- 1Red Sea Research Center, King Abdullah University of Science and Technology, Thuwal, Saudi Arabia
- 2Division of Biological and Environmental Sciences and Engineering, King Abdullah University of Science and Technology, Thuwal, Saudi Arabia
Anthropogenic disturbances have led to the degradation of coral reef systems globally, calling for proactive and progressive local strategies to manage individual ecosystems. Although restoration strategies such as assisted evolution have recently been proposed to enhance the performance of coral reef populations in response to current and future stressors, scalability of these concepts and implementation in habitat or ecosystem-wide management remains a major limitation for logistical and financial reasons. We propose to implement these restoration efforts into an ecotourism approach that embeds land-based coral gardening efforts as architectural landscape elements to enhance and beautify coastal development sites, providing additional value and rationale for ecotourism stakeholders to invest. Our approach extends and complements existing concepts integrating coral reef restoration in ecotourism projects by creating a participatory platform that can be experienced by the public, while effectively integrating numerous restoration techniques, and providing opportunities for long-term restoration and monitoring studies. In this context, we discuss options for pre-selection of corals and systematic, large-scale monitoring of coral genotype performance targeting higher resilience to future stressors. To reduce operating costs during out-planting, we suggest to create coral seeding hubs, clusters of closely transplanted conspecifics, to quickly and efficiently restore/enhance active reproduction. We discuss our land-based coral gardening approach in the context of positive impacts beyond reef restoration. By restoring and strengthening resilience of local populations, we believe this strategy will contribute to a net positive conservation impact, create a culture on restoration and enhance and secure blue economical investments that rely on healthy marine systems.
Introduction
Coral reef systems have undergone severe global degradation and loss during the past decades (Wilkinson, 2004; De’ath et al., 2012; Hughes et al., 2017). They are predicted to experience a further loss of up to 99% of remaining reefs under moderate climate predictions (Bindoff et al., 2019). The development of new strategies for coral reef management have become a conservation imperative to secure the vital ecological and economical services they provide for the livelihoods of hundreds of millions of people (Moberg and Folke, 1999). The increasing frequency and severity of coral bleaching events (Hughes et al., 2003, 2017; Heron et al., 2016) has forced scientists to consider more radical interventions to mitigate the impacts of global warming and other cumulative anthropogenic stressors (van Oppen et al., 2015; Anthony et al., 2017; Damjanovic et al., 2017; van Oppen et al., 2017; National Academies of Sciences, Engineering, and Medicine, 2019). Although strategies such as assisted gene flow (Palumbi et al., 2014; Dixon et al., 2015) and selective breeding (van Oppen et al., 2015) target climate resilience, the biggest challenge of these efforts to date remains the identification of economically viable approaches to implement and scale-up restoration efforts to achieve a zero-net loss or, ideally, an increase in coral reef area (Duarte et al., 2020).
Coral reef restoration costs are amongst the highest for marine coastal habitat restoration efforts (Bayraktarov et al., 2016). Estimates based on scientific literature vary substantially with a median cost of 404,147 $US ha–1 (at base year 2010; Bayraktarov et al., 2019). However, these estimates have limitations as little data exists on the costs of non-scientific programs. These high costs, resulting from high labor intensity associated with coral restoration, limit the scale at which it is conducted, with a current median size of only 100 m2 (based on scientific literature; Bayraktarov et al., 2019) to 500 m2 per project (including both scientific and gray literature; Boström-Einarsson et al., 2018). Additionally, current projects seem to lack proportionate cost savings when production is increased (Bayraktarov et al., 2016). One of the biggest criticisms of coral restoration is the lack of meaningful scalability. While the expense may be high, reported survival rates of restoration efforts are considerable (64.5%; Bayraktarov et al., 2019). However, limitations of these estimations are the short observational period of most studies (median duration of 12 months) and lack of sustained monitoring to evaluate long-term success (Boström-Einarsson et al., 2020). Further, these estimates may be biased as failure in restoration is often not reported (Hobbs, 2009).
Motivations for coral restoration are diverse, ranging from biodiversity enhancement, social outcomes, scientific projects, to projects restoring or maintaining ecosystem services for fisheries, tourism, coastal protection among others (Bayraktarov et al., 2020). While ecological outcomes are often reported, reports on economic and social outcomes are often neglected (Bayraktarov et al., 2019). Aside from the ecological impacts at local scales, most coral reef restoration operators emphasize education and public awareness as a main benefit (Young et al., 2012; Hein et al., 2019). Involvement of the public in restoration through citizen-science projects reduces costs and adds socio-economic benefits, such as creating employment and generating stewardship for local coral habitats (dela Cruz et al., 2014; Lirman and Schopmeyer, 2016; Hein et al., 2018).
We advocate for an approach that builds upon the outreach potential of coral restoration efforts and integrates coral gardens into ecotourism and coastal development as architectural and landscape features that are shared and open to public participation. We believe that the use of land based, ex situ, coral gardens as landscape elements will be a powerful tool to educate and raise awareness to a greater audience while contributing to the scalability of coral reef restoration projects. Implementing coral garden efforts as an economic driver to attract visitors via the creation of unique citizen and visitor experiences, will provide additional values and rationale for hotels and tourism businesses to invest in coral reef restoration. This, will generate economic revenues that can be utilized to scale-up and optimize efforts. Our idea aligns with the goals of the “UN Decade of Ecological Restoration” (UNEP, 2019) starting in 2021 calling to create a culture toward ecological restoration.
As increasing ocean warming and marine heatwaves threaten coral reefs at a global scale (Hughes et al., 2017, 2018, Leggat et al., 2019), long-term success of coral restoration efforts relies on the tolerance of local populations for future climate scenarios. Using landscape embedded coral gardens as a platform, we discuss a structured approach integrating a systematic assessment and monitoring to optimize coral gardening efforts and to build local populations with higher resilience. Restoring and strengthening resilience will contribute to secure ecological and blue economical assets, those associated to a sustainable use of ocean resources which target the improvement of livelihoods while preserving the health of marine ecosystems (Spalding, 2016).
Coral Gardening
The most common and effective approach to coral reef restoration is coral gardening (Young et al., 2012). Corals are grown in an intermediate nursery phase, before being transplanted for restoration (Rinkevich, 1995). In the initial phase of the coral gardening, corals are fragmented or recruited and grown in sheltered sites, before they are transplanted at reasonable size to natural habitats in the second phase. Mid-shelf nurseries have been shown to be very successful in growing a variety of species (Levy et al., 2010; Rinkevich, 2014).
Land-based coral gardening efforts are less explored and have been mostly used for sexual reproduction of corals (O’Neil, 2015) or asexual reproduction via micro-fragmentation (Forsman et al., 2015). The great advantage of ex situ coral gardening facilities is the ability to engineer the environment to enhance growth and survival (Leal et al., 2016), and reduce costs by avoiding SCUBA diving during the gardening stage. Land-based coral nurseries have also been suggested to serve as genetic repositories (Schopmeyer et al., 2012). Providing easy access to the cultured organisms, they allow detailed monitoring of abiotic conditions (light and temperature), organism performance, and quick intervention if problems arise. Additionally, land-based nurseries allow the co-culturing of beneficial biota that reduce competing algae (Craggs et al., 2019) and mimic co-existing partnerships found in situ (within their natural environment), increasing coral health. To date there is a vast knowledge base of successful ex situ coral culture practices, within the aquaria industry, which can be optimized and possibly scaled-up (Leal et al., 2016).
Integration of Adaptive Management
Adaptive management refers to strategies, whereby practices get iteratively better overtime by incorporating and maximizing opportunities to learn from systematic monitoring (Holling, 1978; Hicks et al., 2009). With respect to adaptive coral gardening, examining stress resilience of the donor colonies and growth, survival, reproductive activity, etc., of propagated individuals can provide a feedback loop to inform and guide better selection of stocks. This strengthens populations on a site by site basis (Figure 1). Applying adaptive management strategies may ultimately help to reduce costs in the long term and make restoration efforts economically more viable. Using this strategy, restoration projects may accumulate vast datasets over time that allow informed and optimized restoration efforts and guide the development of innovative new approaches and technologies. This approach allows unprecedented, large-scale experimentation to advance knowledge on the drivers of resilience.
Ideally, restoration projects should record and characterize the original habitat and genotype of each donor/paternal colony (Johnson et al., 2011). Baums et al. (2019) showed that modern sequencing techniques provide a vast array of genotyping technologies, allowing to examine a wide spectrum of genotypic characteristics that may improve restoration efforts, such as symbiont association or genetic traits associated with phenotypic performance. Considering hidden species diversity is often found in corals (Schmidt-Roach et al., 2012), these technologies can also provide taxonomical confirmation, guarantying fertilization compatibility during sexual propagation.
The creation of detailed records is vital to carefully track the performance of transplantation efforts (IUCN, 2002; Boström-Einarsson et al., 2018). Records for each individual coral ramet transplanted should be cataloged and examined. We suggest employing Radio-frequency identification (RFID) transponders that can be attached/cemented into the base of ramets/juveniles. RFID transponders have been shown to be functional underwater (Benelli et al., 2009) and could provide an innovative and cheap way to track individuals during gardening efforts and after transplantation. Furthermore, conventional plastic/metal tags are esthetically not very appealing, add pollution and can also be hard to recover.
Although an increasing number of projects include sexual propagation (Horoszowski-Fridman et al., 2011; Linden and Rinkevich, 2011; Guest et al., 2014; Omori and Iwao, 2014; dela Cruz and Harrison, 2017; Linden and Rinkevich, 2017; Calle-Trivino et al., 2018), most coral restoration projects still rely on asexual reproduction (fragmentation) due to a lack of feasibility and scalability (Young et al., 2012). Careful consideration is required when choosing genotypes for restoration efforts to maintain genotypic diversity and avoid genotypic depletion by inbreeding (Baums, 2008). In this context, finding strategies for reliable tagging is vital in order to identify individual genotypes.
Enhancing Stress Resilience and Reproductive Activity
Selective reproduction/breeding has been suggested as a mechanism to build resilience toward climatic stressors (van Oppen et al., 2015; van Oppen et al., 2017). Selection of resilient genotypes could improve restoration efficiency, reduce costs and, ensure that restored populations do not meet the same fate as their predecessors. Although recommended in restoration guidelines (e.g., Baums et al., 2019; Coral Restoration Consortium, 2020), few projects report pre-selecting genotypes via an assessment of stress resilience before conducting nursery efforts (e.g., Morikawa and Palumbi, 2019; Fundemar and Iberostar case studies in Bayraktarov et al., 2020). Restoration efforts show genotypic driven phenotypic divergence in survivorship and response to thermal stress (Drury et al., 2017; Ladd et al., 2017), yet our understanding of which traits may assure resilience to future climate conditions remains rudimentary.
Strategies need to be identified that allow high-throughput and reliable phenotypic assessments to identify resilient genotypes. The spectrum of approaches used to identify suitable genotypes ranges from stress assessments (e.g., Morikawa and Palumbi, 2019), valuations of the genotypic performance based on habitat origin (Drury et al., 2017) or past bleaching/disease susceptibility, to genetic tools (Baums, 2008; Figure 1). Global warming is likely the most severe future stressor for coral populations (Hughes et al., 2017). A large amount of literature has focused on understanding the drivers of thermal tolerance (see Cziesielski et al., 2019). Thus, selection approaches should consider multi-stressor resilience, as bleaching responses may be coupled with other stressors (DeCarlo and Harrison, 2019).
Based on the current knowledge limitations, Baums et al. (2019) proposed to target the increase of genetic diversity in restoration efforts in order to maximize levels of standing genetic variation that may counteract future stressors. Incorporating sexual reproduction into restoration could have the biggest benefit, but is currently still limited due to feasibility at larger scales and high costs (Guest et al., 2014; Randall et al., 2020). New strategies have recently been proposed to enhance sexual propagation in restoration using seeding tiles that can be out planted with low efforts and cost (Chamberland et al., 2017). Although these techniques seem successful, they are limited to species that easily settle on artificial substrates. For coral species with larvae not easily settled ex situ, techniques such as direct seeding of depleted reefs with relocated larvae harvested ex situ (dela Cruz and Harrison, 2017) or wild-caught from coral larvae slicks (Doropoulos et al., 2019) may be applicable.
Most coral restorations approaches are very expensive as they try to recreate natural habitats often transplanting vast numbers of corals. In contrast to large scale transplantation, we suggest to revive/strengthen populations by restoring/enhancing reproductive activity using corals nurtured in land-based coral gardens. Coral seeding hubs (CSH), aggregations of conspecific corals transplanted within proximate distances to one another, may be a cheap and effective measure for coral restoration. Sperm seems to be the limiting factor in the sea, with decreasing sperm concentration over distance due to dilution resulting in lower fertilization rates (Levitan, 1993; Levitan and Petersen, 1995). Consequently, the number of conspecifics in a reef may be insufficient to guarantee successful reproduction after catastrophic events. Concentrating the number of reproductive conspecifics within the CSH could dramatically increase fertilization rates and reproductive success.
Shearer et al. (2009) estimated that ten randomly selected donor colonies could be sufficient to retain 50% of the original allelic diversity, while 35 colonies could provide 90%. Thus, a few closely transplanted genotypes may be sufficient to recover relatively high genetic diversity and contribute to standing genetic diversity. Corals seem to predominantly recruit locally (Miller and Mundy, 2003; Miller and Ayre, 2008) and, transplantation has been shown to increase recruitment (Maya et al., 2016). CSHs may be a powerful and cost-effective strategy to recover the reproductive potential within populations. Strategic placement, considering local current regimes, may lead to self-sustaining populations which can be quickly applied across different taxa. CSHs can be established for a diversity of taxa, supplied by the land-based gardens and linked via citizen science programs.
Informed selection of genotypes placed in the CSHs may increase the standing genetic diversity of local populations ultimately building up resilience. The advantage of this strategy over conventional restoration via direct large-scale transplantation is that the likelihood of sexual propagation is maximized and allows for natural selection to act on the offspring generated in the CSHs. Consequently, the genotypic architecture of a reef may be less altered than by conventional strategies.
Land-Based Coral Gardens as Blue Economical Assets
Ex situ based coral gardening efforts are predominantly concentrated within research or industrial settings in land-based facilities. Commercial efforts cater toward the aquarium industry (private and public), explore restoration as a business (e.g., CoralVita), or seek to engage guests in hotels (Hein et al., 2018). Although some operators facilitate partial public access, few systems are specifically built to cater to a greater audience including non-swimmers or non-divers. However, operators are starting to explore this avenue, as for example the Wave of Change project of the Iberostar Group in Bayahibe, Dominican Republic, which creates an ex situ, land-based, nursery that caters to both, research as well as educational purposes (Bayraktarov et al., 2020). Nevertheless, the outreach, entertainment and educative value to a greater public audience of coastal land-based coral gardens remains vastly unexplored. Arguably public aquaria cater toward this purpose, but their main role in coral conservation besides education has been to serve as repositories (Petersen et al., 2008) rather than to participate in active restoration.
In preparation for the UN Decade on Ecosystem Restoration aiming at enhancing the role of restoration as a path to rebuild blue natural capital (Duarte et al., 2020; Waltham et al., 2020), we propose to embed coral gardening efforts within coastal ecotourism and developmental projects at large scale (Figure 2). Powered by renewable energies, we envisage extended land-based coral garden nurseries with flow through seawater cascading through public spaces, essentially replacing flowerbeds with the ornamental values of reef organisms. In addition to beautification, this will directly expose citizens and visitors to the marine environment, thereby serving as a tool to create stewardship and awareness. The global tourism revenue of coral reefs is estimated at approximately $36 billion annually (Spalding et al., 2016, 2017), reflecting a large public interest for these unique ecosystems and a great economic potential if a larger audience of non-swimmers and non-divers could be engaged. Creating land-based coral gardens as a participatory platforms that can be experienced by the public, may be a tool to further explore the economic potential of coral reefs and generate economic profits for these areas (e.g., hotels, resorts, golf courses, and public parks) that host these unique elements. Resulting economic revenues and profits may entice private and corporate stakeholders to invest in these concepts and by default assist to scale-up and advance restoration efforts to achieve meaningful ecological outcomes. Adding to blue urbanism, practices of creating a mutually sustainable relationship between coastal populations and the marine environment (Beatley, 2014), this approach may ultimately assist to counteract the degradation of and species loss in coral reefs often observed due to coastal urbanization (Poquita-Du et al., 2019).
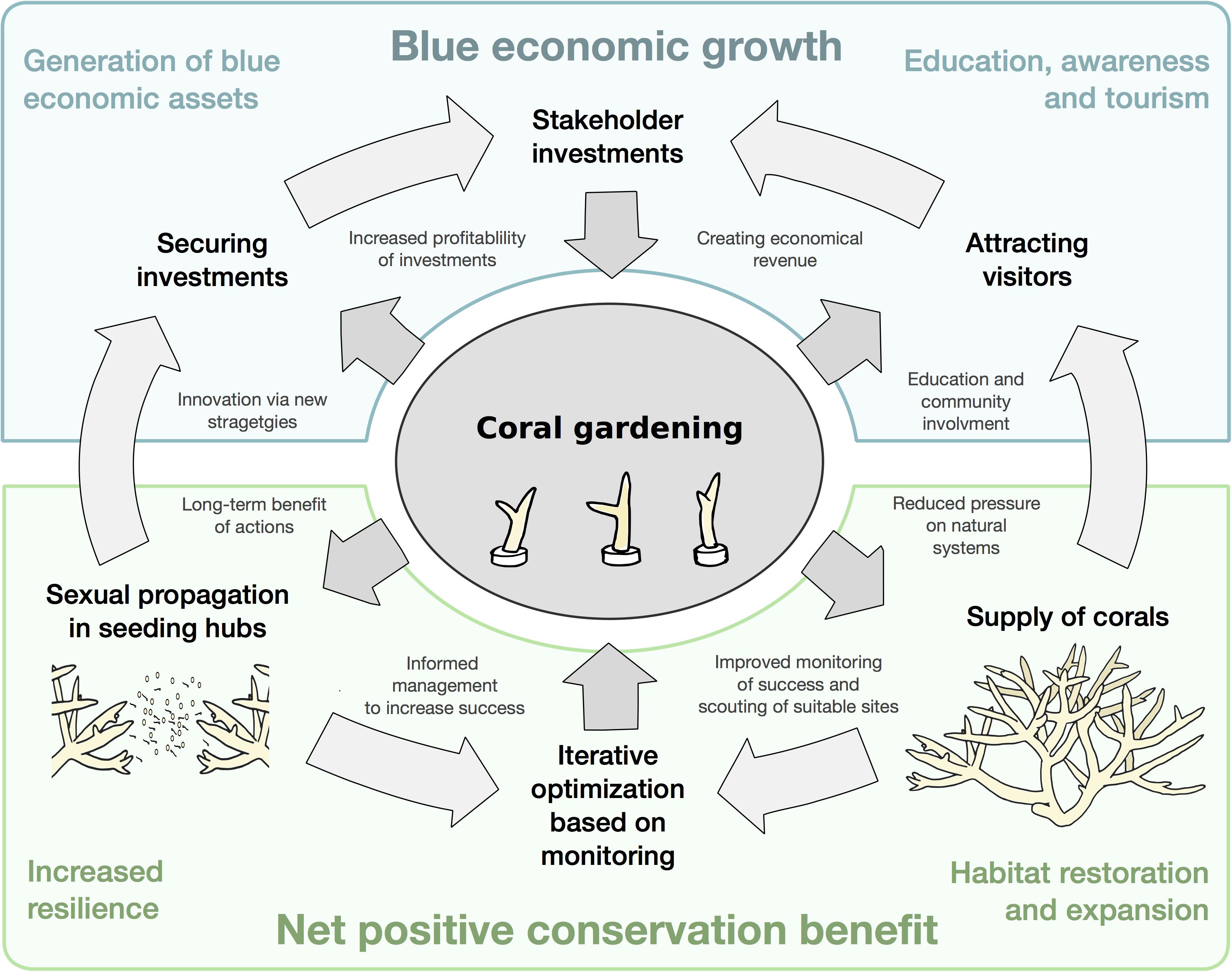
Figure 2. Land-based coral nurseries as an entity to serve toward blue economic growths as well as conservation.
In addition to socio-ecological benefits (Hein et al., 2018), restoring and maintaining healthy coral reef ecosystems will also contribute to preserving the services they provide for coastal protection and fisheries. Further, by enhancing awareness and changing the interactions between people and the ecosystem novel services can emerge (Woodhead et al., 2019). Creation of an interdisciplinary environment that reaches out to a variety of experts, from engineers to landscape architects, may ultimately drive innovation and foster the exploration of novel services. Consequently, generating coral garden landscaping as blue economic assets that support in situ restoration efforts may drive blue economic growth and help achieve net positive conservation benefits for coral reefs (Figure 2). Land-based coral nurseries have been suggested as ecological and economically viable solutions for scalable reef restoration (CoralVita, 2019). The novelty in our approach is the embedment of these efforts as landscape elements within ecotourism, developmental and urban landscapes, ultimately serving a triple mission: educate, restore, and enhance.
Conclusion
A global reduction of greenhouse gas (GHG) emissions would be the most effective and economically viable long-term strategy to mitigate climate change effects and protect vulnerable ecosystems such as coral reefs (Bindoff et al., 2019). Restoration efforts may have reduced effects in mitigating climate change impacts globally (Morrison et al., 2020). However, assuming the global community will comply with the GHG emission pathway, this will not suffice to either conserve coral reefs or restore their previous abundance. Hence, relying solely on decarbonization to counteract the degradation of tropical habitats is unrealistic (Gordon et al., 2020). Therefore, an action plan involving reduction of pressures, including climate change, pollution and overfishing, protection and active restoration provides the pathway to the best possible future for tropical coral reefs (Duarte et al., 2020).
Embedding coral gardening into architectural landscapes will integrate engineers, architects and urban developers, laying the foundation for an interdisciplinary community of practice that would be best able to deliver innovative solutions incorporating bio-printing, material science, architecture and design, as well as renewable energy and pathways to scalability of these solutions to coral reef restoration.
Author Contributions
SS-R drafted the manuscript. All authors read and commented on the final version.
Funding
This work was funded by King Abdullah University of Science and Technology.
Conflict of Interest
The authors declare that the research was conducted in the absence of any commercial or financial relationships that could be construed as a potential conflict of interest.
Acknowledgments
We would like to thank the two reviewers for constructive advice which helped to improve the manuscript.
References
Anthony, K., Bay, L. K., Costanza, R., Firn, J., Gunn, J., Harrison, P., et al. (2017). New interventions are needed to save coral reefs. Nat. Ecol. Evol. 1, 1420–1422.
Baums, I. B. (2008). A restoration genetics guide for coral reef conservation. Mol. Ecol. 17, 2796–2811. doi: 10.1111/j.1365-294x.2008.03787.x
Baums, I. B., Baker, A. C., Davies, S. W., Grottoli, A. G., Kenkel, C. D., Kitchen, S. A., et al. (2019). Considerations for maximizing the adaptive potential of restored coral populations in the western Atlantic. Ecol. Appl. 29:e01978.
Bayraktarov, E., Banaszak, A. T., Montoya Maya, P., Kleypas, J., Arias-González, J. E., Blanco, M., et al. (2020). Coral reef restoration efforts in Latin American countries and territories. bioRxiv [Preprint], doi: 10.1101/2020.02.16.950998
Bayraktarov, E., Saunders, M. I., Abdullah, S., Mills, M., Beher, J., Possingham, H. P., et al. (2016). The cost and feasibility of marine coastal restoration. Ecol. Appl. 26, 1055–1074. doi: 10.1890/15-1077
Bayraktarov, E., Stewart-Sinclair, P. J., Brisbane, S., Bostrom-Einarsson, L., Saunders, M. I., Lovelock, C. E., et al. (2019). Motivations, success, and cost of coral reef restoration. Restor. Ecol. 27, 981–991. doi: 10.1111/rec.12977
Beatley, T. (2014). Blue Urbanism: Exploring Connections Between Cities And Oceans. Washington, DC: Island Press.
Benelli, G., Pozzebon, A., Raguseo, G., Bertoni, D., and Sarti, G. (2009). “An RFID based system for the underwater tracking of pebbles on artificial coarse beaches,” in Proceedings of the 3rd International Conference on Sensor Technologies and Applications (Sensorcomm 2009), Berlin.
Bindoff, N. L., Cheung, W. W. L., Kairo, J. G., Arístegui, J., Guinder, V. A., Hallberg, R., et al. (2019). Changing Ocean, Marine Ecosystems, and Dependent Communities. IPCC Special Report on the Ocean and Cryosphere in a Changing Climate. Geneva: IPCC.
Boström-Einarsson, L., Babcock, R. C., Bayraktarov, E., Ceccarelli, D., Cook, N., Ferse, S. C. A., et al. (2020). Coral restoration - A systematic review of current methods, successes, failures and future directions. PLoS One 15:e0226631. doi: 10.1371/journal.pone.0226631
Boström-Einarsson, L., Ceccarelli, D., Babcock, R. C., Bayraktarov, E., Cook, N., Harrison, P., et al. (2018). Coral Restoration In A Changing World - A Global Synthesis Of Methods And Techniques. Cairns: Reef and Rainforest Research Centre Ltd.
Calle-Trivino, J., Cortes-Useche, C., Sellares-Blasco, R. I., and Arias-Gonzalez, J. E. (2018). Assisted fertilization of threatened staghorn coral to complement the restoration of nurseries in Southeastern Dominican Republic. Reg. Stud. Mar. Sci. 18, 129–134. doi: 10.1016/j.rsma.2018.02.002
Chamberland, V. F., Petersen, D., Guest, J. R., Petersen, U., Brittsan, M., and Vermeij, M. J. A. (2017). New seeding approach reduces costs and time to outplant sexually propagated corals for reef restoration. Sci. Rep. 7:12.
Coral Restoration Consortium (2020). Best Practices. Available online at: http://crc.reefresilience.org/resources/best-practices/ (accessed May 2020).
CoralVita (2019). Developing A Scalable Business Model To Support Large-Scale Global Coral Reef Restoration. UN Secretary General Climate Action Summit. Available online at: http://hdl.handle.net/20.500.11822/28824 (accessed February, 2020).
Craggs, J., Guest, J., Bulling, M., and Sweet, M. (2019). Ex situ co culturing of the sea urchin, Mespilia globulus and the coral Acropora millepora enhances early post-settlement survivorship. Sci. Rep. 9:12984. doi: 10.1038/s41598-019-49447-9
Cziesielski, M. J., Schmidt-Roach, S., and Aranda, M. (2019). The past, present, and future of coral heat stress studies. Ecol. Evol. 9, 10055–10066. doi: 10.1002/ece3.5576
Damjanovic, K., Blackall, L. L., Webster, N. S., and Van Oppen, M. J. H. (2017). The contribution of microbial biotechnology to mitigating coral reef degradation. Microb. Biotechnol. 10, 1236–1243. doi: 10.1111/1751-7915.12769
De’ath, G., Fabricius, K. E., Sweatman, H., and Puotinen, M. (2012). The 27-year decline of coral cover on the great barrier reef and its causes. Proc. Natl. Acad. Sci. U.S.A. 109, 17995–17999. doi: 10.1073/pnas.1208909109
DeCarlo, T. M., and Harrison, H. B. (2019). An enigmatic decoupling between heat stress and coral bleaching on the Great Barrier Reef. PeerJ 7:e07473.
dela Cruz, D. W., and Harrison, P. L. (2017). Enhanced larval supply and recruitment can replenish reef corals on degraded reefs. Sci. Rep. 7:13985.
dela Cruz, D. W., Villanueva, R. D., and Baria, M. V. B. (2014). Community-based, low-tech method of restoring a lost thicket of Acropora corals. ICES J. Mar. Sci. 71, 1866–1875. doi: 10.1093/icesjms/fst228
Dixon, G. B., Davies, S. W., Aglyamova, G. A., Meyer, E., Bay, L. K., and Matz, M. V. (2015). Genomic determinants of coral heat tolerance across latitudes. Science 348, 1460–1462. doi: 10.1126/science.1261224
Doropoulos, C., Elzinga, J., ter Hofstede, R., van Koningsveld, M., and Babcock, R. C. (2019). Optimizing industrial-scale coral reef restoration: comparing harvesting wild coral spawn slicks and transplanting gravid adult colonies. Restor. Ecol. 27, 758–767. doi: 10.1111/rec.12918
Drury, C., Manzello, D., and Lirman, D. (2017). Genotype and local environment dynamically influence growth, disturbance response and survivorship in the threatened coral, Acropora cervicornis. PLoS One 12:e0174000. doi: 10.1371/journal.pone.0174000
Duarte, C. M., Agusti, S., Barbier, E., Britten, G. L., Castilla, J. C., Gattuso, J. P., et al. (2020). Rebuilding marine life. Nature 580, 39–51.
Forsman, Z. H., Page, C. A., Toonen, R. J., and Vaughan, D. (2015). Growing coral larger and faster: micro-colony-fusion as a strategy for accelerating coral cover. Peerj 3:1313. doi: 10.7717/peerj.1313
Gordon, T. A. C., Radford, A. N., Simpson, S. D., and Meekan, M. G. (2020). Marine restoration projects are undervalued. Science 367:635.
Guest, J. R., Baria, M. V., Gomez, E. D., Heyward, A. J., and Edwards, A. J. (2014). Closing the circle: is it feasible to rehabilitate reefs with sexually propagated corals? Coral Reefs 33, 45–55. doi: 10.1007/s00338-013-1114-1
Hein, M. Y., Birtles, A., Willis, B. L., Gardiner, N., Beeden, R., and Marshall, N. A. (2019). Coral restoration: socio-ecological perspectives of benefits and limitations. Biol. Conserv. 229, 14–25. doi: 10.1016/j.biocon.2018.11.014
Hein, M. Y., Couture, F., and Scott, C. M. (2018). “Ecotourism and coral reef restoration: case studies from Thailand and the Maldives,” in Coral Reefs: Tourism, Conservation And Management, eds B. Prideaux and A. Pabel (Oxford: Routledge), 137–150. doi: 10.4324/9781315537320-10
Heron, S. F., Maynard, J. A., Van Hooidonk, R., and Eakin, C. M. (2016). Warming trends and bleaching stress of the world’s coral reefs 1985-2012. Sci. Rep. 6:38402.
Hicks, L. L., Vogel, W. O., Herter, D. R., Early, R. J., and Stabins, H. C. (2009). “Plum creek’s central cascades habitat conservation plan and modeling for the northern spotted owl,” in Models for Planning Wildlife Conservation in Large Landscapes, eds J. J. Millspaugh and F. R. Thomsin (Cambridge, MA: Academic Press), 561–592. doi: 10.1016/b978-0-12-373631-4.00021-6
Hobbs, R. (2009). Looking for the silver lining: making the most of failure. Restor. Ecol. 17, 1–3. doi: 10.1111/j.1526-100x.2008.00505.x
Holling, C. S. (1978). Adaptive Environmental Assessment And Management. New York, NY: John Wiley and Sons.
Horoszowski-Fridman, Y. B., Izhaki, I., and Rinkevich, B. (2011). Engineering of coral reef larval supply through transplantation of nursery-farmed gravid colonies. J. Exp. Mar. Biol. Ecol. 399, 162–166. doi: 10.1016/j.jembe.2011.01.005
Hughes, T. P., Anderson, K. D., Connolly, S. R., Heron, S. F., Kerry, J. T., Lough, J. M., et al. (2018). Spatial and temporal patterns of mass bleaching of corals in the Anthropocene. Science 359:6371.
Hughes, T. P., Baird, A. H., Bellwood, D. R., Card, M., Connolly, S. R., Folke, C., et al. (2003). Climate change, human impacts, and the resilience of coral reefs. Science 301, 929–933. doi: 10.1126/science.1085046
Hughes, T. P., Kerry, J. T., Alvarez-Noriega, M., Alvarez-Romero, J. G., Anderson, K. D., Baird, A. H., et al. (2017). Global warming and recurrent mass bleaching of corals. Nature 543:373.
Johnson, M., Lustic, C., Bartels, E., Baums, I., Gilliam, D., Larson, L., et al. (2011). Caribbean Acropora Restoration Guide: Best Practices For Propagation And Population Enhancement. Arlington, VA: The Nature Conservancy.
Ladd, M. C., Shantz, A. A., Bartels, E., and Burkepile, D. E. (2017). Thermal stress reveals a genotype-specific tradeoff between growth and tissue loss in restored Acropora cervicornis. Mar. Ecol. Prog. Ser. 572, 129–139. doi: 10.3354/meps12169
Leal, M. C., Ferrier-Pages, C., Petersen, D., and Osinga, R. (2016). Coral aquaculture: applying scientific knowledge to ex situ production. Rev. Aquacult. 8, 136–153. doi: 10.1111/raq.12087
Leggat, W., Camp, E. F., Suggett, D. J., Heron, S. F., Fordyce, A. J., Gardener, S., et al. (2019). Rapid coral decay is associated with marine heatwave mortality events on reefs. Curr. Biol. 29, 2723–2730.
Levitan, D. R. (1993). The importance of sperm limitation to the evolution of egg size in marine invertebrates. Am. Natural. 141, 517–536. doi: 10.1086/285489
Levitan, D. R., and Petersen, C. (1995). Sperm limitation in the sea. Trends Ecol. Evol. 10, 228–231. doi: 10.1016/s0169-5347(00)89071-0
Levy, G., Shaish, L., Haim, A., and Rinkevich, B. (2010). Mid-water rope nursery—Testing design and performance of a novel reef restoration instrument. Ecol. Eng. 36, 560–569. doi: 10.1016/j.ecoleng.2009.12.003
Linden, B., and Rinkevich, B. (2011). Creating stocks of young colonies from brooding coral larvae, amenable to active reef restoration. J. Exp. Mar. Biol. Ecol. 398, 40–46. doi: 10.1016/j.jembe.2010.12.002
Linden, B., and Rinkevich, B. (2017). Elaborating an eco-engineering approach for stock enhanced sexually derived coral colonies. J. Exp. Mar. Biol. Ecol. 486, 314–321. doi: 10.1016/j.jembe.2016.10.014
Lirman, D., and Schopmeyer, S. (2016). Ecological solutions to reef degradation: optimizing coral reef restoration in the Caribbean and Western Atlantic. PeerJ 4:e2597. doi: 10.7717/peerj.2597
Maya, P. H. M., Smit, K. P., Burt, A. J., and Frias-Torres, S. (2016). Large-scale coral reef restoration could assist natural recovery in Seychelles, Indian Ocean. Nat. Conserv. 16:1. doi: 10.3897/natureconservation.16.8604
Miller, K. J., and Ayre, D. J. (2008). Population structure is not a simple function of reproductive mode and larval type: insights from tropical corals. J. Anim. Ecol. 77, 713–724. doi: 10.1111/j.1365-2656.2008.01387.x
Miller, K. J., and Mundy, C. N. (2003). Rapid settlement in broadcast spawning corals: implications for larval dispersal. Coral Reefs 22, 99–106. doi: 10.1007/s00338-003-0290-9
Moberg, F., and Folke, C. (1999). Ecological goods and services of coral reef ecosystems. Ecol. Econ. 29, 215–233. doi: 10.1016/s0921-8009(99)00009-9
Morikawa, M. K., and Palumbi, S. R. (2019). Using naturally occurring climate resilient corals to construct bleaching-resistant nurseries. P. Nat. Acad. Sci. U.S.A. 116, 10586–10591. doi: 10.1073/pnas.1721415116
Morrison, T. H., Adger, N., Barnett, J., Brown, K., Possingham, H., and Hughes, T. (2020). Advancing coral reef governance into the Anthropocene. One Earth 2, 64–74. doi: 10.1016/j.oneear.2019.12.014
National Academies of Sciences, Engineering, and Medicine (2019). A Research Review of Interventions to Increase the Persistence and Resilience of Coral Reefs. Washington, DC: National Academies Press.
Omori, M., and Iwao, K. (2014). Methods Of Farming Sexually Propagated Corals And Outplanting For Coral Reef Rehabilitation; With List Of References For Coral Reef Rehabilitation Through Active Restoration Measure. Okinawa: Akajima Marine Science Laboratory.
O’Neil, K. L. (2015). Land-Based Coral Nurseries: A Valuable Tool for Production and Transplantation of Acropora cervicornis. Master’s thesis, Nova Southeastern University, Florida.
Palumbi, S. R., Barshis, D. J., Traylor-Knowles, N., and Bay, R. A. (2014). Mechanisms of reef coral resistance to future climate change. Science 344, 895–898. doi: 10.1126/science.1251336
Petersen, D., Carl, M., Borneman, E., Brittsan, M., Hagedorn, M., Laterveer, M., et al. (2008). Noah’s Ark for the threatened Elkhorn coral Acropora palmata. Coral Reefs 27:715. doi: 10.1007/s00338-008-0385-4
Poquita-Du, R. C., Quek, Z. B. R., Jain, S. S., Schmidt-Roach, S., Tun, K., Heery, E. C., et al. (2019). Last species standing: loss of Pocilloporidae corals associated with coastal urbanization in a tropical city state. Mar. Biodiver. 49, 1727–1741. doi: 10.1007/s12526-019-00939-x
Randall, C. J., Negri, A. P., Quigley, K. M., Foster, T., Ricardo, G. F., Webster, N. S., et al. (2020). Sexual production of corals for reef restoration in the Anthropocene. Mar. Ecol. Progr. Ser. 635, 203–232. doi: 10.3354/meps13206
Rinkevich, B. (1995). Restoration strategies for coral reefs damaged by recreational activities: the use of sexual and asexual recruits. Restor. Ecol. 3, 241–251. doi: 10.1111/j.1526-100x.1995.tb00091.x
Rinkevich, B. (2014). Rebuilding coral reefs: does active reef restoration lead to sustainable reefs? Curr. Opin. Env. Sust. 7, 28–36. doi: 10.1016/j.cosust.2013.11.018
Schmidt-Roach, S., Lundgren, P., Miller, K., Gerlach, G., Noreen, A. M. E., and Andreakis, N. (2012). Assessing hidden species diversity in the coral Pocillopora damicornis from Eastern Australia. Coral Reefs 32, 161–172. doi: 10.1007/s00338-012-0959-z
Schopmeyer, S. A., Lirman, D., Bartels, E., Byrne, J., Gilliam, D. S., Hunt, J., et al. (2012). In situ coral nurseries serve as genetic repositories for coral reef restoration after an extreme cold-water event. Restor. Ecol. 20, 696–703. doi: 10.1111/j.1526-100x.2011.00836.x
Shearer, T. L., Porto, I., and Zubillaga, A. L. (2009). Restoration of coral populations in light of genetic diversity estimates. Coral Reefs 28, 727–733. doi: 10.1007/s00338-009-0520-x
Spalding, M., Burke, L., Wood, S. A., Ashpole, J., Hutchison, J., and Ermgassene, P. Z. (2017). Mapping the global value and distribution of coral reef tourism. Mar. Policy 82, 104–113. doi: 10.1016/j.marpol.2017.05.014
Spalding, M. D., Brumbaugh, R. D., and Landis, E. (2016). Atlas Of Ocean Wealth. Arlington, VA: Nature Conservancy.
Spalding, M. J. (2016). The new blue economy: the future of sustainability. J. Ocean Coastal Econ. 2:8.
UNEP (2019). New UN Decade On Ecosystem Restoration Offers Unparalleled Opportunity For Job Creation, Food Security And Addressing Climate Change. Available online at: https://www.unenvironment.org/news-and-stories/press-release/new-un-decade-ecosystem-restoration-offers-unparalleled-opportunity (accessed May 2020).
van Oppen, M. J. H., Gates, R. D., Blackall, L. L., Cantin, N., Chakravarti, L. J., Chan, W. Y., et al. (2017). Shifting paradigms in restoration of the world’s coral reefs. Glob. Chang. Biol. 23, 3437–3448.
van Oppen, M. J. H., Oliver, J. K., Putnam, H. M., and Gates, R. D. (2015). Building coral reef resilience through assisted evolution. P. Nat. Acad. Sci. U.S.A. 112, 2307–2313. doi: 10.1073/pnas.1422301112
Waltham, N. J., Elliott, M., Lee, S. Y. Y., Lovelock, C., Duarte, C. M., Buelow, C., et al. (2020). UN decade on ecosystem restoration 2021-2030-what chance for success in restoring coastal ecosystems? Front. Mar. Sci. 7:71. doi: 10.3389/fmars.2020.00071
Wilkinson, C. C. (2004). Status Of Coral Reefs Of The World: 2004. Townsville: Australian Institute of Marine Science (AIMS).
Woodhead, A. J., Hicks, C. C., Norström, A. V., Williams, G. J., and Graham, N. A. J. (2019). Coral reef ecosystem services in the Anthropocene. Funct. Ecol. 33, 1023–1034.
Keywords: coral restoration, blue economy, adaptive coral gardening, coral seeding hubs, ecotourism
Citation: Schmidt-Roach S, Duarte CM, Hauser CAE and Aranda M (2020) Beyond Reef Restoration: Next-Generation Techniques for Coral Gardening, Landscaping, and Outreach. Front. Mar. Sci. 7:672. doi: 10.3389/fmars.2020.00672
Received: 29 February 2020; Accepted: 23 July 2020;
Published: 13 August 2020.
Edited by:
Megan Irene Saunders, Oceans and Atmosphere (CSIRO), AustraliaReviewed by:
Ana B. Bugnot, The University of Sydney, AustraliaElisa Bayraktarov, The University of Queensland, Australia
Copyright © 2020 Schmidt-Roach, Duarte, Hauser and Aranda. This is an open-access article distributed under the terms of the Creative Commons Attribution License (CC BY). The use, distribution or reproduction in other forums is permitted, provided the original author(s) and the copyright owner(s) are credited and that the original publication in this journal is cited, in accordance with accepted academic practice. No use, distribution or reproduction is permitted which does not comply with these terms.
*Correspondence: Sebastian Schmidt-Roach, sebastian.schmidtroach@kaust.edu.sa