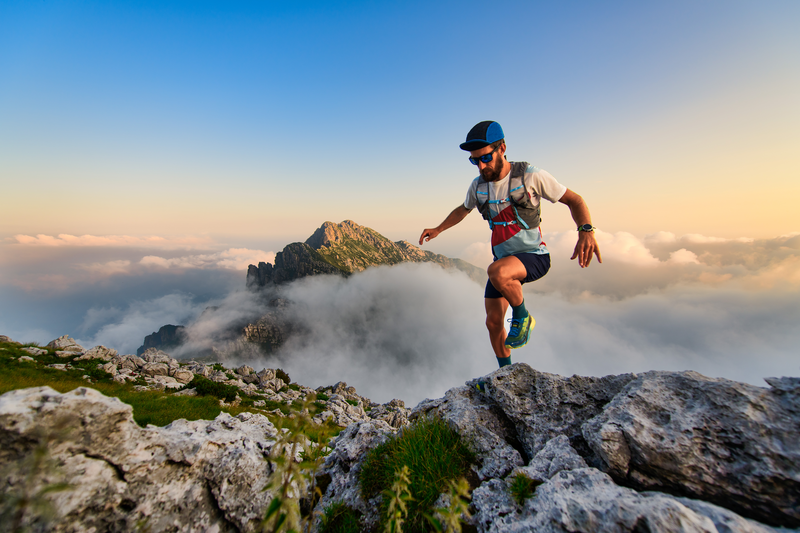
94% of researchers rate our articles as excellent or good
Learn more about the work of our research integrity team to safeguard the quality of each article we publish.
Find out more
ORIGINAL RESEARCH article
Front. Mar. Sci. , 17 September 2020
Sec. Deep-Sea Environments and Ecology
Volume 7 - 2020 | https://doi.org/10.3389/fmars.2020.00701
This article is part of the Research Topic 7th International Symposium on Deep-Sea Corals View all 22 articles
Members of Scleraxonia Studer, 1887 are one of the most dominant megafaunal taxa on seamounts, but their diversity and spatial distribution are poorly known in the tropical Western Pacific. Among this group, the family Victorgorgiidae Moore et al., 2017 is typically characterized by josephinae clubs in their polyp tentacles and a remarkable purple color but remains one of the most poorly known scleraxonian taxa currently. Victorgorgiidae contains only the genus Victorgorgia López-González and Briand, 2002 and six species. Here we describe three new species of Victorgorgia, V. fasciculata sp. nov., V. iocasica sp. nov., V. flabellata sp. nov., and re-describe V. eminens Moore et al., 2017, based on samples collected from four seamounts in the tropical Western Pacific, and evaluate their phylogenetic position using sequence data of mtMutS and COI genes. These new species are distinguished from each other and congeners by the sclerite forms and sizes, colony characters and polyp arrangement, and particularly the sclerites in the polyps and medulla are found to be most informative. Phylogenetic analyses indicate that V. flabellata sp. nov. is the sister group of V. iocasica sp. nov., and V. eminens Moore et al., 2017 showed a close relationship with Victorgorgia sp. GU563313. However, genetic divergence at the species level was found to be inadequate for differentiation of some close species. Each of the four species was found only from a single seamount, suggesting limited biological connectivity among the four seamount gorgoniians. Our study increases our understanding of the species diversity of Victorgorgiidae, and highlights the need for further research on the diversity and zoogeography of the deep-sea gorgonians.
V. fasciculata sp. nov. urn:lsid:zoobank.org:act: 6CDEECC5-B96D-4DDF-95D2-32BEC3DBC938.
V. iocasica sp. nov. urn:lsid:zoobank.org:act: BB11D217-C298-401F-8E13-849C33B03905.
V. flabellata sp. nov. urn:lsid:zoobank.org:act: 2C0FCCF2-33B1-4C2D-AC8E-6737079422F4.
urn:lsid:zoobank.org:pub: 39AF43F8-C0C2-4670-BBA0-DC1A7F1FA01B.
The suborder Scleraxonia Studer, 1887 is a group of octocorals with an axis composed of fused or unfused sclerites (Bayer, 1981; Fabricius and Alderslade, 2001; Daly et al., 2007; Devictor and Morton, 2010). They are among the dominant megafaunal taxa in the hard-bottom environments from shallow water to the deep sea, particularly abundant and diverse on seamounts (e.g., Genin et al., 1986; Stocks, 2004). Scleraxonians can exhibit amazing planar structures or three-dimensional “trees,” function as framework builders, and provide habitats for a variety of invertebrates and fishes (e.g., Koslow et al., 2001; Heifetz, 2002; Buhl-Mortensen and Mortensen, 2005). However, the deficiency of taxonomic studies impedes the understanding of their diversity, phylogeny, zoogeography, as well as ecology (e.g., McFadden et al., 2006; Morgan et al., 2006; Parrish and Baco, 2007; Rogers et al., 2007).
To date, nine families (containing 31 genera and approximately 262 species) have been recognized within Scleraxonia Studer, 1887. Among these, six families possess unconsolidated axes (Anthothelidae Broch, 1916; Briareidae Gray, 1859; Moore et al., 2017; Spongiodermidae Wright and Studer, 1889; Subergorgiidae Gray, 1859; Paragorgiidae Kükenthal, 1916), and three have consolidated axes (Coralliidae Lamouroux, 1812; Melithaeidae Gray, 1870; Parisididae Aurivillius, 1931) (Aurivillius, 1931; López-González and Gili, 2001; Crowther, 2011; Cairns and Wirshing, 2015; Figueroa and Baco, 2015; Moore et al., 2017).
Members of Victorgorgiidae Moore et al., 2017 are restrictedly distributed in deep waters (López-González and Briand, 2002; Moore et al., 2017). Victorgorgia López-González and Briand, 2002, currently the only genus of Victorgorgiidae, is characterized by monomorphic polyps, an extensive boundary space between medulla and cortex, large coelenteric canals in the central medulla, and typical josephinae clubs in tentacles (López-González and Briand, 2002; Moore et al., 2017). The genus Victorgorgia now contains six species: V. josephinae López-González and Briand, 2002 from NE Atlantic at water depth 1,500 m; V. alba (Nutting, 1908) from Hawaii at depths 1,394–1,829 m; V. argentea (Studer, 1894) from the eastern Pacific (off the coast of Mexico) and Hawaii at depths 1,200–1,559 m; V. macrocalyx (Nutting, 1911) from Indonesia at depths 1,165–1,264 m; V. eminens Moore et al., 2017 and V. nyahae Moore et al., 2017 from Southern Australia at depths 899–1,854 m and 590–660 m, respectively (López-González and Briand, 2002; Moore et al., 2017). Among these, only V. josephinae López-González and Briand, 2002, V. eminens Moore et al., 2017 and V. nyahae Moore et al., 2017 were recorded in vivo and all possessed a striking purple color or a variation of purple (López-González and Briand, 2002; Moore et al., 2017).
During the survey on the benthos in the tropical Western Pacific in 2016 and 2019, we collected six remarkable purple gorgonian specimens of Victorgorgia from four seamounts. Based on morphological and molecular phylogenetic analyses, these specimens are described and illustrated as three new and one previously known species. It is worthy of note that each of the species was collected from a single seamount. Their genetic distances and phylogenetic relationships within Victorgorgia were analyzed and discussed. Furthermore, the diagnostic characteristics of Victorgorgia species are summarized.
Specimens were collected by the Remotely Operated Vehicle submersible (ROV) FaXian (Discovery) from four seamounts in the tropical Western Pacific during the cruises of the R/V KEXUE (Science) in 2016 and 2019. The four seamounts are located on the Caroline Ridge (named unofficially as “M5,” “M6,” and “M8”) and near the Mariana Trench (named unofficially as “M2”) (Figure 1). The specimens were photographed in situ before being sampled by the ROV. Post collection, the specimens were immediately photographed and then fixed in 70% ethanol for morphological studies. Small branches were stored at −80°C for DNA Extraction. All examined specimens are deposited in the Marine Biological Museum of Chinese Academy of Sciences (MBMCAS) at Qingdao, China.
Figure 1. Sampling sites of the studied Victorgorgia spp. from seamounts in the tropical Western Pacific.
General morphology and anatomy were studied by means of a stereo dissecting microscope (Zeiss SteREO Discovery. V12). Sclerites were isolated respectively from the polyp tentacles, point and collaret, calyces, cortex and medulla by digestion of the tissues in sodium hypochlorite, and then were washed with distilled water and 70% ethanol. To investigate the ultrastructure of sclerites, sclerites were air-dried, mounted on carbon double adhesive tape, and coated for the Scanning Electron Microscope (SEM). SEM scans were obtained using Hitachi TM3030Plus SEM at 15 kV and the optimum magnification for each kind of sclerites. For each sample, about 40 sclerites were photographed and measured to cover as many shape types, ornament variations and size range as possible. Typical sclerites are illustrated in Figure 2.
Figure 2. Common sclerites found in Victorgorgia López-González and Briand, 2002. (A,B) Josephinae clubs from the tentacle rachis. (C) Club from the tentacle rachis. (D,E) Squat tuberculate rods from the tentacle rachis. (F) Thorn club from the tentacle rachis. (G) Tuberculate stick from the pinnules. (H,I) Flat jagged rods from the pinnules. (J) Cross from the pinnules. (K–R) Smooth to heavily tuberculated spindles and sticks from the points, collarets, calyces, cortex and medulla. Scale bar = 100 μm.
Total genomic DNA was extracted from the polyps of each specimen using the DNeasy Blood and Tissue Kit (Qiagen, Hilden, Germany) following the manufacturer’s instructions. PCR amplifications for the mitochondrial genomic regions 5′-end of the DNA mismatch repair protein − mutS − homolog (mtMutS) was conducted using primers AnthoCorMSH (5′-AGGAGAATTATTCTAAGTATGG-3′; Herrera et al., 2010) and Mut-3458R (5′-TSGAGCAAAAGCCACTCC-3′; Sánchez et al., 2003) as follows: denaturation at 98°C for 2 min, followed by 32 cycles of denaturation at 98°C for 20 s, annealing at 50°C for 20 s, extension at 72°C for 15 s, and a final extension step at 72°C for 2 min. PCR amplification for the 5′-end of the cytochrome coxidase subunit I (COI) were conducted using primers COI8414-F (5′-CCAGGTAGTATGTTAGGRGA-3′; McFadden unpubl.) and HCO2198 (5′-TAAACTTCAGGGTGACCAAAAAATCA-3′; Folmer et al., 1994) as follows: denaturation at 98°C for 2 min, followed by 32 cycles of denaturation at 98°C for 20 s, annealing at 60°C for 20 s, extension at 72°C for 15 s, and a final extension step at 72°C for 2 min. PCR reactions were performed using I-5TM 2 × High-Fidelity Master Mix DNA polymerase (TsingKe Biotech, Beijing, China), and sequencing were performed by TsingKe Biological Technology (TsingKe Biotech, Beijing, China).
The mtMutS and COI may be the most variable mitochondrial genes in octocorals (Herrera et al., 2010; McFadden et al., 2010; Li et al., 2017), and we selected these markers for molecular identification and phylogenetic analyses. All the available mtMutS and COI sequences of Victorgorgia spp. and the out-group species from Anthothela-like genera were downloaded from GenBank. The sequences containing sequencing errors or ambiguous positions (normally marked with “n” or “y” in the original sequences) were omitted from the molecular analyses. To correct possible mistakes, all the selected sequences were visually inspected, and translated to amino acids (AA) to insure all the AA sequences not including stop codons and suspicious substitutions. The nucleotide and AA sequences were aligned using MAFFT v.7 (Katoh and Standley, 2013) with the G-INS-i algorithm. With the guidance of the AA alignment, the nucleotide alignment was refined and trimmed to equal length using BioEdit v7.0.5 (Hall, 1999), and only the nucleotide alignment was used in the subsequent analyses. Genetic distances, calculated as uncorrected “p” distances within each species and among species, were estimated using MEGA 6.0 (Tamura et al., 2013).
For the phylogenetic investigation, the alignment datasets of the mtMutS and the concatenated regions of mtMutS and COI (MutS-COI) were created. The evolutionary model HKY85 + G was the best-fitted model for the both alignments, selected by AIC as implemented in jModeltest2 (Darriba et al., 2012). Maximum likelihood (ML) analysis was carried out using PhyML-3.1 (Guindon et al., 2010). For the ML bootstraps, we consider values <70% as low, 70–94% as moderate and ≥95% as high following Hillis and Bull (1993). Node support came from a majority-rule consensus tree of 1,000 bootstrap replicates. Bayesian inference (BI) analysis was carried out using MrBayes v3.2.3 (Ronquist and Huelsenbeck, 2003) on CIPRES Science Gateway. Posterior probability was estimated using four chains running 10,000,000 generations sampling every 1,000 generations. The first 25% of sampled trees were considered burn-in trees. Convergence was assessed by checking the standard deviation of partition frequencies (<0.01), the potential scale reduction factor (ca. 1.00), and the plots of log likelihood values (no obvious trend was observed over time). For the Bayesian posterior probabilities, we consider values <0.95 as low and ≥0.95 as high following Alfaro et al. (2003). The GenBank accession numbers of the mtMutS and COI sequences were listed next to the species names in Table 1.
Table 1. Interspecific and intraspecific uncorrected pairwise distances at mtMutS (lower left) and COI (upper right) of Victorgorgia species.
The new names established in the manuscript conform to the International Code of Zoological Nomenclature (ICZN). This article and the nomenclatural acts have been registered in ZooBank, the online registration system for the ICZN. The ZooBank LSIDs (Life Science Identifiers) can be resolved and the associated information can be viewed through any standard web browser by appending the LSID to the prefix http://zoobank.org/. The LSID for this publication is: urn:lsid:zoobank.org:pub: 39AF43F8-C0C2-4670-BBA0-DC1A7F1FA01B. The electronic edition of this article was published in a journal with an ISSN, has been archived, and is available from the digital repository PubMed Central. Terminology of the genus Victorgorgia López-González and Briand, 2002 follows Moore et al. (2017).
Phylum Cnidaria Hatschek, 1888
Class Anthozoa Ehrenberg, 1834
Subclass Octocorallia Haeckel, 1866
Order Alcyonacea Lamouroux, 1812
Suborder Scleraxonia Studer, 1887
Family Victorgorgiidae Moore et al., 2017
Victorgorgia López-González and Briand, 2002: 98; Moore et al., 2017: 130.
Monomorphic scleraxonians with arborescent colonies which can be uniplanar or multiplanar; anastomoses absent or rare. Medulla extensively penetrated by large, well-defined coelenteric canals, and separated from a thin cortex by a boundary space formed by anastomosing boundary canals. Calyces always clumped at the branch tips and sometimes also clumped along the branches; usually arranged all over branches in multiplanar colonies, with tendency to be biserially arranged or toward one side of the branches in uniplanar colonies. Josephinae clubs typically present in tentacle rachis and pinnules, but squat rods may also occur. Sclerites from points, calyces, cortex and medulla mainly tuberculate sticks and spindles. Sclerites absent from pharynx.
Victorgorgia josephinae López-González and Briand, 2002, by monotypy.
The generic diagnosis mainly follows Moore et al. (2017), but some characters are redefined (in italics). For example, the colony of the genus is either uniplanar or multiplanar (Table 2). The calyces are always clumped at the branch tips, and also clumped at points along the branches in six of the ten species; the calyces of multiplanar colonies are usually scattered around branches, and those of uniplanar colonies distributed on the three sides of the branches (Table 2). Josephinae clubs are typically present in tentacle rachis and pinnules of some species, but squat rods may be the dominant tentacle sclerites in other species [e.g., V. iocasica sp. nov., V. flabellata sp. nov., V. alba (Nutting, 1908) and V. macrocalyx (Nutting, 1911)].
Table 2. Comparison of species of Victorgorgia López-González and Briand, 2002.
Figures 3, 4 and Supplementary Figures S1–S4.
Figure 3. Morphology of Victorgorgia fasciculata sp. nov. (A) In situ. (B) The specimens when collected, showing contracted polyps in situ. (C) The newly collected holotype, photographed by Xuwen Wu. (D) The holotype in preservation. (E) An enlarged branch of the holotype. (F) The paratype. (G) Cross-section of terminal medulla. (H) Lateral view of polyp head of the holotype. (I) SEM of polyp head of the holotype. Laser dots spaced at 33 cm used for measuring dimensions (A,B). Scale bars = 5 cm (D,F), 1 cm (E), 1 mm (G), 0.5 mm (H), and 0.2 mm (I).
Figure 4. SEM of tentacle and sclerites of Victorgorgia fasciculata sp. nov. (A) Part of tentacle, showing arrangement of sclerites. (B) Sclerites from tentacles of the holotype. (C) Sclerites from point and collaret. (D) Sclerites from calyces of the holotype. (E) Sclerites from cortex of the holotype. (F) Sclerites from medulla of the holotype. (G) Sclerites from tentacles of the paratype. Scale bars = 100 μm.
MBM286395, collected on 26 March 2016 from the station FX-Dive 69 (11°17.23′N, 139°24.16′E) on a seamount (M2) near the Mariana Trench at the water depth of 475 m (temperature 7.24°C; salinity 36.5), rocky bottom.
MBM286409, one specimen, collection data same as the holotype.
Arborescent colony, dichotomous, approximately 260 mm long and 175 mm wide in preservation (Figures 3A–D). Holdfast uncollected. Main stem nearly circular, diameter 18.8–20.5 mm, approximate 36 mm long before two large side branches arise. The large side branches are laterally compressed, the larger one 15.1 mm wide and 18.2 mm deep, the smaller one about 13.1 mm wide and 17.0 mm deep. Terminal branches 15–45 mm in length, 3.0–6.0 mm in diameter exclusive of polyp clumps. Polyp clumps usually contain 10–20 polyps and the clumps 10–15 mm in width. Anastomoses absent. Medulla composed of tightly packed sclerites, separated from cortex by boundary space that consists of anastomoses of boundary canals (Figure 3G). Medulla in terminal branches usually perforated by 1–4 main canals (about 0.2–0.7 mm in diameter), around which sometimes occurred a few smaller, indistinct canals (Figure 3G). Cortex thin, usually 60–200 μm thick.
Polyps scattered around branches and at right angles. Calyces distinct, usually clumped both at branch tips and along branches, resulting in their uneven distribution with notable polyp-free regions (Figures 3D,E). Polyps contracted, and most fully retracted into calyces in preservation, resulting in rounded polyp heads with tentacles folded over the mouths (Figures 3A–E,H,I). In preservation, polyp heads approximately 2.5–4.3 mm wide, and up to 4.5 mm long in partly expanded ones; calyces approximately 3.0–5.0 mm wide and 1.5–3.2 mm long. Each side of tentacles with a row of about 7–9 pinnules, with the middle pinnules the largest.
Tentacles mainly armed with josephinae clubs that have simple tubercles and particularly narrow, mostly smooth handles, ranging 188–419 μm in length. These sclerites densely arranged on the aboral side of the tentacles with the clubbed ends orientated toward tentacle tips; some straight clubs projecting longitudinally into pinnules, small flat rods and lightly tuberculate sticks also common in the pinnules (Figures 4A,B and Supplementary Figure S1). At base of polyp head, some sclerites transversely arranged to form collaret (Figure 3H). The sclerites then grading en chevron up into the points that continue longitudinally along the aboral side of the tentacles (Figures 3H,I). The point and collaret sclerites generally straight to curved tuberculate spindles and sticks ranging 579–771 μm long (Figure 4C and Supplementary Figure S2A). Calyces crowded with tuberculate spindles and sticks, usually 375–788 μm long; most of them straight to slightly curved, and equipped with sparse tubercles (Figure 4D and Supplementary Figure S2B). Cortex with straight to slightly curved tuberculate sticks and spindles, usually 357–800 μm long (Figure 4E and Supplementary Figure S3A). Medulla composed mostly of straight to slightly curved tuberculate sticks and spindles, along with some smooth spindles, occasionally warty forms and fused spindles; sizes ranging 320–960 μm long (Figure 4F and Supplementary Figure S3B).
Polyps and coenenchyme deep purple in vivo and in freshly collected specimen of the holotype, polyps taupe and coenenchyme beige in ethanol preservation (Figure 3). All sclerites transparent and colorless under transmitted light.
The paratype measures 255 mm long and 155 mm wide, shows almost the same characteristics as the holotype in both colony form and sclerites (Figures 3A,B,F, 4G and Supplementary Figure S4).
The Latin adjective fasciculata (fasciculate) refers to the distinct autozooid clumps of the species.
Found only on a seamount (M2) near the Mariana Trench in the tropical Western Pacific (Figure 1), where the water depth was about 475 m, temperature about 7.2°C, and salinity was 36.5 psu. In situ, the specimens attached on a rocky bottom, and each was inhabited by an individual of the ophiuroid order Euryalida.
Among the known species of Victorgorgia López-González and Briand, 2002, V. fasciculata sp. nov. mostly resembles V. eminens Moore et al., 2017 from a morphological point of view. In both species, tentacle sclerites are mainly josephinae clubs, small flat rods, straight clubs, tuberculate sticks; the point and collaret sclerites are tuberculate spindles and sticks of similar sizes; medulla sclerites are mainly tuberculate sticks and spindles (Table 2). V. fasciculata sp. nov. differs from V. eminens Moore et al., 2017 by having somewhat larger tuberculate spindles and sticks in cortex (357–800 μm vs. 350–500 μm long) and slightly larger sclerites in calyces (375–788 μm vs. 220–670 μm long) (Figure 4). In fact, the morphological differences between the two species are very slight, and are probably insufficient to distinguish them. However, the mtMutS genetic distances between V. fasciculata sp. nov. and V. eminens are relatively high (1.39–1.54%, corresponding to 10–11 nucleotide differences), supporting their separation and the establishment of the new species (see the following section “Genetic Distance and Phylogenetic Analysis” for details).
The tentacles of V. alba (Nutting, 1908) and V. macrocalyx (Nutting, 1911) typically possess tuberculate rods, and those of V. argentea (Studer, 1894) and V. nyahae Moore et al., 2017 typically possess thorny clubs, which differ from the josephinae clubs in tentacles of V. fasciculata sp. nov. (Table 2 and Figure 4; Moore et al., 2017). The new species is differentiated from V. josephinae López-González and Briand, 2002 mainly by the polyp arrangement on the branches (clumped around branches vs. mainly isolated with tendency toward bisierial), and conenenchyme color (deep purple vs. yellowish) (Table 2; López-González and Briand, 2002).
Figures 5, 6 and Supplementary Figures S5, S6.
Figure 5. Morphology of Victorgorgia iocasica sp. nov. (A) In situ. Laser dots spaced at 33 cm used for measuring dimensions. (B) Close view in situ, showing expanded polyps. (C) The side with more autozooids of freshly collected specimen, photographed by Shaoqing Wang. (D) The side with fewer autozooids in preservation. (E) Branch tip with clumped autozooids. (F) Polyp in lateral view. (G) Cross-section of terminal medulla. Scale bars = 50 mm (C,D), 2 mm (E), and 1 mm (F,G).
Figure 6. Polyp, tentacle and sclerites of Victorgorgia iocasica sp. nov. (A) SEM of lateral view of polyp head. (B) Tentacle in digestion, showing the location and arrangement of sclerites in rachis and pinnules. (C) Sclerites from tentacles. (D) Sclerites from points and collarets. (E) Sclerites from calyces. (F) Sclerites from cortex. (G) Sclerites from medulla of the branch tip. (H) Sclerites from medulla of the branch close to main stem. Scale bars = 0.5 mm (A), and 100 μm (C–H).
MBM286391, collected on 13 June 2019 from the station FX-Dive 225 (10°36.73′N, 140°3.87′E) on M8 seamount at the water depth of 1,549 m, rocky bottom.
Uniplanar colony, dichotomous, approximately 329 mm long and 340 mm wide in preservation, broken into two pieces from base when collected (Figures 5A,C,D). Holdfast nearly circular, 53–72 mm in diameter, from which two main stems arising. The main stems somewhat compressed, the larger one 12 mm wide and 16 mm deep, the smaller one about 11 mm wide and 12 mm deep. Terminal branches 9–134 mm in length, 2–5 mm in diameter exclusive of polyp clumps. Polyp clumps usually contain 5–11 polyps and the clumps 9–14 mm in width. Anastomoses absent. The colony covered with a thin cuticle. Medulla separated from cortex by boundary space that consists of anastomoses of boundary canals, around which sometimes occurred a few smaller, indistinct canals (Figure 5G). Medulla in the terminal branches usually perforated by 3–6 main canals (0.2–0.5 mm in diameter) (Figure 5G). Cortex thin, usually 150–400 μm thick.
Polyps scattered around branches and at right angles, but one side almost devoid of them. Calyces distinct, mainly isolated, however, clumped and forming clavate clumps mainly at branch tips and rarely along branches (Figures 5C–E). Polyps expanded in live state (Figures 5A,B), contractile, but are not fully retracted into calyces when disturbed or in preservation, resulting in cylindrical polyp heads with tentacles folded over the mouths and bases sited on lips of calyces (Figures 5E,F). Polyp heads 2.0–3.0 mm wide and 1.5–4.2 mm long in preservation; calyces 2.0–4.5 mm wide and 1.0–3.8 mm long in preservation. Each side of tentacles with a row of 7–10 pinnules, with the middle ones largest.
Tentacles armed with mainly tuberculate rods and clubs ranging 267–565 μm in length (Figures 6A–C and Supplementary Figure S5A). Sclerites transversely arranged to form collaret at base of polyp head, and then grading en chevron up into the points that continue longitudinally along the aboral side of the tentacles (Figure 6A). The point and collaret sclerites generally straight to slightly curved sticks and spindles that are sparsely tuberculate, and mostly 306–544 μm long (Figure 6D and Supplementary Figure S5B). Calyces densely arranged with straight to somewhat curved tuberculate sticks, ranging 252–559 μm in length (Figure 6E and Supplementary Figure S5C). Cortex with straight to somewhat curved sticks, rods and spindles, the sclerites moderately tuberculate to almost smooth, and mostly ranging 324–559 μm in length (Figure 6F and Supplementary Figure S6A). Medulla of both the main stem and branch tips composed of spindles and sticks, spindles somewhat curved and almost smooth, sticks nearly straight and sparsely tuberculate, ranging 346–934 μm in length (Figures 6G,H and Supplementary Figures S6B,C).
Polyps and coenenchyme bright purple in vivo and in freshly collected specimen, and taupe in ethanol preservation (Figures 5A–D). All sclerites transparent and colorless under transmitted light.
Composite of IOCAS (the abbreviation of Institute of Oceanology, Chinese Academy of Sciences, Qingdao, China) and the Latin suffix icus (belonging to), in celebration of the 70th anniversary of the founding of the IOCAS.
Found only on a seamount (M8) located on the Caroline Ridge in the tropical Western Pacific, with the water depth about 1,549 m. In situ, the specimen attached on rocky bottoms, and was inhabited by individuals of the ophiuroid order Euryalida.
Victorgorgia iocasica sp. nov. is characterized by the mainly tuberculate rods instead of josephinae clubs in the tentacles, making it most resemble V. alba (Nutting, 1908) and V. macrocalyx (Nutting, 1911; Table 2). The new species is differentiated from V. alba (Nutting, 1908) by the absence of the large and bulky clubs in the points and collarets which are present in V. alba, and the calyx arrangement on branches (mainly isolated on three sides of branches in V. iocasica, while clumped around branches in V. alba); from V. macrocalyx (Nutting, 1911) by the quite tuberculate rods and clubs in the tentacle rachis in V. iocasica while V. macrocalyx has mostly short, flat rods with few, low tubercles, the more smooth and slender spindles and sticks in the medulla in V. iocasica, and the calyx arrangement on branches (on three sides of branches in V. iocasica instead of all around the branches as in V. macrocalyx (Figures 6G,H; Moore et al., 2017).
Figures 7, 8 and Supplementary Figures S7–S9.
Figure 7. Morphology of Victorgorgia flabellata sp. nov. (A) In situ. Laser dots spaced at 33 cm used for measuring dimensions. (B) Close view in situ, showing expanded polyps. (C) The side of the freshly collected holotype with fewer autozooids, photographed by Shaoqing Wang. (D) The side with more autozooids in preservation. (E) A retracted autozooid. (F,G) Lateral view of polyp head. (H) Cross-section of terminal medulla through a polyp. Scale bars = 5 cm (C,D), and 0.5 mm (E–H).
Figure 8. SEM of tentacle and sclerites of Victorgorgia flabellata sp. nov. (A) A tentacle, showing arrangement of sclerites. (B) Sclerites from tentacles. (C) Sclerites from points and collarets. (D) Sclerites from calyces. (E) Sclerites from cortex. (F) Sclerites from medulla. Scale bars = 0.2 mm (A), and 100 μm (B–F).
MBM286393, collected on 29 May 2019 from the station FX-Dive 211 (10°3.15′N, 140°10.57′E) on M5 seamount at the water depth of 1,408 m (temperature 3.3°C; salinity 36.5), rocky bottom.
Uniplanar colony, dichotomous, approximately 313 mm long and 342 mm wide in preservation (Figures 7A,C,D). Holdfast nearly rectangular, side length 24–39 mm. The main stem compressed, approximately 34 mm long, 22.5 mm wide, and 20.2 mm deep. Three large stems arising from the main stem. Terminal branches 10–120 mm in length, 1.9–4.1 mm in diameter exclusive of polyp clumps. Polyp clumps usually contain 3–10 polyps and the clumps 6–9 mm in width. Anastomoses absent. The colony covered with a thin cuticle. Medulla separated from cortex by boundary space that consists of anastomoses of boundary canals, around which sometimes occurred a few smaller, indistinct canals (Figure 7H). Medulla in the terminal branches usually perforated by 1–3 main canals (about 0.2–0.4 mm in diameter) (Figure 7H). Cortex thin, usually 50–200 μm thick.
Polyps distributed mainly on three sides of branches, with one side of colony almost devoid of them. Polyps on the lateral sides of the branches are more prominent than those placed on the face of the branches. Calyces distinct, usually clumped at branch tips, and sometimes along branches (Figures 7B–D). Polyps contractile, most partly to fully retractile into calyces, with a few expanded in preservation (Figures 7E–G). Polyp heads approximately 2.0–4.0 mm wide, and up to 3.5 mm long; calyces 3.0–5.0 mm wide and 2.0–3.5 mm long. Each side of tentacles with a row of 9–14 pinnules, with the middle ones the largest.
Sclerites transversely arranged to form collaret at base of polyp head, and then grading en chevron up into the points that continue longitudinally along the aboral side of the tentacles (Figures 7F,G, 8A and Supplementary Figure S7A). Tentacles armed with squat tuberculate rods, robust, thorn clubs, tuberculate clubs, small tuberculate rods and occasionally tuberculate crosses; sclerites ranging 182–792 μm in length (Figure 8B and Supplementary Figure S7B). The point and collaret sclerites generally straight to slightly curved tuberculate spindles and occasional warty sticks, ranging 550–908 μm long (Figure 8C and Supplementary Figure S8A). Calyces crowded with straight to slightly curved spindles and rods, and occasionally crosses, the sclerites moderately tuberculate to almost smooth with their tubercles rounded to conical, and mostly ranging 289–542 μm in length (Figure 8D and Supplementary Figure S8B). Cortex with slightly curved and almost smooth spindles, nearly straight and moderately tuberculate sticks, and shorter and warty rods; they mostly ranging 292–485 μm in length (Figure 8E and Supplementary Figure S9A). Medulla composed of straight to slightly curved tuberculate spindles and sticks, some furcated on the ends, and mostly ranging 323–915 μm in length (Figure 8F and Supplementary Figure S9B).
Polyps and coenenchyme deep purple in vivo and in freshly collected specimen, and taupe in ethanol preservation (Figures 7A–D). All sclerites transparent and colorless under transmitted light.
The Latin adjective flabellata (flabellate) refers to the planar shape of the colony.
Found on a seamount (M5) located on the Caroline Ridge in the tropical Western Pacific, with water depth of 1,408 m, water temperature about 3.3°C, and salinity at 36.5 psu. In situ, the specimen attached on rocky bottoms. The species was inhabited by individuals of the ophiuroid order Euryalida and the cnidarian order Actiniaria.
The tentacle sclerites of V. flabellata sp. nov. are typically squat tuberculate rods and thorn clubs, making it most similar to V. nyahae Moore et al., 2017. However, the new species differs from V. nyahae by the absence of the large thorn clubs in the points that V. nyahae has, and the fewer large thorn clubs in the tentacle rachis compared with the short thorn clubs in those of V. nyahae. Meanwhile, the top of polyp heads of V. nyahae are much spikier than those of V. flabellata sp. nov. due to the presence of the thorn clubs in the points. Moreover, V. flabellata sp. nov. differs from V. nyahae by the larger calyces width (3.0–5.0 mm vs. 1.4–2.6 mm), the calyx arrangement on branches (mainly isolated on three sides of branches vs. clumped around branches), the shorter calyx sclerites (maximum length 542 μm vs. 880 μm) and cortex sclerites (maximum length 485 μm vs. 710 μm), and the longer medulla sclerites (323–915 μm vs. 120–760 μm) (Table 2; Moore et al., 2017).
V. flabellata sp. nov. differs from V. alba (Nutting, 1908) by the absence of the long clubs with extended tips in the point (vs. presence), the much more diverse and larger sclerites in the tentacles (length 182–792 μm vs. 90–440 μm), the calyx arrangement on branches (mainly isolated on three sides of branches vs. clumped around branches), and the shorter sclerites in the calyces, cortex and medulla (Table 2; Moore et al., 2017).
V. flabellata sp. nov. differs from V. argentea (Studer, 1894) by the presence of thorn clubs in the tentacles (vs. absence), the much more tuberculate spindles and sticks (vs. sparsely tuberculated) and absence of bulky clubs (vs. presence) in the point, the calyx arrangement on branches (mainly isolated on three sides of branches vs. clumped around branches), and the shorter sclerites in the calyces, cortex and medulla (Table 2; Moore et al., 2017).
Both V. flabellata sp. nov. and V. iocasica sp. nov. are characterized by having a uniplanar colonies and squat tuberculate rods in the tentacles, but the former species possesses also robust josephinae clubs, thorn clubs, tuberculate clubs, small tuberculate rods and tuberculate crosses while the latter possesses mainly squat tuberculate rods in the tentacles (Figures 6C, 8B and Supplementary Figures S5A, S7B). Additionally, the medulla sclerites of V. flabellata are more tuberculate than those of V. iocasica (Figures 6G,H, 8F and Supplementary Figures S6B,C).
Figures 9–12 and Supplementary Figures S10–S14.
Figure 9. The specimen MBM286394 of Victorgorgia eminens Moore et al., 2017. (A) In situ. Laser dots spaced at 33 cm used for measuring dimensions. (B) Close view in situ, showing expanded polyps. (C) The freshly collected specimen, photographed by Shaoqing Wang. (D) The preserved specimen. (E) Retracted autozooids. (F) Cross-section of terminal medulla. Scale bars = 5 cm (C,D), 2 mm (E), and 0.5 mm (F).
Figure 10. SEM of polyps, tentacle and sclerites from the specimen MBM286394 of Victorgorgia eminens Moore et al., 2017. (A) Upper view of a polyp head. (B) Lateral view of a polyp head. (C) Part of tentacle, showing arrangement of sclerites. (D) Sclerites from tentacles. (E) Sclerites from point and collaret. (F) Sclerites from calyces. (G) Sclerites from cortex. (H) Sclerites from medulla. Scale bars = 0.5 mm (A,B), 0.2 mm (C), and 100 μm (D–H).
Figure 11. The specimen MBM286392 of Victorgorgia eminens Moore et al., 2017. (A) In situ. Laser dots spaced at 33 cm used for measuring dimensions. (B) Close view in situ, showing expanded polyps. (C) The freshly collected specimen, photographed by Shaoqing Wang. (D) The preserved specimen. (E) Polyp head in lateral view. (F) Cross-section of terminal medulla. Scale bars = 5 cm (C,D), and 1 mm (E,F).
Figure 12. SEM of polyps and sclerites from the specimen MBM286392 of Victorgorgia eminens Moore et al., 2017. (A) Upper view of a polyp head. (B) Lateral view of a polyp head. (C) Tentacular sclerites in situ. (D) Sclerites from tentacles. (E) Sclerites from point and collaret. (F) Sclerites from calyces. (G) Sclerites from cortex. (H) Sclerites from medulla. Scale bars = 0.5 mm (A,B), and 100 μm (C–H).
Victorgorgia eminens Moore et al., 2017: 164–178, figures 172–138.
MBM286394, collected on 7 June 2019 from the station FX-Dive 219 (10°6.68′N, 140°14.53′E) on M6 seamount at the water depth of 890 m (temperature 5.04°C; salinity 36.7), rocky bottom. MBM286392, collected on 6 June 2019 from the station FX-Dive 218 (10°7.23′N, 140°14.42′E) on M6 seamount at the water depth of 813 m (temperature 4.81°C; salinity 36.7), rocky bottom.
Colonies multiplanar, dichotomous, specimen MBM286394 measuring 420 mm long and 265 mm wide, and specimen MBM286392 approximately 395 mm long and 236 mm wide in preservation (Figures 9A–D). Holdfast appears nearly circular in the in situ image, but it was not collected with the colony. Main stem compressed, cross-section nearly rectangular, measuring 14 mm wide, 23 mm deep, and approximately 100 mm before the first branch arises. Terminal branches 15–150 mm in length, 2.2–3.5 mm in diameter exclusive of polyp clumps. Polyp clumps usually contain 6–12 polyps and the clumps 6–10 mm in width. Five anastomoses present among the colony branches of specimen MBM286394, and absent from specimen MBM286392. The colony covered with a thin cuticle. Medulla separated from cortex by boundary space that is composed of anastomoses of boundary canals, around which sometimes occurred a few smaller, indistinct canals (Figure 9F). Medulla in the terminal branches usually perforated by 1–3 main canals (0.3–0.5 mm in diameter) (Figure 9F). Cortex thin, usually 100–300 μm thick.
Polyps scattered around branches and at right angles. Calyces distinct, usually clumped around branches of specimen MBM286392, and commonly isolated around branches of MBM286394 (Figures 9B–E, 11B–E). In preservation, polyps contractile, and most fully retractile into calyces in specimen MBM286394, and almost fully exsert in specimen MBM286392 (Figures 9E, 11E). Polyp heads usually 2.0–4.0 mm wide, and up to 7.0 mm long in expanded ones; calyces usually 3.0–5.0 mm wide and 2.0–3.5 mm long. Each side of tentacles with a row of about 7–10 pinnules, with the middle ones largest.
Tentacle rachis mainly armed with tuberculate josephinae clubs ranging 158–529 μm in length, the tubercles at the heads of josephinae clubs conical and those at the handles rounded; these sclerites densely arranged on the aboral side of the tentacles with the clubbed ends orientated toward tentacle tips. Straight clubs, tuberculate sticks and flat jagged rods commonly present in pinnules, and flat tuberculate crosses rarely detected (Figures 10A–D, 12A–D and Supplementary Figures S10, S13A). At base of polyp head, some sclerites transversely arranged to form collaret. The sclerites then grading en chevron up into the points that continue longitudinally along the aboral side of the tentacles (Figures 10B, 12B). The point and collaret sclerites generally straight to slightly curved tuberculate spindles ranging 282–659 μm long (Figures 10E, 12E and Supplementary Figures S11A, S13B). Calyces crowded with straight to slightly curved spindles, most of them provided with rounded to elliptical tubercles, and ranging 292–670 μm long (Figures 10F, 12F and Supplementary Figures S11B, S14A). Cortex with straight to slightly curved tuberculate to warty spindles and sticks, covered with simple to complex tubercles, and usually 314–520 μm long; the sclerites with simple tubercles are similar to those from calyces (Figures 10G, 12G and Supplementary Figures S12A, S14B). Medulla composed of straight to slightly curved tuberculate spindles and sticks, similar to those from cortex, and mostly ranging 300–794 μm in length (Figures 10H, 12H and Supplementary Figures S12B, S14C).
Polyps and coenenchyme purple in vivo, freshly collected specimens close to pink in specimen MBM286394 and red purple in specimen MBM286392, polyps became taupe and coenenchyme beige in ethanol preservation (Figures 9A–D, 11A–D). All sclerites transparent and colorless under transmitted light.
Our specimens were found on a seamount (M6) located on the Caroline Ridge in the tropical Western Pacific, where the water depths were 813–890 m, water temperature 4.8–5.0°C, and salinity about 36.7 psu. In situ, all specimens attached on rocky bottoms, and the species was always inhabited by individual(s) of the echinoderm order Euryalida. The species is widely distribted from the Southwestern Pacific to the tropical Northwestern Pacific.
Our specimens of Victorgorgia eminens Moore et al., 2017 show differences in anastomoses, polyp retraction and cortex sclerites (some spindles of the specimen MBM286394 are more tuberculate than those from the specimen MBM286392). The variations are considered as intraspecific. Our specimens are recognized as V. eminens, known from the southeastern Australia, due to similarities in colony shape, sclerite forms and sizes of all the parts to that of the holotype of V. eminens (Figures 9–12 and Supplementary Figures S10–S14; Moore et al., 2017). Furthermore, the genetic distances among the V. eminens specimens are also very low (0–0.15%), mirroring the high morphological similarity.
V. eminens typically possesses the josephinae clubs in the tentacles, making it distinguishable from V. alba (Nutting, 1908) and V. macrocalyx (Nutting, 1911) that typically possess tuberculate rods, from V. josephinae López-González and Briand, 2002 that has large josephinae clubs, and from V. nyahae Moore et al., 2017 that typically possess thorny clubs in the tentacles. It differs from V. argentea (Studer, 1894) by the absence of bulky clubs in the points and collarets. Additionally, V. eminens differs from V. josephinae López-González and Briand, 2002 by the thicker and less tuberculate sclerites in the medulla, the smaller sclerites in all examined parts, and the conenenchyme color (deep purple vs. yellowish) (Table 2; López-González and Briand, 2002). V. fasciculata sp. nov. differs morphologically little from V. eminens, but they are separated from each other by molecular sequences (see remarks of V. fasciculata sp. nov.).
A total of 12 sequences from the four species were deposited in GenBank and their accession numbers are provided in Table 1. The alignments of mtMutS, COI and the concatenated mtMutS-COI had lengths of 661, 526, and 1,187 bp, respectively. The inter- and intra-specific genetic divergences of mtMutS and COI were calculated to investigate the genetic distances in Victorgorgia. For the mtMutS alignment, the interspecific distances range from zero to 4.31%, the intraspecific distances are in range of 0–0.15% (Table 1). The genetic distance between V. iocasica sp. nov. and V. flabellata sp. nov is zero. For the COI alignment, the interspecific distances range from zero to 0.95%, the intraspecific distances are in range of 0–0.38% (Table 1). Except for V. eminens MT269897 and V. nyahae, the genetic distances among the other Victorgorgia specimens are zero.
The phylogenetic reconstructions of concatenated mtMutS-COI loci showed quite similar topologies compared to the mtMutS trees and provided higher resolution (Figure 13 and Supplementary Figure S15). Hence, only the topologies of the concatenated sequences are provided here for checking the intrageneric relationships of Victorgorgia. The Victorgorgia species formed a monophyletic clade in both ML and BI trees with low support (ML 62%, BI 0.84). In the ML tree, all the Victorgorgia species were separated into three subclades: (1) Victorgorgia flabellata sp. nov. clustered with V. iocasica sp. nov., followed by V. nyahae Moore et al., 2017 with low support (ML 38%); (2) V. fasciculata sp. nov. independently formed another subclade; (3) V. eminens Moore et al., 2017 and Victorgorgia sp. GU563313 formed a sister subclade with full node support. The BI tree was highly similar to the ML one except for that V. nyahae Moore et al., 2017 formed an independent subclade within Victorgorgia.
Figure 13. Maximum likelihood (ML) and Bayesian inference (BI) trees based on concatenated sequences mtMutS-COI showing phylogenetic relationships among the available Victorgorgia species. Newly sequenced species are in bold.
Both the morphology and molecular phylogenetic analyses support the assignment of the four species to the genus Victorgorgia. The genetic distance analysis of mtMutS and COI is considered as one of the first steps in an integrative identification of octocorals (McFadden et al., 2011). In the present study, nucleotide variation showed that the gene region COI was more conserved than mtMutS for Victorgorgia species (Table 1). Except for V. eminens Moore et al., 2017 MT269897 and V. nyahae KT366865, there is no variation for the rest of COI sequences, indicating its relative usefulness for species delimitation of Victorgorgia species.
Maximum intraspecific distances of mtMutS and minimum interspecific ones have been used to separate Octocorallia species, with the distances greater than 1% confidently used to indicate cryptic species (McFadden et al., 2010; Herrera et al., 2012; Li et al., 2017). Based on this threshold, the establishment of V. fasciculata sp. nov. is supported on basis of the relatively high genetic distances (1.08%). Although there are no distinct morphological differences between the two specimens of V. nyahae described by Moore et al. (2017), they have 24 nucleotide differences (genetic p distance >2%) in mtMutS, indicating that there may be a cryptic species similar to V. nyahae. However, there is no genetic variation between V. iocasica sp. nov. and V. flabellata sp. nov., which are differentiated by the types of tentacle sclerites and the ornamentations of medulla sclerites (Figures 5–8 and Supplementary Figures S5A, S6B,C, S7B, S9B). For those close species without genetic variation, here we summarize the taxonomic characters, such as the colony form, polyp arrangement, and sclerite forms and sizes for the species discrimination.
The vivid purple color is the most striking and recognizable characteristic of Victorgorgia. As all the three known species whose live color were recorded were entirely or partially purple-colored, and the three new species are entirely purple-colored, this character can be used in situ for preliminary identification, and probably a stable taxonomic character of the genus.
Consistent with Moore et al. (2017), the sclerites in the polyps are found to be most diverse and informative, and therefore contribute most to species delineation. In contrast, sclerites from calyx, cortex and medulla are relatively uniform with just ornamental and dimensional variations, but are also useful for species differentiation.
Anastomoses are present in one of the two specimens of V. eminens Moore et al., 2017 known from the tropical Western Pacific, and are more common in the specimens from southeastern Australia, but are absent from all other species of the genus according to the information that we currently have. Therefore, it cannot be taken as a stable character to define species.
Polyp arrangement on branches varies from scattered around branches, distributed on three sides of branches, to almost biserially arranged. According to our observation, all the three species (V. iocasica sp. nov., V. flabellata sp. nov., and V. josephinae) with uniplanar colonies possess polyps distributed on three sides, with one side is almost devoid of polyps and the polyps occurring along the sides of the branches are prominent, while all the other species with multiplanar colonies possess polyps scattered around branches (Table 2). The calyces clumped at the branch tips in all the species of the genus, and usually clumped along the branches in multiplanar colonies.
The ability of a polyp to retract into a calyx is judged by its condition in preservation. The polyps can be retracted or exsert even in the same colony, let alone their variation in different colonies of a species. So it is evidently not a useful diagnostic character at species level.
Medullary canals and boundary space are generic traits used to separate Victorgorgia from the related genera, such as Anthothela Verrill, 1879, Lateothela Moore et al., 2017 and Williamsium Moore et al., 2017. These two characters vary little among Victorgorgia species, so they cannot be utilized to differentiate species.
Among the nine currently known species of Victorgorgia, V. josephinae López-González and Briand, 2002 is the only species recorded from the Atlantic Ocean (López-González and Briand, 2002). All the other species were from the Pacific Ocean: V. macrocalyx (Nutting, 1911) and the three new species from the central Western Pacific; V. alba (Nutting, 1908) from the middle Pacific (Hawaii); V. nyahae Moore et al., 2017 from the Southwestern Pacific (Tasman Sea); V. eminens Moore et al., 2017 from the Southwestern Pacific (Tasman Sea) and the tropical Northwestern Pacific; and V. argentea (Studer, 1894) is the only species distributed in both the Eastern and middle Pacific (Moore et al., 2017; Table 1). In addition, Victorgorgia fasciculata sp. nov. is the shallowest record of the genus (475 m depth), V. eminens Moore et al., 2017 being the deepest record (1,854 m depth).
According to the distribution, it seems that Victorgorgia is widely but sparsely distributed in the deep-sea hard bottom environments. It is noteworthy that the four species were each obtained from a single seamount without overlap, which may indicate a poor biological connectivity among these adjacent seamounts. But the samples are too few to elucidate the hypothesis. More research is needed to evaluate the species diversity and geographic distribution of these deep-sea gorgonians.
In the present study, all the specimens of Victorgorgia were inhabited by individual(s) of the echinoderm order Euryalida (Ophiouridea), and occasionally inhabited by individuals of the cnidarian order Actiniaria. The symbiotic association between Victorgorgia species and euryalids and actiniarians is considered as epibiosis that is defined as “a spatial association between a substrate organism (basibiont) and a sessile organism (epibiont) attached to the basibiont’s outer surface without trophically depending on it” (Wahl, 2009). According to studies on related taxa (e.g., Mosher and Watling, 2009), it is probably a mutually beneficial relationship between the basibionts (Victorgorgia species) and the epibionts (euryalids and actiniarians). The euryalids and actiniarians use gorgonians as a perch for suspension feeding, and probably defend gorgonians from predators and other possible symbionts. The gorgonians seem not to be harmed by the epibionts, and no gorgonian tissues or sclerites were found from the stomach contents of euryalids. The advantages and disadvantages for both Victorgorgia species and their epibionts need to be studied in detail.
We describe three new and one known species, V. fasciculata sp. nov., V. iocasica sp. nov., V. flabellata sp. nov., and V. eminens Moore et al., 2017, from seamounts in the tropical Western Pacific. Based on descriptions of the fully developed and integrally collected specimens, the taxonomic significance of the main characters of Victorgorgia are discussed, with the sclerites in the polyps are reconfirmed to be the most informative characteristic for species delineation. It is found that the level of genetic variation in mtMutS and COI is too low to distinguish close species of Victorgorgia, so more effective markers are necessary for further phylogenetic analysis. The Pacific has the highest species diversity and nine of the ten known Victorgorgia species are distributed here. The results suggest limited biological connectivity among seamount gorgonians and calls for the need of more research on the diversity and distribution of these animals.
The datasets presented in this study are available from online repositories. The names of the repositories and accession numbers can be found in the article/Supplementary Material.
YL, KX, and ZZ conceived and designed the research. KX conducted the sampling. YL studied the morphology. ZZ performed the molecular analysis. All authors contributed to the writing and editing of the final manuscript.
This work was supported by the Science & Technology Basic Resources Investigation Program of China (2017FY100804), the National Natural Science Foundation of China (41930533 and 31970489), and the Senior User Project of RV KEXUE.
The authors declare that the research was conducted in the absence of any commercial or financial relationships that could be construed as a potential conflict of interest.
We thank the assistance of the crew of R/V KEXUE and ROV FaXian for sample collection and our colleagues Mr. Shaoqing Wang and Dr. Xuwen Wu for photographing freshly collected specimens on board. We appreciate Ms Sadie Mills and Di Tracey (National Institute of Water and Atmospheric Research, New Zealand) for helpful identification and editorial revisions. We also appreciate the editor SH (National University of Colombia, Colombia) for his editorial work and three reviewers for their constructive comments on an early version of the manuscript.
The Supplementary Material for this article can be found online at: https://www.frontiersin.org/articles/10.3389/fmars.2020.00701/full#supplementary-material
FIGURE S1 | SEM of tentacle and tentacle sclerites from the holotype of Victorgorgia fasciculata sp. nov. (A) Part of tentacle, showing arrangement of sclerites. (B) Sclerites from tentacles. Scale bars = 100 μm.
FIGURE S2 | Sclerites from the holotype of Victorgorgia fasciculata sp. nov. (A) Sclerites from points and collarets. (B) Sclerites from calyces of the holotype. Scale bars = 100 μm.
FIGURE S3 | Sclerites from the holotype of Victorgorgia fasciculata sp. nov. (A) Sclerites from cortex of the holotype. (B) Sclerites from medulla of the holotype. Scale bars = 100 μm.
FIGURE S4 | Sclerites from tentacles of the paratype of Victorgorgia fasciculata sp. nov. Scale bar = 100 μm.
FIGURE S5 | Sclerites of Victorgorgia iocasica sp. nov. (A) Sclerites from tentacles. (B) Sclerites from points and collarets. (C) Sclerites from calyces. Scale bars = 100 μm.
FIGURE S6 | Sclerites of Victorgorgia iocasica sp. nov. (A) Sclerites from cortex. (B) Sclerites from medulla of the branch tip. (C) Sclerites from medulla of the branch close to main stem. Scale bars = 100 μm.
FIGURE S7 | SEM of tentacle and tentacle sclerites from Victorgorgia flabellata sp. nov. (A) A tentacle, showing arrangement of sclerites. (B) Sclerites from tentacles. Scale bars = 0.2 mm (A), and 100 μm (B).
FIGURE S8 | Sclerites of Victorgorgia flabellata sp. nov. (A) Sclerites from points and collarets. (B) Sclerites from calyces. Scale bars = 100 μm.
FIGURE S9 | Sclerites of Victorgorgia flabellata sp. nov. (A) Sclerites from cortex. (B) Sclerites from medulla. Scale bars = 100 μm.
FIGURE S10 | SEM of tentacle and tentacle sclerites from the specimen MBM286394 of Victorgorgia eminens Moore et al., 2017. (A) Part of tentacle, showing arrangement of sclerites. (B) Sclerites from tentacles. Scale bars = 0.2 mm (A), and 100 μm (B).
FIGURE S11 | Sclerites from the specimen MBM286394 of Victorgorgia eminens Moore et al., 2017. (A) Sclerites from points and collarets. (B) Sclerites from calyces. Scale bars = 100 μm.
FIGURE S12 | Sclerites from the specimen MBM286394 of Victorgorgia eminens Moore et al., 2017. (A) Sclerites from cortex. (B) Sclerites from medulla. Scale bars = 100 μm.
FIGURE S13 | Sclerites from the specimen MBM286392 of Victorgorgia eminens Moore et al., 2017. (A) Sclerites from tentacles. (B) Sclerites from points and collarets. Scale bars = 100 μm.
FIGURE S14 | Sclerites from the specimen MBM286392 of Victorgorgia eminens Moore et al., 2017. (A) Sclerites from calyces. (B) Sclerites from cortex. (C) Sclerites from medulla. Scale bars = 100 μm.
FIGURE S15 | Maximum likelihood (ML) and Bayesian inference (BI) trees based on mtMutS showing phylogenetic relationships among the available Victorgorgia species. Newly sequenced species are in bold.
Alfaro, M. E., Zoller, S., and Lutzoni, F. (2003). Bayes or Bootstrap? A simulation study comparing the performance of Bayesian Markov chain Monte Carlo sampling and bootstrapping in assessing phylogenetic confidence. Mol. Biol. Evol. 20, 255–226. doi: 10.1093/molbev/msg028
Aurivillius, M. (1931). The Gorgonarians from Dr. Sixten Bock’s expedition to Japan and Bonin Islands 1914. Kungliga Svenska Vetenskapsakademiens Handlingar. Stockholm: Almqvist & Wiksell.
Bayer, F. M. (1981). Key to the genera of Octocorallia exclusive of Pennatulacea (Coelenterata: Anthozoa) with diagnoses of new taxa. Proc. Biol. Soc. Washington 94, 902–947.
Broch, H. (1916). Results of Dr E. Mjobergs Swedish Scientific Expeditions to Australia 1910–1913 XI. Alcyonarien. Kungliga Svenska Vetenskapsakademiens Handlingar. Stockholm: Almqvist & Wiksell.
Buhl-Mortensen, L., and Mortensen, P. B. (2005). “Distribution and diversity of species associated with deep-sea gorgonian corals off Atlantic Canada,” in Cold-Water Corals and Ecosystems, eds A. Freiwald and J. M. Roberts (Berlin: Springer-Verlag), 849–879. doi: 10.1007/3-540-27673-4_44
Cairns, S. D., and Wirshing, H. H. (2015). Phylogenetic reconstruction of scleraxonian octocorals supports the resurrection of the family Spongiodermidae (Cnidaria, Alcyonacea). Invertebr. Syst. 29, 345–368. doi: 10.1071/is14063
Crowther, A. L. (2011). “Class anthozoa ehrenberg, 1834,” in Animal Biodiversity: An Outline of Higher-Level Classification and Survey of Taxonomic Richness, ed. Z.-Q. Zhang (Auckland: Zootaxa), 19–23. doi: 10.11646/zootaxa.3148.1.5
Daly, M., Brugler, M. R., Cartwright, P., Collins, A. G., Dawson, M. N., Fautin, D. G., et al. (2007). The phylum Cnidaria: a review of phylogenetic patterns and diversity 300 years after Linnaeus. Zootaxa 1668, 127–182. doi: 10.11646/zootaxa.1668.1.11
Darriba, D., Taboada, G. L., Doallo, R., and Posada, D. (2012). jModelTest 2: more models, new heuristics and parallel computing. Nat. Methods 9:772. doi: 10.1038/nmeth.2109
Devictor, S. T., and Morton, S. L. (2010). Identification guide to the shallow water (0–200 m) octocorals of the South Atlantic Bight. Zootaxa 2599, 1–62. doi: 10.11646/zootaxa.2599.1.1
Ehrenberg, C. G. (1834). Die Corallienthiere Des Rothen Meeres. Berlin: Abhandlungen der Königlichen Akademie der Wissenschaften zu.
Fabricius, K., and Alderslade, P. (2001). Soft Corals and Sea Fans: A Comprehensive Guide to the Tropical Shallow Water Genera of the Central-West Pacific, the Indian Ocean and the Red Sea. Townsville: Australian Institute of Marine Science.
Figueroa, D. F., and Baco, A. R. (2015). Octocoral mitochondrial genomes provide insights into the phylogenetic history of gene order rearrangements, order reversals, and cnidarian phylogenetics. Genome Biol. Evol. 7, 391–409. doi: 10.1093/gbe/evu286
Folmer, O., Black, M., Hoeh, W., Lutz, R., and Vrijenhoek, R. (1994). DNA primers for amplification of mitochondrial cytochrome c oxidase subunit I from diverse metazoan invertebrates. Mol. Mar. Biol. Biotechnol. 3, 294–299.
Genin, A., Dayton, P. K., Lonsdale, P. F., and Spiess, F. N. (1986). Corals on seamount peaks provide evidence of current acceleration over deep-sea topography. Nature 322, 59–61. doi: 10.1038/322059a0
Gray, J. E. (1859). On the arrangement of zoophytes with pinnated tentacles. Ann. Mag. Nat. Hist. 4, 439–444. doi: 10.1080/00222935908697159
Gray, J. E. (1870). Catalogue of Sea-Pens or Pennatulidae in the Collection of the British Museum. London: British Museum.
Guindon, S., Dufayard, J. F., Lefort, V., Anisimova, M., Hordijk, W., and Gascuel, O. (2010). New algorithms and methods to estimate maximum-likelihood phylogenies: assessing the performance of PhyML 3.0. Syst. Biol. 59, 307–321. doi: 10.1080/10635150390235520
Haeckel, E. (1866). Generelle Morphologie der Organismen. Allgemeine Grundzüge der organischen Formen-wissenschaft, mechanisch begründet durch die von Charles Darwin reformirte Descendenz-Theorie. Berlin: Georg Reimer.
Hall, T. A. (1999). BioEdit: a user-friendly biological sequence alignment editor and analysis program for Windows 95/98/NT. Nucleic Acids Symp. Ser. 41, 95–98.
Hatschek, B. (1888). Lehrbuch der Zoologie, eine morphologische Übersicht des Thierreiches zur Einführung in das Studium dieser Wissenschaft. Jena: Gustav Fischer.
Heifetz, J. (2002). Coral in Alaska: distribution, abundance, and species associations. Hydrobiologia 471, 19–28.
Herrera, S., Baco, A., and Sánchez, J. A. (2010). Molecular systematics of the bubblegum coral genera (Paragorgiidae, Octocorallia) and description of a new deep-sea species. Mol. Phylogenet. Evol. 55, 123–135. doi: 10.1016/j.ympev.2009.12.007
Herrera, S., Shank, T. M., and Sánchez, J. A. (2012). Spatial and temporal patterns of genetic variation in the widespread antitropical deep-sea coral Paragorgia arborea. Mol. Ecol. 21, 6053–6067. doi: 10.1111/mec.12074
Hillis, D. M., and Bull, J. J. (1993). An empirical test of bootstrapping as a method for assessing confidence in phylogenetic analysis. Syst. Biol. 42, 182–192. doi: 10.1093/sysbio/42.2.182
Katoh, K., and Standley, D. M. (2013). MAFFT Multiple Sequence Alignment Software version 7: improvements in performance and usability. Mol. Biol. Evol. 30, 772–780. doi: 10.1093/molbev/mst010
Koslow, J. A., Gowlett-Holmes, K., Lowry, J. K., O’Hara, T., Poore, G. C. B., and Williams, A. (2001). Seamount benthic macrofauna off southern Tasmania: community structure and impacts of trawling. Mar. Ecol. Prog. Ser. 213, 111–125. doi: 10.3354/meps213111
Kükenthal, W. (1916). System und stammesgeschichte der Scleraxioner und der ursprung der Holaxonier. Zool. Anzeiger 47, 170–183.
Lamouroux, J. V. F. (1812). Extrait d’un mémoire sur la classification des Polypiers coralligènes non entièrement pierreux. Nouveau Bull. Sci. Soc. Philos. 3, 181–188.
Li, Y., Zhan, Z., and Xu, K. (2017). Morphology and molecular phylogeny of Paragorgia rubra sp. nov. (Cnidaria: Octocorallia), a new bubblegum coral species from a seamount in the tropical Western Pacific. Chinese J. Oceanol. Limnol. 35, 803–814. doi: 10.1007/s00343-017-5320-5
López-González, P. J., and Briand, P. (2002). A new scleraxonian genus from Josephine Bank, north-eastern Atlantic (Cnidaria, Octocorallia). Hydrobiologia 482, 97–105.
López-González, P. J., and Gili, J. M. (2001). Rosgorgia inexspectata, new genus and species of Subergorgiidae (Cnidaria, Octocorallia) from off the Antarctic Peninsula. Polar Biol. 24, 122–126. doi: 10.1007/s003000000176
McFadden, C. S., Benayahu, Y., Pante, E., Thoma, J. N., Nevarez, P. A., and France, S. C. (2011). Limitations of mitochondrial gene barcoding in Octocorallia. Mol. Ecol. Resour. 11, 19–31. doi: 10.1111/j.1755-0998.2010.02875.x
McFadden, C. S., France, S. C., Sánchez, J. A., and Alderslade, P. (2006). A molecular phylogenetic analysis of the Octocorallia (Cnidaria: Anthozoa) based on mitochondrial protein-coding sequences. Mol. Phylogenet. Evol. 41, 513–527. doi: 10.1016/j.ympev.2006.06.010
McFadden, C. S., Sanchez, J. A., and France, S. C. (2010). Molecular phylogenetic insights into the evolution of Octocorallia: a review. Interg. Comp. Biol. 50, 389–410. doi: 10.1093/icb/icq056
Moore, K. M., Alderslade, P., and Miller, K. J. (2017). A taxonomic revision of Anthothela (Octocorallia: Scleraxonia: Anthothelidae) and related genera, with the addition of new taxa, using morphological and molecular data. Zootaxa 4304, 1–212. doi: 10.11646/zootaxa.4304.1.1
Morgan, L. E., Tsao, C.-F., and Guinotte, J. M. (2006). Status of Deep Sea Corals in US Waters. Washington, DC: Marine Conservation Biology Institute.
Mosher, C. V., and Watling, L. (2009). Partners for life: a brittle star and its octocoral host. Mar. Ecol. Prog. Ser. 397, 81–88. doi: 10.3354/meps08113
Nutting, C. C. (1908). Descriptions of the Alcyonaria collected by the U.S. Bureau of Fisheries steamer “Albatross” in the vicinity of the Hawaiian Islands in 1902. Proc. U.S. Natl. Museum 34, 543–601. doi: 10.5962/bhl.title.49592
Nutting, C. C. (1911). The Gorgonacea of the Siboga Expedition. VIII. The scleraxonia. Siboga Expeditie 13b, 1–62.
Parrish, F. A., and Baco, A. R. (2007). “State of deep coral ecosystems in the U.S. Pacific Islands region: Hawaii and the U.S. Pacific Territories,” in The State of Deep Coral Ecosystems of the United States, eds S. E. Lumsden, T. F. Horigan, A. W. Bruckner, and G. Dorr (Silver Spring, MD: NOAA Tech), 155–194.
Rogers, A. D., Baco, A. R., Griffiths, A., Hart, H., and Hall-Spencer, T. (2007). “Corals on Seamounts,” in Seamounts: Ecology, Conservation and Management, eds T. J. Pitcher, T. Morato, P. J. B. Hart, M. R. Clark, N. Haggan, and R. S. Santos (Oxford: Blackwell), 141–169. doi: 10.1002/9780470691953.ch8
Ronquist, F. R., and Huelsenbeck, J. P. (2003). Mrbayes 3: bayesian phylogenetic inference under mixed models. Bioinformatics 19, 1572–1574. doi: 10.1093/bioinformatics/btg180
Sánchez, J. A., Lasker, H. R., and Taylor, D. J. (2003). Phylogenetic analyses among octocorals (Cnidaria): mitochondrial and nuclear DNA sequences (lsu-rRNA, 16S and ssu-rRNA, 18S) support two convergent clades of branching gorgonians. Mol. Biol. Evol. 29, 31–42. doi: 10.1016/s1055-7903(03)00090-3
Stocks, K. (2004). “Seamount invertebrates: composition and vulnerability,” in Seamounts: Biodiversity and Figsheries, eds T. Morato and D. Pauly (Vancouver: University of British Columbia).
Studer, T. (1887). Versuch eines systemes der alcyonaria. Arch. Nat. 53, 1–74. doi: 10.1515/9783111579535-004
Studer, T. (1894). Reports on the dredging operations off the west coast of Central America to the Galapagos, to the west coast of Mexico, and in the Gulf of California, in charge of Alexander Agassiz carried on by the U.S. Fish Commission Steamer “Albatross” during 1891. Bull. Museum Comp. Zool. 25, 53–69.
Tamura, K., Stecher, G., Peterson, D., Filipski, A., and Kumar, S. (2013). MEGA6: molecular evolutionary genetics analysis Version 6.0. Mol. Biol. Evol. 30, 2725–2729. doi: 10.1093/molbev/mst197
Verrill, A. E. (1879). Preliminary Check-List of the Marine Invertebrata of the Atlantic coast, from Cape Cod to the Gulf of St. Lawrence. [Prepared for the United States Commission of Fish and Fisheries.]. Tuttle: Morehouse & Taylor.
Wahl, M. (2009). “Epibiosis: ecology, effects and defences,” in Marine Hard Bottom Communities, ed. M. Wahl (Washington, DC: Ecological Studies), 61–72.
Keywords: Victorgorgia, gorgonians, Scleraxonia, Alcyonacea, cnidaria, new species, biodiversity, seamount
Citation: Li Y, Zhan Z and Xu K (2020) Morphology and Molecular Phylogenetic Analysis of Deep-Sea Purple Gorgonians (Octocorallia: Victorgorgiidae) from Seamounts in the Tropical Western Pacific, with Description of Three New Species. Front. Mar. Sci. 7:701. doi: 10.3389/fmars.2020.00701
Received: 30 March 2020; Accepted: 31 July 2020;
Published: 17 September 2020.
Edited by:
Santiago Herrera, Lehigh University, United StatesReviewed by:
Kirrily M. Moore, Tasmanian Museum and Art Gallery (TMAG), AustraliaCopyright © 2020 Li, Zhan and Xu. This is an open-access article distributed under the terms of the Creative Commons Attribution License (CC BY). The use, distribution or reproduction in other forums is permitted, provided the original author(s) and the copyright owner(s) are credited and that the original publication in this journal is cited, in accordance with accepted academic practice. No use, distribution or reproduction is permitted which does not comply with these terms.
*Correspondence: Kuidong Xu, a3h1QHFkaW8uYWMuY24=
Disclaimer: All claims expressed in this article are solely those of the authors and do not necessarily represent those of their affiliated organizations, or those of the publisher, the editors and the reviewers. Any product that may be evaluated in this article or claim that may be made by its manufacturer is not guaranteed or endorsed by the publisher.
Research integrity at Frontiers
Learn more about the work of our research integrity team to safeguard the quality of each article we publish.