- 1Institute for Marine and Antarctic Studies, University of Tasmania, Hobart, TAS, Australia
- 2Department Primary Industries, NSW Fisheries, Coffs Harbour, NSW, Australia
- 3National Marine Science Centre, Southern Cross University, Coffs Harbour, NSW, Australia
- 4The University of Sydney, School of Life and Environmental Sciences, Coastal and Marine Ecosystems, Sydney, NSW, Australia
- 5Sydney Institute of Marine Science, Mosman, NSW, Australia
- 6Singapore Centre for Environmental Life Sciences Engineering, Nanyang Technological University, Singapore, Singapore
- 7Centre for Marine Science and Innovation, School of Biological Earth and Environmental Sciences, The University of New South Wales, Sydney, NSW, Australia
- 8National Centre for Coasts and Climate, School of Biosciences, The University of Melbourne, Melbourne, VIC, Australia
- 9UWA Oceans Institute and School of Biological Sciences, The University of Western Australia, Crawley, WA, Australia
Kelp forests dominate the rocky coasts of temperate Australia and are the foundation of the Great Southern Reef. Much like terrestrial forests, these marine forests create complex habitat for diverse communities of flora and fauna. Kelp forests also support coastal food-webs and valuable fisheries and provide a suite of additional ecosystem services. In many regions of Australia and around the world, kelp forests are in decline due to ocean warming, overgrazing, and pollution. One potential tool in the conservation and management of these important ecosystems is habitat restoration, the science and practice of which is currently undergoing substantial expansion. We summarize the present state of Australian kelp forests and emphasize that consideration of the initial drivers of kelp decline is a critical first step in restoration. With a focus on Australian examples, we review methods, implementation and outcomes of kelp forest restoration, and discuss suitable measures of success and the estimated costs of restoration activities. We propose a workflow and decision system for kelp forest restoration that identifies alternative pathways for implementation and acknowledges that under some circumstances restoration at scale is not possible or feasible. As a case study, we then apply the Society for Ecological Restoration’s 5-star evaluation to Operation Crayweed, Australia’s primary example of kelp forest restoration. Overall, no single method of kelp forest restoration is suitable for all situations, but outcomes can be optimized by ameliorating the driver(s) of kelp decline and achieving ongoing natural recruitment of kelp. Whilst scalability of kelp forest restoration to the seascape-scale remains a considerable challenge, the present review should provide a platform for future restoration efforts. However, it is also crucial to emphasize that the challenges of restoration place a high value on preventative conservation and protection of existing kelp forest ecosystems – prevention is invariably better than cure.
The Role of Kelp Forests
Kelp1 dominate rocky coastal environments in temperate and subpolar latitudes around the world (Smale et al., 2013; Krumhansl et al., 2016; Wernberg et al., 2019). These habitat-forming macroalgae occur in intertidal and subtidal habitats and range in size from less than a meter to over 40 m in length. Much like terrestrial forests, kelp forests are complex three-dimensional habitats with modified sub-canopy conditions (Gaylord et al., 2007; Layton et al., 2019a) that support diverse communities of associated flora and fauna (Steneck and Johnson, 2014; Teagle et al., 2017; Miller et al., 2018). Kelp also act as the trophic foundation of coastal food-webs by providing food for a suite of grazers, detritivores, and microbes (Schiel and Foster, 2015; Wernberg et al., 2019) – the effects of which can reach to adjacent reef, seagrass, and sediment communities (Bustamente et al., 1995; Bishop et al., 2010), as well as to deep waters and beyond the continental shelf (Harrold et al., 1998; Thompson et al., 2011; Filbee-Dexter et al., 2018).
Most shallow (<30–50 m) rocky reefs in temperate Australia are dominated by kelp (Table 1; Marzinelli et al., 2015; Bennett et al., 2016; Coleman and Wernberg, 2017; Wernberg et al., 2019). Altogether, these kelp-dominated rocky reefs form an ∼8,000 km long interconnected system known as the Great Southern Reef (GSR, Bennett et al., 2016), and sustain high levels of biodiversity and productivity (Ling, 2008; Bennett et al., 2016; Wernberg et al., 2019). One remarkable feature of biodiversity on the GSR is the high levels of endemism, and this is particularly true for macroalgae, with the GSR a global hotspot of macroalgal biodiversity and endemism (Womersley, 1987, 1994; Phillips, 2001; Kerswell, 2006). The GSR is also a global biodiversity hotspot for bryozoans, chordates, crustaceans, echinoderms, molluscs and sponges, with rates of endemism across these taxa ranging from ∼20–60% (Bennett et al., 2016).
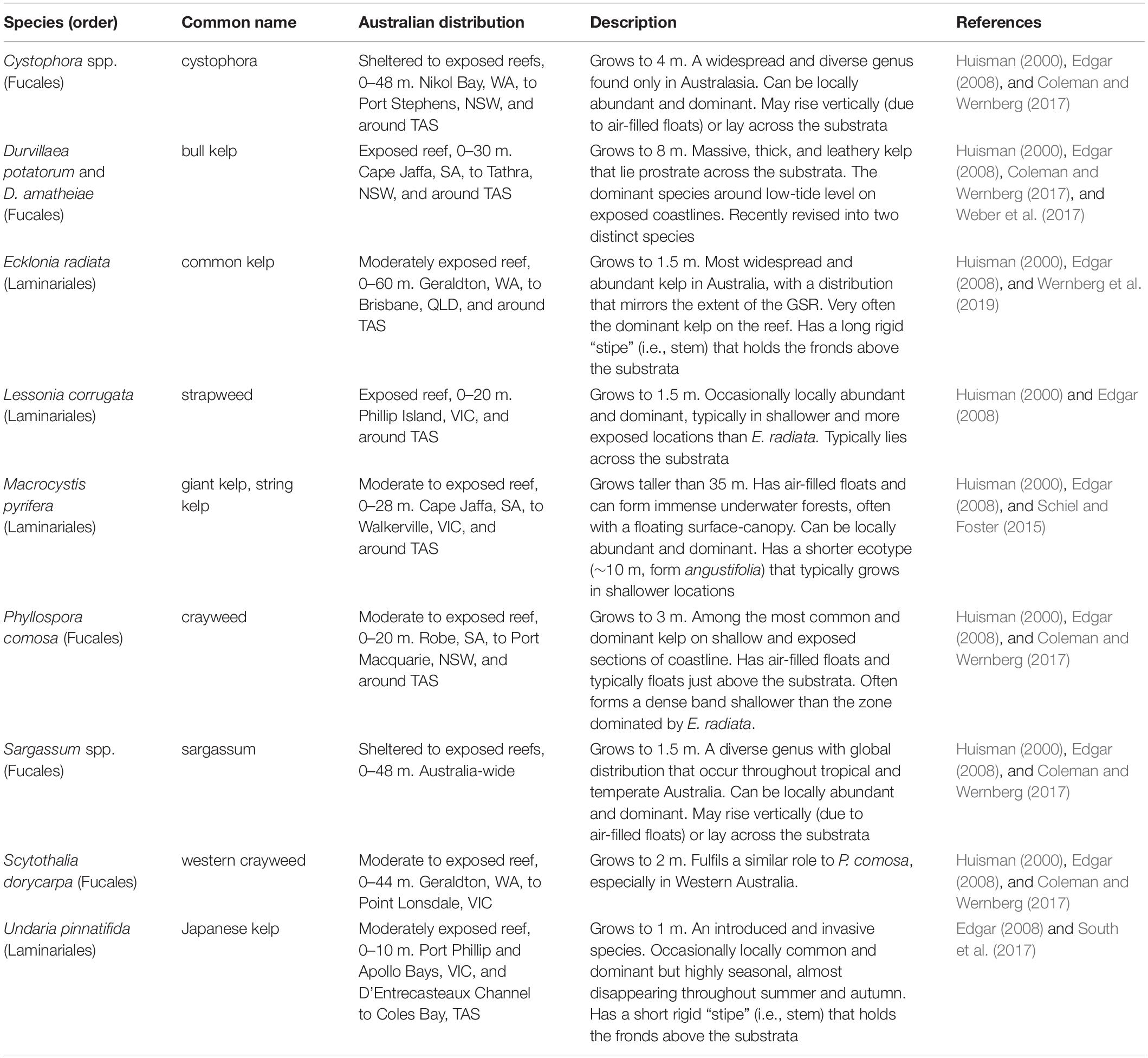
Table 1. The primary genera/species of habitat-forming kelp in Australia. Unlike the northern hemisphere where kelp canopies primarily consist of “true” laminarian kelps, canopy-forming species in Australia constitute both laminarian and fucalean algae, with a larger diversity of fucoids than laminarians.
Australian kelp forests also have high economic value and support many fisheries, including the rock lobster and abalone fisheries that contribute >US$600 million p.a. to the national economy (ABARES, 2019). Beyond direct economic outputs, a lack of data makes it difficult to quantify the full value of ecosystem services provided by kelp forests in Australia. Notably, indirect commercial and social benefits arising from kelp forests are likely to be substantial, especially in coastal communities. These include indirect effects on fisheries (e.g. influence on coastal food-webs and prey species), recreational fishing, ecotourism, and other forms of marine recreation (e.g. scuba-diving) (Bennett et al., 2016). Despite their significant value, Australia temperate marine ecosystems are conspicuously underfunded and understudied relative to their tropical counterparts (Bennett et al., 2016).
Status of Australian Kelp Forests and Drivers of Decline
A strong rationale for considering restoration of Australia kelp forests is that they are in decline in many regions globally. In Australia, significant declines of kelp have occurred in Western Australia (Smale and Wernberg, 2013; Wernberg et al., 2016), South Australia (Connell et al., 2008), Tasmania (Ling, 2008; Johnson et al., 2011), Victoria (Kriegisch et al., 2016; Carnell and Keough, 2019), New South Wales (Andrew and O’Neill, 2000; Coleman et al., 2008; Vergés et al., 2016), and Southern Queensland (Phillips and Blackshaw, 2011). In these areas, and in many locations globally, drivers of kelp forest decline include both physical and biological factors (also see Krumhansl et al., 2016), and these must be understood for restoration efforts to be effective.
In Western Australia, an extreme marine heatwave over the 2010/2011 summer, in combination with southward range extension of subtropical herbivorous fishes associated with ocean warming, resulted in the loss of Ecklonia radiata (the dominant kelp across the GSR) and Scytothalia dorycarpa forests from ∼100 km of coastline between Kalbarri and Geraldton (Smale and Wernberg, 2013; Wernberg et al., 2016). In South Australia, kelp forest losses have been mostly attributed to urbanization and increased runoff of sediments and nutrients (Connell et al., 2008; Gorman and Connell, 2009). Consequently, kelp forests within ∼25 km of Adelaide, consisting mostly of E. radiata, have been largely replaced by less complex and less productive turf algae habitats (Figure 1). There has also been widespread loss of E. radiata in Port Phillip Bay, Victoria, particularly along the western and northern coastlines near the metropolitan areas of Geelong and Melbourne (Kriegisch et al., 2016; Carnell and Keough, 2019). Here, overgrazing by Heliocidaris erythrogramma urchins is the primary cause of kelp destruction, but subsequent proliferation of turf algae, in part due to high nutrient levels, also acts to inhibit kelp recruitment and recovery (Kriegisch et al., 2016; Reeves et al., 2018).
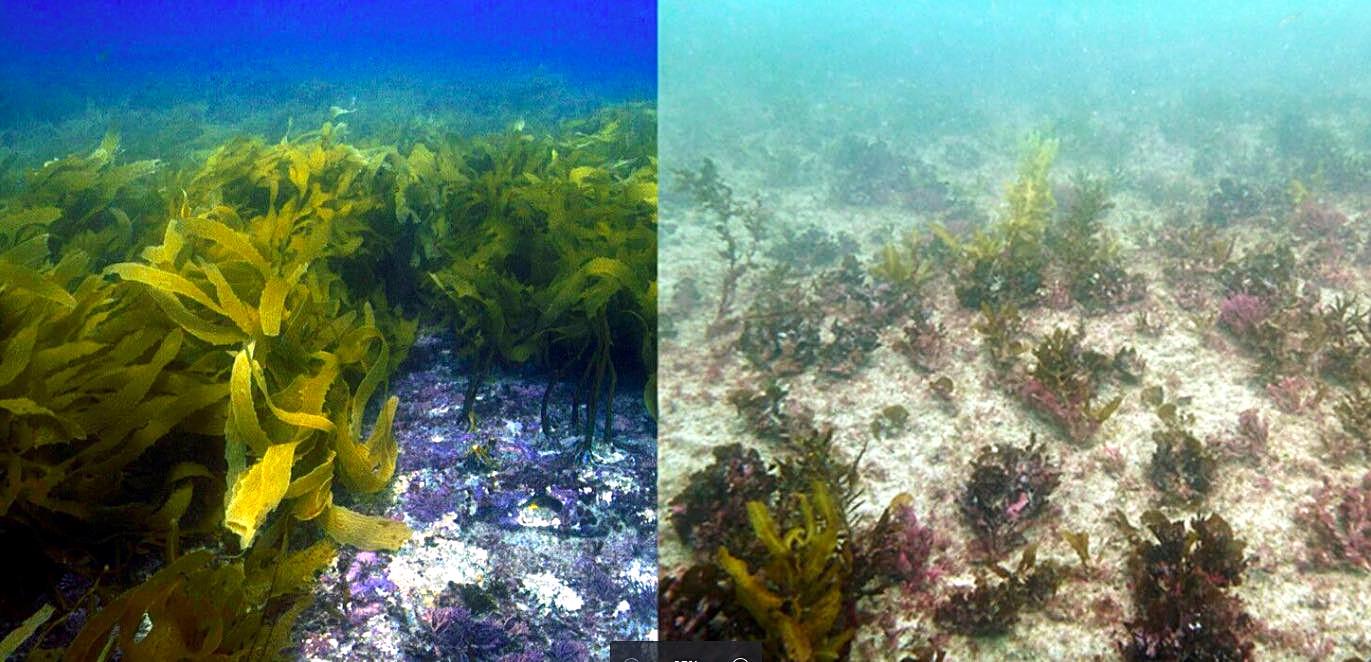
Figure 1. Example of Ecklonia radiata kelp forests (left) replaced by turf algae (right). Putative drivers include increased nutrients, and inhibition of kelp recruitment by the turf algae and entrained sediments. Photos by TW.
Destructive grazing by urchins is also a significant driver of kelp forest loss across Tasmania and the Bass Strait (Johnson et al., 2005, 2011; Ling, 2008), where urchin barrens formed by Centrostephanus rodgersii are now extensive and have replaced formerly lush kelp forests (Ling and Keane, 2018). This urchin, previously only abundant along the New South Wales coast, has undergone southern range extension over the last several decades due to increasing poleward penetration of the East Australia Current (EAC, Johnson et al., 2005, 2011; Ling, 2008). While warming waters and a strengthened EAC are responsible for the incursion of the urchin into southern waters, their local proliferation is linked to ecological overfishing of large southern rock lobster (Jasus edwardsii), which are the primary predator of C. rodgersii urchins in Tasmania (Ling et al., 2009; Johnson et al., 2011). While overgrazing by urchins has mostly affected E. radiata kelp forests, Tasmania has also suffered extensive losses of giant kelp (Macrocystis pyrifera) forests (Johnson et al., 2011). The loss of these iconic underwater forests (Figure 2) is mostly attributed to the increasing influence of the warm, nutrient-poor waters of the EAC in Tasmania, although urchin overgrazing is likely to have exacerbated the problem (also see Ling, 2008; Ling and Keane, 2018) and be impeding recovery in some areas. Overall, more than 95% of Tasmania’s surface canopy-forming giant kelp forests (which also occur to a lesser extent in parts of Victoria and South Australia) have been lost over recent decades, to be replaced by E. radiata forests or urchin barrens (Johnson et al., 2011; Ling and Keane, 2018). Consequently, in 2012 the giant kelp forests of southeast Australia became the first marine community listed as endangered under the Australian Federal Government Environment Protection and Biodiversity Conservation Act (Evans et al., 2017). There is still no Federal Recovery Plan prepared for this community.
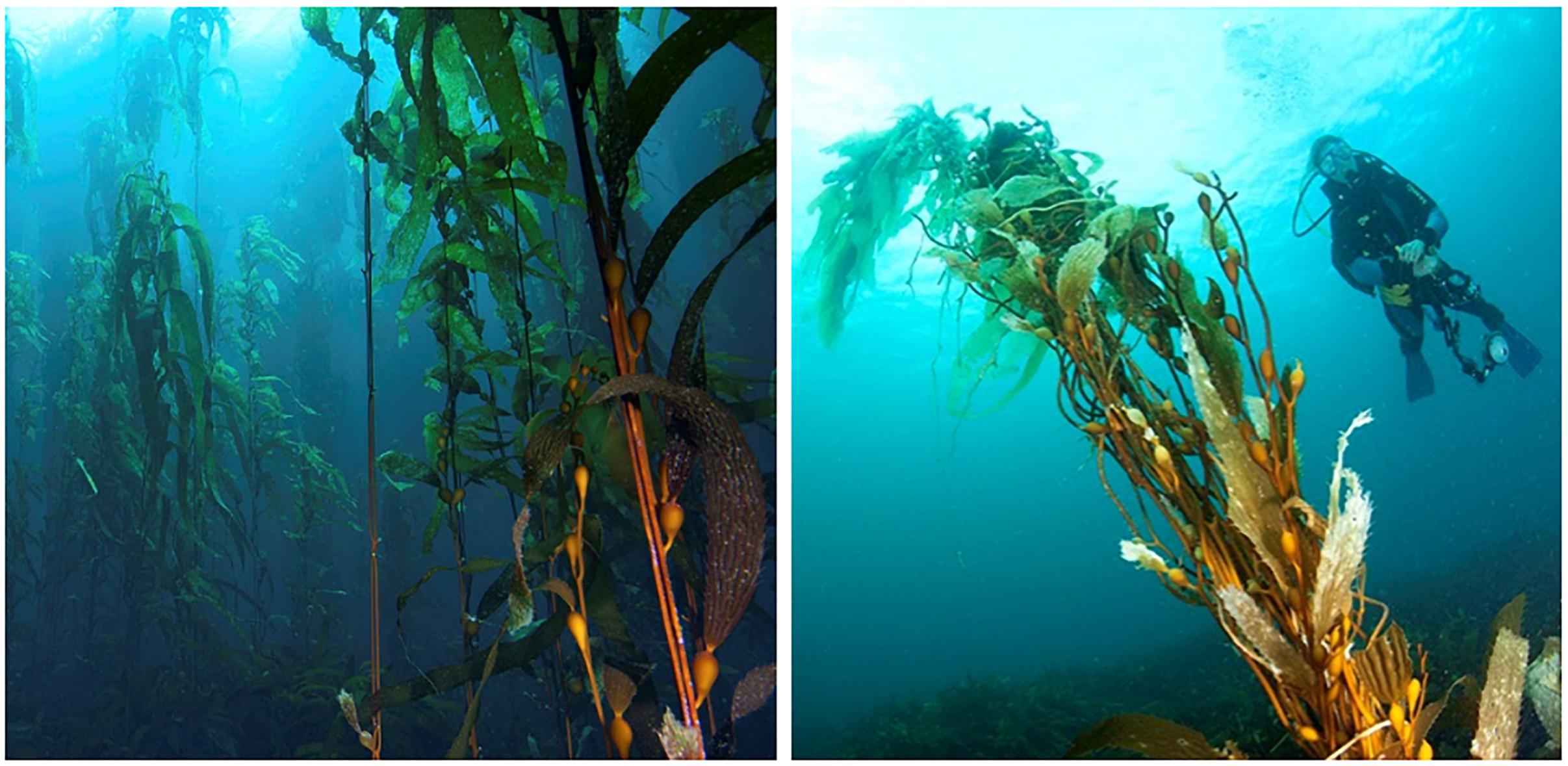
Figure 2. Examples of healthy (left) and degraded (right) giant kelp (Macrocystis pyrifera) forests in Tasmania. Photos reproduced with permission of Matthew Ramaley (left) and Matthew Doggett (right).
The C. rodgersii urchin has also contributed to extensive barren formation across its historical range in New South Wales (Andrew, 1993; Andrew and O’Neill, 2000). Urchin barrens are estimated to extend across >50% of shallow rocky reef habitats along the central and southern coastlines of the state (Andrew and O’Neill, 2000), suggesting widespread losses of the two dominant kelp in the region, E. radiata and Phyllospora comosa. These urchin barrens likely formed over many decades – possibly due to overfishing of urchin predators such as eastern rock lobster (Sagmariasus verreauxi) and eastern blue groper (Achoerodus viridis) (Ling et al., 2009; Ling and Johnson, 2012; Evans et al., 2017) – in conjunction with persistent declines in kelp cover associated with increasing urbanization and ocean warming (Andrew and O’Neill, 2000; Coleman et al., 2008; Mabin et al., 2013).
Urban development on the coasts of metropolitan Sydney, and in particular untreated sewage outfalls, were implicated in the local extinction of P. comosa forests throughout the 1980’s (Coleman et al., 2008). Following improvements in wastewater infrastructure and water quality, these forests are now being restored under the aegis of Operation Crayweed (see below). Losses of E. radiata forests have also occurred on islands off northern New South Wales, toward the northern limit of the species’ distribution, and have been attributed to warming waters and overgrazing by herbivorous subtropical fishes (Vergés et al., 2016). This poleward shift of sub/tropical species into temperate waters is referred to as tropicalization and is expected to increase in the future as oceans continue to warm (Vergés et al., 2016; Zarco-Perello et al., 2017). Indeed, it seems that increasing ocean temperatures – especially in southeast and southwest Australia, which represent global hotspots of ocean warming (Hobday and Pecl, 2014) – are likely to cause continued poleward range contractions of Australian kelp, to be replaced by smaller subtropical macroalgae (Wernberg et al., 2016; Coleman et al., 2017; Martínez et al., 2018) and substantially altering ecosystem functioning (Vergés et al., 2019).
Benefits and Values of Kelp Forest Restoration
Since kelp forests are the foundation of Australia’s rocky reef ecosystems, it follows that maintaining or increasing the abundance and health of kelp forests via restoration could lead to concomitant benefits for production, biodiversity, and fisheries. Work has demonstrated that following urchin removal, small areas of recovered E. radiata kelp forests can support similar communities to natural E. radiata forests (Ling, 2008). However, this is not always the case and “recovered” kelp-dominated communities can be dissimilar to the pre-loss state (Valentine and Johnson, 2003). On artificial reefs in Tasmania, transplanting of adult E. radiata encouraged recruitment of economically and ecologically valuable invertebrates and fishes (including Ostrea angasi oysters and J. edwardsii rock lobsters), and facilitated development of diverse assemblages of flora and fauna (Layton et al., 2019a; Shelamoff et al., 2019). Whilst restoration of P. comosa forests by Operation Crayweed has had cascading benefits on epifaunal community composition, lags in system dynamics mean that restored communities can require time (i.e. years) to match natural P. comosa forests (Marzinelli et al., 2016).
Coastal macroalgal beds – of which kelp are the largest component by biomass – have also been identified as potentially important sinks of marine carbon (so called blue carbon) (Krause-Jensen and Duarte, 2016; Macreadie et al., 2019; Queirós et al., 2019). Recent work suggests that a significant portion of kelp forest biomass can be transported to coastal sediments and the deep ocean (Filbee-Dexter et al., 2018; Queirós et al., 2019). Furthermore, since macroalgal beds comprise such massive biomass, even conservative estimates of carbon transport/storage suggest that sequestration by kelp-dominated macroalgae beds can be a considerable contributor to blue carbon sinks (Macreadie et al., 2019; Queirós et al., 2019).
High biomass and fast growth rates ensure kelp forests also have critical roles in coastal nutrient-cycling (Smale et al., 2013; Bennett et al., 2016) and have great potential to absorb nutrients for bioremediation. Integrated multi-trophic aquaculture is a rapidly emerging field that might be able to utilize kelp to absorb excess nutrients associated with shellfish or finfish aquaculture (Buschmann et al., 2017; Hadley et al., 2018). Other emerging technologies and investments are also positioning kelp and macroalgae as a cornerstone of future blue economy applications, including as food for human consumption, livestock feed, biofuel, nutraceuticals, and pharmaceuticals (Buschmann et al., 2017; Froehlich et al., 2019). These high-value products represent market opportunities and economic incentives to help fund restoration efforts and thus contribute to the “restoration economy” (BenDor et al., 2015).
Kelp forests can also modify local hydrography and bolster coastal defenses by dampening ocean swell and decreasing erosion (Løvås and Tørum, 2001; Gaylord et al., 2007). This service should be given special consideration with regards to forecast increases in sea level and storm activity due to climate change (IPCC, 2014).
Kelp and associated macroalgae also play an important role in Indigenous Australian culture and tradition (reviewed by Thurstan et al., 2018). Uses include ceremonial activities, medicinal practices, clothing, food, shelter, and as domestic devices. Archival records of the use of bull kelp (i.e. Durvillaea spp.) are particularly numerous, and there is considerable contemporary use of this kelp by Indigenous practitioners in artistic and knowledge-sharing activities. Additionally, the culturing, outplanting and monitoring that large-scale kelp forest restoration efforts require, provides ideal opportunities for Indigenous employment, management and custodianship (also see McLeod et al., 2018), and to establish skills and knowledge that underpin macroalgae farming.
Kelp Forest Restoration Attempts: Successes and Failures
The restoration of kelp forests globally has typically followed two broad strategies: assisted recovery and active restoration. Assisted recovery – where natural kelp recovery is facilitated by either the removal of the agent of decline (e.g. culling of sea urchins, Ling, 2008; House et al., 2018) or the installation of substrata for kelp colonization (e.g. artificial reefs, Carter et al., 1985; Ambrose, 1994; Terawaki et al., 2001) – has been successful at increasing kelp recruitment and development of a kelp canopy over the short-term. Results are nonetheless highly variable and site-dependent, and projects involving removal of the agents of decline have seemingly had greater success than those that only provide novel substratum. This may be due to the unsuitability of some artificial substrata for kelp colonization, and/or effects of other colonizing organisms (e.g. filamentous turf algae, mussel, and barnacles) on kelp recruitment. Critically, assisted recovery approaches are often hindered by resource constraints and by hysteresis and feedbacks in the ecological dynamic (see Scheffer et al., 2001; Marzloff et al., 2011), which impair kelp recruitment and reestablishment even after the initial driver of decline has been ameliorated (Gorman and Connell, 2009; Johnson et al., 2017). We are aware of only one example where assisted recovery in isolation has resulted in the long-term restoration of kelp forests (giant kelp in California, see Reed et al., 2006, 2017).
Active restoration efforts have typically had greater success, and typically involve transplanting adult or juvenile kelp from a donor site, or outplanting lab-cultured kelp (North, 1976; Hernández-Carmona et al., 2000; Perkol-Finkel et al., 2012; Zarco-Perello et al., 2017; Verdura et al., 2018). The long-term success of this approach is reliant on either continued transplantation of kelp – which can be cost-prohibitive and dependent on a healthy donor population (North, 1976; Devinny and Leventhal, 1979) – or adequate natural recruitment of juvenile kelp. In the latter case, recruitment could occur from nearby populations of kelp and/or from the transplanted kelp itself (see Operation Crayweed below). Notably, the planting of juvenile kelp (whether lab-cultured or otherwise) has had little success (but see Perkol-Finkel et al., 2012) unless it is combined with the outplanting of adult kelp (North, 1976; Devinny and Leventhal, 1979; Layton et al., 2019a). This may be due to increased herbivory, competition, or abiotic stressors that cause mortality of juvenile kelp in the absence of adults (Hernández-Carmona et al., 2000; Konar and Estes, 2003; Vergés et al., 2016; Layton et al., 2019a).
Within Australia there have been few attempts to restore kelp forests. The earliest reported work comes from the Seacare community group (Sanderson, 2003) who attempted to restore areas of giant kelp (M. pyrifera) in Tasmania. Multiple techniques were used, including transplanting juvenile kelp from donor populations; transplanting artificial substrata on which juvenile giant kelp were growing following natural recruitment; transplanting sporophylls (i.e. the reproductive fronds of giant kelp); and outplanting ropes seeded with small (∼5 mm) lab-cultivated juvenile kelp. Centrostephanus rodgersii urchins were also removed at some restoration sites and improved the likelihood of positive outcomes. Nonetheless, the project realized only marginal success and outcomes varied markedly across the 10 + sites. A single patch of giant kelp was established at one site but subsequently disappeared, in keeping with the ongoing decline of giant kelp in southeast Australia. The methods employed at the site of success did not differ from those at other sites (i.e. transplanting ∼100 juvenile kelp and 3 fertile sporophylls), but it did have the most similar community composition to the donor site and was the most exposed and southerly location of the restoration sites.
Operation Crayweed is the only other reported example of targeted kelp forest restoration in Australia of which we are aware (although there are several projects currently underway, as discussed below). This ongoing project began in 2011 and aims to restore crayweed (P. comosa) forests to metropolitan Sydney where the species was once abundant (Coleman et al., 2008; Campbell et al., 2014; Marzinelli et al., 2014, 2016). Adult crayweed are transplanted from donor populations outside of metropolitan Sydney to restoration sites, with the primary aim to establish sufficient adult individuals to promote recruitment of juvenile crayweed. Despite high variability among sites, survival of transplanted crayweed is typically comparable to natural mortality (Campbell et al., 2014) and, as of 2019, transplanted crayweed has reproduced in six locations such that multiple generations are now identifiable, often hundreds of meters from the original restored patches. These restored crayweed forests have become self-sustaining without the need for additional cost or maintenance, which is a rare result in marine restoration. This relatively small-scale intervention has translated into a large-scale impact/benefit, with crayweed populations continuing to expand and colonize substantial areas, and beginning to function as natural forests (Marzinelli et al., 2016).
Additional work has employed aspects of active restoration and assisted recovery to improve understanding of kelp forests and ecological restoration. Work by Valentine and Johnson (2005) found that even after the removal of urchins, heavy inoculation with E. radiata kelp spores was insufficient to achieve kelp reestablishment – presumably due to recruitment inhibition by the turf algae and sediments that had proliferated in the absence of the kelp (also see Layton et al., 2019b). However, Gorman and Connell (2009) showed that recovery of E. radiata kelp can naturally occur where turf algae are removed. Others have illustrated that urchin removal can facilitate natural recovery of kelp and other macroalgae on Australian temperate reefs when healthy kelp forests are nearby the denuded areas (Fletcher, 1987; Ling, 2008). Layton et al. (2019a) demonstrated successful transplanting of >500 adult E. radiata on artificial reefs in Tasmania. Survivorship of transplants was comparable to natural reefs, and abundant recruitment of juveniles (>750) ensured that many patches became self-sustaining. Crucially however, it was only patches above a certain size and density of adult kelp that facilitated adequate recruitment to maintain the kelp canopy; illustrating the importance of minimum patch sizes and densities when transplanting E. radiata, and likely other kelp species.
Given the rate of environmental change that is influencing coastlines worldwide, and that some drivers of kelp decline cannot be easily ameliorated (e.g. ocean warming), there is growing recognition of the need to plan adaptively and to “future-proof” marine restoration efforts, and potentially even consider the restoration of novel, more-suitable, species (van Oppen et al., 2015; Coleman and Goold, 2019; Wood et al., 2019). While we are aware of no published work implementing these strategies for kelp restoration, research in Australia is currently pioneering the identification of warm water-tolerant seaweed genotypes as the basis of future restoration efforts (IMAS, 2019; Gurgel et al., 2020).
Estimation of the Costs of Restoration
Estimating the costs of implementing effective kelp forest restoration in Australia is difficult given the few examples to date. For Operation Crayweed, workers initially transplanted six 2 m2 patches of P. comosa at each restoration site, with adult kelp densities of 15 m–2. Initial transplanting efforts required ∼5 days at each site and included site marking/preparation, securing of mesh mats for crayweed attachment, collection of adult crayweed from the donor population, and the transplanting itself. Costs of these efforts are estimated at ∼US$6,850 per restoration site (i.e. ∼$570 m–2), which cover a 4-person team, boat and tow-vehicle, SCUBA tank fills, basic equipment and consumables. Project management and monitoring of the multiple Operation Crayweed sites is estimated at an additional ∼US$18,500 p.a. Note that these costs do not include the science necessary to underpin decisions such as choice of donor site, size of patch, etc. Active restoration efforts typically occur at small to medium scales, and not the seascape scale at which kelp forest loss can occur. And so, while Operation Crayweed has demonstrated the translation of small-scale efforts into large-scale outcomes, efficient up-scaling of active restoration remains as a key ongoing consideration.
Assisted recovery techniques such as urchin culling are typically suited to tactical interventions at local spatial scales, such as reducing kelp loss to maintain/bolster the resilience of existing forests (Ling and Johnson, 2012; Layton et al., 2019a), remove incipient barrens (Ling, 2008; Tracey et al., 2015), or support active restoration efforts (Sanderson, 2003). Economic projections indicate that culling of C. rodgersii urchins from densities of 1.5 urchins/m2 to 0.1 urchins/m2 (i.e. the maximum density estimated to allow kelp recovery) across a 1 km2 area of reef and from depths of 0–20 m would take two divers 685 days and cost ∼US$980,478 or US$1,431 day–1 (Tracey et al., 2014). These projections are nonetheless conservative given that urchin densities on barrens are typically closer to 2 urchins/m2 (Ling, 2008; Ling and Johnson, 2012). Novel technology is promising to improve the scalability and cost-effectiveness of urchin culling, and trials of autonomous underwater vehicles designed to locate and kill urchins are in planning. Other alternatives to enhance the value or reduce the costs of urchin-culling operations may involve working in partnership with fisheries/aquaculture industries (Pert et al., 2018) or enlisting the help of citizen scientists, as has been done in Japan (Watanuki et al., 2010) and the United States (House et al., 2018).
Overall, the impetus to consider kelp forest restoration would benefit greatly from environmental accounting to ascertain the (currently unknown) value of kelp forests to human society, and which could underpin rigorous benefit-cost analysis (e.g. Rogers et al., 2018). This is especially since the costs of restoration operations can likely be reduced by minimizing diver labor and increasing automation/efficacy of mass seeding techniques (e.g. mass dispersal of lab-cultured kelp propagules from boat-mounted pumps; North, 1976).
Kelp Forest Restoration: A Workflow
The loss of kelp forests in Australia, and indeed the world, is complex due to high levels of geographic variation and the multitude of different stressors present in any given location. Accordingly, it is useful to apply a workflow and decision framework when approaching restoration (Figure 3), especially where this can also provide an indicator of potential local outcomes. Development and preliminary-testing of this workflow utilized examples of kelp loss from Tasmania, where local restoration interventions must consider two species of dominant kelp (i.e. Ecklonia radiata and Macrocystis pyrifera) and multiple drivers of kelp forest decline (e.g. ocean-warming, overgrazing by urchins).
Our novel workflow and decision support system illustrates multiple alternative pathways and endpoints of restoration, and critically, helps to identify circumstances where restoration is not possible or advisable (also see Johnson et al., 2017). This diversity of pathways and endpoints exists because of environmental factors that are beyond the control of practitioners, such as whether hysteresis is present in the system (e.g. Gorman and Connell, 2009; Johnson et al., 2017). The multiple pathways of kelp forest restoration are also reflective of the diversity of drivers of decline, variability in the resources available for restoration efforts, and scalability of the intervention. For example, the efficacy of assisted recovery techniques is often hindered by resource constraints (e.g. Tracey et al., 2015), while active restoration efforts can be reliant on ongoing transplantation of kelp, which has limited scalability.
The workflow highlights that at each node, research is needed to inform decision making and progression to the next stage, and thus may also help identify knowledge gaps in baseline ecosystem knowledge. Using this workflow to plan restoration efforts should also help practitioners and managers to ensure that interventions are considered within resource constraints and that the driver(s) of kelp forest decline has been addressed.
Progressing through the workflow toward the point of successful kelp restoration incorporates several key questions as decision points. Firstly, is it possible to return the environment to its pre-loss state, such as the improvements in water quality that preceded Operation Crayweed? If not, it will be essential to select and facilitate kelp to survive in the new environmental state, for example, the selection for thermally tolerant kelp from remaining healthy individuals (see IMAS 2019). However, if it is not possible to ameliorate or adapt to the novel ecosystem state, restoration efforts will be, at best, limited to the local scale (Figure 3). Secondly, is there hysteresis present in the dynamics of the system? Knowledge of the capacity for hysteresis is critical since it can prove one of the biggest challenges to kelp forest restoration (see Gorman and Connell, 2009; Marzloff et al., 2011; Johnson et al., 2017). Lastly, for successful restoration to occur at scale, efforts to overcome hysteresis and/or provide a novel source of propagules must also be scalable, and ideally commensurate with the scale of the initial degradation (Steinberg et al., 2016; Johnson et al., 2017; Wood et al., 2019).
Overall, ensuring natural recruitment of juvenile kelp and the continuation of self-sustaining generations is critical to long-term restoration success. Thus, it is important that kelp forest restoration focus on restoring the positive feedbacks that initiate recruitment and facilitation cascades and which promote ecosystem stability (Halpern et al., 2007; Layton et al., 2019a). To this end, restoration actions should also be considered as tools to bolster resilience in existing and/or partially degraded kelp forests where smaller interventions may be adequate to conserve/restore these feedbacks.
Measuring the Success of Kelp Forest Restoration
Since kelp are foundation species that support diverse ecological communities, comparison of community structure between restored and natural “reference ecosystems” can provide comparative indicators of restoration success at the community-level. However, in some instances reference ecosystems may not be identifiable or available, for example, when there are shifting baselines and poor understanding of ecosystem dynamics (Dayton et al., 1998; Johnson et al., 2017), or where ecosystem loss occurs prior to collection of adequate data (e.g. many Australian shellfish reefs, McLeod et al., 2018). Likewise, reference ecosystems may not be sensible targets for restoration when current/predicted environmental parameters (e.g. water temperature) are different to the historical state or community (Perring et al., 2015; Wood et al., 2019).
These diverse circumstances are acknowledged by the Society for Ecological Restoration (SER) who recognize that a reference ecosystem may instead be a conceptual model synthesized from numerous locations, indicators, and historical and predictive records (McDonald et al., 2016). SER have developed International Standards for the practice of ecological restoration, central to which is the “5-star recovery system” (see McDonald et al., 2016). This tool provides a consistent set of criteria against which key ecosystem attributes can be assessed, and acts as a conceptual framework for restoration practitioners, managers, and regulators. The 5-star system also provides a framework for habitat-specific indicators and metrics to be developed. Such indicators for kelp forest restoration might include transplant survival, growth rates, and condition (e.g. fouling, bleaching, and photosynthetic efficiency), genetic diversity, and recruitment. Certainly, recruitment of juvenile kelp is one of the greatest indicators of ongoing success and kelp forest resilience. In most cases, the ideal goal, as demonstrated by Operation Crayweed, is kelp forest recovery and reestablishment beyond the restoration footprint due to spill-over of natural recruitment.
Operation Crayweed – A Case Study of the Ser 5-Star Recovery System
Operation Crayweed has been the most successful kelp restoration program in Australia to date. It has evolved from pilot restoration attempts in 2011 (2 sites) through to restoration at the scale of loss in 2018 (11 sites across ∼70 km) with further expansion ongoing. It provides an ideal opportunity to apply the 5-star recovery system to a kelp restoration project, especially since science was used to rigorously design and test restoration approaches, thus allowing objective assessment of some recovery attributes. While the recovery wheel is site, scale, and temporally specific, here we apply it to the overall Operation Crayweed initiative (∼9 years following initial restoration began) (Figure 4).
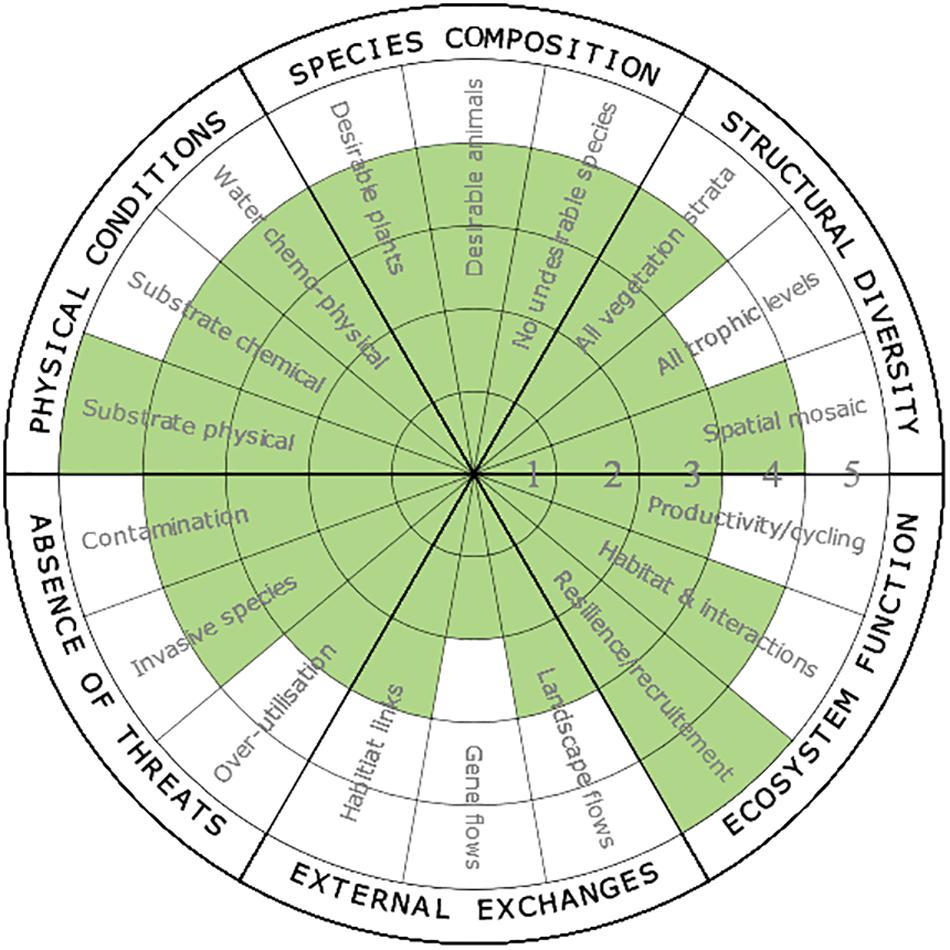
Figure 4. Case study: Assessment of ecosystem recovery by Operation Crayweed using the SER 5-star recovery wheel. Average overall score is 3.7. Conducted by Operation Crayweed researchers (MAC, EMM, PDS, and AV). Individual attribute scores can range from 1 (e.g. biogenic structure restored, and ongoing deterioration prevented, but limited recovery of ecosystem attributes) through to 5 (e.g. restoration has re-established biota and functions that can continue a recovery trajectory without active interventions) (also see McDonald et al., 2016). Ratings are based on both monitoring and scientific publications, as well as expert opinion of the scientists and practitioners involved in restoration.
First, we know that some key aspects of ecosystem function (i.e. recruitment) in restored patches have reached levels found in natural areas (Campbell et al., 2014), warranting a 5-star rating for these attributes. Moreover, species composition of some associated organisms in restored crayweed forests is approaching that of natural forests (Marzinelli et al., 2016) warranting a rating of 4 for “habitat and interactions,” Additionally, ongoing monitoring illustrates that the structural diversity of restored crayweed populations are approaching natural levels (Marzinelli et al., 2016; Steinberg et al., 2016), warranting ratings of 3–4. Whilst the physical conditions that likely precipitated the initial decline (i.e. poor water quality, Campbell et al., 2014) have dramatically improved (following improvements to wastewater infrastructure), given the highly urbanized nature of the restoration locations in metropolitan Sydney there are likely to be ongoing threats from contamination; and therefore warrant a rating of 4 for “absence of threats” (e.g. invasive species and contamination) and “physical conditions” (e.g. water chemo-physical). Moreover, these external attributes cannot be controlled within the restoration framework, but are managed and regulated by government.
Targets for other ecosystem attributes are less well defined, have not been measured, or cannot be measured within the temporal scale defined here (due to prolonged response times). Thus, the recovery rating for some attributes is subjective and based on expert opinion of the Operation Crayweed team (MAC, EMM, PDS, and AV). For example, it is premature to assess “external exchanges” (e.g. gene flow) between restored and pre-existing crayweed populations because insufficient time has passed to restore those connections. The collective scientific knowledge of these systems suggests however that restoration of external connections is occurring, since crayweed cover and extent is expanding at some sites without additional intervention (hence the 2–3 star rating). While recovery across multiple trophic levels is being monitored, it is possible that recovery of higher trophic levels may take decades (e.g. Babcock et al., 2010; Reed et al., 2017), especially for species with limited dispersal (e.g. abalone; Marzinelli et al., 2014). The long timescales over which some attributes respond to restoration may therefore be reflected as a low rating, but can still be indicative of an appropriate trajectory of recovery.
The Society for Ecological Restoration considers the mean attribute score as an appropriate measure of a project’s progress along a trajectory of recovery. However, depending on project and ecosystem-specific requirements, certain criteria could be weighted to provide a more subtle assessment. For Operation Crayweed, the mean attribute score was 3.7, indicating that restoration of crayweed forests at the local of the initial loss is well under way, with high levels of recruitment and good progress toward development of associated communities and ecosystem functions that are on a self-sustaining trajectory. While the mean score and individual recovery attributes could be used as a tool to identify areas requiring ongoing active restoration to accelerate recovery, we believe that it is sufficient to restore populations to a point whereby they can continue on a recovery trajectory naturally, without additional intervention. This is not only cost effective but ensures that limited resources can be strategically directed to maximize restoration efforts across species and ecosystems in need. Regardless, recovery of restored crayweed populations will continue to be measured and assessment of the need for additional interventions will be re-examined on an ongoing basis. The SER recovery system provides assessment of the trajectory of ecosystem recovery but also identifies research gaps. For Operation Crayweed, areas that would benefit from future research include assessing whether crayweed restoration has resulted in recovery of broader ecosystem functions and services (e.g. productivity and recreational fishing) and whether there is increased connectivity among adjacent non-kelp forest habitats (e.g. seagrass).
Conclusion
While kelp forest restoration is not achievable or feasible in all situations, use of a decision framework and consideration of the initial drivers of decline should increase the likelihood of success and the appropriate use of resources. And while past examples of kelp forest restoration are as notable for the failures as the successes, it seems that under many circumstances, small to medium scale restoration is achievable. For example, Operation Crayweed has demonstrated positive ecological outcomes at a scale beyond their initial restoration intervention. However, increasing the scalability of kelp forest restoration to the seascape-scale remains a considerable challenge, as does restoration in response to climate change where drivers of decline cannot be readily ameliorated (e.g. ocean warming). Optimal results will be achieved via thorough planning of restoration interventions and where positive feedbacks in the dynamics of kelp forests can be harnessed to promote habitat resilience and recruitment of juvenile kelp.
It follows however that the challenges and costs inherent in restoring kelp forests ecosystems places a great emphasis on the importance of maintaining and conserving kelp habitats. The difficulty of restoring heavily degraded environments – such as expansive urchin barrens and turf algae habitats – reinforces the notion that “an ounce of prevention is worth more than a ton of cure.” Ultimately, the management and conservation of Australian kelp forests should adopt policies that facilitate early warning and intervention for kelp environments under threat, with the aim to maintain and restore resilience in these critically important habitats.
Author Contributions
CL and CJ conceived the initial idea for the review. CL prepared the manuscript with input from all other authors. All authors contributed to manuscript revision.
Conflict of Interest
The authors declare that the research was conducted in the absence of any commercial or financial relationships that could be construed as a potential conflict of interest.
Acknowledgments
We thank the Australian Government’s National Environmental Science Program Marine Biodiversity Hub for funding and coordinating Project E5 – The role of restoration in conserving matters of national environmental significance where this review was conceived, and project-leader Ian McLeod for encouraging publication of this work. We also thank our colleagues and the workers who contributed the research that forms the basis of this review, and the Australian Research Council, state governments, universities, and private foundations and donors who supported the research described here.
Footnotes
- ^ Kelp are often defined as large, brown macroalgae belonging to the Order Laminariales, however this is a non-taxonomic term. Some authors (e.g. Steneck and Johnson, 2014; Bennett et al., 2016; Wernberg et al., 2019) argue for a broader functional definition that includes other large, brown habitat-forming macroalgae such as those from the Order Fucales. Here we adopt this broader functional definition, especially in light of the significant contribution of fucalean macroalgae to coastal ecosystems in Australia (Table 1; Coleman and Wernberg, 2017).
References
ABARES (2019). Agricultural commodities: March 2019, Annual Fisheries Outlook. Canberra: Australian Government Department of Agriculture and Water Resources.
Ambrose, R. F. (1994). Mitigating the effects of a coastal power plant on a kelp forest community: rational and requirements for an artificial reef. Bulletin of Marine Science 55, 694–708.
Andrew, N. L. (1993). Spatial heterogeneity, sea urchin grazing, and habitat structure on reefs in temperate Australia. Ecology 74, 292–302. doi: 10.2307/1939293
Andrew, N. L., and O’Neill, A. L. (2000). Large-scale patterns in habitat structure on subtidal rocky reefs in New South Wales. Marine and Freshwater Research 51, 255–263.
Babcock, R. C., Shears, N. T., Alcala, A. C., Barrett, N. S., Edgar, G. J., Lafferty, K. D., et al. (2010). Decadal trends in marine reserves reveal differential rates of change in direct and indirect effects. PNAS 107, 18256–18261. doi: 10.1073/pnas.0908012107
BenDor, T., Lester, T. W., Livengood, A., Davis, A., and Yonavjak, L. (2015). Estimating the size and impact of the ecological restoration economy. PLoS ONE 10:e0128339. doi: 10.1371/journal.pone.0128339
Bennett, S., Wernberg, T., Connell, S. D., Hobday, A. J., Johnson, C. R., and Poloczanska, E. S. (2016). The ‘Great Southern Reef’: social, ecological and economic value of Australia’s neglected kelp forests. Marine and Freshwater Research 67, 47–56.
Bishop, M. J., Coleman, M. A., and Kelaher, B. P. (2010). Cross-habitat impacts of species decline: response of estaurine sediment communities to changing detrital resources. Oecologia 163, 517–525. doi: 10.1007/s00442-009-1555-y
Buschmann, A. H., Camus, C., Infante, J., Neori, A., Israel, A., Hernández-González, M. C., et al. (2017). Seaweed production: overview of the global state of exploitation, farming and emerging research activity. European Journal of Phycology 52, 391–406. doi: 10.1080/09670262.2017.1365175
Bustamente, R. H., Branch, G. M., and Eekhout, S. (1995). Maintenance of an exceptional intertidal grazer biomass in South Africa: subsidy by subtidal kelps. Ecology 76, 2314–2329. doi: 10.2307/1941704
Campbell, A. H., Marzinelli, E. M., Vergés, A., Coleman, M. A., and Steinberg, P. D. (2014). Towards restoration of missing underwater forests. PLoS ONE 9:e84106. doi: 10.1371/journal.pone.0084106
Carnell, P. E., and Keough, M. J. (2019). Reconstructing historical marine populations reveals major decline of a kelp forest ecosystem in Australia. Estuaries and Coasts. 42, 765–778. doi: 10.1007/s12237-019-00525-1
Carter, J. W., Carpenter, A. L., Foster, M. S., and Jessee, W. N. (1985). Benthic succession on an artificial reef designed to support a kelp-reef community. Bulletin of Marine Science 37, 86–113.
Coleman, M. A., Cetina-Heredia, P., Roughan, M., Feng, M., Van Sebille, E., and Kelaher, B. P. (2017). Anticipating changes to future connectivity within a network of marine protected areas. Global Change Biology 23, 3533–3542. doi: 10.1111/gcb.13634
Coleman, M. A., and Goold, H. D. (2019). Harnessing synthetic biology for kelp forest conservation. Journal of Phycology 55, 745–751. doi: 10.1111/jpy.1288
Coleman, M. A., Kelaher, B. P., Steinberg, P. D., and Millar, A. J. K. (2008). Absence of a large brown macroalga on urbanized rocky reefs around Sydney. Australia, and evidence for historical decline. Journal of Phycology 44, 897–901. doi: 10.1111/j.1529-8817.2008.00541
Coleman, M. A., and Wernberg, T. (2017). Forgotten underwater forests: The key role of fucoids on Australia temperate reefs. Ecology and Evolution 7, 8406–8418. doi: 10.1002/ece3.3279
Connell, S. D., Russell, B. D., Turner, D. J., Shepherd, S. A., Kildea, T., Miller, D., et al. (2008). Recovering a lost baseline: missing kelp forests from a metropolitan coast. Marine Ecology Progress Series 360, 63–72. doi: 10.3354/meps07526
Dayton, P. K., Tegner, M. J., Edwards, P. B., and Riser, K. L. (1998). Sliding baselines, ghosts, and reduced expectations in kelp forest communities. Ecological Applications 8, 309–322. doi: 10.1890/1051-0761(1998)008[0309:sbgare]2.0.co;2
Devinny, J. S., and Leventhal, J. (1979). New methods for mass culture of Macrocystis pyrifera sporophytes. Aquaculture 17, 241–250. doi: 10.1016/0044-8486(79)90127-3
Edgar, G. J. (2008). Australian Marine Life: The Plants of Animals of Temperate Waters. Sydney: New Holland Publishers.
Evans, K., Bax, N., and Smith, D. C. (2017). Australia state of the environment 2016: marine environment. Canberra: Australian Government Department of the Environment and Energy.
Filbee-Dexter, K., Wernberg, T., Norderhaug, K. M., Ramirez-Llodra, E., and Pedersén, M. F. (2018). Movement of pulsed resource subsidies from kelp forests to deep fjords. Oecologia 187, 291–304. doi: 10.1007/s00442-018-4121-7
Fletcher, W. J. (1987). Interactions among subtidal Australian sea urchins, gastropods, and algae: effects of experimental removals. Ecological Monographs 57, 89–109. doi: 10.2307/1942640
Froehlich, H. E., Afflerbach, J. C., Frazier, M., and Halpern, B. S. (2019). Blue growth potential to mitigate climate change through seaweed offsetting. Current Biology 29, 3087.e–3093.e. doi: 10.1016/j.cub.2019.07.041
Gaylord, B., Rosman, J. H., Reed, D. C., Koseff, J. R., Fram, J., MacIntyre, S., et al. (2007). Spatial patterns of flow and their modification within and around a giant kelp forest. Limnology and Oceanography 52, 1828–1852. doi: 10.4319/lo.2007.52.5.1838
Gorman, D., and Connell, S. D. (2009). Recovering subtidal forests in human-dominated landscapes. Journal of Applied Ecology 46, 1258–1265. doi: 10.1111/j.1365-2664.2009.01711.x
Gurgel, C. F. D., Camacho, O., Minne, A. J. P., Wernberg, T., and Coleman, M. A. (2020). Marine heatwaves drives cryptic loss of genetic diversity in underwater forests. Current Biology accepted
Hadley, S., Wild-Allen, K., Johnson, C., and Macleod, C. (2018). Investigation of broad scale implementation of integrated multitrophic aquaculture using a 3D model of an estuary. Marine Pollution Bulletin 133, 448–459. doi: 10.1016/j.marpolbul.2018.05.045
Halpern, B. S., Silliman, B. R., Olden, J. D., Bruno, J. P., and Bertness, M. D. (2007). Incorporating positive interactions in aquatic restoration and conservation. Frontiers in Ecology and the Environment 5:153–160. doi: 10.1890/1540-9295(2007)5[153:ipiiar]2.0.co;2
Harrold, C., Light, K., and Lisin, S. (1998). Organic enrichment of submarine-canyon and continental-shelf benthic communities by macroalgal drift imported from nearshore kelp forests. Limnology and Oceanography 43, 669–678. doi: 10.4319/lo.1998.43.4.0669
Hernández-Carmona, G., García, O., Robledo, D., and Foster, M. S. (2000). Restoration techniques for Macrocystis pyrifera (Phaeophyceae) populations at the southern limit of their distribution in Mexico. Botanica Marina 43, 273–284.
Hobday, A. J., and Pecl, G. T. (2014). Identification of global marine hotspots: sentinels for change and vanguards for adaptation action. Reviews in Fish Biology and Fishers 24, 415–425. doi: 10.1007/s11160-013-9326-6
House, P., Barilotti, A., Burdick, H., Ford, T., Williams, J., Williams, C., et al. (2018). Palo Verdes kelp forest restoration project, year 5: July 2017–June 2018. Los Angeles CA: The Bay Foundation.
Huisman, J. M. (2000). Marine Plants of Australia. Nedlands, WA. University of Western Australia Press.
IMAS (2019). Assessing the Potential for Restoration and Permaculture of Tasmania’s Giant Kelp Forests. Avaliable at: http://imas.utas.edu.au/kelprestoration. (accessed September 2019).
IPCC (2014). “Climate Change 2014: Impacts, Adaptation, and Vulnerability. Part B: Regional Aspects,” in Contribution of Working Group II to the Fifth Assessment Report of the Intergovernmental Panel on Climate Change, eds V. R. Barros, C. B. Field, D. J. Dokken, M. D. Mastrandea, K. J. Mach, T. E. Bilir, et al. (Cambridge: Cambridge University Press).
Johnson, C. R., Banks, S. C., Barrett, N. S., Cazassus, F., Dunstan, P. K., Edgar, G. J., et al. (2011). Climate change cascades: shifts in oceanography, species’ ranges and subtidal marine community dynamics in eastern Tasmania. Journal of Experimental Marine Biology and Ecology 400, 17–32. doi: 10.1016/j.jembe.2011.02.032
Johnson, C. R., Chabot, R. H., Marzloff, M. P., and Wotherspoon, S. (2017). Knowing when (not) to attempt ecological restoration. Restoration Ecology 24, 140–147. doi: 10.1111/rec.12413
Johnson, C. R., Ling, S. D., Ross, D. J., Shepard, S. A., and Miller, K. (2005). Establishment of the long-spined sea urchin (Centrostephanus rodgersii) in Tasmania: First assessment of potential threats to fisheries. Hobart: University of Tasmania.
Kerswell, A. (2006). Global biodiversity patterns of benthic marine algae. Ecology 87, 2479–2488. doi: 10.1890/0012-9658(2006)87[2479:gbpobm]2.0.co;2
Konar, B., and Estes, J. A. (2003). The stability of boundary regions between kelp beds and deforested areas. Ecology 84, 174–185. doi: 10.1890/0012-9658(2003)084[0174:tsobrb]2.0.co;2
Krause-Jensen, D., and Duarte, C. M. (2016). Substantial role of macroalgae in marine carbon sequestration. Nature Geoscience 9, 737–742. doi: 10.1038/NGEO2790
Kriegisch, N., Reeves, S., Johnson, C. R., and Ling, S. D. (2016). Phase-shift dynamics of sea urchin overgrazing on nutrified reefs. PLoS ONE 11:e0168333. doi: 10.1371/journal.pone.0168333
Krumhansl, K. A., Okamoto, D. K., Rassweiler, A., Novak, M., Bolton, J. J., Cavanaugh, K. C., et al. (2016). Global patterns of kelp forest change over the past half-century. PNAS 113, 13785–13790.
Layton, C., Cameron, M. J., Shelamoff, V., Fernández, P. A., Britton, D., Hurd, C. L., et al. (2019b). Chemical microenvironments within macroalgal assemblages: implications for the inhibition of kelp recruitment by turf algae. Limnol. Oceanogr. 9999, 1–14. doi: 10.1002/lno.11138
Layton, C., Shelamoff, V., Cameron, M. J., Tatsumi, M., Wright, J. T., and Johnson, C. R. (2019a). Resilience and stability of kelp forests: The importance of patch dynamics and environment-engineer feedbacks. PLoS ONE 14:e0210220. doi: 10.1371/journal.pone.0210220
Ling, S. D. (2008). Range expansion of a habitat-modifying species leads to loss of taxonomic diversity: a new and impoverished reef state. Oecologia 156, 883–894. doi: 10.1007/s00442-008-1043-9
Ling, S. D., and Johnson, C. R. (2012). Marine reserves reduce risk of climate-driven phase shift by reinstating size- and habitat-specific trophic interactions. Ecological Applications 22, 1232–1245. doi: 10.1890/11-1587.1
Ling, S. D., Johnson, C. R., Frusher, S. D., and Ridgway, K. R. (2009). Overfishing reduces resilience of kelp beds to climate-driven catastrophic phase shift. PNAS 106, 22341–22345. doi: 10.1073/pnas.0907529106
Ling, S. D., and Keane, J. P. (2018). “Resurvey of the longspined sea urchin (Centrostephanus rodgersii) and associated barren reef in Tasmania,” in Institute for Marine and Antarctic Studies, (Hobart: University of Tasmania).
Løvås, S. M., and Tørum, A. (2001). Effect of the kelp Laminaria hyperborea upon sand dune erosion and water particle velocity. Coastal Engineering 44, 37–63. doi: 10.1016/S0378-3839(01)00021-7
Mabin, C. J. T., Gribben, P. E., Fischer, A., and Wright, J. T. (2013). Variation in the morphology, reproduction and development of the habitat-forming kelp Ecklonia radiata with changing temperature and nutrients. Marine Ecology Progress Series 483, 117–131. doi: 10.3354/meps10261
Macreadie, P. I, Anton, A., Raven, J. A., Beaumont, N., Connolly, R. M., Friess, D. A., et al. (2019). The future of Blue Carbon science. Nature Communications 10, 3998. doi: 10.1038/s41467-019-11693-w
Martínez, B., Radford, B., Thomsen, M. S., Connell, S. D., Carreño, F., Bradshaw, C. J. A., et al. (2018). Distribution models predict large contractions of habitat-forming seaweeds in response to ocean warming. Biodiversity Research 24, 1350–1366. doi: 10.1111/ddi.12767
Marzinelli, E. M., Campbell, A. H., Vergés, A., Coleman, M. A., Kelaher, B. P., and Steinberg, P. D. (2014). Restoring seaweeds: does the declining fucoid Phyllospora comosa support different biodiversity than other habitats? Journal of Applied Phycology 26, 1089–1096. doi: 10.1007/s10811-013-0158-5
Marzinelli, E. M., Leong, M. R., Campbell, A. H., Steinberg, P. D., and Vergés, A. (2016). Does restoration of a habitat-forming seaweed restore associated faunal diversity? Restoration Ecology 24, 81–90. doi: 10.1111/rec.12292
Marzinelli, E. M., Williams, S. B., Babcock, R. C., Barrett, N. S., Johnson, C. R., Jordan, A., et al. (2015). Large-scale geographic variation in distribution and abundance of Australian deep-water kelp forests. PLoS ONE 10:e0118390. doi: 10.1371/journal.pone.0118390
Marzloff, M. P., Dambacher, J. M., Johnson, C. R., Little, L. R., and Frusher, S. D. (2011). Exploring alternative states in ecological systems with a qualitative analysis of community feedback. Ecological Modelling 222, 2651–2662. doi: 10.1016/j.ecolmodel.2011.03.040
McDonald, T., Gann, G. D., Jonson, J., and Dixon, K. W. (2016). International standards for the practice of ecological restoration – including principles and key concepts. Washington, DC: Society for Ecological Restoration.
McLeod, I., Schmider, J., Creighton, C., and Gillies, C. (2018). Seven pearls of wisdom: Advice from Traditional Owners to improve engagement of local Indigenous people in shellfish ecosystem restoration. Ecological Management & Restoration 19, 98–101. doi: 10.1111/emr.12318
Miller, R. J., Lafferty, K. D., Lamy, T., Kui, L., Rassweiler, A., and Reed, D. C. (2018). Giant kelp. Macrocystis pyrifera, increases faunal diversity through physical engineering. Proc. R. Soc. B 285, 3998. doi: 10.1098/rspb.2017.2571
North, W. J. (1976). Aquacultural techniques for creating and restoring beds of giant kelp. Macrocystis spp. Journal of the Fisheries Research Board of Canada 33, 1015–1023. doi: 10.1139/f76-129
Perkol-Finkel, S., Ferrario, F., Nicotera, V., and Airoldi, L. (2012). Conservation challenges in urban seascapes: promoting the growth of threatened species on coastal infrastructures. Journal of Applied Ecology 49, 1457–1466. doi: 10.1111/j.1365-2664.2012.02204.x
Perring, M. P., Standish, R. J., Price, J. N., Craig, M. D., Erickson, T. E., Ruthrof, K. X., et al. (2015). Advances in restoration ecology: rising to the challenge of the coming decades. Ecosphere 6, 1–25. doi: 10.1890/ES15-00121.1
Pert, C. G., Swearer, S. E., Dworjanyn, S. A., Kriegisch, N., Turchini, G. M., Francis, D. S., et al. (2018). Barrens of gold: gonad conditioning of an overabundant sea urchin. Aquaculture Environment Interactions 10, 345–361. doi: 10.3354/aei00274
Phillips, J. A. (2001). Marine macroalgae diversity hotspots: why is there high species richness and endemism in southern Australian marine benthic flora? Biodiversity and Conservation 10, 1555–1577.
Phillips, J. A., and Blackshaw, J. K. (2011). Extirpation of macroalgae (Sargassum spp.) on the subtropical east Australian coast. Conservation Biology 25, 913–921. doi: 10.1111/j.1523-1739.2011.01727.x
Queirós, A. M., Stephens, N., Widdicombe, S., Tait, K., McCoy, S. J., Ingels, J., et al. (2019). Connected macroalgal−sediment systems: blue carbon and food webs in the deep coastal ocean. Ecological Monographs 89, e01366. doi: 10.1002/ecm.1366
Reed, D. C., Schroeter, S. C., and Huang, D. (2006). An experimental investigation of the use of artificial reefs to mitigate the loss of giant kelp forest habitat”. San Diego, CA: University of California.
Reed, D. C., Schroeter, S. C., and Page, M. (2017). Annual Report of the Status of Condition C: Kelp Reef Mitigation. San Diego, CA: University of California.
Reeves, S. E., Kriegisch, N., Johnson, C. R., and Ling, S. D. (2018). Reduced resistance to sediment-trapping turfs with decline of native kelp and establishment of an exotic kelp. Oecologia 188, 1239–1251. doi: 10.1007/s00442-018-4275-3
Rogers, A. A., Gillies, C., Hancock, B., McLeod, I., Nedosyko, A., Reeves, S., et al. (2018). “Benefit-cost analysis for marine habitat restoration: a framework for estimating the viability of shellfish reef repair projects,” in Report to the National Environmental Science Programme, Marine Biodiversity Hub, (Perth: The University of Western Australia).
Sanderson, C. (2003). “Restoration of string kelp (Macrocystis pyrifera) habitats on Tasmania’s east and south coasts,” in Final Report to Natural Heritage Trust for Seacare, (Tasmania).
Scheffer, M., Carpenter, S., Foley, J. A., Folke, C., and Walker, B. (2001). Catastrophic shifts in ecosystems. Nature 413, 591–596. doi: 10.1038/35098000
Schiel, D. R., and Foster, M. S. (2015). The biology and ecology of giant kelp forests. Oakland, California: University of California Press.
Shelamoff, V., Layton, C., Tatsumi, M., Cameron, M. J., Wright, J. T., and Johnson, C. R. (2019). Ecosystem engineering by a canopy-forming kelp facilitates the recruitment of native oysters. Restoration Ecology 27, 1442–1451. doi: 10.1111/rec.13019
Smale, D. A., Burrows, M. T., Moore, P., O’Connor, N., and Hawkins, S. J. (2013). Threats and knowledge gaps for ecosystem services provided by kelp forests: a northeast Atlantic perspective. Ecology and Evolution 3, 4016–4038. doi: 10.1002/ece3.774
Smale, D. A., and Wernberg, T. (2013). Extreme climactic event drives range contraction of a habitat-forming species. Proc R Soc B 280, 20122829. doi: 10.1098/rspb.2012.2829
South, P. M., Floerl, O., Forrest, B. M., and Thomsen, M. S. (2017). A review of three decades of research on the invasive kelp Undaria pinnatifida in Australasia: An assessment of its success, impacts, and status and one of the world’s worst invaders. Marine Environmental Research 131, 243–257. doi: 10.1016/j.marenvres.2017.09.015
Steinberg, P. D., Marzinelli, E. M., Vergés, A., Campbell, A., and Coleman, M. A. (2016). Restoration of the Sydney’s missing underwater forests. Australian Research Council Linkage grant (LP160100836).
Steneck, R. S., and Johnson, C. R. (2014). “Kelp forests: dynamic patterns, processes, and feedbacks,” in Marine Community Ecology and Conservation, eds M. D. Bertness, J. F. Bruno, B. R. Silliman, and J. J. Stachowicz, (Massachusetts: Sinauer Associates, Inc.).
Teagle, H., Hawkins, S. J., Moore, P. J., and Smale, D. A. (2017). The role of kelp species as biogenic habitat formers in coastal ecosystems. Journal of Experimental Marine Biology and Ecology 492, 81–98. doi: 10.1016/j.jembe.2017.01.017
Terawaki, T., Hasegawa, H., Arai, S., and Ohno, M. (2001). Management-free techniques for restoration of Eisenia and Ecklonia beds along the central Pacific coast of Japan. Journal of Applied Phycology 13, 13–17.
Thompson, P. A., Bonham, P., Waite, A. M., Clementson, L. A., Cherukuru, N., Hassler, C., et al. (2011). Contrasting oceanographic conditions and phytoplankton communities on the east and west coasts of Australia. Deep-Sea Research II 58, 645–663. doi: 10.1016/j.dsr2.2010.10.003
Thurstan, R. H., Brittain, Z., Jones, D. S., Cameron, E., Dearnaley, J., and Bellgrove, A. (2018). Aboriginal uses of seaweeds in temperate Australia: an archival assessment. Journal of Applied Phycology 30, 1821–1832. doi: 10.1007/s10811-017-1384-z
Tracey, S. R., Baulch, T., Hartmann, K., Ling, S. D., Lucieer, V., Marzloff, M. P., et al. (2015). Systematic culling controls a climate driven, habitat modifying invader. Biological Invasions 17, 1885–1896. doi: 10.1007/s10530-015-0845-z
Tracey, S. R., Mundy, C., Baulch, T., Marzloff, M. P., Hartmann, K., Ling, S. D., et al. (2014). in Trial of an industry implemented, spatially discrete eradication/control program for Centrostephanus rodgersii in Tasmania, ed. F. R. A. D. Council, (Tasmania: Institute for Marine and Antarctic Studies). doi: 10.1007/s10530-015-0845-z
Valentine, J. F., and Johnson, C. R. (2003). Establishment of the introduced kelp Undaria pinnatifida in Tasmania depends on disturbance to native algal assemblages. Journal of Experimental Marine Biology and Ecology 295, 63–90. doi: 10.1016/S0022-0981(03)00272-7
Valentine, J. P., and Johnson, C. R. (2005). Persistence of sea urchin (Heliocidaris erythrogramma) barrens on the east coast of Tasmania: inhibition of macroalgal recovery in the absence of high densities of sea urchins. Botanica Marina 48, 106–115. doi: 10.1515/bot.2005.025
van Oppen, M. J. H., Oliver, J. K., Putnam, H. M., and Gates, R. D. (2015). Building coral reef resilience through assisted evolution. PNAS 112, 2307–2313. doi: 10.1073/pnas.1422301112
Verdura, J., Sales, M., Ballesteros, E., Cefall, M. E., and Cebrian, E. (2018). Restoration of a canopy-forming alga based on recruitment enhancement: Methods and long-term success assessment. Frontiers in Plant Science 9:1832. doi: 10.3389/fpls.2018.01832
Vergés, A., Doropoulos, C., Malcolm, H. A., Skye, M., Garcia-Pizá, M., Marzinelli, E. M., et al. (2016). Long-term empirical evidence of ocean warming leading to tropicalization of fish communities, increased herbivory, and loss of kelp. PNAS 113, 13791–13796. doi: 10.1073/pnas.1610725113
Vergés, A., McCosker, E., Mayer-Pinto, M., Coleman, M. A., Wernberg, T., Ainsworth, T., et al. (2019). Tropicalisation of temperate reefs: implications for ecosystem functions and management actions. Functional Ecology. 33, 1000–1013. doi: 10.1111/1365-2435.13310
Watanuki, A., Aota, T., Otsuka, E., Kawai, T., Iwahashi, Y., Kuwahara, H., et al. (2010). Restoration of kelp beds on an urchin barren: Removal of sea urchins by citizen divers in southwestern Hokkaido. Bulletin of the Fisheries Research Agency 32, 83–87.
Weber, X. A., Edgar, G. J., Banks, S. C., Waters, J. M., and Fraser, C. I. (2017). A morphological and phylogenetic investigation into divergence among sympatric Australian southern bull kelps (Durvillaea potatorum and D. amatheiae sp. nov.). Molecular Phylogenetics and Evolution 107, 630–643. doi: 10.1016/j.ympev.2016.12.027
Wernberg, T., Bennett, S., Babcock, R., de Bettignies, T., Cure, K., Depczynski, M., et al. (2016). Climate-driven regime shift of a temperate marine ecosystem. Science 353, 169–172. doi: 10.1126/science.aad8745
Wernberg, T., Coleman, M. A., Babcock, R. C., Bell, S. Y., Bolton, J. J., Connell, S. D., et al. (2019). Biology and ecology of the globally significant kelp Ecklonia radiata. Oceanography and Marine Biology: An Annual Review 57, 265-324.
Womersley, H. B. S. (1987). The Marine Benthic Flora of Southern Australia Part II. Adelaide: South Australian Government Printing Division.
Womersley, H. B. S. (1994). The Marine Benthic Flora of Southern Australia Part IIIA. Canberra: Australian Biological Resources Study.
Wood, G., Marzinelli, E. M., Coleman, M. A., Campbell, A. H., Santini, N. S., Kajlich, L., et al. (2019). Restoring subtidal marine macrophytes in the Anthropocene: trajectories and future-proofing. Marine and Freshwater Research
Yoon, J. T., Sun, S. M., and Chung, G. (2014). Sargassum bed restoration by transplantation of germlings grown under protective mesh cage. Journal of Applied Phycology 26, 505–509. doi: 10.1007/s10811-013-0058-8
Keywords: canopy, Great Southern Reef, ecosystem, habitat-forming, macroalga, rehabilitation
Citation: Layton C, Coleman MA, Marzinelli EM, Steinberg PD, Swearer SE, Vergés A, Wernberg T and Johnson CR (2020) Kelp Forest Restoration in Australia. Front. Mar. Sci. 7:74. doi: 10.3389/fmars.2020.00074
Received: 12 September 2019; Accepted: 30 January 2020;
Published: 14 February 2020.
Edited by:
Megan Irene Saunders, CSIRO Oceans and Atmosphere (O&A), AustraliaReviewed by:
Kevin Alexander Hovel, San Diego State University, United StatesWendy Nelson, The University of Auckland, New Zealand
Copyright © 2020 Layton, Coleman, Marzinelli, Steinberg, Swearer, Vergés, Wernberg and Johnson. This is an open-access article distributed under the terms of the Creative Commons Attribution License (CC BY). The use, distribution or reproduction in other forums is permitted, provided the original author(s) and the copyright owner(s) are credited and that the original publication in this journal is cited, in accordance with accepted academic practice. No use, distribution or reproduction is permitted which does not comply with these terms.
*Correspondence: Cayne Layton, cayne.layton@utas.edu.au