- 1Department of Integrative Biology, Oregon State University, Corvallis, OR, United States
- 2Department of Fisheries and Wildlife, Coastal Oregon Marine Experiment Station, Hatfield Marine Science Center, Oregon State University, Newport, OR, United States
- 3College of Earth, Ocean, and Atmospheric Sciences, Oregon State University, Corvallis, OR, United States
- 4Department of Mathematics, Oregon State University, Corvallis, OR, United States
Dungeness crab (Cancer magister) is one of the most lucrative fisheries on the United States (U.S.) west coast. There have been large spatial and temporal fluctuations in catch, which reflect the interconnected influences of the coupled natural-human fishery system. Changing ocean conditions are likely to further alter the magnitude and distribution of Dungeness crab catch, the impacts of which will propagate ecologically and through the social systems of fishing communities. Therefore, the effect of changing ocean conditions on U.S. west coast Dungeness crab catch per unit effort (CPUE) was used as an interdisciplinary case study to examine the susceptibility, a metric that integrates Dungeness crab reliance and social vulnerability indices, of coastal communities to changes in the fishery. Statistical models indicated that ocean conditions influence commercial CPUE 3–5 years later and that CPUE is likely to decline in the future as ocean conditions change. In particular, sea surface temperature scenarios for 2080 (+1.7 and +2.8°C) reduced Dungeness crab CPUE by 30–100%, depending on fishing port latitude. Declines in Dungeness crab CPUE were greater for southern port communities than for northern port communities under both scenarios – demonstrating greater exposure at the southern end of the species range. We show that U.S. west coast communities are differentially susceptible to a decline in Dungeness crab catch, with Washington communities being at least five times more susceptible than California communities. Our overall assessment showed varying levels of risk (a combination of exposure and susceptibility) for Dungeness crab fishing ports that do not necessarily align with regional or fishery management boundaries.
Introduction
Fisheries of the United States (U.S.) west coast are coupled natural-human systems that cross state borders, and integrate private and public sectors. They involve complex relationships between coastal socio-economics, resource management, and environmental factors. The integral role of humans in fishery systems has been formally recognized with a shift toward ecosystem-based management in natural resource programs (ORAP, 2013). In addition, social science research has begun to address vulnerabilities in fishing-reliant communities (Norman et al., 2007; Himes-Cornell et al., 2016; Moore et al., 2019). However, few studies employ the interdisciplinary techniques necessary to understand and address complex problems, like climate change, involving fisheries (except see Ekstrom et al., 2015; Mathis et al., 2015).
The consequences of changing ocean conditions due to climate change are already being experienced across fishery systems (Ritzman et al., 2018; Young et al., 2019). The impacts include, but are not limited to, decreased ocean production, altered food-web dynamics, and shifted species distributions (Hoegh-Guldberg and Bruno, 2010). While there are uncertainties in the spatial and temporal dimensions of future ocean conditions, there are clear trends toward a changing ocean in the California Current System (CCS) (Bograd et al., 2002; Snyder et al., 2003; Bograd et al., 2008; Gruber et al., 2012; Sydeman et al., 2013; Gentemann et al., 2017; Holsman et al., 2019). In particular, sea surface temperature (SST) is expected to increase both globally and within the CCS throughout the 21st century (Holsman et al., 2019). Under the relatively conservative RCP 4.5 W/m2 radiative forcing scenario, the CMIP5 multi-model ensemble predicts a 1.7 ± 0.4°C increase in SST within the CCS by 2080, but other scenarios (e.g., RCP 8.5 W/m2) predict up to a 2.8 ± 0.6°C SST increase by 2080 (reviewed in Jewett and Romanou, 2017). With changing SST and air temperature, other ocean conditions are predicted to change, including upwelling (Upw), physical spring transition (STI), and the way in which these local ocean conditions correlate with basin-scale indexes such as the Pacific Decadal Oscillation (PDO), El Niño Southern Oscillation (ENSO), and North Pacific Gyre Oscillation (NPGO) (Bograd et al., 2002; Bakun et al., 2015; Jacox et al., 2016; Litzow et al., 2020). Oceanographic changes within the next few decades will likely have substantial and cascading implications for ocean systems, fisheries, and coastal communities (Jones et al., 2015; Yen et al., 2016). Distribution shifts have already been documented in species found between 30 and 60°N, and many observed shifts have been correlated with global temperature increases (reviewed in Lenoir and Svenning, 2015). Despite prior expectations, species responses to climate change have not been uniform and species distribution shifts have occurred at different rates and directions (e.g., Perry et al., 2005; Nye et al., 2009; Pinsky et al., 2013; Cheung et al., 2015). Marine species, in particular, show considerable variation in the rate and direction of shifts due to the complexity of changing ocean conditions. This complexity results in considerable spatial variation in the magnitude and direction of ocean condition changes and, ultimately, species have tracked closely with local-scale differences (Pinsky et al., 2013). Understanding the distribution and abundance of marine species is necessary for implementing appropriate fishery management strategies and assessing the potential resilience of a fishery to environmental changes (Ritzman et al., 2018).
Therefore, we aimed to elucidate the impact of changing ocean conditions on coastal communities through the lens of a socio-economically important fishery in the CCS. In this study, we focused on commercial catch of Dungeness crab (Cancer magister) delivered to fishing communities in Washington, Oregon, and California. Currently, Dungeness crab is the most lucrative single species fishery on the U.S. west coast, generating about $200 million per year (Rasmuson, 2013; PSMFC, 2014). Potential impacts associated with climate and oceanographic changes and a resulting shift in the magnitude and distribution of Dungeness crab catch in the CCS, are expected to propagate through ecological and social systems, affecting policy, fishing industries and the greater well-being of many U.S. west coast communities. Major fishing ports for Dungeness crab on the U.S. west coast range from large cities like San Francisco, California to smaller, natural resource dependent communities like Port Orford, Oregon (NOAA, 2017). Some of these areas may be more reliant on the Dungeness crab industry than others, particularly where catch represents a considerable proportion of economic input to the community (PFMC, 2017).
Changes in ocean temperatures and currents may impact survival and dispersal of Dungeness crab larvae in the CCS, and subsequently the magnitude and distribution of commercial catch along the U.S. west coast. The 3- to 4-month pelagic larval stage of Dungeness crab is the most vulnerable life stage, and the crabs become increasingly resilient to environmental factors, like temperature, as they develop into adults (Ebert et al., 1983). In addition to thermally induced physiological effects on crab larvae, there can also be indirect effects, due to changing ocean currents that are correlated with changes in SST in the CCS (Botsford and Lawrence, 2002; Shanks et al., 2010; Shanks, 2013). Therefore, it is possible that the cumulative effect of increasing SST on larval Dungeness crab will alter the abundance and distribution of adults available for harvest within the CCS.
Management of Dungeness crab is atypical compared to other U.S. west coast fisheries because total allowable catch determinations are not based on calculations for sustainable yield and do not incorporate a formal stock assessment (Davis et al., 2017). Instead, managers estimate that the majority (>90%) of legal-sized adult (approximately 4 years of age) male crab are harvested every year (Botsford, 1984; Higgins et al., 1997; Armstrong et al., 2003; Rasmuson, 2013). Even with these uncertainties, the biological condition of the Dungeness crab stock is considered to be healthy based on sustained catch over the past 30+ years (Helliwell, 2009; Richerson et al., 2020). However, there have been large spatial and temporal fluctuations in historical commercial catch of Dungeness crab along the U.S. west coast over the past four decades that reflect the interconnected influences of biological, environmental, and social variability (reviewed in Rasmuson, 2013).
Using an interdisciplinary framework, we investigated how changing ocean conditions affect commercial catch of Dungeness crab and associated fishery communities of the U.S. west coast. Through the process of four steps, our analysis utilized both existing and novel metrics for exposure and susceptibility in the Dungeness crab coupled natural-human system. We defined the potential “exposure” of fishing communities to altered Dungeness crab catch due to changing ocean conditions via the first two steps. First, (1) we determined the spatial and temporal relationship between various ocean conditions and Dungeness crab commercial catch along the CCS from 1981 to 2017. Then, (2) this relationship was used to examine the magnitude of change in Dungeness crab catch for U.S. west coast port communities resulting from two ocean condition scenarios. Next, (3) we assessed the degree to which changes in Dungeness crab commercial catch may impact 18 fishery-reliant CCS communities (Figure 1) by defining a novel “susceptibility” score, which incorporated existing indices for fishery reliance and social vulnerability. Finally, (4) we considered the overall picture of “risk” to coastal communities via a qualitative assessment of the combination of exposure and susceptibility.
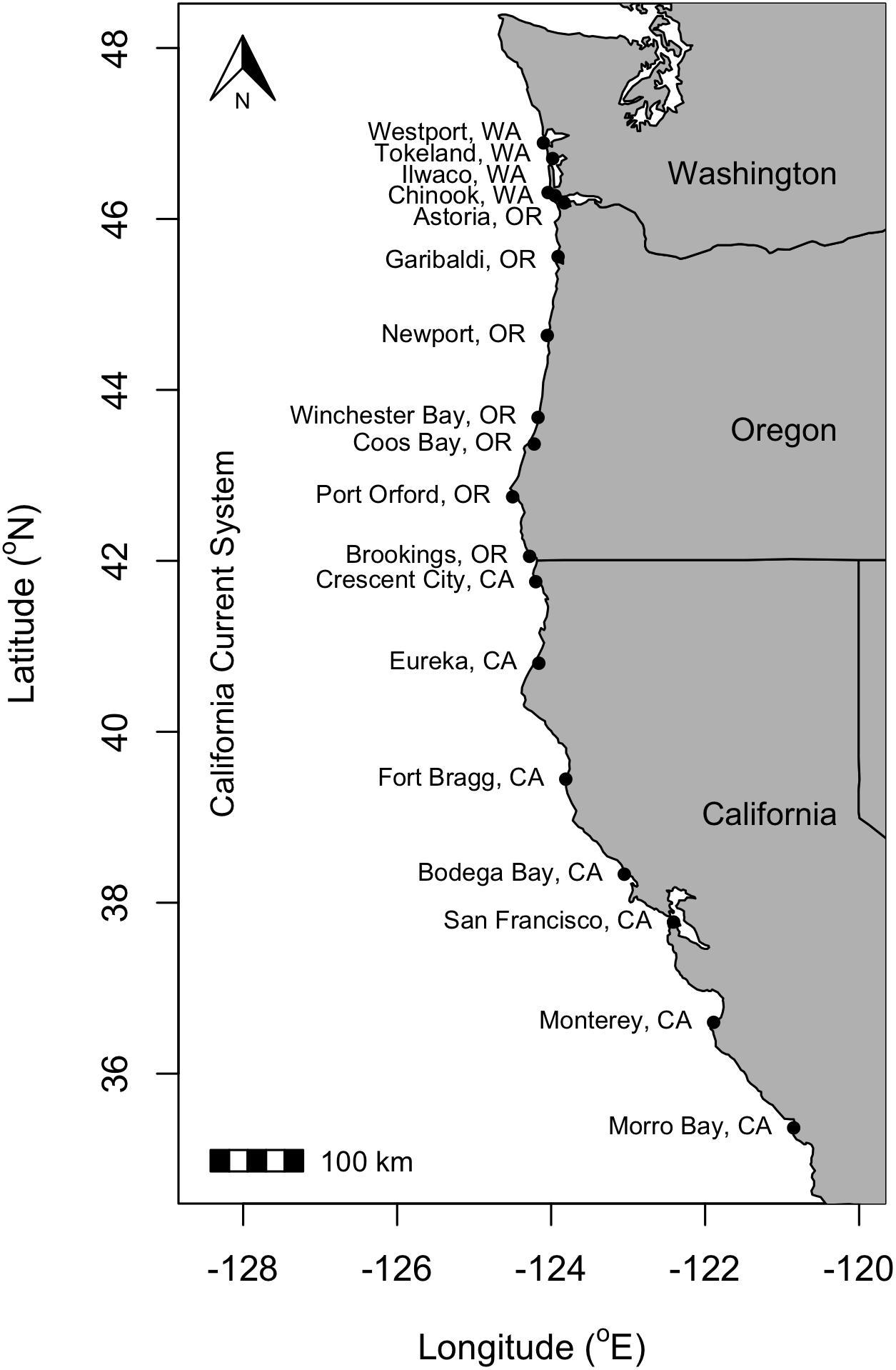
Figure 1. Map of the 18 major U.S. west coast port communities considered in this study within the Washington, Oregon, Northern California, and Central California management units. See Table 4 for port latitudes and management regions.
Materials and Methods
Data were obtained from multiple state, federal, and academic sources (Supplementary Table S1). All analyses were performed in R (v.3.5.0, R Core Development Team, 2018). The data and analyses are described in greater detail below.
Commercial Catch per Unit Effort
We gathered historical Dungeness crab commercial catch data (1981–2017) within the CCS for major U.S. west coast fishing ports (two Washington regions, seven Oregon ports, and seven California ports) (Supplementary Table S1). To normalize commercial catch, we calculated an estimated catch per unit effort (CPUE) by dividing the weight in kilograms (kg) of annual port or region Dungeness crab commercial catch by the number of Dungeness crab fish tickets (i.e., state department of fish and wildlife landing receipts) reported at each location in each year. Additionally, the proportional distribution of CPUE among U.S. west coast ports for each year was determined by dividing each port’s annual CPUE by the total annual CPUE across all ports. Analyses by ODFW (2014) and Richerson et al. (2020) found that fish tickets served as an adequate estimate of effort when compared to logbooks. Limited access and availability of fishery logbooks across all states and years necessitated estimation of CPUE in this way.
Ocean Conditions
A time series of the ocean conditions occurring within or affecting the CCS was compiled for 1977–2017 based on our hypotheses about the impact of ocean conditions on Dungeness crab survival and dispersal (Table 1, Figure 2, and Supplementary Table S1). Four basin-scale ocean condition indices were used in this study: PDO (sum of monthly averages January through June), NPGO (sum of monthly averages January through June), Multivariate ENSO Index (MEI; sum of monthly averages January through June), and Southern Oscillation Index (SOI; sum of monthly averages January through June) (Figure 2, left column). In addition, four latitude-specific measurements were used for 2- or 3-degree latitude increments, including: SST (average of January through June), upwelling (Upw; sum of daily Bakun upwelling index during the April through August upwelling season), spring transition index (STI; day of year), and northward Ekman transport (EkTrans; sum of monthly averages January through June) (Figure 2, right column). We used the northward component of EkTrans (metric tons second–1 kilometer–1) to indicate the relative strength of the California Current, with negative values indicating southward flow (Nelson, 1977). Most of the ocean condition metrics were summarized for January through June (Table 1) because CCS Dungeness crab are planktonic larvae during that time, which is hypothesized to be the life history stage most impacted by ocean conditions (Botsford et al., 1989; Botsford and Lawrence, 2002; Rasmuson, 2013).
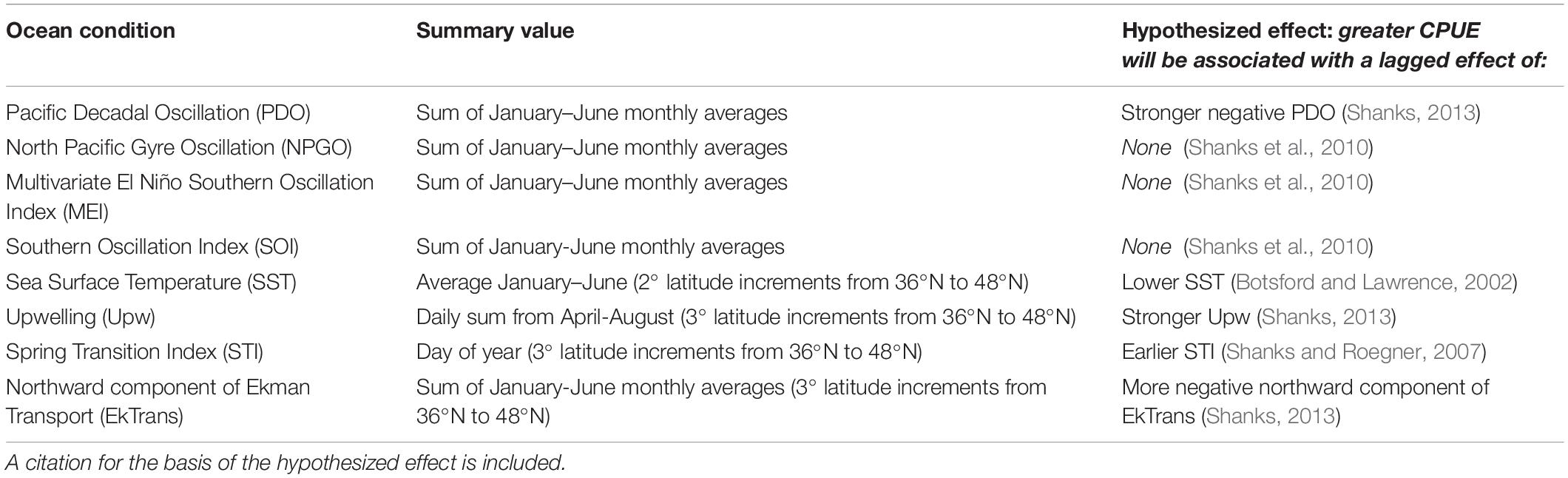
Table 1. List of ocean conditions analyzed for their relationship to Dungeness crab commercial catch per unit effort (CPUE) across the U.S. west coast, description of how the ocean condition value was summarized for analysis, and its hypothesized effect on CPUE.
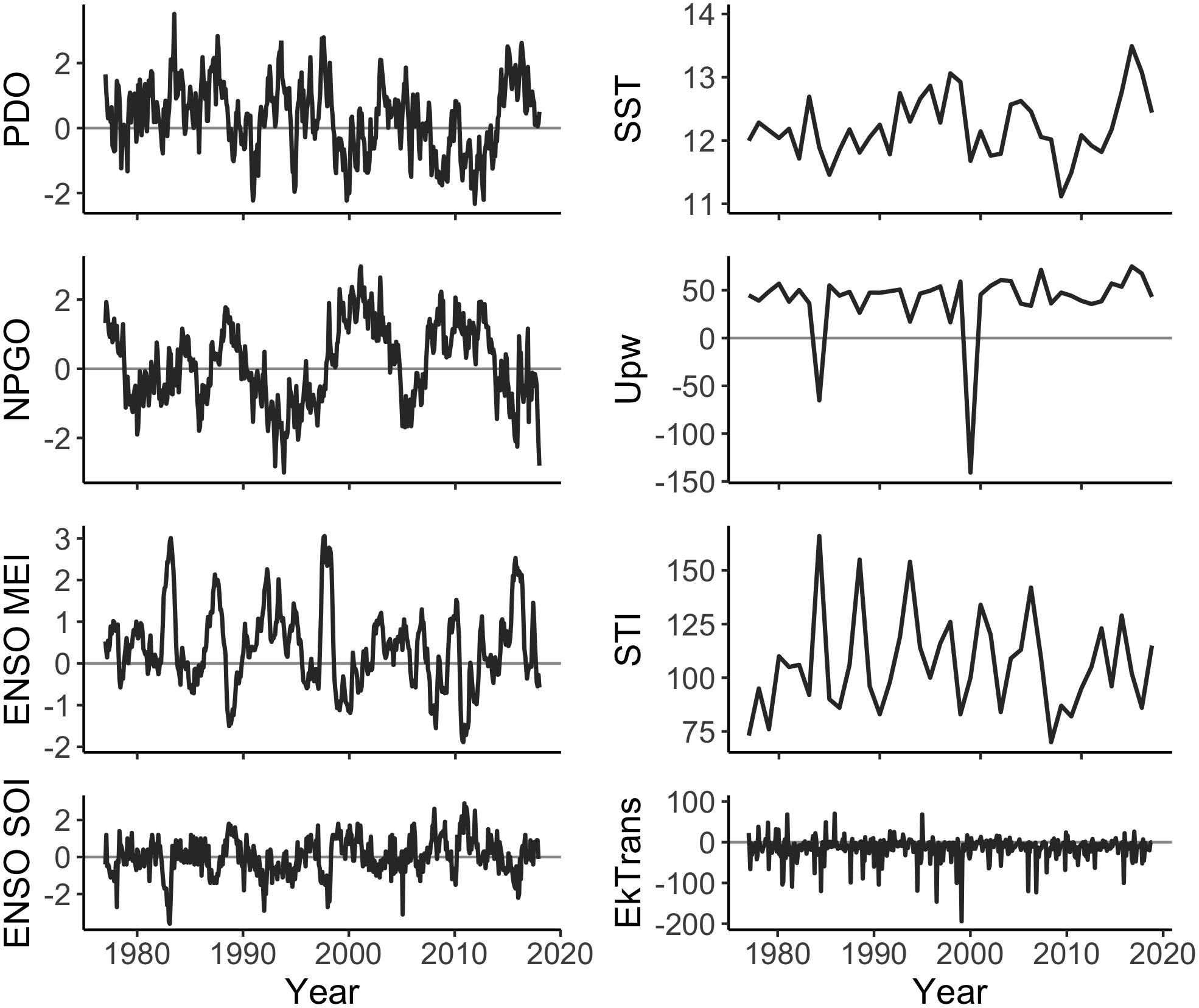
Figure 2. Ocean conditions measurements from 1977 to 2017. Regional factors (PDO, ENSO SOI, ENSO MEI, and NPGO) are monthly index values. Local factors (EkTrans, SST, Upw, and STI) are shown, for example, at 45°N. Northward EkTrans (value × 10 = metric tons second–1 kilometer–1) are shown as monthly values. SST (°C), Upw (meters3 second–1 per 100 m of coastline), and STI (day of year) are annual values.
Spatial and Temporal Analyses of Ocean Conditions and Commercial Catch
Generalized additive mixed models (GAMM) were used to assess the spatial and temporal relationship between ocean conditions and commercial Dungeness crab CPUE along the U.S. west coast. To allow for the spatially variable effects of ocean conditions on Dungeness crab CPUE and to account for simultaneously testing the effects of ocean conditions at multiple locations, we applied a variable coefficient GAMM (VC_GAMM) framework. The VC_GAMM allows for variable model intercepts and variable linear relationships between the response and the design variable (Bacheler et al., 2009; Bartolino et al., 2011). These formulations are ideally suited to test for the presence of either spatially (e.g., Ciannelli et al., 2012) or temporally (e.g., Litzow et al., 2019) variable relationships between ocean conditions and indices of fish productivity or abundance. Dungeness crab span a large distributional range, thus the effect of ocean conditions on catch is likely to be spatially variable across latitude. This outcome could have important societal implications because some fishing communities may be more exposed to changing ocean conditions and associated impacts on fisheries than others. Therefore, our analyses compared two GAMM formulations: one with linear and spatially fixed effects of ocean conditions on Dungeness crab CPUE (stationary GAMM) and another with locally linear and spatially variable effects of ocean conditions on CPUE (VC_GAMM) depending on the port or region of commercial catch (n = 16). The two model formulations are nested and thus can be compared using a likelihood-ratio (LR) test, which tests the null hypothesis that the likelihood of the unconstrained (i.e., VC_GAMM) and constrained (i.e., stationary GAMM) model formulations are not significantly different from each other (Litzow et al., 2019). The two model formulations can also be compared based on the Akaike information criterion (AIC), which is a metric of model performance that accounts for both likelihood of the model parameters and complexity of the model parameterization.
The GAMM and VC_GAMM were built using the R packages ‘mgcv’ (v.1.8-28; Wood, 2017; Wood, 2019) and ‘nlme’ (v. 3.1-139; Pinheiro et al., 2019). The CPUE data were natural log transformed to meet the assumption of normality. The summarized ocean conditions were then lagged 3, 4, and 5 years in accordance with published literature that Dungeness crab are caught in the CCS fishery at about age four (Botsford, 1984; Johnson et al., 1986). For each of the eight ocean conditions, we fit 26 models of latitudinally fixed (i.e., stationary GAMM) and latitudinally variable (i.e., non-stationary VC_GAMM) effects for all three time lags (3, 4, and 5 years). Each ocean condition was considered separately and the suite of 26 models for each ocean condition included all possible combinations of stationary and/or non-stationary effects at one or multiple time lags (Supplementary Table S2). The general formulations for each model type were:
Stationary GAMM:
Variable Coefficient GAMM:
Where yt,lat is Dungeness crab CPUE at year ‘t’ and port latitude (lat). OC is one of the eight ocean condition indices listed in Table 1, at the three examined time lags (3, 4, and 5 years). Each β term is the linear coefficient of the lagged OC variable, and αlat is the model intercept. In the VC_GAMM, model coefficients (αlat and β terms) are assumed to be spatially variable according to a smooth function of lat. In the stationary GAMM formulation, only the model intercept αlat is assumed to be spatially variable, while β terms are fixed. It is necessary for both formulations to allow for spatially variable intercept αlat to account for the overall trend of greater Dungeness crab catch at higher latitudes. The error term (εt,lat) is assumed to be temporally autocorrelated at lag = 1: εt,lat = ∂ + Φεt–1,lat. Including a temporally autocorrelated error structure ensures that potential temporal trends in the model residuals does not bias estimates in the uncertainty of model parameters. For each model, the parameter Φ was assessed to determine if an autocorrelation error variable should be included.
The best model for each ocean condition was chosen based on LR tests and AIC. The model with the lowest AIC value was chosen as the best model if it was the reduced (simpler) model. However, if the model with the lowest AIC value was the more complex model, it had to be significantly different (LR test, p < 0.1) from the reduced model to be chosen as the best model. If the more complex model was not significantly different, then the reduced model was chosen.
Changing Ocean Conditions and Exposure to Reduced Commercial Catch
The CMIP5 multi-model ensemble of the International Panel on Climate Change (IPCC) Fifth Assessment Report (AR5) predicts that SST will increase by 0.3°C decade–1 through the end of the century (Jewett and Romanou, 2017; IPCC, 2018). Therefore, to assess the exposure of CCS port communities to reduced Dungeness crab commercial due to changing ocean conditions, we estimated changes in Dungeness crab CPUE across U.S. west coast ports in relation to expected SST changes in the CCS. Change in Dungeness crab CPUE was estimated based on the predictions determined from the SST VC_GAMM and two SST scenarios (Table 2) using the predict function in the ‘mgcv’ R package (Wood, 2019). The percent change in predicted versus current Dungeness crab CPUE defined the “exposure” component of our framework. We focused the exposure metric on SST because of the high explanatory power of SST on Dungeness crab CPUE and the degree of confidence on the projected range of SST increase in the CCS (Jewett and Romanou, 2017).
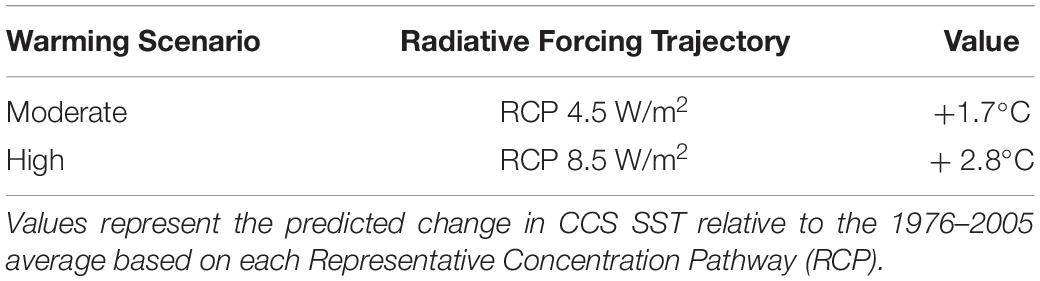
Table 2. Possible future (2080) sea surface temperature (SST) scenarios for the California Current System (CCS) based on the IPCC AR5.
Reliance and Social Vulnerability of Fishery-Dependent Communities
To determine the impact of a change in Dungeness crab CPUE (exposure) on fishery dependent coastal communities along the CCS, we first assessed the current importance of Dungeness crab to these communities by utilizing existing indices for reliance and vulnerability. The National Oceanic and Atmospheric Administration designed frameworks to evaluate fishery reliance and engagement of coastal communities (Norman et al., 2007; Jepson and Colburn, 2013). In response to recent fishery closures, the frameworks were adapted to produce indices specific to the Dungeness crab commercial fishery (K. Norman and A. Varney, personal communication; NOAA, 2017; Moore et al., 2019). Dungeness crab commercial fishery reliance indices for 2015 from 64 U.S. west coast communities were shared with our team (Supplementary Table S1; Norman and Varney, personal communication; NOAA, 2017; Moore et al., 2019). Of these communities, the major Dungeness crab commercial fishing ports from California (7), Oregon (7), and Washington (4) were selected (Figure 1). Reliance indices were used to determine the quantitative importance of the Dungeness crab fishery to each coastal community and were based on the following information: Crab catch (pounds) per capita, crab revenue (USD) per capita, and count of crab processors per capita. Although reliance indices were calculated for only 1 year, the total Dungeness crab catch in the CCS in 2015 (1.92 × 107 kg) was similar to the average catch from 1981 to 2017 (1.90 × 107 kg) (based on Pacific Fisheries Information Network annual data; Supplementary Table S1).
A multi-point social vulnerability analysis in 2014 provided information on the current overall socio-economic stability of each coastal community (Supplementary Table S1; Varney, 2018). Social vulnerability refers to the socioeconomic and demographic factors that determine a community’s ability to withstand hardships and is commonly used in risk assessment. In this case, we used social vulnerability to assess the level of disruption that would occur in a community given an interference in the lucrative Dungeness crab fishery. Community Social Vulnerability Index (CSVI) was calculated for all U.S. west coast communities using the following information: Metrics of personal disruption, population composition, poverty, labor force, housing characteristics, housing disruption, and retiree migration (Varney, 2018). CSVI values were rescaled to be positive.
Coastal Community Susceptibility to a Change in Dungeness Crab CPUE
To determine the relative degree to which a change in Dungeness crab CPUE could impact coastal communities, we combined Dungeness crab-specific fishery reliance indices with CSVI into a novel “susceptibility” score. Our susceptibility score for each community was calculated as the product of CSVI and reliance. CSVI encapsulates the relative ability of communities to weather hardships, where lower indices indicate a community that is less vulnerable in the face of an unwanted event due to its social well-being. The reliance index denotes the per capita presence of the Dungeness crab fishery in the community relative to other communities, which expresses how important the fishery is to the economic and social well-being of the community. The value of the product, susceptibility, was used to represent the vulnerability of a community, scaled by reliance on Dungeness crab and is a quantitative representation of the degree to which each community would be influenced by a change in Dungeness crab CPUE. Communities that are both heavily reliant on Dungeness crab and socially vulnerable would have high susceptibility scores, highlighting the scaling effect of these two properties. In contrast, communities with either high vulnerability and low reliance on Dungeness crab, or low vulnerability and high reliance on crab would have similar susceptibility scores. Finally, a community with both low reliance and vulnerability, would be the least susceptible because they are generally resilient to change, and a change in the Dungeness crab fishery would not have a large impact on the community. The compounded risk for each community was examined by comparing the exposure to a change in Dungeness crab CPUE under increased SST scenarios with a region-specific susceptibility score. Although exact terminology differs, similar approaches have been adopted in other recent studies examining the exposure-vulnerability of coastal communities to changing ocean conditions (e.g., Ekstrom et al., 2015; Mathis et al., 2015).
Results
Relationship Between Ocean Conditions and Dungeness Crab Commercial Catch
Commercial Catch per Unit Effort
From 1981 to 2017, Dungeness crab commercial catch within 16 major ports/regions of the CCS varied annually (total U.S. west coast mean catch = 985,183 kg; Figure 3). Standardized catch (kg of crab landed per number of fish tickets), or CPUE, also varied annually (Figure 3 and Supplementary Figures S1–S4). As a result, there was variation in the annual proportional distribution of CPUE along the CCS (defined as each port’s percent of the total U.S. west coast CPUE) (Figure 4). Total U.S. west coast CPUE was significantly correlated with total U.S. west coast catch (Pearson’s r = 0.92, p < 0.001).
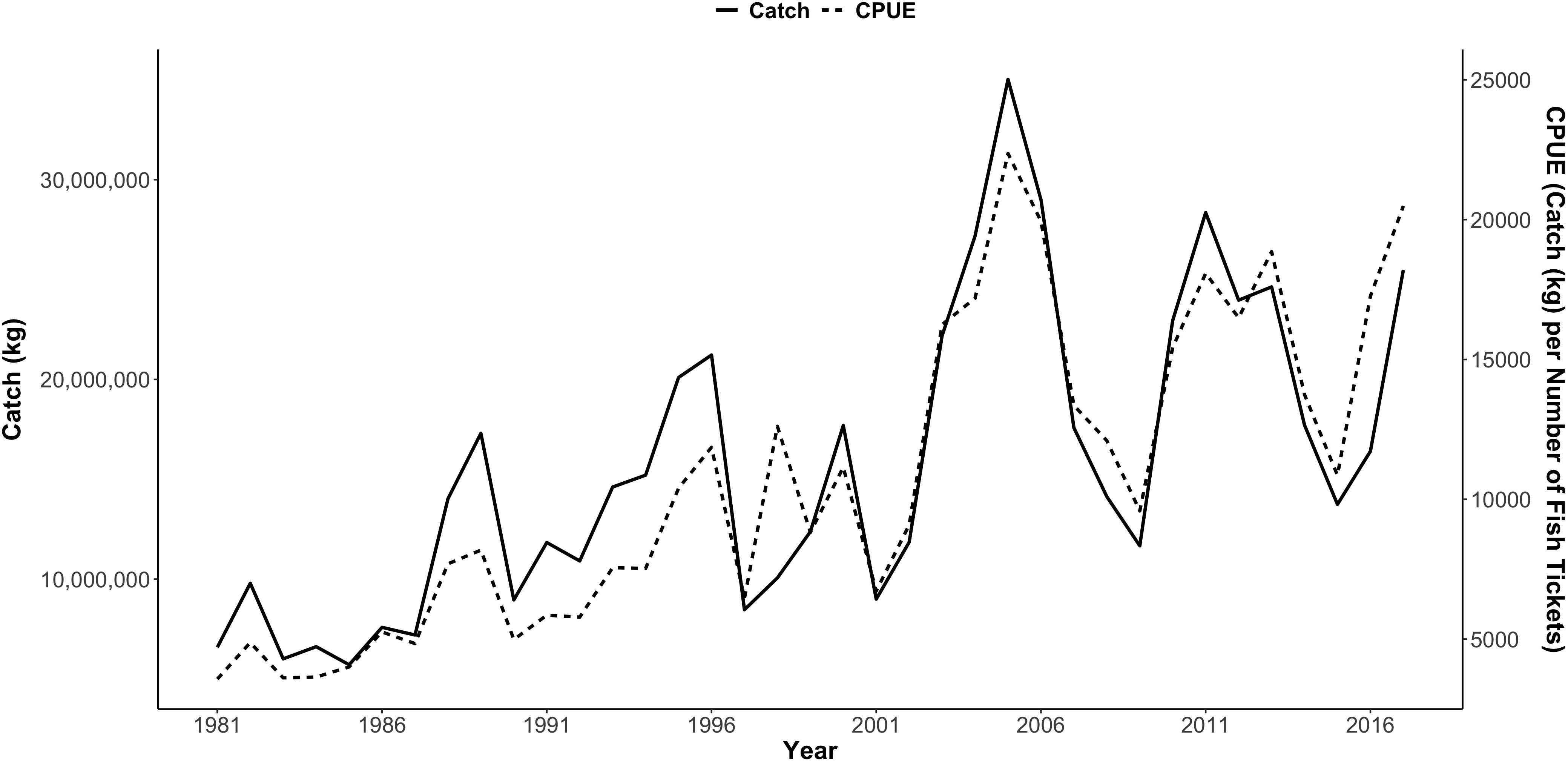
Figure 3. Total commercial catch of Dungeness crab in kilograms (kg) per year (solid line) and catch per unit effort (dashed line) from 1981 to 2017 for the U.S. west coast, including 16 ports/regions in Washington, Oregon, and California. Catch per unit effort (CPUE) was calculated as kg caught per number of fish tickets per year. Data were provided by WDFW 2018, ODFW 2018, and CDFW 2018. Supplementary Figures S1–S4 show CPUE by port for each region.
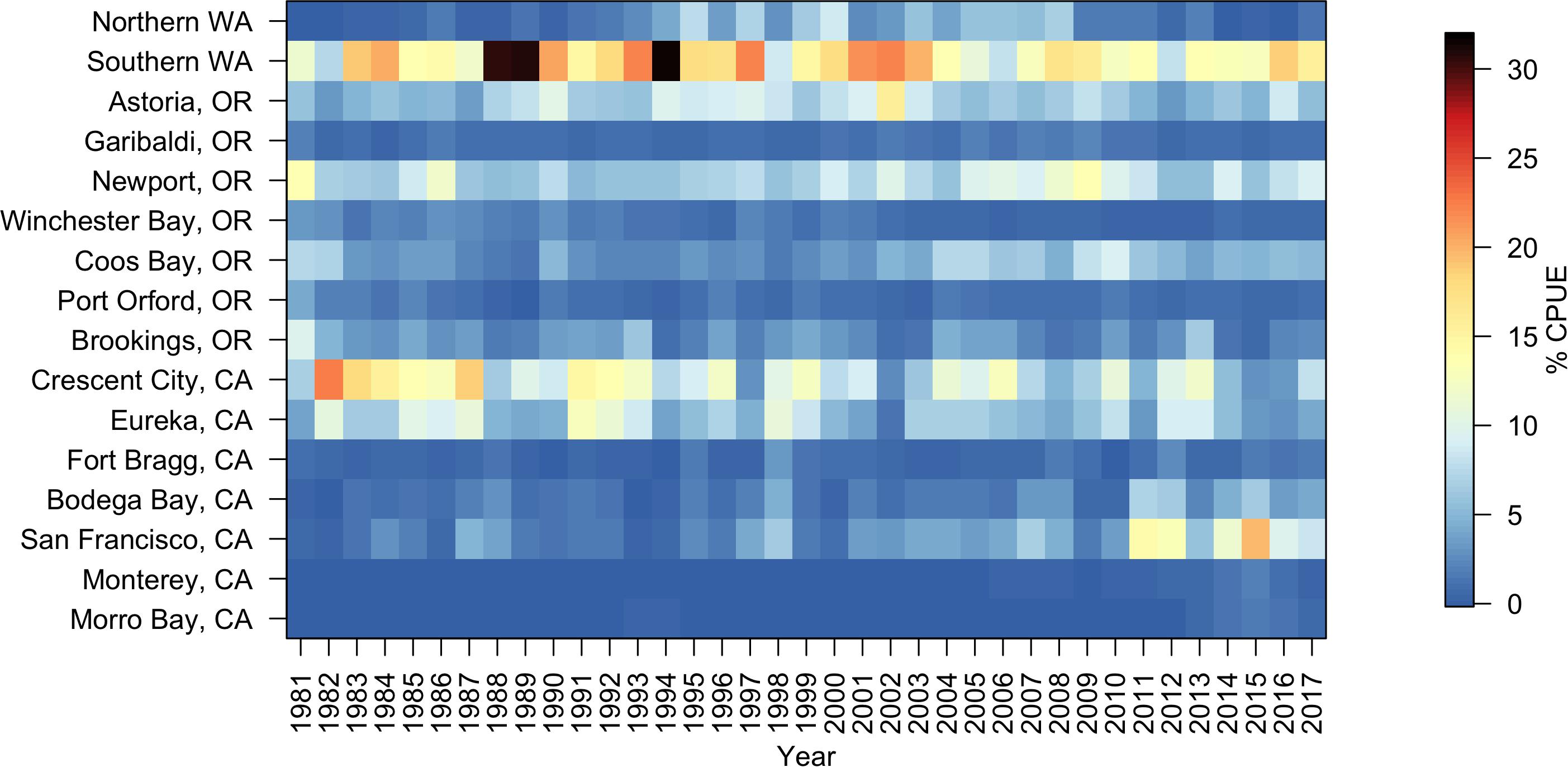
Figure 4. Percent of total Dungeness crab commercial catch per unit effort (CPUE) landed annually in 16 U.S. west coast ports from 1981 to 2017. Warm colors indicate a high percentage of the total annual CPUE and cool colors indicate a low percentage of the total annual CPUE. Total annual CPUE was calculated as kilograms caught per number of fish tickets per year. Ports correspond to those shown in Figure 1 and latitudes are listed in Table 4.
Spatial and Temporal Analyses of Ocean Conditions and Commercial Catch
The results of the stationary GAMM and non-stationary VC_GAMM and selected models are listed in Table 3 and described below. There was support (R2adj = 0.54, LR test: p = 0.09) for a model of Dungeness crab ln(CPUE) along the U.S. west coast that includes a spatially (latitudinally) homogeneous effect of PDO lagged by 4 years and a spatially variable effect of PDO lagged by 5 years (Supplementary Figure S5). At a 4-year lag, there was an inverse relationship between Dungeness crab ln(CPUE) and PDO (coefficient: −0.029). Furthermore, at a 5-year lag the coefficient ranged from −0.042 to −0.0085, and was a stronger negative correlation at southern latitudes compared to northern latitudes (Supplementary Figure S5).
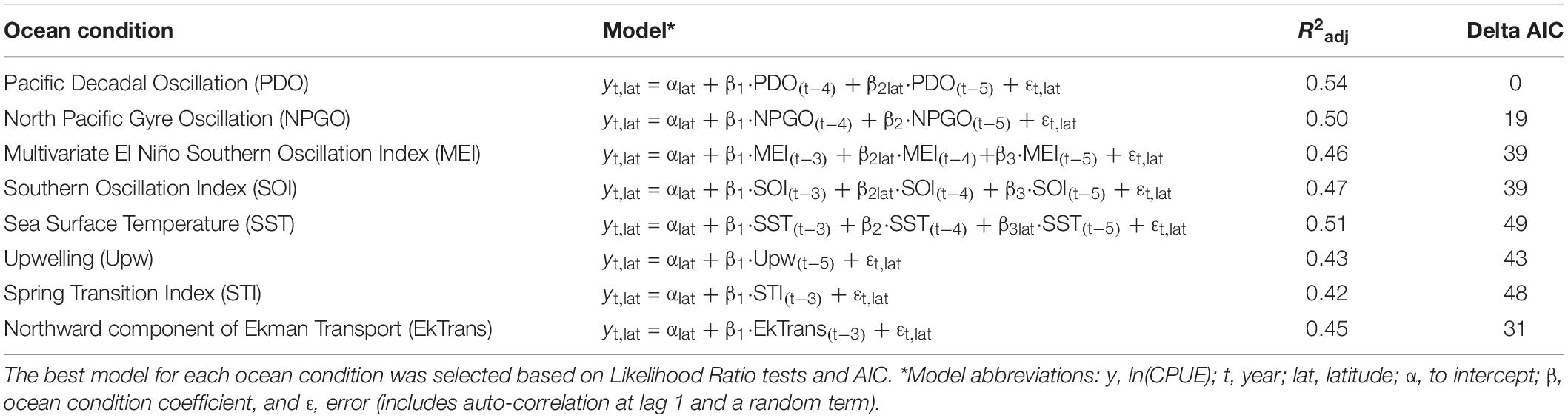
Table 3. Selected models of individual ocean conditions lagged 3, 4, or 5 years and Dungeness crab commercial catch per unit effort ln(CPUE) along the U.S. west coast, including the adjusted R2 and delta Akaike information criterion (AIC) for each model.
There was support (R2adj = 0.50, LR test: p = 0.06) for a model that includes a spatially homogeneous and positive effect of NPGO lagged by 4 (coefficient: 0.173) and 5 (coefficient: 0.068) years (Supplementary Figure S6). There was support (R2adj = 0.46, LR test: p = 0.07) for a model that includes a spatially homogeneous and negative effect of MEI lagged by 3 and 5 years (coefficient: −0.12 at both lags) (Supplementary Figure S7). In addition, there was a spatially variable effect of MEI lagged by 4 years and the coefficient ranged from −0.206 to 0.065, indicating a strong negative relationship at northern latitudes and a weak positive relationship was observed at southern latitudes (Supplementary Figure S7). The effect of ENSO was also examined using SOI and support was found (R2adj = 0.47, LR test: p = 0.14) for a model that includes a spatially homogeneous effect of SOI lagged 3, 4, and 5 years (Supplementary Figure S8). This model indicates a positive relationship between ln(CPUE) and SOI lagged by 3 years (coefficient: 0.142), 4 years (coefficient: 0.067), and 5 years (coefficient: 0.145).
Consistent with other temperature-related oceanic indices (e.g., PDO), there was support (R2adj = 0.51, LR test: p = 0.02) for a model that includes a spatially homogeneous and negative effect of SST lagged by 3 (coefficient: −0.110) and 4 (coefficient: −0.269) years and a spatially variable effect of SST lagged by 5 years (Figures 5A,B). At lag 5, the coefficient ranged from −0.443 to −0.582 and was stronger at southern latitudes than northern latitudes (Figure 5C).
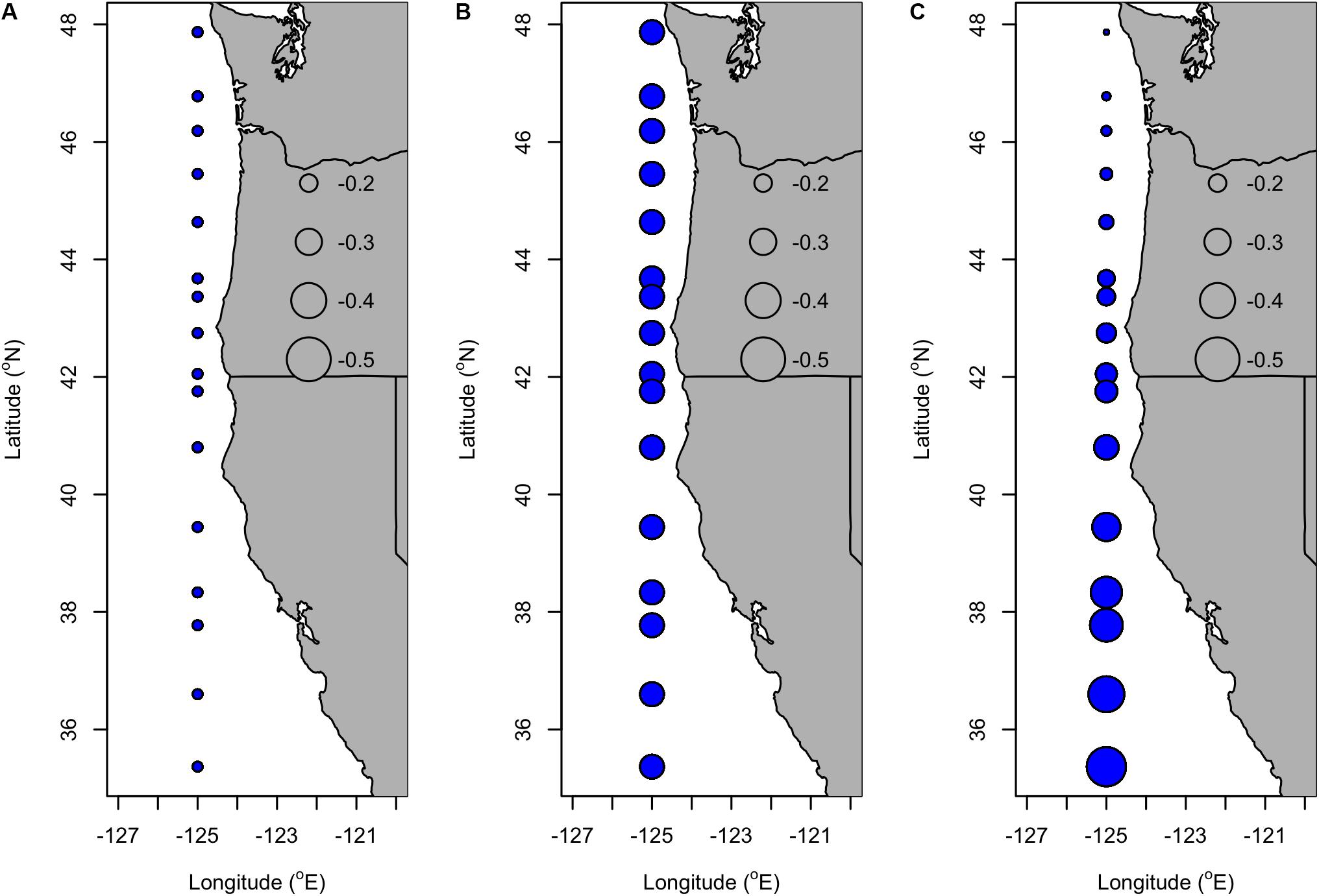
Figure 5. Coefficients of SST lagged by (A) 3 years, (B) 4 years, and (C) 5 years in the selected VC_GAMM of Dungeness crab commercial ln(CPUE) along the U.S. west coast (Table 3). The latitude of ports corresponding to each of the circular symbols are listed in Table 4. Blue symbols represent negative coefficients and the size of the symbol scales with the magnitude of the coefficient.
There was support (R2adj = 0.43, LR test: p = 0.05) for a model that includes a spatially variable effect of Upw lagged by 5 years (coefficient range: −1.12e–5 to 4.04e–5; Supplementary Figure S9). There is a stronger, positive relationship at southern latitudes and a weaker, negative relationship at northern latitudes. On the other hand, there was limited support for a model of STI (R2adj = 0.42, LR test: p = 0.46) with a spatially homogeneous and negative effect lagged by 3 years (coefficient: −7.73e–4; Supplementary Figure S10). There was also limited support (R2adj = 0.45, LR test: p = 0.77) for a model of that includes a weak and positive relationship between ln(CPUE) and the northward component of EkTrans lagged by 3 years (coefficient: 7.72e–4; Supplementary Figure S11).
Exposure: Change to Dungeness Crab Commercial Catch Under SST Scenarios
Predictions for a +1.7 or +2.8°C increase in CCS SST indicated that Dungeness crab ln(CPUE) along the U.S. west coast would decrease under these two scenarios (Figure 6A and Supplementary Table S3). This change was latitudinally variable and a larger decrease in ln(CPUE) was found for southern latitudes than northern latitudes. The +1.7°C SST scenario decreased ln(CPUE) by 30–90%, depending on latitude, and the +2.8°C SST scenario decreased ln(CPUE) even more dramatically (45–100%; Figure 6B). Therefore, there was greater exposure (i.e., a larger decrease in CPUE due to increased SST) at the southern end of the species range.
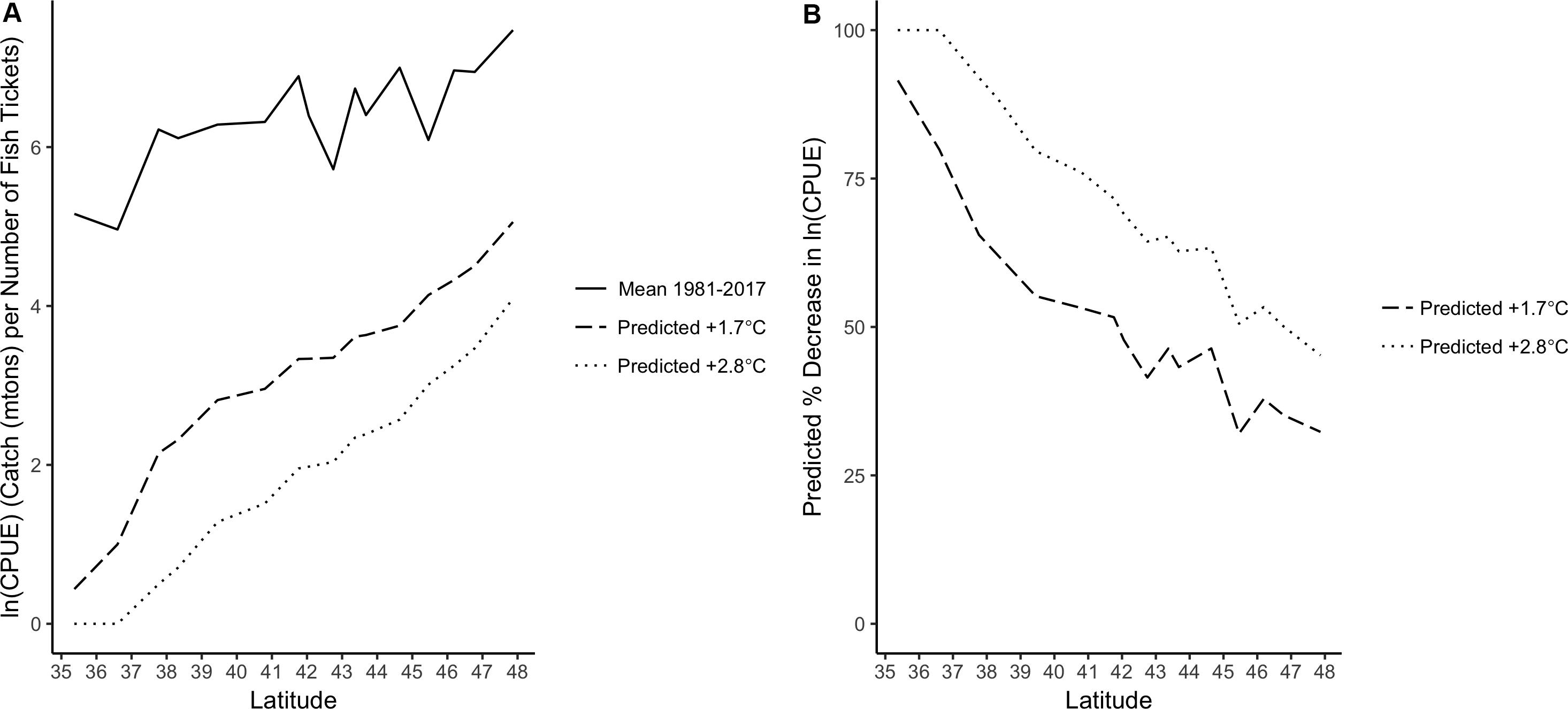
Figure 6. (A) Mean Dungeness crab ln(CPUE) along the U.S. west coast from 1981 to 2017 (solid line) and VC_GAMM predicted CPUE along the U.S. west coast under two future SST scenarios: +1.7°C (dashed) and +2.8°C (dotted). (B) VC_GAMM predicted percent decrease in Dungeness crab ln(CPUE) by latitude along the U.S. west coast based on two future SST scenarios: +1.7°C (dashed) and +2.8°C (dotted).
Risk for Coastal Communities
Quantitative Importance of Dungeness Crab to Coastal Communities
Because paired CPUE data and socio-economic indices (reliance and CSVI) were not available for all 18 selected coastal communities, we divided them into four regions that reflect the U.S. west coast fishery management divisions: Washington, Oregon, Northern California, and Central California. Compared to all communities across the CCS, Westport, Washington was an outlier with a very high Dungeness crab reliance index (Table 4). Regardless of inclusion or exclusion of Westport, Washington port communities had the highest reliance on the Dungeness crab fishery, followed by Oregon, Central California, and Northern California (Table 4 and Figure 7). When social vulnerability was compared between the four U.S. west coast regions, Oregon had the highest CSVI, followed by Washington, Northern California, and Central California (Table 4).
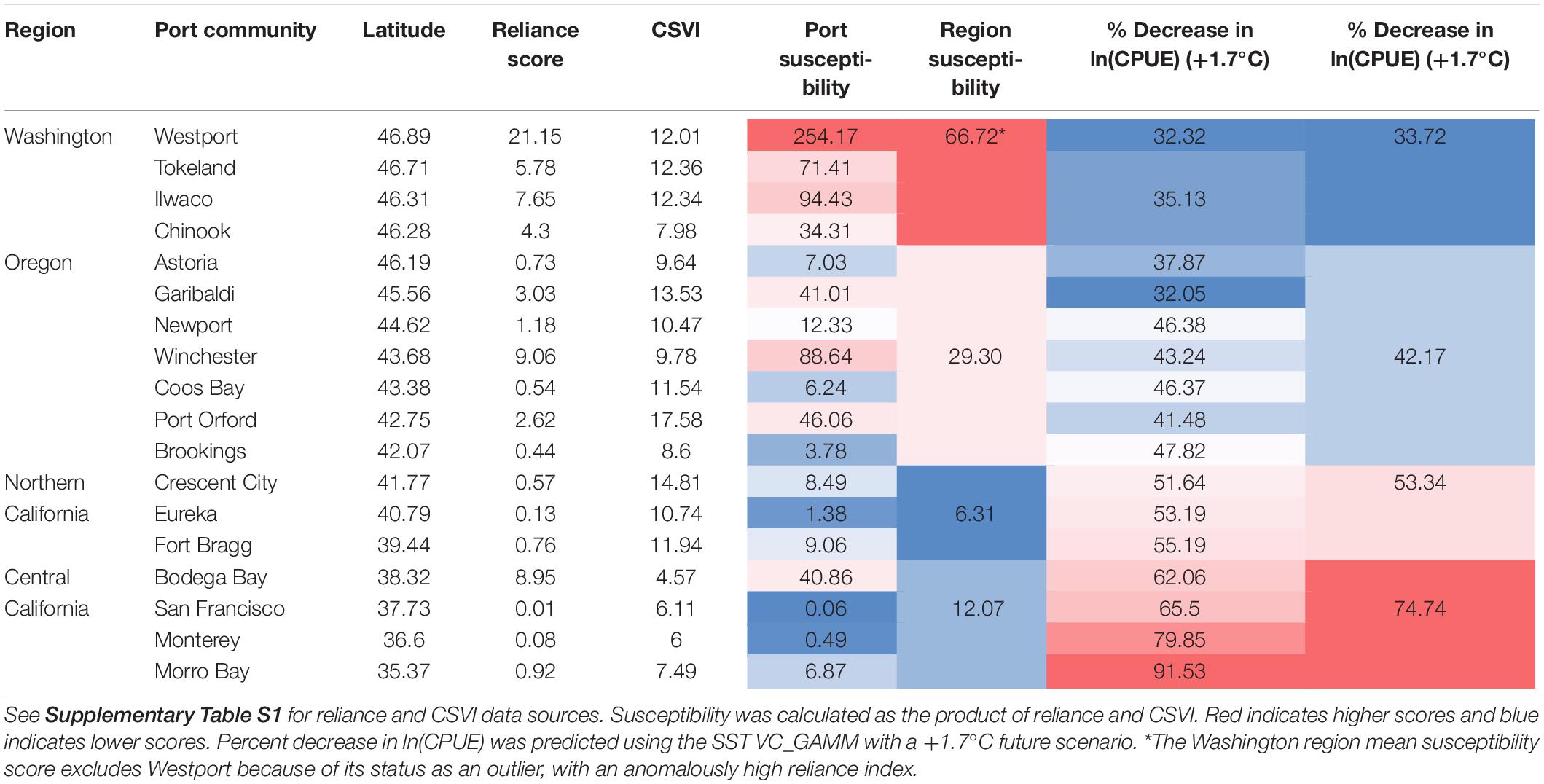
Table 4. Reliance index, community social vulnerability index (CSVI), susceptibility score, and predicted percent decrease in ln(CPUE) by port and by region (NOAA, 2017; Varney, 2018).
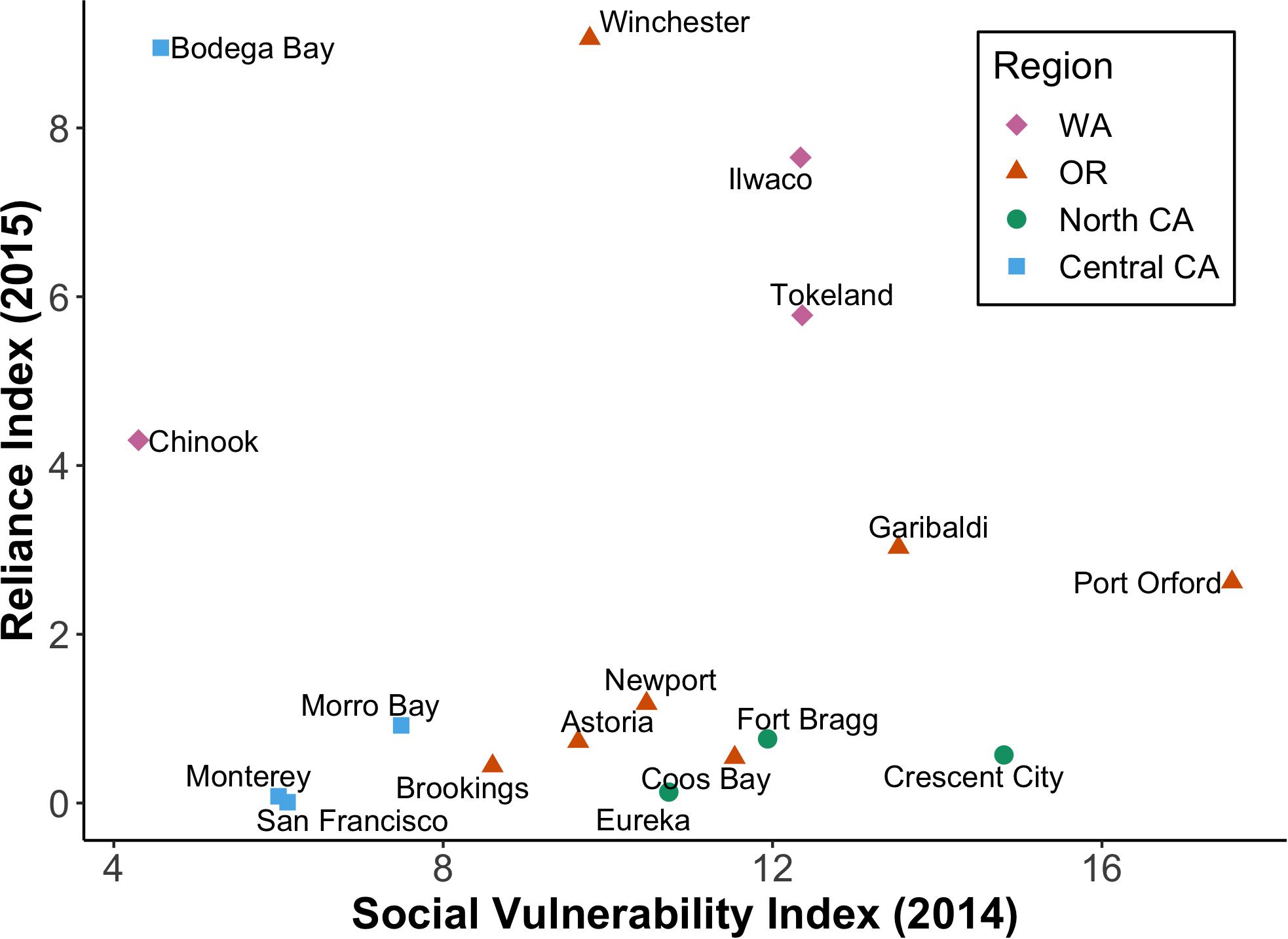
Figure 7. Dungeness crab reliance index for 2015 versus social vulnerability (CSVI) for 2014. Reliance scores consider per capita engagement in the Dungeness crab fishing industry, while CSVI indicates the overall vulnerability of a community. This figure excludes Westport, WA, which had anomalously high reliance and moderate vulnerability (Table 4).
Coastal Community Susceptibility and Risk to Changing Dungeness Crab CPUE
The calculated susceptibility score (product of reliance and CSVI) and the percent decrease in Dungeness crab CPUE under future SST scenarios shows that the overall risk of a shift in Dungeness crab CPUE is moderate to high across the CCS, depending on the level of SST warming (Table 4). Below, we discuss these results specific to each management region.
Washington port communities
Predictions from the SST VC_GAMM indicated that the northern portion of the CCS Dungeness crab fishery is the least exposed to a change in CPUE due to changes in SST (32.31–50.08% decrease in CPUE, depending on latitude and SST scenario; Table 4). The four Washington port communities exhibited high reliance on the Dungeness crab fishery (mean reliance = 9.72), likely due to low human populations (reliance is calculated per capita) and high Dungeness crab catch. Furthermore, social vulnerability of the Washington communities ranked second highest of the four CCS regions examined (mean CSVI = 11.17). Given high reliance and high CSVI, Washington had the highest regional susceptibility score regardless of whether Westport was included or excluded (mean including Westport = 113.58, mean excluding Westport = 66.72). Although exposure is lower for Washington than southern management regions, high susceptibility of the ports in this region indicates higher overall risk that Washington coastal communities would be negatively impacted by a decrease in Dungeness crab CPUE from changing ocean conditions.
Oregon port communities
The SST VC_GAMM predicted higher exposure of Oregon Dungeness crab CPUE to SST changes than for Washington (32.05–69.07% decrease in CPUE, depending on latitude and SST scenario; Table 4). The seven Oregon port communities exhibited wide variability in reliance on the Dungeness crab fishery and CSVI. Examining the region as a whole, reliance of Oregon communities ranked second highest (mean reliance = 2.51) and social vulnerability ranked second highest of the four CCS regions (mean CSVI = 11.59). Combining reliance and CSVI, the susceptibility of Oregon communities was second highest of the four CCS regions (mean susceptibility = 29.30). Similar to Washington, high susceptibility scores reflected the socio-economic importance of Dungeness crab in Oregon port communities, particularly in small, natural resource-based communities like Port Orford and Winchester Bay. Oregon had a lower susceptibility score than Washington, but greater exposure indicates that the risk of this region to a change in Dungeness crab CPUE from changing ocean conditions is still relatively high.
Northern California port communities
Northern California port communities showed higher exposure to changes in CPUE predicted by the SST VC_GAMM than Washington or Oregon (51.64–79.62% decrease in CPUE, depending on latitude and SST scenario; Table 4). The three Northern California communities had the lowest reliance on the Dungeness crab fishery (mean reliance = 0.48) and the highest social vulnerability score (mean CSVI = 12.50). Taken together, however, the susceptibility of this region ranked lowest of the four CCS regions (mean susceptibility = 6.31). Despite having greater exposure than Washington or Oregon, northern California communities may be at lower risk to a decrease in Dungeness crab CPUE due to low susceptibility – driven primarily by low reliance.
Central California port communities
The southernmost Dungeness crab ports had the greatest exposure to CPUE changes due to SST increases, according to the VC_GAMM (62.06–100.00% decrease in CPUE, depending on latitude and future SST scenario; Table 4). Large human populations within central California and generally lower Dungeness crab catch likely resulted in lower reliance on the fishery (mean reliance = 2.38). Furthermore, the overall social vulnerability within this region is also low compared to other CCS regions (mean CSVI = 6.04). The overall susceptibility of the central California region to decreased Dungeness crab CPUE was low (mean susceptibility = 12.07). Despite high exposure for the southern portion of the CCS, central California coastal communities exhibit low risk of being negatively impacted by a decrease in Dungeness crab CPUE due to changing ocean conditions. Although the overall risk of a distribution shift to central California is low, it is also important to consider ports individually. For instance, Bodega Bay has high susceptibility and exposure. Therefore, this specific port is at a much higher risk to a change in Dungeness crab CPUE compared to other ports in the same region.
Discussion
The impacts of climate change on marine systems will alter ecosystem-scale functions including productivity, food web and disease dynamics, and species distributions (Hoegh-Guldberg and Bruno, 2010). Many of these changes are already occurring in the CCS (Snyder et al., 2003) and our analyses indicate that changing ocean conditions could impact the abundance and distribution of commercial catch of the socio-economically important Dungeness crab. These effects will not be confined to the Dungeness crab fishery, but will impact many economically important fisheries on the U.S. west coast and globally. In particular, studies have projected a greater than 10% reduction in catch potential for continental U.S. exploited fisheries by mid-century (Cheung et al., 2010; Barange et al., 2014). Although global fishery assessments are critical to our understanding of climate change, there is also value in addressing fine-scale impacts at the level of individual fisheries. Our study provides a framework for evaluating an individual fishery and the risks for the communities that rely on it, and will be useful when adapting fisheries management strategies in response to climate change.
Importance of Ocean Conditions for Dungeness Crab
Statistical analysis of Dungeness crab CPUE and ocean conditions identified a mix of relationships that were lagged by 3–5 years. This finding upholds previous work and confirms the importance of favorable ocean conditions, such as negative PDO and positive SOI indices, for promoting larval crab survival and transport in the CCS (Botsford and Lawrence, 2002; Shanks et al., 2010; Shanks, 2013). We found support for a mix of latitudinally stationary and non-stationary models, indicating that the influences of various ocean conditions on Dungeness crab CPUE are spatially variable, and are likely specific to different time lags. However, U.S. west coast fishery management often operates under the assumption that harvestable-sized Dungeness crabs are age 4 (Rasmuson, 2013). This analysis suggests that the relationship between ocean conditions and Dungeness crab commercial harvest may be more dynamic and could explain inaccuracies in commercial catch projected using only larval abundance 4 years prior (i.e., Shanks et al., 2010).
Overall, we found that the models explaining the largest amount of variance were those with PDO, SST, and NPGO, individually. Consistent with our findings, negative PDO conditions during the vulnerable early life history stages of Dungeness crab, and other exploited marine fish, have been shown to increase survival to harvestable size (Mantua et al., 1997; Botsford and Lawrence, 2002; Shanks, 2013). Although SST can be correlated to large scale ocean conditions, like PDO, most previous work on Dungeness crab has not explicitly examined SST (except see Botsford and Lawrence, 2002). However, our analysis indicates a strong, negative relationship between Dungeness crab CPUE and lagged SST. In addition, we identified a positive relationship with NPGO, which was unexpected given that Shanks et al. (2010) found no correlation between NPGO and Dungeness crab larval recruitment. However, this newly identified association corroborates other indications that, since the late 1980’s, NPGO has had a greater influence on regional physical and biological variables in the North Pacific Ocean (Di Lorenzo et al., 2008; Litzow et al., 2020), including novel impacts to salmon (Kilduff et al., 2015) and seabirds (Schmidt et al., 2014). The lack of strong support for models of STI and EkTrans was also contrary to our expectations given previous work of Shanks and Roegner (2007) and Shanks (2013). Although we did find support for an upwelling model, the influence of Upw was spatially variable and indicated a greater dependence of southern Dungeness crab CPUE to onshore advection of larvae and upwelling-fueled primary productivity (Shanks, 2013).
While we focus on the SST model for the purpose of determining exposure of CPUE to changing ocean conditions, it is important to recognize that SST is closely correlated with other climate-related phenomena. For example, increasing SST is likely to increase occurrences of hypoxic events (Grantham et al., 2004; Chan et al., 2008) and algal blooms that release domoic acid in the CCS (McKibben et al., 2017). These SST-related phenomena have already had detrimental ecological and socio-economic consequences for the Dungeness crab industry and are likely to influence future abundance, distribution, and accessibility of Dungeness crab along its entire range. Hypoxia causes mass mortality events in adult and juvenile Dungeness crab (Grantham et al., 2004). Harmful algal blooms that release domoic acid result in the bioaccumulation of the neurotoxin in Dungeness crab and other shellfish (Schultz et al., 2013), leading to fishery closures due to the risks of human consumption (Trainer et al., 2007). The occurrence of domoic acid events on the U.S. west coast are expected to increase with warmer ocean conditions (McKibben et al., 2017). Ocean acidification is also expected to negatively impact Dungeness crab, primarily through indirect effects via shellfish prey species (Marshall et al., 2017), but Bednaršek et al. (2020) found that larval crabs are already experiencing exoskeleton dissolution under present acidification levels. The lack of sufficient time series precluded our ability to explore the relationship between ocean acidification, hypoxia, and Dungeness crab. Similar to the impact of ocean conditions considered in this study, the impacts of these climate-related phenomena are likely to vary spatially and temporally. Therefore, further analyses are needed to determine how these complex events will shape the future of the U.S. west coast Dungeness crab industry and other important fisheries.
Exposure of the CCS to Ocean Condition-Driven Changes in Dungeness Crab Catch
Our analysis showed that increasing SST in the CCS would decrease U.S. west coast CPUE of Dungeness crab with larger decreases in CPUE projected for the RCP 8.5 W/m2 radiative forcing scenario (+2.8°C) compared to RCP 4.5 W/m2 (+1.7°C). Decreases in CPUE would likely be caused by a shift in offshore distribution and accessibility of Dungeness crab to the fishing industry. However, the decrease in CPUE may not be monotonic along the coast, as the best model included a non-stationary effect of SST at a 5-year lag. Under both warming scenarios, our model found greater exposure of Dungeness crab CPUE to SST for southern latitude ports than northern latitude ports and indicates the possibility for a northward distribution shift of U.S. west coast Dungeness crab. Furthermore, there may be regional differences in the rate of SST warming, which could accentuate a northward distribution shift.
There is a generally good consensus that SST will increase in the CCS (IPCC, 2018) and other studies have also used SST to project or analyze species movements under climate change (e.g., Pinsky et al., 2013; Yen et al., 2016). It is less clear how large scale ocean-atmosphere climate patterns, such as PDO and NPGO, will shift in the future (Newman et al., 2016). In addition, local-scale differences in shelf dynamics could cause important spatial variability in temperature and upwelling (timing and strength). These uncertainties are not captured in our models. Reported changes in coastal winds and upwelling are spatially variable and have not resulted in a clear intensification of upwelling (Bakun et al., 2010; Sydeman et al., 2014). This spatial variability may explain why there was less support for the models of Upw and STI. However, the importance of upwelling dynamics in driving Dungeness crab dynamics, as well as other important fishery species, is well documented (Barth et al., 2007; Shanks and Roegner, 2007) and the relationship between climate, upwelling, and Dungeness crab is an important avenue for future investigation. Recent advances in upwelling index calculations may further elucidate the importance of upwelling (Jacox et al., 2018). Overall, Dungeness crab are affected by a variety of oceanic conditions and our findings highlight important implications of a changing ocean for fishing communities involved in the Dungeness crab industry.
Importance of Dungeness Crab to Coastal Communities
Economically valuable fisheries provide a direct link between changing ocean conditions and communities. U.S. west coast communities showed varying levels of social vulnerability and reliance on the Dungeness crab fishery that did not necessarily align with regional or management boundaries. Combining these metrics into a susceptibility score allowed us to determine that the risk of a change in Dungeness crab distribution or availability is unequal, with generally greater consequences for northern communities.
Given the projected decrease in Dungeness crab catch with increased SST, communities with the greatest potential for exposure were those in the central California management region. Although the likelihood of communities such as Morro Bay and Monterey experiencing a reduction in catch of Dungeness crab was relatively high, their overall susceptibility was low. On the other hand, Bodega Bay had higher reliance on the Dungeness crab fishery and was the most susceptible of the central California communities.
Oregon and Washington were less exposed to changes in Dungeness crab catch due to increased SST compared to California. However, many of these communities showed high social vulnerability and, ultimately, higher susceptibility. Therefore, the risk of reduced Dungeness crab catch would likely be more detrimental to communities in Oregon and Washington. As noted above, Bodega Bay, California represents a potential exception to this pattern. In addition, communities across the CCS have already been impacted by other climate-related phenomena and the subsequent management responses, including closures for the presence of domoic acid in crabs and hypoxia-related mass mortality of adult crab. Expected increases in the potency, duration, and occurrence rate of harmful algal blooms in marine systems (Gobler et al., 2017) point to increased domoic acid-related fishery closures across the U.S. west coast (McKibben et al., 2017). These closures can be economically damaging and may be the most immediate threat from changing ocean conditions to the Dungeness crab industry and associated coastal communities (Ritzman et al., 2018; Moore et al., 2019).
One caveat of this discussion is the fact that CSVI captures overall vulnerability of the “community of place” and is not specific to participants in the commercial fishing industry, which represents the “community of interest” (McMillan and Chavis, 1986; Nasar and Julian, 1995). Our analysis has captured only the potential risk for the larger community, but it is likely that individual community members would be differentially impacted depending on their connection to the fishery. For instance, fishermen and others directly involved in the fishing industry may experience more impacts than the larger community. This limitation also applies to other recent studies of exposure-vulnerability analyses of fisheries (e.g., Ekstrom et al., 2015; Mathis et al., 2015), but has not received much attention in the literature due to the challenges of characterizing sub-community level impacts (PFMC, 2017). Furthermore, socio-economic indices may not adequately capture intricate natural-human connections woven into fishing communities (Lavoie et al., 2018). However, by calculating a susceptibility score, we are able to apply the notion of vulnerability specifically to the relative importance of Dungeness crab in each community. This provides a general picture of how communities might be differentially susceptible to a change in the Dungeness crab fishery and may be useful for anticipating the needs of individual communities in the face of declining catch potential.
Study Limitations
In general, it is analytically challenging to capture the effect of individual ocean conditions on marine species due to the myriad of interacting physical and biological factors, variability in ocean conditions at multiple spatial and temporal scales, and often limited data on the distribution and abundance. Given these complexities and limited data on certain important ocean conditions, our modeling approach allowed us to describe historical trends and project CPUE under increased SST scenarios, but lacks mechanistic explanation for these changes. In the absence of mechanistic understanding, our projected future changes in CPUE with increased SST are based on the assumption that underlying mechanisms are linear and will continue unchanged into the future. However, synergies between temperature, ocean acidification, and hypoxia have been shown to alter the thermal tolerance of marine species (Pörtner et al., 2005). If the relationship between CPUE and SST has an energetic basis, then there are likely to be non-linearities and thresholds in the response of Dungeness crab to temperature. For example, Metzger et al. (2007) showed that elevated CO2 concentrations dramatically reduced the heat tolerance of edible crabs (Cancer pagurus).
For this analysis, we had to limit our inference to that of Dungeness crab CPUE due to the lack of available information about the actual offshore abundance and distribution of crab. Management of Dungeness crab does not include a formal stock assessment and adequate spatial information captured in fishing vessel logbooks is not available for all regions along the U.S. west coast. Without consistent logbook information, it is difficult to estimate fishing effort across all three states. Therefore, we standardized catch across ports and years using fish tickets following ODFW (2014). We assumed that if fishermen delivered crab to a port close to where it was caught, then an estimate of Dungeness crab commercial CPUE across U.S. west coast ports would be reflective of offshore abundance and distribution along the coast. A recent analysis of the Dungeness crab fishery logbooks and fish tickets by Richerson et al. (2020) found that delivery port was a good proxy for offshore catch area in Oregon. In addition, total catch was a good indication of the abundance of legal-sized male crabs (Richerson et al., 2020). Thus, a change in the magnitude or distribution of CPUE across ports could indicate a change in the offshore abundance or distribution of Dungeness crab associated with ocean conditions.
Although our data covered 36 years, some ocean condition factors operate on longer time scales. For instance, the data only spanned two major oscillations in PDO, which may have limited our ability to effectively describe the relationship between PDO and CPUE. Other ocean conditions not included in this analysis, due to insufficient data, may also contribute to Dungeness crab survival and distribution along the U.S. west coast, including hypoxia and ocean acidification. Such data could improve our mechanistic understanding of changes in Dungeness crab CPUE. These limitations reiterate the value of collecting long-term data series related to the success of socio-economically important fisheries under climate change.
Socio-economic data in our analysis were temporally limited and represent only a snapshot of each community. Indices obtained were based on 2014 (CSVI) and 2015 (Dungeness crab reliance) and may not be a comprehensive representation of the community condition from 1981 to 2017; however, these data represent warm ocean years in the CCS that may be common in the future (Cavole et al., 2016; Moore et al., 2019). In particular, 2015 was an anomalous year with the presence of “The Blob” of warm water in the N.E. Pacific Ocean (Kintisch, 2015; Di Lorenzo and Mantua, 2016; McCabe et al., 2016) and significant commercial fishery closures due to the presence of domoic acid (Ritzman et al., 2018). Future work should evaluate whether and how communities have historically fluctuated in their social vulnerability and reliance on fisheries and, furthermore, what community scenarios may be observed in the future. Long term data of reliance and vulnerability would allow for a more complete evaluation of community susceptibility, risk, and adaptive capacity.
Future Directions
Climate change is creating scenarios that are environmentally, ecologically, and socially novel. We found that decreased Dungeness crab catch from increasing ocean temperature has the potential to greatly impact fishery participants and U.S. west coast communities. In addition, substantial risks to the Dungeness crab fishery exist from other climate-related phenomena (e.g., domoic acid, hypoxia, and ocean acidification) that often co-occur with temperature. This study provides a valuable framework for understanding the social and ecological impacts of one important fishery in a single analysis, however, we recognize that this examination is insufficient to capture the full risk of climate change on U.S. west coast communities. For instance, many exposure-vulnerability analyses suffer from limited understanding of social and ecological adaptive capacity, particularly the timescales over which they operate. The social-ecological context of marine fisheries provides a particularly promising avenue for exploring adaptive capacity and untangling the complexity of coupled natural-human systems. There is great potential for future work to understand the possibility for resilience and adaptation in both coastal communities and target species. Among other social factors that contribute to adaptive capacity, flexibility to change strategies (Cinner and Barnes, 2019) is particularly salient to fishery systems where catch diversification (Kasperski and Holland, 2013; Cline et al., 2017) and the ability to adapt the distribution of fishery effort to track target species’ distributions as they move with climate (Perry et al., 2005) can reduce economic risk. Successful adaptive fishery management in a time of ocean change should rely on an understanding of projected fishery changes as well as the risks to coastal communities across the species’ current and future ranges.
Data Availability Statement
The datasets generated for this study are available on request to the corresponding author.
Author Contributions
All authors contributed to the conceptualization and design of the study. CM and EL obtained the fishery and environmental data and performed the analysis. AS and AJ obtained the social data and performed the analysis. CM, EL, AS, KS, and AJ wrote and revised the manuscript.
Funding
This project was made possible by the NSF-NRT award #1545188, “Risk and Uncertainty Quantification and Communication in Marine Science and Policy.”
Conflict of Interest
The authors declare that the research was conducted in the absence of any commercial or financial relationships that could be construed as a potential conflict of interest.
Acknowledgments
Engagement with numerous stakeholders and scientists was necessary for the success of this project. Data and guidance were provided by Kelly Corbett (ODFW), Troy Buell (ODFW), Mitch Vance (ODFW), Matt Hunter (ODFW), Thomas Swearingen (ODFW), Christy Jahasz (CDFW), Daniel Ayers (WDFW), Karma Norman (NOAA), Anna Varney (PSMFC), Jack Barth (OSU), Jennifer Fisher (NOAA), Gil Sylvia (OSU), Chris Cusack (OSU), and Malin Pinsky (Rutgers). Special thanks to the Oregon State University National Science Foundation Research Traineeship staff and faculty for supporting this project. Feedback from Francis Chan, Flaxen Conway, Sally Hacker, David Koslicki, Kathleen O’Malley, Su Sponaugle, and Lorenzo Ciannelli helped guide this research toward a productive and meaningful outcome.
Supplementary Material
The Supplementary Material for this article can be found online at: https://www.frontiersin.org/articles/10.3389/fmars.2020.00401/full#supplementary-material
References
Armstrong, D. A., Rooper, C., and Gunderson, D. (2003). Estuarine production of juvenile Dungeness crab (Cancer magister) and contribution to the Oregon-Washington coastal fishery. Estuaries 26, 1174–1188. doi: 10.1007/bf02803372
Bacheler, N. M., Bailey, K. M., Ciannelli, L., Bartolino, V., and Chan, K. S. (2009). Density-dependent, landscape, and climate effects on spawning distribution of walleye pollock Theragra chalcogramma. Mar. Ecol. Prog. Ser. 391, 1–12. doi: 10.3354/meps08259
Bakun, A., Black, B. A., Bograd, S. J., García-Reyes, M., Miller, A. J., Rykaczewski, R. R., et al. (2015). Anticipated effects of climate change on coastal upwelling ecosystems. Curr. Clim. Change Rep. 1, 85–93. doi: 10.1007/s40641-015-0008-4
Bakun, A., Field, D., Redondo-Rodriguez, A., and Weeks, S. (2010). Greenhouse gas, upwelling-favorable winds, and the future of coastal ocean upwelling ecosystems. Glob. Change Biol. 16, 1213–1228. doi: 10.1111/j.1365-2486.2009.02094.x
Barange, M., Merino, G., Blanchard, J. L., Scholtens, J., Harle, J., Allison, E. H., et al. (2014). Impacts of climate change on marine ecosystem production in societies dependent on fisheries. Nat. Clim. Change 4, 211–216. doi: 10.1111/gcb.14512
Barth, J., Menge, B., Lubchenco, J., Chan, F., Bane, J., Kirincich, A., et al. (2007). Delayed upwelling alters nearshore coastal ocean ecosystems in the northern California Current. Proc. Natl. Acad. Sci. U.S.A. 104, 3719–3724. doi: 10.1073/pnas.0700462104
Bartolino, V., Ciannelli, L., Bacheler, N. M., and Chan, K. S. (2011). Ontogeny and sex disentangle density-dependent and density-independent spatiotemporal dynamics of a marine fish population. Ecology 92, 189–200. doi: 10.1890/09-1129.1
Bednaršek, N., Feely, R. A., Beck, M. W., Alin, S. R., Siedlecki, S. A., Calosi, P., et al. (2020). Exoskeleton dissolution with mechanoreceptor damage in larval Dungeness crab related to severity of present-day ocean acidification vertical gradients. Sci. Total Environ. 716:136610. doi: 10.1016/j.scitotenv.2020.136610
Bograd, S., Schwing, F., Mendelssohn, R., and Green-Jessen, P. (2002). On the changing seasonality over the North Pacific. Geophys. Res. Lett. 29:47. doi: 10.1371/journal.pone.0067596
Bograd, S. J., Castro, C. G., Di Lorenzo, E., Palacios, D. M., Bailey, H., Gilly, W., et al. (2008). Oxygen declines and the shoaling of the hypoxic boundary in the California Current. Geophys. Res. Lett. 35:L12607.
Botsford, L. W. (1984). Effect of individual growth rates on expected behavior of the northern California Dungeness crab (Cancer magister) fishery. Can. J. Fish. Aquat. Sci. 41, 99–107. doi: 10.1139/f84-009
Botsford, L. W., Armstrong, D. A., and Shenker, J. M. (1989). Oceanographic influences on the dynamics of commercially fished populations. Elsevier Oceanogr. Ser. 47, 511–565. doi: 10.1016/s0422-9894(08)70355-6
Botsford, L. W., and Lawrence, C. (2002). Patterns of co-variability among California Current chinook salmon, coho salmon, Dungeness crab, and physical oceanographic conditions. Prog. Oceanogr. 53, 283–305. doi: 10.1016/s0079-6611(02)00034-4
Cavole, L. M., Demko, A. M., Diner, R. E., Giddings, A., Koester, I., Pagniello, C. M., et al. (2016). Biological impacts of the 2013–2015 warm-water anomaly in the Northeast Pacific: Winners, losers, and the future. Oceanography 29, 273–285.
Chan, F., Barth, J., Lubchenco, J., Kirincich, A., Weeks, H., Peterson, W., et al. (2008). Emergence of anoxia in the California Current large marine ecosystem. Science 319:920. doi: 10.1126/science.1149016
Cheung, W. W., Brodeur, R. D., Okey, T. A., and Pauly, D. (2015). Projecting future changes in distributions of pelagic fish species of Northeast Pacific shelf seas. Prog. Oceanogr. 130, 19–31. doi: 10.1016/j.pocean.2014.09.003
Cheung, W. W., Lam, V. W., Sarmiento, J. L., Kearney, K., Watson, R. E. G., Zeller, D., et al. (2010). Large-scale redistribution of maximum fisheries catch potential in the global ocean under climate change. Glob. Change Biol. 16, 24–35. doi: 10.1111/j.1365-2486.2009.01995.x
Ciannelli, L., Bartolino, V., and Chan, K. S. (2012). Non-additive and non-stationary properties in the spatial distribution of a large marine fish population. Proc. Biol. Sci. 279, 3635–3642. doi: 10.1098/rspb.2012.0849
Cinner, J. E., and Barnes, M. L. (2019). Social dimensions of resilience in social-ecological systems. One Earth 1, 51–56. doi: 10.1016/j.oneear.2019.08.003
Cline, T. J., Schindler, D. E., and Hilborn, R. (2017). Fisheries portfolio diversification and turnover buffer Alaskan fishing communities from abrupt resource and market changes. Nat. Commun. 8:14042. doi: 10.1038/ncomms14042
Davis, S., Sylvia, G., Yochum, N., and Cusack, C. (2017). Oregon Dungeness Crab Fishery Bioeconomic Model: A Fishery Interactive Simulator Learning Tool. Available at: http://oregondungeness.org/wp-content/uploads/2017/06/ODCC-crab-model-report-ver.-5.7.pdf (accessed August 9, 2018).
Di Lorenzo, E., and Mantua, N. (2016). Multi-year persistence of the 2014/15 North Pacific marine heatwave. Nat. Clim. Change 6, 1042–1047. doi: 10.1038/nclimate3082
Di Lorenzo, E., Schneider, N., Cobb, K. M., Franks, P. J. S., Chhak, K., Miller, A. J., et al. (2008). North Pacific Gyre Oscillation links ocean climate and ecosystem change. Geophys. Res. Lett. 35:L08607. doi: 10.1111/gcb.13171
Ebert, E., Haseltine, A., Houk, J., and Kelly, R. (1983). Laboratory cultivation of the Dungeness crab, Cancer magister. Fish. Bull. 172, 259–310.
Ekstrom, J. A., Suatoni, L., Cooley, S. R., Pendleton, L. H., Waldbusser, G. G., Cinner, J. E., et al. (2015). Vulnerability and adaptation of US shellfisheries to ocean acidification. Nat. Clim. Change 5, 207–214. doi: 10.1038/nclimate2508
Gentemann, C. L., Fewings, M. R., and García-Reyes, M. (2017). Satellite sea surface temperatures along the West Coast of the United States during the 2014–2016 northeast Pacific marine heat wave. Geophys. Res. Lett. 44, 312–319. doi: 10.1002/2016gl071039
Gobler, C. J., Doherty, O. M., Hattenrath-Lehmann, T. K., Griffith, A. W., Kang, Y., and Litaker, R. W. (2017). Ocean warming since 1982 has expanded the niche of toxic algal blooms in the North Atlantic and North Pacific oceans. Proc. Natl. Acad. Sci. U.S.A. 114, 4975–4980. doi: 10.1073/pnas.1619575114
Grantham, B. A., Chan, F., Nielsen, K. J., Fox, D. S., Barth, J. A., Huyer, A., et al. (2004). Upwelling-driven nearshore hypoxia signals ecosystem and oceanographic changes in the northeast Pacific. Nature 429, 749–754. doi: 10.1038/nature02605
Gruber, N., Hauri, C., Lachkar, Z., Loher, D., Frölicher, T. L., and Plattner, G.-K. (2012). Rapid progression of ocean acidification in the California Current System. Science 337, 220–223. doi: 10.1126/science.1216773
Helliwell, V. (2009). Fisheries management for California Dungeness Crab—adapting to change. Coast. Manag. 37, 491–500. doi: 10.1080/08920750903269280
Higgins, K., Hastings, A., Sarvela, J., and Bostford, L. (1997). Stochastic dynamics and deterministic skeletons: population behavior of Dungeness crab. Science 276, 1431–1435. doi: 10.1126/science.276.5317.1431
Himes-Cornell, A., Maguire, C., Kasperski, S., Hoelting, K., and Pollnac, R. (2016). Understanding vulnerability in Alaska fishing communities: a validation methodology for rapid assessment of indices related to well-being. Ocean Coast. Manag. 124, 53–65. doi: 10.1016/j.ocecoaman.2016.02.004
Hoegh-Guldberg, O., and Bruno, J. (2010). The impact of climate change on the world’s marine ecosystems. Science 328, 1523–1528. doi: 10.1126/science.1189930
Holsman, K., Hollowed, A., Ito, S., Bograd, S., Hazen, E., King, J., et al. (2019). Climate Change Impacts, Vulnerabilities and Adaptations: North Pacific and Pacific Arctic Marine Fisheries. In Impacts of Climate Change on Fisheries and Aquaculture, eds M. Barange, T. Bahri, M. C. M. Beveridge, K. L. Cochrane, S. F. Smith, and F. Poulain, (Geneva: Food and Agriculture Organization of the United Nations).
IPCC (2018). “Summary for Policymakers,” in Global warming of 1.5°C. An IPCC Special Report on the Impacts of Global Warming of 1.5°C Above Pre-Industrial Levels and Related Global Greenhouse Gas Emission Pathways, in the Context of Strengthening the Global Response to the Threat of Climate Change, Sustainable Development, and Efforts to Eradicate Poverty, eds V. Masson-Delmotte, P. Zhai, H. O. Pörtner, D. Roberts, J. Skea, P. R. Shukla, et al. (Geneva: IPCC), 32.
Jacox, M. G., Edwards, C. A., Hazen, E. L., and Bograd, S. J. (2018). Coastal upwelling revisited: Ekman, Bakun, and improved upwelling indices for the U.S. west coast. J. Geophys. Res. Oceans 123, 7332–7350. doi: 10.1029/2018jc014187
Jacox, M. G., Hazen, E. L., Zaba, K. D., Rudnick, D. L., Edwards, C. A., Moore, A. M., et al. (2016). Impacts of the 2015-2016 El Niño on the California Current System: early assessment and comparison to past events. Geophys. Res. Lett. 43, 7072–7080. doi: 10.1002/2016gl069716
Jepson, M., and Colburn, L. (2013). Development of Social Indicators of Fishing Community Vulnerability and Resilience in the US Southeast and Northeast Regions. Silver Spring, MD: NOAA
Jewett, L., and Romanou, A. (2017). “Ocean acidification and other ocean changes,” in Climate Science Special Report: Fourth National Climate Assessment, Vol. I, eds D. J. Wuebbles, D. W. Fahey, K. A. Hibbard, D. J. Dokken, B. C. Stewart, and T. K. Maycock, (Washington, DC: U.S. Global Change Research Program), 364–392. doi: 10.7930/J0QV3JQB
Johnson, D. F., Botsford, L. W., Methot, R. D. Jr., and Wainwright, T. C. (1986). Wind stress and cycles in Dungeness crab (Cancer magister) catch off California, Oregon, and Washington. Can. J. Fish. Aquat. Sci. 43, 838–845. doi: 10.1139/f86-103
Jones, M. C., Dye, S. R., Pinnegar, J. K., Warren, R., and Cheung, W. W. (2015). Using scenarios to project the changing profitability of fisheries under climate change. Fish Fish. 16, 603–622. doi: 10.1111/faf.12081
Kasperski, S., and Holland, D. S. (2013). Income diversification and risk for fishermen. Proc. Natl. Acad. Sci. U.S.A. 110, 2076–2081. doi: 10.1073/pnas.1212278110
Kilduff, D. P., Di Lorenzo, E., Botsford, L. W., and Teo, S. L. H. (2015). Changing central Pacific El Ninos reduce stability of North American salmon survival rates. Proc. Natl. Acad. Sci. U.S.A. 112, 10962–10966. doi: 10.1073/pnas.1503190112
Kintisch, E. (2015). ‘The Blob’ invades Pacific, flummoxing climate experts. Science 348, 17–18. doi: 10.1126/science.348.6230.17
Lavoie, A., Sparks, K., Kasperski, S., Himes-Cornell, A., Hoelting, K., and Maguire, C. (2018). Ground-truthing social vulnerability indices of Alaska fishing communities. Coast. Manag. 46, 359–387. doi: 10.1080/08920753.2018.1498710
Lenoir, J., and Svenning, J. (2015). Climate-related range shifts - a global multidimensional synthesis and new research directions. Ecography 38, 15–28. doi: 10.1111/ecog.00967
Litzow, M. A., Ciannelli, L., Cunningham, C. J., Johnson, B., and Puerta, P. (2019). Nonstationary effects of ocean temperature on Pacific salmon productivity. Can. J. Fish. Aquat. Sci. 76, 1923–1928. doi: 10.1139/cjfas-2019-0120
Litzow, M. A., Hunsicker, M. E., Bond, N. A., Burke, B. J., Cunningham, C. J., Gosselin, J. L., et al. (2020). The changing physical and ecological meanings of North Pacific Ocean climate indices. Proc. Natl. Acad. Sci. U.S.A. 117, 7665–7671. doi: 10.1073/pnas.1921266117
Mantua, N., Hare, S., Zhang, Y., Wallace, J., Francis, R., Mantua, N., et al. (1997). A Pacific interdecadal climate oscillation with impacts on salmon production. Bull. Am. Meteorol. Soc. 78, 1069–1079. doi: 10.1175/1520-0477(1997)078<1069:apicow>2.0.co;2
Marshall, K., Kaplan, I., Hodgson, E., Hermann, A., Busch, D., McElhany, P., et al. (2017). Risks of ocean acidification in the California Current food web and fisheries: ecosystem model projections. Glob. Change Biol. 23, 1525–1539. doi: 10.1111/gcb.13594
Mathis, J. T., Cooley, S. R., Lucey, N., Colt, S., Ekstrom, J., Hurst, T., et al. (2015). Ocean acidification risk assessment for Alaska’s fishery sector. Prog. Oceanogr. 136, 71–91. doi: 10.1016/j.pocean.2014.07.001
McCabe, R. M., Hickey, B. M., Kudela, R. M., Lefebvre, K. A., Adams, N. G., Bill, B. D., et al. (2016). An unprecedented coastwide toxic algal bloom linked to anomalous ocean conditions. Geophys. Res. Lett. 43, 10366–10376.
McKibben, S., Peterson, W., Wood, A., Trainer, V., Hunter, M., and White, A. (2017). Climatic regulation of the neurotoxin domoic acid. Proc. Natl. Acad. Sci. U.S.A. 114, 239–244. doi: 10.1073/pnas.1606798114
McMillan, D. W., and Chavis, D. M. (1986). Sense of community: a definition and theory. J. Community Psychol. 14, 6–23. doi: 10.1002/1520-6629(198601)14:1<6::aid-jcop2290140103>3.0.co;2-i
Metzger, R., Sartoris, F. J., Langenbuch, M., and Pörtner, H. O. (2007). Influence of elevated CO2 concentrations on thermal tolerance of the edible crab Cancer pagurus. J. Therm. Biol. 32, 144–151. doi: 10.1016/j.jtherbio.2007.01.010
Moore, S. K., Cline, M. R., Blair, K., Klinger, T., Varney, A., and Norman, K. (2019). An index of fisheries closures due to harmful algal blooms and a framework for identifying vulnerable fishing communities on the U.S. West Coast. Mar. Policy 110:103543. doi: 10.1016/j.marpol.2019.103543
Nasar, J. L., and Julian, D. A. (1995). The psychological sense of community in the neighborhood. J. Am. Plann. Assoc. 61, 178–184. doi: 10.1080/01944369508975631
Nelson, C. (1977). Wind Stress and Wind Stress Curl Over the California Current. U.S. Department of Commerce. National Oceanic and Atmospheric Administration. NOAA Technical Report NMFS SSRF-714. Available at: https://swfsc.noaa.gov/publications/CR/1977/7737.PDF (accessed February 6, 2019).
Newman, M., Alexander, M., Ault, T., Cobb, K., Deser, C., Di Lorenzo, E., et al. (2016). The pacific decadal oscillation, revisited. Am. Meteorol. Soc. 29, 4399–4427. doi: 10.1007/s00382-018-4240-1
NOAA, (2017). Engagement and Reliance. National Oceanic and Atmospheric Administration Fisheries. Silver Spring, MD: NOAA.
Norman, K., Sepez, J., Lazrus, H., Milne, N., Package, C., Russell, S., et al. (2007). Community Profiles for West Coast and North Pacific Fisheries. Washington, Oregon, California, and other U.S. States. Silver Spring, MD: NOAA, 1–617.
Nye, J., Link, J., Hare, J., and Overholtz, W. (2009). Changing spatial distribution of fish stocks in relation to climate and population size on the Northeast United States continental shelf. Mar. Ecol. Prog. Ser. 393, 111–129. doi: 10.3354/meps08220
ODFW, (2014). Commercial Ocean Dungeness Crab Fisher Logbook CPUE Preliminary Assessment- 3rd Iteration. Oregon Department of Fish and Wildlife Marine Resources Program.Available at: https://dfw.state.or.us/agency/commission/minutes/14/08_august/Exhibit F_Attachment 11_Commercial Ocean Dungeness Crab Fishery Logbook.pdf(accessed March, 2014).
ORAP, (2013). Implementing Ecosystem-Based Management: A Report to the National Ocean Council. Arlington, VA: NOPP, 33.
Perry, A., Low, P., Ellis, J., and Reynolds, J. (2005). Climate change and distribution shifts in marine fishes. Science 308, 1912–1915. doi: 10.1126/science.1111322
PFMC, (2017). Habitat and Communities: Fishing Communities. Available at: https://www.pcouncil.org/habitat-and-communities/fishing-communities/ (accessed August 08, 2019)Google Scholar
Pinheiro, J., Bates, D., DebRoy, S., Sarkar, D., and R Core Team (2019). nlme: Linear and Nonlinear Mixed Effects Models. R package version 3:1-139. Available at: https://CRAN.R-project.org/package=nlme (accessed September 13, 2019).
Pinsky, M., Worm, B., Fogarty, M., Sarmiento, J., and Levin, S. (2013). Marine taxa track local climate velocities. Science 341, 1239–1242. doi: 10.1126/science.1239352
Pörtner, H. O., Langenbuch, M., and Michaelidis, B. (2005). Synergistic effects of temperature extremes, hypoxia, and increases in CO2 on marine animals: From Earth history to global change. J. Geophys. Res. Oceans 110, 1–15.
PSMFC, (2014). Dungeness Crab Report. Available at: http://www.psmfc.org/crab/2014-2015%20files/DUNGENESS%20CRAB%20REPORT2014.pdf (accessed November 09, 2019).
Rasmuson, L. (2013). The biology, ecology and fishery of the Dungeness crab, Cancer magister. Adv. Mar. Biol. 65, 95–148. doi: 10.1016/B978-0-12-410498-3.00003-3
Richerson, K., Punt, A. E., and Holland, D. S. (2020). Nearly a half century of high but sustainable exploitation in the Dungeness crab (Cancer magister) fishery. Fish. Res. 226:105528. doi: 10.1016/j.fishres.2020.105528
Ritzman, J., Brodbeck, A., Brostrom, S., McGrew, S., Dreyer, S., Klinger, T., et al. (2018). Economic and sociocultural impacts of fisheries closures in two fishing-dependent communities following the massive 2015 U.S. West Coast harmful algal bloom. Harmful Algae 80, 35–45. doi: 10.1016/j.hal.2018.09.002
Schmidt, A. E., Botsford, L. W., Eadie, J. M., Bradley, R. W., Di Lorenzo, E., and Jahncke, J. (2014). Non-stationary seabird responses reveal shifting ENSO dynamics in the northeast Pacific. Mar. Ecol. Prog. Ser. 499, 249–258. doi: 10.3354/meps10629
Schultz, I., Skillman, A., Sloan-Evans, S., and Woodruff, D. (2013). Domoic acid toxicokinetics in Dungeness crabs: new insights into mechanisms that regulate bioaccumulation. Aquat. Toxicol. 140–141, 77–88. doi: 10.1016/j.aquatox.2013.04.011
Shanks, A. (2013). Atmospheric forcing drives recruitment variation in the Dungeness crab (Cancer magister), revisited. Fish. Oceanogr. 22, 263–272. doi: 10.1111/fog.12020
Shanks, A., Roegner, G., and Miller, J. (2010). Using megalopae abundance to predict future commercial catches of Dungeness crabs (Cancer magister) in Oregon. CalCOFI Rep. 51, 1–13.
Shanks, A. L., and Roegner, G. C. (2007). Recruitment limitation in Dungeness crab populations is driven by variation in atmospheric forcing. Ecology 88, 1726–1737. doi: 10.1890/06-1003.1
Snyder, M., Sloan, L., Diffenbaugh, N., and Bell, J. (2003). Future climate change and upwelling in the California Current. Geophys. Res. Lett. 30:1823.
Sydeman, W., Garcia-Reyes, M., Schoeman, D., Rykaczewski, D., Thompson, S., Black, B., et al. (2014). Climate change and wind intensification in coastal upwelling ecosystems. Science 345, 77–80. doi: 10.1126/science.1251635
Sydeman, W. J., Santora, J. A., Thompson, S. A., Marinovic, B., and Lorenzo, E. D. (2013). Increasing variance in North Pacific climate relates to unprecedented ecosystem variability off California. Glob. Change Biol. 19, 1662–1675. doi: 10.1111/gcb.12165
Trainer, V., Cochlan, W., Erickson, A., Bill, B., Cox, F., Borchert, J., et al. (2007). Recent domoic acid closures of shellfish harvest areas in Washington State inland waterways. Harmful Algae 6, 449–459. doi: 10.1016/j.hal.2006.12.001
Varney, A. (2018). Community Social Vulnerability Indices. National Oceanic and Atmospheric Administration, Northwest Fisheries Science Center. Available at: https://www.webapps.nwfsc.noaa.gov/apex/parrdata/inventory/tables/table/community_social_vulnerability_indicies (accessed May 14, 2019).
Wood, S. N. (2017). Generalized Additive Models: An Introduction with R, 2nd Edn. London: Chapman and Hall. doi: 10.1201/9781315370279
Wood, S. N. (2019). Package ‘mgcv’. Available at: http://cran.r-project.org/web/packages/mgcv/mgcv.pdf (accessed August 08, 2019).
Yen, K. W., Su, N. J., Teemari, T., Lee, M. A., and Lu, H. J. (2016). Predicting the catch potential of skipjack tuna in the western and central Pacific Ocean under different climate change scenarios. J. Mar. Sci. Technol. 24, 1053–1062.
Keywords: Dungeness crab, climate change, species distribution, ocean conditions, fisheries, coupled natural-human system
Citation: Magel CL, Lee EMJ, Strawn AM, Swieca K and Jensen AD (2020) Connecting Crabs, Currents, and Coastal Communities: Examining the Impacts of Changing Ocean Conditions on the Distribution of U.S. West Coast Dungeness Crab Commercial Catch. Front. Mar. Sci. 7:401. doi: 10.3389/fmars.2020.00401
Received: 28 February 2020; Accepted: 08 May 2020;
Published: 16 June 2020.
Edited by:
Maria Grazia Pennino, Oceanographic Center of Vigo, Spanish Institute of Oceanography, SpainReviewed by:
Blaine D. Griffen, Brigham Young University, United StatesKevin Alexander Hovel, San Diego State University, United States
Copyright © 2020 Magel, Lee, Strawn, Swieca and Jensen. This is an open-access article distributed under the terms of the Creative Commons Attribution License (CC BY). The use, distribution or reproduction in other forums is permitted, provided the original author(s) and the copyright owner(s) are credited and that the original publication in this journal is cited, in accordance with accepted academic practice. No use, distribution or reproduction is permitted which does not comply with these terms.
*Correspondence: Caitlin L. Magel, whitecai@oregonstate.edu