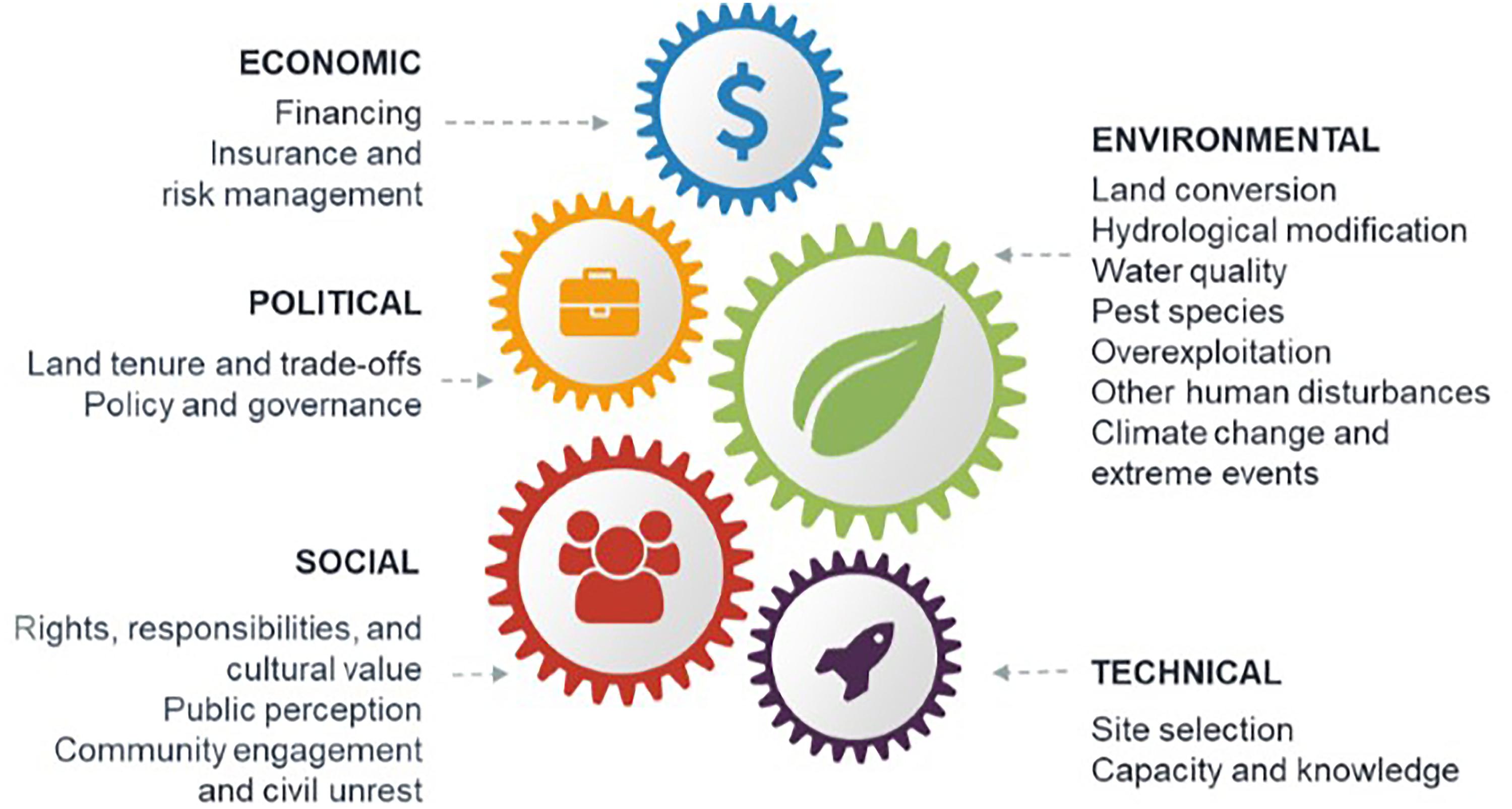
Figure 1. Common barriers to successful blue restoration. Barriers are shown for the restoration of saltmarsh, mangroves, seagrass, shellfish reefs, coral reefs, and kelp.
• Coral reef restoration in Sulawesi, Indonesia, where technical barriers were overcome using new, relatively inexpensive technology (Box 1);
• Mangrove restoration in Sulawesi, Indonesia, where environmental (hydrological) barriers were overcome using creative solutions that were based on sound technical knowledge (Box 2);
• Seagrass restoration in Chesapeake Bay, United States, where technical knowledge was used to increase restoration capacity by implementing seed propagation technology (Box 3); and
• Shellfish restoration in Chesapeake Bay, United States, to illustrate where political barriers were overcome by fostering collaboration between stakeholders (Box 4).
BOX 1. Sulawesi, Indonesia – coral reef restoration.
Ecosystem: Coral reef.
Background: The site (Pulau Badi, South Sulawesi, Indonesia) was damaged by storms, blast-fishing, coral mining, and the construction of a boat channel, resulting in a coral rubble field (Williams et al., 2019). Restoration success was measured against a reference site which was a nearby, undisturbed reef.
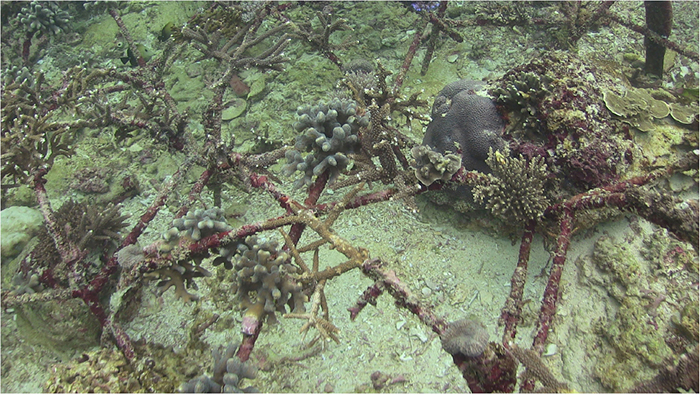
Photo: ‘Spiders’ used to stabilize rubble for coral transplantation.
Barrier 1: Technical – low levels of substrate availability for coral recruits.
Context and solution: The project deployed small, modular, open structures (‘spiders’) to stabilize rubble and to support transplanted coral fragments over 2 years. The structures allowed unrestricted water flow, trapped broken coral fragments and rubble, and stabilized the substratum to support coral recruitment, growth, and diversity. Live coral cover on the structures increased from less than 10% to greater than 60%, although this varied depending on depth, deployment date, location, and disturbances.
BOX 2. Sulawesi, Indonesia – mangrove restoration.
Ecosystem: Mangrove.
Background: Mangrove restoration in two locations – Tiwoho Village, North Sulawesi (site referenced in Brown and Djamaluddin, 2017); and in Tanakeke Island, South Sulawesi (site referenced in Brown et al., 2014). While these sites have a similar history of clearing and land-use conversion, they represent very different conditions for mangrove restoration. Tiwoho is a high productivity mangrove system where the soil is silty and deep, whereas Tanakeke is a low productivity mangrove system on shallow coral sands (Cameron et al., 2019).
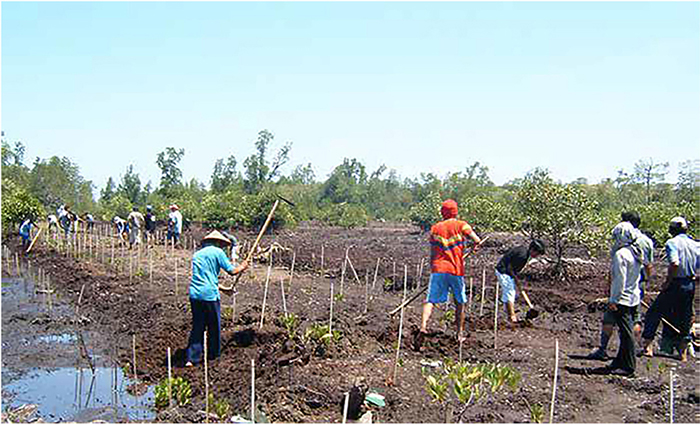
Photo: By Rignolda Djamaluddin of hydrological restoration of mangroves (Brown and Djamaluddin, 2017).
Barrier: Environmental – hydrodynamics.
Context and solutions: Collaboration among scientists at Blue Forests (Yayasan Hutan Biru), Charles Darwin University, and the National University of Singapore, focused on mangrove restoration by implementing Ecological Mangrove Restoration (EMR) (Lewis, 2005). This methodology involves hydrological modifications following an assessment of the current conditions and site-specific needs. Restoration of hydrology included strategic pond wall breaching, creating hand-dug drainage and tidal channels, and creating mounds of substrate to facilitate mangrove colonization in deeper areas of ponds. These techniques simulated the natural conditions to allow mangrove propagules to naturally recruit to the restoration sites. These projects are successful examples of overcoming complicated hydrological challenges with creative solutions.
BOX 3. Chesapeake Bay, United States – seagrass restoration.
Ecosystems: Seagrass
Background: Chesapeake Bay ecosystems have been overexploited and degraded since European settlement over 400 years ago (Cameron et al., 2019). The seagrass, Zostera marina, was lost from 50% of its distribution, followed by 30% decline in the 1990’s (Orth et al., 2012). In response to bay-wide environmental degradation, the Chesapeake Bay Program Partnership was formed in 1983. The Chesapeake Bay Foundation estimated the economic benefits of cleaning up the bay watershed would total US$130 billion annually.
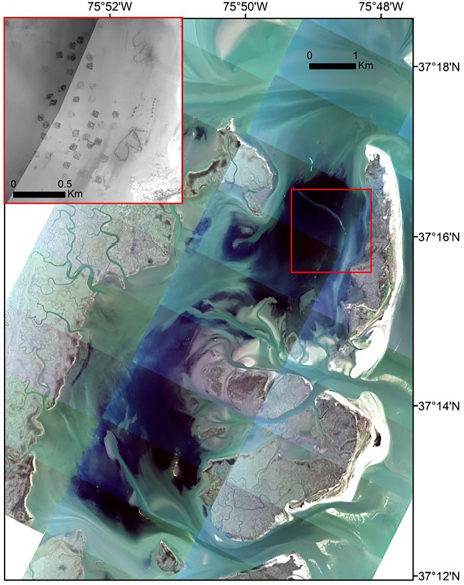
Photo: Zostera marina restoration in South bay, Chesapeake Bay, United States.
Barrier: Technical – seagrass recruitment
Context and solution: Natural recovery of seagrass was slow, despite improvements to water quality. This prompted interventions to assist seagrass recovery. Seed-based restoration provides a greater abundance of genetically diverse propagules. Large-scale restoration using seeds collected from areas adjacent to Chesapeake Bay was initiated in the late 1990s (Orth et al., 2012). This enhanced recruitment above natural levels. Currently, 72 million seeds have been added between 1999 and 2015, to plots ranging in size from 0.01 to 2 ha, totaling 200 ha across four coastal bays. Expansion from these initial plots to approximately 2500 ha of seafloor is attributable to seed dispersal and reproduction from the original plots (Orth and Reeves, 2018, pers. comm.).
BOX 4. Chesapeake Bay, United States – shellfish.
Ecosystems: Shellfish
Background: In Chesapeake Bay, the eastern oyster (Crassostrea virginica) provides valuable services to commercial fisheries, water filtration, and habitat. Over-harvesting, disease, and habitat loss has led to oyster populations <1% of their historic levels (Wilberg et al., 2011). Oyster restoration in the Chesapeake Bay started in 1914 and has led to restoration of 100s of hectares (Wilberg et al., 2011).
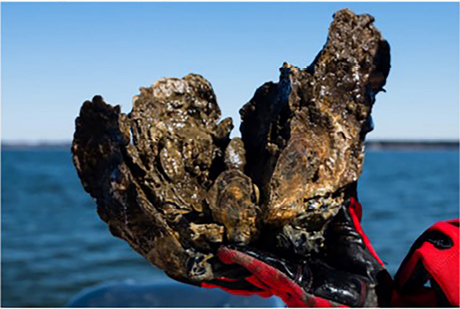
Photo: By Will Parson/Chesapeake Bay Program of clump of oysters from Harris Creek (Chesapeake Bay Program, 2019).
Barrier 1: Political.
Context and solution: Oyster restoration/management in the Chesapeake has been a collaborative and coordinated approach across political jurisdictions. This was driven by a 2009 US Executive Order (no. 13508), which was implemented by the Chesapeake Bay Watershed Agreement (2014), Chesapeake Bay Program (2019). Within this, the Oyster Outcome is to Continually increase finfish and shellfish habitat and water quality benefits from restored oyster populations, restore native oyster habitat and populations in 10 tributaries by 2025 and ensure their protection’ this has catalyzed oyster restoration at scale: by 2018, 289 and 194 hectares had been restored in Maryland and Virginia, respectively. The outcomes of this restoration has been quantified and in Maryland alone, US$51 milion has been invested in oyster restoration, with a fisheries output benefit valued at US$22.3 milion per year, an additional 313 jobs per year, and between US$3-18 million in nitrogen removal value (Chesapeake Bay Program, 2019).
The case studies presented here are examples of success in overcoming barriers and are not intended to be portrayed as representative of the norm in the blue restoration industry. For example, seed propagation is not always as successful in large-scale seagrass restoration as it was in Chesapeake Bay (e.g., only 12% survival on Kangaroo Island in Australia: Tanner et al., 2014). This is due to the relatively young nature of the blue restoration industry, where solution to some barriers have not yet been widely implemented. We expect that more solutions will emerge as the blue restoration industry matures.
Environmental Barriers
Environmental barriers are physical, chemical, biological, or hydrological characteristics that decrease the likelihood of restoration success (Perrow and Davy, 2002). These barriers include land conversion, hydrological modification, poor water quality, pest species, overexploitation, climate change, and extreme weather events (Figure 1).
Land Conversion
Land conversion has been one of the key causes of loss of approximately one third of the world’s saltmarsh, seagrass, and mangroves (Valiela et al., 2001; Gedan et al., 2009; Waycott et al., 2009). Environmental barriers associated with changes in land-use include changes in the structure (compaction) and biogeochemistry of soils (nutrients and other pollutants), which may be difficult to reverse, particularly in the cases of blue ecosystems where the original ecosystem is lost through land reclamation. In cases that do not include land reclamation, solutions that have been identified include adding sediment in saltmarshes where soils and their elevations are inappropriate for plant growth (Berkowitz et al., 2017). The negative impacts of mobilizing pollutants can be minimized through staged reflooding and liming (treating with lime) in acid sulfate soils (Portnoy and Giblin, 1997).
Payments for ecosystem services (including carbon sequestration) have been successfully used to motivate and finance the restoration of converted agricultural landscapes in terrestrial systems, as in China’s Grain-to-Green Program (Chen et al., 2009) and Madagascar’s Ankeniheny-Mantadia-Zahamena Biodiversity Conservation and Restoration Project (Wendland et al., 2010). Carbon finance is applicable to blue ecosystems (Wylie et al., 2016), and methodologies are available for a range of restoration activities for mangroves, saltmarshes, and seagrass (e.g., rewetting landscapes to restore coastal wetlands (Emmer et al., 2015; Needelman et al., 2018). A wide range of projects are emerging to restore mangroves and saltmarshes on converted agricultural and aquaculture landscapes, both for carbon payments, for example Conservation International’s project in Cispatá, Colombia (Bernal et al., 2017), and restoration of saltmarsh in Canada (Wollenberg et al., 2018), and also for supporting biodiversity and other co-benefits, including nutrient cycling (e.g., Land Restoration Fund projects in Queensland, Australia; Queensland Government, 2020).
Hydrological Modification
Degradation of coastal wetlands is often the result of changes in hydrology to support aquaculture and agriculture; for example, drainage, impoundment with seawalls, or excavation (Lewis et al., 2016). Changes to hydrology can cause major alterations to the suitability for plants and animals of blue ecosystems. Mangrove ecosystems can be difficult to restore where aquaculture ponds and channels excavated for shrimp aquaculture result in hydrological modifications of the landscape (Brown et al., 2015). Re-establishing hydrological regimes that are suitable for plant communities has been achieved in a range of saltmarsh settings (Glamore, 2012; Esteves and Williams, 2017), although success has been variable (Wolters et al., 2005). There remain technical barriers to predicting outcomes of hydrological change (e.g., because of difficulties in predicting tidal attenuation in complex environments; Rodríguez et al., 2017) and development of accessible hydrological models is key, although often not possible due to limitations in resources, data, or capacity (Boumans et al., 2002).
Water Quality
Water quality has large effects on how blue ecosystems function and persist, and often preclude successful blue restoration (van Katwijk et al., 2016). Eutrophication (excess nutrient loads resulting in significant reductions in dissolved oxygen) is one of the most prominent cause of seagrass loss (Waycott et al., 2009), and has also contributed to loss of oyster reefs (Beck et al., 2011). Seagrass restoration in the Wadden Sea was more feasible with a higher likelihood of success at sites where water quality issues such as eutrophication and turbidity had been improved in the late 1980s, after extensive seagrass losses had occurred (van Katwijk et al., 2009).
Pest Species
Pest species have caused widespread damage to blue ecosystems, often threatening the success of restoration projects. Pest species can reduce the survival and persistence of restored blue ecosystems. For example, Crown-of-Thorns starfish (Acanthaster planci) and Indo-Pacific lionfish (Pterois volitans) have threatened reef restoration projects in Australia and the Caribbean, respectively (Omori, 2010), green crabs (Carcinus maenas) have reduced survival of restored seagrass (Davis et al., 1998), sea urchins have damaged kelp restoration projects (Watanuki et al., 2010), and the common reed (Phragmites australis) has reduced success of saltmarsh restoration in North American (Silliman and Bertness, 2004). In some cases, the only solution to reducing the impact of pest species on restoration projects is by avoidance through careful site selection (e.g., green crabs in seagrass; Davis et al., 1998). In other cases, manual removal is necessary (e.g. Crown-of-Thorns starfish, Great Barrier Reef, Australia), although this can increase the cost of restoration projects (Johnson et al., 1990). Restoring the biodiversity of blue ecosystems may help resist invasion of pest species, such as in kelp forests (Steneck et al., 2002), while reduced biodiversity is associated with invasions in saltmarsh (e.g., in New England; Silliman and Bertness, 2004). This demonstrates a positive feedback loop between restoration of biodiversity and reduced incidence of pest species.
Overexploitation
In many nations, overharvesting has led to degradation of mangroves and reduction in important ecosystem services, such as coastal protection and stabilization. Restoration is unlikely to be successful unless harvesting of timber from restoration sites is prevented, either through regulation with enforcement or the provision of local people with alternatives resources. Provision of alternative livelihoods has proven a valuable tool to reduce over-exploitation of mangroves, for example in the Mikoko Pamoja mangrove restoration project in Kenya, complementary planting of terrestrial species for timber production reduces pressure on the restored forest (Wylie et al., 2016; Owuor et al., 2019).
Other Human Disturbances
Direct physical damage by human activities can reduce success of restoration projects through increased costs or decreased survival. Physical damage, such as that done by boat propellers and chain moorings, can have a large impact on the success of restoration by reducing the density of seagrass – in some areas to zero (Demers et al., 2013). Eco-mooring systems that prevent damage or designating certain sensitive areas as “low-impact” (i.e., no high-impact moorings), may provide a solution to damage of restoration sites (Cullen-Unsworth and Unsworth, 2016). Implementation of policy that protects seagrass from damage has been successful in Tampa Bay, where areas of restored seagrass were closed to boats with combustion engines, perhaps providing a model for other restoration projects (Stowers et al., 2000).
Larger-scale damage, such as damage to corals by ship groundings, can in some cases be managed with appropriate site selection for restoration (e.g., in the Florida Keys). In this example, areas of high relief did not recover on their own and required restoration, while low-relief, hardground coral sites recovered without the need for assisted restoration. Restoration with appropriate site selection (i.e., that focuses on damaged high-relief habitat) can reduce the cost and increase the feasibility of restoration in cases of ship groundings (Precht et al., 2001).
Climate Change and Extreme Events
Climate change can have a direct impact on blue ecosystems through gradual increased ocean temperatures, sea level rise, and ocean acidification, as well as increasing intensity and frequency of extreme weather events. Restoration projects can be vulnerable to extreme weather events if sites are not chosen carefully to include areas that are less vulnerable (see “Site Selection;” van Katwijk et al., 2016). The projected impacts of climate change should be incorporated into restoration planning, with consideration given to species’ ability to adapt or range shift. Blue ecosystems are diverse and will suffer diverse impacts of climate change and, as such, appropriate restoration techniques and management will depend upon the specific stressors to that system (Erwin, 2008). For example, corals will be impacted by increased temperatures, seagrass and kelps are sensitive to marine heatwaves, while wetland ecosystems may be sensitive to salt water intrusion and sea-level rise [including the impacts of “coastal squeeze” (Mills et al., 2016)].
Modern portfolio theory, which seeks to maximize return and minimize risk, has been applied to selected sites to minimize risks for establishing mangroves under projected climate change scenarios (Runting et al., 2018). A similar approach was used to assess priorities with lowest climatic risks (i.e., risk management) for global investment in conservation of coral reefs (Beyer et al., 2018), but other approaches include species niche modeling with assessment of benefits and costs (Johnston et al., 2002; Adams et al., 2016). Restoration practitioners must consider not only which restoration techniques should be used, but also how effective these are under multiple potential future climate projections, while leaving room in projects for adaptive management of restoration projects (Perry et al., 2015). More knowledge is needed in all blue ecosystems on how restoration practices should change and be adapted to incorporate the effects of climate change (Harris et al., 2006).
Technical Barriers
Technical barriers to blue restoration threaten project success most often when there is poor site selection or limited capacity and knowledge to support restoration projects (Figure 1).
Site Selection
Site selection has been responsible for many failed mangrove restoration projects in low and middle income countries (Lee et al., 2019), where the average survival of restored mangrove seedlings is only 11% (Bayraktarov et al., 2016). However, appropriate site selection is not necessarily an easily solved problem. For example, many failed mangrove plantings were on sites selected to avoid conflicts with private land use (see “Land tenure and trade-offs;” Lewis, 2005; Lovelock and Brown, 2019). Mangrove restoration success has been increased by development of technical guidance including initiatives such as Ecological Mangrove Restoration (EMR; Lewis, 2005) and the UN-led Restoration Opportunities Assessment Methodology (ROAM; Srivastava, 2017). Similar tools are available for shellfish reefs, such as the Ocean Resilience application (The Nature Conservancy, 2018) and the Restoration Guidelines for Shellfish Reefs (Fitzsimons et al., 2019). In coral reefs, matching of coral phenotypic traits with site conditions may lead to increased survival and overall success of restoration projects (Ladd et al., 2018). For example, sites affected by high water temperatures due to climate change could require outplanting of coral genotypes with high thermal tolerance. Further successes may be achieved by strategic placement of coral reef restoration in areas where water currents minimize bleaching of restored corals (Precht, 2006).
Capacity and Knowledge
Limited knowledge of restoration processes and skills can hinder restoration success and implementation.
A solution to a lack of knowledge is increased investment into research and development of blue restoration methods, and training of project personnel. For example, AUD$100 million has been allocated to coral reef restoration research and development on the Great Barrier Reef, which has been focused on technological solutions for climate change impacts, such as the restoration of bleached areas using assisted translocation of coral larvae (RRAP, 2018). This example also underscores an information and investment bias toward blue restoration in nations with high-income economies. For example, Bayraktarov et al. (2016) found that investment is greater in countries with high income economies compared to low/lower middle economy nations for coral (median 282,719 US$ ha–1 cf. 162,455 US$ ha–1) and mangrove restoration (52,006 US$ ha–1 cf. 1,771 US$ ha–1), while in seagrass, saltmarsh, and oyster reef restoration there was no published evidence of investment in low/lower middle income nations. There is limited information on blue restoration from Africa, Asia, and South America. There needs to be increased communication of restoration outside of the developed world (Zhang et al., 2018). Many locally run projects have limited access to communication with restoration experts, and so may waste valuable time and resources developing restoration techniques that work, or the project may suffer from reduced success as a result. Information-sharing and publicly available best-practice guidelines may reduce this limitation.
Social Barriers
Social approval and participation in blue restoration is often overlooked but can be central to successful implementation of large-scale blue restoration (Figure 1).
Rights, Responsibilities, and Cultural Value
Restoration has the potential to greatly impact the human populations living in proximity to restoration projects and those who are dependent on the habitats being restored. As such, consideration of the rights and responsibilities of coastal peoples, and inclusion of all the relevant stakeholders, is crucial to blue restoration success (Lundquist and Granek, 2005).
The cultural value placed on blue ecosystems has the potential to cause conflict with restoration project objectives, particularly where indigenous communities have rights to resource use (e.g., burning mangrove wood for fuel; Torpey-Saboe et al., 2015). For example, the use of mangrove tree resources was criminalized in Para state, northern Brazil (Glaser et al., 2003). The ban was aimed at commercial users; however, it also restricted access to local communities who required wood for their subsistence. The ban created social conflict, resource and economic insecurity, and threatened the local use and restoration of the mangrove habitat (Glaser et al., 2003). Similar concerns have been raised by REDD + (Reduced Emissions from Deforestation and Degradation) projects for mangrove conservation, noting that restoration may compromise access to land and livelihoods by limiting indigenous and local people from traditional use of mangrove land (Griffiths and Martone, 2008). Cultural harvest (i.e., resource use by local or traditional communities) is one of the key cultural values of restored habitats (Wehi and Lord, 2017). Inclusion of sustainable harvest within restored habitats may increase the cultural value of restoration projects and thereby increase community support for restoration projects (Wehi and Lord, 2017). In Puget Sound, in the United States, people’s “sense of place” was an important motivation for resident support of restoration and sustainable harvesting of shellfish (Poe et al., 2016). In North America’s Laurentian Great Lakes, cultural ecosystem services from restoration have guided investment of more than US$1.5 billion into restoration, which increased uptake and success of restoration projects (Allan et al., 2015).
Research into the cultural ecosystem services provided by blue ecosystems is biased toward examples from nations with developed economies and thus does not often include cultural use and value placed on blue ecosystems beyond western values (Rodrigues et al., 2017). The inclusion of local peoples in the planning and implementation of restoration projects has enhanced the success of mangrove restoration projects in Indonesia (Brown, 2017).
Public Perception
Public perception of the impacts of restoring ecosystems has large implications for restoration of blue ecosystems. In Florida, mosquitoes associated with saltmarsh and mangrove forests can become abundant, which has negative impacts on human health and well-being (Rey et al., 2012). Due to negative public perception of saltmarshes and mangroves, because of their association with mosquitoes and other biting insects, much of the historic areas of coastal saltmarsh were drained and mangroves impounded to reduce mosquito numbers. Restoration of coastal wetlands therefore had to overcome the concerns of the public, and thus as a solution, restoration of these marsh areas was paired with mosquito control (Rey et al., 2012).
Public perceptions of the benefits of blue ecosystems are likely to differ from those quantified by ecosystem service valuation (Costanza et al., 1997), where the public values recreation and access to food/fuel over services such as increasing water quality. In order to bring differing perspectives together, it is important to engage a variety of stakeholders (e.g., indigenous and community groups) when planning and implementing blue restoration projects (Lundquist and Granek, 2005).
Community Engagement and Civil Unrest
Civil unrest and conflict can prevent restoration efforts due to weak governance, corruption, and disputes over land tenure (Herr et al., 2019). For example, upstream dam construction and prolonged drought in Senegal has stimulated estuarine mangrove restoration, which has led to a range of social conflicts (Manikowski and Strapasson, 2016; Cormier-Salem, 2017). Non-government organizations have initiated large scale restoration of mangroves, which has had co-benefits of restoring rice and seafood sources for local livelihoods (Livelihoods funds, 2019), although social conflict remains over the carbon financing used for the project (Cormier-Salem, 2017).
Active community engagement in blue restoration projects can combat the negative influence of social conflict and be mutually beneficial to both restoration practitioners and other stakeholders in the community. For example, the effectiveness of strong community engagement for successful restoration was demonstrated in Hawaiian coral restoration, where coral reef restoration benefited the local community by creating jobs, reviving cultural practices, harvest and use of coral-associated algae, increased awareness of the marine environment, and overall increased adaptive capacity for global change (Kittinger et al., 2013).
Other strategies to increase community engagement, such as citizen science, use volunteers to support river restoration activities (Huddart et al., 2016), seagrass (Disney et al., 2017), and coral reef restoration (Hesley et al., 2017). The use of citizen science programs that engage with volunteers can reduce levels of investment needed for monitoring of restoration projects. While there may be a trade-off in data quality and consistency when data collection is done by many volunteers, citizen science can contribute to project success by improving cost effectiveness as well as increasing public engagement and support (Dickinson et al., 2012).
Economic Barriers
Financing and risk management of blue restoration are major barriers to implementation of projects at a meaningful scale (Figure 1). Financing the costs of all components of projects (including capital and operating costs) depends on funding availability, financial benefit, risk management (e.g., through feasibility estimates or insurance), and a stable political climate (Vanderklift et al., 2019).
Financing
Lack of long-term funding for restoration has been identified as one of the major barriers to restoration success (Iftekhar et al., 2017). Most restoration projects are limited to short-term funding (i.e., less than 3 years) and, as a result of this short duration, successful establishment, maintenance, and ongoing monitoring is significantly restricted. This lack of long-term funding has potentially contributed to the relatively low rates of success in blue restoration (Bayraktarov et al., 2016). Financing for blue restoration has historically been acquired through a multitude of ways, including valuation of ecosystem services, carbon financing (e.g., REDD +), debt-for-nature-swaps, green taxes, biodiversity offsets, payment for ecosystem services, impact investments, green bonds, and parametric insurance (Herr et al., 2019). Organizations that have provided finance include: (1) private for-profit, (2) private non-profit, (3) public sector expenditure, and (4) multilateral and bilateral donors (Srivastava, 2017). In some cases, government policy is developed to support financing of blue restoration. For example, in Kenya, a Climate Compatible Development policy was applied to mangrove forests in the Kwale District, which uses valuation of mangroves and estimated mangrove loss to predict the future benefits of sustainable mangrove management, including restoration (Herr et al., 2019).
Insurance and Risk Management
Investment in blue restoration depends on reducing and managing the risk of failure, since investors are generally risk-averse and restoration success (quantified as survival of restored organisms) varies widely from 0 to 65% (Bayraktarov et al., 2016). Reduction of risks can be achieved through insurance in situations where it is possible to charge private stakeholders for ecosystem services (Colgan et al., 2017). The insurance industry is developing tools for managing environmental risks, such as catastrophe bonds, that could be used to manage risks associated with large-scale restoration projects (Colgan et al., 2017). Insurance of mangroves, based on the coastal protection they provide, has been found to be technically feasible in Australia, but thus far has not been implemented (Bell and Lovelock, 2013). Risk management for valuable coral reef habitat occurred in Quintana Roo, Mexico, through parametric insurance where the coral reef was insured for restoration by a private-public partnership (Lab, 2017). The insured party (i.e., the hotel owners) benefit from the tourism income provided by a healthy reef, while the benefit for blue restoration is that if the coral reef is damaged (for example by storms), then restoration programs will be paid out (Lab, 2017). Thus, insuring blue ecosystems for restoration offers a solution to management of risk, and could improve restoration success through increases in the funding available for restoration projects including post-establishment maintenance and ongoing monitoring.
Political Barriers
Political barriers to implementation of blue restoration interventions, such as trade-offs between conflicting objectives, and issues with political delay of approvals and permits, can be significant barriers (Figure 1).
Land Tenure and Trade-Offs
Land conversion to agriculture, aquaculture, and urbanization often results from a change in land ownership from public or indigenously managed land to private land (Esteves and Williams, 2017). In mangroves converted to aquaculture, private landowners do not necessarily live locally and thus may not directly benefit from ecosystem services arising from mangrove restoration, while the local community may feel disempowered by land-use regimes that do not consider community involvement (Lovelock and Brown, 2019). Restoration of blue ecosystems on common land reduces the social barriers, but can result in over-exploitation (Crooks et al., 2011). Land-tenure bottlenecks can result in plantings in inappropriate, low intertidal areas, leading to high failure rates (Lee et al., 2019). One of the solutions to land tenure barriers is payment for ecosystem services of restored habitat to multiple stakeholders, which may include separating carbon and fisheries rights from land rights (Bell-James and Lovelock, 2019).
Conflicting objectives for usage of restored ecosystems (e.g., recreation, fisheries, carbon or conservation) can also lead to project failure. In the Chesapeake Bay, native oysters have been restored but trade-offs occur where fisheries want to harvest the restored oyster stocks, directly inhibiting restoration efforts, and ultimately leading to restoration failure (Beck et al., 2011). A solution to this is early stakeholder engagement and consultation, resulting in agreeable compromise, where oyster stocks (for example) are harvested sustainably, allowing for increases in the cultural value of the restoration project, while still achieving the environmental goals (see Box 4).
Policy and Governance
Political issues that may delay or prevent approvals and permits can delay or prevent blue restoration projects. For example, governments can alter national or regional planning strategies thereby reducing priority for restoration, reducing available funds, or relaxing regulations protecting restored sites (Vanderklift et al., 2019). Despite promotion of blue restoration through national policies (e.g., REDD +), they can be ineffective when not consistently applied across landscapes, or due to a lack of political will (Vanderklift et al., 2019). In Vietnam and Madagascar (Markets and Mangroves project) the planning, time and resources required to implement a REDD + project was so extensive that the REDD + model was discarded (Wylie et al., 2016). Failure of the REDD + scheme in Vietnam was due to the length of time it took to fulfill the requirements of the scheme, whereas in Madagascar government policy, which does not classify mangroves as forests, was the cause (Wylie et al., 2016).
The governance of blue restoration has rarely been explored in literature, but lessons from terrestrial restoration indicate that blue restoration would benefit from considering the cultural context of the restoration, incorporating social science into restoration planning, collaborating with interdisciplinary stakeholders, and combining knowledge from scientific and local experts (France, 2016). However, governance of blue restoration differs from terrestrial restoration in some key ways. For example, land tenure rights are more complicated in blue ecosystems, since restoration sites may not be privately owned, leaving responsibility for restoration projects in the hands of communities and governments. Threats and impacts on blue ecosystems are different to those in the terrestrial environment, and feasibility of blue restoration is reduced by higher costs and risk of failure than in terrestrial restoration. Finally, blue ecosystems may take longer to recover than terrestrial ecosystems, leading to a slower rate of returns and ultimately reduced investment (Bell-James, 2016). Governments and non-government organizations could encourage blue restoration through subsidies, partnerships, or payment for ecosystems services schemes to overcome these barriers (e.g., for blue carbon; Bell-James, 2016).
Conclusion
Blue restoration can make an important contribution to meeting global environmental targets. Blue restoration is a less established field than terrestrial restoration, which has moved on from simple interventions at local scales and based on a static environment, to more advanced interventions acknowledging and planning for changing environments. It is a field that has been relatively under-funded, and predominantly limited to smaller-scale and somewhat experimentally localized projects. Conversely, terrestrial restoration is trending toward large-scale restoration aiming to fulfill multiple goals (e.g., conservation, ecosystem function, climate change mitigation and adaptation), with an increased sensitivity to the societal context of restoration (Perring et al., 2015). The success of blue restoration depends upon progressing quickly along this trajectory, while acknowledging unique characteristics of blue ecosystems (Bell-James, 2016). Understanding which restoration interventions will be most successful and where, is a crucial priority for the field (Perring et al., 2015). For example, the ecological, technical, and financial feasibility of a project is redundant if socio-political contexts make projects unworkable (Lovelock and Brown, 2019). Numerous barriers are regularly encountered during blue restoration projects and are not confined to a single ecosystem type. Understanding these barriers, as well as utilizing the knowledge gained from projects that have approached innovative and multi-faceted solutions to overcoming those barriers, is key to improving the future success of blue restoration, as well as the ability for restoration projects to be scaled up. Successful scaling-up of blue restoration requires an inter-disciplinary approach that addresses the barriers outlined in this paper and doing so is the only solution to meeting global restoration goals. Demonstrations of blue restoration projects where solutions to common barriers have been discovered can inspire, generate innovative solutions, and provide guidance to the global blue restoration community.
Author Contributions
PS-S conceived of and initiated the workshop that all authors contributed to and attended. The bulk of the writing and figure creation was done by PS-S. All authors contributed to the development and writing of the manuscript for their area of expertise. The case studies were researched and written by groups within the author list, and then the overall manuscript was edited by all authors.
Funding
We would like to acknowledge funding to enable the workshop from The University of Queensland – Centre of Excellence for Environmental Decisions. NW was funded through the Australian Government’s National Environment Sciences Programme (Tropical Water Quality Hub) Project 3.3.2. JP was funded through the Australian Government’s National Environmental Sciences Programme (Marine Biodiversity Hub).
Conflict of Interest
The authors declare that the research was conducted in the absence of any commercial or financial relationships that could be construed as a potential conflict of interest.
Supplementary Material
The Supplementary Material for this article can be found online at: https://www.frontiersin.org/articles/10.3389/fmars.2020.541700/full#supplementary-material
References
Adams, M. P., Saunders, M. I., Maxwell, P. S., Tuazon, D., Roelfsema, C. M., Callaghan, D. P., et al. (2016). Prioritizing localized management actions for seagrass conservation and restoration using a species distribution model. Aqu. Conserv. Mar. Freshw. Ecosyst. 26, 639–659. doi: 10.1002/aqc.2573
Allan, J. D., Smith, S. D., McIntyre, P. B., Joseph, C. A., Dickinson, C. E., Marino, A. L., et al. (2015). Using cultural ecosystem services to inform restoration priorities in the Laurentian Great Lakes. Front. Ecol. Environ. 13:418–424. doi: 10.1890/140328
Alleman, L. K., and Hester, M. W. (2011). Refinement of the fundamental niche of black mangrove (Avicennia germinans) seedlings in Louisiana: applications for restoration. Wetlands Ecol. Manag. 19, 47–60. doi: 10.1007/s11273-010-9199-6
Arias-Ortiz, A., Serrano, O., Masqué, P., Lavery, P. S., Mueller, U., Kendrick, G. A., et al. (2018). A marine heatwave drives massive losses from the world’s largest seagrass carbon stocks. Nat. Clim. Change 8, 338–344. doi: 10.1038/s41558-018-0096-y
Barbier, E. B., Hacker, S. D., Kennedy, C., Koch, E. W., Stier, A. C., and Silliman, B. R. (2011). The value of estuarine and coastal ecosystem services. Ecol. Monogr. 81, 169–193.
Bayraktarov, E., Saunders, M. I., Abdullah, S., Mills, M., Beher, J., Possingham, H. P., et al. (2016). The cost and feasibility of marine coastal restoration. Ecol. Appl. 26, 1055–1074. doi: 10.1890/15-1077
Beck, M. W., Brumbaugh, R. D., Airoldi, L., Carranza, A., Coen, L. D., Crawford, C., et al. (2011). Oyster reefs at risk and recommendations for conservation, restoration, and management. BioScience 61, 107–116. doi: 10.1525/bio.2011.61.2.5
Bell, J., and Lovelock, C. E. (2013). Insuring mangrove forests for their role in mitigating coastal erosion and storm-surge: an Australian case study. Wetlands 33, 279–289. doi: 10.1007/s13157-013-0382-4
Bell-James, J. (2016). Developing a framework for blue carbon in Australia: legal and policy considerations. UNSWLJ 39:1583.
Bell-James, J., and Lovelock, C. E. (2019). Legal barriers and enablers for reintroducing tides: an Australian case study in reconverting ponded pasture for climate change mitigation. Land Use Policy 88:104192. doi: 10.1016/j.landusepol.2019.104192
Berkowitz, J., VanZomeren, C., and Piercy, C. (2017). Marsh restoration using thin layer sediment addition: initial soil evaluation. Wetland Sci. Pract. 34, 13–17.
Bernal, B., Sidman, G., and Pearson, T. (2017). Assessment of Mangrove Ecosystems in Colombia and Their Potential for Emissions Reductions and Restoration. Morrilton, AR: Winrock International.
Beyer, H. L., Kennedy, E. V., Beger, M., Chen, C. A., Cinner, J. E., Darling, E. S., et al. (2018). Risk-sensitive planning for conserving coral reefs under rapid climate change. Conserv. Lett. 11:e12587. doi: 10.1111/conl.12587
Boumans, R. M. J., Burdick, D. M., and Dionne, M. (2002). Modeling habitat change in salt marshes after tidal restoration. Restorat. Ecol. 10, 543–555. doi: 10.1046/j.1526-100X.2002.02032.x
Brown, B. (2017). “Ecological rehabilitation in mangrove systems: the evolution of the practice and the need for strategic reform of policy and planning,” in Routledge Handbook of Ecological and Environmental Restoration, eds S. K. Allison and S. D. Murphy (London: Routledge), 295–311. doi: 10.4324/9781315685977-20
Brown, B., and Djamaluddin, R. (2017). A site History & Field Guide for Ecological Mangrove Rehabilitation in Tiwoho Village, Bunaken National Marine Park, North Sulawesi, Indonesia. Indonesia: Center for International Forestry Research (CIFOR).
Brown, B., Fadillah, R., Nurdin, Y., and Soulsby, I. (2014). Community based ecological mangrove rehabilitation (cbemr) in indonesia: From small (12–33 ha) to medium scales (400 ha) with pathways for adoption at larger scales (>5000 ha). Sapiens 7:1–12.
Brown, B., Yuniati, W., Ahmad, R., and Soulsby, I. (2015). “Observations of natural recruitment and human attempts at mangrove rehabilitation after seismic (Tsunami and earthquake) events in Simeulue Island and Singkil Lagoon, Aceh, Indonesia,” in Advances in Natural and Technological Hazards Research, eds V. Santiago-Fandiño, Y. Kontar, and Y. Kaneda (Cham: Springer).
Cameron, C., Hutley, L. B., Friess, D. A., and Brown, B. (2019). Community structure dynamics and carbon stock change of rehabilitated mangrove forests in sulawesi, indonesia. Ecol. Appl. 29:e01810. doi: 10.1002/eap.1810
CBD (2010). Strategic Plan for Biodiversity 2011-2020, including Aichi Biodiversity Targets. Nagoya: Secretariat of the Convention on Biological Diversity.
Chan, A., and Hodgson, P. A. (2017). “A systematic analysis of blast fishing in South-East Asia and possible solutions,” in Proceedings of the 2017 IEEE Underwater Technology (UT) Busan, 1–6.
Chesapeake Bay Watershed Agreement (2014) Chesapeake Bay Watershed Agreement. (accessed December, 2019).
Chesapeake Bay Program (2019). Chesapeake Progress. Available online at: https://www.chesapeakeprogress.com/
Chen, X., Lupi, F., He, G., Ouyang, Z., and Liu, J. (2009). Factors affecting land reconversion plans following a payment for ecosystem service program. Biol. Conserv. 142, 1740–1747. doi: 10.1016/j.biocon.2009.03.012
Colgan, C., Beck, M., and Narayan, S. (2017). Financing Natural Infrastructure for Coastal Flood Damage Reduction. London: Lloyd’s Tercentenary Research Foundation.
Cormier-Salem, M.-C. (2017). Let the women harvest the mangrove. Carbon policy, and environmental injustice. Sustainability 9:1485. doi: 10.3390/su9081485
Costanza, R., d’Arge, R., De Groot, R., Farber, S., Grasso, M., Hannon, B., et al. (1997). The value of the world’s ecosystem services and natural capital. Nature 387:253.
Crooks, S., Herr, D., Tamelander, J., Laffoley, D., and Vandever, J. (2011). Mitigating Climate Change Through Restoration and Management of Coastal Wetlands and Near-Shore Marine Ecosystems: Challenges and Opportunities. Washington, DC: World Bank.
Cullen-Unsworth, L. C., and Unsworth, R. K. (2016). Strategies to enhance the resilience of the world’s seagrass meadows. J. Appl. Ecol. 53, 967–972. doi: 10.1111/1365-2664.12637
Davis, R. C., Short, F. T., and Burdick, D. M. (1998). Quantifying the effects of green crab damage to eelgrass transplants. Restorat. Ecol. 6, 297–302. doi: 10.1046/j.1526-100x.1998.00634.x
Demers, M.-C. A., Davis, A. R., and Knott, N. A. (2013). A comparison of the impact of ‘seagrass-friendly’boat mooring systems on Posidonia australis. Mar. Environ. Res. 83, 54–62. doi: 10.1016/j.marenvres.2012.10.010
Dickinson, J. L., Shirk, J., Bonter, D., Bonney, R., Crain, R. L., Martin, J., et al. (2012). The current state of citizen science as a tool for ecological research and public engagement. Front. Ecol. Environ. 10:291–297. doi: 10.1890/110236
Disney, J. E., Fox, E. L., Farrell, A., LeDuc, C., and Bailey, D. (2017). “Engagement in marine conservation through citizen science: a community-based approach to eelgrass restoration in Frenchman Bay, Maine, USA,” in Citizen Science for Coastal and Marine Conservation, eds J. A. Cigliano, and H. R. Ballard (Abingdon: Routledge), 171–195.
Emmer, I., Needelman, B., Emmett-Mattox, S., Crooks, S., Megonigal, P., Myers, D., et al. (2015). Methodology for Tidal Wetland and Seagrass Restoration. Washington, DC: Verified Carbon Standard.
Erwin, K. L. (2008). Wetlands and global climate change: the role of wetland restoration in a changing world. Wetlands Ecol. Manag. 17:71. doi: 10.1007/s11273-008-9119-1
Esteves, L. S., and Williams, J. J. (2017). “Managed realignment in Europe: a synthesis of methods, achievements and challenges,” in Living Shorelines: The Science and Management of Nature-based Coastal Protection, eds D. M. Bilkovic, M. M. Mitchell, J. D. Toft, and M. K. La Peyre (Boca Raton, FL: CRC Press), 157–180. doi: 10.1201/9781315151465-11
Ferrol-Schulte, D., Gorris, P., Baitoningsih, W., Adhuri, D. S., and Ferse, S. C. A. (2015). Coastal livelihood vulnerability to marine resource degradation: a review of the Indonesian national coastal and marine policy framework. Mar. Policy 52, 163–171. doi: 10.1016/j.marpol.2014.09.026
Fitzsimons, J., Branigan, S., Brumbaugh, R. D., McDonald, T., and Zu Ermgassen, P. S. E. (2019). Restoration Guidelines for Shellfish Reefs. Arlington VA: Society for Ecological Restoration.
France, R. L. (2016). From land to sea: governance-management lessons from terrestrial restoration research useful for developing and expanding social-ecological marine restoration. Ocean Coast. Manag. 133, 64–71. doi: 10.1016/j.ocecoaman.2016.08.022
Gedan, K. B., Silliman, B. R., and Bertness, M. D. (2009). Centuries of human-driven change in salt marsh ecosystems. Annu. Rev. Mar. Sci. 1, 117–141. doi: 10.1146/annurev.marine.010908.163930
Glamore, W. C. (2012). “Incorporating innovative engineering solutions into tidal restoration studies,” in Tidal Marsh Restoration, eds C. T. Roman and D. M. Burdick (Washington, DC: Island Press), 277–295. doi: 10.5822/978-1-61091-229-7_17
Glaser, M., Berger, U., and Macedo, R. (2003). Local vulnerability as an advantage: mangrove forest management in Pará state, north Brazil, under conditions of illegality. Reg. Environ. Change 3, 162–172. doi: 10.1007/s10113-003-0057-4
GMA (2019). Global Mangrove Alliance [Online]. Available online at: http://www.mangrovealliance.org/gma/ (accessed September 2019)
Griffiths, T., and Martone, F. (2008). Seeing’REDD’: Forests, Climate Change Mitigation and the Rights of Indigenous Peoples and Local Communities. Moreton-in-Marsh: Forest Peoples Programme.
Harris, J. A., Hobbs, R. J., Higgs, E., and Aronson, J. (2006). Ecological restoration and global climate change. Restorat. Ecol. 14, 170–176.
Herr, D., Blum, J., Himes-Cornell, A., and Sutton-Grier, A. (2019). An analysis of the potential positive and negative livelihood impacts of coastal carbon offset projects. J. Environ. Manage. 235, 463–479. doi: 10.1016/j.jenvman.2019.01.067
Hesley, D., Burdeno, D., Drury, C., Schopmeyer, S., and Lirman, D. (2017). Citizen science benefits coral reef restoration activities. J. Nat. Conserv. 40, 94–99. doi: 10.1016/j.jnc.2017.09.001
Huddart, J. E. A., Thompson, M. S. A., Woodward, G., and Brooks, S. J. (2016). Citizen science: from detecting pollution to evaluating ecological restoration. Wiley Interdiscipl. Rev. Water 3, 287–300. doi: 10.1002/wat2.1138
Hughes, T. P., Barnes, M. L., Bellwood, D. R., Cinner, J. E., Cumming, G. S., Jackson, J. B., et al. (2017). Coral reefs in the Anthropocene. Nature 546:82.
Iftekhar, M. S., Polyakov, M., Ansell, D., Gibson, F., and Kay, G. M. (2017). How economics can further the success of ecological restoration. Conserv. Biol. 31, 261–268. doi: 10.1111/cobi.12778
Johnson, D., Moran, P., and Driml, S. (1990). Evaluation of a crown-of-thorns starfish (Acanthaster planci) control program at Grub Reef (central Great Barrier Reef). Coral Reefs 9, 167–171. doi: 10.1007/bf00258231
Johnston, R. J., Magnusson, G., Mazzotta, M. J., and Opaluch, J. J. (2002). Combining economic and ecological indicators to prioritize salt marsh restoration actions. Am. J. Agric. Econ. 84, 1362–1370. doi: 10.1111/1467-8276.00403
Kittinger, J. N., Bambico, T. M., Minton, D., Miller, A., Mejia, M., Kalei, N., et al. (2013). Restoring ecosystems, restoring community: socioeconomic and cultural dimensions of a community-based coral reef restoration project. Reg. Environ. Change 16, 301–313. doi: 10.1007/s10113-013-0572-x
Konnerup, D., Betancourt-Portela, J. M., Villamil, C., and Parra, J. P. (2014). Nitrous oxide and methane emissions from the restored mangrove ecosystem of the Ciénaga Grande de Santa Marta, Colombia. Estuar. Coast. Shelf Sci. 140, 43–51. doi: 10.1016/j.ecss.2014.01.006
Krumhansl, K. A., Okamoto, D. K., Rassweiler, A., Novak, M., Bolton, J. J., Cavanaugh, K. C., et al. (2016). Global patterns of kelp forest change over the past half-century. Proc. Natl. Acad. Sci. U.S.A. 113, 13785–13790.
Ladd, M. C., Miller, M. W., Hunt, J. H., Sharp, W. C., and Burkepile, D. E. (2018). Harnessing ecological processes to facilitate coral restoration. Front. Ecol. Environ. 16:239–247. doi: 10.1002/fee.1792
Lee, S. Y., Hamilton, S., Barbier, E. B., Primavera, J., and Lewis, R. R. (2019). Better restoration policies are needed to conserve mangrove ecosystems. Nat. Ecol. Evol. 3:870. doi: 10.1038/s41559-019-0861-y
Lewis, R. R. III (2005). Ecological engineering for successful management and restoration of mangrove forests. Ecol. Eng. 24, 403–418. doi: 10.1016/j.ecoleng.2004.10.003
Lewis, R. R. III, Milbrandt, E. C., Brown, B., Krauss, K. W., Rovai, A. S., and Beever, J. W. III, et al. (2016). Stress in mangrove forests: early detection and preemptive rehabilitation are essential for future successful worldwide mangrove forest management. Mar. Pollut. Bull. 109, 764–771. doi: 10.1016/j.marpolbul.2016.03.006
Livelihoods funds (2019). Senegal: the Largest Mangrove Restoration Programme in the World [Online]. Available online at: http://www.livelihoods.eu/projects/oceanium-senegal/ (accessed on September 2019)
Lovelock, C. E., and Brown, B. M. (2019). Land tenure considerations are key to successful mangrove restoration. Nat. Ecol. Evol. 3, 1135–1135. doi: 10.1038/s41559-019-0942-y
Lundquist, C. J., and Granek, E. F. (2005). Strategies for successful marine conservation: integrating socioeconomic, political, and scientific factors. Conserv. Biol. 19, 1771–1778. doi: 10.1111/j.1523-1739.2005.00279.x
Manikowski, S., and Strapasson, A. (2016). Sustainability assessment of large irrigation dams in Senegal: a cost-benefit analysis for the Senegal River Valley. Front. Environ. Sci. 4:18. doi: 10.3389/fenvs.2016.00018
Mills, M., Leon, J. X., Saunders, M. I., Bell, J., Liu, Y., O’Mara, J., et al. (2016). Reconciling development and conservation under coastal squeeze from rising sea level. Conserv. Lett. 9, 361–368. doi: 10.1111/conl.12213
Murray, N. J., Phinn, S. R., DeWitt, M., Ferrari, R., Johnston, R., Lyons, M. B., et al. (2019). The global distribution and trajectory of tidal flats. Nature 565:222. doi: 10.1038/s41586-018-0805-8
Needelman, B. A., Emmer, I. M., Emmett-Mattox, S., Crooks, S., Megonigal, J. P., Myers, D., et al. (2018). The science and policy of the verified carbon standard methodology for tidal wetland and seagrass restoration. Estuar. Coasts 41, 2159–2171. doi: 10.1007/s12237-018-0429-0
Omori, M. (2010). Degradation and restoration of coral reefs: experience in Okinawa. Japan. Mar. Biol. Res. 7, 3–12. doi: 10.1080/17451001003642317
Orth, R. J., and Reeves, S. (ed.). (2018). Chesapeake Bay Personal Communication. Carlton, VIC: The Nature Conservancy.
Orth, R. J., Moore, K. A., Marion, S. R., Wilcox, D. J., and Parrish, D. B. (2012). Seed addition facilitates eelgrass recovery in a coastal bay system. Mar. Ecol. Prog. Ser. 448, 177–195. doi: 10.3354/meps09522
Owuor, M. A., Icely, J., and Newton, A. (2019). Community perceptions of the status and threats facing mangroves of Mida Creek, Kenya: implications for community based management. Ocean Coast. Manag. 175, 172–179. doi: 10.1016/j.ocecoaman.2019.03.027
Perring, M. P., Standish, R. J., Price, J. N., Craig, M. D., Erickson, T. E., Ruthrof, K. X., et al. (2015). Advances in restoration ecology: rising to the challenges of the coming decades. Ecosphere 6, 1–25.
Perrow, M. R., and Davy, A. J. (2002). Handbook of Ecological Restoration. Cambridge: Cambridge University Press.
Perry, L. G., Reynolds, L. V., Beechie, T. J., Collins, M. J., and Shafroth, P. B. (2015). Incorporating climate change projections into riparian restoration planning and design. Ecohydrology 8, 863–879. doi: 10.1002/eco.1645
Poe, M. R., Donatuto, J., and Satterfield, T. (2016). “Sense of Place”: human wellbeing considerations for ecological restoration in puget sound. Coast. Manag. 44, 409–426. doi: 10.1080/08920753.2016.1208037
Portnoy, J. W., and Giblin, A. E. (1997). Biogeochemical effects of seawater restoration to diked salt marshes. Ecol. Appl. 7, 1054–1063. doi: 10.1890/1051-0761(1997)007%5B1054:beosrt%5D2.0.co;2
Precht, W. F., Aronson, R. B., and Swanson, D. W. (2001). Improving scientific decision-making in the restoration of ship-grounding sites on coral reefs. Bull. Mar. Sci. 69, 1001–1012.
Queensland Government (2020). The Land Restoration Fund [Online]. Available online at: https://www.qld.gov.au/environment/climate/climate-change/land-restoration-fund (accessed on January 2020)
Rey, J. R., Carlson, D., and Brockmeyer, R. (2012). Coastal wetland management in Florida: environmental concerns and human health. Wetlands Ecol. Manag. 20, 197–211. doi: 10.1007/s11273-011-9235-1
Rodrigues, J. G., Conides, A. J., Rivero Rodriguez, S., Raicevich, S., Pita, P., Kleisner, K. M., et al. (2017). Marine and coastal cultural ecosystem services: knowledge gaps and research priorities. One Ecosyst. 2:e12290. doi: 10.3897/oneeco.2.e12290
Rodríguez, J. F., Saco, P. M., Sandi, S., Saintilan, N., and Riccardi, G. (2017). Potential increase in coastal wetland vulnerability to sea-level rise suggested by considering hydrodynamic attenuation effects. Nat. Commun. 8:16094.
RRAP (2018). Reef Restoration and Adaptation Program [Online]. Available online at: https://www.gbrrestoration.org/ (accessed September 2019)
Runting, R. K., Beyer, H. L., Dujardin, Y., Lovelock, C. E., Bryan, B. A., and Rhodes, J. R. (2018). Reducing risk in reserve selection using Modern Portfolio theory: coastal planning under sea-level rise. J. Appl. Ecol. 55, 2193–2203. doi: 10.1111/1365-2664.13190
Salvador, E. (2018). UN Decade of Ecosystem Restoration 2021–2030: Initiative Proposed by El Salvador with the Support of Countries from the Central American Integration System (SICA)-Concept Note. Guatemala: MARN.
SER (2004). The SER International Primer on Ecological Restoration, 1st Edn. Washington, DC: Society for Ecological Restoration International.
Silliman, B. R., and Bertness, M. D. (2004). Shoreline development drives invasion of Phragmites australis and the loss of plant diversity on New England salt marshes. Conserv. Biol. 18, 1424–1434. doi: 10.1111/j.1523-1739.2004.00112.x
Srivastava, R. (2017). Restoration Opportunities Assessment Methodology (ROAM) for Landscape Stewardship From Natural Disasters: A Way Forward. Gland: IUCN.
Steneck, R. S., Graham, M. H., Bourque, B. J., Corbett, D., Erlandson, J. M., Estes, J. A., et al. (2002). Kelp forest ecosystems: biodiversity, stability, resilience and future. Environ. Conserv. 29, 436–459. doi: 10.1017/s0376892902000322
Stowers, J. F., Fehrmann, E., and Squires, A. (2000). “Seagrass scarring in Tampa Bay: impact analysis and management options,” in Proceedings of a Symposium seagrass management: it’s not just nutrients! St. Petersburg, FL.
Tanner, J. E., Irving, A. D., Fernandes, M., Fotheringham, D., McArdle, A., and Murray-Jones, S. (2014). Seagrass rehabilitation off metropolitan Adelaide: a case study of loss, action, failure and success. Ecol. Manag. Restor. 15, 168–179. doi: 10.1111/emr.12133
Torpey-Saboe, N., Andersson, K., Mwangi, E., Persha, L., Salk, C., and Wright, G. (2015). Benefit sharing among local resource users: the role of property rights. World Dev. 72, 408–418. doi: 10.1016/j.worlddev.2015.03.005
UN (2019). United Nations Decade of Ocean Science for Sustainable Development (2021-2030) [Online]. Available online at: http://www.oceandecade.org/ (accessed on September 2019)
Valiela, I., Bowen, J. L., and York, J. K. (2001). Mangrove forests: one of the world’s threatened major tropical environments: at least 35% of the area of mangrove forests has been lost in the past two decades, losses that exceed those for tropical rain forests and coral reefs, two other well-known threatened environments. Bioscience 51, 807–815. doi: 10.1641/0006-3568(2001)051%5B0807:mfootw%5D2.0.co;2
van Katwijk, M. M., Bos, A. R., de Jonge, V. N., Hanssen, L. S. A. M., Hermus, D. C. R., and de Jong, D. J. (2009). Guidelines for seagrass restoration: importance of habitat selection and donor population, spreading of risks, and ecosystem engineering effects. Mar. Pollut. Bull. 58, 179–188. doi: 10.1016/j.marpolbul.2008.09.028
van Katwijk, M. M., Thorhaug, A., Marbà, N., Orth, R. J., Duarte, C. M., Kendrick, G. A., et al. (2016). Global analysis of seagrass restoration: the importance of large-scale planting. J. Appl. Ecol. 53, 567–578. doi: 10.1111/1365-2664.12562
Vanderklift, M. A., Marcos-Martinez, R., Butler, J. R., Coleman, M., Lawrence, A., Prislan, H., et al. (2019). Constraints and opportunities for market-based finance for the restoration and protection of blue carbon ecosystems. Mar. Policy 107:103429. doi: 10.1016/j.marpol.2019.02.001
Waltham, N. J., Elliott, M., Lee, S. Y., Lovelock, C., Duarte, C. M., Buelow, C., et al. (2020). UN decade on ecosystem restoration 2021–2030—what chance for success in restoring coastal ecosystems? Front. Mar. Sci. 7:71. doi: 10.3389/fmars.2020.00071
Watanuki, A., Aota, T., Otsuka, E., Kawai, T., Iwahashi, Y., Kuwahara, H., et al. (2010). Restoration of kelp beds on an urchin barren: removal of sea urchins by citizen divers in southwestern Hokkaido. Bull. Fish. Res. Agent 32, 83–87.
Waycott, M., Duarte, C. M., Carruthers, T. J. B., Orth, R. J., Dennison, W. C., Olyarnik, S., et al. (2009). Accelerating loss of seagrasses across the globe threatens coastal ecosystems. Proc. Natl. Acad. Sci. U.S.A. 106, 12377–12381. doi: 10.1073/pnas.0905620106
Wehi, P. M., and Lord, J. M. (2017). Importance of including cultural practices in ecological restoration. Conserv. Biol. 31, 1109–1118. doi: 10.1111/cobi.12915
Wendland, K. J., Honzák, M., Portela, R., Vitale, B., Rubinoff, S., and Randrianarisoa, J. (2010). Targeting and implementing payments for ecosystem services: opportunities for bundling biodiversity conservation with carbon and water services in Madagascar. Ecol. Econ. 69, 2093–2107. doi: 10.1016/j.ecolecon.2009.01.002
Wilberg, M. J., Livings, M. E., Barkman, J. S., and Morris, B. T. (2011). Overfishing, disease, habitat loss, and potential extirpation of oysters in upper Chesapeake Bay. Mar. Ecol. Prog. Ser. 436, 131–144. doi: 10.3354/meps09161
Williams, S. L., Sur, C., Janetski, N., and Hollarsmith, J. (2019). Large-scale coral reef rehabilitation after blast fishing in indonesia: coral reef rehabilitation. Restor. Ecol. 27, 447–456. doi: 10.1111/rec.12866
Winterwerp, H., Wilms, T., Siri, H., Vries, J., Noor, Y., Van Wesenbeeck, B., et al. (2016). Building with nature: sustainable protection of mangrove coasts. Terra et Aqua 1, 5–15.
Wollenberg, J. T., Ollerhead, J., and Chmura, G. L. (2018). Rapid carbon accumulation following managed realignment on the Bay of Fundy. PLoS One 13:e0193930. doi: 10.1371/journal.pone.0193930
Wolters, M., Garbutt, A., and Bakker, J. P. (2005). Salt-marsh restoration: evaluating the success of de-embankments in north-west Europe. Biol. Conserv. 123, 249–268. doi: 10.1016/j.biocon.2004.11.013
Wylie, L., Sutton-Grier, A. E., and Moore, A. (2016). Keys to successful blue carbon projects: lessons learned from global case studies. Mar. Policy 65, 76–84. doi: 10.1016/j.marpol.2015.12.020
Keywords: marine coastal restoration, mangroves, seagrasses, saltmarsh, corals, kelp, shellfish, ecosystem services
Citation: Stewart-Sinclair PJ, Purandare J, Bayraktarov E, Waltham N, Reeves S, Statton J, Sinclair EA, Brown BM, Shribman ZI and Lovelock CE (2020) Blue Restoration – Building Confidence and Overcoming Barriers. Front. Mar. Sci. 7:541700. doi: 10.3389/fmars.2020.541700
Received: 10 March 2020; Accepted: 18 August 2020;
Published: 15 September 2020.
Edited by:
Tjisse Van Der Heide, Royal Netherlands Institute for Sea Research (NIOZ), NetherlandsReviewed by:
Eduardo Infantes, University of Gothenburg, SwedenCarolyn J. Lundquist, National Institute of Water and Atmospheric Research (NIWA), New Zealand
Copyright © 2020 Stewart-Sinclair, Purandare, Bayraktarov, Waltham, Reeves, Statton, Sinclair, Brown, Shribman and Lovelock. This is an open-access article distributed under the terms of the Creative Commons Attribution License (CC BY). The use, distribution or reproduction in other forums is permitted, provided the original author(s) and the copyright owner(s) are credited and that the original publication in this journal is cited, in accordance with accepted academic practice. No use, distribution or reproduction is permitted which does not comply with these terms.
*Correspondence: Phoebe J. Stewart-Sinclair, cC5zdGV3YXJ0c2luY2xhaXJAdXEuZWR1LmF1