- 1Marine Biology, Norwegian Institute for Water Research, Oslo, Norway
- 2Centre for Environmental and Marine Studies, Department of Biology, University of Aveiro, Aveiro, Portugal
- 3NTNU University Museum, Norwegian University of Science and Technology, Trondheim, Norway
- 4Centre of Autonomous Marine Operations and Systems, Trondheim Biological Station, Department of Biology, Norwegian University of Science and Technology, Trondheim, Norway
- 5Department of Arctic Biology, The University Centre in Svalbard, Longyearbyen, Norway
- 6Department of Biological Sciences and K.G. Jebsen Centre for Deep-Sea Research, University of Bergen, Bergen, Norway
- 7Norwegian Research Centre AS, NORCE Environment, Bergen, Norway
The Mohn’s Treasure, described as an inactive sulfide mound, was discovered at 2,600-m depth on the Arctic Mid-Ocean Ridge (AMOR) in 2002. In 2015, we conducted the first biological survey of Mohn’s Treasure using remotely operated vehicle (ROV) photo transects and sampling. This site is covered by a thick layer of fine sediments, where hard substratum is only visible as rocky outcrops on ridges. The observed benthic community was typical of Arctic bathyal systems. A total of 46 species (identified as morphospecies) were recorded, with densities varying from 12.2 to 31.6 ind.m–2. The two most abundant phyla were Porifera and Echinodermata. The sediment is dominated by fields of the stalked crinoid Bathycrinus carpenterii, whereas areas of hard substratum were characterized by high abundances of several sponge species and associated fauna. Interest in commercial exploration and exploitation of minerals from massive sulfide deposits is rising globally, and the AMOR is being targeted for mineral exploration within Norwegian waters. Gathering baseline ecological data from these poorly known sites is thus urgent and essential if robust resource management measures are to be developed and implemented. The results of this ecological survey are discussed in relation to the designation of deep-sea vulnerable marine ecosystems (VMEs) and their implication in management and conservation of areas targeted by the emerging deep-sea mining industry.
Introduction
Since hydrothermal vents were discovered in 1977, scientific research has been the primary source of anthropogenic disturbance in these ecosystems. However, there is increasing interest in commercial exploration of seabed minerals in general and a special focus on potential exploitation of seafloor massive sulfide (SMS) deposits that also host vent communities and habitats (Van Dover et al., 2014). SMS are large deposits of polymetallic-bearing sulfides on and below the seabed, formed through precipitation of metals contained in the hydrothermal fluids emanating from active vents. SMS deposits can be found associated to active vents, as well as at inactive ones, where fluid flow has stopped, and the chemosynthetic-based faunal communities have disappeared (Van Dover, 2019). At active hydrothermal vents, large biomass of highly adapted organisms is sustained by the primary production of chemoautotrophic microorganisms, both free-living and in symbiosis with the fauna (Van Dover, 2010). Mining SMS deposits represents a whole new level of impact on these unique and rare vent ecosystems (Van Dover et al., 2018), and a better understanding of the community composition, ecosystem function, and population connectivity is essential for the development of sound environmental management, conservation measures, and decision making.
The ultraslow-spreading Arctic Mid-Ocean Ridge (AMOR) comprises the Kolbeinsey Ridge, Mohn’s Ridge, and Knipovich Ridge (Figure 1), with the sections from Jan Mayen to off Svalbard within the Norwegian exclusive economic zone (EEZ) and Extended Continental Shelf. Several active areas have been discovered and studied in the AMOR since 2005, ranging from upper bathyal (140–700 m) vents at the Jan Mayen Vent Field and Seven Sisters Vent Field, to deep bathyal sites (2,400–2,200 m) at the Loki’s Castle and AEgir vent sites (Pedersen et al., 2010a; Schander et al., 2010; Olsen et al., 2016). In 2018, a new active site was observed at more than 3,000-m depth on the Mohn’s Ridge between Loki’s Castle and AEgir, during an exploration survey for potential mineral deposits in the AMOR commissioned by the Norwegian Petroleum Directorate. In addition to the active sites, two inactive sites have been reported so far along the AMOR: Copper Hill (900-m depth) on the central Mohn’s Ridge, for which very limited geological information is available (Pedersen et al., 2010b) and Mohn’s Treasure (2,600 m). Mohn’s Treasure is located at the edge of an inner rift wall, 30 km southwest of Loki’s Castle, and was described as a massive inactive sulfide deposit based on hydrothermal deposits collected in a rock dredge in 2002 (Pedersen et al., 2010b). Lim et al. (2019) provided magnetic data of the fossil sedimented-hosted hydrothermal deposit, delineating a deposit of approximately 200 × 150 m buried under 15 m of sediments, as well as two new deposits. The ecology of AMOR vents has been studied for the shallow active vent fields and Loki’s Castle. While the shallower vents at Seven Sisters and Jan Mayen vent fields do not support typical vent communities, the Loki’s Castle vent field is characterized by chemosynthetic-based fauna, including gastropods, siboglinid polychaetes, and an endemic species of amphipod (Pedersen et al., 2010a; Tandberg et al., 2011, 2018; Kongsrud and Rapp, 2012; Kongsrud et al., 2017). The AEgir vent field, discovered in 2015 and poorly studied to date, seems to host a similar fauna to Loki’s Castle communities, with, however, a lower diversity of vent specialists (Olsen et al., 2016). No biological information was available for the inactive Copper Hill and Mohn’s Treasure sites.
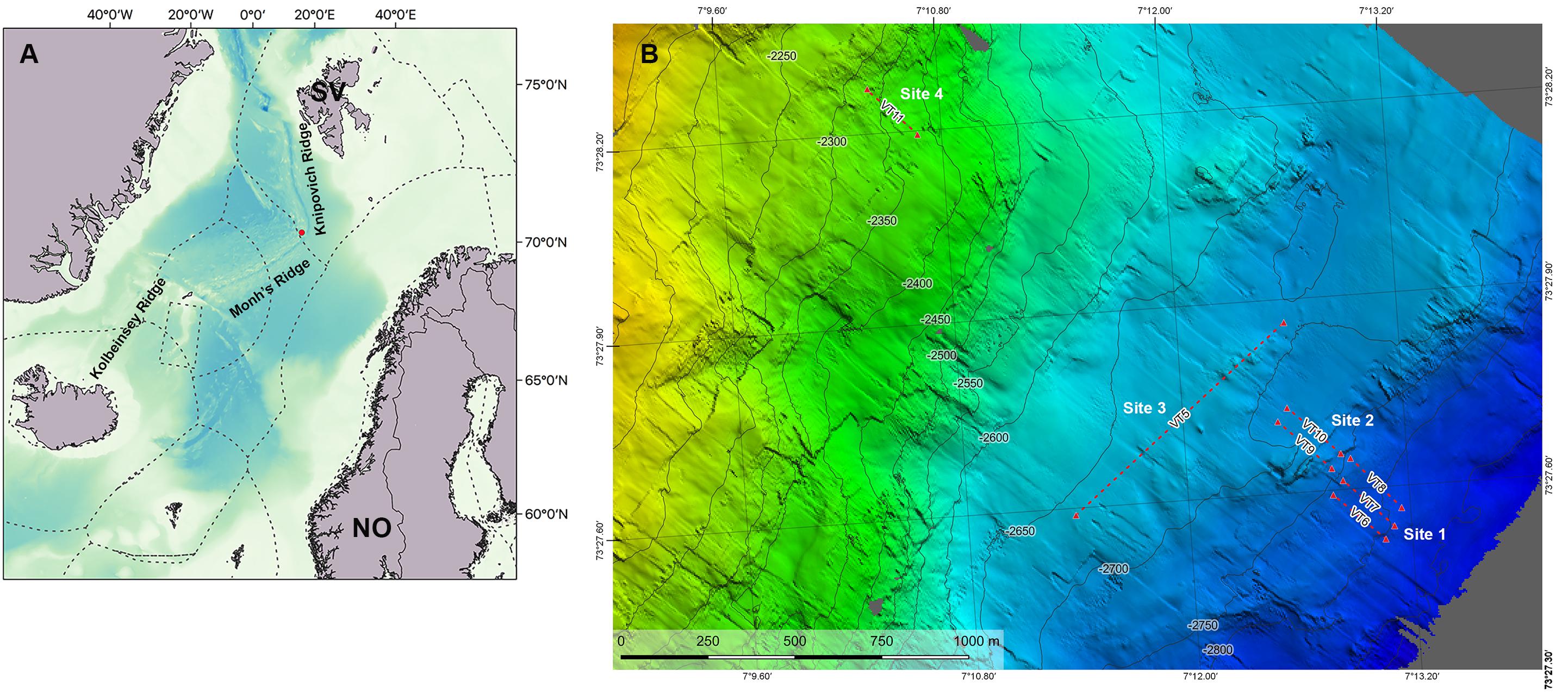
Figure 1. Map of the study area. (A) Regional map showing the Arctic Mid-Ocean Ridge with the location of Mohn’s Treasure (red dot) and the three main ridges: KOR, Kolbeinsey Ridge; MR, Mohn’s Ridge; KR, Knipovich Ridge on the Mohn’s Treasure. (B) Bathymetry of the study area showing the video transects (VT). Mohn’s Treasure map made by Christian Malmquist, NTNU AUR Lab.
Interest in potential seabed mineral resources has increased rapidly in the last decades, and 30 exploration licenses are currently (November 2019) active within international waters, seven of which are for SMS deposits (ISA, 2019). The International Seabed Authority (ISA) Mining Code that will regulate mineral exploitation in The Area (i.e., the seafloor in the area beyond national jurisdiction) is being developed based on the United Nations Convention on the Law of the Sea (UNCLOS). The Mining Code contains environmental regulations that should ensure that the marine environment is protected from any harmful effects, which may arise during mining activities, including, among others, a precautionary approach and the use of best environmental practices such as environmental impact assessments (EIAs), baseline data collection that follows an ecosystem-based approach and monitoring programs (Jaeckel, 2015; UNCLOS articles 137, 145, 153). Norway’s interest in its potential seabed resources has also increased rapidly, and a new “Act on Mineral Activities on the Continental Shelf” entered into force on 1 July 2019, regulating all exploration for and exploitation of subsea minerals on the Norwegian Continental Shelf (Seabed Mineral Law, 2019). The act includes provisions to avoid damage to the marine environment and seabed cultural heritage, as well as avoiding pollution and litter. These provisions include, among others, the requirement for an EIA prior to opening a geographic area for mineral activity. The EIA should contribute to highlight interests from different stakeholders in the area, identify to what degree the mining activity would impact the environment, and estimate potential industrial, economic, and societal impact. However, knowledge on the biological composition, ecological functions, and population connectivity of microbial and faunal communities at many vent sites in international waters and most of the AMOR active and inactive sites within Norwegian jurisdiction is still utterly scarce (Eilertsen et al., 2018), strongly limiting the robustness of any environmental regulations and/or management measures. This is particularly true for inactive sites, as most research so far has focused on the abundant and exotic communities of active vents. Van Dover (2019) reviews current knowledge of inactive sites worldwide and highlights the limited geological and ecological understanding currently available of these habitats and a lack of consensus of what characterizes inactive hydrothermal sites. No biological information was available for Mohn’s Treasure prior to this study. The aim of this study was to provide the first biological data of the region by assessing the faunal composition, community structure, and biodiversity of the megabenthic community to provide initial baseline information that can inform management measures related to seabed mining in the AMOR region.
Materials and Methods
Study Area
The Mohn’s Treasure site is situated at 73°44′N–07°27′E, at the edge of an inner rift wall 30 km southwest of the active Loki’s Castle vent field, at 2,600- to 2,800-m depth (Figure 1). All data were gathered on board the multipurpose subsea vessel CSV Polar King during a research cruise in the framework of the MarMine project in the summer of 2016. The study area was divided into four sampling sites, based on differences in depth and seafloor steepness identified from the low-resolution multibeam data that were available prior to the cruise (Figure 1). Site 1 comprises the deepest parts of the Mohn’s Treasure region, with a gentle slope at ca. 2,810-m depth. Site 2 is located between 2,745- and 2,750-m depth and is located at the start of what resembles a plateau because of its very low steepness. Site 3 is in the middle of the plateau at 2,720 m, and Site 4 is located at 2,385-m depth and has the greatest steepness gradient.
Sampling
All data were gathered during systematic remotely operated vehicles (ROVs)-based video/photo transects using low light navigation cameras Kongsberg OE13-124 and a CCD color camera Kongsberg 14-366 for still photos (Ludvigsen et al., 2016) mounted on a Triton work-class ROV (XLR 02). The ROV was equipped with two green lasers (532 nm), projecting two parallel lines on the seafloor with a distance of 10 cm, which were used to calibrate the field of view and size of object of interest. The video transects (VTs) were conducted flying the ROV at 1 m above the seafloor at a constant speed of 0.4 knots (0.2 m s–1), using the approach detailed by Ludvigsen et al. (2016). Because of the low resolution of the video cameras on the ROV, color photographs were taken at ca. 15-s interval (one photo every 3 m of seafloor). A total of eight transects were conducted (Table 1), with one transect of 800-m length (VT5, Site 3) perpendicular to the other shorter transects (200-m length): three replicates (VT6–VT8) at Site 1, two replicates (VT9–VT10) at Site 2, and two replicates (VT11–VT12) at Site 4. However, we had a technical issue in the still camera during VT12, so this transect is not included in the analyses. Between 68 and 108 images were taken during transects VT6–VT11, but overlapping images were excluded from the data set (Table 1). On transect VT5, 560 images were taken, but only 70 randomly selected photographs (average of number of photographs analyzed from the other transects) were included in the analyses. The field of view (area covered by each image in m2) was calculated based on the ROV height above seafloor, seafloor angle, camera angle, image width/height, and camera vertical/horizontal field of view.
Analyses
The transects were analyzed both qualitatively and quantitatively. All specimens observed were identified to the lowest taxonomic level possible from the ROV images, based on expert knowledge (HR, TB, and EP) and additional literature (Clark, 1970; Clark and Downey, 1992; Madsen and Hansen, 1994; Cárdenas et al., 2013; Hestetun et al., 2017; Plotkin et al., 2018).
All individuals in each image along each transect were identified to the lowest taxonomic level possible and counted. The total abundance within a transect was divided by the transect area (Table 1) to obtain the density (ind.m–2) for each morphospecies per transect. The density data were grouped by phylum for graphic representation (Table 2). The community structure was assessed with multidimensional scaling (MDS) using PRIMER v.6 based on Bray–Curtis similarity and square-root transformation of the morphospecies density matrix. Basic biodiversity indices [Margalef’s species richness, Shannon–Weaver diversity, ES(50), and Pielou’s evenness] were computed for each transect based on morphospecies data. The lack of replicates in Sites 3 and 4 did not allow conducting statistical analyses so the data could only be compared qualitatively among sites.
Results
Environmental and Biological Observations
The ROV video surveys analyzed four sites at different depth ranges on Mohn’s Treasure (Table 1). The survey showed a highly sedimented habitat as would be typical for lower bathyal and abyssal regions, with sparse rocky outcrops (Figure 2). Site 1 in the deeper surveyed area (average depth 2,783 m) was covered by soft sediments. At the end of the transects in Site 1 (VT6, VT7, and VT8), we came across a small rocky ridge, with hard substrate making up approximately 8% of the area. Site 2 started at this ridge and progressed to shallower depths (average depth 2,724 m) on a steeper sedimented slope. Hard substrate covered approximately 22% of the surveyed area. Site 3 (average depth 2,678 m) was surveyed by a long (800 m) perpendicular transect to the other transects, along a low steepness area covered with fine sediment. This area was intercepted by rocky outcrops (10%) colonized by sponges and associated fauna. Site 4 was the shallowest area surveyed, on a steeper slope (average depth 2,353 m). This region had a mixture of coarse and fine sediment with more rocky outcrops. Site 4 had approximately 35% of its surface represented by hard substrate.
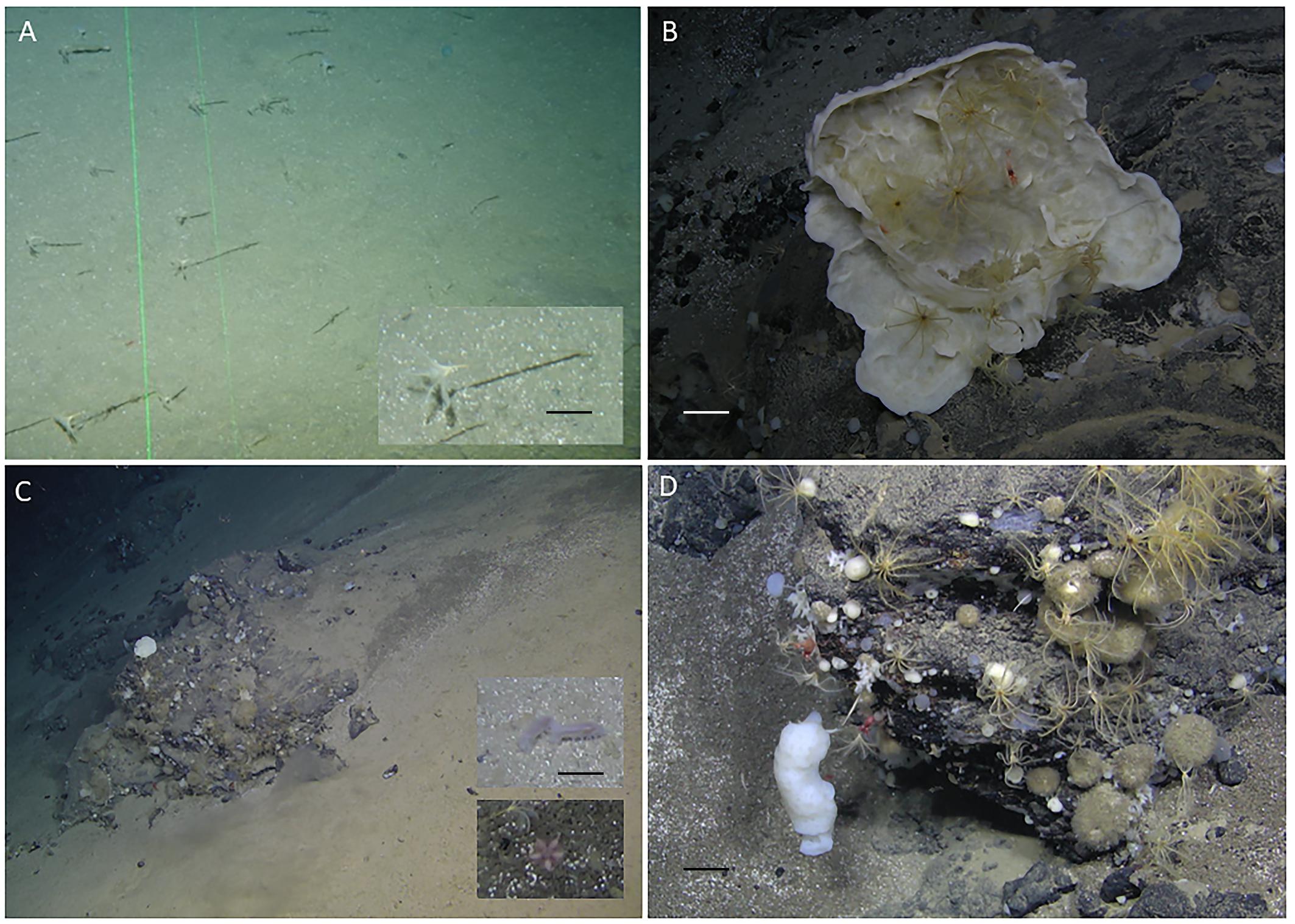
Figure 2. Mohn’s Treasure fauna. (A) Sedimentary plain at Site 1 with dense aggregations of the stalked crinoid Bathycrinus carpenterii. Insert: close-up of one individual (scale bar = 2 cm). (B) Rocky outcrop with sponges and associated fauna on Site 2 (scale bar = 10 cm). (C) Site 3 was dominated by fine sediments with holothurians and asteroids as well as rocky outcrops with sponges. The insert shows the holothurian Kolga species and the asteroid Hymenaster pellucidus (scale bar = 5 cm). (D) Site 4 was dominated by hard substrates with high abundance of filter feeders, in particular sponges (scale bar = 10 cm).
In general, areas of soft sediment were mainly inhabited by stalked crinoids and mobile fauna such as holothurians, asteroids, and crustaceans, whereas the rocky outcrops were colonized by abundant sponges of different species/morphotypes, non-stalked crinoids, and crustaceans. Site 1 was mostly covered by high densities (21.4 ind.m–2) of the stalked crinoid Bathycrinus carpenterii on the soft sediment (Figure 2A). This species was observed forming in dense crinoid fields in the deepest part of Monh’s Treasure, both along the VTs and during other ROV dives that were not dedicated to linear VTs. Site 2 included also large abundances of stalked crinoids on the sediment, while sponges and associated fauna dominated the rocky outcrops (Figure 2B). Site 3 was covered mostly by fine sediment with deposit feeders such as holothurians and asteroids, and rocky outcrops with sponges (Figure 2C). Site 4 had a higher proportion of hard substrate and the communities shifted to more sessile, filter feeder communities, mostly of a variety of sponges, crinoids, and associated fauna (Figure 2D).
Community Structure
A total of 491 ROV photos were analyzed, comprising a seafloor area of 741 m2 (Table 1) with mean area per photo of 1.51 ± 0.41 m2 (±SD). The mean density per site varied from 12.2 to 31.6 ind. m–2 in Sites 3 and 2, respectively (Figure 3). A total of 46 species or morphotypes were identified, belonging to seven phyla. Porifera was the most diverse phylum with 25 different species/morphotypes, followed by Echinodermata with seven different species/morphotypes. Porifera and Echinodermata were also the most abundant groups in all sites representing, respectively, 42 and 47% of the total density. Sponges were most abundant in Site 2 (16.8 ind.m–2), Site 3 (9.1 ind.m–2), and Site 4 (22.4 ind.m–2), whereas echinoderms were most abundant in Site 1 (22.6 ind.m–2). Within these two phyla, the sponges Lissodendoryx complicata and Hymedesmia sp. were the most abundant in all transects, representing 17% of all observed individuals, and the stalked crinoid B. carpenterii was the most abundant echinoderm, representing 43% of all observations. The latter was particularly abundant in Site 1, reaching an average density of 21.4 ind.m–2.
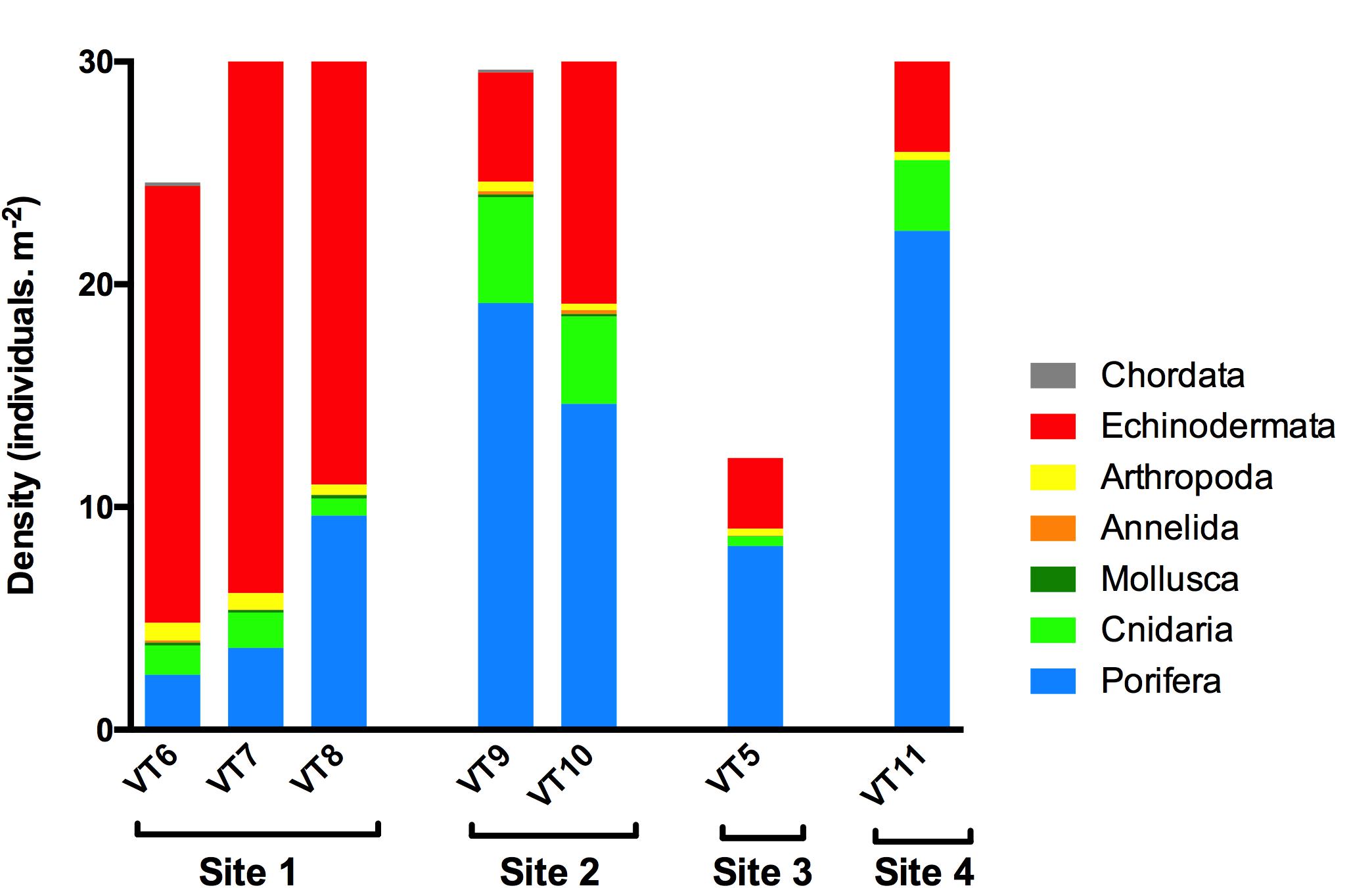
Figure 3. Density (individuals.m–2) of the different phyla observed during the photo transects on Mohn’s Treasure.
The species accumulation curves indicated that the 200-m VTs were a good representation of the megafauna community found in the surveyed area of Mohn’s Treasure. Most of the curves started leveling off after 75 m (25 photos) of survey (Figure 4). In VT5, the species accumulation curve had a steeper increase in number of species, potentially caused by the number of rocky ridges that were crossed by this longer transect. The multidimensional analysis showed that the communities from the three transects in Site 1 and the two transects in Site 3 grouped well together and were different from the communities in Sites 3 and 4 at 60% similarity (Figure 5).
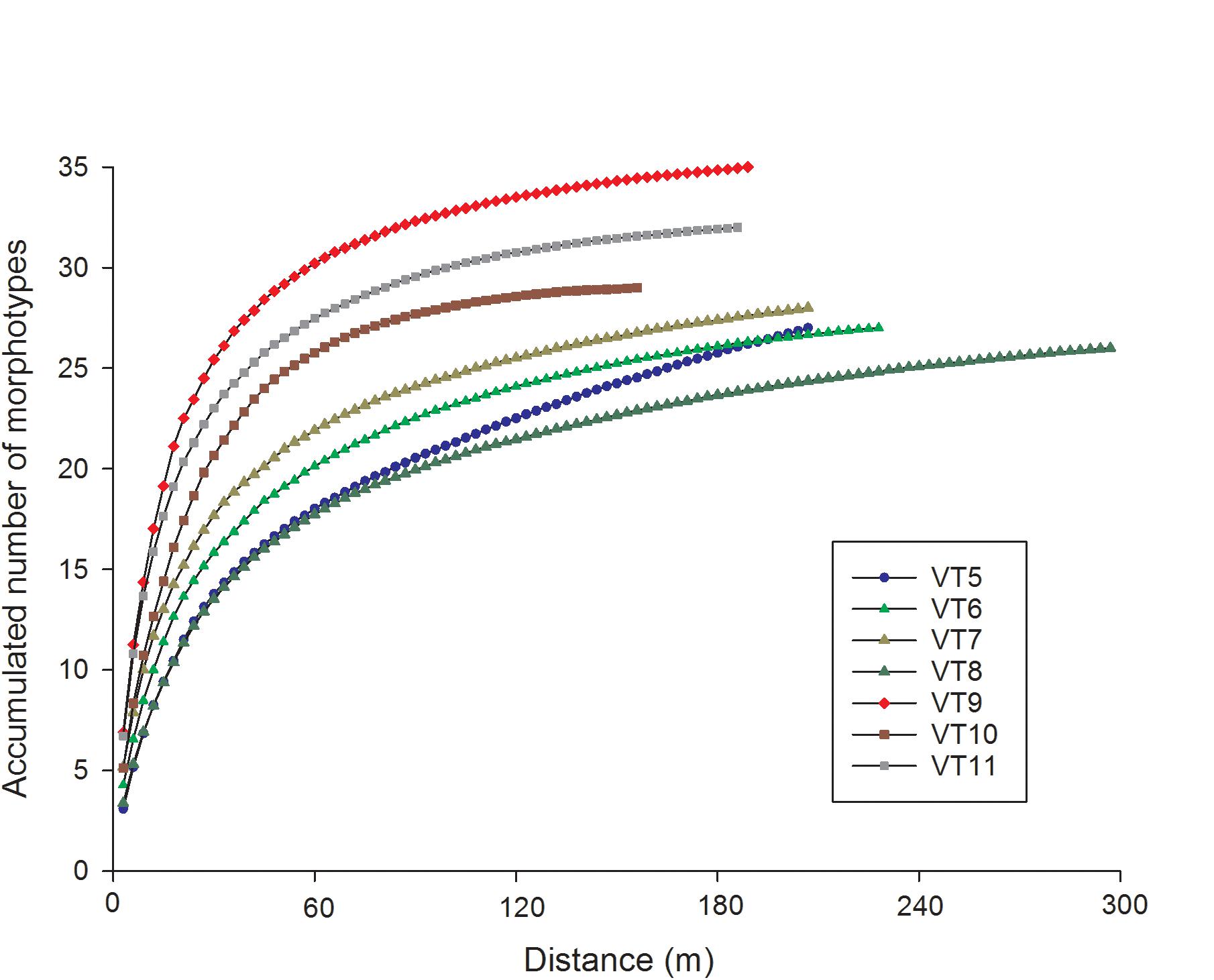
Figure 4. Species accumulation curves for the six ROV transects in Site 1 (VT6, 7, and 8), Site 2 (VT9 and 10), Site 3 (VT5), and Site 4 (VT11).
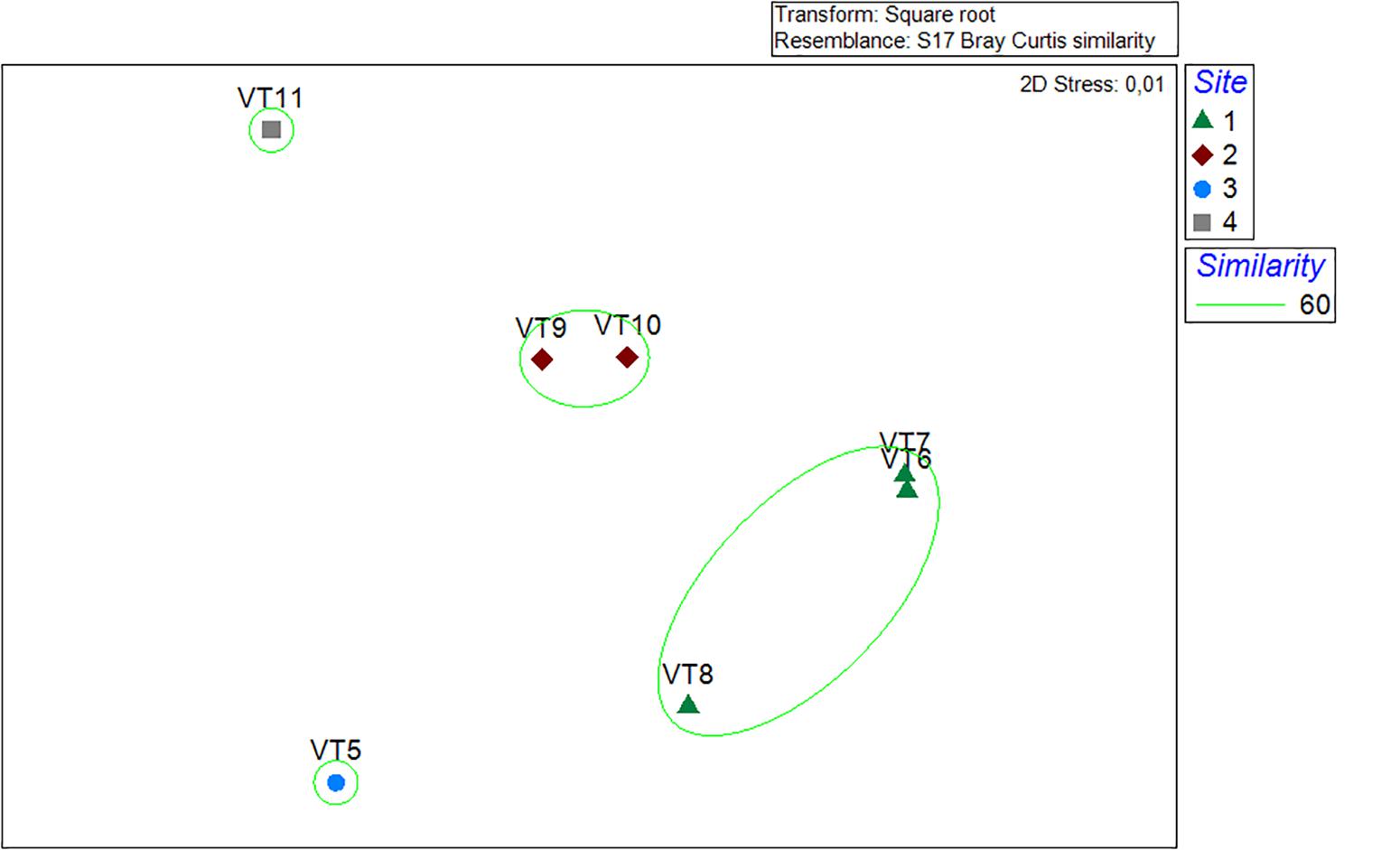
Figure 5. Multidimensional scaling plot. Green circle represents 60% similarity. Site 1: VT6, 7, and 8. Site 2: VT8 and 9. Site 3: VT5. Site 4: VT11.
Biodiversity
Although no statistical analyses could be conducted because of the lack of replicates in Sites 3 and 4, the four computed indices followed a similar pattern, with Margalef’s species richness, Pielou’s evenness, Shannon diversity, and ES(30) lower at Site 1 (Figure 6). This site was characterized by fields of stalked crinoids, which explain the low species richness, the low evenness caused by the dominance of one species, and low Shannon diversity.
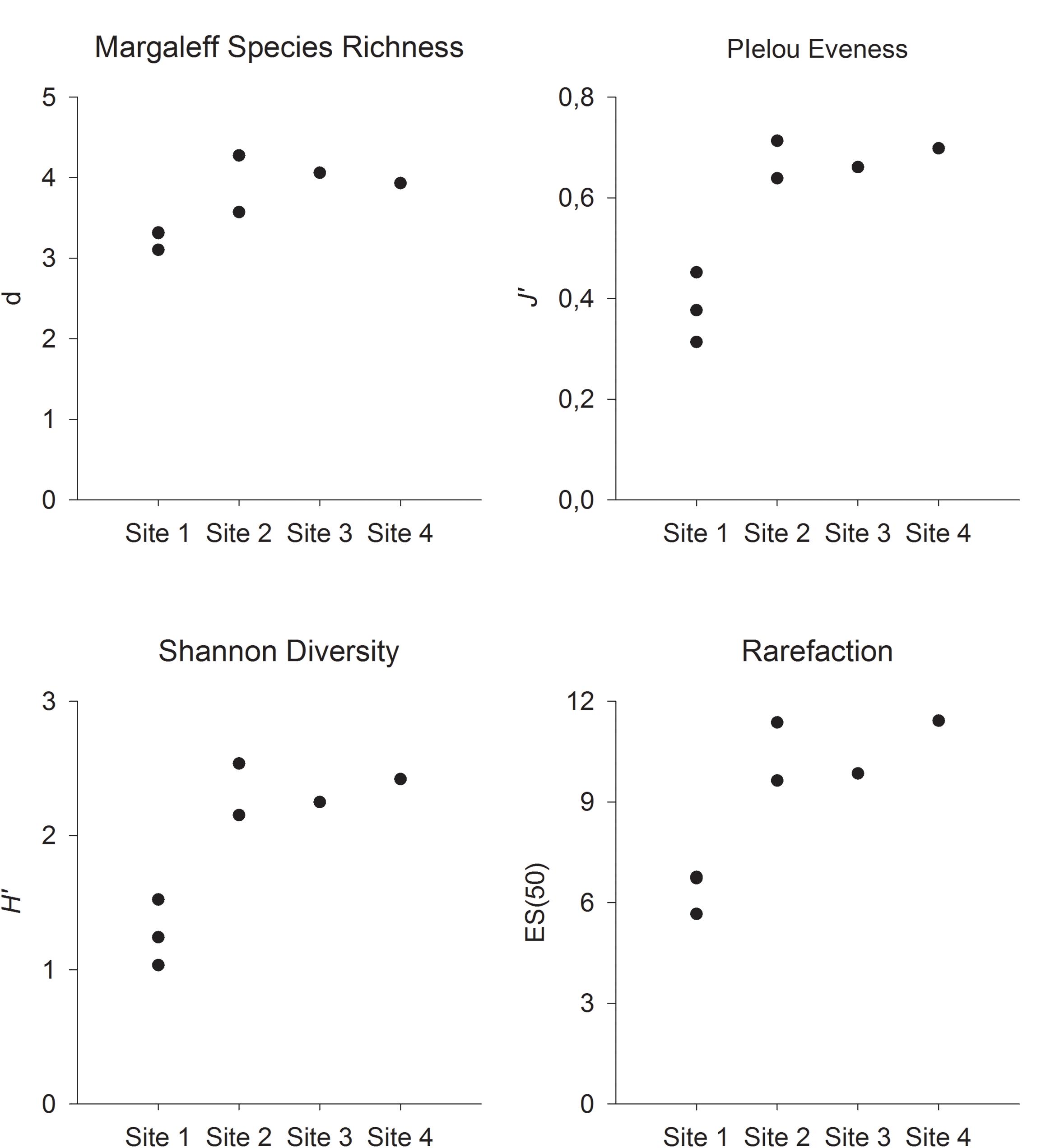
Figure 6. Margalef’s species richness (d), Pielou’s evenness (J’), Shannon biodiversity (H’), and estimated number of species (ES50) at the four sites on Mohn’s Treasure. Site 1: VT6, 7, and 8. Site 2: VT8 and 9. Site 3: VT5. Site 4: VT11.
Discussion
Although Mohn’s Treasure has been described as a massive sulfide deposit, the only evidence of mineralization found during the entire MarMine survey over the area (13 ROV dives) was a few meters of oxidized basalt outcrops comprising minor pyrites and features possibly formed by diffuse discharges (Ludvigsen et al., 2016). Subsequent analyses of magnetic data collected during the cruise suggest the presence of deposits buried under 15 m of sediments (Lim et al., 2019). The general seascape was that of a sedimented rift valley, with the seafloor composed mainly of soft sediment with basalt outcrops. Although biological data of the region around Monh’s Treasure are relatively scarce, the fauna observed in our survey resembles that of communities described from other lower bathyal Arctic regions such as the Shultz Bank, a seamount to the east of Mohn’s Treasure (Meyer et al., 2019), or a deep-water reef in the Fram Straight, west of Svalbard (Meyer et al., 2014). In all these regions, echinoderms dominate the sedimentary habitats, whereas suspension feeders, mostly sponges, and their associated fauna such as crinoids and arthropods dominate the hard substratum (Meyer et al., 2014, 2019; Roberts et al., 2018). In Mohn’s Treasure, the most abundant taxa were sponges, representing 42% of the fauna and dominated by desmosponges, similar to what has been described for the Shultz Bank communities (Meyer et al., 2019), and echinoderms in the lower region of Mohn’s Treasure where high-density fields of the stalked crinoid B. carpenterii were observed. The fauna is very different from that of the active Loki’s castle vent field, which is characterized by chemosynthetic-based fauna, including the amphipod Exitomelita sigynae, the siboglinid worm Sclerolinum contortum, and the rissoid gastropod Pseudosetia griegi (Tandberg et al., 2011, 2018; Kongsrud and Rapp, 2012; Olsen et al., 2016; Kongsrud et al., 2017). The Mohn’s Treasure fauna differs also from the communities described from the Seven Sisters and Jan Mayen vent fields. The fauna in these active, upper-bathyal hydrothermal vents (120–700 m) is characterized by non-vent endemic fauna dominated by anemones and calcareous sponges (Schander et al., 2010; Sweetman et al., 2013). The difference to the Mohn’s Treasure fauna is probably related to depth and enhanced food supply at the active vents through microbial production (Sweetman et al., 2013). Within Mohn’s Treasure, the differences observed among the sites in the MDS can reflect local variability, where the VT crossed one or more rocky ridges at which different sponge species dominate (Beazley et al., 2018; Meyer et al., 2019). The sponge grounds support a rich community of other invertebrates, providing habitat and food (Maldonado et al., 2016). In contrast, the sedimentary areas are characterized by scarce megafauna that can be analyzed from ROV imaging, with only some echinoderms or fish observed sporadically. In addition, the deeper site surveyed at Mohn’s Treasure was characterized by fields of the stalked crinoid B. carpenterii, not observed at the other sites. These differences result in lower biodiversity indices at the deepest site where B. carpenterii dominates.
In relation to the increasing interest for seabed minerals, seven licenses for exploration of SMS deposits in areas beyond national jurisdiction have been granted by the ISA (2019), whereas exploration is also rapidly developing in national waters of some countries such as Japan and Norway. Following the publication of the Norwegian Minerals Act in July 2019 (Seabed Mineral Law, 2019), interest in the seafloor mineral resources of Norway has been increasing dramatically, leading to two exploration cruises in the AMOR, in 2018 and 2019. Although previously described as a massive sulfide deposit (Pedersen et al., 2010b), no resource exploration has been conducted at Mohn’s Treasure to date. In addition, our ROV observations and magnetic data collected during the MarMine cruise (Lim et al., 2019) indicate that this is a highly sedimented system, which would not be, in principle, interesting to mineral exploration in the current technological and economical context. However, Mohn’s Treasure is situated only 30 km to the south of Loki’s Castle active hydrothermal vent field and other active vents with potential sulfide deposits, which have been identified along the Mohn’s Ridge since 2015 (Olsen et al., 2016). The exploitation of mineral resources from the seabed is expected to lead to widespread environmental impacts. These include both direct impacts on the targeted habitat and associated fauna caused by the mining machinery and indirect impacts on adjacent ecosystems caused by the creation and dispersal of sediment plumes with high concentrations of particles, potentially including the dispersal of toxic chemicals (Boschen et al., 2013; Van Dover et al., 2018). If commercial exploration for, and subsequent exploitation of, mineral resources was to be conducted in the AMOR region, robust environmental baseline studies and EIAs will need to be conducted. Our survey provided the first biological study of the Mohn’s Treasure region that can be used to design and support future baselines studies in the region.
In the framework of a full-scale baseline study of the region, an important component would be the assessment of the benthic communities, including the potential presence of vulnerable marine ecosystems (VMEs) (Burgos et al., 2020). VMEs are characterized by the uniqueness or rarity of the ecosystem, its fragility, the functional significance of its habitat, and the presence of key species with life-history traits that make recovery difficult and structural complexity (FAO, 2009). The VME guidelines identify a series of taxa as indicators, including, among others, cold-water corals, sponges, ophiuroids and stalked crinoids, bryozoans, ascidians, brachiopods, and xenophyophore foraminifera (FAO, 2019). Dense aggregations of stalked crinoids are considered VMEs when present in dense aggregations, as they can provide habitat for small invertebrates and are vulnerable to physical impact (Murillo et al., 2011). In our survey of Mohn’s Treasure, we observed dense aggregations of the stalked crinoid B. carpenterii with 21.4 individuals per m2, whereas other observations in the NE Atlantic/Arctic of B. carpenterii report much lower densities: 0.76 ind.m–2 on the Hausgarten Observatory in the Fram Strait (Taylor et al., 2016) and 1.3 ind.m–2 on a deep-water reef in the Fram Strait (Meyer et al., 2014). Although our survey of the lower Mohn’s Treasure area was limited to three transects, we suggest that the observations of dense fields of stalked crinoids, together with the presence of sponges aggregations on rocky outcrops throughout our transects, would need to be taken under consideration prior to any commercial activity for the exploitation of seabed minerals in the central Mohn’s Ridge. In the last years, interest on the mineral resources along the AMOR within Norwegian jurisdiction has increased rapidly, as evidenced by the two seabed mineral mapping surveys of the Norwegian Petroleum Directorate on behalf of the Ministry of Petroleum and Energy in 2018 and 2019. Although mining exploitation on Mohn’s Treasure seems unlikely currently, as any potential resources would be covered by a thick (ca. 15 m) sediment layer (Lim et al., 2019), new sites may be found in proximity. The potential exploitation of mineral resources in the region may result in harmful effects on the crinoid and sponge communities that we observed and on the ecosystem services they provide, such as nutrient regeneration and habitat provision. For example, close associations between ground-forming sponges and specialized microbes have proven to be key players in the biogeochemical cycling of nutrients along the ridge (Rooks et al., 2020). Stressors such as enhanced sedimentation or release of bioavailable metals caused by commercially exploited seabed minerals may have serious effects on ecosystem function, potentially changing these sponge communities from being nutrient sources to nutrient sinks (Rooks et al., 2020).
In addition, it is already well established that hydrothermally active and non-active areas of the AMOR host unique and poorly studied macrofauna, as well as a wide diversity of novel and uncultured microbial biodiversity (e.g., Dahle et al., 2015, 2018; Steen et al., 2016; Stokke et al., 2020). Metagenomic studies from the AMOR have identified novel archaeal lineages, which have challenged our views on the origin and evolution of eukaryotes and the topology of the tree of life (Spang et al., 2015). In addition, recent in situ enrichment and culture of rare sedimentary microbes at AMOR vents have revealed great potential for biodiscovery of novel enzymes for use in biorefining and bioconversion of industrially relevant substrates such as those produced in fish farming and wood-pulping industries (Stokke et al., 2020). Given the high degree of novel findings during work in the area over the most recent years, it is likely that they represent only the tip of the iceberg when it comes to the amount of potentially valuable genetic resources present in the AMOR region. Potential biodiversity loss from seabed mining (Niner et al., 2018), particularly in poorly investigated regions such as the AMOR, may result in the loss of biological knowledge, ecosystem services, and valuable marine genetic resources before we know them (Arrieta et al., 2010; Rabone et al., 2019).
Biological surveys such as the one conducted in this study are essential as a first step toward robust, ecosystem approach, baseline studies that would improve understanding of the ecosystems composition and functioning and their response to stressors (Levin and Le Bris, 2015). Although our study had limitations in terms of replication of transects and analyses of other community components (microbes and meio- and macro-infauna), we provide a first overview of the megabenthos communities of the Mohn’s Treasure and in particular observations of dense stalked crinoid populations and abundant sponge aggregations, which can inform future studies and instigate a dialog with the relevant stakeholders, including industry, authorities, and non-governmental organizations. Conducting thorough environmental baselines and EIAs of these poorly known regions, including both the target area and adjacent areas that may be indirectly impacted by exploitation activities, are necessary to avoid harmful effects to the ecosystem and the services it provides (Van Dover, 2010; Boschen et al., 2013; Van Dover et al., 2018). In addition to currently used technologies and methods for environmental baselines and EIAs, the development of new technologies such as the use of autonomous underwater vehicles (AUVs) and hyperspectral cameras mounted on AUVs and ROVs (Dumke et al., 2018) will greatly contribute to time- and cost-effective large-scale surveys of unknown regions, such as the AMOR, and their faunal communities. These data will provide essential information that is necessary to develop management measures for seabed mining.
Data Availability Statement
This article contains previously unpublished data. The name of the repository and accession number(s) are not available. The data can be available upon request to the lead author.
Author Contributions
EP and CC conducted the image analyses and preliminary data analyses. All authors contributed to the in-depth data analyses and to the writing of the manuscript.
Dedication
This manuscript is dedicated to the memory of Hans Tore Rapp, who passed away on 7 March 2020.
Funding
The research was conducted in the framework of the project MarMine, funded by the Research Council of Norway (Project No. 247626/O30) and associated industrial partners. AH was funded by CESAM (UIDP/50017/2020 + UIDB/50017/2020) that is financed by FCT/MCTES through national funds. HR was funded by the European Research Council (ERC) under the European Union’s Horizon 2020 research and innovation program (Grant Agreement No. 679849; the SponGES project). GJ was supported by the Research Council of Norway through the Centres of Excellence funding scheme (Grant No. 223254–NTNU AMOS).
Conflict of Interest
The authors declare that the research was conducted in the absence of any commercial or financial relationships that could be construed as a potential conflict of interest.
Acknowledgments
The authors would like to acknowledge the support of the Officers, crew, and scientific party on board CVS Polar King during the MarMine cruise for assistance at sea. The authors would like to thank Stein Nornes Melvaer and Øystein Sture (NTNU) for their assistance in the calculation of the image field of view area. We thank Christian Malmquist (NTNU AUR Lab) for making the Mohn’s Treasure map in Figure 1. The authors would like to thank the two reviewers for their valuable comments that have contributed to considerably improving the manuscript.
References
Arrieta, J. M., Arnaud-Haond, S., and Duarte, C. M. (2010). What lies underneath: conserving the oceans’ genetic resources. PNAS 107, 18318–18324. doi: 10.1073/pnas.0911897107
Beazley, L. I., Wang, Z., Kenchington, E. L., Yashayaev, I., Rapp, H. T., Xavier, J. R., et al. (2018). Predicted distribution and climatic tolerance of the glass sponge Vazella pourtalesi on the Scotian Shelf and its persistence in the face of climatic variability. PLoS One 13:e0205505. doi: 10.1371/journal.pone.0205505
Boschen, R. E., Rowden, A. A., Clark, M. R., and Gardner, J. P. A. (2013). Mining of deep-sea seafloor massive sulfides: a review of the deposits, their benthic communities, impacts from mining, regulatory frameworks and management strategies. Ocean Coast. Manag. 84, 54–67. doi: 10.1016/j.ocecoaman.2013.07.005
Burgos, J. M., Buhl-Mortensen, L., Buhl-Mortensen, P., Ólafsdóttir, S. H., Steingrund, P., and Ragnarsson, S. Á, et al. (2020). Predicting the distribution of indicator taxa of vulnerable marine ecosystems in the Arctic and Sub-Arctic waters of the Nordic Seas. Front. Mar. Sci. 7:131. doi: 10.3389/fmars.2020.00131
Cárdenas, P., Rapp, H. T., Klitgaard, A. B., Best, M., Thollesson, M., and Tendal, O. S. (2013). Taxonomy, biogeography and DNA barcodes for Geodia species (Porifera, Demospongiae, Astrophorida) in the Atlantic Boreo-Arctic region. Zool. J. Linnean Soc. 169, 251–311. doi: 10.1111/zoj.12056
Clark, A. M., and Downey, M. E. (1992). Starfishes of the Atlantic. Natural History Museum Publications. London: Chapman and Hall.
Dahle, H., Le Moine Bauer, S., Baumberger, T., Stokke, R., Pedersen, R. B., Thorseth, I. H., et al. (2018). Energy landscapes in hydrothermal chimneys shape distributions of primary producers. Front. Microbiol. 9:1570. doi: 10.3389/fmicb.2018.01570
Dahle, H., Økland, I. E., Thorseth, I. H., Pedersen, R. B., and Steen, I. H. (2015). Energy landscapes shape microbial communities in hydrothermal systems on the Arctic Mid-Ocean Ridge. ISME J. 9, 1593–1606. doi: 10.1038/ismej.2014.247
Dumke, I., Purser, A., Marcon, Y., Nornes, S. M., Johnsen, G., Ludvigsen, M., et al. (2018). Underwater hyperspectral imaging as an in situ taxonomic tool for deep-sea megafauna. Sci. Rep. 8:12860. doi: 10.1038/s41598-018-31261-4
Eilertsen, M. H., Georgieva, M. N., Kongsrud, J. A., Wiklund, H., Glover, A. G., and Rapp, H. T. (2018). Genetic connectivity from the Arctic to the Antarctic: Sclerolinum contortum and Nicomache lokii (Annelida) are both widespread in reducing environments. Sci. Rep. 8:4810. doi: 10.1038/s41598-018-23076-0
FAO (2009). International Guidelines For The Management Of Deep-Sea Fisheries In The High Seas. Rome: Food and Agricultural Organisation of the United Nations.
FAO (2019). VME Indicators, Thresholds And Encounter Responses Adopted By R(F)Mos In Force During 2019. Avaliavle at http://www.fao.org/in-action/vulnerable-marine-ecosystems/vme-indicators/en/ (accessed December 3, 2019).
Hestetun, J. T., Tompkins-MacDonald, G., and Rapp, H. T. (2017). A review of carnivorous sponges from the boreal North Atlantic and Arctic Oceans. Zool. J. Linnean Soc. 181, 1–69. doi: 10.1093/zoolinnean/zlw022
ISA (2019). International Seabed Authority Website. Available online at: https://www.isa.org.jm/deep-seabed-minerals-contractors?qt-contractors_tabs_alt=1#qt-contractors_tabs_alt (accessed November 15, 2019).
Jaeckel, A. (2015). An environmental management strategy for the international seabed authority? the legal basis. Intern. J. Mar. Coast. Law 30, 1–27.
Kongsrud, J. A., and Rapp, H. T. (2012). Nicomache (Loxochona) lokii sp. nov. (Annelida, Polychaeta, Maldanidae) from the Loki’s Castle vent field – an important structure builder in an Arctic vent system. Pol. Biol. 35, 161–170. doi: 10.1007/s00300-011-1048-4
Kongsrud, J. A., Eilertsen, M. H., Alvestad, T., and Rapp, H. T. (2017). Two new species of Ampharetidae (Polychaeta) from the loki castle vent field. Deep Sea Res. Part II 137, 232–245. doi: 10.1016/j.dsr2.2016.08.015
Levin, L. A., and Le Bris, N. (2015). The deep ocean under climate change. Science 350, 766–768. doi: 10.1126/science.aad0126
Lim, A., Bronner, M., Johansen, S. E., and Dumais, M. A. (2019). Hydrothermal activity at the ultraslow-spreading Mohns ridge: new insights from near-seafloor magnetics. Geochem. Geophys. Geosyst. 20, 5691–5709. doi: 10.1029/2019GC008439
Ludvigsen, M., Aasly, K., Ellefmo, S. L., Hilário, A., Ramirez-Llodra, E., Søreide, F. X., et al. (2016). MarMine Cruise Report-Arctic Mid-Ocean Ridge. Trondheim, NO: NTNU.
Madsen, F. J., and Hansen, B. (1994). Echinodermata Holothuroidea. Oslo: Scandinavian University Press.
Maldonado, M., Aguilar, R., Bannister, R. J., Bell, J. J., Conway, K. W., and Dayton, P. K., et al. (eds) (2016). “Sponge grounds as key marine habitats: a synthetic review of types, structure, functional roles, and conservation concerns,” in Marine Animal Forests: the Ecology of Benthic Biodiversity Hotspots, (Berlin: Springer), 1–39. doi: 10.1007/978-3-319-17001-5_24-1
Meyer, H. K., Roberts, E. M., Rapp, H. T., and Davies, A. J. (2019). Spatial patterns of arctic sponge ground fauna and demersal fish are detectable in autonomous underwater vehicle (AUV) imagery. Deep Sea Res. 153:103137. doi: 10.1016/j.dsr.2019.103137
Meyer, K. S., Soltwedel, T., and Bergmann, M. (2014). High Biodiversity on a deep-water reef in the Eastern fram strait. PLoS One 9:e105424. doi: 10.1371/journal.pone.0105424
Murillo, F. J., Kenchington, E., Sacau, M., Piper, D. J. W., Wareham, V., and Muñoz, A. (2011). New VME Indicator Species (Excluding Corals And Sponges) and Some Potential VME Elements of the NAFO Regulatory Area. SC WG on the Ecosystem Approach To Approach Fisheries Management. Dartmouth: Northwest Atlantic Fisheries Organization.
Niner, H. J., Ardron, J. A., Escobar, E. G., Gianni, M., Jaeckel, A., Jones, D. O. B., et al. (2018). Deep-sea mining with no net loss of biodiversity—an impossible aim. Front. Mar. Sci. 5:53. doi: 10.3389/fmars.2018.00053
Olsen, B. R., Økland, I. E., Thorseth, I. H., Pedersen, R. B., and Rapp, H. T. (2016). Environmental Challenges Related To Offshore Mining And Gas Hydrate Extraction. Norway: Norwegian Environment Agency.
Pedersen, R. B., Rapp, H. T., Thorseth, I. H., Lilley, M., Barriga, F., Baumberger, T., et al. (2010a). Discovery of a black smoker field and a novel vent fauna at the ultraslow spreading Arctic Mid-Ocean Ridges. Nat. Commun. 1, 1–6.
Pedersen, R. B., Thorseth, I. H., Nygård, T. E., Lilley, M. D., and Kelley, D. S. (2010b). “Hydrothermal activity at the arctid mid-ocean ridges,” in Diversity of Hydrothermal Systems on Slow Spreading Ocean Ridges. Geophysical Monographs Series, Vol. 118, eds P. A. Rona, C. W. Devey, J. Dyment, and B. J. Murton (Washington, DC: American Geophysical Union), 67–89.
Plotkin, A., Gerasimova, E., and Rapp, H. T. (2018). Polymastiidae (Porifera: Demospongiae) of the Nordic and Siberian Seas. J. Mar. Biol. Assoc. 98, 1273–1335. doi: 10.1017/S0025315417000285
Rabone, M., Harden-Davies, H., Collins, J. E., Zajderman, S., Appeltans, W., Droege, G., et al. (2019). Access to marine genetic resources (MGR): raising awareness of best-practice through a new agreement for biodiversity beyond national jurisdiction (BBNJ). Front. Mar. Sci. 6:520. doi: 10.3389/fmars.2019.00520
Roberts, E. M., Mienis, F., Rapp, H. T., Hanz, U., Meyer, H. K., and Davies, A. J. (2018). Oceanographic setting and short-timescale environmental variability at an Arctic seamount sponge ground. Deep Sea Res. I 138, 98–113. doi: 10.1016/j.dsr.2018.06.007
Rooks, C., Fang, J. K.-H., Mørkved, P. T., Zhao, R., Rapp, H. T., Xavier, J. R., et al. (2020). Deep-sea sponge grounds as nutrient sinks: denitrification is common in boreo-Arctic sponges. Biogeosciences 17, 1231–1245. doi: 10.5194/bg-17-1231-2020
Schander, C., Rapp, H. T., Kongsrud, J. A., Bakken, T., Berge, J., Cochrane, S., et al. (2010). The fauna of the hydrothermal vents on the Mohn Ridge (North Atlantic). Mar. Biol. Res. 6, 155–171. doi: 10.1080/17451000903147450
Seabed Mineral Law (2019). Lov om Mineralvirksomhet På Kontinentalsokkelen (Law On Mineral Activities On The Continental Shelf), LOV-2019-03-22-7 (in Norwegian), Norwegian Ministry of Oil and Energy. Available online at: https://lovdata.no/dokument/NL/lov/2019-03-22-7 (accessed April 2020).
Spang, A., Saw, J., Jørgensen, S., Zaremba-Niedzwiedzka, K., Martijn, K., Lind, A. E., et al. (2015). Complex archaea that bridge the gap between prokaryotes and eukaryotes. Nature 521, 173–179. doi: 10.1038/nature14447
Steen, I. H., Dahle, H., Stokke, R., Roalkvam, I., Daae, F. L., Rapp, H. T., et al. (2016). Novel barite chimneys at the Loki’s Castle vent field shed light on key factors shaping microbial communities and functions in hydrothermal systems. Front. Microbiol. 6:1510. doi: 10.3389/fmicb.2015.01510
Stokke, R., Reeves, E., Dahle, H., Fedoy, A.-E., Viflot, T., Onstad, S. L., et al. (2020). Tailoring hydrothermal vent biodiversity toward improved biodiscovery using a novel in situ enrichment strategy. Front. Microbiol. 11:249. doi: 10.3389/fmicb.2020.00249
Sweetman, A. K., Levin, L. A., Rapp, H. T., and Schander, C. (2013). Faunal trophic structure at hydrothermal vents on the southern Mohn’s Ridge, Arctic Ocean. Mar. Ecol. Prog. Ser. 473, 115–131. doi: 10.3354/meps10050
Tandberg, A. H. S., Vader, W., Olsen, B. R., and Rapp, H. T. (2018). Monoculodes bousfieldi n. sp. from the arctic hydrothermal vent Loki’s Castle. Mar. Biodiv. 48, 927–937. doi: 10.1007/s12526-018-0869-6
Tandberg, A. H., Rapp, H. T., Schander, C., Vader, W., Sweetman, A. K., and Berge, J. (2011). Exitomelita sigynae gen. et sp. nov.: a new amphipod from the Arctic Loki castle vent field with potential gill ectosymbionts. Pol. Biol. 35, 705–716. doi: 10.1007/s00300-011-1115-x
Taylor, J., Krumpen, T., Soltwedel, T., Gutt, J., and Bergmann, M. (2016). Regional- and local-scale variations in benthic megafaunal composition at the Arctic deep-sea observatory Hausgarten. J. Deep Sea Res. I 108, 58–72. doi: 10.1016/j.dsr.2015.12.009
Van Dover, C. L. (2010). Mining seafloor massive sulphides and biodiversity: what is at risk? ICES J. Mar. Sci. 68, 341–348. doi: 10.1093/icesjms/fsq086
Van Dover, C. L. (2019). Inactive sulfide ecosystems in the deep sea: a review. Front. Mar. Sci. 6:461. doi: 10.3389/fmars.2019.00461
Van Dover, C. L., Arnaud-Haond, S., Gianni, M., Helmreich, S., Huber, J. A., Jaeckel, A., et al. (2018). Scientific rationale and international obligations for protection of active hydrothermal vent ecosystems from deep-sea mining. Mar. Policy 90, 20–28. doi: 10.1016/j.marpol.2018.01.020
Keywords: deep-sea benthos, Arctic Mid-Ocean Ridge, inactive hydrothermal vent, seabed mining, vulnerable marine ecosystem
Citation: Ramirez-Llodra E, Hilario A, Paulsen E, Costa CV, Bakken T, Johnsen G and Rapp HT (2020) Benthic Communities on the Mohn’s Treasure Mound: Implications for Management of Seabed Mining in the Arctic Mid-Ocean Ridge. Front. Mar. Sci. 7:490. doi: 10.3389/fmars.2020.00490
Received: 06 December 2019; Accepted: 02 June 2020;
Published: 07 July 2020.
Edited by:
Sarah Mincks Hardy, University of Alaska Fairbanks, United StatesReviewed by:
Lauren Sutton, University of Alaska Fairbanks, United StatesMalcolm Ross Clark, National Institute of Water and Atmospheric Research (NIWA), New Zealand
Copyright © 2020 Ramirez-Llodra, Hilario, Paulsen, Costa, Bakken, Johnsen and Rapp. This is an open-access article distributed under the terms of the Creative Commons Attribution License (CC BY). The use, distribution or reproduction in other forums is permitted, provided the original author(s) and the copyright owner(s) are credited and that the original publication in this journal is cited, in accordance with accepted academic practice. No use, distribution or reproduction is permitted which does not comply with these terms.
*Correspondence: Eva Ramirez-Llodra, ZXZhLnJhbWlyZXpAbml2YS5ubw==