- Molecular Biology of Microbial Consortia, Institute of Plant Science and Microbiology, Universität Hamburg, Hamburg, Germany
The genus Hydrogenovibrio consists of chemolithotrophic sulfur- and hydrogen-oxidizing bacteria that are found in diverse marine environments including hydrothermal vents where they can reach high cell numbers. Although several vent Hydrogenovibrio genomes encode for [NiFe]-hydrogenases (enzymes catalyzing the reversible reaction of hydrogen into protons and electrons), different attempts to grow these strains on hydrogen failed for a long time. Not long ago it was shown that some Hydrogenovibrio strains from hydrothermal vents are indeed able to oxidize hydrogen, which broadens their physiological spectrum in a competitive environment for energy sources. We here identify two active hydrogen consuming bacteria of the Hydrogenovibrio genus with different hydrogenase genes from vents in the South Pacific Ocean. Based on our results, hydrogen consuming Hydrogenovibrio species seem to be much more widespread in the oceans than expected.
Introduction
Hydrogenovibrio species are common in hydrothermal vent environments (Brazelton and Baross, 2010; Böhnke et al., 2019). They were originally described as Thiomicrospira species and based on physiology, morphology and phylogeny only recently reclassified as Hydrogenovibrio (Boden et al., 2017). Initially they were described as chemolithotrophic sulfur-oxidizers capable of using hydrogen sulfide, thiosulfate, tetrathionate and sulfur under aerobic and/or microaerobic conditions (e.g., Ruby and Jannasch, 1982; Jannasch et al., 1985; Brinkhoff and Muyzer, 1997; Takai et al., 2004). Mostly, they were shown to be autotrophic CO2 fixers, but some were posited to be chemolithomixotrophs (Takai et al., 2004). Their ability to utilize alternative inorganic electron donors was expanded by hydrogen oxidation and iron oxidation in the last few years (Hansen and Perner, 2015; Barco et al., 2017). Recent incubation experiments with hydrothermal fluids demonstrate that when spiked with hydrogen or iron their numbers as well as biomass synthesis can increase considerably in some hydrothermal fluids (Böhnke et al., 2019). Consequently, they appear to be important at hydrothermal vents for carbon turnover.
The first Hydrogenovibrio species from a vent for which a hydrogenase (enzyme converting H2 < - > 2H+ + 2e–) was discovered on its genome was H. crunogenus XCL-2 (previously Thiomicrospira crunogena XCL-2) (Scott et al., 2006), although repetitive experiments under different conditions could not detect hydrogen consumption in cultivation experiments (Hansen and Perner, 2016a, b). Hydrogenases from other hydrothermal vent Hydrogenovibrio species were later identified and respective hydrogen consumption ability verified (Hansen and Perner, 2016a). The hydrogenases of the actively consuming hydrogen oxidizing Hydrogenovibrio vent species were identified as [NiFe]-hydrogenases of the subgroup 1, associated with hydrogen uptake (Vignais and Billoud, 2007). Two major subgroups among the Hydrogenovibrio were recognized: those forming cluster I with MA2-6 and SP-41 (from the Mid-Atlantic Ridge, MAR) (Brinkhoff and Muyzer, 1997; Hansen and Perner, 2015) and H. marinus (from the water column) (Nishihara et al., 1991), other Gammaproteobacteria, Alpha- and Zetaproteobacteria (Hansen and Perner, 2016a). The cluster II combines the H. crunogenus hydrogenases encoded on the genomes of MA-3 (Wirsen et al., 1998), SP-41 (Hansen and Perner, 2015) from the MAR and TH-55 (Jannasch et al., 1985) and L-12 (Ruby and Jannasch, 1982) from the East Pacific Vents (Hansen and Perner, 2016a). According to Greening et al. (2016), cluster I hydrogenases are associated with group 1d and cluster II with group 1b. Just recently a new Hydrogenovibrio thermophilus strain from the Southwest Indian Ridge (SWIR) was isolated, namely S5 which encodes a hydrogenase associated with cluster I and which can consume hydrogen (Jiang et al., 2017).
To date no hydrothermal vent Hydrogenovibrio species have been enriched from vents in the Southern Pacific that encode a hydrogenase of cluster I or II and/or exhibit hydrogen consumption ability. Here, we expand the geographic range of hydrogen oxidizing Hydrogenovibrio crunogenus species, so far only found along mid-ocean ridges (MOR), to an island arc venting system.
Materials and Methods
Sample Collection
A hydrothermal fluid (45 ROV 5F/6F KIPS A/B; 70°C, water depth 1318 m; −34°52.73383’S 179°04.26386’E) was taken with the remotely operative vehicle ROV Quest (MARUM, University Bremen) during the HYDROTHERMADEC cruise (SO253, December 2016/January 2017) with the RV Sonne. The hydrothermal fluid was retrieved with the pumped flow-through system KIPS (Kiel Pumping System) (Garbe-Schönberg et al., 2006) from Brothers lower cone in the Kermadec region. For further details on sampling see Perner et al. (2009). The sample was immediately processed after retrieval on board.
Enrichment, Cultivation and Isolations Attempts
Initially an enrichment culture was grown by inoculating artificial seawater medium (MJ medium, 10 ml) and T-ASW medium (10 ml) under an atmosphere of H2:CO2:O2 (79:20:1) (Westfalen AG, Münster, Germany) at standard pressure with 1 ml of the diffuse fluid sample. MJ was prepared as described before without yeast extract and trypticase peptone, but with addition of 10 ml vitamin solution (Balch et al., 1979). The reduction state of the MJ medium was monitored with resazurin (0.5 mg l–1). We prepared the T-ASW medium like described previously (Dobrinski et al., 2005) but with raised Ni and Fe concentrations (0.003 mM and 0.03 mM final concentration, same as in MJ medium). After incubation at 28°C for 1 week a color change in the MJ medium (from blue to colorless), which accompanies the consumption of oxygen, could be observed. Serum bottles with 50 ml of MJ and T-ASW medium were then inoculated with 2 mL of the pre-cultures and supplemented with the gas mixture. The enrichment culture was routinely cultivated on the respective media as described above.
To obtain a pure culture, we used the PALM MicroTweezers microscope (Carl Zeiss AG, Oberkochen, Germany) for picking single cells. Additionally we made six-fold dilution series with T-ASW and MJ medium to 10–10. Since both methods were not successful for isolating the strains in MJ medium, we additionally plated the cultures on MJ agar plates.
Hydrogen Consumption Measurements
The cultures were grown on the respective medium (MJ or T-ASW) in serum bottles with an H2:CO2:O2:He (2:20:1:77) (Westfalen AG, Münster, Germany) atmosphere at standard pressure. The experiment was set up in triplicate and sterilized medium was used as a control. The concentration of hydrogen was measured using gas chromatography and cell numbers were determined using an Olympus BX41 (Olympus, K.K., Tokyo, Japan) microscope as described before (Hansen and Perner, 2015).
DNA and RNA Extraction, cDNA Generation and Amplification of 16S rRNA and hynL Gene Fragments
At the end of the hydrogen consumption experiments the active organisms were determined by simultaneous DNA and RNA extraction using TRIzolTM Reagent (Fisher Scientific, Schwerte, Germany) in combination with a phenol-chloroform extraction method and the Direct-zolTM RNA Miniprep Kit (Zymo Research, Irvine, United States). Complementary DNA synthesis was performed using the SuperScriptTM VILOTM cDNA Synthesis Kit (Fisher Scientific, Schwerte, Germany). Polymerase chain reaction and sequencing of 16S rRNA and hynL genes were performed (see Supplementary Figure 1; Hansen and Perner, 2015, 2016a). The 16S rRNA and hynL gene sequences were deposited under the NCBI accession numbers MN923280, MN923281, MN935466, and MN935467.
Results and Discussion
We used hydrothermal fluids (45ROV5F/6F) from the Brothers volcano on the Kermadec island arc (New Zealand) to enrich for Hydrogenovibrio species. Two types of artificial seawater media were used: (i) T-ASW medium and (ii) MJ medium (cf. Sako et al., 1996; Dobrinski et al., 2005; Hansen and Perner, 2015) – for differences of media compositions see Hansen and Perner (2016b). Since we aimed at culturing hydrogen oxidizers, the medium was supplemented with hydrogen gas (79H2:20CO2:1O2) and kept at room temperature. From these enrichments, two new hydrogen consuming Hydrogenovibrio species were identified: Hydrogenovibrio sp. 45ROV5F/6F-TASW and Hydrogenovibrio sp. 45ROV5F/6F-MJ. According to 16S rRNA genes, the former was related to H. crunogenus species and the latter to H. thermophilus (Figure 1). Both strains encoded a hydrogen uptake group 1 [NiFe]-hydrogenase (Figure 2). The 45ROV5F/6F-TASW hydrogenase grouped in cluster II common for other H. crunogenus hydrogenases. 45ROV5F/6F-MJ’s hydrogenase was related to the hydrogenase from MA2-6 in cluster I (Figure 2). It actually exhibited the highest similarity (99% AA identity) to an environmental hydrogenase sequence reported from the Lilliput venting field at 9°S on the MAR (Perner et al., 2007).
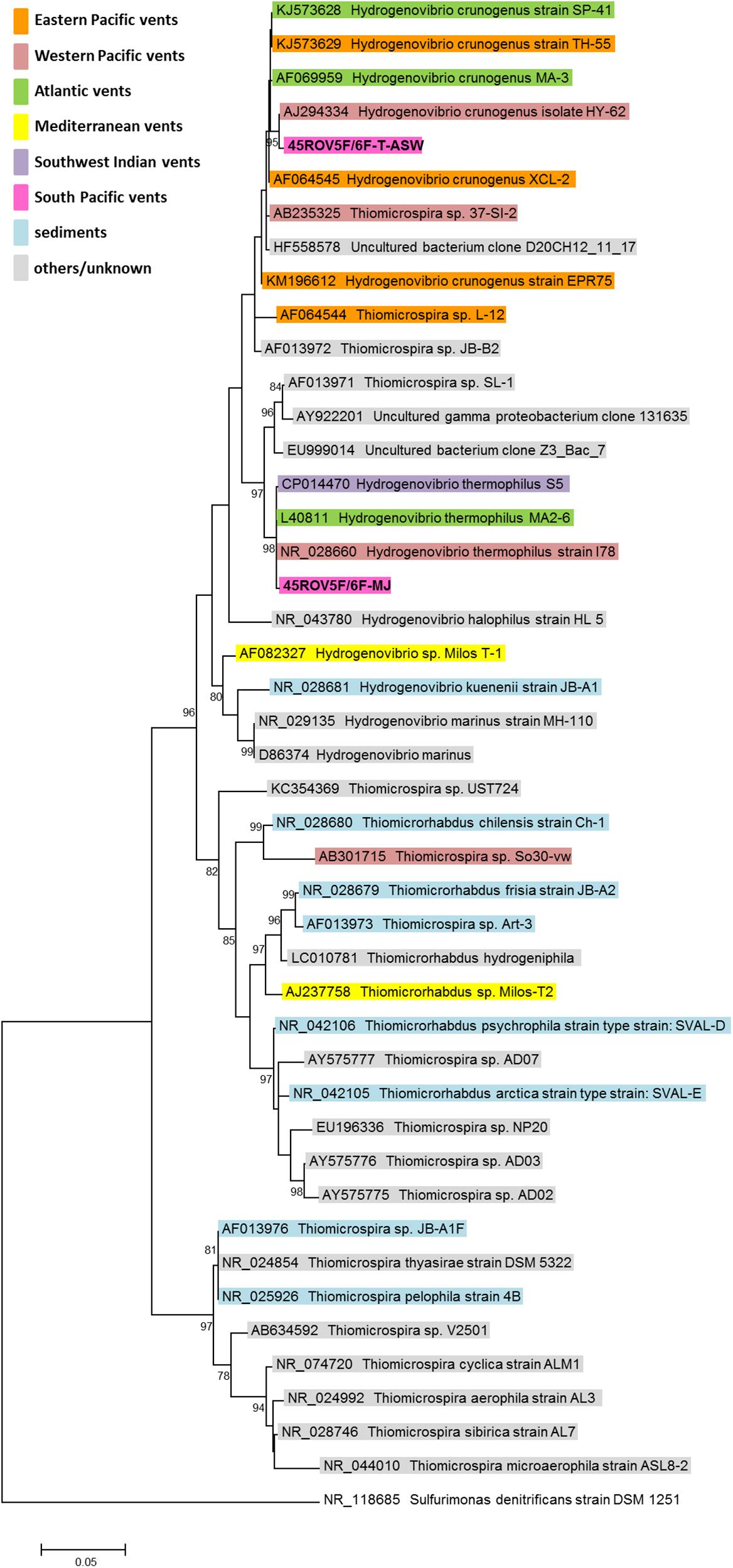
Figure 1. Phylogenetic relationship of Thiomicrospira and Hydrogenovibrio species based on 16S rRNA genes. Sequences were aligned using the multiple sequence alignment web server T-Coffee (Notredame et al., 2000) with followed construction of the phylogenetic tree using Mega-X (Kumar et al., 2018) and 1000 bootstraps. The Thiomicrospira and Hydrogenovibrio species are color-coded according to their isolation source. The strains described here are written in bold. The scale bar represents the changes per nucleotide. Only bootstrap values higher than 75 are given.
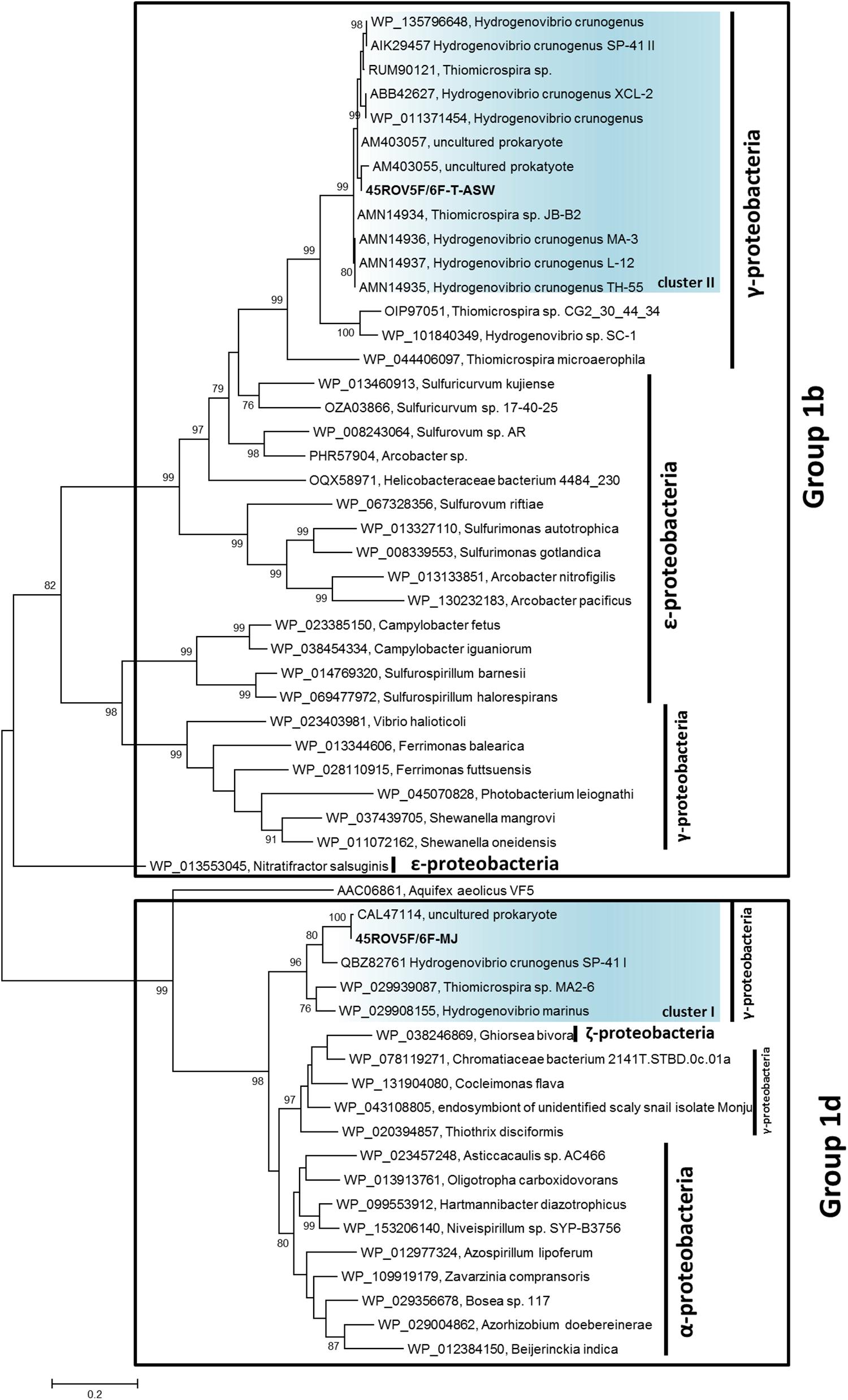
Figure 2. Phylogenetic relationship of Thiomicrospira and Hydrogenovibrio species based on hynL (large subunit of the [NiFe]-hydrogenase) genes. Sequences were aligned using the multiple sequence alignment web server T-Coffee (Notredame et al., 2000) with followed construction of the phylogenetic tree using Mega-X (Kumar et al., 2018) and 1000 bootstraps. The strains described here are written in bold. The scale bar represents the changes per amino acid. Only bootstrap values higher than 75 are given.
Both strains can consume hydrogen (Figures 3, 4). 45ROV5F/6F-TASW uses hydrogen in MJ-medium regardless of whether thiosulfate is present or not suggestive that in any case hydrogen oxidation is utilized as an energy source (Figure 3). In contrast, 45ROV5F/6F-MJ appears to use hydrogen only if hydrogen oxidation is the only available energy source, while hydrogen is not consumed if thiosulfate is present (Figure 4). This indicates that hydrogen oxidation is not the preferred energy source under the provided incubation conditions when thiosulfate is present. Respective hydrogenase transcripts were identified in both strains when grown in hydrogen supplemented MJ-medium (Supplementary Figure 1). This makes it highly likely that the hydrogenases from cluster I and II are responsible for the hydrogen uptake in the cultures, as has been documented for other uptake hydrogenases from vent Hydrogenovibrio strains (Hansen and Perner, 2016a).
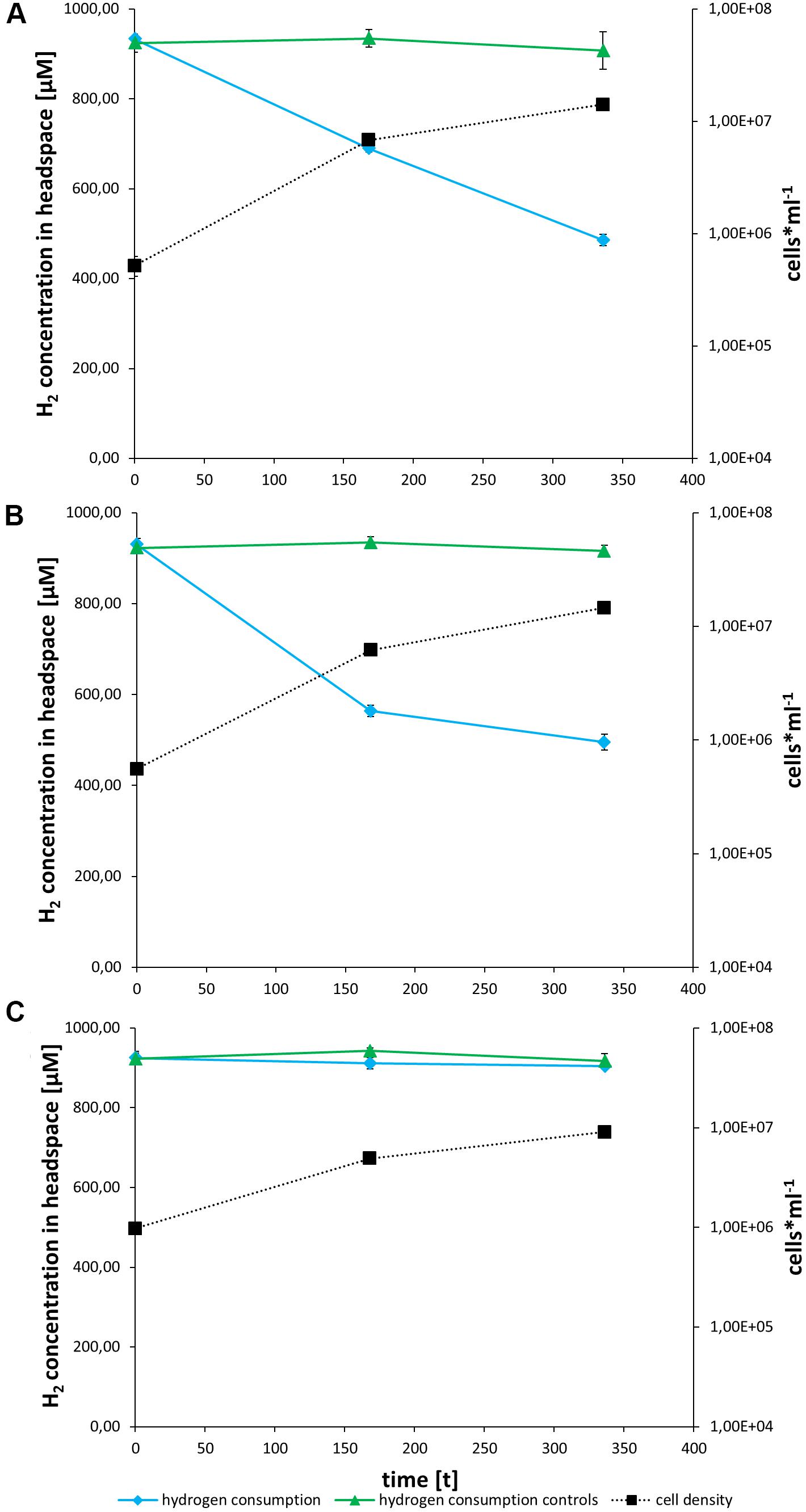
Figure 3. In vivo hydrogen consumption and growth of the T-ASW enrichment culture on MJ (A), MJ-T (B) and T-ASW (C) medium. Hydrogen consumption of the cultures is shown in blue with diamonds, controls containing only the respective medium in green with triangles and cell number of the cultures as dotted line in black with squares. The experiments were performed in triplicate, followed by polymerase chain reaction and sequencing of 16S rRNA and hynL genes (Hansen and Perner, 2015). Hydrogen consumption was determined by using gas chromatography and cell numbers were detected as described before (Hansen and Perner, 2015).
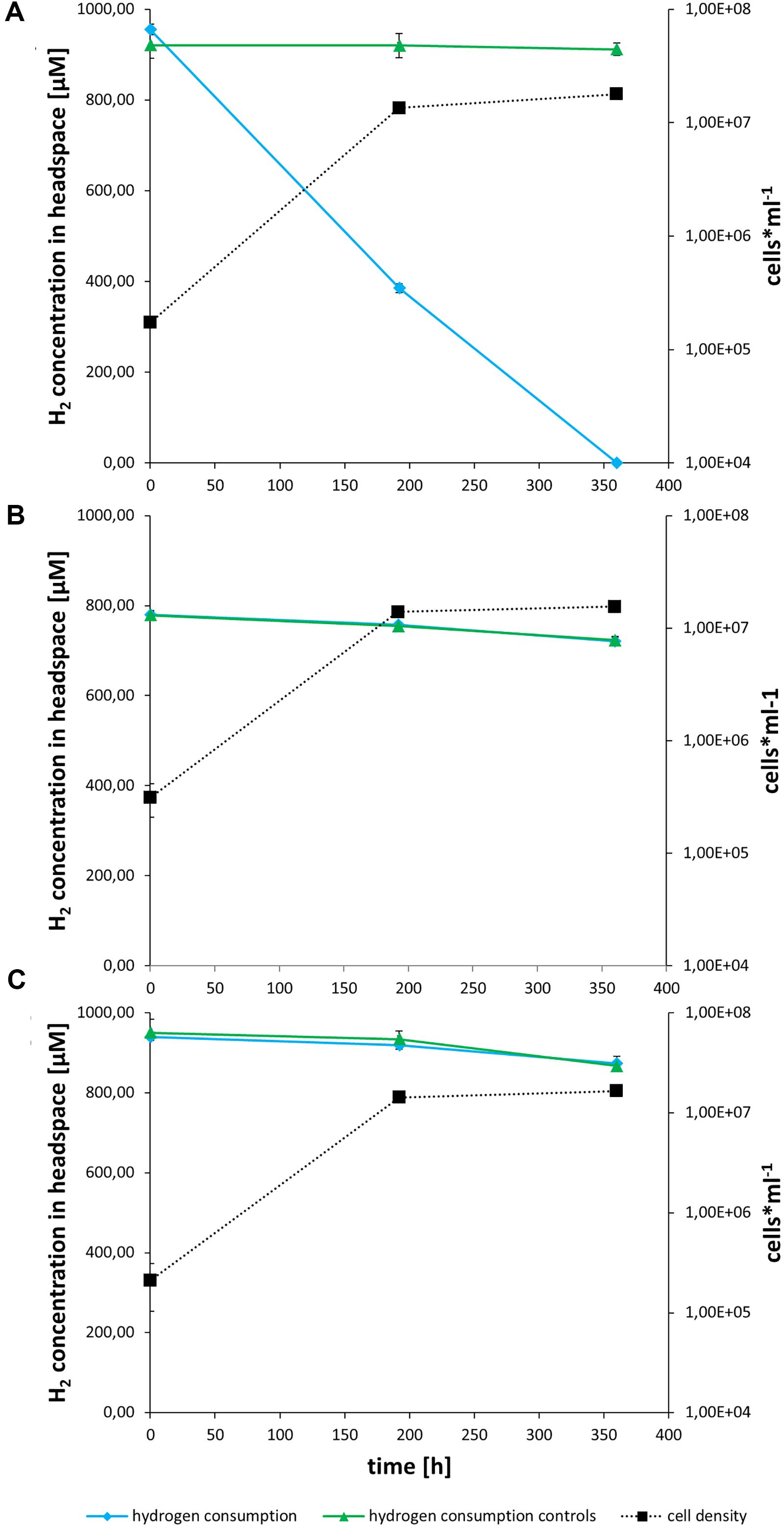
Figure 4. In vivo hydrogen consumption and growth of the MJ enrichment culture on MJ (A), MJ-T (B) and T-ASW (C) medium. Hydrogen consumption of the cultures is shown in blue with diamonds, controls containing only the respective medium in green with triangles and cell number of the cultures as dotted line in black with squares. The experiments were performed in triplicate, followed by polymerase chain reaction and sequencing of 16S rRNA and hynL genes (Hansen and Perner, 2015). Hydrogen consumption was determined by using gas chromatography and cell numbers were detected as described before (Hansen and Perner, 2015).
Expanding the Geographic Range of Hydrogen Oxidizing Vent Hydrogenovibrio Species
We here expand the geographic range of hydrogen oxidizing hydrothermal vent Hydrogenovibrio species by two strains from the Southern Pacific. 45ROV5F/6F-TASW is also the first strain grouping with Hydrogenovibrio crunogenus exhibiting hydrogen uptake ability that is not from a MOR expanding this trait to an Island arc in the Southern Pacific and suggesting that dispersal limitation does not apply for this phenotype in this species. These hydrogenases form a monophyletic clade with those from Epsilonproteobacteria (Figure 2). This supports the previous assumption that hydrogenases in H. crunogenus species were taken up from Epsilonproteobacteria or were acquired from the same bacterium that Epsilonproteobacteria took up this trait from initially (Hansen and Perner, 2016a). Horizontal gene transfer appears to be a common feature in Hydrogenovibrio species (Petri et al., 2001; Scott et al., 2006, 2018).
Hydrogenases of cluster I form a monophyletic clade incorporating H. marinus, which was isolated from the water column (Nishihara et al., 1991) and S5 from a vent along the SWIR, MA2-6 from the MAR and 45ROV5F/6F-MJ from the Kermadec island arc (Figure 2). According to 16S rRNA genes the three vent Hydrogenovibrio species are all affiliated with the described species H. thermophilus (Figure 1). Different scenarios can explain the hydrogenase distribution in cluster I. H. marinus (i) took up its hydrogenase from a vent Hydrogenovibrio, (ii) the vent Hydrogenovibrio took it up from H. marinus or (iii) H. marinus was originally a vent-colonizing organism that emitted with the fluids into the open ocean from which it was essentially isolated. The latter may be supported by the fact that other (endemic) vent organisms have been detected in the open ocean (Gonnella et al., 2016).
It is interesting that all tested hydrogen oxidizing H. crunogenus species have the cluster II and all so far tested hydrogen oxidizing H. thermophilus related species have the cluster I hydrogenases regardless of biogeographic distribution. The only so far known exception is SP-41, which encodes both hydrogenases on its genome (Gonnella et al., 2019). Those from cluster II resemble hydrogenases from hydrothermal vent Epsilonproteobacteria indicating horizontal gene exchange in the respective environment across classes. In contrast, the cluster I hydrogenase clusters with hydrogenases from free-living and symbiotic species associated with hydrothermal vents across different classes including Zetaproteobacteria and Gammaproteobacteria. Hence, at least two events of horizontal gene transfer in the two species groups resulted in the hydrogenase acquisition. Given that H. marinus’ hydrogenase has proven to be extremely oxygen stable (Nishihara et al., 1997), it may transfer a major advantage in a thermally and chemically dynamic vent environment that is influenced by mixing processes of endmember fluids with oxygenated ambient seawater.
This is the first report of Hydrogenovibrio species isolated from deep-sea vents located in the Southern Pacific that express active hydrogenases and can consume hydrogen. We here expand the geographic range of the hydrogen-oxidizing Hydrogenovibrio species. Hydrogenovibrio strains can be abundant in hydrothermal vent habitats and they have been considerably enriched in incubation experiments where hydrogen was amended (Brazelton and Baross, 2010; Perner et al., 2011; Böhnke et al., 2019). For example, they were shown to be among the dominant species in incubations supplemented with hydrogen, where 27 ± 4 nmol H2 ml–1 h–1 was consumed and 0.93 ± 0.1 nmol CO2 ml–1 h–1 was fixed autotrophically. This suggests that in these incubations 15% of the energy gained by hydrogen oxidation could be used for biomass synthesis most likely by Hydrogenovibrio strains. Hydrogenovibrio’s ability to use hydrogen, additionally to reduced sulfur compounds, is a major advantage in these environments because it enhances their competitiveness and furthers their substrate spectrum.
Data Availability Statement
The datasets generated for this study can be found in the NCBI, MN923280, MN923281, MN935466, and MN935467.
Author Contributions
KS collected the samples, performed the experiments and molecular analyses. MP developed the idea and designed the experiments. KS and MP wrote the manuscript.
Funding
This work was granted by the Federal Ministry of Education and Research (BMBF, project 03G0253D), Germany.
Conflict of Interest
The authors declare that the research was conducted in the absence of any commercial or financial relationships that could be construed as a potential conflict of interest.
Acknowledgments
We thank the captain and crew members of the RV Sonne as well as the ROV Quest team (MARUM, University of Bremen) for helping us to obtain the Kermadec samples. Permission to work in the Kermadec Arc by the New Zealand authorities is gratefully acknowledged.
Supplementary Material
The Supplementary Material for this article can be found online at: https://www.frontiersin.org/articles/10.3389/fmars.2020.00295/full#supplementary-material
References
Balch, W. E., Fox, G. E., Magrum, L. J., Woese, C. R., and Wolfe, R. S. (1979). Methanogens: reevaluation of a unique biological group. Microbiol. Rev. 43, 260–296.
Barco, R. A., Hoffman, C. L., Ramírez, G. A., Toner, B. M., Edwards, K. J., and Sylvan, J. B. (2017). In-situ incubation of iron-sulfur mineral reveals a diverse chemolithoautotrophic community and a new biogeochemical role for Thiomicrospira. Environ. Microbiol. 19, 1322–1337. doi: 10.1111/1462-2920.13666
Boden, R., Scott, K. M., Williams, J., Russel, S., Antonen, K., Rae, A. W., et al. (2017). An evaluation of Thiomicrospira, Hydrogenovibrio and Thioalkalimicrobium: reclassification of four species of Thiomicrospira to each Thiomicrorhabdus gen. nov. and Hydrogenovibrio, and reclassification of all four species of Thioalkalimicrobium to Thiomicrospira. Int. J. Syst. Evol. Microbiol. 67, 1140–1151. doi: 10.1099/ijsem.0.001855
Böhnke, S., Sass, K., Gonnella, G., Diehl, A., Kleint, C., Bach, W., et al. (2019). Parameters governing the community structure and element turnover in kermadec volcanic ash and hydrothermal fluids as monitored by inorganic electron donor consumption, autotrophic CO2 fixation and 16S tags of the transcriptome in incubation experiments. Front. Microbiol. 10:2296. doi: 10.3389/fmicb.2019.02296
Brazelton, W. J., and Baross, J. A. (2010). Metagenomic comparison of two Thiomicrospira lineages inhabiting contrasting deep-sea hydrothermal environments. PLoS One 5:e13530. doi: 10.1371/journal.pone.0013530
Brinkhoff, T., and Muyzer, G. (1997). Increased species diversity and extended habitat range of sulfur-oxidizing Thiomicrospira spp. Appl. Environ. Microbiol. 63, 3789–3796.
Dobrinski, K. P., Longo, D. L., and Scott, K. M. (2005). The carbon-concentrating mechanism of the hydrothermal vent chemolithoautotroph Thiomicrospira crunogena. J. Bacteriol. 187, 5761–5766. doi: 10.1128/JB.187.16.5761-5766.2005
Garbe-Schönberg, D., Jähmlich, H., Koschinsky, A., Ratmeyer, V., and Westernstroer, U. (2006). KIPS - A new multiport valve-based all-teflon fluid sampling system for ROVs. Geophys. Res. Abstr. 8:07032.
Gonnella, G., Adam, N., and Perner, M. (2019). Horizontal acquisition of hydrogen conversion ability and other habitat adaptations in the Hydrogenovibrio strains SP-41 and XCL-2. BMC Genomics 20:339. doi: 10.1186/s12864-019-5710-5
Gonnella, G., Böhnke, S., Indenbirken, D., Garbe-Schönberg, D., Seifert, R., Mertens, C., et al. (2016). Endemic hydrothermal vent species identified in the open ocean seed bank. Nat. Microbiol. 1:16086. doi: 10.1038/nmicrobiol.2016.86
Greening, C., Biswas, A., Carere, C. R., Jackson, C. J., Taylor, M. C., Stott, M. B., et al. (2016). Genomic and metagenomic surveys of hydrogenase distribution indicate H2 is a widely utilised energy source for microbial growth and survival. ISME J. 10, 761–777. doi: 10.1038/ismej.2015.153
Hansen, M., and Perner, M. (2015). A novel hydrogen oxidizer amidst the sulfur-oxidizing Thiomicrospira lineage. ISME J. 9, 696–707. doi: 10.1038/ismej.2014.173
Hansen, M., and Perner, M. (2016a). Hydrogenase gene distribution and H2 consumption ability within the Thiomicrospira lineage. Front. Microbiol. 7:99. doi: 10.3389/fmicb.2016.00099
Hansen, M., and Perner, M. (2016b). Reasons for Thiomicrospira’s recalcitrance towards previous attempts to detect its hydrogen consumption ability. Environ. Microbiol. Rep. 8, 53–57. doi: 10.1111/1758-2229.12350
Jannasch, H. W., Wirsen, C. O., Nelson, D. C., and Robertson, L. A. (1985). Thiomicrospira crunogena sp. nov., a colorless, sulfur-oxidizing bacterium from a deep-sea hydrothermal vent. Intern. J. Syst. Bacteriol. 35, 422–424. doi: 10.1099/ijs.0.64255-0
Jiang, L. H., Lyu, J., and Shao, Z. (2017). Sulfur metabolism of Hydrogenovibrio thermophilus strain S5 and its adaptationb to deep-sea hydrothermal vent environment. Front. Microbiol. 8:2513. doi: 10.3389/fmicb.2019.02513
Kumar, S., Stecher, G., Li, M., Knyaz, C., and Tamura, K. (2018). MEGA X: molecular evolutionary genetics analysis across computing platforms. Mol. Biol. Evol. 35, 1547–1549. doi: 10.1093/molbev/msy096
Nishihara, H., Igarashi, Y., and Kodema, T. (1991). Hydrogenovibrio marinus gen. nov., sp. nov. a marine obligately chemolithoautotrophic hydrogen oxidizing bacterium. Intern. J. Syst. Bacteriol. 41, 130–133.
Nishihara, H., Miyashita, Y., Aoyama, K., Kodama, T., Igarashi, Y., and Takamura, Y. (1997). Characterization of an extremely thermophilic and oxygen-stable membrane-bound hydrogenase from a marine hydrogen-oxidizing bacterium Hydrogenovibrio marinus. Biochem. Biophys. Res. Commun. 232, 766–770. doi: 10.1006/bbrc.1997.6369
Notredame, C., Higgins, D. G., and Heringa, J. (2000). T-Coffee: a novel method for fast and accurate multiple sequence alignment. J. Mol. Biol. 302, 205–217. doi: 10.1006/jmbi.2000.4042
Perner, M., Bach, W., Koschinsky, A., Garbe-Schönberg, D., Streit, W. R., and Strauss, H. (2009). Short-term temporal microbial and physico-chemical variability in low-temperature hydrothermal fluids near 5°S on the Mid-Atlantic Ridge. Environ. Microbiol. 11, 2526–2541. doi: 10.1111/j.1462-2920.2009.01978.x
Perner, M., Hentscher, M., Rychlik, N., Seifert, R., Strauss, H., and Bach, W. (2011). Driving forces behind the biotope structures in two low-temperature hydrothermal venting sites on the southern Mid-Atlantic Ridge. Environ. Microbiol. Rep. 3, 727–737. doi: 10.1111/j.1758-2229.2011.00291.x
Perner, M., Seifert, R., Weber, S., Koschinsky, A., Schmidt, K., Strauss, H., et al. (2007). Microbial CO2 fixation and sulfur cycling associated with low-temperature emissions at the Lilliput hydrothermal field, southern Mid-Atlantic Ridge (9°S). Environ. Microbiol. 9, 1186–1201. doi: 10.1111/j.1462-2920.2007.01241.x
Petri, R., Podgorsek, L., and Imhoff, J. F. (2001). Phylogeny and distribution of the soxB gene among thiosulfate-oxidizing bacteria. FEMS MIcrobiol. Lett. 197, 171–178. doi: 10.1111/j.1574-6968.2001.tb10600.x
Ruby, E. G., and Jannasch, H. W. (1982). Physiological-characteristics of Thiomicrospira sp strain L-12 isolated from deep-sea hydrothermal vents. J. Bacteriol. 149, 161–165.
Sako, Y., Takai, K., Ishida, Y., Uchida, A., and Katayama, Y. (1996). Rhodothermus obamensis sp. nov., a modern lineage of extremely thermophilic bacteria. Intern. J. Syst. Bacteriol. 46, 1099–1104. doi: 10.1099/00207713-46-4-1099
Scott, K. M., Sievert, S. M., Abril, F. N., Ball, L. A., Barrett, C. J., Blake, R. A., et al. (2006). The genome of deep-sea vent chemolithoautotroph Thiomicrospira crunogena XCL-2. PLoS Biol. 4:383. doi: 10.1371/journal.pbio.0040383
Scott, K. M., Williams, J., Porter, C. M. B., Russel, S., Harmer, T. L., Paul, J. H., et al. (2018). Genomes of ubiquitous marine and hypersaline Hydrogenovibrio, Thiomicrorhabdus and Thiomicrospira spp. encode a diversity of mechanisms to sustain chemolithoautotrophy in heterogeneous environments. Environ. Microbiol. 20, 2686–2708. doi: 10.1111/1462-2920.14090
Takai, K., Hirayama, H., Nakagawa, T., Suzuki, Y., Nealson, K. H., and Horikoshi, K. (2004). Thiomicrospira thermophila sp. nov., a novel microaerobic, thermotolerant, sulfur-oxidizing chemolithomixotroph isolated from a deep-sea hydrothermal fumarole in the TOTO caldera, Mariana Arc, Western Pacific. Intern. J. Syst. Evol. Microbiol. 54, 2325–2333.
Vignais, P. M., and Billoud, B. (2007). Occurrence, classification, and biological function of hydrogenases: an overview. Chem. Rev. 107, 4206–4272. doi: 10.1021/cr050196r
Keywords: Hydrogenovibrio, [NiFe]-hydrogenase, geographic range, microbial hydrogen oxidation, Kermadec Arc
Citation: Sass K and Perner M (2020) Characterization of Two Hydrogen-Oxidizing Hydrogenovibrio Strains From Kermadec Volcanic Island Arc Hydrothermal Vents. Front. Mar. Sci. 7:295. doi: 10.3389/fmars.2020.00295
Received: 05 February 2020; Accepted: 14 April 2020;
Published: 19 May 2020.
Edited by:
Daphne Cuvelier, Center for Marine and Environmental Sciences (MARE), PortugalReviewed by:
William J. Brazelton, The University of Utah, United StatesKathleen Scott, University of South Florida, United States
Copyright © 2020 Sass and Perner. This is an open-access article distributed under the terms of the Creative Commons Attribution License (CC BY). The use, distribution or reproduction in other forums is permitted, provided the original author(s) and the copyright owner(s) are credited and that the original publication in this journal is cited, in accordance with accepted academic practice. No use, distribution or reproduction is permitted which does not comply with these terms.
*Correspondence: Mirjam Perner, bXBlcm5lckBnZW9tYXIuZGU=
†Present address: Mirjam Perner, Geomicrobiology, GEOMAR Helmholtz Centre for Ocean Research Kiel, Kiel, Germany