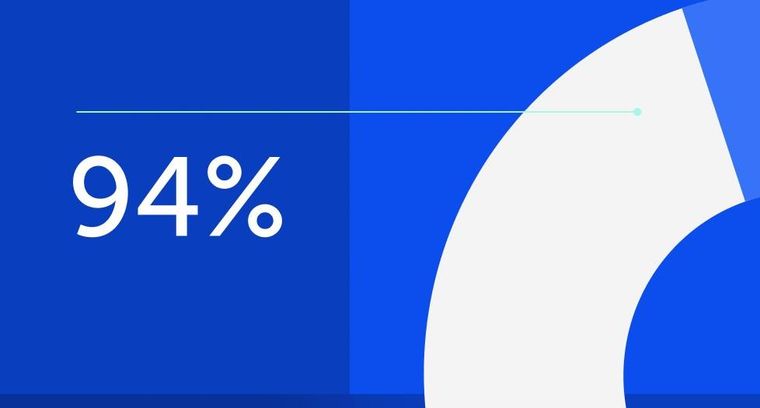
94% of researchers rate our articles as excellent or good
Learn more about the work of our research integrity team to safeguard the quality of each article we publish.
Find out more
MINI REVIEW article
Front. Mar. Sci., 21 May 2020
Sec. Marine Megafauna
Volume 7 - 2020 | https://doi.org/10.3389/fmars.2020.00333
This article is part of the Research TopicPathologic Findings in Stranded Marine Mammals: A Global PerspectiveView all 29 articles
Variably buoyant, dead Cetacea may float, or sink and later bloat to refloat if ambient temperature and pressure allow sufficient decomposition gas formation and expansion. Mortality can result from acute or chronic disease, fishery entanglement, vessel collision, noxious noises, or toxicant spills. Investigators often face the daunting task of elucidating a complex series of events, in reverse order, from when and where an animal is found, and to diagnose the cause of death. Various scenarios are possible: an animal could die at sea remaining there or floating ashore, or strand on a beach alive, where it dies and, if cast high enough, remain beached to be scavenged or decompose. An animal that rests low on a beach may refloat again, through increased buoyancy from decomposition gas and favorable tides, currents, and wind. Here we review the factors responsible for the different outcomes, and how to recognize the provenance of a cetacean mortality found beached, or floating at sea. In conclusion, only some carcasses strand, or remain floating. Negatively buoyant animals that die at depth, or on the surface, and sink, may never surface, even after decomposition gas accumulation, as in cold, deep waters gas may fail to adequately reduce the density of a carcass, precluding it from returning to the surface.
Until scavenging, decomposition and the mechanical action of surface waves render its negatively buoyant parts free to sink, positively buoyant carcasses remain afloat (Reisdorf et al., 2012). A sunken whole or partial carcass will either: decay on the bottom, aided by internal and sediment micro-organisms and other scavengers, or bloat sufficient to refloat, and continue to roam, or wash ashore, decomposing and perhaps being scavenged with time or break up on the beach and percolate into the substrate, or the remains will either float or sink again if still at sea (Reisdorf et al., 2012). The sometimes extended and complex spatial and temporal meanderings of dead animals and their disassociating parts through time, can make it difficult to link potentially injurious events with morbidity or mortality. Furthermore, animals that can be closely examined may only be a small subset of actual mortality – floating animals being more likely to be observed than those that sink, and animals on the beach being more likely to be closely examined than those that remain at sea. For instance, sonar has been linked to atypical mass stranding of beaked whales (Frantzis, 1998; Jepson et al., 2003; Fernandez et al., 2005). Concern has been raised that many more cetaceans are fatally impacted than observed because they sink at the time of death (Parsons et al., 2008). Likewise it has been suggested that strandings can seriously underestimate mortality during an unusual mortality event (Williams et al., 2011). Even where a dolphin population was well-studied within a series of relatively enclosed embayments, carcass recovery only included one-third of known mortalities, as calibrated by a long-standing photo-ID catalog (Wells et al., 2015).
While study of whale carcasses that have been deposited naturally or anchored by humans on the ocean floor has become a major discipline (Smith et al., 2015), there has been less study of carcasses before they reach their final resting place.
An analysis of beached marine mammals (Bernaldo de Quirós et al., 2018) in the Cape Cod, United States region show only 1% (2/173) of stranded small cetaceans were dead on the beach after drowning in fishing gear and floating ashore (Bogomolni et al., 2010). In contrast, 61% (253/415) of cetaceans on the beaches of SW England, United Kingdom were determined to have died due to bycatch in fishing gear (Leeney et al., 2008). Thus, the prevailing SW United Kingdom winds blowing over fishing grounds, enable bycaught carcasses to more readily float ashore: in contrast the offshore (SW in summer and NW in winter) prevailing winds around Cape Cod drive carcasses out to sea. Wind direction also determined carcass behavior for bird carcasses in the North Sea (Bibby, 1981). A study of turtle carcasses on the North Carolina coast concluded that beach strandings can be a poor indicator of at sea mortalities (Epperly et al., 1996), where strong offshore bottom currents were thought to move carcasses away from the beach. Seasonal marine turtle strandings are predictable from physical oceanography and drift bottle experiments (Hart et al., 2006). Efforts to track turtle mortalities using satellite tracked animals have been controversial (Hays et al., 2004).
Data on the proportion of marine mammals that float versus sink at sea are not very common, however, 18% of dead bycaught common dolphins were estimated to be buoyant on release: six per cent of them beached (Peltier et al., 2012; Peltier et al., 2016). Modeling their drift and stranding increased fishery bycatch estimates ten-fold compared to fishery observer estimates (Peltier et al., 2016). Furthermore, recent hind-cast drift models were able to potentially identify the fisheries responsible for the bycatch (Peltier et al., 2020). Historically, whalers reported that certain species of whales float after death (right and sperm whales) while most others sink. Right whales have also been shown to normally need to actively swim down until lung collapse depth, where they can then glide to deeper depths (Nowacek et al., 2001). Blue and fin whales in particular were reported to sink early in the whaling season and float at the end (Slijper and Pomerans, 1962). Generally, buoyancy is considered to be a function of the animal’s body condition and fat content, especially of bone, and blubber and lung inflation. These parameters vary between and within species. In one study, the weight of an immersed dolphin was measured shortly after death (Woodcock and McBride, 1951). According to calculations and measurements the animal would either be buoyed up by roughly 12 lbs or dragged down by slightly over 9 lbs of weight depending on whether its lungs were empty or full. Captive dolphins can rest motionless on the bottom of pools or float inactive at the surface by lung-controlled buoyancy. It is also unclear if dolphins and small whales undergo a respiratory expiration at death or not, given their upper respiratory tract is closed in the relaxed state.
In vivo generated decompression gas bubbles observed in freshly asphyxiated dolphins and seals (Moore et al., 2009) could affect carcass buoyancy, along with post mortem decomposition gas, which is seen first in the abdomen (Bernaldo de Quirós et al., 2013). Rorqual whales with their expandable throat pleats may grossly distend and float belly-up until structural integrity is lost due to mechanical action of wind and waves, degradation by sunlight and scavenger damage. Once a carcass deflates it may sink, or if enough buoyancy remains in fatty tissues, they may float at or near the surface while denser tissues such as bones are shed.
A study of whale carcass temperature showed an increase from 37–38 to 41°C after 24 h, reaching 48°C after two to 3 days with heat and autolysis splitting neutral fatty acids in the blubber causing it to darken and produce an unpleasant smell (Tonnessen and Johnsen, 1982). As temperature rises, the cells of the blubber rupture, and oil leaks from the tissue. This reduces its oil content, and acts to reduce the buoyancy and insulating capacity of the remaining blubber (Tonnessen and Johnsen, 1982). Given allometric scaling and surface area to volume ratio this effect would seem to be more pronounced in small animals with proportionally larger blubber layers per body size, than larger animals that would have more fat content in bone (Smith et al., 2015).
In a mass mortality of 14 humpback whales that died during a 5-week period (Geraci et al., 1989), all but one whale (that was towed immediately after death) sunk after death and were re-floated by gas produced during decomposition. The majority of these carcasses (9 of 14) were recovered from the relatively shallow and restricted confines of Cape Cod Bay, MA United States.
Observations of whale carcasses in very deep water (∼2000 m) indicate that at extreme depths decomposition is slow and carcasses may remain stable for many years (or decades; Allison et al., 1991). In one study a skeletonized whale carcass in 400 m of water indicated that it had remained undisturbed for as long as 30 years. The belly-up position of the carcass seemed to indicate that at one time the carcass had been buoyed up by gas in the body cavity that likely originated from naturally occurring gut flora. Figure 1 summarizes the potential fates and factors impinging on dead whale carcasses.
Figure 1. Schematic summary of the fates of whale carcasses, and the wind, tide, current, temperature, and pressure impinging on them. Depending on carcass buoyancy and location at death, it will either float or sink. If it floats, it will bloat, drift ashore if at sea, or eventually decompose at sea to where the negatively buoyant components sink to the ocean floor. If it is negatively buoyant at death it will sink. If it sinks in shallow water, decomposition gas may expand sufficiently to bloat, and refloat the carcass. If the depth is too great and the water too cold, then the gas volume may not be sufficient to make it buoyant and the carcass will decompose and be scavenged in situ on the bottom. Image: Natalie Renier, Woods Hole Oceanographic Institution.
The depth at which hydrostatic pressure outweighs the ability of decomposition to produce a volume of gas sufficient to refloat a carcass was estimated to be around 1000 m (Allison et al., 1991). Recent observations of both naturally discovered and experimentally planted large and small whale carcasses (Smith et al., 2015) have revised that estimate to 100 m (Reisdorf et al., 2012).
While decomposition may originate with bacterial fermentation of gut bacteria, methanogenisis may also contribute or dominate – depending on temperature or pressure (Allison et al., 1991). These processes produce gas at different rates; therefore, the potential of a carcass to re-float may depend on what process dominates. Gut microbes are best adapted to, and will grow best at warm temperatures with growth slowing at cooler temperatures and halting altogether below15°C. At lower temperatures other decomposition pathways predominate. Therefore, gas production depends on temperature and type of bacterial decomposition activity taking place.
Increasing pressure also increases the solubility of gas, such that as pressure increases more gas will dissolve into surrounding tissue – and not produce gas bubbles that would create buoyancy. Thus, the buoyancy of a carcass at depth will depend on abiotic (temperature, depth or pressure, and structural integrity of the carcass) as well as biotic (bacterial type and activity) factors. An experimental approach is needed to better model the process (Allison et al., 1991).
One location of interest in terms of the potential impact of acoustic stressors is the Tongue of the Ocean, Andros, Bahamas, an area of beaked whale habitat, where defense organizations deploy sonar routinely. The maximum depth ranges from 1300 to 1800 m in this basin. Thus, if carcasses were to sink it is very likely that they will not refloat. Other locations of interest where defense training overlaps sensitive and unique ecosystems and deep waters are the Southern California Bight and offshore waters near the Hawaii main islands.
Tools such as chemical analysis used for determination of time of death in terrestrial animals are of remarkably little value in dead cetaceans (Madea, 2005), as is degree of insect invasion which is of real use in terrestrial carcass evaluation (Gennard, 2007). Similarly in addition to degree of disarticulation, observation of invasion by crustaceans, including isopods, and bacteria may be the best measures in floating and sunken marine mammal carcasses and rates of scavenger activity may vary by location with higher rates of scavenging in areas of frequent carcass deposition (Kemp et al., 2006). Studies of deposited carcasses have shed some light on this process (Kemp et al., 2006; Anderson and Bell, 2014).
The relative lack of food at the deep-sea floor means that the scavenging rate on carcasses can be much higher than in shallow marine habitats, whereas physical, chemical, and microbial decomposition are significantly more important than scavenging in the shallow-water (Reisdorf et al., 2012). Estimation of time since death is also poorly understood for beached marine mammal carcasses, where intestinal putrefaction can lead to inflation and detonation (Reisdorf et al., 2012).
Schafer (Schafer, 1972) presents some general observations of decomposing carcasses at sea and on shore. However, there are no detailed descriptions of the process or order of disarticulation (Allison et al., 1991) on which one could base a reasonable reconstruction of time since death.
Estimation of time since death of a beached whale carcass can be misled by surf maceration. North Atlantic right whale 3107 (Moore et al., 2004) floated ashore with the majority of the body volume still present. Overnight it rolled 10 miles down a beach in heavy surf. After which the majority of the soft tissues and bones had been macerated and extruded via the oral cavity from within the blubber coat. The skin was still attached. Thus, deflated whale carcasses with skin attached should not be assumed to have died many days prior, in that they can deflate very quickly in surf.
While developing a hyperbaric chamber compatible with computer tomography (Moore et al., 2011), initial cadaver trials were undertaken without the carcass fixed to the inside of the chamber (Figure 2). A dolphin was artificially given a measured lung inflation post mortem, with a tube and valve system tied in to the trachea, so it had a fixed volume of lung air, at surface pressure. As the pressure was increased in the chamber, the lungs collapsed and the buoyancy became negative. This was seen by the position of the carcass in the chamber dropping as the pressure increased. Thus, one could envisage an animal dying at greater depth that was negatively buoyant whereas the same animal, with the same surface inspiration volume, that died at the surface could float. What we don’t know is if animals that die near the surface actually retain air in their lungs.
Figure 2. CT sections through head (left) and chest (right) of a common dolphin in a pressure chamber (Moore et al., 2011). Note lung is compressed at higher pressure (shrinking dark areas on right series), whereas trachea remains the same size (dark circle on left series). Note also loss of buoyancy with increasing pressure, and shunting of air into one lung, resulting in the carcass rotating such that the more inflated lung is uppermost, having made that side of the body more buoyant. Chamber pressure for each pair of images is shown in kiloPascals (kPa).
Another feature reported in the hyperbaric chamber CT experiment (Moore et al., 2011) was that the orientation of the animal being on its side, with the upper lung more expanded than the lower lung, suggested that in a dead but not decomposed cadaver at least, the air will follow the small but real pressure difference across the width of the animal in the vertical axis, seeking the highest point (Figure 1). Thus, not surprisingly many fresh carcasses are observed floating on their side, and very rarely belly down. Later as intestinal gas accumulates they tend to then roll onto their backs. With only a small sample size it is speculative to suggest this asymmetrical lung compression is also found in live animals. But if so, while diving deeper, as lung compression increases, when the body is more horizontal than vertical, they may need to use flipper and fluke surfaces to counter the tendency to passively roll and adopt a one side down orientation due to unequal buoyancy.
Given all of the above, Cetacea most likely to float or refloat and be cast ashore after death are a relatively small subset of animals that die at sea (Moore et al., 2011). Animals that are most likely to float would tend to be large, robust, positively buoyant and either die or refloat near shore. The least likely animals to be discovered after death would be sick or naturally lean animals sinking over water deeper than 100 meters. This is the vast majority of offshore odontocetes.
• Considering the above observations, the hypothesis seems reasonable that a whale that dies during a dive may well not come to the surface immediately, nor after a period of time, if sunk in deep, and cold water. Similarly, for many species, dying at the surface could also result in sinking and not refloating if over deep, cold water. In light of this the following would seem to be useful next steps:
• Gather more data on the sink or float behavior of marine mammals discarded from fishing vessels after removal from fishing gear. Documenting their condition with increasing time after death.
• Retrospectively analyze data from large whale necropsy cases that were found floating at sea, for information about how carcass condition changed with time, and prospectively document changes through time of marine mammal carcasses floating at sea and beached.
MM drafted the manuscript. GM, TR, and GE contributed perspectives and assisted with manuscript review.
The authors declare that the research was conducted in the absence of any commercial or financial relationships that could be construed as a potential conflict of interest.
Allison, P. A., Smith, C., Kukert, H., Deming, J., and Bennett, J. (1991). Deep-water taphonomy of vertebrate carcasses: a whale skeleton in the bathyal Santa Catalina Basin. Paleobiology 17, 78–89.
Anderson, G. S., and Bell, L. S. (2014). Deep coastal marine taphonomy: investigation into carcass decomposition in the saanich inlet, British Columbia Using a Baited Camera. PLoS One 9:e110710. doi: 10.1371/journal.pone.0110710
Bernaldo de Quirós, Y., Hartwick, M., Rotstein, D. S., Garner, M. M., and Bogomolni, A. (2018). Discrimination between bycatch and other causes of cetacean and pinniped stranding. Dis. Aquat. Org. 127, 83–95. doi: 10.3354/dao03189
Bernaldo de Quirós, Y., Seewald, J. S., Sylva, S. P., Greer, B., Niemeyer, M., Bogomolni, A. L., et al. (2013). Compositional discrimination of decompression and decomposition gas bubbles in bycaught seals and dolphins. PLoS One 8:e83994. doi: 10.1371/journal.pone.0083994
Bibby, C. J. (1981). An experiment on the recovery of dead birds from the North Sea. Ornis Scand. 12, 261–265.
Bogomolni, A. L., Pugliares, K. R., Patchett, K., Herzig, S. M., Harry, C. T., LaRocque, J. M., et al. (2010). Mortality trends of stranded marine mammals on Cape Cod and Southeastern Massachusetts between 2000-2006. Dis. Aquat. Org. 88, 143–155. doi: 10.3354/dao02146
Epperly, S., Braun, J., Chester, A., Cross, F., Merriner, J., Tester, P., et al. (1996). Beach strandings as an indicator of at-sea mortality of turtles. Bull. Mar. Sci. 59, 289–297. doi: 10.1371/journal.pone.0056776
Fernandez, A., Edwards, J. F., Rodriguez, F., Espinosa De Los Monteros, A., and Herraez, P. (2005). Gas and fat embolic syndrome involving a mass stranding of beaked whales (Family Ziphiidae) exposed to anthropogenic sonar signals. Vet. Pathol. 42, 446–457. doi: 10.1354/vp.42-4-446
Geraci, J. R., Anderson, D. M., Timperi, R. J., St-Aubin, D. J., Early, G. A., Prescott, J. H., et al. (1989). Humpback whales (Megaptera novaeangliae) fatally poisoned by dinoflagellate toxin. Can. J. Fish. Aquat. Sci. 46, 1895–1898.
Hart, K. M., Mooreside, P., and Crowder, L. B. (2006). Interpreting the spatio-temporal patterns of sea turtle strandings: going with the flow. Biol. Conserv. 129, 283–290.
Hays, G. C., Broderick, A. C., Godley, B. J., Luschi, P., and Nichols, W. J. (2004). Tracking turtles to their death. Mar. Ecol. Prog. Ser. 283, 299–300.
Jepson, P. D., Arbelo, M., Deaville, R., Patterson, A. P. I, Castro, P., Baker, J. R., et al. (2003). Gas-bubble lesions in stranded cetaceans. Nature 425, 575–576. doi: 10.1038/425575a
Kemp, K. M., Jamieson, A. J., Bagley, P. M., McGrath, H., Bailey, D. M., Collins, M. A., et al. (2006). Consumption of large bathyal food fall, a six month study in the NE Atlantic. Mar. Ecol. Prog. Ser. 310, 65–76.
Leeney, R. H., Amies, R., Broderick, A., Witt, M. J., Loveridge, J., Doyle, J., et al. (2008). Spatio-temporal analysis of cetacean strandings and bycatch in a UK Fisheries hotspot. Biodivers. Conserv. 17, 2323–2338.
Madea, B. (2005). Is there recent progress in the estimation of the postmortem interval by means of thanatochemistry? Forensic Sci. Int. 151, 139–149. doi: 10.1016/j.forsciint.2005.01.013
Moore, M., Hammar, T., Arruda, J., Cramer, S., Dennison, S., Montie, E. W., et al. (2011). Hyperbaric computed tomographic measurement of lung compression in seals and dolphins. J. Exp. Biol. 214, 2390–2397. doi: 10.1242/jeb.055020
Moore, M., Knowlton, A., Kraus, S., McLellan, W., and Bonde, R. (2004). Morphometry, gross morphology and available histopathology in Northwest Atlantic right whale (Eubalaena glacialis) mortalities (1970 to 2002). J. Cetacean Res. Manag. 6, 199–214.
Moore, M. J., Bogomolni, A. L., Dennison, S. E., Early, G., Garner, M. M., Hayward, B. A., et al. (2009). Gas bubbles in seals, dolphins and porpoises entangled and drowned at depth in gill nets. Vet. Pathol. 46, 536–547. doi: 10.1354/vp.08-VP-0065-M-FL
Nowacek, D. P., Johnson, M. P., Tyack, P. L., Shorter, K., McLellan, W. A., and Pabst, D. A. (2001). Buoyant balaenids: the ups and downs of buoyancy in right whales. Proc. R. Soc. Lond. B 268, 1811–1816. doi: 10.1098/rspb.2001.1730
Parsons, E. C. M., Dolman, S. J., Wright, A. J., Rose, N. A., and Burns, W. (2008). Navy sonar and cetaceans: just how much does the gun need to smoke before we act? Mar. Pollut. Bull. 56, 1248–1257. doi: 10.1016/j.marpolbul.2008.04.025
Peltier, H., Authier, M., Deaville, R., Dabin, W., Jepson, P. D., van Canneyt, O., et al. (2016). Small cetacean bycatch as estimated from stranding schemes: the common dolphin case in the northeast Atlantic. Environ. Sci. Policy 63, 7–18.
Peltier, H., Authier, M., Willy, D., Cécile, D., Fabien, D., Ghislain, D., et al. (2020). Can modelling the drift of bycaught dolphin stranded carcasses help identify involved fisheries? An exploratory study. Glob. Ecol. Conserv. 21:e00843.
Peltier, H., Dabin, W., Daniel, P., Van Canneyt, O., Dorémus, G., Huon, M., et al. (2012). The significance of stranding data as indicators of cetacean populations at sea: modelling the drift of cetacean carcasses. Ecol. Indic. 18, 278–290. doi: 10.1371/journal.pone.0062180
Reisdorf, A., Bux, R., Wyler, D., Benecke, M., Klug, C., Maisch, M., et al. (2012). Float, explode or sink: postmortem fate of lung-breathing marine vertebrates. Palaeobiodivers. Palaeoenviron. 92, 67–81.
Schafer, W. (1972). Ecology and Paleoecology of Marine Environments. Chicago, IL: University of Chicago Press.
Smith, C. R., Glover, A. G., Treude, T., Higgs, N. D., and Amon, D. J. (2015). Whale-fall ecosystems: recent insights into ecology, paleoecology, and evolution. Annu. Rev. Mar. Sci. 7, 571–596. doi: 10.1146/annurev-marine-010213-135144
Wells, R. S., Allen, J. B., Lovewell, G., Gorzelany, J., Delynn, R. E., Fauquier, D. A., et al. (2015). Carcass-recovery rates for resident bottlenose dolphins in Sarasota Bay, Florida. Mar. Mamm. Sci. 31, 355–368.
Williams, R., Gero, S., Bejder, L., Calambokidis, J., Kraus, S. D., Lusseau, D., et al. (2011). Underestimating the damage: interpreting cetacean carcass recoveries in the context of the Deepwater Horizon/BP incident. Conserv. Lett. 4, 228–233.
Keywords: cadaver, beach, sink, float, fate, cetacean
Citation: Moore MJ, Mitchell GH, Rowles TK and Early G (2020) Dead Cetacean? Beach, Bloat, Float, Sink. Front. Mar. Sci. 7:333. doi: 10.3389/fmars.2020.00333
Received: 12 February 2020; Accepted: 22 April 2020;
Published: 21 May 2020.
Edited by:
Alvin Cyril Camus, University of Georgia, United StatesReviewed by:
Gail Schofield, Queen Mary University of London, United KingdomCopyright © 2020 Moore, Mitchell, Rowles and Early. This is an open-access article distributed under the terms of the Creative Commons Attribution License (CC BY). The use, distribution or reproduction in other forums is permitted, provided the original author(s) and the copyright owner(s) are credited and that the original publication in this journal is cited, in accordance with accepted academic practice. No use, distribution or reproduction is permitted which does not comply with these terms.
*Correspondence: Michael J. Moore, bW1vb3JlQHdob2kuZWR1
Disclaimer: All claims expressed in this article are solely those of the authors and do not necessarily represent those of their affiliated organizations, or those of the publisher, the editors and the reviewers. Any product that may be evaluated in this article or claim that may be made by its manufacturer is not guaranteed or endorsed by the publisher.
Research integrity at Frontiers
Learn more about the work of our research integrity team to safeguard the quality of each article we publish.