- 1Marine Science Group, Department of Biological, Geological and Environmental Sciences, University of Bologna, Bologna, Italy
- 2School of BioSciences, University of Melbourne, Parkville, VIC, Australia
- 3Department of Chemistry “Giacomo Ciamician”, University of Bologna, Bologna, Italy
- 4The Mina and Everard Goodman Faculty of Life Sciences, Bar-Ilan University, Ramat Gan, Israel
- 5Fano Marine Center, Department of Biological, Geological and Environmental Sciences, University of Bologna, Fano, Italy
The Intergovernmental Panel on Climate Change (IPCC) predicted that ocean surface temperature will rise of 0.6–2.0°C by 2100. Ocean warming is expected to produce strong impacts on marine ecosystems such as coral reefs, affecting their physiological events including reproductive processes. To date, relatively few studies have examined the effects of climate change on the reproductive success of temperate corals and even less in the azooxanthellate ones. This study examined the reproductive output of the azooxanthellate Mediterranean coral Caryophyllia inornata along a wide latitudinal gradient of seawater temperature and solar radiation. A total of 260 samples, collected from five populations along the Western Italian coast, have been analyzed through histological techniques. The intriguing aspects characterizing all populations of C. inornata along the latitudinal gradient are a strong male-biased sex ratio and the presence of embryos in all stages of development throughout the year in females, males, and sexually inactive individuals. This peculiarity could suggest a mixed strategy of sexual and asexual reproduction in this species as has been observed for some anemones of the genus Actinia. Fecundity and spermary abundance (i.e., the number of reproductive elements per body volume unit), gonadal index (i.e., the percentage of body volume occupied by the germ cells) and fertility (i.e., the number of embryos per body volume unit) in females, males and sexually inactive individuals were unrelated to solar radiation and temperature along the latitudinal gradient. These results suggest that the reproduction in C. inornata is not affected by increasing solar radiation and temperature. The lack of zooxanthellae could make this species less dependent on these environmental parameters, as previously hypothesized for another azooxanthellate species, Leptopsammia pruvoti, investigated along the same gradient.
Introduction
Climate change is today one of the most serious threats to the biodiversity of our planet. Since the Industrial revolution, the human use of fossil fuels, deforestation and the massive advent of the intensive agriculture have dramatically increased the concentration of greenhouse gases in the atmosphere (Sabine et al., 2004) that cause an increase in global mean temperature and, consequently, in ocean surface temperature (Harley et al., 2006). If the anthropogenic emissions keep growing, a further increase of 0.6–2.0°C is expected by 2100 (IPCC, 2014). According to the Intergovernmental Panel on Climate Change (IPCC), ocean warming is severely impacting marine ecosystems, which are among the most ecologically and socio-economically vital on the planet (Harley et al., 2006).
Climatic models predict a greater influence of the global warming in the temperate areas than tropical ones (IPCC, 2014). In particular, the small enclosed Mediterranean Sea that is considered a “biodiversity hotspot,” is one of the most strongly affected regions by increasing temperature (Field et al., 2012). Indeed, the warming rates of the Mediterranean Sea are three times higher than those of the oceans (IPCC, 2014). These traits make the Mediterranean Sea one of the most emblematic natural model for studying the interactions between marine life and environmental changes (Feely et al., 2004; Lejeusne et al., 2010).
Reproduction, which is affected by environmental fluctuations, is considered the most sensitive stage of the life cycle and can provide information on how organisms react to stress (Harrison and Wallace, 1990; Ward, 1995; Van Woesik, 2010). The reproductive cycle of corals can be affected by ocean warming through reduction of fertility, eggs quality, fertilization and recruitment success, threatening the ability of corals to recover after disturbances (Linares et al., 2008; Albright and Mason, 2013). For example, the tropical coral Acropora digitifera exposed to higher temperatures shows a decrease in egg volume and sperm number (Paxton et al., 2016). An increase in temperature has been demonstrated to increase the development of abnormal embryos in the coral Platygyra acuta (Chui and Ang, 2015). Larval development, survival and settlement of A. palmata are negatively affected by temperature increase in laboratory experiments (Randall and Szmant, 2009). Sexual reproduction and recruitment are the most critical processes driving population change (Doherty and Fowler, 1994), thus, it is crucial to gain a better understanding if this vulnerable stage of corals will be impacted by ocean warming.
While several studies show the effects of ocean warming on sexual reproduction of tropical scleractinians (Bassim et al., 2002; Krupp et al., 2006; Negri et al., 2007; Nozawa and Harrison, 2007; Albright and Mason, 2013), only a few examined the response to elevated temperatures in temperate corals who will like be the most impacted by ongoing climate changes. To date, the only studies performed on the effect of increasing seawater temperature on the reproductive output of temperate corals have been performed on the zooxanthellate Balanophyllia europaea and on the azooxanthellate Leptopsammia pruvoti investigated along the same latitudinal gradient considered here. These studies showed that the gametogenesis of the former is negatively affected by increased seawater temperature, while the same parameter investigated in the latter species is unaffected (Airi et al., 2014, 2017).
The present study examined the azooxanthellate solitary coral Caryophyllia inornata, which is widely distributed in the eastern and western Mediterranean Sea (Zibrowius, 1980), extending to the northeast of the Atlantic coast (Cairns, 1999) and from the Canary Islands to the southern coasts of England (Zibrowius, 1980). It colonizes caves, walls, and wrecks, from the surface down to 100 m depth in dimly lit or dark environments, representing one of the main species that populate the walls and the vaults of caves and in some cases is the dominant species (Zibrowius, 1978). It is a slow growing coral with a population density that can reach thousands of individuals per m2 and its abundance along the Western Italian coasts varies from 100 to 1500 individuals per m2 (Caroselli et al., 2015). C. inornata is gonochoric and brooding (Goffredo et al., 2012; Marchini et al., 2015), showing peculiar traits such as a male biased sex ratio (1:3) (Goffredo et al., 2012) and the presence of embryos in coelenteric cavity and mesenteric septa of females, males, and sexually inactive individuals (without germ cells), throughout the year (Marchini et al., 2015). Previous studies reveal that increasing temperature and solar radiation do not affect population density, growth parameters, net calcification rate, bulk skeletal density, and linear extension rate of C. inornata (Caroselli et al., 2015, 2016a,b), while variations have been observed in its population dynamics (Caroselli et al., 2016b). Considering the results of these previous studies, we expect to find a similar response to the effects of temperature regarding gametogenesis. The specific aim of this study was to quantify the reproductive output of C. inornata along a latitudinal gradient of temperature and solar radiation.
Materials and Methods
Sample Collection and Environmental Parameters
Solitary polyps of C. inornata with an average size of 7.0 ± 0.1 mm (mean ± SE) have been collected from five sites along a latitudinal gradient, from 44°20′N to 36°46′N (Figure 1). Coral collection occurs from June 2010 to November 2012. During this period, 18 monthly samplings were performed for four populations (Genova: April 2011–September 2012; Calafuria: February 2011–July 2012; Scilla: July 2010–November 2012; Pantelleria: September 2010–November 2012), with a minimum of 15 polyps collected during each excursion. Data from Elba Island population came from a previous study (Marchini et al., 2015) for which samples were collected between May 2009 and October 2010. Sampling was performed within a depth range (11–16 m) known to have high population density (Caroselli et al., 2015).
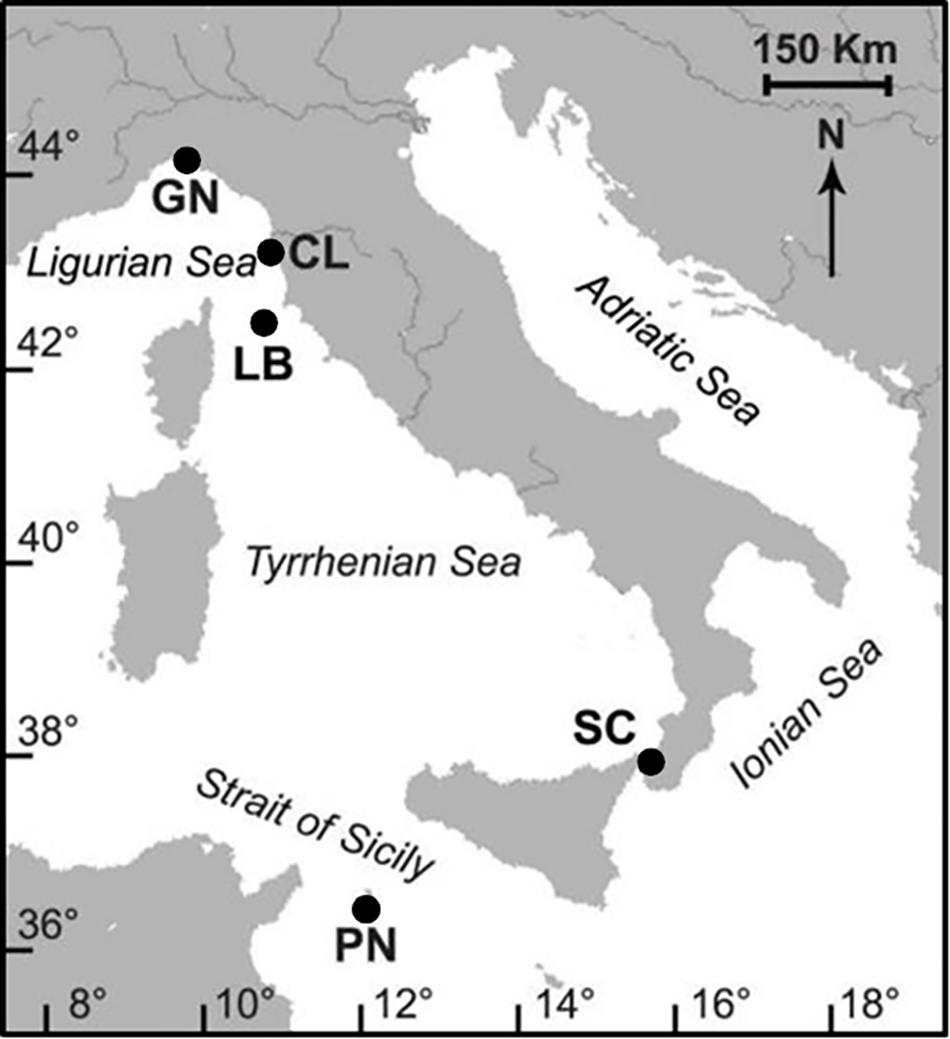
Figure 1. Map of the Italian coastline indicating the sites of coral collection. Abbreviations and coordinates of the sites in decreasing order of latitude: GN Genova, 44°20′N, 9°08′E; CL Calafuria, 43°27′N, 10°21′E; LB Elba Island, 42°45′N, 10°24′E; SC Scilla, 38°01′N, 15°38′E; PN Pantelleria Island, 36°45′N, 11°57′E. Map was created by the authors using the software Adobe® Illustrator® CS3.
Depth Thermometer Temperature (DTT;°C) was measured by digital thermometers (I-Button DS1921H, Maxim Integrated Products), placed at the sampling location for each population. Sensors recorded seawater temperature during the entire experimental period. Sea Surface Temperature data (SST;°C) for each site were recorded hourly from the National Mareographic Network of the Institute for the Environmental Protection and Research (ISPRA)1. These data were measured by mareographic stations (SM3810 manufactured by the Society for the Environmental and Industrial monitoring; SIAP + MICROS), placed close to the sampling sites. For each location, a linear regression was obtained between DTT and SST data (Genova: ; Calafuria: ; Elba Island: DT = 0.8071∗SST + 2.6234; Scilla: DT = 0.988∗SST−0.3225; Pantelleria: DT = 0.7635∗SST + 3.3132) to estimate historical at-depth temperatures (DT;°C) of the 3 years preceding the sampling (Supplementary Table S1).
Solar radiation (W/m2) was recorded from the archives of the Satellite Application Facility on Climate Monitoring (CM-SAF/EUMETSAT)2, using real time data sets based on intersensor calibrated radiances from MFG satellites. Mean annual solar radiation of each site was obtained for the 15 km square associated with each of the five sites. As for temperature, also for solar radiation we considered the average of the 3 years preceding the sampling.
Monthly average values of chlorophyll-a and organic matter were retrieved through the GIOVANNI data system (MODIS-Aqua MODISA_L3m_CHL 4 km; MODIS-Aqua MODISA_L3m_FLH v2014 http://giovanni.gsfc.nasa.gov/giovanni/). Monthly average values of nutrients concentration (nitrate and phosphate) were retrieved using the Copernicus Marine Service Product GLOBAL_ANALYSIS_FORECAST_PHYS_001_002 (http:// marine.copernicus.eu/), and visualized through the Panoply software v. 4.5 (http://www.giss.nasa.gov/tools/panoply/).
Biometric and Histological Analysis
Biometric analyses were performed by measuring length (L, maximum axis of the oral disk), width (W, minimum axis of the oral disk) and height (h, oral–aboral axis) of each sampled polyp. The volume (V) of the individual polyp was calculated using the formula V = h*(L/2)*(W/2)*π (Goffredo et al., 2002).
Polyps were post-fixed in Bouin solution to ensure a better fixation and staining of the tissues. After 4 h decalcification in EDTA and dehydration steps that took place every 2 h in a graded alcohol series from 80 to 100%, polyps were clarified in histolemon and embedded in paraffin. Serial transverse sections were cut at 7 μm intervals along the oral-aboral axis, from the oral to the aboral pole of the entire polyp. Tissues were then stained with Mayer’s hematoxylin and eosin (Goffredo et al., 2002; Marchini et al., 2015).
Cytohistometric Analysis
Cytometric analyses were made with an optical microscope using the image analyzer NIKON NIS-Elements D 3.2. The maximum and minimum diameters of the oocytes in nucleated sections and spermaries were measured and the presence of embryos was recorded. Spermaries were classified into five developmental stages according to earlier studies on gametogenesis in scleractinians (Goffredo et al., 2005, 2012).
Definitions
Reproductive output was defined through four reproductive parameters: (a) fecundity rate and spermary abundance, both defined as the number of reproductive elements per body volume unit (100 mm3); (b) “gonadal” index, defined as the percentage of body volume occupied by the germ cells; (c) reproductive element size, defined as the average of the maximum and minimum diameter of spermaries and oocytes in nucleated section; (d) fertility, defined as the number of embryos per body volume unit (100 mm3) (Marchini et al., 2015).
In accordance with the annual reproductive cycle previously described for C. inornata (Marchini et al., 2015), gametal development was characterized by two gamete activity periods. The gamete recruitment period (Korta et al., 2010; Lowerre-Barbieri et al., 2011; Airi et al., 2017), occurring between February and April, was characterized by the development of early stages of oocytes (<100 μm) and spermaries (I, II, and III maturation stage; Supplementary Figure S1). The gamete maturity period (Korta et al., 2010; Lowerre-Barbieri et al., 2011; Airi et al., 2017), between May and July, was characterized by the presence of larger oocytes (>100 μm) and advanced stage of maturation of spermaries (IV and V maturation stage; Supplementary Figure S1) that reached maturity for fertilization.
Statistical Analysis
A 2-sample Kolmogorov–Smirnov test was used to compare the stage/size-frequency distribution of reproductive elements between gamete recruitment and gamete maturity periods for all populations (Olea and Pawlowsky-Glahn, 2009). Data were tested for normality using a single Kolmogorov–Smirnov test and for homogeneity of variance or homoscedasticity using a Levene’s test. When assumptions for parametric statistics were not fulfilled, a non-parametric test was used. The non-parametric Kruskal–Wallis test was used to compare reproductive parameters among study sites. The Mann–Whitney U test was used as a non-parametric alternative to the Student’s t test to compare the mean oocyte and spermary size of populations between reproductive periods. Spearman’s rank correlation coefficient (ρ) was used to calculate the significance of the correlations between reproductive and environmental parameters. Spearman’s rank correlation coefficient is an alternative used for data that do not meet the assumptions of Pearson’s correlation coefficient (Ludbrook, 1991; Potvin and Roff, 1993). All analyses were computed using SPSS 22.0 (Apache Computer Software Foundation). A one-way permutation multivariate analysis of variance (PERMANOVA) based on Euclidean distances was performed with 999 permutation, to test differences in oocyte size distribution and spermary maturation stage distribution among populations. BEST BIO-ENV routine was carried out using a Spearman rank correlation to identify the best environmental variables (i.e., temperature, solar radiation, chlorophyll a, organic matter, and nutrients) that explained the observed reproductive patterns on 999 permutations. All analyses were computed using the software Primer v6 – Quest Research Limited (Anderson et al., 2008).
Results
Mean annual depth temperature (DT;°C), ranging from 16.6 to19.0°C, and mean annual solar radiation (W/m2), ranging from 156.9 to 218.2 W/m2, were significantly different among sites (DT, Kruskal–Wallis, p < 0.05; solar radiation, ANOVA, p < 0.001; Table 1).
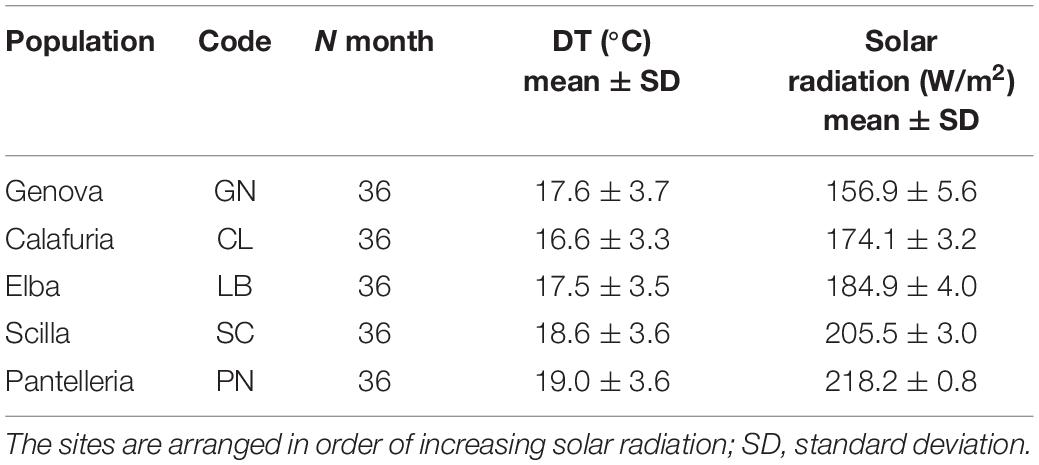
Table 1. Mean annual depth temperature (DT;°C) and solar radiation (W/m2) from the sampled populations.
All populations showed gonochoric and sexually inactive polyps in both reproductive periods (Table 2). All populations along the latitudinal gradient were characterized by a strong male-biased sex ratio (1:6 for Genova, chi-square test: χ2 = 9.80, p < 0.01; 1:6 for Calafuria, chi-square test: χ2 = 7.14, p < 0.01; 1:3 for Elba Island, chi-square test: χ2 = 9.00, p < 0.01; 1:5 for Scilla, chi-square test: χ2 = 9.78, p < 0.01; 1:7 for Pantelleria, chi-square test: χ2 = 12.57, p < 0.001; Table 2).
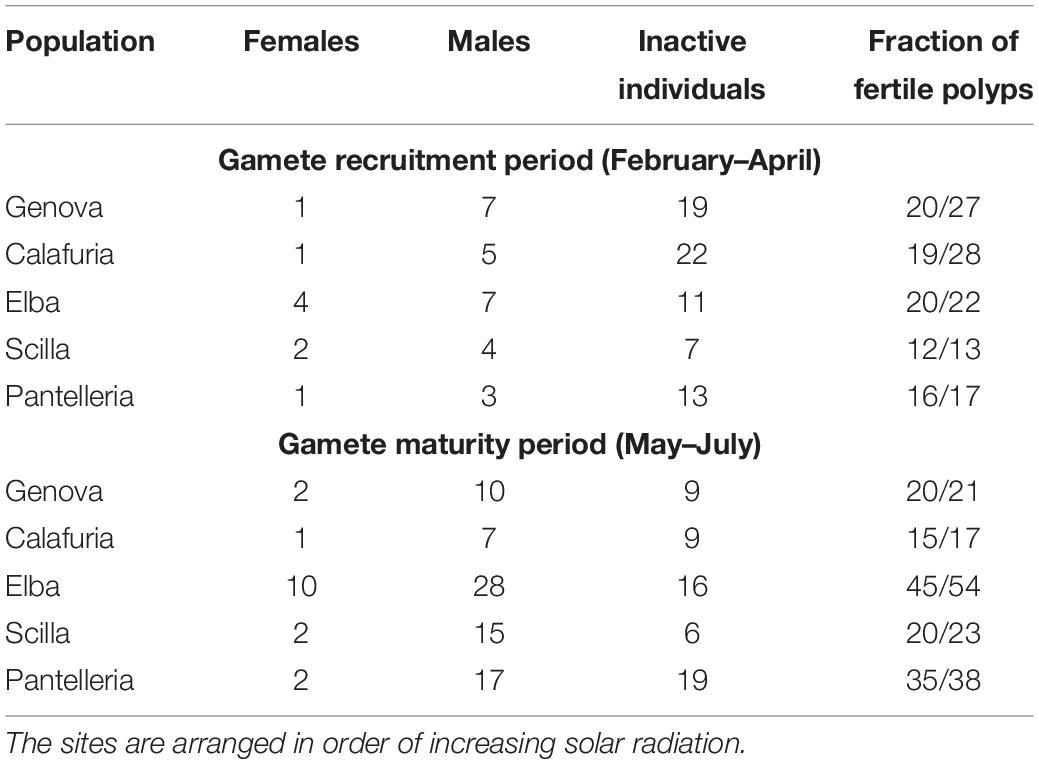
Table 2. Number of females, males, inactive individuals and fraction of fertile polyps in each population for gamete recruitment and gamete maturity periods.
Oocyte size/frequency distribution during February–April (gamete recruitment period) was significantly different from that of May–July (gamete maturity period), in all populations (Kolmogorov–Smirnov, p < 0.001; Figure 2A). The mean oocyte size during the gamete recruitment period was significantly lower than that of gamete maturity period in all populations (Mann–Whitney U test, p < 0.001; Table 3 and Figure 2A), and there were no significant differences in size/frequency distribution among populations (PERMANOVA, p = 0.916 for gamete recruitment period; p = 0.154 for gamete maturity period; Figure 2A).
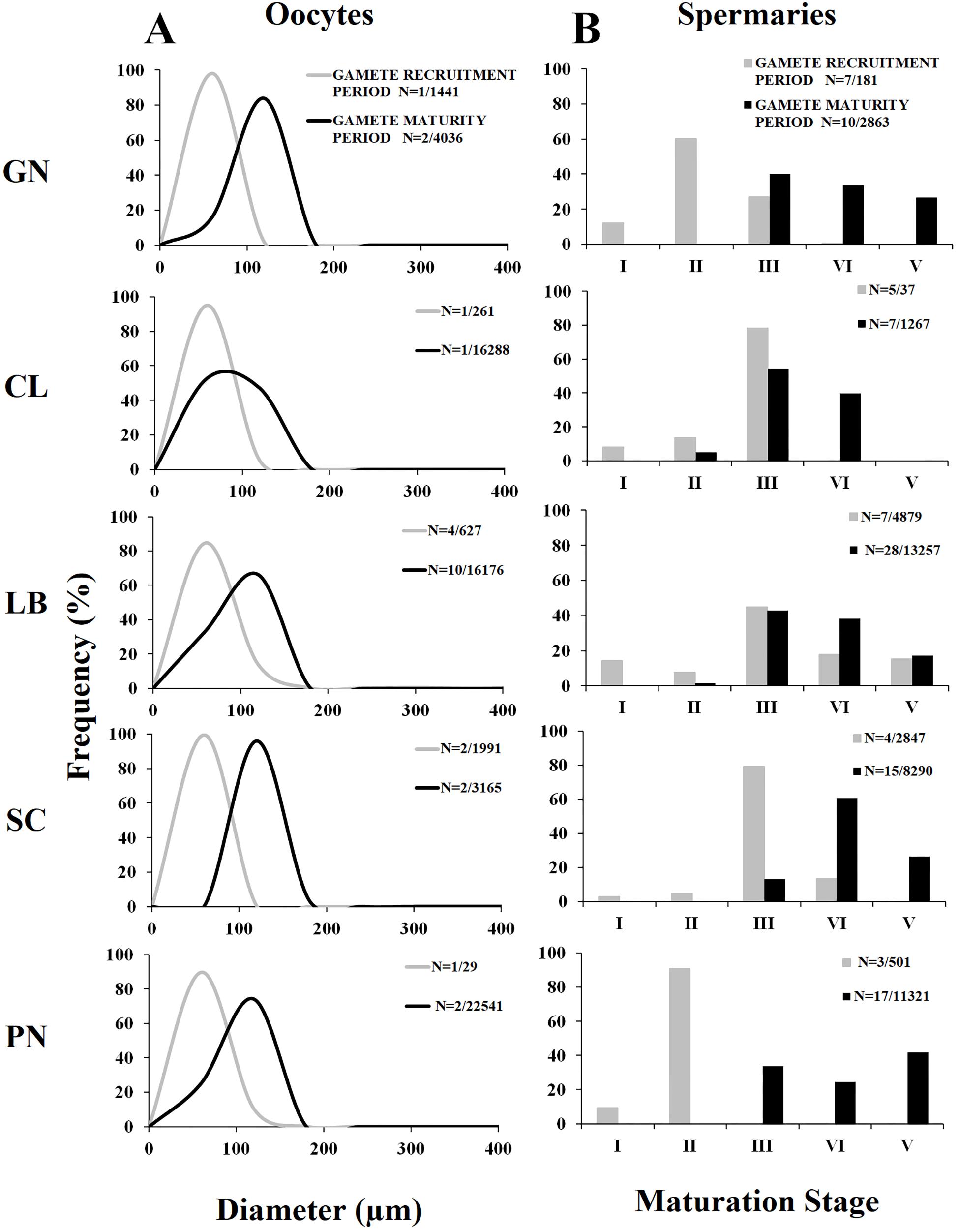
Figure 2. Oocyte size and spermary maturation stage distributions for the two reproductive periods. (A) Distribution of oocyte size during gamete recruitment (gray line) and gamete maturity (black line) periods. N = number of polyps/oocytes. (B) Distribution of the five maturation stages of spermaries during gamete recruitment (gray histogram bars) and gamete maturity (black histogram bars) periods. N = number of polyps/spermaries.
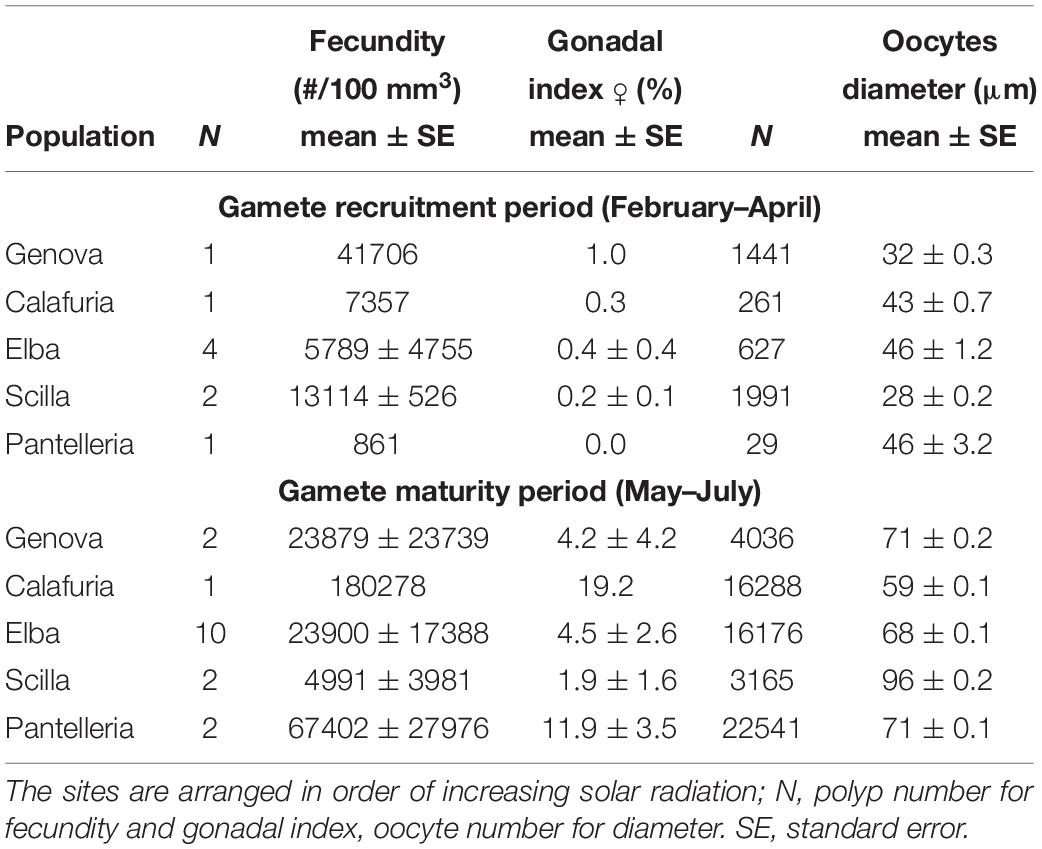
Table 3. Mean fecundity, gonadal index and diameter of oocytes in each population for gamete recruitment and gamete maturity periods.
The distribution of spermary maturation stages was significantly different between gamete recruitment and maturity periods in all populations (Kolmogorov–Smirnov, p < 0.001; Figure 2B). From February to April (gamete recruitment period), each population was characterized by small spermaries belonging to the earliest maturation stages (stages I, II, and III; Figure 2B). During May–July (gamete maturity period) all populations were characterized by more advanced maturation stages (stage III, IV, and V; Figure 2B). The mean spermary size during the gamete recruitment period was significantly lower than that of gamete maturity period in all populations (Mann–Whitney U test, p < 0.05; Table 4 and Figure 2B). Spermary maturation stage distributions were homogeneous among populations for both reproductive periods (PERMANOVA, p = 0.144 for gamete recruitment period; PERMANOVA, p = 0.334 for gamete maturity period; Figure 2B).
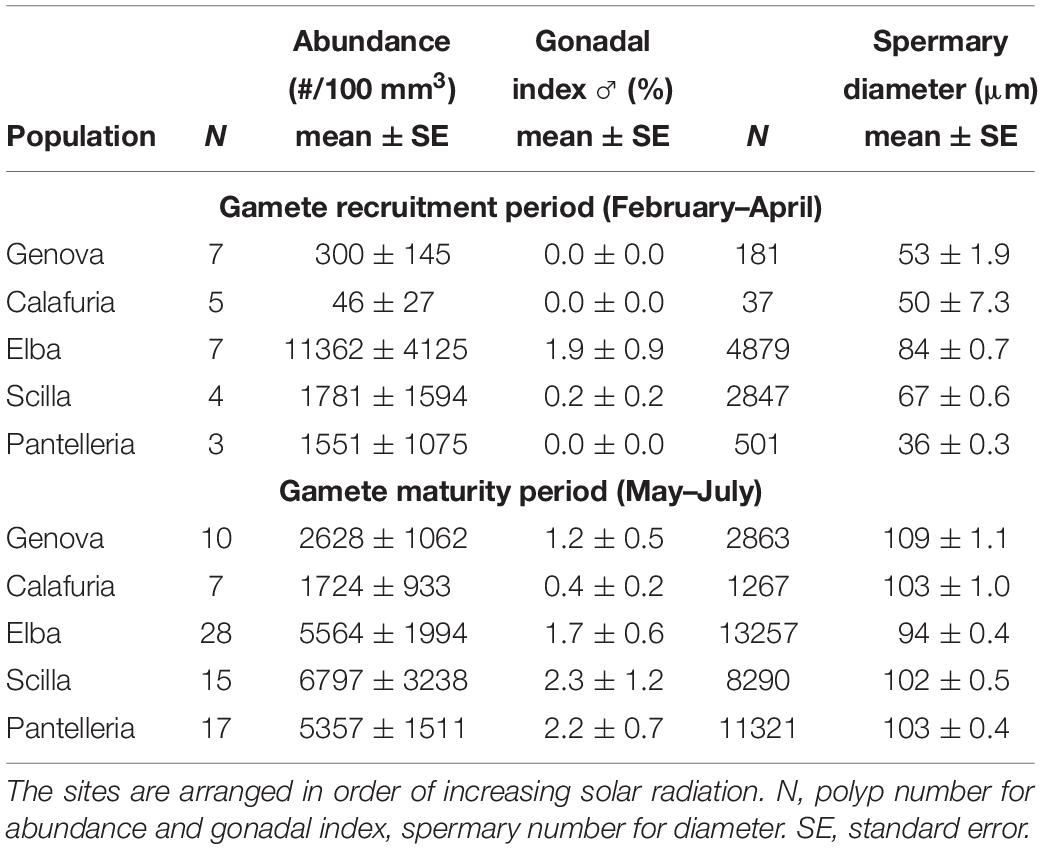
Table 4. Mean abundance, gonadal index and diameter of spermaries in each population for gamete recruitment and gamete maturity periods.
The presence of embryos in the gastrovascular cavity and mesenterial septa (Supplementary Figure S1) were recorded throughout the year without significant differences between reproductive periods, in females, males, and sexually inactive individuals of almost all populations, except for sexually inactive individuals of Scilla that showed more embryos in gamete recruitment period (Mann–Whitney U test, p < 0.05; Table 5 and Figure 3) and for males of Pantelleria Island that showed more embryos in gamete maturity period (Mann–Whitney U test, p < 0.05; Table 5 and Figure 3).
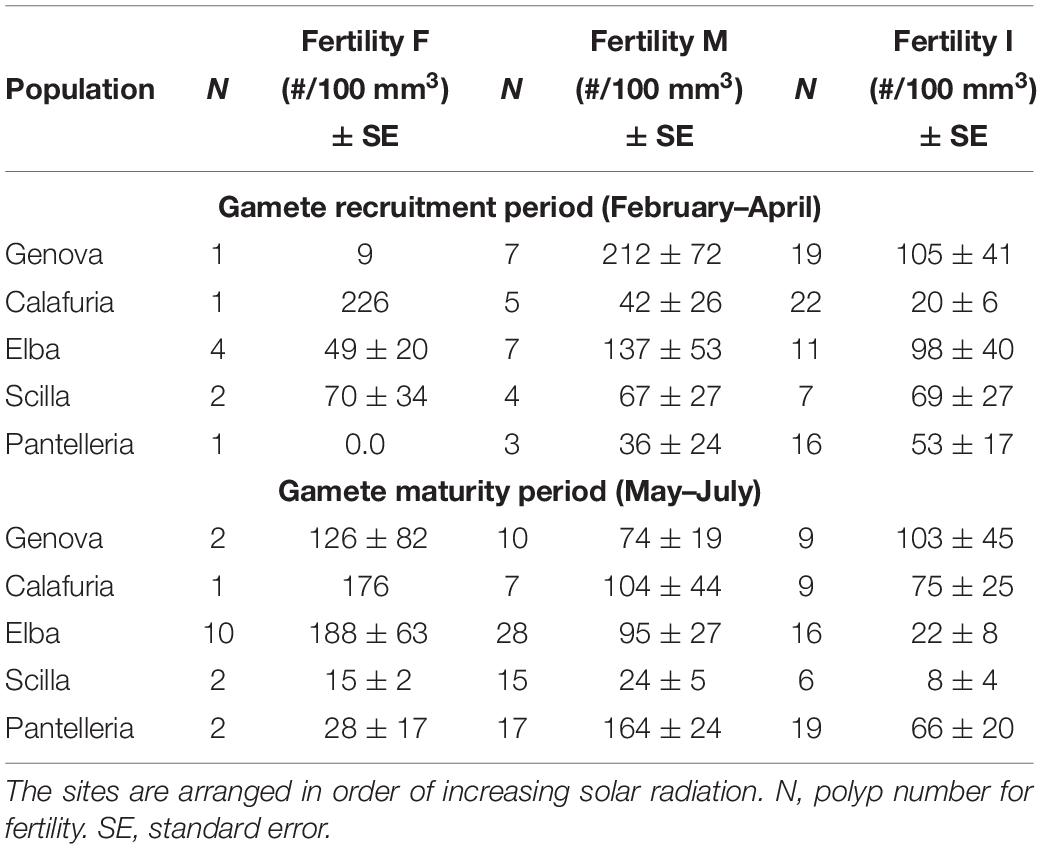
Table 5. Mean fertility of females (F), males (M) and inactive individuals (I) in each population for gamete recruitment and gamete maturity periods.
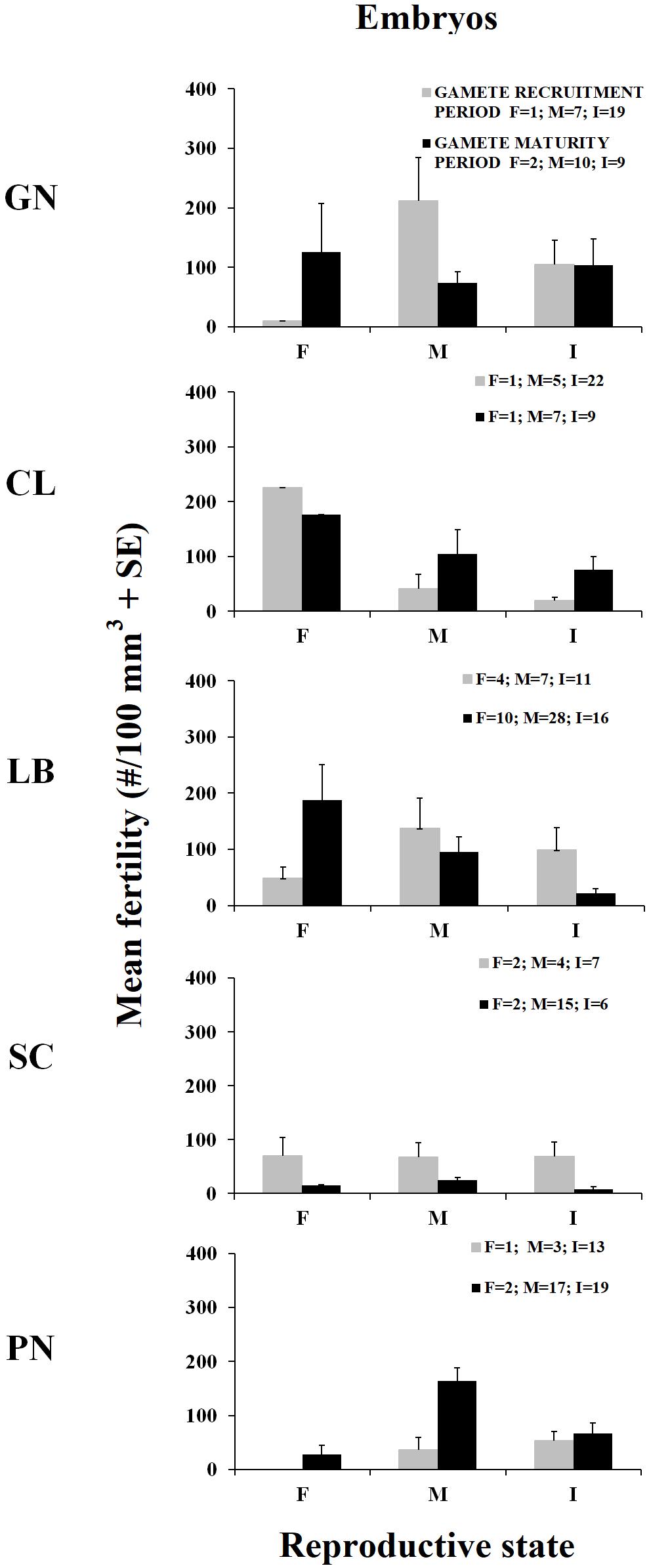
Figure 3. Mean fertility in females (F), males (M), and sexually inactive individuals (I) ± standard error (SE) for the two reproductive periods. Mean fertility during gamete recruitment period (gray histogram bars) and gamete maturity period (black histogram bars). N = number of polyps.
Female fecundity and gonadal index were the same during both gamete recruitment and gamete maturity periods, in all populations along the latitudinal gradient (Supplementary Table S2 and Figure 4). The BEST BIO/ENV analysis confirmed this pattern (p > 0.05; Supplementary Table S5). The mean oocyte diameter was significantly different during both reproductive periods among populations (Kruskal–Wallis test, p < 0.001; Supplementary Table S2 and Figure 4). While in the gamete recruitment period the mean oocyte diameter was negatively correlated with the DT and the solar radiation, during gamete maturity period, it was positively correlated with the same environmental parameters (Spearman correlation test, p < 0.01; Supplementary Table S2 and Figure 5). However, diameters did not correlate with any environmental variables using the BEST BIO/ENV analysis (p > 0.05; Supplementary Table S5).
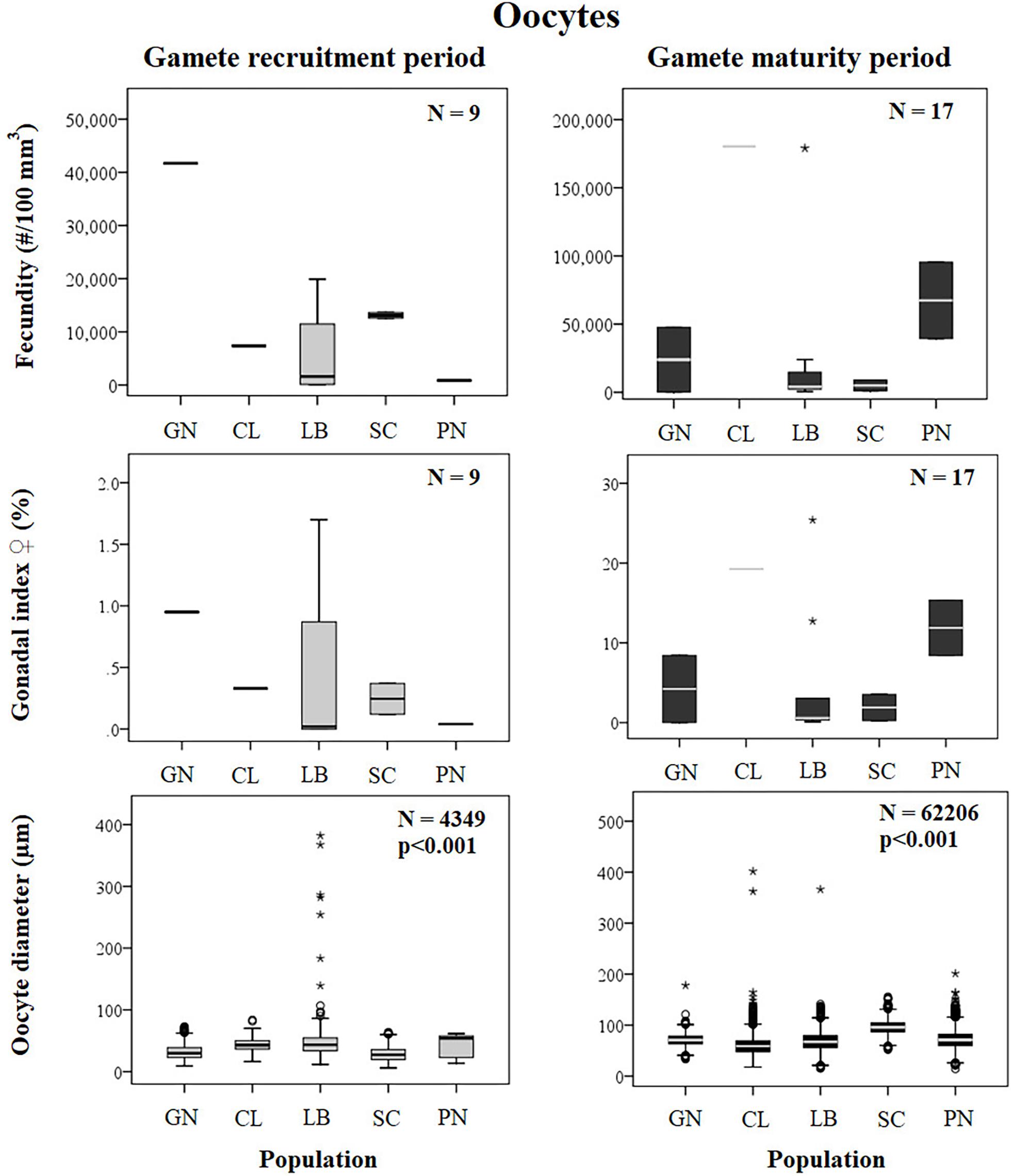
Figure 4. Oocytes. Boxplots of reproductive parameters per study site (GN Genova; CL Calafuria; LB Elba Island; SC Scilla; PN Pantelleria Island) during the gamete recruitment and gamete maturity periods. Each boxplot diagram shows the median (solid horizontal line), the first and third quartiles (box outline), the minimum and maximum values (whiskers) and the outliers (stars and circles). N, polyp number for fecundity and gonadal index, oocyte number for diameter; p, significance of the ANOVA/Kruskal–Wallis test.
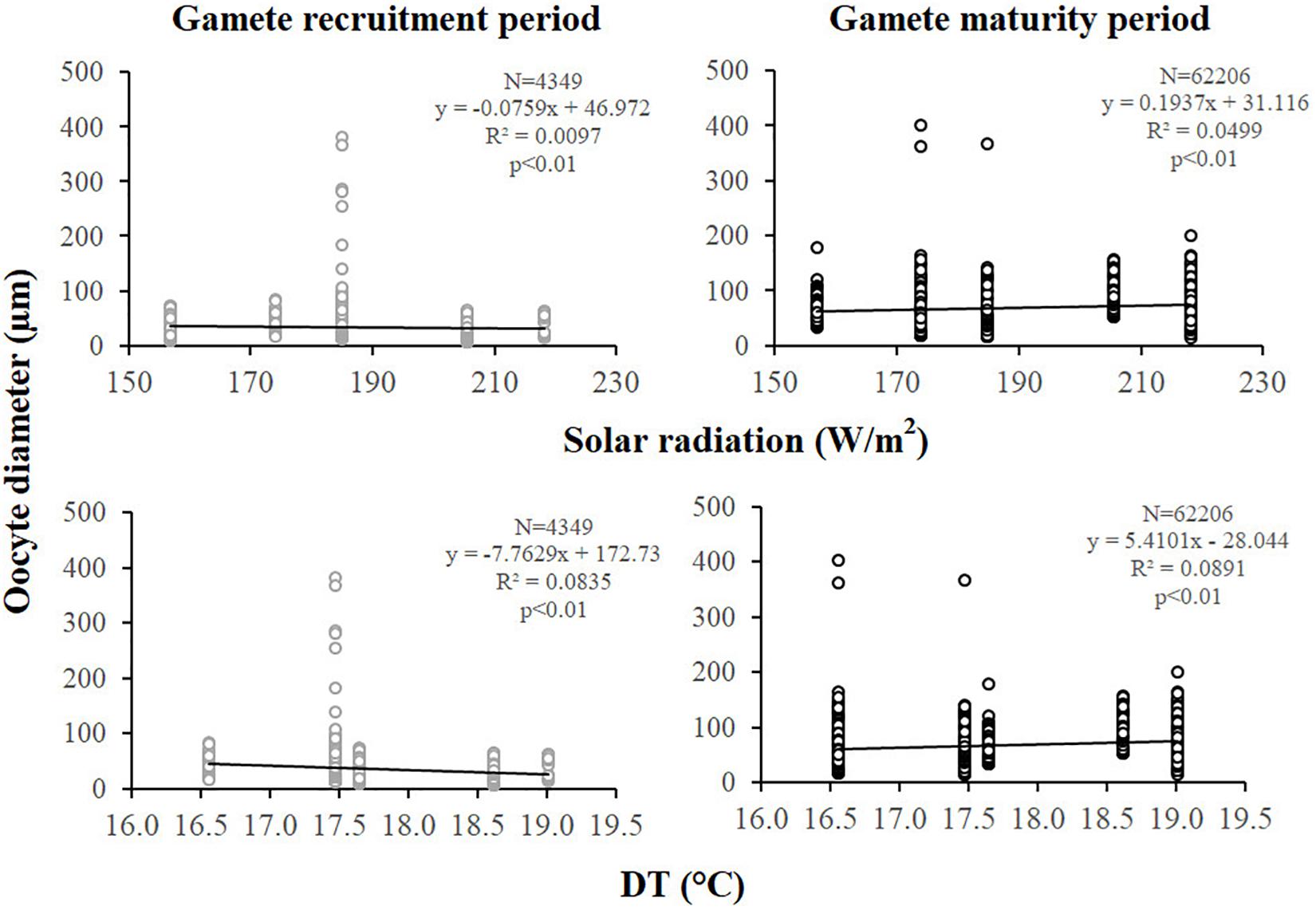
Figure 5. Oocytes. Correlation analysis between oocyte diameter and environmental parameters for gamete recruitment and maturity periods. N, oocyte number; R2, coefficient of determination; p, significance of p-value.
Spermary abundance during gamete recruitment period was significantly different among populations (Kruskal–Wallis test, p < 0.05; Supplementary Table S3 and Figure 6), showing a positive correlation with solar radiation (Spearman correlation test, p < 0.05; Supplementary Table S3 and Figure 7). The BEST BIO/ENV analysis showed no significant relation with environmental parameters (p > 0.05; Supplementary Table S5). Differently, spermary abundance of gamete maturity period and gonadal index of both reproductive periods did not vary among populations (Supplementary Table S3 and Figure 6) and these results were confirmed by the BEST BIO/ENV analysis (p > 0.05; Supplementary Table S5). The mean spermary diameter was significantly different during gamete recruitment and gamete maturity periods among populations (Kruskal–Wallis test, p < 0.001; Supplementary Table S3 and Figure 6). In southern populations, males showed smaller spermaries during February–April (Spearman correlation test, p < 0.01; Supplementary Table S3 and Figure 8) that became larger during May–July (Spearman correlation test, p < 0.01; Supplementary Table S3 and Figure 8). However, diameters did not correlate with any environmental variables using the BEST BIO/ENV analysis (p > 0.05; Supplementary Table S5).
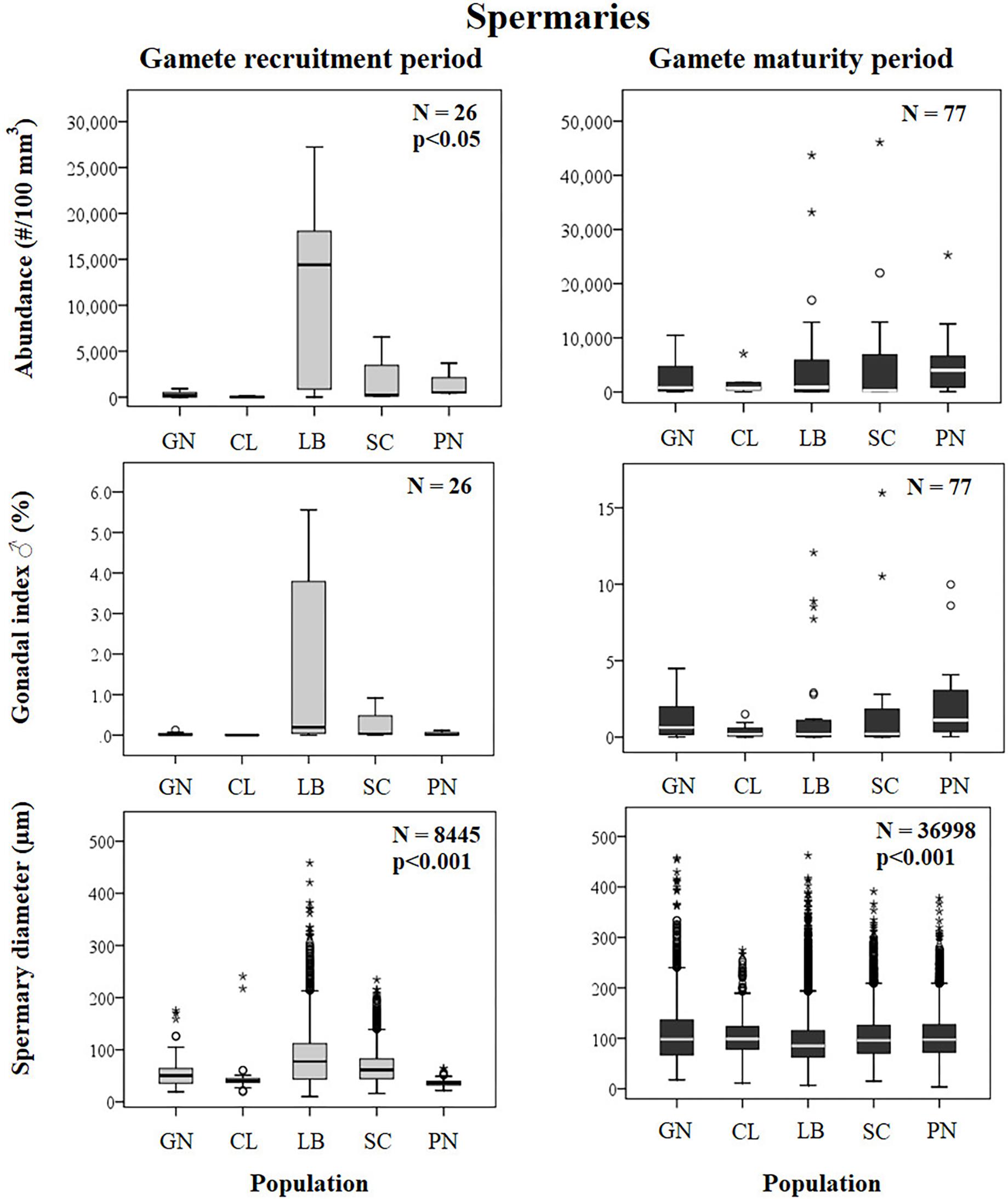
Figure 6. Spermaries. Boxplots of reproductive parameters per study site (GN Genova; CL Calafuria; LB Elba Island; SC Scilla; PN Pantelleria Island) during the gamete recruitment and gamete maturity periods. Each boxplot diagram shows the median (solid horizontal line), the first and third quartiles (box outline), the minimum and maximum values (whiskers) and the outliers (stars and circles). N, polyp number for abundance and gonadal index, spermary number for diameter; p, significance of the ANOVA/Kruskal–Wallis test.
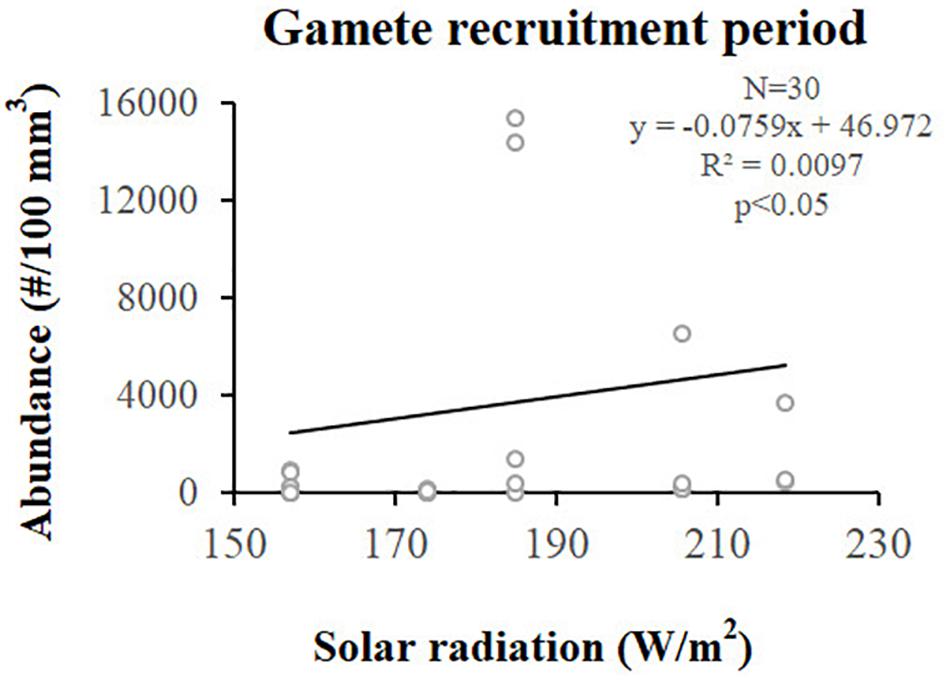
Figure 7. Spermaries. Correlation analysis between spermary abundance and solar radiation (W/m2) for gamete recruitment period. N, polyp number; R2, coefficient of determination; p, significance of p-value.
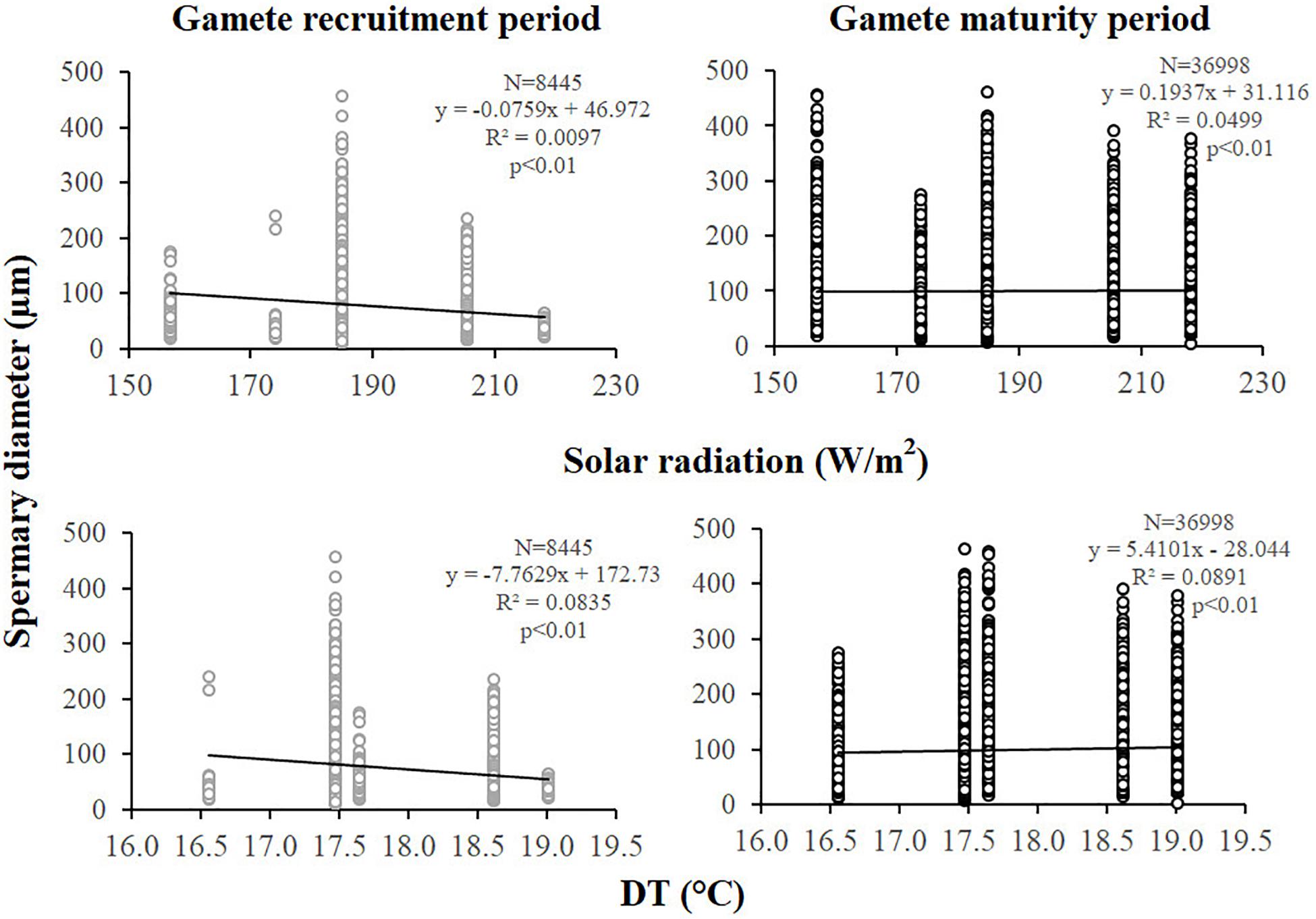
Figure 8. Spermaries. Correlation analysis between spermary diameter and environmental parameters for gamete recruitment and maturity period. N, spermary number; R2, coefficient of determination; p, significance of p-value.
Fertility of females and inactive individuals during both reproductive periods and fertility of males in gamete recruitment period, did not differ among all populations along the gradient (Supplementary Table S4 and Figure 9). Only male fertility during gamete maturity period was significantly different among populations, without any correlation with solar radiation and DT variations (Kruskal–Wallis test, p < 0.001; Supplementary Table S4 and Figure 9). These results were confirmed by the BEST BIO/ENV analysis (p > 0.05; Supplementary Table S5).
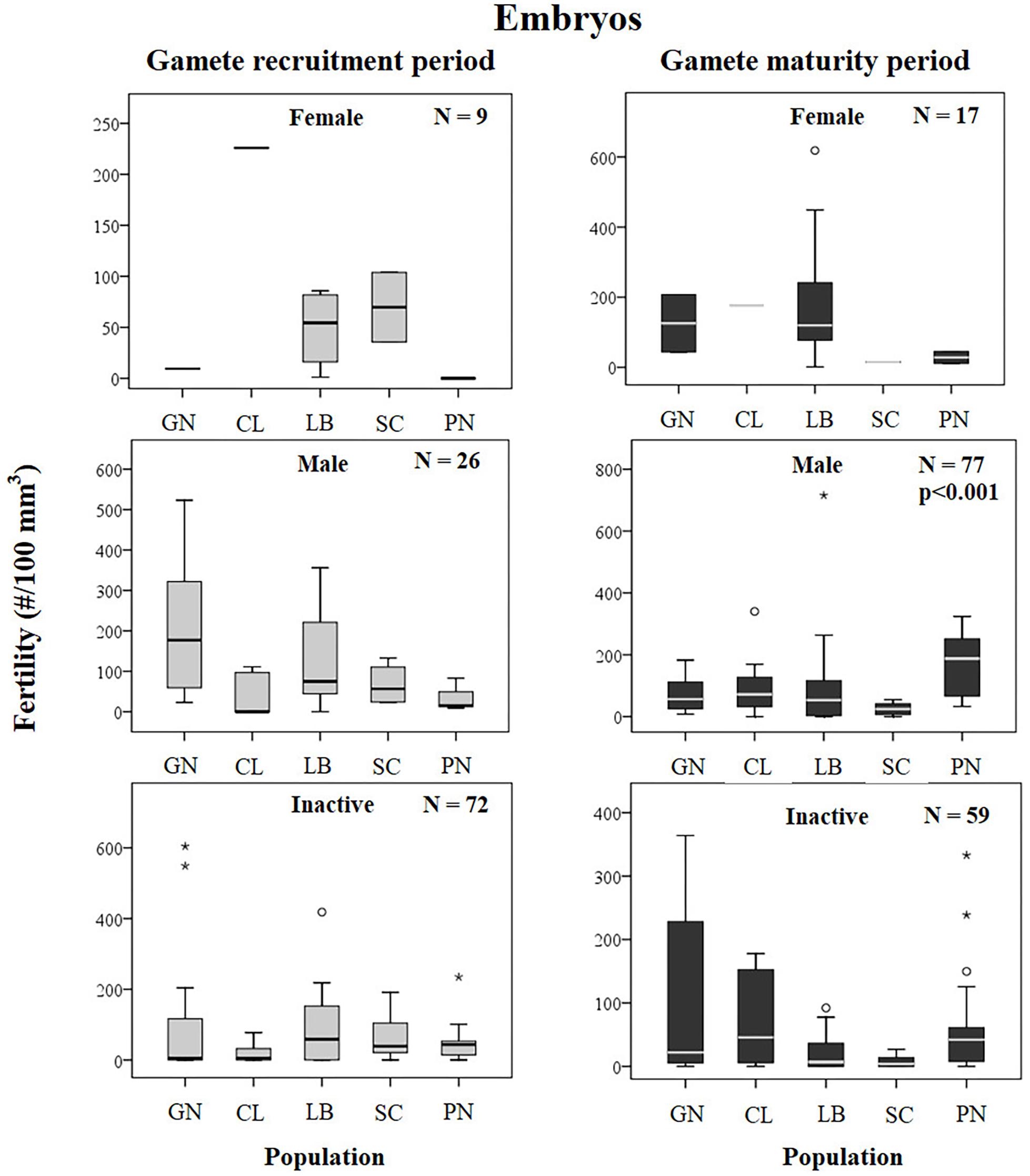
Figure 9. Embryos. Boxplots of reproductive parameters per study site (GN Genova; CL Calafuria; LB Elba Island; SC Scilla; PN Pantelleria Island) during the gamete recruitment and gamete maturity periods. Each boxplot diagram shows the median (solid horizontal line), the first and third quartiles (box outline), the minimum and maximum values (whiskers) and the outliers (stars and circles). N, number of polyps; p, significance of the ANOVA/Kruskal–Wallis test.
Discussion
All the analyzed populations of C. inornata along the latitudinal gradient displayed a strong male-biased sex ratio, the presence of sexually inactive individuals throughout the year, and a rapid oogenesis (mainly represented by oocytes smaller than 100 μm). The strong male-biased sex ratio and apparent lack of females could suggest that sexual reproduction, and hence larval recruitment, must be rare in these populations, highlighting the need to study the population genetics of C. inornata along this latitudinal gradient using microsatellite markers. All the populations of C. inornata analyzed for this study showed a spermatogenesis distinct in two reproductive periods (gamete recruitment and gamete maturity periods), and a reproductive cycle influenced by annual variation of seawater temperature and photoperiod, as previously reported for the population of Elba Island (Marchini et al., 2015). Relations between reproductive cycle and environmental conditions have been observed also for the temperate scleractinians B. europaea (Goffredo et al., 2002), L. pruvoti (Goffredo et al., 2006), Astroides calycularis (Caroselli et al., 2011), Cladocora caespitosa (Kružić et al., 2008; Kersting et al., 2013), and Balanophyllia elegans in the temperate waters of California (Fadlallah and Pearse, 1982; Beauchamp, 1993). At present, several studies assert that sexual reproduction of corals, including gametogenesis, gamete release, fertilization, and planulation, depends on seasonal environmental cues (Babcock et al., 1986; Harrison and Wallace, 1990; Penland et al., 2004; Van Woesik et al., 2006). The main environmental factors involved in the control of reproductive processes are seawater temperature and solar radiation, as they may exert a significant influence on physiological processes (Harrison and Wallace, 1990; Brown, 1997). Despite mean solar radiation and annual depth temperature were significantly different among sites, the seasonal trend of these parameters seems to not produce temporal shifts of the reproductive cycle of C. inornata. Indeed, all populations showed a similar periodicity for gamete development during both gamete recruitment and maturity periods, suggesting an overlap in reproductive seasonality of C. inornata along the latitudinal gradient. Similarly, B. europaea and L. pruvoti exhibited an overlap in gonadal development stages among the same populations analyzed for this study (Airi et al., 2014, 2017).
Since C. inornata is characterized by a strong male-biased sex ratio, the analysis of the oocytes was challenging and was performed on a small number of females. Polyps of C. inornata showed the same reproductive output in all the populations analyzed, since oocyte fecundity, spermary abundance (in the gamete maturity period), female, and male gonadal index were not affected by increasing temperature and solar radiation (Supplementary Tables S2, S3, and Figures 4, 6). However, for oocyte fecundity and gonadal index we cannot draw conclusions since in some populations (Genova, Calafuria, and Pantelleria in the gamete recruitment period and Calafuria in the gamete maturity period) we found only one female. The only parameter that varied in oogenesis was oocyte diameter in both reproductive periods, displaying a relation with both DT and solar radiation (Supplementary Table S2 and Figures 4, 5): southern populations showed smaller oocytes in the gamete recruitment period and larger ones in the gamete maturity period. This apparently contrasting result may suggest that temperature and solar radiation could even have a positive effect on oogenesis, since the oocyte size (in the period close to fertilization) seems greater in populations exposed to higher temperatures. Nevertheless, the percentage of the polyp body volume occupied by the “gonads” was homogeneous among populations along the gradient (Supplementary Table S2 and Figure 4). Furthermore, the correlation between size and the environmental parameters should be considered with caution since it could depend on the high amount of data analyzed, and the slope of the regression line is almost horizontal (Figure 5) without providing any ecological interpretation. In spermatogenesis, as for the oogenesis, spermary diameters displayed the same trend with environmental parameters (southern populations with smaller spermaries in the gamete recruitment period and larger ones in the gamete maturity period; Supplementary Table S3 and Figure 8) but also spermary abundance varied during the gamete recruitment period, increasing in the most irradiated populations (Supplementary Table S3 and Figure 7). This suggests that, during the initial stage of gametogenesis, when southern populations showed more but smaller spermaries than northern populations, C. inornata likely invested the same amount of energy along the gradient, since the percentage of body volume occupied by the “gonads” was the same (Supplementary Table S3 and Figure 6). Then, during the final stage of gametogenesis, spermaries of warmer populations become larger than coldest ones but again, the gonadal index was homogeneous along the latitudinal gradient (Supplementary Table S3 and Figures 6, 8), suggesting that increasing temperature and solar radiation did not affect reproductive energy investment. Moreover, to better understand possible relations between all the reproductive and the environmental parameters we did a BEST BIO/ENV with further variables (chlorophyll a, organic matter, and nutrients) which, however, has not confirmed any significant correlation. The absence of an evident relation with environmental parameters displayed by C. inornata confirms previous findings on population density, net calcification rate (that is linear extension rate × skeletal bulk density) and growth parameters of this species, which are not influenced by increasing solar radiation and temperature (Caroselli et al., 2015, 2016a,b). In fact, the reproductive capacity of a species is strongly influenced by the growth rate and the ecological dynamics of populations (Madin et al., 2012). Thus, C. inornata seems quite tolerant to environmental variations, as observed for L. pruvoti, studied along the same latitudinal gradient (Airi et al., 2017). On the contrary, the zooxanthellate coral B. europaea studied along the same gradient was less abundant and its populations less stable (with loss of young individuals) (Goffredo et al., 2007, 2008, 2009; Caroselli et al., 2011) and less efficient in using the energy invested for gonadal development with increasing temperature (Airi et al., 2014). Indeed, during the gamete recruitment period, the warmest populations showed more and larger oocytes, while at the end of the maturation process of the germ cells, these populations were characterized by the same number of oocytes compared to the populations with lower solar radiation and temperature. This reduced efficiency in energy allocation toward reproduction, highlighted in the warmer populations, could be due to an inhibition of the photosynthetic activity of the zooxanthellae that when exposed to extreme temperatures, reduces the available energy (Goffredo et al., 2007, 2008; Airi et al., 2014). However, C. inornata and L. pruvoti are both azooxanthellate corals and the absence of symbiosis with the dinoflagellates unicellular algae would make them less dependent to solar radiation and temperature variations.
The intriguing aspect that was found in the population of Elba Island of C. inornata is the presence of embryos in all stages of development throughout the year in females, males, and sexually inactive individuals. The same pattern was found in all populations studied along the latitudinal gradient. The absence of seasonality in embryo development and the presence of embryos before the fertilization period (gamete recruitment period) suggests the possibility of an agamic production of embryos in this species (Goffredo et al., 2012; Marchini et al., 2015). Agamic brooded embryos simultaneously with sexual reproduction is a peculiar trait of the genus Actinia (see A. equina: Orr et al., 2007; A. tenebrosa: Black and Johnson, 1979; Sherman et al., 2007; Sherman and Ayre, 2008; A. bermudensis: Monteiro et al., 1998) that, in some case, can display different levels of clonality in response to the habitat heterogeneity (based on a different number of microhabitats or different levels of disturbances) (Sherman et al., 2007; Sherman and Ayre, 2008). Fertility (number of embryos per body volume unit) of C. inornata was not related to the environmental parameters considered for this study (Supplementary Table S4 and Figure 9), confirming that reproduction of this temperate coral is unaffected by increasing temperature and solar radiation. A species with a mixed strategy of sexual and asexual reproduction, seems to merge the evolutionary advantages of both propagation modes into a single organism. Asexual reproduction allows the rapid propagation of many individuals well adapted to local conditions (Smith, 1978) and allow the species to reproduce in absence of both sexes (Francis, 1979). In contrast, sexual reproduction contributes to the formation of new genotypes (Williams, 1975), allowing organisms to adapt to the surrounding environment variations (Van Woesik, 2010). This could be a reproductive assurance for C. inornata, which may favor one or another reproductive tactic based on the different surrounding habitats. Moreover, the absence of symbiosis with zooxanthellae that are sensitive to increasing temperature, may allow C. inornata to cope more successfully than zooxanthellate corals with global environmental changes.
Data Availability Statement
All data are available from the corresponding author upon request.
Author Contributions
SG conceived and designed the experiments and contributed to the reagents, materials, and analysis tools. CM and SG collected the samples. CM, VA, GT, and EG analyzed the data. CM, GF, ZD, and SG wrote the manuscript. GF and ZD gave conceptual advice.
Funding
The research leading to these results has received funding from the European Research Council under the European Union’s Seventh Framework Programme (FP7/2007–2013)/ERC Grant Agreement No. 249930 – CoralWarm: Corals and Global Warming: the Mediterranean versus the Red Sea. The funders had no role in study design, data collection and analysis, decision to publish, or preparation of the manuscript.
Conflict of Interest
The authors declare that the research was conducted in the absence of any commercial or financial relationships that could be construed as a potential conflict of interest.
Acknowledgments
We would like to thank E. Caroselli, F. Gizzi, S. Prantoni, S. Branchini, M. Ghelia, M. Pisconti, A. Picariello, S. Guerrieri, F. Croci, F. Fasoli and F. Sesso who collaborated in the underwater activities. We would also like to thank Centro Immersioni Pantelleria, Il Pesciolino, Bubble Lounge Diving, and Submaldive, which supplied logistic assistance in the field and The Scientific Diving School (www.sdseducational.org) which supplied scientific, technical, and logistic support. The experiments were complied with current Italian law.
Supplementary Material
The Supplementary Material for this article can be found online at: https://www.frontiersin.org/articles/10.3389/fmars.2019.00785/full#supplementary-material
Footnotes
- ^ Available to http://www.mareografico.it
- ^ Available to http://www.cmsaf.eu
References
Airi, V., Gizzi, F., Falini, G., Levy, O., Dubinsky, Z., and Goffredo, S. (2014). Reproductive efficiency of a mediterranean endemic zooxanthellate coral decreases with increasing temperature along a wide latitudinal gradient. PLoS One 9:e91792. doi: 10.1371/journal.pone.0091792
Airi, V., Prantoni, S., Calegari, M., Lisini Baldi, V., Gizzi, F., Marchini, C., et al. (2017). Reproductive output of a non-zooxanthellate temperate coral is unaffected by temperature along an extended latitudinal gradient. PLoS One 12:e0171051. doi: 10.1371/journal.pone.0171051
Albright, R., and Mason, B. (2013). Projected near-future levels of temperature and pCO2 reduce coral fertilization success. PLoS One 8:e56468. doi: 10.1371/journal.pone.0056468
Anderson, M. J., Gorley, R. N., and Clarke, K. R. (2008). PERMANOVA+ for PRIMER: Guide to Software and Statistical Methods. Plymouth: PRIMER-E Ltd.
Babcock, R. C., Bull, G. D., Harrison, P. L., Heyward, A. J., Oliver, J. K., Wallace, C. C., et al. (1986). Synchronous spawnings of 105 scleractinian coral species on the great barrier reef. Mar. Biol. 90, 379–394. doi: 10.1007/BF00428562
Bassim, K. M., Sammarco, P. W., and Snell, T. L. (2002). Effects of temperature on success of (self and non-self) fertilization and embryogenesis in Diploria strigosa (Cnidaria, Scleractinia). Mar. Biol. 140, 479–488. doi: 10.1007/s00227-001-0722-4
Beauchamp, K. A. (1993). Gametogenesis, brooding and plantation in laboratory populations of a temperate scleractinian coral Balanophyllia elegans maintained under contrasting photoperiod regimes. Invertebr. Reprod. Dev. 23, 171–182. doi: 10.1080/07924259.1993.9672312
Black, R., and Johnson, M. S. (1979). Asexual viviparity and population genetics of Actinia tenebrosa. Mar. Biol. 53, 27–31. doi: 10.1007/BF00386526
Brown, B. E. (1997). Coral bleaching: causes and consequences. Coral Reefs 16, S129–S138. doi: 10.1007/s003380050249
Cairns, S. D. (1999). Species richness of recent Scleractinia. Atoll Res. Bull. 459, 1–46. doi: 10.5479/si.00775630.459.1
Caroselli, E., Brambilla, V., Ricci, F., Mattioli, G., Levy, O., Falini, G., et al. (2016a). Inferred calcification rate of a temperate azooxanthellate caryophylliid coral along a wide latitudinal gradient. Coral Reefs 35, 919–928. doi: 10.1007/s00338-016-1422-3
Caroselli, E., Ricci, F., Brambilla, V., Mattioli, G., Levy, O., Falini, G., et al. (2016b). Relationships between growth, population dynamics, and environmental parameters in the solitary non-zooxanthellate scleractinian coral Caryophyllia inornata along a latitudinal gradient in the Mediterranean Sea. Coral Reefs 35, 507–519. doi: 10.1007/s00338-015-1393-9
Caroselli, E., Nanni, V., Levy, O., Falini, G., Dubinsky, Z., and Goffredo, S. (2015). Latitudinal variations in biometry and population density of a mediterranean solitary coral. Limnol. Oceanogr. 60, 1356–1370. doi: 10.1002/lno.10100
Caroselli, E., Prada, F., Pasquini, L., Marzano, F. N., Zaccanti, F., Falini, G., et al. (2011). Environmental implications of skeletal micro-density and porosity variation in two Scleractinian corals. Zoology 114, 255–264. doi: 10.1016/j.zool.2011.04.003
Chui, A. P. Y., and Ang, P. (2015). Elevated temperature enhances normal early embryonic development in the coral Platygyra acuta under low salinity conditions. Coral Reefs 34, 461–469. doi: 10.1007/s00338-014-1247-x
Doherty, P., and Fowler, T. (1994). An empirical test of recruitment limitation in a coral reef fish. Science 263, 935–939. doi: 10.1126/science.263.5149.935
Fadlallah, Y. H., and Pearse, J. S. (1982). Sexual reproduction in solitary corals: overlapping oogenic and brooding cycles, and benthic planulas in Balanophyllia elegans. Mar. Biol. 71, 223–231. doi: 10.1007/BF00397039
Feely, R. A., Sabine, C. L., Lee, K., Berelson, W., Kleypas, J., Fabry, V. J., et al. (2004). Impact of anthropogenic CO2 on the CaCO3 system in the oceans. Science 305, 362–366. doi: 10.1126/science.1097329
Field, C. B., Barros, V., Stocker, T. F., Dahe, Q., Jon Dokken, D., Ebi, K. L., et al. (2012). Managing the Risks of Extreme Events and Disasters to Advance Climate Change Adaptation: Special Report of the Intergovernmental Panel on Climate Change. Cambridge, MA: Cambridge University Press.
Francis, L. (1979). Contrast between solitary and clonal lifestyles in the sea anemone Anthopleura elegantissima. Integr. Comp. Biol. 19, 669–681. doi: 10.1093/icb/19.3.669
Goffredo, S., Airi, V., Radetić, J., and Zaccanti, F. (2006). Sexual reproduction of the solitary sunset cup coral Leptopsammia pruvoti (Scleractinia, Dendrophylliidae) in the Mediterranean. 2. Quantitative aspects of the annual reproductive cycle. Mar. Biol. 148, 923–931. doi: 10.1007/s00227-005-0137-8
Goffredo, S., Arnone, S., and Zaccanti, F. (2002). Sexual reproduction in the Mediterranean solitary coral Balanophyllia europaea (Scleractinia, Dendrophylliidae). Mar. Ecol. Prog. Ser. 229, 83–94. doi: 10.3354/meps229083
Goffredo, S., Caroselli, E., Mattioli, G., Pignotti, E., Dubinsky, Z., and Zaccanti, F. (2009). Inferred level of calcification decreases along an increasing temperature gradient in a Mediterranean endemic coral. Limnol. Oceanogr. 54, 930–937. doi: 10.4319/lo.2009.54.3.0930
Goffredo, S., Caroselli, E., Mattioli, G., Pignotti, E., and Zaccanti, F. (2008). Relationships between growth, population structure and sea surface temperature in the temperate solitary coral Balanophyllia europaea (Scleractinia, Dendrophylliidae). Coral Reefs 27, 623–632. doi: 10.1007/s00338-008-0362-y
Goffredo, S., Caroselli, E., Pignotti, E., Mattioli, G., and Zaccanti, F. (2007). Variation in biometry and population density of solitary corals with solar radiation and sea surface temperature in the Mediterranean Sea. Mar. Biol. 152, 351–361. doi: 10.1007/s00227-007-0695-z
Goffredo, S., Marchini, C., Rocchi, M., Airi, V., Caroselli, E., Falini, G., et al. (2012). Unusual pattern of embryogenesis of Caryophyllia inornata (scleractinia, caryophylliidae) in the mediterranean sea: maybe agamic reproduction? J. Morphol. 273, 943–956. doi: 10.1002/jmor.20039
Goffredo, S., Radetić, J., Airi, V., and Zaccanti, F. (2005). Sexual reproduction of the solitary sunset cup coral Leptopsammia pruvoti (Scleractinia: Dendrophylliidae) in the Mediterranean. 1. Morphological aspects of gametogenesis and ontogenesis. Mar. Biol. 147, 485–495. doi: 10.1007/s00227-005-1567-z
Harley, C. D. G., Hughes, A. R., Hultgren, K. M., Miner, B. G., Sorte, C. J. B., Thornber, C. S., et al. (2006). The impacts of climate change in coastal marine systems. Ecol. Lett. 9, 228–241. doi: 10.1111/j.1461-0248.2005.00871.x
Harrison, P. L., and Wallace, C. C. (1990). “Reproduction, dispersal and recruitment of scleractinian corals,” in Ecosystems of the World 25, ed. Z. Dubinsky (Amsterdam: Elsevier), 133–207.
Kersting, D. K., Casado, C., López-Legentil, S., and Linares, C. (2013). Unexpected patterns in the sexual reproduction of the Mediterranean scleractinian coral Cladocora caespitosa. Mar. Ecol. Prog. Ser. 486, 165–171. doi: 10.3354/meps10356
Korta, M., Murua, H., Kurita, Y., and Kjesbu, O. S. (2010). How are the oocytes recruited in an indeterminate fish? Applications of stereological techniques along with advanced packing density theory on European hake (Merluccius merluccius L.). Fish. Res. 104, 56–63. doi: 10.1016/j.fishres.2010.01.010
Krupp, D. A., Hollingsworth, L. L., and Peterka, J. (2006). “Elevated temperature sensitivity of fertilization and early development in the mushroom coral Fungia scutaria Lamarck 1801,” in Proceedings of the 10th International Coral Reef Symposium, Vol. 1, (Gurugram: Okinawa), 71–77.
Kružić, P., Žuljević, A., and Nikolić, V. (2008). Spawning of the colonial coral Cladocora caespitosa (Anthozoa, Scleractinia) in the Southern Adriatic Sea. Coral Reefs 27, 337–341. doi: 10.1007/s00338-007-0334-7
Lejeusne, C., Chevaldonné, P., Pergent-Martini, C., Boudouresque, C. F., and Pérez, T. (2010). Climate change effects on a miniature ocean: the highly diverse, highly impacted Mediterranean Sea. Trends Ecol. Evol. 25, 250–260. doi: 10.1016/j.tree.2009.10.009
Linares, C., Coma, R., and Zabala, M. (2008). Effects of a mass mortality event on gorgonian reproduction. Coral Reefs 27, 27–34. doi: 10.1007/s00338-007-0285-z
Lowerre-Barbieri, S. K., Ganias, K., Saborido-Rey, F., Murua, H., and Hunter, J. R. (2011). Reproductive timing in marine fishes: variability, temporal scales, and methods. Mar. Coast. Fish. 3, 71–91. doi: 10.1080/19425120.2011.556932
Ludbrook, J. (1991). Practical statistics for medical research. Aust. N. Z. J. Surg. 61, 963–964. doi: 10.1111/j.1445-2197.1991.tb00019.x
Madin, J. S., Hughes, T. P., and Connolly, S. R. (2012). Calcification, storm damage and population resilience of tabular corals under climate change. PLoS One 7:e46637. doi: 10.1371/journal.pone.0046637
Marchini, C., Airi, V., Fontana, R., Tortorelli, G., Rocchi, M., Falini, G., et al. (2015). Annual reproductive cycle and unusual embryogenesis of a temperate coral in the Mediterranean Sea. PLoS One 10:e0141162. doi: 10.1371/journal.pone.0141162
Monteiro, F. A., Russo, C. A. M., and Solé-Cava, A. M. (1998). Genetic evidence for the asexual origin of small individuals found in the coelenteron of the sea anemone Actinia bermudensis mcmurrich. Bull. Mar. Sci. 63, 257–264.
Negri, A. P., Marshall, P. A., and Heyward, A. J. (2007). Differing effects of thermal stress on coral fertilization and early embryogenesis in four Indo Pacific species. Coral Reefs 26, 759–763. doi: 10.1007/s00338-007-0258-2
Nozawa, Y., and Harrison, P. L. (2007). Effects of elevated temperature on larval settlement and post-settlement survival in scleractinian corals, Acropora solitaryensis and Favites chinensis. Mar. Biol. 152, 1181–1185. doi: 10.1007/s00227-007-0765-2
Olea, R. A., and Pawlowsky-Glahn, V. (2009). Kolmogorov-Smirnov test for spatially correlated data. Stoch. Environ. Res. Risk Assess. 23, 749–757. doi: 10.1007/s00477-008-0255-1
Orr, J., Thorpe, J., and Carter, M. (2007). Biochemical genetic confirmation of the asexual reproduction of brooded offspring in the Sea Anemone Actinia equine. Mar. Ecol. Prog. Ser. 7, 227–229. doi: 10.3354/meps007227
Paxton, C. W., Baria, M. V. B., Weis, V. M., and Harii, S. (2016). Effect of elevated temperature on fecundity and reproductive timing in the coral Acropora digitifera. Zygote 24, 511–516. doi: 10.1017/S0967199415000477
Penland, L., Kloulechad, J., Idip, D., and Van Woesik, R. (2004). Coral spawning in the western Pacific Ocean is related to solar insolation: evidence of multiple spawning events in Palau. Coral Reefs 23, 133–140. doi: 10.1007/s00338-003-0362-x
Potvin, C., and Roff, D. A. (1993). Distribution-free and robust statistical methods: viable alternatives to parametric statistics? Ecology 74, 1617–1628. doi: 10.2307/1939920
Randall, C. J., and Szmant, A. M. (2009). Elevated temperature affects development, survivorship, and settlement of the elkhorn coral, Acropora palmata (Lamarck 1816). Biol. Bull. 217, 269–282. doi: 10.1086/BBLv217n3p269
Sabine, C. L., Feely, R. A., Gruber, N., Key, R. M., Lee, K., Bullister, J. L., et al. (2004). The oceanic sink for anthropogenic CO2. Science 305, 367–371. doi: 10.1126/science.1097403
Sherman, C. D. H., and Ayre, D. J. (2008). Fine-scale adaptation in a clonal sea anemone. Evolution 62, 1373–1380. doi: 10.1111/j.1558-5646.2008.00375.x
Sherman, C. D. H., Peucker, A. J., and Ayre, D. J. (2007). Do reproductive tactics vary with habitat heterogeneity in the intertidal sea anemone Actinia tenebrosa? J. Exp. Mar. Bio. Ecol. 340, 259–267. doi: 10.1016/j.jembe.2006.09.016
Smith, J. M. (1978). GRH volume 32 issue 3 cover and back matter. Genet. Res. 32, b1–b9. doi: 10.1017/S0016672300018693
Van Woesik, R. (2010). Calm before the spawn: global coral spawning patterns are explained by regional wind fields. Proc. R. Soc. B Biol. Sci. 277, 715–722. doi: 10.1098/rspb.2009.1524
Van Woesik, R., Lacharmoise, F., and Köksal, S. (2006). Annual cycles of solar insolation predict spawning times of Caribbean corals. Ecol. Lett. 9, 390–398. doi: 10.1111/j.1461-0248.2006.00886.x
Ward, S. (1995). The effect of damage on the growth, reproduction and storage of lipids in the Scleractinian coral Pocillopora damicornis (Linnaeus). J. Exp. Mar. Bio. Ecol. 187, 193–206. doi: 10.1016/0022-0981(94)00180-L
Zibrowius, H. (1978). No TitleLes scléractiniaires des grottes sous-marines en Méditerranée et dans l’Atlantique nord-oriental (Portugal, Madère, Canaries, Açores). Mar. Ecol. 40, 516–545.
Keywords: Mediterranean Sea, global warming, scleractinia, gametogenesis, embryogenesis, asexual reproduction
Citation: Marchini C, Tortorelli G, Guidi E, Airi V, Falini G, Dubinsky Z and Goffredo S (2020) Reproduction of the Azooxanthellate Coral Caryophyllia inornata Is Not Affected by Temperature Along an 850 km Gradient on the Western Italian Coast. Front. Mar. Sci. 6:785. doi: 10.3389/fmars.2019.00785
Received: 28 June 2019; Accepted: 05 December 2019;
Published: 08 January 2020.
Edited by:
Raquel Peixoto, Federal University of Rio de Janeiro, BrazilReviewed by:
Claudia Pogoreutz, Universität Konstanz, GermanyAnna Roik, GEOMAR – Helmholtz Centre for Ocean Research Kiel, Germany
Copyright © 2020 Marchini, Tortorelli, Guidi, Airi, Falini, Dubinsky and Goffredo. This is an open-access article distributed under the terms of the Creative Commons Attribution License (CC BY). The use, distribution or reproduction in other forums is permitted, provided the original author(s) and the copyright owner(s) are credited and that the original publication in this journal is cited, in accordance with accepted academic practice. No use, distribution or reproduction is permitted which does not comply with these terms.
*Correspondence: Stefano Goffredo, s.goffredo@unibo.it