- 1The SeaDoc Society, UC Davis School of Veterinary Medicine, Karen C. Drayer Wildlife Health Center, Eastsound, WA, United States
- 2The Whale Museum, Friday Harbor, WA, United States
- 3Friday Harbor Laboratories, University of Washington, Friday Harbor, WA, United States
- 4Animal Health Centre, British Columbia Ministry of Agriculture, Abbotsford, BC, Canada
- 5Ecological Restoration Program, British Columbia Institute of Technology, Burnaby, BC, Canada
- 6Marine Mammal Investigations, Washington Department of Fish and Wildlife, Tacoma, WA, United States
The harbor seal (Phoca vitulina richardii) population in the Salish Sea has been at equilibrium since the mid-1990s. This stable population of marine mammals resides relatively close to shore near a large human population and offers a novel opportunity to evaluate whether disease acts in a density-dependent manner to limit population growth. We conducted a retrospective analysis of harbor seal stranding and necropsy findings in the San Juan Islands sub-population to assess age-related stranding trends and causes of mortality. Between January 01, 2002 and December 31, 2018, we detected 882 harbor seals that stranded and died in San Juan County and conducted necropsies on 244 of these animals to determine primary and contributing causes of death. Age-related seasonal patterns of stranded animals were evident, with pups found in the summer, weaned pups primarily recovered during fall, and adults and sub-adults recovered in summer and fall. Pups were the most vulnerable to mortality (64% of strandings). Pups predominantly died of nutritional causes (emaciation) (70%), whereas sub-adults and adults presented primarily with clinical signs and gross lesions of infectious disease (42%) and with non-anthropogenic trauma (27%). Primary causes of weaned pup mortality were distributed equally among nutritional, infectious, non-anthropogenic trauma, and anthropogenic trauma categories. Nutritional causes of mortality in pups were likely related to limitations in mid- and late-gestational maternal nutrition, post-partum mismothering, or maternal separation possibly related to human disturbance. Infectious causes were contributing factors in 33% of pups dying of nutritional causes (primarily emaciation–malnutrition syndrome), suggesting an interaction between poor nutritional condition and enhanced susceptibility to infectious diseases. Additional primary causes of harbor seal mortality were related to congenital disorders, predation, human interaction, and infections, including zoonotic and multidrug-resistant pathogens. Bottom-up nutritional limitations for pups, in part possibly related to human disturbance, as well as top-down predatory influences (likely under-represented through strandings) and infectious disease, are important regulators of population growth in this stable, recovered marine mammal population.
Introduction
Carrying capacity is a density-dependent phenomenon shaped by the interdependent relationships between consumers and the finite resources that control their population growth (del Monte-Luna et al., 2004). For abundant and stable populations, growth is limited by effects on reproduction and survivorship (Fowler, 1981). In birds and mammals, especially those with low reproductive rates and long lifespans, infection, starvation, and predation can act independently or synergistically in a density-dependent manner to limit growth in populations near carrying capacity (Leader-Williams, 1982; Sinclair, 1985; Sullivan, 1989; Goss-Custard et al., 2002; Benskin et al., 2009). Across vertebrate taxa, juveniles appear to be the age class most susceptible to density-dependent mortality for populations at carrying capacity (Guinness et al., 1978; Sullivan, 1989; Linnell et al., 1995). Although it is well accepted that disease is an important factor that can control wild population status and growth (e.g., May and Anderson, 1979; Deem et al., 2001), few studies have assessed cause-specific mortality in wild animal populations at carrying capacity.
Harbor seals (Phoca vitulina) are small phocids distributed along the temperate coastal regions of Asia, Europe, and North America. While infectious diseases are well documented in harbor seals, few reports detail causes of mortality in populations at carrying capacity. Even a major epizootic caused by an influenza virus (A/Seal/Mass/1/80 H7N7) that killed hundreds of seals along the New England coast (United States) in 1979 had a conspicuous local geographic effect but no apparent population-level impact (Geraci et al., 1982). In contrast, the 1988 and 2002 phocine distemper virus epizootics in Europe killed 23,000 and 30,000 harbor seals, respectively, with estimated mortality as high as 57–66% in some populations (Härkönen et al., 2006). In some locations, predation may regulate harbor seal populations. For example, a major decline in harbor seals in the western Gulf of Alaska has been attributed to increased predation by killer whales (Orcinus orca) (Springer et al., 2003), though this claim has been debated (DeMaster et al., 2006). On Sable Island, Nova Scotia (Canada), shark predation was a significant cause of mortality in harbor seal pups and adults and contributed to a decline in the productivity of this small, localized population (Lucas and Stobo, 2000).
Within the Salish Sea, a 16,925 km2 inland sea shared by Washington (United States) and British Columbia (Canada) (Gaydos et al., 2008), the harbor seal population (totaling around 50,000 individuals) has been at equilibrium for over two decades (Jeffries et al., 2003; DFO, 2010; Majewski and Ellis, 2019). Pre-exploitation harbor seal population size in the Salish Sea is unknown, but after the cessation of bounty programs in the early 1960s and the adoption of protective measures in the early 1970s, harbor seal numbers on both sides of the international border increased exponentially until reaching presumed carrying capacity in the mid-1990s. These populations have remained at equilibrium to date.
This stable population of marine mammals that resides relatively close to shore and near large human populations offers a novel opportunity to evaluate mortality in a population at carrying capacity to better understand how disease could limit population growth. We conducted a retrospective analysis of harbor seal stranding data and necropsy findings in the San Juan Islands sub-population to assess age-related stranding trends and causes of mortality. San Juan County, Washington is located in the center of the Salish Sea, is comprised entirely of islands, has nearly 150 haul-out sites used by harbor seals (Jeffries et al., 2000), and has one of the highest harbor seal densities within this inland sea (Jeffries et al., 2003).
Materials and Methods
Study Site and Sub-Population
San Juan County (SJC), Washington is comprised of more than 400 rocks and islands with elevations above mean high tide. The county’s land and ocean occupy 450 km2 and 1,160 km2, respectively1. Harbor seals found in SJC are part of Washington’s Inland Stock, specifically the Northern Inland Waters sub-stock (Huber et al., 2012). Aerial surveys were used to count harbor seals in SJC as previously described in Jeffries et al. (2003). Aerial surveys were flown 2 h before to 2 h after low tide during the pupping season when the maximum numbers of animals were hauled out. All known haul-out sites were surveyed, and potential new sites were examined on each survey. A standardized correction factor was applied to correct for the proportion of the population not ashore during the survey.
Carcass Collection and Necropsy
The SJC Marine Mammal Stranding Network is authorized by the United States National Oceanic and Atmospheric Administration, National Marine Fisheries Service to respond to stranded marine mammals in SJC (Permit #18786). Members of the public and field biologists typically report strandings, and network personnel recover carcasses. We analyzed harbor seal stranding and necropsy data from January 01, 2002 to December 31, 2018. Stranding response, necropsy effort, and necropsy procedures were consistent over the study period. Cases used in this study included dead stranded animals or animals that stranded live, were admitted to rehabilitation, and then died or were euthanized (n = 882). Data collected for all recovered carcasses included the date, time, and coordinates of the stranding, age class, sex, weight, axillary girth, straight body length, body condition, postmortem condition, and evidence of human interaction (Geraci and Lounsbury, 2005). Complete necropsies were performed for all seals found in suitable postmortem condition (carcass code 2 or 3; Geraci and Lounsbury, 2005) to determine cause of death and to document health and natural history findings. Briefly, external examinations were performed on fresh, chilled or frozen and thawed carcasses. While sample collection varied based on lesions noted grossly, a standard set of tissues were systematically collected and preserved in 10% neutral buffered formalin for microscopic examination. If present, these included, but were not limited to: adrenal gland, bladder, brain (cerebellum, cerebrum, and brain stem), diaphragm, eye, heart, large and small intestine, kidney, liver, lung, lymph nodes, skeletal muscle, skin, spleen, testicle, thymus, thyroid, tongue, trachea, umbilicus, and uterus. Samples also were placed fresh in sterile packs and frozen for ancillary testing. Aerobic bacteriology was routinely performed and virology if histopathology warranted. As previously described (Ashley et al., 2020), liver was collected from a subset of animals and tested for trace elements. Feces were collected for biotoxins screening as previously described (Lefebvre et al., 2016), macroscopic metazoan parasites were identified to genus or species, and age for some sub-adult and adult seals was determined by cementum analysis of canine teeth. Histopathology and laboratory testing were conducted at the British Columbia Ministry of Agriculture, Animal Health Center (Abbotsford, BC, Canada). Because all harbor seals were dead prior to inclusion in this study, an Institutional Animal Care and Use Committee protocol was not required by the UC Davis Office of Research for this work.
Age and Cause of Death Classifications
As previously described (Lambourn et al., 2013), harbor seals were classified as pups (<2 months), weaned pups (2–12 months), sub-adults (12–48 months), or adults (>48 months). Pups were considered weaned if they (1) had a standard length >90 cm; and/or (2) stranded after October 1 of their first year (Geraci and Lounsbury, 2005). Non-pups were considered adults if they (1) had a standard length >150 cm, (2) were greater than 4 years old based on cementum analysis of a canine tooth, or (3) were females that were pregnant or showed evidence of past reproduction.
Two authors (JG and SR) collaboratively assigned cause of death for each animal using initial stranding reports, gross photographs of lesions, necropsy notes, histopathology, and ancillary diagnostic results. For each animal, we determined the most likely proximate (inciting) cause of death, defined as the disease, disorder, or injury that initiated the animal’s decline and subsequent death. Classifications for proximate cause of death included: congenital; infectious (e.g., bacteria, fungi, and viruses); metabolic; neoplastic; nutritional; parasitic (e.g., metazoan and protozoan); reproductive; non-anthropogenic trauma; anthropogenic trauma; or unknown (Table 1). These categories were adapted from the DAMNIT-V classification system used in veterinary medicine (Lorenz, 2009; Huggins et al., 2015). The same classification scheme was used to identify most likely contributing (secondary and tertiary) causes of death when observed. If an animal was humanely euthanized or died incidentally during rehabilitation, cause of death was assigned based on the pre-existing condition that necessitated euthanasia or rehabilitation. Where possible, we provided additional details on the specific etiology or cause of disease (or death) for each case.
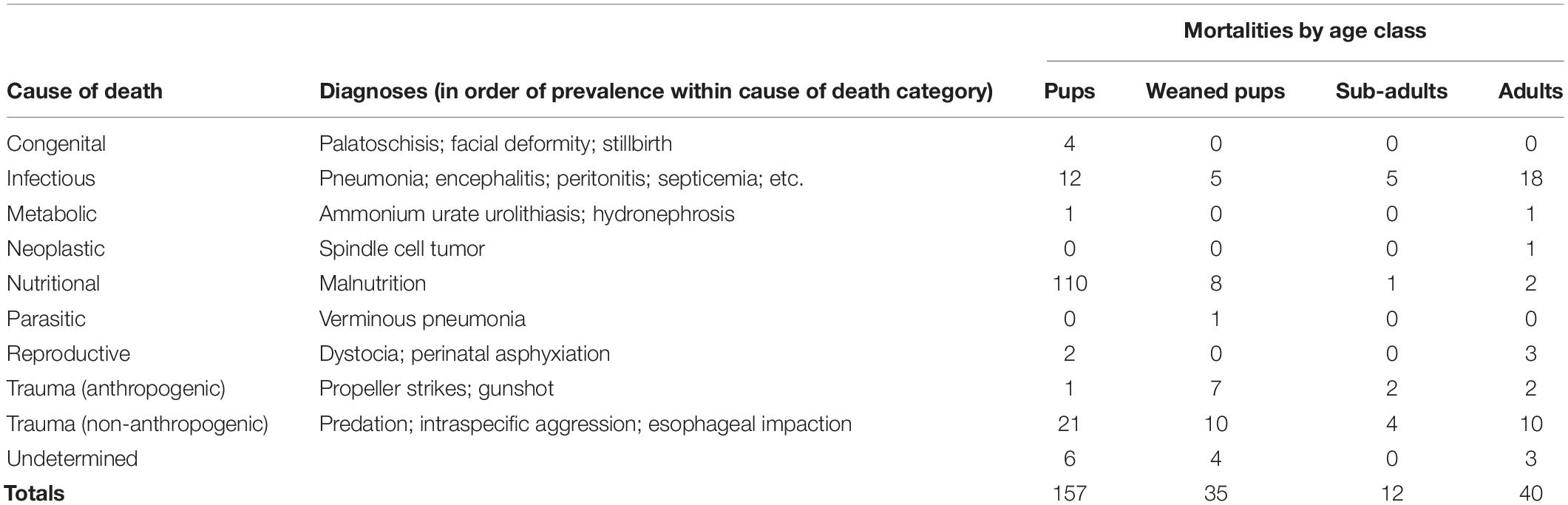
Table 1. Causes of death for harbor seals necropsied between 2002 and 2018 in San Juan County, Washington.
Statistical Analyses
Analyses were performed with R v3.3.3 (R Core Team 2017) using an α-level of 0.05. To analyze seasonal variation in frequency of mortality by age class for all stranded harbor seals, we summarized data by season: winter (December–February); spring (March–May); summer (June–August); and fall (September–November). We performed the chi-square goodness-of-fit test to assess sex bias within each age class for all stranded harbor seals (n = 388 stranded animals with known age and sex). Assuming the harbor seal population has an even (1:1) sex ratio, we used 100 as the expected frequency of males per 100 females in each age class of stranded animals.
We also performed the chi-square goodness-of-fit test to assess whether the sex ratio of necropsied seals (n = 243) differed from those of non-necropsied, stranded seals (n = 145) with known age and sex. We used the proportion of males to females in each age class of stranded harbor seals (excluding necropsied animals) as the expected values. The observed values were the number of males and females in each age class of necropsied seals.
Multiple correspondence analysis (MCA) is a form of multivariate exploratory data analysis used to reveal underlying patterns in datasets encompassing several categorical variables (Greenacre and Blasius, 2006). For MCA, we used only stranding cases categorized into one of the four most common causes of death (infectious, nutritional, anthropogenic trauma, and non-anthropogenic trauma), representing 89% of necropsied harbor seals (144 of 157 pups, 30 of 35 weaned pups, 12 of 12 sub-adults, and 32 of 40 adults). Once categorical variables were transformed into continuous principal components through MCA, hierarchical clustering was performed using the Ward’s criterion to group individuals with shared characteristics. A minimum of three clusters was selected based on partitioning of a hierarchical tree. Statistical analyses and graphics were computed using three R packages: (1) FactoMineR (Lê et al., 2008), (2) factoextra (Kassambara and Mundt, 2017), and (3) ggplot2 (Wickham, 2016).
Results
The SJC harbor seal sub-population grew exponentially between 1970 and the mid-1990s (Figure 1) (Jeffries et al., 2003). Aerial surveys were not conducted again until 2019, when 5,202 harbor seals were reported in SJC (Figure 1) (S. Jeffries, unpublished data).
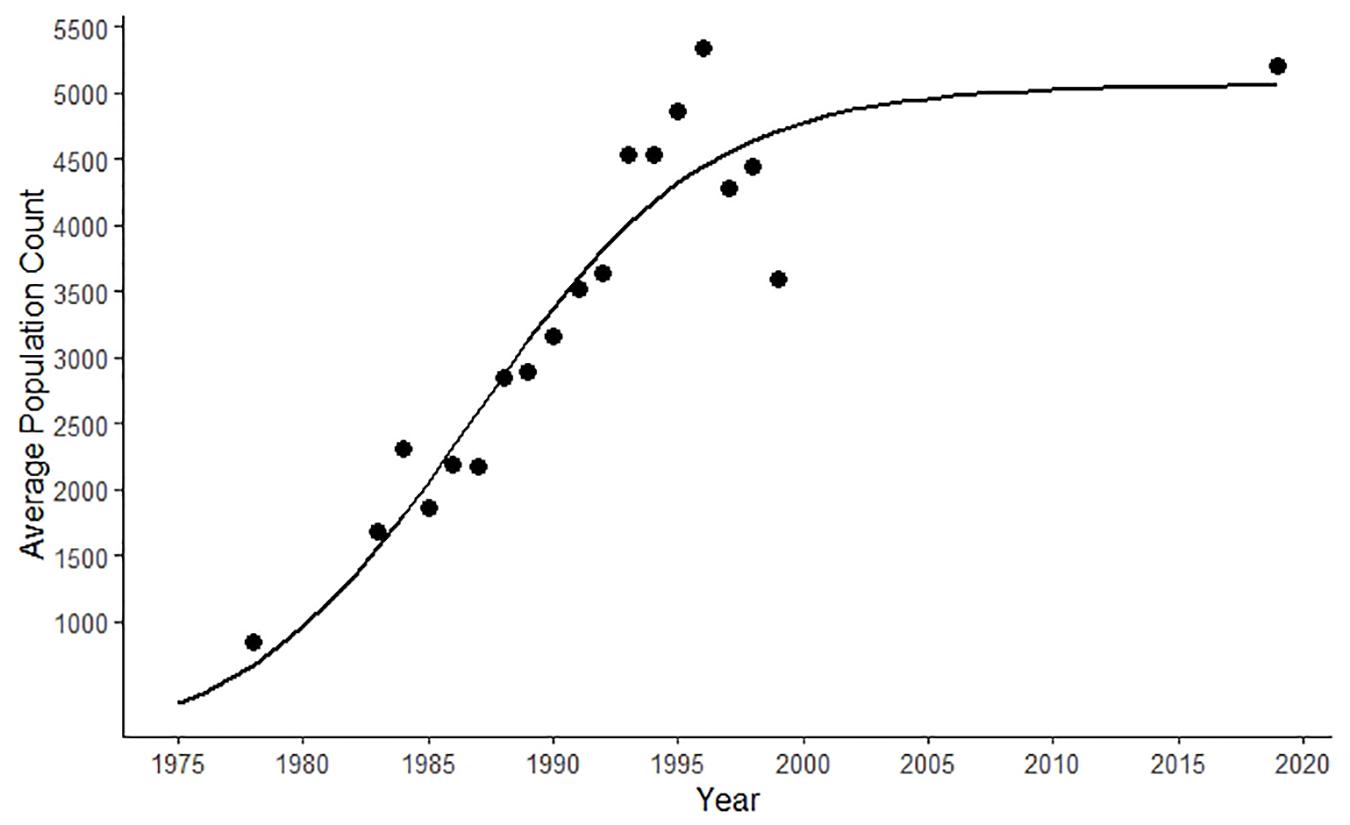
Figure 1. Logistic growth curve of the harbor seal population in the San Juan Islands (i.e., San Juan County) generated using population counts from aerial surveys conducted between 1970 and 2019 (Jeffries et al., 2003; S. Jeffries, unpublished data).
Between January 01, 2002 and December 31, 2018, we found 847 dead-stranded harbor seals in SJC. An additional 35 stranded and died or were humanely euthanized in rehabilitation. Annually, dead strandings (“strandings” or “stranded” hereafter) ranged from 27 to 93 cases per year with an average of 51.8 (SD = 16.8), and complete necropsies were performed on an average of 28.8% (SD = 6.9%) of stranded seals (Figure 2). Cases of stranded seals and those that were necropsied were well distributed throughout the county (Figure 3).
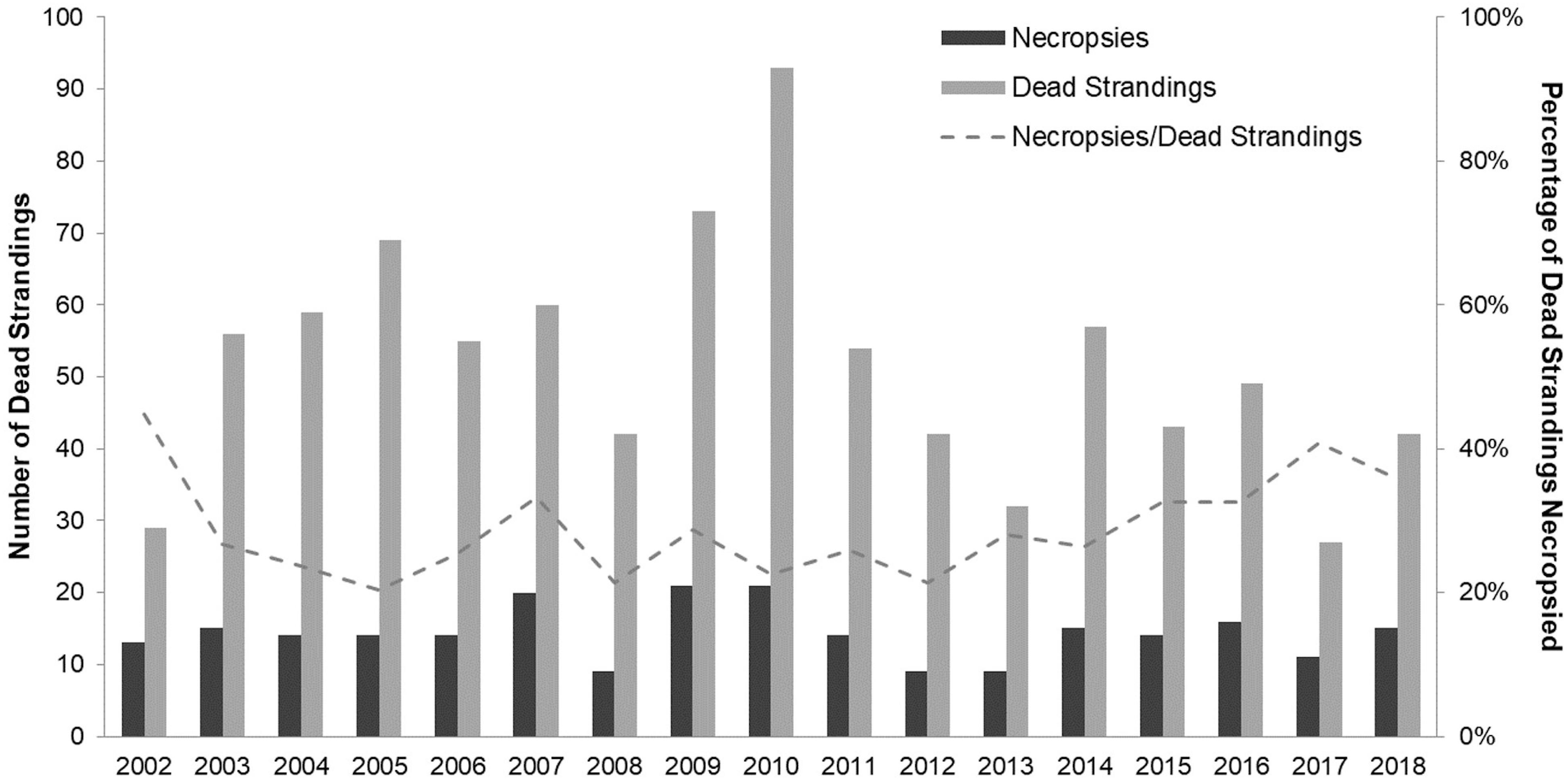
Figure 2. Annual count of dead stranded harbor seals (including live seals that died or were euthanized in rehabilitation) by year (mean = 51.8 ± SD = 16.8) and annual number of harbor seal necropsies performed (mean = 14.3 ± SD = 3.74) in San Juan County between 2002 and 2018. Line graph is the ratio of harbor seals necropsied per year to the total number of harbor seals dead-stranded per year (mean = 28.8% ± SD = 6.9%).
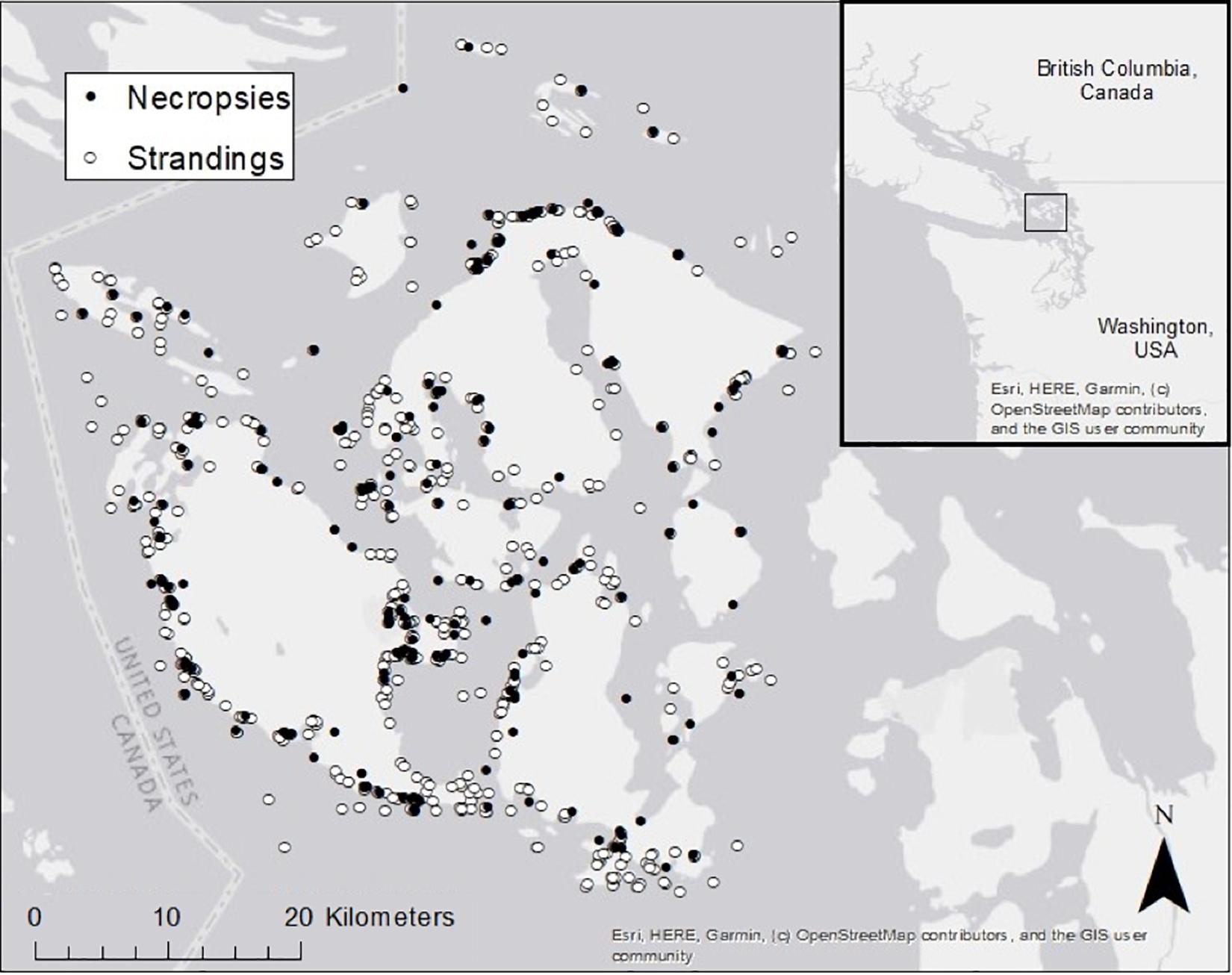
Figure 3. Known stranding locations of dead harbor seals that were necropsied (closed circles, 85% represented) or were not necropsied (open circles, 79% represented) in San Juan County between 2002 and 2018.
Seasonal patterns were evident for stranded seals by age class: pups were found in the summer, weaned pups were mainly found during fall, and adults and sub-adults were mainly found in summer and fall (Figure 4).
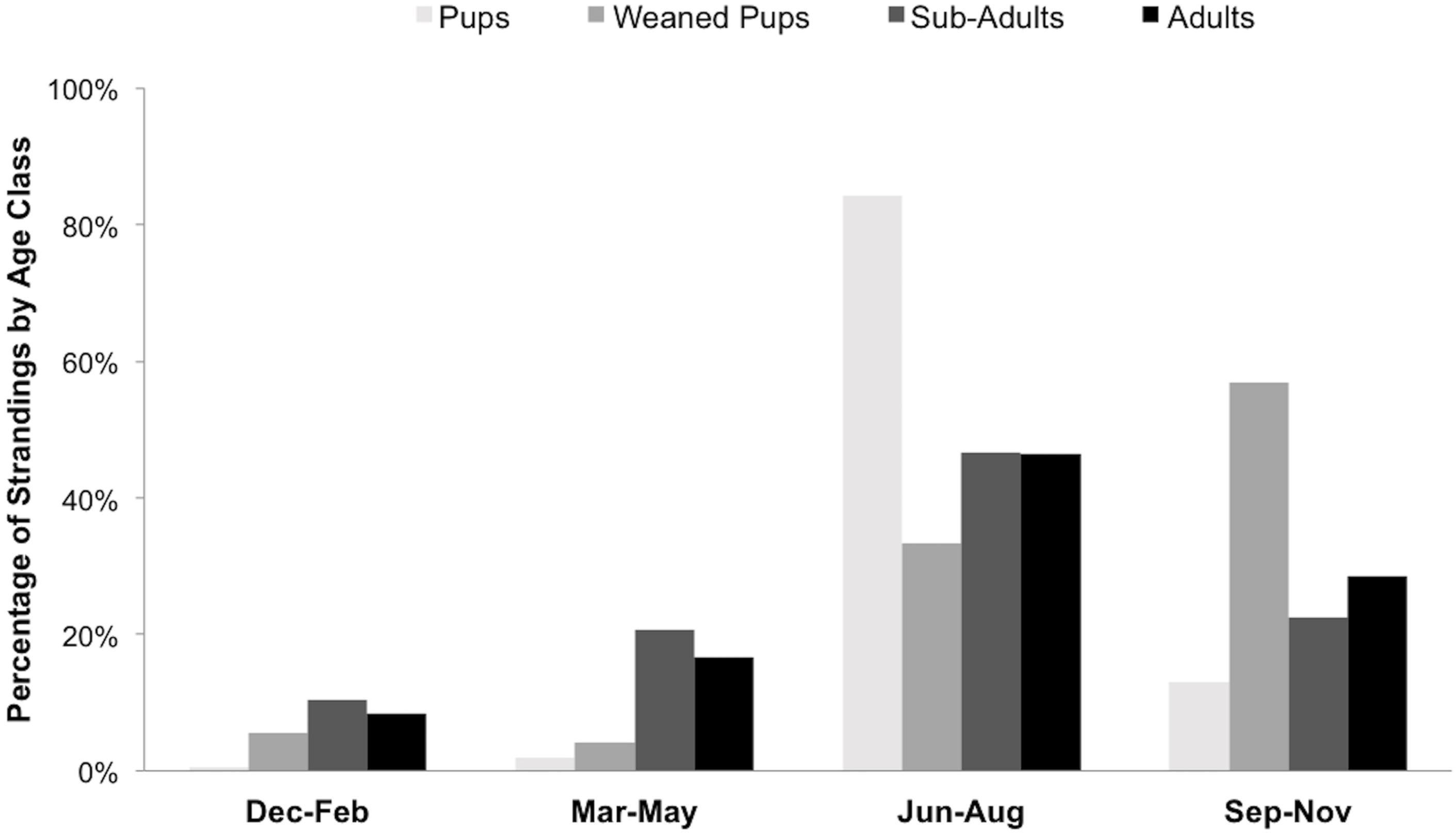
Figure 4. Percentage of stranded harbor seals (n = 882) in San Juan County per age class by season between 2002 and 2018.
For all stranded harbor seals (necropsied animals included), the sex ratio (males per 100 females) for stranded harbor seals where sex and age class were known (n = 388) was 88:100 for pups, 74:100 for weaned pups, 36:100 for sub-adults, and 78:100 for adults. Compared to a hypothetical even sex ratio for stranded animals, only sub-adults diverged [X2 (1, n = 38) = 8.52, p = 0.004]; however, sex was unknown for 36% (21 of 59) of stranded sub-adults.
For necropsied seals with known age class and sex (n = 243), sex ratios diverged from the expected values (proportion of males to females per age class of stranded seals, excluding necropsied seals) only for adults, with the male:female ratio for necropsied seals being 43:100 and expected ratio 188:100 [X2 (1, n = 40) = 20.1, p < 0.001].
The most frequently observed causes of mortality were infectious, nutritional, anthropogenic trauma, and non-anthropogenic trauma, which differed by age class and accounted for 89% of necropsies (Table 1 and Figure 5). Pups primarily died of nutritional causes (70%), whereas sub-adults and adults mainly died of infectious causes (42%) and non-anthropogenic trauma (27%). Weaned pup mortality was distributed equally among the four predominant causes of death. Cause of death could not be determined for thirteen cases (6 pups, 4 weaned pups, and 3 adults).
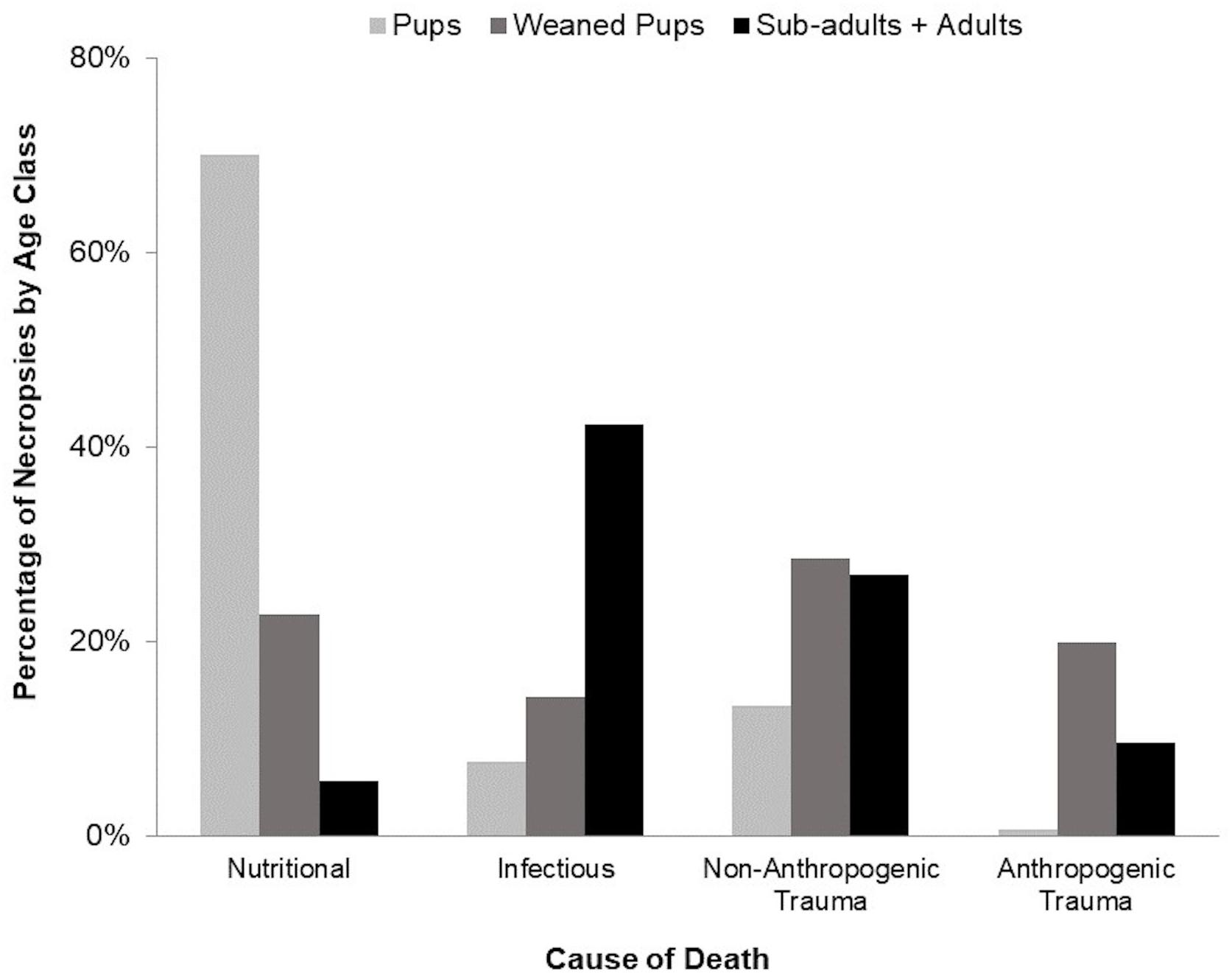
Figure 5. Major cause of death categories by age class, which accounted for 89% of all deaths in necropsied harbor seals (144 of 157 pups, 30 of 35 weaned pups, 12 of 12 sub-adults, and 32 of 40 adults). Sub-adults are combined with adults due to the small sample size.
The two-dimension MCA solution expressed 33.8% of the total variation contained in the dataset, which was significantly greater than the variation obtained by the 0.95 quantile of random distributions (24.5%). The first and second dimensions used were eigenvalue, 0.54 and 0.39, and inertia, 19.6 and 14.2%. The variables correlated most strongly with each dimension are shown in Supplementary Table 1. Hierarchical clustering analysis on principal components of the MCA revealed that most harbor seal mortalities could be grouped into three clusters based on shared age class, season of stranding, and cause of death. Stranded seals belonging to cluster 1 were pups (p < 0.001, v = 14.6) that died in summer (p < 0.001, v = 10.8) of nutritional causes (p < 0.001, v = 9.69). Cluster 2 was characterized by weaned pups (p < 0.001, v = 10.8) that died of anthropogenic trauma (p < 0.001, v = 6.6) in the fall (p < 0.001, v = 6.69). In cluster 3, adults (p < 0.001, v = 10.7) and sub-adults (p < 0.001, v = 3.76) that died of infectious causes (p < 0.001, v = 6.83) and non-anthropogenic trauma (p = 0.04, v = 2.04) in spring (p < 0.001, v = 6.03) and fall (p = 0.004, v = 2.86) were most strongly represented.
Most pups (70%) exhibited nutritional causes of death with 97% of these diagnosed with emaciation-malnutrition syndrome. Our case definition for this diagnosis was suboptimal body weight compared to known average birth weight and expected daily weight gain based on a random sample published by Cottrell et al. (2002), or as evidenced by lack of subcutaneous adipose layer and prominent boney protuberances, where insufficient nutrition was the driver of body condition loss. A contributing cause of death was identified in 46 cases where the primary cause of death was nutritional, including infection (n = 37) and non-anthropogenic trauma (n = 9). Important contributing infectious diagnoses included omphalitis or omphalophlebitis (n = 25) and phocine herpesvirus-1 associated adrenal adenitis (n = 4) (Himworth et al., 2010). Non-anthropogenic trauma was an important primary cause of death for pups (n = 21). Often the cause of trauma could not be determined, but incomplete predation by mammal-eating killer whales (Orcinus orca) was documented in four cases in addition to the two pups and three weaned pups previously described in Gaydos et al. (2005). As expected, all four cases where congenital disease was the primary cause of death occurred in pups.
The weaned pup age class had the highest number of deaths from anthropogenic trauma (n = 7); all were suspected propeller strikes. Weaned pups also died from non-anthropogenic trauma including suspected predation events. Of the five weaned pups that died of infectious causes, all were in the fall, three were cases of bronchopneumonia, one of pleuropneumonia, and one of encephalitis.
Infectious primary causes of death in adults and sub-adults (affecting 44% of seals in these age classes) were dominated by bacterial bronchopneumonia (17 of 23 cases). With the exception of suspected killer whale predation and one case of esophageal perforation by a spotted ratfish spine (Akmajian et al., 2012), the underlying cause of most non-anthropogenic trauma in adults and sub-adults (n = 14) could not be determined. The only case of neoplasia was an emaciated adult (29-year-old) female that had a perigastric spindle cell tumor in the muscularis at the junction of the esophagus and cardia, which likely impinged upon and interfered with normal gastrointestinal transit.
Of the 12 cases for which the primary cause of death was anthropogenic trauma, nine were propeller strikes (1 pup, 7 weaned pups, and 1 adult) and three were gunshot cases (2 sub-adults, one adult).
Toxins were not identified as a primary cause of death for any harbor seal necropsied in this study. However, one adult female had a liver cadmium (Cd) concentration of 22.6 μg/g wet weight, which exceeds the putative lower limit for renal dysfunction in marine mammals (20 μg/g wet weight; Law, 1996) and is over four times greater than the mean liver Cd concentration observed in adult harbor seals in SJC (Ashley et al., 2020). This seal died of septicemia caused by Streptococcus phocae. Because possible immunosuppression from elevated liver Cd concentrations cannot be discounted, toxins were identified as a contributing cause of death.
Zoonotic pathogens were detected at a low frequency in all age classes and may reflect potential environmental and public health concerns. As previously described, Brucella pinnipedialis was isolated or detected by PCR in two sub-adults and one weaned pup (Lambourn et al., 2013). Salmonella enterica subsp. Arizonae and subsp. Litchfield were isolated from the small intestine of two pups, as was Salmonella typhimurium from the small intestine of a weaned pup. One adult and three pups were infected with parapoxviruses, and it is likely that two of the pups were exposed to the viruses while in rehabilitation. One of these pups was simultaneously infected with methicillin-resistant Staphylococcus aureus (MRSA) that we suspect was acquired during rehabilitation as well. Genotyping of the isolate revealed spa type 1 that could be either CMRSA-5 or CMRSA 7/10, which is equivalent to USA300, the most common community-associated clone in people in some areas, including British Columbia. Multidrug-resistant enterococci, which have emerged as a formidable pathogen associated with serious human infections in hospital settings (Grassotti et al., 2018), were isolated from an adult male seal. Parasitic nematodes in the family Anisakidae were identified in 12 adults, seven sub-adults, and two weaned pups. While not directly transmitted to humans by harbor seals, unembryonated nematode eggs shed by seals can infect crustaceans, squid, and fish when they ingest free-living second-stage larvae. Fish and squid infected with third-stage nematode larvae can then infect humans (Dailey, 2005).
Mucormycosis, an emerging fungal disease in marine mammals of the Pacific Northwest (Huggins et al., 2018), was identified in one sub-adult harbor seal in 2009 and one adult in 2019 (the latter was not included in the dataset for this study). During a 2007 Cryptococcus gattii epizootic in Dall’s porpoises (Phocoenoides dalli) and harbor porpoises (Phocoena phocoena), a sub-adult harbor seal was diagnosed with multisystemic C. gattii infection. The clinical presentation and pathologic findings of this animal were consistent with those described by Rosenberg et al. (2016).
Discussion
Rapid population growth in the SJC harbor seal population plateaued in the mid-1990s (Jeffries et al., 2003). Surveys conducted in 2019 found the harbor seal population to be approximately the same size almost two decades later (Figure 1). While population density could have oscillated during the time when no surveys were conducted (2000–2018), the relatively stable annual stranding patterns we documented (Figure 2) suggest that any changes in harbor seal density were neither as pronounced nor as lasting as the dramatic asymptotic growth documented between 1970 and the mid-1990s, suggesting a population at equilibrium. Strandings represent an unknown percentage and bias of the population that dies. That said, our consistent stranding response and the lack of major variation seen in annual stranding rate (Figure 2) support that periodic steep population declines, such as those expected after an epizootic (Eguchi, 2002; Osinga et al., 2012), followed by years of population rebound were not responsible for maintaining this harbor seal population at equilibrium. Instead, the high level of mortality in pups observed every summer suggests that stability is maintained by a small annual population recruitment just large enough to compensate for some low level of mortality occurring in older seals.
Marine mammal strandings are an excellent opportunity to gather mortality data from a population that can be otherwise challenging to study. However, stranded animals represent a potential sample bias, as not all stranded seals are reported or are in suitable condition for postmortem examination once found (Eguchi, 2002). As has been done in other studies (e.g., Osinga et al., 2012), bias was minimized by responding to all reported strandings in all seasons, collecting all animals in suitable condition, and performing consistent complete postmortem examinations on animals.
The observed high percentage of pups dying of malnutrition during summer (Table 1 and Figures 4–6) suggests that a large fraction of pups born annually are unable to survive. While we were unable to identify the precipitating factors for each emaciation-malnutrition case, possibilities include in utero malnutrition, placental insufficiency, primiparous dams, insufficient alimentation and subsequent milk production by dams during the 32 ± 1.5 days of lactation prior to weaning (Cottrell et al., 2002), and post-partum maternal neglect or separation, which may or may not have been related to human interaction.
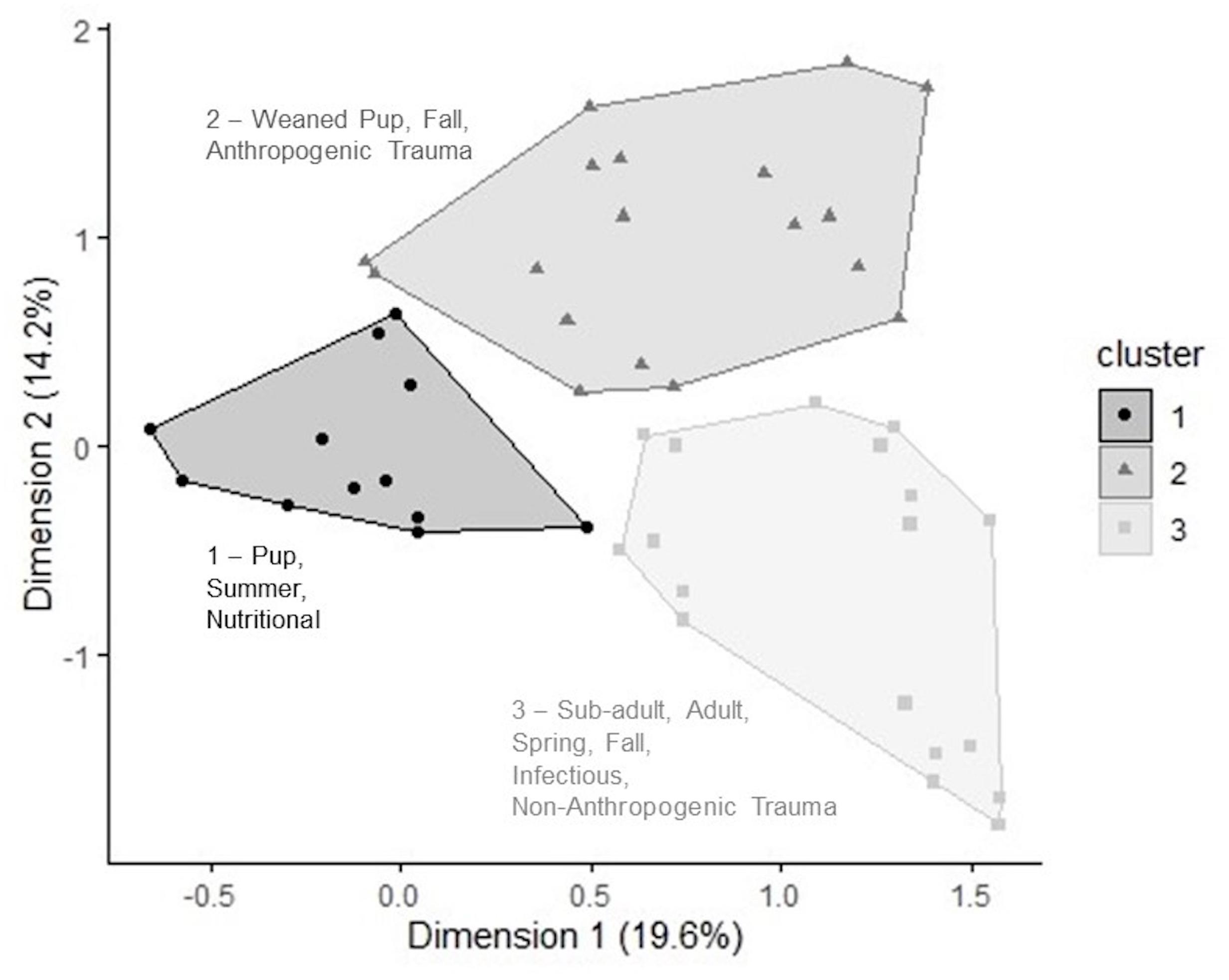
Figure 6. Hierarchical clustering performed on the resulting principal components of a multiple correspondence analysis. Clusters 1–3 represent groups of harbor seals sharing high frequency for categories within the variables: age class, cause of death, and season of stranding. Inertia values for each dimension are labeled on axes.
While malnutrition mainly drives pup mortality in SJC, infectious diseases also contribute to pup loss. Of the 110 pups that died of nutritional causes, 34% (n = 37) had contributing secondary infectious causes, suggesting an interaction between poor nutritional condition and enhanced susceptibility to infectious diseases, likely mediated through immunosuppression, generalized debilitation, or agonal metabolic derangements. The nutrition- and infection-mediated mortality we identify in pups operates in a density-dependent manner in juveniles in other vertebrate populations at or near carrying capacity, such as reindeer (Rangifer tarandus) introduced to the sub-Antarctic island of South Georgia, (Leader-Williams, 1982), some African plains species that compete for forage (Sinclair, 1985), and yellow-eyed juncos (Junco phaeonotus; Sullivan, 1989). Likewise, juveniles often appear to be the age class most susceptible to density-dependent mortality for populations at carrying capacity, as has been shown with red deer (Cervus elaphus scoticus) in Scotland (Guinness et al., 1978), multiple species of mule deer (Odocoileus hemionus spp.; Robinette et al., 1957), and yellow-eyed juncos (Sullivan, 1989). For some mammalian populations at carrying capacity, juvenile mortality is higher among males than females (e.g., Clutton-Brock et al., 1985); however, this was not apparent in our data.
While nutritional factors are considered bottom-up constraints on population growth, in many of the above-cited examples, it is difficult to separate the density-dependent force of nutritional limitation from the top-down influence of predation. In our 17-year retrospective analysis, we only detected cases of predation-related trauma from mammal-eating killer whales in two adults and nine pups or weaned pups. We suspect a far higher level of killer whale-associated predation on harbor seals occurs in SJC (Shields et al., 2018), but this influence is not demonstrable via stranding data as most carcasses are likely consumed rather than beach cast. Even with limited available stranding data on predation, we hypothesize that the factors keeping the harbor seal population in SJC at equilibrium are probably a combination of bottom-up nutritional limitations and top-down predatory influences.
Within the Salish Sea, adult harbor seals display moderate to high site fidelity (Hardee, 2008), usually remaining within 30 km of their primary haul-out sites (DFO, 2010; Peterson et al., 2012). Thus, it is unclear whether our observed age-related causes of mortality are specific to SJC or more widespread in the Salish Sea. It is possible that specific causes of mortality vary regionally with factors such as harbor seal density (Jeffries et al., 2003), human disturbance (Acevedo-Gutierrez and Cendejas-Zarelli, 2011), pathogen and contaminant exposure (Ross et al., 2013; Ashley et al., 2020), and mammal-eating killer whale presence (London et al., 2012; Shields et al., 2018). Regardless of regional variation in the contribution of these factors, malnutrition-related mortality likely contributes to harbor seal population regulation elsewhere in the Salish Sea, as harbor seals are at carrying capacity throughout the ecosystem (DFO, 2010; Jeffries et al., 2003). Over a 7-year period at a site south of SJC, the primary causes of harbor seal mortality were infections followed by malnutrition (Huggins et al., 2013). They also documented up to 25% perinatal mortality in some years. We therefore hypothesize that patterns noted in SJC are likely applicable to populations throughout the Salish Sea.
When looking at harbor seal populations outside of the Salish Sea, a somewhat similar pattern was noted in a study that looked at disease in European harbor seals at two different time points on their population growth curve (Siebert et al., 2007). They found no difference in the pathological changes noted in stranded seals when the population was recovering or recovered from a past epizootic (1996–2002) compared to those examined after a 2002 phocine distemper virus epizootic (2003–2005) when the population was at a much lower density. The major cause of death in all seals over both time periods was bronchopneumonia (Siebert et al., 2007). Interestingly, for the time period when the population was highest (1996–2002), the second most common causes of death were perinatal mortality and cachexia. When the population density was low (2003–2005), the second most common cause of death was infectious (septicemia), and fewer newborns were found to be weak.
It is important to note that while we identified and evaluated mortality factors in a population at equilibrium, we were not able to test for density-dependent effects. Mortality factors might be regulating population density in this population but be present in other harbor seal populations without regulating density. For example, one multi-decadal study looking at mortality in a population of harbor seals that was mostly increasing (with the exception of two phocine distemper virus outbreaks) (Osinga et al., 2012) found multiple categories of disease similar to our findings. These included a wide suite of infectious diseases, trauma cases, and nutrition-related mortality (pup starvation) (Osinga et al., 2012).
Identifying and tracking zoonotic pathogens in harbor seals is important to assess disease risk in other wildlife species, domestic animals, and humans (Greig et al., 2014). For example, while we do not believe that Brucella pinnipedialis affects harbor seals at the population level in the Salish Sea, humans may be at increased risk of contracting brucellosis when they handle weaned harbor seal pups, are exposed to their feces through rehabilitation facilities, or consume raw marine fish or invertebrates (Waltzek et al., 2012; Lambourn et al., 2013). Furthermore, exposure to B. pinnipedialis could pose a risk to mammal-eating or endangered southern resident (fish-eating) killer whales by reducing their fecundity (Gaydos et al., 2004). S. enterica (subsp. Arizonae and Litchfield) and Salmonella typhimurium are common bacteria in pinnipeds that are not typically associated with illness (Greig et al., 2014), but their isolation in harbor seals suggests land-based fecal pollution may also expose humans that swim or fish in SJC. Similarly, while gastrointestinal roundworms of the family Anisakidae are common and usually innocuous in healthy marine mammals, they are a potential concern for human health; humans that consume raw or undercooked seafood infected with the third stage larvae could develop anisakiasis (Dailey, 2005). Pinniped parapoxviruses are widespread globally and can be transmitted to humans via contact, causing painful cutaneous lesions, fever, and myalgia (Hicks and Worthy, 1987; Clark et al., 2005). The presence of poxviruses in SJC harbor seals emphasizes the importance of using personal protective equipment during stranding response and rehabilitation, as well as continued public education to prevent human-seal pup contact.
We did not identify pathogens known to cause large-scale epizootics in harbor seals from other regions, such as morbilliviruses (Härkönen et al., 2006). While bottom-up nutrition-related mortality and top-down predation are likely the major constraints to Salish Sea harbor seal population growth, exposure to a pathogen capable of causing a large epizootic could dramatically shift the SJC harbor seal population away from equilibrium. For example, during the 1988 and 2002 phocine distemper virus (PDV) outbreaks in the North Sea, the harbor seal population plummeted 60 and 50%, respectively, driving population size far below carrying capacity (Reijnders et al., 1997). Remaining harbor seal populations required 5–10 years of rapid exponential growth to recover (Reijnders et al., 1997; Brasseur et al., 2018).
Harbor seals are important components of the Salish Sea ecosystem, occupying a variety of habitats and serving as high trophic level predators. The decades-long stability of the SJC harbor seal population suggests that growth is limited by some combination of prey availability and mammal-eating killer whale predation. However, the corollary that the harbor seal population limits growth of one or more of the sixty or so species of fish and invertebrates they consume has not been demonstrated (Lance et al., 2012). The current factors keeping the harbor seal population at equilibrium could change rapidly and dramatically with the introduction of a pathogen capable of causing a large-scale epizootic.
Data Availability Statement
The datasets generated for this study are available on request to the corresponding author.
Ethics Statement
IACUC approval was not required by the UC Davis Office of Research for this work, as all carcasses used in this study were found dead prior to inclusion in the study.
Author Contributions
JG, JO, EMA, and TA conceived the central ideas guiding the project. EAA assisted with 2 years of carcass collection and necropsies, scrubbed, collated, and analyzed data, and co-wrote the manuscript with JG. JO oversaw the stranding network and helped conduct gross necropsies for 6 years. TA collated and scrubbed data, conducted preliminary analyses, and wrote the initial framework for the manuscript. For all 17 years of data collection, SR analyzed histology samples and oversaw ancillary testing. SR also assigned causes of death with JG. EMA helped conceive the project and supervised TA’s work on the direction of the manuscript. SJ provided harbor seal population data and expertise. For all 17 years, JG co-authored all grants supporting the work, conducted necropsies and prepared final necropsy reports, assigned final causes of death with SR, and co-wrote the manuscript with EAA. All authors contributed to manuscript revision and approved the submitted version.
Conflict of Interest
SR has served as consulting pathologist for the San Juan County Marine Mammal Stranding Network since its inception and is a guest editor for the special edition of “Pathologic Findings in Stranded Marine Mammals: A Global Perspective” for Frontiers in Marine Science.
The remaining authors declare that the research was conducted in the absence of any commercial or financial relationships that could be construed as a potential conflict of interest.
Funding
All samples were collected under permit from the National Marine Fisheries Service Marine Mammal Health and Stranding Response Program (#18786). This study was started while TA was an intern through the Friday Harbor Laboratories Adopt-a-Student Program. Harbor seal stranding response and necropsies were performed using funding from numerous John H. Prescott Marine Mammal Rescue Assistance Grants with in-kind support from The Whale Museum and the SeaDoc Society, a program of the Karen C. Drayer Wildlife Health Center, University of California, Davis School of Veterinary Medicine.
Acknowledgments
We thank the numerous SJC Marine Mammal Stranding Network volunteers and student interns who collected carcasses and assisted with necropsies.
Supplementary Material
The Supplementary Material for this article can be found online at: https://www.frontiersin.org/articles/10.3389/fmars.2020.00319/full#supplementary-material
Footnotes
References
Acevedo-Gutierrez, A., and Cendejas-Zarelli, S. (2011). Nocturnal haul-out patterns of harbor seals (Phoca vitulina) related to airborne noise levels in Bellingham, Washington, USA. Aquat. Mamm. 37, 167–174. doi: 10.1578/AM.37.2.2011.167
Akmajian, A. M., Lambourn, D. M., Lance, M. M., Raverty, S. S., and Gaydos, J. K. (2012). Mortality related to spotted ratfish (Hydrolagus colliei) in Pacific harbor seals (Phoca vitulina) in Washington State. J. Wild. Dis. 48, 1057–1062. doi: 10.7589/2011-12-348
Ashley, E. A., Olson, J. K., Raverty, S., Wilkinson, K., and Gaydos, J. K. (2020). Trace element concentrations in livers of Pacific harbor seals (Phoca vitulina richardii) from San Juan County, Washington, USA. J. Wildl. Dis. 56, 429–436. doi: 10.7589/2019-04-087
Benskin, C. M. H., Wilson, K., Kones, K., and Hartley, I. H. (2009). Bacterial pathogens in wild birds: a review of the frequency and effects of infection. Biol. Rev. 84, 349–373. doi: 10.1111/j.1469-185X.2008.00076.x
Brasseur, S. M. J. M., Reijnders, P. J. H., Cremer, J., Meesters, E., Kirkwood, R., Jensen, L. F., et al. (2018). Echoes from the past: regional variations in recovery within a harbor seal population. PLoS One 13:e0189674. doi: 10.1371/journal.pone.0189674
Clark, C., McIntyre, P. G., Evans, A., McInnes, C. J., and Lewis-Jones, S. (2005). Human sealpox resulting from a seal bite: confirmation that sealpox virus is zoonotic. Br. J. Dermatol. 152, 791–793. doi: 10.1111/j.1365-2133.2005.06451.x
Clutton-Brock, T. H., Albon, S. D., and Guinness, F. E. (1985). Parental investment and sex differences in juvenile mortality in birds and mammals. Nature 313:131.
Cottrell, P., Jeffries, S., Beck, B., and Ross, P. S. (2002). Growth and development in free-ranging harbor seal (Phoca vitulina) pups from southern British Columbia, Canada. Mar. Mamm. Sci. 18, 721–733. doi: 10.1111/j.1748-7692.2002.tb01069.x
Dailey, M. D. (2005). “Parasites of marine mammals,” in Marine Parasitology, ed. K. Rohde (Clayton, VIC: Csiro Publishing), 408–414.
Deem, S. L., Karesh, W. B., and Weisman, W. (2001). Putting theory into practice: wildlife health in conservation. Conserv. Biol. 15, 1224–1233.
del Monte-Luna, P., Brook, B. W., Zetina-Rejón, M. J., and Cruz-Escalona, V. H. (2004). The carrying capacity of ecosystems. Glob. Ecol. Biogeogr. 13, 485–495.
DeMaster, D. P., Trites, A. W., Clapham, P., Mizroch, S., Wade, P., Small, R. J., et al. (2006). The sequential megafaunal collapse hypothesis: testing with existing data. Prog. Oceanogr. 68, 329–342.
DFO (2010). Population Assessment Pacific Harbour Seal (Phoca vitulina richardsi): Canadian Science Advisory Secretariat Science Advisory Report 2009/011. Vancouver, BC: Fisheries and Oceans Canada.
Eguchi, T. (2002). A method for calculating the effect of a die off from stranding data. Mar. Mamm. Sci. 18, 698–e709. doi: 10.1111/j.1748-7692.2002.tb01067.x
Gaydos, J. K., Balcomb, K. C. III, Osborne, R. W., and Dierauf, L. (2004). Evaluating potential infectious disease threats for southern resident killer whales, Orcinus orca: a model for endangered species. Biol. Conserv. 117, 253–262.
Gaydos, J. K., Dierauf, L., Kirby, G., Brosnan, D., Gilardi, K., and Davis, G. E. (2008). Top 10 principles for designing healthy coastal ecosystems like the Salish Sea. EcoHealth 5, 460–471. doi: 10.1007/s10393-009-0209-1
Gaydos, J. K., Raverty, S., Baird, R. W., and Osborne, R. W. (2005). Suspected surplus killing of harbor seal pups (Phoca vitulina) by killer whales (Orcinus orca). Northwest Nat. 86, 150–154.
Geraci, J. R., and Lounsbury, V. J. (2005). Marine Mammals Ashore: A Field Guide for Strandings. Baltimore, MD: National Aquarium in Baltimore, Inc.
Geraci, J. R., St Aubin, D. J., Barker, I. K., Webster, R. G., Hinshaw, V. S., Bean, W. J., et al. (1982). Mass mortality of harbor seals: pneumonia associated with influenza-a virus. Science 215, 1129–1131. doi: 10.1126/science.7063847
Goss-Custard, J. D., Stillman, R. A., West, A. D., Caldow, R. W. G., and McGrorty, S. (2002). Carrying capacity in overwintering migratory birds. Biol. Conserv. 105, 27–41.
Grassotti, T. T., Zvoboda, D. D., Costa, L. D. X., de Araujo, A. J. G., Pereira, R. I., Soares, R. O., et al. (2018). Antimicrobial resistance profiles in Enterococcus spp. isolates from fecal samples of wild and captive black capuchin monkeys (Sapajus nigritus) in south Brazil. Front. Microbiol. 9:2366. doi: 10.3389/fmicb.2018.02366
Greenacre, M., and Blasius, J. eds (2006). Multiple Correspondence Analysis and Related Methods. Boca Raton, FL: Chapman & Hall.
Greig, D. J., Gulland, F. M. D., Smith, W. A., Conrad, P. A., Field, C. L., Fleetwood, M., et al. (2014). Surveillance for zoonotic and selected pathogens in harbor seals Phoca vitulina from central California. Dis. Aquat. Org. 111, 93–106. doi: 10.3354/dao02762
Guinness, F. E., Clutton-Brock, T. H., and Albon, S. D. (1978). Factors affecting calf mortality in red deer (Cervus elaphus). J. Anim. Ecol. 47, 817–832.
Hardee, S. E. (2008). Movements and Home Ranges of Harbor Seals (Phoca vitulina) in the inland waters of the Pacific Northwest. Ph.D. thesis, Western Washington University, Bellingham, WA, 148.
Härkönen, T., Dietz, R., Reijnders, P., Teilmann, J., Harding, K., Hall, A., et al. (2006). A review of the 1988 of 2002 phocine distemper virus epidemics in European harbor seals. Dis. Aquat. Org. 68, 115–130.
Hicks, B. D., and Worthy, G. A. J. (1987). Sealpox in captive grey seals (Halichoerus grypus) and their handlers. J. Wildl. Dis. 23, 1–6. doi: 10.7589/0090-3558-23.1.1
Himworth, C., Haulena, M., Lambourn, D., Gaydos, J. K., Huggins, J., Calambokidis, J., et al. (2010). Pathology and epidemiology of phocid herpesvirus-1 in wild and rehabilitating harbor seals (Phoca vitulina richardsi) in the northeastern Pacific. J. Wildl. Dis. 46, 1046–1051. doi: 10.7589/0090-3558-46.3.1046
Huber, H. S., Jeffries, S., Lambourn, D., and Dickerson, B. (2012). Population substructure of harbor seals (Phoca vitulina richardsi) in Washington State using mtDNA. Can. J. Zool. 88, 280–288.
Huggins, J. L., Lambourn, D. M., Raverty, S. J., Hanson, M. B., Rhodes, L. D., Norman, S. A., et al. (2018). “Mucormycosis: an emerging disease in marine mammals of the Pacific Northwest,” in Proceedings of the 49th Annual International Association for Aquatic Animal Medicine, Long Beach, CA.
Huggins, J. L., Leahy, J., Calambokidis, J., Lambourn, D., Jeffries, S. J., Norman, S. A., et al. (2013). Causes and patterns of harbor seal (Phoca vitulina) pup mortality at Smith Island, Washington, 2004–2010. Northwest Nat. 94, 198–208.
Huggins, J. L., Raverty, S., Norman, S. A., Calambokidis, J., Gaydos, J. K., Duffield, D. A., et al. (2015). Increased harbor porpoise mortality in the Pacific Northwest, USA: understanding when higher levels may be normal. Dis. Aquat. Org. 115, 93–102. doi: 10.3354/dao02887
Jeffries, S., Gearin, P. J., Huber, H. R., Saul, D. L., and Pruett, D. A. (2000). Atlas of Seal and Sea Lion Haulout Sites in Washington. Olympia WA: Washington Department of Fish and Wildlife, 150.
Jeffries, S., Huber, H., Calambokidis, J., and Laake, J. (2003). Trends and status of harbor seals in Washington State: 1978-1999. J. Wildl. Manage. 67, 207–218.
Kassambara, A., and Mundt, F. (2017). Package “factoextra”. Available online at: https://cran.r-project.org/web/packages/factoextra/index.html (accessed November 1, 2019).
Lambourn, D. M., Garner, D., Ewalt, J., Rhyan, S., Raverty, S., Jeffries, S., et al. (2013). Brucella pinnipedialis infections in Pacific harbor seals (Phoca vitulina richardsi) from Washington State. J. Wildl. Dis. 49, 802–815. doi: 10.7589/2012-05-137
Lance, M. M., Chang, W. Y., Jeffries, S., and Pearson, S. (2012). Harbor seal diet in northern Puget sound: implications for the recovery of depressed fish stocks. Mar. Ecol. Prog. Ser. 464, 257–271. doi: 10.3354/meps09880
Law, R. J. (1996). “Metals in marine mammals,” in Environmental Contaminants in Wildlife: Interpreting Tissue Concentrations, eds W. N. Beyer, G. Heinz, and A. Redmon-Norwood (Boca Raton, FL: Lewis Publishers), 357–376.
Lê, S., Josse, J., and Husson, F. (2008). Package factoMineR: a package for multivariate analysis. J. Stat. Softw. 25, 1–18. doi: 10.18637/jss.v025.i01
Leader-Williams, N. (1982). Relationship between a disease, host density and mortality in a free-living deer population. J. Anim. Ecol. 51, 235–240.
Lefebvre, K. A., Quakenbush, L., Frame, E., Burek-Huntington, K., Sheffield, G., Stimmelmayr, R., et al. (2016). Prevalence of algal toxins in Alaskan marine mammals foraging in a changing arctic and subarctic environment. Harmful Algae 55, 13–24. doi: 10.1016/j.hal.2016.01.007
Linnell, J. D., Aanes, R., and Anderson, R. (1995). Who killed Bambi? The role of predation in the neonatal mortality of temperate ungulates. Wildl. Biol. 1, 209–223.
London, J. M., Ver Hoef, J. M., Jeffries, S. J., Lance, M. M., and Boveng, P. L. (2012). Haul-Out behavior of harbor seals (Phoca vitulina) in Hood Canal, Washington. PLoS One 7:e38180. doi: 10.1371/journal.pone.0038180
Lorenz, M. D. (2009). “The problem-oriented approach,” in Small Animal Medical Diagnosis, eds M. D. Lorenz, T. M. Neer, and P. DeMars (Ames, IA: Wiley-Blackwell), 3–12.
Lucas, Z., and Stobo, W. (2000). Shark-inflicted mortality on a population of harbour seals (Phoca vitulina) at Sable Island, Nova Scotia. J. Zool. 252, 405–414.
Majewski, S. P., and Ellis, G. M. (2019). Abundance and Distribution of Harbour Seals (Phoca vitulina). British Columbia, VIC: Prep Publishing.
May, R. M., and Anderson, R. M. (1979). Population biology of infectious diseases: part II. Nature 280, 455–461. doi: 10.1038/280455a0
Osinga, N., Shahi Ferdous, M. M., Morick, D., Garcia Hartmann, M., Ulloa, J. A., Vedder, L., et al. (2012). Patterns of stranding and mortality in common seals (Phoca vitulina) and grey seals (Halichoerus grypus) in the Netherlands between 1979 and 2008. J. Comp. Path. 147, 550–565. doi: 10.1016/j.jcpa.2012.04.001
Peterson, S. H., Lance, M. M., Jeffries, S. J., and Acevedo-Gutierrez, A. (2012). Long distance movements and disjunct spatial use of harbor seals (Phoca vitulina) in the inland waters of the Pacific Northwest. PLoS One 7:e39046. doi: 10.1371/journal.pone.0039046
R Core Team (2017). R: A Language and Environment for Statistical Computing. Vienna: R Foundation for Statistical Computing.
Reijnders, P. J. H., Ries, E. H., Tougaard, S., Norgaard, N., Heidemann, G., Schwarz, J., et al. (1997). Population development of harbor seals Phoca vitulina in the Wadden Sea after the 1988 virus epizootic. J. Sea Res. 38, 161–168.
Robinette, W. L., Gashwiler, J. S., Low, J. B., and Jones, A. (1957). Differential mortality by sex and age among mule deer. J. Wildl. Manage. 21, 1–16.
Rosenberg, J., Haulena, M., Hoang, L. M. N., Morshed, M., Zabek, E., and Raverty, S. (2016). Cryptococcus gattii type VGIIa infection in harbor seals (Phoca vitulina) in British Columbia, Canada. J. Wildl. Dis. 52, 677–681. doi: 10.7589/2015-11-299
Ross, P. S., Noel, M., Lambourn, D., Dangerfield, N., Calambokidis, J., and Jeffries, S. (2013). Declining concentrations of persistent PCBs, PBDEs, PCDEs, and PCNs in harbor seals (Phoca vitulina) from the Salish Sea. Prog. Oceanogr. 115, 160–170. doi: 10.1016/j.pocean.2013.05.027
Shields, M. W., Hysong-Shimazu, S., Shields, J. C., and Woodruff, J. (2018). Increased presence of mammal-eating killer whales in the Salish Sea with implications for predator-prey dynamics. PeerJ 6:c6062. doi: 10.7717/peerj.6062
Siebert, U., Wohlseiny, P., Lehnert, K., and Baumgartnery, W. (2007). Pathological findings in harbour seals (Phoca vitulina): 1996 – 2005. J. Comp. Path 137, 47–58. doi: 10.1016/j.jcpa.2007.04.018
Sinclair, A. R. E. (1985). Does interspecific competition or predation shape the African Ungulate community? J. Anim. Ecol. 54, 899–918.
Springer, A. M., Estes, J. A., Van Vliet, G. B., Williams, T. M., Doak, D. F., Danner, E. M., et al. (2003). Sequential megafaunal collapse in the North Pacific Ocean: an ongoing legacy of industrial whaling? PNAS 100, 12223–12228. doi: 10.1073/pnas.1635156100
Sullivan, K. A. (1989). Predation and starvation: age-specific mortality in juvenile juncos (Junco phaenotus). J. Anim. Ecol 58, 275–286.
Waltzek, T. B., Cortes-Hinojosa, G., Wellehan, J. F. X. Jr., and Gray, G. C. (2012). Marine mammal zoonoses: a review of disease manifestations. Zoonoses Public Health 59, 521–535. doi: 10.1111/j.1863-2378.2012.01492.x
Keywords: carrying capacity, equilibrium population, harbor seal, marine mammals, pathology, Salish Sea, San Juan Islands, stranding network
Citation: Ashley EA, Olson JK, Adler TE, Raverty S, Anderson EM, Jeffries S and Gaydos JK (2020) Causes of Mortality in a Harbor Seal (Phoca vitulina) Population at Equilibrium. Front. Mar. Sci. 7:319. doi: 10.3389/fmars.2020.00319
Received: 15 February 2020; Accepted: 20 April 2020;
Published: 13 May 2020.
Edited by:
Alastair Martin Mitri Baylis, South Atlantic Environmental Research Institute, Falkland IslandsReviewed by:
Mauricio Seguel, University of Georgia, United StatesElizabeth McHuron, University of California, Santa Cruz, United States
Peter Douglas Shaughnessy, South Australian Museum, Australia
Copyright © 2020 Ashley, Olson, Adler, Raverty, Anderson, Jeffries and Gaydos. This is an open-access article distributed under the terms of the Creative Commons Attribution License (CC BY). The use, distribution or reproduction in other forums is permitted, provided the original author(s) and the copyright owner(s) are credited and that the original publication in this journal is cited, in accordance with accepted academic practice. No use, distribution or reproduction is permitted which does not comply with these terms.
*Correspondence: Joseph K. Gaydos, jkgaydos@ucdavis.edu