- 1Department of Marine Science and Applied Biology, University of Alicante, Alicante, Spain
- 2Murcia University Aquarium, University of Murcia, Murcia, Spain
For decades, inputs of nutrients and organic matter into the Mar Menor coastal lagoon have favored the change from an original oligotrophic to a eutrophic state. The lagoon reached a stage of severe eutrophication and “environmental collapse” during the spring of 2016. This paper describes the massive growth of Serpulidae (Annelida, Polychaeta) forming large aggregations and reef structures after the environmental collapse caused by the eutrophic crisis. Four species belonging to the genera Hydroides and Serpula were identified; the identified species are Hydroides elegans, Hydroides dianthus, Serpula concharum, and Serpula vermicularis. The presence of bi-operculate and tri-operculate specimens is also documented in this study.
Introduction
Eutrophication and dystrophic crises are increasingly occurring in coastal lagoons around the world (Amanieu et al., 1975; Reyes and Merino, 1991; Ferrari et al., 1993; Mesnage and Picot, 1995; Boynton et al., 1996; Bachelet et al., 2000; Nakamura and Kerciku, 2000; Naldi and Viaroli, 2002; Newton et al., 2003). This is also the case for the Mar Menor, a Mediterranean hypersaline lagoon located in the southwest of Spain and declared as a protected area in several lists, such as the RAMSAR Convention on Wetlands of International Importance, Specially Protected Areas of Mediterranean Importance, European Union Bird Directive (Council Directive 79/409/EEC), and Natura 2000 Network (Figure 1). The Mar Menor is the largest coastal lagoon in the western Mediterranean and covers an area of 135 km2 with an average depth of 3.5 m and a 7-m maximum depth. This lagoon is the main collector of the Cartagena field watershed, and it has a reduced interconnection with the Mediterranean Sea due to the presence of a sandbar called La Manga (Martín de Agar et al., 1986). The lagoon undergoes sudden temperature changes throughout the year (10–32°C), and their salinity varies from 38 to 47 psu. Historically, this lagoon was oligotrophic, with transparent water, low levels of nutrients, and a benthic dominance of the seagrass Cymodocea nodosa, (Ucria) (Ascherson, 1870). The enlargement of the connection channel between the Mediterranean and the lagoon in 1972 increased the water renewal rates, reducing the salinity and extreme temperatures (Terrados and Ros, 1991). Anthropogenic pressures over the last few decades have led to nutrient and organic matter inputs into this lagoon, changing its original oligotrophic state into a eutrophic condition with an associated loss of ecological integrity (Velasco et al., 2006). These pressures have increased in recent years with the growth of intensive agricultural activity causing a drastic reduction in water quality in Mar Menor from the second half of 2015 (Aguilar Escribano et al., 2016). Significant nutrient inputs into the lagoon (mainly due to chemical fertilizers) caused a massive proliferation of phytoplankton, reducing the water column visibility from around 6 m to <0.5 m. The scarce light availability resulting from the exponential growth of the phytoplankton, limited photosynthetic activity to a shallow area (2–4 m depth), with the consequent mortality of the seagrass beds located below this depth limit. In addition, the increased deposition of dead organisms at the bottom of the lagoon intensified the bacterial activity and oxygen consumption (Moreno-González et al., 2013; Jiménez-Martínez et al., 2016; Belando et al., 2017). This situation led to an “environmental collapse” with the water column dominated by phytoplankton and the disappearance of the benthic community below 3 m depth (Hilton et al., 2006; Aguilar Escribano et al., 2016). The benthic vegetation in the lagoon was mainly responsible for controlling the flow of nutrients between the water column and the sediments, so its loss increased the vulnerability and consequently decreased the resilience of the lagoon ecosystem (Meyer and Kump, 2008). These eutrophication processes also caused organic matter and mineralized nutrients to be stored in the sediment, producing a supply of nutrients that can be kept for years (Duarte et al., 2015; McCrackin et al., 2017).
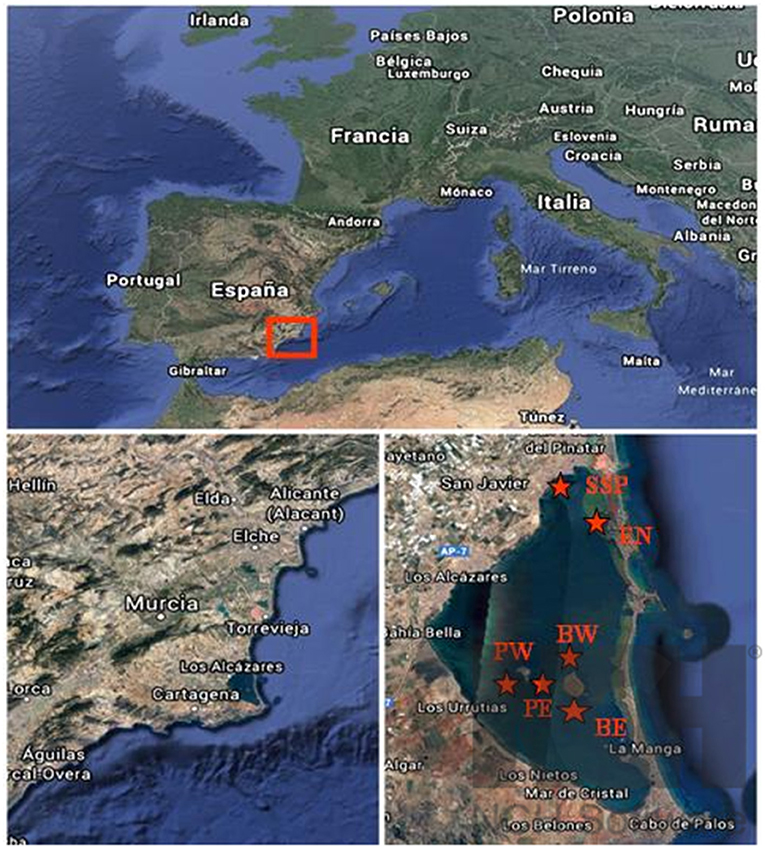
Figure 1. Location of the Mar Menor coastal lagoon: Two localities in the surrounding of the Baron Island: BE (37°41′27.14″N, 0°45′01″W) and BW (37°41′45.89″N, 0°46′37.8″W); two in La Perdiguera Island: PE (37°41′55.7″N, 0°47′47.18″W) and PW (37°41′55.03″N, 0°48′05.46″W), one locality in Las Encañizadas EN (37°47′41.26″N, 0°45′54.31″W) and the port of San Pedro del Pinatar marina SSP (37°49′00.13″N; 0°47′03.71″W).
Between 2016 and 2018, after the eutrophic crisis, a slight recovery of vegetation in the shallow areas of the lagoon and a primary recolonization of invertebrates in the sediment were detected (Ruiz-Fernandez et al., 2019). Serpulid reefs were observed on the sedimentary bottom, and some fan mussel shells (Pinna nobilis; Linnaeus, 1758) were also covered with serpulid aggregations. However, during 2019, the concentration of chlorophyll a in the water column progressively increased to levels similar to those recorded during 2016 (Ruiz-Fernandez et al., 2019). This situation worsened in October 2019, when a heavy flood caused a massive inflow of fresh water into the lagoon, resulting in stratification of the water body (i.e., high salinity with active eutrophication processes in the deeper waters and lower salinity and high nutrient levels of the flood runoff in the shallower waters). This event caused euxinic conditions in the lagoon (Schouten et al., 2001). Euxinic conditions occur when the water is anoxic, and hydrogen sulfide (H2S) levels increase. In this particular case, it has caused the death of macrofaunal community below 3 m, due to the lack of oxygen and the presence of toxins as a result of the decomposition of organic matter by anaerobic bacteria (Meyer and Kump, 2008; Ruiz-Fernandez et al., 2019).
Just weeks after the 2019 Mar Menor euxinic episode, large aggregations of polychaetes from the family Serpulidae Rafinesque (1815) (Annelida, Polychaeta), concentrated in the central coastal region, reappeared.
Polychaetes are one of the most diverse and abundant groups in marine sediments from the intertidal to deep zones, and they are decisively maintaining the structure and function of the marine environment (Bàez and Ardila, 2003). Furthermore, these organisms help to decompose, incorporate, and replace organic matter on the seabed, contributing to the nutrient recycling (Liñero-Arana and Reyes-Vásquez, 1979). Serpulidae is a family of sedentary polychaetes that construct calcareous tubes attached to hard substrata. Immediately after settlement, the worm forms a calcareous tube, which extends as the worm grows. The body is divided into the so-called branchial crown, the limited segment thorax, and the abdomen. The branchial crown bears ciliated pinnulate tentacles, and between them is the operculum (Ghobashy and Ghobashy, 2005). Some genera are found exclusively in estuarine or hypersaline environments and form true reefs with varying structures and dimensions. These “reefs” are usually colonized by epibiont organisms such as bryozoans, sponges, and bivalves, also predators and scavengers (crustaceans, echinoderms, and fish), which are provided with shelter and food (Ten Hove and Van den Hurk, 1993). Serpulids are usually recognized by the presence of an operculum that serves as a plug when the worms withdraw into its tube (Bastida-Zavala, 2009). However, the family includes both operculate and non-operculate taxa, and it is also common to find specimens with pseudo- or rudimentary opercula in addition to the functional operculum. When necessary, the pseudoperculum can develop into a functional operculum, replacing the previous one (Rzhavsky et al., 2014). This is the case for the genera Serpula (Linnaeus, 1758) and Hydroides (Gunnerus, 1768), that are characterized by having a pseudoperculum.
The purpose of this study is to provide an overview of the Serpullidae aggregates that emerged in Mar Menor in multiple habitats (boat hulls, intertidal rocks, and artificial substrates, etc.) over a 3-years period. This study has been carried out after two environmental crises in the coastal lagoon. The environmental conditions that have favored the growth and expansion of serpulids in the lagoon have been investigated. The first record of bi- and tri-operculus specimens in the lagoon is also documented.
Materials and Methods
Samples were collected during 3 years (2017, 2018, and 2019) from five localities in the Mar Menor. Two localities in Baron Island: BE (37°41′27.14″N, 0°45′01″W) and BW (37°41′45.89″N, 0°46′37.8″W); two localities in La Perdiguera Island: PE (37°41′55.7″N, 0°47′47.18″W) and PW (37°41′55.03″N, 0°48′05.46″W), and one locality in Las Encañizadas EN (37°47′41.26″N, 0°45′54.31″W) (Figure 1). All locations have been chosen according to the habitat structure and depth. BE and EN are shallow localities with a depth of 1.5 to 3 m and are characterized by a muddy sand sediment covered by a mixed meadow of Caulerpa prolifera (Forsskål) (Lamouroux, 1809) and Cymodocea nodosa. The PE, PW, and BW localities are characterized by muddy bottoms with empty shells of P. nobilis specimens covered with serpulids aggregations. PE and PW are deeper locations with a depth of 5–7 m, while the BW area has a depth of 1.5–2.5 m.
Two different sampling strategies have been used. In the first, collectors installed during 2017 (A1) and 2018 (A2) were used. In total, 12 collectors were installed, four in each of the three locations (BE, BW, and EN), between 1.5 and 2.5 m deep (Figure 1). The collectors consisted of 1 m2 nets and plates that were anchored to the bottom by concrete blocks. In the second sampling strategy, organisms were collected directly from their natural substrates. Sampling was carried out in three different periods during 2019 (March, July, and October), in deeper areas of the lagoon (PE and PW) between 5 and 7 m. depth (Figures 1, 2A). Six P. nobilis shells covered with serpulids aggregates and four specific reef structures were collected from the sediment. Sample B1, collected in March, consisted of an aggregation of serpulids and a shell of P. nobilis; sample B2 was collected in July and included two aggregations of serpulids and three shells of P. nobilis; and sample B3 included an aggregation and two shells of P. nobilis collected in October. These samples were collected prior to the 2019 euxinic event. Furthermore, two structures were sampled in December 2019 (C1) from a boat incrustation in the marina of San Pedro del Pinatar: (SSP: 37°49′00.13″N; 0°47′03.71″W). This sample was collected after the euxinic process (Figures 1, 2B).
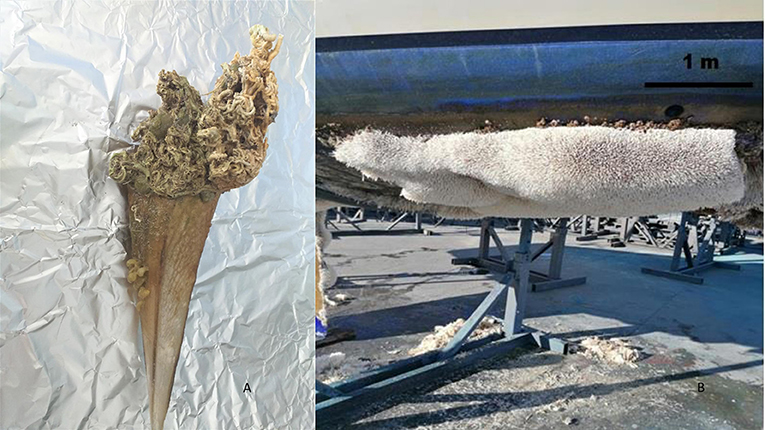
Figure 2. (A) Pinna nobilis shell partially jettisoned and covered with Hydroides dianthus aggregations. (B) Hulls of boats in the port of San Pedro del Pinatar from which the December 2019 samples were taken (photo: Ramon Pagan).
All samples were placed in seawater and stored in a refrigerator during transport to the laboratory, where they were fixed and preserved in 10% formalin until identification. Prior to identification, the samples were washed on a 0.5 mm sieve with non-demineralized water. Individuals were then removed from the tubes with the help of tweezers and identified at the genus or species level under a stereoscopic microscope (Bianchi and Morri, 2001; Bastida-Zavala, 2009; Ten Hove and Kupriyanova, 2009; Del Pilar Ruso et al., 2014; Martínez and San Martín, 2019). After taxonomic identification, the organisms were preserved in 70% ethanol.
The calcification rate was estimated for each aggregation by removing all worms from their tubes and drying them in the oven at 80°C for 48 h, after which the weight of the tubes was determined (Riedi and Smith, 2015). In addition, the wet weights of the animals were measured by drying the individuals on tissue paper and weighing them with a digital balance with a 0.001 g accuracy. With the data obtained, the relationship between the dry weight of the tube structure and the live organisms was calculated, obtaining the calcium carbonate values based on the Solís-Weiss (1998) methodology.
In this study, the two most abundant species are Hydroides elegans (Haswell, 1883) and Hydroides dianthus (Verrill, 1873). To estimate the density of the two species, the following measurements were made: the surface area of the reef structure, using a millimeter sheet as a quadrat (m2); the length of each individual tube; and the number of worms found in the structures and their weight. With the data obtained, the number of worms was divided by the area of the structure, and then it was multiplied with the value of the specimens' length and weight: N/A * L * W (N = number of specimens, A = area of the structure, L = length of tubes, W = weight of worms). Density was calculated with reference to the work of Vega et al. (2014) with minor modifications.
Bi-operculate forms of the species Hydroides elegans (Haswell, 1883), Hydroides dianthus (Verrill, 1873), Serpula vermicularis (Linnaeus, 1767), and tri-operculate Hydroides cf elegans have been deposited at the National Museum of Natural Sciences (MNCN), Madrid with the collection numbers: Hydroides elegans MNCN 16.01/18917 and MNCN 16.01/18919, Hydroides dianthus MNCN 16.01/18920, Serpula vermicularis MNCN 16.01/18921, and tri-operculate of Hydroides cf elegans MNCN 16.01/18918.
Results
A total of 4,372 serpulids were quantified from the samples collected in 2017, 2018, and 2019, of which 99.8% were identified at the species level. These belonged to four species in the genera Serpula and Hydroides: S. concharum (Langerhans, 1880), S. vermicularis, H. elegans, and H. dianthus.
In total, 2,830 serpulid specimens were quantified from the fouling collector samples analyzed during 2017 and 2018 (in A1: 887 of which 381 were H. elegans and 173 H. dianthus; in A2: 1.943 of which 693 belonged to H. elegans and 286 to H. dianthus). In both samples (A1 and A2), H. elegans was the most abundant species (67.6%), followed by H. dianthus (19.6%), S. vermicularis (2.1%), and S. concharum (1.7%).
By contrast, H. dianthus was the main species identified in the samples collected by the second sampling strategy, with a total of 451 specimens in the four aggregations on the sediment and 481 in the P. nobilis fouling. In the aggregations on the sediment, H. dianthus constituted 81% of the community in the B1 sample and 61% of the community in the B2 sample, but only 9.4% of the community in the B3 sample. In the structures growing on P. nobilis shells, these were all monospecific, with the exclusive presence of H. dianthus. Finally, the samples collected from the boat fouling during December 2019 (C1) consisted of a monospecific colony of H. elegans. A total of 640 specimens were counted making up 92% of the community present in the structure (Figure 2B).
Bi-operculate (Figures 3A,B) were documented in the fouling collector samples during 2017 and 2018 (A1 and A2), at rates of 4.7% for H. dianthus (Figure 3A), 4.1% for H. elegans (Figure 3B), in relation to the total number of specimens of each species. Moreover, bi-operculate specimens of H. dianthus were collected in 2019, using the second sampling strategy (directed sampling), at a rates of 2.3% in March (B1), 2.2% in July (B2), and 0.1% in October (B3), while bi-operculate specimens of H. elegans were collected from ship fouling in December 2019 (C1) at a rate of 2.0%. In addition, two specimens of Hydroides cf elegans analyzed from the fouling collector samples were tri-operculate, bearing one operculum that looked like a Serpula-type operculum, one that was a typical Hydroides cf. elegans operculum, and one pseudo-operculum.
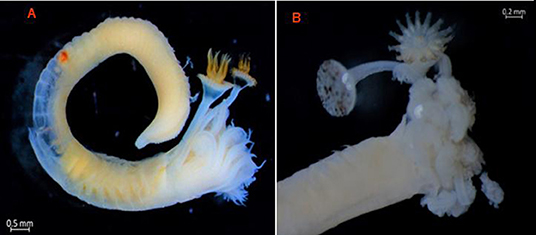
Figure 3. (A) Bi-operculate Hydroides dianthus exhibiting two opercula with the same morphology on the gill crown. The verticil is composed of eight pointed spines that are completely smooth, the distal ends of which curve toward the ventral side of the operculum, so that the longer spines appear to curve toward the inside of the verticil and the shorter ventral spines appear to curve toward the outside. (B) Bi-operculate Hydroides elegans specimen exhibiting two opercula with different morphologies on the gill crown. The first operculum has a typical H. elegans morphology with two levels: the verticil develops first and then the basal funnel differentiates and expands to fit the hole in the tube. The second operculum presents the typical Serpula morphology.
The calcification rate at A1 was 3.04 in 2017, with an increase to 6.14 in sample A2 in 2018, when the aggregations were dominated by H. elegans. Samples B collected in 2019 during three different periods B1 (March), B2 (July), and B3 (October), showed a calcification rate of 14.9 in the samples (B1), increasing to 25, 8 in samples (B2) and dropping below 7.1 in samples B3. In these B samples, the aggregations were dominated by H. dianthus. In the samples collected from ship fouling in December 2019 (C1), the calcification rate reached an extraordinary value of 38.7, with monospecific structures of H. elegans.
Three quantitative samples B1, B2, and B3 were taken to determine the population density of H. dianthus collected in the sediment. The density of H. dianthus was 43,000 individuals/m2 in B1, 44,400 individuals/m2 in sample B2, and 24,375 individuals/m2 in sample B3.
The density value of H. elegans collected in the ship fouling in December 2019 (C1) was 154,967 ± 86,570 individuals/m2.
The changes that occurred in physical parameters during the study period from 2017 to 2020 in Mar Menor were summarized (Figure 4). In the 1st months of 2017, the temperature fluctuated between 20 and 30°C, salinity remained between 38 and 44 psu, chlorophyll a content remained low at 5 mg/m3, and the oxygen value in the water column was between 7 and 8 mg/L.
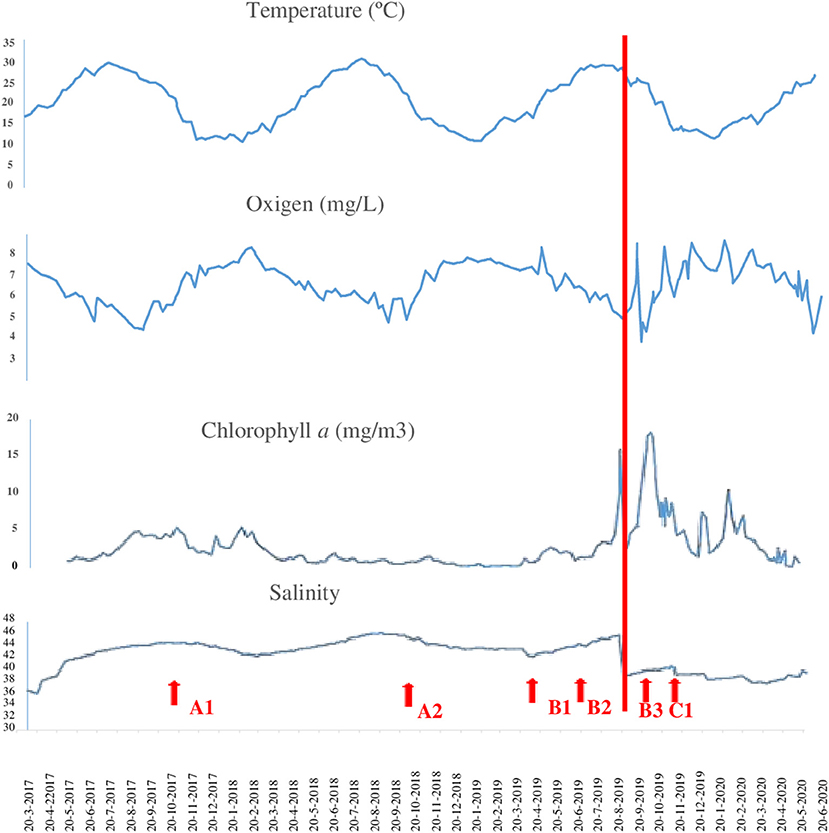
Figure 4. Changes in the concentration of chlorophyll a, oxygen, temperature, and salinity in the Mar Menor water column during the study period. Data were obtained from the General Directorate of the Mar Menor of the Ministry of Water, Agriculture, Livestock, Fisheries, and Environment of the Autonomous Community of the Region of Murcia (https://www.canalmarmenor.es). The red line indicates the extreme rainfall event in 2019. The red arrows indicate the different sampling times.
The relative abundance of serpulids in the polychaeta assemblages by sampling period (Figure 5) evidenced that samples collected before the extreme rainfall event and the euxinic crisis that occurred in 2019 showed a dominance of serpulids and specifically of H. elegans and H. Dianthus. Polychaeta assemblage gathered in 2017 (A1) showed a relative abundance of serpulids of 20% in relation to the total number of polychaetes, but an increase in the proportion of serpulids was observed in the samples of 2018 (A2) 45% and 2019 (B1) 80%, (B2) 60%. By the end of the summer of 2019, the level of chlorophyll a had risen to values higher than 15 mg/m3, salinity had decreased between 34 and 36 psu and oxygen values had dropped to 3–4 mg/L. These high values of chlorophyll a and the low values of oxygen reflected again an increase in phytoplankton and other algae, and a consequent decrease in oxygen (https//www.canalmarmenor.es). The dominant species in samples B3 collected in the 1st week of October 2019 (2 weeks before the second environmental crisis suffered in Mar Menor) was H. dianthus but a drop of up to 10% was observed in the relative abundance of serpulids in relation to the total number of polychaetes (Figure 5). The samples collected at the end of December in the sports marina of San Pedro, almost 3 months after the euxian crisis that occurred in the Mar Menor, were dominated by monospecific colonies of H. elegans with a relative abundance of 90% in relation to the total of polychaetes identified (Figures 4, 5).
Discussion
The species of Hydroides described in the present study in the Mar Menor coastal lagoon can be clearly differentiated by the morphology of the operculum and verticil spines. However, these structures are often very similar and present a great variability (Bastida-Zavala and Salazar-Vallejo, 2000; Ten Hove and Eliahu, 2005) as in the case of H. elegans and H. norvegica (Gunnerus, 1768). Nevertheless, these two species can only be safely distinguished by examining the special setae: H. norvegica special setae has two large subapical teeth, rarely accompanied by finer denticles and H. elegans special setae has a densely toothed subapical area, ending in two or more slightly thicker upper teeth (Zibrowius, 1971; Ten Hove, 1974). In the two-tier operculum, the verticil develops first, after which the basal funnel differentiates and expands to fit the tube bore (Hedgpeth, 1950; Ten Hove and Wolf, 1984; Bastida-Zavala and Salazar-Vallejo, 2000; Bastida-Zavala and Ten Hove, 2002; Trott, 2004; Ten Hove and Eliahu, 2005; Link et al., 2009). In this study, some specimens of H. elegans showed a modified operculum: the operculum displayed a soft whorl on the top of a funnel not yet fully developed. For similar reasons, H. dianthus is one of the many species that have been confused under the name of H. uncinata (Philippi, 1844), likely due to the remarkable morphological variation of its operculum. However, specimens from hypohaline lagoons have smaller bodies and poorly developed opercula, with dorsal verticil spines that have a blunt tip and are sometimes formed irregularly.
Two species of Serpula were also identified in the lagoon: S. concharum and S. vermicularis. These species differ in aspects related to the operculum, the tube, the abdominal hooks, and the special collar setae. Serpula concharum was easily identified by its very characteristic operculum and tube. However, there has been some taxonomic confusion between the species S. vermicularis and S. cavernicola (Fassari and Mòllica, 1991) due to erroneous morphological descriptions of the collar setae and its basal teeth (Imajima and ten Hove, 1984; Ten Hove and Jansen-Jacobs, 1984; Kupriyanova and Jirkov, 1997; Kupriyanova, 1999; Ten Hove and Kupriyanova, 2009). Serpula vermicularis collar setae usually have two large basal teeth and a serrated edge, while S. cavernicola collar setae have one large basal tooth and a smooth edge (Alcázar and San Martín, 2016). In the present study, no Serpula specimens were found with only one large basal tooth on the collar setae, which would be typical of S. cavernicola. However, all the specimens that were clearly identified as S. vermicularis based on the collar setae had pink and salmon-colored tubes with a series of circular sections that were sometimes clearly denticulate, while previous studies have shown that S. vermicularis specimens have completely smooth white tubes (with the exception of the growth lines) (Bastida-Zavala, 2012). The radiolar crown consists of two halves and includes a finely serrated cone-shaped inverted operculum on the upper edge. The color of the radioles can vary, but reddish or pink tones with white bands predominate.
Hydroids elegans and H. dianthus were the dominant species in the lagoon samples, and are both considered potentially invasive and opportunistic species (Read and Gordon, 1991). Both species have a great capacity to survive and reproduce rapidly in polluted environments with high temperatures and salinities (Link et al., 2009).
Hydroids dianthus was originally described (as Serpula dianthus) by Verrill (1873) from the New England coast. It appears to be native to the east coast of North America, where it is widely distributed in a variety of habitats including open coasts, as well as in partially brackish bays, lagoons, and harbors. In Europe, however, its distribution occurs only in ports and lagoons or immediately adjacent areas (Bastida-Zavala and Ten Hove, 2003b). It is found both in estuaries temperate and subtropical, and does not seem to have the temperature restrictions of other species of the genus Hydroides (Bastida-Zavala et al., 2017). The species have a threshold of tolerance to salinity between 28 and 50 psu, and a temperature range between 5 and 30°C (Zibrowius, 1971), and it presents a rapid growth during the hottest summer periods (Bastida-Zavala and Ten Hove, 2002).
Hydroides elegans populations grow at high densities, changing the ecosystems they colonize (Bastida-Zavala, 2009) and competing for food and space with native species. Although H. elegans has originally been described from Australia (Haswell, 1883), and some authors speculate that it is native from there (Zibrowius, 1992; Ben-Eliahu and ten Hove, 2011; Sun et al., 2015), there is no clear evidence of its Australian origin because this species was also found in the Hawaiian coast at natural and artificial substrates (Long, 1974; Bailey-Brock, 1976; Sun et al., 2015), which suggests a broader Indo-Western Pacific origin. The true origin of its geographical distribution is unknown, so it is impossible to classify it as a native species of a territory. Both species, H. elegans and H. dianthus, are considered to be cryptogenic (Bastida-Zavala et al., 2017). Hydroides elegans has spread in subtropical and temperate waters around the world through transport by ship. Its calcareous tubes and its rapid reproduction cause problems for ships and other maritime structures (Nedved and Hadfield, 2008) (Figure 3B). Indeed, this is one of the dominant species in aquaculture earthen ponds, where the organic content is very high, and the environmental conditions are quite stressful. It has been classified as tolerant species to organic enrichment (Carvalho et al., 2006). It has a tolerance range of salinity between 31 and 37 psu and temperature tolerance range between 5 and 30°C (Zibrowius, 1971). This polychaete can mature and reproduce in only 3 weeks in a favorable environment (Ten Hove, 1974; Hadfield et al., 1994; Unabia and Hadfield, 1999; Bastida-Zavala and Ten Hove, 2002, 2003a; Sun et al., 2012). Some studies have shown that the diversity of the bacterial community in the marine environment produces a signal that is received by H. elegans larvae improving their settlement and survival (Unabia and Hadfield, 1999). Hydroides elegans is also considered a bioindicator of polluted waters because it can live in areas that have suffered a high level of environmental degradation and active bacterial growth (Carpizo-Ituarte and Hadfield, 1998). Usually, these polychaetes have a significant impact on the ecosystems they colonize, threatening native biological diversity and even the economy (Villalobos-Guerrero et al., 2014). Both species are distributed throughout the western Mediterranean, many of the locations cited being coast lagoons (Table 1). Changes in temperature, oxygen, salinity, and especially chlorophyll a indicate the eutrophic state of a body of water, which is related to the development of plankton in the water column, and this development, in turn, is related to the availability of nutrients (Figure 4). Thus, for example, a higher content of chlorophyll a indicates a higher supply of nutrients. Since 2017, aggregations of H. dianthus have been observed on live and dead P. nobilis shells in shallow areas of Mar Menor, and numerous remains of partially “dissolved” shells that were covered with polychaete aggregations have been found in deep areas of the lagoon, below 3 m depth (pers. Obs.). These aggregations of H. dianthus could be a consequence of eutrophication in the lagoon. Eutrophication has serious implications for calcium carbonate production and dissolution among organisms with calcareous structures, which have weaker shells, at low pH levels (Sanchez Gomez-Leon and Mejia-Piña, 2018). Some authors who have concluded that the high reduction in calcium carbonate production is likely caused by the dissolution of previously deposited calcium carbonate (Brown and Elderfields, 1996; Glas et al., 2012), and Reymond et al. (2013), found that a pH below the threshold of 7.6 can lead to a decline in calcification of invertebrate structures (Gazeau et al., 2013). Mar Menor reached pH values below this limit during the eutrophication event in 2016 (Álvarez Rogel et al., 2016). Thus, the rapid formation of H. dianthus carbonate aggregations may have been at the expense of the affected P. nobilis shells, due to the sudden change in pH associated with this eutrophication crisis.
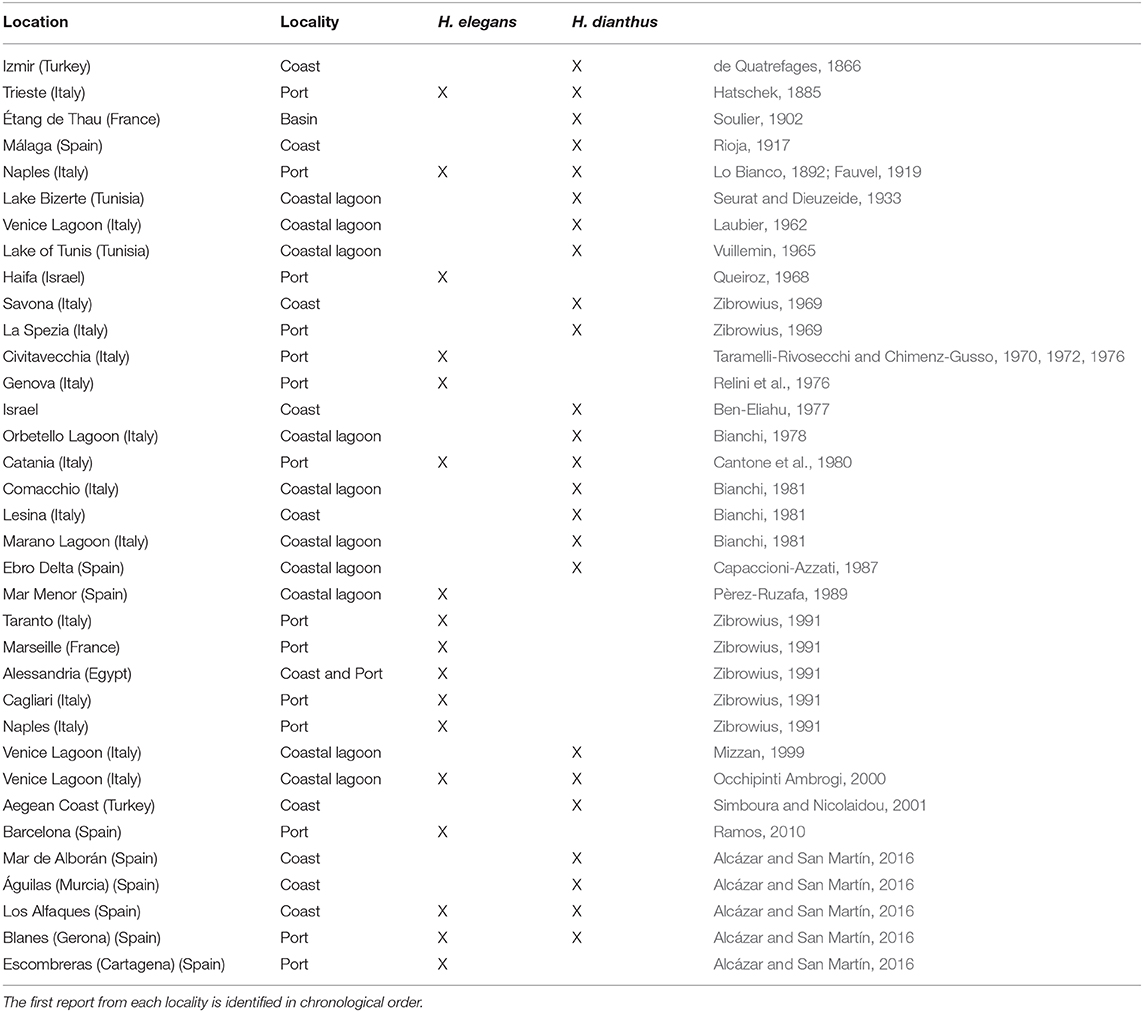
Table 1. Locations where Hydroides elegans and H. dianthus have previously been recorded in the Mediterranean.
In the marine environment, the invasive ability of a given species is determined by not only its biological characteristics (resistance to changes in physical–chemical factors, competition, reproductive, demographic strategy, etc.) but also the state of conservation of the potential host ecosystem (Giménez-Casalduero, 2006). In this sense, ports and some lagoons are an unstable system with high anthropogenic pressures that generally have reduced diversity and frequently present symptoms of environmental dysfunction (eutrophic episodes, hypoxia, etc.), increasing their propensity for establishing populations of non-native species (Kocak et al., 1999). Consequently, the study of marine biota of port and lagoon systems is an important tool for monitoring species distribution and abundance, assessing their invasive potential, and establishing protection measures against associated environmental risks (El Haddad et al., 2012).
Changes in various physical and chemical parameters due to the effects of anthropogenic inputs can lead to modifications in the structure of communities, with some species being favored over others and becoming dominant (Martínez-López et al., 2001). Polychaetes are used as indicators of pollution and of the sensitivity of marine ecosystems to changes in the environment, including those induced by human activities (Liñero-Arana and Reyes-Vásquez, 1979). Thus, knowledge of the polychaete community is a fundamental requirement of environmental studies, not only in those places that experience alterations of anthropogenic or natural origin, to calculate the degree and type of damage, but also in ecologically healthy areas to estimate the richness or productivity of the systems (Del Pilar et al., 2009).
Calcium carbonate precipitation is one of the biological ways in which carbon dioxide can be seized in the ocean. On the contrary, the increase in carbon dioxide that is generated by anthropogenic activities is considered one of the precursors of the acidity of the oceans. In coastal environments, anthropogenic influences can cause unfavorable conditions for the conservation of calcium carbonate due to excessive contributions of organic matter and/or nutrients (Sanchez Gomez-Leon and Mejia-Piña, 2018). In the present study, the carbonate concentration aggregations were calculated considering the relationship between the dry weight of the tubes of the dominant species, so as not to create confusion between the largest and smallest tubes, and the number of individuals found. This yielded very different values between the years of study, ranging from 3.04 in 2017 (A1) to 6.14 in 2018 (A2) and different values in the different months of 2019, 14.9 in March (B1), 25.8 in July (B2), 7.12 in October (B3), and 38.07 in December (C1). The low concentration of carbonates in the 2017 samples could be associated with the dominant species, as well as with the low number of serpulids (Figure 5), and it is also associated with the different physical parameters of the water column. In the following years, the number of specimens increased, perhaps in relation to the increase in eutrophication, which causes a high availability of nutrients, an increase in pH and, consequently, an increase in dissolved calcium carbonate, which could favor the tube formation (Fabry et al., 2008; Doney et al., 2009; Wicks and Roberts, 2012).
In this study, the presence of specimens of H. elegans, H. dianthus, and S. vermicularis with two opercula was documented. Hydroides elegans has a particular propensity for developing bi-operculate specimens (Rioja, 1919; Sentz, 1962; Alcázar and San Martín, 2016). In some bi-operculate organisms, both opercula have similar morphological characteristics, while in others, each operculum has a different morphology. Ten Hove and Eliahu (2005) coined the term “bi-operculate chimera” to indicate the ontogenetic state of having two different types of operculum that occurs in some species of Hydroides. Okada (1933) noted the presence of bi-operculate individuals while studying the phenomenon he called “compensatory regulation” and explained their presence under natural conditions as a phylogenetically primitive condition as opposed to being a result of malformations. The presence of two opercula was considered a result from the regeneration of a damaged operculum, activated by the developmental inhibition that is exerted by the operculum on the pseudo-operculum, and this theory was also maintained by La Greca (1949). However, although this theory seems, in principle, to be a plausible explanation for the presence of bi-operculate individuals, no evident breaks were found in the upper part of the secondary operculum. Indeed, the only anomaly that was found occurred in the tri-operculate specimens of Hydroides cf elegans, in which the primitive operculum was duplicated. According to Ten Hove and Eliahu (2005), this state concerns a transient and very short ontogenetic state due to a guarantee of the reproduction and, therefore, the continuity of life in environments with high contamination. Previous studies reported that malformations or anomalies in polychaetes were related to exposure to pollutants and its effects at many levels: individual, specific, population, and community (Coutinho and Santos, 2014). More recent studies on samples collected elsewhere have shown that when two opercula are present, the largest operculum directly or indirectly inhibits the developmental power of the smallest, leading to a state of equilibrium (Kubal et al., 2012). Molecular studies have attributed this inhibition to the presence of a high concentration of the nucleotide 5′-adenosine monophosphate in the functional operculum, with the inhibitory effect only occurring when the nucleotide concentration increases above a certain level and regeneration occurring when this level is reduced (Puccia et al., 1976). The relatively abundant presence of biperculated and trioperculated specimens in the Mar Menor could be related to the environmental conditions, low pH, availability of CO2, and the presence of high bacterial concentration. It would be necessary to analyze the effect of these environmental conditions on the reproduction rates of the serpulids, possible self-fertilization, and genetic drift in the lagoon populations, and the effect of all these over the presence of bi and trioperculated specimens.
Data Availability Statement
The raw data supporting the conclusions of this article will be made available by the authors, without undue reservation.
Author Contributions
JS and FG conceptualized the study. FG and EC collected samples. JS processed samples and analyzed the data. JS and FG wrote the manuscript with contribution from EC and YD.
Funding
This study was supported by the research fund of the University of Alicante.
Conflict of Interest
The authors declare that the research was conducted in the absence of any commercial or financial relationships that could be construed as a potential conflict of interest.
Acknowledgments
The cooperation and technical support of all the staff of the Marine Science and Applied Biology Department and the AQUARIUM of the University of Murcia was appreciated, in particular Vincent Pardo, Rosa Canales, Carmen Barberá, and Eleuterio Abellan, Also Dra Cristina Almansa Carrascosa from the microscopy unit Technical Services of UA. The manuscript benefitted from useful criticism and helpful suggestions from the two reviewers and the editor Susana Carvalho. We especially thanks to Dr. Harry Ten Hove for solving some doubts about the species described.
References
Aguilar Escribano, J., Gimenez-Casalduero, F., Mas Hernández, J., and Ramos-Esplá, A. A. (2016). Evaluación del estado y composición de la comunidad Fitoplanctónica de las agua del Mar Menor, Murcia (mayo de 2016).
Alcázar, J., and San Martín, G. (2016). Serpúlidos (Annelida, Serpulidae) colectados en la Campaña Oceanográfica “Fauna II” y catálogo actualizado de especies íbero-baleares de la familia Serpulidae. Graellsia 72:053. doi: 10.3989/graellsia.2016.v72.120
Álvarez Rogel, J., González Alcaraz, M. N., Conesa Alcaraz, H. M., Tercero Gómez, M. D. C., P-árraga Aguado, I. M., María Cervantes, A., et al. (2016). Eutrofización y contaminación por residuos mineros en humedales del Mar Menor: comprendiendo los procesos biogeoquímicos para plantear posibles actuaciones de manejo. Madrid, Instituto Español de Oceanografía, Ministerio de Economía y Competitividad, 2016. Temas Oceanogr. 9, 251–270. Available online at: http://hdl.handle.net/10317/7162
Amanieu, M., Baleux, B., Guelorguet, O., and Michel, P. (1975). Etude biologique et hydrologique d'une crise dystrophique (malaigue) dans l'étang du Prévost a Palavas (Hérault). Vie Milieu 25, 175–204.
Ascherson, P. (1870). Sitzungs-Bericht der Gesellschaft naturforschender Freunde zu Berlin am 16. Februar 1869 – Sitzungsber. Ges. Naturf. Freunde Berlin 1869, 3–6.
Bachelet, G., Montaudouin, X., Auby, I., and Labourg, P.-J. (2000). Seasonal changes in macrophyte and macrozoobenthos assemblages in three coastal lagoons under varying degrees of eutrophication. J. Mar. Sci. 57, 1495–1506. doi: 10.1006/jmsc.2000.0902
Bàez, D., and Ardila, N. E. (2003). Polychaetes (Annelida: Polychaeta) of the Colombian Caribbean. Biota Colombiana 4, 89–109. Available online at: http://revistas.humboldt.org.co/index.php/biota/article/view/126
Bailey-Brock, J. H. (1976). Habitats of tubicolous polychaetes from the Hawaiian Islands and Johnston Atoll. Pac. Sci. 30, 69–81.
Bastida-Zavala, J. R., and Ten Hove, H. A. (2003a). Revision of Hydroides Gunnerus, (1768) (Polychaeta: Serpulidae) from the eastern pacific region and hawaii. Beaufortia 53, 67–110. Available online at: https://repository.naturalis.nl/pub/505009
Bastida-Zavala, J. R. (2009). “Capítulo 43: serpulidae rafinesque, 1815,” in Poliquetos (Annelida: Polychaeta) de México y América Tropical, eds J.A. de León-González, J. R. BastidaZavala, L. F. Carrera-Parra, M. E. García-Garza, A. Peña-Rivera, S. I. Salazar-Vallejo, and V. Solís-Weiss (Monterrey: Universidad Autónoma de Nuevo León), 511–544.
Bastida-Zavala, J. R., McCann, L. D., Keppel, E., and Ruiz, G. M. (2017). The fouling serpulids (Polychaeta: Serpulidae) from United States coastal waters: an overview. Eur. J. Taxonomy 344, 1–76. doi: 10.5852/ejt.2017.344
Bastida-Zavala, J. R., and Salazar-Vallejo, S. (2000). Serpúlidos (Polychaeta: Serpulidae) del caribe noroccidental: hydroides y serpula. Rev. Biol. Trop. 48, 841−858. doi: 10.15517/RBT.V48I4.18901
Bastida-Zavala, J. R., and Ten Hove, H. A. (2002). Revision of Hydroides Gunnerus, 1768 (Polychaeta: Serpulidae) from the Western Atlantic Region. Beaufortia 52, 103–178. doi: 10.3897/zookeys.642.10443
Bastida-Zavala, J. R., and Ten Hove, H. A. (2003b). Revision of Hydroides Gunnerus, (1768) (Polychaeta: Serpulidae) from the western Atlantic region. Beaufortia 52, 103–178. Available online at: https://repository.naturalis.nl/pub/504958
Bastida-Zavala, R. (2012). Serpula and Spiraserpula (Polychaeta, Serpulidae) from the Tropical Western Atlantic and Gulf of Guinea. ZooKeys 198, 1–23. doi: 10.3897/zookeys.198.3030
Belando, M. D., Bernardeau-Esteller, J., García-Muñoz, R., Ramos-Segura, A., Santos-Echeandía, J., García-Moreno, P., et al. (2017). Evaluación del Estado de Conservación de las Praderas de Cymodocea Nodosa en la Laguna Costera del Mar Menor. 2014-2016. Murcia: Instituto Español de Oceanografía y Asociación de Naturalistas del Sureste.
Ben-Eliahu, M. N., and ten Hove, H. A. (2011). Serpulidae (Annelida: Polychaeta) from the Suez Canal From a Lessepsian migration perspective. Zootaxa 2848, 1–147. doi: 10.11646/zootaxa.2848.1.1
Ben-Eliahu, N. M. (1977). Polychaete cryptofauna from rims of similar intertidal vermetid reefs on the Mediterranean coast of Israel and in the gulf of Eilat: Sedentaria. Israel J. Zool. 25, 121–155.
Bianchi, C. N. (1978). Serpuloidea (Annelida, Polyehaeta) delle aeque italiane: elenco delle specie e chiavi per la determinazione. Ann. Mus. Civ. Star: Nat. 82, 266–294.
Bianchi, C. N. (1981). Policheti Serpuloidei. Guideper il Riconoscimento Delle Specie Animali Delle Acque Lagunari E Costiere Italiane. AQ/1/96. Genova: Consiglio Nazionale delle Ricerche.
Bianchi, C. N., and Morri, C. (2001). The battle is not to the strong: Serpulid reefs in the lagoon of Orbetello (Tuscany, Italy). Estuarine Coast. Shelf Sci. 53, 215–220. doi: 10.1006/ecss.2001.0793
Boynton, W. R., Hagy, J. D., Murray, L., Stokes, C., and Kemp, W. M. (1996). A comparative analysis of eutrophication patterns in a temperate coastal lagoon. Estuaries 19, 408–421. doi: 10.2307/1352459
Brown, S. J., and Elderfields, H. (1996). Variations in Mg/Ca and Sr/Ca ratios of planktonic foraminifera caused by post depositional dissolution: evidence of shallow Mg-dependent dissolution. Paleoceanography 11, 543–551. doi: 10.1029/96PA01491
Cantone, G., Cormaci, M., Fassari, G., Furnari, G., Galluzzo, G., Pavone, P., and Brigandì, S. (1980). Primi dati sul fouling del porto di Catania. Thalassia Salentina 10, 3–44.
Capaccioni-Azzati, R. (1987). Anélidos Poliquetos de la Ensenada de los Alfaques (Delta del Ebro, Mediterráneo Occidental). (Ph. D. Thesis doctoral). Universidad de Valencia. Valencia, España.
Carpizo-Ituarte, E., and Hadfield, M. G. (1998). Stimulation of metamorphosis in the polychaete Hydroides elegans Haswell (Serpulidae). Biol. Bull. 194, 14–24. doi: 10.2307/1542509
Carvalho, S., Barata, M., Pereira, F., Gaspar, M. B., da Fonseca, L. C., and Pousao-Ferreira, P. (2006). Distribution patterns of macrobenthic species in relation to organic enrichment within aquaculture earthen ponds. Marine Pollut. Bull. 52, 1573–1584. doi: 10.1016/j.marpolbul.2006.09.005
Coutinho, M. C. L., and Santos, C. S. G. (2014). Morphological anomalies in polychaetes: Perinereis species (Polychaeta: Annelida) examples from the Brazilian coast. Mem. Mus. Victoria 71, 45–51. doi: 10.24199/j.mmv.2014.71.05
del Pilar Ruso, Y., Casalduero, M. F. G., de la Ossa Carretero, J. A., Lizaso, J. L. S., and Esplá, A. Á. R. (2014). Guía práctica Para la Identificación de familias de poliquetos. Alicante: Editorial Club Universitario.
Del Pilar, Y., De la Ossa, J. A., Loya-Fernandez, A., Ferrero-Vicente, L. M., and Giménez-Casalduero, F. (2009). Assessment of soft-bottom Polychaeta assemblage affected by a spatial confluence of impacts: Sewage and brine discharges. Marine Pollut. Bull. 58, 776–782. doi: 10.1016/j.marpolbul.2009.03.002
Doney, S. C., Fabry, V. J., Feely, R. A., and Kleypas, J. A. (2009). Ocean acidification: the other CO2 problem. Ann. Rev. Mar. Sci. 1, 169–192. doi: 10.1146/annurev.marine.010908.163834
Duarte, C., Borja, A., Carstensen, J., Elliott, M., Krause-Jensen, D., and Marbà, N. (2015). Paradigms in the recovery of estuarine and coastal ecosystems. Estuar. Coasts 38, 1202–1212. doi: 10.1007/s12237-013-9750-9
El Haddad, M., Bermell, V. T., Carmona, J. A. G., and García, C. A. (2012). The use of georeferenced underwater TV devices for the study of the exotic invasive species Branchiomma luctuosum (Grube, 1869) (Polychaeta, Sabellidae) in ports from the Eastern Iberian coast (Western Mediterranean Sea). BioInvasions Records 1, 277–281. doi: 10.3391/bir.2012.1.4.06
Fabry, V. J., Seibel, B. A., Feely, R. A., and Orr, J. C. (2008). Impacts of ocean acidification on marine fauna and ecosystem processes. ICES J. Marine Sci. 65, 414–432. doi: 10.1093/icesjms/fsn048
Fassari, G., and Mòllica, E. (1991). Una nuova specie di Serpulidae (Annelida Polychaeta) di grotta. Animalia 18, 261–267.
Fauvel, P. (1919). Annélides Polychètes de Madagascar, de Djibouti, et du Golfe Persique. Arch. Zool. Exp. Générl. 58, 315–473. doi: 10.5962/bhl.part.8154
Ferrari, I., Ceccherelli, V. U., Naldi, M., and Viaroli, P. (1993). Planktonic and benthic communities in a shallow-water dystrophic lagoon. Verh. Internat. Verein. Limnol. 25, 1043–1047. doi: 10.1080/03680770.1992.11900318
Gazeau, F., Parker, L. M., Comeau, S., Gattuso, J.-P., O'Connor, W. A., and Martin, S. (2013). Impacts of ocean acidification on marine shelled molluscs. Marine Biol 160, 2207–2245. doi: 10.1007/s00227-013-2219-3
Ghobashy, A. F. A., and Ghobashy, M. A. (2005). Marine fouling studies in Egypt. a. Serpulids. Egypt. J. Aqu. Res. 31, 89–102.
Giménez-Casalduero, F. (2006). “Gestión de la franja costera: un enfoque integra- do,” in Contrastes Naturales en la Región Bioclimática del Mediterráneo, eds A. de Murcia, M. de la Ciencia y del Agua (Murcia: Museo de la Ciencia y del Agua), 261–272.
Glas, M. S., Langer, G., and Keul, N. (2012). Calcification acidifies the microenvironment of a benthic foraminifer (Ammonia sp.). J. Exp. Marine Biol. Ecol. 424-425, 53–58. doi: 10.1016/j.jembe.2012.05.006
Gunnerus, J. E. (1768). Om nogle Norske coraller. KGL. Norske Videnskabers Selskabs Skrifter 4, 38–73.
Hadfield, M. G., Unabia, C. C., Smith, C. M, and Michael, T. M. (1994). Settlement preferences of the ubiquitous fouler Hydroides elegans,” in Recent Development in Biofouling Control, eds M. Thompson, R. Nagabhushanam, R. Sarojini, M. Fingerman (Rotterdam: August Aimé Balkema), 65–74.
Haswell, W. A. (1883). A monograph of the Australian Aphroditea. Proc. Linnaean Soc. New South Wales 7, 250–299. doi: 10.5962/bhl.part.22751
Hatschek, B. (1885). Entwicklung der Trochophora von Eupomatus uncinatus (Phil.). Arbeiten aus den Zoologischen lnstituten der Universitdt Wien und der Zoologischen Station in Triest 6, 121–148.
Hedgpeth, J. W. (1950). “Annotated list of certain marine invertebrates found on Texas jetties,” in The Invertebrate Fauna of Texas Coast Jetties; A Preliminary Survey, eds H. L. Whitten, H. F. Rosene, J. W. Hedgpeth (University of Texas, Texas: Publications of the Institute of Marine Science), 53–87.
Hilton, J., O'Hare, M., Bowes, M. J., and Jones, J. I. (2006). How green is my river? a new paradigm of eutrophication in rivers. Sci. Total Environ. 365, 66–83. doi: 10.1016/j.scitotenv.2006.02.055
Imajima, M., and ten Hove, H. A. (1984). Serpulinae (Annelida, Polychaeta) from the Truk Islands, Ponape and Majuro Atoll, with some other new Indo-Pacific records. Proc. Japan. Soc. Syst. Zool. 27, 35–66.
Jiménez-Martínez, J., García-Aróstegui, J. L., Hunink, J. E., Contreras, S., Baudron, P., and Candela, L. (2016). The role of groundwater in highly human-modified hydrosystems: a review of impacts and mitigation options in the Campo de Cartagena-Mar Menor coastal plain (SE Spain). Environ. Rev. 24, 377–392. doi: 10.1139/er-2015-0089
Kocak, F., Ergen, Z., and Çinar, ME. (1999). Fouling organisms and their developments in a polluted and an unpolluted marina in the Aegean Sea (Turkey). Ophelia 50, 1–20. doi: 10.1080/00785326.1999.10409385
Kubal, P., Sukumaran, S., and Vijapure, T. (2012). Record of bioperculate fouling serpulid polychaete, Hydroides operculatus (Treadwell, 1929) from Diu, west coast of India. Indian J. Geo-Marine Sci. 41, 381–384.
Kupriyanova, E. K. (1999). The taxonomic status of Serpula cf. columbiana Johnson, 1901 from the American and Asian coasts of the North Pacific Ocean (Polychaeta: Serpulidae). Ophelia 50, 21–34. doi: 10.1080/00785326.1999.10409386
Kupriyanova, E. K., and Jirkov, I. A. (1997). Serpulidae (Annelida, Polychaeta) of the Arctic Ocean. Sarsia 82, 203–236. doi: 10.1080/00364827.1997.10413651
La Greca, M. (1949). Ricerche sull'origine degli individui biopercolati di Hydroides (Anellidi Policheti). Italian J. Zool. 16, 101–106. doi: 10.1080/11250004909439086
Lamouroux, J. V. F. (1809). Observations sur la physiologie des algues marines, et description de cinq nouveaux genres de cette famille. Nouv. Bull. Sci. Soc. Phil. Paris 1, 330–333.
Langerhans, P. (1880). Die Wurmfauna von Madeira. III. Zeitschrift für wissenschaftliche Zool. 34, 87–143.
Laubier, L. (1962). Quelques Annélides Polychètes de la Lagune de Venise. description de Prionospio caspersi n. sp. Vie et Milieu 13, 123–159.
Liñero-Arana, I., and Reyes-Vásquez, G. (1979). Nereidae (Polychaeta: Errantia) del Golfo de Cariaco, Venezuela. Bol. Inst. Oceanogr. Venezuelas 18, 3–12.
Link, H., Nishi, E., Tanaka, K., Bastida-Zavala, R., Kupriyanova, E. K., and Yamakita, T. (2009). Hydroides dianthus (Polychaeta: Serpulidae), and alien species introduced into Tokyo Bay, Japan. Mar. Biodivers. Rec. 2, 1–5. doi: 10.1017/S1755267209000931
Linnaeus, C. (1758). “Systema Naturae per regna tria naturae, secundum classes, ordines, genera, species, cum characteribus, differentiis, synonymis, locis,” in Editio decima, reformata 10th Edn, Vol. 1, (Laurentius Salvius: Holmiae), 824. doi: 10.5962/bhl.title.542
Linnaeus, C. (1767). Systema naturae per regna tria naturae: secundum classes, ordines, genera, species, cum characteribus, differentiis, synonymis, locis. Ed. 12. 1., Regnum Animale. 1 & 2. Holmiae, Laurentii Salvii. Holmiae (Stockholm), 533–1327.
Lo Bianco, S. (1892). Gli Anellidi tubicoli trovati nel Golfo di Napoli. Atti R. Acad. Se. Fisic. Mal. Napoli, ser. 2, 1–97.
Martín de Agar, P., Torres Martínez, A., and Ramírez-Díaz, L. (1986). Ecología y ordenación del territorio: El caso del Mar Menor y ecosistemas adyacentes (Murcia: SE España) I: Prospección y sectorización del Medio Físico. Anales Biol. 8, 71–84.
Martínez, M. J., and San Martín, G. (2019). Serpúlidos (Annelida, Serpulidae) colectados en las campañas oceanográficas de Fauna Ibérica. Graellsia 75:085. doi: 10.3989/graellsia.2019.v75.224
Martínez-López, A., Cervantes-Duarte, R., Reyes-Salinas, A., and Valdez-Holguín, J. E. (2001). Cambio estacional de clorofila a en la Bahía de La Paz, BCS, México. Hidrobiológica 11, 45–52.
McCrackin, M. L., Jones, H. P., Jones, P. C., and Moreno-Mateos, D. (2017). Recovery of lakes and coastal marine ecosystems from eutrophication: a global meta-analysis. Limnol. Oceanogr. 62, 507–518. doi: 10.1002/lno.10441
Mesnage, V., and Picot, B. (1995). The distribution of phosphate in sediments and its relation with eutrophication of a Mediterranean coastal lagoon. Hydrobiologia 297, 29–41. doi: 10.1007/BF00033499
Meyer, K. M., and Kump, L. R. (2008). Oceanic euxinia in Earth history: causes and consequences. Annu. Rev. Earth Planet. Sci. 36, 251–288. doi: 10.1146/annurev.earth.36.031207.124256
Mizzan, L. (1999). Le specie alloctone del macrozoobenthos della Laguna di Venezia: il punto della situazione. Bollettino del Museo Civico di Storia Naturale di Venezia 49, 145–177.
Moreno-González, R., Campillo, J. A., and León, V. M. (2013). Influence of an intensive agricultural drainage basin on the seasonal distribution of organic pollutants in seawater from a -Mediterranean coastal lagoon (Mar Menor, SE Spain). Marine Pollut. Bull. 77, 400–411. doi: 10.1016/j.marpolbul.2013.09.040
Nakamura, Y., and Kerciku, F. (2000). Effects of filter-feeding bivalves on the distribution of water quality and nutrient cycling in a eutrophic coastal lagoon. J. Mar. Syst. 26, 209–221. doi: 10.1016/S0924-7963(00)00055-5
Naldi, M., and Viaroli, P. (2002). Nitrate uptake and storage in the seaweed Ulva rigida C. Agardh in relation to nitrate availability and thallus nitrate content in a eutrophic coastal lagoon (Sacca di Goro, Po River Delta, Italy). J. Exp. Mar. Biol. Ecol. 269, 65–83. doi: 10.1016/S0022-0981(01)00387-2
Nedved, B. T., and Hadfield, M. G. (2008). “Hydroides elegans (Annelida: Polychaeta): a model for biofouling research,” in Marine and Industrial Biofouling Springer Series on Biofilms, Vol. 4, eds H.C. Flemmig, P. Syrutha Murthy, R. Venkatesan, and K. E. Cooksey (Berlin, Heidelberg: Springer), 203–217. doi: 10.1007/978-3-540-69796-1_11
Newton, A., Icely, J. D., Falcão, M., Nobre, A., Nunes, J. P., Ferreira, J. G., et al. (2003). Evaluation of eutrophication in the Ria Formosa coastal lagoon, Portugal. Cont. Shelf Res. 23, 1945–1961. doi: 10.1016/j.csr.2003.06.008
Occhipinti Ambrogi, A. (2000). Biotic invasions in a Mediterranean lagoon. Biol. Invasions 2, 165–176. doi: 10.1023/A:1010004926405
Okada, Y. K. (1933). Remarks on the reversible asymmetry in the opercula of the polychaete Hydroides. J. Marine Biol. Assoc. 18, 655–670. doi: 10.1017/S0025315400043976
Pèrez-Ruzafa, A. (1989). Estudio Ecológico y Bionómico de los Poblamientos Bentónicos del Mar Menor (Murcia, SE de España). (Ph. D. Thesis), Universidad de Murcia, Murcia, Spain.
Philippi, A. (1844). Einige bemerkungen iiber die gattung serpula, nebst aufzahlung der von mir im mittelmeermit dem thier beobachteten arten. Arch. Naturg. 10, 186–198. doi: 10.5962/bhl.part.29558
Puccia, E., Durante, M., and Mazzola, C. (1976). Synthesis of adenine nucleotides during opercular compensatory regeneration in the polychete Hydroides norvegica. Atti della Accademia Nazionale dei Lincei. Classe di Scienze Fisiche, Matematiche e Naturali. Rendiconti 60, 510–517.
Quatrefages, A. D. (1866). Histoire Naturelle des Annelés Marins et d'eau Douce. Annélides et Géphyriens. Paris: Tome second Deuxième partie Librarie Encyclopédique de Roret, 337–794. doi: 10.5962/bhl.title.122818
Queiroz, D. (1968). Preliminary note on the Polychaeta larvae in Haifa Harbour, Israel. Rapp. Comm. int. Mer Médit. 19, 193–194.
Rafinesque, C. S. (1815). Analyse de la Nature, ou Tableau de l'univers et des Corps Organisés. Palaermo: Published privately. doi: 10.5962/bhl.title.106607
Ramos, M. (2010). IBERFAUNA. The Iberian Fauna Databank. Available online at: http://iberfauna.mncn.csic.es (accessed June 11, 2020).
Read, G. B., and Gordon, D. P. (1991). Adventive occurrence of the fouling serpulid Ficopomatus enigmaticus (Polychaeta) in New Zealand. N. Z. J. Marine Freshwater Res. 25, 269–273. doi: 10.1080/00288330.1991.9516478
Relini, G., Geraci, S., Montanari, M., and Romairone, V. (1976). Variazioni stagionali del fouling sulle piattaforme off-shore di Ravenna e Crotone. Bollettino di Pesca, Piscicoltura e Idrobiologia, 31, 227–256.
Reyes, E., and Merino, M. (1991). Diel dissolved-oxygen dynamics and eutrophication in a shallow, well-mixed tropical lagoon (Cancun, Mexico). Estuaries 14, 372–381. doi: 10.2307/1352262
Reymond, C. E., Lloyd, A., Kline, D. I., Dove, S. G., and Pandolfi, J. M. (2013). Decline in growth of foraminifer M arginopora rossi under eutrophication and ocean acidification scenarios. Glo. Change Biol. 19, 291–302. doi: 10.1111/gcb.12035
Riedi, M. A., and Smith, A. M. (2015). Tube growth and calcification of two reef-building ecosystem engineers in southern New Zealand: Galeolaria hystrix and Spirobranchus cariniferus (Polychaeta: Serpulidae). Marine Geol. 367, 212–219. doi: 10.1016/j.margeo.2014.10.010
Rioja, E. (1919). Una curiosa anomalía de Hydroides norvegica Gunnerus y algunas consideraciones acerca de la filogenia de los serpúlidos. Boletín de la Real Sociedad Española de Historia Natural 19, 445–449.
Rioja, E. (1917). D atos para el conocim iento de la fauna de Anélidos poliquetos del C antábrico. Trab. Mus. Nac. Cieñe. Nat. Madre (ser. Zool.) 29.
Ruiz-Fernandez, J. M., León, V. M., Marín-Guirao, L., Giménez-Casalduero, F., Alvárez-Rogel, J., Esteve-Selma, M. A., et al. (2019). “Informe de síntesis sobre el estado actual del mar menor y sus causas en relación a los contenidos de nutrientes,” in Projects of Sustainability and Conservation of Mar Menor Lagoon and Its Basin. Alicante, Spain: Universidad de Alicant. Available online at: https://dcmba.ua.es/es/documentos/carteles-seminariosdoctorado/informe-estado-mar-menor.pdf (accessed November 25, 2019).
Rzhavsky, A. V., Kupriyanova, E. K., Sikorski, A. V., and Dahle, S. (2014). Calcareous Tubeworms (Polychaeta, Serpulidae) of the Arctic Ocean. Moscow: KMK Scientific Press.
Sanchez Gomez-Leon, A., and Mejia-Piña, K. (2018). “Limitada preservación de carbonato de calcio en una laguna costera tropical del Golfo de California,” in Estado Actual del Conocimiento del Ciclo del Carbono y sus Interacciones en México: Síntesis a 2018. Serie Síntesis Nacionales, eds F. Paz, A. Velazquez, M. Rojo (Texcoco: Programa Mexicano del Carbono-Centro del Cambio Global y la Sustentabilidad en el Sureste, AC-Universidad Juárez Autónoma de Tabasco), 177–181.
Schouten, S., Wakeham, S. G., and Damsté, J. S. S. (2001). Evidencia de oxidación anaeróbica de metano por arqueas en aguas euxínicas del Mar Negro. Geoquímica orgánica 32, 1277–1281. doi: 10.1016/S0146-6380(01)00110-3
Sentz, E. (1962). Étude morphologique des Serpulidae Hydroides norvegica (Gunnerus) et Serpula concharum Langerhans et de leurs forms intermédiares. Vie et Milieu 13, 441–452.
Seurat, L. G., and Dieuzeide, R. (1933). Sur la présence de I'Oncidiella celtica Cuvicr sur les côtes algériennes. Bulletin des Travaux de la Station d'Aquiculture et de Pêche de Castiglione 1–10.
Simboura, N., and Nicolaidou, A. (2001). The polychaetes (Annelida, Polychaeta) of Greece: checklist, distribution and ecological characteristics. Monogr. Marine Sci. 4:106.
Solís-Weiss, V. (1998). Atlas de anélidos poliquetos de la plataforma continental del Golfo de México. México: DF Universidad Nacional Autónoma de México. Instituto de Ciencias del Mar y Limnología. Informe final SNIBCONABIO proyecto, (P052).
Soulier, A. (1902). Revision des Annélides de la région de Cette, fas. i. Trav. de instit. de Zoologie de Montpellier et de la Station maritime de Cette Mémoire (10).
Sun, Y., ten Hove, HA., and Qiu, J. (2012). Serpulidae (Annelida: Polychaeta) from Hong Kong. Zootaxa 3424, 1–42. doi: 10.11646/zootaxa.3424.1.1
Sun, Y., Wong, E., ten Hove, H. A., Hutchings, P. A., Williamson, J. E., and Kupriyanova, E. K. (2015). Revision of the genus Hydroides (Annelida: Serpulidae) from Australia. Zootaxa 4009, 1–99. doi: 10.11646/zootaxa.4009.1.1
Taramelli-Rivosecchi, E., and Chimenz-Gusso, c. (1970). Nuove ricerche sul fouling del porto di Civitavecchia. II. Osservazioni sulle com unità incrostanti piastre m etalliche verniciate, im m erse a vai re profondità. Rend. Ac. Naz. XL ser. IV. 20, 1–20.
Taramelli-Rivosecchi, E., and Chimenz-Gusso, C. (1972). Nuove ricerche sul fouling del porto di Civitavecchia. III. osservazioni sulle biocenosi incrostanti substra ti di m ateriali diversi. Rend. Acc. Naz. XL ser. IV. 22, 1–16.
Taramelli-Rivosecchi, E., and Chimenz-Gusso, c. (1976). “Etudes sur la salissure m arine et sur les perforants du bois dans le port de Civitavecchia,” in Proc. Int. Congr. Mar. Corr. and Fauling (Antibes-Juan-les-Pins), 513–518.
Ten Hove, H. A. (1974). Notes on Hydroides elegans (Haswell, 1883) and Mercierella enigmatica Fauvel, 1923. alien serpulid polychaetes introduced into the Netherlands. Bull. Zool. Mus. Amsterdam 4, 45–51.
Ten Hove, H. A., and Jansen-Jacobs, M. J. (1984). “A revision of the genus Crucigera (Polychaeta; Serpulidae); a proposed methodical approach of serpulids, with special reference to variation in Serpula and Hydroides,” in Proceedings of the First International Polychaete Conference, ed P. A. Hutchings (Sydney: The Linnean Society of New South Wales), 143–180.
Ten Hove, H. A., and Van den Hurk, P. (1993). A review of recent and fossil serpulid “reef”, actuopalaentology and the “Upper Malm” serpulid limestone in NW Germany. Geology 72, 23–67.
Ten Hove, H. A., and Wolf, P. S. (1984). “Chapter 55 Family Serpulidae Johnston, 1865,” in Taxonomic Guide to the Polychaetes of the Northern Gulf of Mexico. Final report to the Minerals Management Service, Contract 14-12-001-29091, eds J. M. Uebelacker, and P. G. Johnson (Alabama: Barry A Vittor & Ass Inc Mobile), 1–34.
Ten Hove, H. A., and Kupriyanova, E. K. (2009). Taxonomy of Serpulidae (Annelida, Polychaeta): the state of affairs. Zootaxa 2036, 1–126. doi: 10.11646/zootaxa.2036.1.1
Ten Hove, H. A., and Eliahu, B. (2005). On the identity of Hydroides priscus Pillai 1971-taxonomic confusion due to ontogeny in some serpulid genera (Annelida: Polychaeta: Serpulidae). Senckenbergiana Biol. 85, 127–145.
Terrados, J., and Ros, J. D. (1991). Production dynamics in a macrophyte-dominated ecosystem: The Mar Menor coastal lagoon (SE Spair). Oecol. Aqu. 10.
Trott, T. J. (2004). Cobscook Bay inventory: a historical checklist of marine invertebrates spanning 162 years. Northeast Nat. 11, 261–324. doi: 10.1656/1092-6194(2004)11[261:CBIAHC]2.0.CO;2
Unabia, C. R. C., and Hadfield, M. G. (1999). Role of bacteria in larval settlement and metamorphosis of the polychaete Hydroides elegans. Marine Biol. 133, 55–64. doi: 10.1007/s002270050442
Vega, Á. J., Robles, Y. A., and Torres, L. (2014). Anàlisis Biòlogico y Pesquero de la extracciòn del poliqueto Americonuphis reesei en el golfo de Montijo, Pacifico Panameño. Tecnosciencia. 16, 77–93. Available online at: https://revistas.up.ac.pa/index.php/tecnociencia/article/view/1193
Velasco, J., Lloret, J., Millán, A., Marin, A., Barahona, J., Abellán, P., et al. (2006). Nutrient and particulate inputs into the Mar Menor lagoon (SE Spain) from an intensive agricultural watershed. Water Air Soil Pollut. 176, 37–56. doi: 10.1007/s11270-006-2859-8
Verrill, A. E. (1873). Results of the recent dredging expeditions on the coast of New England. Am. J. Sci. Arts 6, 435–441. doi: 10.2475/ajs.s3-5.26.98
Villalobos-Guerrero, T. F., Tovar-Hernández, M. A., and Tovar-Hernàndez, M. A. (2014). Poliquetos errantes (Polychaeta: Errantia) esclerobiontes del puerto de Mazatlán, Sinaloa (México). Boletín de Investigaciones Marinas y Costeras 43, 43–87. doi: 10.25268/bimc.invemar.2014.43.1.30
Vuillemin, S. (1965). Contribution à l'étude écologique du lac de Tunis. Biologie de Mercierella enigmatica Fauvel. (Ph. D. Thèse). Faculté des Sciences, Paris.
Wicks, L. C., and Roberts, M. J. (2012). “Benthic invertebrates in a high-CO2 world,” in Oceanography and Marine Biology - An Annual Review, eds R. N. Gibson, R. J. A. Atkinson, J. D. M. Gordon, and R. N. Hughes, (Milton Park: Taylor & Francis), 127–188.
Zibrowius, H. (1971). Les espèces Méditerranéennes du genre Hydroides (Polychaeta Serpulidae). Remarques sur le prétendu polymorphisme de Hydroides uncinata. Téthys 2, 691–746.
Zibrowius, H. (1991). Ongoing modification of the Mediterranean marine fauna and flora by the establishment of exotic species. Mésogée 51, 83–107.
Zibrowius, H. (1992). Ongoing modification of the Mediterranean fauna and flora by the establishment of exotic species. Mésogée 51, 83–107.
Keywords: eutrophication, invasive species, coastal lagoon, biogenic aggregation, serpulidae
Citation: Sandonnini J, Del Pilar Ruso Y, Cortés Melendreras E and Giménez Casalduero F (2021) Massive Aggregations of Serpulidae Associated With Eutrophication of the Mar Menor, Southeast Iberian Peninsula. Front. Mar. Sci. 7:531726. doi: 10.3389/fmars.2020.531726
Received: 01 February 2020; Accepted: 10 December 2020;
Published: 21 January 2021.
Edited by:
Susana Carvalho, King Abdullah University of Science and Technology, Saudi ArabiaReviewed by:
Eduardo Lopez Garcia, Autonomous University of Madrid, SpainAscensão Ravara, University of Aveiro, Portugal
Copyright © 2021 Sandonnini, Del Pilar Ruso, Cortés Melendreras and Giménez Casalduero. This is an open-access article distributed under the terms of the Creative Commons Attribution License (CC BY). The use, distribution or reproduction in other forums is permitted, provided the original author(s) and the copyright owner(s) are credited and that the original publication in this journal is cited, in accordance with accepted academic practice. No use, distribution or reproduction is permitted which does not comply with these terms.
*Correspondence: Francisca Giménez Casalduero, francisca.gimenez@ua.es