- Southern Seas Ecology Laboratories and the Environment Institute, School of Biological Sciences, The University of Adelaide, Adelaide, SA, Australia
Climatic effects on the growth of apex marine predators – such as sharks – are poorly understood; moreover, shifts in shark growth are primarily attributed to fishing pressure. This paucity of information impedes management and conservation planning for these taxa. Using vertebral increment patterning as a proxy of somatic growth, this study reconstructed mean growth of the philopatric and demersal Heterodontus portusjacksoni population from Gulf St Vincent (South Australia). A biochronology of shark growth spanning a 15 year period (1996–2010) was developed using mixed effects models. The biochronology showed considerable year-to-year deviations in growth that were significantly and negatively correlated with mean sea surface temperatures during the species’ breeding season (July to November). These findings are consistent with mesocosm experiments and support the influence of changing climates on shark growth; particularly in an inshore, demersal, and highly philopatric shark species. It is likely that the effects of environmental variation occur in a species-specific manner, governed by life history strategies and ecological requirements. In this manner, life history traits might aid in estimating species vulnerability to climate change.
Introduction
Environmental controls on the phenology and biology of terrestrial and aquatic taxa are well established (Parmesan, 2006). Key biological processes, such as growth, have been linked to temperature, with fluctuations in external conditions correlated to variations in growth. This is well-established in teleosts; with particular interest in climate change effects (Morrongiello et al., 2012). However, in top order predators such as sharks and marine mammals, there is little investigation of environmental controls on growth due to logistical constraints of controlled experimental studies and long-term observational work (Wittmann et al., 2016). Moreover, shifts in shark growth are primarily attributed to fishing effects (Walker et al., 1998), resulting in a paucity of information on the environmental correlates of growth in top order aquatic taxa.
Biochronologies provide a means of measuring growth deviations though time, using the incremental patterns of calcified structures as proxies of somatic growth (Morrongiello et al., 2012). For example, biochronologies of growth, based on tooth incremental patterns, have demonstrated growth-temperature relationships in New Zealand fur seals (Wittmann et al., 2016). Shark vertebrae, possess analogous incremental growth patterns and are widely used to estimate chronological age (Cailliet and Goldman, 2004), with vertebral increments potentially providing suitable proxies of somatic shark growth when allometry is validated.
Biochronologies developed using mixed effects models allows assessment of the simultaneous effects of biological, environmental and temporal factors on population growth (Morrongiello et al., 2012). This approach enables quantification of growth responses to environmental fluctuation and/or management action (reduced fishing effort); while accounting for biological effects (age-/sex-based growth). This is advantageous compared to previous approaches for assessing temporal variation in shark growth, which are largely limited to comparisons of growth (von Bertalanffy) functions (Parsons, 1993) or length-at-age dynamics (Walker et al., 1998) across multiple sampling periods; thus prone to study-level confounding factors and failing to account for potentially influential covariates.
This study develops a biochronology of growth deviations of the Port Jackson shark subpopulation from Gulf St Vincent, using mixed effects models of vertebrae growth. Port Jackson sharks (Heterodontus portusjacksoni) provide an excellent shark species for biochronology development. This oviparous species is highly philopatric within its temperate southern Australian distribution, returning to natal sites to breed in the austral winter after migrating (Powter and Gladstone, 2008a; Powter and Gladstone, 2009). The extreme site fidelity of the species ensures that it does not alter its distribution even when exposed to unfavorable environmental conditions, and thus climate variation should be reflected in population growth. Moreover, there is no direct fishing for Port Jackson sharks and negligible fishing mortality as most sharks caught are discarded (Powter and Gladstone, 2008a; Tovar-Ávila et al., 2010); therefore, we assume that observed deviations in growth are primarily influenced by environmental processes. To test this assumption, we related year-to-year growth deviations to ecologically relevant periods of sea surface temperature data to explore the influence of climatic variation on growth in situ.
Materials and Methods
Sharks (n = 50) were collected from the bycatch of commercial prawn trawlers operating in Gulf St Vincent (South Australia) between 2006 and 2011 (Figure 1). Gulf St Vincent is an inverse estuary that is largely sheltered from oceanic waves. Demographic data and tagging indicates that Port Jackson sharks inhabiting Gulf St Vincent and the adjacent Spencer Gulf form discrete subpopulations (Rodda and Svane, 2007; Izzo and Rodda, 2012).
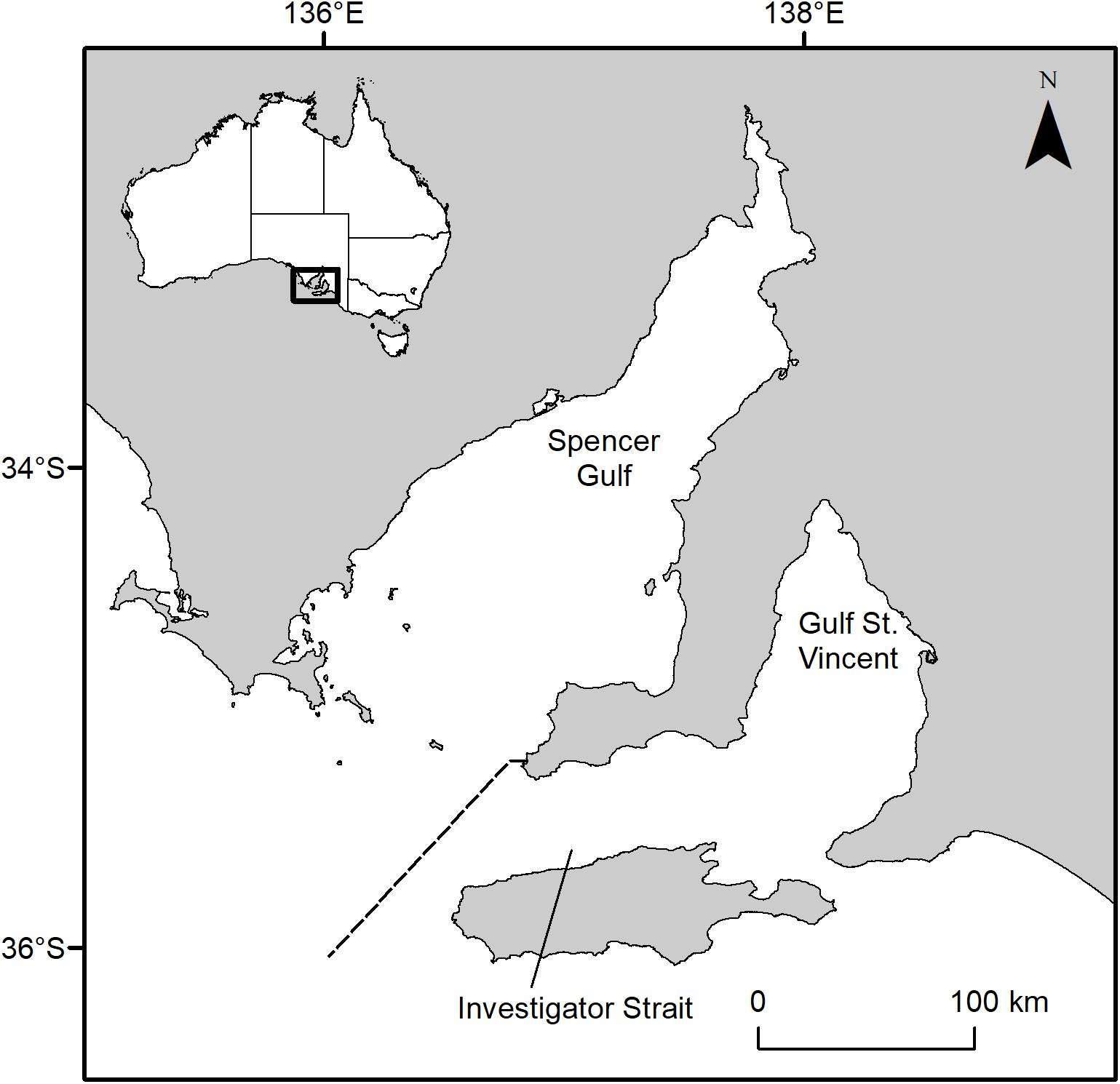
Figure 1. Map of South Australia’s gulf systems. The dashed line separates Spencer Gulf and Gulf St Vincent. Inset, map of Australia showing the study area along the southern coastline.
Shark total length (in mm) and sex were recorded, and a section (n = 8–12) of vertebrae collected from under the first dorsal fin, were prepared for age determination using standard protocols (Izzo and Rodda, 2012). Vertebral centrum diameter was measured (in mm). Briefly, individual vertebra were cleaned of adhering tissue, dried and then embedded in epoxy resin to enable a transverse section (thickness = ∼500 μm) to be cut.
Growth increments (paired opaque and translucent bands) were counted, and widths measured under a dissecting microscope with transmitted light and image analysis software. Port Jackson shark vertebrae possess clear growth increments, and chemical marking has been used to verify that growth increments form annually (Tovar-Ávila et al., 2009; Izzo and Rodda, 2012), enabling the temporal resolution of shark growth to be validated.
Growth increments were assigned a year relative to the date of capture. Marginal increment and pre-natal vertebral growth were excluded from biochronology development as they may not represent a complete annual growth cycle, defined as January to December, based on a January hatch date (Rodda and Seymour, 2008).
Biochronologies, quantifying year-to-year growth deviations, were developed using linear mixed effects models (following Weisberg et al., 2010; Morrongiello and Thresher, 2015). Increment widths were log-transformed to meet model assumptions, with all continuous parameters mean centered to facilitate model convergence (Morrongiello and Thresher, 2015). Model fitting was undertaken in R (R Core Team, 2018), using the lme4 package (Bates et al., 2013).
The random effects model structure was optimized by comparing a series of models with all combinations of the random terms that included: Year, Cohort, and SharkID (see Table 1 for a description of the model terms). In all models, the SharkID term was fit with a random Age slope, enabling each shark to have its own age correction term, negating potential inter-individual differences that may arise from examining different centra in the vertebral column. Models were ranked using Akaike’s Information Criterion corrected for small sample sizes (AICc) (Burnham and Anderson, 2004), using the MuMIn package (Bartoń, 2013; Supplementary Table S1). Model parameters were estimated using restricted maximum likelihood (REML).
The fixed effects structure was optimized by comparing a series of models that contained the optimized random effects structure (see above) and all combinations of the fixed covariates that included: Sex and Age-at-capture (see Table 1). All models contained a fixed Age term to account for ontogenetic variation in increment widths. Models were ranked using AICc (Supplementary Table S1). Comparisons among models with differing fixed effects terms were fitted using maximum likelihood, with the best ranked model re-fitted using REML (Zuur et al., 2009). Random Year effects representing the year-to-year deviations in population growth (the biochronology) were extracted from the model containing the optimized random and fixed effects structure.
The influence of sea surface temperature (SST in°C) on shark growth was assessed by relating temperature over three ecological periods: (i) annual growth cycle (January–December); (ii) austral winter to spring (July–November), coinciding with the breeding season (Powter and Gladstone, 2008b); and (iii) austral summer (December–February), coinciding with the timing of neonate hatching (Rodda and Seymour, 2008). Daily SST data (from –34.234, 138.078: see Supplementary Table S2) were averaged over the three ecological time periods. Temperature data for the three periods were correlated against the random Year effects (the year-to-year growth deviations) from the growth biochronology.
Results
Increments were measured on vertebrae of 50 sharks (female = 24; male = 26) ranging from 2 to 14 years of age (Figure 2A) and 207 to 794 mm TL (Figure 2B). In total, 185 increments were measured (Figure 2C). Centrum diameter was significantly correlated to total length (Figure 3), confirming that Port Jackson shark vertebrae provide suitable proxies of somatic growth, developing in proportion to total length (Figure 3).
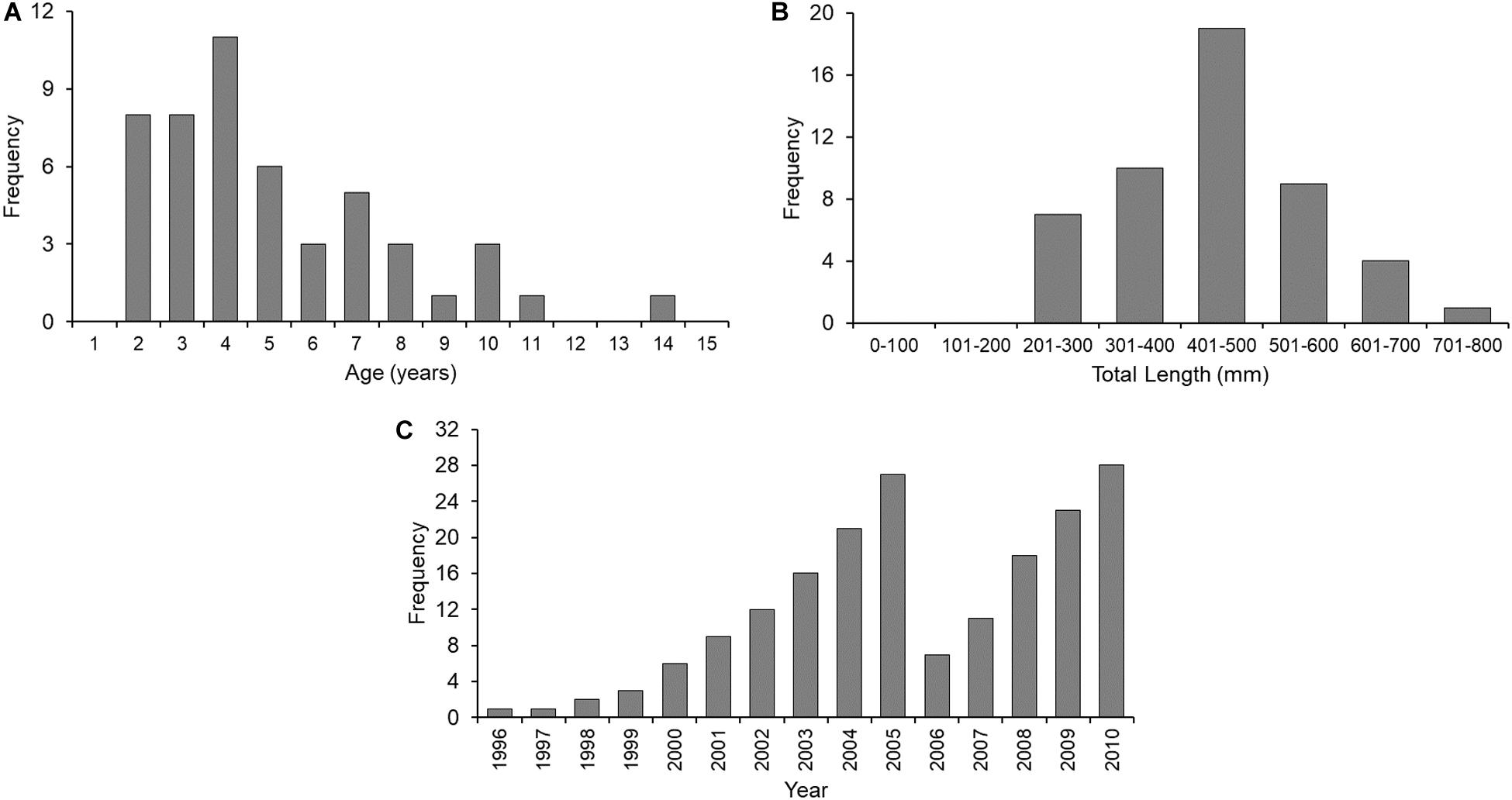
Figure 2. Absolute frequency plots of (A) age (in years), (B) total length (in mm), and (C) increment measurements (per year), of Port Jackson shark samples examined in this study (n = 50 sharks).
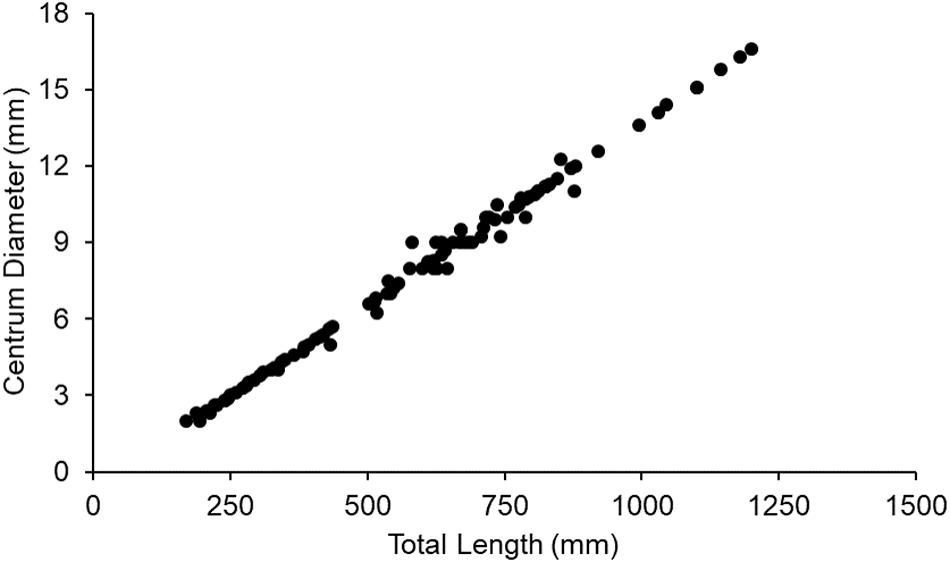
Figure 3. Linear relationship between Port Jackson shark vertebral centrum diameter and total length (r = 0.99).
The shark biochronology spanned a 15 year period, between 1996 and 2010 (Figure 4) and showed considerable deviations in year-to-year growth based on the Year term (Table 1), with an extended period of above average growth between 2001 and 2004. The SharkID term suggested a large degree of inter-individual growth differences; with the correlation statistic indicating increasing shark growth with increasing age (Table 1). Neither specimen Sex nor Age-at-capture influenced shark growth (Supplementary Table S1).
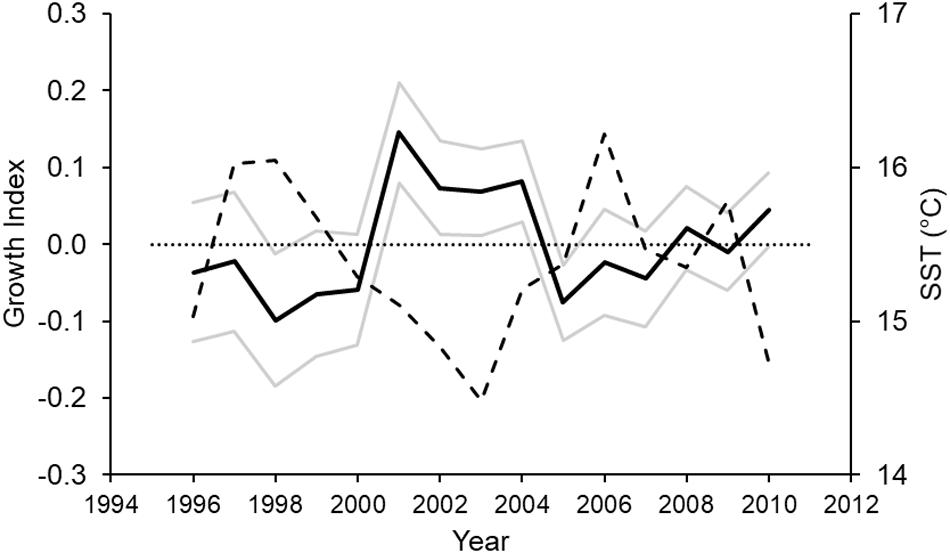
Figure 4. Biochronology of Port Jackson shark growth variation (a unitless index; solid black line) around the population mean (dotted line) for the duration of the period studied (model intercept). Standard errors are shown as solid gray lines. The dashed line is the mean sea surface temperature (in°C) over the breeding season (austral winter to spring) of the species.
Mean SST for the breeding season (austral winter to spring) was significantly and negatively correlated to shark growth (p = 0.020, r = –0.59) (Table 2). Mean annual SST had a similar negative influence on shark growth (r = –0.50). Water temperature had a negative effect on shark growth over the temperature conditions experienced. Conversely, mean summer SST had a weak positive correlation with growth (r = 0.18) (Table 2).

Table 2. Correlation among Port Jackson shark Growth (the extracted random Year effects from the mixed effects growth model) and sea surface temperature over three ecological periods: (i) Annual growth cycle (January–December); (ii) Breeding season (July–November); and (iii) Summer (December–February).
Discussion
This study demonstrates that in the absence of targeted fishing pressure, shark growth fluctuates through time; with Port Jackson shark population growth negatively correlated with sea surface temperature over a 15 year period (between 1996 and 2010). This temperature effect was most prevalent in the species’ winter/spring breeding period, whereby the species undergoes extended periods of residency on natal sites and are unlikely to actively regulate their external environment (i.e., through migration) (O’Gower, 1995; Powter and Gladstone, 2009). This study focused on an inshore, demersal and highly philopatric shark species, traits that while assumed to make the Port Jackson sharks conducive to developing biochronologies; likely render the species highly susceptible to localized climatic change. Conversely highly mobile, pelagic species may be better suited to actively mitigate the impacts of environmental fluctuation by undertaking range shifts to habitats with preferable environmental conditions (Last et al., 2011). It is likely that the effects of environmental variation on sharks (and elasmobranchs more generally) occur in a species-specific manner, governed by individual life history strategies and ecological requirements (Izzo et al., 2016). Thus, species life history traits might aid in assessing the vulnerability of elasmobranchs to climate change (Chin et al., 2010). However, intraspecific differences in growth (and metabolic performance) of subpopulations exposed to different local environmental conditions suggest elasmobranchs have the potential to demonstrate local adaptation to climatic stressors (Frisk and Miller, 2006; Di Santo, 2016).
Temperature was significantly and negatively related to Port Jackson shark growth. These findings are consistent with decreased growth reported for juvenile Port Jackson sharks held in laboratory and mesocosm experiments (Rodda, 2000; Pistevos et al., 2015) and empirical studies of global marine fish growth trends (Cheung et al., 2012) whereby, when faced with increasing water temperature, growth is reduced and fish attain smaller maximum sizes. The observed negative correlation between temperature and growth aligns with patterns of increasing growth with increasing latitudes reported in elasmobranch species (e.g., Lombari-Carlson et al., 2003; Frisk and Miller, 2006; Winton et al., 2014). This potentially reflects the species need to increase growth rates to account for shorter periods of optimal environmental conditions (Lombari-Carlson et al., 2003) consistent with a counter-gradient variation of growth rates (Conover and Present, 1990). It is anticipated that under forecast climate scenarios, species are likely to be increasingly exposed to periods of suboptimal local environmental conditions, which in turn exacerbates the frequency and magnitude of variability in population growth (Neuheimer et al., 2011; Martino et al., 2019). More broadly, these findings provide indirect support of Bergmann’s Rule in poikilotherms (Conover, 1990; Meiri, 2011), and suggest that in the absence of genetic variability within the Gulf St Vincent Port Jackson shark subpopulation (Izzo, unpublished data), population growth is primarily influenced by local environmental factors (Lombari-Carlson et al., 2003).
While this study explored the relationship between temperature and shark growth in isolation, experiments demonstrate that multiple parameters interact to affect shark growth; e.g., temperature and ocean acidification (Di Santo, 2015; Pistevos et al., 2015). Therefore, as species approach physiological temperature thresholds, growth will likely also be influenced by synergistic ocean acidification effects (Pistevos et al., 2015) and shifts in other biological and phenotypic processes; e.g., metabolism, reproductive timing, and/or juvenile survival (e.g., Rosa et al., 2014; Di Santo, 2015, 2016; Rosa et al., 2017). Given the logistical challenges of working with elasmobranchs in situ, few of these biological parameters are captured and thus, linkages between changing environmental conditions and biological processes are not well understood. Extrinsic influences on shark growth are highly complex, and when considered in conjunction with the effects of fishing (which may alter a population’s phenotypic and/or genotypic composition: Walker et al., 1998), it is likely that a myriad of simultaneous factors may act upon the population to alter resilience through time.
The growth modeling did not identify an age cohort effect on population growth, suggesting rates of growth among year classes of Port Jackson sharks did not differ considerably. However, juvenile survival in oviparous (egg-laying) or viviparous (live-bearing) elasmobranch species has been shown to be adversely impacted under increased water temperatures and, or acidified ocean conditions (Rosa et al., 2014; Di Santo, 2015; Johnson et al., 2016). Nevertheless, the influence of external factors on year class strength may be explored using the approach employed in this study.
Port Jackson sharks are commonly encountered as a bycatch species throughout their southern Australian distribution (Powter and Gladstone, 2008a; Jones et al., 2008; Tovar-Ávila et al., 2010; Izzo and Rodda, 2012), however, the species is seldom retained, is highly robust, and not prone to post-capture mortality (Frick et al., 2010). This study assumed negligible fishing induced mortality in the Port Jackson shark population in Gulf St Vincent, and hence deviations in population growth were primarily attributed to environmental factors. However, targeted fishing pressure has been attributed to shifts in shark population growth (Lombari-Carlson et al., 2003) and length-at-age dynamics (Walker et al., 1998), as well as other broader ecological processes (Stevens et al., 2000). Biochronologies provide a means to explore the influence of fishing on population growth (e.g., Martino et al., 2019).
The model optimization process indicated that the age at capture term was not an important explanatory growth term, inferring the absence of an age trend in the growth data. We also interpreted this as confirmation of the absence of an age-bias in the sample data (Morrongiello and Thresher, 2015). Yet we acknowledge that the samples were predominately small to medium (juvenile/immature) individuals, as large adults are infrequently encountered in Gulf St Vincent (Izzo and Rodda, 2012). This impeded our ability to explore relative growth responses across all Port Jackson shark life history stages through the omission of potential growth phenotypes; and therefore, future studies should seek to access all life stages to assess the potential for differing growth responses throughout development (Morrongiello et al., 2012). Nevertheless, the biochronology approach employed here is advantageous, as it facilitates the examination of sharks from all life stages without requiring logistically challenging controlled experiments on large animals to be undertaken.
This study has demonstrated that in a demersal shark that encounters indirect fishing pressure and negligible fishing mortality, population growth is negatively related to sea surface temperature, consistent with mesocosm experiments. The direct consequences of this relationship are unknown, however, it is expected to result in a shift in the species phenology. Further studies are required to explore growth-temperature relationships in elasmobranch species with varying life history strategies and exposed to varying degrees of fishing pressure to understand the generality of the trends seen here. The mixed effects modeling approach used here provides a means of exploring elasmobranch growth time series, and might be expanded upon to explore how multiple environmental and, or biological parameters interact to influence shark growth. While this study explored the relationship between temperature and Port Jackson shark growth in isolation, future studies that include synergistic relationships among a suite of simultaneously interacting factors will better reflect natural conditions.
Data Availability Statement
The datasets generated for this study are available on request to the corresponding author.
Ethics Statement
Sample collection and processing was reviewed and approved by The University of Adelaide Animal Ethics Committee (S-022-2006).
Author Contributions
CI and BG conceived, designed the study, and wrote the manuscript. CI collected and prepared the samples, collected and analyzed the data, and applied the statistical analyses.
Funding
Funding provided by the Sea World Research and Rescue Foundation Inc. (SWR/8/2007), awarded to CI.
Conflict of Interest
The authors declare that the research was conducted in the absence of any commercial or financial relationships that could be construed as a potential conflict of interest.
Acknowledgments
We thank J. Queiroz for assistance with sample preparation and D. Matthews for providing the map. We thank the crews of the numerous prawn trawlers that assisted in sample collection. We also thank the two reviewers for providing insightful comments which improved the manuscript.
Supplementary Material
The Supplementary Material for this article can be found online at: https://www.frontiersin.org/articles/10.3389/fmars.2020.00240/full#supplementary-material
References
Bartoń, K. (2013). MuMIn: Multi-Model Inference. R Package Version 1.9.13. Avaliable at: http://CRAN.R-project.org/package=MuMIn/. (accessed December20, 2018)Google Scholar
Bates, D., Maechler, M., Bolker, B., and Walker, S. (2013). lme4: Linear Mixed-Effects Models Using Eigen and s4. Vienna: The R Foundation for Statistical Computing.
Burnham, K. P., and Anderson, D. R. (2004). Multimodel inference: understanding AIC and BIC in model selection. Sociol. Methods Res. 33, 261–304. doi: 10.1177/0049124104268644
Cailliet, G. M., and Goldman, K. J. (2004). “Age determination and validation in chondrichthyan fishes,” in Biology of Sharks and their Relatives, eds J. Carrier, J. A. Musick, and M. Heithaus (San Diego, CA: CRC Press), 399–439.
Cheung, W. W. L., Sarmiento, J. L., Dunne, J., Frölicher, T. L., Lam, V. W. Y., Deng Palomares, M. L., et al. (2012). Shrinking of fishes exacerbates impacts of global ocean changes on marine ecosystems. Nat. Clim. Change 3, 254–258. doi: 10.1038/nclimate1691
Chin, A., Kyne, P. M., Walker, T. I., and McAuley, R. B. (2010). An integrated risk assessment for climate change: analysing the vulnerability of sharks and rays on Australia’s Great Barrier Reef. Global Change Biol. 16, 1936–1953. doi: 10.1111/j.1365-2486.2009.02128.x
Conover, D. O. (1990). The relation between capacity for growth and length of growing season: evidence for and implications of countergradient variation. Trans. Am. Fish. Soc. 119, 416–430. doi: 10.1577/1548-8659(1990)119<0416:trbcfg>2.3.co;2
Conover, D. O., and Present, T. M. C. (1990). Countergradient variation in growth rate: compensation for length of the growing season among Atlantic silversides from different latitudes. Oecologia 83, 316–324. doi: 10.1007/BF00317554
Di Santo, V. (2015). Ocean acidification exacerbates the impacts of global warming on embryonic little skate, Leucoraja erinacea (Mitchill). J. Exp. Mar. Biol. Ecol. 463, 72–78. doi: 10.1016/j.jembe.2014.11.006
Di Santo, V. (2016). Intraspecific variation in physiological performance of a benthic elasmobranch challenged by ocean acidification and warming. J. Exp. Biol. 219, 1725–1733. doi: 10.1242/jeb.139204
Frick, L. H., Reina, R. D., and Walker, T. I. (2010). Stress related physiological changes and post-release survival of Port Jackson sharks (Heterodontus portusjacksoni) and gummy sharks (Mustelus antarcticus) following gill-net and longline capture in captivity. J. Exp. Mar. Biol. Ecol. 385, 29–37. doi: 10.1016/j.jembe.2010.01.013
Frisk, M. G., and Miller, T. J. (2006). Age, growth, and latitudinal patterns of two Rajidae species in the northwestern Atlantic: little skate (Leucoraja erinacea) and winter skate (Leucoraja ocellata). Can. J. Fish. Aqu. Sci. 63, 1078–1091. doi: 10.1139/f06-005
Izzo, C., Doubleday, Z. A., Grammer, G. L., Barnes, T. C., Delean, S., Ferguson, G. J., et al. (2016). Multi-species response to rapid environmental change in a large estuary system: a biochronological approach. Ecol. Indic. 69, 739–748. doi: 10.1016/j.ecolind.2016.05.019
Izzo, C., and Rodda, K. R. (2012). Comparative rates of growth of the Port Jackson shark throughout its southern Australian range. Mar. Freshw. Res. 63, 687–694.
Johnson, M. S., Kraver, D. W., Renshaw, G. M. C., and Rummer, J. L. (2016). Will ocean acidification affect the early ontogeny of a tropical oviparous elasmobranch (Hemiscyllium ocellatum)? Conserv. Physiol. 4:cow003. doi: 10.1093/conphys/cow003
Jones, A. A., Hall, N. G., and Potter, I. C. (2008). Size compositions and reproductive biology of an important bycatch shark species (Heterodontus portusjacksoni) in south-western Australian waters. J. Mar. Biol. Assoc. U. K. 88, 189–197. doi: 10.1017/s0025315408000209
Last, P. R., White, W. T., Gledhill, D. C., Hobday, A. J., Brown, R., Edgar, G. J., et al. (2011). Long-term shifts in abundance and distribution of a temperate fish fauna: a response to climate change and fishing practices. Global Ecol. Biogeogr. 20, 58–72. doi: 10.1111/j.1466-8238.2010.00575.x
Lombari-Carlson, L. A., Cortés, E., Parsons, G. R., and Manire, C. A. (2003). Latitudinal variation in life-history traits of bonnethead sharks, Sphyrna tiburo, (Carcharhiniformes: Sphyrnide) from the eastern Gulf of Mexico. Mar. Freshw. Res. 54, 875–883.
Martino, J. C., Fowler, A. J., Doubleday, Z. A., Grammer, G. L., and Gillanders, B. M. (2019). Using otolith chronologies to understand long-term trends and extrinsic drivers of growth in fisheries. Ecosphere 10:e02553.
Morrongiello, J. R., and Thresher, R. E. (2015). A statistical framework to explore ontogenetic growth variation among individuals and populations: a marine fish example. Ecol. Monogr. 85, 93–115. doi: 10.1890/13-2355.1
Morrongiello, J. R., Thresher, R. E., and Smith, D. C. (2012). Aquatic biochronologies and climate change. Nat. Clim. Change 2, 849–857. doi: 10.1038/nclimate1616
Neuheimer, A., Thresher, R., Lyle, J., and Semmens, J. (2011). Tolerance limit for fish growth exceeded by warming waters. Nat. Clim. Change 1, 110–113. doi: 10.1038/nclimate1084
O’Gower, A. K. (1995). Speculations on a spatial memory for the Port Jackson shark (Heterodontus portusjacksoni) (Meyer) (Heterodontidae). Mar. Freshw. Res. 46, 861–871.
Parmesan, C. (2006). Ecological and evolutionary responses to recent climate change. Annu. Rev. Ecol. Evol. Syst. 37, 637–669. doi: 10.1146/annurev.ecolsys.37.091305.110100
Parsons, G. R. (1993). Age determination and growth of the bonnethead shark Sphyrna tiburo: a comparison of two populations. Mar. Biol. 117, 23–31. doi: 10.1007/bf00346422
Pistevos, J. C. A., Nagelkerken, I., Rossi, T., Olmos, M., and Connell, S. D. (2015). Ocean acidification and global warming impair shark hunting behaviour and growth. Sci. Rep. 5:16293. doi: 10.1038/srep16293
Powter, D. M., and Gladstone, W. (2008a). Demographic analysis of the Port Jackson shark Heterodontus portusjacksoni in the coastal waters of eastern Australia. Mar. Freshw. Res. 59, 444–455.
Powter, D., and Gladstone, W. (2008b). Embryonic mortality and predation on egg capsules of the port jackson shark Heterodontus portusjacksoni (Meyer). J. Fish Biol. 72, 573–584. doi: 10.1111/j.1095-8649.2007.01721.x
Powter, D. M., and Gladstone, W. (2009). Habitat-mediated use of space by juvenile and mating adult Port Jackson sharks. Heterodontus portusjacksoni, in Eastern Australia. Pacific Sci. 63, 1–14. doi: 10.2984/1534-6188(2009)63
R Core Team (2018). R: A Language and Environment for Statistical Computing, v3.5, 1st Edn. Vienna: R Foundation for Statistical Computing.
Rodda, K. (2000). Development in the Port Jackson Shark Embryo. Adelaide, SA: University of Adelaide.
Rodda, K., and Svane, I. (2007). “Tagging and movement in spencer gulf,” in Prawn Fishery By-Catch and Discards: Marine Ecosystem Analysis - Population Effects. FRDC Project No. 2003/023, eds I. Svane, K. Rodda, and P. Thomas (Adelaide: SARDI Aquatic Sciences Research Report Series; no. 199), 89–111.
Rodda, K. R., and Seymour, R. S. (2008). Functional morphology of embryonic development in the Port Jackson shark Heterodontus portusjacksoni (Meyer). J. Fish Biol. 72, 961–984. doi: 10.1111/j.1095-8649.2007.01777.x
Rosa, R., Baptista, M., Lopes Vanessa, M., Pegado Maria, R., Ricardo Paula, J., Trübenbach, K., et al. (2014). Early-life exposure to climate change impairs tropical shark survival. Proc. R. Soc. B Biol. Sci. 281, 20141738. doi: 10.1098/rspb.2014.1738
Rosa, R., Rummer, J. L., and Munday, P. L. (2017). Biological responses of sharks to ocean acidification. Biol. Lett. 13:20160796. doi: 10.1098/rsbl.2016.0796
Stevens, J. D., Bonfil, R., Dulvy, N. K., and Walker, P. A. (2000). The effects of fishing on sharks, rays, and chimaeras (chondrichthyans), and the implications for marine ecosystems. J. Mar. Sci. 57, 476–494. doi: 10.1006/jmsc.2000.0724
Tovar-Ávila, J., Day, R. W., and Walker, T. I. (2010). Using rapid assessment and demographic methods to evaluate the effects of fishing on Heterodontus portusjacksoni off far-eastern Victoria. Austr. J. Fish Biol. 77, 1564–1578. doi: 10.1111/j.1095-8649.2010.02788.x
Tovar-Ávila, J., Izzo, C., Walker, T. I., Braccini, J. M., and Day, R. W. (2009). Assessing growth band counts from vertebrae and dorsal-fin spines for ageing sharks: comparison of four methods applied to Heterodontus portusjacksoni. Mar. Freshw. Res. 60, 898–903.
Walker, T. I., Taylor, B. L., Hudson, R. J., and Cottier, J. P. (1998). The phenomenon of apparent change of growth rate in gummy shark (Mustelus antarcticus) harvested off southern Australia. Fish. Res. 39, 139–163. doi: 10.1016/s0165-7836(98)00180-5
Weisberg, S., Spangler, G., and Richmond, L. S. (2010). Mixed effects models for fish growth. Can. J. Fish. Aqu. Sci. 67, 269–277. doi: 10.1139/f09-181
Winton, M. V., Natanson, L. J., Kneebone, J., Cailliet, G. M., and Ebert, D. A. (2014). Life history of Bathyraja trachura from the eastern Bering Sea, with evidence of latitudinal variation in a deep-sea skate species. Mar. Biol. Assoc. U. K. J. Mar. Biol. Assoc. U. K. 94, 411. doi: 10.1017/s0025315413001525
Wittmann, T. A., Izzo, C., Doubleday, Z. A., McKenzie, J., Delean, S., and Gillanders, B. M. (2016). Reconstructing climate–growth relations from the teeth of a marine mammal. Mar. Biol. 163, 1–11.
Keywords: climate change, growth, mixed effects modeling, phenology, Port Jackson shark
Citation: Izzo C and Gillanders BM (2020) Port Jackson Shark Growth Is Sensitive to Temperature Change. Front. Mar. Sci. 7:240. doi: 10.3389/fmars.2020.00240
Received: 08 December 2019; Accepted: 27 March 2020;
Published: 21 April 2020.
Edited by:
Esteban Avigliano, National Council for Scientific and Technical Research (CONICET), ArgentinaReviewed by:
André Martins Vaz-dos-Santos, Federal University of Paraná, BrazilGustavo Enrique Chiaramonte, Museo Argentino de Ciencias Naturales “Bernardino Rivadavia”, Argentina
Copyright © 2020 Izzo and Gillanders. This is an open-access article distributed under the terms of the Creative Commons Attribution License (CC BY). The use, distribution or reproduction in other forums is permitted, provided the original author(s) and the copyright owner(s) are credited and that the original publication in this journal is cited, in accordance with accepted academic practice. No use, distribution or reproduction is permitted which does not comply with these terms.
*Correspondence: Christopher Izzo, Y2hyaXN0b3BoZXIuaXp6b0BmcmRjLmNvbS5hdQ==
† Christopher Izzo, Fisheries Research and Development Corporation, Adelaide, SA, Australia