- 1Department of Marine Biology, Texas A&M University at Galveston, Galveston, TX, United States
- 2Department of Wildlife and Fisheries Sciences, Texas A&M University, College Station, TX, United States
- 3Department of Oceanography and Coastal Sciences, Louisiana State University, Baton Rouge, LA, United States
- 4Galveston Laboratory, Southeast Fisheries Science Center, National Oceanic and Atmospheric Administration, Galveston, TX, United States
Offshore coral reefs in the Gulf of Mexico (GOM) are unique model systems for examining the mechanisms structuring reef fish communities due to their substantial geographic isolation, and the presence of replicate experimental units of both low (mid-shelf) and high coral diversity (shelf-edge) reefs. Here, we examined the species assemblage structure of juvenile and adult fishes at two mid-shelf reefs (Sonnier and Stetson Bank) and two shelf-edge reefs (East and West Flower Garden Banks) in the northwestern GOM to evaluate the relative importance of habitat (i.e., coral diversity) vs. recruitment in structuring resident fish assemblages. Visual reef fish surveys (n = 400) were conducted at the four coral reefs during two seasons, spring-early summer and late summer-fall in 2009 and 2010. Two depth zones were surveyed at each reef, representing the reef crest (15–23 m depth) and upper slope (23–30 m depth) habitats. Seasonal variability in recruitment to both mid-shelf and shelf-edge reefs was observed, with higher mean juvenile density and diversity (H′) observed during the late season at all reefs in 2009 and all but Stetson in 2010, supporting an early fall recruitment peak. Likewise, considerable inter-annual variability in juvenile recruitment was observed, with significantly lower juvenile density and H′ observed at mid-shelf reefs in 2010 relative to 2009. Species diversity was strongly linked to coral diversity, with greater reef fish diversity consistently observed at shelf-edge relative to mid-shelf reefs. Observed differences in the composition of juvenile and adult assemblages at mid-shelf reefs suggest that reef fish communities at these reefs were more strongly influenced by post-settlement processes (e.g., juvenile mortality) than shelf-edge reefs, which may be a function of several limiting factors (e.g., predation, coral diversity, water quality). Results indicate that reef fish assemblages associated with mid-shelf and shelf-edge reefs in the northwestern GOM may have sufficient stabilizing mechanisms in place to facilitate recovery from anomalous recruitment events. The strong, reef type-specific differences in assemblage composition observed throughout the study indicate that mid-shelf and shelf-edge coral reefs may fill different functional roles for demersal fishes in the northwestern GOM.
Introduction
An extensive body of community-level research conducted in numerous coral reef ecosystems reveals substantial variability in the species composition, spatial distribution, and temporal stability of resident fish assemblages (e.g., Sale, 2004; Mellin et al., 2016). As a result, it has been widely suggested that the primary mechanisms regulating reef fish community structure may vary considerably as a function of reef type, reef context, and geographic region (e.g., Beukers and Jones, 1998; Friedlander et al., 2003; Darling et al., 2017). A comprehensive understanding of local community dynamics is necessary for the development of effective and spatially explicit management strategies (Bohnsack and Ault, 1996; Sale, 2004). Thus, identifying processes responsible for maintaining community structure and biodiversity within reef-associated fish assemblages is critical as coral reef ecosystems worldwide are threatened by overfishing and habitat degradation (Newman et al., 2006; MacNeil et al., 2015).
Patterns of fish recruitment to coral reefs typically show a large degree of spatiotemporal variability (Doherty and Williams, 1988; Sale, 2004), and the relative importance of pre- and post-recruitment mechanisms in structuring reef fish communities has been widely debated in the literature (Armsworth, 2002; Smallhorn-West et al., 2017). Multiple small-scale studies on patch reefs have indicated that the community structure of adult fishes may be determined almost entirely by the initial species composition of juveniles recruiting from the plankton, with little evidence of post-settlement population regulation (reviewed in Doherty, 2002; Shima et al., 2018). Several larger-scale studies have also suggested that cross-shelf gradients in the species composition of reef-associated fish communities (e.g., Williams, 1983) appear to be structured primarily by offshore-inshore gradients in the delivery or survival of planktonic recruits, rather than subsequent movement or differential mortality of juvenile and adult fishes between offshore and inshore reef habitats (Roberts, 1991; see Wismer et al., 2009; Burgess et al., 2010). However, other long-term studies have found the distribution and abundance of adult reef fish populations to be largely independent of spatiotemporal fluctuations in recruitment and relatively consistent within a given reef type, suggesting that habitat characteristics and biotic interactions may ultimately determine the community structure and persistence of coral reef-associated fish assemblages in some systems (e.g., Robertson et al., 1988; Yeager et al., 2017).
Biotic interactions such as competition and predation are inherently density dependent (Carr et al., 2002; Hixon, 2015), and therefore the relative importance of post-recruitment processes in structuring a fish assemblage will be strongly influenced by the local quality, composition, and structural complexity of available habitat (e.g., Grober-Dunsmore et al., 2007, 2008). Newly settled reef fishes typically experience high predator-induced mortality during the first few weeks of life (Almany and Webster, 2006), and the availability of appropriately sized refuges within the reef structure can greatly influence the survival rates of new recruits as well as the species composition of individuals reaching maturity from a given cohort (Jones, 1988; Beukers and Jones, 1998; Syms and Jones, 2000). Refuge availability may be limiting even on a relatively large reef complex if the structured habitat varies greatly in quality, and numerous studies on coral reefs have found positive correlations between topographic complexity and the local density and diversity of resident fishes (Almany, 2004; Bejarano et al., 2015). Similar positive relationships between reef fish abundance and live coral cover have also been documented (Roberts and Ormond, 1987; Holbrook et al., 2008), and recent research has demonstrated that in certain coral reef systems, the diversity and species composition of corals within a given reef habitat may directly determine the diversity and community structure of the resident fish assemblage (Messmer et al., 2011).
The aim of the current study was to characterize the community structure of fish assemblages associated with mid-shelf and shelf-edge coral reefs in the northwestern Gulf of Mexico (GOM). Offshore coral reefs in the GOM represent a unique model system for examining the mechanisms structuring reef fish communities because they show an unusual degree of geographic isolation, but also contain replicate experimental units of both low coral diversity (mid-shelf) and high coral diversity (shelf-edge) reef habitats. Additionally, these coral reefs represent much of the hard-bottom habitat available to demersal fishes in the northern GOM, and they may be critical to the maintenance of reef fish populations throughout this region (Dennis and Bright, 1988; Schmahl et al., 2008; Hickerson et al., 2012; Voss et al., 2014). Here, we examined the species composition of juvenile and adult fishes at two low diversity coral reefs (located on the mid shelf) and two high diversity coral reefs (located on the shelf edge) in the northwestern GOM to evaluate the relative importance of habitat (i.e., coral diversity, depth) vs. recruitment in structuring resident fish assemblages. We also compared large-scale settlement patterns between reef types in an attempt to characterize the functional roles of mid-shelf and shelf-edge reef habitats within this continental shelf system.
Materials and Methods
Study Area
Four coral reefs were included in the study: two mid-shelf reefs [Sonnier Bank (SONN), Stetson Bank (STET)] and two shelf-edge reefs [East Flower Garden Bank (EFGB), West Flower Garden Bank (WFGB)]. Based on the species richness of scleractinian corals, both mid-shelf reefs were considered to be low coral diversity (<12 species), while both shelf-edge reefs were classified as high coral diversity (>20 species).
The two mid-shelf reefs (SONN, STET) are located approximately 110 km offshore, cresting at depths of 18–20 m (Figure 1). Both reefs consist of relatively bare siltstone-claystone outcroppings characterized in limited areas of the reef crest and upper slope by Millepora-Sponge benthic communities (Rezak et al., 1990). Apart from the encrusting hydrozoan Millepora spp., which represents approximately 30% of benthic coverage on the crests of SONN and STET, the majority of scleractinian corals species occur only in small numbers as isolated colonies (Schmahl et al., 2008; Hickerson et al., 2012). The establishment of more extensive hermatypic coral communities within this reef type is precluded by the high levels of turbidity and low winter temperatures (<16°C) associated with mid-shelf waters in the GOM (Rezak et al., 1990).
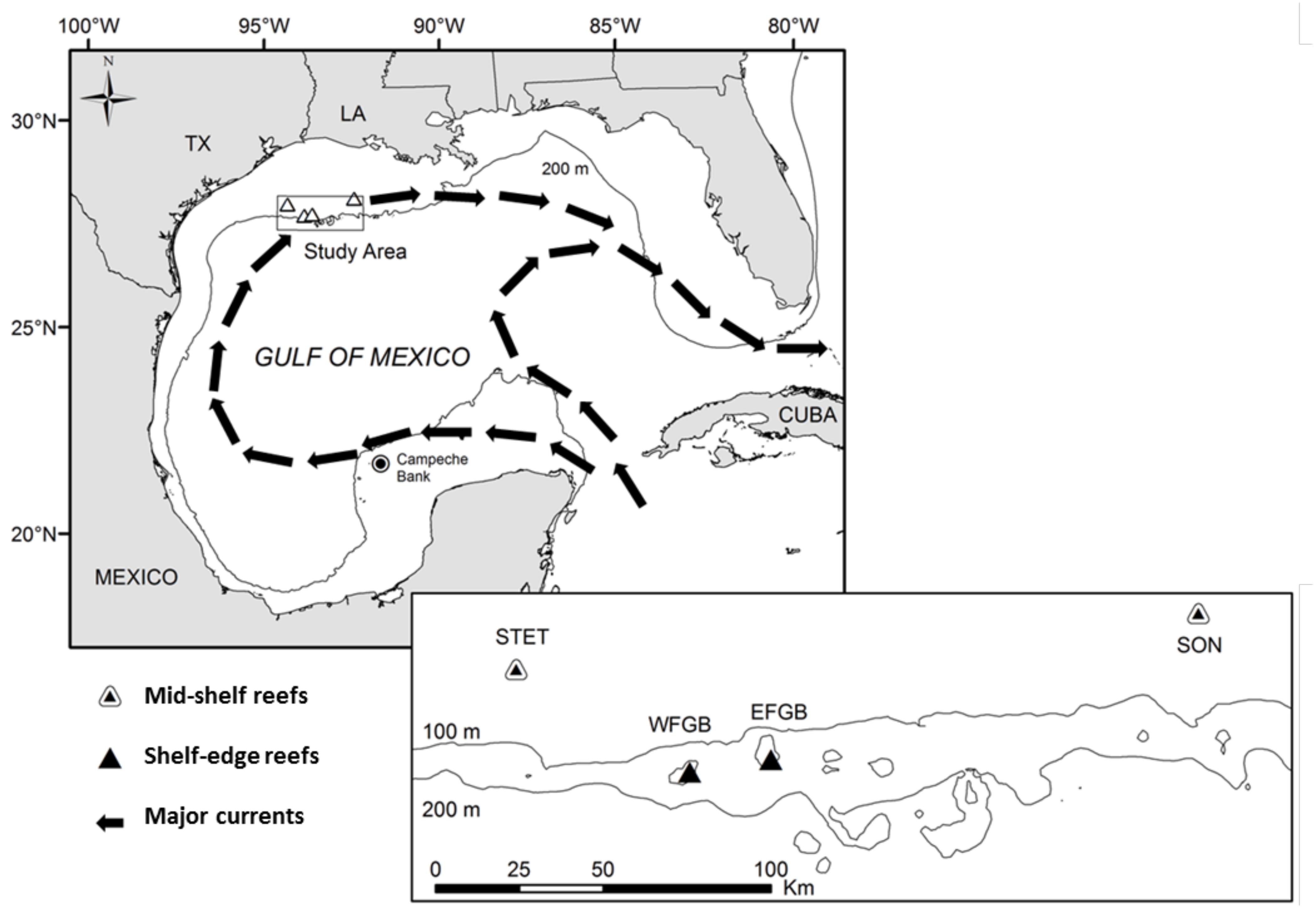
Figure 1. Regional context of study reefs in the Gulf of Mexico (GOM). Arrows denote the directional flow of major basin-wide currents, and a rectangle delineates the study area (and location of map inset) along the northern continental shelf. Map inset illustrates the bathymetry of the study area in greater detail and shows the spatial arrangement of mid-shelf (Sonnier, SON; Stetson, STET) and shelf-edge (East Flower Garden Bank, EFGB; West Flower Garden Bank, WFGB) reef sites.
The two shelf-edge reefs (EFGB, WFGB) are complex carbonate reef caps located approximately 185 km offshore on the outer continental shelf, where oceanic water and a more stable temperature regime facilitate the development of extensive Diploria-Montastrea-Porites coral communities throughout the reef crest (≈ 20 m) and upper slope habitat zones (Rezak et al., 1990; Johnston et al., 2016). Both EFGB and WFGB are largely dominated by colonies of the hermatypic brain corals Montastrea franski and Montastrea faveolata and the star coral Diploria strigosa, although several other species (particularly Porites spp.) are also present in fairly extensive colonies (Schmahl et al., 2008). The benthic coral communities at shelf-edge reefs in the northwestern GOM are less diverse but similar in species composition to coral communities in the Caribbean (Schmahl et al., 2008), and coral coverage at both EFGB and WFGB is high (45–52%) (Johnston et al., 2016).
Three of the reefs represented in this study (EFGB, WFGB, and STET) are located within the boundaries of the Flower Garden Banks National Marine Sanctuary (FGBNMS), where federal regulations prohibit anchoring of vessels, commercial fishing, and spearfishing, although recreational hook-and-line angling is still permitted. Only SONN is located outside of the sanctuary boundaries, and thus this reef is vulnerable to habitat damage as a result of vessel anchorage and commercial fishing activities, as well as selective removal of large, upper-trophic level fishes by recreational spearfishers (Schmahl et al., 2008). Recent sanctuary expansion has been proposed which would result in the future inclusion of SONN in the FGBNMS.
Reef Fish Surveys
Fish surveys were conducted from May to October in 2009 and 2010. This sampling period was chosen to correspond with potential periods of high recruitment for juvenile reef fishes, based on documented peaks in larval abundance that occur from spring to early fall in the FGBNMS when water temperatures are the warmest (McGowan, 1985). The sampling period was divided into spring-early summer (May 15–July 15) and late summer-fall (August 1–October 1) sampling seasons, and all reefs in the study were surveyed at least once during each sampling season (Table 1). Two depth zones were surveyed at each reef, representing the reef crest (15–23 m depth) and upper slope (23–30 m depth) habitats.
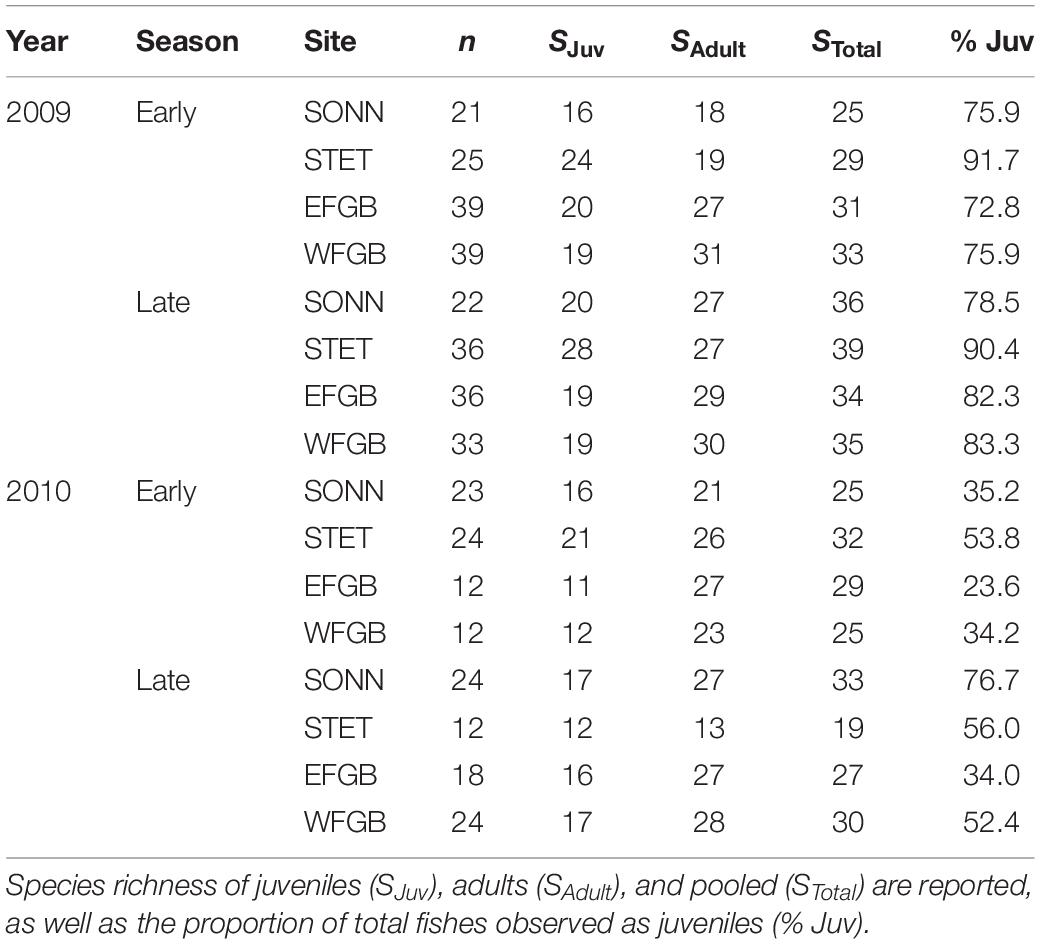
Table 1. Summary of survey effort in 2009 and 2010, with the number of transects (n) conducted at East Flower Garden Bank (EFGB), West Flower Garden Bank (WFGB), Sonnier Bank (SONN), and Stetson Bank (STET) during each sampling period.
Visual fish counts were conducted with SCUBA on line transects 5-m in length and 2-m in width. All fish within a given transect area were identified to the lowest possible taxa and assigned an age class based on body size and coloration, either juvenile (for juveniles and young-of-the year) or adult (for sub-adult and adult individuals). Fish were enumerated in order of encounter to minimize diver bias or double counting, and transect locations were designated using a stratified random sampling design, with divers assigned randomly generated compass headings and numbers of fin-kick cycles within each depth zone prior to every dive (approximately 2–3 transects were conducted per dive). Sampling surveys took place at multiple mooring locations at each reef in order to facilitate representative sampling across the reef crest.
In 2009, measurements of rugosity (defined here as the ratio between substrate contour and straight-line distance) were taken along each transect to serve as an indicator of habitat complexity. Rugosity was measured by draping a weighted chain along the contour of the reef and measuring the length of chain needed to cover a 1-m linear distance. Three measurements of rugosity were taken at regular intervals along each transect, and the rugosity estimate was reported as the mean value of these three measurements. In 2010, vessel limitations resulting from the Deepwater Horizon oil spill limited our sampling effort and rugosity measurements were not conducted during this survey year.
Data Analysis
Density was calculated for each transect as the total number of fishes (all species combined) encountered per square meter. The assumptions of normality and homogeneity of variance were tested and values were ln-transformed before analysis to correct for heteroscedasticity of variance among transects. Diversity was also calculated for each transect using the Shannon diversity index (H′):
where pi is the proportion of the total number of individuals encountered on the transect represented by species i. Seasonal, within-reef, and among-reef trends in fish density and diversity for each year of the study were analyzed using multivariate analysis of variance (MANOVA), with season, depth zone, and reef as the main effects. Post hoc differences in mean density and diversity were examined using Tukey’s honestly significant difference (HSD) method (α = 0.05). Because juvenile recruitment patterns are often influenced by different mechanisms than those structuring mature reef fish assemblages (Sale, 1991), juvenile and adult age classes were analyzed separately. The effects of rugosity on overall fish density and diversity were analyzed using linear regression.
Comparisons of assemblage structure among reefs for each year, sampling period, and age class were conducted in PRIMER v5 (Clarke and Warwick, 2001) using non-metric multidimensional scaling (MDS) models of ln-transformed density data. Data ordination was based on a Bray–Curtis similarity matrix and stress coefficients (residual modeling error) of 0.2 were treated as critical values to evaluate goodness-of-fit for each MDS model in two dimensions (Clarke and Warwick, 2001). Pairwise analysis of similarity (ANOSIM) permutation procedures were used to analyze the degree of overlap and test for differences in juvenile and adult assemblage structure among reefs for each sampling season and year of the study (999 permutations, α = 0.05). Pairwise ANOSIM procedures were also performed on untransformed juvenile and adult presence absence data within each reef in order to compare the species present in the mature fish assemblage with the species composition of newly settled recruits.
Results
Assemblage Composition
In total, 11,234 fishes representing 70 species from 24 families were observed in 2009, and 7,027 fishes representing 64 species from 23 families were observed in 2010 (Supplementary Table S1). Overall species richness (all transects pooled) was fairly similar among reefs during both survey years, ranging from 40 to 43 species observed at each reef in 2009 (43–72 transects surveyed per reef in 2009) and 34–39 species observed at each reef in 2010 (30–47 transects surveyed per reef in 2010). Pomacentrids and labrids were the dominant families at both mid-shelf and shelf-edge reefs in 2009, together accounting for 88–92% of the total fish assemblage at each reef (Figures 2A,B). In 2010, these two families remained numerically dominant (75–85%) at both shelf-edge reefs (EFGB, WFGB); however, at the mid-shelf reefs (SONN, STET), blenniids (primarily the seaweed blenny Parablennius marmoreus) also made up a significant proportion of the fish assemblage, representing 21% of all fish observed at SONN and 12% at STET. Relative abundances of pomacentrids and labrids were fairly equal at the two shelf-edge reefs in 2009, while pomacentrids (65–69%) were notably more abundant than labrids at the two mid-shelf reefs. In 2010, pomacentrids were more abundant (46–63%) than labrids at all four reefs.
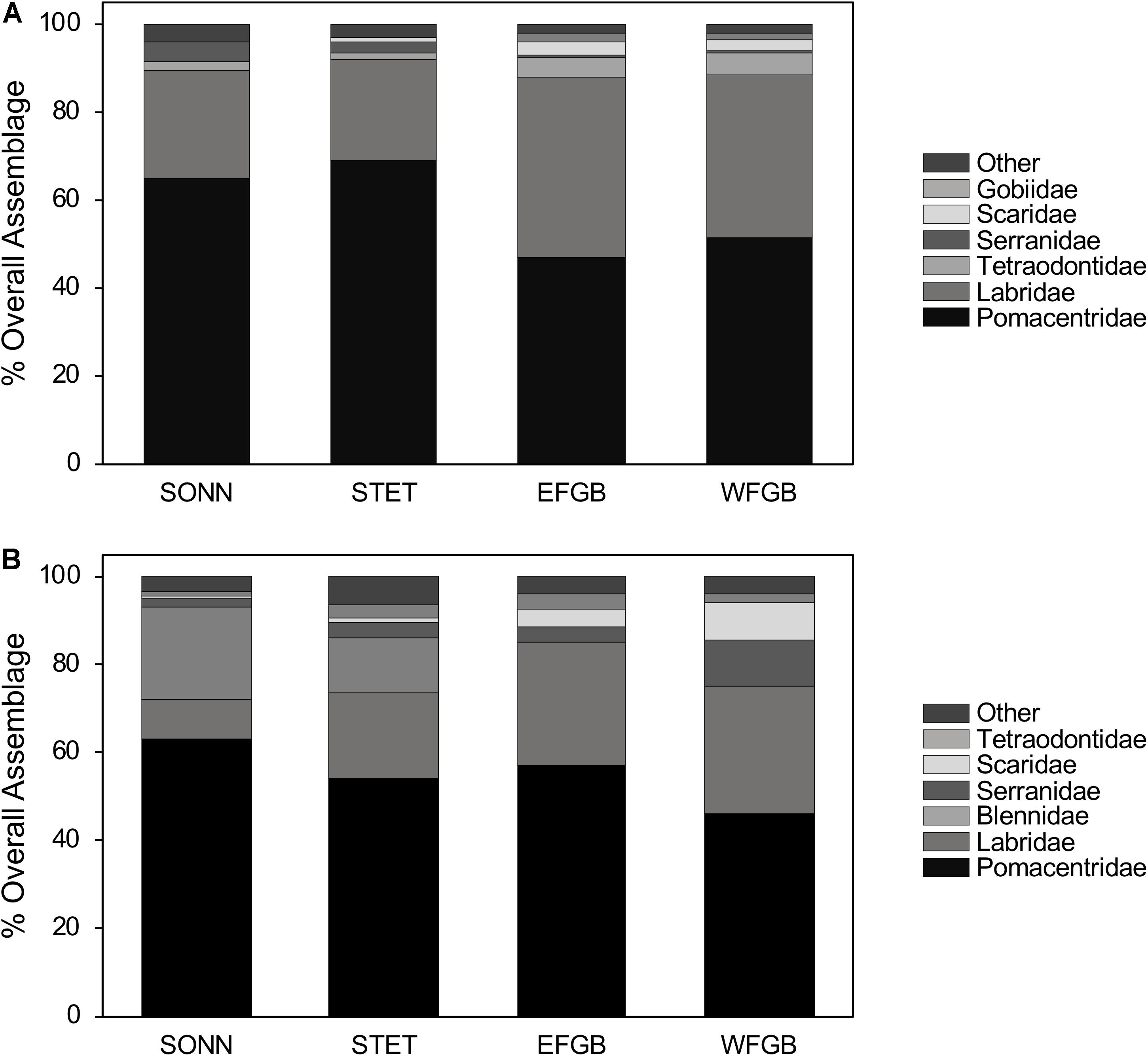
Figure 2. Percent composition of the six numerically dominant reef fish families present at each reef for 2009 (A) and 2010 (B). Both juveniles and adults were included in the analysis, and figures represent the pooled values from all transects conducted within each reef during a given year.
Spatiotemporal Trends in Abundance and Diversity
Mean densities of juvenile reef fishes (all species pooled) varied significantly by reef, season, and depth zone for both years of the study (ANOVAs, P < 0.01; Figures 3A–D). In 2009, mean juvenile fish density was significantly higher at STET than at the other mid-shelf reef (SONN) or either of the shelf-edge reefs (EFGB, WFGB) (Tukey HSD, P < 0.05). Mean density was also significantly higher during the late season surveys and in the shallow depth zone. There was a significant interaction between reef and depth zone (ANOVA, P < 0.001); juvenile fish densities were relatively similar between shallow and deep habitats at EFGB and WFGB, but were significantly higher on the shallow reef crest at both mid-shelf reefs (SONN and STET). Mean densities of juvenile fishes were generally lower in 2010 than 2009 (Figures 3C,D), although significant differences were again detected among study sites (ANOVA, P < 0.001). Patterns of seasonality and depth distribution in 2010 were similar to those observed in 2009, with significantly higher densities during the late sampling season and in the shallow depth zone. The one notable exception was STET, showing an opposite trend with lower densities of juveniles during the late sampling season and no apparent difference in juvenile densities between depth zones. The lowest mean densities of juvenile fishes were observed at the two shelf-edge reefs (EFGB, WFGB) for both years of the study.
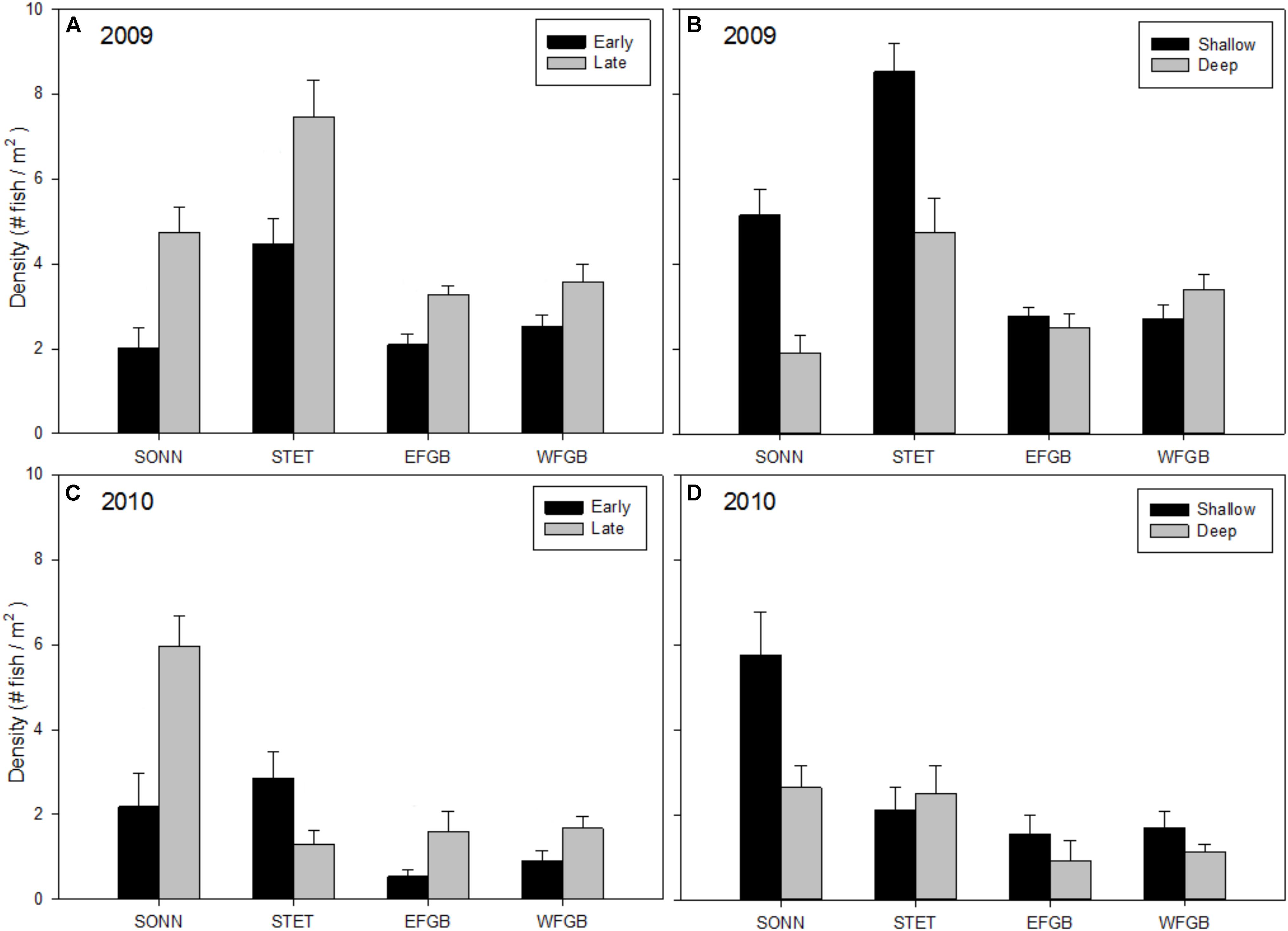
Figure 3. Mean densities of juvenile reef fish (all species pooled) observed at each reef for 2009 (A,B) and 2010 (C,D). Results were compared across seasons (A,C) and depth zones (B,D) for each year of the study, and error bars represent one standard error of the mean. Significant differences among study sites (if applicable) are denoted by uppercase letters in panel (A) for 2009 and (C) for 2010.
Mean densities of adult fishes showed less consistent patterns with respect to reef, season, and depth but all three factors were found to be significant (ANOVAs, P < 0.01; Figures 4A–D). In 2009, mean densities of adult fishes were significantly higher at the mid-shelf reef SONN than at the other three reefs (Tukey HSD, P < 0.05). There were also significant differences between seasons and depth zones, with higher densities of adults recorded in the shallow depth zone and the late sampling season. Again, these differences were apparent only at the two mid-shelf reefs. In 2010, densities of adult reef fishes were highly variable within all reefs surveyed, largely due to an increased presence of schooling planktivorous fishes on transects, and there were no significant differences among study sites, sampling seasons, or depth zones.
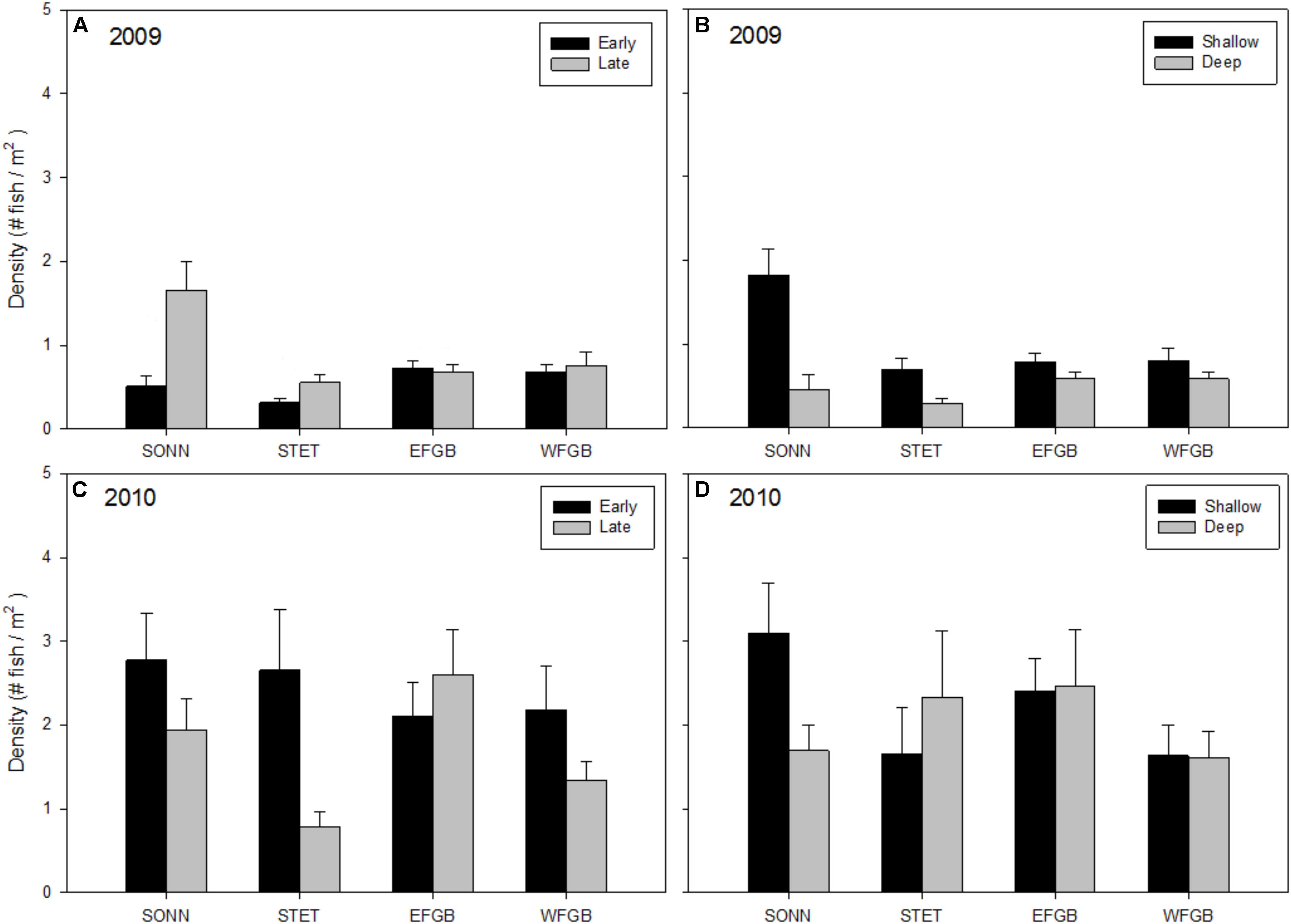
Figure 4. Mean densities of adult reef fish (all species pooled) observed at each reef for 2009 (A,B) and 2010 (C,D). Results were compared across seasons (A,C) and depth zones (B,D) for each year of the study, and error bars represent one standard error of the mean. Significant differences among study sites are denoted by uppercase letters in panel (A) for 2009. No significant differences among study sites were detected in 2010.
Shannon diversity (H′) for juvenile fishes differed markedly between the two survey years (Figures 5A–D). This was particularly evident at the mid-shelf reefs (SONN, STET) where certain tropical species observed as juveniles in 2009 were either absent (e.g., blue chromis Chromis cyanea, ocean surgeon Acanthurus bahianus, queen angelfish Holacanthus ciliaris) or present at markedly lower densities (e.g., bluehead wrasse, dusky damselfish Stegastes adustus, French angelfish Pomacanthus paru). A notable shift was also observed in juvenile species composition toward sub-tropical/temperate recruits, in particular, the cocoa damselfish (Stegastes variabilis), which represented a combined 29% of the juvenile fishes enumerated at the mid-shelf reefs in 2009 but accounted for nearly 60% of all juveniles observed in 2010 (and over 74% at SONN). In 2009, no significant difference in mean H′ was detected for juvenile fishes among study reefs, although mean H′ was significantly higher during the late sampling season (ANOVA, P < 0.001) and in the shallow depth zone (ANOVA, P < 0.01). In 2010, mean H′ differed among reefs (ANOVA, P < 0.001), and diversity of juvenile fishes was significantly lower at SONN than at the other three reefs surveyed (Tukey’s HSD, P < 0.05). However, no significant seasonal or depth-related effects on mean H′ were detected in 2010. For adult fishes, H′ remained fairly consistent between years (Figures 6A–D). Significant differences in H′ were found among reefs in both 2009 and 2010, and H′ of adult fishes at the two shelf-edge reefs (EFGB, WFGB) was significantly higher than at the two mid-shelf reefs (SONN, STET) for both years of the study (Tukey’s HSD, P < 0.05). In 2009, H′ was also significantly higher in the shallow depth zone at SONN and STET (ANOVA, P < 0.01), but was relatively similar between depth zones at EFGB and WFGB. For adult fishes, H′ was also statistically similar between depth zones on both mid-shelf and shelf-edge reefs in 2010.
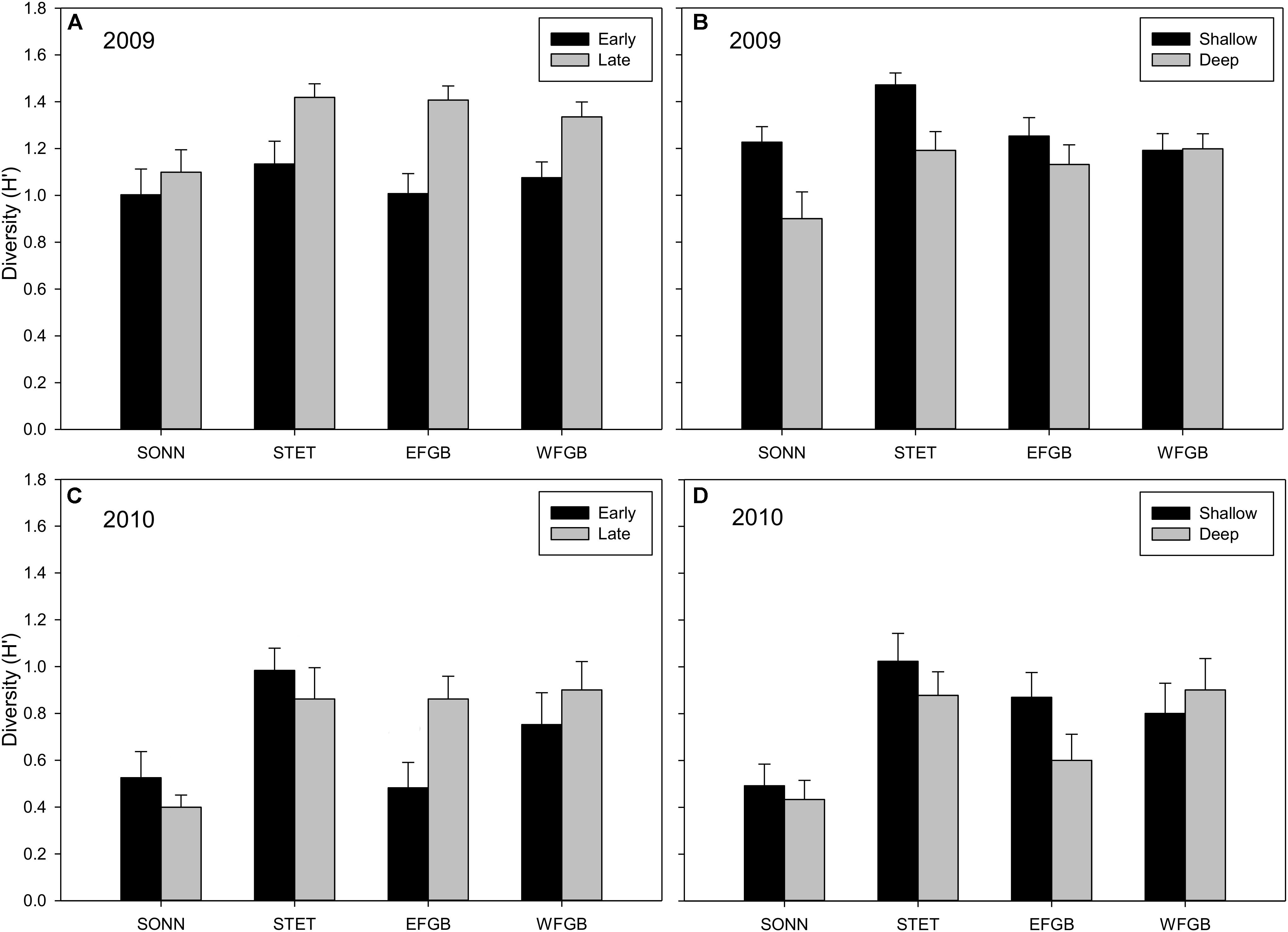
Figure 5. Mean Shannon–Wiener diversity estimates (H′) for juvenile reef fish observed at each reef in 2009 (A,B) and 2010 (C,D). Results were compared across seasons (A,C) and depth zones (B,D) for each year of the study, and error bars represent one standard error of the mean. Significant differences among study sites are denoted by uppercase letters in panel (C) for 2010. No significant differences among study sites were detected in 2009.
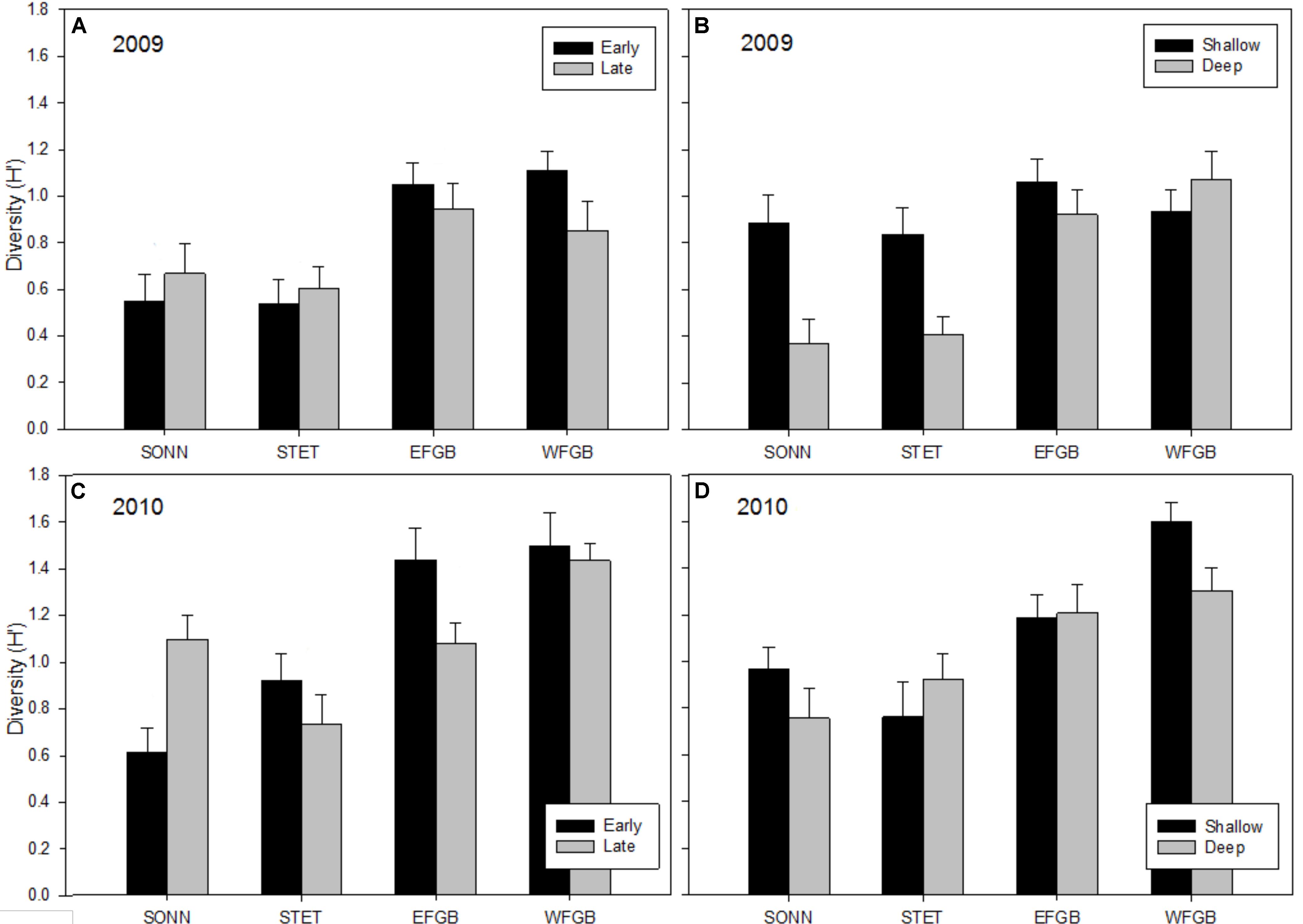
Figure 6. Mean Shannon–Wiener diversity estimates (H′) for adult reef fish observed at each reef in 2009 (A,B) and 2010 (C,D). Results were compared across seasons (A,C) and depth zones (B,D) for each year of the study, and error bars represent one standard error of the mean. Significant differences among study sites are denoted by uppercase letters in panel (A) for 2009 and (C) for 2010.
Microhabitat Selection
The effects of habitat complexity (i.e., rugosity) on overall reef fish density and H′ were examined during the first year of surveys (2009) and the influence of rugosity on fish distribution differed between mid-shelf and shelf-edge reefs (Figures 7A,B). At the mid-shelf reefs (SONN, STET), there was a positive relationship between rugosity and fish distribution; linear regressions of rugosity against density and diversity (H′) were both significant (P < 0.001). No significant relationships between rugosity and reef fish density or diversity were detected within the shelf-edge reefs (P = 0.524, 0.361).
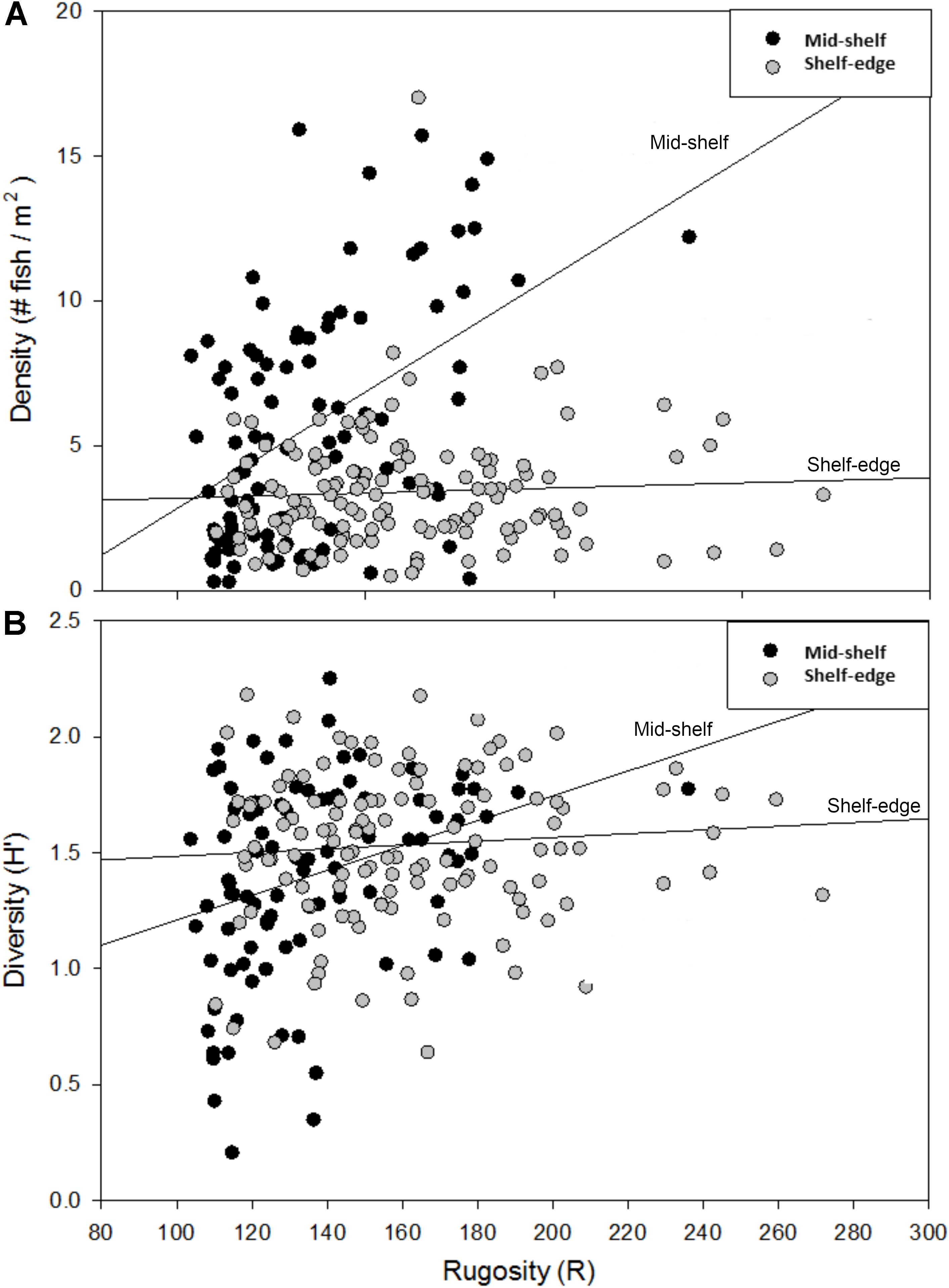
Figure 7. Relationship between habitat rugosity and the density (A) and diversity (B) of reef fishes (both age classes pooled) observed at mid-shelf and shelf-edge reefs during the 2009 study year. Trend lines represent the linear regression of transect density and diversity (H′) against transect rugosity within each bank type (mid-shelf vs. shelf edge). Relationships were statistically significant for both density (r2 = 0.23) and diversity (r2 = 0.10) at mid-shelf reefs, while neither relationship was significant at shelf-edge reefs.
Comparison of Assemblage Structure Among Reefs
Density data for juvenile and adult reef fishes were analyzed separately both by survey year and by sampling season within each year using non-metric MDS ordination (Figure 8). Stress coefficients for all MDS models were below the experimental cutoff of 0.20 with the exception of the two juvenile models from 2009, which showed slightly higher values (0.22 for both sampling seasons). In general, MDS models with stress coefficients ranging from 0.20 to 0.30 are considered marginal for interpretation, particularly for ordinations based on a small to moderate sample size (<50 data points) (Clarke and Warwick, 2001). However, because both MDS models in 2009 were based on relatively large samples (124 and 127 transects, respectively), the juvenile models from this year were considered acceptable for analysis.
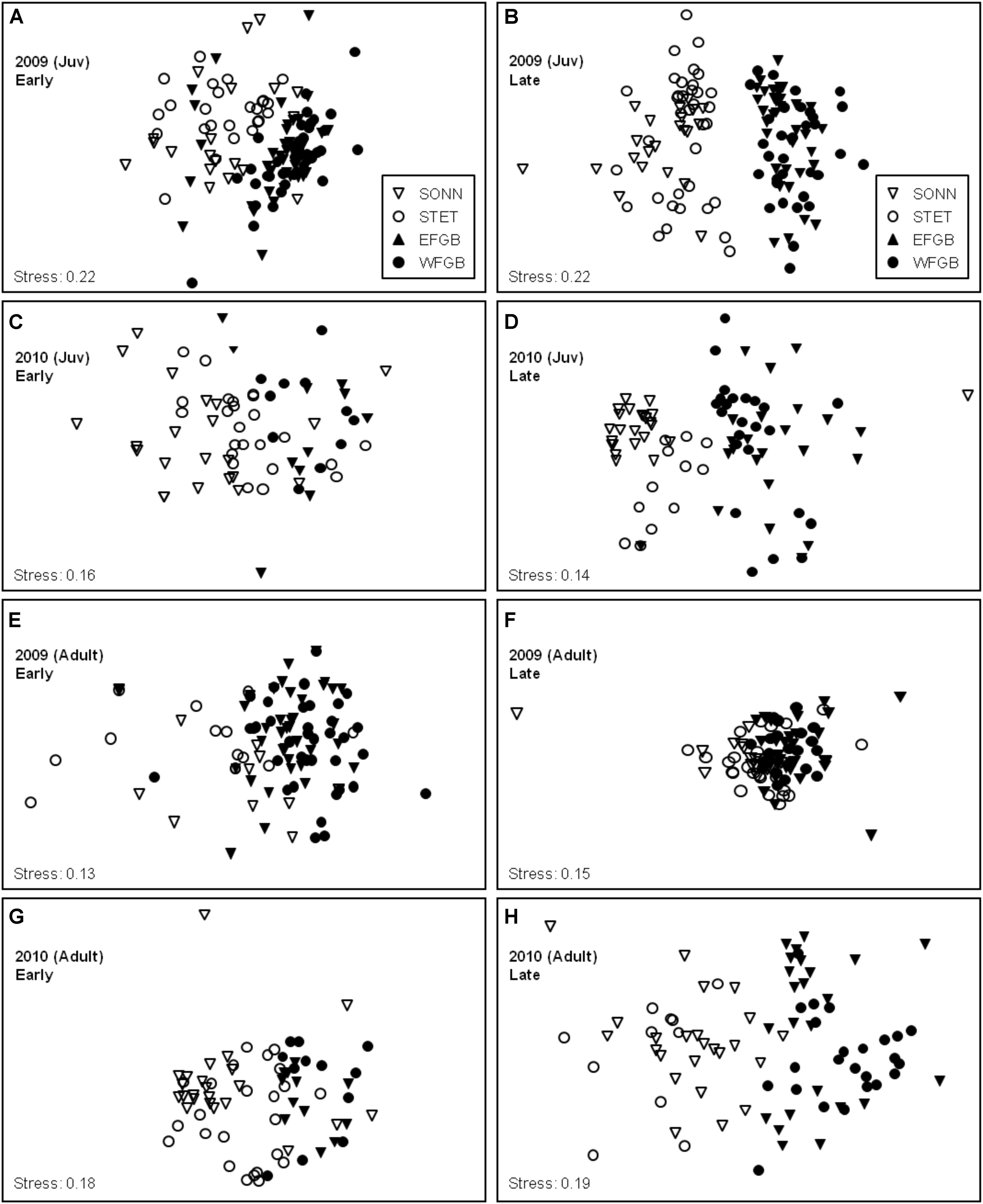
Figure 8. Non-metric multidimensional scaling (MDS) ordinations of juvenile (A–D) and adult (E–H) species density data for all four study reefs for 2009 (A,B,E,F) and 2010 (C,D,G,H). Early and late sampling seasons were analyzed separately within each study year for both age classes. Symbol legends are shown in panels (A) and (B). Replicates from low diversity (mid-shelf) study sites (SON and STET) are represented by open symbols (▽, ∘, respectively) and high diversity (shelf edge) study sites (EFGB and WFGB) are represented by filled symbols (▼, ∙, respectively).
Overall, MDS plots of both juvenile and adult data revealed remarkably consistent distinctions in species composition between the mid-shelf reefs (SONN, STET) and the shelf-edge reefs (EFGB, WFGB), but considerable overlap between the two sites within a given reef type (Figure 8). Pairwise ANOSIM permutation procedures were used to test the significance of these apparent groupings, and results from these pairwise tests were largely in agreement with the spatial ordinations observed in our MDS models. For both juvenile and adult fishes over the two years of surveys, all pairwise comparisons between different (i.e., mid-shelf vs. shelf-edge) reef types revealed significant differences in the community structure of fishes associated with each reef type (ANOSIM, P < 0.001). In contrast, few significant differences in community structure were found in pairwise comparisons within a given reef type (e.g., between two mid-shelf reefs), particularly for adult fishes.
In 2009, no significant differences in juvenile community composition were detected between the two shelf-edge reefs (EFGB, WFGB) for either sampling season (ANOSIM, P > 0.05). Community composition of juvenile fishes at the two mid-shelf reefs (SONN, STET) differed significantly during the early sampling season (ANOSIM, R = 0.242, P < 0.001) but was similar during the late sampling season (P > 0.05). Juvenile assemblages at both mid-shelf reefs during the early season were numerically dominated by three species; bluehead wrasse Thalassoma bifasciatum, cocoa damselfish, and purple reef fish Chromis scotti, and differences in the relative abundances of these species at SONN and STET were responsible for structuring the majority (61%) of the dissimilarity in juvenile assemblage structure within the mid-shelf reef type during early season surveys (SIMPER). No significant within-reef type differences were found for adult fishes in 2009; pairwise comparisons of adult assemblage structure showed similar species compositions between the two mid-shelf reefs (SONN, STET) and between the two shelf-edge reefs (EFGB, WFGB) during both early and late sampling seasons (ANOSIM, P > 0.05). In 2010, findings closely resembled those from 2009, with one notable difference. While both age classes at the two shelf-edge reefs (EFGB, WFGB) were again similar in species composition for each sampling season (ANOSIM, P > 0.05), significant differences in assemblage structure between the two mid-shelf reefs (SONN, STET) were observed for both juveniles and adults during the early sampling season in 2010 (ANOSIM, RJuv = 0.170, P < 0.001; RAdult = 0.212, P < 0.01). The majority (53%) of early season dissimilarity in adult assemblage structure between SONN and STET was attributed to differences in the relative abundance of the seaweed blenny P. marmoreus (SIMPER). The effects of this event were short-lived and by the late 2010 sampling season, seaweed blenny densities had returned to 2009 levels and adult assemblages within the low-diversity reef type (i.e., SONN, STET) were once again similar (P > 0.05).
Juvenile and Adult Species Presence Within Each Reef
For both survey years, 85–100% of fish species observed as juveniles at the two shelf-edge reefs (EFGB, WFGB) were also represented in the adult assemblages (Figures 9A,B). In 2009, just three species at EFGB and two at WFGB were present only as juveniles, and in 2010, there were no such species at EFGB and only one at WFGB. However, at the two mid-shelf reefs, the number of species observed only as juveniles was markedly higher (9 species at SONN and 12 at STET in 2009; 14 species at SONN and 7 at STET in 2010) (Figure 9). During each year of surveys, 27–61% of all juvenile fish species recruiting to each mid-shelf reef were never observed as adults, and pairwise comparisons between age classes at both mid-shelf reefs (SONN, STET) revealed significant differences in species presence between the adult fish assemblage and juvenile recruits (ANOSIM, P > 0.05). Examples of taxa observed at mid-shelf reefs as juveniles but not adults included cherubfish Centropyge argi, painted wrasse Halichoeres caudalis, sailfin blenny Emblemaria pandionis, yellowtail damselfish Microspathodon chrysurus, and Sparisoma spp. In contrast, only 0–13% of juvenile fish species recruiting to shelf-edge reefs over each survey year were never observed as adults, and pairwise within-reef comparisons revealed similar juvenile and adult species presence at both EFGB and WFGB (ANOSIM, P < 0.05).
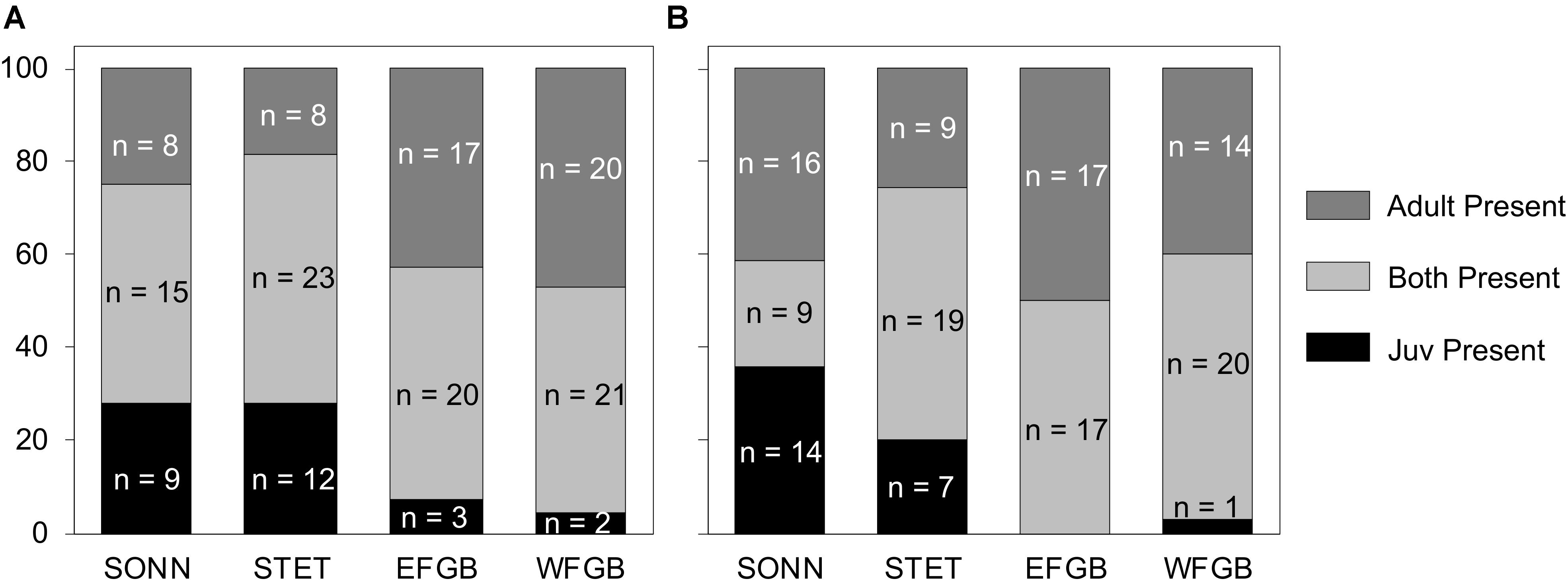
Figure 9. Percentage of reef fish species present only as juveniles (black), only as adults (dark gray), and as both adults and juveniles (light gray) within the two low diversity (mid-shelf) reef sites (SONN, STET) and the two high diversity (shelf edge) reef sites (EFGB, WFGB) for 2009 (A) and 2010 (B). The raw species counts (n) within each category at each reef type are also given.
Discussion
Recruitment to both mid-shelf and shelf-edge reefs showed a strong seasonal component, with higher juvenile density and assemblage diversity (H′) observed during the late (August 1–October 1) season at all reefs in 2009 and all but STET in 2010, supporting the early fall recruitment peak previously suggested for coral reef fishes in the northwestern GOM (Rooker et al., 1997). The period of increased juvenile density observed in our study corresponds with a period of elevated mean sea surface temperature and annual peak eddy activity in the region (Limer et al., in review), and similar increases in recruitment have been widely observed in other coral reef systems during the summer months when oceanographic conditions are optimal for larval growth and survival (e.g., Doherty and Williams, 1988; Adjeroud et al., 1998; Chittaro and Sale, 2003). Seasonality in recruitment is generally most pronounced on high-latitude reefs occupying tropical-temperate transition zones (Doherty, 1991; Beck, 2015; Komyakova and Swearer, 2019), which is the case for both the mid-shelf and shelf-edge reefs in our study. However, the most pronounced late-season increases in juvenile density for both survey years occurred at the two mid-shelf reefs (SONN, STET), where oceanographic conditions are influenced by seasonal coastal processes [e.g., freshwater input from the Mississippi-Atchafalaya River System (MARS) is higher during late spring/early summer] and water temperatures are cooler during the winter months, potentially restricting the time frame of successful recruitment (see Rezak et al., 1990).
Vertical zonation of coral and reef fish community structure is well documented (Edmunds and Leichter, 2016; MacDonald et al., 2016). Still, depth-related differences in reef fish density and diversity were primarily observed at the mid-shelf (low coral diversity) reefs, with greater juvenile and adult densities observed at shallow depths near the reef crest. Perhaps this is not surprising due to the fact that hermatypic corals are primarily restricted to the shallower portions of the reef crest at both SONN and STET, with deeper areas characterized by less complex bedrock outcroppings and benthic algae. In contrast, coral coverage was high and more ubiquitous across both depth zones at the two shelf edge reefs (EFGB, WFGB), which may explain the relative similarity in reef fish density and diversity across the two depth zones sampled at these reefs. Still, our surveys were limited to the upper 30 m of the water column and it is likely that shifts in reef fish community structure may have been more evident at mesophotic depths (>30 m).
Juvenile density on both reef types displayed considerable inter-annual variability, with significantly reduced density and H′ observed in 2010. Previous studies on coral reef fishes have documented declines in the density and condition of newly settled recruits in response to cooler water temperatures (e.g., Sponaugle et al., 2006), and it is possible that poor recruitment in 2010 may have been influenced by the exceptionally cold preceding winter, which caused regional sea surface temperatures to remain 2–4°C below 2009 values until mid-May (Texas Automated Buoy System; TABS, Buoy V). Although reef fish assemblages in the northern GOM are composed of both tropical and sub-tropical/temperate taxa, decreased recruitment in 2010 appeared to be driven primarily by poor representation of tropical recruits, which may be particularly sensitive to sub-optimal thermal conditions (Sponaugle et al., 2006). The greatest inter-annual differences in recruitment occurred at the two mid-shelf reefs (SONN, STET) where winter water temperatures are lowest, and at each of these reefs in 2010, several tropical species observed as juveniles in 2009 were either absent or present at markedly lower densities. This was accompanied by a notable shift in juvenile species composition toward sub-tropical/temperate recruits, in particular, the cocoa damselfish, which dominated juvenile assemblages at mid-shelf reefs in 2010 relative to 2009 (60% of all juveniles observed in 2010). Considering that congeneric juveniles (Stegastes spp.) have been shown to aggressively inhibit recruitment of other tropical reef fish species (Risk, 1998), the exceptional success of cocoa damselfish at SONN in 2010 may have contributed to the seemingly anomalous increase in juvenile density, but significantly lower juvenile diversity, observed at this reef. Nevertheless, natural variability in recruitment is common in reef fishes (Sponaugle, 2015) and observed inter-annual differences may simply be a function of inherent stochasticity in the remote production and planktonic delivery of larval recruits. Indeed, oceanographic conditions in 2009 were likely more favorable for larval subsidy from Campeche Bank and Yucatan Shelf reefs to reach the northern GoM as the Loop Current pushed further northward, with eddies produced from this mesoscale feature crossing over the study reefs with greater frequency in 2009 relative to 2010 (Limer et al., in review). It is also important to note that the Deepwater Horizon oil spill occurred in the northern GOM in May of 2010, potentially explaining the decrease in juvenile densities observed in that year. However, given the lack of geographical overlap between the study reefs and the oil spill footprint, it is likely that the impact of the spill on fish density and community structure at the study sites was minimal (Johnston et al., 2013). Invasive Indo-Pacific lionfish (Pterois sp.) were also first observed at Sonnier Bank in fall of 2010; however, individuals were not documented at the other reefs until 2011 (Johnston et al., 2013). While potential negative effects of lionfish on reef fish community structure are well documented, it is unclear to what extent lionfish may have affected juvenile community structure and/or densities at our study reefs in 2010.
The assemblage structure of adult reef fishes in our study appeared to be fairly resilient to short-term fluctuations in recruitment, remaining consistent within and distinct between the two reef types. H′ of adult fishes was consistently highest at the two shelf-edge reefs (EFGB, WFGB) over both years of surveys, and MDS ordination revealed distinct differences in species composition between mid-shelf and shelf-edge reefs, but little to no separation within either reef type. Similar inshore-offshore gradients in diversity and species composition have been widely reported for reef fishes inhabiting tropical-temperate transition zones (e.g., Cheal et al., 2012), and cross-shelf gradients in diversity are often accompanied (or driven) by clear shifts from sub-tropical/temperate reef fish communities inshore (i.e., SONN, STET) to primarily tropical, coral reef-associated assemblages near the shelf edge (i.e., EFGB, WFGB), where the effects of winter cold fronts and terrigenous influences (e.g., sedimentation, nutrient enrichment) are buffered by deeper water and exposure to warm oceanographic currents (Dennis and Bright, 1988; Malcolm et al., 2010). These conditions also promote coral growth, and similar patterns of diversity between corals and fishes (higher fish diversity associated with higher coral diversity) consistent with those observed in the current study have been noted in multiple geographic regions (Bellwood and Hughes, 2001; Connolly, 2005; Burgess et al., 2010). Still, it has been widely debated whether cross-shelf gradients in reef fish community structure are established post-settlement as a direct result of changes in the benthic coral community (e.g., Adjeroud et al., 1998) or whether they are simply produced by cross-shelf gradients in recruitment (James et al., 2002; Burgess et al., 2010).
Similar to studies conducted in other regions (reviewed Burgess et al., 2010), natural correlations between reef type and distance offshore prevent us from definitively separating the effects of shelf position and coral diversity; however, the current study is also unique from many previous efforts (see James et al., 2002) in that our life stage-specific data allowed for the direct comparison of juvenile settlement patterns with mature fish assemblages at reefs occupying different shelf positions. Distinct differences in the composition of juveniles observed between the mid-shelf reefs (SONN, STET) and the shelf-edge reefs (EFGB, WFGB) indicates that an inshore-offshore recruitment gradient may exist for reef-dependent fishes in the northern GOM, likely established by decreased larval delivery or survivorship closer to the coast (see Roberts, 1991), where pelagic conditions are often strongly influenced by cold, low-salinity, nutrient-rich input from MARS (Rezak et al., 1990; reviewed in D’Sa and Dimarco, 2009). It is also possible that regional patterns of fish diversity are influenced by increased exposure of the shelf-edge reefs (EFGB, WFGB) to tropical larvae originating from the southern GOM or Caribbean Sea (Rezak et al., 1990; Schmahl et al., 2008; Kitchens et al., 2017). However, the notable distinction between juvenile and adult assemblages observed within both mid-shelf reefs (SONN, STET) also suggests that the species composition of mature fish communities associated with this reef type may not directly resemble the initial species composition of settlers. Therefore, while the supply of larval recruits to reefs in the northern GOM is likely influenced to some extent by shelf position, recruitment limitation may not be the primary determinant of adult community structure throughout this system, and initial patterns of settlement may be modified to a considerable extent by intrinsic characteristics of the reefs.
Habitat characteristics have been widely implicated in determining the local abundance and diversity of coral reef fishes in other regions (e.g., Grober-Dunsmore et al., 2008; Bejarano et al., 2015), and the relative influence of habitat-mediated interactions on juvenile survivorship and distribution is generally strongest when densities of recruits are at or near carrying capacity (see Beukers and Jones, 1998; Almany, 2003). Several studies have reported that while pre-settlement processes (i.e., larval supply and dispersal) appear to be the primary determinants of community structure on reef types characterized by high rugosity and extensive coral coverage (e.g., EFGB, WFGB), post-settlement processes (e.g., competition, predation) may be more critical on reef types where habitat complexity is low and refuge spaces are limited (e.g., SONN, STET) (Benfield et al., 2008). Predation is the primary agent of post-settlement mortality for reef fishes (Almany and Webster, 2006; Hixon, 2015), and at both SONN and STET, the large proportion (20–36%) of species observed as juveniles but not adults indicates that mortality rates of new recruits to these mid-shelf reefs may be substantial. Intense local predation on coral reefs can significantly reduce assemblage diversity by eliminating rarer species of recruits from persisting into the adult assemblage (e.g., Heinlein et al., 2010), and this may explain the consistently lower diversity of adult fishes observed at the two mid-shelf reefs (SONN, STET), as well as the strong associations between fish distribution and habitat rugosity (i.e., prey refuges). It is also possible that suboptimal physicochemical conditions (e.g., temperature, salinity) on mid-shelf reefs during cooler months contribute to greater overwinter mortality and vulnerability to predation for tropical species. In contrast, little evidence of post-settlement regulation was detected on the shelf-edge reefs (EFGB, WFGB), where the low densities and even spatial distribution (i.e., weak habitat associations) observed for juvenile fishes indicate levels of recruitment well below saturation. Adult reef fish assemblages at both EFGB and WFGB showed strong resemblance to the species composition of juvenile assemblage and well over 90% of all species recruiting to each reef over the course of the study appeared to successfully persist as mature populations, suggesting that community structure on these reefs is determined largely at time of settlement.
Both shelf-edge reefs in the current study (EFGB, WFGB) appeared to maintain stable resident fish assemblages that were more consistent and predictable in community structure than those observed at the mid-shelf reefs (SONN, STET). One possible explanation for this incongruity is a high rate of self-recruitment at EFGB and WFGB due to the extreme biogeographic isolation and regional oceanographic conditions along the outer continental shelf (Cowen et al., 2006; Schmahl et al., 2008; Hickerson et al., 2012). The closest coral reef-associated fish assemblages upstream from EFGB and WFGB occur on the offshore reefs of Campeche Bank (approximately 700 km to the south; Figure 1). Under ideal oceanographic conditions, predicted transport time (Lugo-Fernández, 2006) from this region would be expected to exceed the reported planktonic larval duration (PLD) for many of the dominant species in our surveys of the EFGB and WFGB (Victor, 1986; Wellington and Victor, 1989), and considering that ecologically relevant scales of connectivity for reef fish populations in the region are typically on the order of 10 to 100 km (Cowen et al., 2006), the contribution of larvae derived from the Caribbean is likely to be minimal. Nevertheless, the degree to which larval contribution from the southern GoM or Caribbean Sea affects juvenile recruitment (density and assemblage structure) at northern GOM reefs is likely temporally variable and dependent on the position of the Loop Current and associated eddies (see Kitchens et al., 2017; Limer et al., in review). This may explain the greater density and diversity of juvenile recruits observed in 2009 compared to 2010 across all reefs, as the Loop Current penetrated farther northward in 2009 (Rooker et al., 2013; Limer et al., in review).
The only other potential source of larval contribution to EFGB and WFGB is the extensive network of petroleum platforms spanning the northern GOM, but because the major currents approaching the edge of the continental shelf are oceanic in origin, the majority of these platforms are hydrologically downstream from EFGB and WFGB (Lugo-Fernández et al., 2001). Furthermore, surveys conducted on both mid-shelf and shelf-edge platforms have indicated that the diversity of reef fishes recruiting to these structures is markedly depleted relative to natural reefs in the region (Sonnier et al., 1976; Rooker et al., 1997; Voss et al., 2014), and therefore, it is likely that petroleum platforms generally act as a sink rather than a source of tropical recruits (see Lugo-Fernández et al., 2001). Given the low likelihood of EFGB or WFGB receiving significant subsidies of larval recruits from remote source locations, fish communities at these two shelf-edge reefs must be largely replenished through local production, which is possible given that oceanographic conditions in this region are generally favorable for larval retention (Lugo-Fernández et al., 2001).
If resident fish assemblages at our two shelf-edge coral reefs (EFGB, WFGB) are primarily self-replenishing, it is likely that larval subsidies originating from these reefs may also be largely responsible for the replenishment and regional persistence of many tropical species across the continental shelf (Dennis and Bright, 1988; see Malcolm et al., 2010). However, the magnitude and inshore extent of larval export from the EFGB and WFGB can be expected to vary from year to year as a function of regional oceanographic conditions in the GOM (Lugo-Fernández et al., 2001), and this may have contributed to observed interannual variability in the composition of newly recruited juveniles at our two mid-shelf reefs (SONN, STET). By chance, our surveys appeared to occur over one “good” recruitment year (2009) with favorable oceanographic conditions and one “poor” recruitment year (2010) where lower sea surface temperatures and increased freshwater inflow likely had a negative impact on the delivery and survivorship of pelagic larvae closer to the coast. Both mid-shelf reefs in 2010 showed decreased juvenile diversity and a marked shift in recruitment composition toward species commonly observed on platforms and nearshore reefs (e.g., cocoa damselfish, seaweed blennies) (Rooker et al., 1997; Dance et al., 2011), suggesting that in years or seasons when conditions are unfavorable, tropical recruits originating from shelf-edge coral reefs may be poorly represented across the continental shelf, and our mid-shelf reefs (SONN, STET) may receive a greater proportion of sub-tropical/temperate recruits from other mid-shelf reefs or production platforms. Such spatiotemporal variability in both the magnitude and source of recruitment is typical of coral-reef associated fishes and fluctuations in recruitment of tropical reef fishes have been widely attributed to stochastic oceanographic and climatic conditions in other regions (e.g., Victor, 1986; Doherty and Williams, 1988; Fontes et al., 2016; Shulzitski et al., 2016). However, results from the current study also suggest that the reef fish assemblages associated with mid-shelf and shelf-edge reefs in the northwestern GOM may have sufficient stabilizing mechanisms in place to facilitate recovery from anomalous recruitment events. The strong, reef type-specific differences in community composition observed throughout the study indicate that mid-shelf and shelf-edge coral reefs may fill different functional roles for demersal fishes in the northwestern GOM.
Data Availability Statement
Data supporting the conclusions of this article will be made available by the authors, without undue reservation, to any qualified researcher.
Ethics Statement
Ethical review and approval was not required for this animal study in accordance with the local legislation and institutional requirements. No animals were sampled or handled as part of this project.
Author Contributions
All authors designed the study and contributed to the final version of the manuscript. LW, MD, and JR performed the sampling surveys and wrote the manuscript. LW analyzed the data.
Funding
This study was funded in part by grants to JR from the NOAA Coral Reef Conservation Grant Program through the Gulf of Mexico Fishery Management Council.
Conflict of Interest
The authors declare that the research was conducted in the absence of any commercial or financial relationships that could be construed as a potential conflict of interest.
Acknowledgments
This work was carried out in cooperation with the Flower Garden Banks National Marine Sanctuary. We thank Emma Hickerson, the crew of the M.V. Fling, Sam Waltman, Marissa Nuttall, Jessica Lee, Anja Schulze, Sam Davenport, Larissa Kitchens, Richard Kraus, R. J. David Wells, Amie Hufton-Louchouarn, Kevin Buch, Sepp Haukebo, Brad Smith, Liam Carr, and several scientific divers from the Texas A&M University for their help in completing field work.
Supplementary Material
The Supplementary Material for this article can be found online at: https://www.frontiersin.org/articles/10.3389/fmars.2020.00152/full#supplementary-material
References
Adjeroud, M., Letourneur, Y., Porcher, M., and Salvat, B. (1998). Factors influencing spatial distribution of fish communities on a fringing reef at Mauritius, S.W. Indian Ocean. Environ. Biol. Fishes 53, 169–182.
Almany, G. R. (2003). Priority effects in coral reef fish communities. Ecology 84, 1920–1935. doi: 10.1890/0012-9658(2003)084%5B1920:peicrf
Almany, G. R. (2004). Differential effects of habitat complexity, predators and competitors on abundance of juvenile and adult coral reef fishes. Oecologia 141, 105–113. doi: 10.1007/s00442-004-1617-0
Almany, G. R., and Webster, M. S. (2006). The predation gauntlet: early post-settlement mortality in reef fishes. Coral Reefs 25, 19–22. doi: 10.1007/s00338-005-0044-y
Armsworth, P. R. (2002). Recruitment limitation, population regulation, and larval connectivity in reef fish metapopulations. Ecology 83, 1092–1104. doi: 10.1890/0012-9658(2002)083
Beck, H. J. (2015). Tropical Fish Recruitment Success Varies Among Temperate Reef Habitats, Potentially Impacting their Range Expansion. Ph.D. dissertation, University of Technology Sydney, Sydney.
Bejarano, S., Lohr, K., Hamilton, S., and Manfrino, C. (2015). Relationships of invasive lionfish with topographic complexity, groupers, and native prey fishes in Little Cayman. Mar. Biol. 162, 253–266. doi: 10.1007/s00227-014-2595-3
Bellwood, D. R., and Hughes, T. P. (2001). Regional-scale assembly rules and biodiversity of coral reefs. Science 292, 1532–1535. doi: 10.1126/science.1058635
Benfield, S., Baxter, L., Guzman, H. M., and Mair, J. M. (2008). A comparison of coral reef and coral community fish assemblages in Pacific Panama and environmental factors governing their structure. J. Mar. Biol. Assoc. U.K. 88, 1331–1341. doi: 10.1017/s0025315408002002
Beukers, J. S., and Jones, G. P. (1998). Habitat complexity modifies the impact of piscivores on a coral reef fish population. Oecologia 114, 50–59. doi: 10.1007/s004420050419
Bohnsack, J. A., and Ault, J. S. (1996). Management strategies to conserve marine biodversity. Oceanography 9, 73–82. doi: 10.5670/oceanog.1996.30
Burgess, S. C., Osborne, K., and Caley, M. J. (2010). Similar regional effects among local habitats on the structure of tropical reef fish and coral communities. Glob. Ecol. Biogeogr. 19, 363–375. doi: 10.1111/j.1466-8238.2009.00516.x
Carr, M. H., Anderson, T. W., and Hixon, M. A. (2002). Biodiversity, population regulation, and the stability of coral-reef fish communities. Proc. Natl. Acad. Sci. U.S.A. 99, 11241–11245. doi: 10.1073/pnas.162653499
Cheal, A., Emslie, M., Miller, I., and Sweatman, H. (2012). The distribution of herbivorous fishes on the Great Barrier Reef. Mar. Biol. 159, 1143–1154. doi: 10.1007/s00227-012-1893-x
Chittaro, P. M., and Sale, P. F. (2003). Structure of patch-reef fish assemblages at St. Croix, US Virgin Islands, and One Tree Reef, Australia. Mar. Ecol. Prog. Ser. 249, 277–287. doi: 10.3354/meps249277
Clarke, K. R., and Warwick, R. M. (2001). Change in Marine Communities: An Approach to Statistical Analysis and Interpretation, 2nd Edn. Plymouth: Plymouth Marine Laboratory.
Connolly, S. R. (2005). Process-based models of species distributions and the mid-domain effect. Am. Nat. 166, 1–11. doi: 10.1086/430638
Cowen, R. K., Paris, C. B., and Srinivasan, A. (2006). Scaling of connectivity in marine populations. Science 311, 522–527. doi: 10.1126/science.1122039
Dance, M. A., Patterson, W. F., and Addis, D. T. (2011). Fish community and trophic structure at artificial reef sites in the northeastern Gulf of Mexico. Bull. Mar. Sci. 87, 301–324. doi: 10.5343/bms.2010.1040
Darling, E. S., Graham, N. A. J., Januchowski-Hartley, F. A., Nash, K. L., Pratchett, M. S., Wilson, S. K., et al. (2017). Relationships between structural complexity, coral traits, and reef fishes. Coral Reefs 36, 561–575. doi: 10.1007/s00338-017-1539-z
Dennis, G. D., and Bright, T. J. (1988). Reef Fish Assemblages on Hard Banks in the Northwestern Gulf of Mexico. Bull. Mar. Sci. 43, 280–307.
Doherty, P. J. (1991). “Spatial and temporal patterns in recruitment,” in The Ecology of Fishes on Coral Reefs, ed. P. F. Sale, (San Diego, CA: Academic Press), 261–293. doi: 10.1016/b978-0-08-092551-6.50015-5
Doherty, P. J. (2002). “Variable replenishment and the dynamics of reef fish populations,” in Coral Reef Fishes: Dynamics and Diversity in a Complex Ecosystem, ed. P. F. Sale, (San Diego, CA: Academic Press), 327–358.
Doherty, P. J., and Williams, D. M. B. (1988). The replenishment of coral reef fish populations. Oceanogr. Mar. Biol. Annu. Rev. 26, 487–551.
D’Sa, E. J., and Dimarco, S. F. (2009). Seasonal variability and controls on chromophoric dissolved organic matter in a large river-dominated coastal margin. Limnol. Oceanogr. 54, 2233–2242. doi: 10.4319/lo.2009.54.6.2233
Edmunds, P. J., and Leichter, J. J. (2016). Spatial scale-dependent vertical zonation of coral reef community structure in French Polynesia. Ecosphere 7:e01342.
Fontes, J., Semmens, B., Caselle, J. E., Santos, R. S., and Shree, R. P. (2016). Ocean productivity may predict recruitment of the rainbow wrasse (Coris julis). PLoS One 11:e0165648. doi: 10.1371/journal.pone.0165648
Friedlander, A. M., Brown, E. K., Jokiel, P. L., Smith, W. R., and Rodgers, K. S. (2003). Effects of habitat, wave exposure, and marine protected area status on coral reef fish assemblages in the Hawaiian archipelago. Coral Reefs 22, 291–305. doi: 10.1007/s00338-003-0317-2
Grober-Dunsmore, R., Frazer, T. K., Beets, J. P., Lindberg, W. J., Zwick, P., and Funicelli, N. A. (2008). Influence of landscape structure on reef fish assemblages. Landsc. Ecol. 23, 37–53. doi: 10.1007/s10980-007-9147-x
Grober-Dunsmore, R., Frazer, T. K., Lindberg, W. J., and Beets, J. (2007). Reef fish and habitat relationships in a Caribbean seascape: the importance of reef context. Coral Reefs 26, 201–216. doi: 10.1007/s00338-006-0180-z
Heinlein, J. M., Stier, A. C., and Steele, M. A. (2010). Predators reduce abundance and species richness of coral reef fish recruits via non-selective predation. Coral Reefs 29, 527–532. doi: 10.1007/s00338-010-0592-7
Hickerson, E. L., Schmahl, G. P., Johnston, M. A., Nuttall, M. F., Embesi, J. A., and Eckert, R. J. (2012). “Flower Garden Banks – A refuge in the Gulf of Mexico?” in Proceedings of the 12th International Coral Reef Symposium, Cairns.
Hixon, M. (2015). “Predation: Piscivory and the ecology of coral reef fishes,” in Ecology of Fishes on Coral Reefs, ed. C. Mora, (Cambridge: Cambridge University Press), 41–52. doi: 10.1017/CBO9781316105412.007
Holbrook, S. J., Schmitt, R. J., and Brooks, A. J. (2008). Resistance and resilience of a coral reef fish community to changes in coral cover. Mar. Ecol. Prog. Ser. 371, 263–271. doi: 10.3354/meps07690
James, M. K., Armsworth, P. R., Mason, L. B., and Bode, L. (2002). The structure of reef fish metapopulations: modelling larval dispersal and retention patterns. Proc. R. Soc. Lond. Ser. B Biol. Sci. 269, 2079–2086. doi: 10.1098/rspb.2002.2128
Johnston, M. A., Embesi, J. A., Eckert, R. J., Nuttall, M. F., Hickerson, E. L., and Schmahl, G. P. (2016). Persistence of coral assemblages at east and west flower garden banks, Gulf of Mexico. Coral Reefs 35, 821–826. doi: 10.1007/s00338-016-1452-x
Johnston, M. A., Nuttall, M. F., Eckert, R. J., Embesi, J. A., Slowey, N. C., Hickerson, E. L., et al. (2013). Long-term monitoring at the East and West Flower Garden Banks National Marine Sanctuary. Silver Spring, MD: NOAA, 202.
Jones, G. P. (1988). Experimental evaluation of the effects of habitat structure and competitive interactions on the juveniles of two coral reef fishes. J. Exp. Mar. Biol. Ecol. 123, 115–126. doi: 10.1016/0022-0981(88)90164-5
Kitchens, L. L., Paris, C. B., Vaz, A. C., Ditty, J. G., Cornic, M., Cowan, J. H., et al. (2017). Occurrence of invasive lionfish (Pterois volitans) larvae in the northern Gulf of Mexico: characterization of dispersal pathways and spawning areas. Biol. Invasions 19, 1971–1979. doi: 10.1007/s10530-017-1417-1
Komyakova, V., and Swearer, S. E. (2019). Contrasting patterns in habitat selection and recruitment of temperate reef fishes among natural and artificial reefs. Mar. Environ. Res. 143, 71–81. doi: 10.1016/j.marenvres.2018.11.005
Limer, B., Bloomberg, J., and Holstein, D. M. (in review). The influence of eddies on coral larval retention in the Flower Garden Banks. Front. Mar. Sci.
Lugo-Fernández, A. (2006). Travel times of passive drifters from the Western Caribbean to the Gulf of Mexico and Florida-Bahamas. Gulf M. Sci. 24, 61–67.
Lugo-Fernández, A., Morin, M. V., Ebesmeyer, C. C., and Marshall, C. F. (2001). Gulf of Mexico Historic (1955-1987) Surface Drifter Data Analysis. J. Coast. Res. 17, 1–16.
MacDonald, C., Bridge, T. C. L., and Jones, G. P. (2016). Depth, bay position and habitat structure as determinants of coral reef fish distributions: Are deep reefs a potential refuge? Mar. Ecol. Prog. Ser. 561, 217–231. doi: 10.3354/meps11953
MacNeil, M., Graham, N., Cinner, J., Wilson, S. K., Williams, I. D., Maina, J., et al. (2015). Recovery potential of the world’s coral reef fishes. Nature 520, 341–344. doi: 10.1038/nature14358
Malcolm, H. A., Jordan, A., and Smith, S. D. A. (2010). Biogeographical and cross-shelf patterns of reef fish assemblages in a transition zone. Mar. Biodivers. 40, 181–193. doi: 10.1007/s12526-010-0042-3
McGowan, M. F. (1985). Ichthyoplankton of the Flower Garden Banks, Northwest Gulf of Mexico. Ph.D. thesis, University of Miami, Coral Gables, FL, 358.
Mellin, C., Cheal, A. J., Emslie, M. J., and Caley, M. J. (2016). Marine protected areas increase resilience among coral reef communities. Ecol. Lett. 19, 629–637. doi: 10.1111/ele.12598
Messmer, V., Jones, G. P., Munday, P. L., Holbrook, S. J., Schmitt, R. J., and Brooks, A. J. (2011). Habitat biodiversity as a determinant of fish community structure on coral reefs. Ecology 92, 2285–2298. doi: 10.1890/11-0037.1
Newman, M. J. H., Paredes, G. A., Sala, E., and Jackson, J. B. C. (2006). Structure of Caribbean coral reef communities across a large gradient of fish biomass. Ecol. Lett. 9, 1216–1227. doi: 10.1111/j.1461-0248.2006.00976.x
Rezak, R., Gittings, S. R., and Bright, T. J. (1990). Biotic Assemblages and Ecological Controls on Reefs and Banks of the Northwest Gulf of Mexico1. Integr. Comp. Biol. 30, 23–35. doi: 10.1093/icb/30.1.23
Risk, A. (1998). The effects of interactions with reef residents on the settlement and subsequent persistence of ocean surgeonfish, Acanthurus bahianus. Environ. Biol. Fishes 51, 377–389.
Roberts, C. M. (1991). Larval mortality and the composition of coral reef fish communities. Trends Ecol. Evol. 6, 83–87. doi: 10.1016/0169-5347(91)90180-6
Roberts, C. M., and Ormond, R. F. G. (1987). Habitat complexity and coral reef fish diversity and abundance on Red Sea fringing reefs. Mar. Ecol. Prog. Ser. 41, 1–8. doi: 10.3354/meps041001
Robertson, D. R., Green, D. G., and Victor, B. C. (1988). Temporal coupling of production and recruitment of larvae of a caribbean reef fish. Ecology 69, 370–381. doi: 10.2307/1940435
Rooker, J. R., Dokken, Q. R., Pattengill, C. V., and Holt, G. J. (1997). Fish assemblages on artificial and natural reefs in the Flower Garden Banks National Marine Sanctuary, USA. Coral Reefs 16, 83–92. doi: 10.1007/s003380050062
Rooker, J. R., Kitchens, L. L., Dance, M. A., Wells, R. J. D., Falterman, B., and Cornic, M. (2013). Spatial, temporal, and habitat-related variation in abundance of pelagic fishes in the Gulf of Mexico: potential implications of the Deepwater Horizon oil spill. PLoS One 8:e76080. doi: 10.1371/journal.pone.0076080
Sale, P. F. (1991). “Habitat structure and recruitment in coral reef fishes,” in Habitat Structure: The Physical Arrangement of Objects in Space, eds S. S. Bell, E. D. Mccoy, and H. R. Mushinsky, (Dordrecht: Springer), 197–210. doi: 10.1007/978-94-011-3076-9_10
Sale, P. F. (2004). Connectivity, recruitment variation, and the structure of reef fish communities1. Integr. Comp. Biol. 44, 390–399. doi: 10.1093/icb/44.5.390
Schmahl, G. P., Hickerson, E. L., and Precht, W. F. (2008). “Biology and ecology of coral reefs and coral communities in the flower garden banks region, Northwestern Gulf of Mexico,” in Coral Reefs of the USA, eds B. M. Riegl and R. E. Dodge, (Dordrecht: Springer), 221–261. doi: 10.1007/978-1-4020-6847-8_6
Shima, J. S., Noonburg, E. G., Swearer, S. E., Alonzo, S. H., and Osenberg, C. W. (2018). Born at the right time? A conceptual framework linking reproduction, development, and settlement in reef fish. Ecology 99, 116–126. doi: 10.1002/ecy.2048
Shulzitski, K., Sponaugle, S., Hauff, M., Walter, K. D., and Cowen, R. K. (2016). Encounter with mesoscale eddies enhances survival to settlement in larval coral reef fishes. Proc. Natl. Acad. Sci. U.S.A. 113, 6928–6933. doi: 10.1073/pnas.1601606113
Smallhorn-West, P. F., Bridge, T. C. L., Munday, P. L., and Jones, G. P. (2017). Depth distribution and abundance of a coral-associated reef fish: roles of recruitment and post-recruitment processes. Coral Reefs 36, 157–166. doi: 10.1007/s00338-016-1509-x
Sonnier, F., Teerling, J., and Hoese, H. D. (1976). Observations on the offshore reef and platform fish fauna of Louisiana. Copeia 1976, 105–111.
Sponaugle, S. (2015). “Recruitment of coral reef fishes: linkages across stages,” in Ecology of Fishes on Coral Reefs, ed. C. Mora, (Cambridge: Cambridge University Press), 28–33. doi: 10.1017/cbo9781316105412.005
Sponaugle, S., Grorud-Colvert, K., and Pinkard, D. (2006). Temperature-mediated variation in early life history traits and recruitment success of the coral reef fish Thalassoma bifasciatum in the Florida Keys. Mar. Ecol. Prog. Ser. 308, 1–15. doi: 10.3354/meps308001
Syms, C., and Jones, G. P. (2000). Disturbance, habitat structure, and the dynamics of a coral-reef fish community. Ecology 81, 2714–2729. doi: 10.1890/0012-9658(2000)081
Victor, B. (1986). Duration of the planktonic larval stage of one hundred species of Pacific and Atlantic wrasses (family Labridae). Mar. Biol. 90, 317–326. doi: 10.1007/bf00428555
Voss, J. D., Williams, M. A., Reed, J. K., and Clark, R. (2014). “Benthic and fish communities in the mid and lower mesophotic zone of the sanctuary,” in Fish and Benthic Communities of the Flower Garden Banks National Marine Sanctuary: Science to Support Sanctuary Management, eds R. Clark, J. C. Taylor, C. A. Buckel, and L. M. Kracker, (Silver Spring, MD: NOAA), 201–260.
Wellington, G. M., and Victor, B. C. (1989). Planktonic larval duration of one hundred species of Pacific and Atlantic damselfishes (Pomacentridae). Mar. Biol. 101, 557–567. doi: 10.1007/bf00541659
Williams, D. M. (1983). Daily, monthly and yearly variability in recruitment of a guild of coral reef fishes. Mar. Ecol. Prog. Ser. 10, 231–237. doi: 10.3354/meps010231
Wismer, S., Hoey, A. S., and Bellwood, D. R. (2009). Cross-shelf benthic community structure on the Great Barrier Reef: relationships between macroalgal cover and herbivore biomass. Mar. Ecol. Prog. Ser. 376, 45–54. doi: 10.3354/meps07790
Keywords: reef fish, Flower Garden Banks, Stetson Bank, Sonnier Bank, assemblage structure, recruitment, coral reef, biodiversity
Citation: Wetmore LS, Dance MA, Hill RL and Rooker JR (2020) Community Dynamics of Fish Assemblages on Mid-Shelf and Outer-Shelf Coral Reefs in the Northwestern Gulf of Mexico. Front. Mar. Sci. 7:152. doi: 10.3389/fmars.2020.00152
Received: 16 August 2019; Accepted: 27 February 2020;
Published: 27 March 2020.
Edited by:
Adrienne M. S. Correa, Rice University, United StatesReviewed by:
Austin T. Humphries, University of Rhode Island, United StatesDominic A. Andradi-Brown, World Wildlife Fund, United States
Copyright © 2020 Wetmore, Dance, Hill and Rooker. This is an open-access article distributed under the terms of the Creative Commons Attribution License (CC BY). The use, distribution or reproduction in other forums is permitted, provided the original author(s) and the copyright owner(s) are credited and that the original publication in this journal is cited, in accordance with accepted academic practice. No use, distribution or reproduction is permitted which does not comply with these terms.
*Correspondence: Michael A. Dance, bWRhbmNlMUBsc3UuZWR1
†Present address: Department of Biology, San Diego State University, San Diego, CA, USA