- 1Greenland Institute of Natural Resources, Nuuk, Greenland
- 2Greenland Climate Research Centre, Greenland Institute of Natural Resources, Nuuk, Greenland
- 3Department of Physics and Astronomy, Aarhus University, Aarhus, Denmark
To maintain a sustainable harvest of marine mammals, knowledge on key life history parameters such as age is essential. In West Greenland, humpback whale numbers were low during the 1980s due to past commercial whaling. However, the moratorium, which was enforced in 1986, had a positive effect on their abundance and by 2010 it was possible for Greenland to reopen the subsistence hunt on humpback whales in West Greenland. In this study, eyes were collected from 12 humpback whales taken in the subsistence hunt from 2010 to 2015 and used for age and growth estimation. Aspartic acid racemization (AAR) and bomb radiocarbon dating techniques were performed on the eye lens nuclei to obtain independent age estimates. Through AAR, 11 individuals were estimated to be younger than 20 years old and a single individual estimated to be 46 years old. Radiocarbon dating supported these estimates as all individuals had post-bomb pMC values. The Gompertz growth curve showed that humpback whales reach their asymptotic lengths around age ten and hence at a relatively young age. In conclusion, half a century after commercial whaling ended, the humpback whales feeding off West Greenland appear to consist mainly of young individuals. An age distribution that is likely attributed to a historical overexploitation and since a rapid increase in abundance following the moratorium.
Introduction
To decrease the risk of unsustainable harvest of marine mammals, there is a need to obtain knowledge on key life history parameters. This includes age and derived attributes such as longevity, age at sexual maturity, fertility peak and age distribution within a population (e.g., Barlow and Clapham, 1997; Punt et al., 2006; Nielsen et al., 2013).
The large whales of the North Atlantic were subject to an intense hunt for centuries. This was also the case for the humpback whale (Megaptera novaeangliae) until commercial whaling on the species ended during the 1960’s. In Greenland, the subsistence hunt for humpback whales persisted for another two decades (Martin et al., 1984; Stevick et al., 2003). In 1986, a moratorium was, however, enforced by the International Whaling Commission (IWC) and the quota was reduced to zero (IWC, 1986). At this time, the humpback whales feeding off West Greenland had declined dramatically, and was down to less than 200 individuals when the moratorium was implemented (Perkins et al., 1984). The following years with no hunt led to an increase in abundance and Heide-Jørgensen et al. (2012) estimated the annual rate of increase in West Greenland humpbacks to be 9.4% year–1 for the period 1984–2007. By 2007, the stock was estimated to more than 2,700 individuals (Heide-Jørgensen and Laidre, 2015). With the increase in numbers followed a reopening in 2010 of the subsistence hunt on humpback whales in West Greenland and hunters have for the last 10 years been allowed to harvest approximately ten individuals annually.
Throughout time, several methods have been applied to investigate the age of large cetaceans. For toothed whales annually deposited growth bands or growth layer groups (GLGs) counted in the hard dentine structure have been used to estimate age (e.g., Grue and Jensen, 1979; Evans et al., 2002). For baleen whales, GLGs counted in tissues such as the earplug, the baleen, or the ear bone have been used to produce age estimates (Konrádsson and Sigurjónsson, 1989; Lubetkin et al., 2008; Nielsen et al., 2013). However, for large baleen whales, these tissues are often difficult to access. Furthermore, counting GLG layers in e.g., ear plugs becomes increasingly difficult when dealing with older individuals and enhances the chance of unreliable age estimates (Lockyer, 1974). Therefore, alternative aging techniques based on more easy-to-attain tissues have been developed and encompass fatty acid analysis (Herman et al., 2009), epigenetic analysis based on methylation of specific age-related genes (Polanowski et al., 2014; Riekkola et al., 2018), bomb radiocarbon dating (Stewart et al., 2006), and aspartic acid racemization (AAR) on metabolic inert tissues such as eye lens nuclei obtained from post-mortem specimens (Bada et al., 1980; George et al., 1999).
The eye lens nucleus is composed of metabolically inert crystalline proteins, and the nucleus center is formed during embryonic development (Lynnerup et al., 2008; Bassnett et al., 2011). Therefore, the center of the eye lens nucleus retains proteins synthetized at approximately age 0 – a feature which has been utilized in several aging studies using AAR or bomb radiocarbon of difficult-to-age marine vertebrates, such as bowhead whales (Balaena mysticetus), narwhals (Monodon monoceros) and the Greenland shark (Somniosus microcephalus) (George et al., 1999; Garde et al., 2007; Nielsen et al., 2016). The AAR technique utilizes that the D isomer of aspartic acid in metabolically inactive tissue accumulate over time as L-aspartic acids transform into D-aspartic acid at a constant rate (Masters et al., 1977). This rate is species specific and depends largely on body temperature (Bada and Schroeder, 1975; Rosa et al., 2013; Garde et al., 2018). Eye lenses have previously been used when applying the AAR on baleen whales such as bowhead whales (Balaena mysticetus; George et al., 1999; Rosa et al., 2013), fin whales (Balaenoptera physalus; Nielsen et al., 2013) and minke whales (Balaenoptera acutorostrata; Olsen and Sunde, 2002). Bomb radiocarbon dating has been used in age determination and particularly age validation of beluga whale (Delphinapterus leucas; Stewart et al., 2006) as well as multiple shark species (Campana et al., 2002; Hamady et al., 2014; Nielsen et al., 2016). Bomb radiocarbon dating is based on the abrupt increase in the atmospheric 14C content following thermonuclear testing in the late 1950’s (De Vries, 1958). This shift quickly propagated through food webs (i.e., “Bomb pulse”), including marine systems of the North Atlantic Ocean (Campana et al., 2002; Scourse et al., 2012). The bomb pulse is nowadays established as a chemical time mark in carbon based time series of the marine environment (Kalish, 1993; Campana et al., 2002), meaning that tissue from for example eye lenses, can be dated as of either pre- or post-bomb origin (Nielsen, 2018).
The subsistence hunt on West Greenland humpback whales offers a unique opportunity to sample hunted individuals and gain knowledge on age and growth of the animals. In this study, we use AAR and bomb radiocarbon in combination on tissue obtained from the eye lens nucleus to attain age estimates of West Greenland humpback whales. These two methods have never been used on humpback whales nor combined for validation and age estimates of the West Greenland feeding aggregation is non-existent. This study gives a first insight on the age profile of the West Greenland stock half a century post commercial whaling.
Materials and Methods
Sampling
Eyes for age estimation using AAR and bomb radiocarbon technique were collected from 12 humpback whales taken in the subsistence hunt in West Greenland (Figure 1). All individuals were caught in the period September 2010 – April 2015 (Table 1). Eight whales were taken by hunters off Nuuk, two were taken near Paamiut south of Nuuk and two in the Disko Bay area (one from Qeqertarsuaq and one from Ilulissat). Nine whales were females and three were males. The eyes were either removed by the researchers if present in the area or by the hunters themselves and the eyes were then frozen at −20°C as soon as possible. Both eyes were collected from seven of the whales and one eye collected from five whales. Previous studies have shown that there is no significant difference in D/L measurements from the right and the left eye (Garde et al., 2007, 2018) and a random eye was therefore used for the AAR and bomb radiocarbon age estimations. The remaining eyes were stored in a −20°C freezer. Body lengths for 10 of the 12 whales were measured by the hunters in the field. One whale (Mn_007) was measured by the authors.
Eye Lens Dissection
In the laboratory, eye lenses were dissected out of the eyes. The outer lens layers were removed by rolling the lens on paper, and any remaining layers were removed under a stereoscope leaving only the lens nucleus for hydrolysis and subsequent high-performance liquid chromatography (HPLC) analysis as described in detail in Garde et al. (2007, 2012). The lens nuclei were parted in two – one half was used for analyses and the other half was archived at −20°C.
Aspartic Acid Racemization
Hydrolysis and High-Performance Liquid Chromatography Analysis
Procedures of Zhao and Bada (1995) and Garde et al. (2007, 2010) were followed for hydrolysis of samples and analysis by HPLC. Eye lens nuclei were hydrolyzed in glass tubes containing 1 ml 6 M HCl for 6 h at 100°C. Chromatography and data analysis was performed using an Agilent 1100 Series HPLC system (Agilent Technologies, Walbronn, Germany). Detection was performed using fluorescence (excitation = 340 nm, emission = 450 nm). The column was a Zorbax Eclipse XDB-C18, 4.6 150 mm, with particle size 3.5 μm.
The D/L ratios measured by HPLC were calibrated using the following D/L standards: 0.5/99.5, 1/99, 2/98, 5/95, 10/90, and 15/85, which were run at the beginning and end of the HPLC run. Measured D/L ratios from the eye lens nuclei were recalculated using calibration equations (linear regression) for the D/L standards. Two standard curves were produced using D/L standards as described above. Linear regression equations for each run were calculated by regression of theoretical D/L ratios versus the measured D/L ratios from the D/L standards. The coefficient was r2 = 1 for each run. The equation with the slope closest to 1 (range: 1.1404–1.1418) was used to recalculate D/L ratios for the samples.
Aspartic Acid Racemization Age Estimation
Humpback whale ages were estimated using the AAR age equation (1) derived for fin whales using GLG counts from earplugs from 15 fin whales and 15 fin whale fetuses (0 year) (Nielsen et al., 2013).
Where A is age (years) and X is ln[(1 + D/L)/(1 − D/L)]. This approach by Ohtani and Yamamoto (2011) has previously been used for AAR age estimation of marine and other mammals (e.g., Garde et al., 2015, 2018).
Standard errors on age estimates was done following Nielsen et al. (2013), where uncertainty of the earplug GLG counts was evaluated for each animal (between ± 1 and ± 3 year) and the standard error of A was calculated as SE = √(938.1(X-0.05749)∧2 + 57.70).
A Gompertz growth function was fitted to the data using equation (2):
where a defines the maximum of the curve (i.e., maximum length), μ defines the maximum slope, λ is related to the lag-phase (e.g., the location of the maximum slope along the time axis). Model uncertainty was estimated by 95% confidence interval, which were added to the growth curve using simulations from the R package “propagate.”
Radiocarbon Dating
The preparation procedure follows that described in Nielsen et al. (2016), and the 14C content is reported as percentage Modern Carbon (pMC). A pMC value of 95 was used as the criteria separating individuals born before (<95 pMC) or after (>95 pMC) the bomb pulse (Scourse et al., 2012). In organically based radiocarbon chronologies representing continental shelf food webs of the northern North Atlantic, the bomb pulse onset (i.e., when bomb-produced radiocarbon becomes detectable in a chronology) is no later than 1963 (e.g., Scourse et al., 2012; Nielsen et al., 2016).
Sighting History
Two individuals, Mn006 and Mn009, has a sighting history in the waters outside Nuuk where they were caught in 2013. Mn006 was first registered in 2006 and Mn009 was first registered in 2004. At the time of registration both whales were solitaire and independent. Hence, Mn006 was at least 8 years when caught in 2013 and Mn009 at least 10 years old.
Results
Aspartic acid racemization ages for the 12 humpback whales and their associated SE’s are listed in Table 1 along with pMC values derived from radiocarbon dating. Based on AAR, five of the humpback whales were between 0 and 9 years, six between 10 and 19 years and one was approximately 46 years. The eye lens of this older individual was yellow and hard, which is a sign of old age, compared to the other more soft and clear or milky colored lenses, typically of younger individuals. The Gompertz growth parameters were (estimate ± SE): α = 12.46 ± 0.52; μ = 3.08 ± 0.85 and λ = −1.40 ± 0.38 and the asymptotic length (α) was reached at approximately age 10 (Figure 2).
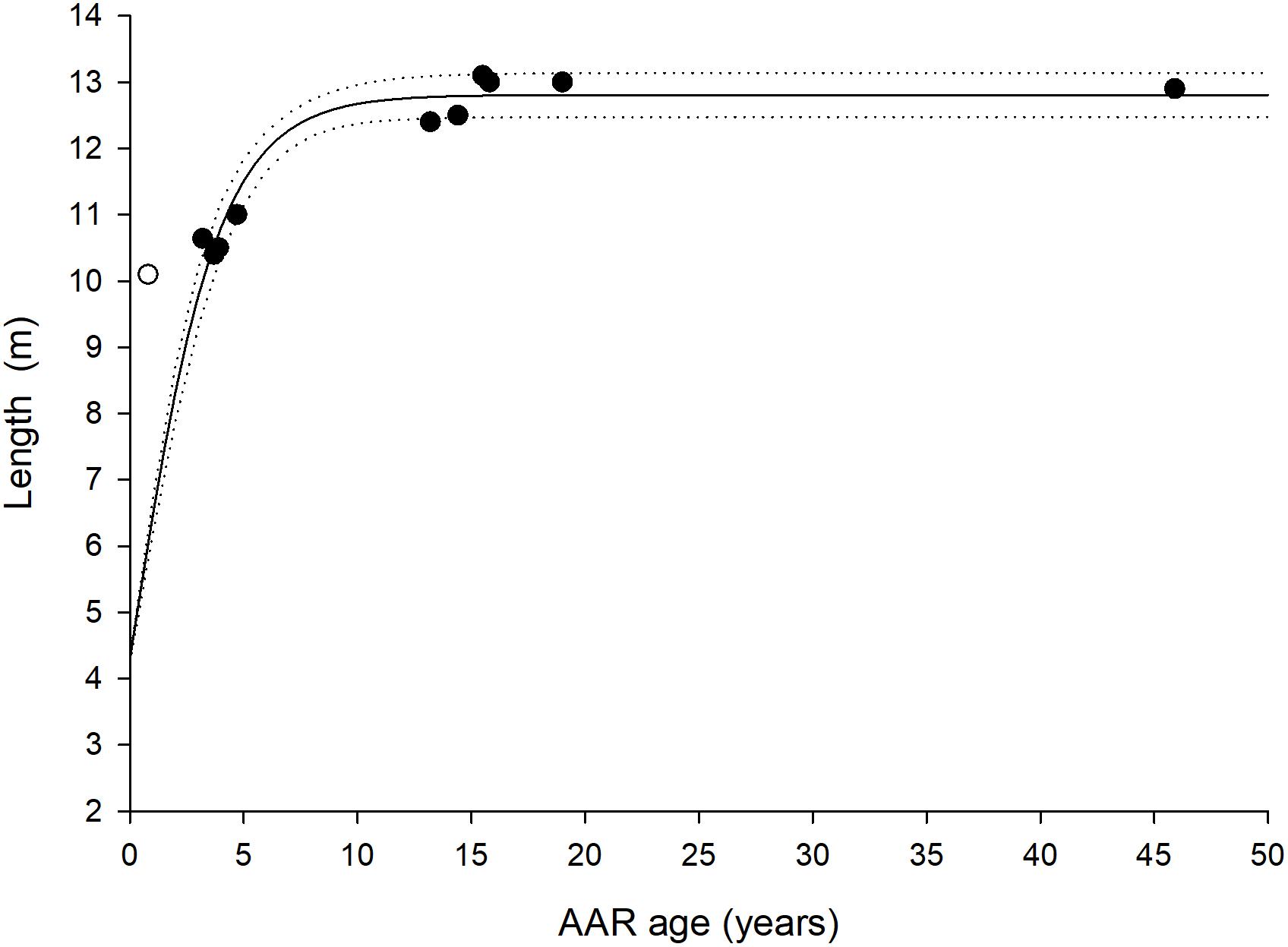
Figure 2. Age and length relation of the 11 humpback whales of know size (black circles). The solid line is a Gompertz growth curve fitted to the data and dashed lines indicate 95% confidence intervals. The white circle represents whale ID Mn002 where there is uncertainty in regards to the registered length. A birth length (i.e., intercept) of 4.3 m is assumed (Chittleborough, 1965).
The radiocarbon dating results showed that all individuals had post-bomb pMC values above 95 and varied between 103 and 106 (Table 1). Hence, all individuals were estimated to have been born after the bomb pulse (i.e., born after 1963). There was no trend in pMC values with length.
Discussion
The aspartic acid racemization age estimates showed that 11 of 12 individuals were younger than 20 years old while one individual was 46 years. These age estimates were supported by bomb radiocarbon dating finding all animals to be of post-bomb pulse origin. Humpback whales likely attain sexual maturity around age nine and typically produce one offspring every other year or third year with a gestation time of approximately 1 year (Chittleborough, 1958; Zerbini et al., 2010). The maximum life span of humpback whales is unknown. The oldest individual in a study by Riekkola et al. (2018) based on epigenetic aging was estimated to be 67 years old. Gabriele et al. (2010) estimated a humpback whale to be 45 years based on earplug growth layers and hence similar to the oldest individual in this study. However, other Balaenopteridae, their closest relatives (e.g., fin whales), have estimated ages above 100 years old (Mizroch, 1981; Nielsen et al., 2013) and we would expect humpback whales to have similar potential longevity. Also, humpback whales have very low adult mortality with an estimated annual survival rate of 96% (Larsen and Hammond, 2004). As previously noted the humpback whale abundance in West Greenland increased 10 fold from 200 in the early 80’s to 2,700 by 2007 (Whitehead et al., 1983; Perkins et al., 1984; Heide-Jørgensen and Laidre, 2015). With a rapid increase in population size seeded by a relatively small population we could expect the many years of overexploitation to have skewed the age distribution toward younger individuals. This is further supported by Taylor et al. (2007). They calculated the generation time (the average age of parents to newborns within the same year) of North Atlantic humpback whales, based on life history parameters, to be 14.5 years at present date contrary to 21.5 years pre-whaling. Similar age distribution as seen in present study have been found for humpback whales on the Southern Ocean feeding grounds which were also historically overexploited by commercial whalers (Riekkola et al., 2018). Riekkola et al. (2018) found a majority of younger individuals in the Southern Ocean and they estimated average age to be 14 years similar to our 12.5 years. They argue that the age distribution of humpbacks in the Southern Ocean is attributed to historical whaling as they compare their findings of a current young age distribution to an older estimated pre-whaling age distributions for the area. We have not come across pre-whaling age distributions for the West Greenland humpback whales. Yet, our results, bearing in mind the small sample size, show that the majority of the individuals appear to be relatively young and this could indicate a population in West Greenland that has not yet returned to a pre-whaling age structure.
Alternatively, the samples could be skewed toward younger individuals due to selective hunting. However, nothing indicates that hunters specifically target smaller (i.e., younger) individuals. Smaller individuals are easier to handle but larger individuals are worth more as the yield in meat is larger. Yet, it is more important to be close to a flensing area to assure a quick flensing process as the whales are hunted from small vessels that are unable to process the catch on board. This makes the hunt more opportunistic, simply targeting individuals in the vicinity. Also, in many cases only a single individual may be present and the hunters will target that individual. By law, hunters are not allowed to take a mother with a calf and vice versa. This should have little effect on this study, as humpback whale calves usually only follow their mother during most of their first year (Weinrich, 1993).
The two different aging techniques provide similar conclusions with none of the whales estimated to have been born before 1963. Also, the two individuals, which had a sighting history and were known to be older than at least eight and 10 years, were estimated by AAR to be older than these given ages (9.6 ± 7.6 and 15.5 ± 7.6 years, respectively). This further supports the estimated AAR ages. Naturally, having an individual that was estimated to have been born before 1963 would have added even more support to the aging techniques, and additional sampling is encouraged. Additional sampling on both young and old individuals would also strengthen the estimated growth curve.
One individual has an AAR age of less than a year (Table 1 and Figure 2). However the given whale has a noted length of 10.10 m which does not align with a whale within its natal year according to both present and previously published growth curves for humpback whales (Stevick, 1999). Humpback whales are only around 4.3 m in length at birth (Chittleborough, 1965) and it is unlikely that the whale will grow additional 6 m in length within its natal year. Hence, we must assume that the whale is either older than estimated or smaller than reported by the hunters. The estimated ages of similar sized whales cluster around 3–4 years, and the estimate of 0.8 ± 7.6 years and 10.10 m appears to be an outlier. The lengths of the whales were in all but one occasion measured by the hunters in the field. Lengths have not been verified by the researchers and we speculate that this particular measurement has been reported incorrectly. Yet, the AAR method is more reliable for older than for younger individuals (George et al., 1999; Garde et al., 2010, 2018) probably as a consequence of the eye lens not being fully developed upon birth and continue to grow for a period postnatal (Heinämäki and Lindfors, 1988; Garde et al., 2018). This could be the case here.
There are no published AAR racemization rates nor (D/L)0 values specific for the humpback whale and in this study it was not possible to estimate a humpback whale specific racemization rate or (D/L)0 value. Estimation of species-specific racemization rates requires samples covering a range of known ages including near-term fetuses or postpartum individuals. For this study neither earplugs nor baleen plates were collected for age estimation. Previous studies have shown that body temperature is the main driver of the racemization process (Rosa et al., 2013; Garde et al., 2018). The humpback whale has a body temperature of 36.0°C (Morrison, 1962) which is similar to that of 36.1°C for fin whales (Balaenoptera physalus) (Brodie and Paasche, 1985). Even though it is recommended to use values of other species with care (Garde et al., 2018), we here chose to use age equation (1) and a SE equation developed based on GLG count from fin whale earplugs (Nielsen et al., 2013). We chose this considering the similarities in body temperature, biology and ecological life style of the two species but also acknowledge that there is a chance of introducing unwanted errors to the age estimates.
Proteins of the eye lens are of dietary origin and hence, the isotopic profile of the embryonic nucleus represent the food web of the mother during fetal development (Nielsen et al., 2016). To convert the radiocarbon levels of the eye lens nucleus into age estimates, it is crucial knowing the timing of the bomb pulse in the environment. Reference chronologies of both organic and inorganic origin have shown that the bomb pulse penetrated into the North Atlantic food webs no later than early 1960s (Campana et al., 2002; Scourse et al., 2012; Hamady et al., 2014; Nielsen et al., 2016). Humpback whales in West Greenland feed in the pelagic food web on prey such as capelin (Mallotus villosus) and euphausiids (Larsen and Hammond, 2004) at depths down to 260 m (Simon et al., 2012). Although no reference chronologies, specific for pelagic food webs of West Greenland, have been established, it is a fair assumption that the bomb pulse penetrated into this environment similar to other regions of the northern North Atlantic and Arctic – a crucial assumption which also has been applied by Nielsen et al. (2016). This assumption implies that samples of post-bomb origin are from humpbacks born later than 1963, whereas pre-bomb samples must be from earlier than 1963. However, because the exact response to the bomb pulse (i.e., the amplitude of change) and the pMC trend in the decades following the bomb pulse appears to be highly varying across the Atlantic (Nielsen, 2018) it is not possible to derive an exact age of individuals born after 1963. The very distinct bomb pulse timing in combination with AAR age estimation provides a valuable tool for age validation in difficult-to-age animals such as long-lived baleen whales and suggests that the AAR age provided are not underestimated. If so, the oldest individual of 46 years, would likely have had pre-bomb pMC values. Clearly, more future samples are wanted to support this finding.
From a management perspective, it is the goal to minimize the effect of the subsistence hunting on the feeding aggregation. However, as the humpback whales obtain their maximum size at a relatively young age (10 years) compared to expected longevity, it is not practically possible to use length as a proxy for age. Rather, if managers want to harvest selectively, compared to the expected life span and age at maturity, one suggestion could be to develop and maintain a catalog of individual whales and from that, select individuals that can be harvested. Such a catalog could be a combination of easily sampled and analyzed photos (Boye et al., 2014) and a concurrent genetic sampling that could possibly allow for aging of live individuals (Riekkola et al., 2018). The combination of all techniques on both live and dead individuals will validate the age estimation and inform management. Such considerations could further take into account that individual humpback whales seem to have a high degree of site fidelity in some areas, like Nuup Kangerlua, the Nuuk fjord system (Boye et al., 2010, 2014). In this way, the ecosystem services provided by the whales could be optimized on several parameters.
Half a century post commercial whaling, this study gives an insight on the age distribution on West Greenland humpback whales. Bomb radiocarbon supports the ages found through AAR and depicts a feeding aggregation skewed toward younger ages. A distribution that is likely caused by a rapid increase in population size following depletion after many years of hunt.
Data Availability Statement
All datasets generated for this study are included in the article/supplementary material.
Ethics Statement
Samples in this study were exported from Greenland to Denmark for analysis under the Cites science permit number 16GL1167034.
Author Contributions
MS, TB, and RH conceived the study. MS and TB sampled/facilitated the sampling of eyes and the corresponding hunting data. JO was responsible for the bomb radiocarbon dating. JN and EG conducted all laboratory work and age calculations. RH and TB conducted the age and growth analysis. TB drafted the manuscript with contributions from all authors.
Funding
Aspartic acid racemization and radiocarbon dating analysis were funded by the Danish Ministry of Higher Education and Science.
Conflict of Interest
The authors declare that the research was conducted in the absence of any commercial or financial relationships that could be construed as a potential conflict of interest.
Acknowledgments
We thank the hunters in West Greenland for sampling and assistance of sampling of their hunt, and laboratory coordinator Kirsten Andersen from the Department of Pharmacy at the University of Copenhagen, for her technical assistance in relation to AAR measurements. We also thank senior scientist Lars Witting from the Greenland Institute of Natural Resources for a constructive discussion on age distribution in humpback whales, and Peter Hegelund and Carl “Bror” Isaksen for their assistance in sampling.
References
Bada, J. L., Brown, S., and Maters, P. M. (1980). Age determination of marine mammals based on aspartic acid racemization in the teeth and lens nucleus. Rep. Int. Whal. Comm. Special Issue 3, 113–118.
Bada, J. L., and Schroeder, A. R. (1975). Amino acid racemization reactions and their geo-chemical implications. Naturwissenschaften 62, 71–79. doi: 10.1007/bf00592179
Barlow, J., and Clapham, P. J. (1997). A new birth-interval approach to estimating demographic parameters of humpback whales. Ecology 78, 535–546. doi: 10.1890/0012-9658(1997)078
Bassnett, S., Shi, Y., and Vrensen, G. F. J. M. (2011). Biological glass: structural determinants of eye lens transparency. Philos. Trans. R. Soc. Lond. B Biol. Sci. 366, 1250–1264. doi: 10.1098/rstb.2010.0302
Boye, T. K., Simon, M., and Madsen, P. T. (2010). Habitat use of humpback whales in Godthaabsfjord, West Greenland, with implications for commercial exploitation. J. Mar. Biol. Assoc. U.K. 90, 1529–1538. doi: 10.1017/S0025315410000755
Boye, T. K., Simon, M., and Witting, L. (2014). How may an annual removal of humpback whales from Godthaabsfjord, West Greenland, affect the within-fjord sighting rate? J. Cetacean Res. Manag. 14, 51–56.
Brodie, P., and Paasche, A. (1985). Thermoregulation and energetics of fin and sei whales based on postmortem, stratified temperature measurements. Can. J. Zool. 63, 2267–2269. doi: 10.1139/z85-336
Campana, S. E., Natanson, L. J., and Myklevoll, S. (2002). Bomb dating and age determination of large pelagic sharks. Can. J. Fish. Aquat. Sci. 59, 450–455. doi: 10.1139/f02-027
Chittleborough, R. G. (1958). The breeding cycle of the female humpback whale Megaptera nodosa (Bonnaterre). Aust. J. Mar. Freshw. Res. 9, 1–18. doi: 10.1071/MF9580001
Chittleborough, R. G. (1965). Dynamics of two populations of the humpback whale, Megaptera novaeangliae (Borowski). Aust. J. Mar. Freshw. Res. 16, 33–128.
De Vries, H. (1958). Atomic bomb effect: variation of radiocarbon in plants, shells, and snails in the past 4 years. Sicence 128, 250–251. doi: 10.1126/science.128.3318.250
Evans, K., Hindell, M. A., Robertson, K., Lockyer, C., and Rice, D. (2002). Factors affecting the precision of age determination of sperm whales (Physeter macrocephalus). J. Cetacean Res. Manag. 4, 193–201.
Gabriele, C. M., Lockyer, C., Straley, J. M., Jurasz, C. M., and Kato, H. (2010). Sighting history of a naturally marked humpback whale (Megaptera novaeangliae) suggests ear plug growth layer groups are deposited annually. Mar. Mamma. Sci. 26, 443–450. doi: 10.1111/j.1748-7692.2009.00341.x
Garde, E., Bertelsen, M. F., Ditlevsen, S., Heide-Jørgensen, M. P., Nielsen, N. H., Frie, A. K., et al. (2018). Accuracy of the aspartic acid racemization technique in age estimation of mammals and the influence of body temperature. NAMMCO Sci. Publ. 10, 1–22. doi: 10.7557/3.4400
Garde, E., Frie, A. K., Dunshea, G., Hansen, S. H., Kovacs, K. M., and Lydersen, C. (2010). Harp seal ageing techniques - teeth, aspartic acid racemization, and telomere sequence analysis. J. Mammal. 91, 1365–1374. doi: 10.1644/10-MAMM-A-080.1
Garde, E., Hansen, S. H., Ditlevsen, S., Tvermosegaard, K. B., Hansen, J., Harding, K. C., et al. (2015). Life history parameters of narwhals (Monodon monoceros) from Greenland. J. Mammal. 96, 866–879. doi: 10.1093/jmammal/gyv110
Garde, E., Heide-Jørgensen, M. P., Ditlevsen, S., and Hansen, S. H. (2012). Aspartic acid racemization rate in narwhal (Monodon monoceros) eye lens nuclei estimated by counting of growth layers in tusks. Polar Res. 31:15865. doi: 10.3402/polar.v31i0.15865
Garde, E., Heide-Jørgensen, M. P., Hansen, S. H., Nachman, G., and Forchammer, M. C. (2007). Age-specific growth and remarkable longevity in narwhals (Monodon monoceros) from West Greenland as estimated by aspartic acid racemization. J. Mammal. 88, 49–58. doi: 10.1644/06-MAMM-A-056R.1
George, J. C., Bada, J., Zeh, J., Scott, L., Brown, S. E., O’Hara, T., et al. (1999). Age and growth estimates of bowhead whales (Balaena mysticetus) via aspartic acid racemization. Can. J. Zool. 77, 571–580. doi: 10.1139/z99-015
Grue, H., and Jensen, B. (1979). Review of the formation of incremental lines in tooth cementum of terrestrial mammals. Dan. Rev. Game Biol. 11, 1–47.
Hamady, L. L., Natanson, L. J., Skomal, G. B., and Thorrold, S. R. (2014). Vertebral bomb radiocarbon suggests extreme longevity in white sharks. PLoS One 9:e84006. doi: 10.1371/journal.pone.0084006
Heide-Jørgensen, M. P., and Laidre, K. L. (2015). Surfacing time, availability bias and abundance of humpback whales in West Greenland. J. Cetacean Res. Manag. 15, 1–8.
Heide-Jørgensen, M. P., Laidre, K. L., Hansen, R. G., Burt, M. L., Simon, M., Borchers, D. L., et al. (2012). Rate of increase and current abundance of humpback whales in West Greenland. J. Cetacean Res. Manag. 12, 1–14.
Heinämäki, A. A., and Lindfors, A. S. H. (1988). Free amino acids in rat ocular tissues during postnatal development. Biochem. Int. 16, 405–412.
Herman, D. P., Ylitalo, G. M., Robbins, J., Straley, J. M., Gabriele, C. M., Clapham, P. J., et al. (2009). Age determination of humpback whales Megaptera novaeangliae through blubber fatty acid compositions of biopsy samples. Mar. Ecol. Prog. Ser. 392, 277–293. doi: 10.3354/meps08249
IWC (1986). Chairman’s report of the thirty-seventh annual meeting. Rep. Int. Whal. Commn. 36, 10–29.
Kalish, J. M. (1993). Pre- and post-bomb radiocarbon in fish otoliths. Earth Planet. Sci. Lett. 114, 549–554. doi: 10.1016/0012-821x(93)90082-k
Konrádsson, A., and Sigurjónsson, J. (1989). Studies on growth layers in tympanic bullae in fin whales (Balaenoptera physalus) caught off Iceland. Rep. Int. Whal. Commn. 39, 277–279.
Larsen, F., and Hammond, P. (2004). Distribution and abundance of West Greenland humpback whales (Megaptera novaeangliae). J. Zool. 263, 343–358. doi: 10.1017/s095283690400531x
Lockyer, C. (1974). Investigation of the ear plug of the southern sei whale, Balaenoptera borealis, as a valid means of determining age. ICES J. Mar. Sci. 36, 71–81. doi: 10.1093/icesjms/36.1.71
Lubetkin, S. C., Zeh, J. E., Rosa, C., and George, J. C. (2008). Age estimation for young bowhead whales (Balaena mysticetus) using annual baleen growth increments. Can. J. Zool. 86, 525–538. doi: 10.1139/Z08-028
Lynnerup, N., Kjeldsen, H., Heegaard, S., Jacobsen, C., and Heinemeier, J. (2008). Radiocarbon dating of the human eye lens crystallines reveal proteins without carbon turnover throughout life. PLoS One 1529:e1529. doi: 10.1371/journal.pone.0001529
Martin, A. R., Katona, S. K., Mattila, D. K., Hembree, D., and Waters, T. D. (1984). Migration of humpback whales between the caribbean and Iceland. J. Mammal. 65, 330–333. doi: 10.2307/1381174
Masters, P. M., Bada, J. L., and Zigler, J. S. (1977). Aspartic acid racemization in the human lens during ageing and in cataract formation. Nature 268, 71–73. doi: 10.1038/268071a0
Mizroch, S. A. (1981). Analysis of some parameters of the Antarctic fin whale (Balaenoptera physalus). Rep. Int. Whal. Commn. 31, 425–435.
Morrison, P. (1962). Body temperatures in some Australian mammals. Biol. Bull. 123: 484–497. doi: 10.2307/1539512
Nielsen, H. N., Garde, E., Heide-Jørgensen, M. P., Lockyer, C. H., Ditlevsen, S., Òlafsdottir, D., et al. (2013). Application of a novel method for age estimation of a baleen whale and a porpoise. Mar. Mammal. Sci. 29, E1–E23. doi: 10.1111/j.1748-7692.2012.00588.x
Nielsen, J. (2018). The Greenland Shark (Somniosus microcephalus): Diet, Tracking and Radiocarbon Age Estimates Reveal the World’s Oldest Vertebrate. Ph.D. thesis, University of Copenhagen.
Nielsen, J., Hedeholm, R. B., Heinemeier, J., Bushnell, P. G., Christiansen, J. S., Olsen, J., et al. (2016). Eye lens radiocarbon reveals centuries of longevity in the Greenland shark (Somniosus microcephalus). Science 353:6300. doi: 10.1126/science.aaf1703
Ohtani, S., and Yamamoto, T. (2011). Comparison of age estimation in Japanese and scandinavian teeth using amino acid racemization. J. Forensic Sci. 56, 244–247. doi: 10.1111/j.1556-40292010.01545.x
Olsen, E., and Sunde, J. (2002). Age determination of minke whales (Balaenoptera acutorostrata) using the aspartic acid racemization technique. Sarsia 87, 1–8. doi: 10.1080/003648202753631686
Perkins, J. S., Balcomb, K. C. III, Nichols, G. Jr., and DeAvilla, M. (1984). Abundance and distribution of humpback whales (Megaptera novaeangliae) in West Greenland waters. Can. Fish. Aquat. Sci. 41, 533–536. doi: 10.1139/f84-065
Polanowski, A. M., Robbins, J., Chandler, D., and Jarman, S. N. (2014). Epigenetic estimation of age in humpback whales. Mol. Ecol. 14, 976–817. doi: 10.1111/1755-0998.12247
Punt, A. E., Friday, N. A., and Smith, T. D. (2006). Reconciling data on the trends and abundance of North Atlantic humpback whales within a population modelling framework. J. Cetacean Res. Manag. 8, 145–159.
Riekkola, L., Zerbini, A. N., Andrews, O., Andrews-Goff, V., Baker, C. S., Chandler, D., et al. (2018). Application of a multi-disciplinary approach to reveal population structure and Southern Ocean feeding grounds of humpback whales. Ecol. Indic. 89, 455–465. doi: 10.1016/j.ecolind.2018.02.030
Rosa, C., Zeh, J., George, J. C., Botta, O., Zausher, M., Bada, J., et al. (2013). Age estimates based on aspartic acid racemization for bowhead whales (Balaena mysticetus) harvested in 1998-2000 and the relationship between racemization rate and temperature. Mar. Mammal. Sci. 29, 424–445. doi: 10.1111/j.1748-7692.2012.00593.x
Scourse, J. D., Wanamaker, A. Jr., Weidman, C., Heinemeier, J., and Reimer, P. (2012). The marine radiocarbon bomb pulse across the temperate North Atlantic: a compilation of Δ14C time histories from Arctica islandica growth increments. Radiocarbon 54, 165–186. doi: 10.2458/azu_js_rc.v54i2.16026
Simon, M., Johnson, M., and Madsen, P. T. (2012). Keeping momentum with a mouthful of water: behavior and kinematics of humpback whale lunge feeding. J. Exp. Biol. 215, 3786–3798. doi: 10.1242/jeb.071092
Stevick, P. T. (1999). Age-length relationships in humpback whales: a comparison of strandings in the Western North Atlantic with commercial catches. Mar. Mamm. Sci. 15, 725–737. doi: 10.1111/j.1748-7692.1999.tb00839.x
Stevick, P. T., Allen, J., Clapham, P. H., Friday, N., Katona, T. K., Larsen, F., et al. (2003). North Atlantic humpback whale abundance and the rate of increase four decades after protection from whaling. Mar. Ecol. Prog. Ser. 258, 263–273. doi: 10.3354/meps258263
Stewart, R. E. A., Campana, S. E., Jones, C. M., and Stewart, B. E. (2006). Bomb radiocarbon dating calibrates beluga (delphinapterus leucas) age estimates. Can. J. Zool. 84, 1840–1852. doi: 10.1139/z06-182
Taylor, B. L., Chivers, S. J., Larese, J., and Perrin, W. F. (2007). Generation Length and Percent Mature Estimates for IUCN Assessments of Cetaceans. Administrative Report LJ-07-01. Silver Spring, MD: National Marine Fisheries.
Weinrich, M. (1993). Separation of humpback whale mothers and calves on a feeding ground in early autumn. Mar. Mammal Sci. 9, 325–328. doi: 10.1111/j.1748-7692.1993.tb00476.x
Whitehead, H., Kevin, C., Perkins, J., Bryant, P., and Nichols, G. (1983). Population size, stock identity, and distribution of the humpback whales off West Greenland – summer 1981. Rep. Int. Whal. Commn. 33, 497–501.
Zerbini, A. N., Clapham, P. J., and Wade, P. R. (2010). Assessing plausible rates of population growth in humpback whales from life-history data. Mar. Biol. 157, 1225–1236. doi: 10.1007/s00227-010-1403-y
Keywords: humpback whales, West Greenland, age, radiocarbon dating, aspartic acid racemization, post whaling
Citation: Boye TK, Garde E, Nielsen J, Hedeholm R, Olsen J and Simon M (2020) Estimating the Age of West Greenland Humpback Whales Through Aspartic Acid Racemization and Eye Lens Bomb Radiocarbon Methods. Front. Mar. Sci. 6:811. doi: 10.3389/fmars.2019.00811
Received: 30 September 2019; Accepted: 16 December 2019;
Published: 14 January 2020.
Edited by:
Lyne Morissette, M – Expertise Marine, CanadaReviewed by:
Sabrina Fossette, Department of Biodiversity, Conservation and Attractions (DBCA), AustraliaGail Schofield, Queen Mary University of London, United Kingdom
Copyright © 2020 Boye, Garde, Nielsen, Hedeholm, Olsen and Simon. This is an open-access article distributed under the terms of the Creative Commons Attribution License (CC BY). The use, distribution or reproduction in other forums is permitted, provided the original author(s) and the copyright owner(s) are credited and that the original publication in this journal is cited, in accordance with accepted academic practice. No use, distribution or reproduction is permitted which does not comply with these terms.
*Correspondence: Tenna K. Boye, tennakb@gmail.com