- 1Frontiers Science Center for Deep Ocean Multispheres and Earth System, and Key Laboratory of Marine Chemistry Theory and Technology, Ministry of Education/Institute for Advanced Ocean Studies, Ocean University of China, Qingdao, China
- 2Laboratory for Marine Ecology and Environmental Science, Qingdao National Laboratory for Marine Science and Technology, Qingdao, China
- 3Department of Earth Sciences, Geological Institute, ETH Zürich, Zurich, Switzerland
- 4Biogeochemistry Program, Research Institute for Marine Resources Utilization, Japan Agency for Marine-Earth Science and Technology, Yokosuka, Japan
Natural abundance radiocarbon (14C) is an increasingly widely used tool for investigating the organic carbon (OC) cycle in the contemporary ocean. Recent studies have provided extensive information on the 14C characteristics of organic matter (OM) in sinking particles and sediments in the East Asian Seas including studies from the Bohai Sea, Yellow Sea, East China Sea, South China Sea, Japan Sea, and Japan Trench. 14C investigations have provided insights into biogeochemical processes controlling the fate of sedimentary OM in these settings. Here, we highlight these insights from oceanic landscapes stretching across deltas, shelves, abyssal oceans, and the hadal zones of the East Asian Seas; share our perspectives on the source-to-sink dynamics of sedimentary OM in the ocean; and outline the challenges that need to be faced to make the most out of interpreting 14C signals in sedimentary OC.
Introduction
The net amount of carbon that is reduced and buried in the form of sedimentary organic carbon (OC) is one key flux controlling global biogeochemical cycles over geologic timescales (Berner, 1990). The journey begins with fixation by autotrophs incorporating the radiocarbon (14C) signature of their sources. This carbon propagates through the food web until it is respired or enters non-living pools of marine OC, which are subject to 14C radioactive decay. Expressed as a large spread in 14C patterns in the ocean, sedimentary OC contains a spectrum of pools at varying states of (radioactive) decay (McNichol and Aluwihare, 2007). The cosmogenic origin and 14C decay provide insight into the timescales of source-to-sink carbon dynamics and the response of the carbon cycle to perturbations (Eglinton and Repeta, 2014). Natural abundance 14C has established itself as a cornerstone for assessing sources and processes governing the fate of sedimentary OC in the oceans (Williams et al., 1992; Wang et al., 1996; Eglinton et al., 1997; Masiello and Druffel, 1998; Drenzek et al., 2009; Griffith et al., 2010). Recent technical developments in accelerator mass spectrometry reducing sample size requirements (Synal et al., 2007; Xu et al., 2016; Yamane et al., 2019) and increasing throughput by interfacing with direct CO2 intake systems by elemental analyzers (McIntyre et al., 2017) or gas bench-type systems (Wacker et al., 2013) are poised to continue this progress.
In marine environments, interpretations of 14C in sedimentary OC often require going beyond a straightforward chronological approach and are convoluted owing to a series of factors. Across the vast expanses of oceanic landscapes stretching deltas, shelves, abyssal plains, and hadal trenches, “radiocarbonscapes” (here, defined as spatiotemporal variations, characteristics, and patterns of radiocarbon) of sedimentary OC are markedly different, providing insight into the stories that carbon has to tell as it traverses from sedimentary source to sink. The East Asian Seas host natural laboratories characterized by a range of depositional environments from vast shelves spanning hundreds of kilometers to the deepest trenches scarring the face of our planet. To date, extensive 14C datasets exist for these natural laboratories with starkly contrasting underpinning controls, which we divide into four different themes shaping radiocarbonscapes including (A) provenance, (B) hydrodynamic processes, (C) event-driven sedimentation, and (D) organic matter (OM)–mineral interactions. In this contribution, we highlight several OC 14C studies from the East Asian Seas and review these distinct radiocarbonscapes from the perspectives of these four themes.
Discussion
Provenance
The East Asian marginal seas receive continentally derived sedimentary OM exported from some of the world’s largest rivers including the Yangtze, Yellow, and Pearl Rivers (Wang et al., 2012; Tao et al., 2015; Wu et al., 2018; Lin et al., 2019). After fixation from the atmosphere, terrigenous OC may undergo long storage times on land, reducing its 14C content. For example, particulate OM from the Yellow River contains a higher percentage of aged OC (Tao et al., 2015, 2016; Yu et al., 2019); hence, the corresponding deltaic area shows lower 14C content than elsewhere in the Bohai Sea (Bao et al., 2016; Figure 1A). Similarly, based on cluster analysis of combined sedimentary OC content and its 14C concentration across the vast expanses of the East China Sea, the radiocarbonscape emanating from the Yangtze River reflects heavy terrestrial influence (Van der Voort et al., 2018). An extreme case of provenance-dominated oceanic radiocarbonscape is exemplified by the export of 14C-free OC of petrogenic origin (i.e., kerogen) (Kao and Liu, 1996; Hilton et al., 2008; Lin et al., 2020). Surrounding Taiwan island, these contributions of petrogenic OC appear as anomalously low 14C signatures in sedimentary OC (Figure 1A; Bao et al., 2016; Zheng et al., 2017). Therefore, low 14C concentrations in sedimentary OC of deltaic and land-proximal settings may reflect a provenance-based effect with the addition of pre-aged sedimentary OM from terrestrial sources to recently synthesized marine OM (see also South China Sea investigation by Mollenhauer et al., 2005).
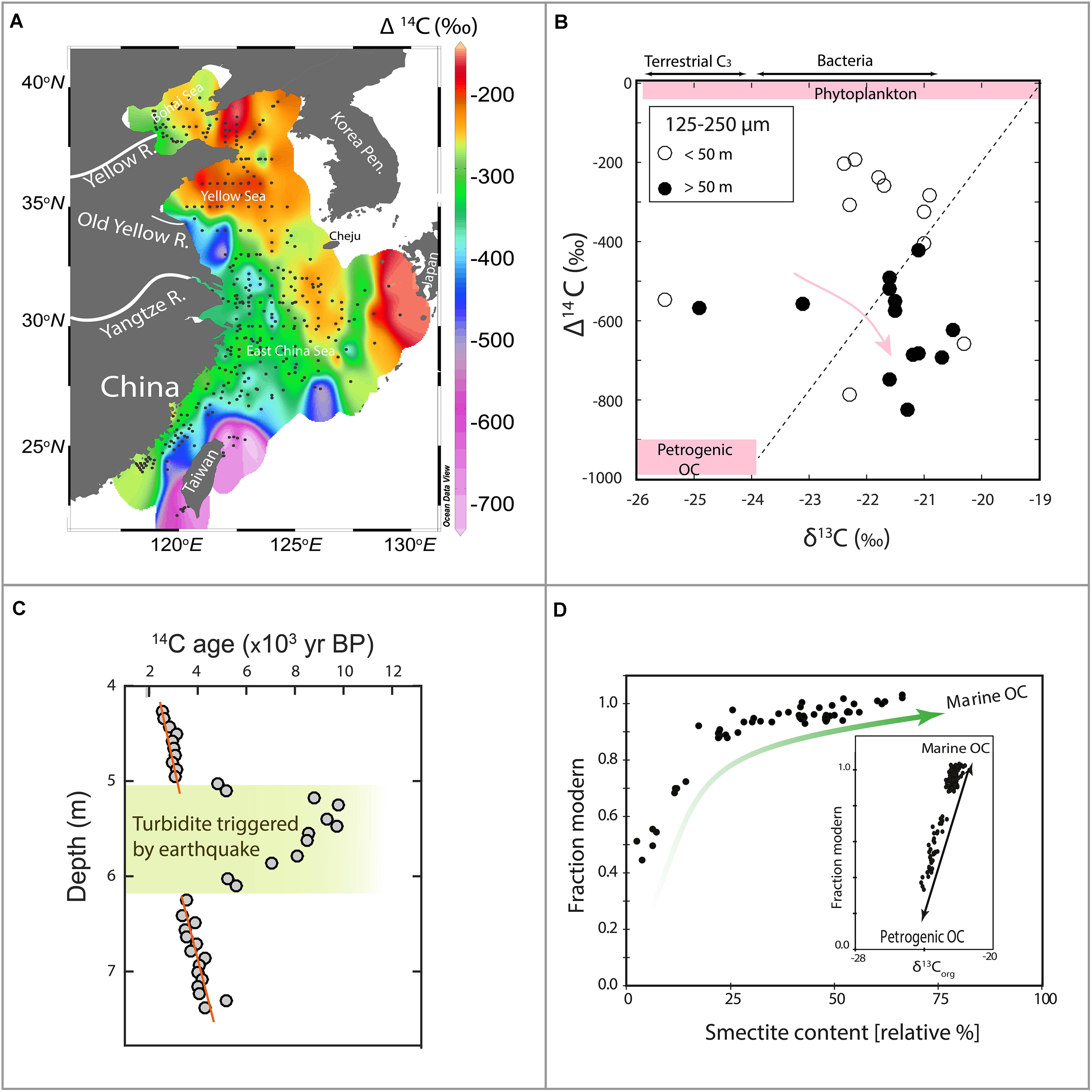
Figure 1. 14C study highlights from East Asian Seas with (A) illustrating the spatial distribution of 14C concentration in sedimentary OC in the Bohai, Yellow, East China Seas (Bao et al., 2016). (B) Shows the 14C concentrations of sedimentary OC in 125–250-μm grain size fractions of surface sediments in the East China Sea, with the dashed line separating the result of mixing of OC sources on the left from the effect of sedimentary reworking on the right (water depth >50 m; Bao et al., 2019a). (C) Illustrates a sedimentary profile in the Japan trench showing the 14C imprint on sedimentary OC in a turbidite deposit triggered by one earthquake (after Bao et al., 2018b). (D) Shows the relationship between 14C content of sedimentary OC and phyllosilicate composition in abyssal South China Sea sinking particles with modern marine OM associated with soil-derived smectite (after Blattmann et al., 2019).
Hydrodynamic Processes
Often, the 14C content of sedimentary OC is to a first-order determined by provenance; however, when viewing spatiotemporal patterns in radiocarbon contents of sedimentary OC (Figure 1A), it is apparent that highly variable 14C contents in distal shelf settings are prevalent. Sedimentary redistribution processes (e.g., resuspension and dispersal) affect particles with different hydrodynamic properties differently, resulting in these highly variable patterns. Thus, there is size-dependent redistribution, as well as lateral transport time, recorded by the 14C “clock,” leading to a highly variable radiocarbonscape dependent on transport pathways (Bao et al., 2018c). Resuspension remobilizes aged sedimentary OM associated especially with intermediate grain size fractions in shallow inner-shelf settings, whereas in deeper regions and erosional areas, bedload transport exerts the strongest influence on redistribution of aged sedimentary OM, especially for the coarser fractions (Bao et al., 2016; Figure 1B). The aged sedimentary OM spreads into deep-sea settings that receive sedimentary input from these areas. In the East Asian Seas, based on 14C analyses of specific compounds and OM thermal decomposition windows on specific grain size fractions, lateral transport of sedimentary OM over millennial year timescales is evident across hundreds of kilometers’ distance in the Bohai, Yellow Sea, and East China Sea (Bao et al., 2018c,2019a,b). Such contributions of aged sedimentary OM are also apparent in deeper waters adjacent to and receiving detrital input from East Asian shelves, such as for aged suspended sedimentary OM arriving in the Okinawa trough (Honda et al., 2000). Laterally derived aged suspended sedimentary OM is also observed in the lower water column of the Japan Sea (Kim et al., 2017, 2020). Hydrodynamic processes redistribute sedimentary OM with aged 14C signatures, thereby overprinting 14C of OC in surface sediments into which redeposition takes place leading to considerable spatial variability in radiocarbonscapes and uncertainty in 14C age-related information.
Event-Driven Sedimentation
Mass wasting events such as those triggered by earthquakes and typhoons mobilize enormous pulses of sediments into the deep sea (Carter et al., 2012; Kioka et al., 2019), carrying with it vast amounts of OM (Tsai et al., 2010; Liu et al., 2013). The heavily incised active margin shelves along the Japan Trench offer little accommodation space for sediment storage, facilitating landslides triggered by tectonic events. This old sedimentary OM, which is mobilized, a consequence of protracted storage in intermediate reservoirs on land (e.g., soils) and/or on the continental margin, strongly influences the 14C contents of OC in the hadal zone such as the Japan Trench (∼8,000-m water depth; Bao et al., 2018b). Such events blanket the seafloor with turbidites containing aged OM (Figure 1C; see also Nakamura et al., 1990). Similarly, within the Gaoping canyon, directly south of Taiwan, event-driven sedimentary inputs of terrestrial OC to the ocean are common. In the case of typhoons, hyperpycnal flow conditions directly export large contributions of land-derived biospheric and petrogenic OC into the South China Sea via submarine canyons (Hilton et al., 2008; Kao et al., 2014; Zheng et al., 2017; Lin et al., 2020). Over hundreds of kilometers’ distance, sedimentary OC is carried by turbidites (Zhang et al., 2018) or entrained in eddies (Zhang et al., 2014), reflecting an event-driven overlay observed over time series of sinking particles (Blattmann et al., 2018). Therefore, these episodic aged or even petrogenic OM exports, triggered by events such as earthquakes and typhoons, exert large-scale control on radiocarbonscapes in the deep ocean.
OM–Mineral Interactions
Mineral ballast exerts key control over the sedimentary transport and deposition of OM in aquatic environments (Ittekkot et al., 1990; Wakeham et al., 2009). Investigations have revealed the association of marine OM with lithogenic minerals (Keil et al., 1997; Kennedy and Wagner, 2011), which is also observed in the sinking particles of the South China Sea, which impart a strong effect on 14C contents of sedimentary OC (Blattmann et al., 2018). The systematic radiocarbonscapes with contrasting modern and ancient forms of sedimentary OM (see insert in Figure 1D), representing marine and petrogenic OM forms, respectively, display strong relationships with mineralogical composition, revealing mineral-specific behavior on the retention and release of terrestrial OM in the marine environment by loss-and-replacement reactions (Figure 1D; Blattmann et al., 2019). Smectite, a pedogenic mineral, loses its association with pedogenic OM and associates with marine OM in distal marine settings. By way of this mechanism, sedimentary OM sourced from land is desorbed and/or degraded, leaving marine OM with a straightforward source-to-sink trajectory to repopulate the particulate phase (c.f., Zhang et al., 2019). Organic matter–mineral interactions impart a systematic effect on the fate of sedimentary OM in the ocean and govern the type of OM that is stabilized, thereby changing the overall radiocarbonscape.
Synthesis and Perspectives
14C is a one-dimensional value traditionally used to express age based on its decay constant (Libby et al., 1949). Rather than 14C expressing a single property (i.e., age, which is commonly assumed), sedimentary OC in oceanic settings integrates an overlay of processes, which contribute to an overall radiocarbonscape. Delineating the manifold processes involved given the scalar nature of this measurement often arrives at a non-unique set of solutions. Toward resolving these ambiguities by extending 14C into multidimensional space, sedimentary OM can be dissected into different physical fractions [e.g., density (Wakeham et al., 2009); grain size (Bao et al., 2019a), and hydrodynamic (Coppola et al., 2007)], reactivity pools [e.g., chemical oxidation (Ohkouchi and Eglinton, 2006), thermal treatment (Hemingway et al., 2019; Bao et al., 2018a)], and individual compounds that can be targeted (e.g., Eglinton et al., 1997; Tao et al., 2016). Based on the evidence accumulated thus far, the “radiocarbonscapes” of the East Asian Seas are controlled by a combination of (A) provenance, (B) hydrodynamics, (C) event-driven sedimentation, (D) OM–mineral interactions, and/or other potentially important and less-well understood processes. 14C is a tracer that intersects with all of these themes, and it is apparent that we need to continue our efforts to deconvolve the complex tapestry of radiocarbonscapes in the marine environment to resolve basic questions including the following:
1. How do the four themes provenance, hydrodynamics, event-driven sedimentation, and OM–mineral interactions weigh against each other in shaping radiocarbonscapes?
2. How can quantitative information on these four themes be extracted from radiocarbonscapes?
Based on the East Asian Sea natural laboratories, we have developed our own perspectives toward answering these questions and judged the relative influences of the four themes on seafloor radiocarbonscapes (Figure 2). It appears that the relative importance of these four themes varies greatly across delta to deep-sea transects for active and passive margin settings (c.f., Blair and Aller, 2012), with provenance-based influence strongest in deltas and more diffuse across passive margins with hydrodynamic processes, leading to extensive imprints on radiocarbonscapes on wide, high-energy shelf settings. In contrast, event-driven sedimentation markedly impacts radiocarbonscapes across active margin settings where episodic mobilization and redistribution of vast amounts of sediment occur along with a pronounced inheritance of a provenance signal. With their areas of greatest influence difficult to pinpoint, OM–mineral interactions control loss and replacement of terrestrial with marine OM, thereby rewriting the organic geochemical fingerprint of sedimentary OM. Based on individual case studies of the East Asian Seas natural laboratories, the operation of these four themes crystallizes out of radiocarbonscape patterns. However, quantitative approaches for disentangling radiocarbonscapes are needed where these four themes are more intertwined, ultimately key for understanding marine biogeochemical cycles.
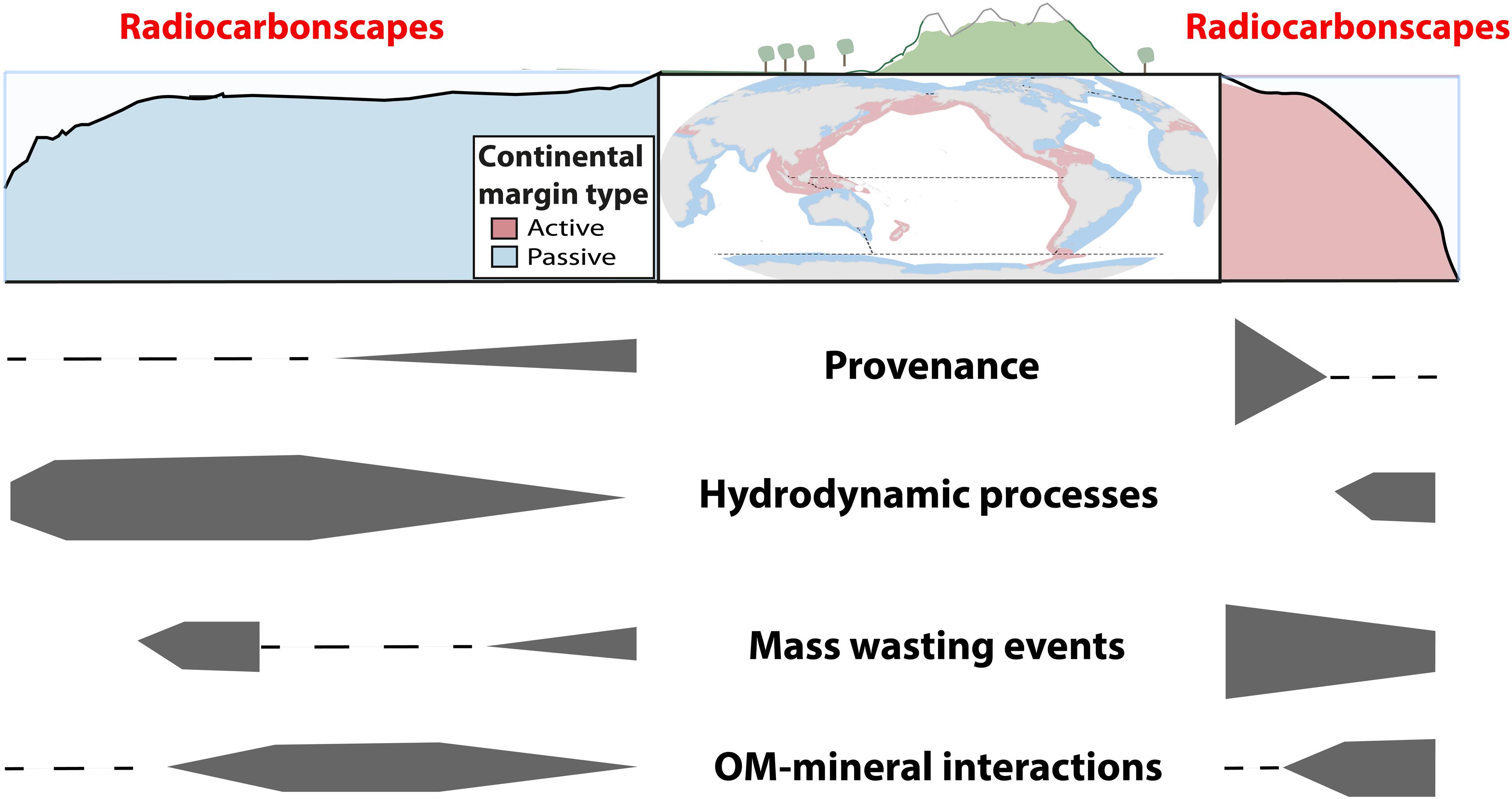
Figure 2. Radiocarbonscapes of sedimentary OC are influenced by several underpinning controls, with their relative influence represented by the thickness of the tapered bars across source-to-sink sediment pathways as hypothesized by the authors. Top panel is modified from Bao et al. (2018d) and Blair and Aller (2012).
Author Contributions
RB and TB contributed equally to discussion and writing. Both authors contributed to the article and approved the submitted version.
Funding
The authors acknowledge funding from Stockholm University, Harvard University, JAMSTEC, Ocean University of China (No. 862001013124), and ETH Zürich. Additional financial support from the Hanse-Wissenschaftskolleg, the Geochemical Society, and the Deutsche Forschungsgemeinschaft (DFG, German Research Foundation) project number 422798570 made this publication possible.
Conflict of Interest
The authors declare that the research was conducted in the absence of any commercial or financial relationships that could be construed as a potential conflict of interest.
The reviewer AM declared a past co-authorship with one of the authors RB to the handling editor.
Acknowledgments
The authors are deeply indebted to Timothy Eglinton for his many years of tutelage and gracious support and we are thankful for the stimulating and dedicated reviews we received from GM and AM that have greatly improved this contribution. We thank the organizers and attendees of the Marine Organic Geochemistry workshop held in Delmenhorst, Germany for inspiring discussions.
References
Bao, R., Blattmann, T. M., McIntyre, C., Zhao, M., and Eglinton, T. I. (2019a). Relationships between grain size and organic carbon 14C heterogeneity in continental margin sediments. Earth Planet. Sci. Lett. 505, 76–85. doi: 10.1016/j.epsl.2018.10.013
Bao, R., Zhao, M., McNichol, A., Galy, V., McIntyre, C., Haghipour, N., et al. (2019b). Temporal constraints on lateral organic matter transport along a coastal mud belt. Organic Geochem. 128, 86–93. doi: 10.1016/j.orggeochem.2019.01.007
Bao, R., McIntyre, C., Zhao, M., Zhu, C., Kao, S.-J., and Eglinton, T. I. (2016). Widespread dispersal and aging of organic carbon in shallow marginal seas. Geology 44, 791–794. doi: 10.1130/g37948.1
Bao, R., McNichol, A. P., Hemingway, J. D., Lardie Gaylord, M. C., and Eglinton, T. I. (2018a). Influence of different acid treatments on the radiocarbon content spectrum of sedimentary organic matter determined by rpo/accelerator mass spectrometry. Radiocarbon 61, 395–413. doi: 10.1017/rdc.2018.125
Bao, R., Strasser, M., McNichol, A. P., Haghipour, N., McIntyre, C., Wefer, G., et al. (2018b). Tectonically-triggered sediment and carbon export to the Hadal zone. Nat. Commun. 9:121. doi: 10.1038/s41467-017-02504-1
Bao, R., Uchida, M., Zhao, M., Haghipour, N., Montlucon, D., McNichol, A., et al. (2018c). Organic carbon aging during across-shelf transport. Geophys. Res. Lett. 45, 8425–8434. doi: 10.1029/2018gl078904
Bao, R., van der Voort, T. S., Zhao, M., Guo, X., Montluçon, D. B., McIntyre, C., et al. (2018d). Influence of hydrodynamic processes on the fate of sedimentary organic matter on continental margins. Global Biogeochem. Cycles 32, 1420–1432. doi: 10.1029/2018gb005921
Berner, R. A. (1990). Atmospheric carbon dioxide levels over phanerozoic time. Science 249, 1382–1386. doi: 10.1126/science.249.4975.1382
Blair, N. E., and Aller, R. C. (2012). The fate of terrestrial organic carbon in the marine environment. Annu. Rev. Mar. Sci. 4, 401–423. doi: 10.1146/annurev-marine-120709-142717
Blattmann, T. M., Liu, Z., Zhang, Y., Zhao, Y., Haghipour, N., Montluçon, D. B., et al. (2019). Mineralogical control on the fate of continentally derived organic matter in the ocean. Science 366, 742–745. doi: 10.1126/science.aax5345
Blattmann, T. M., Zhang, Y., Zhao, Y., Wen, K., Lin, S., Li, J., et al. (2018). Contrasting fates of petrogenic and biospheric carbon in the South China Sea. Geophys. Res. Lett. 45, 9077–9086. doi: 10.1029/2018gl079222
Carter, L., Milliman, J. D., Talling, P. J., Gavey, R., and Wynn, R. B. (2012). Near-synchronous and delayed initiation of long run-out submarine sediment flows from a record-breaking river flood, offshore Taiwan. Geophys. Res. Lett. 39:L12603. doi: 10.1029/2012GL051172
Coppola, L., Gustafsson, Ö, Andersson, P., Eglinton, T. I., Uchida, M., and Dickens, A. F. (2007). The importance of ultrafine particles as a control on the distribution of organic carbon in Washington Margin and Cascadia Basin sediments. Chem. Geol. 243, 142–156. doi: 10.1016/j.chemgeo.2007.05.020
Drenzek, N. J., Hughen, K. A., Montluçon, D. B., Southon, J. R., dos Santos, G. M., Druffel, E. R., et al. (2009). A new look at old carbon in active margin sediments. Geology 37, 239–242. doi: 10.1130/g25351a.1
Eglinton, T. I., Benitez-Nelson, B. C., Pearson, A., McNichol, A. P., Bauer, J. E., and Druffel, E. R. M. (1997). Variability in radiocarbon ages of individual organic compounds from marine sediments. Science 277, 796–799. doi: 10.1126/science.277.5327.796
Eglinton, T. I., and Repeta, D. J. (2014). “Organic matter in the contemporary ocean,” in Treatise on Geochemistry, eds H. D. Holland and K. K. Turekian (Oxford: Elsevier), 151–189. doi: 10.1016/b978-0-08-095975-7.00606-9
Griffith, D. R., Martin, W. R., and Eglinton, T. I. (2010). The radiocarbon age of organic carbon in marine surface sediments. Geochim. Cosmochim. Acta 74, 6788–6800. doi: 10.1016/j.gca.2010.09.001
Hemingway, J. D., Rothman, D. H., Grant, K. E., Rosengard, S. Z., Eglinton, T. I., Derry, L. A., et al. (2019). Mineral protection regulates long-term global preservation of natural organic carbon. Nature 570, 228–231. doi: 10.1038/s41586-019-1280-6
Hilton, R. G., Galy, A., Hovius, N., Chen, M.-C., Horng, M.-J., and Chen, H. (2008). Tropical-cyclone-driven erosion of the terrestrial biosphere from mountains. Nat. Geosci. 1, 759–762. doi: 10.1038/ngeo333
Honda, M. C., Kusakabe, M., Nakabayashi, S., and Katagiri, M. (2000). Radiocarbon of sediment trap samples from the Okinawa trough: lateral transport of 14C-poor sediment from the continental slope. Mar. Chem. 68, 231–247. doi: 10.1016/s0304-4203(99)00080-8
Ittekkot, V., Haake, B., and Wiesner, M. G. (1990). Organic-mineral interactions in the sea: implications for atmospheric CO2-transfer to the deep ocean. Mitteilun. Geol. Paläontol. Inst. Univ. Hamburg 69, 167–175.
Kao, S.-J., Hilton, R. G., Selvaraj, K., Dai, M., Zehetner, F., Huang, J.-C., et al. (2014). Preservation of terrestrial organic carbon in marine sediments offshore Taiwan: mountain building and atmospheric carbon dioxide sequestration. Earth Surface Dyn. 2, 127–139. doi: 10.5194/esurf-2-127-2014
Kao, S.-J., and Liu, K.-K. (1996). Particulate organic carbon export from a subtropical mountainous river (Lanyang Hsi) in Taiwan. Limnol. Oceanogr. 41, 1749–1757. doi: 10.4319/lo.1996.41.8.1749
Keil, R. G., Mayer, L. M., Quay, P. D., Richey, J. E., and Hedges, J. I. (1997). Loss of organic matter from riverine particles in deltas. Geochim. Cosmochimi. Acta 61, 1507–1511. doi: 10.1016/s0016-7037(97)00044-6
Kennedy, M. J., and Wagner, T. (2011). Clay mineral continental amplifier for marine carbon sequestration in a greenhouse ocean. Proc. Natl. Acad. Sci. U.S.A. 108, 9776–9781. doi: 10.1073/pnas.1018670108
Kim, M., Hwang, J., Rho, T., Lee, T., Kang, D.-J., Chang, K.-I., et al. (2017). Biogeochemical properties of sinking particles in the southwestern part of the East Sea (Japan Sea). J. Mar. Syst. 167, 33–42. doi: 10.1016/j.jmarsys.2016.11.001
Kim, M., Kim, Y.-I., Hwang, J., Choi, K. Y., Kim, C. J., Ryu, Y., et al. (2020). Influence of sediment resuspension on the biological pump of the Southwestern East Sea (Japan Sea). Front. Earth Sci. 8:144. doi: 10.3389/feart.2020.00144
Kioka, A., Schwestermann, T., Moernaut, J., Ikehara, K., Kanamatsu, T., McHugh, C. M., et al. (2019). Megathrust earthquake drives drastic organic carbon supply to the hadal trench. Sci. Rep. 9:1553. doi: 10.1038/s41598-019-38834-x
Libby, W. F., Anderson, E. C., and Arnold, J. R. (1949). Age determination by radiocarbon content - world-wide assay of natural radiocarbon. Science 109, 227–228. doi: 10.1126/science.109.2827.227
Lin, B., Liu, Z., Eglinton, T. I., Kandasamy, S., Blattmann, T. M., Haghipour, N., et al. (2019). Perspectives on provenance and alteration of suspended and sedimentary organic matter in the subtropical Pearl River system. South China. Geochim. Cosmochim. Acta 259, 270–287. doi: 10.1016/j.gca.2019.06.018
Lin, B., Liu, Z., Eglinton, T. I., Kandasamy, S., Blattmann, T. M., Haghipour, N., et al. (2020). Island-wide variation in provenance of riverine sedimentary organic carbon: a case study from Taiwan. Earth Planet. Sci. Lett. 539:116238. doi: 10.1016/j.epsl.2020.116238
Liu, J. T., Kao, S.-J., Huh, C.-A., and Hung, C.-C. (2013). gravity flows associated with flood events and carbon burial: taiwan as instructional source area. Annu. Rev. Mar. Sci. 5, 47–68. doi: 10.1146/annurev-marine-121211-172307
Masiello, C. A., and Druffel, E. R. M. (1998). Black carbon in deep-sea sediments. Science 280, 1911–1913. doi: 10.1126/science.280.5371.1911
McIntyre, C. P., Wacker, L., Haghipour, N., Blattmann, T. M., Fahrni, S., Usman, M., et al. (2017). Online 13C and 14C gas measurements by EA-IRMS–AMS at ETH Zürich. Radiocarbon 59, 893–903. doi: 10.1017/rdc.2016.68
McNichol, A. P., and Aluwihare, L. I. (2007). The power of radiocarbon in biogeochemical studies of the marine carbon cycle: Insights from studies of dissolved and particulate organic carbon (DOC and POC). Chem. Rev. 107, 443–466. doi: 10.1021/cr050374g
Mollenhauer, G., Kienast, M., Lamy, F., Meggers, H., Schneider, R. R., Hayes, J. M., et al. (2005). An evaluation of 14C age relationships between co-occurring foraminifera, alkenones, and total organic carbon in continental margin sediments. Paleoceanography 20:A1016. doi: 10.1029/2004PA001103
Nakamura, T., Shiki, T., and Nakai, N. (1990). Variations in 14C ages of various organic fractions in a turbidite sediment core from Suruga Trough. Geochem. J. 24, 47–56. doi: 10.2343/geochemj.24.47
Ohkouchi, N., and Eglinton, T. I. (2006). Radiocarbon constraint on relict organic carbon contributions to Ross Sea sediments. Geochem. Geophys. Geosyst. 7:Q04012. doi: 10.1029/2005GC001097
Synal, H.-A., Stocker, M., and Suter, M. (2007). MICADAS: a new compact radiocarbon AMS system. Nuclear Instrum. Methods Phys. Res. Section BBeam Interact. Mater. Atoms 259, 7–13. doi: 10.1016/j.nimb.2007.01.138
Tao, S., Eglinton, T. I., Montluçon, D. B., McIntyre, C., and Zhao, M. (2015). Pre-aged soil organic carbon as a major component of the Yellow River suspended load: regional significance and global relevance. Earth Planet. Sci. Lett. 414, 77–86. doi: 10.1016/j.epsl.2015.01.004
Tao, S., Eglinton, T. I., Montluçon, D. B., McIntyre, C., and Zhao, M. (2016). Diverse origins and pre-depositional histories of organic matter in contemporary Chinese marginal sea sediments. Geochim. Cosmochim. Acta 191, 70–88. doi: 10.1016/j.gca.2016.07.019
Tsai, F., Hwang, J.-H., Chen, L.-C., and Lin, T.-H. (2010). Post-disaster assessment of landslides in southern Taiwan after 2009 Typhoon Morakot using remote sensing and spatial analysis. Nat. Hazards Earth Syst. Sci. 10, 2179–2190. doi: 10.5194/nhess-10-2179-2010
Van der Voort, T. S., Mannu, U., Blattmann, T. M., Bao, R., Zhao, M., and Eglinton, T. I. (2018). Deconvolving the fate of carbon in coastal sediments. Geophys. Res. Lett. 45, 4134–4142. doi: 10.1029/2018gl077009
Wacker, L., Fahrni, S. M., Hajdas, I., Molnar, M., Synal, H. A., Szidat, S., et al. (2013). A versatile gas interface for routine radiocarbon analysis with a gas ion source. Nuclear Instrum. Methods Phys. Res. Section BBeam Interact. Mater. Atoms 294, 315–319. doi: 10.1016/j.nimb.2012.02.009
Wakeham, S. G., Canuel, E. A., Lerberg, E. J., Mason, P., Sampere, T. P., and Bianchi, T. S. (2009). Partitioning of organic matter in continental margin sediments among density fractions. Mar. Chem. 115, 211–225. doi: 10.1016/j.marchem.2009.08.005
Wang, X., Ma, H., Li, R., Song, Z., and Wu, J. (2012). Seasonal fluxes and source variation of organic carbon transported by two major Chinese Rivers: the yellow river and changjiang (Yangtze) River. Global Biogeochem. Cycles 26:GB2025. doi: 10.1029/2011GB004130
Wang, X.-C., Druffel, E. R. M., and Lee, C. (1996). Radiocarbon in organic compound classes in particulate organic matter and sediment in the deep northeast Pacific Ocean. Geophys. Res. Lett. 23, 3583–3586. doi: 10.1029/96gl03423
Williams, P. M., Robertson, K. J., Soutar, A., Griffin, S. M., and Druffel, E. R. M. (1992). Isotopic signatures (14C, 13C, 15N) as tracers of sources and cycling of soluble and particulate organic matter in the Santa Monica Basin. California. Prog. Oceanogr. 30, 253–290. doi: 10.1016/0079-6611(92)90015-r
Wu, Y., Eglinton, T. I., Zhang, J., and Montlucon, D. B. (2018). Spatiotemporal variation of the quality, origin, and age of particulate organic matter transported by the Yangtze River (Changjiang). J. Geophys. Res. Biogeosci. 123, 2908–2921. doi: 10.1029/2017jg004285
Xu, X., Gao, P., and Salamanca, E. G. (2016). Ultra small-mass graphitization by sealed tube zinc reduction method for AMS 14C Measurements. Radiocarbon 55, 608–616. doi: 10.1017/s0033822200057751
Yamane, M., Yokoyama, Y., Hirabayashi, S., Miyairi, Y., Ohkouchi, N., and Aze, T. (2019). Small- to ultra-small-scale radiocarbon measurements using newly installed single-stage AMS at the University of Tokyo. Nuclear Instrum. Methods Phys. Res. Section BBeam Interact. Mater. Atoms 455, 238–243. doi: 10.1016/j.nimb.2019.01.035
Yu, M., Eglinton, T. I., Haghipour, N., Montluçon, D. B., Wacker, L., Wang, Z., et al. (2019). Molecular isotopic insights into hydrodynamic controls on fluvial suspended particulate organic matter transport. Geochim. Cosmochim. Acta 262, 78–91. doi: 10.1016/j.gca.2019.07.040
Zhang, J., Li, H., Xuan, J., Wu, Z., Yang, Z., Wiesner, M. G., et al. (2019). Enhancement of mesopelagic sinking particle fluxes due to upwelling, aerosol deposition, and monsoonal influences in the northwestern South China Sea. J. Geophys. Res. Oceans 124, 99–112. doi: 10.1029/2018jc014704
Zhang, Y., Liu, Z., Zhao, Y., Colin, C., Zhang, X., Wang, M., et al. (2018). Long-term in situ observations on typhoon-triggered turbidity currents in the deep sea. Geology 46, 675–678. doi: 10.1130/g45178.1
Zhang, Y., Liu, Z., Zhao, Y., Wang, W., Li, J., and Xu, J. (2014). Mesoscale eddies transport deep-sea sediments. Sci. Rep. 4:5937. doi: 10.1038/srep05937
Zheng, L.-W., Ding, X., Liu, J. T., Li, D., Lee, T.-Y., Zheng, X., et al. (2017). Isotopic evidence for the influence of typhoons and submarine canyons on the sourcing and transport behavior of biospheric organic carbon to the deep sea. Earth Planet. Sci. Lett. 465, 103–111. doi: 10.1016/j.epsl.2017.02.037
Keywords: 14C, sediment, source-to-sink, shelf, margin, abyssal, hadal, turbidite
Citation: Bao R and Blattmann TM (2020) Radiocarbonscapes of Sedimentary Organic Carbon in the East Asian Seas. Front. Mar. Sci. 7:517. doi: 10.3389/fmars.2020.00517
Received: 13 January 2020; Accepted: 05 June 2020;
Published: 14 July 2020.
Edited by:
Cindy Lee, Stony Brook University, United StatesReviewed by:
Ann P. McNichol, Woods Hole Oceanographic Institution, United StatesGesine Mollenhauer, Alfred Wegener Institute, Helmholtz Center for Polar and Marine Research, Germany
Copyright © 2020 Bao and Blattmann. This is an open-access article distributed under the terms of the Creative Commons Attribution License (CC BY). The use, distribution or reproduction in other forums is permitted, provided the original author(s) and the copyright owner(s) are credited and that the original publication in this journal is cited, in accordance with accepted academic practice. No use, distribution or reproduction is permitted which does not comply with these terms.
*Correspondence: Rui Bao, baorui@ouc.edu.cn; Thomas M. Blattmann, blattmannt@jamstec.go.jp