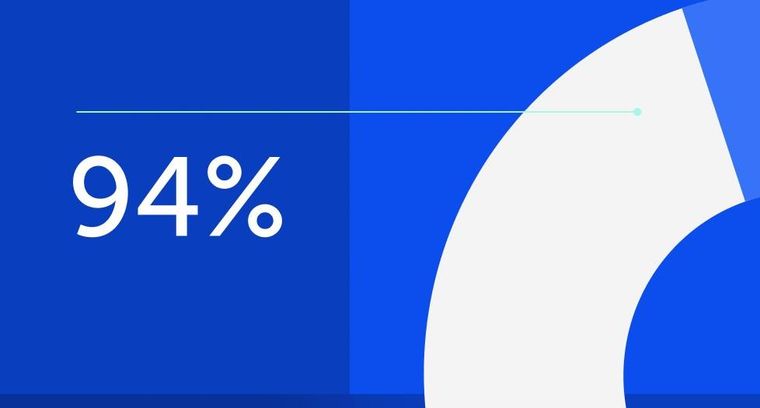
94% of researchers rate our articles as excellent or good
Learn more about the work of our research integrity team to safeguard the quality of each article we publish.
Find out more
ORIGINAL RESEARCH article
Front. Mar. Sci., 19 November 2020
Sec. Marine Megafauna
Volume 7 - 2020 | https://doi.org/10.3389/fmars.2020.00582
This article is part of the Research TopicPathologic Findings in Stranded Marine Mammals: A Global PerspectiveView all 29 articles
We compiled findings from 15 years (1998–2012) of southern sea otter (Enhydra lutris nereis) necropsies, incorporating data from 560 animals. Sensitive diagnostic tests were used to detect biotoxins, bacteria, parasites and fungi. Methods to classify primary and contributing causes of death (COD) and sequelae utilized an updated understanding of health risks affecting this population. Several interesting patterns emerged, including identification of coastal regions of high mortality risk for sea otter mortality due to shark bite, cardiomyopathy, toxoplasmosis, sarcocystosis, acanthocephalan peritonitis and coccidioidomycosis. We identified demographic attributes that enhanced the risk of disease in relation to age, sex, and reproductive stage. Death due to white shark (Carcharodon carcharias) bite increased dramatically during the study period and was the most common primary COD. However, when primary and contributing COD were combined, the most prevalent COD was infectious disease (affecting 63% of otters), especially fatal infections by acanthocephalans (Profilicollis spp.) and protozoa (e.g., Sarcocystis neurona and Toxoplasma gondii). Fatal bacterial infections were also extremely common as a primary process or a sequela, affecting 68% of examined otters. Substantial advances were made in identifying sea otters that died following exposure to the pervasive marine neurotoxin domoic acid (DA), and DA intoxication was conservatively estimated as a primary or contributing COD for 20% of otters. Cardiomyopathy was also highly prevalent as a primary or contributing COD (41%) and exhibited significant associations with DA intoxication and protozoal infection. For adult and aged adult females in late pup care through post-weaning at the time of death, 83% had end lactation syndrome (ELS) as a primary or contributing COD. This comprehensive longitudinal dataset is unique in its depth and scope. The large sample size and extensive time period provided an opportunity to investigate mortality patterns in a changing environment and identify spatial and temporal disease “hot spots” and emerging threats. Our findings will help improve estimates of population-level impacts of specific threats and optimize conservation and environmental mitigation efforts for this threatened species.
As a federally listed threatened species, southern sea otters (Enhydra lutris nereis) have been necropsied by veterinary pathologists at the California Department of Fish and Wildlife (CDFW) since 1998, providing a robust longitudinal dataset that can be used to identify threats to population recovery. As a keystone (Reisewitz et al., 2006) and sentinel species (Jessup et al., 2007), sea otters are bioindicators of nearshore ecosystem health (Jessup et al., 2004). Despite decades of protection, this population has attained only a fraction of its pre-harvest abundance, and range expansion has stalled (USFWS, 2015). Clarifying factors limiting population growth can help inform management actions to help recover this population.
Nearly two decades have passed since the last comprehensive analysis of southern sea otter mortality patterns (Kreuder et al., 2003), and much has been learned about the effects of marine predators (Tinker et al., 2016), viruses (Tuomi et al., 2014; Ng et al., 2015; Siqueira et al., 2017), bacteria (Johnson et al., 2003; Stavely et al., 2003; Miller et al., 2010a, 2018; Bartlett et al., 2016), parasites (Miller et al., 2004, 2008, 2010b, 2018; Shapiro et al., 2012, 2016, 2019; Shockling-Dent et al., 2019), fungi (Huckabone et al., 2015), and biotoxins (Miller et al., 2010c; Fire and Van Dolah, 2012) on sea otters. Novel health conditions have been identified, and some idiopathic conditions have become better-characterized (Kreuder et al., 2005; Tuomi et al., 2014; Ng et al., 2015; Chinn et al., 2016; Shockling-Dent et al., 2019; Miller et al., in review). Due to new discoveries and improved diagnostic tests, and because mortality patterns are not static in wild populations, periodic reassessment is vital. Detailed review of core mortality patterns should be performed every 10–15 years, with more frequent re-assessment performed upon identification of mortality events, novel health threats, or new tests that are informative for shaping conservation policy. Interplay between hosts, pathogens, biotoxins, and the environment is ever-changing, and some hazards can interact, accentuating impacts on individuals and populations. Effective management requires monitoring fundamental causes of mortality and their biological and environmental associations, and rapid identification of potential emerging threats.
For some diseases, estimation of population impacts has been hindered by limited understanding and lack of criteria to facilitate case recognition. Many of these more ill-defined processes have associations with anthropogenic activity, including introduction of invasive species and their endogenous pathogens, coastal urbanization, manipulation of coastal watersheds and climate change. Examples include parasites shed by non-native felids and marsupials (Kreuder et al., 2003; Miller et al., 2010b, 2018), and aquatic biotoxins where bloom frequency and severity can be accentuated by nutrification and climate change (Moore et al., 2008; Hinder et al., 2012; Miller et al., 2010c; McKibben et al., 2017). Biotoxins known to threaten humans and other animals in California include microcystin, saxitoxin and domoic acid (DA). Domoic acid is an important cause of marine wildlife mortality (Fire and Van Dolah, 2012), and a threat to human food safety (McKibben et al., 2017). If population-level impacts could be more precisely measured, these threats could be remediated through cooperative management between state and federal agencies entrusted with sea otter conservation and protection of human health.
A major challenge was the complexity of disease processes affecting southern sea otters. Due to their physiology and diverse diet (Yeates et al., 2007; Tinker et al., 2008; Thometz et al., 2014), sea otters are exposed to a wide range of stressors and infectious agents. As a result, it is common to identify multiple significant, independent disease processes during necropsy. Accurately capturing, describing and analyzing this complexity was an important challenge. This was accomplished by revising methods used to categorize disease to include a primary cause of death (COD), up to three contributing COD and sequelae, with assessments based on our most current understanding of southern sea otter biology, ecology, and disease. Where possible mortality patterns were interpreted in the context of smaller-scale regional population dynamics.
We analyzed COD for 560 southern sea otters with comprehensive postmortem examinations spanning 15 years (1998–2012). Geographical and/or temporal stranding patterns were identified for several conditions; investigation of these patterns may help scientists identify disease drivers. We aimed to accurately capture this complexity and utilize the longitudinal nature of this dataset to inform management and facilitate efforts to achieve southern sea otter population recovery.
A subset of stranded southern sea otters recovered in California during 1998–2012 were included in this study. Cause of death and other findings were compiled for all otters >1 years old (e.g., subadults, adults, and aged adults) that died or were found dead, were minimally decomposed, were not frozen, and received a detailed necropsy. Otters < 1 year old were excluded due to limited sample size. The stranding date and location were considered, along with sex, age class; subadult (1–3 years), adult (4–10 years), and aged adult (>10 year), and nutritional condition (emaciated, poor, fair, good, excellent) (Kreuder et al., 2003; Tinker et al., 2016). Reproductive stage was assessed for all females: 0 = immature, 1 = first half of post-implantation pregnancy, 2 = second half of pregnancy, 3 = first half of pup care, 4 = second half of pup care and 5 = post-weaning/estrus/delayed implantation (Chinn et al., 2016).
Our collaborative stranding program achieved approximately the same level of carcass search effort across the study time frame. Necropsy case selection utilized a randomized sampling protocol, with any changes implemented at the beginning of applicable calendar years to minimize within-year sampling effects. Case selection was based on stranding order (for example, every third fresh otter), with allowances for inclusion of tagged research animals and otters from areas that were comparatively under-represented, such as the Big Sur Coast. After careful consideration, we felt that the benefits of including these two very important subgroups (radio-tagged sea otters and geographically under-represented stranding areas) outweighed the risks of adding some sample bias. The study population was restricted to subadults through aged adults to include the most broadly sampled age classes.
Tissue samples were formalin-fixed and processed for microscopic examination as previously described (Kreuder et al., 2003). Histopathology was incomplete for some cases due to zoonotic risk or postmortem scavenging; these partial cases were included only when primary and contributing COD were readily apparent. Tissues and body fluids were also cryoarchived for diagnostic tests.
For otters with available serum, immunofluorescent antibody tests were performed to assess IgG seroreactivity to Toxoplasma gondii and Sarcocystis neurona (Miller et al., 2002), and titers ≥ 1:320 serum dilution were used to define infection. Immunohistochemistry was performed where indicated to confirm the identity of protozoa observed in tissue sections (Miller et al., 2018).
Aerobic and anaerobic bacterial characterization were performed as needed to determine the COD, assess infection severity and trace pathogen invasion from wounds or other sources within the body. Fungal culture was also performed as needed to identify pathogens, ensure safe carcass disposal and protect human health. Bacterial and fungal isolation and identification were performed as previously described (Miller et al., 2010a) and (Huckabone et al., 2015), respectively.
For all otters with available urine and/or gastrointestinal (GI) content, liquid chromatography-tandem mass spectrometry (LCMS/MS) was used to detect DA using validated methods (Torr et al., 2003). Matrix spiking/recovery and blinded controls were included with each run, and most testing was completed within 2 years of necropsy to minimize error due to sample degradation. Urine was prioritized when available because it reflects DA ingestion, absorption and excretion (Fire and Van Dolah, 2012); GI content was tested when urine was unavailable.
Limited LCMS/MS testing for microcystin and saxitoxin was performed in a subset of samples in order to explore potential toxin exposure and to facilitate future investigations. Accurate detection of both biotoxins is challenging post-ingestion, as there are no definitive criteria to aid diagnoses, and little is known about possible health effects (Miller et al., 2010c). In this study, potential cases of fatal microcystin intoxication were noted.
Cause(s) of death were determined based on clinical history, gross necropsy findings, histopathology and diagnostics. The primary COD was the most severe process that likely caused stranding. Secondary, tertiary and quaternary (cumulatively “contributing”) COD represented independent processes that were also present at necropsy, were deemed moderate or severe and likely contributed to stranding. Combined primary and contributing (“top four”) COD were used for cause-specific statistical models. When the primary COD was acute trauma, any pre-existing conditions that may have contributed to the risk of traumatic death were included as contributing causes if they were moderate to severe and were considered significant enough to have led to death in the absence of trauma (e.g., DA intoxication, acanthocephalan peritonitis [AP] or protozoal COD).
Necropsy findings were categorized as sequelae if they occurred as a direct consequence of a primary or contributing COD and contributed to the risk of death (e.g., white shark [Carcharodon carcharias] bite as a primary COD with bacterial infection as a sequela). Because sequelae are not independent processes, they were used for descriptive analyses, but excluded from cause-specific mortality models.
Shark bite wounds were classified as confirmed if tooth fragments or parallel serrated scratches on bone were found. Presumptive cases had no visible tooth fragments or bone scratches, but the external wounds were characteristic of shark bite (Ames and Morejohn, 1980). All shark wounds were presumed to be from white sharks (Tinker et al., 2016). Care was taken to distinguish shark bite from propeller wounds or other trauma using previously established criteria (Ames and Morejohn, 1980). Wound chronicity was determined based on presence/absence of healing and secondary bacterial infection. Lacerations with minimal wound healing or infection were considered acute; partially healed and/or infected wounds were considered subacute or chronic.
In addition to interspecific trauma, sea otters are also affected by intraspecific trauma. Fatal fight trauma can occur when male sea otters defend territory or compete for females. Fight trauma was characterized by lacerations on the face, feet, testicles and penis. Mating trauma occurs to females during copulation when males grasp the female’s snout with their teeth; nasal and facial lacerations are common. Copulation occurs in water, and females occasionally drown (Staedler and Riedman, 1993). Lesions indicative of mating trauma include nose wounds and facial, vaginal or rectal lacerations. Fatal vaginal or rectal perforations have been reported (Harris et al., 2010). The above lesion patterns, plus sex and age criteria were considered when assessing fight or mating trauma as a primary or contributing COD.
Primary bacterial infection (by any combination of bacteria) was defined as a COD if the infection was moderate to severe and no predisposing condition was found. Bacterial infections that were considered secondary to pre-existing conditions were classified as sequelae.
Although southern sea otters can be infected by several acanthocephalan species, intestinal perforation has been attributed only to Profilicollis altmani or P. kenti (Mayer et al., 2003). These parasites infect sea otters via crustacean intermediate hosts. Once infected, trans-intestinal parasite migration often causes fatal peritonitis (Mayer et al., 2003). Acanthocephalan peritonitis was considered a COD when moderate to high numbers of Profilicollis spp. were observed in the peritoneal cavity in conjunction with peritonitis.
Protozoal infection and death due to T. gondii and S. neurona were diagnosed from necropsy, histopathology, and serology. Protozoa were identified using morphological criteria, and occasionally, immunohistochemistry (Miller et al., 2018). Histopathologic assessments included relative parasite burden (none, low, medium or high), protozoal stages (tissue cysts, schizonts and/or zoites) and the character and severity of parasite-associated inflammation and tissue damage. Lymphoplasmacytic inflammation typically dominates in T. gondii infections, while a broader mix of inflammatory cells is indicative of S. neurona infection (Miller et al., 2018).
Any putative Sarcocystis spp. infection was classified as S. neurona based on results of prior sea otter studies (Thomas et al., 2007; Miller et al., 2010b). Sarcocystis neurona was considered a COD when S. neurona-associated inflammation and pathology in the brain, spinal cord, meninges and any other tissues were moderate or severe. In contrast, chronic sublethal T. gondii infection is common in southern sea otters and is often accompanied by CNS meningitis and perivasculitis without significant brain or spinal cord pathology (Miller et al., 2018). Toxoplasma gondii was considered a COD when parasite-associated pathology in the brain, spinal cord or other tissues was moderate or severe, in addition to any observed meningeal or perivascular inflammatory infiltrate. In some cases, S. neurona and T. gondii were identified as concurrent COD based on findings from serology and histopathology.
Although detailed characterization of all viral causes of sea otter death was not feasible during this study, genomic screening of archival tissues to identify viruses circulating in this population was completed as a first step (Ng et al., 2015; Siqueira et al., 2017). Sera were also screened for immunoreactivity to influenza A viruses (Capuano et al., 2017), and limited PCR was performed to screen tissues for morbilliviruses. A few otters were assessed for rabies via brain histopathology and immunofluorescence, but no cases were identified.
Viral infection was diagnosed as a COD when suspect inclusions were observed in association with moderate to severe pathology that was compatible with viral disease (e.g., epithelial necrosis, lympholysis or interstitial pneumonia). This preliminary assessment likely under-recognizes the impact of viruses on southern sea otters, but future studies can expand on our initial findings.
All adult and aged adult females were evaluated for end lactation syndrome (ELS) (Chinn et al., 2016) as a COD. A physical expression of the high energetic cost of reproduction (Thometz et al., 2014), ELS was defined as females stranding during late pup care or post-weaning with moderate or severe emaciation that was not attributable to an independent disease process (Chinn et al., 2016). Lesions indicative of mating trauma included nose wounds and facial, vaginal or rectal lacerations.
Although DA toxicosis has been well-described in California sea lions (Scholin et al., 2000; Silvagni et al., 2005; Goldstein et al., 2008; Pulido, 2008), no diagnostic criteria have been proposed to diagnose fatal DA intoxication in sea otters. A fundamental aim of this study was to estimate the health impacts of DA intoxication on southern sea otters. Interpretation of DA concentration in biological samples is challenging due to rapid toxin clearance from tissues and body fluids, DA persistence in sediment and biota, and lack of validated criteria to define toxicity (Fire and Van Dolah, 2012). To address these knowledge gaps, we systematized our approach, testing available urine or GI content, reviewing case records for ante-mortem clinical signs, and examining tissues microscopically for DA-associated pathology based on reports in other species (Scholin et al., 2000; Silvagni et al., 2005; Goldstein et al., 2008; Pulido, 2008).
All otters were classified with respect to the likelihood of DA intoxication as a primary or contributing COD as probable, possible, or negative; cases of probable DA intoxication were used in epidemiological analyses. The probable DA intoxication category was considered a conservative estimate of the effects of DA on southern sea otters; actual impacts are likely to be higher. Possible DA cases had a lower degree of certainty regarding diagnosis; some animals in this category likely died from DA intoxication, while others may not have. Challenges in making a diagnosis included missing clinical data, test results, and limited/sub-optimal histopathology. Guidelines to facilitate diagnosis of DA intoxication and interpretation of DA concentrations in biological samples are being compiled as complimentary publications (Miller et al., in review).
A diagnosis of cardiomyopathy (Kreuder et al., 2005) was characterized grossly by a variably dilated heart, engorged coronary veins and patchy myocardial pallor. Microscopic lesions included cardiac myofiber degeneration, stromal collapse, fibrosis and inflammation. To investigate associations with other conditions, lesions supportive of cardiomyopathy were assessed independently of other fatal conditions.
Otters with neoplasia or GI erosions/ulcers as COD were also pooled into groups to facilitate analysis; compiled cases will be further examined as separate studies.
Proportionate mortality (proportion of deaths in this study population attributable to each defined condition) was used to assess the prevalence of the primary COD. This was expressed as the number of otters with a given primary COD divided by all otters in the study. The sum of the primary COD proportionate mortalities was 100%, as each primary COD was mutually exclusive. In addition, the proportion of a defined condition present in each otters’ top four (pooled primary and contributing) COD was calculated to assess the overall prevalence in the study population. This was expressed as the number of otters with a given condition as one of the top four COD, divided by the number of animals that could be examined with sufficient detail to confirm whether that condition was present at necropsy. The denominator varied for cases with missing components required to confirm specific conditions (e.g., limited histopathology due to scavenging or zoonoses), and for COD that can only occur in some animals (e.g., ELS can only occur in reproductively mature females). For the pooled top four COD, the total proportions do not sum to 100% because conditions are not mutually exclusive, as individuals contributed to more than one category of mortality; however, the calculations are important for showing the burden of significant conditions affecting this species.
Some common causes of southern sea otter death were assessed relative to regional population estimates collected each year (Tinker and Hatfield, 2017). Due to variable viewing conditions, these counts are considered a population index, with 3-year running means used to assess trends.
Sea otter stranding dates were grouped by stranding period to assess risk associated with seasonal weather patterns: spring (February – April), summer (May – July), fall (August – October), and winter (November – January). During most years, precipitation is highest during the winter and spring months along the Central California coast. Otters in the study were also pooled by stranding location to assess risk in relation to stranding area: north (San Francisco to Pacific Grove), central (Pacific Grove to Cayucos), and south (Cayucos to Santa Barbara). Most stranded animals included in this study were recovered within the established geographic range of the southern sea otter, which spanned approximately from Half Moon Bay to Santa Barbara during this study. The stranding site for each otter was recorded to the nearest pre-defined 0.5 km location along the central California coast (Kreuder et al., 2003). Regional and temporal population indices (3-year running means) provided graphical comparisons with mortality data for some figures (USFWS, 2015; Tinker and Hatfield, 2017).
Spatial and temporal clustering of cause-specific mortality were evaluated using SaTScanTM software v9.4.4 (Kulldorff M. and Information Management Services, Inc., 2009)1. The spatial scan statistic (Kulldorff, 1997) was used to test whether a primary or contributing COD was randomly distributed or clustered in time or space. This method scanned for clusters with high or low rates of occurrence (relative risk = RR) using the Bernoulli model. A Monte Carlo iterative technique was used to determine distribution of the likelihood ratio test statistic (Kulldorff and Nagarwalla, 1995). Spatial-temporal clusters were assessed for select pooled top four COD by evaluating the distribution of cases as a binary response variable (positive or negative) for all stranding locations and dates.
Cluster analysis included assessment of COD with respect to stranding location (spatial analysis), stranding date (temporal analysis), and location and date (space-time or spatiotemporal analysis). The spatial window was defined by a maximum cluster size of 50% of the population at risk, and/or a 75-km radius. The temporal window was defined by a minimum (1 month) and maximum (50% of the study period; 7.5 years) cluster size. Spatial and temporal cluster analyses were conducted for the most prevalent CODs: shark bite, AP, protozoal infection (S. neurona and/or T. gondii), ELS, probable DA intoxication, and cardiomyopathy. Patterns for coccidioidomycosis (Coccidioides sp. infection) were also evaluated due to the importance of this fungus as a human pathogen.
A coastal map was produced for each COD that yielded statistically significant spatial and spatiotemporal clusters. Cluster centroids were plotted over heatmaps, representing smoothed spatial distributions of the relative frequency of each condition. Heatmaps were produced using a kernel smoothing algorithm (a local polynomial density function with normal kernel and 50 km bandwidth) (Wand and Jones, 1995) fit to the dataset, interpolating the proportion of positive or negative cases at each coastal location. Kernel smoothing was conducted using R programming language (R Core Team, 2016) with the “locpoly” function of the “KernSmooth” library2. Heatmaps and cluster centroids were produced using QGIS software (QGIS Development Team, 2018).
To clarify temporal patterns, we produced heat maps showing the mean proportional incidence of each COD by time period. Two temporal scales were evaluated, with data grouped by year (illustrating inter-annual variation), and by month (illustrating seasonal variation). To clarify temporal patterns, we fit a smoothing spline to the proportional incidence data by time period, with the smoothing parameter derived using the generalized cross-validation method (Green and Silverman, 1993). Spline smoothing and temporal heatmap plots were achieved using R programming software (R Core Team, 2016) and “ggplot” libraries (Wickham, 2016).
Potential risk factors were evaluated for associations with the most prevalent primary and contributing COD: shark bite, AP, systemic protozoal infections (S. neurona and T. gondii), ELS, probable DA intoxication, and cardiomyopathy. Independent logistic regression models were used to examine the associations between cause-specific mortality and putative demographic and environmental risk factors, incorporating data from nearshore environment models for the California Coast (Burgess et al., 2018; Tinker et al., unpublished data) (Supplementary Table 1). Additional information on environmental variables and specialized assessments for some COD are summarized in the Supplementary Materials.
The outcome for all models was presence or absence of a specific COD. Logistic regression models adjusted for confounding variables to estimate odds ratios (OR), 95% confidence intervals (CIs), and P-values using Stata software, version 14.2 (STATACorp, College Station, TX, United States). The following explanatory variables were assessed: (1) age class, (2) sex, (3) reproductive stage, (4) nutritional condition, (5) stranding season, (6) stranding year, (7) stranding area, and (8) SaTScan high-risk clusters (spatial, temporal, spatiotemporal). For certain COD (where biologically appropriate), we also evaluated the co-occurrence of other disease conditions and/or contributing COD as potential predictor variables, including: (9) protozoal infection (S. neurona and/or T. gondii), (10) bacterial infection (combined primary infections and sequelae), (11) viral infection, (12) GI erosions/ulcers, (13) neoplasia, (14) probable DA intoxication, and (15) saxitoxin-positive test results.
Previous research suggests that environmental variables and local population density were potential risk factors for ELS (Laidre et al., 2006; Chinn et al., 2016; Tinker et al., 2017, 2019), so we also evaluated the effects of local population status and habitat type (benthic substrate and kelp canopy cover) in the ELS mortality model (Laidre et al., 2006). Due to substantial co-linearity between environmental and demographic explanatory variables, we used principal components analysis (PCA) (Smith, 2002) to create an index variable representing status with respect to carrying capacity (K), denoted as the “K-index.” The K-index was scaled from zero to one, with values close to one corresponding to areas with high sea otter population density, prolonged occupation by sea otters, high pup to adult ratio, poor individual body condition, and stable or slightly negative abundance trends. A binomial version of the variable, K-status, was also created by rounding K-index to zero or one. Details of model development and the PCA and K-index derivation are provided in the Supplementary Figures 1–3 and Table 1.
Table 1. Sex and age class distribution for 560 stranded southern sea otters (Enhydra lutris nereis) necropsied during 1998–2012.
Univariate analyses were used to generate descriptive statistics, examine frequencies and distributions, and determine the most appropriate form of variables. Next, bivariate analyses were conducted to examine associations between variables and each COD using Fisher’s exact or Pearson’s chi square tests. Variables with P ≤ 0.3 were considered for inclusion in multivariate logistic regression models using a forward stepwise approach. Variables were removed through backward selection if they lost significance within the multivariate model (likelihood ratio test, P ≤ 0.2), or inclusion did not significantly improve model fit. Models were compared using deviance and Akaike Information Criteria. The Hosmer–Lemeshow goodness-of-fit test was used to assess overall model fit. Where appropriate, the strength of associations was estimated by the OR, 95% CI and P-value, with P-values ≤ 0.05 considered significant. For multivariate logistic regression analyses, factors that were not significant individually, but optimized overall model fit were retained.
From 1998–2012, 3,774 southern sea otter deaths were reported throughout California (annual mean 252 ±62 SD), and 560 cases met criteria for study inclusion (annual mean 37 ±14 SD), including 535 full necropsies, and 25 partial necropsies due to zoonoses or mild scavenging. Some otters could not be accurately assessed for every condition, but all potential COD were evaluated for nearly all cases. For example, 547 otters were assessed for protozoal disease, 549 for DA intoxication and 552 for cardiomyopathy.
Of the 560 otters in this study, 364 were found dead and 196 stranded alive (108 died subsequent to stranding and 88 were euthanized). Sex and age distribution can be seen in Table 1. The nutritional condition of otters in this study were: 224 emaciated, 63 thin, 61 fair, 92 good, and 116 excellent (four unknown). Eighty-six otters were previously tagged for rehabilitation or research.
Sea otters in this study represented a subset of all carcasses reported annually, with the proportion ranging from 7% (n = 15) in 1998 to 23% (n = 59) in 2003 (Figure 1). Although the stranding locations spanned 1,035 km, when three outliers were excluded (one otter each from Marin, Orange, and San Diego Counties), all others were found across 535 km of the central California coast from San Francisco to Santa Barbara. Similar to prior reports (Kreuder et al., 2003; Tinker et al., 2016), fewer carcasses were recovered from the range center between Carmel and Cayucos (e.g., Big Sur Coast; Figure 2). This remote region has a high sea otter density (Tinker and Hatfield, 2017), but low carcass recovery due to sparse beaches and a small human population, so epidemiological analyses were used to account for differential sampling.
Figure 1. Comparison of annual southern sea otter (Enhydra lutris nereis) carcass stranding patterns, the annual population index (data missing for 2011), and the number of subadult (SA), adult (A) and aged adult (AA) carcasses collected vs. examined for this study, along with all animals of unknown age class (UNKN), pups (PUP), and immature (IMM) sea otters that were excluded because limited numbers received detailed necropsies during this period (1998–2012).
Figure 2. Coastal California, United States stranding patterns from San Francisco Bay south to Santa Barbara for southern sea otter (Enhydra lutris nereis) carcasses examined 1998–2012 for this study (black bars). The superimposed black line is an index of the sea otter population during the same years across this same geographical area; note that although the sea otter population was high in the center of the range (Big Sur Coast), carcass recovery was low because this region is relatively sparsely populated and has few beaches to facilitate post-stranding carcass recovery. At center right is a small inset map of central California; the black-outlined portion of the inset shows the location of the study area (enlarged and shown in light gray at right center) along the California coast.
To facilitate interpretation, primary and contributing COD were grouped as trauma, pathogens, age and sex-associated conditions, biotoxins, multicausal conditions, direct anthropogenic causes and other conditions. Annual mortality patterns are summarized in Supplementary Figure 4.
The trauma category includes shark bite and trauma of unknown origin. Intraspecific and anthropogenic trauma are included in other categories. Since most cases in the trauma category were shark bite, only that COD is discussed in detail.
Shark bite was the most common primary COD (28%; 161/560) and was a contributing cause for 1% of cases (Table 2). Based on wound healing and bacterial invasion, more than half (53%; 84/161) of fatal shark bite cases survived the initial trauma but succumbed to secondary infections days to weeks later.
Table 2. Combined primary and contributing causes of death (COD) for 560 necropsied southern sea otters (Enhydra lutris nereis) examined 1998–2012.
Table 3. Spatial and temporal clustering patterns for stranded southern sea otters (Enhydra lutris nereis) in relation to key causes of death (COD), for 560 animals necropsied 1998–2012.
Spatial SaTScan analysis showed that otters are at highest risk for fatal shark bite at the northern and southern ends of the southern sea otter range (Table 3 and Figure 3). Two overlapping high-risk clusters were detected to the north at Pescadero (RR = 2.95; P < 0.0001) and Pacifica (RR = 2.52; P = 0.01), and two overlapping high-risk clusters were detected to the south at Pismo Beach (RR = 2.00; P = 0.001) and Vandenberg Air Force Base (RR = 1.91; P = 0.006). Shark bite mortality had pronounced seasonal (Figure 4A) and yearly trends (Figure 4B). Shark-related death increased in the fall (August – October) and winter (November – January). Temporal SaTScan analysis demonstrated that stranded sea otters from 2008-2012 had 1.80 times higher risk of shark bite mortality than otters that stranded earlier (P = 0.01). Spatiotemporal SaTScan analysis revealed that Pescadero was a high-risk region from 2009 – 2012 (RR = 2.83; P = 0.02).
Figure 3. Map of the central California coast, showing significant high (red) and low (blue) risk spatial (diamonds) and space-time (stars) clusters for select health conditions affecting 560 southern sea otters (Enhydra lutris nereis) necropsied 1998-2012. The color gradient indicates relative mortality risk (with highest risk red and lowest risk blue). The relative width of the gradient in each region corresponds with the extent of usable sea otter habitat, delineated by the area of coastal habitat located within ≤60 m seawater depth. Numbers adjacent to diamonds or stars correspond with the index numbers in Table 3, where additional details can be found. A high-risk cluster is shown for Coccidioidomycosis, although this cluster is not quite significant (P = 0.055).
Figure 4. Temporal trends for common causes of southern sea otter (Enhydra lutris nereis) death for 560 animals necropsied 1998 – 2012. Temporal trends are depicted as heat maps of the mean proportional incidence of each cause of death (pooled top 4 COD). Cases were grouped by month (A): illustrating seasonal variation, and by year (B): illustrating inter-annual variation. Warmer colors (dark orange and red) illustrate higher proportional incidence, while cooler colors (light orange and yellow) illustrate lower incidence. Domoic acid intox. = Domoic acid intoxication.
The multivariate model evaluating factors associated with shark bite mortality included sex, age class, nutritional condition, season, year, and the high-risk spatial clusters (n = 560; Table 4). Subadults were 4.43 times more likely to die of shark trauma than aged adults (P ≤ 0.001). Otters in good to excellent nutritional condition were 2.64–3.71 times more likely to have died of shark bite than otters in a worse nutritional state (P ≤ 0.001). Males had 1.5-fold higher risk of shark bite mortality compared to females, but this finding was not statistically significant (P = 0.1). Individuals that stranded in fall and winter had substantially higher odds of fatal shark bite (OR = 5.20 and 2.48, respectively) than those in spring and summer (P ≤ 0.004). Otters that stranded between 2008 and 2012 were 2.32 times more likely to die of shark bite than those between 1998 and 2007 (P < 0.001). Otters that stranded in the northern and southern high-risk spatial clusters had 10.23 and 3.06 times higher odds of fatal shark bite than otters that stranded elsewhere (P < 0.001).
Table 4. Shared measures of effect (odds ratios) for seven common causes of southern sea otter (Enhydra lutris nereis) death 1998 – 2012.
When all infectious causes of death cases were combined, infectious disease was the most prevalent mortality category as a primary or contributing cause (63%; 354/560), not including additional cases where pathogens invaded tissues following traumatic or other events (e.g., sequelae) (Table 2).
Acanthocephalan peritonitis associated with Profilicollis sp. was the primary COD for 11% of otters (61/560) and was a pooled top four COD for 24% (127/541). Bacterial co-infection was common; 61% of fatal AP cases also had bacterial peritonitis as a concurrent COD or sequela, compared with 4% for otters without AP. Fatal AP also co-occurred with fatal enteritis, enterocolitis or proctitis: Concurrent intestinal disease (possibly of bacterial and/or viral origin), was observed in 20% of AP cases, compared with 7% of otters without this condition.
As previously presented by others (Mayer et al., 2003), our study found a pattern of AP occurrence in sea otters with stranding near sandy beach habitat favored by crustacean intermediate hosts. Spatiotemporal SaTScan analysis showed a high-risk cluster for fatal AP near Moss Landing in 2002–2007 (RR = 2.31; P = 0.04) (Table 3 and Figure 3). However, portions of this same region were relatively low-risk for AP from 2007 to 2010 (RR = 0.067; P = 0.05). Seasonally, AP mortality was most prevalent in April and May (Figure 4A). The annual prevalence of AP varied in a cyclical pattern over the study period (Figure 4B).
The multivariate model evaluating factors associated with fatal AP included age class, nutritional condition, bacterial infection, GI erosions/ulcers, season, and the high-risk spatiotemporal cluster (n = 487; Table 4). Subadults were 4.13 times more likely to die from AP than adults or aged adults (P < 0.001). Emaciated animals had 1.84 greater odds of fatal AP than otters in better nutritional condition (P = 0.02). AP mortality was positively associated with bacterial infection (OR 2.54; P = 0.002) and GI erosions/ulcers (OR 2.06; P = 0.009). Otters that stranded in the high-risk spatiotemporal cluster had 3.61 times higher odds of fatal AP than those stranding elsewhere (P < 0.001) (Figure 3). Otters stranding during spring and summer were 1.6 times more likely to have fatal AP than those stranding in fall or winter, although this finding was not statistically significant (P = 0.1).
Fatal S. neurona and/or T. gondii infection accounted for 9% (50/560) of otter deaths as a primary cause, and 20% (108/547) as a primary or contributing cause (Table 2). Sarcocystis neurona was more than five times more common than T. gondii as a primary COD (Table 5). Because T. gondii and S. neurona commonly co-infect southern sea otters, it is not always possible to separate the impacts of each parasite; for at least 3% of cases (14/547), protozoal co-infection was considered a top four COD.
Table 5. Findings for terrestrial protozoan parasite (Toxoplasma gondii and Sarcocystis neurona or S. sp.) seroprevalence, infection and mortality for 560 southern sea otters (Enhydra lutris nereis), 1998 – 2012. Some seronegative sea otters (T. gondii or S. neurona titers ≤1:320 serum dilution) had microscopically-confirmed infection with T. gondii, S. neurona/S. sp., or both parasite groups, resulting in higher totals for confirmed-infected cases than seropositive cases. Fewer otters had confirmed infection with T. gondii only than were seropositive for T. gondii only because some seropositive animals were subsequently confirmed to have concurrent infection with S. neurona and moved to the dual infection column. Please see the text for additional details.
Many additional sea otters were infected with T. gondii and/or S. neurona, but the associated pathology was not considered severe enough to cause death. A total of 306 sea otters (57%) were seropositive for T. gondii and 244 (45%) were seropositive for S. neurona at a cutoff of ≥ 1:320 serum dilution (Table 5). Additional seronegative otters were confirmed to be infected with T. gondii (n = 28) and S. neurona (n = 63) on histopathology. In total, 12% of T. gondii-seronegative otters were infected with T. gondii, and 21% of S. neurona-seronegative otters were infected with S. neurona or S. spp. on histopathology. Based on pooled results from serology and microscopic examination, 62% (334/542) of all sampled sea otters were infected with T. gondii, and over half (57%; 307/539) were infected with S. neurona (Table 5). Over half (52%; 220/541) of otters with serological or histological evidence of protozoal infection were co-infected with both parasites (Table 5), and 78% (421/541) of all sampled sea otters were infected with S. neurona, T. gondii, or both parasites concurrently. Because histology and serology are less sensitive methods of parasite detection than PCR (Arkush et al., 2003; Rejmanek et al., 2010; Miller et al., 2018), the prevalence of T. gondii and S. neurona infection are likely higher than we have reported.
Spatial analysis of S. neurona infection (independent of COD) revealed a high-risk spatial cluster near Watsonville (RR = 1.68; P < 0.001), and a low-risk cluster along the central Big Sur coast (RR = 0.49; P = 0.001). A high-risk spatiotemporal cluster was also noted near Watsonville during 2007–2011 (RR = 1.89; P < 0.001).
Sarcocystis neurona mortality and seropositivity showed the greatest prevalence from March through May (Figure 4A), and 2004 had the highest annual S. neurona mortality (Table 3 and Figure 4B). Spatiotemporal SaTScan analysis of S. neurona mortality patterns revealed two overlapping high-risk clusters centered near Guadalupe and Cayucos in April 2004 (Table 3 and Figure 3). Otters stranding within these clusters were approximately 10.5 times more likely to have fatal S. neurona infection (P < 0.001), reflective of a previously described epizootic (Miller et al., 2010b).
The multivariate model evaluating factors associated with S. neurona mortality included sex, age class, nutritional condition and season (n = 547, Table 4). Subadults were 3.66 times more likely to die of S. neurona than adults or aged adults (P < 0.001), and males had 2.23-fold higher odds than females (P = 0.01). Emaciated otters were 2.12 times more likely to have died of S. neurona than animals in better nutritional condition (P = 0.01). Sarcocystis neurona mortality was highest during spring and summer, with 7.18 and 5.22 times higher odds compared with otters that stranded during fall/winter (P < 0.001), respectively.
Toxoplasma gondii seropositivity was highest in aged adults (72%; 76/105) compared with subadults (29%; 35/122), suggestive of chronic infection. SaTScan analyses for T. gondii infection (independent of COD) revealed a low-risk spatial cluster near Seaside (RR = 0.64; P = 0.05), and two low-risk spatiotemporal clusters near Seaside during 2005–2012 (RR = 0.43; P = 0.034), and northern Big Sur during 2006-2012 (RR = 0.44; P = 0.034).
Spatial analysis of T. gondii mortality identified three overlapping high-risk clusters at Oso Flaco, Morro Bay, and Cambria (RR = 3.00–3.49; P ≤ 0.008) (Table 3 and Figure 3). To the north, three overlapping low-risk clusters were identified at Año Nuevo, Aptos and Point Lobos (RR = 0.25–0.35; P ≤ 0.04); spatiotemporal analysis showed these areas were especially low-risk between 2005 and 2012 (RR = 0.085–0.13; P ≤ 0.03). In contrast with S. neurona, T. gondii mortality patterns were relatively constant over time, with little annual and inter-annual variation (Figures 4A,B).
The multivariate model evaluating factors associated with T. gondii mortality included age class and the high-risk spatial clusters (n = 547; Table 4). Subadult and adult sea otters were 1.89 times more likely to have died of T. gondii than aged adults, although this was not statistically significant (P = 0.2). The combined high-risk spatial clusters was a highly significant predictor of T. gondii-related mortality: otters that died near Oso Flaco, Morro Bay, and Cambria had 3.26 times higher odds of dying of systemic T. gondii infection than otters that died outside of these areas (P < 0.001).
Nasopulmonary acariasis (Halarachne halocheri infestation; Pesapane et al., 2018; Shockling-Dent et al., 2019) was a contributing COD for three otters. Larva migrans (brain infection by Baylisascaris sp. nematode larvae, most likely B. procyonis from raccoon [Procyon lotor] feces) was a contributing COD for an additional three otters. Verminous hepatitis associated with the nematode Capillaria hepatica was also observed in three cases, contributing to the death of one otter (Miller et al., 2020).
Bacterial infection was common as a primary or contributing COD, and as a sequela to other COD. Bacterial invasion was a primary COD for 6% (33/558) of otters, and a primary or contributing COD for 12% of animals (68/558) (Table 2). Over half (56%; 314/558) of otters had bacterial infection as sequelae, and 68% (377/558) had bacterial infection as a COD or sequela. Because this category encompassed diverse pathogens, space-time and multivariate regression analyses were not performed.
Fatal bacterial lesions included septicemia, abscesses, peri- or endocarditis, osteomyelitis, mastitis, endometritis, pyometra, nephritis, prostatitis, posthitis, cholecystitis, laryngitis, and pneumonia. Bacterial infection was most common as sequelae to AP, shark bite, mating trauma and fight trauma. Bacteria associated with fatal lesions included Streptococcus spp. (especially beta-hemolytic strains such as S. phocae), Erysipelothrix sp. (e.g., E. rhusiopathiae), Klebsiella pneumoniae, Pasteurella multocida, Salmonella sp., hemolytic Escherichia coli, Campylobacter spp., Staphylococcus spp. (e.g., S. delphini, S. intermedius, and S. schleiferi ss coagulans), Vibrio spp. (e.g., V. parahaemolyticus and V. alginolyticus), Fusobacterium spp. (e.g., F. necrophorum and F. nucleatum), Clostridium sp. (e.g., C. septicum, C. difficile and C. perfringens), Peptostreptococcus anaerobius, and Leptospira sp.
In addition to diagnostically confirmed bacterial infections, other less well-characterized conditions could have bacteria as an underlying cause, especially GI diseases. Due to limited knowledge, idiopathic enteric conditions were assessed separately from known bacterial or viral COD (Table 2), but many could have resulted from bacterial and/or viral infections.
The zoonotic fungus Coccidioides sp. (C. immitis or C. posadasii) was a primary COD for nine otters, and a primary or contributing COD for 2% (11/556) of animals (Table 2). Incidental Coccidioides sp. infections were not observed, and most cases received abbreviated necropsies to minimize zoonotic risk. Spatial SaTScan analysis revealed four overlapping high-risk clusters, one of which was marginally significant (Table 3). Otters stranding near Pismo Beach were 15.11 times more likely to have coccidioidomycosis than those stranding elsewhere (P = 0.06) (Table 3 and Figure 3). Nearly all cases (10/11) occurred between Morro Bay and Pismo Beach; a single case occurred in Moss Landing. Our findings emphasize the localized occurrence of this zoonotic pathogen in coastal California.
Viral infection was a primary COD for three otters, and a primary or contributing COD for 1% (8/550) of animals (Table 2). Canine distemper virus was suspected in most cases, although diagnostic test results were inconclusive (data not shown), prompting further investigation. Uncharacterized viral deaths included infections associated with oropharyngitis, esophagitis, adrenalitis, lympholysis, and enterocolitis.
Age and sex-associated conditions included ELS, mating trauma and abortion or uterine torsion (females), fight trauma (males), and dental disease (aged adults) (Table 2).
End lactation syndrome affects only adult and aged adult females (183/231 females in the study were in these age groups), exerting substantial negative impacts on this demographic cohort (Chinn et al., 2016). End lactation syndrome accounted for 23% (42/183) of reproductive-age female deaths as a primary cause, and 58% (106/183) as a primary or contributing COD (Table 2). Of adult and aged adult females in late pup care through post-weaning at the time of death (otters eligible for ELS assessment), 83% (104/124) had ELS as a primary or contributing COD. When examined in the overall context of this 560 animal study, ELS accounted for 19% of all otter deaths as a primary and contributing cause.
No high-risk ELS spatial or temporal clusters were identified, but a low-risk spatiotemporal ELS cluster was found near Morro Bay during 2007–2012 (RR = 0.082; P = 0.01) (Table 3). ELS mortality was most prevalent in the late spring and early summer (Figure 4A) with little inter-annual variation, likely due to peak pupping during winter (Chinn et al., 2016).
The multivariate model evaluating factors associated with ELS mortality included age class, GI erosions/ulcers, season and the interaction of kelp canopy cover and the K-index (n = 168; Table 4 and Supplementary Figures 1–3). For this model, season was defined slightly differently in which late spring/summer spanned from April – July (reflecting seasonal peaks in southern sea otter reproduction and associated ELS mortality). Aged adult females were 12.70 times more likely to have died from ELS than adults (P < 0.001). Fatal ELS was associated with stranding in late spring and early summer (OR = 2.47; P = 0.02) and having GI erosions/ulcers (OR = 7.46; P < 0.001). The best-supported model indicated that an interaction between kelp cover and K-index was more useful for predicting fatal ELS than either variable alone. An increase in kelp canopy had little effect on ELS death for females inhabiting areas below K, but was associated with increased ELS risk for females from regions at/near K (Supplementary Figure 6). Females from areas of the range that were at or near K (mostly within the range center) and with dense kelp cover were 4.21 times more likely to have died from ELS than females from areas of the range that were well below K, and having limited kelp cover (P = 0.002; Table 4 and Supplementary Figures 7, 8).
Serious fight wounds were common for adult and aged adult males: 89% (41/46) of cases were in these age classes. Fight trauma was a COD for 14% of males (46/329; Table 2) and 8% (46/560) of all enrolled otters. Fight-associated wounds on the head, feet, testicles and penis occasionally contained sea otter tooth fragments, especially the tips of canine teeth. Older males often had healed baculum and digit fractures indicative of prior sublethal fight trauma.
Mating trauma represented 24% (56/231) of COD among females (Table 2), and 10% (56/560) of deaths among all otters. Many females with fatal mating trauma were also ELS cases. Nearly all 98% (55/56) females with fatal mating trauma had moderate/severe nose wounds; fatal vaginal or rectal perforations were less common. Portions of the nose and face were sometimes severely lacerated by males. Severe tissue swelling, pulmonary emphysema and bacterial infection were common sequelae; pneumothorax, pneumoabdomen and subcutaneous emphysema were less common.
Biotoxin poisoning of any type (DA, microcystin or saxitoxin) was a primary COD for 10% (57/560) of otters (Table 2).
Probable DA intoxication was a primary COD for 9% (52/560) of otters and was a primary or contributing COD for 20% (112/549) (Table 2). Possible DA intoxication was a primary COD for 1% (8/560) of otters and was a primary or contributing COD for 21% (116/549). Pooled results for probable and possible DA intoxication yielded 11% (60/560) as a primary COD, and 41% as a primary or contributing COD (228/549).
Spatial, temporal, or spatiotemporal clusters were not detected for probable DA intoxication. Sea otter death from probable DA intoxication occurred in all seasons (Figure 4A) and years (Figure 4B) of the study. Otter deaths from probable DA intoxication were especially prominent during 1998 – 2003, and 2009 – 2010, although these peaks were not statistically significant.
The multivariate model evaluating factors associated with probable DA intoxication as a primary or contributing COD included sex, age class and nutritional condition (n = 208; Table 4). Terrestrial land-cover and marine environmental variables were not significant predictors of DA-associated mortality. Males had 2.39-fold higher odds of death from DA than females (P = 0.007). Adults were 1.57 times more likely to have died from DA intoxication than subadults or aged adults, although this finding was not statistically significant (P = 0.2). Otters in fair to excellent nutritional condition were 4.99 times more likely to have died from DA than those in worse nutritional states (P < 0.001). A sub-analysis of 86 otters with identifiable prey in their GI tracts included sex, age class, nutritional condition, clam and crab presence in digesta (Supplementary Table 2). In this model, clams (OR = 8.07; P = 0.07) and crabs (OR = 2.01; P = 0.3) increased the odds of fatal DA intoxication, although not statistically significant at the α = 0.05 level, likely due to small sample size (Supplementary Table 3).
Based on biochemistry, necropsy, and histopathology, possible microcystin intoxication was a primary COD for <1% (5/560) of enrolled otters, and a primary or contributing COD for 2.5% (14/547; Table 2). Although additional animals were microcystin-positive based on biochemical testing of tissues, this toxin was not necessarily considered a primary or contributing COD.
Although no otter deaths were attributed to saxitoxin in the current study (136 animals were tested), this neurotoxin can cause death with no discernible gross or microscopic lesions, so fatal cases may have been missed; additional research is necessary.
Cardiomyopathy was a primary COD for 8% (44/560) of otters and was a primary and contributing COD for 41% (229/552) (Table 2). SaTSCan analyses identified a high-risk spatial cluster for fatal cardiomyopathy near Moss Landing (RR = 1.69; P = 0.01), and a high-risk temporal cluster between March – June 2004 (RR = 2.12; P = 0.01) (Table 3 and Figure 3). Fatal cardiomyopathy was prevalent in all months, especially April through June, and December (Figure 4A) and all study years, especially 2004 – 2006 (Figure 4B). The annual prevalence of fatal cardiomyopathy varied in a cyclical pattern over the study period (Figure 4B).
The multivariate model evaluating factors associated with fatal cardiomyopathy included age class, probable DA intoxication, S. neurona infection, T. gondii infection and the high-risk spatial and temporal clusters (n = 529; Table 4). Aged adults were 11.48 times more likely to have died of cardiomyopathy than subadults (P < 0.001), and adults were 2.98 times more likely than subadults (P = 0.001). Sea otters with probable DA intoxication were 4.64 times more likely to have died of cardiomyopathy than animals that were not considered DA cases (P < 0.001). Sea otters with T. gondii infection had 2.31-fold higher odds of fatal cardiomyopathy (P < 0.001) and those with S. neurona infection were 1.91 times more likely to have fatal cardiomyopathy (P = 0.004) than uninfected otters. Otters that stranded within the high-risk Moss Landing cluster were almost three-times more likely to have died from cardiomyopathy than those stranding elsewhere (P < 0.001). Animals that stranded from March – June 2004 were 11.88 times more likely to have fatal cardiomyopathy than otters that stranded outside of this temporal window (P < 0.001).
Although GI erosions/ulcers and melena were not a primary COD for any otters, they were a common contributing COD that may be a non-specific expression of severe stress, inanition, emaciation, poor health, or Helicobacter sp. mucosal bacterial infections. Of 500 otters assessed for this condition, 59% (295/500) had gastric erosions/ulcers, and 47% (237/500) had lesions severe enough to be a contributing COD (Table 2), representing the most prevalent top four COD in this study. Erosions and ulcers were twice as common in otters that were in poor or emaciated nutritional condition at necropsy (56%; 144/258), when compared with otters in fair, good or excellent nutritional condition (28%; 27/238).
Emaciation/starvation was an important contributing COD, affecting 23% (130/560) of animals (Table 2). This condition is distinct from ELS-associated emaciation, and may be the physical expression of multiple stressors, including competition, food scarcity and disease.
Direct anthropogenic (human-caused) sea otter mortality was documented for management and mitigation purposes. In this study, direct anthropogenic mortality affected 7% (38/560) of otters as a primary or contributing COD (Table 2). Boat strike was the most common anthropogenic COD; 16/25 boat strike cases were within three km of harbors at Santa Cruz, Moss Landing, Monterey or Morro Bay. Other anthropogenic COD (fishing gear entanglement, gunshot and fishhook ingestion) were relatively uncommon (Table 2). This category excluded medical complications related to permitted capture, surgery and other veterinary procedures (7/560).
Other less common and less well-characterized COD included tumors (3%; 17/556) and various non-specific respiratory conditions (pulmonary emphysema, pulmonary edema and terminal drowning, possibly as sequelae to other, unknown conditions), affecting 7% (40/560) of sampled otters (Table 2). Fatal tumors included lymphosarcoma and large cervical or uterine leiomyomas that were associated with dystocia or pelvic obstruction. Miscellaneous COD (5%; 28/560) included idiopathic anemia, dyspnea and pneumonia, osteosclerosis/osteoarthritis, dehydration, lymphadenopathy, nephrosclerosis, blindness, rectal prolapse, rhabdomyolysis, splenic torsion and possible zinc toxicosis.
We compiled findings from 15 years of southern sea otter postmortem examinations, incorporating data from 560 animals; this large sample and extended time period enabled us to assess longitudinal mortality patterns in a changing environment. Using knowledge gained from necropsy, histopathology and research, we updated case assessments and performed comprehensive testing for biotoxins and parasites. Novel analytical techniques were used to identify mortality patterns and coastal areas of high mortality risk. We investigated associations between common conditions and biological and environmental variables that might enhance the risk of adverse health outcomes. Characterizing factors that increase the risk of sea otter death from common health conditions can help focus population recovery and environmental mitigation efforts.
A fundamental goal of this study was to update methods of classifying causes of death in southern sea otters. Accurately assessing the complex interplay between free-ranging animals and their dynamic habitat, pathogens, toxins, prey and predators can be challenging, especially for this threatened species with unique biology. These small marine mammals have very high metabolic demands (Yeates et al., 2007) and spend their entire lives along the interface between land and sea. Southern sea otters inhabit the coastal ecosystem and consume large amounts of benthic invertebrates from the nearshore subtidal zone. Because some preferred prey can concentrate terrestrial and aquatic pathogens or toxins through filtration or other feeding methods, the diet and ecology of sea otters places them at the confluence of two ecosystems, where they are sensitive to, and reflective of, changes in either domain. This study also identified several spatial and temporal “hot spots” for many diseases, including terrestrial pathogens of sea otters and humans (e.g., T. gondii and Coccidioides sp.). Investigation of these high-risk zones may reveal opportunities to facilitate sea otter recovery and protect human health.
Our work has confirmed that the list of terrestrial pathogens infecting southern sea otters includes land-based fungi (Coccidioides spp.), viruses (putative canine distemper virus), and parasites associated with cats (T. gondii), opossums (S. neurona), rodents (C. hepatica) and raccoons (putative B. procyonis). These terrestrial pathogens can be fatal, and the parasites likely infect sea otters through a similar route; land-based eggs/oocysts that flow downstream into marine habitats, where they can concentrate in invertebrates consumed by sea otters (Miller et al., 2008; Shapiro et al., 2012). Spatiotemporal patterns of fatal Coccidioides spp. infection in sea otters mirror those of sympatric marine mammals, humans, and terrestrial animals (Huckabone et al., 2015). Our work has confirmed that infection by land-based parasites is common in sea otters, and novel terrestrial pathogens were detected in this study, underscoring the principle of land-sea pathogen flow as a risk for sea otter fitness and survival.
Shark-associated mortality increased dramatically during our study period, becoming the most common primary COD. Many otters that died of shark bite appeared to be otherwise healthy, and good body condition was predictive of shark bite mortality (Table 4). The 1994 closure of the nearshore gillnet fishery in southern California, and closer regulation of the offshore driftnet fishery, resulted in greatly reduced mortality for juvenile white sharks in the late 1990s, just before our study began (Heneman and Glazer, 1996; Lowe et al., 2012). As these large marine predators increased and/or redistributed through time, negative impacts on southern sea otters have grown.
We have identified high-risk zones for shark mortality at both ends of the otter range, consistent with previous analyses that suggest shark/sea otter conflict may be slowing population growth and preventing range expansion (Tinker et al., 2016; Nicholson et al., 2018; Moxley et al., 2019). Our findings were consistent with a report encompassing a larger set of stranded southern sea otters from 1985 to 2013 (Tinker et al., 2016) that identified similar hot spots for shark bite mortality, and a dramatic increase in shark bite mortality over time. Two factors have been suggested as possibly contributing to the spatial pattern of higher shark bite mortality near the edges of the range: (1) proximity to pinniped breeding and haul-out aggregations, and (2) a greater preponderance of kelp-free habitat (e.g., open sandy embayments) near the range-ends, combined with the fact that presence of kelp canopy may lower the risk of shark-bite mortality (Tinker et al., 2016; Nicholson et al., 2018; Moxley et al., 2019).
A progressive increase in shark-associated otter deaths and broadening of seasonal prevalence was also observed during our 15 year study; if this pattern continues, seasonal peaks may become less apparent over time (Supplementary Figure 5). Although there are no clear management options for reducing otter-shark interactions, this increasingly important source of mortality may require greater focus on mitigating causes of mortality that are amenable to conservation efforts, including direct and indirect anthropogenic factors.
A notable finding of our study was that over half (53%) of otters with fatal shark bite died from post-traumatic bacterial infections, and we have no evidence to confirm sea otter tissue consumption by sharks. These findings support a previously proposed hypothesis that sharks are not biting sea otters with the intention of killing and consuming them, but instead are investigating surface silhouettes through mouthing or bumping (Tinker et al., 2016).
Profilicollis sp.-associated peritonitis was a primary or contributing COD for nearly a quarter of all otters in this study, and many of these cases also had bacterial peritonitis or enteritis, suggesting that enteric acanthocephalans may facilitate infection by other opportunistic pathogens. Interestingly, the prevalence of AP varied throughout the study in a cyclical fashion (Figure 4B), suggestive of underlying ecological drivers and/or fluctuating population immunity.
Our findings from protozoal serology and histopathology revealed that >75% (408/541) of sampled sea otters were infected with S. neurona, T. gondii, or both parasites concurrently. These methods of parasite detection are cost-effective, but relatively insensitive. Because these protozoal detection methods are less sensitive than PCR (Arkush et al., 2003; Rejmanek et al., 2010; Miller et al., 2018), the prevalence of T. gondii and S. neurona infection are likely higher than we have reported.
The southern portion of the otter range was higher risk for fatal T. gondii than the northern and central regions (Figure 3). Shapiro et al. (2019) showed a similar pattern and revealed that 79% of T. gondii genotypes from southern sea otters necropsied 1998–2015, including animals from the current study, were atypical Type X or Type X variants. In our double-blind comparison, all otters that died from T. gondii as a primary COD were infected with Type X or X-variant T. gondii, and identical strains were detected in terrestrial felids from adjacent watersheds. Sarcocystis neurona was a more common COD than T. gondii (Table 5), and protozoal co-infection was fatal for at least 6% of cases. Although we did not measure associations for this study, otters with concurrent S. neurona and T. gondii infection often exhibited more severe lesions than animals with monoparasitic disease, supporting a report by Gibson et al. (2011).
Because we focused on assessing mortality patterns for subadults, adults, and aged adults in the current study, causes of mortality that are more common in younger sea otters (pups and juveniles) were not fully represented in this overview. For example, S. neurona and AP prevalence (Kreuder et al., 2003; Mayer et al., 2003; Miller et al., 2010b) are likely to be lower in our study population relative to the wild population in its entirety.
Domoic acid intoxication is an important emerging threat associated with climate change that has the potential to negatively affect southern sea otter population recovery. This potent neurotoxin, produced by the diatom Pseudo-nitzschia sp., is pervasive throughout California marine waters and biota (Bargu et al., 2010; McKibben et al., 2017), so it was critical to evaluate the health impacts of DA for southern sea otters. Many of the invertebrates consumed by sea otters (including crabs and bivalves) have been shown to effectively concentrate and maintain DA in tissues for weeks to months after exposure to toxic blooms (Goldberg, 2003), and fisheries are often closed to protect humans from DA-associated health risks (Mariën, 1996; Trainer et al., 2007). Sea otters continue eating marine invertebrates during toxic blooms when fisheries are closed for public safety. Because sea otters consume approximately 25% of their body weight in prey every day (Yeates et al., 2007), it is likely that all wild southern sea otters are intermittently or chronically exposed to DA. Due to their small body size, high metabolism and diverse prey preferences, sea otters are particularly susceptible to DA exposure, though the toxic dose for sea otters may differ from human alert levels (Mariën, 1996).
We utilized a preponderance of evidence approach to assess DA intoxication in this study and we describe our case definition in a companion paper (Miller et al., in review). Based on our conservative estimate, probable DA intoxication was a primary or contributing COD for 20% of sea otters in this study. It is important to note that our case definition focused on acute/subacute DA impacts. Because DA can cause acute, subacute, and chronic disease, and lifelong damage can occur to fetuses exposed in utero (Pulido, 2008), the population-level effects of DA on southern sea otters are likely to be far greater than we have reported.
Our findings suggest that clams and crabs may be high-risk prey for fatal DA intoxication in sea otters, and high DA concentrations were detected in otters year-round, including periods when no toxic Pseudo-nitzschia blooms were detected by public health monitoring programs. This study provides critical insight into a previously uncharacterized threat for southern sea otter recovery. Because humans and sea otters consume similar marine foods, our findings may also help inform human health precautions. Sea otter mortality patterns often reflect environmental perturbations (Miller et al., 2010a, b; Huckabone et al., 2015). Pseudo-nitzschia bloom frequency, severity, and persistence appear to be increasing along Pacific Coastal North America in relation to climate change (Moore et al., 2008; Bargu et al., 2010; Hinder et al., 2012; McKibben et al., 2017), suggesting that DA-associated otter deaths are also likely to increase over time.
We have demonstrated significant associations between DA intoxication and cardiomyopathy in southern sea otters, which supports our previous research identifying DA as a potential risk factor for dilated cardiomyopathy (Kreuder et al., 2005). Although it is challenging to estimate the proportion of cardiomyopathy deaths that were partially or solely attributable to DA intoxication, our data suggests that many animals survived the acute impacts of DA exposure, but died later from severe, progressive, DA-mediated cardiomyopathy. Cardiomyopathy is also a common finding in California sea lions with acute and chronic DA toxicity (Zabka et al., 2009). Cardiomyopathy is likely a multifactorial condition and it is challenging to isolate the effects of DA exposure on heart disease development. The prevalence of fatal cardiomyopathy varied in a cyclical pattern over the study period. Interestingly, the cardiomyopathy case peaks and troughs mirrored cyclical patterns observed for fatal AP (Figure 4B). These conditions are not known to be causally related, so climatic conditions or other exogenous factors may influence the prevalence of both conditions.
Our findings demonstrate similarities and differences from prior sea otter mortality surveys. Kreuder et al. (2003), which included a subset of the earliest animals examined for our study, reported that 4.8% (5/105) of otters had DA intoxication as a COD, compared with >20% in the current study. These differences reflect improvements in case detection, broader access to diagnostic tests, and perhaps increased case frequency over time, although this cannot be confirmed.
Similarly, the number of fatal microcystin intoxication cases in this study (14/547) was less than reported in an earlier study (Miller et al., 2010c; n = 21) due to differences in case selection and more stringent case definition criteria in the current study. The toxic dose of microcystin is unknown for sea otters and potential lesions are poorly characterized, so additional cases could have been missed. There are many different congeners of microcystin and saxitoxin, and these toxins can be difficult to detect and quantify in body fluids and tissues. In addition, detection of low concentrations of microcystin and saxitoxins in tissues or body fluids does not necessarily confirm these toxins as a COD.
Kreuder et al. (2003) reported a significantly elevated risk for traumatic death in sea otters with pooled cases of pre-existing moderate to severe brain inflammation or necrosis from any cause. This relationship was not assessed as part of the current study. Instead, utilizing a larger sample size and more stringent pathology coding criteria, we assessed associations between certain fatal conditions known to cause CNS dysfunction (e.g., probable DA intoxication or protozoal COD) and trauma as a possible risk factor. No association was found using pairwise comparisons (univariate analyses), so conditions causing CNS dysfunction were not included as risk factors in the multivariate models for traumatic causes of death. Kreuder et al. (2003) also found that AP was the most prevalent COD for southern sea otters. We found that AP was the second most prevalent COD, possibly due to a progressive increase in shark bite COD, and because our study excluded juvenile otters that are more likely to die from AP.
White et al. (2018) reviewed COD for 333 northern sea otters (E. l. kenyoni) from Washington and Oregon that were necropsied between 2002-2015; infectious disease was the most common COD in that sample (56%), especially those caused by protozoa (S. neurona) and bacteria (S. phocae). Shark bite was rare (two cases), and although fatal cardiomyopathy was noted, no DA-associated mortality, AP, or ELS cases were reported. Some subpopulations of northern sea otters are growing faster than the southern subspecies in part due to habitat differences, including a relatively undeveloped coastline, lower sea otter population density and minimal shark predation pressure (White et al., 2018). Although no in-depth pathology survey is available for northern sea otters in Alaska, exposure to S. neurona, T. gondii, and Profilicollis sp. are much lower (Miller et al., 2002; Hanni et al., 2003; Mayer et al., 2003; Burgess et al., 2018, 2020). For Alaskan sea otters tested 2004–2011, 25% (43/172) were DA-positive, 23% (37/163) were saxitoxin-positive, and saxitoxin-associated otter deaths were reported (Lefebvre et al., 2016).
Compiled data from tagged and live-stranded otters can also provide insight on mortality factors (Nicholson et al., 2018), although conditions requiring histopathology for confirmation (e.g., DA intoxication, cardiomyopathy, ELS, neoplasia and many parasitic, bacterial, viral, and fungal infections) will be underrepresented. Where possible, future population models should incorporate data from both antemortem and postmortem monitoring programs (e.g., Tinker et al., in review).
Our research identified key sources of mortality that are potential obstacles for southern sea otter population recovery. Because southern sea otter carcass recovery is opportunistic and uneven across a >400 km geographic range, and because demographic processes and population status vary considerably within this range (Tinker et al., 2006, 2019), mortality patterns were interpreted in the context of regional population indices where possible. Some COD can negatively influence population growth as an expression of resource limitation in densely occupied habitat, such as fight trauma and ELS, while others such as shark bite or DA exposure do not appear to be density dependent. Mortality from shark bite has increased and is now the most common primary COD; the concentration of shark bite deaths at the range peripheries may impede future population recovery by limiting expansion into unoccupied habitats. Additional COD, such as DA intoxication and cardiomyopathy, appear to be inter-related, suggesting that their combined impacts are more substantial than previously understood.
Several COD exert effects across the entire southern sea otter range, but may be especially severe in regions that are more impacted by humans (Miller et al., 2010a, b; Oates et al., 2012; Huckabone et al., 2015; Shapiro et al., 2019). Similar to previous reports (Thomas and Cole, 1996; Kreuder et al., 2003), infectious agents were the most prevalent cause of mortality when considering both primary and contributing COD in our study (Table 2). Multi-pathogen infections were extremely common, and mortality due to concurrent terrestrial (e.g., T. gondii and S. neurona) and marine pathogen exposure (e.g., Profilicollis sp., S. phocae, and Vibrio sp.) was documented throughout the southern sea otter range.
In addition to impacts on survival, sublethal polymicrobial infections may reduce fitness and lower resistance to other threats. Sea otters have the highest mass-specific energy requirements of any marine mammal (Thometz et al., 2014), and reproduction, pathogen or toxin exposure and human disturbance can exacerbate nutritional stress. Efforts to mitigate indirect anthropogenic threats, such as terrestrial pathogen, nutrient and other pollutant discharges can provide strong collateral benefits to sea otters, marine ecosystems and humans who rely on these habitats for food and other resources. The potential for anthropogenic nutrient discharges (e.g., sewage and fertilizer) to stimulate or enhance toxic algal blooms is increasingly recognized (Trainer et al., 2007; Cochlan et al., 2008; Kudela et al., 2008). Other occult sources of anthropogenic sea otter mortality may include drowning in fishing traps, as some of these carcasses could sink rather than float to shore (Hatfield et al., 2011).
Ultimately, a key component of southern sea otter population recovery efforts will be range expansion. To the extent that some potential future habitats (e.g., estuaries) may also have lower risk of shark bite mortality (Hughes et al., 2019; Mayer et al., 2019), this conservation approach may address several of the emerging challenges identified by our analyses. As management actions are taken to enhance range expansion, we recommend continued population monitoring and careful assessment of potential threats in currently unoccupied habitat.
This is one of the most comprehensive and detailed reviews of mortality patterns and disease risk factors in a free-ranging wildlife species. Our research has revealed insight on mortality trends, emerging threats, and spatial and temporal disease “hot spots,” and confirmed the importance of southern sea otters as sentinels for terrestrial-origin pollution. It has also demonstrated the importance of consistent, long-term monitoring efforts that influence conservation priorities and animal care; our 15 year perspective illustrates how predominant disease patterns and their ecological drivers change over time. Identifying mechanisms by which sea otters are exposed to pollutants is critical to develop effective management actions, improve clinical care, and decrease health risks for humans and wildlife at the land-sea interface. Knowledge gained from this study has been used in an integrated population model (Tinker et al., in review) that will help clarify processes influencing southern sea otter survival, inform policy, and guide consideration of this population for de-listing as a threatened species under the Federal Endangered Species Act.
The raw data supporting the conclusions of this article will be made available by the authors, without undue reservation.
Ethical review and approval was not required for the animal study because the specimens used were from post-mortem samples and were collected as part of California Department of Fish and Wildlife’s sea otter necropsy program.
MAM directed, administered, and funded the project, conducted the histological analyses, completed all necropsy case reviews, and wrote the manuscript. MEM served as technical staff lead for the project, completed some epidemiological analyses, and helped substantially with manuscript completion. LH helped substantially with updating coding techniques, COD and sequelae, and led on non-epidemiological data compilation and analysis. MT had a substantial role in complex model development and drafting explanatory text and figures, especially for ELS and shark bite. TB led completion of spatial/temporal cluster analysis and aided development of the DA regression model and some of the core figures. FB assisted substantially with sea otter necropsy, data entry, data crosschecking, case coding, diagnostic testing, developing case definitions for key COD and sequelae, manuscript preparation and submission. ED assisted substantially with necropsy, data entry, data crosschecking, case coding, diagnostic testing, developing case definitions, and manuscript preparation. CY assisted substantially with necropsy, data crosschecking, developing case definitions, developing figures related to annual mortality proportions, and manuscript preparation. MH assisted substantially with necropsy, data crosschecking, developing case definitions, helped develop figures related to annual mortality proportions, and manuscript preparation. DJ provided core physical and financial support for development and funding of the CDFW sea otter pathology program and assisted with necropsies, sampling, testing, carcass recovery, and manuscript preparation. JA substantially assisted with sea otter capture, carcass recovery and necropsies, helped develop the spatial mapping system that was central to epidemiological analyses, and assisted with developing case definitions and manuscript preparation. PC and AP provided intellectual input on testing and sampling for serum collection, storage, evaluation, and interpretation, and organized and tested all serum samples. CJ served in a lead role for project design, method development, and completion of epidemiological analyses, and served as the core mentor and provided funding support for the collaborating graduate students (MEM and TB). She also had a lead role in optimizing sections of the manuscript pertinent to statistical analyses, modeling and sea otter disease. All the authors reviewed, edited, and approved the manuscript.
We thank the citizens of California for making this work possible through contributions to the California Sea Otter Fund (Sea Otter Tax Checkoff). Tax checkoff funding to support this work was made available through the California State Coastal Conservancy, and the California Department of Fish and Wildlife – Office of Spill Prevention and Response. Megan Moriarty was supported by the Morris Animal Foundation Fellowship Training Grant (D17ZO-413) during this study.
MT was employed by the company Nhydra Ecological Consulting. TB was employed by the company Acadia Wildlife Services.
The remaining authors declare that the research was conducted in the absence of any commercial or financial relationships that could be construed as a potential conflict of interest.
This project would not have been possible without assistance from many contributors, especially (by organization): University of California, Davis: Tracey Goldstein, Walter Boyce, Alyssa Capuano, Stori Oates, Jonna Mazet, Mike Ziccardi, Matthew Blake, Kirsten Gilardi, Linda Lowenstein, Lavonne Hull, Woutrina Miller; California Department of Fish and Wildlife: Katherine Greenwald, Angelina Reed, Jessica Kunz, Eva Berberich, Sharon Toy-Choutka, Christen Bechert, Sara Huckabone, Sarah Chinn; University of California, Santa Cruz: Raphe Kudela; U.S. Geological Survey: Brian Hatfield, Joe Tomoleoni, Ben Weitzman; Monterey Bay Aquarium: Mike Murray, Michelle Staedler, Karl Mayer, Andy Johnson, Marissa Young. Central Coast Water Quality Control Board: Karen Worcester, David Paradies, Mary Adams; U.S. Fish and Wildlife Service: Lilian Carswell; The Marine Mammal Center: Frances Gulland, Padraig Duignan, Barbie Halaska; Greenwater Laboratories: Amanda Foss, Mark Aubel; Blood Systems Research Institute: Eric Delwart, Juliana Siqueira, Terry Ng, Linlin Li, Xutao Deng; University of Illinois: Katie Colegrove; NOAA Northwest Fisheries Science Center: Kathi Lefebvre; Alaska Veterinary Pathology Services: Kathy Burek. We thank the University of California, Davis VMTH histology and microbiology staff, especially Becky Griffey, and the CAHFS staff, especially Robert Poppenga, Karen Sverlow, and Leslie Woods for their outstanding support.
The Supplementary Material for this article can be found online at: https://www.frontiersin.org/articles/10.3389/fmars.2020.00582/full#supplementary-material
Ames, J. A., and Morejohn, G. V. (1980). Evidence of white shark, Carcharodon carcharias, attacks on sea otters, Enhydra lutris. Calif. Fish Game 66, 196–209.
Arkush, K. D., Miller, M. A., Leutenegger, C. M., Gardner, I. A., Packham, A. E., Heckeroth, A. R., et al. (2003). Molecular and bioassay-based detection of Toxoplasma gondii oocyst uptake by mussels (Mytilus galloprovincialis). Int. J. Parasitol. 33, 1087–1097. doi: 10.1016/S0020-7519(03)00181-4
Bargu, S., Silver, M., Goldstein, T., Roberts, K., and Gulland, F. (2010). Complexity of domoic acid-related sea lion strandings in Monterey Bay, California: foraging patterns, climate events, and toxic blooms. Mar. Ecol. Prog. Ser. 418, 213–222. doi: 10.3354/meps0881
Bartlett, B., Miller, W., Dominik, C., Batac, F., Dodd, E., Byrne, B., et al. (2016). Prevalence, pathology and potential risk factors associated with Streptococcus phocae infection in southern sea otters (Enhydra lutris nereis) (2004-2010). J. Wildl. Dis. 52, 1–9. doi: 10.7589/2015-02-048
Burgess, T. L., Tinker, M. T., Miller, M. A., Bodkin, J. L., Murray, M. J., Saarinen, J. A., et al. (2018). Defining the risk landscape in the context of pathogen pollution: Toxoplasma gondii in sea otters along the Pacific Rim. R. Soc. Open Sci. 5:171178. doi: 10.1098/rsos.171178
Burgess, T. L., Tinker, M. T., Miller, M. A., Smith, W. A., Bodkin, J. L., Murray, M. J., et al. (2020). Spatial epidemiological patterns suggest mechanisms of land-sea transmission for Sarcocystis neurona in a coastal marine mammal. Sci. Rep. 10, 1–9. doi: 10.1038/s41598-020-60254-5
Capuano, A. M., Miller, M. A., Stallknecht, D. E., Moriarty, M., Plancarte, M., Dodd, E., et al. (2017). Serologic detection of subtype-specific antibodies to influenza A viruses in southern sea otters (Enhydra lutris nereis). J. Wildl. Dis. 53, 906–910. doi: 10.7589/2017-01-011
Chinn, S. M., Miller, M. A., Tinker, M. T., Staedler, M. M., Batac, F. I., Dodd, E. M., et al. (2016). The high cost of motherhood: end-lactation syndrome in southern sea otters (Enhydra lutris nereis) on the central California Coast. USA. J. Wildl. Dis. 52, 307–318. doi: 10.7589/2015-06-158
Cochlan, W. P., Herndon, J., and Kudela, R. M. (2008). Inorganic and organic nitrogen uptake by the toxigenic diatom Pseudo-nitzschia australis (Bacillariophyceae). Harm. Algae 8, 111–118. doi: 10.1016/j.hal.2008.08.008
Fire, S. E., and Van Dolah, F. M. (2012). Marine biotoxins: Emergence of Harmful Algal Blooms as Health Threats to Marine Wildlife. Washington, DC: U.S. Department of Commerce, 553.
Gibson, A. K., Raverty, S., Lambourn, D. M., Huggins, J., Magargal, S. L., and Grigg, M. E. (2011). Polyparasitism is associated with increased disease severity in Toxoplasma gondii-infected marine sentinel species. PLoS Negl. Trop. Dis. 5:e1142. doi: 10.1371/journal.pntd.0001142
Goldberg, J. D. (2003). Domoic acid in the benthic food web of Monterey Bay, California. Master’s Thesis, California State University Monterey Bay, Seaside, CA.
Goldstein, T., Mazet, J. A. K., Zabka, T. S., Langlois, G., Colegrove, K. M., Silver, M., et al. (2008). Novel symptomatology and changing epidemiology of domoic acid toxicosis in California sea lions (Zalophus californianus): an increasing risk to marine mammal health. P. Roy. Soc. B-Biol. Sci. 275, 267–276. doi: 10.1098/rspb.2007.1221
Green, P. J., and Silverman, B. W. (1993). Nonparametric Regression and Generalized Linear Models: A Roughness Penalty Approach. London: CRC Press.
Hanni, K. D., Mazet, J. A., Gulland, F. M., Estes, J., Staedler, M., Murray, M. J., et al. (2003). Clinical pathology and assessment of pathogen exposure in southern and Alaskan sea otters. J. Wildl. Dis. 39, 837–850. doi: 10.7589/0090-3558-39.4.837
Harris, H. S., Oates, S. C., Staedler, M. M., Tinker, T., Jessup, D. A., Harvey, J. T., et al. (2010). Lesions of juvenile harbor seals associated with forced copulation by southern sea otters. J. Aquat. Mamm. 36, 219–229. doi: 10.7589/0090-3558-39.4.837
Hatfield, B. B., Ames, J. A., Estes, J., and Tinker, M. T. (2011). Sea otter mortality in fish and shellfish traps: estimating potential impacts and exploring possible solutions. Endang. Sp. Res. 13, 219–229. doi: 10.3354/esr00327
Heneman, B., and Glazer, M. (1996). “More rare than dangerous: a case study of white shark conservation in California,” in Great White Sharks: The Biology of Carcharodon Carcharias, eds A. P. Klimley, and D. G. Ainley (Amsterdam: Elsevier Inc), 481–491. doi: 10.1016/b978-012415031-7/50046-x
Hinder, S. L., Hays, G. C., Edwards, M., Roberts, E. C., Walne, A. W., and Gravenor, M. B. (2012). Changes in marine dinoflagellate and diatom abundance under climate change. Nat. Clim. Change 2, 271–275. doi: 10.1038/NCLIMATE1388
Huckabone, S. E., Gulland, F. M., Johnson, S. M., Colegrove, K. M., Dodd, E. M., Pappagianis, D., et al. (2015). Coccidioidomycosis and other systemic mycoses of marine mammals stranding along the central California, USA coast: 1998–2012. J. Wildl. Dis. 51, 295–308. doi: 10.7589/2014-06-143
Hughes, B. B., Wasson, K., Tinker, M. T., Williams, S. L., Carswell, L. P., Boyer, K. E., et al. (2019). Species recovery and recolonization of past habitats: lessons for science and conservation from sea otters in estuaries. PeerJ 7:e8100. doi: 10.7717/peerj.8100
Jessup, D. A., Miller, M., Ames, J., Harris, M., Kreuder, C., Conrad, P. A., et al. (2004). Southern sea otter as a sentinel of marine ecosystem health. EcoHealth 1, 239–245. doi: 10.1007/s10393-004-0093-7
Jessup, D. A., Miller, M. A., Kreuder-Johnson, C., Conrad, P. A., Tinker, M. T., Estes, J., et al. (2007). Sea otters in a dirty ocean. JAVMA 231, 1648–1652. doi: 10.2460/javma.231.11.1648
Johnson, S., Jang, S., Gulland, F., Miller, M. A., Casper, D., Lawrence, J., et al. (2003). Characterization and clinical manifestations of Archanobacterium phocae infections in marine mammals stranded along the California coast. J. Wild. Dis. 39, 136–144. doi: 10.7589/0090-3558-39.1.136
Kreuder, C., Miller, M. A., Jessup, D. A., Lowenstein, L. J., Harris, M. D., Ames, J. A., et al. (2003). Patterns of mortality in southern sea otters (Enhydra lutris nereis) from 1998-2001. J. Wildl. Dis. 39, 495–509. doi: 10.7589/0090-3558-39.3.495
Kreuder, C. M., Miller, M. A., Lowenstine, L. J., Conrad, P. A., Carpenter, T. E., Jessup, D. A., et al. (2005). Evaluation of cardiac lesions and risk factors associated with myocarditis and dilated cardiomyopathy in southern sea otters (Enhydra lutris nereis). Am. J. Vet. Res. 66, 289–299. doi: 10.2460/ajvr.2005.66.289
Kudela, R. M., Lane, J. Q., and Cochlan, W. P. (2008). The potential role of anthropogenically derived nitrogen in the growth of harmful algae in California. USA. Harm. Algae 8, 103–110. doi: 10.1016/j.hal.2008.08.019
Kulldorff, M. (1997). Bernoulli, discrete Poisson and continuous Poisson models: a spatial scan statistic. Commun. Stat. Theory Methods 26, 1481–1496.
Kulldorff, M., and Nagarwalla, N. (1995). Spatial disease clusters: detection and inference. Stat. Med. 14, 799–810. doi: 10.1002/sim.4780140809
Laidre, K. L., Estes, J. A., Tinker, M. T., Bodkin, J. L., Monson, D. H., and Schneider, K. B. (2006). Patterns of growth and body condition in sea otters from the Aleutian archipelago before and after the recent population decline. J. Anim. Ecol. 75, 978–989. doi: 10.1111/j.1365-2656.2006.01117.x
Lefebvre, K. A., Quakenbush, L., Frame, E., Burek-Huntington, K., Sheffield, G., Stimmelmayr, R., et al. (2016). Prevalence of algal toxins in Alaskan marine mammals foraging in a changing arctic and subarctic environment. Harm. Algae 55, 13–24. doi: 10.1016/j.hal.2016.01.007
Lowe, C. G., Blasius, M. E., Jarvis, E. T., Mason, T. J., Goodman, G. D., and O’Sullivan, J. B. (2012). “Historic fishery interactions with white sharks in the Southern California Bight,” in Global Perspectives on the Biology and Life History of the White Shark, ed. M. L. Domeier (Boca Raton, FL: CRC Press), 169–186. doi: 10.1201/b11532-17
Mariën, K. (1996). Establishing tolerable dungeness crab (Cancer magister) and razor clam (Siliqua patula) domoic acid contaminant levels. Envir. Health Perspec. 104, 1230–1236. doi: 10.1289/ehp.104-1469507
Mayer, K. A., Dailey, M. D., and Miller, M. A. (2003). Helminth parasites of the southern sea otter Enhydra lutris nereis in central California: abundance, distribution and pathology. Dis. Aq. Org. 53, 77–88. doi: 10.3354/dao053077
Mayer, K. A., Tinker, M. T., Nicholson, T. E., Murray, M. J., Johnson, A. B., Staedler, M., et al. (2019). Surrogate rearing a keystone species to enhance population and ecosystem restoration. Oryx 2019, 1–11. doi: 10.1017/S0030605319000346
McKibben, S. M., Peterson, W., Wood, A. M., Trainer, V. L., Hunter, M., and White, A. E. (2017). Climatic regulation of the neurotoxin domoic acid. PNAS 114, 239–244. doi: 10.1073/pnas.1606798114
Miller, M. A., Byrne, B. A., Jang, S. S., Dodd, E. M., Dorfmeier, E., Harris, M. D., et al. (2010a). Enteric bacterial pathogen detection in southern sea otters (Enhydra lutris nereis) is associated with coastal urbanization and freshwater runoff. Vet. Res. 41, 1–13. doi: 10.1051/vetres/2009049
Miller, M. A., Conrad, P. A., Harris, M., Hatfield, B., Langlois, G., Jessup, D. A., et al. (2010b). A protozoal-associated epizootic impacting marine wildlife: mass-mortality of southern sea otters (Enhydra lutris nereis) due to Sarcocystis neurona infection. Vet. Parasitol. 172, 83–194. doi: 10.1016/j.vetpar.2010.05.019
Miller, M. A., Kudela, R. M., Mekebri, A., Crane, D., Oates, S. C., Tinker, M. T., et al. (2010c). Evidence for a novel marine harmful algal bloom: Cyanotoxin (microcystin) transfer from land to sea otters. PLoS One 5:e12576. doi: 10.1371/journal.pone.0012576
Miller, M. A., Duignan, P. J., Dodd, E., Batac, F., Staedler, M., Tomoleoni, J. A., et al. (2020). Emergence of a zoonotic pathogen in a coastal marine sentinel: Capillaria hepatica (syn. Calodium hepaticum)-associated hepatitis in southern sea otters (Enhydra lutris neries). Front. Mar. Sci. 7:335. doi: 10.3389/fmars.2020.00335
Miller, M. A., Gardner, I. A., Packham, A. E., Mazet, J. K., Hanni, K. D., Jessup, D. A., et al. (2002). Evaluation of an indirect fluorescent antibody test (IFAT) for demonstration of antibodies to Toxoplasma gondii in the sea otter (Enhydra lutris). J. Parasitol. 88, 594–599. doi: 10.2307/3285456
Miller, M. A., Grigg, M. E., Kreuder, C., James, E. R., Melli, A. C., Crosbie, P. R., et al. (2004). An unusual genotype of Toxoplasma gondii is common in California sea otters (Enhydra lutris nereis) and is a cause of mortality. Int. J. Parasitol. 34, 275–284. doi: 10.1016/j.ijpara.2003.12.008
Miller, M. A., Miller, W. A., Conrad, P. A., James, E. R., Melli, A. C., Leutenegger, C. M., et al. (2008). Type X Toxoplasma gondii in a wild mussel and terrestrial carnivores from coastal California: new linkages between terrestrial mammals, runoff and toxoplasmosis of sea otters. Int. J. Parasitol. 38, 1319–1328. doi: 10.1016/j.ijpara.2008.02.005
Miller, M. A., Raverty, S., and Shapiro, K. (2018). “Protozoan parasites of marine mammals,” in CRC Handbook of Marine Mammal Medicine: Health, Disease, and Rehabilitation, 3rd Edn, eds L. Dierauf, F. M. Gulland, and K. I. Whitman (Boca Raton, FL: CRC Press).
Moore, S. K., Trainer, V. L., Mantua, N. L., Parker, M. S., Laws, E. A., Backer, L. C., et al. (2008). Impacts of climate variability and future climate change on harmful algal blooms and human health. Proceedings of the Centers for Oceans and Human Health Investigators Meeting. Envir. Health 7:S4. doi: 10.1186/1476-069X-7-S2-S4
Moxley, J. H., Nicholson, T. E., Van Houtan, K. S., and Jorgensen, S. J. (2019). Non-trophic impacts from white sharks complicate population recovery for sea otters. Ecol. Evol. 9, 6378–6388. doi: 10.1002/ece3.5209
Ng, T., Miller, M. A., Kondova, N. O., Dodd, E., Manzer, M., Ivesa, S., et al. (2015). Oral papillomatosis caused by Enhydra lutris papillomavirus 1 (ElPV-1) in Southern sea otters. J. Wildl. Dis. 51, 446–453. doi: 10.7589/2014-06-152
Nicholson, T. E., Mayer, K. A., Staedler, M. M., Fujii, J. A., Murray, M. A., Johnson, A. B., et al. (2018). Gaps in kelp cover may threaten the recovery of California sea otters. Ecography 41, 1751–1762. doi: 10.1111/ecog.03561
Oates, S. C., Miller, M. A., Byrne, B. A., Chouicha, N., Hardin, D., Jessup, D., et al. (2012). Epidemiology and potential land-sea transfer of enteric bacteria from terrestrial to marine species in the Monterey Bay region of California. J. Wildl. Dis. 48, 654–668. doi: 10.7589/0090-3558-48.3.654
Pesapane, R., Dodd, E., Javeed, N., Miller, M., and Foley, J. (2018). Infestation with the nasopulmonary mite Halarachne halichoeri in threatened southern sea otters (Enhydra lutris nereis). Int. J. Parasitol. 7, 386–390. doi: 10.1016/j.ijppaw.2018.09.009
Pulido, O. M. (2008). Domoic acid toxicologic pathology: a review. Mar. Drugs 6, 180–219. doi: 10.3390/md20080010
QGIS Development Team (2018). QGIS Geographic Information System. Open Source Geospatial Foundation Project. Chicago, IL: QGIS Development Team.
R Core Team, (2016). R: A Language and Environment for Statistical Computing. Vienna: R Foundation for Statistical Computing.
Reisewitz, S. E., Estes, J. A., and Simenstad, C. A. (2006). Indirect food web interactions: sea otters and kelp forest fishes in the Aleutian archipelago. Oecologia 146, 623–631. doi: 10.1007/s00442-005-0230-1
Rejmanek, D., Miller, M. A., Grigg, M. E., Crosbie, P. R., and Conrad, P. A. (2010). Molecular characterization of Sarcocystis neurona strains from opossums (Didelphis virginiana) and intermediate hosts from Central California. Vet. Parasitol. 170, 20–29. doi: 10.1016/j.vetpar.2009.12.045
Scholin, C. A., Gulland, F., Doucette, G. J., Benson, S., Busman, M., Chavez, F. P., et al. (2000). Mortality of sea lions along the central California coast linked to a toxic diatom bloom. Nature 403, 80–84. doi: 10.1038/47481
Shapiro, K., Miller, M. A., Aguilar, B., Packham, A., Conrad, P., VanWormer, E., et al. (2016). Dual congenital transmission of Toxoplasma gondii and Sarcocystis neurona in a late-term aborted pup from a chronically infected southern sea otter (Enhydra lutris nereis). J. Parasitol. 43, 276–288. doi: 10.1017/S0031182015001377
Shapiro, K., Miller, M. A., and Mazet, J. K. (2012). Temporal association between land-based runoff events and California sea otter (Enhydra lutris nereis) protozoal mortalities. J. Wildl. Dis. 48, 394–404. doi: 10.7589/0090-3558-48.2.394
Shapiro, K., VanWormer, E., Packham, A., Aguilar, B., Dodd, E., Conrad, P. A., et al. (2019). Type X strains of Toxoplasma gondii are virulent for southern sea otters (Enhydra lutris nereis) and present in felids from nearby watersheds. P. Roy. Soc. B. 286, 1–10. doi: 10.1098/rspb.2019.1334
Shockling-Dent, C. E., Miller, M. A., Batac, F. I., Dodd, E., Smith, W., Pesapane, R., et al. (2019). Pathology and epidemiology of nasopulmonary acariasis (Halarachne sp.) in southern sea otters (Enhydra lutris nereis). IJP 9, 60–67. doi: 10.1016/j.ijppaw.2019.03.009
Silvagni, P. A., Lowenstine, L. J., Spraker, T., Lipscomb, T. P., and Gulland, F. M. D. (2005). Pathology of domoic acid toxicity in California sea lions (Zalophus californianus). Vet. Pathol. 42, 184–191. doi: 10.1354/vp.42-2-184
Siqueira, J. D., Ng, T., Miller, M., Li, L., Deng, X., Dodd, E., et al. (2017). Endemic infection of stranded southern sea otters (Enhydra lutris nereis) with novel parvovirus, polyomavirus, and adenovirus. J. Wildl. Dis. 53, 532–542. doi: 10.7589/2016-04-082
Smith, L. I. (2002). A tutorial on principal components analysis. Technical Report OUCS-2002-12. Dunedin: University of Otago.
Staedler, M., and Riedman, M. (1993). Fatal mating injuries in female sea otters (Enhydra lutris nereis). Mammalia 57, 135–139.
Stavely, C. S., Register, K. B., Miller, M. A., Brockmeier, S. L., Jessup, D., and Jang, S. (2003). Molecular and antigenic characterization of Bordetella bronchiseptica isolated from a wild southern sea otter (Enhydra lutris nereis) with suppurative bronchopneumonia. J. Vet. Diag. Invest. 15, 570–574. doi: 10.1177/104063870301500610
Thomas, N. J., and Cole, R. A. (1996). The risk of disease and threats to the wild population. Endanger. Species Update 13, 23–27.
Thomas, N. J., Dubey, J. P., Lindsay, D. S., Cole, R. A., and Meteyer, C. U. (2007). Protozoal meningoencephalitis in sea otters (Enhydra lutris): a histopathological and immunohistochemical study of naturally occurring cases. J. Comp. Pathol. 137, 102–121. doi: 10.1016/j.jcpa.2007.05.001
Thometz, N. M., Tinker, M. T., Staedler, M. M., Mayer, K. A., and Williams, T. M. (2014). Energetic demands of immature sea otters from birth to weaning: implications for maternal costs, reproductive behavior and population-level trends. J. Exper. Biol. 217, 2053–2061. doi: 10.1242/jeb.099739
Tinker, M. T., Bentall, G., and Estes, J. A. (2008). Food limitation leads to behavioral diversification and dietary specialization in sea otters. Proc. Natl. Acad. Sci. U.S.A. 105, 560–565. doi: 10.1073/pnas.0709263105
Tinker, M. T., Doak, D. F., Estes, J. A., Hatfield, B. B., Staedler, M. M., and Bodkin, J. L. (2006). Incorporating diverse data and realistic complexity into demographic estimation procedures for sea otters. Ecol. Appl. 16, 2293–2312. 10.1890/1051-0761(2006)016%5B2293:iddarc%5D2.0.co;2
Tinker, M. T., and Hatfield, B. B. (2017). California sea otter (Enhydra lutris nereis) census results, spring 2017: US Geological Survey Data Series 1067. Reston, VI: U.S. Geological Survey, doi: 10.3133/ds1067
Tinker, M. T., Hatfield, B. B., Harris, M. D., and Ames, J. A. (2016). Dramatic increase in sea otter mortality from white sharks in California. Mar. Mamm. Sci. 32, 309–326. doi: 10.1111/mms.12261
Tinker, M. T., Tomoleoni, J., LaRoche, N., Bowen, L., Miles, A. K., Murray, M., et al. (2017). Southern Sea Otter Range Expansion and Habitat Use in the Santa Barbara Channel. California. US Geological Survey Open File Report 201, Reston, VI: U.S. Geological Survey, doi: 10.3133/ofr20171001
Tinker, M. T., Tomoleoni, J. A., Weitzman, B. P., Staedler, M., Jessup, D., Murray, M. J., et al. (2019). Southern sea otter (Enhydra lutris nereis) population biology at Big Sur and Monterey, California: Investigating the Consequences of Resource Abundance and Anthropogenic Stressors for Sea Otter Recovery. U.S. Geological Survey Open File Report 2019-1022, Reston, VI: U.S. Geological Survey, doi: 10.3133/ofr20191022
Torr, E. R., Puschner, B., and Whitehead, W. (2003). Rapid Determination of Domoic Acid in Serum and Urine by Liquid Chromatography-Electrospray Tandem Mass Spectrometry. J. Agric. Food Chem. 51, 1791–1796. doi: 10.1021/jf020947f
Trainer, V. L., Cochlan, W. P., Erickson, A., Bill, B. D., Cox, F. H., Borchert, J. A., et al. (2007). Recent domoic acid closures of shellfish harvest areas in Washington State inland waterways. Harm. Algae 6, 449–459. doi: 10.1016/j.hal.2006.12.001
Tuomi, P. A., Murray, M. J., Garner, M. M., Goertz, C. E., Nordhausen, R. W., Burek-Huntington, K. A., et al. (2014). Novel poxvirus infection in northern and southern sea otters (Enhydra lutris kenyoni and Enhydra lutris nereis), Alaska and California, USA. J. Wildl. Dis. 50, 607–615. doi: 10.7589/2013-08-217
USFWS (2015). Southern sea otter (Enhydra lutris nereis)-5 year review: Summary and evaluation. California: U.S. Fish and Wildlife Service Ventura Fish and Wildlife Office Ventura.
Wand, M. P., and Jones, M. C. (1995). Monographs on Statistics and Applied Probability. Kernel smoothing. London: Chapman and Hall/CRC.
White, L., Lankau, E. W., Lynch, D., Knowles, S., Schuler, K. L., Dubey, J. P., et al. (2018). Mortality trends in northern sea otters (Enhydra lutris kenyoni) collected from the coasts of Washington and Oregon (2002–15). J. Wildl. Dis. 54, 238–247. doi: 10.7589/2017-05-122
Wickham, H. (2016). Programming with ggplot2. In ggplot2. Cham: Springer, 241–253. doi: 10.1007/978-3-319-24277-4_12
Yeates, L. C., Williams, T. M., and Fink, T. L. (2007). Diving and foraging energetics of the smallest marine mammal, the sea otter (Enhydra lutris). J. Exper. Biol. 210, 1960–1970. doi: 10.1242/jeb.02767
Keywords: cardiomyopathy, domoic acid, infectious disease, mortality, pathology, risk factors, southern sea otter, white shark bite
Citation: Miller MA, Moriarty ME, Henkel L, Tinker MT, Burgess TL, Batac FI, Dodd E, Young C, Harris MD, Jessup DA, Ames J, Conrad PA, Packham AE and Johnson CK (2020) Predators, Disease, and Environmental Change in the Nearshore Ecosystem: Mortality in Southern Sea Otters (Enhydra lutris nereis) From 1998–2012. Front. Mar. Sci. 7:582. doi: 10.3389/fmars.2020.00582
Received: 16 April 2020; Accepted: 23 June 2020;
Published: 19 November 2020.
Edited by:
Alvin Cyril Camus, University of Georgia, United StatesReviewed by:
Rebecca Ruth McIntosh, Phillip Island Nature Parks, AustraliaCopyright © 2020 Miller, Moriarty, Henkel, Tinker, Burgess, Batac, Dodd, Young, Harris, Jessup, Ames, Conrad, Packham and Johnson. This is an open-access article distributed under the terms of the Creative Commons Attribution License (CC BY). The use, distribution or reproduction in other forums is permitted, provided the original author(s) and the copyright owner(s) are credited and that the original publication in this journal is cited, in accordance with accepted academic practice. No use, distribution or reproduction is permitted which does not comply with these terms.
*Correspondence: Melissa A. Miller, bWVsaXNzYS5taWxsZXJAd2lsZGxpZmUuY2EuZ292
Disclaimer: All claims expressed in this article are solely those of the authors and do not necessarily represent those of their affiliated organizations, or those of the publisher, the editors and the reviewers. Any product that may be evaluated in this article or claim that may be made by its manufacturer is not guaranteed or endorsed by the publisher.
Research integrity at Frontiers
Learn more about the work of our research integrity team to safeguard the quality of each article we publish.