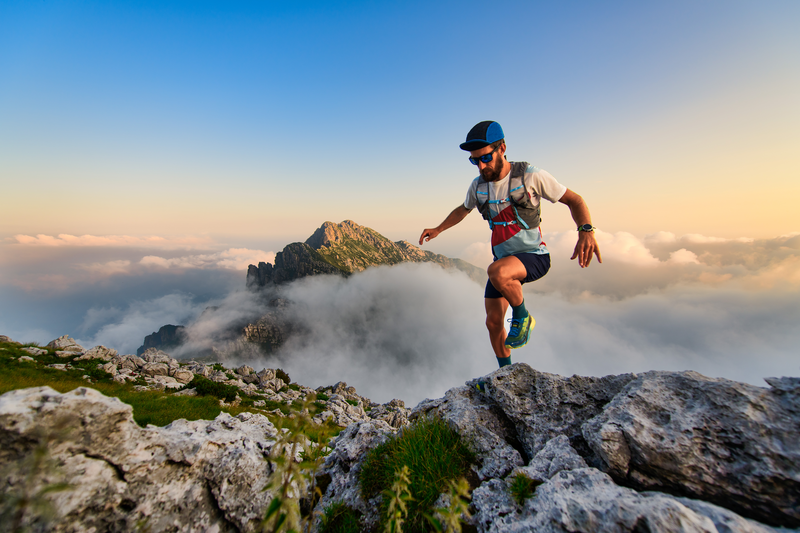
94% of researchers rate our articles as excellent or good
Learn more about the work of our research integrity team to safeguard the quality of each article we publish.
Find out more
ORIGINAL RESEARCH article
Front. Mar. Sci. , 28 May 2020
Sec. Aquatic Physiology
Volume 7 - 2020 | https://doi.org/10.3389/fmars.2020.00394
This article is part of the Research Topic Environmental Stress-Promoting Responses in Algae View all 9 articles
The timing of the transition from growth to reproduction is essential for the regulation of the seaweed life cycle. Variable environmental conditions can stress seaweeds and promote trade-offs between their growth and reproduction. Here, we demonstrate that reproductive responses can be induced by environmental stresses in the gametophytic thalli of the marine red alga Pyropia yezoensis. Excision of explants accelerated release of asexual monospores and sexual carpospores. The algal sensitivity to wounding was enhanced by a 3-day dark treatment prior to the excision of the explants. By contrast, heat stress at 25°C stimulated the production of a callus, a three-dimensional aggregation of randomly divided cells with multiple cell layers. This callus produced new gametophytic thalli with a normal shape; therefore, callus formation is thought to be one of the asexual reproductive strategies used by the alga to increase the number of thalli under heat stress conditions. Our results demonstrate that wounding and heat stress can reset the timing of reproduction and that gametophytic thalli therefore use a variety of distinct reproductive strategies under different stress conditions. These findings provide insights into the induction of reproduction by environmental stresses as a life cycle trade-off in seaweeds.
Plants and seaweeds control the timing of their growth and reproduction in response to environmental stresses, trading off these phases to optimize their life cycle progression and best adapt to the variable natural conditions they experience (Liu et al., 2017; Mohring et al., 2013; Karasov et al., 2017; Shaar-Moshe et al., 2019). The elucidation of the mechanisms regulating the trade-off between growth and reproduction in response to changes in the environmental conditions is fundamental for understanding how seaweeds integrate environmental signals and adjust their life cycle accordingly.
The Bangiales, including the marine red algal genera Pyropia and Porphyra, undergo a haploid-diploid heteromorphic life cycle, in which the haploid leafy gametophyte (thallus) and the diploid filamentous sporophyte (conchocelis) occur in a mutually exclusive manner (Blouin et al., 2011; Mikami et al., 2012; Takahashi and Mikami, 2017; Adams et al., 2018). The life cycle of Pyropia is in fact triphasic consisting of gametophyte, sporophyte and diploid conchosporophyte parasitically generated on sporophyte to produce gametophytes by apospory (Mikami et al., 2019). It is well known that the fertilization of male and female gametes, both of which develop on gametophytic thalli, results in the establishment of the sporophyte generation through the production and germination of diploid carpospores (Blouin et al., 2011; Takahashi and Mikami, 2017; Mikami et al., 2019). Gametogenesis, performed by the gametophytes, is reportedly stimulated by a temperature upshift and changes in the daylight length in the marine red alga Pyropia yezoensis and other Bangiophycean species (Avila et al., 1986; Brawley and Johnson, 1992; Sidirelli-Wolf, 1992; Notoya and Nagaura, 1998; Notoya and Miyashita, 1999; Monotilla and Notoya, 2004, 2010; Kakinuma et al., 2006; Liu et al., 2017). Since we have observed that the stimulation of gametogenesis by environmental cues lowers the growth rate of the thalli by reducing the number of vegetative cells, sexual reproduction is thought to represent a life cycle trade-off in gametophytic thalli.
In addition to sexual reproduction, P. yezoensis also undergoes asexual reproduction, producing gametophytic clones via the differentiation of vegetative cells to asexual spores called monospores or archespores (Li et al., 2008, 2009; Takahashi et al., 2010; Mikami et al., 2012; Takahashi and Mikami, 2017). This process also reduces the number of vegetative cells available for the growth of the existing thalli; therefore, asexual propagation also involves trade-offs between growth and reproduction. The production of monospores in P. yezoensis is strongly stimulated by an increase in light intensity (Ying, 1984), wounding (Hafting, 1999), hypotonic stress (Li et al., 2008, 2009), reduction in extracellular calcium ion concentrations (Takahashi et al., 2010), and oxidative stress (Takahashi and Mikami, 2017). The asexual life cycle of P. yezoensis is therefore activated by environmental stresses and is dependent on trade-offs between growth and reproduction.
Despite the accumulation of evidence suggesting the existence of life cycle trade-offs during reproduction, little is known about the promotion of sexual and asexual reproduction by environmental stress in the Bangiales. In this study, we examined the effects of wounding and heat stress on the life cycle trade-offs in P. yezoensis gametophytes. Our results demonstrate the presence of asexual and sexual life cycle trade-off strategies, which were accelerated by the wounding of the gametophytes and further enhanced by a dark treatment prior to wounding. In addition, a wounding-independent but heat-dependent activation of the asexual strategy was also observed. These findings indicate an intrinsic ability for the flexible selection of different reproductive strategies involving life cycle trade-offs in P. yezoensis, which maximize survival under various stress conditions.
Gametophytic blades and spores of the P. yezoensis strain U-51 were cultured in sterilized artificial seawater (SEALIFE; Marinetech, Tokyo, Japan) containing the nutritional mixture ESS2 with NaNO3 as a nitrogen source, vitamins, and trace metal elements (Takahashi et al., 2010; Li et al., 2019). The samples were cultured in 200 ml of medium under 60–70 μmol photons m–2 s–1 in a short-day photoperiod (10 h light/14 h dark) at various temperatures, aerated with air filtered through a 0.22 μm filter (Whatman; GE Healthcare, Chicago, IL, United States). The culture medium was changed weekly.
Thalli were cultured in 9 cm dishes [Asnol petri dishes: 90 mm (diameter) × 20 mm (height); As One Corporation, Osaka, Japan] containing 30 ml seawater for a week at 15°C or 4°C in darkness or in light. A razor blade was used to excise 1 mm2 gametophytic portions from the tip, middle (0.8 cm from the tip), and bottom (1.6 cm from the tip) of thalli 2.0–2.4 cm in length, inducing a wounding response. The number of spores released and adhered to the bottom of culture dishes was counted by observation using microscope. Explants were also excised from the tips of thalli 0.7, 1.7, 2.4, and 3.2 cm in length. The gametogenesis and callus formation processes were observed in all samples.
To further explore the callus formation process, nonwounded thalli were also incubated at 15°C with or without a 3-day pretreatment in the dark and at 25°C without any pretreatment. The samples were observed and imaged using an Olympus IX73 light microscope (Olympus, Tokyo, Japan) equipped with an Olympus DP22 camera.
Values are indicated with ± SD from triplicated experiments for counting the number of released monospores. Since we proposed that effects of experimental conditions on reproductive responses is able to be evaluated by comparison of the number of released monospores among treatments every day (1–7 days) using excised portions from the same thallus as performed in our experiments, a one-way ANOVA followed by a Tukey–Kramer test was used for multiple comparisons, and significant differences were determined using a cutoff value of p < 0.05.
First, we reexamined the wounding-dependent acceleration of asexual spore release reported by Hafting (1999). We excised 1 mm2 explants from the tips of thalli grown at 15°C and incubated them at 15°C for a further seven days under short-day photoperiod conditions, resulting in the release of a large number of spores (Figures 1, 2), indicating the promotion of spore release by wounding. In addition, these spores developed into gametophytic thalli or sporophytic conchocelis (Figure 1B), the latter of which were rare and dependent on the gametogenesis and fertilization of male and female gametes in the wounded thalli (Figure 1C). Wounding therefore stimulated both asexual and sexual reproduction, resulting in the production of monospores and carpospores developing into leafy thalli and conchocelis filaments, respectively.
Figure 1. Wounding-promoted asexual and sexual reproduction in gametophytic thalli. (A) Discharge of spores from a thallus observed 3 days after wounding. (B) Confirmation of the presence of monospores by the observation of a developing thallus and carpospores indicated by a developing conchocelis (arrow) 7 days after wounding. (C) Promotion of gametogenesis observed 5 days after wounding. Scale bars, 100 μm in (A,C), 25 μm in (B).
Figure 2. Differences in the sensitivity to wounding in the top, middle, and bottom regions of the gametophytic thallus. Explants were excised from the tips, middles (0.8 cm from the tip), and bottoms (1.6 cm from the tip) of thalli and incubated for 7 days. Discharged spores were counted daily. Error bars indicate the standard deviation of data from triplicate experiments (n = 3). Different letters indicate significant differences at p < 0.05.
When explants from three different areas of the thallus were cultured for 7 days, the total number of spores released was highest from the samples excised from the tip, with the fewest spores released from the tissues taken from the bottom of the thallus (Figure 2). Monospores comprised the majority of the spores released by the samples extracted from all three regions (Supplementary Figure S1). These findings indicate that all regions of the thallus can respond to wounding through the acceleration of the asexual life cycle, however, the number of reproductive spores produced in response to wounding stress varied between the portions. Moreover, when the wounding response in thalli at different growth stages was examined using the tips of 0.7, 1.7, 2.4, and 3.2 cm thalli, more spores were found to be released from the thalli 2.4 and 3.2 cm in length than by the shorter and potentially less well-developed thalli (Supplementary Figure S2). The sensitivity to wounding therefore increases during thallus growth development. Based on the above findings, we used explants from the tips of 2–3 cm thalli in the following experiments.
We noticed that when we used thalli sent via a refrigerated delivery service for experiments immediately after their arrival, they released a markedly increased number of spores. Since these thalli were exposed to both cold and dark conditions for 3 days during this transportation, we hypothesized that low-temperature stress and/or dark treatment could increase the sensitivity of the thalli to the wound stress.
To address this possibility, the wounding-dependent production and release of spores was examined using thalli pretreated for 3 days with darkness or light at 4 or 15°C prior to wounding. The thalli pretreated with darkness at 4°C released more spores than those cultured under the normal conditions of 15°C and light (Figure 3). To distinguish whether this increased spore release was accelerated by the cold or the dark treatment, we compared the number of spores released by thalli pretreated in the light at 4°C or in darkness at 15°C. The thalli pretreated at 15°C in the dark released significantly more spores than the control thalli cultured in light, while those pretreated at 4°C in the light released a similar number of spores to the control.
Figure 3. Effects of 3-day pretreatments of thalli with low temperature (4°C) or darkness prior to wounding. Discharged spores were counted daily. Error bars indicate the standard deviation of data from triplicate experiments (n = 3). Different letters indicate significant differences at p < 0.05.
We further showed that the dark treatment enhanced the release of both the monospores and carpospores (Supplementary Figure S3). Moreover, nonwounded thalli also released more spores when pretreated in darkness at 15°C (Supplementary Figure S4), although much fewer spores were released from these thalli than from the wounded explants under the same conditions (Figure 3 and Supplementary Figures S3, S4). We therefore concluded that the 3-day pretreatment in darkness is responsible for enhancing spore release and increasing the sensitivity of thalli to wounding.
When the thallus explants were incubated at 25°C for 1 week, they produced calli composed of many brownish-red cells that differed from the surrounding vegetative and sexually mature cells (Figure 4A). The calli were usually produced at the edge of the explants (Figures 4B,C) and comprised a three-dimensional structure with randomly aggregated brownish-red cells (Figures 4D,E). Normal thalli developed from the calli produced at 25°C (Figure 4F), indicating that the production of calli under heat stress conditions is an asexual reproductive response to generate new gametophytic thalli.
Figure 4. Heat stress-induced callus production in wounded explants. (A) Production of calli at the edge of explants cultured at 25°C for 1 week. (B) Magnified view of calli at the edge of the thallus. (C,D) Vertical (C) and cross-section (D) views of the callus displaying the random and multilayer aggregation of cells. (E) Generation of a gametophytic thallus from a callus. Scale bars, 200 μm for all panels.
Moreover, calli were also frequently produced by nonwounded whole thalli under heat stress conditions (Figure 5). We therefore concluded that heat stress promotes callus production to accelerate the asexual propagation of gametophytic thalli, independent of wounding. Spore release was not observed at 25°C either with or without the dark pretreatment (data not shown).
Figure 5. Heat stress-induced callus production in a nonwounded thallus. When a nonwounded thallus (A) was incubated at 25°C for 2 weeks, many calli were produced at its top (B) and bottom (C). Blue circles correspond to top and bottom positions used for the observations. The development of a normal thallus from a callus is indicated in (B) by an arrow. Scale bars, 0.5 cm for (A), 100 μm for (B,C).
Here, we demonstrated that spore release and the gametogenesis of male and female gametes was enhanced by wounding the gametophytic thalli of P. yezoensis and that the formation of calli in these tissues could be induced by heat stress. These findings indicate that the gametophytic thallus has the ability to respond to environmental stresses by accelerating asexual and sexual reproduction, with different strategies of reproductive activation induced by different stresses. It is clear that wounding directs the transition of the life history strategy from growth to reproduction. Similarly, the heat stress-induced formation of calli with accelerated and nonorganized cell division to generate three-dimensional structures was an asexual reproductive process, resulting in the production of new thalli that can survive and grow under high-temperature conditions. We therefore concluded that the gametophytic thallus P. yezoensis can recognize different stresses and select an asexual or sexual reproductive response as a life cycle trade-off.
As shown in Figure 1B, the thallus explants released both monospores and carpospores, unlike a previous report (Hafting, 1999) that did not observe wounding-promoted induction of gametogenesis and production of carpospores. Since wounding promoted gametogenesis (Figure 1C), we hypothesized that the carpospores were produced by the fertilization of the male and female gametes, however, we previously showed that oxidative stress-dependent diploid apogamy produced carpospores without gametogenesis in P. yezoensis (Takahashi and Mikami, 2017; Mikami, 2019). This means that we cannot exclude the possibility that wounding may partially promote diploid apogamy, since wounding produces reactive oxygen species (ROS) that cause oxidative stress in plants (León et al., 2001; Beneloujaephajri et al., 2013; Baxter et al., 2014) as well as in seaweeds (Mcdowell et al., 2014a, b). To explore this possibility, a new experimental system should be developed to separate spore release and gametogenesis, both of which were promoted by wounding. In this respect, mutants lacking gametogenesis could be useful for exploring the possible inducibility of apogamy by wounding, however, we are not aware of any mutants lacking gametogenesis in Pyropia.
The promotion of gametogenesis by wounding has also been observed in the green algal genus Ulva, including in U. linza, U. lactuca, U. prolifera, and U. mutanilis (Gao et al., 2010; Wichard and Oertel, 2010; Vesty et al., 2015; Katsaros et al., 2017). In U. linza, for example, chopping gametophytes into 3–5 mm fragments induced gametogenesis within 48 h and gamete discharge in 3 days (Vesty et al., 2015). Thus, the sexual reproductive response to wounding could be conserved in the red and green macroalgae. Species in the genus Ulva produce sporulation inhibitors (SI-1 and SI-2) and a swarming inhibitor (SW1) that inhibit gametogenesis and gamete discharge, respectively (Stratmann et al., 1996; Wichard and Oertel, 2010; Vesty et al., 2015). It is plausible that wounding might prevent the production or function of these inhibitors, promoting the reproductive response. It is currently unclear whether P. yezoensis possesses homologs of the sporulation and swarming inhibitors found in Ulva; therefore, it would be valuable to determine whether these factors exist in the Bangiales and to explore whether wounding or other stresses inhibit their production or activities. This would help to elucidate the mechanisms responsible for the regulation of the life cycle trade-offs in these algae.
We previously demonstrated that the asexual reproductive response in P. yezoensis was enhanced under hypotonic and oxidative stress conditions, as well as by a decrease in the extracellular concentration of calcium ions (Li et al., 2008, 2009; Takahashi et al., 2010; Takahashi and Mikami, 2017). The effects of hypotonic stress on the monospore release were originally observed in Porphyra pulchella (Ackland et al., 2007). In addition, the promotion of the asexual life cycle by heat stress was previously observed in the red alga Bangia fuscopurpurea (Notoya and Iijima, 2003; Wang et al., 2008; Mikami and Kishimoto, 2018), although in this study we found that heat stress did not stimulate monospore release in P. yezoensis (data not shown). These findings indicate that some Bangiales species can respond to environmental stresses using life cycle trade-offs. The reproductive processes promoted by the various stresses differ between species, suggesting that different stresses target distinct regulatory components involved in the promotion of asexual or sexual reproduction in each species. To explore this possibility, it will be necessary to elucidate the systems regulating the stress-dependent promotion of asexual reproduction and the release of monospores in the Bangiales.
We demonstrated that wounding also promoted the gametogenesis in thalli to produce conchocelis filaments in P. yezoensis (Figure 1). This process involved the conversion of vegetative cells in the thalli to male and female gametangia (Takahashi and Mikami, 2017), meaning that gametogenesis usually results in the retardation of growth because of the reduction in vegetative cell numbers. Wounding therefore promotes the sexual life cycle using a growth trade-off in P. yezoensis. It is still unknown whether wounding promotes gametogenesis directly or if it causes growth retardation that in turn triggers gametogenesis. Little is known about regulatory mechanisms of gametogenesis in thalli; therefore, the elucidation of the wound perception and intracellular signaling pathways could help us to understand the regulation of gametogenesis and the environmental stress-promoted life cycle trade-offs in P. yezoensis.
Our results clearly indicated positive effects of dark pretreatment on the promotion of sexual and asexual propagation by wounding (Figure 3 and Supplementary Figures S3, S4), experimentally confirming our preliminary observation of the effect of the refrigerated delivery service. Certain physiological changes may therefore be induced within 3 days in response to darkness, enhancing the sensitivity of the thalli to wounding. However, little is currently known about the factors responsible for the effect of darkness in P. yezoensis. As shown in Figure 3, the effects of wounding and darkness were almost additive but not synergistic, suggesting that these stresses make different contributions to the reproductive strategy. In terrestrial plants, darkness induces the production of ROS (Rosenvasser et al., 2006; Zhang et al., 2016), meaning that a dark-induced oxidizing status in the P. yezoensis cells might enhance spore release. Oxidative stress was previously shown to promote the production and release of monospores in P. yezoensis (Takahashi and Mikami, 2017); therefore, future studies should address whether P. yezoensis produces ROS in the dark and whether any dark-induced ROS production enhances gametogenesis and monospore release.
Callus production was observed in thalli under heat stress conditions (Figures 4, 5). Normal leafy gametophytic thalli were produced from these calli, which comprised three-dimensional tissues with aggregations of proliferating cells (Figure 4); therefore, callus formation seems to be an asexual strategy to maintain the gametophyte generation. In terrestrial plants, wounding promotes callus formation, and the regeneration of tissues from calli depending on the actions of plant hormones such as auxin and cytokinin (Ikeuchi et al., 2013, 2017). We previously demonstrated the presence of plant hormones such as auxin and cytokinin in P. yezoensis (Mikami et al., 2016; Mori et al., 2017), although the physiological functions of these hormones are yet to be resolved. Future studies should therefore explore whether the promotion of calli formation by heat stress and the subsequent regeneration of the thalli is regulated by hormones in P. yezoensis.
The increase in culture temperature from 15 to 25°C stimulated the division of nonorganized cells but did not alter the identity of the life cycle generation, suggesting that heat stress can disrupt the rigidly determined direction of cell division required for the maintenance of the two-dimensional leafy thallus shape. Thus, the promotion of callus formation by heat stress could be a useful experimental system for the elucidation of unresolved important issues, such as why and how the gametophytic thalli of the Bangiales maintain a two-dimensional structure using an invariable direction of cell division. The exploration of the formation of calli and regeneration from them will therefore provide new insights into fundamental biological aspects, such as how wounding enables abnormal cell proliferation and how polarity-dependent cell division is regulated in the thalli of P. yezoensis.
The gametophytic thalli of P. yezoensis employ multiple life history strategies to cope with the negative impacts of environmental stresses by accelerating asexual and sexual reproductive responses using life cycle trade-offs. One such strategy is the promotion of changes in the life cycle strategy from vegetative growth to monospore release and gametogenesis, while the other is the production of an aberrantly growing callus as an abnormal asexual propagation mechanism. The elucidation of the regulatory mechanisms by which stresses promote the various reproductive responses in P. yezoensis could therefore enhance our understanding of the significance of flexible changes in life cycle strategies that enable the Bangiales to respond to and survive stressful conditions by producing asexual and sexual progenies.
The datasets generated for this study are available on request to the corresponding author.
KM conceptualized and designed the study, made the figures, and wrote the manuscript. MS performed the experiments, collected and analyzed the data, and made the figures.
The authors declare that the research was conducted in the absence of any commercial or financial relationships that could be construed as a potential conflict of interest.
We are grateful to the Marine Resources Research Center of the Aichi Fisheries Research Institute for kindly providing the gametophytic thalli of P. yezoensis strain U51.
The Supplementary Material for this article can be found online at: https://www.frontiersin.org/articles/10.3389/fmars.2020.00394/full#supplementary-material
FIGURE S1 | The majority of spores discharged following wounding were monospores. Explants were excised from the tips, middles (0.8 cm from the tip), and bottoms (1.6 cm from the tip) of thalli and incubated for 7 days. Discharged spores were counted daily. The pink and green bars correspond to the numbers of monospores and nongerminated spores, respectively. Error bars indicate the standard deviation of data from triplicate experiments (n = 3).
FIGURE S2 | Growth stage dependency of sensitivity to wounding in thalli. Explants were excised from thalli 0.7, 1.7, 2.4, or 3.2 cm in length and incubated for 7 days. Discharged spores were counted daily. Error bars indicate the standard deviation of data from triplicate experiments (n = 3). Different letters indicate significant differences at p < 0.05.
FIGURE S3 | Dark-enhanced discharge of monospores and carpospores. The thalli were pretreated at 4°C or in darkness for 3 days. Discharged spores were counted daily. Pink and green bars correspond to the numbers of monospores and nongerminated spores, respectively. Error bars indicate the standard deviation of data from triplicate experiments (n = 3).
FIGURE S4 | Effect of darkness on spore discharge in a wounding-independent manner. The number of spores released from nonwounded whole thalli following a 3-day pretreatment in darkness or light at 15°C. Discharged spores were counted daily. Error bars indicate the standard deviation of data from triplicate experiments (n = 3). Different letters indicate significant differences at p < 0.05.
Ackland, J. C., West, J. A., and Pickett-Heaps, J. (2007). Actin and myosin regulate pseudopodia of Porphyra pulchella (Rhodophyta) archeospores. J. Phycol. 43, 129–138.
Adams, E., Mikami, K., and Shin, R. (2018). Selection and functional analysis of a Pyropia yezoensis ammonium transporter PyAMT1 in potassium deficiency. J. Appl. Phycol. 29, 2617–2626.
Avila, M., Santelices, B., and McLachlan, J. (1986). Photoperiod and temperature regulation of the life history of Porphyra columbina (Rhodophyta, Bangiales) from central Chile. Can. J. Bot. 64, 1867–1872.
Baxter, A., Mittler, R., and Suzuki, N. (2014). ROS as key players in plant stress signalling. J. Exp. Bot. 65, 1229–1240. doi: 10.1093/jxb/ert375
Beneloujaephajri, E., Costa, A., L’Haridon, F., Métraux, J. P., and Binda, M. (2013). Production of reactive oxygen species and wound-induced resistance in Arabidopsis thaliana against Botrytis cinerea are preceded and depend on a burst of calcium. BMC Plant Biol. 13:160. doi: 10.1186/1471-2229-13-160
Blouin, N. A., Brodie, J. A., Grossman, A. C., Xu, P., and Brawley, S. H. (2011). Porphyra: a marine crop shaped by stress. Trends Plant Sci. 16, 29–37. doi: 10.1016/j.tplants.2010.10.004
Brawley, S. H., and Johnson, L. E. (1992). Gametogenesis, gametes and zygotes: an ecological perspective on sexual reproduction in the algae. Br. Phycol. J. 27, 233–252. doi: 10.1080/00071619200650241
Gao, S., Chen, X., Yi, Q., Wang, G., Pan, G., Lin, A., et al. (2010). A strategy for the proliferation of Ulva prolifera, main causative species of green tides, with formation of sporangia by fragmentation. PLoS One 5:e8571. doi: 10.1371/journal.pone.0008571
Hafting, J. T. (1999). A novel technique for propagation of Porphyra yezoensis Ueda blades in suspension cultures via monospores. J. Appl. Phycol. 11, 361–367.
Ikeuchi, M., Iwase, A., Rymen, B., Lambolez, A., Kojima, M., Takebayashi, Y., et al. (2017). Wounding triggers callus formation via dynamic hormonal and transcriptional changes. Plant Physiol. 175, 1158–1174. doi: 10.1104/pp.17.01035
Ikeuchi, M., Sugimoto, K., and Iwase, A. (2013). Plant callus: mechanisms of induction and repression. Plant Cell 25, 3159–3173. doi: 10.1105/tpc.113.116053
Kakinuma, M., Izumi Kaneko, I., Coury, D. A., Suzuki, T., and Amano, H. (2006). Isolation and identification of gametogenesis-related genes in Porphyra yezoensis (Rhodophyta) using subtracted cDNA libraries. J. Appl. Phycol. 18, 489–496.
Karasov, T. L., Chae, E., Herman, J. J., and Bergelson, J. (2017). Mechanisms to mitigate the trade-off between growth and defense. Plant Cell 29, 666–680. doi: 10.1105/tpc.16.00931
Katsaros, C., Weiss, A., Llangos, I., Theodorou, I., and Wichard, T. (2017). Cell structure and microtubule organisation during gametogenesis of Ulva mutabilis Føyn (Chlorophyta). Bot. Mar. 60, 123–135.
León, J., Rojo, E., and Sánchez-Serrano, J. J. (2001). Wound signalling in plants. J. Exp. Bot. 52, 1–9.
Li, C., Ariga, I., and Mikami, K. (2019). Difference in nitrogen starvation-inducible expression patterns among phylogenetically diverse ammonium transporter genes in the red seaweed Pyropia yezoensis. Am. J. Plant Sci. 10, 1325–1349.
Li, L., Saga, N., and Mikami, K. (2008). Phosphatidylinositol 3-kinase activity and asymmetrical accumulation of F-actin are necessary for establishment of cell polarity in the early development of monospores from the marine red alga Porphyra yezoensis. J. Exp. Bot. 59, 3575–3586. doi: 10.1093/jxb/ern207
Li, L., Saga, N., and Mikami, K. (2009). Ca2+ influx and phosphoinositide signalling are essential for the establishment and maintenance of cell polarity in monospores from the red alga Porphyra yezoensis. J. Exp. Bot. 60, 3477–3489. doi: 10.1093/jxb/erp183
Liu, X., Bogaert, K., Engelen, A. H., Leliaert, F., Roleda, M. Y., and De Clerck, O. (2017). Seaweed reproductive biology: environmental and genetic controls. Bot. Mar. 60, 89–108.
Mcdowell, R., Amsler, C. D., Dickinson, D. A., McClintock, J. B., and Baker, B. J. (2014a). Reactive oxygen species and the Antarctic macroalgal wound response. J. Phycol. 50, 71–80. doi: 10.1111/jpy.12127
Mcdowell, R., Amsler, C. D., McClintock, J. B., and Baker, B. J. (2014b). Reactive oxygen species as a marine grazing defense: H2O2 and wounded Ascoseira mirabilis both inhibit feeding by an amphipod grazer. J. Exp. Mar. Biol. Ecol. 458, 34–38.
Mikami, K. (2019). Diploid apogamy in red algal species of the genus Pyropia. J. Aquat. Res. Mar. Sci. 2019, 206–208.
Mikami, K., and Kishimoto, I. (2018). Temperature promoting the asexual life cycle program in Bangia fuscopurpurea (Bangiales, Rhodophyta) from Esashi in the Hokkaido Island, Japan. Algal Resour. 11, 25–32.
Mikami, K., Li, C., Irie, R., and Hama, Y. (2019). A unique life cycle transition in the red seawed Pyropia yezoensis depends on apospory. Commun. Biol. 2:229. doi: 10.1038/s42003-019-0549-545
Mikami, K., Li, L., and Takahashi, M. (2012). “Monospore-based asexual life cycle in Porphyra yezoensis,” in Porphyra yezoensis: Frontiers in Physiological and Molecular Biological Research, ed. K. Mikami (New York, NY: Nova Science Publishers), 15–37.
Mikami, K., Mori, I. C., Matsuura, T., Ikeda, Y., Kojima, M., Sakakibara, H., et al. (2016). Comprehensive quantification and genome survey reveal the presence of novel phytohormone action modes in red seaweeds. J. Appl. Phycol. 28, 2539–2548.
Mohring, M. B., Kendrick, G. A., Wernberg, T., Rule, M. J., and Vanderklift, M. A. (2013). Environmental influences on kelp performance across the reproductive period: an ecological trade-off between gametophyte survival and growth? PLoS One 8:e65310. doi: 10.1371/journal.pone.0065310
Monotilla, W. D., and Notoya, M. (2004). Morphological and physiological responses of Porphyra suborbicular a Kjellman (Bangiales, Rhodophyta) blades from five locations. Bot. Mar. 47, 323–334.
Monotilla, W. D., and Notoya, M. (2010). Growth and development of Porphyra marcosii (Bangiales, Rhodophyta) under different temperature and photoperiod. Philippine J. Sci. 139, 197–206.
Mori, I. C., Ikeda, Y., Matsuura, T., Hirayama, T., and Mikami, K. (2017). Phytohormones in red seaweeds: a technical review of methods for analysis and a consideration of genomic data. Bot. Mar. 60, 153–170.
Notoya, M., and Iijima, N. (2003). Life history and sexuality of archeospore and apogamy of Bangia atropurpurea (Roth) Lyngbye (Bangiales, Rhodophyta) from Fukaura and Enoshima, Japan. Fish. Sci. 69, 799–805.
Notoya, M., and Miyashita, A. (1999). Life history, in culture, of the obligate epiphyte Porphyra moriensis (Bangiales, Rhodophyta). Hydrobiology 398, 121–125.
Notoya, M., and Nagaura, K. (1998). Life history and growth of the epiphytic thallus of Porphyra lacerate (Bangiales, Rhodophyta) in culture. Algae 13, 207–211.
Rosenvasser, S., Mayak, S., and Friedman, H. (2006). Increase in reactive oxygen species (ROS) and in senescence-associated gene transcript (SAG) levels during dark-induced senescence of Pelargonium cuttings, and the effect of gibberellic acid. Plant Sci. 170, 873–879.
Shaar-Moshe, L., Hayouka, R., Roessner, U., and Peleg, Z. (2019). Phenotypic and metabolic plasticity shapes life-history strategies under combinations of abiotic stresses. Plant Direct 3, 1–13. doi: 10.1002/pld3.113
Sidirelli-Wolf, M. (1992). The influence of temperature, irradiation, and photoperiod on the reproductive life history of Porphyra leucosticte (Bangiales, Rhodophyta) in laboratory culture. Bot. Mar. 35, 251–257.
Stratmann, J., Paputsoglu, G., and Oertel, W. (1996). Differentiation of Ulva mutabilis (Chlorophyta) gametangia and gamete release are controlled by extracellular inhibitors. J. Phycol. 32, 1009–1021.
Takahashi, M., and Mikami, K. (2017). Oxidative stress promotes asexual reproduction and apogamy in the red seaweed Pyropia yezoensis. Front. Plant Sci. 8:62. doi: 10.3389/fpls.2017.00062
Takahashi, M., Saga, N., and Mikami, K. (2010). Photosynthesis-dependent extracellular Ca2+ influx triggers an asexual reproductive cycle in the marine red macroalga Porphyra yezoensis. Am. J. Plant Sci. 1, 1–11.
Vesty, E. F., Kessler, R. W., Wichard, T., and Coates, J. C. (2015). Regulation of gametogenesis and zoosporogenesis in Ulva linza (Chlorophyta): comparison with Ulva mutabilis and potential for laboratory culture. Front. Plant Sci. 6:15. doi: 10.3389/fpls.2015.00015
Wang, W. J., Zhuc, J.-Y., Xuc, P., Xuc, J. R., Line, Z.-Z., Huange, C.-K., et al. (2008). Characterization of the life history of Bangia fuscopurpurea (Bangiaceae, Rhodophyta) in connection with its cultivation in China. Aquaculture 278, 101–109.
Wichard, T., and Oertel, W. (2010). Gametogenesis and gamete release of Ulva mutabilis and Ulva lactuca (chlorophyta): regulatory effects and chemical characterization of the “swarming inhibitor”. J. Phycol. 46, 2248–2259.
Ying, L. S. (1984). “The ecological characteristics of monospores of Porphyra yezoensis Ueda and their use in cultivation,” in Proceedings of the Eleventh International Seaweed Symposium, Developments in Hydrobiology, eds C. J. Bird, and M. A. Ragan (Dordrecht: Springer).
Zhang, J., Li, H., Xu, B., Li, J., and Huang, B. (2016). Exogenous melatonin suppresses dark-induced leaf senescence by activating the superoxide dismutase-catalase antioxidant pathway and down-regulating chlorophyll degradation in excised leaves of perennial ryegrass (Lolium perenne L.). Front. Plant Sci. 7:1500. doi: 10.3389/fpls.2016.01500
Keywords: callus, heat stress, life cycle, Pyropia yezoensis, reproduction, wounding
Citation: Suda M and Mikami K (2020) Reproductive Responses to Wounding and Heat Stress in Gametophytic Thalli of the Red Alga Pyropia yezoensis. Front. Mar. Sci. 7:394. doi: 10.3389/fmars.2020.00394
Received: 20 August 2019; Accepted: 07 May 2020;
Published: 28 May 2020.
Edited by:
Ester A. Serrao, University of Algarve, PortugalReviewed by:
Michael L. Fine, Virginia Commonwealth University, United StatesCopyright © 2020 Suda and Mikami. This is an open-access article distributed under the terms of the Creative Commons Attribution License (CC BY). The use, distribution or reproduction in other forums is permitted, provided the original author(s) and the copyright owner(s) are credited and that the original publication in this journal is cited, in accordance with accepted academic practice. No use, distribution or reproduction is permitted which does not comply with these terms.
*Correspondence: Koji Mikami, a29taWthbWlAZmlzaC5ob2t1ZGFpLmFjLmpw
Disclaimer: All claims expressed in this article are solely those of the authors and do not necessarily represent those of their affiliated organizations, or those of the publisher, the editors and the reviewers. Any product that may be evaluated in this article or claim that may be made by its manufacturer is not guaranteed or endorsed by the publisher.
Research integrity at Frontiers
Learn more about the work of our research integrity team to safeguard the quality of each article we publish.