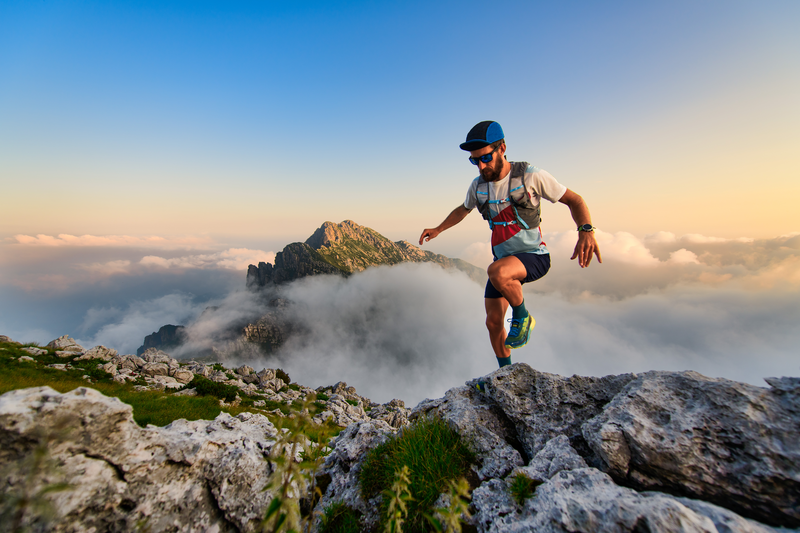
94% of researchers rate our articles as excellent or good
Learn more about the work of our research integrity team to safeguard the quality of each article we publish.
Find out more
ORIGINAL RESEARCH article
Front. Mar. Sci. , 19 March 2020
Sec. Marine Fisheries, Aquaculture and Living Resources
Volume 7 - 2020 | https://doi.org/10.3389/fmars.2020.00161
This article is part of the Research Topic Fish Nutrition, Metabolism and Physiology View all 20 articles
In Eriocheir sinensis aquaculture, crabs feeding on an artificial diet without steroid hormones available in ice-fresh fish or snails show delayed ovarian maturation and late listing. Icariin (ICA), a safe plant-derived monomer compound, can effectively promote estradiol (E2) synthesis and ovarian development in mammals, but its effect in crustaceans has not been reported. We investigated the effects of ICA on estrogen synthesis, vitellogenesis, and oocyte development in Chinese mitten crabs fed with one of four diets containing 0, 50, 100, and 200 mg/kg ICA, respectively, for 8 weeks. Results showed that vitellogenin (VTG) contents, gonadosomatic indexes (GSIs), and oocyte volumes in ICA50 and ICA 100 crabs were significantly higher than those in the control. The highest upregulated expression of VTG in hepatopancreases and ovaries was detected in ICA100 crabs. Hemolymph E2 contents were also significantly increased in ICA100 crabs. Enzyme-linked immunosorbent assay (ELISA) and western blot analysis indicated that the increase in E2 concentrations was attributable to an elevation in aromatase protein levels in ovaries through the cyclic adenosine monophosphate (cAMP)/protein kinase A (PKA)/cAMP response element binding (CREB) protein pathway. These findings suggest that ICA could serve as a natural additive to resolve the problem of delayed ovarian maturation of crabs raised in ponds.
The Chinese mitten crab, Eriocheir sinensis, is a commercially important cultured freshwater species with a huge consumer market in China. Annual production of E. sinensis has increased steadily in the past few years, reaching 812,103 tons in 2016 (Chinese Fishery Statistical Yearbook, 2017), reflecting strong market demand and popularity among Chinese consumers. To date, the dominant E. sinensis aquaculture feeding model remains the traditional feeding of crabs with natural diets such as ice-fresh fish and snails. However, the long-term overfishing has led to a growing shortage of ice-fresh fish resources and an increase in breeding costs. In addition, the improper feeding of natural baits is likely to cause eutrophication and water pollution, and even outbreak of diseases. By contrast, artificial formula feed has the advantages of comprehensive nutrients, controllable quality and safety, easy storage, and low cost. Therefore, it is urgent to popularize and apply artificial formula feed at a large scale. Furthermore, in China, consumers prefer to consume the gonads and hepatopancreas of E. sinensis because of their distinctive aroma and taste, and the maturity and fullness of ovaries determine market prices when crabs are on sale. Consumers also prefer to consume crabs during traditional holidays such as the Mid-Autumn Festival and the National Day, which generally fall in September or October. However, during this same period, harvested crabs fed an artificial diet show poor ovarian development and delayed ovarian maturation, which is characterized by lower gonadosomatic index (GSI) and less vitellin (Vn) deposition in ovaries (Wu et al., 2010). This leads to a delay in listing, and ultimately, lower prices for these crabs in contrast to those fed with a traditional diet of ice-fresh fish and snails. This also represents a technical bottleneck for wide-spread adoption of artificial crab feed. The key to overcoming this bottleneck is to find a functional feed additive that can promote the ovary development of crabs; however, to date, research on this field is limited. Therefore, we chose the critical period before listing (form early August to early October) to carry out this study to find a functional feed additive that can promote ovarian development in E. sinensis for use in the crab aquaculture industry.
Vitellogenesis is a key process in the development and maturation of ovaries in female crustaceans, and occurs in two stages in E. sinensis: early vitellogenesis mainly occurs in ovaries and is called the endogenous vitellogenic stage, and late vitellogenesis mainly occurs in hepatopancreas and is called the exogenous vitellogenic stage (Chen et al., 2004). The main yolk protein stored in oocytes is Vn, composed of carbohydrates, phospholipids, carotenoid components, and protein; its precursor is vitellogenin (VTG) (Chen et al., 2004). During the exogenous vitellogenic stage, abundant VTG synthesized by the hepatopancreas is transported through the hemolymph and internalized in developing oocytes via receptor-mediated endocytosis. Eventually, VTG is enzymolyzed and modified into Vn (Okuno et al., 2010). The synthesis and rapid accumulation of yolk protein directly result in a significant increase in GSI and the accumulation of nutrients, including amino acids, carbohydrates, vitamins, and trace elements in developing oocytes, thereby promoting ovarian development (Tsukimura, 2001). The exogenous vitellogenic phase occurs from September to November, and the GSI of E. sinensis changes from 0.5 to 11% during this critical physiological process (Wu et al., 2014).
In view of the importance of vitellogenesis to ovary maturation, many studies have focused on exploring the regulation of vitellogenesis in crustaceans. Reproductive hormones are important physiological indicators in regulating ovarian development and vitellogenesis. Previous studies have shown that wild broodstock tends to have healthy ovarian development because they ingest natural foods such as Artemia Nauplii and polychaetes Perinereis that contain sex steroid hormones. In contrast, feeding of artificial diets that lack steroid hormones may result in delayed ovarian maturation in pond-reared crustaceans (Wouters et al., 2001; Meunpol et al., 2007). Reproductive hormones such as methyl farnesoate (Rodríguez et al., 2002), ecdysteroid (Dong et al., 2009), progesterone (Reddy et al., 2006), and gonad inhibition hormone (Urtgam et al., 2015) have been found to play a vital role in gonadal development and vitellogenesis of crustaceans. Among them, estradiol (E2), whether endogenous or exogenous, is particularly important to promoting ovarian development and vitellogenesis (Warrier et al., 2001). Numerous reports have shown that exogenous E2 could shorten the ovarian maturation period of crustacean species such as Cherax albidus (Coccia et al., 2010), Penaeus monodon (Merlin et al., 2015), Panulirus interruptus (Nan et al., 2005), Marsupenaeus japonicus (Yano and Hoshino, 2006), and E. sinensis (Shen et al., 2010). The effects of E2 are dose-dependent and stage-specific, with higher E2 levels required in ovaries and hemolymph when oocytes are transiting from endogenous vitellogenesis to exogenous vitellogenesis. E2 strictly regulates VTG gene transcription mainly by binding to ligand-dependent transcription factors, estrogen receptors (ERs), in the target organ of vertebrates (Nelson and Habibi, 2013), and the promoter region of the VTG gene contains a specific E2 receptor binding site, designated as estrogen-responsive elements (EREs) (Evans et al., 1987; Klein-Hitpass et al., 1988). This ER gene has been lost during the evolution of arthropods, and no functional equivalents for this gene have been identified in crustaceans (Thornton et al., 2003). However, the mechanism of E2-ER signaling during ovarian development in crustaceans is still unknown, and it is unclear whether ER plays an important role in crustacean E2-VTG signaling.
Estrogen biosynthesis is catalyzed by aromatase, which is the final and unique rate-limiting enzyme in estrogen biosynthesis. Aromatase is encoded by the CYP19 gene (Means et al., 1989), and the transcriptional regulation of the CYP19 gene is tissue-specific because different promoters are present (Simpson et al., 2002). Multiple signaling pathways are involved in the regulation of aromatase activity and CYP19 gene transcripts through specific promoters and different cohorts of transcription factors (Simpson et al., 1997). In ovarian tissues, CYP19 expression is mediated primarily at promoter II by a cyclic adenosine monophosphate (cAMP) response element-like sequence (CLS) and the downstream steroidogenic factor-1 (SF-1) motif, which bind to the transcription factor, cAMP response element binding (CREB) protein, and SF-1, respectively, as well as by gonadotropin follicle-stimulating hormone (FSH), through the classical cAMP/protein kinase A (PKA)/CREB pathway (Gore-Langton and Dorrington, 1981; Michael et al., 1995; Guiguen et al., 2010). To sum up, the key criterion for selecting a functional feed additive for ovarian development in E. sinensis is the ability to promote estrogen synthesis and vitellogenesis. How to promote ovarian development, vitellogenesis, and reproductive hormone expression, and to shorten gonad maturation time in crustaceans remain to be explored. Previous studies on crustacean ovarian development and vitellogenesis have focused mainly on the effects of various lipids and exogenous hormones (Reddy et al., 2006; Wu et al., 2007a; Zmora et al., 2009; Lu et al., 2010; Liu et al., 2018). However, the effects of Chinese herbal medicine additives on ovarian development and reproductive hormones in crustaceans are rarely reported.
Epimedium brevicornum is a traditional Chinese herbal medicine plant used to treat reproductive diseases such as sexual dysfunction, anestrus, infertility, and estrogen deficiency-related diseases in Chinese veterinary practice and human medicine (Zhang et al., 2007). Icariin (ICA) is the most abundant bioactive component and main flavonoid in E. brevicornum (Li et al., 2015). Previous studies have confirmed that ICA and E. brevicornum can promote estrogen biosynthesis in human ovarian granulosa-like KGN cells and osteoblastic UMR-106 cells in a concentration- and time-dependent manner by enhancing aromatase mRNA and protein expression (Yang et al., 2013), similar to forskolin, which is well known to activate AC to increase intracellular cAMP production, in turn, resulting in the activation of PKA/CREB for aromatase transcription (Watanabe and Nakajin, 2004). However, whether ICA can promote ovarian tissue estrogen biosynthesis and aromatase expression in crustaceans through interacting with the cAMP/PKA/CREB pathway is still unknown.
In this study, we focused on the classical cAMP/PKA/CREB/aromatase signaling pathway to explore the effects of ICA on estradiol synthesis and aromatase expression by western blot analysis. We also investigated the effects of ICA on synthesis and deposition of VTG and oocyte development in female Chinese mitten crabs using molecular biological and histological methods, respectively. We focused on the critical period before listing (form early August to early October), with the aim of developing a new additive to solve the problem of delayed ovarian maturation and late listing of pond-reared E. sinensis and promoting the application of artificial formula feed in the crab breeding industry.
The procedures involving animal care in this study were approved by the Animal Care and Use Committee of Nanjing Agricultural University (Nanjing, China) [Permit Number: SYXK (Su) 2017-0007].
Icariin (purity ≧ 98%) purchased from Nantong Feiyu Biological Technology Co., Ltd. (Nantong, China) was supplemented at 50, 100, and 200 mg/kg feed weight (designated as ICA 50, ICA 100, and ICA 200, respectively). A diet without ICA was used as the control (CON). The ingredients and proximal composition of the CON diet are the same as those reported previously (Zheng et al., 2019). The 32% fishmeal, 20% soybean meal, 13% peanut meal, 4.5% blood powder, and 2% rapeseed meal served as protein sources, and equal portions of fish oil and soybean oil (2.4%) were included as lipid sources. The crude protein and lipid contents of the diet were 41.96 and 7.03%, respectively. All dry ingredients were finely ground, thoroughly mixed, and squeezed into strips by a single-screw meat grinder extruder with a 2.5 mm diameter die, and then air-dried with ventilation, ground, and sieved to appropriate sizes (2 cm length and 2 mm diameter) for feeding crabs. The food was stored at −20°C in sealed black plastic bags until use.
Non-precocious female crabs with similar size and initial weight (the average weight was about 28.93 g), and the same ovary maturation status were caught in the same locality at the same time. In this study, the method for judging whether the ovarian development stage of these crabs with uniform weight is roughly in synchronization was to dissect the ovarian tissues from 30 crabs randomly and conduct morphological and histological observations in strict conformity with the staging criteria of a previous study (Pan et al., 2017). Morphological observation revealed that these ovary tissues were thin and translucent, and thus were difficult to recognize. The mean value of these GSIs was about 0.26. Histological observation of the ovary showed that the dominant type of gametocytes was oogonia, followed by previtellogenic oocytes and endogenous vitellogenic oocytes. According to the random sampling results of morphological and histological observation, and GSI values, we concluded that these crabs were all in the early stage of ovarian development (Stage I). Prior to the experimental period, intermolt crabs were acclimated to experimental conditions for 1 week of hand-feeding with a commercial diet thrice daily (6:30, 15:30, and 19:30) (Haipurui Feed Co., Ltd., Jiangsu, China). After environmental acclimatization, a total of 200 healthy crabs (initial weight: 33.58 ± 0.05 g) were randomly classified into four treatment groups with 10 individuals per cement pool (1.0 × 1.0 × 0.8 m, L: W: H) and five replicates each. There were in total 20 cement pools. During the experimental period, water temperature, pH, and dissolved oxygen were monitored daily using a YSI 556 MPS multi-probe field meter (Geotech, United States). To maintain these indicators within the normal range, we changed the water every other day, and the water temperature ranged from 24 to 28°C, pH fluctuated between 8.5 and 8.6, and dissolved oxygen was maintained approximately at 5.0 mg/L by using an AIR 1000 oxygenation pump (EHIEIM, Germany) during the feeding trial.
At the end of the 2-month feeding trial, crabs were deprived of food for 24 h and then put on ice for hypothermia anesthesia. Each sampled crab was gently blotted by a towel to remove surface moisture and weighed using a standard electric balance with 0.0001 g accuracy. Then the ovaries and hepatopancreas of each crab were dissected aseptically and weighed. The hepatosomatic index (HSI) and GSI of each crab were calculated as the percentage of tissue wet weight to body wet weight. Subsequently, a small piece of fresh ovary tissue was collected and fixed in Bouin’s solution for ovarian histological analysis, and the remaining ovarian tissue and all the hepatopancreas tissue were quick-frozen in liquid nitrogen and stored at -80°C for further analysis. Next, 0.5 mL of hemolymph was collected from each crab’s second last pair of walking legs using a 1-mL sterile syringe, and mixed with pre-inhaled precooling crab anticoagulant solution at the ratio of 1:1 (Zheng et al., 2019), and immediately centrifuged at 9000 r/min and 4°C for 20 min. The supernatant was collected and stored at -20°C for later determination of VTG and gonadotropin releasing hormone (GnRH), FSH, and E2 contents.
The ovarian tissues fixed in Bouin’s solution for 24 h were progressively dehydrated in ascending concentrations of ethanol solutions and then cleared with xylene before being embedded in paraffin wax (melting point: 56–58°C). Sections (5–7 μm) of ovaries were prepared with a microtome (Leica 2016, Leica Microsystems Inc., Bannockburn, IL, United States) and then stained with hematoxylin-eosin (H&E), as previously reported (Zhou et al., 2018). Eight individuals were analyzed from each group. Histological observations and measurements were performed under a light microscope (Olympus BX-41, Olympus Corporation, Shinjuku-ku, Tokyo, Japan) equipped with an automated digital camera system (Nikon IDXM 1200, Nikon Co., Shinagawa-ku, Tokyo, Japan). Only dominant oocytes with nuclei were used for measurement of oocyte sizes. The long diameter of an oocyte (LO), shorter diameter of an oocyte (SO), long diameter of a nuclei (LN), shorter diameter of a nuclei (SN), volume of an oocyte (VO), and volume of a nuclei (VN) were measured using Image-Pro Plus 6.0 (Media Cybernetics Inc., Bethesda, MD, United States). The nucleo-cytoplasmic ratio (NCR) was calculated as the percentage of volume of nucleus to volume of cell, where volume = 0.523 × W2 × L (W, maximum width; L, maximum length) (Wu et al., 2007b). Fifty oocytes from eight replicate crabs and eight slides were analyzed, and the relative frequency of the most advanced oocytes appearing in the ovarian sections was used for ovarian staging (Wu et al., 2014).
Levels of FSH, E2 and VTG in the hemolymph supernatants were quantified by magnetic particle-based enzyme-linked immunosorbent assay (ELISA) kits (Mouse FSH ELISA Kit RGB and CHN lot: 20190505. 60341M; Mouse E2 ELISA kit RGB and CHN lot: 20190505. 60307M and Crab VTG ELISA kit RGB and CHN lot: 20200103. 60520CR, respectively) according to the manufacturer’s instructions (Beijing Rigor Bioscience Development Ltd., China). Optical densities were read at 450 nm by a microplate reader (Spectramax M5 Molecular Devices, United States) within 15 min of the addition of a color development reagent. The ELISA sensitivities for FSH, E2, and VTG were 0.1 mIU × mL–1, 1 ng × L–1, and 0.05 μg/mL, respectively.
Approximately 0.3 g of ovarian tissue was minced and homogenized in ice-cold 0.86% stroke-physiological saline solution (w/v, 1:9) using an Ultra-Turrax homogenizer (Tekmar Co., Cincinnati, OH, United States), and then centrifuged at 5000 r/min at 4°C for 10 min to obtain the supernatant for further analysis. Total protein content of the ovarian tissue was measured using the Biuret method (Kingsley, 1939). Levels of AC, cAMP, and PKA in ovarian supernatants were quantified by ELISA according to the kit manufacturer’s instructions (Nanjing Jiancheng Bioengineering Institute, China). Levels of aromatase in the ovarian supernatants were quantified by ELISA according to the kit manufacturer’s instructions (Shanghai Enzyme-linked Biotechnology Co., Ltd., China). The levels of ovarian AC, cAMP, PKA, and aromatase were expressed as nmol/mg protein.
Total RNA was isolated from ovary and hepatopancreas samples from E. sinensis using TRIzol (Invitrogen, CA, United States) according to the manufacturer’s protocols, and then treated with RQ1 RNase free DNase (Takara Co., Ltd., Japan) to remove genomic DNA. RNA integrity was tested by 1% agarose gel electrophoresis. RNA concentration and purity were determined based on OD 260/280 readings (ratio > 1.8) using a Nano Drop ND-1000 UV Spectrophotometer (Nano Drop Technologies, Wilmington, DE, United States). cDNA was synthesized using a Perfect Real Time SYBR Prime Script TM RT Reagent Kit (TaKaRa Biotechnology, Dalian, China) as per the manufacturer’s instructions and stored at −20°C. RTqPCR was conducted in the ABI Step One Plus TM Real Time PCR System (Applied Biosystems, Grand Island, NY, United States) as follows: pre-run at 95°C for 15 min, followed by 40 cycles of denaturation at 95°C for 15 s and a 60°C annealing step for 34 s. The reaction mixture comprised 2 μL cDNA, 0.4 μL forward primer, 0.4 μL reverse primer, 10 μL SYBR Premix Ex TaqTM (TaKaRa, Dalian, Liaoning, China), 0.4 μL ROX Reference Dye (TaKaRa, Dalian, Liaoning, China), and 6.8 μL double-distilled water. Each sample was tested in triplicate. The gene-specific primers of VTG (F: 5′-CGCTCCCTGCTGACAAACA-3′ and R: 5′-TGGGGCGAAATAGGAAAGG-3′) (Pan et al., 2018) and reference gene S27 (F: 5′-GGTCGATGACAATGGCAAGA-3′ and R: 5′-CCACAGTACTGGCGGTCAAA-3′) (Huang et al., 2017) were synthesized by Shanghai Generay Biotech Co., Ltd. (Shanghai, China). The relative levels of mRNA expression were calculated using the 2–Δ Δ CT method (Livak and Schmittgen, 2001).
Hepatopancreas and ovarian tissues were homogenized using a glass Tenbroeck tissue grinder (Kimble Chase) on ice and lyzed with 50 mM Tris⋅HCl, 150 mM NaCl, 0.5% NP-40, 0.1% SDS, and 1 mM EDTA (pH 7.5), supplemented with protease and a phosphatase inhibitor cocktail (Roche Diagnostics GmbH, Penzberg, Germany) at 4°C for 1 h and then cleared by centrifugation at 4°C and 12,000 × g for 15 min. Protein concentrations were quantified using a BCA protein assay kit (Tiangen, China). Heat denatured protein lysates (20 μg of protein) were loaded into each well, separated on 5–10% gradient sodium dodecyl sulfate-polyacrylamide electrophoresis gels for 1–2 h at 100 V using a Mini-Protean System (Bio-Rad, United States), and transferred to 0.45 μm polyvinylidene fluoride (PVDF) membranes (Millipore, MA, United States). Each PVDF membrane was rinsed three times in TBS for 10 min, blocked with 5% non-fat milk in TBST buffer (20 mM Tris⋅HCl, 500 mM NaCl, 0.1% Tween 20) for 1 h at room temperature, and then incubated overnight at 4°C with primary antibodies against AC (#AF9001, Affinity), cAMP (#ab76238, Abcam), PKA (#ab75991, Abcam), CREB (#ab31387, Abcam), p-CREB (#ab32096, Abcam), SF-1 (#AF7895, Affinity), ARO (#ab124776, Abcam), VTG (#abs119855, Absin), and β-actin (#ab8226, Abcam). The next day, secondary antibodies conjugated with horseradish peroxidase (HRP) (#ab6789, Abcam and # ab6271, Abcom) were added and visualized using ECL reagents (Beyotime Biotechnology, China). Western blot images were captured with a digital camera (EOS D60; Canon, Tokyo, Japan), and the intensity of immunoreactive bands was quantified using Image J 1.44p software (U.S. National Institutes of Health, Bethesda, MD, United States).
All data were expressed as treatment means with SEMs. Before statistical analysis, all data were tested for the normality of distribution and homogeneity of variances with the Levene test. Then, one-way ANOVA and Tukey’s honest significant difference (Tukey’s HSD) test were used to analyze differences between the four groups, and a P-value < 0.05 was considered significant difference. All statistical analyses were performed using SPSS 20.0 software (Chicago, IL, United States; Version 20.0).
The HSI and GSI of E. sinensis subjected to different levels of dietary ICA for 8 weeks are presented in Figure 1. Compared to the CON group (HSI ∼8.41%), no significant differences were found in HSI of crabs fed with ICA50 (HSI ∼8.25%), ICA100 (HSI ∼7.89%), or ICA200 diets (HSI ∼8.36%) (P > 0.05). The GSI increased significantly in both the ICA50 (GSI ∼3.81%) and ICA100 (GSI ∼4.65%) groups compared to that in the CON (GSI ∼2.02%) group (P < 0.05). Contrastingly, there was no significant difference in GSI (GSI ∼2.33%) of crabs fed with ICA200 diets compared to that in the CON group (P > 0.05).
Figure 1. Hepatosomatic index (HSI, A1) and gonadosomatic index (GSI, A2) of E. sinensis subjected to different levels of dietary ICA for 8 weeks. Each datum represents the mean of twenty replicates. Bars assigned different superscripts are significantly different (P < 0.05).
Ovarian histological observation was used to evaluate and compare the ovarian development stage, and the deposition status of yolk granules (YGs) in the oocytes. As shown in Figure 2, all crabs were in the exogenous vitellogenesis stage of ovary development, which is characterized by eosinophilic exogenous vitellogenic oocytes surrounded by follicular cells. The oocytes were oval or irregular in shape at this stage, and many eosinophilic YGs were present in the cytoplasm of oocytes, and only a small amount of basophilic endogenous vitellogenic oocytes existed. Under the same photomicrographic magnification (10×), the numbers of exogenous vitellogenic stage oocytes in the ICA50 and ICA100 groups were obviously less than those in the ICA200 and CON groups. By contrast, the oocytes and YGs in crabs fed with ICA50 and ICA100 diets were obviously bigger than those in the CON group. Further, oocytes in crabs in the ICA50 and ICA100 groups appeared to be in a state of mutual extrusion and deformation.
Figure 2. H&E staining of ovarian tissue from E. sinensis subjected to different levels of dietary ICA. Images from the CON (fed the basal diet without ICA), ICA50, ICA100, and ICA200 (fed the basal diet supplemented with 50, 100, and 200 mg/kg ICA, respectively) groups. Eight replicates were performed for each group. EN, endogenous vitellogenic oocyte; EX, exogenous vitellogenic oocyte; NO, nearly mature oocyte; FC, follicle cell; YG, yolk granule; N, nucleus. Photomicrographs (10×) with scale bars (100 μm).
Histological measurements of oocytes were used to analyze and compare the parameters of oocytes. As shown in Table 1, the parameters, LO, SO, and VO were significantly increased in the ICA50 and ICA100 groups compared to those in the control group (P < 0.05), and peaked in the ICA100 group. LN and VN were significantly increased in the ICA100 group as compared with that in the CON group (P < 0.05). In terms of NCR, the highest NCR was found in the CON group, and there were no significant differences among the ICA50, ICA100, and ICA200 groups (P > 0.05).
As shown in Figure 3, the levels of FSH were increased in the ICA50 and ICA100 groups compared to those in the CON group, albeit no significant difference (P > 0.05). E2 concentrations in hemolymph increased with ICA supplementation levels from 50 to 100 mg/kg, and ICA100 crabs showed significantly higher E2 concentrations than those in the CON group (P < 0.05). VTG concentrations in hemolymph were significantly increased both in the ICA50 and ICA100 groups (P < 0.05). No significant differences were found in E2 and VTG concentrations in crabs fed with the ICA200 diet compared to those in the CON group (P > 0.05).
Figure 3. Determination of follicle-stimulating hormone (FSH), estradiol, and vitellogenin concentrations (FSH, (A1); Estradiol, (A2); Vitellogenin, (A3)) in hemolymph of E. sinensis subjected to different levels of dietary ICA. Each datum represents the mean of eight replicates. Bars assigned different superscripts are significantly different (P < 0.05).
As shown in Figure 4, ICA50 and ICA100 supplementation induced VTG gene expression in both hepatopancreases and ovaries compared to those in the CON group (P < 0.05). The highest levels of VTG mRNA in both organs were observed in the ICA100 group. By contrast, there was no significant difference between the ICA200 and CON groups (P > 0.05).
Figure 4. Gene expression of VTG in hepatopancreas (A1) and ovary (A2) of E. sinensis subjected to different levels of dietary ICA. The relative expression levels of VTG were normalized to that of S27. Values represent means ± SEM (n = 8). Bars assigned with different superscripts are significantly different (P < 0.05).
Vitellogenin protein expression in hemocytes and hepatopancreases is presented in Figure 5. Compared to that in the CON group, VTG protein was significantly upregulated in the ovaries of crabs with ICA supplementation levels of 50 and 100 mg/kg (P < 0.05), and showed the highest levels in the ICA100 group. VTG protein expression in hepatopancreases of crabs fed with the ICA100 diet was higher than that in the CON group (P < 0.05). There was no significant difference in VTG expression between crabs fed with the ICA200 diet and those in the CON group (P > 0.05).
Figure 5. Protein expression of VTG in ovary and hepatopancreas of E. sinensis subjected to different levels of dietary ICA. The relative expression levels of VTG protein in ovary and hepatopancreas were normalized to that of β-actin (ovary, A1; hepatopancreas, A2). Values represent means ± SEM (n = 3). Bars assigned different superscripts are significantly different (P < 0.05).
As shown in Figure 6, the ELISA results showed that levels of AC, cAMP, and PKA in ovarian tissues of crabs in both the ICA50 and ICA100 groups were significantly higher than those in the CON group (P < 0.05), with the highest levels observed in the ICA100 group. Aromatase levels in ovarian tissues increased with increasing ICA supplementation levels of 50 to 100 mg/kg, and ICA100 crabs showed significantly higher aromatase levels than those in the CON group (P < 0.05). There were no significant differences in these four parameters in crabs fed with the ICA200 diet compared to those in the CON group (P > 0.05).
Figure 6. Determination of AC (A1), cAMP (A2), PKA (A3), and Aromatase (A4) levels in ovarian tissue of E. sinensis subjected to different levels of dietary ICA. Each datum represents the mean of eight replicates. Bars assigned different superscripts are significantly different (P < 0.05).
As shown in Figure 7, ovary aromatase protein levels were upregulated with increasing ICA supplementation levels increasing of 0 to 100 mg/kg; only ICA100 crabs showed significantly higher aromatase protein levels than those in the CON group (P < 0.05). The ICA200 group showed no difference in aromatase protein expression levels compared with the CON group (P > 0.05). Ovary AC, cAMP, PKA, and SF-1 proteins levels in both the ICA50 and ICA100 groups were significantly increased compared to those in the CON group (P < 0.05). Ovary p-CREB/CREB ratios were upregulated with increasing ICA supplementation levels of 50 to 100 mg/kg; only ICA100 crabs showed significantly higher ratios than those in the CON group (P < 0.05).
Figure 7. Protein expression of Aromatase and AC/cAMP/PKA/CREB/SF-1 pathway in ovarian tissue of E. sinensis subjected to different levels of dietary ICA. The relative expression levels of aromatase were normalized to that of β-actin. Values represent means ± SEM (n = 3). Bars assigned different superscripts are significantly different (P < 0.05).
In crustaceans, ovary maturation is marked by increases in GSI, oocyte volume, and VTG level, with GSI and HSI being the two critical indicators of maturity and stage of ovarian development in female crustaceans (Lee and Chang, 1997; Boucard et al., 2002; Okumura et al., 2007). A previous study showed that, with the development and maturation of ovaries, the HSI of E. sinensis gradually decreased, while GSI dramatically increased, especially during the exogenous vitellogenic stage, which is characterized by GSI levels of approximately 0.5–11% and HSI levels of 7–10% (Li et al., 2001). In the present study, significant increases in GSI were found in crabs fed with ICA50 (GSI ∼3.81%) and ICA100 (GSI ∼4.65%) diets compared to those in the CON group (GSI ∼2.02%), but crabs fed with ICA200 (GSI ∼2.33%) did not show any difference in GSI compared with those in the CON group. A previous study showed that the mean GSI ranged from 0.52 to 4.74 during the stage III of E. sinensis ovary development (Pan et al., 2017), and it is noteworthy that the final mean GSIs of each group in this study ranged from 2.02 to 4.65%. This suggested that all crabs were in the stage III of ovary development, and that certain doses of ICA (50–100 mg/kg) could accelerate the ovary development of E. sinensis. Notably, however, a high dose of ICA (200 mg/kg) did not increase GSIs in E. sinensis. Similar results have been reported from previous studies on sexually mature female rats; these studies showed that ICA can increase ovarian indexes and uterine weight in a dose-dependent manner (Zhang et al., 2007; Kang et al., 2012). Previous studies on ICA for cell proliferation by 3-(4,5-dimethylthiazol-2-yl)-2,5-diphenyltetrazolium bromide (MTT) cell viability assay may explain this. Research on SKBr3 ER-negative breast cancer cells showed that ICA at doses of 10–9–10–5 M could stimulate cell proliferation in a dose-dependent manner; the maximal cell proliferative effect was exerted at a dose of 10–7 M ICA, and then began to decline with higher concentrations (Ma et al., 2014). Another research on MC3T3-E1 osteoblastic cells showed that ICA treatment could increase cell viability as compared to that of an untreated control group in a dose-dependent manner; the maximal cell viability was observed at 10 nM ICA, and a higher dose led to decreased cell viability (Song et al., 2013). These results suggest that ICA might have a diphasic regulatory action in a dose-dependent manner; and that overdose of ICA may have a cytotoxic effect on cells.
In terms of GSI, a previous study also showed that there was a significantly positive correlation between GSI and LO (Pan et al., 2017). Similarly, in this study, mean LO, SO, and VO values were significantly increased in the ICA50 and ICA100 groups, whereas NCR showed an opposite trend. This result was expected because NCR gradually decreased with oocyte development in E. sinensis (Pan et al., 2017). Meanwhile, histological observations of oocytes showed that oocytes and YGs in the cytoplasm were bigger, and consequently the numbers of oocytes were less in a visual field in the ICA50 and ICA100 groups than in the CON group. Therefore, we inferred that the appropriate dose of ICA could promote the synthesis of VTG, as well as the transport and deposition of nutrients into oocytes. This was supported by the rapid increase in GSI and oocyte size, which could be directly attributed to the transportation of abundant nutrients by a carrier of VTG from hepatopancreases to developing ovaries and rapid accumulation of yolk protein and other essential nutrients such as carotenoids, steroid hormones, and phospholipids in E. sinensis oocytes (Pan et al., 2018).
Vitellogenin is considered as a vital biomarker of vitellogenesis in oviparous animals, including crustaceans (Gerber-Huber et al., 1987; Coccia et al., 2010). In E. sinensis, vitellogenesis is divided into two stages, endogenous and exogenous vitellogenesis, with the ovary and hepatopancreas, respectively, serving as the major sites of VTG synthesis. During exogenous vitellogenesis, VTG synthesis is very active in the hepatopancreas. VTG is then transported through the hemolymph to developing oocytes via endocytosis, when the cytoplasms of exogenous vitellogenic oocytes are filled with YG (Chen et al., 2004; Pan et al., 2018). In the present study, significantly elevated hemolymph VTG concentrations were found in the ICA50 and ICA100 groups compared with those in the CON group, but a high dose of ICA (200 mg/kg) showed no significant effect on VTG synthesis. This result was consistent with the up-regulation of VTG mRNA and protein expression in both hepatopancreases and ovaries. This suggested that only an appropriate dose of ICA supplementation can promote endogenous and exogenous vitellogenesis in E. sinensis by promoting VTG expression at both the transcriptional and translation levels and transportation of VTG from the hepatopancreas to ovaries. Moreover, similar trends in E2 levels in hemolymph were found in this study. Thus, we concluded that the increase in vitellogenesis in ICA-supplemented crabs was attributable to stimulation of E2 biosynthesis and elevated E2 contents in circulation. This view is supported by results of prior studies, which found that E2 levels were positively correlated with VTG contents in the hemolymph (Huang et al., 2009; Zhang, 2011), and were closely associated with vitellogenesis during different ovary developmental stages in crustaceans. The changes of both E2 and VTG levels showed the following trend: an initial increase, reaching a peak in the exogenous vitellogenesis stage, and then a decline (Fairs et al., 1990; Martins et al., 2007; Liu et al., 2017; Pan et al., 2018). In addition, numerous studies of crustaceans have shown that exogenous 17β-E2 can significantly induced vitellogenesis in E. sinensis, P. monodon, and M. japonicus (Yano and Hoshino, 2006; Shen et al., 2010; Merlin et al., 2015). In some oviparous vertebrates, E2 activates VTG expression at the transcriptional level by binding to ERs and specific EREs in the VTG promoter (Evans et al., 1987; Klein-Hitpass et al., 1988). Based on the above findings, we conclude that ICA may indirectly affect vitellogenesis by promoting E2 biosynthesis, but the precise mechanism underlying this relationship will require further study.
E2 biosynthesis is specifically catalyzed by a unique rate-limiting enzyme called aromatase, which is encoded by the CYP19 gene (Simpson et al., 1994). To explore the mechanism by which ICA regulates E2 biosynthesis in E. sinensis, we investigated the effects of different doses of ICA on aromatase protein expression and its potential regulatory role. In this study, ELISA and western blot analysis results showed that only 100 mg/kg of ICA supplementation significantly increased gene and protein expression levels of aromatase in ovarian tissue; and that doses beyond 100 mg/kg did not exhibit similar effects to the 100 mg/kg dose, which was in line with findings on the E2 levels. The decline of aromatase mRNA and protein expression levels at 200 mg/kg of ICA likely represents a cytotoxic effect. These results indicate that ICA can promote the biosynthesis of E2 by stimulating aromatase expression in a concentration-dependent manner. This conclusion is supported by previous studies in human ovarian granulosa cells (KGN cells), human osteoblastic cells (UMR-106 cells), rat ovarian granulosa cells, and TGF-β1-treated rat Leyding cells that showed that ICA promotes E2 biosynthesis by enhancing aromatase mRNA and protein expression in a concentration-dependent manner (Yang et al., 2013, 2016; Nie et al., 2018). In this study, to investigate the potential mechanism by which ICA regulates aromatase expression, we focused on proteins in the cAMP/PKA/CREB pathway because CYP19 expression in ovaries is mediated by the classical cAMP/PKA/CREB pathway (Gore-Langton and Dorrington, 1981; Guiguen et al., 2010). Our results showed that supplementation with 50–100 mg/kg ICA increased AC, cAMP, and PKA mRNA levels in ovarian tissues, and that at the translational level, ICA activated the AC/cAMP/PKA/CREB pathway by increasing intracellular cAMP and PKA expression and CREB phosphorylation. These findings were consistent with those of several previous studies, which showed that ICA treatment could activate AC/cAMP/PKA/CREB signaling by increasing intracellular cAMP levels and facilitating phosphorylation of both PKA and CREB in rat calvarial osteoblasts (Shi et al., 2017) and human ovarian granulosa-like KGN cells (Zaccolo and Movsesian, 2007; Li et al., 2017). Together, these results suggest that ICA may promote E2 synthesis by promoting aromatase expression through cAMP/PKA/CREB signaling in ovaries of E. sinensis in a concentration-dependent manner.
In conclusion, the results obtained in this study indicated that ICA supplementation at 100 mg/kg can efficiently promote estrogen biosynthesis, vitellogenesis, and oocyte development in female E. sinensis. Therefore, ICA has the potential to serve as an additive that promotes ovarian development in pond-reared crabs. However, hepatopancreas and oocyte cell models must be constructed to thoroughly evaluate the exact molecular mechanisms underlying the effect of ICA in indirectly stimulating vitellogenesis by promoting E2 biosynthesis. In addition, it is necessary to further compare the efficacy between feeding with artificial diets, including ICA as an ovarian development regulator, and natural diets such as ice-fresh fish and snails by conducting further experiments in aquaculture.
All datasets generated for this study are included in the article/supplementary material.
CC and WL conceptualized this experiment, reviewed and edited the manuscript. XZ performed the feeding experiment, analyzed the data, and wrote the manuscript. JL, CZ, LZ, FG, and DZ analyzed the data. All authors discussed and approved the final version of the manuscript.
This work was supported by the earmarked fund for Jiangsu Agricultural Industry Technology System [Grant No. JATS (2019) 441], the Jiangsu Agricultural Science and Technology Innovation Fund [Grant No. CX (19) 1006], the Modern Fisheries Industry Technology System Project of Jiangsu Province (Grant No. JFRS-01), the Fundamental Research Funds for the Central Universities (Grant No. KJQN201937), the National Natural Science Foundation of China (Grant No. 31802347), and the Aquatic Three New Project of Jiangsu Province (Grant No. D2018-4).
The authors declare that the research was conducted in the absence of any commercial or financial relationships that could be construed as a potential conflict of interest.
We thank Dr. Kang Cheng (Nanjing Agricultural University) for help with histological observation. We thank Wan Liu (Global Tone Communication Technology Co., Ltd.) for help with Language revision.
Boucard, C. G. V., Levy, P., Ceccaldi, H. J., and Brogren, C. H. (2002). Developmental changes in concentrations of vitellin, vitellogenin, and lipids in hemolymph, hepatopancreas, and ovaries from different ovarian stages of Indian white prawn Fenneropenaeus indicus. J. Exp. Mar. Biol. Ecol. 281, 63–75. doi: 10.1016/s0022-0981(02)00409-4
Chen, L. Q., Jiang, H. B., Zhou, Z. L., Li, K., Deng, G. Y., and Liu, Z. J. (2004). Purification of vitellin from the ovary of Chinese mitten-handed crab (Eriocheir sinensis) and development of an antivitellin ELISA. Comp. Biochem. Physiol. B Biochem. Mol. Biol. 138, 305–311. doi: 10.1016/j.cbpc.2004.04.012
Chinese Fishery Statistical Yearbook, (2017). Fisheries Agency of China Agriculture Ministry. Beijing: China Agriculture Press, 24.
Coccia, E., De, L. E., Di, C. C., Di, C. A., and Paolucci, M. (2010). Effects of estradiol and progesterone on the reproduction of the freshwater crayfish Cherax albidus. Biol. Bull. 218, 36–47. doi: 10.1086/BBLv218n1p36
Dong, S. Z., Ye, G. Y., Guo, J. Y., and Hu, C. (2009). Roles of ecdysteroid and juvenile hormone in vitellogenesis in an endoparasitic wasp, Pteromalus puparum (Hymenoptera: Pteromalidae). Gen. Comp. Endocrinol. 160, 102–108. doi: 10.1016/j.ygcen.2008.11.007
Evans, M. I., O’Malley, P. J., Krust, A., and Burch, J. B. (1987). Developmental regulation of the estrogen receptor and the estrogen responsiveness of five yolk protein genes in the avian liver. Proc. Natl. Acad. Sci. U.S.A. 84, 8493–8497. doi: 10.1073/pnas.84.23.8493
Fairs, N. J., Quinlan, P. T., and Goad, L. J. (1990). Changes in ovarian unconjugated and conjugated steroid titers during vitellogenesis in Penaeus monodon. Aquaculture 89, 83–99. doi: 10.1016/0044-8486(90)90235-f
Gerber-Huber, S., Nardelli, D., Haefliger, J. A., Cooper, D. N., Givel, F., Germond, J. E., et al. (1987). Precursor-product relationship between vitellogenin and the yolk proteins as derived from the complete sequence of a Xenopus vitellogenin gene. Nucleic Acids Res. 15, 4737–4753. doi: 10.1093/nar/15.12.4737
Gore-Langton, R. E., and Dorrington, J. H. (1981). FSH induction of aromatase in cultured rat granulosa cells measured by a radiometric assay. Mol. Cell. Endocrinol. 22, 135–151. doi: 10.1016/0303-7207(81)90087-3
Guiguen, Y., Fostier, A., Piferrer, F., and Chang, C. F. (2010). Ovarian aromatase and estrogens: a pivotal role for gonadal sex differentiation and sex change in fish. Gen. Comp. Endocrinol. 165, 352–366. doi: 10.1016/j.ygcen.2009.03.002
Huang, H. Y., Ye, H. H., Han, S. Z., and Wang, G. Z. (2009). Profiles of gonadotropins and steroid hormone-like substances in the hemolymph of mud crab Scylla paramamosain during the reproduction cycle. Mar. Freshw. Behav. Physiol. 42, 297–305. doi: 10.1080/10236240903174792
Huang, S., Chen, X. W., Wang, J., Chen, J., Yue, W. C., Lu, W. Q., et al. (2017). Selection of appropriate reference genes for qPCR in the Chinese mitten crab, Eriocheir sinensis (Decapoda,Varunidae). Crustaceana 90, 275–296. doi: 10.1163/15685403-00003651
Kang, H. K., Lee, S. B., Kwon, H. S., Sung, C. K., Park, Y. I., and Dong, M. S. (2012). Peripubertal administration of icariin and icaritin advances pubertal development in female rats. Biomol. Ther. 20, 189–195. doi: 10.4062/biomolther.2012.20.2.189
Kingsley, G. R. (1939). The determination of serum total protein, albumin and globulin by the biuret reaction. J. Biol. Chem. 131, 197–200. doi: 10.1515/bchm2.1940.263.6.267
Klein-Hitpass, L., Ryffel, G. U., Heitlinger, E., and Cato, A. C. (1988). A 13 bp palindrome is a functional estrogen responsive element and interacts specifically with estrogen receptor. Nucleic Acids Res. 16, 647–663. doi: 10.1093/nar/16.2.647
Lee, F. Y., and Chang, C. F. (1997). The concentrations of vitellogenin (vitellin) and protein in hemolymph, ovary and hepatopancreas in different ovarian stages of the freshwater prawn, Macrobrachium rosenbergii. Comp. Biochem. Physiol. Part A Mol. Integr. Physiol. 117, 433–439. doi: 10.1016/s0300-9629(96)00260-5
Li, C., Li, Q., Mei, Q. B., and Lu, T. L. (2015). Pharmacological effects and pharmacokinetic properties of icariin, the major bioactive component in Herba Epimedii. Life Sci. 126, 57–68. doi: 10.1016/j.lfs.2015.01.006
Li, F., Du, B. W., Lu, D. F., Wu, W. X., Wongkrajang, K., Wang, L., et al. (2017). Flavonoid glycosides isolated from Epimedium brevicornum and their estrogen biosynthesis-promoting effects. Sci. Rep. 7:7760. doi: 10.1038/s41598-017-08203-7
Li, S. F., Cheng, H. W., and Zhao, N. G. (2001). Studies on gonad developmental rule of lake stocked mitten crab of yangtze population (in Chinese with English abstract). Acta Hydrobiol. Sin. 25, 350–357.
Liu, M. M., Pan, J., Liu, Z. J., Cheng, Y. X., Gong, J., and Wu, X. G. (2018). Effect of estradiol on vitellogenesis and oocyte development of female swimming crab, Portunus trituberculatus. Aquaculture 486, 240–245. doi: 10.1016/j.aquaculture.2017.12.034
Liu, M. M., Wu, X. G., Pan, J., and Cheng, Y. X. (2017). Immunolocalization and changes in 17β-estradiol in Portunus trituberculatus during ovarian development (in Chinese with English abstract). J. Fish. Sci. China 24, 239–247.
Livak, K. J., and Schmittgen, T. D. (2001). Analysis of relative gene expression data using realtime quantitative PCR and the 2-ΔΔCT method. Methods 25, 402–408. doi: 10.1006/meth.2001
Lu, J. F., Chang, G. L., Wu, X. G., Yang, X. Z., Zhao, W. X., and Cheng, Y. X. (2010). Hormonal regulations of ovarian development and vitellogenesis in Chinese mitten crab Eriocheir sinensis fed on two different diets (in Chinese with English abstract). Oceanol. Limnol. Sin. 41, 505–512.
Ma, H. R., Wang, J., Chen, Y. F., Wang, W. S., and Aisa, H. A. (2014). Icariin and icaritin stimulate the proliferation of SKBr3 cells through the GPER1-mediated modulation of the EGFR-MAPK signaling pathway. Int. J. Mol. Med. 33, 1627–1634. doi: 10.3892/ijmm.2014.1722
Martins, J., Ribeiro, K., Rangel-Figueiredo, T., and Coimbra, J. (2007). Reproductive cycle, ovarian development, and vertebrate-type steroids profile in the freshwater prawn Macrobrachium rosenbergii. J. Crustacean Biol. 27, 220–228. doi: 10.1651/c-2597.1
Means, G. D., Mahendroo, M. S., Corbin, C. J., Mathis, J. M., Powell, F. E., Mendelson, C. R., et al. (1989). Structural analysis of the gene encoding human aromatase cytochrome P-450, the enzyme responsible for estrogen biosynthesis. J. Biol. Chem. 264, 19385–19391. doi: 10.1016/0006-291X(89)91832-9
Merlin, J., Mohanlal, D. L., Balasubramanian, C. P., Sherly, T., Subramoniam, T., Syamadayal, J., et al. (2015). Induction of vitellogenesis and reproductive maturation in tiger shrimp, Penaeus monodon by 17β-estradiol and 17α-hydroxyprogesterone: in vivo and in vitro studies. Invertebr. Reprod. Dev. 59, 166–175. doi: 10.1080/07924259.2015.1051192
Meunpol, O., Iampai, S., Suthikrai, W., and Piyatiratitivorakul, S. (2007). Identification of progesterone and 17α-hydroxyprogesterone in polychaetes (Perinereis sp.) and the effects of hormone extracts on penaeid oocyte development in vitro. Aquaculture 270, 485–492. doi: 10.1016/j.aquaculture.2007.05.031
Michael, M. D., Kilgore, M. W., Morohashi, K., and Simpson, E. R. (1995). Ad4BP/SF-1 regulates cyclic AMP-induced transcription from the proximal promoter (PII) of the human aromatase P450 (CYP19) gene in the ovary. J. Biol. Chem. 270, 13561–13566. doi: 10.1074/jbc.270.22.13561
Nan, F. H., Wu, Y. S., and Chang, N. C. (2005). The effect of steroid hormone feeds on the reproductive biology of the spiny lobster, Panulirus interruptus (J. W. Randall, 1840) (Decapoda, Palinura). Crustaceana 88, 1367–1386. doi: 10.1163/15685403-00003489
Nelson, E. R., and Habibi, H. R. (2013). Estrogen receptor function and regulation in fish and other vertebrates. Gen. Comp. Endocrinol. 192, 15–24. doi: 10.1016/j.ygcen.2013.03.032
Nie, X., Sheng, W., Hou, D., Liu, Q., Wang, R., and Tan, Y. (2018). Effect of hyperin and icariin on steroid hormone secretion in rat ovarian granulosa cells. Clin. Chim. Acta 495, 646–651. doi: 10.1016/j.cca.2018.05.004
Okumura, T., Yamano, K., and Sakiyama, K. (2007). Vitellogenin gene expression and hemolymph vitellogenin during vitellogenesis, final maturation, and oviposition in female kuruma prawn, Marsupenaeus japonicus. Comp. Biochem. Physiol. Part A Mol. Integr. Physiol. 147, 1028–1037. doi: 10.1016/j.cbpa.2007.03.011
Okuno, A., Yang, W. J., Jayasankar, V., Saido-Sakanaka, H., Huong, D. T., Jasmani, S., et al. (2010). Deduced primary structure of vitellogenin in the giant freshwater prawn Macrobrachium rosenbergii, and yolk processing during ovarian maturation. J. Exp. Zool. 292, 417–429. doi: 10.1002/jez.10083
Pan, J., Cheng, Y. X., Zeng, C. S., Liu, M. M., Wu, X. G., and Chen, H. (2017). The ovarian development pattern of pond-reared Chinese mitten crab, Eriocheir sinensis H. Milne-Edwards, 1853. Crustaceana 90, 449–470. doi: 10.1163/15685403-00003662
Pan, J., Liu, M. M., Chen, T., and Wu, X. G. (2018). Immunolocalization and changes of 17beta-estradiol during ovarian development of Chinese mitten crab Eriocheir Sinensis. Cell Tissue Res. 373, 509–520. doi: 10.1007/s00441-018-2834-x
Reddy, P. R., Kiranmayi, P., Kumari, K. T., and Reddy, P. S. (2006). 17α-hydroxyprogesterone induced ovarian growth and vitellogenesis in the freshwater rice field crab Oziotelphusa senex senex. Aquaculture 254, 768–775. doi: 10.1016/j.aquaculture.2005.11.023
Rodríguez, E. M., López Greco, L. S., Medesani, D. A., Laufer, H., and Fingerman, M. (2002). Effect of methyl farnesoate, alone and in combination with other hormones, on ovarian growth of the red swamp crayfish, Procambarus clarkii, during vitellogenesis. Gen. Comp. Endocrinol. 125, 34–40. doi: 10.1006/gcen.2001.7724
Shen, B. J., Yang, X. Z., Wu, X. G., Cheng, Y. X., and Tang, B. R. (2010). The effects of exogenous 17β-estradiol on ovary development and on the level of endogenous 17β-estradiol in Eriocheir sinensis (in Chinese with English abstract). J. Shanghai Ocean Univ. 19, 289–295.
Shi, W. G., Gao, Y. H., Wang, Y. Y., Zhou, J., Wei, Z. L., Ma, X. N., et al. (2017). The flavonol glycoside icariin promotes bone formation in growing rats by activating the cAMP signaling pathway in primary cilia of osteoblasts. J. Biol. Chem. 292, 20883–20896. doi: 10.1074/jbc.M117.809517
Simpson, E. R., Clyne, C., Rubin, G., Boon, W. C., Robertson, K., Britt, K., et al. (2002). Aromatase-a brief overview. Annu. Rev. Physiol. 64, 93–127. doi: 10.1146/annurev.physiol.64.081601.142703
Simpson, E. R., Mahendroo, M. S., Means, G. D., Kilgore, M. W., Hinshelwood, M. M., Graham-Lorence, S., et al. (1994). Aromatase cytochrome P450, the enzyme responsible for estrogen biosynthesis. Endocr. Rev. 15, 342–355. doi: 10.1210/edrv-15-3-342
Simpson, E. R., Zhao, Y., Agarwal, V. R., Michael, M. D., Bulun, S. E., Hinshelwood, M. M., et al. (1997). Aromatase expression in health and disease. Recent Prog. Horm. Res. 52, 185–213.
Song, L. G., Zhao, J. S., Zhang, X. Z., Li, H., and Zhou, Y. (2013). Icariin induces osteoblast proliferation, differentiation and mineralization through estrogen receptor-mediated ERK and JNK signal activation. Eur. J. Pharmacol. 714, 15–22. doi: 10.1016/j.ejphar.2013.05.039
Thornton, J. W., Need, E., and Crews, D. (2003). Resurrecting the ancestral steroid receptor: ancient origin of estrogen signaling. Science 301, 1714–1717. doi: 10.1126/science.1086185
Tsukimura, B. (2001). Crustacean vitellogenesis: its role in oocyte development. Am. Zool. 41, 465–476. doi: 10.2307/3884477
Urtgam, S., Treerattrakool, S., Roytrakul, S., Wongtripop, S., Prommoon, J., Panyim, S., et al. (2015). Correlation between gonad-inhibiting hormone and vitellogenin during ovarian maturation in the domesticated Penaeus monodon. Aquaculture 437, 1–9. doi: 10.1016/j.aquaculture.2014.11.014
Warrier, S. R., Tirumalai, R., and Subramoniam, T. (2001). Occurrence of vertebrate steroids, estradiol 17beta and progesterone in the reproducing females of the mud crab Scylla serrata. Comp. Biochem. Physiol. Part A Mol. Integr. Physiol. 130, 283–294. doi: 10.1016/s1095-6433(01)00385-3
Watanabe, M., and Nakajin, S. (2004). Forskolin up-regulates aromatase (CYP19) activity and gene transcripts in the human adrenocortical carcinoma cell line H295R. J. Endocrinol. 180, 125–133. doi: 10.1677/joe.0.1800125
Wouters, R., Lavens, P., Nieto, J., and Sorgeloos, P. (2001). Penaeid shrimp broodstock nutrition: an updated review on research and development. Aquaculture 202, 1–21. doi: 10.1016/s0044-8486(01)00570-1
Wu, X. G., Chen, H., Liu, Z. J., and Cheng, Y. X. (2014). Immunorecognition and distribution of progesterone receptors in the Chinese Mitten Crab, Eriocheir sinensis, during ovarian development. J. Shellfish Res. 33, 35–43. doi: 10.2983/035.033.0105
Wu, X. G., Cheng, Y. X., Sui, L. Y., Yang, X. Z., Nan, T. Z., and Wang, J. Q. (2010). Biochemical composition of pond-reared and lake-stocked Chinese mitten crab Eriocheir sinensis (H. Milne-Edwards) broodstock. Aquacult. Res. 38, 1459–1467. doi: 10.1111/j.1365-2109.2007.01728.x
Wu, X. G., Cheng, Y. X., Sui, L. Y., Zeng, C. S., Southgate, P. C., and Yang, X. Z. (2007a). Effect of dietary supplementation of phospholipids and highly unsaturated fatty acids on reproductive performance and offspring quality of Chinese mitten crab, Eriocheir sinensis (H. Milne-Edwards), female broodstock. Aquaculture 273, 602–613. doi: 10.1016/j.aquaculture.2007.09.030
Wu, X. G., Yao, G. G., Yang, X. Z., Cheng, Y. X., and Wang, C. L. (2007b). A study on the ovarian development of Portunus trituberculatus in East Sea during the first reproductive cycle (in Chinese with English abstract). Acta Oceanol. Sin. 29, 120–127.
Yang, L. J., Lu, D. F., Guo, J. J., Meng, X. L., Zhang, G. L., and Wang, F. (2013). Icariin from Epimedium brevicornum Maxim promotes the biosynthesis of estrogen by aromatase (CYP19). J. Ethnopharmacol. 145, 715–721. doi: 10.1016/j.jep.2012.11.031
Yang, Q. Q., Ma, J., Liu, M. L., Cao, L., Li, Y. Q., Liu, A. Q., et al. (2016). Protective effect of icariin against TGF-β1-induced injury of rat Leydig cells. Natl. J. Androl. 22, 867–871.
Yano, I., and Hoshino, R. (2006). Effects of 17β-estradiol on the vitellogenin synthesis and oocyte development in the ovary of kuruma prawn (Marsupenaeus japonicus). Comp. Biochem. Physiol. Part A Mol. Integr. Physiol. 144, 18–23. doi: 10.1016/j.cbpa.2006.01.026
Zaccolo, M., and Movsesian, M. A. (2007). cAMP and cGMP signaling cross-talk: role of phosphodiesterases and implications for cardiac pathophysiology. Circ. Res. 100, 1569–1578. doi: 10.1161/CIRCRESAHA.106.144501
Zhang, S., Wang, X., Yin, P. F., Yu, H. F., Li, B., Li, X. Y., et al. (2007). Effects of icariin on the development of ovary and uterus in sexually matured female rats. J. Tradit. Chin. Vet. Med. 26, 15–18.
Zhang, Y. (2011). Developmental Changes in Concentrations of Vitellin, Vitellogenin and Vitellogenin Gene Expression During the Ovarian Development of Swimming Crab, Portunus trituberculatus. Master’s thesis, Shanghai Ocean University, Shanghai.
Zheng, X. C., Chi, C., Xu, C. Y., Liu, J. D., Zhang, C. Y., Zhang, L., et al. (2019). Effects of dietary supplementation with icariin on growth performance, antioxidant capacity and non-specific immunity of Chinese mitten crab (Eriocheir sinensis). Fish Shellfish Immunol. 90, 264–273. doi: 10.1016/j.fsi.2019.04.296
Zhou, W. H., Rahimnejad, S., Lu, K. L., Wang, L. N., and Liu, W. B. (2018). Effects of berberine on growth, liver histology, and expression of lipid-related genes in blunt snout bream (Megalobrama amblycephala) fed high-fat diets. Fish Physiol. Biochem. 45, 83–91. doi: 10.1007/s10695-018-0536-7
Keywords: Eriocheir sinensis, icariin, estrogen synthesis, vitellogenesis, oocyte development
Citation: Zheng X, Liu W, Liu J, Zhang C, Zhang L, Gao F, Zhang D and Chi C (2020) Dietary Supplementation With Icariin Affects Estrogen Synthesis, Vitellogenesis, and Oocyte Development in the Chinese Mitten Crab, Eriocheir sinensis. Front. Mar. Sci. 7:161. doi: 10.3389/fmars.2020.00161
Received: 01 November 2019; Accepted: 02 March 2020;
Published: 19 March 2020.
Edited by:
Bahram Falahatkar, University of Guilan, IranReviewed by:
Erchao Li, Hainan University, ChinaCopyright © 2020 Zheng, Liu, Liu, Zhang, Zhang, Gao, Zhang and Chi. This is an open-access article distributed under the terms of the Creative Commons Attribution License (CC BY). The use, distribution or reproduction in other forums is permitted, provided the original author(s) and the copyright owner(s) are credited and that the original publication in this journal is cited, in accordance with accepted academic practice. No use, distribution or reproduction is permitted which does not comply with these terms.
*Correspondence: Cheng Chi, Y2hpY2hlbmdAbmphdS5lZHUuY24=
Disclaimer: All claims expressed in this article are solely those of the authors and do not necessarily represent those of their affiliated organizations, or those of the publisher, the editors and the reviewers. Any product that may be evaluated in this article or claim that may be made by its manufacturer is not guaranteed or endorsed by the publisher.
Research integrity at Frontiers
Learn more about the work of our research integrity team to safeguard the quality of each article we publish.