- 1Unidad Académica de Ecología y Biodiversidad Acuática, Instituto de Ciencias del Mar y Limnología, Universidad Nacional Autónoma de México, Mexico City, Mexico
- 2Posgrado en Ciencias del Mar y Limnología, Universidad Nacional Autónoma de México, Mexico City, Mexico
Nitrogen (N) is a key element for life in the oceans. It controls primary productivity in many parts of the global ocean, consequently playing a crucial role in the uptake of atmospheric carbon dioxide. The marine N cycle is driven by multiple biogeochemical transformations mediated by microorganisms, including processes contributing to the marine fixed N pool (N2 fixation) and retained N pool (nitrification, assimilation, and dissimilatory nitrate reduction to ammonia), as well as processes contributing to the fixed N loss (denitrification, anaerobic ammonium oxidation and nitrite-dependent anaerobic methane oxidation). The N cycle maintains the functioning of marine ecosystems and will be a crucial component in how the ocean responds to global environmental change. In this review, we summarize the current understanding of the marine microbial N cycle, the ecology and distribution of the main functional players involved, and the main impacts of anthropogenic activities on the marine N cycle.
Introduction
0Nitrogen (N) is a key element for life and the functioning of marine ecosystems. It plays a crucial role in marine biogeochemistry, and because of its connections to the cycles of other elements such as carbon (C) it has a strong impact on Earth’s climate (Gruber, 2008; Voss et al., 2013). Fixed N limits marine productivity in many parts of the global ocean. Its availability also regulates the strength of the biological pump, one of the mechanisms contributing to oceanic uptake of atmospheric C dioxide (CO2) (Falkowski, 1997).
N is present in different oxidation states in the ocean, ranging from -III in reduced forms like ammonium (NH4+) and organic N to +V in fully oxidized nitrate (NO3–), which highlights its importance as both an electron acceptor and donor for energy metabolism in marine ecosystems (Figure 1). Microorganisms mainly mediate the redox transformations of N, changing the concentrations of N compounds in the environment. The major sources of fixed N for the ocean are biological N2 fixation (BNF) and atmospheric deposition, while the major sinks are denitrification and anaerobic ammonium oxidation (anammox) (Gruber and Galloway, 2008). Because alterations of this balance caused by anthropogenic activity may pose significant impact on marine ecosystem health, biodiversity and climate change, the study of microbial communities involved in marine N cycling has gained great interest in recent years (Lam and Kuypers, 2011; Zehr and Kudela, 2011; Voss et al., 2013).
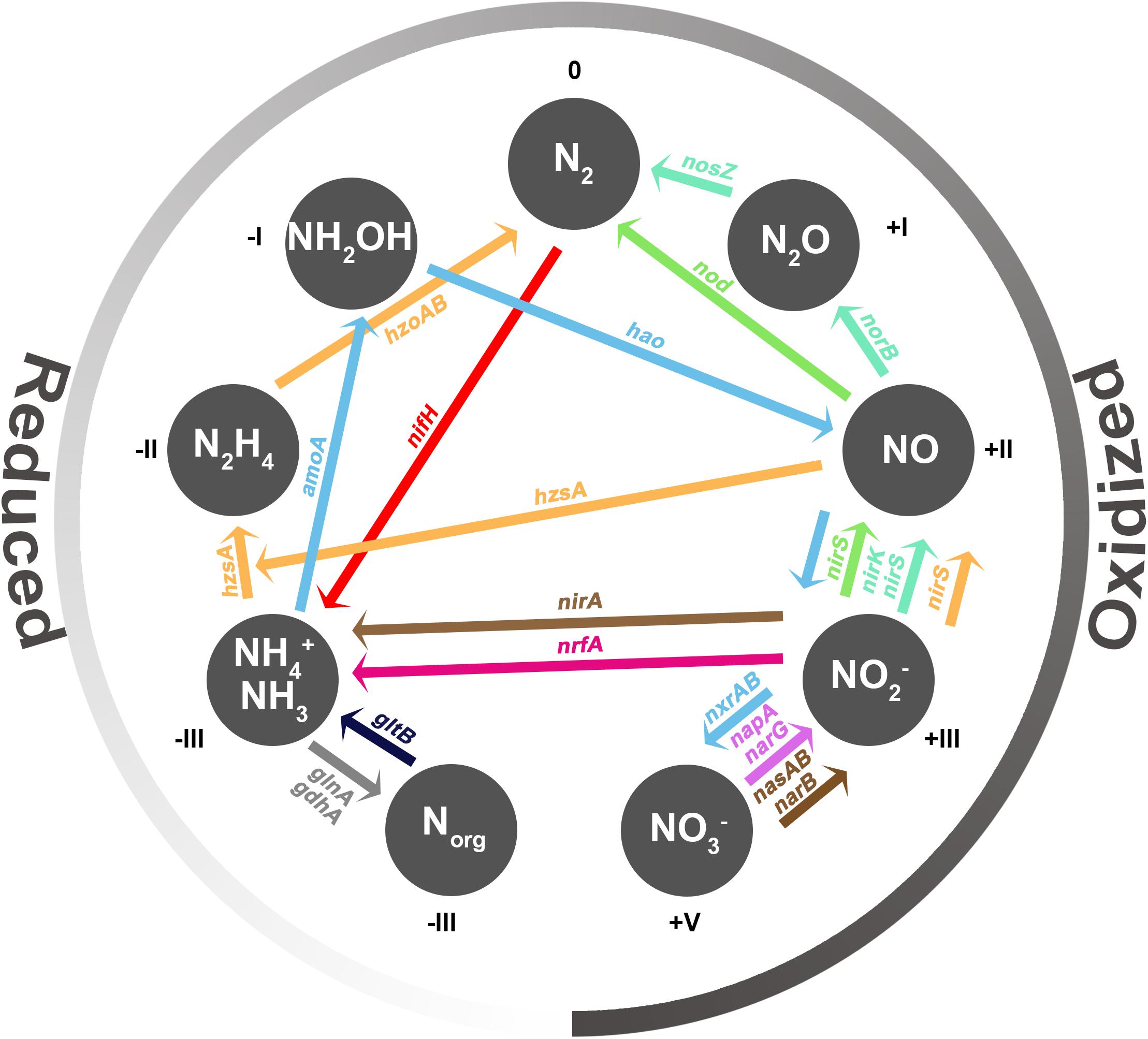
Figure 1. Nitrogen species involved in N cycling and its transformations. Each gray circle represents a N species, and the number next to each N species indicates its oxidation state. Colored arrows represent each N transformation and the main marker genes involved: N2 fixation (nifH) in red, nitrification (amoA, hao, nxrA, nxrB) in light blue, nitrate reduction (narG, napA) in violet, DNRA (nrfA) in magenta, assimilatory nitrate and nitrite reduction to ammonia (nasA, nasB, narB, nirA) in brown, ammonium assimilation (glnA, gdhA) in gray, remineralization (gltB) in dark blue, denitrification (nirK, nirS, norB, nosZ) in aquamarine, anammox (nirS, hzsA, hzoA, hzoB) in orange, and N-damo (nirS, nod) in green.
Microbial communities related to marine N cycling have been studied extensively using both culture-dependent and independent techniques. These technologies have allowed the attainment of a wealth of genomic data, revealing enormous metabolic versatility within N-transforming microorganisms. Furthermore, the study of genes encoding key metabolic proteins along with rate measurements of N processes has provided important discoveries about the genomic potential of microorganisms participating in N processes, as well as their biogeography and activity in marine systems (Table 1; Lam and Kuypers, 2011; Devol, 2015; Damashek and Francis, 2018). However, some discrepancies have been found between different studies, which may be due to the use of different methodologies (e.g., primer-based approaches versus untargeted approaches with meta-omic signatures or direct incubation experiments versus isotopic mass balance approach). As a contribution to this understanding, this review provides a general survey of the key microbial processes that comprise the marine N cycle and the N genes involved that are used as biomarkers, as well as the ecology and distribution of participating microorganisms in different marine ecosystems. We also identify several of the knowledge gaps that we still face in the study of microbial marine N processes. We end our review with a discussion of the impacts of anthropogenic activity on the microbially mediated marine N cycle.
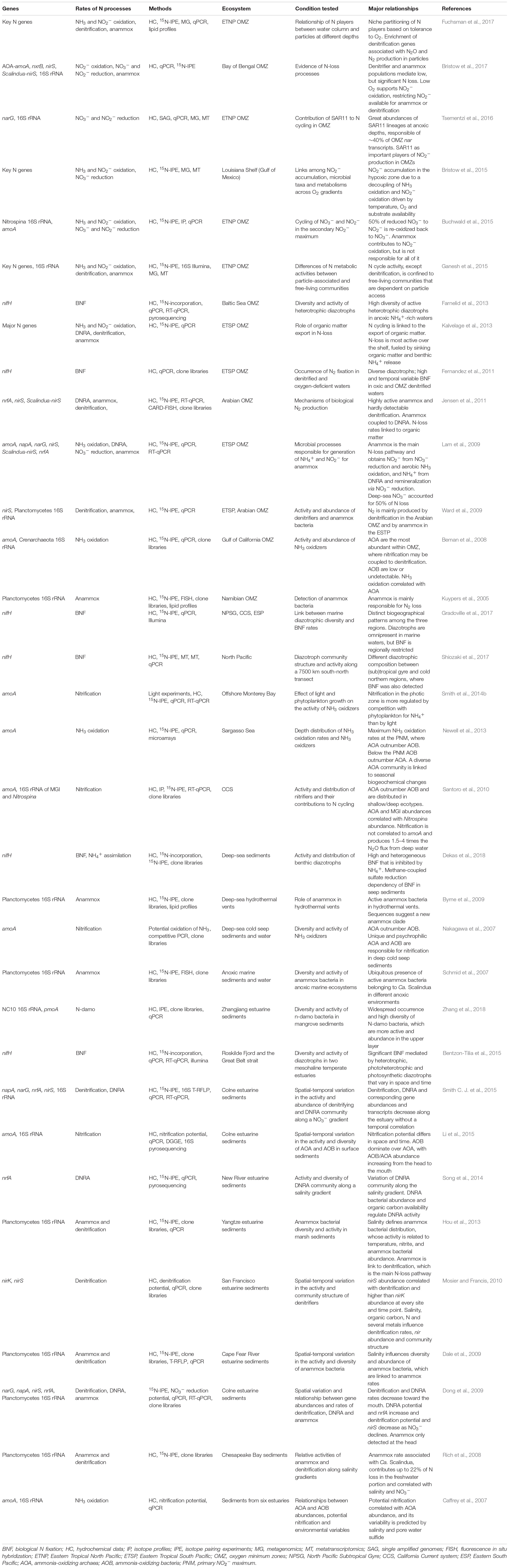
Table 1. Representative studies using N cycling genes and rate measurements of N processes in marine ecosystems: ocean minimum zones, open ocean, deep-sea, and estuarine sediments.
Microbial Processes in the Marine N Cycle
The marine N cycle is driven by multiple microbial transformations, and N-converting enzymes are found in very diverse microorganisms globally distributed throughout marine systems (Figure 2). The microbial transformations of N compounds have important effects on the balance of marine and global N budgets. Thus, understanding how they occur, their distribution and the factors making them possible is essential to comprehend the fate of marine ecosystems and the future Earth. In this section, we give a general overview of the biochemistry and ecology of the main marine N processes and the microorganisms involved in them. In order to emphasize the role of these processes in the marine and global budgets, we divide them into processes contributing to the marine fixed N (BNF) and retained N pool (derived from processes that transform one N species to another without causing its loss from the system) and processes contributing to the fixed N loss.
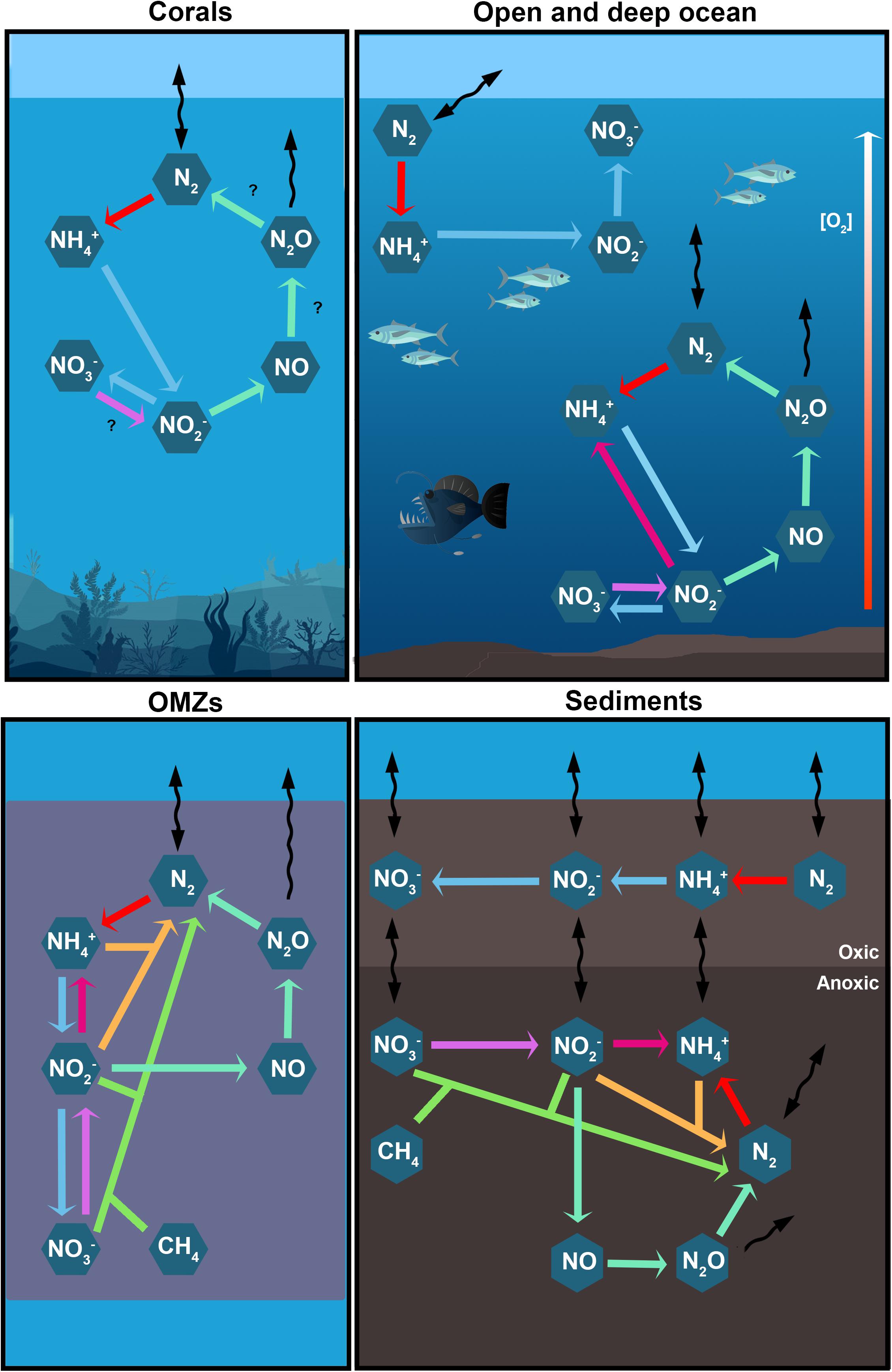
Figure 2. The main studied processes of the N cycle in different marine environments. Every colored arrow represents a N transformation: N2 fixation (red), nitrification (light blue), nitrate reduction (violet), DNRA (magenta), denitrification (aquamarine), N-damo (green), and anammox (orange). Black curved arrows represent physical processes such as advection and diffusion.
Bioavailable N-Gain and Retention Processes
BNF is the only biotic process adding N to marine systems and it has been estimated of ∼140 Tg N yr–1 (Eugster and Gruber, 2012; Luo et al., 2012). However, this estimate remains highly uncertain due to the incomplete knowledge of the organisms involved and their ecological controls (Gruber, 2016; Landolfi et al., 2018). Other microbial N processes such as nitrification, dissimilatory nitrate reduction to ammonia (DNRA) and N assimilation retain the fixed N. Most marine microorganisms can assimilate inorganic N forms, and also dissolved organic N compounds in estuaries and coastal waters (Damashek and Francis, 2018) and even in oligotrophic open ocean waters (Benavides et al., 2017b). Thus, addressing this process is beyond the scope of this review, but it is covered elsewhere (e.g., Allen et al., 2001; Zehr and Ward, 2002; Martiny et al., 2009; Zehr and Kudela, 2011; Damashek and Francis, 2018).
Biological N2 Fixation (BNF)
Marine N2 fixers (diazotrophs) convert dissolved N2 gas into bioavailable ammonia (NH3). This is an intensely energy-requiring process that only a small but diverse group of bacteria and archaea are able to carry out. Marine diazotrophs mainly include non-heterocystous filamentous cyanobacteria (e.g., Trichodesmium, Oscillatoria, Lyngbya), heterocystous filamentous cyanobacteria (e.g., Aphanizomenon, Nodularia), diatom symbiotic cyanobacteria (e.g., Richelia, Calothrix), and unicellular cyanobacteria (Ca. Atelocyanobacterium thalassa [UCYN-A], Crocosphaera watsonii [UCYN-B] and Cyanothece [UCYN-C]) (Villareal, 1991; Capone, 1997; Zehr et al., 2001; Thompson et al., 2012). UCYN-A is further divided into at least four sublineages; two of them (UCYN-A1 and UCYN-A2) live symbiotically with distinct prymnesiophyte microalgae (Zehr et al., 2016, and references therein). Other marine diazotrophs include heterotrophic bacteria (e.g., Klebsiella, Vibrio), phototrophic bacteria (e.g., Chlorobium, Chromatium, Rhodospirillum), strict anaerobes (e.g., Clostridium, Desulfovibrio), iron (Fe) oxidizers (e.g., Thiobacillus), methanogenic Euryarchaeota, and even members of Planctomycetes (Zehr and Paerl, 2008; Bombar et al., 2016; Delmont et al., 2018). These microorganisms share a common feature: the nitrogenase complex, which catalyzes N2 fixation.
Nitrogenase is composed of two metalloproteins: the molybdenum (Mo)–Fe protein (dinitrogenase) encoded in the nifDK genes; and the Fe protein (dinitrogenase reductase) encoded in the nifH gene. Alternative nitrogenases replace Mo with vanadium or solely contain Fe, and are encoded in the vnf and anf genes, respectively (Zehr et al., 2003). The nifH gene is the preferred biomarker in the study of diazotroph diversity because it remains highly conserved (Zehr et al., 2003; Turk et al., 2011; Jayakumar et al., 2012).
Factors affecting BNF in marine systems
Oxygen (O2), light, temperature, inorganic N forms, phosphorus (P), Fe, and organic matter are the main factors that affect marine diazotroph distribution. Nitrogenase is sensitive to O2, and diazotrophs have developed numerous protective strategies against this. For example, several cyanobacteria generate specialized N2-fixing cells called heterocysts that provide an almost anoxic environment. Temporal separation is another protection mechanism; most photosynthetic diazotrophs and certain proteobacterial diazotrophs fix N2 at night (Moisander et al., 2014), whereas Trichodesmium and heterocyst-forming cyanobacteria fix N2 during the day (Staal et al., 2007). The ability of Trichodesmium to fix N2 during daytime remains enigmatic. Several mechanisms have been considered, such as its nitrogenase is confined to specialized cells called diazocytes (Bergman and Carpenter, 1991), the photoreduction of O2 to H2O in the photosystem I (the Mehler reaction) (Milligan et al., 2007), the uncoupling of CO2 and N2 fixation in cyanophycin granules (Finzi-Hart et al., 2009), or the down-regulation of photosynthesis during the period of maximum nitrogenase activity at midday (Berman-Frank et al., 2001b), which suggests that light may also be a determinant factor for BNF. Interestingly, the symbiosis between UCYN-A (which lacks genes for oxygenic photosynthesis and C fixation, Zehr et al., 2008) and its algal host has led to enable daytime BNF (Muñoz-Marín et al., 2018), although nifH expression of UCYN-A has also been observed at night (Moisander et al., 2014).
Temperature is an important factor determining the distribution of different diazotrophs in the ocean. Trichodesmium appears to be distributed mainly in (sub)tropical surface waters (Capone, 1997); while small diazotrophs have been found in a wider latitudinal span (Moisander et al., 2010; Luo et al., 2012), from colder surface waters (e.g., Holl et al., 2007; Fernández-Méndez et al., 2016; Harding et al., 2018) to (sub)tropical marine waters (e.g., Bonnet et al., 2009; Messer et al., 2016; Berthelot et al., 2017; Stenegren et al., 2018). It is important to note that temperature may be correlated with other factors that control the distribution patterns of marine diazotrophs such as light, NO3– or O2 (Stal, 2009; Sohm et al., 2011b).
There is increasing evidence that BNF may not be as sensitive to inorganic N as previously thought (Dekaezemacker and Bonnet, 2011; Turk-Kubo et al., 2018), especially when P is not limited (Knapp, 2012). P availability influences BNF (e.g., Riemann et al., 2010; Gradoville et al., 2017) and the distribution of diazotrophs (Sohm et al., 2011b). For instance, cyanobacterial diazotrophs have been associated with high P concentration in the Arctic Ocean (Fernández-Méndez et al., 2016) and the Baltic Sea (Moisander et al., 2007b). High BNF might be also associated with denitrified waters in oxygen minimum zones (OMZs), which are limited in N relative to P (Deutsch et al., 2007; Fernandez et al., 2011; Loescher et al., 2014). Thus, N:P ratio may play a critical role, as BNF rates at high inorganic N concentrations can be offset when P is available (Knapp et al., 2012).
Diazotrophs require much more Fe for growth than other microbes, and its bioavailability directly affects BNF in many areas of the ocean (Berman-Frank et al., 2001a; Kustka et al., 2002; Knapp et al., 2016). Fe is generally depleted in surface waters of the open ocean, and Fe additions can stimulate diazotrophic activity (Turk-Kubo et al., 2012); thus the delivery of dust rich in Fe to the ocean may ultimately control the rate and distribution of marine BNF (Monteiro et al., 2011; Sohm et al., 2011b). For instance, diazotrophs, especially Trichodesmium, are abundant in the North Atlantic Ocean, in which dissolved Fe concentrations are relatively high because dust inputs are greater than in the South Atlantic Ocean, where dissolved Fe concentrations are extremely low (Langlois et al., 2008; Moore et al., 2009). Furthermore, direct experimental measurements have demonstrated that marine BNF can be co-limited by both Fe and P availability (Mills et al., 2004).
Finally, dissolved organic matter seems to stimulate heterotrophic diazotrophs in aphotic environments (Bonnet et al., 2013; Rahav et al., 2013; Benavides et al., 2015, 2016a) and coastal waters (Bentzon-Tilia et al., 2015; Severin et al., 2015) due to the high-energy requirements of the reaction. Furthermore, the interior of C-rich particles may be suitable for heterotrophic BNF (Moisander et al., 2017; Farnelid et al., 2019; and references therein). Additionally, organic matter could promote the mixotrophic nutrition of Trichodesmium when inorganic nutrients are scarce (Benavides et al., 2017b).
Distribution of diazotrophs in marine environments
The global presence of diazotrophs has been documented in recent years (Table 1; Monteiro et al., 2010; Sohm et al., 2011b; Luo et al., 2012). BNF is not only present in tropical surface waters (Capone et al., 2005; Goebel et al., 2010), but also in hypoxic basins (Hamersley et al., 2011; Farnelid et al., 2013), OMZs (Fernandez et al., 2011; Jayakumar et al., 2012), deep sea (Dekas et al., 2009), hydrothermal vents (Mehta and Baross, 2006), the Arctic Ocean (Sipler et al., 2017; Harding et al., 2018) and coral reefs (Benavides et al., 2017a), as well as in estuaries and nutrient-rich coastal upwelling regions (Mulholland et al., 2012; Bentzon-Tilia et al., 2015). These discoveries have challenged the classical paradigm that diazotrophy is constrained to warm, oligotrophic, surface waters.
Open oceans
BNF is particularly important in extremely oligotrophic environments such as open-ocean gyres, in which bioavailable N is scarce (Karl et al., 2002; Halm et al., 2012). Much of the research on diazotroph distribution has focused on Trichodesmium, which is mainly found in the North and Tropical Atlantic Ocean and the Arabian Sea, where it often forms massive surface blooms (Capone et al., 1998, 2005). The dominance of Trichodesmium in warm oligotrophic waters of the Tropical Atlantic Ocean seems to be due to a reduction of the N:P ratio by an increased uptake of inorganic N forms by non-diazotrophic cyanobacteria (Goebel et al., 2010; Singh et al., 2017), while its dominance in the Northern Atlantic Ocean seems to be due to high dissolved Fe concentrations (Langlois et al., 2008; Moore et al., 2009).
Diatom symbiotic cyanobacteria have a much patchier distribution, probably because the diatom hosts require silicon to build their cell walls (Monteiro et al., 2010; Sohm et al., 2011b). They predominate in the warm ocean, with the largest densities in the plumes of Amazon (Foster et al., 2007), Congo (Foster et al., 2009), and Mekong rivers (Grosse et al., 2010).
UCYN groups display different distributions in the ocean. UCYN-A was originally described from partial nifH fragments recovered from the North Pacific (Zehr et al., 1998) and has been recently detected in all ocean basins (Cabello et al., 2016; Farnelid et al., 2016; Martínez-Pérez et al., 2016), where UCYN-A1 dominates and frequently co-occurs with UCYN-A3 in the (sub)tropical open ocean (Thompson et al., 2014; Turk-Kubo et al., 2017). UCYN-B has been also detected in the global ocean, but dominates in (sub)tropical surface waters (e.g., Zehr et al., 2007; Bonnet et al., 2015; Stenegren et al., 2018). UCYN-C seems to be constrained to (sub)tropical open regions (e.g., Goebel et al., 2010; Berthelot et al., 2017), where is adapted to low P and high salinity waters (Turk-Kubo et al., 2015; Cheung et al., 2017).
Heterotrophic diazotrophs are also important N2 fixers in the global ocean (Bombar et al., 2016), where they are ubiquitous in the marine sunlit layer (Riemann et al., 2010; Farnelid et al., 2011; Langlois et al., 2015). Heterotrophic Proteobacteria are widespread in oceanic environments, but dominate in the Pacific coastal upwelling systems (Gradoville et al., 2017), the South Pacific Gyre (Halm et al., 2012), the Indian Ocean (Shiozaki et al., 2014), and the Arabian Sea (Kumar et al., 2017). Diverse and active heterotrophic diazotrophs are also found in sinking particles (Farnelid et al., 2019), aphotic waters (Hamersley et al., 2011; Bonnet et al., 2013; Rahav et al., 2013), OMZs (Jayakumar et al., 2012; Loescher et al., 2014; Fernandez et al., 2015), NH4+-rich sulfidic-anoxic waters of the Baltic Sea (Farnelid et al., 2013), and colder waters such as those in the Arctic Ocean (Blais et al., 2012; Fernández-Méndez et al., 2016), where nifH sequences related to anaerobic bacteria predominate (Farnelid et al., 2011; Shiozaki et al., 2018).
Estuaries and coastal zones
Little is known about the distribution and activity of diazotrophs in estuaries and coastal regions. BNF in these systems is affected by high nutrient inputs from land, so it is assumed to be unimportant (Howarth et al., 1988). However, recent studies suggest that BNF may be important in a number of estuaries and nutrient-repleted coastal upwelling regions (Fulweiler et al., 2007; Wen et al., 2017; Tang et al., 2019), where complicated relationships exist between environmental factors and distribution of different diazotrophs (Short et al., 2004; Moisander et al., 2007a; Severin et al., 2015). In the Baltic Sea, for instance, planktonic heterotrophic diazotrophs are common in a eutrophic estuary, while cyanobacterial diazotrophs are more abundant in a lower-nutrient estuary (Bentzon-Tilia et al., 2015). Furthermore, UCYN-A is the most abundant diazotroph in coastal upwelling ecosystems (Sohm et al., 2011a; Agawin et al., 2014; Moreira-Coello et al., 2019), and other nutrient-rich coastal waters (Mulholland et al., 2012, 2019; Shiozaki et al., 2015, 2017), where UCYN-A2 dominates and usually co-occurs with UCYN-A1 and sometimes with UCYN-A4 (Messer et al., 2015; Turk-Kubo et al., 2017; Henke et al., 2018). Additionally, diatom symbiotic cyanobacteria may be abundant in upwelling regions such as the Taiwan Strait (Wen et al., 2017).
BNF is also important in estuarine and coastal sediments, where heterotrophic bacteria are the dominant diazotrophs (e.g., Burns et al., 2002; Fan et al., 2015a). Finally, BNF is a key process in coastal microbial mats, whose diazotrophic community is composed mainly of filamentous cyanobacteria, anoxygenic phototrophic bacteria, and sulfate-reducing bacteria (Severin et al., 2010; Woebken et al., 2012, 2015).
Seeps and deep-sea vents
Mehta et al. (2003) provided the first evidence of nifH genes in deep-sea hydrothermal vents, where methanogenic archaea are the dominant diazotrophs (Mehta and Baross, 2006). Further, a consortium composed of an anaerobic methanotrophic archaeon (ANME) and a sulfate-reducing bacterium seems to mediate both sulfate-dependent anaerobic methane (CH4) oxidation (S-damo) and BNF in CH4 seep sediments (Dekas et al., 2009, 2014, 2018). Proteobacterial diazotrophs are also abundant in deep-sea hydrothermal vents (Wu et al., 2014; Cao et al., 2015) and CH4 seep sediments (Dang et al., 2009b).
Coral reefs
Corals are found in N-depleted (sub)tropical coastal waters; thus, BNF should play an important role in these ecosystems, providing an additional source of N for symbiotic dinoflagellates and thus improving the productivity. Corals harbor diverse communities of diazotrophs consisting mostly of heterotrophic bacteria, which are host species-specific (Rädecker et al., 2015; Benavides et al., 2017a). Diazotrophs participate in coral nutrition by three main mechanisms: as endosymbionts fixing N2 in several compartments of the holobiont (Olson et al., 2009; Lema et al., 2012; Grover et al., 2014), pelagic diazotroph ingestion (Benavides et al., 2016b) or N2 fixation products assimilated by corals (Cardini et al., 2015). Additionally, the ecological importance of N2-fixing symbionts may be determined by the trophic functional group of the host. For instance, Pogoreutz et al. (2017) found that autotrophic Pocilloporidae exhibited nifH copies and gene expression 100 times higher than those in heterotrophic Fungiidae, suggesting that BNF compensates for the low N uptake in autotrophic corals.
Nitrification
Nitrification is an aerobic process and involves three types of microorganisms: those who oxidize NH3 to nitrite (NO2–), those who oxidize NO2– to NO3–, and those who completely oxide NH3 to NO3– via NO2– (comammox, complete ammonia oxidation).
The oxidation of NH3 is carried out by two groups of chemolithotrophic microorganisms: ammonia-oxidizing bacteria (AOB) belonging to a few genera within the Betaproteobacteria (Nitrosomonas and Nitrosospira) and Gammaproteobacteria (Nitrosococcus) classes (Purkhold et al., 2000), and ammonia-oxidizing archaea (AOA) belonging to the Thaumarchaeota phylum, such as Nitrosopumilus maritimus (Könneke et al., 2005) and Cenarchaeum symbiosum (Hallam et al., 2006). The discovery of AOA solved the long-standing mystery of the apparently rare ammonia oxidizers in the ocean (Wuchter et al., 2006).
Until recently, NH3 oxidation was thought to be a two-step process in AOB, with large gaps still remaining in the AOA pathway (Walker et al., 2010; Vajrala et al., 2013; Kozlowski et al., 2016). The first and usually rate-limiting step is the conversion of NH3 to hydroxylamine (NH2OH), catalyzed by the ammonia monooxygenase (AMO). The second step was once believed to be the conversion of NH2OH to NO2– by the hydroxylamine oxidoreductase (HAO), but recent studies indicate that NH2OH is first converted to nitric oxide (NO), and then to NO2– (Kozlowski et al., 2016; Caranto and Lancaster, 2017), raising the possibility that NH3 oxidation in AOA is also a three-step process (Carini et al., 2018). The pathways for the oxidation of NH2OH to NO and then to NO2– must be characterized to fully understand the bioenergetics of NH3 oxidation in AOB and AOA (Lancaster et al., 2018).
The AMO enzyme is encoded by the amoABC operon. The amoA gene has been widely used as a molecular marker for studying ammonia oxidizers in the environment (Rotthauwe and Witzel, 1997). The HAO enzyme is a homotrimer and is encoded in the hao gene, present in AOB with multiple copies (Arp et al., 2007). The hao gene has been little-used as a molecular marker of AOB in marine systems (Lüke et al., 2016; Rasigraf et al., 2017). No homologs of hao gene have been identified in the genome from any known AOA, suggesting that AOA probably catalyze NH2OH oxidation via a novel enzyme complex (Vajrala et al., 2013; Kozlowski et al., 2016) or produce a reactive nitroxyl (HNO) intermediate instead of NH2OH (Walker et al., 2010).
Ammonia oxidizers are important contributors to marine nitrous oxide (N2O) production (Santoro et al., 2011; Löscher et al., 2012; Trimmer et al., 2016); although the exact metabolic pathways and their relative importance for global N2O production remain unclear. AOB can produce N2O by nitrifier denitrification (see section Denitrification) through NO2– reduction via a NO intermediate (Stein, 2011), as well as a by-product of the nitrification through incomplete NH2OH oxidation to either HNO or NO (Caranto and Lancaster, 2017) or via the enzymatic oxidation of NH2OH (Caranto et al., 2016). AOA also produce N2O by pathways that are apparently similar but less well understood (Stieglmeier et al., 2014; Kozlowski et al., 2016).
The NO2– oxidation is catalyzed by the nitrite oxidoreductase (NXR), which is present in nitrite-oxidizing bacteria (NOB), a phylogenetically diverse group belonging to Chloroflexi, Nitrospirae, Nitrospinae and several classes of Proteobacteria (Daims et al., 2016). The NXR enzyme is closely related to the membrane-bound nitrate reductases (NAR) (Lücker et al., 2010), and is comprised of three subunits: NxrA, NxrB and NxrC. The nxrAB genes, along with the 16S rRNA gene, have been the most used markers for studying NOB in marine environments (e.g., Bristow et al., 2015; Rani et al., 2017). Some marine NOB, such as Nitrospira, are mixotrophs and can produce NH3 from urea, thus sustaining ammonia oxidizers that provide NO2– to Nitrospira (Koch et al., 2015). NOB of the genus Nitrospina have exclusively been found in marine environments (Mincer et al., 2007; Beman et al., 2013; Levipan et al., 2014) and have only two known cultured species to date: N. gracilis, isolated from ocean surface waters (Watson and Waterbury, 1971), and N. watsonii, isolated from the suboxic zone of the Black Sea (Spieck et al., 2014). Nitrospina is the most abundant and globally distributed marine NOB and has a key role in the oceanic NO2– oxidation and C fixation (Pachiadaki et al., 2017).
Comammox bacteria were discovered in 2015 (Daims et al., 2015; van Kessel et al., 2015) and have been classified as members of lineage II within Nitrospira (Daims et al., 2015). These organisms have a high affinity for NH3 and may be well adapted to oligotrophic environments (Kits et al., 2017). A metagenomic survey recently found a relatively high proportion of comammox bacteria in coastal water and sediment samples, whereas they were nearly undetectable in open-ocean samples (Xia et al., 2018). Further research of comammox bacteria is needed in marine environments to assess their contribution to marine nitrification.
Factors affecting nitrification in marine systems
Oxygen, NH4+, NO2–, light, pH, temperature, and salinity are among the main factors allowing niche differentiation of the main nitrifier groups (Bouskill et al., 2012) and the decoupling of the two steps of nitrification in some marine environments (Heiss and Fulweiler, 2016; Zakem et al., 2018). Nitrifiers require O2, but they seem to do well in microaerophilic conditions. In this context, it has been observed that NH3 oxidation and NO2– oxidation persist even at nanomolar O2 levels in OMZs, suggesting that specialist nitrifiers have developed an exceptionally high affinity for O2 in this habitat (Füssel et al., 2012; Bristow et al., 2016, 2017; Sun et al., 2017). Furthermore, O2 and NO2– might be the primary drivers of niche differentiation of marine NOB species, since OMZ NOB species seem to be more adapted to lower O2 but higher NO2– concentrations than non-OMZ NOB species (Watson and Waterbury, 1971; Sun et al., 2017).
Photoinhibition of marine nitrifiers has been also reported (e.g., Merbt et al., 2012; Pedneault et al., 2015; Peng et al., 2016; Horak et al., 2018), but observations of nitrification and the frequent retrieval of amoA genes close to the sea surface (Mincer et al., 2007; Christman et al., 2011; Shiozaki et al., 2016) suggest that this is not universally the case. Thus, light might be an indirect control on nitrification (Zakem et al., 2018), and the base of the euphotic zone might be an optimal location for nitrifiers in stratified water columns (Santoro et al., 2010; Beman et al., 2012; Peng et al., 2016): phytoplankton outcompete slow-growing nitrifiers for NH4+ and NO2– in the sunlit ocean, but not deeper where light limits phytoplankton (Smith et al., 2014b). Differential photoinhibition has been also proposed as the mechanism that maintains the oceanic primary NO2– maximum at the base of the euphotic zone (where high NH3 oxidation rates and AOA abundance have been found; Newell et al., 2013; Peng et al., 2015) because NOB are more sensitive to light than ammonia oxidizers (Olson, 1981). Additionally, light might be an important factor structuring marine AOA in two distinct ecotypes by depth; the water column group A (WCA) or “shallow” ecotype, with putative adaptive mechanisms to reduce light-induced damage, and the water column group B (WCB) or “deep” ecotype (Luo et al., 2014). Other factors, such as different substrate affinities (Sintes et al., 2013; Smith et al., 2016) or the ability to use organic substrates (Alonso-Sáez et al., 2012; Qin et al., 2014), also influence the vertical distribution of AOA ecotypes.
Elevated temperature may drives decoupling of NH3 and NO2– oxidation, leading to NO2– accumulation in some coastal waters (Bristow et al., 2015; Schaefer and Hollibaugh, 2017), and can affect the composition and abundance of AOA communities in several bays (Mosier and Francis, 2008; He et al., 2018). Temperature also seems to be an important factor influencing the diversity of ammonia oxidizers in sponge associations (Cardoso et al., 2013) and deep-sea seep sediments (Dang et al., 2010b). Marine NOB can grow in a broad temperature range and sequences from this group have been recovered from polar environments (Rani et al., 2017) to deep-sea brines with temperatures up to 65°C (Ngugi et al., 2016).
Other factors affecting marine nitrifier communities are site-specific. For example, in deep-sea environments, total C, N and sulfur (S) seem to control the distribution of ammonia oxidizers (Xu et al., 2014; Luo et al., 2015). Moreover, salinity is an important factor driving amoA gene diversity in estuaries (e.g., Francis et al., 2003; Bernhard et al., 2005, 2010; Hou et al., 2018). High salinity also seems to affect NOB communities (Oren, 2011; Monteiro et al., 2017), although Ca. Nitromaritima RS can thrive over 11% salinity in the Red Sea brines (Luo et al., 2015).
Distribution of nitrifier communities in marine environments
Open oceans
The distribution and activity of ammonia oxidizing communities in the open ocean has been widely studied (Table 1). In this NH4+-limited environment, AOA are present throughout the water column and dominate over AOB (e.g., Beman et al., 2008; Newell et al., 2011; Pajares et al., 2019), which sometimes go undetected (Mincer et al., 2007; De Corte et al., 2009; Molina et al., 2010). A potential explanation for the marine AOA dominance is their high substrate affinity that could provide a competitive advantage over AOB (Martens-Habbena et al., 2009).
There is not a clear pattern of depth distribution of both AOB and AOA in the open ocean. For example, AOA are mainly present in the euphotic layer and AOB are confined to higher depths in the Sargasso Sea, the North Pacific Ocean, the Arctic Ocean and the Gulf of California (Beman et al., 2012; Newell et al., 2013; Shiozaki et al., 2016), while AOA are predominantly distributed below the euphotic zone in the central and subtropical Pacific Ocean (Mincer et al., 2007; Church et al., 2010). Moreover, AOA form vertical and latitudinal gradients throughout the North Atlantic, where their abundance and diversity decrease in meso- and bathypelagic waters from the north toward the equator (Agogué et al., 2008).
Abundant and active ammonia oxidizers have been found in OMZs (Newell et al., 2011; Peng et al., 2015, 2016), such as those in the Arabian Sea and the Eastern Tropical North Pacific (ETNP), where ammonia oxidizers and anammox bacteria have different vertical distributions in the anoxic layer (Pitcher et al., 2011a; Pajares et al., 2019), the Eastern Tropical South Pacific (ETSP), where both AOA and AOB exhibit a strong amoA expression in the upper OMZ (Lam et al., 2009), and the Baltic Sea, where the high abundance of AOA suggests a tolerance of this group to anoxic and sulfidic waters (Berg et al., 2015).
The two ecotypes of AOA are distributed at different depths in the open ocean (Francis et al., 2005; Luo et al., 2014). The WCA is typically more abundant in the sunlit ocean, while the WCB dominates the meso- and bathypelagic, where light is absent and the NH4+ flux is very low but the availability of other growth substrates (e.g., urea) is high (Sintes et al., 2013; Santoro et al., 2017). This distribution of AOA ecotypes has been found in different marine systems such as OMZs (Beman et al., 2008; Molina et al., 2010), coastal upwelling region (Santoro et al., 2010), warmer waters with P deficit (De Corte et al., 2009), and colder waters (Shiozaki et al., 2016; Smith et al., 2016). WCA abundance has been associated with the highest nitrification rates, suggesting that this ecotype is responsible for most of the NH3 oxidation in the ocean (Santoro et al., 2017).
In contrast with ammonia oxidizers, NOB distribution is less understood in marine environments. Nitrococcus, with only one species known to date (N. mobilis) (Watson and Waterbury, 1971), can dominate in certain marine areas such as the Namibian OMZ (Füssel et al., 2012, 2017). Nitrospina-like NOB have been found in abundance in different areas of the global ocean, such as at open-ocean benchmark research sites as the Hawaii Ocean Time-series (HOT) (DeLong et al., 2006) and San Pedro Ocean Time-series (SPOT) (Beman et al., 2010), but exhibits a preference for mesopelagic waters (Mincer et al., 2007), sediments (Reyes et al., 2017) and OMZs (Füssel et al., 2012; Levipan et al., 2014; Ganesh et al., 2015; Lüke et al., 2016). Two novel uncultured Nitrospina-like species have been recently identified in high abundance in OMZs (Sun et al., 2019), which is consistent with the detection of higher NO2– oxidation rates in OMZs than in oxic seawaters and suggests novel adaptations of specialist NOB to anoxic environments. Furthermore, it was hypothesized that NOB in OMZs might benefit from utilizing alternative terminal electron acceptors for NO2– oxidation, such as iodate, manganese or Fe (Lam and Kuypers, 2011; Casciotti and Buchwald, 2012). Their significance in OMZs, specifically in counteracting N loss through denitrification and anammox, has been recognized in recent years (Beman et al., 2013; Buchwald et al., 2015; Bristow et al., 2017).
Estuaries and coastal environments
The diversity of ammonia oxidizers in estuaries might be greater than that in the adjacent open oceans due to nutrient discharge from rivers (Cao et al., 2011; He et al., 2018). Both AOA and AOB are frequently found together in estuarine and coastal sediments, although the AOA community is usually more diverse than AOB community (Beman and Francis, 2006; Jin et al., 2011; Zheng et al., 2014; Zhang Y. et al., 2014). However, the dominance of one group over the other is unclear. In some estuaries and coasts, AOA form the most abundant ammonia oxidizer community (e.g., Caffrey et al., 2007; Lipsewers et al., 2014; Tait et al., 2014; Urakawa et al., 2014; Zhang Y. et al., 2014), while in others they are less abundant than or equal to AOB (e.g., Zheng et al., 2014; Fan et al., 2015b; Li et al., 2015; Smith J. M. et al., 2015). The salinity gradient is one of the main factors controlling the distribution and activity of nitrifier communities in estuarine sediments, where AOA and AOB seem to occupy different niches (Table 1). AOB are usually more abundant where salinity and N content are higher, while AOA dominates in the part exhibiting low salinity and N content (Mosier and Francis, 2008). Further, AOA belonging to “sediment” and “marine” clades (Nitrosopumilus-like sequences) are more abundant in the mouth, whereas AOA belonging to the “low salinity” (Nitrosoarchaeum-like sequences) and “soil” (group 1.1b) clades are more abundant in the head of many estuaries (e.g., Francis et al., 2003; Beman and Francis, 2006; Mosier and Francis, 2008; Bernhard et al., 2010). Similarly, AOB sequences belonging to the Nitrosospira-like cluster have mostly been obtained at high salinities, while the Nitrosomonas-like cluster is dominant at low salinities (Francis et al., 2003; Bernhard et al., 2005).
The structure of AOA populations follows different patterns in coastal waters. For instance, WCA organisms seem to be distributed at all depths, whereas WCB organisms are confined to low-O2 and low-chlorophyll deeper waters in the Pacific coasts (Smith et al., 2014a; Bertagnolli and Ulloa, 2017). Additionally, AOA populations fluctuate seasonally, with abundance peaks during winter in the coastal Arctic and North Sea (Christman et al., 2011; Pitcher et al., 2011b), whereas in the Chilean coast, WCB organisms are abundant during spring and summer and non-detectable during winter (Bertagnolli and Ulloa, 2017).
NOB diversity in estuaries is also strongly influenced by salinity. For instance, distinctive NOB phylotypes have been detected along a salinity gradient in estuarine sediments, where NOB increase their abundance as salinity decreases (Monteiro et al., 2017). Additionally, salinity and NO2– flux may be major factors causing niche differentiation of NOB groups in estuarine waters, where Nitrospina could be more adapted to low-nutrients and high-salinity conditions, while Nitrospira could be well adapted to eutrophic estuarine conditions (Hou et al., 2018). Finally, NH3 and NO2– oxidation are not always coupled in coastal waters (Heiss and Fulweiler, 2016), which may be due to a decoupling between AOA and Nitrospina populations (Bristow et al., 2015).
Deep-sea environments
Deep-sea sediments and hydrothermal vents are sites of active nitrification that harbor diverse ammonia-oxidizing prokaryotes (Table 1; Nakagawa et al., 2007; Dang et al., 2009a; Baker et al., 2012). In these systems, AOA are much more diverse but less abundant than AOB (Cao et al., 2012; Xu et al., 2014; Luo et al., 2015), although several exceptions have been found (Dang et al., 2010b; Nunoura et al., 2013). NOB community has been understudied in deep-sea environments, where they seem to be very abundant (Baker et al., 2013; Nunoura et al., 2013; Ngugi et al., 2016; Tully and Heidelberg, 2016). Further, distinctive distribution of NOB has been found, with Nitrospina more abundant in the bathyal waters and Nitrospira more abundant in the hadal waters (Nunoura et al., 2015).
Symbiosis with coral reefs and other marine organisms
The few studies of the nitrifying ecology in coral reefs suggest that AOA may be the main contributors to N cycling in these systems (Beman et al., 2007), with N. maritimus as an important player that oxidizes NH3 during daytime when the conditions in coral mucus are oxic (Siboni et al., 2008). However, AOB seem to dominate nitrifier communities in other coral species (Yang et al., 2013). Further research in this direction would help understand the importance of both ammonia oxidizer groups in a variety of coral species.
Ammonia oxidizers have also been studied in symbiosis with other marine organisms. The first genome assembly of a marine AOA was generated from C. symbiosum, which accounts for 65% of microorganisms associated with the sponge Axinella mexicana (Preston et al., 1996; Hallam et al., 2006). However, Nitrosospira and Nitrosopumilus-related AOA seem to be the major drivers of nitrification in different types of sponges (Mohamed et al., 2010; Radax et al., 2012; Zhang F. et al., 2014). In addition, AOA play an important nitrification role within the tissue of colonial ascidians (Martínez-García et al., 2008).
Dissimilatory Nitrate Reduction to Ammonia (DNRA)
Respiratory ammonification or DNRA is an anaerobic process in which NO3– is reduced to NO2– and then to NH4+, though the reaction may begin with NO2– directly. DNRA is mainly a heterotrophic process, but it can also be an autotrophic process driven by inorganic compounds such as sulfide (S–2), elemental S, or Fe+2 (Robertson et al., 2016; Slobodkina et al., 2017).
NO3– reduction is a major source of NO2– for other N-cycling processes, including aerobic NO2– oxidation and anammox, and in DNRA occurs in the same way as in denitrification. It is mainly catalyzed by either the NarGHI complex, present in a wide variety of microorganisms, or the periplasmic nitrate reductase complex (NapAB), present mainly in Gram-negative bacteria (Moreno-Vivián et al., 1999; Simon and Klotz, 2013). The active sites of both complexes are encoded in narG and napA genes, which have been used as biomarkers for the marine microorganisms involved in NO3– reduction (e.g., Smith et al., 2007; Lam et al., 2011).
The reduction of NO2– to NH4+ in the heterotrophic DNRA is catalyzed by the cytochrome C nitrite reductase (ccNIR), encoded in the nrfA gene, which is frequently used as a biomarker for the DNRA process (e.g., Papaspyrou et al., 2014; Welsh et al., 2014). The reduction of NO2– to NH4+ in the chemolithotrophic DNRA is mainly catalyzed by the octaheme tetrathionate reductase (Otr) (Atkinson et al., 2007) or the octaheme cytochrome C nitrite reductase (Onr) (Tikhonova et al., 2006).
A broad diversity of microorganisms is capable of DNRA, mainly prokaryotic organisms belonging to Proteobacteria, Firmicutes, Verrucomicrobia, Planctomycetes, Acidobacteria, Chloroflexi, and Chlorobia (Tiedje, 1988; Welsh et al., 2014). Marine eukaryotes capable of DNRA include diatoms, which use DNRA to enter a resting stage for long-term survival in dark anoxic sediments (Kamp et al., 2011), and fungi such as Aspergillus terreus isolated from the Arabian Sea OMZ (Stief et al., 2014). Evidence of chemolithotrophic DNRA in marine sediments has been found in Beggiatoa (Preisler et al., 2007), as well as in Thermosulfurimonas dismutans and Dissulfuribacter thermophilus, thermophilic anaerobic bacteria isolated from deep-sea hydrothermal vents (Slobodkina et al., 2017).
Factors affecting DNRA in marine systems
DNRA produces NH4+ without the release of N2 and contributes to nitrification and anammox. Therefore, understanding the mechanisms controlling the DNRA community and its interactions with other N-cycling communities is critical for understanding the fate of N in marine systems.
DNRA and denitrification compete for NO3– and there are several factors favoring DNRA over denitrification, such as high S–2 concentrations and C:NO3– ratio, elevated temperatures, salinity and anoxic conditions (e.g., Dong et al., 2011; Song et al., 2014; Yin et al., 2017). Although DNRA has a lower energetic yield than denitrification, it can accept a greater number of electrons per NO3– molecule (eight, compared to five for denitrification). For this reason, DNRA may be energetically favored over denitrification in anoxic environments where NO3– is limiting and electron donors (organic C or S–2) are in excess (Dong et al., 2011; Kraft et al., 2014). It has been suggested that DNRA is not affected by NH4+ (Tiedje, 1988) and NO2– (van den Berg et al., 2017), but it has been demonstrated that NH4+ and NO2– concentrations are positively correlated with DNRA rates in estuaries (Song et al., 2014; Lisa et al., 2015; Yin et al., 2017). Salinity seems to be another factor affecting DNRA, although there is not a clear pattern. In some estuaries DNRA rates increase with increasing salinity (Gardner et al., 2006; Giblin et al., 2010; Lisa et al., 2015), while in others DNRA rates decrease (Dong et al., 2009). Finally, elevated temperatures favor DNRA rates; therefore, DNRA may be an important pathway for NO3– reduction in (sub)tropical estuaries (Gardner and McCarthy, 2009; Dong et al., 2011; Yin et al., 2017).
Distribution of DNRA communities in marine environments
Since DNRA is an anaerobic process, marine DNRA communities are mostly restricted to anoxic environments such as sediments and OMZs (Table 1). However, there are relatively few studies on DNRA communities in those marine systems compared to the number of studies on microbes involved in other N-cycling processes.
Open oceans and deep-sea environments
Only a few studies on DNRA distribution have been conducted in open oceans, most of them in OMZs. In the Arabian sea OMZ, DNRA is coupled with anammox (Jensen et al., 2011), and is carried out by microorganisms with divergent nrfA genes (Lüke et al., 2016). In Baltic Sea sediments nrfA has been found in lower abundance than genes involved in denitrification, possibly due to the combination of low-sulfide, oligotrophic and hyposaline conditions of this environment (Rasigraf et al., 2017; Reyes et al., 2017). DNRA has also been detected in the ETNP and ETSP OMZs, where it may supply most of the NH4+ needed for anammox (Lam et al., 2009; Pajares et al., 2019). However, several studies have reported low or undetectable nrfA genes and low DNRA rates in the ETSP, suggesting that this process may be sporadic (Kalvelage et al., 2013; Schunck et al., 2013) or that anammox bacteria could perform both DNRA and anammox producing their own NH4+ from NO3– (Kartal et al., 2007; De Brabandere et al., 2014).
DNRA communities have also been found in deep-sea sediments, where microbes involved in DNRA, nitrification and anammox are more abundant than in shallow sediments (Yu et al., 2018). More studies are needed to confirm the importance of DNRA in deep-sea environments.
Estuarine zones
Diversity and abundance of the nrfA gene in estuaries are high and often change along salinity and NO3– gradients (e.g., Papaspyrou et al., 2014; Song et al., 2014; Smith C. J. et al., 2015). There are exceptions such as in the Yellow River estuary, where the abundance and activity of DNRA communities are not affected by either type of gradient (Bu et al., 2017). Additionally, DNRA communities from estuarine sediments are site-specific and could vary significantly at a small spatial scale (Decleyre et al., 2015; Zheng et al., 2016). For example, in the well-studied Colne estuary (United Kingdom), DNRA communities embedded in deeper anoxic sediments are more homogeneous compared to those in the surface (Takeuchi, 2006). Furthermore, in situ rates of denitrification and DNRA along with gene markers for nitrate reduction (narG), denitrification (nirS), and DNRA (nrfA) decrease toward the mouth of that estuary where the NO3– concentration is lower, while denitrification potential also decrease but DNRA potential increase (Smith et al., 2007; Dong et al., 2009; Papaspyrou et al., 2014; Smith C. J. et al., 2015), indicating that DNRA microorganisms are more competitive than denitrifiers when the ratio of electron donors to electron acceptors increase, which stimulates DNRA relative to denitrification. Finally, higher nrfA abundance and DNRA rates have been found in estuarine sediments richer in organic C and S–2 (Song et al., 2014; Yin et al., 2017).
N-Loss Processes
The oceanic N budget balance leans more toward higher losses (between ∼275–481 Tg N yr–1) than inputs (between ∼265–294 Tg N yr–1) (Codispoti et al., 2001; Codispoti, 2007; Gruber and Galloway, 2008; Voss et al., 2013). The N release from the ocean is mainly caused by denitrification and anammox in a theoretical 71:29 ratio, assuming that anammox consumes all NH4+ produced from mineralization of C and N in a 106:16 ratio coupled to denitrification (Dalsgaard et al., 2012). The most important factor over marine N loss is O2 given that denitrification and anammox occur in environments where O2 is nearly or fully depleted, such as OMZs and sediments, which are responsible for 30–50% and 50–70% of N loss, respectively (Codispoti et al., 2001; Devol, 2015; Na et al., 2018). The interaction of denitrification and anammox with other N processes also affects marine N loss. As discussed below, DNRA and denitrification coexist in anoxic environments, but DNRA may be favored in environments with low NO3– and high organic C (Kraft et al., 2014). DNRA interaction with anammox could enhance N loss, because DNRA feeds anammox by increasing NH4+ concentrations (Jensen et al., 2011). Nitrate/nitrite-dependent anaerobic methane oxidation (N-damo) has recently identified as a N-loss process (Padilla et al., 2016), but its contribution to the global marine N loss has not yet been investigated.
Among dissolved gaseous N compounds, marine N2O has caught the most attention of the scientific community in the last years (Bange et al., 2010; Martinez-Rey et al., 2015), because it is a potent greenhouse gas and an ozone-depleting agent that is mainly produced by NH3 oxidation and denitrification (Freing et al., 2012). Estimates of global oceanic N2O emissions range between 3.8 Tg N yr–1 (Ciais et al., 2013) and 4.3 Tg N yr–1 (Battaglia and Joos, 2018), ∼35% of total N2O natural emissions (Syakila and Kroeze, 2011). The highest oceanic N2O concentrations and fluxes occur in coastal upwelling ecosystems (Nevison et al., 2004; Arévalo-Martínez et al., 2015) and OMZs (e.g., Naqvi and Noronha, 1991; Kock et al., 2016; Bourbonnais et al., 2017; Casciotti et al., 2018), in which it is produced near the oxycline where a decoupling between N2O production from both NH3 oxidation and denitrification and consumption by denitrification occurs (Farías et al., 2009; Dalsgaard et al., 2014; Babbin et al., 2015; Ji et al., 2015), as the latter process is less O2 tolerant.
Denitrification
Denitrification is a respiratory pathway performed by diverse facultative anaerobic microorganisms in which NO3– is respired to NO2– (discussed in the DNRA section), followed by stepwise reductions to NO, N2O, and N2 (Zumft, 1997). Denitrification is a modular pathway, in which a microorganism may not always possess the full set of enzymes and thus perform only a subset of steps within the pathway (Graf et al., 2014).
Two isofunctional but structurally divergent periplasmic enzymes catalyze the reduction of NO2– to NO (Simon and Klotz, 2013): a Cu-containing nitrite reductase (encoded by nirK) and a haem-containing cd1 nitrite reductase (encoded by nirS), which are thought to be mutually exclusive in the genome of denitrifying organisms (Jones et al., 2008), although some exceptions have been found (Graf et al., 2014). Because this is the first committed step of the pathway to a N gaseous product, nir genes are the most widely used markers for denitrifiers (e.g., Braker et al., 2000; Mosier and Francis, 2010; Pajares et al., 2019). The nir genes are present in many other microorganisms, including anammox bacteria, nitrite and methane-oxidizing bacteria, AOA and AOB (Kuypers et al., 2018). Moreover, several studies have shown evidence of niche differentiation between nirK and nirS communities (Jones and Hallin, 2010; Wittorf et al., 2016; Pajares et al., 2017). Furthermore, nirS-denitrifiers seem to have a complete denitrification pathway (including nor and nos genes); thus, they are more likely to completely reduce NO2– to N2 (Graf et al., 2014).
The conversion of NO to N2O is carried out mainly by the nitric oxide reductases cNOR (a cytochrome c-dependent complex) or qNOR (a quinol-dependent complex), whose active sites are encoded by two variants of the same gene (cnorB and qnorB, respectively) (Simon and Klotz, 2013). The cNOR has also been found in AOB (Casciotti and Ward, 2005), while qNOR has also been found in N-damo and non-denitrifying microorganisms, where it may play a detoxifying role (Jones et al., 2008; Wu et al., 2011). Both qnorB and cnorB have been little used as biomarkers for marine NO-reducing denitrifiers (Braker and Tiedje, 2003; Ganesh et al., 2015).
The final step in the denitrification pathway is the reduction of N2O to N2. It is catalyzed by the nitrous oxide reductase (NOS), which is encoded in the nosZ gene and is frequently used as a biomarker of N2O-reducing denitrifiers in marine systems (e.g., Bowles et al., 2012; Wittorf et al., 2016). There are two phylogenetically distinct nosZ clades: clade I includes organisms with a complete denitrification pathway, whereas clade II includes organisms that frequently lack other denitrification genes (Jones et al., 2013).
Denitrification is mainly a heterotrophic process, although autotrophic denitrifiers, which use H2 or S compounds as electron donors, have been found in many marine environments, including OMZs (Lam and Kuypers, 2011, and references therein), hydrothermal vent sediments and microbial mats (e.g., Shao et al., 2011; Bowles et al., 2012).
Finally, a partial denitrification pathway has been reported in marine AOA and AOB. In the called “nitrifier denitrification,” NO2– is reduced to N2O via a NO intermediate under suboxic or anoxic conditions (Frame and Casciotti, 2010; Zhu et al., 2013). Nitrifier denitrification was originally thought to be restricted within some AOB harboring Nir and Nor enzymes (Stein, 2011). However, the observation of high N2O production rates under low O2 concentrations for N. maritimus suggests that marine AOA might play an important role in N2O production via this pathway (Löscher et al., 2012) or via a hybrid formation (Kozlowski et al., 2016). Nitrifier denitrification has been observed in the lower euphotic zone of the open ocean (Wilson et al., 2014) and OMZs (Löscher et al., 2012; Bourbonnais et al., 2017).
Factors affecting denitrification in marine systems
Denitrification is limited to environments where O2 is nearly fully depleted. It has been reported that nirS, norB and nosZ transcripts strongly decrease in O2 concentrations > 200 nM (Dalsgaard et al., 2014), and nirS is rarely present in well-oxygenated waters (Jayakumar et al., 2004; Ward et al., 2009). Furthermore, O2 availability is associated with the habitat partitioning of NO2– and N2O reducers, with nirS- and nosZI-type denitrifiers prevailing under lower O2 regimes (Kim et al., 2011; Wittorf et al., 2016).
Heterotrophic denitrifiers depend on a supply of organic matter, and incubation experiments in OMZs suggest that organic C is a major driver of denitrification, which might be linked to the episodic supply of organic substrates from productive surface waters (Ward et al., 2008; Babbin et al., 2014). The composition and stoichiometry of the source organic matter may also determine the dominant N-loss process: fresh organic matter with higher C:N ratio stimulates denitrification over anammox because denitrification uses organic matter directly while anammox uses NH4+ from organic matter degradation (Babbin et al., 2014; Chang et al., 2014). Furthermore, N2O and N2 production associated with denitrifying communities have been found in particles, whereas other N processes are more associated with free-living communities in OMZs (Ganesh et al., 2015; Fuchsman et al., 2017). The structure and abundance of denitrifiers in OMZs is also correlated with NO3– and NO2– concentrations (Liu et al., 2003; Castro-Gonzalez et al., 2005; Jayakumar and Ward, 2013). Additionally, chemolithotrophic denitrification may be responsible for N2 production in OMZs where hydrogen sulfide (H2S) accumulates (Galán et al., 2014).
The input and type of organic matter and salinity are among the key factors controlling denitrification rates and denitrifier community distribution in estuaries (e.g., Mosier and Francis, 2010; Eyre et al., 2013; Francis et al., 2013; Zhang Y. et al., 2014; Lee and Francis, 2017). Since NirK and NosZ require Cu, it could represent a regulating factor in denitrification and the production of N2O in marine environments (Granger and Ward, 2003). However, it has been observed that increasing concentrations of Cu decrease the abundance and diversity of denitrifiers and inhibit denitrification rates in estuarine sediments (Magalhães et al., 2011). As mentioned before, denitrification and DNRA compete for NO3–, and factors such as low S–2 concentrations (which inhibit the last two steps of denitrification), cold temperatures, and a low C:NO3– ratio favor denitrification over DNRA (Burgin and Hamilton, 2007; Smith C. J. et al., 2015).
Distribution of denitrifier communities in marine environments
Although denitrifying microorganisms can be found in any marine environment, denitrification is typically restricted to suboxic or anoxic environments such as OMZs and sediments (Table 1).
Open oceans and deep-sea environments
OMZs are considered one of the major oceanic sites of denitrification. For example, denitrification is the dominant N-loss pathway in the Arabian Sea OMZ (Ward et al., 2009), where denitrifiers dominate over anammox bacteria (Jayakumar et al., 2009; Sokoll et al., 2012); although a recent report has found higher abundance of anammox genes over denitrifying genes in this OMZ (Bandekar et al., 2018). Depth distributions of nir genes follow the same pattern in the Arabian Sea, ETNP and ETSP, where they are associated with the secondary NO2– maximum in oxygen-depleted waters (Bandekar et al., 2018; Pajares et al., 2019). However, the denitrifier community composition of these sites seems to be different (Jayakumar and Ward, 2013; Bandekar et al., 2018). In the ETNP, narG belonging to SAR11 clades is the most abundant denitrifying gene (Tsementzi et al., 2016), while nirK dominates over nirS (Fuchsman et al., 2017). In the Black Sea, nirK-based communities vary with depth, while the composition of both nirK and nirS genes changes at the bottom of this suboxic zone (Oakley et al., 2007). Great genomic potential for full denitrification to N2 but less genomic potential for anammox and DNRA have been found in Baltic Sea sediments (Rasigraf et al., 2017; Reyes et al., 2017). Additionally, the composition of nirS-based communities is site-specific in this environment and varies along biogeochemical gradients in the water column, while it is uniform in the sediment (Falk et al., 2006; Hannig et al., 2006). Finally, the nosZ-based communities have a strong biogeographical separation, thus the communities from surface ocean waters differ from those in OMZs (Jayakumar et al., 2018).
Few studies of denitrifier communities have been conducted in deep-sea sediments and hydrothermal vents, where chemolithotrophic denitrification seems to be an important process given the high concentration of reduced S species in such environments (Shao et al., 2011; Bowles et al., 2012). In addition, nirS sequences have been retrieved from these systems (Bourbonnais et al., 2014), where nirK-type denitrifiers were undetected (Tamegai et al., 2007).
Estuaries and coastal environments
Denitrification is often the major process driving N removal from coastal and estuarine environments (Damashek and Francis, 2018, and references therein). Sediments provide ideal conditions for this process, due to the narrow spatial scale for diffusion across redox boundaries. Therefore, most studies have documented the diversity and activity of denitrifiers in estuary sediments (e.g., Abell et al., 2010; Magalhães et al., 2011; Wang et al., 2014; Smith J. M. et al., 2015), with a few of them in estuary waters (e.g., Santoro et al., 2006; Zhang Y. et al., 2014; Smith C. J. et al., 2015). Denitrifiers often change along the estuarine salinity gradient, with distinct communities in fresh and marine regions (e.g., Abell et al., 2013; Francis et al., 2013; Lee and Francis, 2017). For instance, in the San Francisco Bay estuary, the abundance of nirK is higher in the riverine zone, whereas nirS is more abundant in marine zones (Mosier and Francis, 2010). Additionally, denitrification rates and the abundance of nitrate and nitrite genes usually decline from the estuary head toward the mouth, where NO3– concentrations are lower (Smith et al., 2007; Dong et al., 2009).
Other environments
Denitrification also occurs in other niches outside anoxic sediments and OMZs. For example, the low-oxygen environment within Trichodesmium colonies allows the growth of active denitrifiers harboring nosZ genes, representing a potential sink for N2O within oceanic surface waters (Wyman et al., 2013; Coates and Wyman, 2017). Diverse bacteria harboring nir genes have been also found in corals and sponges (Hoffmann et al., 2009; Yang et al., 2013). Additionally, sinking copepod carcasses have anoxic interiors that support the expression of nirS genes, representing hotspots of pelagic denitrification (Glud et al., 2015). Finally, certain benthic foraminifera are capable of accumulating and respiring NO3– through denitrification (Risgaard-Petersen et al., 2006; Pina-Ochoa et al., 2010), which is their preferred respiration pathway in OMZs, contributing substantially to total benthic NO3– loss in these environments (Glock et al., 2013, 2019).
Nitrate/Nitrite-Dependent Anaerobic Methane Oxidation (N-Damo)
Anaerobic CH4 oxidation coupled with denitrification (N-damo) was discovered in 2006 and constitutes a unique link between the C and N cycles (Raghoebarsing et al., 2006).
The N-damo process is carried out by ANME archaea and NC10 bacteria. Ca. Methanoperedens nitroreducens (ANME-2d) is one of the microorganisms capable of coupling the anaerobic CH4 oxidation to NO3– reduction to NO2– (Haroon et al., 2013), while Ca. Methylomirabilis oxyfera (a member of the NC10 phylum) is capable of coupling the anaerobic CH4 oxidation to NO2– reduction to N2 without the presence of ANME archaea (Ettwig et al., 2010). The metabolism of Ca. M. nitroreducens includes genes related to reverse methanogenesis (Haroon et al., 2013; Timmers et al., 2017), such as the methyl coenzyme-M reductase gene (mcrA), which has been recently used as a biomarker for methanogens and ANME archaea in marine sediments (Vaksmaa et al., 2017).
The metabolism of Ca. M. oxyfera is also complex and unusual, because despite being considered an anaerobic microorganism, it is able to oxidize CH4 using enzymes found in aerobic methanotrophs (Ettwig et al., 2010) such as the particulate methane-monooxygenase (pMMO), encoded in the pmoA gene, which has been used as a biomarker of N-damo bacteria in marine systems (e.g., Chen et al., 2016; Padilla et al., 2016). Metagenomic evidence suggests that Ca. M. oxyfera possesses an “intra-aerobic” metabolism consisting of an intracellular production of O2 by dismutating NO into O2 and N2 (Ettwig et al., 2010), although this is yet to be further proven. Its genome also encodes NirS and qNOR, which may participate in detoxifying processes (Wu et al., 2011).
Factors affecting N-damo and its distribution in marine systems
The N-damo process has just begun to be studied, and only a few works on these microorganisms in marine environments are available (Table 1). Most of these works has focused on NC10 bacteria and thus information on N-damo archaea is very scarce. The mcrA gene and nar transcripts affiliated to M. nitroreducens-like archaea were recently retrieved from the North Sea sediments (Vaksmaa et al., 2017) and the ETNP OMZ (Thamdrup et al., 2019), respectively, revealing that ANME-2d archaea have niches in marine ecosystems and their roles need to be further explored.
N-damo has been detected in OMZs, which represent a niche for NC10 bacteria (Padilla et al., 2016; Chronopoulou et al., 2017). For example, in the ETNP OMZ, active NC10 bacteria are abundant in the anoxic zone with high NO2– and CH4 concentrations, suggesting that anaerobic NH4+ oxidation is coupled to NO3– reduction and denitrification (Padilla et al., 2016; Thamdrup et al., 2019). M. oxyfera-like bacteria have also been detected in estuarine and coastal sediments, where they show great diversity and a depth-specific distribution influenced by redox potential, water content and total organic C (Li-dong et al., 2014; Zhang et al., 2018). Moreover, the activity of this community in coastal sediments vary seasonally and spatially and seem to be highly influenced by NO3– (Shen et al., 2016; Wang et al., 2017), NH4+, NO2– (Chen et al., 2014, 2015b) and salinity (Chen et al., 2015a; Shen et al., 2016).
The importance of N-damo microorganisms in the C and N cycles, in addition to the small number of studies on their distribution, clearly warrant further study to ascertain the drivers of these communities in different marine ecosystems.
Anammox
Anammox consists in the conversion of NH4+ and NO2– to N2 in the absence of O2. In this process, the initial NO2– reduction to NO is believed to be catalyzed by NirS, which has been confirmed in the genomes of Ca. Kuenenia stuttgartiensis (Strous et al., 2006) and Ca. Scalindua (van de Vossenberg et al., 2008). The production of N2H4 from NH4+ and NO is catalyzed by the hydrazine synthase (HZS). The N2H4 is subsequently oxidized to N2 by the hydrazine dehydrogenase (HDH), also known as hydrazine oxidase (HZO) (Jetten et al., 2009; Kartal et al., 2011; Simon and Klotz, 2013). Various functional genes have been used as anammox biomarkers in marine systems: Scalindua-like nirS, coding for a NirS specific to Ca. Scalindua, the dominant anammox bacteria in marine OMZs (Lam et al., 2009); hzoAB (Hirsch et al., 2011; Lisa et al., 2014), coding for part of the HDH and with numerous divergent copies in a number of anammox bacteria (Strous et al., 2006); and hzsA, coding for part of the HZS, which has been suggested as the most suitable biomarker for the process (Harhangi et al., 2012; Han et al., 2017).
Until now, 10 Candidatus species belonging to five genera have been reported as responsible for anammox, all of them within a deep, monophyletic branch in the order Planctomycetales: Kuenenia, Anammoxoglobus and Jettenia with one species each, Brocadia with three species, and Scalindua with four (Kartal et al., 2012; van de Vossenberg et al., 2013). Anammox bacteria are slow-growing anaerobic autotrophs with great affinity for NO2– and NH4+ (Jetten et al., 2009) that possess a unique capability of producing and converting N2H4 in a ladderane lipid membrane called anammoxosome (Kartal et al., 2012).
Anammox coupled to the reduction of sulfate (Sulfammox) and Fe+3 (Feammox) has been recently reported in coastal sediments, where both processes may promote significant N losses (Rios-Del Toro et al., 2018). Further studies are required to elucidate the key microbial organisms and mechanisms involved in N2 production by Sulfammox and Feammox.
Factors affecting anammox in marine systems
Although the presence of anammox bacteria may not be indicative of high anammox activity, several studies have shown that anammox bacterial abundance correlates with anammox rates in marine environments (e.g., Hou et al., 2013; Bale et al., 2014; Lisa et al., 2014). In general, anammox activity is mainly regulated by O2 and inorganic N concentrations and seems to be coupled with the NO2– liberated during aerobic NH4+ oxidation (Lam et al., 2007) and NO3– reduction (Thamdrup and Dalsgaard, 2002), and the NH4+ liberated during denitrification (Dalsgaard et al., 2003) and DNRA (Jensen et al., 2011). Despite being obligated anaerobes active only at O2 concentrations below 2 μM, anammox bacteria are resistant to O2 exposure; nevertheless, the process is inhibited at high O2 concentrations (Jetten et al., 2009). For example, studies have shown that O2 and NO2– co-limit the distribution of anammox bacteria in OMZs (Dalsgaard et al., 2003; Lam et al., 2007; Pitcher et al., 2011a; Kong et al., 2013), although NH4+ appears to limit the process in these systems (Lam and Kuypers, 2011). Furthermore, the availability of inorganic N regulates anammox activity in coastal and estuarine sediments (e.g., Trimmer et al., 2005; Nicholls and Trimmer, 2009; Teixeira et al., 2016) in which the fluctuating availability of O2, NO3– and NO2– typically favors denitrifying microorganisms over anammox bacteria (Risgaard-Petersen et al., 2005).
In many marine environments anammox is highly dependent on salinity (Rich et al., 2008; Dale et al., 2009; Sonthiphand et al., 2014), temperature (Shehzad et al., 2016; Qian et al., 2018), but mostly on organic matter content (see Section Factors affecting denitrification in marine systems; e.g., Trimmer and Engström, 2011; Babbin et al., 2014). For example, recent studies have demonstrated that organic N substrates could support anammox in OMZs (Babbin et al., 2017; Ganesh et al., 2018), particularly in productive shelf waters (Kalvelage et al., 2013). The contribution of anammox to N2 loss in sediments seems to be higher at greater water depths where mineralization rates are lower and, therefore, denitrification too. However, anammox rates tend to decrease in deeper sediments because is limited by the NH4+ availability (Thamdrup, 2012, and references therein). Conversely, high organic C concentrations in shallow sediments usually stimulate denitrification while suppressing anammox because of the competition for NO2– (Nicholls and Trimmer, 2009; Brin et al., 2014). Nevertheless, a number of studies have found positive correlations between organic C content and anammox rates in marine sediments caused by high production of NH4+ or NO2– from remineralization and nitrification (Trimmer et al., 2003; Hou et al., 2013; Lisa et al., 2015). These contradictions show that understanding the relationship between organic C content and anammox is still an open question.
Distribution of anammox bacteria in marine environments
Anammox bacteria are present and active in a wide range of oxygen-depleted marine environments (Table 1) such as the OMZs (e.g., Jensen et al., 2011; Rich et al., 2018), eutrophic bays (e.g., Dang et al., 2010a; Lisa et al., 2014), estuarine sediments (e.g., Trimmer et al., 2005; Li et al., 2011), fjord sediments (Risgaard-Petersen et al., 2004; Brandsma et al., 2011), Arctic sediments (Rysgaard et al., 2004), deep-sea sediments (Hong et al., 2011a; Shao et al., 2014), and hydrothermal vents (Byrne et al., 2009). Below, we summarize the main findings from many studies on the distribution of anammox bacteria in representative marine environments.
Open oceans
OMZs are ideal environments for the growth of anammox bacteria. These bacteria are found in different OMZs such as those in the Black Sea (Kuypers et al., 2003), Golfo Dulce (Dalsgaard et al., 2003), ETNP (Rush et al., 2012; Kong et al., 2013), ETSP (Galán et al., 2009; Kalvelage et al., 2013), Colombian Pacific (Castro-González et al., 2014), Arabian Sea (Jaeschke et al., 2007; Lam et al., 2011), Eastern Indian Ocean (Qian et al., 2018) and Namibian upwelling system (Woebken et al., 2007; Kalvelage et al., 2011), where the anammox process accounts for between one-fifth and all of N2 production (Dalsgaard et al., 2005). In fact, anammox may be the dominant N-loss pathway in the OMZs of Namibia (Kuypers et al., 2005) and ETSP (Hamersley et al., 2007); although higher denitrification rates have also been found in the ETSP, suggesting that both processes may be temporally and/or spatially separated (Dalsgaard et al., 2012).
A low diversity of anammox communities has been detected in OMZs (Schmid et al., 2007; Kong et al., 2013), in which two clades of Ca. Scalindua typically predominate: Clade 1 (Ca. Scalindua sorokinii/brodae) and clade 2 (Ca. Scalindua arabica) (Woebken et al., 2008). Ca. Scalindua species also split into two clusters in the Black Sea: Ca. Scalindua richardsii, present in the upper suboxic zone at high NO2– and NO3– and low NH4+ concentrations, and Ca. Scalindua sorokinii, present in the lower suboxic zone at high NH4+ and low NO3– concentrations (Fuchsman et al., 2012).
Estuaries and coastal environments
Anammox has been reported mainly in eutrophic estuaries (e.g., Trimmer et al., 2003; Risgaard-Petersen et al., 2004; Lisa et al., 2015) and coastal sediments (e.g., Engström et al., 2005; Tal et al., 2005; Dang et al., 2013), where the distribution of anammox bacterial diversity and activity is mostly affected by temperature, salinity, NO3– and organic N substrates (Hou et al., 2013; Brin et al., 2014; Sonthiphand et al., 2014). Ca. Scalindua typically dominates throughout estuarine sediments (Tal et al., 2005; Rich et al., 2008; Dang et al., 2010a) while Ca. Brocadia, Ca. Kuenenia, Ca. Anammoxoglobous and Ca. Jettenia are mainly found in fresh to oligohaline sediments (Dale et al., 2009; Hirsch et al., 2011).
Deep-sea and other environments
Deep-sea and other extreme environments harbor a great diversity and abundance of Ca. Scalindua species (Hong et al., 2011a, b; Shehzad et al., 2016). Anammox bacteria are also active in hydrothermal vent areas such as those in the Mid-Atlantic Ridge, where anammox occurs as high as 85°C (Byrne et al., 2009), the Okhotsk Sea, where hzo is highly abundant (Shao et al., 2014), and the Guaymas Basin, where Ca. Scalindua species are more abundant in cold hydrocarbon-rich sediments than hydrothermal vents (Russ et al., 2013).
Effects of Anthropogenic Activity on the Marine Nitrogen Cycle
The marine N cycle is being largely perturbed by human activity. Anthropogenic activities pertaining to the production of artificial fertilizers and fossil fuel combustion are mainly responsible for this imbalance, affecting the marine N cycle directly or indirectly (Figure 3). Direct alterations include the N inputs through riverine discharges and atmospheric deposition (Duce et al., 2008; Lee et al., 2016; Jickells et al., 2017) that cause eutrophication and the formation of anoxic or hypoxic areas in coastal areas (“dead zones”), impacting primary production and the marine trophic web (Vaquer-Sunyer and Duarte, 2008). Indirect alterations include activities that increase the atmospheric concentration of greenhouse gases, leading to ocean warming, acidification and deoxygenation. The impacts of these alterations on the marine N cycle remain highly uncertain (Gruber, 2016; Hutchins and Fu, 2017) and have been covered by multiple reviews (e.g., Voss et al., 2013; Wannicke et al., 2018).
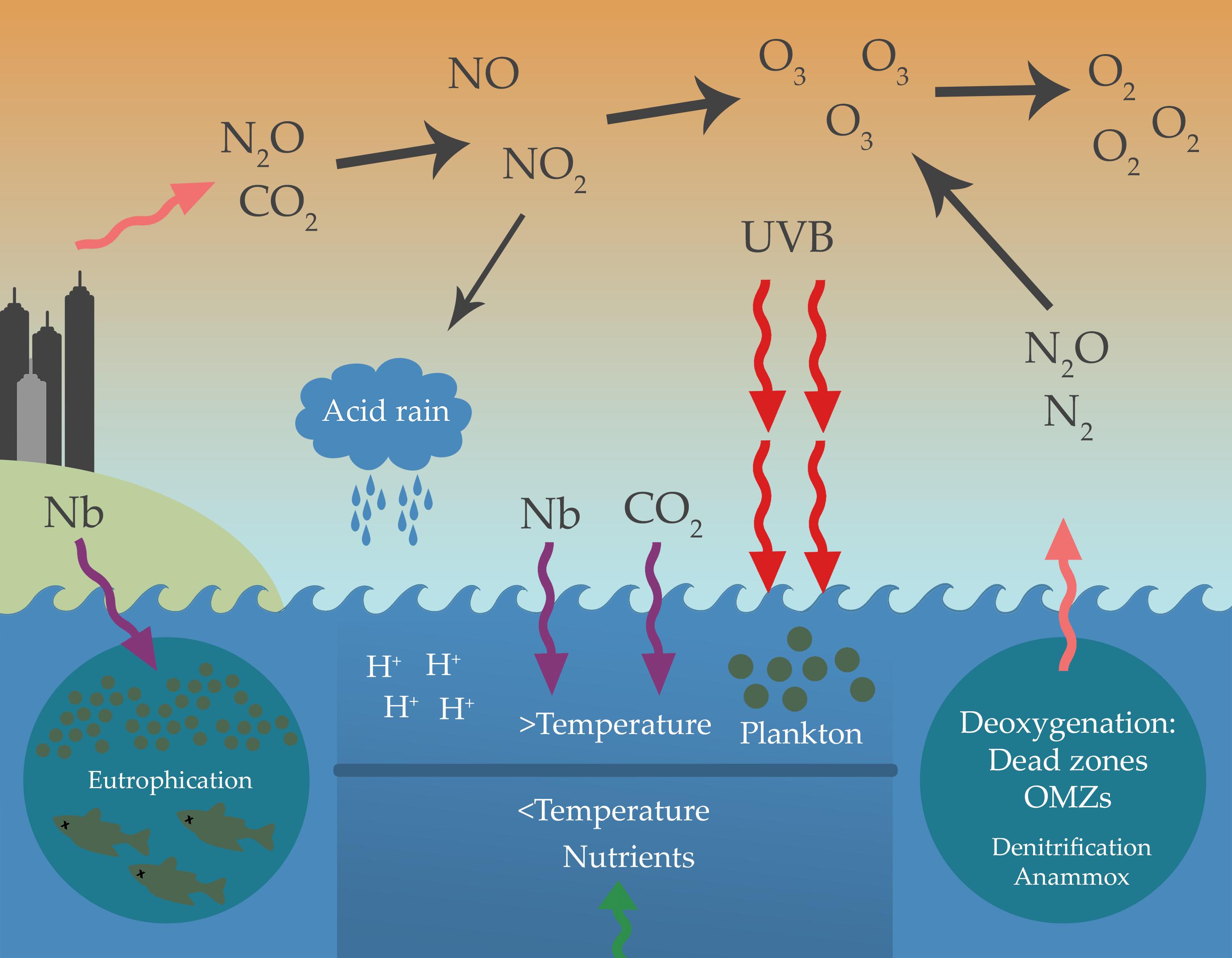
Figure 3. Anthropogenic activities and their effects on the marine N cycling. Bioavailable N (Nb) is introduced into the marine ecosystems by runoff or atmospheric deposition, causing eutrophication, the formation of dead zones and the expansion of the ocean minimum zones (OMZs). The release of N oxides (N2O, NO) from anthropogenic activities and oxygen-depleted zones causes stratospheric ozone depletion leading to higher UVB exposition, which produces the damage of marine life, acid rain and ocean warming. Ocean warming causes water stratification, deoxygenation, and the formation of dead zones. Dead zones and OMZs are hotspots for anammox and denitrification, causing N loss (N2 and N2O). Elevated atmospheric CO2 acidifies seawater, decreasing pH-dependent N-cycling processes such as nitrification, and enhancing N2 fixation.
Many studies have been conducted on the response of BNF in the future ocean. Recent models indicate that increased atmospheric N deposition will slow down BNF rates due to the enhanced availability of fixed N in the surface ocean (Somes et al., 2016; Yang and Gruber, 2016; Jickells et al., 2017). However, these models do not take in consideration other future ocean scenarios such as ocean acidification or warming. Several studies show that ocean acidification does not seem to have an effect on C and N2 fixation rates in mixed diazotrophic assemblages (Law et al., 2012; Böttjer et al., 2014). Conversely, other studies have documented the increase of C and N2 fixation rates in Trichodesmium and UCYN-B cultures when CO2 levels are increased to those expected in the future ocean (e.g., Lomas et al., 2012; Hutchins et al., 2015; Rees et al., 2017). This species-specific response of N2 fixation to ocean acidification may impact on the dominance of diazotrophic groups and also alter new N supply to the ocean; although this response seems to be dependent on light, P, and Fe (Hutchins et al., 2013). More studies on this topic are needed, since ocean acidification may lead to a decrease in the bioavailability of Fe (Shi et al., 2010), which may in turn lead to a decrease in BNF. Furthermore, ocean warming will likely cause an expansion of habitats suitable for diazotrophs and an increase of BNF (Hutchins et al., 2009; Sohm et al., 2011b), leading to an increase of available N for further processes in the N cycle.
Nitrification rates may decrease as a consequence of ocean acidification. Experiments have demonstrated decreases in NH3 oxidation due to the incremental protonation of NH3 to NH4+ as seawater pH decreases (Beman et al., 2011; Kitidis et al., 2011). A decrease in nitrification rates may reduce the supply of NO2– and NO3– to other N-cycling processes such as denitrification, anammox and DNRA, turning nitrification into a “N cycle bottleneck” (Hutchins et al., 2009) and putting NO3–-consumers in disadvantage over NH4+-consumers (Yool et al., 2007). Furthermore, a decrease in nitrification rates due to ocean acidification could reduce N2O production between 2.4 and 44% (Beman et al., 2011; Rees et al., 2016). In contrast, nitrification rates might exhibit an increase due to a “CO2-fertilization” effect (Hutchins et al., 2009); however, this assumption need to be further demonstrated, as evidence suggest that this is unlikely to happen given that AOA lack RuBisCo (Walker et al., 2010) and AOB possess mechanisms to reduce their sensibility to CO2 (Chain et al., 2003).
Little is known about how ocean warming may affect nitrification, but studies suggest that ammonia oxidizers may be relatively insensitive to changing temperature (Horak et al., 2013; Baer et al., 2014). Conversely, the global proliferation of suboxic waters as consequence of deoxygenation could promote nitrification, since this process occurs in transitional regions around OMZs where O2 is low but not fully depleted (Newell et al., 2011; Peng et al., 2015; Bristow et al., 2016).
Ammonia oxidation and denitrification are the major sources of marine N2O, but their contribution to the global N2O budget and the factors controlling its production and consumption are still being investigated. Moreover, the consequences of anthropogenic activities on N-loss processes have not been properly investigated. No direct effect of ocean acidification has been observed on these processes (Wannicke et al., 2018); although an increase in marine pCO2 may result in an elevated C:N ratio, indirectly enhancing denitrification (Hutchins et al., 2009) and decreasing anammox (Babbin et al., 2014). Models have also suggested that increased atmospheric N deposition and deoxygenation could enhance denitrification (Keeling et al., 2010; Somes et al., 2016; Yang and Gruber, 2016; Jickells et al., 2017), which in turn may increase by 21% the marine N2O production (Battaglia and Joos, 2018).
Alterations in the N cycling will also have mayor consequences for marine C cycling. However, the understanding of these consequences is poor due to the lack of enough global models. Some of these collateral alterations include modifications to CO2 budgets as result of elevated primary production due to the increase of available N entering marine ecosystems. Calculations suggest that atmospheric N deposition represents ∼32% of the total N entering the ocean, which translates into ∼3% of the annual new marine production (∼0.3 Pg C yr–1) (Duce et al., 2008). Other authors have estimated that anthropogenic atmospheric N inputs are currently leading to an increase in primary production and CO2 uptake of 0.15 Pg C yr–1 (Jickells et al., 2017). However, the resulting reduction in radiative forcing will be offset by increases in marine N2O emissions (Suntharalingam et al., 2012). Further, the CO2 budgets could also be altered as result of an increase of hypoxia and denitrification generated by N inputs in coastal environments. In addition, the dominance of either denitrification or anammox in expanding dead zones and OMZs will impact global C and N budgets. In marine environments dominated by denitrification, CO2 and N2O emissions are likely to increase, while in environments dominated by anammox, atmospheric CO2 uptake is likely to increase (Koeve and Kähler, 2010; Suntharalingam et al., 2012).
Conclusion and Perspectives
In this review, we have provided a panorama of the genetics, ecology and distribution of marine N-cycling microbes and the processes they mediate. These processes are more widely distributed than previously thought, given that they have been found in unpredicted marine environments. Plus, many new metabolic N pathways have been reported over the past few years, completely changing the paradigm of the classic marine N cycle. Additionally, we have examined the potential effects of human activity on N processes; such activity has led to an alteration of the natural balance of the marine N cycle, with consequences that we are just beginning to experience and comprehend. Many models have been developed to help us foresee the consequences of anthropogenic activities on the marine N cycle. However, these mathematical representations of the future cannot be completely trusted as they have been developed based on our current understating of the N processes, and we still have a long way to go given that new discoveries are made every day. Thus, we need to build models based on an holistic view of the processes and scenarios, using all the available information and taking into consideration all the possible natural settings. To do this, we should first understand the functioning of the microorganisms involved in the marine N processes. Finally, we identify potential priority research topics regarding marine microbial N cycle in which future investigation should be directed (Box 1).
BOX 1. Priority research topics on the marine N cycle.
∙ Further studying of the physiology, metabolism, genetics, and ecology of microorganisms participating in novel and previously established N-cycling processes (e.g., UCYN groups, heterotrophic diazotrophs, AOA clades, NOB, DNRA communities, ANME-2d, NC10 bacteria, anammox bacteria).
∙ Exploring N processes in understudied marine systems (e.g., BNF in coastal zones, nitrification and denitrification in coral reefs, comammox in the ocean, anaerobic N processes in sinking particles, and N processes in deep sea environments).
∙ Investigating the interactions and couplings between N cycle processes.
∙ A more comprehensive understanding of the environmental and ecological interaction between N-gain and N-loss processes in order to advance toward a more robust estimates and predictions of the marine N budget. ∙ A mechanistic understanding of the potential effects of anthropogenic activity on marine N processes and their interaction with other biogeochemical processes.
Author Contributions
All authors listed have made a substantial, direct and intellectual contribution to the work, and approved it for publication.
Funding
Funding for this work was granted by PAPIIT-UNAM No. IA201617. RR’s Master studies were supported by a CONACYT scholarship. Financial support for language editing and article processing fee was provided by the Instituto de Ciencias del Mar y Limnología (UNAM).
Conflict of Interest
The authors declare that the research was conducted in the absence of any commercial or financial relationships that could be construed as a potential conflict of interest.
Acknowledgments
This manuscript has been released as a pre-print at https://www.biogeosciences-discuss.net/bg-2018-279/ (Ramos and Pajares, 2018). We would like to thank the reviewers and the scientific community for their valuable feedback that have greatly improved this manuscript.
References
Abell, G., Revill, A. T., Smith, C., Bissett, A. P., Volkman, J. K., and Robert, S. S. (2010). Archaeal ammonia oxidizers and nirS-type denitrifiers dominate sediment nitrifying and denitrifying populations in a subtropical macrotidal estuary. ISME J. 4, 286–300. doi: 10.1038/ismej.2009.105
Abell, G., Ross, D., Keane, J., Oakes, J., Eyre, B., Robert, S., et al. (2013). Nitrifying and denitrifying microbial communities and their relationship to nutrient fluxes and sediment geochemistry in the Derwent Estuary, Tasmania. Aquat. Microb. Ecol. 70, 63–75. doi: 10.3354/ame01642
Agawin, N. S. R., Benavides, M., Busquets, A., Ferriol, P., Stal, L. J., and Arístegui, J. (2014). Dominance of unicellular cyanobacteria in the diazotrophic community in the Atlantic Ocean. Limnol. Oceanogr. 59, 623–637. doi: 10.4319/lo.2014.59.2.0623
Agogué, H., Brink, M., Dinasquet, J., and Herndl, G. J. (2008). Major gradients in putatively nitrifying and non-nitrifying archaea in the deep North Atlantic. Nature 456, 788–791. doi: 10.1038/nature07535
Allen, A. E., Booth, M. G., Frischer, M. E., Verity, P. G., Zehr, J. P., and Zani, S. (2001). Diversity and detection of nitrate assimilation genes in marine bacteria. Appl. Environ. Microbiol. 67, 5343–5348. doi: 10.1128/AEM.67.11.5343-5348.2001
Alonso-Sáez, L., Waller, A. S., Mende, D. R., Bakker, K., Farnelid, H., Yager, P. L., et al. (2012). Role for urea in nitrification by polar marine Archaea. Proc. Natl. Acad. Sci. U.S.A. 109, 17989–17994. doi: 10.1073/pnas.1201914109
Arévalo-Martínez, D. L., Kock, A., Löscher, C. R., Schmitz, R. A., and Bange, H. W. (2015). Massive nitrous oxide emissions from the Tropical South Pacific Ocean. Nat. Geosci. 8, 530–533. doi: 10.1038/ngeo2469
Arp, D. J., Chain, P. S. G., and Klotz, M. G. (2007). The impact of genome analyses on our understanding of ammonia-oxidizing bacteria. Annu. Rev. Microbiol. 61, 503–528. doi: 10.1146/annurev.micro.61.080706.093449
Atkinson, S. J., Mowat, C. G., Reid, G. A., and Chapman, S. K. (2007). An octaheme c-type cytochrome from Shewanella oneidensis can reduce nitrite and hydroxylamine. FEBS Lett. 581, 3805–3808. doi: 10.1016/j.febslet.2007.07.005
Babbin, A. R., Bianchi, D., Jayakumar, A., and Ward, B. B. (2015). Rapid nitrous oxide cycling in the suboxic ocean. Science 348, 1127–1129. doi: 10.1126/science.aaa8380
Babbin, A. R., Keil, R. G., Devol, A. H., and Ward, B. B. (2014). Organic matter stoichiometry, flux, and oxygen control nitrogen loss in the ocean. Science 344, 406–408. doi: 10.1126/science.1248364
Babbin, A. R., Peters, B. D., Mordy, C. W., Widner, B., Casciotti, K. L., and Ward, B. B. (2017). Multiple metabolisms constrain the anaerobic nitrite budget in the Eastern Tropical South Pacific. Global Biogeochem. Cycles 31, 258–271. doi: 10.1002/2016GB005407
Baer, S. E., Connelly, T. L., Sipler, R. E., Yager, P. L., and Bronk, D. A. (2014). Effect of temperature on rates of ammonium uptake and nitrification in the western coastal Arctic during winter, spring, and summer. Global Biogeochem. Cycles 28, 1455–1466. doi: 10.1002/2013GB004765
Baker, B. J., Lesniewski, R. A., and Dick, G. J. (2012). Genome- enabled transcriptomics reveals archaeal populations that drive nitrification in a deep-sea hydrothermal plume. ISME J. 6, 2269–2279. doi: 10.1038/ismej.2012.64
Baker, B. J., Sheik, C. S., Taylor, C. A., Jain, S., Bhasi, A., Cavalcoli, J. D., et al. (2013). Community transcriptomic assembly reveals microbes that contribute to deep-sea carbon and nitrogen cycling. ISME J. 7, 1962–1973. doi: 10.1038/ismej.2013.85
Bale, N. J., Villanueva, L., Fan, H., Stal, L. J., Hopmans, E. C., Schouten, S., et al. (2014). Occurrence and activity of anammox bacteria in surface sediments of the southern North Sea. FEMS Microbiol. Ecol. 89, 99–110. doi: 10.1111/1574-6941.12338
Bandekar, M., Ramaiah, N., and Meena, R. M. (2018). Diversity and abundance of denitrifying and anammox bacteria from the Arabian Sea oxygen minimum zone. Deep Sea Res. Part II Top. Stud. Oceanogr. 156, 19–26. doi: 10.1016/j.dsr2.2018.08.008
Bange, H. W., Freing, A., Kock, A., and Löscher, C. R. (2010). “Marine pathways to nitrous oxide,” in Nitrous Oxide and Climate Change, ed. K. Smith, (London: Earthscan), 36–62.
Battaglia, G., and Joos, F. (2018). Marine N2O emissions from nitrification and denitrification constrained by modern observations and projected in multimillennial global warming simulations. Global Biogeochem. Cycles 32, 92–121. doi: 10.1002/2017GB005671
Beman, J. M., and Francis, C. A. (2006). Diversity of ammonia-oxidizing archaea and bacteria in the sediments of a hypernutrified subtropical estuary: bahia del Tobari, Mexico. Appl. Environ. Microbiol. 72, 7767–7777. doi: 10.1128/AEM.00946-946
Beman, J. M., Chow, C.-E., King, A. L., Feng, Y., Fuhrman, J. A., Andersson, A., et al. (2011). Global declines in oceanic nitrification rates as a consequence of ocean acidification. Proc. Natl. Acad. Sci. U.S.A. 108, 208–213. doi: 10.1073/pnas.1011053108
Beman, J. M., Leilei Shih, J., and Popp, B. N. (2013). Nitrite oxidation in the upper water column and oxygen minimum zone of the Eastern Tropical North Pacific Ocean. ISME J. 7, 2192–2205. doi: 10.1038/ismej.2013.96
Beman, J. M., Popp, B. N., and Alford, S. E. (2012). Quantification of ammonia oxidation rates and ammonia-oxidizing archaea and bacteria at high resolution in the Gulf of California and Eastern Tropical North Pacific Ocean. Limnol. Oceanogr. 57, 711–726. doi: 10.4319/lo.2012.57.3.0711
Beman, J. M., Popp, B. N., and Francis, C. A. (2008). Molecular and biogeochemical evidence for ammonia oxidation by marine crenarchaeota in the Gulf of California. ISME J. 2, 429–441. doi: 10.1038/ismej.2007.118
Beman, J. M., Roberts, K. J., Wegley, L., Rohwer, F., and Francis, C. A. (2007). Distribution and diversity of archaeal ammonia monooxygenase genes associated with corals. Appl. Environ. Microbiol. 73, 5642–5647. doi: 10.1128/AEM.00461-467
Beman, J. M., Sachdeva, R., and Fuhrman, J. A. (2010). Population ecology of nitrifying archaea and bacteria in the Southern California bight. Environ. Microbiol. 12, 1282–1292. doi: 10.1111/j.1462-2920.2010.02172.x
Benavides, M., Bednarz, V. N., and Ferrier-Pagès, C. (2017a). Diazotrophs: overlooked key players within the coral symbiosis and tropical reef ecosystems? Front. Mar. Sci. 4:10. doi: 10.3389/fmars.2017.00010
Benavides, M., Berthelot, H., Duhamel, S., Raimbault, P., and Bonnet, S. (2017b). Dissolved organic matter uptake by Trichodesmium in the Southwest Pacific. Sci. Rep. 7:41315. doi: 10.1038/srep41315
Benavides, M., Bonnet, S., Hernández, N., Martínez-Pérez, A. M., Nieto-Cid, M., Álvarez-Salgado, X. A., et al. (2016a). Basin-wide N2 fixation in the deep waters of the Mediterranean Sea. Global Biogeochem. Cycles 30, 952–961. doi: 10.1002/2015GB005326
Benavides, M., Houlbrèque, F., Camps, M., Lorrain, A., Grosso, O., and Bonnet, S. (2016b). Diazotrophs: a non-negligible source of nitrogen for the tropical coral Stylophora pistillata. J. Exp. Biol. 219, 2608–2612. doi: 10.1242/jeb.139451
Benavides, M., Moisander, P. H., Berthelot, H., Dittmar, T., Grosso, O., and Bonnet, S. (2015). Mesopelagic N2 fixation related to organic matter composition in the Solomon and Bismarck Seas (Southwest Pacific). PLoS One 10:e0143775. doi: 10.1371/journal.pone.0143775
Bentzon-Tilia, M., Traving, S. J., Mantikci, M., Knudsen-Leerbeck, H., Hansen, J. L. S., Markager, S., et al. (2015). Significant N2 fixation by heterotrophs, photoheterotrophs and heterocystous cyanobacteria in two temperate estuaries. ISME J. 9, 273–285. doi: 10.1038/ismej.2014.119
Berg, C., Vandieken, V., Thamdrup, B., and Jürgens, K. (2015). Significance of archaeal nitrification in hypoxic waters of the Baltic Sea. ISME J. 9, 1319–1332. doi: 10.1038/ismej.2014.218
Bergman, B., and Carpenter, E. J. (1991). Nitrogenase confined to randomly distributed trichomes in the marine cyanobacterium Trichodesmium thiebautii. J. Phycol. 27, 158–165. doi: 10.1111/j.0022-3646.1991.00158.x
Berman-Frank, I., Cullen, J. T., Shaked, Y., Sherrell, R. M., and Falkowski, P. G. (2001a). Iron availability, cellular iron quotas, and nitrogen fixation in Trichodesmium. Limnol. Oceanogr. 46, 1249–1260. doi: 10.4319/lo.2001.46.6.1249
Berman-Frank, I., Lundgren, P., Chen, Y.-B., Küpper, H., Kolber, Z., Bergman, B., et al. (2001b). Segregation of nitrogen fixation and oxygenic photosynthesis in the marine cyanobacterium Trichodesmium. Science 294, 1534–1537. doi: 10.1126/science.1064082
Bernhard, A. E., Donn, T., Giblin, A. E., and Stahl, D. A. (2005). Loss of diversity of ammonia-oxidizing bacteria correlates with increasing salinity in an estuary system. Environ. Microbiol. 7, 1289–1297. doi: 10.1111/j.1462-2920.2005.00808.x
Bernhard, A. E., Landry, Z. C., Blevins, A., de la Torre, J. R., Giblin, A. E., and Stahl, D. A. (2010). Abundance of ammonia-oxidizing archaea and bacteria along an estuarine salinity gradient in relation to potential nitrification rates. Appl. Environ. Microbiol. 76, 1285–1289. doi: 10.1128/AEM.02018-2019
Bertagnolli, A. D., and Ulloa, O. (2017). Hydrography shapes community composition and diversity of amoA-containing thaumarchaeota in the coastal waters off central Chile. Environ. Microbiol. Rep. 9, 717–728. doi: 10.1111/1758-2229.12579
Berthelot, H., Benavides, M., Moisander, P. H., Grosso, O., and Bonnet, S. (2017). High-nitrogen fixation rates in the particulate and dissolved pools in the Western Tropical Pacific (Solomon and Bismarck Seas). Geophys. Res. Lett. 44, 8414–8423. doi: 10.1002/2017GL073856
Blais, M., Tremblay, J.-E., Jungblut, A. D., Gagnon, J., Martin, J., Thaler, M., et al. (2012). Nitrogen fixation and identification of potential diazotrophs in the Canadian Arctic. Global Biogeochem. Cycles 26:GB3022. doi: 10.1029/2011GB004096
Bombar, D., Paerl, R. W., and Riemann, L. (2016). Marine non-cyanobacterial diazotrophs: moving beyond molecular detection. Trends Microbiol. 24, 916–927. doi: 10.1016/j.tim.2016.07.002
Bonnet, S., Biegala, I. C., Dutrieux, P., Slemons, L. O., and Capone, D. G. (2009). Nitrogen fixation in the Eestern Equatorial Pacific: rates, diazotrophic cyanobacterial size class distribution, and biogeochemical significance. Global Biogeochem. Cycles 23:GB3012. doi: 10.1029/2008GB003439
Bonnet, S., Dekaezemacker, J., Turk-Kubo, K. A., Moutin, T., Hamersley, R. M., Grosso, O., et al. (2013). Aphotic N2 fixation in the Eastern Tropical South Pacific Ocean. PLoS One 8:e81265. doi: 10.1371/journal.pone.0081265
Bonnet, S., Rodier, M., Turk-Kubo, K. A., Germineaud, C., Menkes, C., Ganachaud, A., et al. (2015). Contrasted geographical distribution of N2 fixation rates and nifH phylotypes in the Coral and Solomon Seas (southwestern Pacific) during austral winter conditions. Global Biogeochem. Cycles 29, 1874–1892. doi: 10.1002/2015GB005117
Böttjer, D., Karl, D. M., Letelier, R. M., Viviani, D. A., and Church, M. J. (2014). Experimental assessment of diazotroph responses to elevated seawater pCO2 in the North Pacific subtropical gyre. Global Biogeochem. Cycles 28, 601–616. doi: 10.1002/2013GB004690
Bourbonnais, A., Juniper, S. K., Butterfield, D. A., Anderson, R. E., and Lehmann, M. F. (2014). Diversity and abundance of bacteria and nirS-encoding denitrifiers associated with the juan de fuca ridge hydrothermal system. Ann. Microbiol. 64, 1691–1705. doi: 10.1007/s13213-014-0813-813
Bourbonnais, A., Letscher, R. T., Bange, H. W., Échevin, V., Larkum, J., Mohn, J., et al. (2017). N2O production and consumption from stable isotopic and concentration data in the peruvian coastal upwelling system. Global Biogeochem. Cycles 31, 678–698. doi: 10.1002/2016GB005567
Bouskill, N. J., Eveillard, D., Chien, D., Jayakumar, A., and Ward, B. B. (2012). Environmental factors determining ammonia-oxidizing organism distribution and diversity in marine environments. Environ. Microbiol. 14, 714–729. doi: 10.1111/j.1462-2920.2011.02623.x
Bowles, M. W., Nigro, L. M., Teske, A. P., and Joye, S. B. (2012). Denitrification and environmental factors influencing nitrate removal in Guaymas Basin hydrothermally altered sediments. Front. Microbiol. 3:377. doi: 10.3389/fmicb.2012.00377
Braker, G., and Tiedje, J. M. (2003). Nitric oxide reductase (norB) genes from pure cultures and environmental samples. Appl. Environ. Microbiol. 69, 3476–3483. doi: 10.1128/AEM.69.6.3476-3483.2003
Braker, G., Zhou, J., Wu, L., Devol, A. H., and Tiedje, J. M. (2000). Nitrite reductase genes (nirK and nirS) as functional markers to investigate diversity of denitrifying bacteria in Pacific northwest marine sediment communities. Appl. Environ. Microbiol. 66, 2096–2104. doi: 10.1128/AEM.66.5.2096-2104.2000
Brandsma, J., van de Vossenberg, J., Risgaard-Petersen, N., Schmid, M. C., Engström, P., Eurenius, K., et al. (2011). A multi-proxy study of anaerobic ammonium oxidation in marine sediments of the Gullmar Fjord. Sweden. Environ. Microbiol. Rep. 3, 360–366. doi: 10.1111/j.1758-2229.2010.00233.x
Brin, L. D., Giblin, A. E., and Rich, J. J. (2014). Environmental controls of anammox and denitrification in southern New England estuarine and shelf sediments. Limnol. Oceanogr. 59, 851–860. doi: 10.4319/lo.2014.59.3.0851
Bristow, L. A., Callbeck, C. M., Larsen, M., Altabet, M. A., Dekaezemacker, J., Forth, M., et al. (2017). N2 production rates limited by nitrite availability in the Bay of Bengal oxygen minimum zone. Nat. Geosci. 10, 24–29. doi: 10.1038/ngeo2847
Bristow, L. A., Dalsgaard, T., Tiano, L., Mills, D. B., Bertagnolli, A. D., Wright, J. J., et al. (2016). Ammonium and nitrite oxidation at nanomolar oxygen concentrations in oxygen minimum zone waters. Proc. Natl. Acad. Sci. U.S.A. 113, 10601–10606. doi: 10.1073/pnas.1600359113
Bristow, L. A., Sarode, N., Cartee, J., Caro-Quintero, A., Thamdrup, B., and Stewart, F. J. (2015). Biogeochemical and metagenomic analysis of nitrite accumulation in the Gulf of Mexico hypoxic zone. Limnol. Oceanogr. 60, 1733–1750. doi: 10.1002/lno.10130
Bu, C., Wang, Y., Ge, C., Ahmad, H. A., Gao, B., and Ni, S.-Q. (2017). Dissimilatory nitrate reduction to ammonium in the yellow river estuary: rates, abundance, and community diversity. Sci. Rep. 7:6830. doi: 10.1038/s41598-017-06404-6408
Buchwald, C., Santoro, A. E., Stanley, R. H. R., and Casciotti, K. L. (2015). Nitrogen cycling in the secondary nitrite maximum of the Eastern Tropical North Pacific off Costa Rica. Global Biogeochem. Cycles 29, 2061–2081. doi: 10.1002/2015GB005187
Burgin, A. J., and Hamilton, S. K. (2007). Have we overemphasized the role of denitrification in aquatic ecosystems? A review of nitrate removal pathways. Front. Ecol. Environ. 5, 89–96. doi: 10.1890/1540-929520075
Burns, J. A., Zehr, J. P., and Capone, D. G. (2002). Nitrogen-fixing phylotypes of chesapeake bay and neuse river estuary sediments. Microb. Ecol. 44, 336–343. doi: 10.1007/s00248-002-1000-1009
Byrne, N., Strous, M., Crépeau, V., Kartal, B., Birrien, J.-L., Schmid, M., et al. (2009). Presence and activity of anaerobic ammonium-oxidizing bacteria at deep-sea hydrothermal vents. ISME J. 3, 117–123. doi: 10.1038/ismej.2008.72
Cabello, A. M., Cornejo-Castillo, F. M., Raho, N., Blasco, D., Vidal, M., Audic, S., et al. (2016). Global distribution and vertical patterns of a prymnesiophyte–cyanobacteria obligate symbiosis. ISME J. 10, 693–706. doi: 10.1038/ismej.2015.147
Caffrey, J. M., Bano, N., Kalanetra, K., and Hollibaugh, J. T. (2007). Ammonia oxidation and ammonia-oxidizing bacteria and archaea from estuaries with differing histories of hypoxia. ISME J. 1, 660–662. doi: 10.1038/ismej.2007.79
Cao, H., Hong, Y., Li, M., and Gu, J. D. (2011). Diversity and abundance of ammonia-oxidizing prokaryotes in sediments from the coastal Pearl River Estuary to the South China Sea. Antonie Van Leeuwenhoek 100, 545–556. doi: 10.1007/s10482-011-9610-9611
Cao, H., Hong, Y., Li, M., and Gu, J. D. (2012). Lower abundance of ammonia-oxidizing archaea than ammonia-oxidizing bacteria detected in the subsurface sediments of the northern South China Sea. Geomicrobiol. J. 29, 332–339. doi: 10.1080/01490451.2011.559304
Cao, H., Shao, Z., Li, J., Zhang, W., and Qian, P. Y. (2015). Phylogenetic diversity of nitrogen-utilizing genes in hydrothermal chimneys from 3 middle ocean ridges. Extremophiles 19, 1173–1182. doi: 10.1007/s00792-015-0788-784
Capone, D. G. (1997). Trichodesmium, a globally significant marine cyanobacterium. Science 276, 1221–1229. doi: 10.1126/science.276.5316.1221
Capone, D. G., Burns, J. A., Montoya, J. P., Subramaniam, A., Mahaffey, C., Gunderson, T., et al. (2005). Nitrogen fixation by Trichodesmium spp.: an important source of new nitrogen to the tropical and subtropical North Atlantic Ocean. Global Biogeochem. Cycles 19:GB2024. doi: 10.1029/2004GB002331
Capone, D., Subramaniam, A., Montoya, J., Voss, M., Humborg, C., Johansen, A., et al. (1998). An extensive bloom of the N2-fixing cyanobacterium Trichodesmium erythraeum in the central Arabian Sea. Mar. Ecol. Prog. Ser. 172, 281–292. doi: 10.3354/meps172281
Caranto, J. D., and Lancaster, K. M. (2017). Nitric oxide is an obligate bacterial nitrification intermediate produced by hydroxylamine oxidoreductase. Proc. Natl. Acad. Sci. U.S.A. 114, 8217–8222. doi: 10.1073/pnas.1704504114
Caranto, J. D., Vilbert, A. C., and Lancaster, K. M. (2016). Nitrosomonas europaea cytochrome P460 is a direct link between nitrification and nitrous oxide emission. Proc. Natl. Acad. Sci. U.S.A. 113, 14704–14709. doi: 10.1073/pnas.1611051113
Cardini, U., Bednarz, V. N., Naumann, M. S., van Hoytema, N., Rix, L., Foster, R. A., et al. (2015). Functional significance of dinitrogen fixation in sustaining coral productivity under oligotrophic conditions. Proc. Biol. Sci. 282:20152257. doi: 10.1098/rspb.2015.2257
Cardoso, J., van Bleijswijk, J., Witte, H., and van Duyl, F. (2013). Diversity and abundance of ammonia-oxidizing archaea and bacteria in tropical and cold-water coral reef sponges. Aquat. Microb. Ecol. 68, 215–230. doi: 10.3354/ame01610
Carini, P., Dupont, C. L., and Santoro, A. E. (2018). Patterns of thaumarchaeal gene expression in culture and diverse marine environments. Environ. Microbiol. 20, 2112–2124. doi: 10.1111/1462-2920.14107
Casciotti, K. L., and Buchwald, C. (2012). Insights on the marine microbial nitrogen cycle from isotopic approaches to nitrification. Front. Microbiol. 3:356. doi: 10.3389/fmicb.2012.00356
Casciotti, K. L., and Ward, B. B. (2005). Phylogenetic analysis of nitric oxide reductase gene homologues from aerobic ammonia-oxidizing bacteria. FEMS Microbiol. Ecol. 52, 197–205. doi: 10.1016/j.femsec.2004.11.002
Casciotti, K. L., Forbes, M., Vedamati, J., Peters, B. D., Martin, T. S., and Mordy, C. W. (2018). Nitrous oxide cycling in the Eastern Tropical South Pacific as inferred from isotopic and isotopomeric data. Deep Sea Res. Part II Top. Stud. Oceanogr. 156, 155–167. doi: 10.1016/j.dsr2.2018.07.014
Castro-Gonzalez, M., Braker, G., Farias, L., and Ulloa, O. (2005). Communities of nirS-type denitrifiers in the water column of the oxygen minimum zone in the Eastern South Pacific. Environ. Microbiol. 7, 1298–1306. doi: 10.1111/j.1462-2920.2005.00809.x
Castro-González, M., Molina, V., Rodríguez-Rubio, E., and Ulloa, O. (2014). The first report of a microdiverse anammox bacteria community in waters of Colombian Pacific, a transition area between prominent oxygen minimum zones of the Eastern Tropical Pacific. Environ. Microbiol. Rep. 6, 595–604. doi: 10.1111/1758-2229.12165
Chain, P., Lamerdin, J., Larimer, F., Regala, W., Lao, V., Land, M., et al. (2003). Complete genome sequence of the ammonia-oxidizing bacterium and obligate chemolithoautotroph Nitrosomonas europaea. J. Bacteriol. 185, 2759–2773. doi: 10.1128/JB.185.9.2759-2773.2003
Chang, B. X., Rich, J. R., Jayakumar, A., Naik, H., Pratihary, A. K., Keil, R. G., et al. (2014). The effect of organic carbon on fixed nitrogen loss in the eastern tropical South Pacific and Arabian Sea oxygen deficient zones. Limnol. Oceanogr. 59, 1267–1274. doi: 10.4319/lo.2014.59.4.1267
Chen, J., Dick, R., Lin, J.-G., and Gu, J.-D. (2016). Current advances in molecular methods for detection of nitrite-dependent anaerobic methane oxidizing bacteria in natural environments. Appl. Microbiol. Biotechnol. 100, 9845–9860. doi: 10.1007/s00253-016-7853-7855
Chen, J., Jiang, X.-W. W., and Gu, J.-D. D. (2015a). Existence of novel phylotypes of nitrite-dependent anaerobic methane-oxidizing bacteria in surface and subsurface sediments of the South China Sea. Geomicrobiol. J. 32, 1–10. doi: 10.1080/01490451.2014.917742
Chen, J., Zhou, Z., and Gu, J.-D. (2015b). Complex community of nitrite-dependent anaerobic methane oxidation bacteria in coastal sediments of the Mai Po wetland by PCR amplification of both 16S rRNA and pmoA genes. Appl. Microbiol. Biotechnol. 99, 1463–1473. doi: 10.1007/s00253-014-6051-6056
Chen, J., Zhou, Z.-C., and Gu, J.-D. (2014). Occurrence and diversity of nitrite-dependent anaerobic methane oxidation bacteria in the sediments of the South China Sea revealed by amplification of both 16S rRNA and pmoA genes. Appl. Microbiol. Biotechnol. 98, 5685–5696. doi: 10.1007/s00253-014-5733-5734
Cheung, S., Suzuki, K., Saito, H., Umezawa, Y., Xia, X., and Liu, H. (2017). Highly heterogeneous diazotroph communities in the kuroshio current and the Tokara Strait Japan. PLoS One 12:e0186875. doi: 10.1371/journal.pone.0186875
Christman, G. D., Cottrell, M. T., Popp, B. N., Gier, E., and Kirchman, D. L. (2011). Abundance, diversity, and activity of ammonia-oxidizing prokaryotes in the coastal arctic ocean in summer and winter. Appl. Environ. Microbiol. 77, 2026–2034. doi: 10.1128/AEM.01907-1910
Chronopoulou, P. M., Shelley, F., Pritchard, W. J., Maanoja, S. T., and Trimmer, M. (2017). Origin and fate of methane in the Eastern Tropical North Pacific oxygen minimum zone. ISME J. 11, 1386–1399. doi: 10.1038/ismej.2017.6
Church, M. J., Wai, B., Karl, D. M., and DeLong, E. F. (2010). Abundances of crenarchaeal amoA genes and transcripts in the Pacific Ocean. Environ. Microbiol. 12, 679–688. doi: 10.1111/j.1462-2920.2009.02108.x
Ciais, P., Sabine, C., Bala, G., Bopp, L., Brovkin, V., Canadell, J., et al. (2013). “Carbon and other biogeochemical cycles,” in Climate Change 2013: The Physical Science Basis. Contribution of Working Group I to the Fifth Assessment Report of the Intergovernmental Panel on Climate Change, eds T. F. Stocker, D. Qin, G. K. Plattner, M. Tignor, S. K. Allen, J. Boschung, et al. (Geneva: IPCC), 465–570.
Coates, C. J., and Wyman, M. (2017). A denitrifying community associated with a major, marine nitrogen fixer. Environ. Microbiol. 19, 4978–4992. doi: 10.1111/1462-2920.14007
Codispoti, L. A. (2007). An oceanic fixed nitrogen sink exceeding 400 Tg N a−1 vs the concept of homeostasis in the fixed-nitrogen inventory. Biogeosciences 4, 233–253. doi: 10.5194/bg-4-233-2007
Codispoti, L. A., Brandes, J. A., Christensen, J. P., Devol, A. H., Naqvi, S. W. A., Paerl, H. W., et al. (2001). The oceanic fixed nitrogen and nitrous oxide budgets: moving targets as we enter the anthropocene? Sci. Mar. 65, 85–105. doi: 10.3989/scimar.2001.65s285
Daims, H., Lebedeva, E. V., Pjevac, P., Han, P., Herbold, C., Albertsen, M., et al. (2015). Complete nitrification by Nitrospira bacteria. Nature 528, 504–509. doi: 10.1038/nature16461
Daims, H., Lücker, S., and Wagner, M. (2016). A new perspective on microbes formerly known as nitrite-oxidizing bacteria. Trends Microbiol. 24, 699–712. doi: 10.1016/j.tim.2016.05.004
Dale, O. R., Tobias, C. R., and Song, B. (2009). Biogeographical distribution of diverse anaerobic ammonium oxidizing (anammox) bacteria in Cape Fear River Estuary. Environ. Microbiol. 11, 1194–1207. doi: 10.1111/j.1462-2920.2008.01850.x
Dalsgaard, T., Canfield, D. E., Petersen, J., Thamdrup, B., and Acuña-González, J. (2003). N2 production by the anammox reaction in the anoxic water column of Golfo Dulce Costa Rica. Nature 422, 606–608. doi: 10.1038/nature01526
Dalsgaard, T., Stewart, F. J., Thamdrup, B., De Brabandere, L., Revsbech, N. P., Ulloa, O., et al. (2014). Oxygen at nanomolar levels reversibly suppresses process rates and gene expression in anammox and denitrification in the oxygen minimum zone off northern Chile. mBio 5:e1966-14. doi: 10.1128/mBio.01966-1914
Dalsgaard, T., Thamdrup, B., and Canfield, D. E. (2005). Anaerobic ammonium oxidation (anammox) in the marine environment. Res. Microbiol. 156, 457–464. doi: 10.1016/j.resmic.2005.01.011
Dalsgaard, T., Thamdrup, B., Farías, L., and Revsbech, N. P. (2012). Anammox and denitrification in the oxygen minimum zone of the Eastern South Pacific. Limnol. Oceanogr. 57, 1331–1346. doi: 10.4319/lo.2012.57.5.1331
Damashek, J., and Francis, C. A. (2018). Microbial nitrogen cycling in estuaries: from genes to ecosystem processes. Estuaries Coasts 41, 626–660. doi: 10.1007/s12237-017-0306-302
Dang, H., Chen, R., Wang, L., Guo, L., Chen, P., Tang, Z., et al. (2010a). Environmental factors shape sediment anammox bacterial communities in hypernutrified Jiaozhou Bay, China. Appl. Environ. Microbiol. 76, 7036–7047. doi: 10.1128/AEM.01264-1210
Dang, H., Li, J., Zhang, X., Li, T., Tian, F., and Jin, W. (2009a). Diversity and spatial distribution of amoA-encoding archaea in the deep-sea sediments of the tropical west Pacific continental margin. J. Appl. Microbiol. 106, 1482–1493. doi: 10.1111/j.1365-2672.2008.04109.x
Dang, H., Luan, X., Chen, R., Zhang, X., Guo, L., and Klotz, M. G. (2010b). Diversity, abundance and distribution of amoA-encoding archaea in deep-sea methane seep sediments of the Okhotsk Sea. FEMS Microbiol. Ecol. 72, 370–385. doi: 10.1111/j.1574-6941.2010.00870.x
Dang, H., Luan, X., Zhao, J., and Li, J. (2009b). Diverse and novel nifH and nifH-like gene sequences in the deep-sea methane seep sediments of the Okhotsk Sea. Appl. Environ. Microbiol. 75, 2238–2245. doi: 10.1128/AEM.02556-2558
Dang, H., Zhou, H., Zhang, Z., Yu, Z., Hua, E., Liu, X., et al. (2013). Molecular detection of Candidatus Scalindua pacifica and environmental responses of sediment anammox bacterial community in the Bohai Sea China. PLoS One 8:e61330. doi: 10.1371/journal.pone.0061330
De Brabandere, L., Canfield, D. E., Dalsgaard, T., Friederich, G. E., Revsbech, N. P., Ulloa, O., et al. (2014). Vertical partitioning of nitrogen-loss processes across the oxic-anoxic interface of an oceanic oxygen minimum zone. Environ. Microbiol. 16, 3041–3054. doi: 10.1111/1462-2920.12255
De Corte, D., Yokokawa, T., Varela, M. M., Agogué, H., and Herndl, G. J. (2009). Spatial distribution of bacteria and archaea and amoA gene copy numbers throughout the water column of the eastern Mediterranean Sea. ISME J. 3, 147–158. doi: 10.1038/ismej.2008.94
Decleyre, H., Heylen, K., Van Colen, C., and Willems, A. (2015). Dissimilatory nitrogen reduction in intertidal sediments of a temperate estuary: small scale heterogeneity and novel nitrate-to-ammonium reducers. Front. Microbiol. 6:1124. doi: 10.3389/fmicb.2015.01124
Dekaezemacker, J., and Bonnet, S. (2011). Sensitivity of N2 fixation to combined nitrogen forms (NO3– and NO4+) in two strains of the marine diazotroph Crocosphaera watsonii (Cyanobacteria). Mar. Ecol. Prog. Ser. 438, 33–46. doi: 10.3389/fmicb.2012.00374
Dekas, A. E., Chadwick, G. L., Bowles, M. W., Joye, S. B., and Orphan, V. J. (2014). Spatial distribution of nitrogen fixation in methane seep sediment and the role of the ANME archaea. Environ. Microbiol. 16, 3012–3029. doi: 10.1111/1462-2920.12247
Dekas, A. E., Fike, D. A., Chadwick, G. L., Green-Saxena, A., Fortney, J., Connon, S. A., et al. (2018). Widespread nitrogen fixation in sediments from diverse deep-sea sites of elevated carbon loading. Environ. Microbiol. 20, 4281–4296. doi: 10.1111/1462-2920.14342
Dekas, A. E., Poretsky, R. S., and Orphan, V. J. (2009). Deep-sea archaea fix and share nitrogen in methane-consuming microbial consortia. Science 326, 422–426. doi: 10.1126/science.1178223
Delmont, T. O., Quince, C., Shaiber, A., Esen, O. C., Lee, S. T., Rappé, M. S., et al. (2018). Nitrogen-fixing populations of Planctomycetes and Proteobacteria are abundant in surface ocean metagenomes. Nat. Microbiol. 3, 804–813. doi: 10.1038/s41564-018-0176-179
DeLong, E. F., Preston, C. M., Mincer, T., Rich, V., Hallam, S. J., Frigaard, N. U., et al. (2006). Community genomics among stratified microbial assemblages in the ocean’s interior. Science 311, 496–503. doi: 10.1126/science.1120250
Deutsch, C., Sarmiento, J. L., Sigman, D. M., Gruber, N., and Dunne, J. P. (2007). Spatial coupling of nitrogen inputs and losses in the ocean. Nature 445, 163–167. doi: 10.1038/nature05392
Devol, A. H. (2015). Denitrification, anammox, and N2 production in marine sediments. Ann. Rev. Mar. Sci. 7, 403–423. doi: 10.1146/annurev-marine-010213-135040
Dong, L. F., Naqasima Sobey, M., Smith, C. J., Rusmana, I., Phillips, W., Stott, A., et al. (2011). Dissimilatory reduction of nitrate to ammonium, not denitrification or anammox, dominates benthic nitrate reduction in tropical estuaries. Limnol. Oceanogr. 56, 279–291. doi: 10.4319/lo.2011.56.1.0279
Dong, L. F., Smith, C. J., Papaspyrou, S., Stott, A., Osborn, A. M., and Nedwell, D. B. (2009). Changes in benthic denitrification, nitrate ammonification, and anammox process rates and nitrate and nitrite reductase gene abundances along an estuarine nutrient gradient (the Colne Estuary, United Kingdom). Appl. Environ. Microbiol. 75, 3171–3179. doi: 10.1128/AEM.02511-2518
Duce, R. A., LaRoche, J., Altieri, K., Arrigo, K. R., Baker, A. R., Capone, D. G., et al. (2008). Impacts of atmospheric anthropogenic nitrogen on the open ocean. Science 320, 893–897. doi: 10.1126/science.1150369
Engström, P., Dalsgaard, T., Hulth, S., and Aller, R. C. (2005). Anaerobic ammonium oxidation by nitrite (anammox): implications for N2 production in coastal marine sediments. Geochim. Cosmochim. Acta 69, 2057–2065. doi: 10.1016/j.gca.2004.09.032
Ettwig, K. F., Butler, M. K., Le Paslier, D., Pelletier, E., Mangenot, S., Kuypers, M. M. M., et al. (2010). Nitrite-driven anaerobic methane oxidation by oxygenic bacteria. Nature 464, 543–548. doi: 10.1038/nature08883
Eugster, O., and Gruber, N. (2012). A probabilistic estimate of global marine N-fixation and denitrification. Global Biogeochem. Cycles 26:GB4013. doi: 10.1029/2012GB004300
Eyre, B. D., Maher, D. T., and Squire, P. (2013). Quantity and quality of organic matter (detritus) drives N2 effluxes (net denitrification) across seasons, benthic habitats, and estuaries. Global Biogeochem. Cycles 27, 1083–1095. doi: 10.1002/2013GB004631
Falk, S., Hannig, M., Braker, G., Wardenga, R., Köster, M., Jürgens, K., et al. (2006). nirS-containing denitrifier communities in the water column and sediment of the Baltic Sea. Biogeosci. Discuss. 3, 697–727. doi: 10.5194/bgd-3-697-2006
Falkowski, P. G. (1997). Evolution of the nitrogen cycle and its influence on the biological sequestration of CO2 in the ocean. Nature 387, 272–275. doi: 10.1038/387272a0
Fan, H., Bolhuis, H., and Stal, L. J. (2015a). Drivers of the dynamics of diazotrophs and denitrifiers in North Sea bottom waters and sediments. Front. Microbiol. 6:738. doi: 10.3389/fmicb.2015.00738
Fan, H., Bolhuis, H., and Stal, L. J. (2015b). Nitrification and nitrifying bacteria in a coastal microbial mat. Front. Microbiol. 6:1367. doi: 10.3389/fmicb.2015.01367
Farías, L., Castro-González, M., Cornejo, M., Charpentier, J., Faúndez, J., Boontanon, N., et al. (2009). Denitrification and nitrous oxide cycling within the upper oxycline of the Eastern Tropical South Pacific oxygen minimum zone. Limnol. Oceanogr. 54, 132–144. doi: 10.4319/lo.2009.54.1.0132
Farnelid, H., Andersson, A. F., Bertilsson, S., Al-Soud, W. A., Hansen, L. H., Sørensen, S., et al. (2011). Nitrogenase gene amplicons from global marine surface waters are dominated by genes of non-cyanobacteria. PLoS One 6:e19223. doi: 10.1371/journal.pone.0019223
Farnelid, H., Bentzon-Tilia, M., Andersson, A. F., Bertilsson, S., Jost, G., Labrenz, M., et al. (2013). Active nitrogen-fixing heterotrophic bacteria at and below the chemocline of the central Baltic Sea. ISME J. 7, 1413–1423. doi: 10.1038/ismej.2013.26
Farnelid, H., Turk-Kubo, K., Muñoz-Marín, M., and Zehr, J. (2016). New insights into the ecology of the globally significant uncultured nitrogen-fixing symbiont UCYN-A. Aquat. Microb. Ecol. 77, 125–138. doi: 10.3354/ame01794
Farnelid, H., Turk-Kubo, K., Ploug, H., Ossolinski, J. E., Collins, J. R., Van Mooy, B. A. S., et al. (2019). Diverse diazotrophs are present on sinking particles in the North Pacific subtropical gyre. ISME J. 13, 170–182. doi: 10.1038/s41396-018-0259-x
Fernandez, C., Farías, L., and Ulloa, O. (2011). Nitrogen fixation in denitrified marine waters. PLoS One 6:e20539. doi: 10.1371/journal.pone.0020539
Fernandez, C., González, M. L., Muñoz, C., Molina, V., and Farias, L. (2015). Temporal and spatial variability of biological nitrogen fixation off the upwelling system of central Chile (35-38.5°S). J. Geophys. Res. Ocean. 120, 3330–3349. doi: 10.1002/2014JC010410
Fernández-Méndez, M., Turk-Kubo, K. A., Buttigieg, P. L., Rapp, J. Z., Krumpen, T., Zehr, J. P., et al. (2016). Diazotroph diversity in the sea ice, melt ponds, and surface waters of the Eurasian Basin of the central Arctic Ocean. Front. Microbiol. 7:1884. doi: 10.3389/fmicb.2016.01884
Finzi-Hart, J. A., Pett-Ridge, J., Weber, P. K., Popa, R., Fallon, S. J., Gunderson, T., et al. (2009). Fixation and fate of C and N in the cyanobacterium Trichodesmium using nanometer-scale secondary ion mass spectrometry. Proc. Natl. Acad. Sci.U.S.A. 106, 6345–6350. doi: 10.1073/pnas.0810547106
Foster, R. A., Subramaniam, A., and Zehr, J. P. (2009). Distribution and activity of diazotrophs in the Eastern Equatorial Atlantic. Environ. Microbiol. 11, 741–750. doi: 10.1111/j.1462-2920.2008.01796.x
Foster, R. A., Subramaniam, A., Mahaffey, C., Carpenter, E. J., Capone, D. G., and Zehr, J. P. (2007). Influence of the Amazon River plume on distributions of free-living and symbiotic cyanobacteria in the western tropical north Atlantic Ocean. Limnol. Oceanogr. 52, 517–532. doi: 10.4319/lo.2007.52.2.0517
Frame, C. H., and Casciotti, K. L. (2010). Biogeochemical controls and isotopic signatures of nitrous oxide production by a marine ammonia-oxidizing bacterium. Biogeosciences 7, 2695–2709. doi: 10.5194/bg-7-2695-2010
Francis, C. A., O’Mullan, G. D., and Ward, B. B. (2003). Diversity of ammonia monooxygenase (amoA) genes across environmental gradients in Chesapeake Bay sediments. Geobiology 1, 129–140. doi: 10.1046/j.1472-4669.2003.00010.x
Francis, C. A., O’Mullan, G. D., Cornwell, J. C., and Ward, B. B. (2013). Transitions in nirS-type denitrifier diversity, community composition, and biogeochemical activity along the Chesapeake Bay estuary. Front. Microbiol. 4:237. doi: 10.3389/fmicb.2013.00237
Francis, C. A., Roberts, K. J., Beman, J. M., Santoro, A. E., and Oakley, B. B. (2005). Ubiquity and diversity of ammonia-oxidizing archaea in water columns and sediments of the ocean. Proc. Natl. Acad. Sci. U.S.A. 102, 14683–14688. doi: 10.1073/pnas.0506625102
Freing, A., Wallace, D. W. R., and Bange, H. W. (2012). Global oceanic production of nitrous oxide. Philos. Trans. R. Soc. B Biol. Sci. 367, 1245–1255. doi: 10.1098/rstb.2011.0360
Fuchsman, C. A., Devol, A. H., Saunders, J. K., McKay, C., and Rocap, G. (2017). Niche partitioning of the N cycling microbial community of an offshore oxygen deficient zone. Front. Microbiol. 8:2384. doi: 10.3389/fmicb.2017.02384
Fuchsman, C. A., Staley, J. T., Oakley, B. B., Kirkpatrick, J. B., and Murray, J. W. (2012). Free-living and aggregate-associated Planctomycetes in the Black Sea. FEMS Microbiol. Ecol. 80, 402–416. doi: 10.1111/j.1574-6941.2012.01306.x
Fulweiler, R. W., Nixon, S. W., Buckley, B. A., and Granger, S. L. (2007). Reversal of the net dinitrogen gas flux in coastal marine sediments. Nature 448, 180–182. doi: 10.1038/nature05963
Füssel, J., Lam, P., Lavik, G., Jensen, M. M., Holtappels, M., Günter, M., et al. (2012). Nitrite oxidation in the Namibian oxygen minimum zone. ISME J. 6, 1200–1209. doi: 10.1038/ismej.2011.178
Füssel, J., Lücker, S., Yilmaz, P., Nowka, B., van Kessel, M. A. H. J., Bourceau, P., et al. (2017). Adaptability as the key to success for the ubiquitous marine nitrite oxidizer Nitrococcus. Sci. Adv. 3:e1700807. doi: 10.1126/sciadv.1700807
Galán, A., Faúndez, J., Thamdrup, B., Santibáñez, J. F., and Farías, L. (2014). Temporal dynamics of nitrogen loss in the coastal upwelling ecosystem off central Chile: evidence of autotrophic denitrification through sulfide oxidation. Limnol. Oceanogr. 59, 1865–1878. doi: 10.4319/lo.2014.59.6.1865
Galán, A., Molina, V., Thamdrup, B., Woebken, D., Lavik, G., Kuypers, M. M. M., et al. (2009). Anammox bacteria and the anaerobic oxidation of ammonium in the oxygen minimum zone off northern Chile. Deep Sea Res. Part II Top. Stud. Oceanogr. 56, 1021–1031. doi: 10.1016/j.dsr2.2008.09.016
Ganesh, S., Bertagnolli, A. D., Bristow, L. A., Padilla, C. C., Blackwood, N., Aldunate, M., et al. (2018). Single cell genomic and transcriptomic evidence for the use of alternative nitrogen substrates by anammox bacteria. ISME J. 12, 2706–2722. doi: 10.1038/s41396-018-0223-229
Ganesh, S., Bristow, L. A., Larsen, M., Sarode, N., Thamdrup, B., and Stewart, F. J. (2015). Size-fraction partitioning of community gene transcription and nitrogen metabolism in a marine oxygen minimum zone. ISME J. 9, 2682–2696. doi: 10.1038/ismej.2015.44
Gardner, W. S., and McCarthy, M. J. (2009). Nitrogen dynamics at the sediment-water interface in shallow, sub-tropical Florida Bay: why denitrification efficiency may decrease with increased eutrophication. Biogeochemistry 95, 185–198. doi: 10.1007/s10533-009-9329-9325
Gardner, W. S., McCarthy, M. J., An, S., Sobolev, D., Sell, K. S., and Brock, D. (2006). Nitrogen fixation and dissimilatory nitrate reduction to ammonium (DNRA) support nitrogen dynamics in Texas estuaries. Limnol. Oceanogr. 51, 558–568. doi: 10.4319/lo.2006.51.1_part_2.0558
Giblin, A. E., Weston, N. B., Banta, G. T., Tucker, J., and Hopkinson, C. S. (2010). The effects of salinity on nitrogen losses from an oligohaline estuarine sediment. Estuaries Coasts 33, 1054–1068. doi: 10.1007/s12237-010-9280-9287
Glock, N., Roy, A.-S., Romero, D., Wein, T., Weissenbach, J., Revsbech, N. P., et al. (2019). Metabolic preference of nitrate over oxygen as an electron acceptor in foraminifera from the Peruvian oxygen minimum zone. Proc. Natl. Acad. Sci. U.S.A. 116, 2860–2865. doi: 10.1073/pnas.1813887116
Glock, N., Schönfeld, J., Eisenhauer, A., Hensen, C., Mallon, J., and Sommer, S. (2013). The role of benthic foraminifera in the benthic nitrogen cycle of the Peruvian oxygen minimum zone. Biogeosciences 10, 4767–4783. doi: 10.5194/bg-10-4767-2013
Glud, R. N., Grossart, H.-P., Larsen, M., Tang, K. W., Arendt, K. E., Rysgaard, S., et al. (2015). Copepod carcasses as microbial hot spots for pelagic denitrification. Limnol. Oceanogr. 60, 2026–2036. doi: 10.1002/lno.10149
Goebel, N. L., Turk, K. A., Achilles, K. M., Paerl, R., Hewson, I., Morrison, A. E., et al. (2010). Abundance and distribution of major groups of diazotrophic cyanobacteria and their potential contribution to N2 fixation in the Tropical Atlantic Ocean. Environ. Microbiol. 12, 3272–3289. doi: 10.1111/j.1462-2920.2010.02303.x
Gradoville, M. R., Bombar, D., Crump, B. C., Letelier, R. M., Zehr, J. P., and White, A. E. (2017). Diversity and activity of nitrogen-fixing communities across ocean basins. Limnol. Oceanogr. 62, 1895–1909. doi: 10.1002/lno.10542
Graf, D. R. H., Jones, C. M., and Hallin, S. (2014). Intergenomic comparisons highlight modularity of the denitrification pathway and underpin the importance of community structure for N2O emissions. PLoS One 9:e114118. doi: 10.1371/journal.pone.0114118
Granger, J., and Ward, B. B. (2003). Accumulation of nitrogen oxides in copper-limited cultures of denitrifying bacteria. Limnol. Oceanogr. 48, 313–318. doi: 10.4319/lo.2003.48.1.0313
Grosse, J., Bombar, D., Doan, H. N., Nguyen, L. N., and Voss, M. (2010). The Mekong River plume fuels nitrogen fixation and determines phytoplankton species distribution in the South China Sea during low and high discharge season. Limnol. Oceanogr. 55, 1668–1680. doi: 10.4319/lo.2010.55.4.1668
Grover, R., Ferrier-Pagès, C., Maguer, J. F., Ezzat, L., and Fine, M. (2014). Nitrogen fixation in the mucus of Red Sea corals. J. Exp. Biol. 217, 3962–3963. doi: 10.1242/jeb.111591
Gruber, N. (2008). The Marine Nitrogen Cycle: Overviews and Challenges in Nitrogen in the Marine Environment. Amsterdam: Elsevier.
Gruber, N. (2016). Elusive marine nitrogen fixation. Proc. Natl. Acad. Sci. U.S.A. 113, 4246–4248. doi: 10.1073/pnas.1603646113
Gruber, N., and Galloway, J. (2008). An Earth-system perspective of the global nitrogen cycle. Nature 451, 293–296. doi: 10.1038/nature06592
Hallam, S. J., Mincer, T. J., Schleper, C., Preston, C. M., Roberts, K., Richardson, P. M., et al. (2006). Pathways of carbon assimilation and ammonia oxidation suggested by environmental genomic analyses of marine Crenarchaeota. PLoS Biol. 4:e95. doi: 10.1371/journal.pbio.0040095
Halm, H., Lam, P., Ferdelman, T. G., Lavik, G., Dittmar, T., Laroche, J., et al. (2012). Heterotrophic organisms dominate nitrogen fixation in the South Pacific Gyre. ISME J. 6, 1238–1249. doi: 10.1038/ismej.2011.182
Hamersley, M. R., Lavik, G., Woebken, D., Rattray, J. E., Lam, P., Hopmans, E. C., et al. (2007). Anaerobic ammonium oxidation in the Peruvian oxygen minimum zone. Limnol. Ocean. 52, 923–933. doi: 10.4319/lo.2007.52.3.0923
Hamersley, M. R., Turk, K. A., Leinweber, A., Gruber, N., Zehr, J. P., Gunderson, T., et al. (2011). Nitrogen fixation within the water column associated with two hypoxic basins in the Southern California Bight. Aquat. Microb. Ecol. 63, 193–205. doi: 10.3354/ame01494
Han, P., Klümper, U., Wong, A., Li, M., Lin, J.-G. G., Quan, Z., et al. (2017). Assessment of molecular detection of anaerobic ammonium-oxidizing (anammox) bacteria in different environmental samples using PCR primers based on 16S rRNA and functional genes. Appl. Microbiol. Biotechnol. 101, 7689–7702. doi: 10.1007/s00253-017-8502-8503
Hannig, M., Braker, G., Dippner, J., and Jürgens, K. (2006). Linking denitrifier community structure and prevalent biogeochemical parameters in the pelagial of the central Baltic Proper (Baltic Sea). FEMS Microbiol. Ecol. 57, 260–271. doi: 10.1111/j.1574-6941.2006.00116.x
Harding, K., Turk-Kubo, K. A., Sipler, R. E., Mills, M. M., Bronk, D. A., and Zehr, J. P. (2018). Symbiotic unicellular cyanobacteria fix nitrogen in the Arctic Ocean. Proc. Natl. Acad. Sci. U.S.A. 115, 13371–13375. doi: 10.1073/pnas.1813658115
Harhangi, H. R., Le Roy, M., van Alen, T., Hu, B., Lan Groen, J., Kartal, B., et al. (2012). Hydrazine synthase, a unique phylomarker with which to study the presence and biodiversity of anammox bacteria. Appl. Environ. Microbiol. 78, 752–758. doi: 10.1128/AEM.07113-7111
Haroon, M. F., Hu, S., Shi, Y., Imelfort, M., Keller, J., Hugenholtz, P., et al. (2013). Anaerobic oxidation of methane coupled to nitrate reduction in a novel archaeal lineage. Nature 500, 567–570. doi: 10.1038/nature12375
He, H., Zhen, Y., Mi, T., Fu, L., and Yu, Z. (2018). Ammonia-oxidizing archaea and bacteria differentially contribute to ammonia oxidation in sediments from adjacent waters of Rushan Bay China. Front. Microbiol. 9:116. doi: 10.3389/fmicb.2018.00116
Heiss, E. M., and Fulweiler, R. W. (2016). Coastal water column ammonium and nitrite oxidation are decoupled in summer. Estuar. Coast. Shelf Sci. 178, 110–119. doi: 10.1016/j.ecss.2016.06.002
Henke, B. A., Turk-Kubo, K. A., Bonnet, S., and Zehr, J. P. (2018). Distributions and abundances of sublineages of the N2-fixing cyanobacterium Candidatus Atelocyanobacterium thalassa (UCYN-A) in the new caledonian coral lagoon. Front. Microbiol. 9:554. doi: 10.3389/fmicb.2018.00554
Hirsch, M. D., Long, Z. T., and Song, B. (2011). Anammox bacterial diversity in various aquatic ecosystems based on the detection of hydrazine oxidase genes (hzoA/hzoB). Microb. Ecol. 61, 264–276. doi: 10.1007/s00248-010-9743-9741
Hoffmann, F., Radax, R., Woebken, D., Holtappels, M., Lavik, G., Rapp, H. T., et al. (2009). Complex nitrogen cycling in the sponge Geodia barretti. Environ. Microbiol. 11, 2228–2243. doi: 10.1111/j.1462-2920.2009.01944.x
Holl, C. M., Waite, A. M., Pesant, S., Thompson, P. A., and Montoya, J. P. (2007). Unicellular diazotrophy as a source of nitrogen to leeuwin current coastal eddies. Deep Sea Res. Part II Top. Stud. Oceanogr. 54, 1045–1054. doi: 10.1016/j.dsr2.2007.02.002
Hong, Y. G., Li, M., Cao, H., and Gu, J. D. (2011a). Residence of habitat-specific anammox bacteria in the deep-sea subsurface sediments of the South China Sea: analyses of marker gene abundance with physical chemical parameters. Microb. Ecol. 62, 36–47. doi: 10.1007/s00248-011-9849-9840
Hong, Y. G., Yin, B., and Zheng, T. L. (2011b). Diversity and abundance of anammox bacterial community in the deep-ocean surface sediment from Equatorial Pacific. Appl. Microbiol. Biotechnol. 89, 1233–1241. doi: 10.1007/s00253-010-2925-2924
Horak, R. E. A., Qin, W., Bertagnolli, A. D., Nelson, A., Heal, K. R., Han, H., et al. (2018). Relative impacts of light, temperature, and reactive oxygen on thaumarchaeal ammonia oxidation in the North Pacific Ocean. Limnol. Oceanogr. 63, 741–757. doi: 10.1002/lno.10665
Horak, R. E. A., Qin, W., Schauer, A. J., Armbrust, E. V., Ingalls, A. E., Moffett, J. W., et al. (2013). Ammonia oxidation kinetics and temperature sensitivity of a natural marine community dominated by archaea. ISME J. 7, 2023–2033. doi: 10.1038/ismej.2013.75
Hou, L., Xie, X., Wan, X., Kao, S.-J., Jiao, N., and Zhang, Y. (2018). Niche differentiation of ammonia and nitrite oxidizers along a salinity gradient from the Pearl River Estuary to the South China Sea. Biogeosciences 15, 5169–5187. doi: 10.5194/bg-15-5169-2018
Hou, L., Zheng, Y., Liu, M., Gong, J., Zhang, X., Yin, G., et al. (2013). Anaerobic ammonium oxidation (anammox) bacterial diversity, abundance, and activity in marsh sediments of the Yangtze Estuary. J. Geophys. Res. Biogeosci. 118, 1237–1246. doi: 10.1002/jgrg.20108
Howarth, R. W., Marino, R., Lane, J., and Cole, J. J. (1988). Nitrogen fixation in freshwater, estuarine, and marine ecosystems. 1. Rates and importance. Limnol. Oceanogr. 33, 669–687. doi: 10.4319/lo.1988.33.4part2.0669
Hutchins, D. A., and Fu, F. X. (2017). Microorganisms and ocean global change. Nat. Microbiol. 2:17058. doi: 10.1038/nmicrobiol.2017.58
Hutchins, D. A., Fu, F.-X., Webb, E. A., Walworth, N., and Tagliabue, A. (2013). Taxon-specific response of marine nitrogen fixers to elevated carbon dioxide concentrations. Nat. Geosci. 6, 790–795. doi: 10.1038/NGEO1858
Hutchins, D. A., Walworth, N. G., Webb, E. A., Saito, M. A., Moran, D., McIlvin, M. R., et al. (2015). Irreversibly increased nitrogen fixation in Trichodesmium experimentally adapted to elevated carbon dioxide. Nat. Commun. 6:8155. doi: 10.1038/ncomms9155
Hutchins, D., Mulholland, M., and Fu, F. (2009). Nutrient cycles and marine microbes in a CO2-enriched ocean. Oceanography 22, 128–145. doi: 10.5670/oceanog.2009.103
Jaeschke, A., Hopmans, E. C., Wakeham, S. G., Schouten, S., and Sinninghe Damsté, J. S. (2007). The presence of ladderane lipids in the oxygen minimum zone of the Arabian Sea indicates nitrogen loss through anammox. Limnol. Oceanogr. 52, 780–786. doi: 10.4319/lo.2007.52.2.0780
Jayakumar, A., Al-Rshaidat, M. M. D., Ward, B. B., and Mulholland, M. R. (2012). Diversity, distribution, and expression of diazotroph nifH genes in oxygen-deficient waters of the Arabian Sea. FEMS Microbiol. Ecol. 82, 597–606. doi: 10.1111/j.1574-6941.2012.01430.x
Jayakumar, A., Balachandran, D., Rees, A. P., Kearns, P. J., Bowen, J. L., and Ward, B. B. (2018). Community composition of nitrous oxide reducing bacteria investigated using a functional gene microarray. Deep Sea Res. Part II Top. Stud. Oceanogr. 156, 44–50. doi: 10.1016/j.dsr2.2018.10.002
Jayakumar, A., O’Mullan, G. D., Naqvi, S. W. A. A., and Ward, B. B. (2009). Denitrifying bacterial community composition changes associated with stages of denitrification in oxygen minimum zones. Microb. Ecol. 58, 350–362. doi: 10.1007/s00248-009-9487-y
Jayakumar, D., and Ward, B. B. (2013). Community composition of bacteria involved in fixed nitrogen loss in the water column of two major oxygen minimum zones in the ocean. Aquat. Microb. Ecol. 70, 245–259. doi: 10.3354/ame01654
Jayakumar, D., Francis, C. A., Naqvi, S. W. A., and Ward, B. B. (2004). Diversity of nitrite reductase genes (nirS) in the denitrifying water column of the coastal Arabian Sea. Aquat. Microb. Ecol. 34, 69–78. doi: 10.3354/ame034069
Jensen, M. M., Lam, P., Revsbech, N. P., Nagel, B., Gaye, B., Jetten, M. S. M. S., et al. (2011). Intensive nitrogen loss over the Omani Shelf due to anammox coupled with dissimilatory nitrite reduction to ammonium. ISME J. 5, 1660–1670. doi: 10.1038/ismej.2011.44
Jetten, M. S. M., Niftrik, L., van, Strous, M., Kartal, B., Keltjens, J. T., et al. (2009). Biochemistry and molecular biology of anammox bacteria. Crit. Rev. Biochem. Mol. Biol. 44, 65–84. doi: 10.1080/10409230902722783
Ji, Q., Babbin, A. R., Jayakumar, A., Oleynik, S., and Ward, B. B. (2015). Nitrous oxide production by nitrification and denitrification in the Eastern Tropical South Pacific oxygen minimum zone. Geophys. Res. Lett. 42:764. doi: 10.1002/2015GL066853
Jickells, T. D., Buitenhuis, E., Altieri, K., Baker, A. R., Capone, D., Duce, R. A., et al. (2017). A reevaluation of the magnitude and impacts of anthropogenic atmospheric nitrogen inputs on the ocean. Global Biogeochem. Cycles 31, 289–305. doi: 10.1002/2016GB005586
Jin, T., Zhang, T., Ye, L., Lee, O. O., Wong, Y. H., and Qian, P. Y. (2011). Diversity and quantity of ammonia-oxidizing archaea and bacteria in sediment of the Pearl River Estuary, China. Appl. Microbiol. Biotechnol. 90, 1137–1145. doi: 10.1007/s00253-011-3107-3108
Jones, C. M., and Hallin, S. (2010). Ecological and evolutionary factors underlying global and local assembly of denitrifier communities. ISME J. 4, 633–641. doi: 10.1038/ismej.2009.152
Jones, C. M., Graf, D. R., Bru, D., Philippot, L., and Hallin, S. (2013). The unaccounted yet abundant nitrous oxide-reducing microbial community: a potential nitrous oxide sink. ISME J. 7, 417–426. doi: 10.1038/ismej.2012.125
Jones, C. M., Stres, B., Rosenquist, M., and Hallin, S. (2008). Phylogenetic analysis of nitrite, nitric oxide, and nitrous oxide respiratory enzymes reveal a complex evolutionary history for denitrification. Mol. Biol. Evol. 25, 1955–1966. doi: 10.1093/molbev/msn146
Kalvelage, T., Jensen, M. M., Contreras, S., Revsbech, N. P., Lam, P., Günter, M., et al. (2011). Oxygen sensitivity of anammox and coupled N-cycle processes in oxygen minimum zones. PLoS One 6:e29299. doi: 10.1371/journal.pone.0029299
Kalvelage, T., Lavik, G., Lam, P., Contreras, S., Arteaga, L., Loscher, C. R., et al. (2013). Nitrogen cycling driven by organic matter export in the South Pacific oxygen minimum zone. Nat. Geosci. 6, 228–234. doi: 10.1038/ngeo1739
Kamp, A., de Beer, D., Nitsch, J. L., Lavik, G., and Stief, P. (2011). Diatoms respire nitrate to survive dark and anoxic conditions. Proc. Natl. Acad. Sci. U.S.A. 108, 5649–5654. doi: 10.1073/pnas.1015744108
Karl, D., Michaels, A., Bergman, B., Capone, D., Carpenter, E., Letelier, R., et al. (2002). Dinitrogen fixation in the world’s oceans. Biogeochemistry 57, 47–98. doi: 10.1007/978-94-017-3405-9-2
Kartal, B., Kuypers, M. M. M. M., Lavik, G., Schalk, J., Op Den, Camp, H. J. M. M., et al. (2007). Anammox bacteria disguised as denitrifiers: nitrate reduction to dinitrogen gas via nitrite and ammonium. Environ. Microbiol. 9, 635–642. doi: 10.1111/j.1462-2920.2006.01183.x
Kartal, B., Maalcke, W. J., de Almeida, N. M., Cirpus, I., Gloerich, J., Geerts, W., et al. (2011). Molecular mechanism of anaerobic ammonium oxidation. Nature 479, 127–130. doi: 10.1038/nature10453
Kartal, B., van Niftrik, L., Keltjens, J. T., Op, den Camp, H. J. M., and Jetten, M. S. M. (2012). Anammox–growth physiology, cell biology, and metabolism. Adv. Microb. Physiol. 60, 211–262. doi: 10.1016/B978-0-12-398264-3.00003-6
Keeling, R. F., Körtzinger, A., and Gruber, N. (2010). Ocean deoxygenation in a warming world. Ann. Rev. Mar. Sci. 2, 199–229. doi: 10.1146/annurev.marine.010908.163855
Kim, O.-S., Imhoff, J. F., Witzel, K.-P., and Junier, P. (2011). Distribution of denitrifying bacterial communities in the stratified water column and sediment–water interface in two freshwater lakes and the Baltic Sea. Aquat. Ecol. 45, 99–112. doi: 10.1007/s10452-010-9335-9337
Kitidis, V., Laverock, B., McNeill, L. C., Beesley, A., Cummings, D., Tait, K., et al. (2011). Impact of ocean acidification on benthic and water column ammonia oxidation. Geophys. Res. Lett. 38:L21603. doi: 10.1029/2011GL049095
Kits, D. K., Sedlacek, C. J., Lebedeva, E. V., Han, P., Bulaev, A., Pjevac, P., et al. (2017). Kinetic analysis of a complete nitrifier reveals an oligotrophic lifestyle. Nature 549, 269–272. doi: 10.1038/nature23679
Knapp, A. N. (2012). The sensitivity of marine N2 fixation to dissolved inorganic nitrogen. Front. Microbiol. 3:374. doi: 10.3389/fmicb.2012.00374
Knapp, A. N., Casciotti, K. L., Berelson, W. M., Prokopenko, M. G., and Capone, D. G. (2016). Low rates of nitrogen fixation in Eastern Tropical South Pacific surface waters. Proc. Natl. Acad. Sci. U.S.A. 113, 4398–4403. doi: 10.1073/pnas.1515641113
Knapp, A. N., Dekaezemacker, J., Bonnet, S., Sohm, J. A., and Capone, D. G. (2012). Sensitivity of Trichodesmium erythraeum and Crocosphaera watsonii abundance and N2 fixation rates to varying NO3– and PO43– concentrations in batch cultures. Aquat. Microb. Ecol. 66, 223–236. doi: 10.3354/ame01577
Koch, H., Lücker, S., Albertsen, M., Kitzinger, K., Herbold, C., Spieck, E., et al. (2015). Expanded metabolic versatility of ubiquitous nitrite-oxidizing bacteria from the genus Nitrospira. Proc. Natl. Acad. Sci. U.S.A. 112, 11371–11376. doi: 10.1073/pnas.1506533112
Kock, A., Arévalo-Martínez, D. L., Löscher, C. R., and Bange, H. W. (2016). Extreme N2O accumulation in the coastal oxygen minimum zone off Peru. Biogeosciences 13, 827–840. doi: 10.5194/bg-13-827-2016
Koeve, W., and Kähler, P. (2010). Heterotrophic denitrification vs. autotrophic anammox – quantifying collateral effects on the oceanic carbon cycle. Biogeosciences 7, 2327–2337. doi: 10.5194/bg-7-2327-2010
Kong, L., Jing, H., Kataoka, T., Buchwald, C., and Liu, H. (2013). Diversity and spatial distribution of hydrazine oxidoreductase (hzo) gene in the oxygen minimum zone off Costa Rica. PLoS One 8:e78275. doi: 10.1371/journal.pone.0078275
Könneke, M., Bernhard, A. E., de la Torre, J. R., Walker, C. B., Waterbury, J. B., and Stahl, D. A. (2005). Isolation of an autotrophic ammonia-oxidizing marine archaeon. Nature 437, 543–546. doi: 10.1038/nature03911
Kozlowski, J. A., Stieglmeier, M., Schleper, C., Klotz, M. G., and Stein, L. Y. (2016). Pathways and key intermediates required for obligate aerobic ammonia-dependent chemolithotrophy in bacteria and Thaumarchaeota. ISME J. 10, 1836–1845. doi: 10.1038/ismej.2016.2
Kraft, B., Tegetmeyer, H. E., Sharma, R., Klotz, M. G., Ferdelman, T. G., Hettich, R. L., et al. (2014). The environmental controls that govern the end product of bacterial nitrate respiration. Science 345, 676–679. doi: 10.1126/science.1254070
Kumar, P. K., Singh, A., Ramesh, R., and Nallathambi, T. (2017). N2 fixation in the eastern Arabian Sea: probable role of heterotrophic diazotrophs. Front. Mar. Sci. 4:80. doi: 10.3389/fmars.2017.00080
Kustka, A., Carpenter, E. J., and Sañudo-Wilhelmy, S. A. (2002). Iron and marine nitrogen fixation: progress and future directions. Res. Microbiol. 153, 255–262. doi: 10.1016/S0923-2508(02)01325-1326
Kuypers, M. M. M., Lavik, G., Woebken, D., Schmid, M., Fuchs, B. M., Amann, R., et al. (2005). Massive nitrogen loss from the Benguela upwelling system through anaerobic ammonium oxidation. Proc. Natl. Acad. Sci. U.S.A. 102, 6478–6483. doi: 10.1073/pnas.0502088102
Kuypers, M. M. M., Marchant, H. K., and Kartal, B. (2018). The microbial nitrogen-cycling network. Nat. Rev. Microbiol. 16, 263–276. doi: 10.1038/nrmicro.2018.9
Kuypers, M. M. M., Sliekers, A. O., Lavik, G., Schmid, M., Jørgensen, B. B., Kuenen, J. G., et al. (2003). Anaerobic ammonium oxidation by anammox bacteria in the Black Sea. Nature 422, 608–611. doi: 10.1038/nature01472
Lam, P., and Kuypers, M. M. M. (2011). Microbial nitrogen cycling processes in oxygen minimum zones. Ann. Rev. Mar. Sci. 3, 317–345. doi: 10.1146/annurev-marine-120709-142814
Lam, P., Jensen, M. M., Kock, A., Lettmann, K. A., Plancherel, Y., Lavik, G., et al. (2011). Origin and fate of the secondary nitrite maximum in the Arabian Sea. Biogeosciences 8, 1565–1577. doi: 10.5194/bg-8-1565-2011
Lam, P., Jensen, M. M., Lavik, G., McGinnis, D. F., Muller, B., Schubert, C. J., et al. (2007). Linking crenarchaeal and bacterial nitrification to anammox in the Black Sea. Proc. Natl. Acad. Sci. U.S.A. 104, 7104–7109. doi: 10.1073/pnas.0611081104
Lam, P., Lavik, G., Jensen, M. M., van de Vossenberg, J., Schmid, M., Woebken, D., et al. (2009). Revising the nitrogen cycle in the peruvian oxygen minimum zone. Proc. Natl. Acad. Sci. U.S.A. 106, 4752–4757. doi: 10.1073/pnas.0812444106
Lancaster, K. M., Caranto, J. D., Majer, S. H., and Smith, M. A. (2018). Alternative bioenergy: updates to and challenges in nitrification metalloenzymology. Joule 2, 421–441. doi: 10.1016/j.joule.2018.01.018
Landolfi, A., Kähler, P., Koeve, W., and Oschlies, A. (2018). Global marine N2 fixation estimates: from observations to models. Front. Microbiol. 9:2112. doi: 10.3389/fmicb.2018.02112
Langlois, R. J., Hummer, D., and LaRoche, J. (2008). Abundances and distributions of the dominant nifH phylotypes in the Northern Atlantic Ocean. Appl. Environ. Microbiol. 74, 1922–1931. doi: 10.1128/AEM.01720-1727
Langlois, R., Großkopf, T., Mills, M., Takeda, S., and LaRoche, J. (2015). Widespread distribution and expression of Gamma A (UMB), an uncultured, diazotrophic, γ-proteobacterial nifH phylotype. PLoS One 10:e0128912. doi: 10.1371/journal.pone.0128912
Law, C. S., Breitbarth, E., Hoffmann, L. J., McGraw, C. M., Langlois, R. J., LaRoche, J., et al. (2012). No stimulation of nitrogen fixation by non-filamentous diazotrophs under elevated CO2 in the south Pacific. Glob. Chang. Biol. 18, 3004–3014. doi: 10.1111/j.1365-2486.2012.02777.x
Lee, J. A., and Francis, C. A. (2017). Deep nirS amplicon sequencing of san francisco bay sediments enables prediction of geography and environmental conditions from denitrifying community composition. Environ. Microbiol. 19, 4897–4912. doi: 10.1111/1462-2920.13920
Lee, R. Y., Seitzinger, S., and Mayorga, E. (2016). Land-based nutrient loading to LMEs: a global watershed perspective on magnitudes and sources. Environm. Develop. 17, 220–229. doi: 10.1016/j.envdev.2015.09.006
Lema, K. A., Willis, B. L., and Bourneb, D. G. (2012). Corals form characteristic associations with symbiotic nitrogen-fixing bacteria. Appl. Environ. Microbiol. 78, 3136–3144. doi: 10.1128/AEM.07800-7811
Levipan, H. A., Molina, V., and Fernandez, C. (2014). Nitrospina-like bacteria are the main drivers of nitrite oxidation in the seasonal upwelling area of the Eastern South Pacific (Central Chile 36°S). Environ. Microbiol. Rep. 6, 565–573. doi: 10.1111/1758-2229.12158
Li, J., Nedwell, D. B., Beddow, J., Dumbrell, A. J., McKew, B. A., Thorpe, E. L., et al. (2015). amoA gene abundances and nitrification potential rates suggest that benthic ammonia-oxidizing bacteria and not archaea dominate N cycling in the colne estuary. U. K. Appl. Environ. Microbiol. 81, 159–165. doi: 10.1128/AEM.02654-2614
Li, M., Cao, H., Hong, Y.-G., and Gu, J.-D. (2011). Seasonal dynamics of anammox bacteria in estuarial sediment of the Mai Po nature reserve revealed by analyzing the 16S rRNA and hydrazine oxidoreductase (hzo) genes. Microb. Environ. 26, 15–22. doi: 10.1264/jsme2.ME10131
Li-dong, S., Qun, Z., Shuai, L., Ping, D., Jiang-ning, Z., Dong-qing, C., et al. (2014). Molecular evidence for nitrite-dependent anaerobic methane-oxidising bacteria in the Jiaojiang Estuary of the East Sea (China). Appl. Microbiol. Biotechnol. 98, 5029–5038. doi: 10.1007/s00253-014-5556-5553
Lipsewers, Y. A., Bale, N. J., Hopmans, E. C., Schouten, S., Sinninghe Damsté, J. S., and Villanueva, L. (2014). Seasonality and depth distribution of the abundance and activity of ammonia oxidizing microorganisms in marine coastal sediments (North Sea). Front. Microbiol. 5:472. doi: 10.3389/fmicb.2014.00472
Lisa, J. A., Song, B., Tobias, C. R., and Hines, D. E. (2015). Genetic and biogeochemical investigation of sedimentary nitrogen cycling communities responding to tidal and seasonal dynamics in cape fear river estuary. Estuar. Coast. Shelf Sci. 167, A313–A323. doi: 10.1016/j.ecss.2015.09.008
Lisa, J., Song, B., Tobias, C., and Duernberger, K. (2014). Impacts of freshwater flushing on anammox community structure and activities in the New River Estuary. U.S.A. Aquat. Microb. Ecol. 72, 17–31. doi: 10.3354/ame01682
Liu, X., Tiquia, S. M., Holguin, G., Wu, L., Nold, S. C., Devol, A. H., et al. (2003). Molecular diversity of denitrifying genes in continental margin sediments within the oxygen-deficient zone off the Pacific coast of Mexico. Appl. Environ. Microbiol. 69, 3549–3560. doi: 10.1128/AEM.69.6.3549-3560.2003
Loescher, C. R., Großkopf, T., Desai, F. D., Gill, D., Schunck, H., Croot, P. L., et al. (2014). Facets of diazotrophy in the oxygen minimum zone waters off Peru. ISME J. 8, 2180–2192. doi: 10.1038/ismej.2014.71
Lomas, M., Hopkinson, B., Losh, J., Ryan, D., Shi, D., Xu, Y., et al. (2012). Effect of ocean acidification on cyanobacteria in the Subtropical North Atlantic. Aquat. Microb. Ecol. 66, 211–222. doi: 10.3354/ame01576
Löscher, C. R., Kock, A., Könneke, M., Laroche, J., Bange, H. W., and Schmitz, R. A. (2012). Production of oceanic nitrous oxide by ammonia-oxidizing archaea. Biogeosciences 9, 2419–2429. doi: 10.5194/bg-9-2419-2012
Lücker, S., Wagner, M., Maixner, F., Pelletier, E., Koch, H., and Vacherie, B. (2010). A Nitrospira metagenome illuminates the physiology and evolution of globally important nitrite-oxidizing bacteria. Proc. Natl. Acad. Sci. U.S.A. 107, 13479–13484. doi: 10.1073/pnas.1003860107
Lüke, C., Speth, D. R., Kox, M. A. R., Villanueva, L., and Jetten, M. S. M. (2016). Metagenomic analysis of nitrogen and methane cycling in the Arabian Sea oxygen minimum zone. PeerJ 4:e1924. doi: 10.7717/peerj.1924
Luo, H., Tolar, B. B., Swan, B. K., Zhang, C. L., Stepanauskas, R., Moran, A. M., et al. (2014). Single-cell genomics shedding light on marine Thaumarchaeota diversification. ISME J. 8, 732–736. doi: 10.1038/ismej.2013.202
Luo, Y.-W., Doney, S. C., Anderson, L. A., Benavides, M., Berman-Frank, I., Bode, A., et al. (2012). Database of diazotrophs in global ocean: abundance, biomass and nitrogen fixation rates. Earth Syst. Sci. Data 4, 47–73. doi: 10.5194/essd-4-47-2012
Luo, Z.-H., Xu, W., Li, M., Gu, J.-D., and Zhong, T.-H. (2015). Spatial distribution and abundance of ammonia-oxidizing microorganisms in deep-sea sediments of the Pacific Ocean. Antonie Van Leeuwenhoek 108, 329–342. doi: 10.1007/s10482-015-0485-484
Magalhães, C. M., Machado, A., Matos, P., and Bordalo, A. A. (2011). Impact of copper on the diversity, abundance and transcription of nitrite and nitrous oxide reductase genes in an urban European estuary. FEMS Microbiol. Ecol. 77, 274–284. doi: 10.1111/j.1574-6941.2011.01107.x
Martens-Habbena, W., Berube, P. M., Urakawa, H., de la Torre, J. R., and Stahl, D. A. (2009). Ammonia oxidation kinetics determine niche separation of nitrifying archaea and bacteria. Nature 461, 976–979. doi: 10.1038/nature08465
Martínez-García, M., Stief, P., Díaz-Valdés, M., Wanner, G., Ramos-Esplá, A., Dubilier, N., et al. (2008). Ammonia-oxidizing crenarchaeota and nitrification inside the tissue of a colonial ascidian. Environ. Microbiol. 10, 2991–3001. doi: 10.1111/j.1462-2920.2008.01761.x
Martínez-Pérez, C., Mohr, W., Löscher, C. R., Dekaezemacker, J., Littmann, S., Yilmaz, P., et al. (2016). The small unicellular diazotrophic symbiont. UCYN-A, is a key player in the marine nitrogen cycle. Nat. Microbiol. 1:16163. doi: 10.1038/nmicrobiol.2016.163
Martinez-Rey, J., Bopp, L., Gehlen, M., Tagliabue, A., and Gruber, N. (2015). Projections of oceanic N2O emissions in the 21st century using the IPSL earth system model. Biogeosciences 12, 4133–4148. doi: 10.5194/bg-12-4133-2015
Martiny, A. C., Kathuria, S., and Berube, P. M. (2009). Widespread metabolic potential for nitrite and nitrate assimilation among Prochlorococcus ecotypes. Proc. Natl. Acad. Sci. U.S.A. 106, 10787–10792. doi: 10.1073/pnas.0902532106
Mehta, M. P., and Baross, J. A. (2006). Nitrogen fixation at 92°C by a hydrothermal vent archaeon. Science 314, 1783–1786. doi: 10.1126/science.1134772
Mehta, M. P., Butterfield, D. A., and Baross, J. A. (2003). Phylogenetic diversity of nitrogenase (nifH) genes in deep-sea and hydrothermal vent environments of the Juan de Fuca Ridge. Appl. Environ. Microbiol. 69, 960–970. doi: 10.1128/AEM.69.2.960-970.2003
Merbt, S. N., Stahl, D. A., Casamayor, E. O., Martí, E., Nicol, G. W., and Prosser, J. I. (2012). Differential photoinhibition of bacterial and archaeal ammonia oxidation. FEMS Microbiol. Lett. 327, 41–46. doi: 10.1111/j.1574-6968.2011.02457.x
Messer, L. F., Mahaffey, C., Robinson, C., Jeffries, T. C., Baker, K. G., Bibiloni Isaksson, J., et al. (2016). High levels of heterogeneity in diazotroph diversity and activity within a putative hotspot for marine nitrogen fixation. ISME J. 10, 1499–1513. doi: 10.1038/ismej.2015.205
Messer, L., Doubell, M., Jeffries, T., Brown, M., and Seymour, J. (2015). Prokaryotic and diazotrophic population dynamics within a large oligotrophic inverse estuary. Aquat. Microb. Ecol. 74, 1–15. doi: 10.3354/ame01726
Milligan, A. J., Berman-Frank, I., Gerchman, Y., Dismukes, G. C., and Falkowski, P. G. (2007). Light-dependent oxygen consumption in nitrogen-fixing cyanobacteria plays a key role in nitrogenase protection 1. J. Phycol. 43, 845–852. doi: 10.1111/j.1529-8817.2007.00395.x
Mills, M. M., Ridame, C., Davey, M., La Roche, J., and Geider, R. J. (2004). Iron and phosphorus co-limit nitrogen fixation in the Eastern Tropical North Atlantic. Nature 429, 292–294. doi: 10.1038/nature02550
Mincer, T. J., Church, M. J., Taylor, L. T., Preston, C., Karl, D. M., and DeLong, E. F. (2007). Quantitative distribution of presumptive archaeal and bacterial nitrifiers in Monterey Bay and the North Pacific Subtropical Gyre. Environ. Microbiol. 9, 1162–1175. doi: 10.1111/j.1462-2920.2007.01239.x
Mohamed, N. M., Saito, K., Tal, Y., and Hill, R. T. (2010). Diversity of aerobic and anaerobic ammonia-oxidizing bacteria in marine sponges. ISME J. 4, 38–48. doi: 10.1038/ismej.2009.84
Moisander, P. H., Beinart, R. A., Hewson, I., White, A. E., Johnson, K. S., Carlson, C. A., et al. (2010). Unicellular cyanobacterial distributions broaden the oceanic N2 fixation domain. Science 327, 1512–1514. doi: 10.1126/science.1185468
Moisander, P. H., Benavides, M., Bonnet, S., Berman-Frank, I., White, A. E., and Riemann, L. (2017). Chasing after non-cyanobacterial nitrogen fixation in marine pelagic environments. Front. Microbiol. 8:1736. doi: 10.3389/fmicb.2017.01736
Moisander, P. H., Morrison, A. E., Ward, B. B., Jenkins, B. D., and Zehr, J. P. (2007a). Spatial-temporal variability in diazotroph assemblages in Chesapeake Bay using an oligonucleotide nifH microarray. Environ. Microbiol. 9, 1823–1835. doi: 10.1111/j.1462-2920.2007.01304.x
Moisander, P. H., Paerl, H., Dyble, J., and Sivonen, K. (2007b). Phosphorus limitation and diel control of nitrogen-fixing cyanobacteria in the Baltic Sea. Mar. Ecol. Prog. Ser. 345, 41–50. doi: 10.3354/meps06964
Moisander, P. H., Serros, T., Paerl, R. W., Beinart, R. A., and Zehr, J. P. (2014). Gammaproteobacterial diazotrophs and nifH gene expression in surface waters of the South Pacific Ocean. ISME J. 8, 1962–1973. doi: 10.1038/ismej.2014.49
Molina, V., Belmar, L., and Ulloa, O. (2010). High diversity of ammonia-oxidizing archaea in permanent and seasonal oxygen-deficient waters of the Eastern South Pacific. Environ. Microbiol. 12, 2450–2465. doi: 10.1111/j.1462-2920.2010.02218.x
Monteiro, F. M., Dutkiewicz, S., and Follows, M. J. (2011). Biogeographical controls on the marine nitrogen fixers. Global Biogeochem. Cycles 25:GB2003. doi: 10.1029/2010GB003902
Monteiro, F. M., Follows, M. J., and Dutkiewicz, S. (2010). Distribution of diverse nitrogen fixers in the global ocean. Global Biogeochem. Cycles 24:GB3017. doi: 10.1029/2009GB003731
Monteiro, M., Séneca, J., Torgo, L., Cleary, D., Gomes, N., Santoro, A., et al. (2017). Environmental controls on estuarine nitrifying communities along a salinity gradient. Aquat. Microb. Ecol. 80, 167–180. doi: 10.3354/ame01847
Moore, M. C., Mills, M. M., Achterberg, E. P., Geider, R. J., LaRoche, J., Lucas, M. I., et al. (2009). Large-scale distribution of Atlantic nitrogen fixation controlled by iron availability. Nat. Geosci. 2, 867–871. doi: 10.1038/ngeo667
Moreira-Coello, V., Mouriño-Carballido, B., Marañón, E., Fernández-Carrera, A., Bode, A., Sintes, E., et al. (2019). Temporal variability of diazotroph community composition in the upwelling region off NW Iberia. Sci. Rep. 9:3737. doi: 10.1038/s41598-019-39586-39584
Moreno-Vivián, C., Cabello, P., Martínez-Luque, M., Blasco, R., and Castillo, F. (1999). Prokaryotic nitrate reduction: molecular properties and functional distinction among bacterial nitrate reductases. J. Bacteriol. 181, 6573–6584.
Mosier, A. C., and Francis, C. A. (2008). Relative abundance and diversity of ammonia-oxidizing archaea and bacteria in the San Francisco Bay Estuary. Environ. Microbiol. 10, 3002–3016. doi: 10.1111/j.1462-2920.2008.01764.x
Mosier, A. C., and Francis, C. A. (2010). Denitrifier abundance and activity across the San Francisco Bay Estuary. Environ. Microbiol. Rep. 2, 667–676. doi: 10.1111/j.1758-2229.2010.00156.x
Mulholland, M. R., Bernhardt, P. W., Blanco-Garcia, J. L., Mannino, A., Hyde, K., Mondragon, E., et al. (2012). Rates of dinitrogen fixation and the abundance of diazotrophs in North American coastal waters between cape hatteras and georges Bank. Limnol. Oceanogr. 57, 1067–1083. doi: 10.4319/lo.2012.57.4.1067
Mulholland, M. R., Bernhardt, Widner, B. N., Selden, C. R., Chappell, P. D., Clayton, S., et al. (2019). High rates of N2 fixation in temperate, Western North Atlantic coastal waters expand the realm of marine diazotrophy. Global Biogeochem. Cycles 33, 826–840. doi: 10.1029/2018GB006130
Muñoz-Marín, M., del, C., Shilova, I. N., Shi, T., Farnelid, H., Cabello, A. M., et al. (2018). The transcriptional cycle is suited to daytime N2 fixation in the unicellular cyanobacterium “Candidatus Atelocyanobacterium thalassa” (UCYN-A). mBio 10:e2495-18. doi: 10.1128/mBio.02495-2418
Na, T., Thamdrup, B., Kim, B., Kim, S.-H., Vandieken, V., Kang, D.-J., et al. (2018). N2 production through denitrification and anammox across the continental margin (shelf-slope-rise) of the Ulleung Basin. East Sea. Limnol. Oceanogr. 63, S410–S424. doi: 10.1002/lno.10750
Nakagawa, T., Mori, K., Kato, C., Takahashi, R., and Tokuyama, T. (2007). Distribution of cold-adapted ammonia-oxidizing microorganisms in the deep-ocean of the northeastern Japan Sea. Microbes Environ. 22, 365–372. doi: 10.1264/jsme2.22.365
Naqvi, S. W. A., and Noronha, R. J. (1991). Nitrous oxide in the Arabian Sea. Deep Sea Res. Part A Oceanogr. Res. Pap. 38, 871–890. doi: 10.1016/0198-0149(91)90023-90029
Nevison, C. D., Lueker, T. J., and Weiss, R. F. (2004). Quantifying the nitrous oxide source from coastal upwelling. Global Biogeochem. Cycles 18:GB1018. doi: 10.1029/2003GB002110
Newell, S. E., Babbin, A. R., Jayakumar, D., Ward, B. B., Jayakumar, A., and Ward, B. B. (2011). Ammonia oxidation rates and nitrification in the Arabian Sea. Global Biogeochem. Cycles 25:GB4016. doi: 10.1029/2010GB003940
Newell, S. E., Fawcett, S. E., and Ward, B. B. (2013). Depth distribution of ammonia oxidation rates and ammonia-oxidizer community composition in the Sargasso Sea. Limnol. Oceanogr. 58, 1491–1500. doi: 10.4319/lo.2013.58.4.1491
Ngugi, D. K., Blom, J., Stepanauskas, R., and Stingl, U. (2016). Diversification and niche adaptations of Nitrospina-like bacteria in the polyextreme interfaces of Red Sea brines. ISME J. 10, 1383–1399. doi: 10.1038/ismej.2015.214
Nicholls, J., and Trimmer, M. (2009). Widespread occurrence of the anammox reaction in estuarine sediments. Aquat. Microb. Ecol. 55, 105–113. doi: 10.3354/ame01285
Nunoura, T., Nishizawa, M., Kikuchi, T., Tsubouchi, T., Hirai, M., Koide, O., et al. (2013). Molecular biological and isotopic biogeochemical prognoses of the nitrification-driven dynamic microbial nitrogen cycle in hadopelagic sediments. Environ. Microbiol. 15, 3087–3107. doi: 10.1111/1462-2920.12152
Nunoura, T., Takaki, Y., Hirai, M., Shimamura, S., Makabe, A., Koide, O., et al. (2015). Hadal biosphere: insight into the microbial ecosystem in the deepest ocean on Earth. Proc. Natl. Acad. Sci. U.S.A. 112, E1230–E1236. doi: 10.1073/pnas.1421816112
Oakley, B. B., Francis, C. A., Roberts, K. J., Fuchsman, C. A., Srinivasan, S., and Staley, J. T. (2007). Analysis of nitrite reductase (nirK and nirS) genes and cultivation reveal depauperate community of denitrifying bacteria in the Black Sea suboxic zone. Environ. Microbiol. 9, 118–130. doi: 10.1111/j.1462-2920.2006.01121.x
Olson, N. D., Ainsworth, T. D., Gates, R. D., and Takabayashi, M. (2009). Diazotrophic bacteria associated with Hawaiian Montipora corals: diversity and abundance in correlation with symbiotic dinoflagellates. J. Exp. Mar. Bio. Ecol. 371, 140–146. doi: 10.1016/j.jembe.2009.01.012
Olson, R. (1981). Differential photoinhibition of marine nitrifying bacteria: a possible mechanism for the formation of the primary nitrite maximum. J. Mar. Res. 39, 227–238.
Oren, A. (2011). Thermodynamic limits to microbial life at high salt concentrations. Environ. Microbiol. 13, 1908–1923. doi: 10.1111/j.1462-2920.2010.02365.x
Pachiadaki, M. G., Sintes, E., Bergauer, K., Brown, J. M., Record, N. R., Swan, B. K., et al. (2017). Major role of nitrite-oxidizing bacteria in dark ocean carbon fixation. Science 358, 1046–1051. doi: 10.1126/science.aan8260
Padilla, C. C., Bristow, L., Sarode, N., Garcia-Robledo, E., Gómez Ramírez, E., Benson, C. R., et al. (2016). NC10 bacteria in marine oxygen minimum zones. ISME J. 10, 2067–2071. doi: 10.1038/ismej.2015.262
Pajares, S., Merino-Ibarra, M., Macek, M., and Alcocer, J. (2017). Vertical and seasonal distribution of picoplankton and functional nitrogen genes in a high-altitude warm-monomictic tropical lake. Freshw. Biol. 62, 1180–1193. doi: 10.1111/fwb.12935
Pajares, S., Soto-Jiménez, M., and Merino-Ibarra, M. (2019). Molecular and isotopic evidence of the distribution of nitrogen-cycling microbial communities in the oxygen minimum zone of the Tropical Mexican Pacific. FEMS Microbiol. Ecol. 95:fiz143. doi: 10.1093/femsec/fiz143
Papaspyrou, S., Smith, C. J., Dong, L. F., Whitby, C., Dumbrell, A. J., and Nedwell, D. B. (2014). Nitrate reduction functional genes and nitrate reduction potentials persist in deeper estuarine sediments Why? PLoS One 9:e94111. doi: 10.1371/journal.pone.0094111
Pedneault, E., Galand, P. E., Potvin, M., Tremblay, J., and Lovejoy, C. (2015). Archaeal amoA and ureC genes and their transcriptional activity in the Arctic Ocean. Sci. Rep. 4:4661. doi: 10.1038/srep04661
Peng, X., Fuchsman, C. A., Jayakumar, A., Oleynik, S., Martens-Habbena, W., Devol, A. H., et al. (2015). Ammonia and nitrite oxidation in the Eastern Tropical North Pacific. Global Biogeochem. Cycles 29, 2034–2049. doi: 10.1002/2015GB005278
Peng, X., Fuchsman, C. A., Jayakumar, A., Warner, M. J., Devol, A. H., and Ward, B. B. (2016). Revisiting nitrification in the Eastern Tropical South Pacific: a focus on controls. J. Geophys. Res. Ocean 121, 1667–1684. doi: 10.1002/2015JC011455
Pina-Ochoa, E., Hogslund, S., Geslin, E., Cedhagen, T., Revsbech, N. P., Nielsen, L. P., et al. (2010). Widespread occurrence of nitrate storage and denitrification among Foraminifera and Gromiida. Proc. Natl. Acad. Sci. U.S.A. 107, 1148–1153. doi: 10.1073/pnas.0908440107
Pitcher, A., Villanueva, L., Hopmans, E. C., Schouten, S., Reichart, G. J., and Sinninghe Damsté, J. S. (2011a). Niche segregation of ammonia-oxidizing archaea and anammox bacteria in the Arabian Sea oxygen minimum zone. ISME J. 5, 1896–1904. doi: 10.1038/ismej.2011.60
Pitcher, A., Wuchter, C., Siedenberg, K., Schouten, S., and Sinninghe Damsté, J. S. (2011b). Crenarchaeol tracks winter blooms of ammonia-oxidizing Thaumarchaeota in the coastal North Sea. Limnol. Oceanogr. 56, 2308–2318. doi: 10.4319/lo.2011.56.6.2308
Pogoreutz, C., Rädecker, N., Cárdenas, A., Gärdes, A., Wild, C., and Voolstra, C. R. (2017). Nitrogen fixation aligns with nifH abundance and expression in two coral trophic functional groups. Front. Microbiol. 8:1187. doi: 10.3389/fmicb.2017.01187
Preisler, A., de Beer, D., Lichtschlag, A., Lavik, G., Boetius, A., and Jørgensen, B. B. (2007). Biological and chemical sulfide oxidation in a Beggiatoa inhabited marine sediment. ISME J. 1, 341–353. doi: 10.1038/ismej.2007.50
Preston, C. M., Wu, K. Y., Molinski, T. F., and DeLong, E. F. (1996). A psychrophilic crenarchaeon inhabits a marine sponge: Cenarchaeum symbiosum gen. nov., sp. nov. Proc. Natl. Acad. Sci. U.S.A. 93, 6241–6246. doi: 10.1073/pnas.93.13.6241
Purkhold, U., Pommerening-Roser, A., Juretschko, S., Schmid, M. C., Koops, H.-P., and Wagner, M. (2000). Phylogeny of all recognized species of ammonia oxidizers based on comparative 16S rRNA and amoA sequence analysis: implications for molecular diversity surveys. Appl. Environ. Microbiol. 66, 5368–5382. doi: 10.1128/AEM.66.12.5368-5382.2000
Qian, G., Wang, J., Kan, J., Zhang, X., Xia, Z., Zhang, X., et al. (2018). Diversity and distribution of anammox bacteria in water column and sediments of the eastern Indian Ocean. Int. Biodeterior. Biodegr. 133, 52–62. doi: 10.1016/j.ibiod.2018.05.015
Qin, W., Amin, S. A., Martens-Habbena, W., Walker, C. B., Urakawa, H., Devol, A. H., et al. (2014). Marine ammonia-oxidizing archaeal isolates display obligate mixotrophy and wide ecotypic variation. Proc. Natl. Acad. Sci. U.S.A. 111, 12504–12509. doi: 10.1073/pnas.1324115111
Radax, R., Hoffmann, F., Rapp, H. T., Leininger, S., and Schleper, C. (2012). Ammonia-oxidizing archaea as main drivers of nitrification in cold-water sponges. Environ. Microbiol. 14, 909–923. doi: 10.1111/j.1462-2920.2011.02661.x
Rädecker, N., Pogoreutz, C., Voolstra, C. R., Wiedenmann, J., and Wild, C. (2015). Nitrogen cycling in corals: the key to understanding holobiont functioning? Trends Microbiol. 23, 490–497. doi: 10.1016/j.tim.2015.03.008
Raghoebarsing, A. A., Pol, A., van de Pas-Schoonen, K. T., Smolders, A. J. P., Ettwig, K. F., Rijpstra, W. I. C., et al. (2006). A microbial consortium couples anaerobic methane oxidation to denitrification. Nature 440, 918–921. doi: 10.1038/nature04617
Rahav, E., Bar-Zeev, E., Ohayon, S., Elifantz, H., Belkin, N., Herut, B., et al. (2013). Dinitrogen fixation in aphotic oxygenated marine environments. Front. Microbiol. 4:227. doi: 10.3389/fmicb.2013.00227
Ramos, R., and Pajares, S. (2018). Reviews and syntheses: processes and functional genes involved in nitrogen cycling in marine environments. Biogeosci. Discuss. [Preprint]. doi: 10.5194/bg-2018-2279
Rani, S., Koh, H. W., Rhee, S. K., Fujitani, H., and Park, S. J. (2017). Detection and diversity of the nitrite oxidoreductase alpha subunit (nxrA) gene of Nitrospina in marine sediments. Microb. Ecol. 73, 111–122. doi: 10.1007/s00248-016-0897-893
Rasigraf, O., Schmitt, J., Jetten, M. S. M. M., and Lüke, C. (2017). Metagenomic potential for and diversity of N-cycle driving microorganisms in the Bothnian Sea sediment. Microbiologyopen 6:e00475. doi: 10.1002/mbo3.475
Rees, A. P., Brown, I. J., Jayakumar, A., and Ward, B. B. (2016). The inhibition of N2O production by ocean acidification in cold temperate and polar waters. Deep. Res. Part II Top. Stud. Oceanogr. 127, 93–101. doi: 10.1016/j.dsr2.2015.12.006
Rees, A. P., Turk-Kubo, K. A., Al-Moosawi, L., Alliouane, S., Gazeau, F., Hogan, M. E., et al. (2017). Ocean acidification impacts on nitrogen fixation in the coastal western Mediterranean Sea. Estuar. Coast. Shelf Sci. 186, 45–57. doi: 10.1016/j.ecss.2016.01.020
Reyes, C., Schneider, D., Lipka, M., Thürmer, A., Böttcher, M. E., and Friedrich, M. W. (2017). Nitrogen metabolism genes from temperate marine sediments. Mar. Biotechnol. 19, 175–190. doi: 10.1007/s10126-017-9741-9740
Rich, J. J., Arevalo, P., Chang, B. X., Devol, A. H., and Ward, B. B. (2018). Anaerobic ammonium oxidation (anammox) and denitrification in Peru margin sediments. J. Mar. Syst. (in press). doi: 10.1016/j.jmarsys.2018.09.007
Rich, J. J., Dale, O. R., Song, B., and Ward, B. B. (2008). Anaerobic ammonium oxidation (anammox) in Chesapeake Bay sediments. Microb. Ecol. 55, 311–320. doi: 10.1007/s00248-007-9277-9273
Riemann, L., Farnelid, H., and Steward, G. F. (2010). Nitrogenase genes in non-cyanobacterial plankton: prevalence, diversity and regulation in marine waters. Aquat. Microb. Ecol. 61, 235–247. doi: 10.3354/ame01431
Rios-Del Toro, E. E., Valenzuela, E. I., López-Lozano, N. E., Cortés-Martínez, M. G., Sánchez-Rodríguez, M. A., Calvario-Martínez, O., et al. (2018). Anaerobic ammonium oxidation linked to sulfate and ferric iron reduction fuels nitrogen loss in marine sediments. Biodegradation 29, 429–442. doi: 10.1007/s10532-018-9839-9838
Risgaard-Petersen, N., Langezaal, A. M., Ingvardsen, S., Schmid, M. C., Jetten, M. S. M., Op, et al. (2006). Evidence for complete denitrification in a benthic foraminifer. Nature 443, 93–96. doi: 10.1038/nature05070
Risgaard-Petersen, N., Meyer, R., and Revsbech, N. (2005). Denitrification and anaerobic ammonium oxidation in sediments: effects of microphytobenthos and NO3–. Aquat. Microb. Ecol. 40, 67–76. doi: 10.3354/ame040067
Risgaard-Petersen, N., Meyer, R., Schmid, M., Jetten, M., Enrich-Prast, A., Rysgaard, S., et al. (2004). Anaerobic ammonium oxidation in an estuarine sediment. Aquat. Microb. Ecol. 36, 293–304. doi: 10.3354/ame036293
Robertson, E. K., Roberts, K. L., Burdorf, L. D. W., Cook, P., and Thamdrup, B. (2016). Dissimilatory nitrate reduction to ammonium coupled to Fe(II) oxidation in sediments of a periodically hypoxic estuary. Limnol. Oceanogr. 61, 365–381. doi: 10.1002/lno.10220
Rotthauwe, J., and Witzel, K. (1997). The ammonia monooxygenase structural gene amoA as a functional marker: molecular fine-scale analysis of natural ammonia-oxidizing populations. Appl. Environ. Microbiol. 63, 4704–4712.
Rush, D., Wakeham, S. G., Hopmans, E. C., Schouten, S., and Sinninghe Damsté, J. S. (2012). Biomarker evidence for anammox in the oxygen minimum zone of the Eastern Tropical North Pacific. Org. Geochem. 53, 80–87. doi: 10.1016/j.orggeochem.2012.02.005
Russ, L., Kartal, B., op den Camp, H. J. M., Sollai, M., Le Bruchec, J., Caprais, J. C., et al. (2013). Presence and diversity of anammox bacteria in cold hydrocarbon-rich seeps and hydrothermal vent sediments of the Guaymas Basin. Front. Microbiol. 4:219. doi: 10.3389/fmicb.2013.00219
Rysgaard, S., Glud, R. N., Risgaard-Petersen, N., and Dalsgaard, T. (2004). Denitrification and anammox activity in Arctic marine sediments. Limnol. Oceanogr. 49, 1493–1502. doi: 10.4319/lo.2004.49.5.1493
Santoro, A. E., Boehm, A. B., and Francis, C. (2006). Denitrifier community composition along a nitrate and salinity gradient in a coastal aquifer. Appl. Environ. Microbiol. 72, 2102–2109. doi: 10.1128/AEM.72.3.2102-2109.2006
Santoro, A. E., Buchwald, C., McIlvin, M. R., and Casciotti, K. L. (2011). Isotopic signature of N2O produced by marine ammonia-oxidizing archaea. Science 333, 1282–1285. doi: 10.1126/science.1208239
Santoro, A. E., Casciotti, K. L., and Francis, C. A. (2010). Activity, abundance and diversity of nitrifying archaea and bacteria in the central California current. Environ. Microbiol. 12, 1989–2006. doi: 10.1111/j.1462-2920.2010.02205.x
Santoro, A. E., Saito, M. A., Goepfert, T. J., Lamborg, C. H., Dupont, C. L., and DiTullio, G. R. (2017). Thaumarchaeal ecotype distributions across the equatorial Pacific Ocean and their potential roles in nitrification and sinking flux attenuation. Limnol. Oceanogr. 62, 1984–2003. doi: 10.1002/lno.10547
Schaefer, S. C., and Hollibaugh, J. T. (2017). Temperature decouples ammonium and nitrite oxidation in coastal waters. Environ. Sci. Technol. 51, 3157–3164. doi: 10.1021/acs.est.6b03483
Schmid, M. C., Risgaard-Petersen, N., van de Vossenberg, J., Kuypers, M. M. M., Lavik, G., Petersen, J., et al. (2007). Anaerobic ammonium-oxidizing bacteria in marine environments: widespread occurrence but low diversity. Environ. Microbiol. 9, 1476–1484. doi: 10.1111/j.1462-2920.2007.01266.x
Schunck, H., Lavik, G., Desai, D. K., Großkopf, T., Kalvelage, T., Löscher, C. R., et al. (2013). Giant hydrogen sulfide plume in the oxygen minimum zone off Peru supports chemolithoautotrophy. PLoS One 8:e68661. doi: 10.1371/journal.pone.0068661
Severin, I., Acinas, S. G., and Stal, L. J. (2010). Diversity of nitrogen- fixing bacteria in cyanobacterial mats. FEMS Microbiol. Ecol. 73, 514–525. doi: 10.1111/j.1574-6941.2010.00925.x
Severin, I., Bentzon-Tilia, M., Moisander, P. H., and Riemann, L. (2015). Nitrogenase expression in estuarine bacterioplankton influenced by organic carbon and availability of oxygen. FEMS Microbiol. Lett. 362:fnv105. doi: 10.1093/femsle/fnv105
Shao, M.-F., Zhang, T., Fang, H. H.-P., and Li, X. (2011). The effect of nitrate concentration on sulfide-driven autotrophic denitrification in marine sediment. Chemosphere 83, 1–6. doi: 10.1016/j.chemosphere.2011.01.042
Shao, S., Luan, X., Dang, H., Zhou, H., Zhao, Y., Liu, H., et al. (2014). Deep-sea methane seep sediments in the Okhotsk Sea sustain diverse and abundant anammox bacteria. FEMS Microbiol. Ecol. 87, 503–516. doi: 10.1111/1574-6941.12241
Shehzad, A., Liu, J., Yu, M., Qismat, S., Liu, J., and Zhang, X.-H. (2016). Diversity, community composition and abundance of anammox bacteria in sediments of the north marginal seas of China. Microbes Environ. 31, 111–120. doi: 10.1264/jsme2.ME15140
Shen, L., Hu, B., Liu, S., Chai, X., He, Z., Ren, H., et al. (2016). Anaerobic methane oxidation coupled to nitrite reduction can be a potential methane sink in coastal environments. Appl. Microbiol. Biotechnol. 100, 7171–7180. doi: 10.1007/s00253-016-7627-7620
Shi, D., Xu, Y., Hopkinson, B. M., and Morel, F. M. M. (2010). Effect of ocean acidification on iron availability to marine phytoplankton. Science 327, 676–679. doi: 10.1126/science.1183517
Shiozaki, T., Bombar, D., Riemann, L., Hashihama, F., Takeda, S., Yamaguchi, T., et al. (2017). Basin scale variability of active diazotrophs and nitrogen fixation in the North Pacific, from the tropics to the subarctic Bering Sea. Global Biogeochem. Cycles 31, 996–1009. doi: 10.1002/2017GB005681
Shiozaki, T., Fujiwara, A., Ijichi, M., Harada, N., Nishino, S., Nishi, S., et al. (2018). Diazotroph community structure and the role of nitrogen fixation in the nitrogen cycle in the Chukchi Sea (western Arctic Ocean). Limnol. Oceanogr. 63, 2191–2205. doi: 10.1002/lno.10933
Shiozaki, T., Ijichi, M., Isobe, K., Hashihama, F., Nakamura, K., Ehama, M., et al. (2016). Nitrification and its influence on biogeochemical cycles from the equatorial Pacific to the Arctic Ocean. ISME J. 10, 2184–2197. doi: 10.1038/ismej.2016.18
Shiozaki, T., Ijichi, M., Kodama, T., Takeda, S., and Furuya, K. (2014). Heterotrophic bacteria as major nitrogen fixers in the euphotic zone of the Indian Ocean. Global Biogeochem. Cycles 28, 1096–1110. doi: 10.1002/2014GB004886
Shiozaki, T., Nagata, T., Ijichi, M., and Furuya, K. (2015). Nitrogen fixation and the diazotroph community in the temperate coastal region of the northwestern North Pacific. Biogeosciences 12, 4751–4764. doi: 10.5194/bg-12-4751-2015
Short, S. M., Jenkins, B. D., and Zehr, J. P. (2004). Spatial and temporal distribution of two diazotrophic bacteria in the Chesapeake Bay. Appl. Environ. Microbiol. 70, 2186–2192. doi: 10.1128/AEM.70.4.2186-2192.2004
Siboni, N., Ben-Dov, E., Sivan, A., and Kushmaro, A. (2008). Global distribution and diversity of coral-associated archaea and their possible role in the coral holobiont nitrogen cycle. Environ. Microbiol. 10, 2979–2990. doi: 10.1111/j.1462-2920.2008.01718.x
Simon, J., and Klotz, M. G. (2013). Diversity and evolution of bioenergetic systems involved in microbial nitrogen compound transformations. Biochim. Biophys. Acta Bioenerg. 1827, 114–135. doi: 10.1016/j.bbabio.2012.07.005
Singh, A., Bach, L. T., Fischer, T., Hauss, H., Kiko, R., Paul, A. J., et al. (2017). Niche construction by non-diazotrophs for N2 fixers in the Eastern Tropical North Atlantic Ocean. Geophys. Res. Lett. 44, 6904–6913. doi: 10.1002/2017GL074218
Sintes, E., Bergauer, K., De Corte, D., Yokokawa, T., and Herndl, G. J. (2013). Archaeal amoA gene diversity points to distinct biogeography of ammonia-oxidizing Crenarchaeota in the ocean. Environ. Microbiol. 15, 1647–1658. doi: 10.1111/j.1462-2920.2012.02801.x
Sipler, R. E., Gong, D., Baer, S. E., Sanderson, M. P., Roberts, Q. N., Mulholland, M. R., et al. (2017). Preliminary estimates of the contribution of Arctic nitrogen fixation to the global nitrogen budget. Limnol. Oceanogr. Lett. 2, 159–166. doi: 10.1002/lol2.10046
Slobodkina, G. B., Mardanov, A. V., Ravin, N. V., Frolova, A. A., Chernyh, N. A., Bonch-Osmolovskaya, E. A., et al. (2017). Respiratory ammonification of nitrate coupled to anaerobic oxidation of elemental sulfur in deep-sea autotrophic thermophilic bacteria. Front. Microbiol. 8:87. doi: 10.3389/fmicb.2017.00087
Smith, C. J., Dong, L. F., Wilson, J., Stott, A., Osborn, A. M., and Nedwell, D. B. (2015). Seasonal variation in denitrification and dissimilatory nitrate reduction to ammonia process rates and corresponding key functional genes along an estuarine nitrate gradient. Front. Microbiol. 6:542. doi: 10.3389/fmicb.2015.00542
Smith, C. J., Nedwell, D. B., Dong, L. F., and Osborn, A. M. (2007). Diversity and abundance of nitrate reductase genes (narG and napA), nitrite reductase genes (nirS and nrfA), and their transcripts in estuarine sediments. Appl. Environ. Microbiol. 73, 3612–3622. doi: 10.1128/AEM.028942896
Smith, J. M., Casciotti, K. L., Chavez, F. P., and Francis, C. A. (2014a). Differential contributions of archaeal ammonia oxidizer ecotypes to nitrification in coastal surface waters. ISME J. 8, 1704–1714. doi: 10.1038/ismej.2014.11
Smith, J. M., Chavez, F. P., and Francis, C. A. (2014b). Ammonium uptake by phytoplankton regulates nitrification in the sunlit ocean. PLoS One 9:e108173. doi: 10.1371/journal.pone.0108173
Smith, J. M., Damashek, J., Chavez, F. P., and Francis, C. A. (2016). Factors influencing nitrification rates and the abundance and transcriptional activity of ammonia-oxidizing microorganisms in the dark Northeast Pacific Ocean. Limnol. Oceanogr. 61, 596–609. doi: 10.1002/lno.10235
Smith, J. M., Mosier, A. C., and Francis, C. A. (2015). Spatiotemporal relationships between the abundance, distribution, and potential activities of ammonia-oxidizing and denitrifying microorganisms in intertidal sediments. Microb. Ecol. 69, 13–24. doi: 10.1007/s00248-014-0450-451
Sohm, J. A., Hilton, A. A., Noble, A. E., Zehr, J. P., Saito, M. A., and Webb, E. A. (2011a). Nitrogen fixation in the South Atlantic gyre and the benguela upwelling system. Geophys. Res. Lett. 38:L16608. doi: 10.1029/2011GL048315
Sohm, J. A., Webb, E. A., and Capone, D. G. (2011b). Emerging patterns of marine nitrogen fixation. Nat. Rev. Microbiol. 9, 499–508. doi: 10.1038/nrmicro2594
Sokoll, S., Holtappels, M., Lam, P., Collins, G., Schlüter, M., Lavik, G., et al. (2012). Benthic nitrogen loss in the Arabian Sea off Pakistan. Front. Microbiol. 3:395. doi: 10.3389/fmicb.2012.00395
Somes, C. J., Landolfi, A., Koeve, W., and Oschlies, A. (2016). Limited impact of atmospheric nitrogen deposition on marine productivity due to biogeochemical feedbacks in a global ocean model. Geophys. Res. Lett. 43, 4500–4509. doi: 10.1002/2016GL068335
Song, B., Lisa, J. A., and Tobias, C. R. (2014). Linking DNRA community structure and activity in a shallow lagoonal estuarine system. Front. Microbiol. 5:460. doi: 10.3389/fmicb.2014.00460
Sonthiphand, P., Hall, M. W., and Neufeld, J. D. (2014). Biogeography of anaerobic ammonia-oxidizing (anammox) bacteria. Front. Microbiol. 5:399. doi: 10.3389/fmicb.2014.00399
Spieck, E., Keuter, S., Wenzel, T., Bock, E., and Ludwig, W. (2014). Characterization of a new marine nitrite oxidizing bacterium, Nitrospina watsonii sp. nov., a member of the newly proposed phylum “Nitrospinae. Syst. Appl. Microbiol. 37, 170–176. doi: 10.1016/j.syapm.2013.12.005
Staal, M., Rabouille, S., and Stal, L. J. (2007). On the role of oxygen for nitrogen fixation in the marine cyanobacterium Trichodesmium sp. Environ. Microbiol. 9, 727–736. doi: 10.1111/j.1462-2920.2006.01195.x
Stal, L. J. (2009). Is the distribution of nitrogen-fixing cyanobacteria in the oceans related to temperature? Environ. Microbiol. 11, 1632–1645. doi: 10.1111/j.1758-2229.2009.00016.x
Stein, L. Y. (2011). Surveying N2O-producing pathways in bacteria. Methods Enzymol. 486, 131–152. doi: 10.1016/B978-0-12-381294-0.00006-7
Stenegren, M., Caputo, A., Berg, C., Bonnet, S., and Foster, R. A. (2018). Distribution and drivers of symbiotic and free-living diazotrophic cyanobacteria in the Western Tropical South Pacific. Biogeosciences 15, 1559–1578. doi: 10.5194/bg-15-1559-2018
Stief, P., Fuchs-Ocklenburg, S., Kamp, A., Manohar, C.-S., Houbraken, J., Boekhout, T., et al. (2014). Dissimilatory nitrate reduction by Aspergillus terreus isolated from the seasonal oxygen minimum zone in the Arabian Sea. BMC Microbiol. 14:35. doi: 10.1186/1471-2180-14-35
Stieglmeier, M., Mooshammer, M., Kitzler, B., Wanek, W., Zechmeister-Boltenstern, S., Richter, A., et al. (2014). Aerobic nitrous oxide production through N-nitrosating hybrid formation in ammonia-oxidizing archaea. ISME J. 8, 1135–1146. doi: 10.1038/ismej.2013.220
Strous, M., Pelletier, E., Mangenot, S., Rattei, T., Lehner, A., Taylor, M. W., et al. (2006). Deciphering the evolution and metabolism of an anammox bacterium from a community genome. Nature 440, 790–794. doi: 10.1038/nature04647
Sun, X., Ji, Q., Jayakumar, A., and Ward, B. B. (2017). Dependence of nitrite oxidation on nitrite and oxygen in low-oxygen seawater. Geophys. Res. Lett. 44, 7883–7891. doi: 10.1002/2017GL074355
Sun, X., Kop, L. F. M., Lau, M. C. Y., Frank, J., Jayakumar, A., Lücker, S., et al. (2019). Uncultured nitrospina-like species are major nitrite oxidizing bacteria in oxygen minimum zones. ISME J. 13, 2391–2402. doi: 10.1038/s41396-019-0443-447
Suntharalingam, P., Buitenhuis, E., Le Quéré, C., Dentener, F., Nevison, C., Butler, J. H., et al. (2012). Quantifying the impact of anthropogenic nitrogen deposition on oceanic nitrous oxide. Geophys. Res. Lett. 39, 1–6. doi: 10.1029/2011GL050778
Syakila, A., and Kroeze, C. (2011). The global nitrous oxide budget revisited. Greenh. Gas Meas. Manag. 1, 17–26. doi: 10.3763/ghgmm.2010.0007
Tait, K., Kitidis, V., Ward, B., Cummings, D., Jones, M., Somerfield, P., et al. (2014). Spatio-temporal variability in ammonia oxidation and ammonia-oxidising bacteria and archaea in coastal sediments of the western english channel. Mar. Ecol. Prog. Ser. 511, 41–58. doi: 10.3354/meps10933
Takeuchi, J. (2006). Habitat segregation of a functional gene encoding nitrate ammonification in estuarine sediments. Geomicrobiol. J. 23, 75–87. doi: 10.1080/01490450500533866
Tal, Y., Watts, J. E. M., and Schreier, H. J. (2005). Anaerobic ammonia-oxidizing bacteria and related activity in baltimore inner harbor sediment. Appl. Environ. Microbiol. 71, 1816–1821. doi: 10.1128/AEM.71.4.1816-1821.2005
Tamegai, H., Aoki, R., Arakawa, S., and Kato, C. (2007). Molecular analysis of the nitrogen cycle in deep-sea microorganisms from the nankai trough: genes for nitrification and denitrification from deep-sea environmental DNA. Extremophiles 11, 269–275. doi: 10.1007/s00792-006-0035-30
Tang, W., Wang, S., Fonseca-Batista, D., Dehairs, F., Gifford, S., Gonzalez, A. G., et al. (2019). Revisiting the distribution of oceanic N2 fixation and estimating diazotrophic contribution to marine production. Nat. Commun. 10:831. doi: 10.1038/s41467-019-08640-8640
Teixeira, C., Magalhães, C., Joye, S. B., and Bordalo, A. A. (2016). Response of anaerobic ammonium oxidation to inorganic nitrogen fluctuations in temperate estuarine sediments. J. Geophys. Res. Biogeosci. 121, 1829–1839. doi: 10.1002/2015JG003287
Thamdrup, B. (2012). New pathways and processes in the global nitrogen cycle. Annu. Rev. Ecol. Evol. Syst. 43, 407–428. doi: 10.1146/annurev-ecolsys-102710-145048
Thamdrup, B., and Dalsgaard, T. (2002). Production of N2 through anaerobic ammonium oxidation coupled to nitrate reduction in marine sediments. Appl. Environ. Microbiol. 68, 1312–1318. doi: 10.1128/AEM.68.3.1312-1318.2002
Thamdrup, B., Steinsdóttir, H. G. R., Bertagnolli, A. D., Padilla, C. C., Patin, N. V., Garcia-Robledo, E., et al. (2019). Anaerobic methane oxidation is an important sink for methane in the ocean’s largest oxygen minimum zone. Limnol. Oceanogr. 64, 2569–2585. doi: 10.1002/lno.11235
Thompson, A. W., Foster, R. A., Krupke, A., Carter, B. J., Musat, N., Vaulot, D., et al. (2012). Unicellular cyanobacterium symbiotic with a single-celled eukaryotic alga. Science 337, 1546–1550. doi: 10.1126/science.1222700
Thompson, A., Carter, B. J., Turk-Kubo, K., Malfatti, F., Azam, F., and Zehr, J. P. (2014). Genetic diversity of the unicellular nitrogen-fixing cyanobacteria UCYN-A and its prymnesiophyte host. Environ. Microbiol. 16, 3238–3249. doi: 10.1111/1462-2920.12490
Tiedje, J. M. (1988). “Ecology of denitrification and dissimilatory nitrate reduction to ammonium,” in Environmental Microbiology of Anaerobes, ed. A. J. B. Zehnder, (New York, NY: John Wiley & Sons), 179–244.
Tikhonova, T. V., Slutsky, A., Antipov, A. N., Boyko, K. M., Polyakov, K. M., Sorokin, D. Y., et al. (2006). Molecular and catalytic properties of a novel cytochrome c nitrite reductase from nitrate-reducing haloalkaliphilic sulfur-oxidizing bacterium thioalkalivibrio nitratireducens. Biochim. Biophys. Acta Proteins Proteom. 1764, 715–723. doi: 10.1016/j.bbapap.2005.12.021
Timmers, P. H. A., Welte, C. U., Koehorst, J. J., Plugge, C. M., Jetten, M. S. M., and Stams, A. J. M. (2017). Reverse methanogenesis and respiration in methanotrophic archaea. Archaea 2017, 1–22. doi: 10.1155/2017/1654237
Trimmer, M., and Engström, P. (2011). “Distribution, activity, and ecology of anammox bacteria in aquatic environments,” in Nitrification, eds B. B. Ward, D. J. Arp, and M. G. Klotz, (Washington, D.C: ASM Press), 201–235.
Trimmer, M., Chronopoulou, P.-M., Maanoja, S. T., Upstill-Goddard, R. C., Kitidis, V., and Purdy, K. J. (2016). Nitrous oxide as a function of oxygen and archaeal gene abundance in the North Pacific. Nat. Commun. 7:13451. doi: 10.1038/ncomms13451
Trimmer, M., Nicholls, J. C., and Deflandre, B. (2003). Anaerobic ammonium oxidation measured in sediments along the Thames Estuary, United Kingdom. Appl. Environ. Microbiol. 69, 6447–6454. doi: 10.1128/AEM.69.11.6447-6454-2003
Trimmer, M., Nicholls, J. C., Morley, N., Davies, C. A., and Aldridge, J. (2005). Biphasic behavior of anammox regulated by nitrite and nitrate in an estuarine sediment. Appl. Environ. Microbiol. 71, 1923–1930. doi: 10.1128/AEM.71.4.1923-1930.2005
Tsementzi, D., Wu, J., Deutsch, S., Nath, S., Rodriguez-R, L. M., Burns, A. S., et al. (2016). SAR11 bacteria linked to ocean anoxia and nitrogen loss. Nature 536, 179–183. doi: 10.1038/nature19068
Tully, B. J., and Heidelberg, J. F. (2016). Potential mechanisms for microbial energy acquisition in oxic deep-sea sediments. Appl. Environ. Microbiol. 82, 4232–4243. doi: 10.1128/AEM.01023-1016
Turk, K. A., Rees, A. P., Zehr, J. P., Pereira, N., Swift, P., Shelley, R., et al. (2011). Nitrogen fixation and nitrogenase (nifH) expression in tropical waters of the Eastern North Atlantic. ISME J. 5, 1201–1212. doi: 10.1038/ismej.2010.205
Turk-Kubo, K. A., Achilles, K. M., Serros, T. R. C., Ochiai, M., Montoya, J. P., and Zehr, J. P. (2012). Nitrogenase (nifH) gene expression in diazotrophic cyanobacteria in the Tropical North Atlantic in response to nutrient amendments. Front. Microbiol. 3:386. doi: 10.3389/fmicb.2012.00386
Turk-Kubo, K. A., Connell, P., Caron, D., Hogan, M. E., Farnelid, H. M., and Zehr, J. P. (2018). In situ diazotroph population dynamics under different resource ratios in the North Pacific Subtropical Gyre. Front. Microbiol. 9:1616. doi: 10.3389/fmicb.2018.01616
Turk-Kubo, K. A., Farnelid, H. M., Shilova, I. N., Henke, B., and Zehr, J. P. (2017). Distinct ecological niches of marine symbiotic N2-fixing cyanobacterium Candidatus Atelocyanobacterium thalassa sublineages. J. Phycol. 53, 451–461. doi: 10.1111/jpy.12505
Turk-Kubo, K. A., Frank, I. E., Hogan, M. E., Desnues, A., Bonnet, S., and Zehr, J. P. (2015). Diazotroph community succession during the VAHINE mesocosm experiment (New Caledonia lagoon). Biogeosciences 12, 7435–7452. doi: 10.5194/bg-12-7435-2015
Urakawa, H., Martens-Habbena, W., Huguet, C., de la Torre, J. R., Ingalls, A. E., Devol, A. H., et al. (2014). Ammonia availability shapes the seasonal distribution and activity of archaeal and bacterial ammonia oxidizers in the puget sound estuary. Limnol. Oceanogr. 59, 1321–1335. doi: 10.4319/lo.2014.59.4.1321
Vajrala, N., Martens-Habbena, W., Sayavedra-Soto, L. A., Schauer, A., Bottomley, P. J., Stahl, D. A., et al. (2013). Hydroxylamine as an intermediate in ammonia oxidation by globally abundant marine archaea. Proc. Natl. Acad. Sci. U.S.A. 110, 1006–1011. doi: 10.1073/pnas.1214272110
Vaksmaa, A., Jetten, M. S. M., Ettwig, K. F., and Lüke, C. (2017). mcrA primers for the detection and quantification of the anaerobic archaeal methanotroph ‘Candidatus Methanoperedens nitroreducens.’. Appl. Microbiol. Biotechnol. 101, 1631–1641. doi: 10.1007/s00253-016-8065-8068
van de Vossenberg, J., Rattray, J. E., Geerts, W., Kartal, B., van Niftrik, L., van Donselaar, E. G., et al. (2008). Enrichment and characterization of marine anammox bacteria associated with global nitrogen gas production. Environ. Microbiol. 10, 3120–3129. doi: 10.1111/j.1462-2920.2008.01643.x
van de Vossenberg, J., Woebken, D., Maalcke, W. J., Wessels, H. J. C. T., Dutilh, B. E., Kartal, B., et al. (2013). The metagenome of the marine anammox bacterium ‘Candidatus Scalindua profunda’ illustrates the versatility of this globally important nitrogen cycle bacterium. Environ. Microbiol. 15, 1275–1289. doi: 10.1111/j.1462-2920.2012.02774.x
van den Berg, E. M., Rombouts, J. L., Kuenen, J. G., Kleerebezem, R., and van Loosdrecht, M. C. M. (2017). Role of nitrite in the competition between denitrification and DNRA in a chemostat enrichment culture. AMB Express 7:91. doi: 10.1186/s13568-017-0398-x
van Kessel, M. A. H. J., Speth, D. R., Albertsen, M., Nielsen, P. H., Op, den Camp, H. J. M., et al. (2015). Complete nitrification by a single microorganism. Nature 528, 555–559. doi: 10.1038/nature16459
Vaquer-Sunyer, R., and Duarte, C. M. (2008). Thresholds of hypoxia for marine biodiversity. Proc. Natl. Acad. Sci. U.S.A. 105, 15452–15457. doi: 10.1073/pnas.0803833105
Villareal, T. (1991). Nitrogen-fixation by the cyanobacterial symbiont of the diatom genus Hemiaulus. Mar. Ecol. Prog. Ser. 76, 201–204. doi: 10.3354/meps076201
Voss, M., Bange, H. W., Dippner, J. W., Middelburg, J. J., Montoya, J. P., and Ward, B. B. (2013). The marine nitrogen cycle: recent discoveries, uncertainties and the potential relevance of climate change. Philos. Trans. R. Soc. B 368:20130121. doi: 10.1098/rstb.2013.0121
Walker, C. B., de la Torre, J. R., Klotz, M. G., Urakawa, H., Pinel, N., Arp, D. J., et al. (2010). Nitrosopumilus maritimus genome reveals unique mechanisms for nitrification and autotrophy in globally distributed marine crenarchaea. Proc. Natl. Acad. Sci. U.S.A. 107, 8818–8823. doi: 10.1073/pnas.0913533107
Wang, J., Shen, L., He, Z., Hu, J., Cai, Z., Zheng, P., et al. (2017). Spatial and temporal distribution of nitrite-dependent anaerobic methane-oxidizing bacteria in an intertidal zone of the East China Sea. Appl. Microbiol. Biotechnol. 101, 8007–8014. doi: 10.1007/s00253-017-8521-8520
Wang, L., Zheng, B., Nan, B., and Hu, P. (2014). Diversity of bacterial community and detection of nirS- and nirK-encoding denitrifying bacteria in sandy intertidal sediments along Laizhou Bay of Bohai Sea. China. Mar. Pollut. Bull. 88, 215–223. doi: 10.1016/j.marpolbul.2014.09.002
Wannicke, N., Frey, C., Law, C. S., and Voss, M. (2018). The response of the marine nitrogen cycle to ocean acidification. Glob. Chang. Biol. 24, 5031–5043. doi: 10.1111/gcb.14424
Ward, B. B. B., Devol, A. H., Rich, J. J., Chang, B. X., Bulow, S. E., Naik, H., et al. (2009). Denitrification as the dominant nitrogen loss process in the Arabian Sea. Nature 461, 78–81. doi: 10.1038/nature08276
Ward, B. B., Tuit, C. B., Jayakumar, A., Rich, J. J., Moffett, J., and Naqvi, S. W. A. (2008). Organic carbon, and not copper, controls denitrification in oxygen minimum zones of the ocean. Deep Sea Res. Part I Oceanogr. Res. Pap. 55, 1672–1683. doi: 10.1016/j.dsr.2008.07.005
Watson, S. W., and Waterbury, J. B. (1971). Characteristics of two marine nitrite oxidizing bacteria, nitrospina gracilis nov. gen. nov. sp. and Nitrococcus mobilis nov. gen. nov. sp. Arch. Mikrobiol. 77, 203–230. doi: 10.1007/BF00408114
Welsh, A., Chee-Sanford, J. C., Connor, L. M., Löffler, F. E., and Sanford, R. A. (2014). Refined NrfA phylogeny improves PCR-based nrfA gene detection. Appl. Environ. Microbiol. 80, 2110–2119. doi: 10.1128/AEM.03443-3413
Wen, Z., Lin, W., Shen, R., Hong, H., Kao, S. J., and Shi, D. (2017). Nitrogen fixation in two coastal upwelling regions of the Taiwan Strait. Sci. Rep. 7:17601. doi: 10.1038/s41598-017-18006-18005
Wilson, S. T., del Valle, D. A., Segura-Noguera, M., and Karl, D. M. (2014). A role for nitrite in the production of nitrous oxide in the lower euphotic zone of the oligotrophic North Pacific Ocean. Deep Sea Res. Part I Oceanogr. Res. Pap. 85, 47–55. doi: 10.1016/j.dsr.2013.11.008
Wittorf, L., Bonilla-Rosso, G., Jones, C. M., Bäckman, O., Hulth, S., and Hallin, S. (2016). Habitat partitioning of marine benthic denitrifier communities in response to oxygen availability. Environ. Microbiol. Rep. 8, 486–492. doi: 10.1111/1758-2229.12393
Woebken, D., Burow, L. C., Behnam, F., Mayali, X., Schintlmeister, A., Fleming, E. D., et al. (2015). Revisiting N2 fixation in Guerrero Negro intertidal microbial mats with a functional single-cell approach. ISME J. 9, 485–496. doi: 10.1038/ismej.2014.144
Woebken, D., Burow, L. C., Prufert-Bebout, L., Bebout, B. M., Hoehler, T. M., Pett-Ridge, J., et al. (2012). Identification of a novel cyanobacterial group as active diazotrophs in a coastal microbial mat using NanoSIMS analysis. ISME J. 6, 1427–1439. doi: 10.1038/ismej.2011.200
Woebken, D., Fuchs, B. M., Kuypers, M. M. M., and Amann, R. (2007). Potential interactions of particle-associated anammox bacteria with bacterial and archaeal partners in the Namibian upwelling system. Appl. Environ. Microbiol. 73, 4648–4657. doi: 10.1128/AEM.02774-2776
Woebken, D., Lam, P., Kuypers, M. M. M., Naqvi, S. W. A., Kartal, B., Strous, M., et al. (2008). A microdiversity study of anammox bacteria reveals a novel Candidatus Scalindua phylotype in marine oxygen minimum zones. Environ. Microbiol. 10, 3106–3119. doi: 10.1111/j.1462-2920.2008.01640.x
Wu, M. L., Ettwig, K. F., Jetten, M. S. M., Strous, M., Keltjens, J. T., Niftrik, L., et al. (2011). A new intra-aerobic metabolism in the nitrite-dependent anaerobic methane-oxidizing bacterium Candidatus ‘Methylomirabilis oxyfera.’. Biochem. Soc. Trans. 39, 243–248. doi: 10.1042/BST0390243
Wu, Y., Cao, Y., Wang, C., Wu, M., Aharon, O., and Xu, X. (2014). Microbial community structure and nitrogenase gene diversity of sediment from a deep-sea hydrothermal vent field on the Southwest Indian Ridge. Acta Oceanol. Sin. 33, 94–104. doi: 10.1007/s13131-014-0544-540
Wuchter, C., Abbas, B., Coolen, M. J. L., Herfort, L., van Bleijswijk, J., Timmers, P., et al. (2006). Archaeal nitrification in the ocean. Proc. Natl. Acad. Sci. U.S.A. 103, 12317–12322. doi: 10.1073/pnas.0600756103
Wyman, M., Hodgson, S., and Bird, C. (2013). Denitrifying alphaproteobacteria from the Arabian Sea that express nosZ, the gene encoding nitrous oxide reductase, in oxic and suboxic waters. Appl. Environ. Microbiol. 79, 2670–2681. doi: 10.1128/AEM.03705-3712
Xia, F., Wang, J.-G., Zhu, T., Zou, B., Rhee, S.-K., and Quan, Z.-X. (2018). Ubiquity and diversity of complete ammonia oxidizers (comammox). Appl. Environ. Microbiol. 84:e01390-18. doi: 10.1128/AEM.01390-1318
Xu, W., Li, M., Ding, J.-F., Gu, J.-D., and Luo, Z.-H. (2014). Bacteria dominate the ammonia-oxidizing community in a hydrothermal vent site at the mid-atlantic ridge of the South Atlantic Ocean. Appl. Microbiol. Biotechnol. 98, 7993–8004. doi: 10.1007/s00253-014-5833-5831
Yang, S., and Gruber, N. (2016). The anthropogenic perturbation of the marine nitrogen cycle by atmospheric deposition: nitrogen cycle feedbacks and the 15N haber-bosch effect. Global Biogeochem. Cycles 30, 1418–1440. doi: 10.1002/2016GB005421
Yang, S., Sun, W., Zhang, F., and Li, Z. (2013). Phylogenetically diverse denitrifying and ammonia-oxidizing bacteria in corals Alcyonium gracillimum and Tubastraea coccinea. Mar. Biotechnol. 15, 540–551. doi: 10.1007/s10126-013-9503-9506
Yin, G., Hou, L., Liu, M., Li, X., Zheng, Y., Gao, J., et al. (2017). DNRA in intertidal sediments of the Yangtze Estuary. J. Geophys. Res. Biogeosci. 122, 1988–1998. doi: 10.1002/2017JG003766
Yool, A., Martin, A. P., Fernández, C., and Clark, D. R. (2007). The significance of nitrification for oceanic new production. Nature 447, 999–1002. doi: 10.1038/nature05885
Yu, T., Li, M., Niu, M., Fan, X., Liang, W., and Wang, F. (2018). Difference of nitrogen-cycling microbes between shallow bay and deep-sea sediments in the South China Sea. Appl. Microbiol. Biotechnol. 102, 447–459. doi: 10.1007/s00253-017-8594-8599
Zakem, E. J., Al-Haj, A., Church, M. J., van Dijken, G. L., Dutkiewicz, S., Foster, S. Q., et al. (2018). Ecological control of nitrite in the upper ocean. Nat. Commun. 9:1206. doi: 10.1038/s41467-018-03553-w
Zehr, J. P., and Kudela, R. M. (2011). Nitrogen cycle of the open ocean: from genes to ecosystems. Ann. Rev. Mar. Sci. 3, 197–225. doi: 10.1146/annurev-marine-120709-142819
Zehr, J. P., and Paerl, H. W. (2008). “Molecular ecological aspects of nitrogen fixation in the marine environment,” in Microbial Ecology of the Oceans, ed. D. L. Kirchman, (Hoboken, NJ: John Wiley & Sons, Inc.), 481–525. doi: 10.1002/9780470281840.ch13
Zehr, J. P., Bench, S. R., Carter, B. J., Hewson, I., Niazi, F., Shi, T., et al. (2008). Globally distributed uncultivated oceanic N2-fixing cyanobacteria lack oxygenic photosystem II. Science 322, 1110–1112. doi: 10.1126/science.1165340
Zehr, J. P., Bench, S. R., Mondragon, E. A., McCarren, J., and DeLong, E. F. (2007). Low genomic diversity in tropical oceanic N2-fixing cyanobacteria. Proc. Natl. Acad. Sci. U.S.A. 104, 17807–17812. doi: 10.1073/pnas.0701017104
Zehr, J. P., Jenkins, B. D., Short, S. M., and Steward, G. F. (2003). Nitrogenase gene diversity and microbial community structure: a cross-system comparison. Environ. Microbiol. 5, 539–554. doi: 10.1046/j.1462-2920.2003.00451.x
Zehr, J. P., Mellon, M., and Zani, S. (1998). New nitrogen-fixing microorganisms detected in oligotrophic oceans by amplification of nitrogenase (nifH) genes. Appl. Environ. Microbiol. 64, 3444–3450.
Zehr, J. P., Shilova, I. N., Farnelid, H. M., Muñoz-Marín, M. C., and Turk-Kubo, K. A. (2016). Unusual marine unicellular symbiosis with the nitrogen-fixing cyanobacterium UCYN-A. Nat. Microbiol. 2:16214. doi: 10.1038/nmicrobiol.2016.214
Zehr, J. P., Waterbury, J. B., Turner, P. J., Montoya, J. P., Omoregie, E., Steward, G. F., et al. (2001). Unicellular cyanobacteria fix N2 in the subtropical North Pacific Ocean. Nature 412, 635–638. doi: 10.1038/35088063
Zehr, J., and Ward, B. B. (2002). Nitrogen cycling in the ocean: new perspectives on processes and paradigms. Appl. Environ. Microbiol. 68, 1015–1024. doi: 10.1128/AEM.68.3.1015
Zhang, F., Pita, L., Erwin, P. M., Abaid, S., López-Legentil, S., and Hill, R. T. (2014). Symbiotic archaea in marine sponges show stability and host specificity in community structure and ammonia oxidation functionality. FEMS Microbiol. Ecol. 90, 699–707. doi: 10.1111/1574-6941.12427
Zhang, M., Luo, Y., Lin, L., Lin, X., Hetharua, B., Zhao, W., et al. (2018). Molecular and stable isotopic evidence for the occurrence of nitrite-dependent anaerobic methane-oxidizing bacteria in the mangrove sediment of Zhangjiang Estuary China. Appl. Microbiol. Biotechnol. 102, 2441–2454. doi: 10.1007/s00253-017-8718-8712
Zhang, Y., Xie, X., Jiao, N., Hsiao, S. S. Y., and Kao, S. J. (2014). Diversity and distribution of amoA-type nitrifying and nirS-type denitrifying microbial communities in the Yangtze River Estuary. Biogeosciences 11, 2131–2145. doi: 10.5194/bg-11-2131-2014
Zheng, Y., Hou, L., Liu, M., Liu, Z., Li, X., Lin, X., et al. (2016). Tidal pumping facilitates dissimilatory nitrate reduction in intertidal marshes. Sci. Rep. 6:21338. doi: 10.1038/srep21338
Zheng, Y., Hou, L., Newell, S., Liu, M., Zhou, J., Zhao, H., et al. (2014). Community dynamics and activity of ammonia-oxidizing prokaryotes in intertidal sediments of the Yangtze Estuary. Appl. Environ. Microbiol. 80, 408–419. doi: 10.1128/AEM.03035-3013
Zhu, X., Burger, M., Doane, T. A., and Horwath, W. R. (2013). Ammonia oxidation pathways and nitrifier denitrification are significant sources of N2O and NO under low oxygen availability. Proc. Natl. Acad. Sci. U.S.A. 110, 6328–6333. doi: 10.1073/pnas.1219993110
Keywords: nitrogen processes, marine ecosystems, microbial community, functional nitrogen genes, anthropogenic activity, nitrous oxide
Citation: Pajares S and Ramos R (2019) Processes and Microorganisms Involved in the Marine Nitrogen Cycle: Knowledge and Gaps. Front. Mar. Sci. 6:739. doi: 10.3389/fmars.2019.00739
Received: 03 June 2019; Accepted: 13 November 2019;
Published: 29 November 2019.
Edited by:
Lasse Riemann, University of Copenhagen, DenmarkReviewed by:
Mar Benavides, Institut de Recherche pour le Développement (IRD), FranceBo Thamdrup, University of Southern Denmark, Denmark
Robinson W. (Wally) Fulweiler, Boston University, United States
Copyright © 2019 Pajares and Ramos. This is an open-access article distributed under the terms of the Creative Commons Attribution License (CC BY). The use, distribution or reproduction in other forums is permitted, provided the original author(s) and the copyright owner(s) are credited and that the original publication in this journal is cited, in accordance with accepted academic practice. No use, distribution or reproduction is permitted which does not comply with these terms.
*Correspondence: Silvia Pajares, c3BhamFyZXNAY21hcmwudW5hbS5teA==; c2lsdmlwYW1vQGdtYWlsLmNvbQ==
†These authors have contributed equally to this work