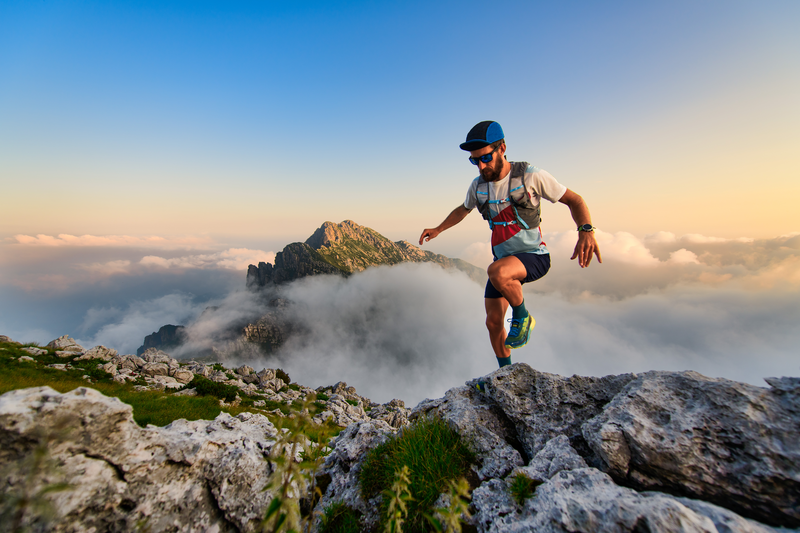
94% of researchers rate our articles as excellent or good
Learn more about the work of our research integrity team to safeguard the quality of each article we publish.
Find out more
ORIGINAL RESEARCH article
Front. Mar. Sci. , 06 February 2020
Sec. Marine Biotechnology and Bioproducts
Volume 7 - 2020 | https://doi.org/10.3389/fmars.2020.00031
Endophytes are an unexploited source of pharmacologically relevant compounds owing to their species richness and diversity. In this study, a total of 26 endophytic fungi were isolated and identified from 10 marine algal samples collected from the Konkan coast, Goa, India. Eighteen of the fungal isolates belonged to phylum Ascomycota while one belonged to phylum Basidiomycota based on ITS sequencing. Further, the genus Aspergillus sp. was the most common and abundant endophyte found in the sampled algal species. A significant antibacterial activity against five pathogenic bacteria was exhibited by the extracts of fungal isolates AG1.1, AG1.1 (G) and VG2.6 (agar diffusion assay). The extracts of fungal endophytes VB1.1, PG1.2 and VG2.6 demonstrated good antioxidant activity (DPPH scavenging assay). Further, cytotoxicity of all the endophytic extracts on human cancer cell lines was determined by MTT and resazurin assay. The crude extract of Aspergillus unguis (AG 1.2) showed the highest cytotoxic potential on cervical cancer (HeLa), breast cancer (MCF-7), lung cancer (A549), and skin cancer (A431) cell lines in a concentration dependent manner. Moreover, Gas Chromatography-Mass Spectroscopy analysis of the extract of A. unguis (AG 1.2) confirmed the presence of several bioactive metabolites including azelaic acid, azetidine, and furopyrans. The extract of A. unguis (AG 1.2) demonstrated G1 phase cell cycle arrest, reactive oxygen species (ROS)-dependent MMP loss and apoptosis-dependent cell death in A431 cells. The algae-derived fungal endophytes of Konkan coast are a rich source of novel pharmaceutically active compounds as indicated by this work.
For centuries treatment of a wide spectrum of maladies depended on natural sources (Mann, 2002; Marris, 2006). In the modern medicine, many effective lead molecules have come out of experimental research on natural products which include complex extracts or isolated compounds from plants, marine organisms, bacteria, and fungi (Lauritano et al., 2016). Due to the wide range of climatic and topological conditions, India offers a variety of flora and fauna, ranked to be one of the richest in the world (Nagarajan, 2014). The Indian coastline extends over 8,000 km with rich marine habitats, not just restricted to the sea but also in the intertidal rocky, sandy and muddy shores, mangroves, algae and coral reefs.
Marine natural products have been the focus of global research owing to their species richness and diversity (Calisto et al., 2019). Marine life is fundamentally robust and tolerant to extreme situations caused by salinity, acidification, chemical pollution, and climate change owing to its symbiotic relationship with endophytes (Jensen and Fenical, 1994).
Marine algae have been demonstrated to be an excellent source of endophytic fungi (Debbab et al., 2011). In the last few decades, interesting bioactive secondary metabolites from marine endophytic fungi have been reported with relevant clinical targets, some of which are novel (Marris, 2006). The Konkan coast of India represents a diverse source of marine algae-derived endophytic fungi. These organisms could be a prospective host to a unique natural productome. Though the endophytes collected from India's eastern coast have been searched for drug prospecting, western coast has not been explored yet. Peptides isolated from a marine fungus Simplicillium obclavatum from the eastern coast of India showed moderate cytotoxic activity against human leukemia cell line (Liang et al., 2016). A mangrove associated fungus, Hypocrea lixii from the south eastern coast of India showed potent anticancer and antimicrobial activity (Bhimba et al., 2012).
Most of the natural products exhibit a myriad of bioactivities like antioxidative, antimitotic, anti-inflammatory, antiangiogenic, and cytotoxic (Simmons et al., 2005; Leman-Loubière et al., 2017). Marine endophytes continue to remain a source of novel and therapeutically important secondary metabolites (Deshmukh et al., 2018) which can be manipulated to generate compounds of interest at higher yield from their natural productome (Hussain et al., 2012).
Cancer is keeping its position alive in the list of the world's most alarming diseases. Every case turns out to be different since this disease demonstrates intricate cellular machinery and chemoresistance making it necessary to search for new cytotoxic compounds (Greve et al., 2010; Demain and Vaishnav, 2011; Afsar et al., 2016) necessary. Indole derivatives isolated from marine algae derived Paecilomyces variotii were cytotoxic against many cancer cell lines with IC50 values between 2.6 and 8.2 μg/mL (Deshmukh et al., 2018). Physcion obtained from red algae-associated fungus Microsporum sp. induced apoptosis in HeLa cells (Wijesekara et al., 2014). Similarly, Wentilactone B from Aspergillus wentii associated with Sargassum sp. displayed cytotoxicity against HeLa, HepG2, MDA-MB-231 cell lines at IC50 17 μM (Zhang et al., 2013).
Methicillin-resistant Staphylococcus aureus (MRSA) and many other deadly pathogenic bacteria have developed antibiotic resistance (Craft et al., 2019) necessitating the development of novel antibiotics. Pestalotia sp., a marine fungus associated with a brown alga was extracted to isolate a novel chlorinated bezophenone compound called pestalone when cocultured with a marine bacterium. It showed appreciable antibiotic activity against MRSA at MIC 37 ng/mL. The ethyl acetate extracts of Penicillium glabarum, Fusarium oxysporum, and Alternaria alternate isolated from different marine plants including algae and invertebrates possessed broad spectrum antimicrobial potential (Zhang et al., 2012).
Generation of free radicals and causing oxidative impairment is a classical stage in cancer, inflammation, senescence, and neurodegenerative disorders (Finkel and Holbrook, 2000). Artificially produced antioxidants are used currently to mitigate these effects. However, there is an unprecedented interest in naturally produced antioxidants. Li et al. (2017) isolated hydroanthraquinones with antioxidative and antimicrobial properties from a marine red alga-associated endophytic fungus Talaromyces islandicus. Extracts of mangrove-associated Pestalotiopsis sp. could produce natural antioxidants with IC50 values of 9.75 ± 0.58 μg/mL (Zhao et al., 2018).
This is the first study that provides insight into the diversity and richness of marine algae -derived fungal endophytes from Konkan coast of Goa and screening for their cytotoxic antimicrobial and antioxidative activities to understand the mechanism of cytotoxicity.
Fresh algal samples were collected in August 2017 from the rocky Konkan coast of Goa, India (Latitude: 28°38′N Longitude: 72°12′E). The coastal areas covered were Terekhol (15.7251°N, 73.6882°E), Vagator (15.6030°N, 73.7336°E), Anjuna (15.5736°N, 73.7407°E), Baga (15.5553°N, 73.7517°E) in North Goa while Cabo de Ram (15.08806°N 73.91991°E) and Palolem (15.0100°N, 74.0232°E) in South Goa (Figure S2). Algal samples along with 50 mL seawater were placed in appropriately labeled polythene bags, transported to the laboratory in CSIR-NIO, Goa where they were identified and processed immediately for isolation of endophytic fungi. The identification of the macroalgal specimens was based on the publications of Pereira and Almeida (2014) and manuals of CSIR-National Institute of Oceanography, Goa, India (Dhargalkar and Kavlekar, 2004) with the help of Dr. C. Ravindran, Sr. Scientist, CSIR-NIO, Goa.
The general methodology used for isolation of endophytic fungi was as reported earlier by Kumari et al. (2018). Briefly, the algal samples were washed with sterile sea water. Further, the sample was rinsed with 70% ethanol for 60 s followed by 0.4% sodium hypochlorite for 30 s. Two washes with sterile sea water for 60 s each were given and then the samples were dabbed with a sterile tissue paper. The alga was aseptically cut into segments of about 2.0 cm with a sterile blade and five pieces were pressed onto PDA plates supplemented with artificial sea salts and streptomycin (250 mg/L). To ensure exclusive growth of endophytic fungi, drops of last rinse of sea water were placed on PDA plates. The plates were incubated for 7 days in dark condition at 28 ± 2°C. The fungal strains used in this study were observed to grow exclusively from algal samples placed on PDA plates, thus ensuring their endophytic nature. The colonization rates (CR%) and isolation rates (IR%) were calculated. The fungal diversity was also calculated using Shannon-Weiner Index (H') following the method of Tan et al. (2018).
Every fungal mycelium emerging from the algal segment was separately plated to obtain its pure culture. The pure endophytic fungi thus obtained were photographed and preserved using sterile PDA containing penicillin and mineral oil (Felicio et al., 2015).
Fungal isolates were identified by sequencing of the 5.8S rDNA internal transcribed region (ITS). To obtain fungal mycelia, all the fungal isolates were grown in 25 mL PDB for 7 days at 28 ± 2°C. Mycelia was filtered and used for DNA extraction following previously described protocol (Kumari et al., 2018). ITS1 (sequence: 5′-TCC GTA GGT GAA CCT GCG G-3′) and ITS4 (5′-TCC TCC GCT TAT TGA TAT GC-3′) primers were used to amplify and sequence the fungal genomic ITS region. The PCR products were purified and sequenced by AgriGenome Labs (AgGenome), India.
The amplified ITS sequences were compared with known sequences in NCBI GenBank using BLASTN. Sequences with high similarity matches were used in taxonomical identification. The sequences of endophytic fungi were submitted to the GenBank and accession numbers were obtained (Leylaie and Zafari, 2018).
For construction of the phylogenetic tree, ITS sequences of the endophytic fungi and their nearest neighbors from NCBI were aligned using ClustalW in MEGA-X. UPGMA method was used to build the tree. Evolutionary history was inferred by the Neighbor-Joining method and the evolutionary distances were computed using Maximum Composite Likelihood method. The anamorphic fungus Sporendocladia bactrospora of the family Ceratocystidaceae was used as an outgroup species.
All the fungal isolates were pre-cultured on PDA plates for 7 days. Thereafter, 3 agar discs of each endophyte were inoculated into 1,000 mL Erlenmeyer flask containing 200 mL PDB. These flasks were then incubated in dark static conditions at 28 ± 2°C for 28 days. Cultures were then filtered through a pre-weighed Whatman No. 1 filter paper. The wet mycelial mat on the filter paper was dried in a hot air oven overnight after which it was weighed again to calculate the dry weight of the fungal biomass. The dried fungus was crushed in liquid nitrogen using mortar and pestle and added to the filtered fermentation broth. This was extracted with twice the volume of ethyl acetate for 2 days by placing on rotary shaker at room temperature. On the following day, the separated organic phase was removed by concentration in vacuo. The extract so obtained was further volatilized in speed vacuum at a mild temperature of 40°C until all the organic solvent was removed. The dried total culture crude extracts were stored at −20°C.
Antibacterial activity was determined by agar well diffusion assay against four Gram-positive pathogenic bacteria (Staphylococcus aureus, Bacillus megaterium, Bacillus subtilis, and Xanthomonas campestris) and two Gram-negative pathogenic bacteria (Escherichia coli and Pseudomonas aeruginosa). Stock cultures were maintained on nutrient agar slants at 4°C. Single colony of each microorganism was picked from the stock slant and transferred into 5 mL of sterile LB broth. The cultures were incubated overnight on rotary shaker at 180 rpm at 37°C. Inoculum (100 μL) of overnight grown cultures was spread plated on LB plates and left to dry for 5 min. Wells (diameter 8 mm) were dug in these plates using a sterile metallic borer. The extracts were tested at a range of concentrations ranging from 10 to 1,000 μg/mL. The extracts were dissolved in DMSO and filtered through 0.22 μm syringe filters and dilutions were made in sterile LB broth and added into the well, respectively. Streptomycin (250 mg/L) was used as a positive control and sterile LB broth with DMSO (0.1% v/v) without addition of extract was used as negative control. The plates were left undisturbed for 30 min to allow diffusion of fungal extract into the agar. Later, the plates were incubated overnight at 37°C. At the end of incubation period, the zone of inhibition (ZOI) formed around the well was measured. The experiment was performed in triplicates. The mean ± SD of ZOI was used to evaluate the antibacterial activity of the fungal crude extract (Hafedh et al., 2008; Punnam et al., 2016).
The antioxidative effect of all the fungal extracts was assessed by the DPPH scavenging assay. Briefly, 100 μL of 0.1 mM methanolic solution of DPPH was added to a 96-well plate. Hundred microliter of four different concentrations of the fungal crude extracts (10, 50, 100, and 200, μg/mL) dissolved in methanol was added to it. The plates were incubated in dark at room temperature for 30 min. The radical scavenging activity was determined by measuring absorbance at 517 nm wavelength against methanol (blank) (Molecular Devices, USA). Ascorbic acid was used as a positive control and untreated DPPH served as a negative control (Hafedh et al., 2008).
The cytotoxic potential of all the fungal crude extracts was measured using MTT on different human cancer cell lines including human lung cancer (A549), human cervical cancer (HeLa), human skin cancer (A431) and human breast cancer (MCF-7), and a normal human embryonic kidney (HEK 293T) cells as non-cancer cell line. The respective cells were cultured in complete DMEM supplemented with 10% FBS, 100 mg/L penicillin, 250 mg/L streptomycin and 2 mM glutamine and grown at 37°C for 24 h in ambient conditions of 5% CO2 and 95% humidity in a CO2 incubator (Kumari et al., 2018).
These cells were seeded in a transparent 96-well plate each at a density of 1 × 104 cells/mL and incubated in the above-mentioned conditions. After 24 h, the cells were treated with four different concentrations of the fungal crude extract (10, 50, 100, and 200 μg/mL), respectively, and incubated at 37°C for 48 h in the ambient conditions of 5% CO2 and 95% humidity in a CO2 incubator. At the end of incubation period, 10 μL of 5 mg/mL MTT solution (5 mg/mL in PBS) was added to each well and incubated again in the CO2 incubator for 2 h. Further, the plates were emptied and 100 μL of DMSO was filled in every well to dissolve the purple MTT-formazan crystals. Absorbance was measured on a 96 well plate reader Infinite M2000 Pro™ (Tecan, Crailsheim, Germany) at 595 nm. The IC50 values were calculated for each cell line. All the assays were performed in triplicates and results are presented as mean ± SD.
The resazurin reduction assay was performed to determine and corroborate the cytotoxic potential of the fungal total culture extracts. A549, MCF-7, A431, and HeLa cells were seeded in 96-well plates at a seeding density of 1 × 104 cells/mL in a total volume of 100 μL. After 24 h, the cells were treated with different concentration of the total culture fungal extract (10, 50, 100, and 200 μg/mL). After 48 h, 20 μL resazurin (0.01% w/v in double distilled water) was added to each well and the plates were incubated in dark at 37°C for 3 h. Fluorescence was measured on an Infinite M2000 Pro™ plate reader (Tecan, Crailsheim, Germany) using an excitation wavelength of 544 nm and an emission wavelength of 590 nm. Cell viability was evaluated based on a comparison with untreated cells and IC50 values were calculated (Kuete et al., 2017).
Based on the cytotoxicity assessment, fungal extract of AG1.2 showed the highest cytotoxicity, which was chosen for further studies.
The biologically active fungal crude ethyl acetate extract was subjected to GC-MS analysis to investigate the presence of volatile metabolites. The sample was derivatized to impart volatility to the compounds for maximum detection. Briefly, 100 μL of 1 mg/mL stock was dried with pure N2 gas to which 50 μl of derivatizing reagent N,O-Bis(trimethylsilyl)trifluoroacetamide:Chlorotrimethylsilane (BSTFA:TMCS (99:1) was added. This mixture was incubated at 60°C for 120 min. Derivatized sample was diluted 10 times with ethyl acetate. One microliter of this was subjected to GC-MS. Thus, the compounds obtained were trimethylsilyl adducts or derivatives (Guo et al., 2006).
GC-MS analysis was performed using Agilent system 7890A equipped with electron impact (EI) and a single quadrupole mass analyzer. Agilent column DB-5MS (30 mL × 0.25 mm ID × 0.25 um film thickness) was used for separation. The oven program had an initial temperature of 40°C for 5 min, then a 10°C/min run to 260°C with a final hold at 260°C for 10 min. The injector temperature was kept at 250°C (splitless mode) The carrier gas was purified Helium (99.99%) at a linear flow rate of 1 mL/min. All detected compounds were putatively identified by mass spectral database search of the National Institute of Standards and Technology (NIST) followed by matching of the mass spectral data.
To understand the effect of incubation time on production of biomass and cytotoxic secondary metabolite, the total culture was harvested at different time intervals (7, 14, 21, 28, and 35 days). Same procedure was followed for inoculation and extraction of fifteen 1 L flasks containing 200 mL PDB with double the volume of ethyl acetate. The mycelial dry weight was measured at each time point. All the total culture crude extracts of A. unguis were evaluated by MTT assay on A431 cell line.
The fungus, A. unguis was grown in fifteen 1 L flasks containing 200 mL of PDB and harvested. However, four different solvents ethyl acetate, dichloromethane, chloroform and hexane were used for extraction of secondary metabolites from total culture based upon their polarity. Finally, all the crude extracts were evaluated for their cytotoxic potential by MTT assay on A431 cell line.
Live dead analysis was carried out in A431 cells following the protocol of Kumari et al. (2018). The experiment was conducted on A431 cells after a treatment with increasing concentrations of fungal crude extract for 24 h. Briefly, the cells were seeded in 24 well plate at a density of 105 cells/well. After the appropriate treatment interval, the cells were trypsinized and washed with PBS two times. Propidium iodide (1 μg/mL) was added just before data acquisition in CytoFlex flow cytometer (Beckman Coulter) by CytExpert 2.0 software.
Effect of the fungal crude extract in the cell cycle progression on A431 cells was evaluated by PI staining following the protocol of Pozarowski and Darzynkiewicz (2004). Cells were seeded in 6-well plate at a density of 1.2 × 106 cells/mL. These cells were treated with increasing concentrations of the crude extract (3–25 μg/mL) for a duration of 24 h. Further, the cells were harvested with trypsin, washed with PBS, fixed overnight with ice cold 70% ethanol and were incubated with RNase A. Finally, the cells were stained with 50 μg/mL propidium iodide for 30 min at 37°C after which data was acquired in CytoFlex flow cytometer (Beckman Coulter) by CytExpert 2.0 software for a minimum of 10,000 cells per sample. Hundred nanometer Taxol treated cells served as positive control while untreated cells served as negative control.
Loss of MMP post treatment with fungal crude extract was measured by FACS using JC-1 stain (Kumari et al., 2018). Briefly, A431 cells were seeded in a 24-well plate at a density of 104 cells/mL. Cells were treated with different concentrations of fungal crude extracts (3–25 μg/mL) for 24 h. Further, the cells were trypsinized and washed with PBS twice. The cells were stained with freshly prepared 2.5 μg/mL of JC-1 in DMEM at 37°C in dark for 15 min before acquiring data in CytoFlex flow cytometer (Beckman Coulter) by CytExpert 2.0 software. Carbonyl cyanide 3-chlorophenylhydrazone (CCCP) treated cells served as positive control while untreated cells were used as negative control.
As described previously by Eruslanov and Kusmartsev (2010), A431 cells seeded at a density of 104 cells/mL in a 24-well plate were treated with various concentrations of the fungal crude extract (3–25 μg/mL) for 24 h. Further, to measure the ROS generation, medium was replaced with freshly prepared 10 μM DCFH-DA in DMEM at 37°C in dark for 15 min in CO2 incubator. Next, the cells were trypsinised and washed with PBS twice. Data was immediately acquired in CytoFlex flow cytometer (Beckman Coulter) by CytExpert 2.0 software. A 15 min treatment of 10 mM H2O2 prepared in DMEM was used as positive control while untreated cells served as negative control.
A classical sign of apoptosis is externalization of PS to the cell surface was measured by FACS by dual staining with annexin V-fluorescein isothiocyanate (annexinV-FITC)/propidium iodide (PI). Annexin V binds to PS and flags the earliest stage of apoptosis. On the other hand, PI enters the cells only after membrane integrity is damaged. Thus, annexin V-FITC positive and PI negative cells are early apoptotic cells while annexin V-FITC/PI-double positive are late apoptotic cells.
For this study, A431 cells were seeded in 24-well plates at a density of 3.0 × 104 cells/mL. After treatment of the fungal crude extract for 24 h (3–25 μg/mL), the cells were trypsinized and according to the manufacturer's instructions (Annexin V-FITC Apoptosis Detection Kit - Sigma Aldrich) the cells were stained with 1 μL of the AnnexinV-FITC stain with or without PI in the annexin binding buffer. After 15 min of incubation in dark, cells were analyzed using CytoFlex flow cytometer (Beckman Coulter) by CytExpert 2.0 software (Dhayanithy et al., 2019).
All the experiments were performed in triplicate and quantitative variables are represented in terms of mean ± S.D. in histograms using the statistical software prism.
Ten different marine algae were sampled from different locations of Goa coast, India (Vagator, Anjuna, Terekhol and Palolem, Baga and Cabe de Ram) in the monsoon season (Figure 1A). Six belonged to Chlorophyceae (green alga) and four others to Phaeophyceae (brown alga). In two locations, Cabe de Ram and Baga, marine algae were not observed in the inter-tidal region. The surface sterilization procedure ensured the isolation of endophytic fungi exclusively. Representative images of endophytic fungi emerging from pieces of alga and their pure culture have been shown in Figures 1B,C. A total of 26 endophytic fungi were isolated from the collected algal samples. As summarized in Table 1 highest percentage of colonization rate (CR%) was observed in the Enteromorpha sp. (green alga) collected from the Vagator coast while the least was observed in one species of Padina (brown alga) from the Palolem coast. Shannon-Weiner diversity indices (H') indicated that the green alga Chaetomorpha sp. hosts eight different types of fungal endophytes implicating the highest endophytic community diversity (1.742) followed by the same algal species collected from Vagator coast (1.329). Least endophytic diversity was observed in algal samples collected from Terekhol coast. Brown alga Dictyota sp. collected from Vagator coast also hosted least fungal diversity. The low fungal diversity in some of the algal hosts could be because of the peculiar slow growth of endophytic fungi, temperature and media requirements or they were intimately associated with the host.
Figure 1. Habitat of marine algae and its fungal endophytes. (A) Chaetomorpha sp. growing on the rocky shore of Vagator. (B) Fungal endophytes emerging from algal segments after 2 weeks on PDA plate. (C) Representative fungal morphophytes isolated from various marine algae growing on PDA for 7 days at 26°C.
All the culturable fungal isolates were identified by sequence analysis of the ITS rDNA region. Subsequently, 19 isolates were categorized at the genus and species level based on sequence similarity analyses, while seven isolates remained unidentified due to low sequence homology in the GenBank database (Table 2). Further, a phylogenetic tree describing the taxonomic relationship between the fungal endophytes identified from various marine algal species was constructed using MEGA X software (Figure 2). All the isolates were cladded with the members from their respective class and genera.
Table 2. Culturable endophytic fungi identified from marine algae collected from Konkan coast, India.
Figure 2. Phylogenetic tree based on neighbor-joining method. The tree was constructed using ITS-region sequences of fungal endophytes isolated from marine algae.
According to the diversity and sequence data of the 19 isolates, 18 belonged to Ascomycota and the isolates matched with 10 different genera mostly belonging to Aspergillus. One isolate belonged to phylum Basidiomycota, genera Perenniporia.
The 18 representatives belonging to phylum Ascomycota belonged to three classes, including Eurotiomycetes, Sordariomycetes, and Dothideomycetes. Eurotiomycetes class was represented by 10 isolates, Sordariomycetes by six and Dothideomycetes by two isolates. Of all the algal species investigated, Enteromorpha sp. from the Palolem coast contained a rich diversity of endophytic fungi, and most of them belonged to the ubiquitous phylum Ascomycota.
Eurotiomycetes was the most prevalent class of endophytic fungi followed by Sordariomycetes and Dothideomycetes. Aspergillus was the most common fungal genus and was abundant in all the algal species. However, fungi belonging to Basidiomycota appeared to be a rare possibly because of their inability to associate with marine algae. This could be because of the absence of lignin which is the most appropriate substrate for fungal growth. Algal thali contains cellulose in high amount. Nevertheless, Perenniporia sp. of the phylum Basidiomycota was found associated with a green alga Enteromorpha sp. This can be corroborated with the findings of Baldrian and Valásková (2008) which demonstrated the potential of certain Basidiomycetes to produce cellulolytic enzymes.
Eurotiomycetes was the most represented class among all the endophytes. Its high frequency in this study is concordant with previous reports (Gnavi et al., 2017). The dominance of this class in marine algae could be attributed to the high growth rate and sporulation.
Fungi belonging to the class Dothideomycetes are usually common in the marine habitat. They commonly associate with brown and red seaweeds. However, only two endophytic fungi belonging to this class were found. One of them was Pleosporales sp. which is a very common representative of this class. The other member of this class was Cladosporium sp. of the order Capnodiales. It mainly includes plant and human pathogens and saprobes (Crous et al., 2009). Their minimal presence could be attributed to the peculiar requirements or specificity of the algal host. Further, it was observed that endophytic fungus Chaetomium globosum (PG1.6) was pigmented with a characteristic yellow color indicating the presence of flavonoids or terpenoids.
Endophytic fungi from marine origin have emerged as a new source for an undiscovered natural productome which can further be used for human welfare. The current study shows a wide diversity of fungal endophytes comprising many genera and species can act as ‘hot factories’ for production of bioactive secondary metabolites, an untapped source for drug discovery.
Ethyl acetate extracts of all isolated fungi were screened for their antibacterial potential against five pathogenic bacteria at a range of concentrations. A total of 12 different isolates showed significant antibacterial activity against the tested pathogens (Table 3). AG1.1, AG1.1 (G), and VG2.6 identified as Aspergillus unguis exhibited antimicrobial activity against both Gram-positive and Gram-negative pathogenic bacteria in a concentration dependent manner. Highest antibacterial activity was demonstrated by PG1.6 identified as Chaetomium globosum against E. coli and P. aeruginosa.
Table 3. Antibacterial activity of fungal endophytes isolated from marine algae collected from Konkan coast, India.
Interestingly, most of the endophytes showing antibacterial potential were isolated from the green algae, irrespective of the locations. Many of the marine green algae are known to produce antibacterial compounds (Shannon and Abu-Ghannam, 2016). Endophytes living in the close vicinity of their host may possibly get the same gene via horizontal gene transfer (HGT) to acquire production of antibiotics. The endophytes showing highest antibacterial activity belonged to genus Aspergillus and Chaetomium. Members of these genera are known to produce a wide range of antimicrobial secondary metabolites (Deshmukh et al., 2018). Mitrephora wangii flowers hosted endophytic Aspergillus sp. which could produce β-thujaplicin at high yield with strong antimicrobial spectrum (Monggoot et al., 2018).
The maximum antioxidative activity was shown by VB1.1 followed by PG1.2 and VG2.6 in a concentration-dependent manner (Figure 3). The DPPH scavenging activity of VB1.1 was comparable to the scavenging activity of ascorbic acid. Endophytic fungi isolated from marine algae are the hot spot for the synthesis of a myriad of bioactive compounds. Earlier, Li et al. (2017) extracted antioxidant hydroanthraquinones from the marine algal-derived endophytic fungus Talaromyces islandicus EN-501.
Figure 3. Antioxidative activity of marine algal-derived endophytic fungi from Konkan coast, India estimated by DPPH scavenging assay.
All the endophytic fungal ethyl acetate extracts were assessed for their cytotoxic potential against four different human cancer cell lines, namely HeLa, MCF-7, A549, and A431 on different concentrations (10, 50, 100, and 200 μg/mL) (Figure 4). The IC50 values exhibited by all the ethyl acetate extracts are given in Table S1. Out of the 26 endophytes screened for cytotoxicity, ethyl extract of eight endophytes showed an IC50 value < 10 μg/mL on all four cell lines. Based on maximum percentage of cell death at 10 μg/mL, AG 1.2 (Aspergillus unguis) was selected for further study. The ethyl acetate extract of AG 1.2 was again evaluated for its cytotoxic potential at lower concentrations (3, 5, 10, and 25 μg/mL) on HeLa, MCF-7, A549, A431, and HEK 293T (Figure S1) which showed an IC50 value of 5.94 ± 0.077 μg/mL on A431 cell line (Table 4). Further, the resazurin reduction assay was conducted to confirm the cytotoxic potential of the crude extract on A431 cells. The results corroborated with those obtained by MTT showing an IC50 value of 6.09 ± 0.051 μg/mL. The extract showed IC50 value > 25 μg/mL on HEK 293T cell line showing the non-cytotoxic nature of the extract on normal non-cancer cell lines.
Figure 4. In vitro cytotoxicity of ethyl acetate total culture fungal extracts against A431, HeLa, A549 and MCF-7 cells. Cells were incubated with different concentrations (25, 50, 100, and 200 μg/mL) of extracts and the cytotoxicity was determined by MTT assay.
Table 4. IC50 value of Aspergillus unguis (AG1.2) on multiple human cancer cell lines by MTT and resazurin assay.
Both resazurin and MTT assays are reliable methods to measure cytotoxicity of drugs on cancer cell lines by detecting change in cellular metabolism and plasma membrane permeability (Gordon et al., 2018). Interestingly, all the fungal isolates irrespective of their place of isolation and genera showed cytotoxic activity on the cancer cell lines tested, though eight isolates fulfilled the criteria of National Cancer Institute showing IC50 value much lesser that 30 μg/mL (Suffness and Pezzuto, 1990). The robust and saline environment of the marine endophytes provided them a unique chemical space to synthesize structurally diverse bioactive secondary metabolites (Sarasan et al., 2017). The unexplored wealth of marine Algicolous endophytic fungi from Konkan coast promises for the discovery of potent and novel bioactive compounds.
The GC-MS analysis of ethyl acetate extract of AG1.2 revealed some compounds of cytotoxic and bioactive nature (Table S2). Azelaic acid, Azetidine, and furo pyrans present in the extract are known for their bioactive properties (Figure 5). Azelaic acid is a dicarboxylic acid known for its effectiveness against inflammatory acne and cytotoxic potential against human malignant melanocyte (Fitton and Goa, 1991). Earlier, Noolvi et al. (2014) reported cytotoxic and antimicrobial activity of novel azetidine-2-one. Decane, dodecene, undecene, octane, and cetene obtained in the extract form a major part of essential oils, known to show antimicrobial and antioxidative properties (Chouhan et al., 2017; Kumari et al., 2017). Dedecane skeleton containing compounds isolated from marine isolate Penicillium bilaiae MA-267 have shown antifungal properties against plant pathogens (Meng et al., 2014). Many peaks obtained from GC-MS remained unidentified indicating the high probability of finding novel bioactive compounds in the crude extract of A. unguis.
Figure 5. Representative compounds found by GC-MS analysis in ethyl acetate extract of AG 1.2 (Aspergillus unguis).
For enhanced yield of bioactive compounds, it is necessary to optimize the growth curve and polarity of organic solvents used. The fungal growth in PDB was monitored in static and dark conditions at 28 ± 2°C up to 35 days. In order to extract the cytotoxic secondary metabolites, several organic polar and non-polar solvents were optimized. Further, the extent of cancer cell growth inhibition by these extracts was monitored by MTT assay after 48 h of treatment. AG1.2 clearly showed maximum biomass as well as cytotoxicity after incubation for 28 days in dichloromethane extract (Figures 6A–C) indicating the semi-polar nature of cytotoxic compounds present in the extract. Growth curve and solvent optimization are often done to manipulate and enhance the yield of bioactive compounds present in the crude extract (Ruiz et al., 2010). Marine algae-derived fungal endophytes may require a specific culture time period to produce certain metabolites of bioactive potential. Xu et al. (2015) found that anti-tumor wentilactones, isolated from marine fungus Aspergillus dimorphicus, require growth period of 25–30 days for production. Organic solvents based upon their polarity can extract secondary metabolites present differentially. Ebada et al. (2008) have focused on the role and polarity of organic solvents for isolation, purification, and structural elucidation of bioactive secondary metabolites from marine invertebrates.
Figure 6. Culture condition optimization of crude extract of A. unguis (AG 1.2). (A) Growth curve analyses of A. unguis grown in PDB. (B) Optimization of incubation time interval for production of cytotoxic secondary metabolites evaluated by MTT assay on A431 cells. (C) Evaluation of appropriate organic solvent for total extraction of cytotoxic metabolites from A. unguis by MTT assay on A431 cells.
Propidium Iodide (PI) is a membrane impermeable dye that is generally excluded from viable cells since their membrane integrity is intact. Such a dye exclusion test makes it possible to estimate the percentage of live and dead cells after treatment (Crowley et al., 2016). AUDE exhibited a dose dependent response in A431 cells line. The cell death percentage increased from 33 to 96.30 ± 2.25% in the concentration range from 3 to 25 μg/mL (Figures 7A,B). To examine whether AUDE induced cell death connects with the changes in cell cycle progression, the distribution of treated cell in the phases of cell cycle were assessed. Analyses of cell population in sub G0, G1, S, and G2M at four different concentrations of fungal extracts were done after 24 h of crude extract treatment. AUDE induced a concentration dependent G1 phase arrest (Figures 7C,D) at four different concentrations. The G1 population increased from 49.14% in control to 82.15% after 24 h of AUDE treatment (25 μg/mL).
Figure 7. Mechanism of cell death induced by AUDE treatment in A431 cells as determined by FACS analyses: (A,B) PI staining after 24 h of treatment. (C,D) Effect of AUDE treatment on cell cycle arrest after 24 h in A431 cells stained with PI. (E,F) Effect of AUDE treatment on mitochondrial membrane potential after 24 h in A431 cells, stained with JC-1. (G,H) Effect of AUDE treatment on ROS production after 24 h in A431 cells, using DCFH-DA dye. (I,J) Effect of AUDE treatment on externalization of phosphatidylserine in A431 cells after 24 h, using annexin FITC/PI dual staining.
The effect of AUDE on the mitochondrial apoptotic pathway, the extent of mitochondrial membrane depolarization in cancer cells was probed with cationic dye JC-1. The dye which exits as aggregates emitting red fluorescence within the electronegative interior of healthy mitochondria and as green fluorescent monomers in depolarized mitochondria. Thus, a reduction in red to green fluorescence ratio clearly indicates loss in mitochondrial membrane potential (MMP) (Ebrahimi-Fakhari et al., 2016). A loss of 18.8 ± 1.8% in MMP was observed after treatment of AUDE (3 μg/mL) while it increased to 65.9 ± 3.4% after treatment of AUDE (25 μg/mL) (Figures 7E,F).
Loss in MMP is also linked with the production of reactive oxygen species (ROS) (Rastogi et al., 2010). The cells were stained with DCFH-DA and further examined by flow cytometry. A concentration-dependent increase in the percentage of ROS production was observed. ROS production increased up to 50.2 ± 2.8% with an increase in the concentration of AUDE from 3 to 25 μg/mL after 24 h (Figures 7G,H).
Further, to examine the externalization of phosphatidylserine (PS) in A431 cells for confirmation of apoptosis, annexin FITC/PI staining was performed. Live and healthy cancer cells being impermeable to PI and having a borderline presence of PS on its extracellular surface, are double negative (lower left quadrant). Early apoptotic cells are just positive for annexin V-FITC due to the flipped-out PS, but an intact cell membrane (lower right quadrant). Cells in the late stages of apoptosis owing to loss of membrane integrity are double positive for PI and annexin V (upper right quadrant). However, necrotic cells are usually positive only for PI, due to complete disruption of cell membrane and leakage of cellular contents, resulting in staining of only the fragmented DNA (upper left quadrant) (Crowley et al., 2016). After AUDE treatment for 24 h, percentage of early apoptotic cells increased from 0.21 ± 0.02% in control to 8.95 ± 1.44 and 59.65 ± 3.77%, respectively, at 3 and 5 μg/mL of AUDE. Further, late apoptotic cell population increased to 8.39 ± 1.55% while 57.38 ± 2.92% early apoptotic cell population was observed after 25 μg/mL of AUDE treatment for 24 h (Figures 7I,J).
Apoptosis is a well-known and extensively studied mechanism of cell death (Rastogi et al., 2010; Ebrahimi-Fakhari et al., 2016). Most of the natural products including those derived from endophytes have shown remarkable apoptotic cell death in different cancer cell lines. Recently, Nagabhishek and Madankumar (2019) isolated a novel apoptosis-inducing metabolite from marine sponge derived endophytic fungus Monascus sp. NMK7 which mediated ROS dependent apoptotic cell death in breast cancer cell line. Apoptosis follows a cascade of reactions ultimately leading to programmed cell death of cancer cells (Reed, 2000; Razak et al., 2019). Loss of ROS dependent mitochondrial membrane potential is one of the early hallmarks of intrinsic pathway of apoptotic cell death. The marine ecosystem is a pandora box for discovery of new and effective bioactive compounds. Cell cycle arrest is a classic tool to induce cytotoxicity by most of the chemo-therapeutic agents used in cancer treatment. The G1, S, or G2/M phase arrest is brought by potent drugs followed by the damaged cell enter mitosis and apoptosis (Nagabhishek and Madankumar, 2019). Mohyeldin et al. (2017) described a marine-derived pachycladin diterpenoid which acted as a novel inhibitor of wild-type and mutant EGFR responsible for metastasis of various types of cancers.
In conclusion, marine algal-derived endophytic fungi of Konkan coast are rich in fungal endophytic diversity. Many of the endophytes can emerge as prospective hosts for novel and potential bioactive compounds. Aspergillus unguis isolated from a green alga Enteromorpha sp., collected from Anjuna coast demonstrated ROS dependent MMP loss and apoptotic cell death in A431 cancer cell lines. Further studies on isolation and purification of bioactive compounds and their yield enhancement are needed to explore their pharmaceutical potential of these endophytes derived from Konkan coast.
The datasets generated for this study can be found in the NCBI GenBank, Accession numbers: MH654996, MH655000, MH655001, MH654992, MH654997, MH654995, MH654998, MH654999, MH655003, MH655004, MH645800.1, MH655002, MH655008, MH654993, MH655009, MH655005, MH655006, MH655007, MH654994.
CJ conceived the idea. SK, ST, and MK did the sampling. SK and MK designed, performed the experiments, analyzed the data, and wrote the manuscript. All authors reviewed the manuscript.
The authors declare that the research was conducted in the absence of any commercial or financial relationships that could be construed as a potential conflict of interest.
Authors thank FACS and GC-MS facility, Division of Biological Sciences, Indian Institute of Science, Bangalore. This study was supported by a grant from the Department of Biotechnology, Govt. of India, New Delhi (Ref. no. BT/PR/14569). We also thank DBT-IISc partnership program, DST-FIST and UGC special assistance program for financial support. SK thanks UGC for her SRF. MK thanks UGC for the award of D S Kothari post-doctoral Fellowship (DSKPDF) and ST thanks DBT for his SRF. Authors also acknowledge Dr. Ravindran C and his team at CSIR-National Institute of Oceanography, Goa, for their help in collection of marine algae samples. The bacterial pathogens were a kind gift from Dr. Kavitha Alapati, Department of Biochemistry, Indian Institute of Science, Bangalore, India. The authors also thank Prof. T. Ramasarma and Prof K. Sankara Rao for their valuable comments and suggestions on this manuscript.
The Supplementary Material for this article can be found online at: https://www.frontiersin.org/articles/10.3389/fmars.2020.00031/full#supplementary-material
Afsar, T., Trembley, J. H., Salomon, S. E., Razak, S., Khan, M. R., and Ahmed, K. (2016). Growth inhibition and apoptosis in cancer cells induced by polyphenolic compounds of Acacia hydaspica: involvement of multiple signal transduction pathways. Sci. Rep. 6:23077. doi: 10.1038/srep23077
Baldrian, P., and Valásková, V. (2008). Degradation of cellulose by basidiomycetous fungi. FEMS Microbiol. Rev. 32, 501–521. doi: 10.1111/j.1574-6976.2008.00106.x
Bhimba, B., Franco, A. D., Mathew, J. M., Jose, G. M., Joel, E. L., and Thanagraj, M. (2012). Anticancer and antimicrobial activity of mangrove derived fungi Hypocrea lixii VB1. Chin. J. Nat. Med. 10, 77–80. doi: 10.1016/S1875-5364(12)60017-X
Calisto, R., Saibo, E. F., Storesund, J. E., Øvreås, L., Herfindal, M., and Lage, O. M. (2019). Anticancer activity in planctomycetes. Front. Mar. Sci. 5:499. doi: 10.3389/fmars.2018.00499
Chouhan, S., Sharma, K., and Guleria, S. (2017). Antimicrobial activity of some essential oils-present status and future perspectives. Medicines 4:58. doi: 10.3390/medicines4030058
Craft, K. M., Nguyen, J. M., Berga, L. J., and Townsend, S. D. (2019). Methicillin-resistant Staphylococcus aureus (MRSA): antibiotic-resistance and the biofilm phenotype. MedChemComm. 10, 1231–1241. doi: 10.1039/C9MD00044E
Crous, P. W., Schoch, C. L., Hyde, K. D., Wood, A. R., Gueidan, C., de Hoog, G. S., et al. (2009). Phylogenetic lineages in the Capnodiales. Studies Mycol. 64, 17–47-S7. doi: 10.3114/sim.2009.64.02
Crowley, L. C., Chojnowski, G., and Waterhouse, N. J. (2016). Measuring the DNA content of cells in apoptosis and at different cell-cycle stages by propidium iodide staining and flow cytometry. Cold Spring Harbor Protoc. 10:087247. doi: 10.1101/pdb.prot087247
Debbab, A., Aly, A. H., and Proksch, P. (2011). Bioactive secondary metabolites from endophytes and associated marine derived fungi. Fun. Divers. 49:1. doi: 10.1007/s13225-011-0114-0
Demain, A. L., and Vaishnav, P. (2011). Natural products for cancer chemotherapy. Microb. Biotechnol. 4, 687–699. doi: 10.1111/j.1751-7915.2010.00221.x
Deshmukh, S. K., Prakash, V., and Ranjan, N. (2018). Marine fungi: a source of potential anticancer compounds. Front. Microbiol. 8:2536. doi: 10.3389/fmicb.2017.02536
Dhargalkar, V. K., and Kavlekar, D. P. (2004). Seaweeds-a Field Manual. Dona Paula: National Institute of Oceanography.
Dhayanithy, G., Subban, K., and Jayabaskaran, C. (2019). Diversity and biological activities of endophytic fungi associated with Catharanthus roseus. BMC Microbiol. 19:22. doi: 10.1186/s12866-019-1386-x
Ebada, S. S., Edrada, R. A., Lin, W., and Proksch, P. (2008). Methods for isolation, purification and structural elucidation of bioactive secondary metabolites from marine invertebrates. Nat. Prot. 3, 1820–1183. doi: 10.1038/nprot.2008.182
Ebrahimi-Fakhari, D., Saffari, A., Wahlster, L., Di Nardo, A., Turner, D., Lewis, T. L. Jr., et al. (2016). Impaired mitochondrial dynamics and mitophagy in neuronal models of tuberous sclerosis complex. Cell Rep. 17, 1053–1070. doi: 10.1016/j.celrep.2016.09.054
Eruslanov, E., and Kusmartsev, S. (2010). “Identification of ROS using oxidized DCFDA and flow-cytometry,” in Advanced Protocols in Oxidative Stress II, ed D. Armstrong (Totowa, NJ: Humana Press), 57–72.
Felicio, R., Pavãoa, G. B., de Oliveira, A. L. L., Erbert, C., Conti, R., Pupo, M. T., et al. (2015). Antibacterial, antifungal and cytotoxic activities exhibited by endophytic fungi from the Brazilian marine red alga Bostrychia tenella (Ceramiales). Rev. Bras. Farmacogn. 25:6. doi: 10.1016/j.bjp.2015.08.003
Finkel, T., and Holbrook, N. J. (2000). Oxidants, oxidative stress and the biology of ageing. Nature 408:239. doi: 10.1038/35041687
Fitton, A., and Goa, K. L. (1991). Azelaic acid. A review of its pharmacological properties and therapeutic efficacy in acne and hyperpigmentary skin disorders. Drugs 41, 780–798. doi: 10.2165/00003495-199141050-00007
Gnavi, G., Garzoli, A., Poli, A., Prigione, V., Burgaud, G., and Varese, G. C. (2017). The culturable mycobiota of Flabellia petiolata: first survey of marine fungi associated to a Mediterranean green alga. PLoS ONE 12:e017594. doi: 10.1371/journal.pone.0175941
Gordon, J. L., Brown, M. A., and Reynolds, M. (2018). Cell-based methods for determination of efficacy for candidate therapeutics in the clinical management of cancer. Diseases 6, 85–98. doi: 10.3390/diseases6040085
Greve, H., Ietidal, E., Alexander, M., Stefan, K., Harald, G., and Gabriele, M. K. (2010). Fungal metabolites: structural diversity as incentive for anticancer drug development. Phytochem. Rev. 9, 537–545. doi: 10.1007/s11101-010-9198-5
Guo, L., Xie, M. Y., Yan, A. P., Wan, Y. Q., and Wu, Y. M. (2006). Simultaneous determination of five synthetic antioxidants in edible vegetable oil by GC–MS. Analytic. and Bioanalytic. Chem. 386:1881. doi: 10.1007/s00216-006-0738-1
Hafedh, H., Snoussi, M., Jannet, H. B., and Mighri, Z. (2008). Comparison of chemical composition and antimicrobial activities of Mentha longifolia L. ssp. longifolia essential oil from two Tunisian localities (Gabes and Sidi Bouzid). Ann. Microbial. 58, 513–520. doi: 10.1007/BF03175551
Hussain, M. S., Fareed, S., Ansari, S., Rahman, M. A., Ahmad, I. Z., and Saeed, M. (2012). Current approaches toward production of secondary plant metabolites. J. Pharm. Bioall. Sci. 4:10. doi: 10.4103/0975-7406.92725
Jensen, P. R., and Fenical, W. (1994). Strategies for the discovery of secondary metabolites from marine bacteria: ecological perspectives. Ann. Rev. Microbiol. 48, 559–584. doi: 10.1146/annurev.mi.48.100194.003015
Kuete, V., Karaosmanoğlu, O., and Sivas, H. (2017). “Anticancer activities of African medicinal spices and vegetables,” in Medicinal Spices and Vegetables From Africa, ed V. Kuete (Academic Press), 271–297.
Kumari, M., Shukla, S., Pandey, S., Giri, V. P., Bhatia, A., Tripathi, T., et al. (2017). Enhanced cellular internalization: a bactericidal mechanism more relative to biogenic nanoparticles than chemical counterparts. ACS Appl. Mat. Interfaces 9, 4519–4533. doi: 10.1021/acsami.6b15473
Kumari, M., Taritla, S., Sharma, S., and Jayabaskaran, C. (2018). Antiproliferative and antioxidative bioactive compounds in extracts of marine-derived endophytic fungus Talaromyces purpureogenus. Front. Microbiol. 9:1777. doi: 10.3389/fmicb.2018.01777
Lauritano, C., Andersen, J. H., Hansen, E., Albrigtsen, M., Escalera, L., Esposito, F., et al. (2016). Bioactivity screening of microalgae for antioxidant, anti-Inflammatory, anticancer, anti-diabetes, and antibacterial activities. Front. Mar. Sci. 3, 1–12. doi: 10.3389/fmars.2016.00068
Leman-Loubière, C., Goffg, L., Debitus, C., and Ouazzani, J. (2017). Sporochartines A–E, a new family of natural products from the marine fungus Hypoxylon monticulosum isolated from a Sphaerocladina sponge. Front. Mar. Sci. 4, 1–9. doi: 10.3389/fmars.2017.00399
Leylaie, S., and Zafari, D. (2018). Antiproliferative and Antimicrobial activities of secondary metabolites and phylogenetic study of endophytic Trichoderma species from vinca plants. Front. Microbiol. 9:1484. doi: 10.3389/fmicb.2018.01484
Li, H., Li, X. M., Li, X., Wang, C. Y., Liu, H., Kassack, M. U., et al. (2017). Antioxidant hydroanthraquinones from the marine algal-derived endophytic fungus Talaromyces islandicus en-501. J. Nat. Prod. 801, 162–168. doi: 10.1021/acs.jnatprod.6b00797
Liang, X., Zhang, X., Nong, X., Wang, J., Huwang, Z., and Qi, S.-H. (2016). Eight linear peptides from the deep-sea-derived fungus Simplicillium obclavatum EIODSF 020. Tetrahedron 72, 3092–3097. doi: 10.1016/j.tet.2016.04.032
Mann, J. (2002). Natural products in cancer chemotherapy: past, present and future. Nat. Rev. Cancer 2, 143–148. doi: 10.1038/nrc723
Marris, E. (2006). Marine natural products: drugs from the deep. Nature 443, 904–905. doi: 10.1038/443904a
Meng, L., Mándi, A., Li, X., and Du, F. (2014). Penicibilaenes A and B, sesquiterpenes with a tricyclo[6.3.1.01,5]dodecane skeleton from the marine isolate of Penicillium bilaiae MA-267. Org. Lett. 1623, 6052–6055. doi: 10.1021/ol503046u
Mohyeldin, M. M., Akl, M. R., Siddique, A. B., Hassan, H. M., and El Sayed, K. A. (2017). The marine-derived pachycladin diterpenoids as novel inhibitors of wild-type and mutant EGFR. Biochem. Pharmacol. 126, 51–68. doi: 10.1016/j.bcp.2016.12.003
Monggoot, S., Pichaitam, T., Tanapichatsakul, C., and Pripdeevech, P. (2018). Antibacterial potential of secondary metabolites produced by Aspergillus sp., an endophyte of Mitrephora wangii. Arch. Microbiol. 200, 951–959. doi: 10.1007/s00203-018-1511-5
Nagabhishek, S. N., and Madankumar, A. (2019). A novel apoptosis-inducing metabolite isolated from marine sponge symbiont Monascus sp. NMK7 attenuates cell proliferation, migration and ROS stress-mediated apoptosis in breast cancer cells. RSC Adv. 9:5878. doi: 10.1039/C8RA09886G
Nagarajan, K. (2014). History of natural products chemistry in India. Ind. J. His. Sci. 49, 377–398.
Noolvi, M., Agrawal, S., Patel, H., Badiger, A., Gaba, M., and Zambre, A. (2014). Synthesis, antimicrobial and cytotoxic activity of novel azetidine-2-one derivatives of 1H-benzimidazole. Arabian J. Chem. 7, 219–226. doi: 10.1016/j.arabjc.2011.02.011
Pereira, N., and Almeida, M. R. (2014). A preliminary checklist of marine algae from the Coast of Goa. Ind. J. Geo Mar. Sci. 43, 665–666.
Pozarowski, P., and Darzynkiewicz, Z. (2004). “Analysis of cell cycle by flow cytometry,” in Checkpoint Controls and Cancer, Vol. 281. eds A. H. Schönthal (Humana Press), 301–311.
Punnam, C. M., Pillai, C. R., Sunish, I. P., and Vijayachari, P. (2016). Antimicrobial and antimalarial properties of medicinal plants used by the indigenous tribes of Andaman and Nicobar Islands, India. Microb. Pathogen. 96, 85–88. doi: 10.1016/j.micpath.2016.04.017
Rastogi, R. P., Singh, S. P., Häder, D. P., and Sinha, R. P. (2010). Detection of reactive oxygen species (ROS) in cyanobacteria using the oxidant-sensing probe 2', 7'-dichlorodihydrofluorescein diacetate (DCFH-DA). Biochem. Biophys. Res. Commun. 397, 603–607. doi: 10.1016/j.bbrc.2010.06.006
Razak, N. A., Abu, N., Ho, W. Y., Zamberi, N. R., Tan, S.W., Alitheen, N. B., et al. (2019). Cytotoxicity of eupatorin in MCF-7 and MDA-MB-231 human breast cancer cells via cell cycle arrest, anti-angiogenesis and induction of apoptosis. Sci. Rep. 9:1514. doi: 10.1038/s41598-018-37796-w
Reed, J. C. (2000). Mechanisms of apoptosis. Am. J. Pathol. 57, 1415–1430. doi: 10.1016/S0002-9440(10)64779-7
Ruiz, B., Chávez, A., Forero, A., García-Huante, Y., Romero, A., Sánchez, M., et al. (2010). Production of microbial secondary metabolites: regulation by the carbon source. Crit. Rev. Microbiol. 36, 46–167. doi: 10.3109/10408410903489576
Sarasan, M., Puthumana, J., Job, N., Han, J., Lee, J. S., and Philip, R. (2017). Marine algicolous endophytic fungi - a promising drug resource of the era. J. Microbiol. Biotechnol. 27, 1039–1052. doi: 10.4014/jmb.1701.01036
Shannon, E., and Abu-Ghannam, N. (2016). Antibacterial derivatives of marine algae: an overview of pharmacological mechanisms and applications. Mar. Drugs 14:81. doi: 10.3390/md14040081
Simmons, T. L., Andrianasolo, E., McPhail, K., Flatt, P., and Gerwick, W. H. (2005). Marine natural products as anticancer drugs. Mol. Can. Therap. 4, 333–342.
Suffness, M., and Pezzuto, J. M. (1990). Methods in Plant Biochemistry: Assays for Bioactivity. London: Academic Press.
Tan, X., Zhou, Y. Q., Zhou, X. L., Xia, X. H., Wei, Y., He, L. L., et al. (2018). Diversity and bioactive potential of culturable fungal endophytes of Dysosma versipellis; a rare medicinal plant endemic to China. Sci. Rep. 8:5929. doi: 10.1038/s41598-018-31009-0
Wijesekara, I., Hang, C., Van, T. Q., Vo, T. S., Li, Y. X., and Kim, S. K. (2014). Physcion from marine-derived fungus Microsporum sp. induces apoptosis in human cervical carcinoma HeLa cells. Microbiol. Res. 169, 255–261. doi: 10.1016/j.micres.2013.09.001
Xu, R., Xu, G., Li, X., Li, C., and Wang, B. (2015). Characterization of a newly isolated marine fungus Aspergillus dimorphicus for optimized production of the anti-tumor agent Wentilactones. Mar. Drugs 13, 7040–7054. doi: 10.3390/md13117040
Zhang, Q., Li, H. Q., Zong, S. C., Gao, J. M., and Zhang, A. L. (2012). Chemical and bioactive diversities of the genus Chaetomium secondary metabolites. Mini Rev. Med. Chem. 12, 127–148. doi: 10.2174/138955712798995066
Zhang, Z., Miao, L., Lv, C., Sun, H., Wei, S., Wang, B., et al. (2013). Wentilactone B induces G2/M phase arrest and apoptosis via the Ras/Raf/MAPK signaling pathway in human hepatoma SMMC-7721 cells. Cell Death Dis. 4:e657. doi: 10.1038/cddis.2013.182
Keywords: Konkan coast, fungal endophyte, diversity, cytotoxicity, apoptosis
Citation: Kamat S, Kumari M, Taritla S and Jayabaskaran C (2020) Endophytic Fungi of Marine Alga From Konkan Coast, India—A Rich Source of Bioactive Material. Front. Mar. Sci. 7:31. doi: 10.3389/fmars.2020.00031
Received: 05 November 2019; Accepted: 20 January 2020;
Published: 06 February 2020.
Edited by:
Chiara Lauritano, Stazione Zoologica Anton Dohrn, ItalyReviewed by:
Perumal Karthick, Sea6 Energy Pvt Ltd, IndiaCopyright © 2020 Kamat, Kumari, Taritla and Jayabaskaran. This is an open-access article distributed under the terms of the Creative Commons Attribution License (CC BY). The use, distribution or reproduction in other forums is permitted, provided the original author(s) and the copyright owner(s) are credited and that the original publication in this journal is cited, in accordance with accepted academic practice. No use, distribution or reproduction is permitted which does not comply with these terms.
*Correspondence: C. Jayabaskaran, Y2piQGlpc2MuYWMuaW4=
Disclaimer: All claims expressed in this article are solely those of the authors and do not necessarily represent those of their affiliated organizations, or those of the publisher, the editors and the reviewers. Any product that may be evaluated in this article or claim that may be made by its manufacturer is not guaranteed or endorsed by the publisher.
Research integrity at Frontiers
Learn more about the work of our research integrity team to safeguard the quality of each article we publish.