- 1Centre for Marine Science and Innovation & Evolution and Ecology Research Centre, School of Biological, Earth and Environmental Sciences, The University of New South Wales, Sydney, NSW, Australia
- 2The University of Sydney, School of Life and Environmental Sciences, Coastal and Marine Ecosystems, Sydney, NSW, Australia
- 3Singapore Centre for Environmental Life Sciences Engineering, Nanyang Technological University, Singapore, Singapore
- 4Sydney Institute of Marine Science, Mosman, NSW, Australia
- 5Institute for Marine and Antarctic Studies, College of Sciences and Engineering, University of Tasmania, Hobart, TAS, Australia
- 6Nicholas School of the Environment, Duke University, Beaufort, NC, United States
Kelp forests are highly productive foundation species along much of the world’s coastline. As a result, kelp are crucial to the ecological, social, and economic well-being of coastal communities. Yet, due to a combination of acute and chronic stressors, kelp forests are under threat and have declined in many locations worldwide. Active restoration of kelp ecosystems is an emerging field that aims to reverse these declines by mitigating negative stressors and then, if needed, introducing biotic material into the environment. To date, few restoration efforts have incorporated positive species interactions. This gap presents a potential shortcoming for the field as evidence from other marine ecosystems illustrates that the inclusion of positive species interactions can enhance restoration success. Additionally, as the climate continues to warm, this approach will be particularly pertinent as positive interactions can also expand the range of physical conditions under which species can persist. Here, we highlight how practitioners can use positive density dependence within and amongst kelp species to increase the chances of restoration success. At higher trophic levels, we emphasize how co-restoring predators can prime ecosystems for restoration. We also investigate how emerging technologies in genetic and microbial selection and manipulation can increase the tolerance of target species to warming and other stressors. Finally, we provide examples of how we can use existing anthropogenic activities to facilitate restoration while performing alternative purposes. As kelp forests continue to decline and the field of kelp restoration continues to develop, it is also important that we monitor these potential advancements and ensure they do not have unintended ecosystem effects, particularly with untested techniques such as genetic and microbial manipulations. Nevertheless, incorporating positive species interactions into future restoration practice stands to promote a more holistic form of restoration that also increases the likelihood of success in a shifting seascape.
Introduction
Significance, Threats, and Declines of Kelp Forests
Kelp, defined here in the broad sense as large brown seaweeds from the orders Laminariales, Fucales, Desmarestiales (Wernberg and Filbee-Dexter, 2019), are habitat-forming marine macroalgae that form the basis for some of the most productive ecosystems in the world’s sub-tropical, temperate and polar seas (Dayton, 1975; Coleman and Wernberg, 2017; Smale et al., 2019; Wernberg et al., 2019). These habitat formers provide a complex three-dimensional habitat (Miller et al., 2018; Layton et al., 2019) that support other macroalgal species (Melville and Connell, 2001; Wernberg et al., 2005), fish, and invertebrates many of which are commercially valuable, e.g., abalone, and lobsters (Graham et al., 2007; Marzinelli et al., 2014; Teagle et al., 2017; Olson et al., 2019). Kelp is also a valuable food source, both through the production of live tissue and of detritus that is often exported to other ecosystems (Dayton, 1985; Bustamante et al., 1995; Bishop et al., 2010). Exportation of carbon into deep sea sediments, combined with their high productivity means kelp can act as a valuable carbon sink (Chung et al., 2013; Filbee-Dexter et al., 2018; Queirós et al., 2019). Other ecosystem services include wave attenuation and reductions in coastal erosion, services that are increasingly important under climate-mediated sea level rise and increases in extreme storm events (Smale et al., 2013). Many kelp species are also part of a wild or farmed harvest economy (Vásquez et al., 2014), are efficient nutrient cyclers (Graham et al., 2007), and provide recreational and cultural value (Smale et al., 2013). Based on these services, kelp ecosystems are currently valued at ∼1 million USD per kilometer of coast per year, though these values are considered underestimates (Wernberg et al., 2019).
Given the great ecological and economic importance of kelp forests, there is growing concern about their disappearance from the world’s oceans. Krumhansl et al. (2016) found that laminarian populations in 38% of studied ecoregions had declined over several decades. Compounding the global average decline, several regions have experienced range contractions and near total losses of their kelp populations in the last 5–10 years (Bennett et al., 2015; Ling and Keane, 2018; Rogers-Bennett and Catton, 2019). These dramatic losses of kelp have already led to severe socioeconomic consequences and resulted in declines, closures or limitations of major fisheries, such as abalone fisheries in eastern Japan and California (Kiyomoto et al., 2013; Rogers-Bennett and Catton, 2019) and rock lobster fisheries in Australia (Hinojosa et al., 2014). An analogous synthesis does not exist for fucoid species, but there have also been notable local declines of Phyllospora, Fucus, Sargassum, and Cystoseira species throughout the world (Thibaut et al., 2005; Coleman and Wernberg, 2017). Natural recovery is not common and is not anticipated at a significant scale (Wernberg et al., 2019; Layton et al., 2020) so without intervention, the loss of kelp and their associated services will likely continue (Smale et al., 2019).
The causes of kelp forest decline and disappearance are complex and range from local, often mitigatable impacts, to global, irreversible changes over the course of decades (Smale et al., 2013; Schiel and Foster, 2015; Wernberg et al., 2019). At local scales, nutrient and contaminant inputs from untreated sewage and agricultural runoff can distribute toxic materials (Burridge et al., 1996; Coleman et al., 2008), increase abundances of competitors (Connell et al., 2008), and cause high turbidity that can prevent kelp from photosynthesizing (Reed and Brzezinski, 2009; Tait, 2019). Construction can also physically remove kelp from the seafloor (Cheney et al., 1994). Local biotic stressors can also play an important role in reducing kelp forest distributions. Overgrazing by herbivores has resulted in the marked decline of kelp forests in many locations around the globe (Filbee-Dexter and Scheibling, 2014; Ling et al., 2015). The main actor, sea urchins, are a natural part of the kelp ecosystem, but their populations can increase in numbers when, for example, their predators (e.g., otters, fishes, lobsters) disappear from an ecosystem (Shurin et al., 2010), or when altered environmental conditions, such as warmer temperatures result range expansion to a new location (Ling et al., 2009). Furthermore, warm water herbivorous fishes have expanded their ranges in many parts of the world in response to ocean warming, causing declines in kelp populations (Vergés et al., 2014, 2019). Climate change poses a major threat to kelp forests, as most kelp are cool water species, and warming temperatures can push them beyond their physiological limit and either kill juvenile or adult plants or prevent further recruitment by killing the spores (Smale et al., 2019).
Ocean warming and other climate-related stressors cannot be mitigated over short time scales and may cause a re-evaluation of which populations are manageable under changing conditions (Coleman and Goold, 2019). For example, along the warm edge of the distribution of many species, management of kelp forests may entail supporting the expansion of naturally warm-adapted genotypes or even alien species. Alternately, management could work to expand the niche of native species, through more interventionist approaches such as introduction of new genotypes via assisted evolution (Coleman and Goold, 2019; Wood et al., 2019) or the introduction of species which are involved in positive species interactions.
Traditional Management Interventions in Kelp Forests
Kelp conservation has a long history and managers across the world have been working to conserve kelp forests since the 1800s (Fujita, 2011), mostly focusing on eliminating the causes of kelp decline such as kelp overharvesting (Buschmann et al., 2014) or water pollution (Coleman et al., 2008). Overharvesting can be a straightforward fix in systems that contain wild harvest industries (e.g., Chile, France, Japan), and appropriate management that regulates kelp extraction can allow for populations to return (Fujita, 2011; Buschmann et al., 2014; Frangoudes and Garineaud, 2015). Enhancing the water quality in an area can also slow kelp loss or sometimes allow it to return (Hawkins et al., 1999). While kelp restoration is not usually a focal motivation for implementing marine protected areas (MPAs) (Woodcock et al., 2017), MPA restrictions may limit the harvest of certain marine predators that can help control herbivore population and thus their installation may promote the resilience of kelp ecosystems (Ferrari et al., 2018). Only some MPAs and harvest restrictions have had success in restoring kelp populations, particularly where food webs are less complicated and there is no nutrient limitation or other stressors present, whereby increases in the populations of urchin predators such as sea otters or lobsters have had a positive cascading impact on kelp (Shears and Babcock, 2002; Watson and Estes, 2011; Caselle et al., 2018; Eger and Baum, 2020). Where kelp does not re-establish following such interventions (Barrett et al., 2009; Campbell et al., 2014a), restoration should be considered.
Restoration of Kelp Forests
Many attempts at preventing further losses of kelp have failed, accelerating the interest in both different forms of kelp forest restoration (Eger et al., 2020a). Successful kelp restoration projects are rare and costs have been high (Bayraktarov et al., 2016; Eger et al., 2020a; Layton et al., 2020). The majority of the work conducted thus far is at spatial scales of less than one hectare and over durations of less than 2 years, and the costs have often exceeded hundreds of thousands of US dollars per hectare (Eger et al., 2020a). Despite these limitations, there is an emerging interest in large scale kelp restoration from universities, non-governmental organizations, governments, and industries. Active efforts to restore kelp forests include the addition of kelp transplants, seeds, or habitat (via artificial reefs) to the marine environment (Basconi et al., 2020), but can also involve the removal of kelp consumers such as urchins and fishes (Terawaki et al., 2001; Tracey et al., 2015; Layton et al., 2020). The main techniques used in these early kelp restoration efforts have been a combination of passive restoration via first eliminating threats and active efforts that focus on supplementary activities such as transplanting (Wilson and North, 1983; Campbell et al., 2014a; Verdura et al., 2018). While these techniques will remain relevant, it is important to consider what further elements might enhance the chances of success and lower the costs of kelp forest restoration, which can be significant (Eger et al., 2020b).
Positive Species Interactions, Stress, and Kelp Forests
One promising method to complement previous ecosystem restoration methods is to incorporate positive species interactions and other synergies into the process. Positive species interactions occur between organisms where at least one individual benefits and the other individual is not harmed (e.g., mutualism, commensalism, facilitation, Bruno et al., 2003) and are increasing documented across almost all marine ecosystems (Thomsen et al., 2018; Gribben et al., 2019). There is now evidence from coastal marine ecosystems (coral reefs, salt marshes, mangroves, seagrasses) that positive interactions can work to enhance restoration success and reduce costs (Silliman et al., 2015; Shaver and Silliman, 2017; Renzi et al., 2019; Valdez et al., 2020). For example, Silliman et al. (2015) showed that enhancing positive interactions, and not suppressing competition was key to coastal wetland recovery. Not only did their facilitation focused planting orientation increase growth by 1–200% but it also reduced oxygen stress and increased resistance to wave erosion. Other examples are found in mangroves whereby clumping saplings also reduces oxygen stress (Gedan and Silliman, 2009). There are also some examples of facilitation between species, as ascidians and sponges growing on mangrove roots, can protect mangroves from isopod grazing (Ellison and Farnsworth, 1990).
According to the Stress Gradient Hypothesis, the frequency of positive interactions should increase with greater levels of stress (Bertness and Callaway, 1994). Positive interactions may thus become more important in the future as conditions become more stressful due to multiple, interactive abiotic (physical disturbance, temperature) and biological (e.g., predation) stressors (He et al., 2013; Wright and Gribben, 2017; Uyà et al., 2019). In particular, positive interactions can influence the physical conditions under which species persist, and thus have the potential to mitigate the effects of warming, drought or acidification on the distribution of species (Silliman et al., 2011; Angelini et al., 2016; Bulleri et al., 2016, 2018). For example, positive species interactions can help biogenic habitats such as salt marsh survive acute abiotic stresses such as drought (He et al., 2017) and might increase the thermal tolerance of some species such as corals to otherwise lethal warming events (Shaver et al., 2018). In intertidal systems, canopies of the fucoid Ascophyllum nodosum can reduce maximum summer rock temperatures in New England by up to 8°C (Leonard, 2000). The presence of such canopies also influences biotic processes and interactions of key grazers in the system (Marzinelli et al., 2012), which in turn can affect kelp recruitment (Hawkins and Hartnoll, 1983). Recognizing and encouraging these interactions may aid in successful restoration of kelp forest ecosystems, especially as ecosystems become more stressed and variable. While these interactions are not regularly considered in a kelp restoration context, there are some well-known positive interactions from ecological literature on kelp forests that may aid restoration efforts.
Interest in kelp restoration is increasing and it is important that managers consider the best available options for developing successful and cost-effective restoration. Incorporating positive species interactions into kelp restoration could help kelp recovery, but also accelerate the re-establishment of associated biodiversity (Angelini et al., 2016) and ecological processes (Thomsen et al., 2018). Given that kelp restoration is an emergent and fast-growing field, the opportunity exists to incorporate positive interactions into the development of management interventions and improve the likelihood of success of future efforts and their cost-effectiveness. The aim of this paper is to catalog known and potential positive interactions in kelp forests and provide context about how future kelp restoration efforts can use these interactions. Our work uses a combination of a structured literature review and expert knowledge to identify several different positive interactions under current and future conditions. These are: (1) facilitation between primary producers; (2) indirect trophic effects; (3) genotypic and microbial interactions; and (4) anthropogenic synergies. For each interaction, we review the existing knowledge for kelp forests and provide advice on how current and future restoration efforts can apply these.
Methods
We first conducted a literature search using SCOPUS on July 12th, 2019, with the following search terms:
kelp∗ OR seaweed∗ OR macroalga∗ OR Laminariales OR Fucales OR Desmarestiales
AND
species interact∗ OR biotic OR connect∗ OR link∗
AND
positiv∗ OR benefic∗ OR facilitat∗ OR density dependen∗ OR mutalis∗ OR synerg∗ OR c
ommensal∗ OR cascad∗
The search returned 156 results. We then conducted a preliminary assessment for suitable papers that might (1) involve a species of seaweed from the order Fucales or Laminariales or Desmarestiales and (2) involve positive interactions (e.g., mutualism, synergism, commensalism). This process refined the initial search results down to 92 possible papers (Figure 1). We then read these papers to ensure they met the same two criteria, and if so, classified the positive interaction detailed in each paper to create a table of all identified positive interactions (Supplementary Table S1). We then created a final list of 14 interactions by combining the returned topics with suggestions from the authors (Supplementary Table S1). Each author then identified which six interactions they thought were most relevant to include. We created a final list of topics by selecting the interactions that had three or more votes; this process resulted in a final list of nine interactions (Supplementary Table S2). We removed the topics on facilitation cascades and settlement because insufficient material exists for kelp and we incorporated the topic “hypothesized interactions from other ecosystems” into the main text.
Synergies in Kelp Forest Restoration
Intraspecific Facilitation – Figure 2-1
There is strong evidence for positive density dependencies in kelp forests, with numerous studies showing that kelp populations have density thresholds that alter the environment and support future generations (Dayton, 1985; Harrold and Reed, 1985; Schiel, 1985; Pearson and Brawley, 1996; Anderson et al., 1997). Indeed, the slow recovery of kelp after large-scale losses (Kirkman, 1981; Toohey et al., 2007; Connell et al., 2008) is often attributed to the breakdown of these positive ‘environment-engineer feedbacks’ (Cuddington et al., 2009; Jones et al., 2010). Likewise, a failure to re-establish this intraspecific facilitation may explain the limited success of some previous kelp restoration efforts (Layton et al., 2019, 2020).
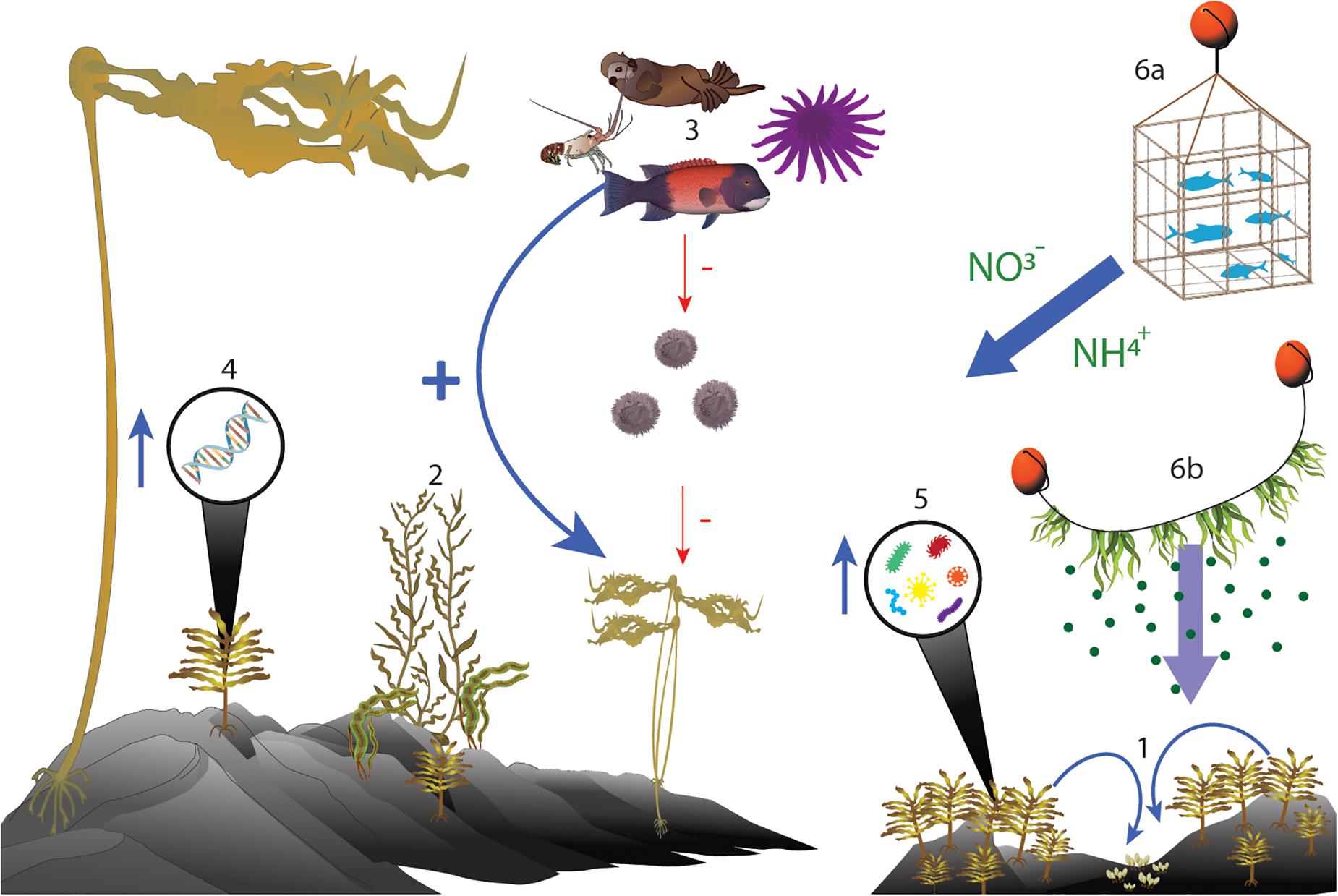
Figure 2. Ecosystem diagram of different positive interactions that may be used in kelp forest restoration. 1: Intraspecific facilitation. 2: Interspecific foundation species facilitation. 3: Trophic cascades. 4: Genetic manipulation. 5: Manipulation of microbial communities. 6a: Fish farms and nutrient cycling. 6b: Kelp farms and spore supply.
One pathway by which this feedback manifests is via the supply and dispersal of reproductive propagules in the environment. In general kelp species, across all three orders considered here, are poor dispersers and only have single generation dispersal ranges of 0.1–10 km (Chan et al., 2013; Schiel and Foster, 2015; Luttikhuizen et al., 2018). Additionally, populations need very high densities of adults to supply propagules to future generations (Dayton, 1985), which, in turn, can enhance fertilization (Pearson and Brawley, 1996). As a result, the lack of a local adult populations limits the unassisted range expansion of a single population as well as the regular long-distance dispersal of buoyant species such as Sargassum. Without adequate propagule supply to enhance recruitment success, the survival of those offspring is thus likely limited (Schiel and Foster, 2006).
The modification of the local physical and chemical environment by the adult kelp canopy can also facilitate the survival and development of juvenile conspecifics within the sub-canopy (Schiel and Foster, 2006; Layton et al., 2019). Degraded kelp canopies (e.g., reduced patch sizes or densities) lower the ability of the canopy to engineer the sub-canopy environment and can cause a reduction or break down of the positive feedback processes (Layton et al., 2019). In turn, this loss can lead to disruption and even collapse of the demographic processes of micro- and macroscopic juvenile kelp and can result in a total loss of habitat stability and resilience.
The importance of intraspecific facilitation, especially for juvenile kelp, might be greater in more stressful environments (Bertness and Callaway, 1994). At local scales, for instance, the importance of facilitation may relate to depth gradients in light, ice scour, or wave exposure (Kitching, 1941; Wood, 1987; Chapman and Johnson, 1990). At larger scales, gradients of abiotic stress across latitudinal gradients, due to changes in water temperature and irradiance, may be more important (Wernberg et al., 2011). Ultimately, the presence of adult kelp in stressful conditions can expand the realized niche of juvenile conspecifics beyond their fundamental niche, thus allowing juveniles to thrive in areas where they would otherwise perish in isolation (Bruno et al., 2003; Layton et al., 2019). This is likely to become more important in the future given projections suggest that the marine environment will become more stressful (Frölicher et al., 2018; Smale et al., 2019).
As we continue to improve and refine active restoration interventions, there are several ways to better harness and re-establish the internal processes that promote the stability of kelp forests. Given the importance of intra-specific facilitation for kelp patch expansion and dispersal (Schiel and Foster, 2006), future restoration attempts might be most successful when they occur nearby intact kelp forests, thus ensuring there is an adequate supply and exchange of propagules between neighboring populations. If new patches are being created, it would be prudent to orient them such that there is connectivity with nearby forests as to enhance the contributions of local propagule supply. Effective dispersal distances vary amongst species, with distances less than 1–2 km in genera such as Saccharina, Alaria, Ecklonia, Sargassum, and Undaria (Norton, 1992; Forrest et al., 2000; Serisawa et al., 2005; Chan et al., 2013; Akino et al., 2015; Luttikhuizen et al., 2018) but up to 10 km in Macrocystis (Reed et al., 2006). Smaller distances between populations may further enhance the likelihood of propagule exchange and restoration success.
Existing kelp canopies can be used in several different ways to facilitate restoration projects. For instance, managers can transplant kelp individuals or propagules to enhance existing but declining kelp populations and help re-establish positive density-dependent processes before they disappear. If successful, this approach avoids a phase shift to a barren or turf-dominated state, after which it may be more difficult to restore (Gorman and Connell, 2009; Johnson et al., 2017; Filbee-Dexter and Wernberg, 2018), and aids dispersal. In restoration attempts using propagules or juveniles, it may also be helpful to transplant (or outplant cultured) adult individuals to help prime the environment for the new recruits, though this step adds additional upfront cost. However, if it leads to increased survival, it can be more cost effective in the long term. Indeed, it seems for some species and locations that juvenile kelp do not recruit nor survive (if transplanted) in the absence of adult conspecifics (Layton et al., 2019). It is unlikely that enhanced intraspecific facilitation alone will be enough to help vulnerable populations survive water temperatures above their physiological threshold and these approaches may need to be applied along with the genetic techniques described in section “Genetics in Kelp Restoration – Figure 2-4.”
Interspecific Facilitation – Figure 2-2
Facilitation cascades, whereby a habitat-forming species promotes a secondary habitat-former with positive effects on associated biodiversity, occur in almost all marine ecosystems (Thomsen et al., 2018; Gribben et al., 2019). Most studies on facilitation cascades have focused on synergistic effects of positive interactions among habitat-forming species on the overall biodiversity they support. In contrast, few studies have explored how interactions between different habitat-forming species influence their own performance (Bulleri, 2009; Gribben et al., 2019), despite such positive interactions being potentially critical for restoring or increasing the resilience of kelp forests.
For instance, in the absence of established kelp beds to facilitate recruitment, other habitat-forming species may be critical recruitment habitats that reduce abiotic (e.g., wave action) or biotic (e.g., herbivory) stress (Bulleri et al., 2011). As an example, recruitment of the habitat-forming fucoid Scytothalia dorycarpa is facilitated by the canopy of the kelp Ecklonia radiata (Bennett and Wernberg, 2014). Interestingly, a similar positive effect is found on recruits of the fucoid Sargassum spp., but only under partial Ecklonia canopies, whereas dense canopies had a negative effect on recruitment of Sargassum (Bennett and Wernberg, 2014). This result suggests that we need to better understand the context and species specifics of positive interactions between habitat-forming kelp before they can be incorporated in management interventions to avoid undesired outcomes.
Experimental tests with artificial kelp blades show that the motion or “whiplash” from frond movement can help deter urchin grazing and facilitate the growth of juveniles (Vasquez and McPeak, 1998). Though this example used artificial blades, the presence of other kelp species nearby could play a similar role, but further testing is required to determine the efficacy. Some kelp species may be better at deterring grazing through such mechanisms and thus outplanting adults of these species alongside focal restoration species or transplanting the focal species near to extant canopies of the grazing-deterrent species, could enhance effective restoration. Working to transplant multiple species requires additional considerations as they may have different environmental requirements and thus necessitate separate cultivation chambers or storage units; however, the additional investment could prove worthwhile if success rates are increased.
There is also some evidence that habitat-forming species can facilitate other spatially distant habitat-formers, that is, facilitation often occurs at larger, seascape scales (Gribben et al., 2019). For example, in soft-sediment environments, beds of mussels can promote the high abundances of other bivalves by altering hydrodynamic regimes at distances of 100s of meters away from the mussel beds (Donadi et al., 2013), and clams provide a hard substratum for fucoid settlement thus extending their distribution from their natal habitat (rocky shore) to a connected novel habitat (Lanham et al., 2020). Therefore, kelp restoration may only succeed if the habitat is made more amenable for growth by the presence of another habitat-forming species (e.g., another kelp species) in the local seascape. It is predicted that these types of interactions will have larger positive seascape-scale effects on habitat-forming species and may thus provide the biggest benefits in ecosystem services and function, but for kelp forests such effects remain unknown. Pragmatically, reinstalling these types of interactions may be more difficult than utilizing other habitat-formers to facilitate restoration of a focal kelp species at smaller scales.
Harnessing positive interspecies interactions has the potential to aid kelp restoration efforts. But before managers can achieve this goal, we require a better understanding of how other species enhance kelp populations, under what conditions do positive interactions perform best. Notably, the target kelp or the facilitative species may eventually experience negative feedbacks as the relationship maybe positive at one life stage or given density and negative at a differing life stage or density (Gribben et al., 2019).
Trophic Cascades – Figure 2-3
Trophic cascades where predators impact the health of foundation species are well documented across many marine ecosystems and often positively affect foundation species (Eger and Baum, 2020). Tri-trophic cascades in which predators promote foundation species by suppressing populations of their grazers are powerful examples and include blue crabs and fish protecting salt marsh plants (Silliman and Bertness, 2002; Altieri et al., 2012) and sharks promoting seagrass growth by deterring turtle grazing (Burkholder et al., 2013). Trophic cascades are particularly relevant in the context of kelp restoration as the loss of predators such as sea otters (Estes and Duggins, 1995), sea stars (Burt et al., 2018), lobsters (Ling et al., 2009), and predatory fish (Caselle et al., 2018), and later expansion of consumers such as sea urchins, is often linked to the initial loss of the kelp habitat. Therefore, controlling herbivore populations and re-establishing predator populations, along with the kelp, may not only be an additional step to increase the success of kelp restoration but a requisite step, without which long term restoration success may never be possible.
Two interventions that have been successful in elevating predator populations are the establishment of strict harvest limits on predators and the creation of MPAs. For example, installing limits on predator harvest has resulted in large scale returns of kelp habitat in Alaska (Estes and Duggins, 1995), California (Caselle et al., 2018), British Columbia (Watson and Estes, 2011), and New Zealand (Shears and Babcock, 2002). MPAs are a common marine management tool to help restore animal populations (Boonzaier and Pauly, 2016). Since both fisheries limits and MPAs are gaining momentum, used in governmental policy (Watson et al., 2014), and are often politically viable (Jones et al., 2013), these two methods have great promise as key mechanisms to help kelp recovery. To date, however, management of kelp through the management of predators has tended to stop at the predator level and have not typically considered kelp populations in the marine planning process (Woodcock et al., 2017). As a result, future MPA designs should consider how their placement can also suit the restoration of primary producers, instead of solely focusing on high trophic levels. For example, restoration efforts can occur within MPAs or managers can situate new MPAs to ensure population connectivity among kelp populations (Coleman et al., 2017). Through these planning adjustments, restoration efforts could also benefit from the increased predator populations.
Often, the restriction or elimination of a harvest pressure is not enough to allow for the return of predators, and in turn, kelp. For example, after the end of the fur trade, and following legal protection as an endangered species, sea otters (Enhydra lutris) failed to return to parts of their previous range. To resolve this problem, managers translocated otters and reintroduced them into parts of the United States and Canada (Bodkin, 2015). Though these efforts were costly, difficult, and resulted in significant otter mortality (VanBlaricom et al., 2015), they have been successful at restoring kelp beds at large scales and maintaining those restored populations (Filbee-Dexter and Scheibling, 2014). To date, no captive breeding program exists for restoration purposes (VanBlaricom et al., 2015) and if otters require introduction, scientists instead advocate for additional otter translocations to help connect the populations and restore kelp ecosystems (Davis et al., 2019). Despite their success, translocating otters or other predators (Hayward and Somers, 2009), can be contentious because they are very likely to interact with humans, eat recreationally and commercially harvested species, and opportunities for development can disappear because of their endangered status and legal protection (Booth, 1988). Additionally, otters can sometimes avoid using urchin barrens as feeding grounds because urchin barrens contain nutritionally poor urchins, and instead hunt in nearby kelp forests, which defeats the purpose of their reintroduction (Hohman et al., 2019). Thus, introduced otters may be most effective at maintaining kelp forests rather than promoting their recovery. As a result, managers are currently hesitant to introduce more otter populations in the Eastern Pacific (Hohman et al., 2019). Potentially, the restoration of a diversity of predators may be needed to control herbivore populations (Katano et al., 2015) and other species could be introduced alongside or in place of otters.
Artificial stock enhancements of marine fishes and invertebrates, often for harvest, have been successful in augmenting the wild populations of many species worldwide (Bell et al., 2008; Lorenzen et al., 2010). As a result, programs focused on other species that consume urchins may prove to be a more cost-effective and politically tenable alternative or supplement to sea otter introduction. In areas such as Tasmania, Australia, where overharvest of the Southern Rock Lobster (Jasus edwardsii) has contributed to increases in urchin populations and declines in canopy-forming algae (Ling et al., 2009), managers could release cultured J. edwardsii into the environment. Although lobsters are unlikely to revert declines (Layton C, Johnson C, personal communication), they can complement other restorative actions and aid in conserving extant kelp forests. While J. edwardsii is not currently used to restore kelp populations, researchers are successfully culturing the species (Hooker et al., 1997; Ritar, 2001; Kittaka et al., 2005) and managers could redirect this practice to a restoration focus. Similar species such as the Eastern rock lobster (Sagmariasus verreauxi), a key predator of Centrostephanus rodgersii, are also cultivable (Jensen et al., 2013) and are candidates for wild enhancement programs.
Other species which are not as developed from an aquaculture standpoint, but that also positively affect kelp ecosystems are the predatory crabs (red king crab, Paralithodes camtchaticus and brown crab Cancer pagurus) in Norway (Christie et al., 2019), the California sheephead (Semicossyphus pulcher) in the Eastern Pacific (Caselle et al., 2018), and sea stars, such as the carnivorous Pycnopodia spp. along the Pacific Coast of North America (Burt et al., 2018). Little work has assessed the feasibility of culturing these species, but preliminary results on other analogous species suggest that it could be feasible (Stevens, 2006; Brooker et al., 2018). For example, large scale cultures of Paralithodes camtchaticus supplement wild fishery populations (Epelbaum et al., 2006; Daly et al., 2009) and maybe adjusted for restoration purposes. In California, the sheephead is a popular target for sports fishers and preliminary work part of a breeding program showed that they can spawn in captivity (Jirsa et al., 2007). However, their social structure, feeding requirements, and hermaphroditism make them difficult to culture and further efforts by the “Hubbs-Seaworld Research Institute and the Ocean Resources Enhancement and Hatchery Program” in California, United States are no longer under investigation (Stuart, Personal communication, 2019). Following the sea star wasting syndrome die off in the Eastern Pacific (Eisenlord et al., 2016; Harvell et al., 2019), scientists at the University of Washington and The Nature Conservancy California are beginning to experiment with culturing wild sea stars Pycnopodia spp., spawning them, and raising the juveniles to maturity, and determining their impact in the ecosystem. If the trials are successful, they plan to scale up the results, incorporate genetic diversity into the breeding program, and work to develop a recovery plan for the species (Eddy, Personal communication, 2020).
The restoration of an ecosystem through restored trophic interactions has been and will continue to be the subject of much debate (Seddon et al., 2007; Lorimer et al., 2015; Svenning et al., 2016). As this conversation continues, any attempt at restoring kelp forests in parallel with one of the prior mentioned species must consider: the ecosystem effects of that species, the genetic diversity of the introduced population, potential disease transmission, actual and opportunity costs, and public perception, and will for reintroduction along with other societal issues. Other authors (McCoy and Berry, 2008; Lorenzen et al., 2010) consider these barriers elsewhere, but this is beyond the scope of our review.
As oceans continue to warm, species ranges and territories will change, and new trophic interactions will form. For example, the tropical rock lobster (Panulirus ornatus) is currently mass cultured for commercial sale (Petersen and Phuong, 2010) but the species is currently restricted from most of South Australia by temperature. As oceans get warmer, there may be the opportunity to introduce P. ornatus into these now habitable areas to help control urchin populations. Such considerations and novel interactions may become important in any attempt to assist in future kelp restoration efforts (Wood et al., 2019).
Genetics in Kelp Restoration –Figure 2-4
Over the past few decades, it has become clear that genetics are an influential component of an individual’s, population’s, or wider ecosystem’s health. For example, genetic diversity and provenance can affect establishment rates and population fitness in many plants and animals (Hughes and Stachowicz, 2004; Forsman and Wennersten, 2016). Restoration efforts can thus benefit by incorporating the mechanisms responsible for these positive health effects (McDonald et al., 2016; Gann et al., 2019). The positive population and ecosystem effects from enhanced genetic diversity may be achieved through the restoration of diverse genotypes or phenotypes or individuals (Gann et al., 2019). The case is particularly strong for foundation species, where enhanced genetic diversity has benefitted not only the survival of the target species but also other components of the ecosystem, such as primary productivity and rates of decay and flux of nutrients (Whitham et al., 2006; Hughes et al., 2008; Reynolds et al., 2012; Kettenring et al., 2014; Gribben et al., 2020).
Although genetic approaches are only now being considered in the context of kelp restoration (Coleman and Goold, 2019), the kelp or broader seaweed aquaculture industry has long used techniques to genetically manipulate kelp, such as selected breeding or strain selection and manipulation to increase yield and flavor (Dai et al., 1993; Liu et al., 2006; Bast, 2014). Phycologists in the industry have used chimeras in Laminaria sp. populations to insert traits for increased tolerance to irradiance, seawater temperatures, and tissue rot (Li et al., 2007, 2008; Robinson et al., 2013). Further work to increase the genetic heterogeneity of seaweeds may potentially allow for increased resistance to abiotic stressors (Medina et al., 2015) and may also confer adaptive capacity to climate stress (Wernberg et al., 2018).
The selection of donor biological material (reproductive tissue, individuals, populations) that contain desirable traits such as tolerance to thermal stress may also be necessary to future-proof populations (Wood et al., 2019). This process might involve sourcing biological material for restoration from warm-adapted populations, breeding under specific conditions designed to achieve “super strains” or even implementing synthetic biology techniques, e.g., using CRISPR-Cas9 genome editing tool to edit the genomes of kelp species to bring out desirable traits (Coleman and Goold, 2019; Wood et al., 2019). Such future-proofing concepts are in development for terrestrial (Aitken and Whitlock, 2013) and coral reef systems (van Oppen et al., 2015), and are now being explored in the context of seaweed restoration as well (Wood et al., 2019).
While the explicit incorporation of genetics in marine restoration is rare (Mijangos et al., 2015), the techniques exist in industry (Robinson et al., 2013) which when coupled with the advancement of other genetic and genomic tools, e.g., rapid DNA sequencing technologies, can enable scientists to understand how to further advance restoration (Mijangos et al., 2015; Wood et al., 2019). For example, Wood et al. (2020) recently demonstrated that genetic diversity and structure of restored Phyllospora comosa (order Fucales) populations mimicked that of a mixture of local extant populations and this provides a platform to effectively “design” populations of this species as desired. While the application of seaweed genetic diversity in a restoration/management context requires further research, there is encouraging evidence for its future application to seaweed restoration programs. As these programs continue to develop, it is important that they proceed with caution and consider the potentially deleterious effects of introducing new genes or altering existing genes within a population (Filbee-Dexter and Smajdor, 2019).
Microbial Interactions and Kelp Restoration – Figure 2-5
Another aspect that may enhance effective restoration and management is the incorporation of kelp–microbiome interactions. Evidence from multiple systems suggests that microorganisms play fundamental roles in the life and performance of their eukaryotic hosts (McFall-Ngai et al., 2013). This knowledge has led to the proposal of the “holobiont” concept (Margulis and Fester, 1991), which argues that ‘macrobial’ hosts and their associated microbiota form a coherent biological entity and we need to considered them together to understand the biology and ecology of hosts (McFall-Ngai et al., 2013). In marine systems, this concept was first applied to reef-forming corals (Rohwer et al., 2002), but recent work highlights its applicability to other marine macroorganisms, including seaweeds (Egan et al., 2013). For instance, surface-associated microorganisms can influence the development, growth, photosynthesis, and reproduction of seaweeds (see review by Egan et al., 2013), and recent work suggests that microbes may even influence interactions between seaweeds and other macroorganisms such as grazers and epiphytes (Campbell et al., 2014b; Marzinelli et al., 2018).
Most studies of kelp-associated microorganisms are, however, descriptive, showing relationships between environmental conditions and/or kelp performance and condition, and the structure of the associated microbiota (Lachnit et al., 2011). Often, the focus is on the negative effects of microbes on kelp (Marzinelli et al., 2015), for example, changes in abundances of several bacterial taxa can cause a bleaching disease of the Australian kelp Ecklonia radiata, and experiments manipulating warming and acidification show that future environmental conditions are likely to exacerbate this (Qiu et al., 2019). Some studies have gone beyond establishing relationships to show causation in seaweed systems via isolation and subsequent experimental inoculation of target microorganisms (Matsuo et al., 2005; Case et al., 2011; Kumar et al., 2016). Despite the focus on negative/harmful interactions, experimental inoculations and similar experimental approaches (e.g., via selective removal of microbial taxa, Singh and Reddy, 2014) are potential techniques to determine positive interactions and isolate microbial taxa that may enhance kelp performance and/or confer resistance or resilience to future environmental conditions (see Rosado et al., 2019 for corals). Microbial communities associated with macroorganisms in marine systems are a “soup” of microbes and this presents manipulation challenges. However, recent work in corals has demonstrated that coral-associated microbiomes are influenceable and can develop in distinct directions following inoculations at early larval stages in experimental conditions (Damjanovic et al., 2017). Thus, focusing microbially guided restoration efforts on early life stages may enhance the feasibility of using such solutions in seaweed systems, either to enhance recruitment or growth, or resilience to abiotic (e.g., temperature) or biotic (e.g., grazing, fouling) stressors. For example, managers could grow kelp zygotes or recruits in the lab and inoculate them with specific taxa until they achieve a desired microbial community and then outplant them as normal.
Finally, host genetics can influence associated microbial communities (Org et al., 2016). Understanding the relative importance of host characteristics versus the environment in shaping the kelp microbiota is critical, as this may have implications on how we design restoration and/or future-proofing programs (Wood et al., 2019). If the environment influences microbial communities or important taxa, attempts to harness microbial interactions to improve restoration or future-proofing outcomes may fail as local microbial taxa swamp the microbial communities (but see Campbell et al., 2015). Alternately, if host specific traits influence microbial communities, harnessing positive microbial interactions may be as simple as including genotypes (or phenotypes) with beneficial microbiota. Another approach could be to tailor microbial manipulations to specific host types, as is in human medicine (Benson et al., 2010; Bonder et al., 2016). Our knowledge of kelp–microbiota interactions is still in its infancy and more experimental work aimed at understanding mechanisms is necessary in order to develop methods that successfully harness the power of beneficial microorganisms in the context of kelp restoration.
Anthropogenic Synergies – Figure 2-6
It is likely that kelp forest restoration can receive ecological and environmental benefits from kelp aquaculture and marine harvest efforts. The use of cultivated populations of kelp as concentrated sources of spores seems particularly promising, especially given that extensive localized losses of kelp in some areas combined with short dispersal distances and Allee effects can slow natural recovery of kelp populations. But these applications require availability of suitable local substratum nearby and that the kelp not be harvested before it is reproductive, two conditions that require additional consideration. The aquaculture of kelp also has direct economic outputs, and this may help incentivize and contribute to the funding of local ‘restoration economies’ (BenDor et al., 2015). Kelp aquaculture would also help to ease pressure on kelp forests (restored or otherwise) that may be the target for wild harvest operations. In addition, kelp cultivation may also be a cost-effective method of trialing whether an area is suitable for kelp growth and re-establishment, especially where local conditions have improved/degraded relative to the established trend.
Another innovative solution is the removal of sea urchins by divers who then sell them as a food product, known as uni in Japanese restaurants (Hohman et al., 2019; Sea Urchin Harvest, 2020). In many instances, however, the edible part of the urchin (the roe) is of poor quality due to limited food availability in the urchin barren (Claisse et al., 2013). Companies are working to solve this problem by establishing land-based aquaculture facilities that take urchins collected from barrens, feed them an adequate diet, improve the quality of the gonads, and then sell the urchins on the market (Urchinomics, 2020). As conservation considers market-based solutions (Huwyler et al., 2016), this approach to kelp restoration holds significant promise and may be especially useful in areas where predators are unable to revert urchin barrens from an alternate stable state while also creating jobs and contributing to local economies.
Kelp forests are especially efficient nutrient cyclers and are thus recognized as sustainable and positive solutions to nutrient loading in aquaculture farms (Chopin et al., 2001; Stévant et al., 2017). While kelp forests do not directly benefit from this relationship (unless nutrient-limited), their services could motivate aquaculture facilities to restore kelp forests next to their operations, thus helping reduce the financial load on other organizations. While these solutions will not be applicable in all circumstances, these practices contribute to the broader idea behind ‘restorative aquaculture’ (Theuerkauf et al., 2019) and might provide a beneficial accompaniment to restoration activities.
Incorporation of Positive Interactions in Kelp Forest Restoration
As managers continue to work to restore kelp forests, they will need to consider novel and adaptive approaches in a bid to achieve success while also crafting cost-efficient solutions. We posit that incorporating facilitative interactions and other synergies into traditional forms of restoration may help achieve these two purposes. Many of the solutions described above, need little to no further research to inform new restoration projects and can be used once the initial causes of decline have been mitigated or removed. To take advantage of intraspecific processes, managers can pair juvenile and adult outplants or combine adult transplants with seeding efforts. We also suggest that future restoration locations be closely spaced to each other or in close vicinity to extant kelp beds. Or, if kelp beds are declining but have not yet disappeared, restoration efforts can instead focus on augmenting existing beds and eliminating the need for future restoration. Depending on the species involved, managers could look for algal species, or genotypes, that promote each other and look to outplant polycultures instead of monocultures. Managers can further consider the benefits of restoring additional elements of the ecosystem in addition to the kelp itself. For example, where urchins are a problem, restoring species like otters, lobsters, crabs, or sea stars incurs a high upfront cost but can likely offset the cost of continual, manual urchin removal in the long term. Additionally, by adopting this approach to restoration, we are advancing the establishment of ecosystem functions beyond those provided by foundation species, an implicit goal in most all ecosystem restoration projects. Kelp and aquaculture farms also provide exploitable synergies to not only restore ecosystems but provide profits for their operators. Working to situate kelp farms near restoration sites can help seed barren grounds and once populations have become established, the kelp itself can work to offset nutrient pollution from aquaculture farms. It is also possible that kelp restoration could be profitable with new companies looking to remove, culture, and sell the urchins from barrens, thus letting the kelp regrow. Future permitting could be contingent on the company adopting best ecosystem practices and restorative aquaculture certifications can incentivize companies to restore kelp forests as part of their business.
Other approaches, namely incorporating genetic adaptation, interactions between specific genotypes and beneficial microbes are not as established, but steady progress is being made on understanding how future efforts can use these approaches. Because these approaches will initially be more costly than traditional restoration, it will be important to consider the added benefits of incorporating them into restoration practices. While this analysis is not completed, it is possible that with rapidly shifting environmental conditions, microbial and genetic approaches will be requisites to future restoration operations.
Managers can start integrating these interactions into restoration during the planning process, first by describing the known or plausible interactions in their system, determining which ones are feasibly included, carefully considering adverse outcomes, experimentally testing them at small scales, and then putting them into practice. As with any new conservation or restoration intervention, it is vital that we pair these approaches with adequate monitoring programs to evaluate them against goal-dependent performance criteria (Basconi et al., 2020; Eger et al., 2020b), and work to determine the marginal gains in success and the associated costs. Additionally, caution should be used whenever manipulating the environment, most notably when introducing foreign or novel species, genotypes, or microbiomes. While the interactions described above carry many potential benefits, they may also cause unintended harm to the ecosystem as novel introductions could replace elements of the ecosystem beyond those intended. Therefore, an essential part of the planning process is the small-scale experimentation and trialing of the proposed methods. In all cases, the precautionary principle should be applied and we do not promote the blind application of these methods. Rather we suggest a careful, case-specific consideration of application of proven methods while also considering the need to make conservation decisions with incomplete information (Grantham et al., 2009).
More generally speaking, kelp restoration efforts would benefit from positive remediation of the environment and other preventative conservation measures. For example, a decrease in land-based nutrient inputs that benefits turf algae or a decrease in sediment deposited in coastal ecosystems which interferes with the recruitment of kelp populations. As alluded to the positive species interactions section, it may indeed be most effective to restore kelp populations on the periphery of existing natural populations. Therefore, any efforts to conserve extant kelp populations may indeed be facilitating future restoration efforts. These efforts are also tied to improvements in water quality but also related to the destruction of rocky reef habitat, overfishing, overharvesting, or introduced species (Wernberg et al., 2019).
While we document the reported positive interactions that are feasibly useable to enhance kelp restoration, there are several other interactions from marine ecosystems that are not yet described. For instance, facilitation cascades (a set of positive species interactions) are well described and hypothesized to apply to salt marsh and coral restoration, but we are unaware of applicable analogs in kelp restoration. Further, as kelp species are typically limited dispersers, any interaction that worked to enhance the dispersal range of kelp forests would be a great aid to restoration efforts as established, restored populations could act as a source population for other areas. Even among the topics included in our review there is very little empirical evidence for most subjects. Of the 54 papers found in our literature search, over half were about trophic cascades and no other topic had more than five papers on that subject. Additionally, aside from trophic cascades, the concepts outlined here, have only been tested at small scales and stand to be trialed at larger, more ecologically relevant scales. Both the topics included and excluded from this literature review require additional research.
The importance of these positive interactions should increase with additional anthropogenic stressors related to coastal development in climate change. Unfortunately, there is little empirical evidence, and these remain theoretical improvements to restoration. Therefore, we encourage scientists and managers not only to attempt to incorporate these approaches into their projects but work to test their efficacy and allow for restoration to act as both an experiment and a conservation outcome. By doing so, we can quickly and efficiently work to determine how to best restore our underwater forests in the face of mounting pressures.
Author Contributions
AE and BS conceived the manuscript concept. All authors contributed to narrowing the scope and selecting the final topics to be covered. AE conducted the literature review and created the figures and tables. AE incorporated comments from all authors and wrote the first draft. All authors read the draft, provided comments, and approved the manuscript for submission.
Funding
Support was provided to AE by the University of New South Wales in the form of a Scientia Ph.D. Fellowship. BS received travel support from the Australian Distinguished Fulbright Chair in Science and Technology CSIRO and from a grant from the Lenfest Ocean Program. Further support was provided by the Australian Research Council through various grants: DP180104041 (PS and EM) and LP160100836 (PS, EM, and AV).
Conflict of Interest
The authors declare that the research was conducted in the absence of any commercial or financial relationships that could be construed as a potential conflict of interest.
The handling Editor declared a past co-authorship with one of the authors PS.
Acknowledgments
The vector graphics were adapted from The Integration and Application Network, University of Maryland Center for Environmental Science (http://ian.umces.edu/imagelibrary/).
Supplementary Material
The Supplementary Material for this article can be found online at: https://www.frontiersin.org/articles/10.3389/fmars.2020.00544/full#supplementary-material
References
Aitken, S. N., and Whitlock, M. C. (2013). Assisted gene flow to facilitate local adaptation to climate change. Annu. Rev. Ecol. Evol. Syst. 44, 367–388. doi: 10.1146/annurev-ecolsys-110512-135747
Akino, H., Kawai, T., Yotsukura, N., and Kono, T. (2015). Transportation and spatial distribution of Saccharina japonica var. religiosa zoospores in surface waters off the coast of Tomari. Hokkaido, Sea of Japan. Fish. Eng. 52, 1–9.
Altieri, A. H., Bertness, M. D., Coverdale, T. C., Herrmann, N. C., and Angelini, C. (2012). A trophic cascade triggers collapse of a salt-marsh ecosystem with intensive recreational fishing. Ecology 93, 1402–1410. doi: 10.1890/11-1314.1
Anderson, R. J., Carrick, P., Levitt, G. J., and Share, A. (1997). Holdfasts of adult kelp Ecklonia maxima provide refuges from grazing for recruitment of juvenile kelps. Mar. Ecol. Prog. Ser. 159, 265–273. doi: 10.3354/meps159265
Angelini, C., Griffin, J. N., van de Koppel, J., Lamers, L. P. M., Smolders, A. J. P., Derksen-Hooijberg, M., et al. (2016). A keystone mutualism underpins resilience of a coastal ecosystem to drought. Nat. Commun. 7, 1–8.
Barrett, N. S., Buxton, C. D., and Edgar, G. J. (2009). Changes in invertebrate and macroalgal populations in Tasmanian marine reserves in the decade following protection. J. Exp. Mar. Bio. Ecol. 370, 104–119. doi: 10.1016/j.jembe.2008.12.005
Basconi, L., Cadier, C., and Guerrero-Limón, G. (2020). “Challenges in marine restoration ecology: how techniques, assessment metrics, and ecosystem valuation can lead to improved restoration success,” in YOUMARES 9-The Oceans: Our Research, Our Future, (Oldenburg: Springer), 83–99. doi: 10.1007/978-3-030-20389-4_5
Bast, F. (2014). An Illustrated Review on Cultivation and Life History of Agronomically Important Seaplants. New York, NY: Nova Publishers.
Bayraktarov, E., Saunders, M. I., Abdullah, S., Mills, M., Beher, J., Possingham, H. P., et al. (2016). The cost and feasibility of marine coastal restoration. Ecol. Appl. 26, 1055–1074. doi: 10.1890/15-1077
Bell, J. D., Leber, K. M., Blankenship, H. L., Loneragan, N. R., and Masuda, R. (2008). A new era for restocking, stock enhancement and sea ranching of coastal fisheries resources. Rev. Fish. Sci. 16, 1–9. doi: 10.1080/10641260701776951
BenDor, T., Lester, T. W., Livengood, A., Davis, A., and Yonavjak, L. (2015). Estimating the size and impact of the ecological restoration economy. PLoS One 10:e0128339. doi: 10.1371/journal.pone.0128339
Bennett, S., and Wernberg, T. (2014). Canopy facilitates seaweed recruitment on subtidal temperate reefs. J. Ecol. 102, 1462–1470. doi: 10.1111/1365-2745.12302
Bennett, S., Wernberg, T., Harvey, E. S., Santana-Garcon, J., and Saunders, B. J. (2015). Tropical herbivores provide resilience to a climate-mediated phase shift on temperate reefs. Ecol. Lett. 18, 714–723. doi: 10.1111/ele.12450
Benson, A. K., Kelly, S. A., Legge, R., Ma, F., Low, S. J., Kim, J., et al. (2010). Individuality in gut microbiota composition is a complex polygenic trait shaped by multiple environmental and host genetic factors. Proc. Natl. Acad. Sci. U.S.A 107, 18933–18938. doi: 10.1073/pnas.1007028107
Bertness, M. D., and Callaway, R. (1994). Positive interactions in communities. Trends Ecol. Evol. 9, 191–193.
Bishop, M. J., Coleman, M. A., and Kelaher, B. P. (2010). Cross-habitat impacts of species decline: response of estuarine sediment communities to changing detrital resources. Oecologia 163, 517–525. doi: 10.1007/s00442-009-1555-y
Bodkin, J. L. (2015). “Historic and contemporary status of sea otters in the north pacific,” in Sea Otter Conserv, eds S. E. Larson, A. Gross, and G. R. VanBlaricom (Washington DC: U.S. Department of the Interior), 43–61. doi: 10.1016/b978-0-12-801402-8.00003-2
Bonder, M. J., Kurilshikov, A., Tigchelaar, E. F., Mujagic, Z., Imhann, F., Vila, A. V., et al. (2016). The effect of host genetics on the gut microbiome. Nat. Genet. 48, 1407–1412.
Boonzaier, L., and Pauly, D. (2016). Marine protection targets: an updated assessment of global progress. Oryx 50, 27–35. doi: 10.1017/s0030605315000848
Booth, W. (1988). Reintroducing a political animal. Science 241, 156–158. doi: 10.1126/science.241.4862.156
Brooker, A. J., Skern-Mauritzen, R., and Bron, J. E. (2018). Production, mortality, and infectivity of planktonic larval sea lice, Lepeophtheirus salmonis (Krøyer, 1837): current knowledge and implications for epidemiological modelling. ICES J. Mar. Sci. 75, 1214–1234. doi: 10.1093/icesjms/fsy015
Bruno, J. F., Stachowicz, J. J., and Bertness, M. D. (2003). Inclusion of facilitation into ecological theory. Trends Ecol. Evol. 18, 119–125. doi: 10.1016/s0169-5347(02)00045-9
Bulleri, F. (2009). Facilitation research in marine systems: state of the art, emerging patterns and insights for future developments. J. Ecol. 97, 1121–1130. doi: 10.1111/j.1365-2745.2009.01567.x
Bulleri, F., Benedetti-Cecchi, L., Jaklin, A., and Iveša, L. (2016). Linking disturbance and resistance to invasion via changes in biodiversity: a conceptual model and an experimental test on rocky reefs. Ecol. Evol. 6, 2010–2021. doi: 10.1002/ece3.1956
Bulleri, F., Cristaudo, C., Alestra, T., and Benedetti-Cecchi, L. (2011). Crossing gradients of consumer pressure and physical stress on shallow rocky reefs: a test of the stress-gradient hypothesis. J. Ecol. 99, 335–344. doi: 10.1111/j.1365-2745.2010.01750.x
Bulleri, F., Eriksson, B. K., Queirós, A., Airoldi, L., Arenas, F., Arvanitidis, C., et al. (2018). Harnessing positive species interactions as a tool against climate-driven loss of coastal biodiversity. PLoS Biol. 16:e2006852. doi: 10.1371/journal.pbio.2006852
Burkholder, D. A., Heithaus, M. R., Fourqurean, J. W., Wirsing, A., and Dill, L. M. (2013). Patterns of top-down control in a seagrass ecosystem: could a roving apex predator induce a behaviour-mediated trophic cascade? J. Anim. Ecol. 82, 1192–1202. doi: 10.1111/1365-2656.12097
Burridge, T. R., Portelli, T., and Ashton, P. (1996). Effect of sewage effluents on germination of three marine brown algal macrophytes. Mar. Freshw. Res. 47, 1009–1014.
Burt, J. M., Tinker, M. T., Okamoto, D. K., Demes, K. W., Holmes, K., and Salomon, A. K. (2018). Sudden collapse of a mesopredator reveals its complementary role in mediating rocky reef regime shifts. Proc. R. Soc. B Biol. Sci. 285:20180553. doi: 10.1098/rspb.2018.0553
Buschmann, A. H., Prescott, S., Potin, P., Faugeron, S., Vasquez, J. A., Camus, C., et al. (2014). “The status of kelp exploitation and marine agronomy, with emphasis on Macrocystis pyrifera, in Chile,” in Advances in Botanical Research, ed. N. Bourgougnon (Amsterdam: Elsevier), 161–188.
Bustamante, R. H., Branch, G. M., and Eekhout, S. (1995). Maintenance of an exceptional intertidal grazer biomass in South Africa: subsidy by subtidal kelps. Ecology 76, 2314–2329. doi: 10.2307/1941704
Campbell, A. H., Marzinelli, E. M., Gelber, J., and Steinberg, P. D. (2015). Spatial variability of microbial assemblages associated with a dominant habitat-forming seaweed. Front. Microbiol. 6:230. doi: 10.3389/fmicb.2015.00230
Campbell, A. H., Marzinelli, E. M., Vergés, A., Coleman, M. A., and Steinberg, P. D. (2014a). Towards restoration of missing underwater forests. PLoS One 9:e84106. doi: 10.1371/journal.pone.0084106
Campbell, A. H., Vergés, A., and Steinberg, P. D. (2014b). Demographic consequences of disease in a habitat-forming seaweed and impacts on interactions between natural enemies. Ecology 95, 142–152. doi: 10.1890/13-0213.1
Case, R. J., Longford, S. R., Campbell, A. H., Low, A., Tujula, N., Steinberg, P. D., et al. (2011). Temperature induced bacterial virulence and bleaching disease in a chemically defended marine macroalga. Environ. Microbiol. 13, 529–537. doi: 10.1111/j.1462-2920.2010.02356.x
Caselle, J. E., Davis, K., and Marks, L. M. (2018). Marine management affects the invasion success of a non-native species in a temperate reef system in California. USA. Ecol. Lett. 21, 43–53. doi: 10.1111/ele.12869
Chan, S. W., Cheang, C. C., Chirapart, A., Gerung, G., Tharith, C., and Ang, P. (2013). Homogeneous population of the brown alga Sargassum polycystum in Southeast Asia: possible role of recent expansion and asexual propagation. PLoS One 8:e77662. doi: 10.1371/journal.pone.0077662
Chapman, A. R. O., and Johnson, C. R. (1990). Disturbance and organization of macroalgal assemblages in the Northwest Atlantic. Hydrobiologia 192, 77–121. doi: 10.1007/bf00006228
Cheney, D., Oestman, R., Volkhardt, G., and Getz, J. (1994). Creation of rocky intertidal and shallow subtidal habitats to mitigate for the construction of a large marina in Puget Sound. Washington. Bull. Mar. Sci. 55, 772–782.
Chopin, T., Buschmann, A. H., Halling, C., Troell, M., Kautsky, N., Neori, A., et al. (2001). Integrating seaweeds into marine aquaculture systems: a key toward sustainability. J. Phycol. 37, 975–986. doi: 10.1046/j.1529-8817.2001.01137.x
Christie, H. C., Andersen, G. S., Bekkby, T., Gitmark, J. K., Gundersen, H., and Rinde, E. (2019). Shifts between sugar kelp and turf algae in Norway: regime shifts or flips between different opportunistic seaweed species? Front. Mar. Sci. 6:72. doi: 10.3389/fmars.2019.00072
Chung, I. K., Oak, J. H., Lee, J. A., Shin, J. A., Kim, J. G., and Park, K.-S. (2013). Installing kelp forests/seaweed beds for mitigation and adaptation against global warming: korean project overview. ICES J. Mar. Sci. 70, 1038–1044. doi: 10.1093/icesjms/fss206
Claisse, J. T., Williams, J. P., Ford, T., Pondella, D. J., Meux, B., Protopapadakis, L., et al. (2013). Kelp forest habitat restoration has the potential to increase sea urchin gonad biomass. Ecosphere 4, 1–19. doi: 10.1890/ES12-00408.1
Coleman, M. A., Cetina-Heredia, P., Roughan, M., Feng, M., van Sebille, E., and Kelaher, B. P. (2017). Anticipating changes to future connectivity within a network of marine protected areas. Glob. Chang. Biol. 23, 3533–3542. doi: 10.1111/gcb.13634
Coleman, M. A., and Goold, H. (2019). Harnessing synthetic biology for kelp forest conservation. J. Phycol 55, 745–751. doi: 10.1111/jpy.12888
Coleman, M. A., Kelaher, B. P., Steinberg, P. D., and Millar, A. J. K. (2008). Absence of a large brown macroalga on urbanized rocky reefs around Sydney, Australia, and evidence for historical decline. J. Phycol. 44, 897–901. doi: 10.1111/j.1529-8817.2008.00541.x
Coleman, M. A., and Wernberg, T. (2017). Forgotten underwater forests: The key role of fucoids on Australian temperate reefs. Ecol. Evol. 7, 8406–8418. doi: 10.1002/ece3.3279
Connell, S. D., Russell, B. D., Turner, D. J., Shepherd, S. A., Kildea, T., Miller, D., et al. (2008). Recovering a lost baseline: missing kelp forests from a metropolitan coast. Mar. Ecol. Prog. Ser. 360, 63–72. doi: 10.3354/meps07526
Cuddington, K., Wilson, W. G., and Hastings, A. (2009). Ecosystem engineers: feedback and population dynamics. Am. Nat. 173, 488–498. doi: 10.1086/597216
Dai, J., Zhang, Q., and Bao, Z. (1993). “Genetic breeding and seedling raising experiments with Porphyra protoplasts,” in Genetics in Aquaculture, eds G. A. E. Gall and H. Chen (Amsterdam: Elsevier), 139–145. doi: 10.1016/0044-8486(93)90032-t
Daly, B., Swingle, J. S., and Eckert, G. L. (2009). Effects of diet, stocking density, and substrate on survival and growth of hatchery-cultured red king crab (Paralithodes camtschaticus) juveniles in Alaska, USA. Aquaculture 293, 68–73. doi: 10.1016/J.AQUACULTURE.2009.04.010
Damjanovic, K., Blackall, L. L., Webster, N. S., and van Oppen, M. J. H. (2017). The contribution of microbial biotechnology to mitigating coral reef degradation. Microb. Biotechnol. 10, 1236–1243. doi: 10.1111/1751-7915.12769
Davis, R. W., Bodkin, J. L., Coletti, H. A., Monson, D. H., Larson, S. E., Carswell, L. P., et al. (2019). Future directions in sea otter research and management. Front. Mar. Sci. 5:510. doi: 10.3389/fmars.2018.00510
Dayton, P. K. (1975). Experimental evaluation of ecological dominance in a rocky intertidal algal community. Ecol. Monogr. 45, 137–159. doi: 10.2307/1942404
Dayton, P. K. (1985). Ecology of kelp communities. Annu. Rev. Ecol. Syst. 16, 215–245. doi: 10.1146/annurev.es.16.110185.001243
Donadi, S., van der Heide, T., van der Zee, E. M., Eklöf, J. S., de Koppel, J., and van Weerman, E. J. (2013). Cross-habitat interactions among bivalve species control community structure on intertidal flats. Ecology 94, 489–498. doi: 10.1890/12-0048.1
Egan, S., Harder, T., Burke, C., Steinberg, P., Kjelleberg, S., and Thomas, T. (2013). The seaweed holobiont: understanding seaweed–bacteria interactions. FEMS Microbiol. Rev. 37, 462–476. doi: 10.1111/1574-6976.12011
Eger, A. M., and Baum, J. K. (2020). Trophic cascades in coastal marine ecosystems: a meta analysis of experimental and observational research. EcoEvoRXiv [Preprint]. doi: 10.32942/osf.io/vbn3x
Eger, A. M., Marzinelli, E., Steinberg, P., and Vergés, A. (2020a). Worldwide Synthesis of Kelp Forest Reforestation. Open Science Framework. Available online at: https://osf.io/5bgtw/
Eger, A. M., Vergés, A., Choi, C. G., Christie, H. C., Coleman, M. A., Fagerli, C. W., et al. (2020b). Financial and institutional support are important for large-scale kelp forest restoration. EcoEvoRXiv [Preprint]. doi: 10.32942/osf.io/5enhy
Eisenlord, M. E., Groner, M. L., Yoshioka, R. M., Elliott, J., Maynard, J., Fradkin, S., et al. (2016). Ochre star mortality during the 2014 wasting disease epizootic: role of population size structure and temperature. Philos. Trans. R. Soc. B Biol. Sci. 371:0150212.
Ellison, A. M., and Farnsworth, E. J. (1990). The ecology of Belizean mangrove-root fouling communities. I. Epibenthic fauna are barriers to isopod attack of red mangrove roots. J. Exp. Mar. Bio. Ecol. 142, 91–104. doi: 10.1016/0022-0981(90)90139-4
Epelbaum, A. B., Borisov, R. R., and Kovatcheva, N. P. (2006). Early development of the red king crab Paralithodes camtschaticus from the Barents Sea reared under laboratory conditions: morphology and behaviour. J. Mar. Biol. Assoc. U. K. 86, 317–333. doi: 10.1017/s0025315406013178
Estes, J. A., and Duggins, D. O. (1995). Sea otters and kelp forests in Alaska: generality and variation in a community ecological paradigm. Ecol. Monogr. 65, 75–100. doi: 10.2307/2937159
Ferrari, R., Marzinelli, E. M., Ayroza, C. R., Jordan, A., Figueira, W. F., Byrne, M., et al. (2018). Large-scale assessment of benthic communities across multiple marine protected areas using an autonomous underwater vehicle. PLoS One 13:e0193711. doi: 10.1371/journal.pone.0193711
Filbee-Dexter, K., and Scheibling, R. E. (2014). Sea urchin barrens as alternative stable states of collapsed kelp ecosystems. Mar. Ecol. Prog. Ser. 495, 1–25. doi: 10.3354/meps10573
Filbee-Dexter, K., and Smajdor, A. (2019). Ethics of assisted evolution in marine conservation. Front. Mar. Sci. 6:20. doi: 10.3389/fmars.2019.00020
Filbee-Dexter, K., and Wernberg, T. (2018). Rise of turfs: a new battlefront for globally declining kelp forests. Bioscience 68, 64–76. doi: 10.1093/biosci/bix147
Filbee-Dexter, K., Wernberg, T., Norderhaug, K. M., Ramirez-Llodra, E., and Pedersen, M. F. (2018). Movement of pulsed resource subsidies from kelp forests to deep fjords. Oecologia 187, 291–304. doi: 10.1007/s00442-018-4121-7
Forrest, B. M., Brown, S. N., Taylor, M. D., Hurd, C. L., and Hay, C. H. (2000). The role of natural dispersal mechanisms in the spread of Undaria pinnatifida (Laminariales. Phaeophyceae). Phycologia 39, 547–553. doi: 10.2216/i0031-8884-39-6-547.1
Forsman, A., and Wennersten, L. (2016). Inter-individual variation promotes ecological success of populations and species: evidence from experimental and comparative studies. Ecography 39, 630–648. doi: 10.1111/ecog.01357
Frangoudes, K., and Garineaud, C. (2015). “Governability of kelp forest small-scale harvesting in Iroise Sea, France,” in Interactive Governance for Small-Scale Fisheries, eds S. Jentoft and R. Chuenpagdee (Berlin: Springer), 101–115. doi: 10.1007/978-3-319-17034-3_6
Frölicher, T. L., Fischer, E. M., and Gruber, N. (2018). Marine heatwaves under global warming. Nature 560, 360–364. doi: 10.1038/s41586-018-0383-9
Gann, G. D., McDonald, T., Walder, B., Aronson, J., Nelson, C. R., Jonson, J., et al. (2019). International principles and standards for the practice of ecological restoration. Restor. Ecol. 27, S3–S46.
Gedan, K. B., and Silliman, B. R. (2009). Using facilitation theory to enhance mangrove restoration. AMBIO A J. Hum. Environ. 38:109. doi: 10.1579/0044-7447-38.2.109
Gorman, D., and Connell, S. D. (2009). Recovering subtidal forests in human-dominated landscapes. J. Appl. Ecol. 46, 1258–1265. doi: 10.1111/j.1365-2664.2009.01711.x
Graham, M. H., Vasquez, J. A., and Buschmann, A. H. (2007). Global ecology of the giant kelp Macrocystis: from ecotypes to ecosystems. Oceanogr. Mar. Biol. 45, 39–88. doi: 10.1201/9781420050943.ch2
Grantham, H. S., Wilson, K. A., Moilanen, A., Rebelo, T., and Possingham, H. P. (2009). Delaying conservation actions for improved knowledge: how long should we wait? Ecol. Lett. 12, 293–301. doi: 10.1111/j.1461-0248.2009.01287.x
Gribben, P., Angelini, C., Altieri, A. H., Bishop, M., Thomsen, M. S., and Bulleri, F. (2019). Facilitation cascades in marine ecosystems: a synthesis and future directions. Oceanogr. Mar. Biol. Annu. Rev. 57, 127–168. doi: 10.1201/9780429026379-3
Gribben, P. E., Bishop, M. J., O’Connor, W. A., Bradley, D. J., and Hughes, A. R. (2020). Intraspecific diversity in prey body size influences survivorship by conferring resistance to predation. Ecosphere 11:e03106.
Harrold, C., and Reed, D. C. (1985). Food availability, sea urchin grazing, and kelp forest community structure. Ecology 66, 1160–1169. doi: 10.2307/1939168
Harvell, C. D., Montecino-Latorre, D., Caldwell, J. M., Burt, J. M., Bosley, K., Keller, A., et al. (2019). Disease epidemic and a marine heat wave are associated with the continental-scale collapse of a pivotal predator (Pycnopodia helianthoides). Sci. Adv. 5:eaau7042. doi: 10.1126/sciadv.aau7042
Hawkins, S. J., Allen, J. R., and Bray, S. (1999). Restoration of temperate marine and coastal ecosystems: nudging nature. Aquat. Conserv. Mar. Freshw. Ecosyst. 9, 23–46. doi: 10.1002/(sici)1099-0755(199901/02)9:1<23::aid-aqc324>3.0.co;2-c
Hawkins, S. J., and Hartnoll, R. G. (1983). Grazing of intertidal algae by marine invertebrates. Oceanogr. Mar. Biol. 21, 195–282.
Hayward, M. W., and Somers, M. (2009). Reintroduction of Top-Order Predators, 1st Edn. West Sussex: John Wiley & Sons.
He, Q., Bertness, M. D., and Altieri, A. H. (2013). Global shifts towards positive species interactions with increasing environmental stress. Ecol. Lett. 16, 695–706. doi: 10.1111/ele.12080
He, Q., Silliman, B. R., Liu, Z., and Cui, B. (2017). Natural enemies govern ecosystem resilience in the face of extreme droughts. Ecol. Lett. 20, 194–201. doi: 10.1111/ele.12721
Hinojosa, I. A., Green, B. S., Gardner, C., and Jeffs, A. (2014). Settlement and early survival of southern rock lobster, Jasus edwardsii, under climate-driven decline of kelp habitats. ICES J. Mar. Sci. 72, i59–i68. doi: 10.1093/icesjms/fsu199
Hohman, R., Hutto, S., Catton, C. A., and Koe, F. (2019). Sonoma-Mendocino Bull Kelp Recovery Plan. Sacramento, CA: California Department of Fish and Wildlife.
Hooker, S. H., Jeffs, A. G., Creese, R. G., and Sivaguru, K. (1997). Growth of captive Jasus edwardsii (Hutton) (Crustacea:Palinuridae) in north-eastern New Zealand. Mar. Freshw. Res. 48, 903–909. doi: 10.1071/MF97156
Hughes, A. R., Inouye, B. D., Johnson, M. T. J., Underwood, N., and Vellend, M. (2008). Ecological consequences of genetic diversity. Ecol. Lett. 11, 609–623. doi: 10.1111/j.1461-0248.2008.01179.x
Hughes, A. R., and Stachowicz, J. J. (2004). Genetic diversity enhances the resistance of a seagrass ecosystem to disturbance. Proc. Natl. Acad. Sci. U.S.A. 101, 8998–9002. doi: 10.1073/pnas.0402642101
Huwyler, F., Käppeli, J., and Tobin, J. (2016). Conservation Finance from Niche to Mainstream. Zurich: The Building of an Institutional Asset Class.
Jensen, M. A., Fitzgibbon, Q. P., Carter, C. G., and Adams, L. R. (2013). The effect of stocking density on growth, metabolism and ammonia–N excretion during larval ontogeny of the spiny lobster Sagmariasus verreauxi. Aquaculture 376–379, 45–53. doi: 10.1016/J.AQUACULTURE.2012.10.033
Jirsa, D., Drawbridge, M., and Stuart, K. (2007). Spawning of a Captive Population of California Sheephead, Semicossyphus pulcher. J. World Aquac. Soc. 38, 122–128. doi: 10.1111/j.1749-7345.2006.00080.x
Johnson, C. R., Chabot, R. H., Marzloff, M. P., and Wotherspoon, S. (2017). Knowing when (not) to attempt ecological restoration. Restor. Ecol. 25, 140–147. doi: 10.1111/rec.12413
Jones, C. G., Gutiérrez, J. L., Byers, J. E., Crooks, J. A., Lambrinos, J. G., and Talley, T. S. (2010). A framework for understanding physical ecosystem engineering by organisms. Oikos 119, 1862–1869. doi: 10.1111/j.1600-0706.2010.18782.x
Jones, P. J. S., Qiu, W., and De Santo, E. M. (2013). Governing marine protected areas: social–ecological resilience through institutional diversity. Mar. Policy 41, 5–13. doi: 10.1016/j.marpol.2012.12.026
Katano, I., Doi, H., Eriksson, B. K., and Hillebrand, H. (2015). A cross-system meta-analysis reveals coupled predation effects on prey biomass and diversity. Oikos 124, 1427–1435. doi: 10.1111/oik.02430
Kettenring, K. M., Mercer, K. L., Reinhardt Adams, C., and Hines, J. (2014). Application of genetic diversity–ecosystem function research to ecological restoration. J. Appl. Ecol. 51, 339–348. doi: 10.1111/1365-2664.12202
Kirkman, H. (1981). The first year in the life history and the survival of the juvenile marine macrophyte, Ecklonia radiata (Turn.). J. Agardh. J. Exp. Mar. Bio. Ecol. 55, 243–254. doi: 10.1016/0022-0981(81)90115-5
Kitching, J. A. (1941). Studies in Sublittoral ecology: III. Laminaria Forest on the West Coast of Scotland; a study of Zonation in Relation to wave action and illumination. Biol. Bull. 80, 324–337. doi: 10.2307/1537719
Kittaka, J., Ono, K., Booth, J. D., and Webber, W. R. (2005). Development of the red rock lobster, Jasus edwardsii, from egg to juvenile. New Zeal. J. Mar. Freshw. Res. 39, 263–277. doi: 10.1080/00288330.2005.9517306
Kiyomoto, S., Tagawa, M., Nakamura, Y., Horii, T., Watanabe, S., Tozawa, T., et al. (2013). Decrease of abalone resources with disappearance of macroalgal beds around the Ojika Islands, Nagasaki, southwestern Japan. J. Shellfish Res. 32, 51–59.
Krumhansl, K. A., Okamoto, D. K., Rassweiler, A., Novak, M., Bolton, J. J., Cavanaugh, K. C., et al. (2016). Global patterns of kelp forest change over the past half-century. Proc. Natl. Acad. Sci. 113, 13785–13790. doi: 10.1073/pnas.1606102113
Kumar, V., Zozaya-Valdes, E., Kjelleberg, S., Thomas, T., and Egan, S. (2016). Multiple opportunistic pathogens can cause a bleaching disease in the red seaweed Delisea pulchra. Environ. Microbiol. 18, 3962–3975. doi: 10.1111/1462-2920.13403
Lachnit, T., Meske, D., Wahl, M., Harder, T., and Schmitz, R. (2011). Epibacterial community patterns on marine macroalgae are host-specific but temporally variable. Environ. Microbiol. 13, 655–665. doi: 10.1111/j.1462-2920.2010.02371.x
Lanham, B. S., Poore, A. G. B., and Gribben, P. E. (2020). Facilitation cascades create a predation refuge for biodiversity in a novel connected habitat. Ecosphere 11:e03053.
Layton, C., Coleman, M. A., Marzinelli, E. M., Steinberg, P. D., Swearer, S. E., Vergés, A., et al. (2020). Kelp forest restoration in Australia. Front. Mar. Sci. 7:74. doi: 10.3389/fmars.2020.00074
Layton, C., Shelamoff, V., Cameron, M. J., Tatsumi, M., Wright, J. T., and Johnson, C. R. (2019). Resilience and stability of kelp forests: the importance of patch dynamics and environment-engineer feedbacks. PLoS One 14:e0210220. doi: 10.1371/journal.pone.0210220
Leonard, G. H. (2000). Latitudinal variation in species interactions: a test in the New England rocky intertidal zone. Ecology 81, 1015–1030. doi: 10.1890/0012-9658(2000)081[1015:lvisia]2.0.co;2
Li, X., Cong, Y., Yang, G., Shi, Y., Qu, S., Li, Z., et al. (2007). Trait evaluation and trial cultivation of Dongfang No. 2, the hybrid of a male gametophyte clone of Laminaria longissima (Laminariales, Phaeophyta) and a female one of L. japonica. J. Appl. Phycol. 19, 139–151. doi: 10.1007/s10811-006-9120-0
Li, X., Liu, J., Cong, Y., Qu, S., Zhang, Z., Dai, H., et al. (2008). Breeding and trial cultivation of Dongfang No. 3, a hybrid of Laminaria gametophyte clones with a more than intraspecific but less than interspecific relationship. Aquaculture 280, 76–80. doi: 10.1016/j.aquaculture.2008.05.005
Ling, S. D., Johnson, C. R., Frusher, S. D., and Ridgway, K. R. (2009). Overfishing reduces resilience of kelp beds to climate-driven catastrophic phase shift. Proc. Natl. Acad. Sci. U.S.A. 106, 22341–22345. doi: 10.1073/pnas.0907529106
Ling, S. D., Scheibling, R. E., Rassweiler, A., Johnson, C. R., Shears, N., Connell, S. D., et al. (2015). Global regime shift dynamics of catastrophic sea urchin overgrazing. Philos. Trans. R. Soc. B Biol. Sci. 370:20130269. doi: 10.1098/rstb.2013.0269
Ling, S., and Keane, J. (2018). Resurvey of the longspined Sea Urchin (Centrostephanus rodgersii) and associated barren reef in Tasmania. Hobart Tasmania 1–52. doi: 10.13140/RG.2.2.16363.80162
Liu, Y., Cui, J., Shen, X., Liu, T., and Gong, Q. (2006). The application of dna molecular marker technique to heritable breeding of Laminaria. Trans. Oceanol. Limnol. 1, 75–81.
Lorenzen, K., Leber, K. M., and Blankenship, H. L. (2010). Responsible approach to marine stock enhancement: an update. Rev. Fish. Sci. 18, 189–210. doi: 10.1080/10641262.2010.491564
Lorimer, J., Sandom, C., Jepson, P., Doughty, C., Barua, M., and Kirby, K. J. (2015). Rewilding: science, practice, and politics. Annu. Rev. Environ. Resour. 40, 39–62. doi: 10.1146/annurev-environ-102014-021406
Luttikhuizen, P. C., van den Heuvel, F. H. M., Rebours, C., Witte, H. J., van Bleijswijk, J. D. L., and Timmermans, K. (2018). Strong population structure but no equilibrium yet: Genetic connectivity and phylogeography in the kelp Saccharina latissima (Laminariales. Phaeophyta). Ecol. Evol. 8, 4265–4277. doi: 10.1002/ece3.3968
Margulis, L., and Fester, R. (1991). Symbiosis as a Source of Evolutionary Innovation: Speciation and Morphogenesis. Cambridge: MIT Press.
Marzinelli, E. M., Burrows, M. T., Jackson, A. C., and Mayer-Pinto, M. (2012). Positive and negative effects of habitat-forming algae on survival, growth and intra-specific competition of limpets. PLoS One 7:e51601. doi: 10.1371/journal.pone.0051601
Marzinelli, E. M., Campbell, A. H., Verges, A., Coleman, M. A., Kelaher, B. P., and Steinberg, P. D. (2014). Restoring seaweeds: does the declining fucoid Phyllospora comosa support different biodiversity than other habitats? J. Appl. Phycol. 26, 1089–1096. doi: 10.1007/s10811-013-0158-5
Marzinelli, E. M., Campbell, A. H., Zozaya Valdes, E., Vergés, A., Nielsen, S., Wernberg, T., et al. (2015). Continental-scale variation in seaweed host-associated bacterial communities is a function of host condition, not geography. Environ. Microbiol. 17, 4078–4088. doi: 10.1111/1462-2920.12972
Marzinelli, E. M., Qiu, Z., Dafforn, K. A., Johnston, E. L., Steinberg, P. D., and Mayer-Pinto, M. (2018). Coastal urbanisation affects microbial communities on a dominant marine holobiont. Biofilms Microbiomes 4, 1–7.
Matsuo, Y., Imagawa, H., Nishizawa, M., and Shizuri, Y. (2005). Isolation of an algal morphogenesis inducer from a marine bacterium. Science 307:1598. doi: 10.1126/science.1105486
McCoy, E. D., and Berry, K. (2008). Using an ecological ethics framework to make decisions about the relocation of wildlife. Sci. Eng. Ethics 14, 505–521. doi: 10.1007/s11948-008-9091-4
McDonald, T., Jonson, J., and Dixon, K. W. (2016). National standards for the practice of ecological restoration in Australia. Restor. Ecol. 24, S4–S32.
McFall-Ngai, M., Hadfield, M. G., Bosch, T. C. G., Carey, H. V., Domazet-Lošo, T., and Douglas, A. E. (2013). Animals in a bacterial world, a new imperative for the life sciences. Proc. Natl. Acad. Sci. U.S.A. 110, 3229–3236.
Medina, F. J., Flores, V., González, A. V., and Santelices, B. (2015). Coalescence increases abiotic stress tolerance in sporelings of Mazzaella laminarioides (Gigartinales, Rhodophyta). J. Appl. Phycol. 27, 1593–1598. doi: 10.1007/s10811-014-0478-0
Melville, A. J., and Connell, S. D. (2001). Experimental effects of kelp canopies on subtidal coralline algae. Austral Ecol. 26, 102–108. doi: 10.1111/j.1442-9993.2001.01089.pp.x
Mijangos, J. L., Pacioni, C., Spencer, P. B. S., and Craig, M. D. (2015). Contribution of genetics to ecological restoration. Mol. Ecol. 24, 22–37. doi: 10.1111/mec.12995
Miller, R. J., Lafferty, K. D., Lamy, T., Kui, L., Rassweiler, A., and Reed, D. C. (2018). Giant kelp, Macrocystis pyrifera, increases faunal diversity through physical engineering. Proc. R. Soc. B Biol. Sci. 285:20172571. doi: 10.1098/rspb.2017.2571
Norton, T. A. (1992). Dispersal by macroalgae. Br. Phycol. J. 27, 293–301. doi: 10.1080/00071619200650271
Olson, A. M., Hessing-Lewis, M., Haggarty, D., and Juanes, F. (2019). Nearshore seascape connectivity enhances seagrass meadow nursery function. Ecol. Appl. 29:e01897.
Org, E., Mehrabian, M., Parks, B. W., Shipkova, P., Liu, X., Drake, T. A., et al. (2016). Sex differences and hormonal effects on gut microbiota composition in mice. Gut Microbes 7, 313–322. doi: 10.1080/19490976.2016.1203502
Pearson, G. A., and Brawley, S. H. (1996). Reproductive ecology, of Fucus distichus (Phaeophyceae): an intertidal alga with successful external fertilization. Mar. Ecol. Prog. Ser. 143, 211–223. doi: 10.3354/meps143211
Petersen, E. H., and Phuong, T. H. (2010). Tropical spiny lobster (Panulirus ornatus) farming in Vietnam - bioeconomics and perceived constraints to development. Aquac. Res. 41, 634–642. doi: 10.1111/j.1365-2109.2010.02581.x
Qiu, Z., Coleman, M. A., Provost, E., Campbell, A. H., Kelaher, B. P., Dalton, S. J., et al. (2019). Future climate change is predicted to affect the microbiome and condition of habitat-forming kelp. Proc. R. Soc. B 286:20181887. doi: 10.1098/rspb.2018.1887
Queirós, A. M., Stephens, N., Widdicombe, S., Tait, K., McCoy, S. J., Ingels, J., et al. (2019). Connected macroalgal-sediment systems: blue carbon and food webs in the deep coastal ocean. Ecol. Monogr. 89:e01366.
Reed, D. C., Kinlan, B. P., Raimondi, P. T., Washburn, L., Gaylord, B., and Drake, P. T. (2006). “A metapopulation perspective on the patch dynamics of giant kelp in Southern California,” in Marine Metapopulations, eds J. P. Kritzer and P. F. Sale (Amsterdam: Elsevier), 353–386. doi: 10.1016/b978-012088781-1/50013-3
Renzi, J. J., He, Q., and Silliman, B. R. (2019). Harnessing positive species interactions to enhance coastal wetland restoration. Front. Ecol. Evol. 7:131. doi: 10.3389/fevo.2019.00131
Reynolds, L. K., McGlathery, K. J., and Waycott, M. (2012). Genetic diversity enhances restoration success by augmenting ecosystem services. PLoS One 7:e38397. doi: 10.1371/journal.pone.0038397
Ritar, A. J. (2001). The experimental culture of phyllosoma larvae of southern rock lobster (Jasus edwardsii) in a flow-through system. Aquac. Eng. 24, 149–156. doi: 10.1016/S0144-8609(00)00072-8
Robinson, N., Winberg, P., and Kirkendale, L. (2013). Genetic improvement of macroalgae: status to date and needs for the future. J. Appl. Phycol. 25, 703–716. doi: 10.1007/s10811-012-9950-x
Rogers-Bennett, L., and Catton, C. A. (2019). Marine heat wave and multiple stressors tip bull kelp forest to sea urchin barrens. Sci. Rep. 9, 1–9.
Rohwer, F., Seguritan, V., Azam, F., and Knowlton, N. (2002). Diversity and distribution of coral-associated bacteria. Mar. Ecol. Prog. Ser. 243, 1–10. doi: 10.3354/meps243001
Rosado, P. M., Leite, D. C. A., Duarte, G. A. S., Chaloub, R. M., Jospin, G., da Rocha, U. N., et al. (2019). Marine probiotics: increasing coral resistance to bleaching through microbiome manipulation. ISME J. 13, 921–936. doi: 10.1038/s41396-018-0323-6
Schiel, D. R. (1985). A short-term demographic study of Cystoseira osmundacea (fucales: cystoseiraceae) in central California. J. Phycol. 21, 99–106. doi: 10.1111/j.0022-3646.1985.00099.x
Schiel, D. R., and Foster, M. S. (2006). The population biology of large brown seaweeds: ecological consequences of multiphase life histories in dynamic coastal environments. Annu. Rev. Ecol. Evol. Syst. 37, 343–372. doi: 10.1146/annurev.ecolsys.37.091305.110251
Schiel, D. R., and Foster, M. S. (2015). The biology and ecology of giant kelp forests. Berkeley, CA: University of California Press.
Sea Urchin Harvest (2020). Sea Urchin Harvest. Available online at: https://seaurchinharvest.com.au/ (accessed February 2, 2020).
Seddon, P. J., Armstrong, D. P., and Maloney, R. F. (2007). Developing the science of reintroduction biology. Conserv. Biol. 21, 303–312. doi: 10.1111/j.1523-1739.2006.00627.x
Serisawa, Y., Imoto, Z., Taino, S., Choi, C. G., Ishikawa, T., Ohno, M., et al. (2005). Marine afforestation of Ecklonia cava by using a spore bag method at an Isoyake area in Tosa Bay, southern Japan. Jpn. J. Phycol. 53, 19–24.
Shaver, E. C., Burkepile, D. E., and Silliman, B. R. (2018). Local management actions can increase coral resilience to thermally-induced bleaching. Nat. Ecol. Evol. 2, 1075–1079. doi: 10.1038/s41559-018-0589-0
Shaver, E. C., and Silliman, B. R. (2017). Time to cash in on positive interactions for coral restoration. PeerJ 5:e3499. doi: 10.7717/peerj.3499
Shears, N. T., and Babcock, R. C. (2002). Marine reserves demonstrate top-down control of community structure on temperate reefs. Oecologia 132, 131–142. doi: 10.1007/s00442-002-0920-x
Shurin, J. B., Markel, R. W., and Mathews, B. (2010). “Comparing trophic cascades across ecosystems,” in Trophic Cascades: Predators, Prey, and the Changing Dynamics of Nature, eds J. W. Terborgh and J. A. Estes (Washington, DC: Island Press), 319–336.
Silliman, B. R., and Bertness, M. D. (2002). A trophic cascade regulates salt marsh primary production. Proc. Natl. Acad. Sci. U.S.A. 99, 10500–10505. doi: 10.1073/pnas.162366599
Silliman, B. R., Bertness, M. D., Altieri, A. H., Griffin, J. N., Bazterrica, M. C., Hidalgo, F. J., et al. (2011). Whole-community facilitation regulates biodiversity on Patagonian rocky shores. PLoS One 6:e24502. doi: 10.1371/journal.pone.0024502
Silliman, B. R., Schrack, E., He, Q., Cope, R., Santoni, A., van der Heide, T., et al. (2015). Facilitation shifts paradigms and can amplify coastal restoration efforts. Proc. Natl. Acad. Sci. U.S.A. 112, 14295–14300. doi: 10.1073/pnas.1515297112
Singh, R. P., and Reddy, C. R. K. (2014). Seaweed–microbial interactions: key functions of seaweed-associated bacteria. FEMS Microbiol. Ecol. 88, 213–230. doi: 10.1111/1574-6941.12297
Smale, D. A., Burrows, M. T., Moore, P., O’Connor, N., and Hawkins, S. J. (2013). Threats and knowledge gaps for ecosystem services provided by kelp forests: a northeast A tlantic perspective. Ecol. Evol. 3, 4016–4038. doi: 10.1002/ece3.774
Smale, D. A., Wernberg, T., Oliver, E. C. J., Thomsen, M., Harvey, B. P., Straub, S. C., et al. (2019). Marine heatwaves threaten global biodiversity and the provision of ecosystem services. Nat. Clim. Chang 9, 306–312.
Stévant, P., Rebours, C., and Chapman, A. (2017). Seaweed aquaculture in Norway: recent industrial developments and future perspectives. Aquac. Int. 25, 1373–1390.
Stevens, B. G. (2006). “King crab cultivation and stock enhancement in Japan and the United States: a brief history,” in Alaska Crab Stock Enhancement and Rehabilitation: Workshop Proceedings. At Kodiak, 23–31.
Svenning, J.-C., Pedersen, P. B. M., Donlan, C. J., Ejrnæs, R., Faurby, S., Galetti, M., et al. (2016). Science for a wilder Anthropocene: synthesis and future directions for trophic rewilding research. Proc. Natl. Acad. Sci. U.S.A. 113, 898–906. doi: 10.1073/pnas.1502556112
Tait, L. W. (2019). Giant kelp forests at critical light thresholds show compromised ecological resilience to environmental and biological drivers. Estuar. Coast. Shelf Sci. 219, 231–241.
Teagle, H., Hawkins, S. J., Moore, P. J., and Smale, D. A. (2017). The role of kelp species as biogenic habitat formers in coastal marine ecosystems. J. Exp. Mar. Bio. Ecol. 492, 81–98. doi: 10.1016/j.jembe.2017.01.017
Terawaki, T., Hasegawa, H., Arai, S., and Ohno, M. (2001). Management-free techniques for restoration of Eisenia and Ecklonia beds along the central Pacific coast of Japan. J. Appl. Phycol. 13, 13–17.
Theuerkauf, S. J., Morris, J. A. Jr., Waters, T. J., Wickliffe, L. C., Alleway, H. K., and Jones, R. C. (2019). A global spatial analysis reveals where marine aquaculture can benefit nature and people. PLoS One 14:e0222282. doi: 10.1371/journal.pone.0222282
Thibaut, T., Pinedo, S., Torras, X., and Ballesteros, E. (2005). Long-term decline of the populations of Fucales (Cystoseira spp. and Sargassum spp.) in the Alberes coast (France, North-western Mediterranean). Mar. Pollut. Bull. 50, 1472–1489.
Thomsen, M. S., Altieri, A. H., Angelini, C., Bishop, M. J., Gribben, P. E., Lear, G., et al. (2018). Secondary foundation species enhance biodiversity. Nat. Ecol. Evol. 2, 634–639.
Toohey, B. D., Kendrick, G. A., and Harvey, E. S. (2007). Disturbance and reef topography maintain high local diversity in Ecklonia radiata kelp forests. Oikos 116, 1618–1630.
Tracey, S. R., Baulch, T., Hartmann, K., Ling, S. D., Lucieer, V., Marzloff, M. P., et al. (2015). Systematic culling controls a climate driven, habitat modifying invader. Biol. Invasions 17, 1885–1896. doi: 10.1007/s10530-015-0845-z
Urchinomics (2020). Urchinomics. Available online at: https://www.urchinomics.com/ (accessed January 12, 2020).
Uyà, M., Bulleri, F., Wright, J. T., and Gribben, P. E. (2019). Facilitation of an invader by a native habitat-former increases along interacting gradients of environmental stress. Ecology 101:e02961.
Valdez, S., Zhang, Y. S., van der Heide, T., Vanderklift, M. A., Tarquinio, F., Orth, R. J., et al. (2020). Positive ecological interactions and the success of seagrass restoration. Front. Mar. Sci. 7:91. doi: 10.3389/fmars.2020.00091
van Oppen, M. J. H., Oliver, J. K., Putnam, H. M., and Gates, R. D. (2015). Building coral reef resilience through assisted evolution. Proc. Natl. Acad. Sci. U.S.A. 112, 2307–2313.
VanBlaricom, G. R., Belting, T. F., and Triggs, L. H. (2015). “Sea otters in captivity: applications and implications of husbandry development, public display, scientific research and management, and rescue and rehabilitation for sea otter conservation,” in Sea Otter Conservation, eds S. E. Larson, J. L. Bodkin, and G. R. VanBlaricom (Amsterdam: Elsevier), 197–234.
Vasquez, J. A., and McPeak, R. H. (1998). A new tool for kelp restoration. Calif. Fish Game 84, 149–158.
Vásquez, J. A., Zuñiga, S., Tala, F., Piaget, N., Rodríguez, D. C., and Vega, J. M. A. (2014). Economic valuation of kelp forests in northern Chile: values of goods and services of the ecosystem. J. Appl. Phycol. 26, 1081–1088.
Verdura, J., Sales, M., Ballesteros, E., Cefalì, M. E., and Cebrian, E. (2018). Restoration of a canopy-forming alga based on recruitment enhancement: methods and long-term success assessment. Front. Plant Sci. 9:1832. doi: 10.3389/fpls.2018.01832
Vergés, A., McCosker, E., Mayer-Pinto, M., Coleman, M. A., Wernberg, T., Ainsworth, T., et al. (2019). Tropicalisation of temperate reefs: implications for ecosystem functions and management actions. Funct. Ecol. 33, 1000–1013. doi: 10.1111/1365-2435.13310
Vergés, A., Tomas, F., Cebrian, E., Ballesteros, E., Kizilkaya, Z., Dendrinos, P., et al. (2014). Tropical rabbitfish and the deforestation of a warming temperate sea. J. Ecol. 102, 1518–1527.
Watson, J., and Estes, J. A. (2011). Stability, resilience, and phase shifts in rocky subtidal communities along the west coast of Vancouver Island. Canada. Ecol. Monogr. 81, 215–239.
Watson, J. E. M., Dudley, N., Segan, D. B., and Hockings, M. (2014). The performance and potential of protected areas. Nature 515, 67–73.
Wernberg, T., Coleman, M. A., Bennett, S., Thomsen, M. S., Tuya, F., and Kelaher, B. P. (2018). Genetic diversity and kelp forest vulnerability to climatic stress. Sci. Rep. 8, 1–8.
Wernberg, T., and Filbee-Dexter, K. (2019). Missing the marine forest for the trees. Mar. Ecol. Prog. Ser. 612, 209–215.
Wernberg, T., Kendrick, G. A., and Toohey, B. D. (2005). Modification of the physical environment by an Ecklonia radiata (Laminariales) canopy and implications for associated foliose algae. Aquat. Ecol. 39, 419–430.
Wernberg, T., Thomsen, M. S., Tuya, F., and Kendrick, G. A. (2011). Biogenic habitat structure of seaweeds change along a latitudinal gradient in ocean temperature. J. Exp. Mar. Bio. Ecol. 400, 264–271.
Wernberg, T., Krumhansl, K., Filbee-Dexter, K., and Pedersen, M. F. (2019). “Status and trends for the world’s kelp forests,” in World Seas: An Environmental Evaluation, ed. C. Sheppard (Amsterdam: Elsevier), 57–78.
Whitham, T. G., Bailey, J. K., Schweitzer, J. A., Shuster, S. M., Bangert, R. K., LeRoy, C. J., et al. (2006). A framework for community and ecosystem genetics: from genes to ecosystems. Nat. Rev. Genet. 7, 510–523.
Wilson, K. C., and North, W. J. (1983). A review of kelp bed management in southern California. J. World Maric. Soc. 14, 345–359.
Wood, G., Marzinelli, E. M., Coleman, M. A., Campbell, A. H., Santini, N. S., Kajlich, L., et al. (2019). Restoring subtidal marine macrophytes in the Anthropocene: trajectories and future-proofing. Mar. Freshw. Res. 70, 936–951.
Wood, W. F. (1987). Effect of solar ultra-violet radiation on the kelp Ecklonia radiata. Mar. Biol. 96, 143–150.
Wood, G., Marzinelli, E. M., Vergés, A., Campbell, A. H., Steinberg, P. D., and Coleman, M. A. (2020). Using genomics to design and evaluate the performance of underwater forest restoration. J. App. Ecol. 1–11. doi: 10.1111/1365-2664.13707
Woodcock, P., O’Leary, B. C., Kaiser, M. J., and Pullin, A. S. (2017). Your evidence or mine? Systematic evaluation of reviews of marine protected area effectiveness. Fish Fish. 18, 668–681.
Keywords: kelp, restoration, positive species interactions, facilitation, synergy
Citation: Eger AM, Marzinelli E, Gribben P, Johnson CR, Layton C, Steinberg PD, Wood G, Silliman BR and Vergés A (2020) Playing to the Positives: Using Synergies to Enhance Kelp Forest Restoration. Front. Mar. Sci. 7:544. doi: 10.3389/fmars.2020.00544
Received: 15 February 2020; Accepted: 15 June 2020;
Published: 10 July 2020.
Edited by:
Rochelle Diane Seitz, College of William & Mary, United StatesReviewed by:
Heather D. Penney, Memorial University of Newfoundland, CanadaStein Fredriksen, University of Oslo, Norway
Copyright © 2020 Eger, Marzinelli, Gribben, Johnson, Layton, Steinberg, Wood, Silliman and Vergés. This is an open-access article distributed under the terms of the Creative Commons Attribution License (CC BY). The use, distribution or reproduction in other forums is permitted, provided the original author(s) and the copyright owner(s) are credited and that the original publication in this journal is cited, in accordance with accepted academic practice. No use, distribution or reproduction is permitted which does not comply with these terms.
*Correspondence: Aaron M. Eger, aaron.eger@unsw.edu.au