- 1Plymouth Marine Laboratory, Plymouth, United Kingdom
- 2Australian Antarctic Division, Department of Agriculture, Water and the Environment, Kingston, TAS, Australia
- 3Australian Antarctic Program Partnership, University of Tasmania, Hobart, TAS, Australia
- 4British Antarctic Survey, Natural Environmental Research Council, Cambridge, United Kingdom
- 5National Oceanography Centre Southampton, Southampton, United Kingdom
- 6National Oceanography Centre Southampton, University of Southampton, Southampton, United Kingdom
Antarctic krill inhabit areas of the Southern Ocean that can exceed 4.0°C, yet they preferentially inhabit regions with temperatures of −1.5 to ≤1.5°C. Successful embryonic development and hatching are key to their life cycle, but despite the rapid climatic warming seen across their main spawning areas, the effects of elevated temperatures on embryogenesis, hatching success, and nauplii malformations are unknown. We incubated 24,483 krill embryos in two independent experiments to investigate the hypothesis that temperatures exceeding 1.5°C have a negative impact on hatching success and increase the numbers of malformed nauplii. Field experiments were on krill collected from near the northern, warm limit of their range and embryos incubated soon after capture, while laboratory experiments were on embryos from krill acclimated to laboratory conditions. The hatching success of embryo batches varied enormously, from 0 to 98% (mean 27%). Both field and laboratory experiments showed that hatching success decreased markedly above 3.0°C. Our field experiments also showed an approximate doubling of the percentage of malformed nauplii at elevated temperatures, reaching 50% at 5.0°C. At 3.0°C or below, however, temperature was not the main factor driving the large variation in embryo hatching success. Our observations of highly variable and often low success of hatching to healthy nauplii suggest that indices of reproductive potential of female krill relate poorly to the subsequent production of viable krill larvae and may help to explain spatial discrepancies between the distribution of the spawning stock and larval distribution.
Introduction
The success or failure of the early life stages is a key part of species’ life cycles. For broadcast spawners, embryonic success is reliant upon internal reserves, parasitism, predation, and the physical environment. In marine crustacean species, some embryonic stages are very sensitive to environmental conditions, specifically temperature (García-Guerrero et al., 2003). High temperatures have been found to cause higher metabolic rates, lower growth and survival rates, and greater numbers of larval malformations in crustacean embryos (Rhodes, 1981; Jones, 1995; Naylor et al., 1999; Kumlu et al., 2000; Paula et al., 2001; Manush et al., 2006). All of these responses to temperatures can lead to reduced embryonic success, higher levels of nauplii failure, and lower recruitment to the larval population. Identifying the relative importance of temperature on embryonic development, success, and nauplii malformations will lead to development of more accurate population dynamic models on both spatial and temporal scales.
Antarctic krill (Euphausia superba), hereafter “krill,” are an ecologically and commercially valuable stenothermal crustacean species in the Southern Ocean where they inhabit a temperature range of −2.0°C to 4.0°C (Tarling et al., 2006; Cuzin-Roudy et al., 2014). Within this 6.0°C temperature envelope, krill are considered to preferentially inhabit regions with temperatures between −1.5°C and ≤1.5°C (Cuzin-Roudy et al., 2014; Perry et al., 2019). Experimental results by Atkinson et al. (2006) show that adult krill growth rates peak at 0.5°C and decrease rapidly to zero above 3.0°C. Yet, the upper temperature tolerance of krill embryos has never been defined. Anecdotal evidence published by George and Stromberg (1985) stated that an incubation temperature of 4.0°C caused complete embryonic failure but their finding requires further investigation. The lower temperature tolerance of krill embryos was more exhaustively tested by Yoshida et al. (2004) who recorded successful hatching down to −2.1°C. Krill embryos are negatively buoyant, and once spawned, they sink until they hatch as nauplii (Fraser, 1936; Marr, 1962). Throughout their roughly 6-day descent, embryos experience a range of temperature conditions that affect water density and hence sinking rate (Ross et al., 1988). The variation in temperature during this descent also affects the speed at which they develop (Marschall, 1983; George, 1984; Ross et al., 1988; Yoshida et al., 2004).
The south-west Atlantic sector of the Southern Ocean is key for krill, being the region with the highest krill densities, where the fishery operates and where the population extends to its warmest thermal limit around the island of South Georgia (Atkinson et al., 2008). Surface water temperatures around South Georgia can reach over 4.0°C, yet its waters contain high densities of sexually mature adult krill that support the fishery (Marr, 1962; Perry et al., 2019). This adult population does not, however, translate to high larval recruitment as expected (Ward et al., 1990; Tarling et al., 2007; Siegel and Wakins, 2016). Suggested reasons for this lack of larval recruitment include the area being unsuitable for spawning, predation on larvae, and larval export during the winter (Ruud, 1932; Tarling et al., 2007). However, the effect of temperature on embryonic development and larval recruitment around South Georgia has not been examined. A wider understanding of the factors that modulate krill embryogenesis and hatching success will allow us to better understand, model, and predict their population distribution and dynamics (Kawaguchi, 2016).
We tested the hypothesis that temperatures approaching the warm distributional limit have a negative impact on egg hatching success and naupliar development. To investigate this, we ran two independent incubation experiments. In one, we incubated embryos from gravid females collected and immediately incubated from around South Georgia and the South Sandwich Islands. In the other, we incubated embryos from females that had been collected and then laboratory acclimated at the aquarium of the Australian Antarctic Division in Tasmania.
Materials and Methods
Overview
For this study, we incubated 24,483 embryos from 20 females across six temperature treatments. These 20 females were collected from two sources. Fourteen gravid females were collected from a field expedition and incubated for short periods at sea to harvest their eggs; experiments carried out on these embryos will hereafter be referred to as field experiments. Embryos from field females were incubated at 0.5, 3.0, and 5.0°C. The remaining six females had been originally collected from the field and were subsequently maintained in controlled conditions for a minimum of 10 months and re-matured in captivity. These are hereafter referred to as laboratory experiments. Embryos from laboratory females were incubated at 0.0, 1.0, 3.0, 5.0, and 7.0°C. Differences in the range of set temperature between our field and laboratory experiments were a result of the different amounts of space and resources available in each context.
Embryos From Our Field Experiments
Sampling Method and Collection of Embryos
Krill were collected from the Scotia Sea using a Rectangular Midwater Trawl with an effective mouth area of 8 m2 net (RMT8), or an RMT 1 + 8 (Baker et al., 1973) on board the RRV Discovery during January and February 2019 (Figure 1). Within 30 min of net recovery, undamaged gravid females were relocated to stable environmental conditions similar to those described in Tarling et al. (2006). Gravid females were transferred individually into perforated 500 ml lidded containers with a 1-mm mesh placed 25 mm from the bottom to allow spawned embryos to sink to the bottom to avoid cannibalism (Ross and Quetin, 1983). These containers were then stacked up to six high inside 9 cm diameter perforated pipes within a 320 L aquarium. Unfiltered ambient temperature seawater, at a flow of 20 L per minute from the ship’s non-toxic intake at 5 m depth, was passed through the aquarium to provide the females with a constant turnover of fresh oxygenated seawater and any available plankton. We did not provide any supplementary food to the females. Water temperature of the aquarium was monitored frequently to ensure it aligned with ambient ocean conditions. Temperature profiles from both South Georgia and the South Sandwich Islands are shown in Figure 1. A cover was placed over the top of the aquarium so that krill were kept in subdued light conditions except for routine maintenance checks.
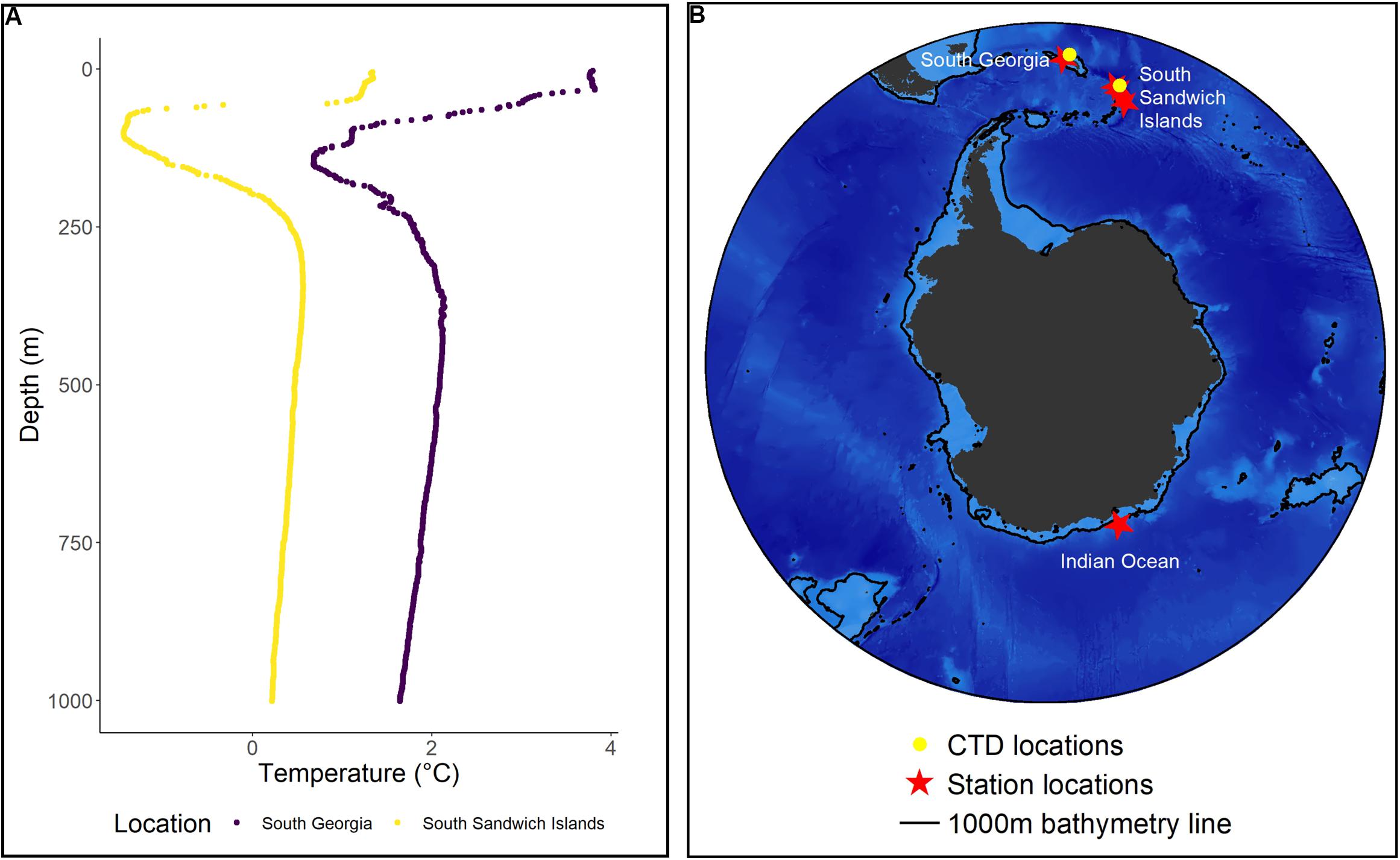
Figure 1. Sample locations for field and laboratory experiments as well as temperature details from the field experiments. (A) Temperature profiles collected from CTD casts at South Georgia and the South Sandwich Islands during the cruise on which the field experiments were undertaken. (B) The station locations at which gravid females were captured for our field and laboratory experiments. Field samples were collected from the Atlantic sector. Laboratory samples were collected from the Indian Ocean sector of the Southern Ocean.
Krill were checked every 12 h (12:00 and 00:00 local time) for embryos, until they had spawned. All females spawned within 7 days of being placed in the aquarium. An embryo batch refers to a complete spawn from a single female that was consequently used for experimentation. No more than a single batch was used per female. All egg batches were checked for fertilization which was determined by looking for the formation of fertilization jelly (Jia et al., 2014). All egg batches checked were fertile and were progressed to the next stage of the experiment. Each of the females, from which embryo batches were taken, was weighed and measured (AT = from anterior edge of eye to tip of telson) (Morris et al., 1988) then frozen at −80°C with any remaining embryos from the batch.
From fertilized embryo batches (of >1000), ∼30 embryos were immediately fixed in 4% formalin as an initial (T0) sample and the remaining embryos were progressed to the experimental stage. Embryo batches from 14 different females were collected from five different stations split between two main locations (South Georgia and the South Sandwich Islands). Of these 14 females, four originated from South Georgia and 10 were from the South Sandwich Islands (Table 1).
Experimental Method
For each of our 14 embryo batches, we investigated embryonic development at three different temperature treatments (Supplementary Figure S1). This allowed hatching success between females to be examined in addition to change caused by temperature. Individual embryo batches (i.e., embryos from a single female) were immediately randomly divided into nine 250 ml bottles, each containing ∼60 embryos. The 250 ml bottles were prepared with 0.45 μm filtered seawater. All seawater was pH tested at this stage of the experiment, but a malfunction resulted in the readings being inaccurate so pH levels are not reported for these experiments. Three bottles from each batch were placed in scientific fridges at each of our treatment temperatures. Fridges maintained temperatures within ±0.5°C of set temperatures. We used three temperature treatments for our field experiments. Our lowest temperature was 0.5°C, representing the temperature optimum for post-larval growth (Atkinson et al., 2006). The other treatment temperatures were 3.0 and 5.0°C chosen to be either side of 4.0°C that George and Stromberg (1985) suggested to be the point of embryonic failure.
Every 24 h, ∼30 embryos were removed from each experimental temperature and fixed using 4% formaldehyde solution. Each bottle started with ∼60 embryos such that each bottle contained 2 days’ worth of embryos. Experiments lasted for 6 days (Ross et al., 1988; Yoshida et al., 2004). Oxygen consumption of 60 krill embryos for 6 days is predicted to be 15–30 μl (Ikeda, 1984; Yoshida et al., 2011). Considering that the oxygen saturation level in seawater would be 2.5 ml of oxygen per 250 ml, a reduction in oxygen of 30 μl would be minimal even at the elevated temperature treatments. Taking this into consideration, we did not measure oxygen levels in the experimental bottles during our field experiments. Our experiments resulted in 19 samples from each egg batch: six from each of the three temperature treatments and one T0 sample. From the 14 embryo batches, three out of the total 266 samples were damaged and impossible to analyze.
Laboratory Analysis
Preserved embryos and larvae were observed using an Olympus SZX16 microscope with Olympus SDF PLAPO 1× PF lens and photographed with a Canon EOS550D camera and captured with EOS utility software. Each sample was photographed and analyzed for different life stages (Supplementary Figure S2). Three embryonic stages were identified prior to hatching: two cell, multiple cell, and limb bud. We used photographs from Jia et al. (2014) to help identify these stages. Hatched nauplii were categorized as either healthy or malformed, with total hatching success being the sum of these two categories. Three additional stages were also identified; entrapped embryos were nauplii that either died while hatching or were fixed in formalin at the time they were hatching; unclassified embryos were those that were damaged and therefore unclassifiable; and all embryos that could not be categorized were recorded simply as embryos.
Embryos From the Laboratory Experiments
Sampling Method and Collection of Embryos
The krill population used in the laboratory experiments originated from the Indian Ocean sector of the Southern Ocean between 64.67 to 64.71°S and 109.44 to 109.69°E during the 2017/2018 field season (Figure 1). Male and female krill were returned to the Australian Antarctic Division’s marine research aquarium and maintained at 0.5°C, where they matured and spawned naturally (Kawaguchi et al., 2010). Embryos were obtained in November 2018. While these krill were caught from different swarms, the locations caught were in close proximity, and they all experienced an identical environment for the 10 months prior to spawning, so we have referred to them as a single entity “Laboratory krill.” Individual gravid females were isolated and floated in 2 L spawning bottles with mesh set 1 cm from the bottom of the bottle to ensure embryos were not cannibalized by the female after spawning. Spawning was checked for twice a day. When spawning was observed, the eggs were gently transferred to 5 L containers and checked under the microscope for fertilization by looking for the formation of fertilization jelly (Jia et al., 2014). Six batches of >1000 embryos originating from six different females were used.
Experimental Method
Each egg batch was randomly divided into 30 experimental bottles (250 ml) with ∼30 embryos per bottle. Each experimental bottle had a window with 400 μm mesh to allow exchange of temperature-controlled seawater in the bottle. Experimental bottles were then randomly placed in five different incubation tanks with recirculating temperature-controlled water at five different temperature settings (six experimental bottles each in 0.0, 1.0, 3.0, 5.0, and 7.0°C, respectively). One bottle from each batch and temperature treatment was sampled every 24 h. The temperature-controlled recirculating seawater was measured for temperature, salinity, and pH prior to pipetting out the embryos and larvae into glass vials and fixing with 4% formaldehyde solution.
Laboratory Analysis
Embryos and larvae were observed with Leica M205C dissecting stereo-microscope with a Leica DFC 450 camera and Leica LAS V4.0 software. Data regarding embryonic developmental stages and nauplii malformations were not collected from the laboratory data.
Statistical Analysis
All statistical analyses were conducted in the R 3.4.1 programming environment (R Core Team, 2017). The relationship between the number of hatched and unhatched eggs was examined using a generalized linear model (GLM) using a quasibinomial distribution following the method used by Mayor et al. (2012). Field and laboratory results were tested independently of each other. The effects of the following variables were also investigated using this model: temperature (field levels = 0.5, 3.0, and 5.0°C, laboratory levels = 1.0, 3.0, 5.0°C) and individual female (field levels = females 1–14, laboratory levels = females 1–6). An optimal model was backward selected from a full model containing all variables.
We examined the relationship between the number of healthy and malformed nauplii at different temperature treatments using a non-parametric Kruskal–Wallis test. We only performed this analysis on embryos from our field experiments where the data were available. The same method of analysis was used to test the difference between weight and length of field females on hatching success and number of nauplii malformations. For all analyses, α = 0.05.
We acknowledge that our sample size of 20 females will affect the statistical power of our analyses. However, we emphasize the difficulty in obtaining field samples of healthy gravid females, which subsequently spawn within an experimental setting that has limited sample sizes in all previous attempts of this sort (Table 2). Furthermore, the numbers obtained in the present study still allow robust statistical analyses to be performed. The design of our field experiments means that repeat sampling could bias our analysis. To account for this, we ran a sensitivity analysis using (1) all days between 1 and 6, (2) odd days only, and (3) even days only. We found that the repeat sampling did not produce false trends, and consequently, we have reported on the analysis that used all 6 days of sampling.
Results
Firstly, we present the results of our quasibinomial GLM which examined how hatching success was affected by female and temperature in both our field and laboratory experiments. Secondly, we describe the effects of temperature on the number of nauplii malformations and egg death.
Variation in Hatching Success
Variation in Hatching Success Between Females
Hatching success was significantly affected by female in both our field (Quasibinomial GLM, F = 4.204, df = 38, p≤0.001) and laboratory results (Quasibinomial GLM, F = 8.6008, df = 38, p≤0.01) (Figure 2). The wet weights and lengths of females from the field experiments ranged from 1.25 to 2.1 g and 52 to 60 mm (Table 1). We found that neither the weight nor length of the field females had a significant effect on the hatching success or the occurrence of nauplii malformation. Weight and length data are not available for the laboratory data.
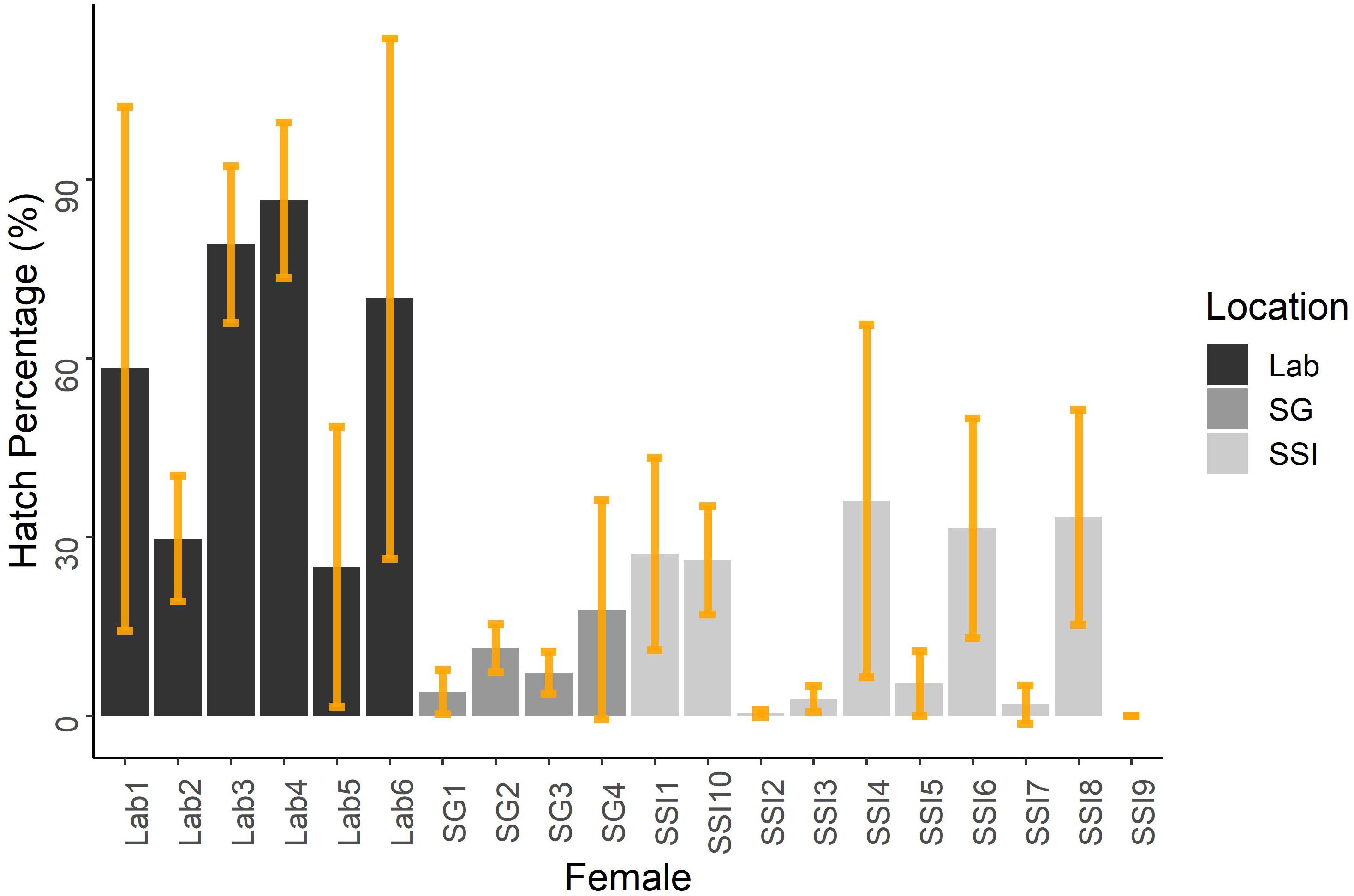
Figure 2. Mean hatch percentages of females from both the field and laboratory experiments. Egg batches from all 20 females were categorized into the two experiments from the laboratory (black bars) or the field. Field experiments are subdivided further into either South Georgia or the South Sandwich Islands. Average hatching success values and the standard deviation (shown by orange bar) were calculated for each female. The laboratory average hatching success was created using results from 1.0, 3.0, and 5.0°C treatments. These temperature treatments were chosen as they were the closest match to the three temperature treatments averaged here in the field experiments (0.5, 3.0, and 5.0°C).
Variation in Hatching Success Between Temperature
Temperatures had a highly significant effect on hatching success in both the field (Quasibinomial GLM, F = 10.435, df = 13, p≤0.001) and the laboratory experiments (Quasibinomial GLM, F = 11.1707, df = 15, p≤0.01).
Embryos from our field experiments had greatest hatching success at 3.0°C, whereas laboratory embryos had greatest hatching success at 1.0°C (Figure 3). The lowest recorded mean hatching success from our field experiments occurred at 0.5°C from embryos collected from South Georgia (mean = 5.7 ± SD 2%). At all temperatures in the field experiments, some embryo batches had no nauplii hatching. The highest mean hatching success in our field experiments occurred at 3.0°C from embryos collected from the South Sandwich Islands (mean = 23. ± SD 21%).
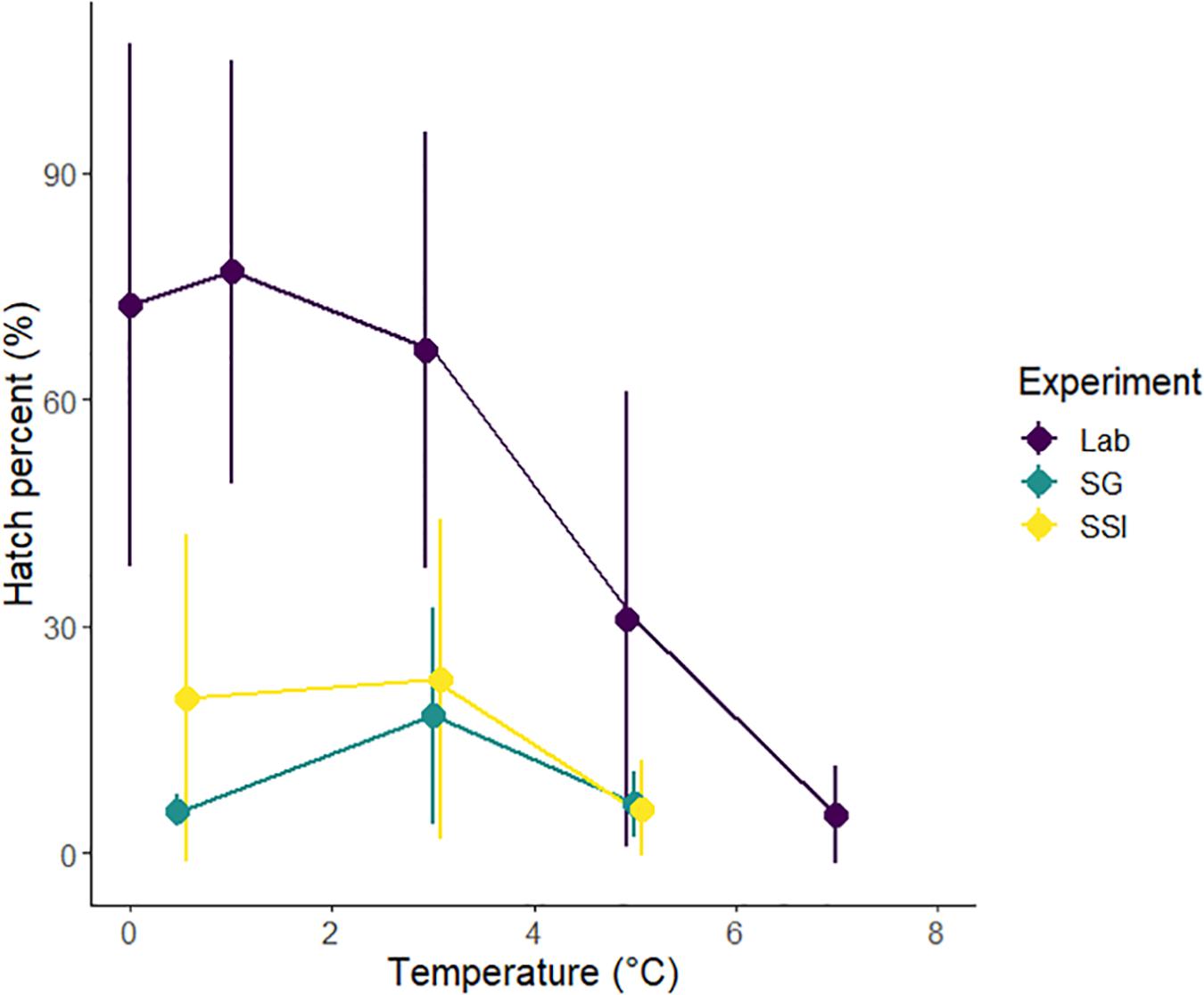
Figure 3. Variation in hatching success with temperature in field and laboratory experiments. Field experiments are divided into South Georgia and the South Sandwich Islands. Mean hatching success values presented here highlight the variation between the two experiments. Each data point has been slightly offset to ensure the bar showing standard deviation is visible.
The minimum mean hatching success between 1.0 and 5.0°C from the laboratory experiments was recorded at 5.0°C (mean = 31 ± SD 30%). The highest recorded mean hatching success occurred at 1.0°C (mean = 77 ± SD 28%) (Table 3).
Embryos from both the field and laboratory experiments showed very similar trends in embryonic development, peaks and development times at both 3.0°C and 5.0°C, even though the hatching successes were different (Figure 4). Day six of both experiments showed that the percentage of larvae hatched at temperatures up to and including 3.0°C were more than double than at 5.0°C. Although hatching success was minimal, hatching did occur at the 7.0°C treatment in the laboratory experiments.
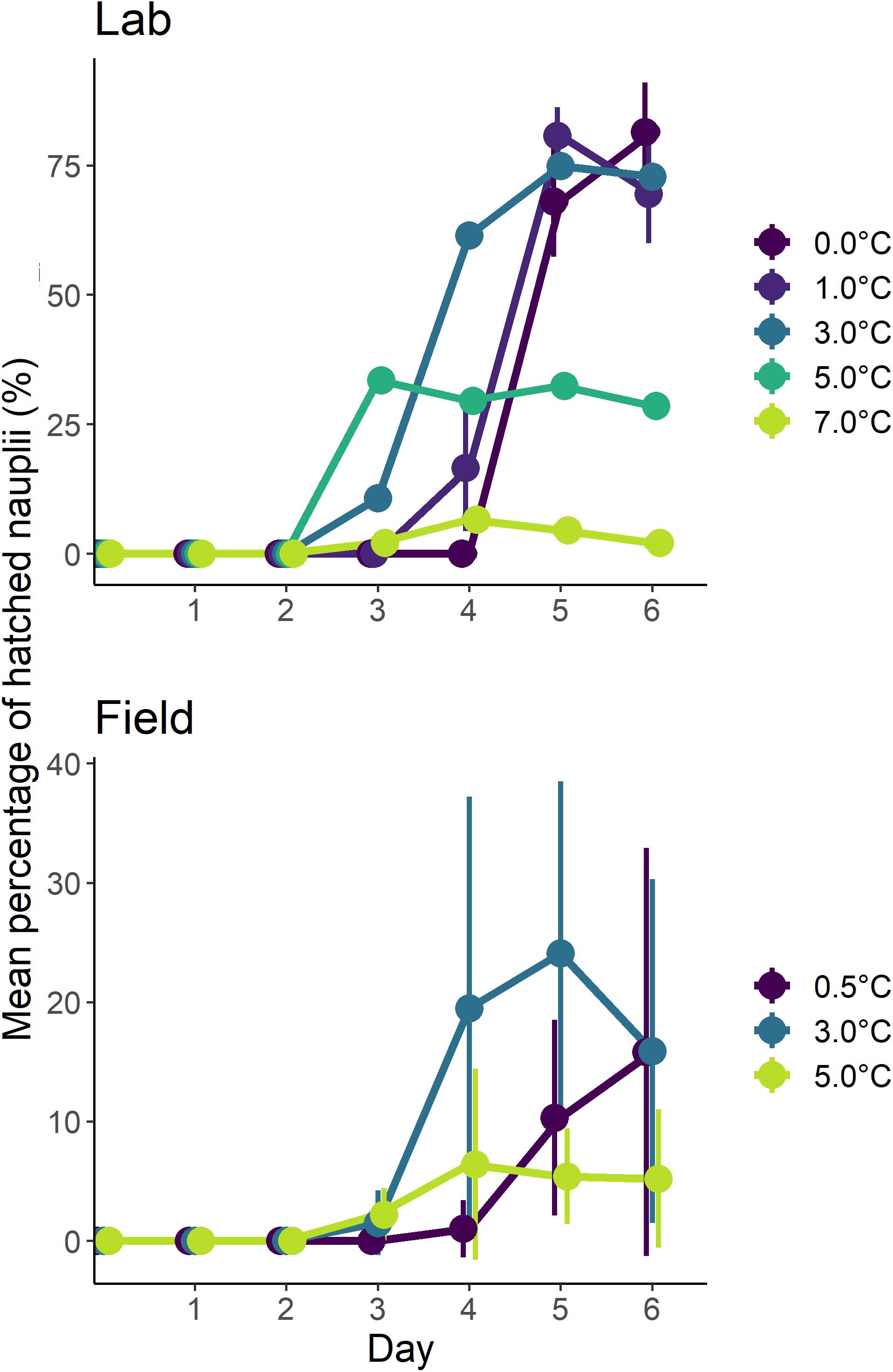
Figure 4. Effect of temperature on rate of embryonic development. The mean percentage of nauplii hatched for each of the temperature replicates for each day of the incubation experiments. Each data point has been slightly offset to ensure the bar showing standard deviation is visible.
Effect of Temperature on Nauplii Malformation and Egg Death
Differentiation of embryos stages and nauplii into healthy or malformed was only done for the field experiments. We found a significant difference in the number of healthy nauplii hatching at different temperature treatments (Kruskal–Wallis, chi-squared = 6.97, df = 2, p = 0.03). Nauplii that hatched at 5.0°C were twice as likely to be malformed than at the two lower temperature treatments (Figure 5). A negative correlation was identified between the total hatch percent and the percentage of hatched malformed nauplii (R2 = 0.11, p = 0.0589, n = 33).
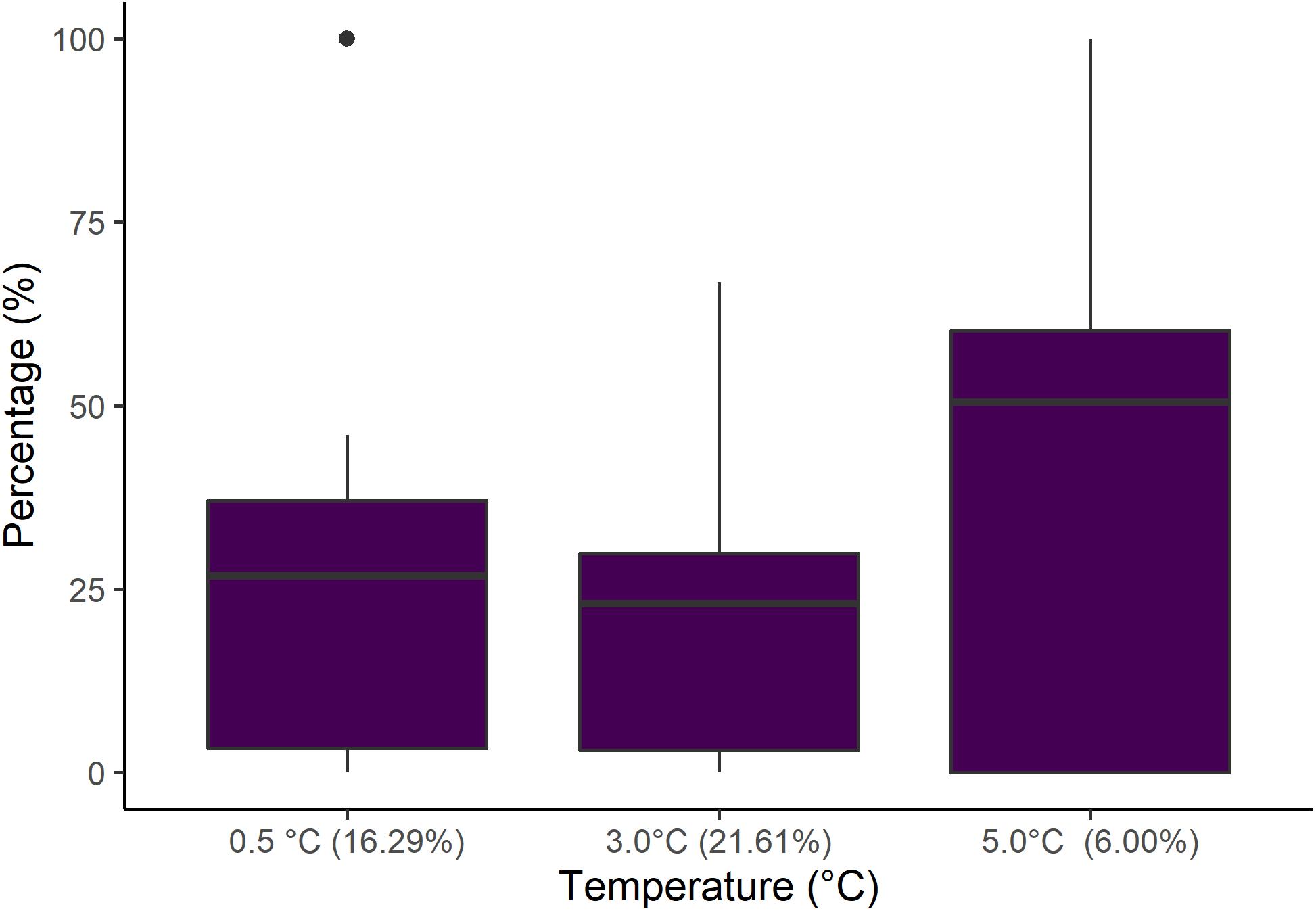
Figure 5. Percentages of malformed nauplii. All hatched nauplii in our field experiments were categorized as either healthy or malformed. This figure presents the percentage of malformed nauplii for each of the three temperature treatments. On the x-axis the number in brackets is the mean total hatch percent at the corresponding temperature. The error bars show standard deviation and the horizontal bar shows the mean percentage values.
Field experiments showed an average of 53% of embryos developing on day one and 34% by day six (Figure 6). Across the 6 days of the experiment, the average number of embryos developing differed at each treatment: 40% (0.5°C), 41% (3.0°C), and 34% (5.0°C). The percentage of embryos reaching the multiple cell stage on day one decreased with increasing temperature, from 56 to 49%. The average percentage of embryos failing to develop from the multiple cell stage to limb bud ranged from 30 to 32% across treatments. The average percentage of entrapped nauplii was 2% at 0.5 and 3.0°C but increased to 5% at 5.0°C.
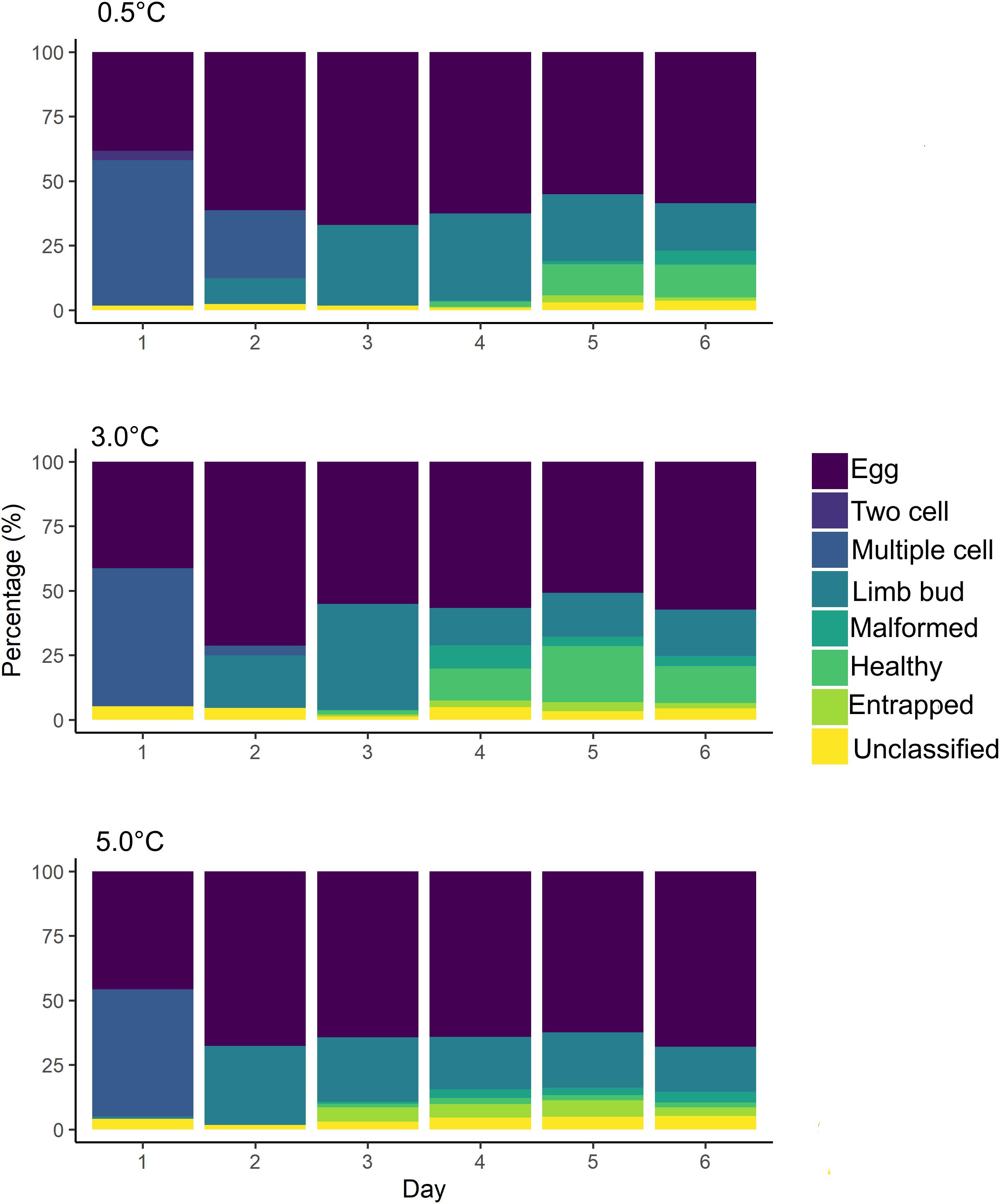
Figure 6. Rate of embryonic development. The rate of embryonic development from each of the temperature treatments in our field experiments. Each of the recorded embryonic development stages is shown in a different color. For each day of the experiments, the mean percentage of each developmental stage is given.
Discussion
Total hatching success in our field experiments was low and variable and the percentage of nauplii hatching with malformations increased significantly above 3.0°C. Total hatching success in our laboratory experiments was higher, but also highly variable. In regard to our hypothesis, we found that both field and laboratory experiments showed the inhibitory effect of temperature above 3.0°C regardless of the reported mean hatching success. From our field experiments, we also found a doubling of the percentage of malformed nauplii between 3.0 and 5.0°C. Below, we describe the influence of temperature on embryonic success in both the field and the laboratory experiments, the implications of our findings for understanding krill recruitment at a regional scale, and how krill reproduction may be affected in a warming climate.
Effect of Temperature on Hatching Success
Krill embryos did not hatch well above 3.0°C, either in the laboratory or the field. However, contrary to previous suggestions (George and Stromberg, 1985), we did not find complete inhibition of embryonic development above 4.0°C in either of our experiments. Embryos hatched at 5.0°C in both our field and laboratory experiments and in very low numbers at 7.0°C in the laboratory experiments, a finding that shows krill embryos are more resilient to temperature than suggested by George and Stromberg (1985). Field experiments also showed other responses to high temperatures, namely a lower percentage of embryos reaching the multiple cell stage, an increase in the percentage of embryos becoming entrapped during hatching, and a significant increase in the percentage of nauplii hatching with visible malformations.
These results suggest that temperatures above 3.0°C have a strong effect on hatching success and the prevalence of nauplii malformations in krill. Antarctic marine species are known for their stenothermy (Peck et al., 2004) reflecting their long evolutionary isolation (Hempel, 1985). Their physiology such as enzyme reactions, diffusion, and membrane transport are adapted for polar temperatures, and warmer temperatures inhibit these processes. This variation of biological function in response to changing temperatures could be the reason for the variation in embryonic development speeds described in Ross et al. (1988), Yoshida et al. (2004), and similarly reported from our own experiments. Adult krill growth rates are also highly sensitive to small temperature changes; growth rates peak at ∼0.5°C and decrease rapidly towards zero above 3.0°C (Atkinson et al., 2006). We suggest that future work should investigate exactly why the physiology of krill is so negatively affected by seemingly small changes in temperature.
Low hatching success recorded from prior incubation experiments have been attributed to handling effects, as shown in Euphausia pacifica (Ross, 1981). We handled the embryos carefully, just once, and in a consistent manner across all experiments. Such methods have been shown to yield high embryo hatching success in several experiments (Harrington and Ikeda, 1986; Yoshida et al., 2004, 2011) as well as in our own laboratory experiments. We found consistent trends in either low or high hatching success of fertilized embryos across multiple krill sampled from different schools which, given our consistent methods, would be hard to explain in terms of handling-based artifacts. Furthermore, studies on copepods have shown that, when embryo success is low, more of the hatching nauplii are malformed (Mayor et al., 2012; Maud et al., 2015) which aligns with our results. Taken together, the evidence suggests that our results are not an artifact of experimental procedures.
Causes of High Variability in Hatching Success
Evidence from our results and from other published studies (Table 2) show that krill embryos exhibit a high variability in the hatching success of their embryos and, on occasion, have extremely high or low success. This phenomenon also holds true for other euphausiid species (Gómez-Gutiérrez, 2006). Hatching success of Euphausia pacifica have been reported to range from 50 to 94% (Feinberg et al., 2006) <5 to >95% (Ross, 1981) and <10 to <40% (Gómez-Gutiérrez, 2002). In accordance with results from prior studies, we also found high levels of variability in mean hatching success between females in both our field (15%, 0–54% ± SD 17%) and laboratory (58%, 0–98% ± SD 34%) studies. Our field results showed that neither the weight nor the length of the female krill had a significant effect on the hatching success or the number of malformed nauplii. The well-defined relationship between female length and brood size in euphausiid species suggests that brood size does not have an effect hatching success and malformed nauplii (Ross and Quetin, 1983; Harrington and Ikeda, 1986; Feinberg et al., 2007). Results from our field experiments also showed a high incidence of malformed nauplii, a factor poorly reported on in the literature.
The reasons behind this high level of variation are unclear; however, we suggest that one or a number of other environmental factors drive hatching success below 3.0°C. The extremely high energetic cost of egg production in krill (Nicol et al., 1995, 2000) makes it likely that food quality and availability has a significant effect on quality of embryos. A modeling study by Rumsey and Franks (1999) suggested that variability of hatching success reported in E. pacifica could be due to female nutrition and the oceanic environment at the time and location of embryo release. Hatching success in krill has also been associated with quantity and composition of fatty acids in the embryos, which in turn is influenced by the maternal diet (Yoshida et al., 2011). Our findings show that future studies should prioritize assessing numbers of healthy nauplii and not just hatching success, as well as investigating the reasons for nauplii malformation. This level of variation is important to take into account when estimating larval recruitment using spawning estimates.
Implications for Understanding Spatial Variability in Krill Recruitment
Because krill are a key species in the food web and exploited commercially, there is much interest in identifying areas of successful spawning and recruitment (Orsi et al., 1995; Hofmann and Hüsrevoglu, 2003; Murphy et al., 2004; Perry et al., 2019; Thorpe et al., 2019). Our results highlight the extremely variable and often low krill hatching success in the field, suggesting that we need to refine our models to account for maternal factors as well as temperature.
As one important example, krill are abundant at South Georgia, where the females are the largest anywhere in the Southern Ocean (Schmidt et al., 2014) and clearly benefit from the high food concentrations (Pond et al., 2005). Yet, larval abundances are typically low here (Ward et al., 2006; Tarling et al., 2007; Perry et al., 2019). A variety of explanations have been proposed, including high predation and mass advection (Tarling et al., 2007). We found the mean hatching success of embryos from South Georgia to be 10% in surface waters (∼150 m) where temperatures can exceed 3.0°C during summer (Whitehouse et al., 1996). While temperatures exceeding 3.0°C will have increasingly negative impacts on hatching success, embryos spawned in the top 100 m of the water column will sink out of these sub-optimal temperature conditions within 24 h (Quetin and Ross, 1984; Hempel and Hempel, 1986; Figure 1). It is also possible that embryos will be spawned below the surface warm layer and avoid interacting with warmer temperatures until they ascend as nauplii (Kawaguchi et al., 2011). To fully understand the interaction between embryonic development and temperature, we must understand how high initial temperatures within 24 h of spawning may affect subsequent hatching success. Nevertheless, the degree of variation exhibited in our experiments strongly suggests that temperature conditions at South Georgia are not the only reason for embryonic failure, reduced nauplii survival, and consequent poor recruitment to larvae.
Recruitment of juvenile krill to the adult population is highly variable from year to year (Quetin et al., 2003; Saba et al., 2014; Atkinson et al., 2019), and the causal factors vary regionally and possibly temporally (Loeb and Santora, 2015) with sea ice extent, ENSO, and the Southern Annular Mode all being invoked. However, there does not appear to be a clear stock–recruit relationship; moderate or high recruitment can follow years with exceptionally low biomass of the spawning stock (Moline et al., 2004; Siegel and Wakins, 2016). Fecundity of krill is known to vary with their age (Cuzin-Roudy, 2000; Tarling et al., 2016), but this study shows that, in addition to consideration of the overall output of eggs, we need to incorporate the large and unexplained variability of egg hatching success into our understanding of krill population dynamics.
Krill Reproduction in a Changing Climate
Irrespective of any maternal effect, temperatures of 5.0°C reduce hatching success by half compared to 3.0°C. Concurrently, nauplii are more likely to be entrapped during hatching and are twice as likely to be malformed. Yet, neither current nor predicted Southern Ocean temperatures for 2100 create conditions whereby the full descent of an embryo will exceed temperatures of 3.0°C (Meredith and King, 2005; Clarke et al., 2007; Barnes and Peck, 2008; Sallée et al., 2013). For the next century, it is unlikely that larval recruitment of krill will be driven directly by temperature, especially due to the smaller predicted changes below the surface waters of the Southern Ocean. However, this statement comes with a caveat, as we did not test temperature in tandem with any other future climate change stressors. Pressure from multiple climate change stressors, including pH and food availability (Atkinson et al., 2004; Quetin et al., 2007; Kawaguchi et al., 2011, 2013), may cumulatively make embryos more sensitive to temperature as they will ultimately have to expend more energy maintaining homeostasis.
Overall, our results show that krill embryos are negatively impacted by incubation temperatures that exceed 3.0°C. Below this temperature, other environmental factors and individual female variability dictate hatching success. Further understanding of these controls may help to explain the discrepancy in the spatial distributions of spawning females and larvae (Perry et al., 2019). To model the distribution of krill in a changing climate to the level required for effective fishing management to operate (Hill et al., 2006), we need to take account of the factors that dictate embryo hatching success.
Data Availability Statement
The datasets generated for this study are available on request to the corresponding author.
Author Contributions
The conceptualization of the experimental design was undertaken by SK, RK, FP, AA, SS, and GT. FP conducted field experiments. AC and RK conducted the laboratory experiments. The analysis of samples in the laboratory was done by FP and AC. DM, GT, and FP undertook the statistical analysis. Project administration and resources supplied by SK, CL, and DM. The original draft of the manuscript was written by FP. All authors contributed significantly to the drafting of this manuscript.
Funding
This work was supported by the Natural Environment Research Council (NERC) (grant number NE/L002531/1) to FP. GT contributed to this work as part of the Ecosystems program at the British Antarctic Survey. DM received funding from the Natural Environment Research Council, United Kingdom (DIAPOD; NE/P0063513/1). AA was supported by the World Wildlife Fund. SK, RK, and AC were supported by Australian Antarctic Program Projects 4037 and 4512.
Conflict of Interest
The authors declare that the research was conducted in the absence of any commercial or financial relationships that could be construed as a potential conflict of interest.
Acknowledgments
The authors are grateful to the officers and crew of the RRV Discovery (DY098), as well as Sophie Fielding and Clara Manno, for their support during the field experiments.
Supplementary Material
The Supplementary Material for this article can be found online at: https://www.frontiersin.org/articles/10.3389/fmars.2020.00501/full#supplementary-material
FIGURE S1 | A schematic showing our field experiment setup. For each batch of eggs (>1000) produced by a gravid female the following experimental setup was used. We placed ∼60 eggs into each of nine treatment bottles. Three bottles were placed in each of the three temperature treatments. Each day ∼30 eggs were removed from each of the bottles and fixed in 4% formalin.
FIGURE S2 | Recorded stages of embryonic development from field experiments. Photographic documentation for the different embryonic development stages recorded when analyzing the eggs from the field experiment in the laboratory.
References
Atkinson, A., Hill, S., Pakhomov, E., Siegel, V., Reiss, C., Loeb, V., et al. (2019). Krill (Euphausia superba) distribution contracts southward during rapid regional warming. Nat. Clim. Chang. 9, 142–147. doi: 10.1038/s41558-018-0370-z
Atkinson, A., Shreeve, R., Hirst, A., Rothery, P., Tarling, G., Pond, D., et al. (2006). Natural growth rates in Antarctic krill (Euphausia superba): II. Predictive models based on food, temperature, body length, sex, and maturity stage. Limnol. Oceanogr. 51, 973–987. doi: 10.4319/lo.2006.51.2.0973
Atkinson, A., Siegel, V., Pakhomov, E., and Rothery, P. (2004). Long-term decline in krill stock and increase in salps within the Southern Ocean. Nature 432, 100–103. doi: 10.1038/nature02950.1
Atkinson, A., Siegel, V., Pakhomov, E. A., Rothery, P., Loeb, V. J., Ross, R. M., et al. (2008). Oceanic circumpolar habitats of Antarctic krill: appendices. Mar. Ecol. Prog. Ser. 362, 1–23. doi: 10.3354/meps07498
Baker, A., Clarke, M., and Harris, M. (1973). The n.i.o. combination net (rmt 1 + 8) and further developments of rectangular midwater trawls. J. Mar. Biol. Assoc. U. K. 53, 167–184. doi: 10.1017/S0025315400056708
Barnes, D., and Peck, L. (2008). Vulnerability of Antarctic shelf biodiversity to predicted regional warming. Clim. Res. 37, 149–163. doi: 10.3354/cr00760
Clarke, A., Murphy, E., Meredith, M., King, J., Peck, L., Barnes, D., et al. (2007). Climate change and the marine ecosystem of the western Antarctic Peninsula. Philos. Trans. R. Soc. B Biol. Sci. 362, 149–166. doi: 10.1098/rstb.2006.1958
Cuzin-Roudy, J. (2000). Seasonal reproduction, multiple spawning, and fecundity in northern krill, Meganyctiphanes norvegica, and Antarctic krill, Euphausia superba. Can. J. Fish. Aquat. Sci. 57, 6–15. doi: 10.1139/f00-165
Cuzin-Roudy, J., Irisson, J., Penot, F., Kawaguchi, S., and Vallet, C. (2014). “Southern Ocean Euphausiids,” in Biogeographic Atlas of the Southern Ocean, eds C. De Broyer, P. Koubbi, H.J. Griffiths, B. Raymond, C. Udekem d’Acoz, A. P. d’ Van de Putte, et al. (Cambridge, MA: Scientific Committee on Antarctic Research), 309–320.
Feinberg, L., Shaw, C., and Peterson, W. (2007). Long-term laboratory observations of Euphausia pacifica fecundity: comparison of two geographic regions. Mar. Ecol. Prog. Ser. 341, 141–152. doi: 10.3354/meps341141
Feinberg, L., Shaw, T., and Peterson, W. (2006). Larval development of Euphausia pacifica in the laboratory: variability in developmental pathways. Mar. Ecol. Prog. Ser. 316, 127–137. doi: 10.3354/meps316127
Fraser, F. (1936). On the development and distribution of the young stages of krill (Euphausia superba). Discov. Rep. 14, 1–192. doi: 10.5962/bhl.part.3886
García-Guerrero, M., Villarreal, H., and Racotta, I. (2003). Effect of temperature on lipids, proteins, and carbohydrates levels during development from egg extrusion to juvenile stage of Cherax quadricarinatus (Decapoda: Parastacidae). Comp. Biochem. Physiol. A Mol. Integr. Physiol. 135, 147–154. doi: 10.1016/S1095-6433(02)00354-9
George, R. (1984). Ontogenetic adaptations in growth and respiration of Euphausia superba in relation to temperature and pressure. J. Crustac. Biol. 4, 252–262. doi: 10.1163/1937240x84x00633
George, R., and Stromberg, J. (1985). Development of eggs of antarctic krill Euphausia superba in relation to pressure. Polar Biol. 4, 125–133.
Gómez-Gutiérrez, J. (2002). Hatching mechanism and delayed hatching of the eggs of three broadcast spawning euphausiid species under laboratory conditions. J. Plankton Res. 24, 1265–1276. doi: 10.1093/plankt/24.12.1265
Gómez-Gutiérrez, J. (2006). Hatching mechanisms and death of euphausiid embryos during hatching process: evidences for evolutionary reversal of the free-living nauplius? CICIMAR Oceánides 21, 63–79.
Harrington, S., and Ikeda, T. (1986). Laboratory observations on spawning, brood size and egg hatchability of the Antarctic krill Euphausia superba from Prydz Bay, Antarctica. Mar. Biol. 92, 231–235. doi: 10.1007/bf00392840
Hempel, G. (1985). “Antarctic marine food webs,” in Antarctic Nutrient Cycles and Food Webs, eds W.R. Siegfried, P.R. Condy, and R.M. Laws (Berlin: Springer), 266–270. doi: 10.1007/978-3-642-82275-9_38
Hempel, I., and Hempel, G. (1986). Field observations on the developmental ascent of larval Euphausia superba (Crustacea). Polar Biol. 6, 121–126. doi: 10.1007/BF00258263
Hill, S., Murphy, E., Reid, K., Trathan, P., and Constable, A. (2006). Modelling Southern Ocean ecosystems: krill, the food-web, and the impacts of harvesting. Biol. Rev. 81, 581–608. doi: 10.1017/S1464793106007123
Hirano, Y., Matsuda, T., and Kawaguchi, S. (2003). Breeding antarctic krill in captivity. Mar. Freshw. Behav. Physiol. 36, 259–269. doi: 10.1080/10236240310001614448
Hofmann, E., and Hüsrevoglu, Y. (2003). A circumpolar modeling study of habitat control of Antarctic krill (Euphausia superba) reproductive success. Deep. Res. Part II Top. Stud. Oceanogr. 50, 3121–3142. doi: 10.1016/j.dsr2.2003.07.012
Ikeda, T. (1984). Development of the lavae of the antarctic krill (Euphausia superba Dana) observation in the laboratory. J. Exp. Mar. Bio. Ecol. 75, 107–117. doi: 10.1016/0022-0981(84)90175-8
Jia, Z., Virtue, P., Swadling, K., and Kawaguchi, S. (2014). A photographic documentation of the development of Antarctic krill (Euphausia superba) from egg to early juvenile. Polar Biol. 37, 165–179. doi: 10.1007/s00300-013-1420-7
Jones, C. (1995). “Effect of temperature on growth and survival of the tropical freshwater crayfish, Cherax quadricarinatus (von Martens) (Decapoda: Parastacidae),” in Proceedings of the Freshwater Crayfish VIII: Papers from the 8th International Symposium on Astacology, Baton Rouge, LA, 391–398.
Kawaguchi, S. (2016). “Reproduction and larval development in Antarctic krill (Euphausia superba),” in Biology and Ecology of Antarctic krill, ed. V. Siegel (Cham: Springer International Publishing), 225–246. doi: 10.1007/978-3-319-29279-3_6
Kawaguchi, S., Ishida, A., King, R., Raymond, B., Waller, N., Constable, A., et al. (2013). Risk maps for Antarctic krill under projected Southern Ocean acidification. Nat. Clim. Chang. 3, 843–847. doi: 10.1038/nclimate1937
Kawaguchi, S., King, R., Meijers, R., Osborn, J., Swadling, K., Ritz, D., et al. (2010). An experimental aquarium for observing the schooling behaviour of Antarctic krill (Euphausia superba). Deep. Res. Part II Top. Stud. Oceanogr. 57, 683–692. doi: 10.1016/j.dsr2.2009.10.017
Kawaguchi, S., Kurihara, H., King, R., Hale, L., Berli, T., Robinson, J., et al. (2011). Will krill fare well under Southern Ocean acidification? Biol. Lett. 7, 288–291. doi: 10.1098/rsbl.2010.0777
Kikuno, T. (1981). Spawning behaviour and early development of the Antarctic krill, Euphausia superba Dana, observed on board R. V. Kaiyo Maru in 1979-1980. Antarc. Rec. 73, 97–102.
Kumlu, M., Eroldogan, O., and Aktas, M. (2000). Effects of temperature and salinity on larval growth, survival and development of Penaeus semisulcatus. Aquaculture 188, 167–173. doi: 10.1016/S0044-8486(00)00330-6
Loeb, V., and Santora, J. (2015). Climate variability and spatiotemporal dynamics of five Southern Ocean krill species. Prog. Oceanogr. 134, 93–122. doi: 10.1016/j.pocean.2015.01.002
Manush, S., Pal, A., Das, T., and Mukherjee, S. (2006). The influence of temperatures ranging from 25 to 36 °C on developmental rates, morphometrics and survival of freshwater prawn (Macrobrachium rosenbergii) embryos. Aquaculture 256, 529–536. doi: 10.1016/j.aquaculture.2006.02.007
Marr, J. (1962). The natural history and geography of the Antarctic Krill (Euphausia superba Dana). Discov. Reports 10, 33–464.
Maud, J., Atkinson, A., Hirst, A., Lindeque, P., Widdicombe, C., Harmer, R., et al. (2015). How does Calanus helgolandicus maintain its population in a variable environment? Analysis of a 25-year time series from the English Channel. Prog. Oceanogr. 137, 513–523. doi: 10.1016/j.pocean.2015.04.028
Mayor, D., Everett, N., and Cook, K. (2012). End of century ocean warming and acidification effects on reproductive success in a temperate marine copepod. J. Plankton Res. 34, 258–262. doi: 10.1093/plankt/fbr107
Meredith, M., and King, J. (2005). Rapid climate change in the ocean west of the Antarctic Peninsula during the second half of the 20th century. Geophys. Res. Lett. 32, 1–5. doi: 10.1029/2005GL024042
Moline, M., Claustre, H., Frazer, T., Schofield, O., and Vernet, M. (2004). Alteration of the food web along the Antarctic Peninsula in response to a regional warming trend. Glob. Chang. Biol. 10, 1973–1980. doi: 10.1111/j.1365-2486.2004.00825.x
Morris, D., Watkins, J., Ricketts, C., Buchholz, F., and Priddle, J. (1988). An assessment of the merits of length and weight measurements of Antarctic krill Euphausia superba. Br. Antarc. Surv. Bull. 79, 27–50.
Murphy, E., Thorpe, S., Watkins, J., and Hewitt, R. (2004). Modeling the krill transport pathways in the Scotia Sea: spatial and environmental connections generating the seasonal distribution of krill. Deep. Res. Part II Top. Stud. Oceanogr. 51, 1435–1456. doi: 10.1016/j.dsr2.2004.06.019
Naylor, J., Taylor, E., and Bennett, D. (1999). Oxygen uptake of developing eggs of Cancer pagurus (Crustacea: Decapoda: Cancridae) and consequent behaviour of the ovigerous females. J. Mar. Biol. Assoc. U.K. 79, 305–315. doi: 10.1017/S0025315498000332
Nicol, S., De La Mare, W., and Stolp, M. (1995). The energetic cost of egg production in Antarctic krill (Euphausia superba Dana). Antarct. Sci. 7, 25–30. doi: 10.1017/s0954102095000058
Nicol, S., Kitchener, J., King, R., Hosie, G., and De La Mare, W. (2000). Population structure and condition of Antarctic krill (Euphausia superba) off East Antarctica (80–150°E) during the Austral summer of 1995/1996. Deep Sea Res. Part II Top. Stud. Oceanogr. 47, 2489–2517. doi: 10.1016/S0967-0645(00)00033-3
Orsi, A., Whitworth, T., and Nowlin, W. (1995). On the meridional extent and fronts of the Antarctic circumpolar current. Deep. Res. Part I 42, 641–673. doi: 10.1016/0967-0637(95)00021-W
Paula, J., Mendes, R., Paci, S., McLaughlin, P., Gherardi, F., and Emmerson, W. (2001). Combined effects of temperature and salinity on the larval development of the estuarine mud prawn Upogebia africana (Crustacea, Thalassinidea). Hydrobiologia 449, 141–148. doi: 10.1023/A:1017505506184
Peck, L., Webb, K., and Bailey, D. (2004). Extreme sensitivity of biological function to temperature in Antarctic marine species. Funct. Ecol. 18, 625–630. doi: 10.1111/j.0269-8463.2004.00903.x
Perry, F., Atkinson, A., Sailley, S., Tarling, G., Hill, S., Lucas, C., et al. (2019). Habitat partitioning in Antarctic krill: spawning hotspots and nursery areas. PLoS One 14:e0219325. doi: 10.1371/journal.pone.0219325
Pond, D., Atkinson, A., Shreeve, R., Tarling, G., and Ward, P. (2005). Diatom fatty acid biomarkers indicate recent growth rates in Antarctic krill. Limnol. Oceanogr. 50, 732–736. doi: 10.4319/lo.2005.50.2.0732
Quetin, L., and Ross, R. (1984). Depth distribution of developing Euphausia superba embryos, predicted from sinking rates. Mar. Biol. 79, 47–53. doi: 10.1007/BF00404984
Quetin, L., Ross, R., Frazer, T., Amsler, M., Wyatt-Evens, C., and Oakes, S. (2003). Growth of larval krill, Euphausia superba, in fall and winter west of the Antarctic Peninsula. Mar. Biol. 143, 833–843. doi: 10.1007/s00227-003-1130-8
Quetin, L., Ross, R., Fritsen, C., and Vernet, M. (2007). Ecological responses of Antarctic krill to environmental variability: can we predict the future? Antarct. Sci. 19, 253–266. doi: 10.1017/S0954102007000363
R Core Team (2017). R: A Language and Environment for Statistical Computing. Vienna: R Foundation for Statistical Computing.
Rhodes, C. (1981). Artificial incubation of the eggs of the crayfish Austropotamobius pallipes (Lereboullet). Aquaculture 25, 129–140. doi: 10.1016/0044-8486(81)90175-7
Ross, R. (1981). Culture and development of Euphausia pacifica. Society 26, 235–246. doi: 10.4319/lo.1981.26.2.0235
Ross, R., and Quetin, L. (1983). Spawning frequency and fecundity of the Antarctic krill Euphausia superba. Mar. Biol. 77, 201–205. doi: 10.1007/BF00395807
Ross, R., Quetin, L., and Kirsch, E. (1988). Effect of temperature on developmental times and survival of early larval stages of Euphausia superba Dana. J. Exp. Mar. Bio. Ecol. 121, 55–71. doi: 10.1016/0022-0981(88)90023-8
Rumsey, S., and Franks, P. (1999). Influence of variability in larval development on recruitment success in the euphausiid Euphausia pacifica: elasticity and sensitivity analyses. Mar. Biol. 133, 283–291. doi: 10.1007/s002270050467
Saba, G., Fraser, W., Saba, V., Iannuzzi, R., Coleman, K., Doney, S., et al. (2014). Winter and spring controls on the summer food web of the coastal West Antarctic Peninsula. Nat. Commun. 5:4318. doi: 10.1038/ncomms5318
Sallée, J., Shuckburgh, E., Bruneau, N., Meijers, A., Bracegirdle, T., Wang, Z., et al. (2013). Assessment of Southern Ocean water mass circulation and characteristics in CMIP5 models: historical bias and forcing response. J. Geophys. Res. Ocean. 118, 1830–1844. doi: 10.1002/jgrc.20135
Schmidt, K., Atkinson, A., Pond, D., and Ireland, L. (2014). Feeding and overwintering of Antarctic krill across its major habitats: the role of sea ice cover, water depth, and phytoplankton abundance. Limnol. Oceanogr. 59, 17–36. doi: 10.4319/lo.2014.59.1.0017
Siegel, V., and Wakins, J. (2016). “Distribution, biomass and demography,” in Biology and Ecology of Antarctic Krill, ed. V. Siegel (Cham: Springer International Publishing), 21–100. doi: 10.1007/978-3-319-29279-3_2
Tarling, G., Cuzin-Roudy, J., Thorpe, S., Shreeve, R., Ward, P., and Murphy, E. (2007). Recruitment of Antarctic krill Euphausia superba in the South Georgia region: adult fecundity and the fate of larvae. Mar. Ecol. Prog. Ser. 331, 161–179. doi: 10.3354/meps331161
Tarling, G., Hill, S., Peat, H., Fielding, S., Reiss, C., and Atkinson, A. (2016). Growth and shrinkage in antarctic krill Euphausia superba is sex-dependent. Mar. Ecol. Prog. Ser. 547, 61–78. doi: 10.3354/meps11634
Tarling, G., Shreeve, R., Hirst, A., Atkinson, A., Pond, D., Murphy, E., et al. (2006). Natural growth rates in Antarctic krill (Euphausia superba): I. Improving methodology and predicting intermolt period. Limnol. Oceanogr. 51, 959–972. doi: 10.4319/lo.2006.51.2.0959
Thorpe, S., Tarling, G., and Murphy, E. (2019). Circumpolar patterns in Antarctic krill larval recruitment: an environmentally driven model. Mar. Ecol. Prog. Ser. 613, 77–96. doi: 10.3354/meps12887
Ward, P., Atkinson, A., Peck, J., and Wood, A. (1990). Euphausiid life cycles and distribution around South Georgia. Antarct. Sci. 2, 43–52. doi: 10.1017/S0954102090000050
Ward, P., Shreeve, R., Atkinson, A., Korb, B., Whitehouse, M., Thorpe, S., et al. (2006). Plankton community structure and variability in the Scotia Sea: austral summer 2003. Mar. Ecol. Prog. Ser. 309, 75–91. doi: 10.3354/meps309075
Whitehouse, M., Priddle, J., and Symon, C. (1996). Seasonal and annual change in seawater temperature, salinity, nutrient and chlorophyll a distributions around South Georgia, South Atlantic. Deep. Res. Part I Oceanogr. Res. Pap. 43, 425–443. doi: 10.1016/0967-0637(96)00020-9
Yoshida, T., Toda, T., Hirano, Y., and Matsuda, T. (2004). Effect of temperature on embryo development time and hatching success of the Antarctic krill Euphausia superba Dana in the laboratory. Mar. Freshw. Behav. Physiol. 37, 137–145. doi: 10.1080/10236240410001705789
Keywords: Antarctic krill, hatching success, nauplii, malformation, temperature
Citation: Perry FA, Kawaguchi S, Atkinson A, Sailley SF, Tarling GA, Mayor DJ, Lucas CH, King R and Cooper A (2020) Temperature–Induced Hatch Failure and Nauplii Malformation in Antarctic Krill. Front. Mar. Sci. 7:501. doi: 10.3389/fmars.2020.00501
Received: 14 February 2020; Accepted: 03 June 2020;
Published: 23 June 2020.
Edited by:
Rui Rosa, University of Lisbon, PortugalReviewed by:
Jaime Gómez-Gutiérrez, Centro Interdisciplinario de Ciencias Marinas (IPN), MexicoRicardo Calado, University of Aveiro, Portugal
Copyright © 2020 Perry, Kawaguchi, Atkinson, Sailley, Tarling, Mayor, Lucas, King and Cooper. This is an open-access article distributed under the terms of the Creative Commons Attribution License (CC BY). The use, distribution or reproduction in other forums is permitted, provided the original author(s) and the copyright owner(s) are credited and that the original publication in this journal is cited, in accordance with accepted academic practice. No use, distribution or reproduction is permitted which does not comply with these terms.
*Correspondence: Frances A. Perry, ZnJwZUBwbWwuYWMudWs=