- 1Department of General Zoology and Neurobiology, ND7/31, Ruhr-University Bochum, Bochum, Germany
- 2Department of Animal Ecology, Evolution and Biodiversity, Ruhr-University Bochum, Bochum, Germany
The flashlight fish Photoblepharon steinitzi inhabit coral reef caves in the Red Sea. During the night they dwell alone or in pairs near their cave entrance, characteristic of territorial like behavior. A special feature of the flashlight fish is a bioluminescent organ located under their eyes, which emits blue green light. This bioluminescence may have various functions including intraspecific communication. To gain a better understanding of how these bioluminescent signals are used during territorial aggression, we investigated the territorial behavior of P. steinitzi in its native environment. Using infrared video recordings at night, we found that P. steinitzi increases its blinking frequency, while attacking intraspecific intruders, different artificial light organs or a fish dummy simulating an intraspecific intruder. All three stimuli presented to P. steinitzi elicited four different types of attack modes (i.e., darting, border crossings, repetitive swimming toward stimuli and aggressive contact with stimuli such as ramming and bites) to varying degrees coupled with high blinking frequencies. These attacks occurred near the entrance of the cave where P. steinitzi mainly resides during the night, suggesting a territorial behavior. Collectively our data show that the intensity of displayed aggression potential in P. steinitzi depends on the signal properties of the intraspecific intruder. A constant glowing light organ dummy increase the aggression level in P. steinitzi whereas a blinking light organ dummy that simulate an intruder and a constant glowing dummy that display the fish shape decrease the aggression level in P. steinitzi.
Introduction
In animals, aggression is a form of adaptive behavior. It is used to establish dominance hierarchies, to compete for food or mating partners, for defense and to protect offspring (Freudenberg et al., 2016). Aggressive interaction normally involves the competition for resources and often occurs in defined personal spaces or territories. For example, in territorial fish aggressive interaction and communication occur in a defined spatial area where the fish normally resides (Grant, 1997; Helfman et al., 2009). The aggression can occur between individuals of the same (intraspecific) or different (interspecific) species and involves visual, acoustic, chemical, tactile and electric communication (Helfman et al., 2009).
One fascinating communication phenomenon in dark marine environments is the use of bioluminescence. The emitted light normally shows wavelengths in the blue green spectral range (Bowlby et al., 1991) and is produced by an oxygen-dependent reaction involving an enzyme (luciferase) and a light-emitting molecule (oxyluciferin) to produce light (Wilson and Hastings, 1998; Haddock et al., 2010; Widder, 2010). More than 40 families of fish use bioluminescence (Haddock et al., 2010) and a recent study reported 27 independent evolutionary events of bioluminescence in ray finned fish (Davies et al., 2016). The bioluminescent light is produced either by specialized organs filled with symbiotic luminescent bacteria (Haygood and Diestel, 1993) or intrinsically such as in hatchetfishes, dragonfishes (Stomiiformes), lanternfishes (Myctophiformes) and luminescent sharks (Herring and Cope, 2005; Haddock et al., 2010; Claes et al., 2012, 2013). One major challenge for understanding the role of bioluminescence in fish behavior is that most bioluminescent fish species live in the deep sea, with a few exceptions. As one example, flashlight fish (Anomalopidae) live in shallow waters and can be studied at night on reef flats or in experimental tanks (Morin et al., 1975; Hellinger et al., 2017). The family Anomalopidae contain 6 genera including Anomalops katoptron and the genus Photoblepharon (McCosker and Rosenblatt, 1987). A. katoptron live in schools between 10 and 50 individuals in the open water and can be studied at moonless night at the water surface on shallow reef flats (Haneda and Tsuji, 1971; McCosker, 1977; Hellinger et al., 2017; Gruber et al., 2019). Their retina and photoreceptors are specialized to detect blue green bioluminescent light (Mark et al., 2018). Photoblepharon consists of two species, P. palpebratum which inhabit the Pacific and Western Indian oceans and Photoblepharon steinitzi which live in the Red Sea and the Western Indian ocean, for example at the Comoros and Maldives (Froese and Pauly, 2019). In contrast to A. katoptron, which occur usually in schools P. palpebratum and P. steinitzi show territorial behavior and can be found alone, in pairs or form groups in coral reefs near to the water surface (Morin et al., 1975). The genus Photoblepharon display a bean-shaped light organ situated below the eye, which is filled with bioluminescent bacteria. The light organs can be covered by a black, eyelid-like shutter (Johnson and Rosenblatt, 1988) to produce blink patterns, which have been described in captured fish living in an isolated tank. These blink patterns are described as infrequent blinking behavior, where the light is on most of the time, equal on and off blinks of around a second and fast blinking behavior during darting swimming behavior. Blink patterns were discussed in the context of territory defense (Morin et al., 1975). If aggression and territory defense in P. steinitzi is triggered by light signals, fish body or both is not known. We therefore analyzed aggression and blinking behavior induced by different light organ and fish dummies in the local population of P. steinitzi in Dahab Egypt, using infrared video recordings. We identified different blink patterns and aggression modes during territorial defense in the flashlight fish P. steinitzi.
Materials and Methods
Recording Sites
Behavioral experiments to investigate the potential role of bioluminescence in the context of intraspecific aggressive and territorial behavior in P. steinitzi were conducted in their natural habitat at the entrance area of small reef caves (Figure 1). Specimens of P. steinitzi were identified by the glow of subocular light organs (Figures 1A–C) during night dives. Field experiments were performed in May 2015–2018 after sunset at a fringing reef in the Gulf of Aqaba (Red Sea) during scuba dives. P. steinitzi showed no signs of avoidance reactions or conspicuous changes in blink frequencies as a result of a slowly approaching diver. All data were recorded at the coast of Dahab on the Sinai Peninsula in Egypt. The entrance areas of reef caves (Figure 1D) were identified during day dives (scuba) beneath the reef edge at depths between 1.5 and 4 m. The size of main reef cave entrance and smaller cave branches (as described below) were estimated using an 80 cm long steel rod as a scale. The main entrance area of reef caves showed an approximated dimension of 1–3 m in length, 0.8–1.4 m in height and 2 m in depth. The openings of smaller branches showed an approximated dimension of 0.4–1 m in diameter. Recordings in reef caves were performed at two sites with coordinates 28° 29.292′ N 034° 30.990′ E and 28° 29.307′ N 034° 30.994′ E. Behavior experiments were performed in two reef cave systems on both coordinates. The reef cave systems showed a similar morphology consisted of a main cave opening and smaller cave branches. The sites were surrounded by numerous rock spurs consisting of limestone deposits from coral polyps and by large entrance areas to the reef caves (Figure 1D). Reef caves and rock spurs spread into numerous smaller reef caves (Figure 1E) occupied and defended by single specimens or pairs of P. steinitzi. Pairs consisted of two specimens of different length. Morin et al. (1975) reported that pairs of P. steinitzi consisted of a larger female and a smaller male. Therefore, two specimens that showed a size difference and occured together in a territory were defined as pairs in this work. Our own observations revealed approximately 4–7 cm for potential males and 7–10 cm for potential females. The difference in body length may reflect reproduction success because larger females produce more eggs. Recording sites were documented by photographs (Canon G15, 12 megapixel). Suitable reef caves were tagged with small buoys for behavioral experiments after sunset. The morphology of the fringing reef is characterized as a wide and shallow reef flat (approximately 1–1.5 m depth and 100 m wide). The reef edge and the steep reef slope show a dense cover with scleractinian corals. In contrast, entrance areas of reef caves are characterized by a decrease in coral cover (Figures 1A,D,E) and an increase in coralline algae (Corallinales). Deeper branches of reef caves are characterized by encrusting sponges. Reef slopes at the recording sites ended in a sandy bottom with solitary coral rocks at depths of approximately 20 m. The entrance areas of reef caves open in a central cave and branch out in smaller caves and crevices where P. steinitzi appear out of the deeper branches after sunset in pairs or single specimens and occupy and defend small territories.
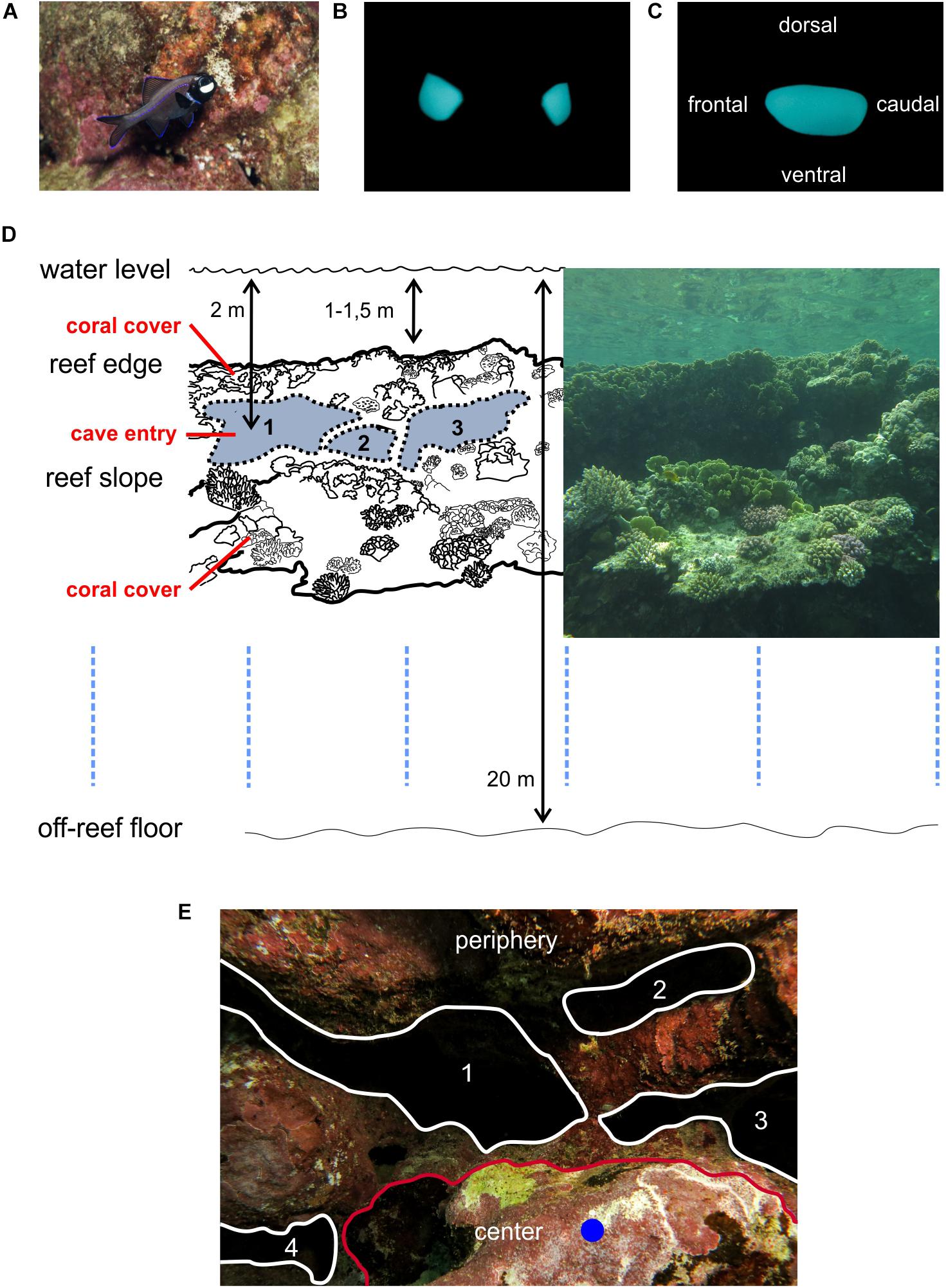
Figure 1. Photoblepharon steinitzi in the Red Sea at the coast of Dahab. Bioluminescence of light organs and natural habitat of P. steinitzi. (A) Flash photograph of P. steinitzi in its natural habitat, a reef cave entrance area on a reef slope at the coast of the Red Sea. The bean shaped light organ appears as a white patch under the eye as a result of a mirror in the light organ and the reflection produced by the camera flash. The specimen showed an approximate length of about 10 cm. (B,C) Frontal and side views of P. steinitzi bean shaped light organs in the dark. The blue green bioluminescent light is produced by symbiotic luminescent bacteria in the light organs. (D) Example of one reef cave system in Dahab located in a shallow fringing reef at the Red Sea coast (N 28° 29.292′, E 034° 30.990′). Photoblepharon steinitzi dwell after sunset in these small reef cave inlets at the reef slope. The scheme on the left represent a replica of the photograph on the right. Cave inlets on the reef slope are indicated by 1–3. Photographs were made with an underwater camera (Canon Powershot G15, 12 megapixel). (E) Example of one reef cave with. The red line indicates the border between the center where the dummies were placed and the peripgery. White lines indicate the entrance area of small branches in the reef cave system. The blue dot shows the dummy position.
Behavioral Experiments
All behavioral observations were recorded using an infrared (IR) sensitive HD-video camera (Sony HDR-CX 730 6.3 mm CMOS-Sensor, 24.1 megapixel, 6544 × 3680, 50 frames/s sampling rate) in an underwater housing (BS Kinetics, Germany). The entrance areas of P. steinitzi territories were illuminated with 3–4 custom made IR-LED lights. Each IR-light consisted of a 5× high power IR-LED with 860 nm peak wavelength (WEPIR1-S1 IR Power 1 W, Winger Electronics GmbH, Germany). IR-LEDs were used to identify and analyze aggression behavior in P. steinitzi. Using high IR-light intensities could potentially influence fish behavior. Therefore, we used the same control as described in a previous study on the related flashlight fish species A. katoptron (Hellinger et al., 2017). Switching the lights on and off prior to the experiments triggered no reactions in P. steinitzi. We built a fish dummy where a glow stick (519 nm peak wavelength) was used to illuminate the artificial light organs mounted in the fish dummy (Figure 2A). The fish dummy was made of a plastic fish (100 mm length) fitted with artificial light organs (10 mm length) made of acrylic glass (3 mm thick) and a central acrylic glass tube (50 mm length, 6 mm diameter) with a glow stick inside to illuminate the artificial light organ. The fish dummy was painted to mimic the natural gray and black pattern of P. steinitzi using acrylic paint (Revell GmbH, Germany). Fish dummy experiments were performed to investigate the potential role of fish shape in the context of blink and aggressive/territory behavior in P. steinitzi. We further used two different light organ dummies to investigate the blink frequencies and aggressive behavior. We used a blinking LED (Nichia 0.12 W, 6 mm length WINGER Electronics GmbH, Germany) with 500 nm peak wavelength mounted in an underwater acrylic glass housing (Figure 2B) that mimics a blinking light organ. This device was used to investigate the role of blink behavior in P. steinitzi. We observed increased blink frequencies in P. steinitzi during agonistic behavior compared to undisturbed fish during preliminary observations in the territory owner as well as in the intruder. Therefore, we used a blink frequency of 1 Hz for the blinking light organ dummy. We further used a glow stick (4.5 mm diameter, 37 mm length and 519 nm peak wavelength, JENZI Germany) mounted on an aluminum rod (Figure 2C) to mimic the display of constant glowing light organs in P. steinitzi. Glow stick and LED peak wavelength was measured using a portable spectrometer (Flame–S-UV-VIS-ES, Ocean Optics, United States). The illuminated surface of the glow stick was reduced to 10 mm in length with black textile tape (tesa SE, Germany) to simulate the light organ size of P. steinitzi. Haneda and Tsuji (1971) reported a light organ length of 9.1 mm in the related species P. palpebratum on the Banda Island in Indonesia. Our own measurements in P. steinitzi specimens revealed a mean light organ size of 9.94 mm ± 0.15 SEM (N = 8) measured along the light organ longitudinal axis. The LED and the glow stick show a wavelength difference of 19 nm. Preliminary observations with constant glowing small green, blue and red light sources showed approaching reactions of P. steinitzi to all three lights. Therefore, we assume no wavelength dependent effects in behavior by using a 500 nm LED and a 519 nm glow stick. The dummies were positioned prior to the experiments in the center region of reef caves (Figure 1E) by a slow moving diver to avoid disturbance in fish behavior. The fish behavior was subsequently observed by the diver. Recordings were started at least 1 min after dummy positioning to avoid influence caused by the diver. All recordings were made in distinct territories of P. steinitzi i.e., an area occupied and defended by the fish. Territories were defined as an area defended by the territory owner as described by Grant (1997). We identified territories by observation of P. steinitzi pairs which occupy and defend an area in the entrance region of reef caves. Prior to the experiments recording sites in the territories were subdivided into 2 areas to analyze aggression behavior. A central and a peripheral part (Figure 1E). The central part was defined as the area were the dummies were presented to P. steinitzi and showed a diameter of approximately 1 m. The peripheral area was defined as the remaining part of the territory defended by pairs or single specimens of P. steinitzi and showed a dimension of approximately 2 m. Structures like rocks, sponges and patches covered with coralline algae were used to define borders between the central part and the periphery. A minimum distance of 1 m between the camera and the dummies was used to avoid evasive reaction by the fish as described in Morin et al. (1975). We never observed any kind of reactions to a slow moving diver in a distance greater than 1 m. Field experiments were started at least 30 min after sunset when flashlight fish activity was observed in the entrance area of reef caves by the diver. Video recordings were captured and analyzed for N = 6 single unstimulated fish, N = 2 fish in pairs, N = 11 fish confronted with a fish dummy, N = 6 fish confronted with a blinking LED, N = 8 fish confronted with a glow stick and N = 5 fish during intraspecific interaction. Every stimulus was recorded in fixed sample time intervals for 5 min in sequential sessions. Blink frequencies were presented in blinks/min. Light organ on/off times were presented in seconds. Aggression behavior were presented in reactions per 5 min trial and occurrence were analyzed for 5 min trials. IR-recordings for each specimen of P. steinitzi were analyzed with the video analysis software (Vidana 1.0) using frame by frame analysis for blink frequencies and an event recorder function for on/off times, occurrence and aggression behavior which allows the analysis of event numbers and duration.
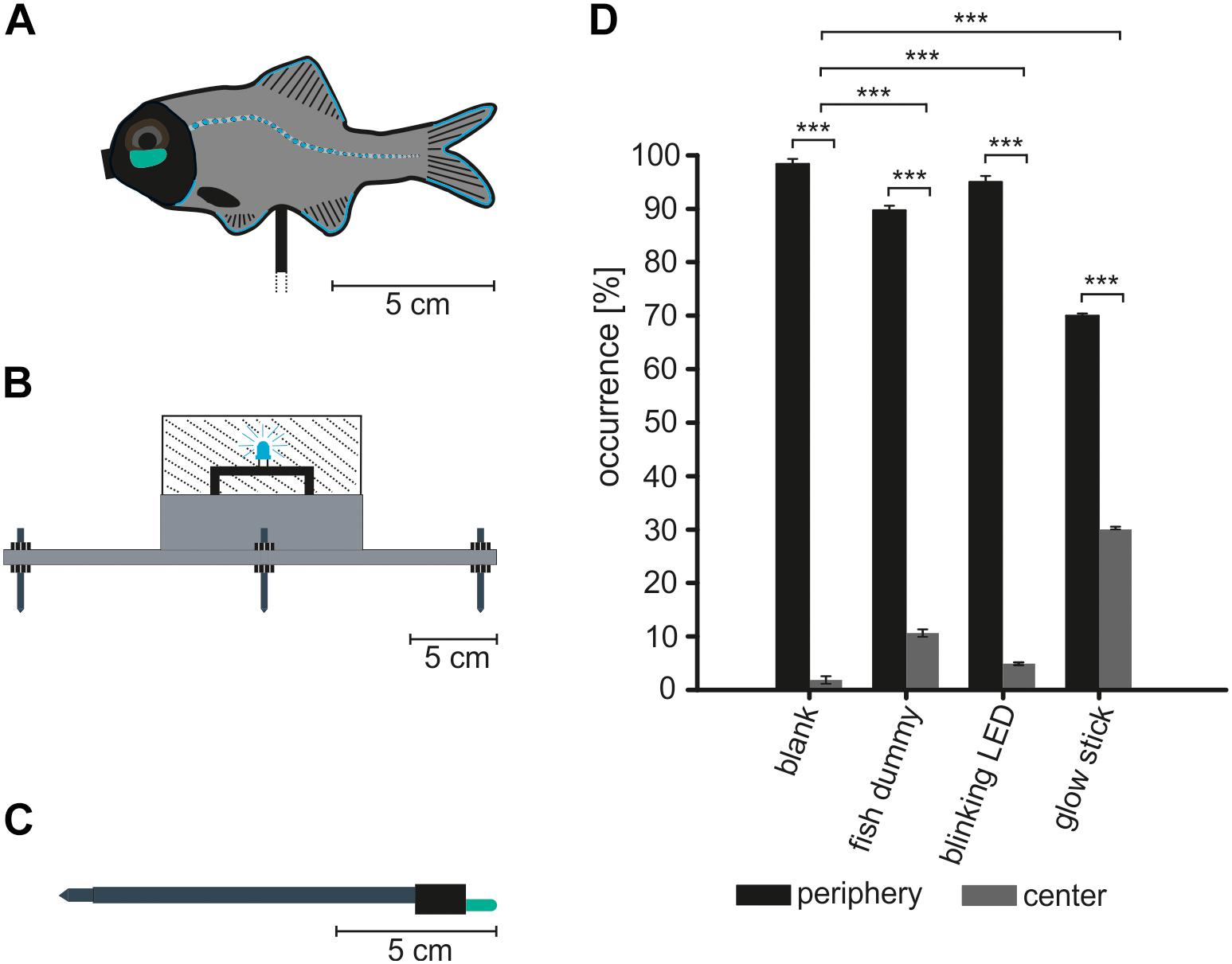
Figure 2. Fish and light organ dummies. Relative occurrence of P. steinitzi in the center and periphery of small territories in reef caves where P. steinitzi reside after sunset. (A–C) The following dummies were used in the experiments. (A) A fish dummy with integrated artificial light organs. Artificial light organs consisted of an acrylic glass pipe with a glow stick and acrylic glass covered the artificial light organs in the fish dummy. (B) Blinking light organ dummy. The dummy consisted of a PVC base and an acrylic glass cylinder. A 5 mm LED produced a 1 Hz blinking signal with 500 nm peak wavelength. (C) Constantly glowing light organ dummy. The dummy was made of an aluminum stick (130 mm length) and a glow stick (4.5 mm Ø, 37 mm length) mounted on the tip. The glow stick surface was reduced to 10 mm to simulate the light organ size in P. steinitzi. (D) Relative occurrence time (in percent of 5 min recording intervals) of P. steinitzi after sunset in the entrance area of reef caves. Comparison of relative times between center and periphery and time in center between the different recording conditions (blank, dummy, blinking LED, and glow stick). Black bars indicate occurrence time in the territory periphery. Gray bars indicate occurrence time in the center of the territory. Error bars indicate mean ± SEM. Significance values are reported as: *p ≤ 0.05; **p ≤ 0.01; ***p ≤ 0.001.
Statistical Analysis
SigmaPlot 12.0 was used to analyze aggression and blink behavior in P. steinitzi. Differences in blink frequency, open and closed times of light organs and occurrence of specimens were compared using a one-way ANOVA and Holm-Sidak post hoc analysis. The data were log-transformed (log(1 + x) before statistical analysis to obtain normalized data. Aggression behavior was compared using a Mann-Whitney rank sum test. The results are reported as mean ± SEM (standard error of mean). Significance values are reported as: ∗p < 0.05, ∗∗p < 0.01; ∗∗∗p < 0.001.
Results
Identification of Reef Caves in the Red Sea and Occurrence Times of Photoblepharon steinitzi
In order to analyze the behavior of a P. steinitzi population in its native environment Dahab, we identified suitable reef caves beyond the reef edge in a fringing reef. The reef edge and caves are located approximately 100 m from the shore at a depth of 1.5–4 m (Figure 1D). We routinely observed P. steinitzi (Figures 1A–C) in the entrance of reef caves (Figures 1A,D,E) independent of the moon phase and the tide with one exception. We observed P. steinitzi during dark and moonless nights outside of reef caves. During these condition P. steinitzi occur outside but close to the reef caves. We never observed specimens of P. steinitzi more than approximately 3 m away from their territories. P. steinitzi are cohabitants with nocturnal soldierfish of the subfamily Myripristinae and squirrelfish of the subfamily Holocentrinae in the reef caves. We did not observe interspecific interactions like aggression, escape responses and agonistic behavior between these species and P. steinitzi. For the behavioral analysis we used different light organ and fish dummies with artificial light organs to represent intruders and observed how the fish responds with changing blinking patterns and territorial aggressive behavior. Therefore, we first measured the occurrence of P. steinitzi in the periphery or center of the caves, where the light organ and fish dummies (Figures 1D,E) were placed during the recordings as mentioned above. P. steinitzi spent 98.32% ± 0.77 SEM of its time in their territories (periphery of the cave) and 1,68% ± 0.77 SEM in the center during the absence of light organ and fish dummies (Figure 2D). In the presence of the light organ dummy (glow stick) P. steinitzi spend significantly more time 30.22% ± 1.8 SEM in the center of the cave (Figure 2D). In the presence of a constant glowing fish dummy P. steinitzi spend 89.47% ± 1.39.2 SEM in the periphery and 10.53% ± 1.37 SEM in the center. During stimulation with a blinking light organ dummy (blinking LED) P. steinitzi spent 95.28% ± 0.14 SEM in the periphery and 4.72% ± 0.14 SEM in the center of the reef cave.
Analysis of Blinking Behavior of Photoblepharon steinitzi
We next recorded the blinking behavior and light organ open and closed times of P. steinitzi in the presence and absence of the dummies and intraspecific interactions with P. steinitzi from contiguous territories within the caves (Figure 3). We found that P. steinitzi either alone (7.46 blinks/min ± 0.56 SEM) or in pairs (6.2 blinks/min ± 0.79 SEM) reveal a low blinking frequency (Supplementary Videos S1, S2 Movie). We found that in single specimens of P. steinitzi the light organs were exposed for 96.57% of recording intervals and revealed averaged single open times of 8.67 s ± 0.75 SEM and closed times of 0.33 s ± 0.05 SEM (Figures 3B,C). We found also nearly constant opened light organs (98.25%) in a pair of P. steinitzi. P. steinitzi revealed mean light organ opening times of 11.37 s ± 0.05 SEM and closed times of 0.164 s ± 0.05 SEM under this condition. Additionally, P. steinitzi revealed nearly similar time ratios while feeding (Supplementary Video S3 Movie) zooplankton (Figures 3B,C). In contrast, the blink frequency in P. steinitzi increased (P < 0.001) to approximately 52 blinks per minute (Figure 3A) in the presence of artificial light organs and fish dummies (Supplementary Videos S4–S6 Movie). The light organ open times are decreased to approximately 0.82 s (Figures 3B,C). No significant differences in blink frequencies were observed between the constant glowing fish dummy, blinking LED light organ dummy, constant glowing light organ dummy (glow stick) and intraspecific intruders (Figure 3A). P. steinitzi responds with increased blinking frequencies to artificial greenish light emitting dummies.
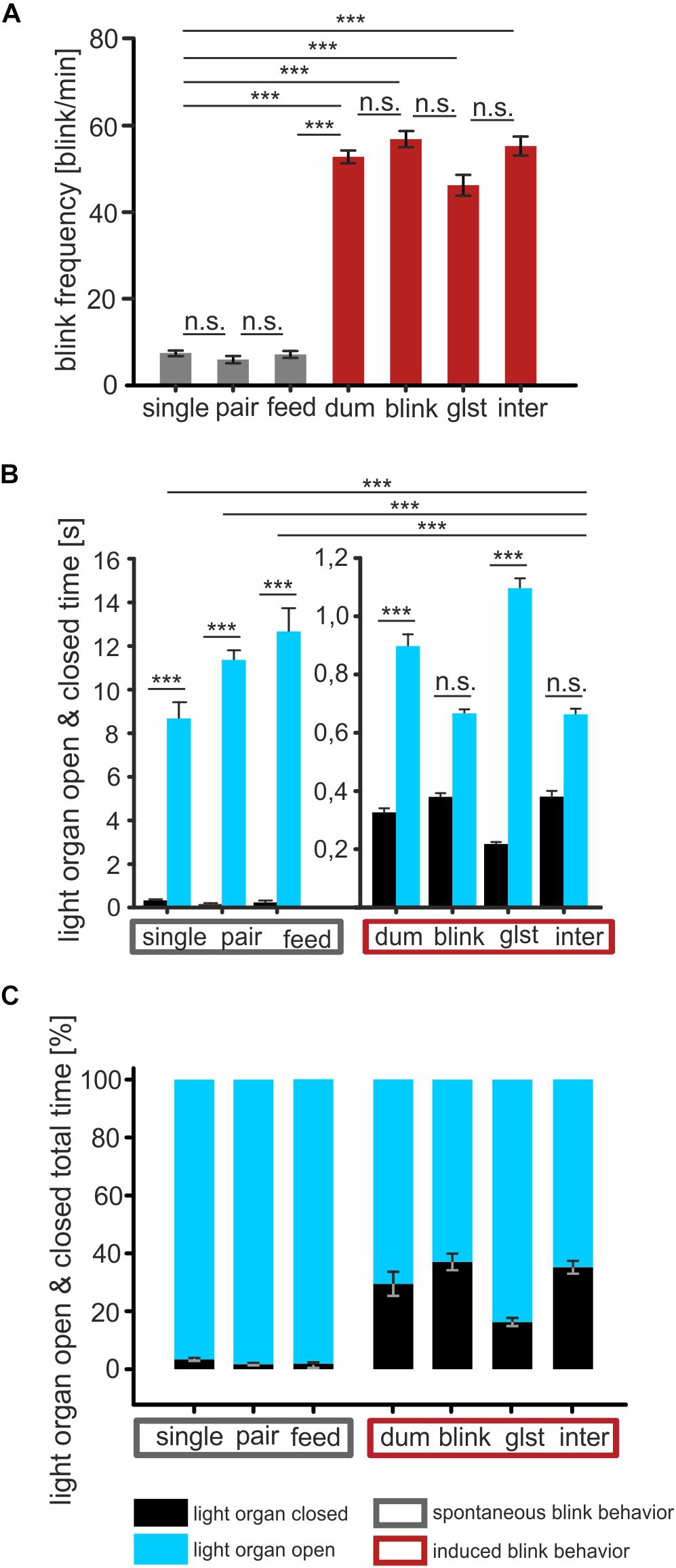
Figure 3. Blink behavior and light organ open and closed times of P. steinitzi during feeding and exposure to artificial and intraspecific intruders. (A) Blink frequency (blinks/min) in P. steinitzi in undisturbed specimens (single) or pairs (pair) and during feeding zooplankton organisms (feed). Photoblepharon steinitzi show increased blink frequencies during confrontation with: a constant glowing fish dummy (dum), a blinking (1 Hz) LED/light organ dummy (blink), a constant glowing glow stick/light organ dummy (glst) and during intraspecific interactions triggered by a conspecific intruder (inter). Gray bars indicate blinking behavior in undisturbed specimens. Red bars indicate blinking behavior during exposure to artificial and intraspecific intruders. (B) Mean time intervals of single open and closed times of light organs. Black bars indicate closed light organs. Blue bars indicate open light organs. Rectangle with gray frame indicate conditions in undisturbed fish. Rectangle with red frame indicate conditions during exposure to artificial and intraspecific intruders as described in above. (C) Total relative distribution of open and closed light organs in percent of 5 min recording intervals. Color coding of bars and rectangles as described in (A,B). Error bars indicate mean ± SEM. Significance values are reported as: *p ≤ 0.05; **p ≤ 0.01; ***p ≤ 0.001.
Monitoring Aggressive Behavior of Photoblepharon steinitzi in Reef Caves of the Red Sea
We next analyzed how the blinking patterns relate to different territorial and aggressive behavior when the dummies were placed in the center of the cave. In general, we observed that dummies as well as intraspecific intruders provoke aggression and were attacked with different behavioral strategies. We could distinguish four different aggression types. Type 1: Darting movement at the border between center and periphery (Figure 4A and Supplementary Video S5 Movie) of reef caves accompanied with high blink frequencies (Figure 3A). This type of aggression was seen for all three dummies, but was most pronounced for the blinking LED with 16.15 ± 1.57 reactions, followed by the fish dummy with 10.37 ± 1.09 attacks and the glow stick, which was attacked 5.56 ± 0.98 times on average. Type 2: Repeated swimming in stimulus direction and border crossing between periphery and center of reef caves (Figures 1E, 4B and Supplementary Video S4 Movie) accompanied by high blink frequencies (Figure 3A). This aggression type was displayed 3.6 ± 1.3 times for the LED and 4.69 ± 0.74 times for the glow stick. The fish dummy triggered 11.15 ± 1.61 type 2 reactions. Type 3: Repeated swimming in stimulus direction with U-turns directly in front of the dummies (Figure 4C and Supplementary Video S6 Movie) accompanied by high blink frequencies (Figure 3A). This aggression type was not observed for the LED, sparsely for the fish dummy (3.26 ± 1.65 attacks), but was significantly (P < 0.001) pronounced for the glow stick, where on average 22.17 ± 4.43 attacks were observed (Figure 4C). Type 4: Direct aggressive physical contact (Figure 4D and Supplementary Video S6 Movie) i.e., ram and bite reactions accompanied by high blink frequencies (Figure 3A). This attack mode was demonstrated in one case for the LED, rarely for the fish dummy (0.296 ± 0.16), but was again significantly (P < 0.001) amplified for the glow stick with on average 14.69 ± 3.66 attacks per trial (Figure 4D). Depending on the quality on the light stimulus (blink or constant glowing) and the shape of the dummies (simulated light organs or fish shape) different aggressive behavior types were provoked and observed in the flashlight fish P. steinitzi.
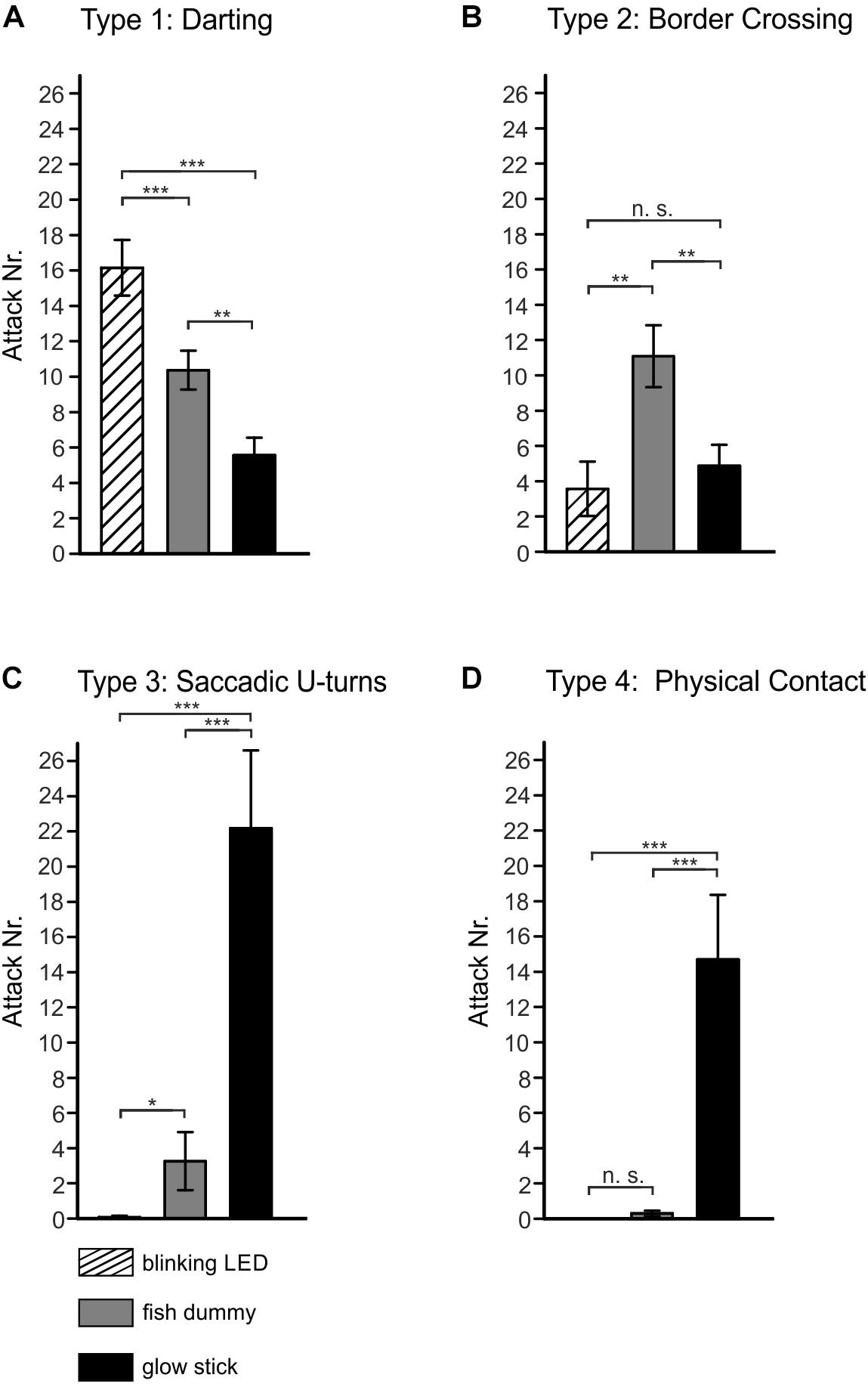
Figure 4. Aggression behavior in Photoblepharon steinitzi during exposure to fish and light organ dummies. Analysis of the aggression behavior of P. steinitzi during exposure to light organ and fish dummies, i.e., blinking LED, fish dummy, and glow stick. Four different types of aggression behavior in P. steinitzi were observed. All types of aggression were correlated with an increase in blink frequency. (A) Type 1: Darting swimming profile in the periphery. (B) Type 2: Border crossing between periphery and center of caves. (C) Type 3: Repeated swimming directly in stimulus direction followed by saccadic U-turns into the opposite direction. (D) Type 4: Aggressive physical contact with hit and bite reactions. Shaded bars indicate trials with an artificial blinking (1 Hz) light organ. Gray bars indicate trials with a fish dummy with constant glowing light organs. Black bars indicate trials with an artificial constant glowing light organ dummy. Error bars indicate mean ± SEM. Significance values are reported as: *p ≤ 0.05; **p ≤ 0.01; ***p ≤ 0.001.
Discussion
The flashlight fish P. steinitzi is a small nocturnal plankton feeder that lives in coral reefs of the Red Sea. The species is characterized by small bean shaped light organs located directly under the eyes where P. steinitzi host symbiotic luminescent bacteria of the genus “Candidatus Photodesmus blepharus” (Hendry and Dunlap, 2014). P. steinitzi and the closely related species P. palpebratum, which occur in the Pacific Ocean (Froese and Pauly, 2019), use a lid like shutter to close the light organs (Johnson and Rosenblatt, 1988) to produce striking blink patterns. Very little information is available on the role of light organs for intraspecific communication for example aggression and territory defense. Morin et al. (1975) suggested several functions for light organs in P. steinitzi such as in assisting predation, avoiding predatory fishes, and intraspecific communication based on field and laboratory observations in the Gulf of Eilat of the Red Sea. In this article we describe the role of luminescence for territorial behavior and the correlation between intraspecific bioluminescent signaling and aggressive behavior in the flashlight fish P. steinitzi in the Gulf of Aqaba of the Red Sea.
Blinking Behavior in P. steinitzi
Behavioral signaling based on visual cues is a widespread mode of behavior in fishes and was demonstrated e.g., for ultraviolet color patterns in damselfish (Pomacentrus amboinensis) in the context of aggression (Siebeck, 2004). Red fluorescence in fishes has also been discussed in the context of intraspecific communication (Michiels et al., 2008). Bioluminescent signaling in fishes was discussed for the flashlight fishes P. steinitzi (Morin et al., 1975) and the splitfin flashlight fish A. katoptron (McCosker, 1977; McCosker and Rosenblatt, 1987; Hellinger et al., 2017). Here we present the first aggression study on P. steinitzi in its native enviroment and its relation to changes in bioluminescent signals during different types of aggression using infrared video monitoring. Our experiments demonstrate a context-dependent change in the blink frequency and occurence in P. steinitzi. Our results show different occurrence times in P. steinitzi depending on different dummies presented in the territories compared to the controls without stimulation. Thus, the results suggest that a constant glowing light organ dummy trigger aggressive approaching behavior in P. steinitzi whereas a constant glowing fish dummy and a blinking light organ dummy attenuates this behavior because P. steinitzi may perceive the fish shape (dummy) and the blinking light organ dummy as an aggressor. The decrease in aggression behavior to the fish dummy could also reflect a consequence of potential dominance, i.e., P. steinitzi may recognize the fish dummy as an dominant conspecific. We found further that the flashlight fish P. steinitzi alter their blink frequencies and light organ opening times in an intruder-specific manner. Our data show that undisturbed specimens of P. steinitzi display long opening times of light organs interrupted by short times with closed light organs and thus low blink frequencies. Morin et al. (1975) also described an infrequent blink behavior with short off times of light organs for Photoblepharon specimens in the Gulf of Aqaba. In contrast to P. steinitzi the related flashlight fish species A. katoptron shows high blink frequencies (Haneda and Tsuji, 1971; McCosker, 1977; Hellinger et al., 2017) and nearly equal opened and closed light organs in the active period during dark nights (Hellinger et al., 2017). The differences in the open and closed times of the light organs of P. steinitzi and A. katoptron are probably related to the different nocturnal behaviors of the two species. A. katoptron form large schools during dark nights and hunt for zooplankton in the open water and reef flats (Haneda and Tsuji, 1971; McCosker, 1977; Hellinger et al., 2017). This behavior in A. katoptron may allow an easier detection of prey but may increase the risk to be attacked by predatory fish species. Different antipredatory responses are described in the literature (Botham et al., 2006; Ioannou et al., 2007; Marras et al., 2011; Larsson, 2012). Fast blinking and schooling behavior in A. katoptron probably distract predators in unprotected areas during the night. Long opening times and the occurrence as single specimens or in pairs in P. steinitzi may enhance the chance of becoming discovered by predatory fishes. In contrast to A. katoptron, P. steinitzi reside in the entrance area of reef caves and can easily seek shelter in the deeper and narrower parts of reef caves if predators appear. We observed that P. steinitzi in Dahab resided exclusively in the entrance areas of reef caves and close to the caves. The pioneering study by Morin et al. (1975) reported that P. steinitzi also dwelled in minimally protected areas of the reef making them more vulnerable to predators. These difference in behavior may reflect different habitat structures. It is also possible that the density of food organisms is higher in unprotected areas during different seasons in the year and P. steinitzi may swim and feed in less protected areas under these conditions. We do not exlude the possibility that P. steinitzi in Dahab display a similar behavior in less protected areas in the reef during different seasons of the year.
We observed that undisturbed P. steinitzi display mostly opened light organs. This constant glowing may assist in feeding and attract zooplankton organisms. In support of this theory we observed that P. steinitzi feed on mysid shrimp illuminated by the bioluminescent light of the light organs (Supplementary Video S3 Movie). Thus, we predict that P. steinitzi use bioluminescent light to detect and feed on zooplankton, as we described for the closely related splitfin flashlight fish A. katoptron. A. katoptron use their subocular light organs to detect planktonic prey organisms by switching their blink behavior from fast blinking to a constant glow (Hellinger et al., 2017). Prey capture by means of bioluminescence was also suggested for non-beryciform fish species. McFall-Ngai and Dunlap (1983) proposed that Gazza minuta attract and locate prey on which the fish nocturnally feeds. The bioluminescent deep-sea anglerfishes display a luminescent lure and are generally thought to attract prey with this glowing lure (Herring and Morin, 1987). The apogonid fish Siphamia versicolor has also been suggested to use their ventral light organs to illuminate and attract prey organisms (Dunlap and Nakamura, 2011).
In contrast to the nearly constant glowing pattern in undisturbed specimens, P. steinitzi significantly increased their blink frequencies in the presence of intraspecific intruders from adjacent territories (Supplementary Video S7 Movie) and artificial blinking or non-blinking light organ dummies and a constant glowing fish dummy. This behavior response was exclusively observed during intraspecific interaction and as a consequence of stimulation by the different dummies. Fast blinking behavior was also reported for A. katoptron and high blink frequencies were discussed in the context of intraspecific communication, schooling behavior and avoiding predation (McCosker, 1977; McCosker and Rosenblatt, 1987; Hellinger et al., 2017). The leiognathid species G. minuta display luminescent light flashes and the light flashes were discussed in the context of startle predators. The flashes could function as an alarming cue for nearby conspecifics in a school of G. minuta (McFall-Ngai and Dunlap, 1983). Leiognathus splendens display a synchronized flashing which was suggested for spatial organization of schooling fish and reduction of attacks by predators (Woodland et al., 2002). Our results in P. steinitzi show that the blink frequencies increased from approximately 7 blinks/min in undisturbed P. steinitzi to approximately 53 blinks/min in specimens confronted with intraspecific intruders or dummies. We found that the change in blink behavior was independent of the stimulus quality, i.e., blinking lights and constant glowing stimuli was exclusively observed in connection with agonistic behavior. Thus we assume that fast blinking in the flashlight fish P. steinitzi is an intraspecific communication signal used in the context of aggression and territory defense.
Territorial and Aggressive Behavior in the Flashlight Fish P. steinitzi
Territoriality is a common behavioral trait in fishes and very frequent in coral reef fish species. Fish often defend resources like food, shelter, mates, spawning sites and offspring (Grant, 1997). We observed numerous pairs and single specimens of P. steinitzi building territories on the reef slopes in Dahab where one territory is often directly adjacent to the neighboring territories. Several explanations for the high density of territories are feasible. The bioluminescent of P. steinitzi makes a small planktivorous fish which lacks defensive structures light sharp spines vulnerable for predation. This vulnerability may increase the benefit to form territories in the entrance area of caves, which provide shelter against predation. A further explanation for territoriality in P. steinitzi is food accessibility. During our night dives we frequently observed high amounts of mesoplankton like mysid shrimps and Chaetognaths in our dive light beams at the reef cave entrances. Thus, we assume that P. steinitzi occupy and defend feeding territories. The observation that P. steinitzi defend the territories also in pairs leads to the assumption that P. steinitzi use the territories in addition for reproduction. High food densities can increase the economic defendability of territoriality (Grant, 1997), i.e., the benefit of food accessibility is higher than the costs of defending a territory. For example, aggressiveness and monopolization of resources in the cichlid fish Cichlasoma nigrofasciatum increase with increasing predictability of food (Grant and Grant, 1994).
Territoriality often includes agonistic behavior like dynamic color displays, fast exposure of hidden structures (e.g., fin erection, gill-cover flaring), lateral displays, aggressive behavior like body beating, a vigorous swimming in place that pushes water at an opponent or physical aggression like biting (Helfman et al., 2009). We observed evasive swimming behavior and darting during intraspecific interaction induced by conspecifics and accompanied by high blink frequencies in P. steinitzi. We never observed direct physical contact like biting because the subdominant fish move back into deeper branches of reef caves. We observed and analyzed four different types of aggression and territorial defense in P. steinitzi (Figure 4) triggered by different types of dummies. All 4 types of aggression were accompanied by an increase in the blink frequency of light organs (see above and Figure 3A) and evasive swimming behavior. We assume that fast blinking is a visual display for aggression and dominance behavior. The different types of aggression occurred in a context dependent manner. For instance, a blinking LED light organ dummy triggered almost exclusively types of aggression without direct physical contact e.g., hit and biting (type 4) or close approach to the dummies associated with U-turns (type 3) in front of the intruder. We found similar results for the constant glowing fish dummy. In contrast a constant glowing light organ dummy (glow stick) triggered especially close approach to the intruder related wit U-turn (type 3) and direct aggressive physical contact (type 4). Ram and bite behavior to the glow stick could potentially also reflect a feeding attack and not territorial aggression to the dummy. Our results show clearly that P. steinitzi display constant glowing during feeding zooplankton organisms and high blink frequencies during aggression behavior. A previous study on the related flashlight fish A. katoptron reported also constant opened light organs during feeding on zooplankton (Hellinger et al., 2017). Thus, we assume that the reaction in P. steinitzi display aggression behavior and no feeding response to the glow stick. The decision for territorial aggression behavior must be made in a context of danger for the defender of a distinct territory. The fish shape (constant glowing fish dummy) may decrease the aggression behavior as a consequence of dominance. In this case the fish shape of the dummy may act as a signal for dominance and the signal may exceed the motivation for aggression triggered by the constant glowing light organ in the dummy. The conflicting demands between aggressively defending a territory and danger for the defender may create a defense-injury risk trade-off. Similar trade-offs were also known in the context of foraging against predation risk (Helfman et al., 2009). A fast blinking light organ dummy (LED) simulates an intruder with an increased aggression level and therefore decreases the internal motivation for a direct approach and physical aggression to avoid an injury risk for the defender. Our results show that a constant glowing fish dummy representing the shape of a fish body, induced a similar aggressive response. Thus, the aggression potential in P. steinitzi depends on more visual signals such as blinking frequency and fish shape. In contrast, a constant glowing light organ dummy (glow stick) without the fish shape appeared to signal a less aggressive intruder and thus encouraging a direct approach and physical aggression for the defender of a territory due to a lower risk of injury. Thus, the lack of visual signals such as fast blinking of light organs or the shape of the fish increase the motivation for physical aggression behavior in the defender of a territory because of the lower risk of injury by a non-aggressive intruder. In contrast, high blink frequencies indicate aggression by the intruder and decrease the motivation for physical aggression in the defender of a territory because of a higher risk of injury. Another factor, which may also affect the level of aggressive behavior, is former experiences in defending territories. Past aggressive encounters and their outcomes can often affect the aggressive behavior and outcomes of subsequent aggressive contests. For example, a fish with more unsuccessful encounters in the past may be less likely to show aggressive attacks compared to a fish with many successful contests (Hsu et al., 2005).
In summary, we show that P. steinitzi use their bioluminescent light organs as a visual intraspecific signal to convey information for aggression, dominance and territorial behavior. Undisturbed P. steinitzi show nearly constant exposed light organs and use the light to feed on planktonic prey in the absence of intraspecific competitors in their territories. In addition, we demonstrate that the intensity of displayed aggression in P. steinitzi depends on the signal properties of the artificial intruder. A constant glowing intruder increase the aggression level in P. steinitzi whereas a blinking light organ dummy that simulate an intruder and a constant glowing dummy that display the fish shape decrease the aggression level in P. steinitzi.
Data Availability Statement
The raw data supporting the conclusions of this article will be made available by the authors, without undue reservation, to any qualified researcher.
Ethics Statement
Ethical review and approval was not required for the animal study because the research represent behavioral studies in the field.
Author Contributions
JH and SH developed the questions and experimental concept, designed the experiments, conducted the data analysis and interpretation of data. JH, PJ, LW, KS, and SH conducted experiments. JH, SH, and MM wrote the manuscript with contributions from all authors.
Funding
This work was supported by funds from the Ruhr-University of Bochum, Germany.
Conflict of Interest
The authors declare that the research was conducted in the absence of any commercial or financial relationships that could be construed as a potential conflict of interest.
Acknowledgments
We would like to thank Dr. Dietrich Kurt Hofmann for the helpful literature research. We would also like to thank Stefan Dobers and Stefan Rasche from the faculty workshop for technical support. We additionally thank our hosts in Dahab for providing excellent working conditions.
Supplementary Material
The Supplementary Material for this article can be found online at: https://www.frontiersin.org/articles/10.3389/fmars.2020.00078/full#supplementary-material
VIDEO S1 | Movie single. Single Photoblepharon steinitzi: Undisturbed behavior in its native environment in a coral reef cave in the Red Sea.
VIDEO S2 | Movie pair. Pair of Photoblepharon steinitzi: Undisturbed behavior in its native environment in a coral reef cave in the Red Sea.
VIDEO S3 | Movie feeding. Feeding behavior of in a pair of Photoblepharon steinitzi in its native environment in a coral reef cave in the Red Sea.
VIDEO S4 | Movie fish dummy. Blink and aggression behavior of a Photoblepharon steinitzi pair during exposure to a fish dummy with constant glowing light organ in its native environment in a coral reef cave in the Red Sea. The dummy is visible in the lower right corner of the screen.
VIDEO S5 | Movie blinking light organ dummy. Blink and aggression behavior of a Photoblepharon steinitzi pair during exposure to a blinking light organ dummy in its native environment in a coral reef cave in the Red Sea.
VIDEO S6 | Movie constant glowing light organ dummy. Blink and aggression behavior of a single Photoblepharon steinitzi during exposure to a constant glowing light organ dummy in its native environment in a coral reef cave in the Red Sea.
VIDEO S7 | Movie intraspecific interaction. Blink and aggression behavior in two pairs of Photoblepharon steinitzi during intraspecific interaction in its native environment in a coral reef cave in the Red Sea. Two specimens of P. steinitzi appear in the first second. The fish on the right side show the intruder. The fish on the left side show the territory owner.
References
Botham, M. S., Kerfoot, C. J., Louca, V., and Krause, J. (2006). The effects of different predator species on antipredator behavior in the Trinidadian guppy, Poecilia reticulata. Naturwissenschaften 93, 431–9. doi: 10.1007/s00114-006-0131-0
Bowlby, M. R., Widder, E. A., and Case, J. F. (1991). Disparate Forms of Bioluminescence from the Amphipods Cyphocaris faurei, Scina crassicornis and S. borealis. Mar. Biol. 108, 247–253. doi: 10.1007/bf01344339
Claes, J. M., Dean, M. N., Nilsson, D., Hart, N. S., and Mallefet, J. (2013). A deepwater fish with ‘lightsabers’-dorsal spine associated luminescence in a counterilluminating lanternshark. Sci. Rep. 3:1308. doi: 10.1038/srep01308
Claes, J. M., Ho, H. C., and Mallefet, J. (2012). Control of luminescence from pygmy shark (Squalus aliae) photophores. J. Exp. Biol. 215, 1691–1699. doi: 10.1242/jeb.066704
Davies, M. P., Sparks, J. S., and Smith, W. L. (2016). Repeated and widespread evolution of bioluminescence in marine fishes. PLoS One 11:e0155154. doi: 10.1371/journal.pone.0155154
Dunlap, P. V., and Nakamura, M. (2011). Functional morphology of the luminescence system of Siphamia versicolor (Perciformes: Apogonidae), a bacterially luminous coral reef fish. J. Morphol. 272, 897–909. doi: 10.1002/jmor.10956
Freudenberg, F., Carreño Gutierrez, H., Post, A. M., Reif, A., and Norton, W. H. J. (2016). Aggression in non-human vertebrates: Genetic mechanisms and molecular pathways. Am. J. Med. Genet. B Neuropsychiatr. Gen. 171, 603–640. doi: 10.1002/ajmg.b.32358
Froese, R., and Pauly, D. eds (2019). FishBase. World Wide Web electronic publication. www.fishbase.org, version (08/2019).
Grant, J. W. A. (1997). “Territoriality” in Behavioral ecology of teleost fishes. ed. J.-G. J. Godin. (Oxford, UK: Oxford university press), 81–104.
Grant, T. C., and Grant, J. W. A. (1994). Spatial predictability of food influences ist monopolisation and defense by juvenile convict cichlids. Anim. Behav. 47, 91–100. doi: 10.1006/anbe.1994.1010
Gruber, D. F., Phillips, B. T., O’Brien, R., Boominathan, V., Veeraraghavan, A., Vasan, G., et al. (2019). Bioluminescent flashes drive nighttime schooling behavior and synchronized swimming dynamics in flashlight fish. PLoS One 14:e0219852. doi: 10.1371/journal.pone.0219852
Haddock, S. H. D., Moline, M. A., and Case, J. F. (2010). Bioluminescence in the Sea. Ann. Rev. Mar. Sci. 2, 443–493.
Haneda, Y., and Tsuji, F. I. (1971). Light Production in the Luminous Fishes Photoblepharon and Anomalops from the Banda Islands. Science 173, 143–145. doi: 10.1126/science.173.3992.143
Haygood, M. D., and Diestel, D. L. (1993). Bioluminescent symbionts of flashlight fishes and deep-sea anglerfishes form unique lineages related to the genus Vibrio. Nature 363, 154–156. doi: 10.1038/363154a0
Helfman, G. S., Collette, B. B., Facey, D. E., and Bowen, B. W. (2009). The Diversity of Fishes: Biology, Evolution, and Ecology. Second Edition (. Hoboken, NJ: Wiley-Blackwell.
Hellinger, J., Jägers, P., Donner, M., Sutt, F., Mark, M. D., Senen, B., et al. (2017). The flashlight fish Anomalops katoptron uses bioluminescent light to detect prey in the dark. PLoS One 12:e0170489. doi: 10.1371/journal.pone.0170489
Hendry, T. A., and Dunlap, P. (2014). Phylogenetic divergence between the obligate luminous symbionts of flashlight fishes demonstrates specificity of bacteria to host genera. Environ. Microbiol. Rep. 6, 331–338. doi: 10.1111/1758-2229.12135
Herring, P. J., and Cope, C. (2005). Red bioluminescence in fishes: on the suborbital photophores of Malacosteus, Pachystomias and Aristostomias. Mar. Biol. 148, 383–394. doi: 10.1007/s00227-005-0085-3
Herring, P. J., and Morin, J. G. (1987). “Bioluminescence in fishes,” in Bioluminescence in action, ed. P. J. Herring, (New York: Academic Press), 273–329.
Hsu, Y., Early, R. L., and Wolf, L. L. (2005). Modulation of aggressive behavior by fighting experience: mechanisms and contest outcomes. Biol. Rev. 81, 33–74.
Ioannou, C. C., Tosh, C. R., Neville, L., and Krause, J. (2007). The confusion effect—from neural networks to reduced predation risk. Behav. Ecol. 19, 126–130. doi: 10.1093/beheco/arm109
Johnson, G. D., and Rosenblatt, R. H. (1988). Mechanisms of light organ occlusion in flashlight fishes, family Anomalopidae (Teleostei: Beryciformes), and the evolution of the group. Zool. J. Linn. Soc. 94, 65–96. doi: 10.1111/j.1096-3642.1988.tb00882.x
Mark, M. D., Donner, M., Eickelbeck, D., Stepien, J., Nowrousian, M., Kück, U., et al. (2018). Visual tuning in the flashlight fish Anomalops katoptron to detect blue, bioluminescent light. PLoS One 13:e0198765. doi: 10.1371/journal.pone.0198765
Marras, S., Batty, R. S., and Domenici, P. (2011). Information transfer and antipredator maneuvers in schooling herring. Adapt. Behav. 20, 44–56. doi: 10.1177/1059712311426799
McCosker, J. E., and Rosenblatt, R. H. (1987). Notes on the biology, taxonomy, and distribution of flashlight fishes (Beryciformes: Anomalopidae). Jpn. J. Ichthyol. 34, 157–164. doi: 10.1007/BF02912410
McFall-Ngai, M. J., and Dunlap, P. V. (1983). Three new modes of luminescence in the leiognathid fish Gazza minuta: Discrete projected luminescence, ventral body flash, and buccal luminescence. Mar. Biol. 73, 227–237. doi: 10.1007/bf00392247
Michiels, N. K., Antes, N., Hart, N. S., Herler, J., Meixner, A. J., Schleifenbaum, F., et al. (2008). Red fluorescence in reef fish: a novel signalling mechanism? BMC Ecol. 8:16. doi: 10.1186/1472-6785-8-16
Morin, J. G., Harrington, A., Nealson, K., Krieger, N., Baldwin, T. O., and Hastings, J. W. (1975). Light for all reasons: versatility in the behavioral repertoire of the flashlight fish. Science 190, 74–76. doi: 10.1126/science.190.4209.74
Siebeck, U. E. (2004). Communication in coral reef fish: the role of ultravioletcolour patterns in damselfish territorial behaviour. Anim. Behav. 68, 273–282. doi: 10.1016/j.anbehav.2003.11.010
Widder, E. A. (2010). Bioluminescence in the Ocean: origins of Biological; Chemical and Ecological Diversity. Science 328, 704–708. doi: 10.1126/science.1174269
Wilson, T., and Hastings, J. W. (1998). Bioluminescence. Ann. Rev. Cell Dev. Biol. 14, 197–230. doi: 10.1146/annurev.cellbio.14.1.197
Keywords: bioluminescence, flashlight fish, aggression behavior, teleost, territorial behavior
Citation: Hellinger J, Jägers P, Spoida K, Weiss LC, Mark MD and Herlitze S (2020) Analysis of the Territorial Aggressive Behavior of the Bioluminescent Flashlight Fish Photoblepharon steinitzi in the Red Sea. Front. Mar. Sci. 7:78. doi: 10.3389/fmars.2020.00078
Received: 06 November 2019; Accepted: 31 January 2020;
Published: 19 February 2020.
Edited by:
Stelios Katsanevakis, University of the Aegean, GreeceReviewed by:
Alexander Kasumyan, Lomonosov Moscow State University, RussiaYuichi Takeuchi, University of Toyama, Japan
Copyright © 2020 Hellinger, Jägers, Spoida, Weiss, Mark and Herlitze. This is an open-access article distributed under the terms of the Creative Commons Attribution License (CC BY). The use, distribution or reproduction in other forums is permitted, provided the original author(s) and the copyright owner(s) are credited and that the original publication in this journal is cited, in accordance with accepted academic practice. No use, distribution or reproduction is permitted which does not comply with these terms.
*Correspondence: Stefan Herlitze, c3hoMTA2QGdtYWlsLmNvbQ==