- 1Institute of Marine Sciences, National Research Council, Bologna, Italy
- 2Department for the Cultural Heritage, University of Bologna, Ravenna, Italy
- 3Department of Biology, Woods Hole Oceanographic Institution, Woods Hole, MA, United States
- 4Stazione Zoologica Anton Dohrn, Naples, Italy
- 5Institute of Polar Sciences, National Research Council, Bologna, Italy
- 6Laboratoire des Sciences du Climat et de l’Environnement, Gif-sur-Yvette, France
- 7Lamont Doherty Earth Observatory, Columbia University, Palisades, NY, United States
Ocean warming is expected to impinge detrimentally on marine ecosystems worldwide up to impose extreme environmental conditions capable to potentially jeopardize the good ecological status of scleractinian coral reefs at shallow and bathyal depths. The integration of literature records with newly acquired remotely operated vehicle (ROV) data provides an overview of the geographic distribution of the temperate coral Dendrophyllia cornigera spanning the eastern Atlantic Ocean to the whole Mediterranean Sea. In addition, we extracted temperature values at each occurrence site to define the natural range of this coral, known to maintain its physiological processes at 16°C. Our results document a living temperature range between ∼7°C and 17°C, suggesting that the natural thermal tolerance of this eurybathic coral may represent an advantage for its survival in a progressively warming ocean.
Introduction
More efficient remote-sensing technologies, advanced SCUBA diving techniques, increasing quality of ROV (Remotely Operated Vehicles) images, and maneuverability have sensibly bettered our in-depth understanding of shallow-to-deep marine habitats. In the Mediterranean Sea, scleractinian corals populate all depths: (i) zooxantellate corals in shallow waters (with Cladocora caespitosa as the major bioconstructor); (ii) zooxanthellate and azooxanthellate corals at intermediate depths, approximately between 30 and 200 m (e.g., Dendrophyllia cornigera and D. ramea); and (iii) azooxanthellate cold-water corals (CWCs) below 200 m depth, mostly dominated by Madrepora oculata and Desmophyllum pertusum (=Lophelia pertusa: Addamo et al., 2016).
However, to date, relatively little information is available on mesophotic-to-twilight coral habitats, though these are known to be defined by specific environmental factors and characterized by high level of biodiversity (Loya et al., 2018; Corriero et al., 2019).
Although our perception of how global climate changes are affecting marine environments is still limited, there is a growing awareness that these may negatively influence marine ecosystems (Poloczanska et al., 2013; Gattuso et al., 2015). This holds true, in particular, for coral biocostructions, known for supporting and enhancing biodiversity, and providing unique ecosystems services (Hoegh-Guldberg et al., 2017). According to the Fifth Assessment Report (AR5) of the Intergovernmental Panel on Climate Change (IPCC) the current global climate change is proceeding at an unprecedented pace compared to those occurred in the geological history (Pachauri et al., 2014). The surface ocean temperatures have increased of about 1°C since the beginning of the 20th century (0.89°C over the period 1901–2012, Pachauri et al., 2014). Available forecasts provide quantitative scenarios of the possible future state of the ocean, prospecting that oceans will be warmer, more acidic and stratified, less productive, and oxygenated than at present by the end of the 21st century (Pachauri et al., 2014). If these predictions come true, stenoecius marine organisms will be facing extreme conditions in the near future, calling for adaptation or failure. Warming ocean temperatures may influence corals fitness and physiological processes, leading to variations in their abundance and distribution (Hughes et al., 2018). Decreasing ocean pH may alter coral calcification capacity and metabolism, though with responses that appear to be species-specific (McCulloch et al., 2012; Rodolfo-Metalpa et al., 2015; Movilla, 2019).
The consequences of global changes have already been observed at tropical latitudes, where coral reefs have experienced several mass bleaching events (e.g., in 1998, 2002, and 2006), with the largest event recorded in 2016 (Lough et al., 2018). Globally, 90% of all reefs is expected to experience severe bleaching with annual incidence by 2055 (Hooidonk et al., 2014). The Mediterranean Sea is also strongly influenced by climate change, with future warming expected to exceed global rates by 25% (Lionello and Scarascia, 2018). Here, global changes are threatening coral ecosystems by exacerbating bleaching events in temperate zooxanthellate species (Kersting et al., 2013), inducing mass mortality of circalittoral coral communities due to heat waves (Coma et al., 2009), and emphasizing the reduction of food supply and oxygen availability for deep-sea corals related to ocean stratification and the restriction of water recycle (Levin and Bris, 2015).
Dendrophyllia cornigera, the yellow coral, is a considerable component of both mesophotic and deep coral ecosystems in the Mediterranean Sea, extending its bathymetric range up to participate to deep-sea ecosystems dominated by white cold-water corals (Roberts et al., 2009; Angeletti et al., 2014; Taviani et al., 2017; Altuna and Poliseno, 2019; Aymà et al., 2019; Chimienti et al., 2019; Puig and Gili, 2019; Rueda et al., 2019). This coral species was observed also as part of the mesophotic-to-deep coral communities in the Atlantic, from the Azores to the Bay of Biscay (Rodolfo-Metalpa et al., 2015). The spatial distribution of D. cornigera (Figure 1), and the wide range of environments that this species populates, suggest that it may have less restrictive environmental needs to settle and grow compared to those of other CWCs, mainly restricted to temperature between 4°C and 13°C (Roberts et al., 2006). In several studies investigating the biological response of different deep-sea corals (mainly M. oculata, D. pertusum, and D. cornigera) to the increasing sea water temperature, D. pertusum showed decreased fitness performances at 15°C (Brooke et al., 2013) while M. oculata appeared more tolerant (Orejas et al., 2011). The same thermal treatments applied on specimens of D. cornigera revealed an higher ability to capture preys (zooplankton) and an increase in the growth and respiration rates at temperature regimes up to over 16°C (Gori et al., 2014; Reynaud and Ferrier-Pagès, 2019). However, information about the spatial distribution of D. cornigera and its temperature preferences from in situ observations are still poor and fragmented, lessening our capability to make predictions regarding the fate of this species in relation with global changes. In this study, we quantify D. cornigera occurrences based on 16 ROV video transects performed in 8 sites spanning different areas of the Mediterranean Sea. These data have been integrated with published D. cornigera records to get a comprehensive picture of the distribution of D. cornigera and of how its abundance varies in relation with depth. Finally, we determine the temperature range of its occurrence. Our contribution aims at providing the first comprehensive overview on the geographic and bathymetric distribution of D. cornigera, strongly focusing to the definition of its thermal tolerance range with in situ observation, and speculating about possible response in face of global ocean warming.
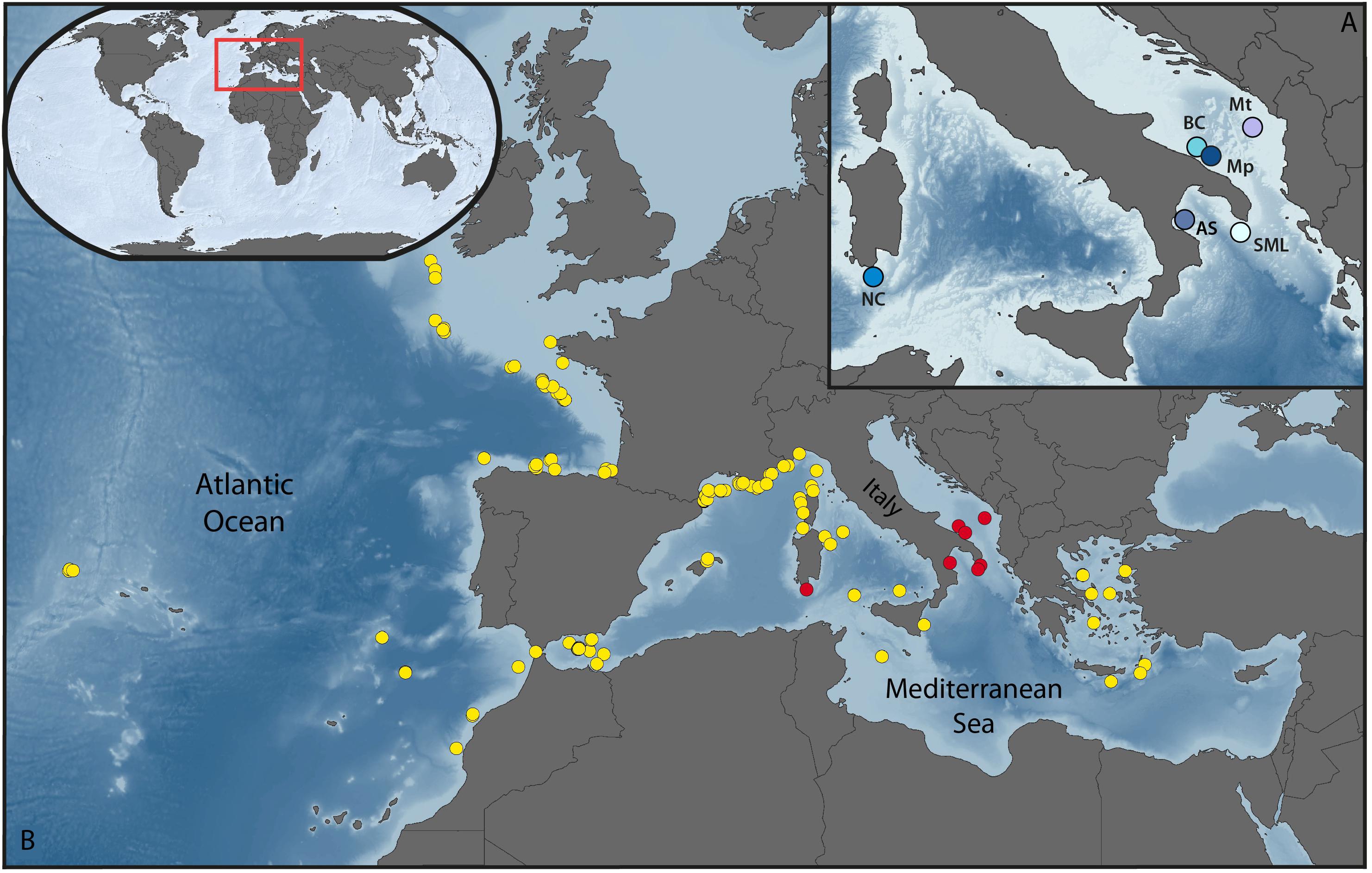
Figure 1. Dendrophyllia cornigera distribution. (A) Detailed view of the sites explored by the ROV dives. BC, Bari Canyon (272–510 m depth); SML, off Santa Maria di Leuca (119–498 m depth); AS, Amendolara Seamount (133–205 m depth); NC, Nora Canyon (441–463 m depth); Mt, off Montenegro (433–504 m depth); Mp, off Monopoli (463–494 m depth). (B) Locations of D. cornigera published records (yellow dots, for reference see Supplementary Table S1), and occurrences obtained from ROV video transects (red dots).
Materials and Methods
Benthic Community Data
Data come from the analysis of 16 ROV videos collected during 8 oceanographic cruises carried out from 2010 to 2017 in 6 different areas (Supplementary Table S1): 9 from the Adriatic Sea (Bari Canyon, off Monopoli and off Montenegro); 6 in the Ionian Sea (Amendolara Seamount and Santa Maria di Leuca); and 1 in the Tyrrhenian Sea (Nora Canyon) (Figure 1A). We analyzed the videos using ArcGIS and Adelie software. The navigation data were simplified using Gaussian smoothing and synchronized with the high-definition ROV videos. The benthic communities were mapped by randomly extracting a frame every 10 s, obtaining over 9700 seafloor images. After removing poor-quality frames, the remaining images were analyzed for the taxonomic identification of sessile megabenthic fauna and to determine D. cornigera sites.
Literature Records
The ROV data were integrated with previous information on the distribution of D. cornigera from available peer-reviewed publications, national and international reports obtained from principal scientific databases (such as ISI Web of Knowledge, Scopus, Google Scholar). We selected only works reporting the geographic position and the depth of the coral occurrences. In older publications, benthic samples were collected using dredges, hauls or other devices, making it impossible to identify the precise coordinates and depths of the samples. We therefore decided to exclude sites without a precise location and with an uncertainty in the sampling depth greater than 100 m, which could generate a bias in the temperature analysis.
Temperature Ranges
Seawater temperature values at water depths close to the D. cornigera sites were sourced from the World Ocean Atlas 2013 version 2 (WOA13v2) dataset at 0.25° (Locarnini et al., 2013). For each site, we extracted seasonal temperature values (winter: January–March; spring: April–June; summer: July–September; autumn: October–December) at the closest available depth to that of D. cornigera records. Temperatures were extracted at the minimum and maximum depth values for those literature records missing a unique depth value but characterized by a range. The annual minimum and maximum temperature of each D. cornigera records were calculated, providing the overall temperature range.
Results
Geographic and Bathymetric Distribution of Dendrophyllia cornigera
The 16 ROV dives explored 90,054 m2 of seabed in the depth range comprised between 119 and 510 m. Overall, the analysis of the benthic communities revealed a notable presence of D. cornigera within the 6 investigated areas, with 61 sites having at least one colony of the yellow coral, for a total of 116 colonies (Supplementary Table S2). The Ionian dives revealed the highest number of D. cornigera specimens (83 colonies in 42 sites). In particular, the Amendolara Seamount area hosts the highest value documented, 67 colonies distributed in 35 sites, while dives performed off Santa Maria di Leuca show the lowest abundance in the Ionian Sea (16 colonies in 7 sites). The occurrences are significantly lower in the Adriatic Sea, where a total of 14 sites host 22 colonies. Here, 5 coral sites were identified in the Bari Canyon area and off Monopoli, which present 6 and 12 colonies, respectively, while dives performed off Montenegro show only one colony for each of the 4 sites. In the Nora Canyon (South Sardinia), 11 colonies were observed in 5 sites along the transect.
Most of the coral occurrences fall within the 100–200 m depth range, where 32 sites and 61 specimens were counted (Figure 2). At this depth, transects performed along the Amendolara Seamount largely contribute to the abundance observed (29 sites and 58 colonies), while Santa Maria di Leuca dives display much lower values (3 sites and 3 colonies). A second peak of abundance is present between 400 and 500 m depth, with a total of 20 sites hosting 42 colonies. Within this bathymetric range, we identified sites with D. cornigera specimens in all the explored areas, with the exception of Amendolara Seamount area. The highest number of sites were detected in Nora Canyon and off Monopoli areas (5 coral sites each), while a greater number of specimens (13 colonies) was reported in the Santa Maria di Leuca area.
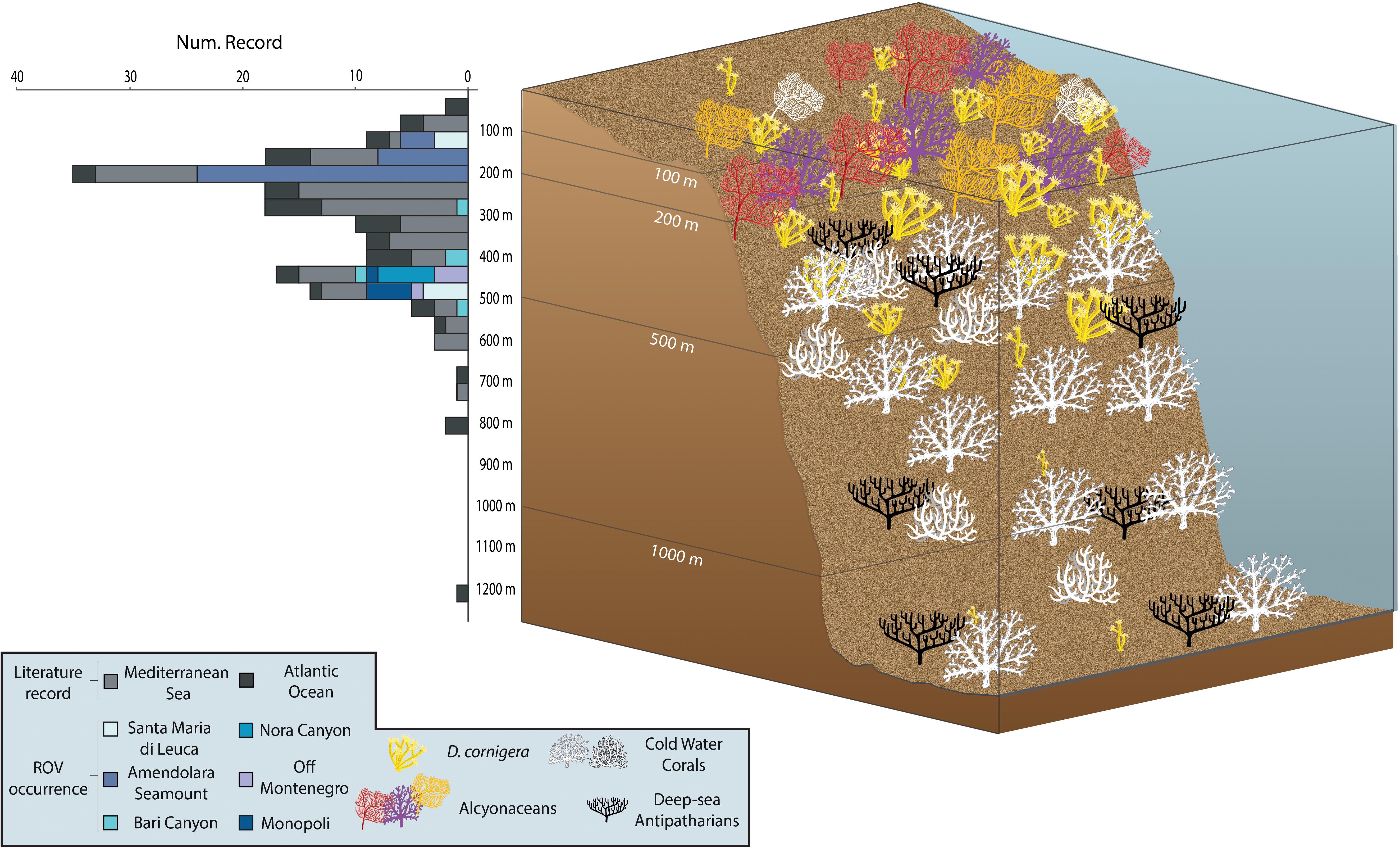
Figure 2. Bathymetric distribution of D. cornigera records. 3D schematic representation showing the bathymetric distribution, and the number of D. cornigera records from literature review and ROV analysis performed in this study, by depth range.
Literature Records
The selection of published documents reporting geographic and bathymetric locations of D. cornigera resulted in 36 publications, from 1948 to 2018, and a total of 120 coral records (Figure 1B). With the exception of two documents targeting both Mediterranean and Atlantic regions, the majority of the selected publications refer to the Mediterranean Sea (27 out of 36; Figure 1B and Supplementary Table S3). Overall, 81 D. cornigera occurrences are reported in the Mediterranean region, with a decreasing trend toward the eastern basin: 18 publications (57 records) for the western Mediterranean, 5 publications (16 records) for the central Mediterranean, and 6 publications (8 records) for the eastern Mediterranean, mainly in the Aegean Sea (Figure 1B).
The Mediterranean D. cornigera records encompass a bathymetric range between 70–100 m and 733 m (Figure 2 and Supplementary Table S2). The majority falls within 200–300 m depth (29 records), with fewer occurrences in the range 100–200 m (15 records), and 300–400 m (13 records).
A much lower number of records concerns the Atlantic Ocean, with 39 sites reported in 9 publications and a bathymetric range spanning from 30 to 1200 m (Figure 2). As for the Mediterranean Sea, a great part of the records (26 of 39) are located between 100 and 400 m depth, but with the highest value between 300 and 400 m depth (10 records).
Finally, we integrated the literature records with the new ROV occurrences from the present study, obtaining a total number of 181 D. cornigera records for the Atlantic-Mediterranean region.
Temperature Range
The seawater temperatures close to the coral sites show a wide variability ranging from ∼7°C to ∼17°C (Figure 3). More than 80% of the coral records occur between ∼13°C and ∼15°C and a second peak of abundance (ca. 15%) was detected between ∼10°C and ∼13°C (Figure 3A).
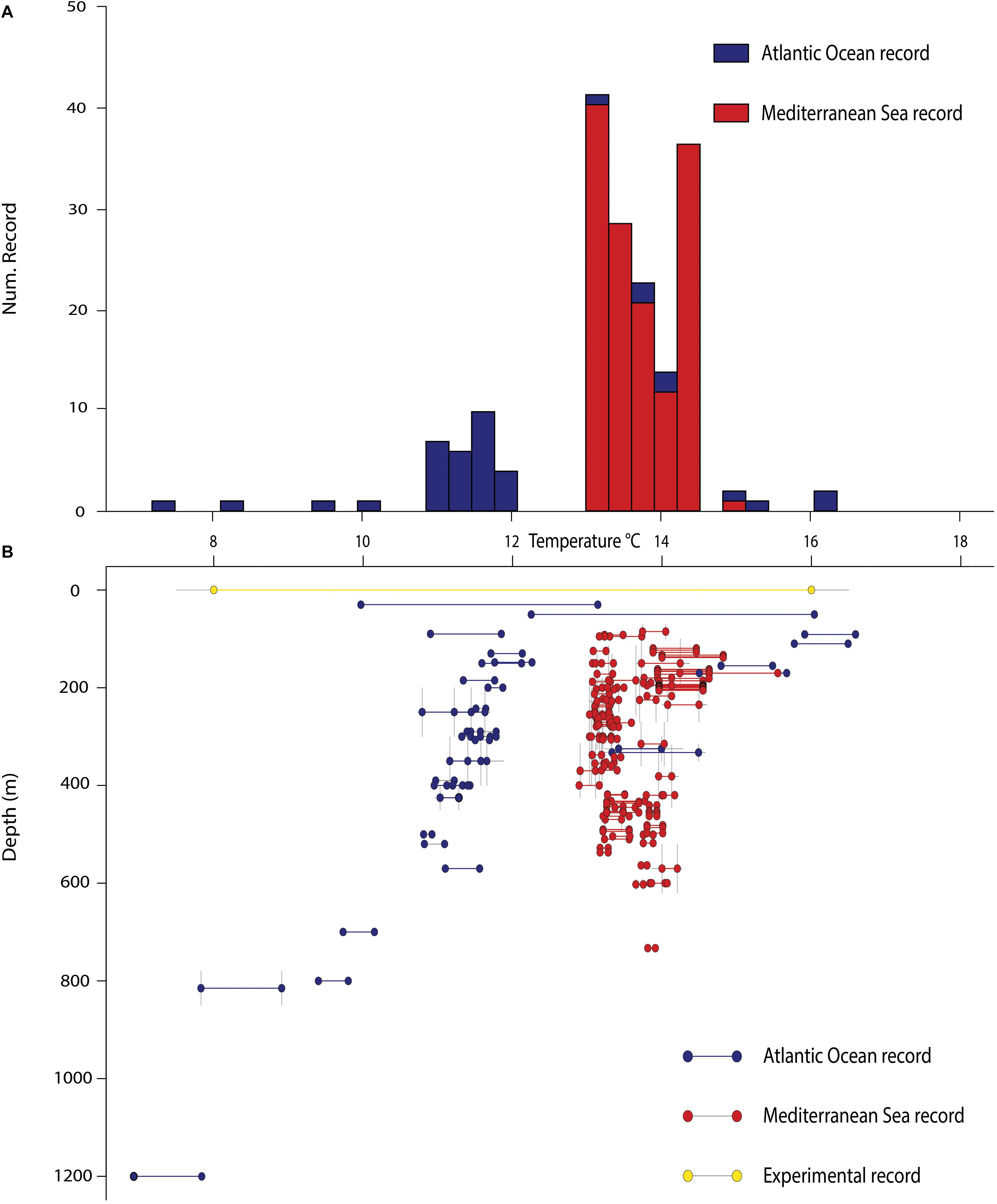
Figure 3. Frequency histogram and temperature annual variability of D. cornigera sites by water depth. (A) Number of D. cornigera records by temperature. (B) Temperature variability ranges defined by annual minimum and maximum values for each D. cornigera site and related with water depth. Yellow bar represents the temperature interval observed from in-tank experiment (Gori et al., 2014); horizontal gray lines represent the uncertainty value related with temperature, while the vertical ones stand for those ralated with depth.
Temperature values show a clear separation between the Atlantic and Mediterranean sites, with the exception of few sites located along the Moroccan coast and off the Azores (Figure 3B). The Mediterranean sites are characterized by well-defined temperature ranges, varying from 12.89°C to 15.55°C. Temperature variations at any individual site are wider at shallower depths and gradually decrease going deeper. The overall range of temperature is wider for the Atlantic sites (from 6.93°C in the Bay of Biscay at 1200 m to 16.59°C off the Azores at 91 m), and the temperature variation does not seem directly related to depth, with few deep sites showing up to 1°C seasonal amplitude.
Discussion
Despite D. cornigera being commonly recognized as an occasional species in CWCs ecosystems dominated by M. oculata and D. pertusum (Chimienti et al., 2019; Rueda et al., 2019, with references therein), corals of the genus Dendrophyllia are a consistent component of temperate coral ecosystems since at least the Miocene. Dendrophyllia corals from this geological period have been observed with high abundances in on-land Mediterranean CWCs fossil occurrences, being also present in the Pliocene and the Early-Middle Pleistocene records (Vertino et al., 2019). At the Pliocene-Pleistocene boundary, the diversity of dendrophylliids remarkably decreased, starting the “golden age” of Lophelia-dominated bioconstructions. To date, most of the D. cornigera rubble facies in the Mediterranean Sea date back to the Late Pleistocene, implying a greater development of this species in the recent geological time (Vertino et al., 2014).
Through the combined analysis of the new ROV videos and the available literature records, we obtained a comprehensive view of the modern geographic distribution of D. cornigera, which extends from the Azores and the eastern Atlantic coast to the whole Mediterranean Sea (Figure 1), in agreement with previously published information (Gori et al., 2014). The lower number of D. cornigera records in the southern Ionian Sea and the Levantine Basin could be an artifact reflecting the paucity of scientific publications on these areas compared to the available literature for the western Mediterranean Sea and the eastern Atlantic Ocean. In principle, this lack of information could bias the present-day D. cornigera distribution, with existing gaps not necessarily associated to unsuitable conditions.
The geographic and bathymetric distribution of temperate scleractinian corals reflects different environmental factors. Radiation levels (for zooxanthellate corals only), geomorphologic and hydrological conditions, seawater temperature and dissolved nutrient, and oxygen concentration are among the major drivers controlling the temperate coral growth (Roberts et al., 2009). In particular, seawater temperature seems to play a major role in determining and limiting the distribution of zooxanthellate and azooxanthellate scleractinian corals (Coma et al., 2009; Roberts et al., 2009; Kersting et al., 2013). C. caespitosa, a significant zooxanthellate scleractinian reef-builder in the Mediterranean Sea, populates shallow waters down to 40 m depth in a temperature range of ∼14–25°C (Montagna et al., 2007; Rodolfo-Metalpa et al., 2008). Several studies have investigated the environmental factors controlling C. caespitosa distribution. Under suboptimal conditions, such as high light intensity and irregular food source, C. caespitosa shows the ability to upregulate its heterotrophy and maintain the symbiosis, presenting light-adapted symbiotic zooxanthellae (Symbiodinium Clade A) (Rodolfo-Metalpa et al., 2008; Hoogenboom et al., 2010). Seawater temperature, on the contrary, clearly influences the distribution of C. caespitosa and causes bleaching events (Kersting et al., 2013).
Temperature also affects the distribution of azooxanthellate CWC, with most of the species living within ∼4–14°C temperature range (Roberts et al., 2006; Taviani et al., 2017). In the Mediterranean Sea, CWCs find suitable conditions to settle below ∼200 m depth, where the temperature is typically lower than 14°C. The near-homeothermic conditions of the deep Mediterranean Sea, that does not get colder than 12°C (Lejeusne et al., 2010), allow CWCs to populate the seabed over 1000 m depth (Mastrototaro et al., 2010).
Field observations and results from culturing experiments in aquaria reveal a higher thermal tolerance of D. cornigera compared to CWCs species. Studies testing the physiological responses of several CWCs to different temperatures show the absence of significant physiological changes in D. cornigera at temperatures up to 16°C (Gori et al., 2014). In situ annual temperature ranges are in line with these experimental results and confirm that this coral is well adapted to relatively warm temperature conditions (Figure 3). A further line of evidence comes from the bathymetric distribution of D. cornigera, which extends from 30 to 1200 m depth. The highest number of occurrences are observed between 100 and 200 m depth (53 coral sites) (Figure 2). These depths probably present the most suitable temperature conditions for the coral (from 13.80 ± 0.05°C to 14.35 ± 0.08°C in the Mediterranean Sea and from 12.89 ± 0.64°C to 13.47 ± 0.71°C in the Atlantic Ocean) (Figure 3A). Therefore, the tolerance to high temperature allows D. cornigera to settle at shallower depths, where temperatures are too warm for more sensitive temperate coral species.
A natural tolerance to warm conditions seems to be shared by corals in family Dendrophylliidae, genus Dendrophyllia. For instance, Dendrophyllia ramea, presents a patchy distribution in the Mediterranean Sea, having been observed in the Adriatic Sea (Kružić et al., 2002), and the Levantine Basin (Salomidi et al., 2010; Orejas et al., 2017). The environmental and ecological factors regulating the distribution of D. ramea are still poorly known, but available information suggest that this species preferentially thrives in the shallower layers of the water column (approximately from 20 to 150 m), thus requiring warmer conditions (Orejas et al., 2019). In addition, Eguchipsammia fistula (family Dendrophylliidae) has been reported in the Red Sea at intermediate depths (230–270 m) at 21.6°C and Dendrophyllia sp. even deeper (570–640 m) in the same basin at 21.5°C (Roder et al., 2013).
The bathymetric distribution of D. cornigera shows a notable peak of abundance between 300 and 400 m, with temperature ranging from 13.24 ± 0.05°C to 13.39 ± 0.05°C in the Mediterranean Sea and 11.73 ± 0.34°C to 12.14 ± 0.41°C in the Atlantic Ocean, indicating the capability of this coral to colonize also deeper and colder layers.
The Mediterranean Sea is experiencing a gradual warming, from the surface down to the deepest layers, as a result of global climate changes (Vargas-Yáñez et al., 2008). The IPCC documented an increasing trend in Mediterranean Sea surface temperature of 0.66°C from 1950 to 2009 (Pachauri et al., 2014). Current predictions, based on different Representative Concentration Pathways (RCPs), indicate a temperature increase from 0.5°C to 2.6°C for the end of the 21st century (Shaltout and Omstedt, 2014). The raise of seawater temperature over its actual range of variability will probably push temperate coral ecosystems beyond the range of natural temperature, potentially influencing their fitness and survival. In-tank experiments testing the response of C. caespitosa to warm conditions reported coral tissue necrosis or mortality appearing at 26–28°C (Kersting et al., 2013). The warming of the seawater temperature over the thermal tolerance of C. caespitosa at its distribution depth (from 0 to ∼40 m) may lead to an increase in the intensity and frequency of bleaching events (Rodolfo-Metalpa et al., 2006). Cladocora caespitosa cannot escape these warmer conditions moving deeper along the water column because of its specific trophic needs (i.e., radiation levels for photosynthesis). As a consequence, this warming might in principle reduce the extent of C. caespitosa reefs, enhancing the importance of coral ecosystems composed by species with higher thermal tolerance and playing a similar ecological role.
The increase in temperature is taking place also in the deep Mediterranean Sea. The most conservative predictions for the 2016–2035 period forecast a temperature increase of about 0.035°C per year for the 150–350 m depth layer (Ozer et al., 2017). Applying this rate of increase to the current thermal conditions of the D. cornigera sites between 150 and 350 m depth, the seawater temperature value will be ∼0.7°C warmer in 2035 (from 14.23 ± 0.04°C to 14.61 ± 0.07°C) than present-day (from 13.57 ± 0.04°C to 13.94 ± 0.07°C). Deep-sea warming will probably lead to the exceeding of CWCs thermal limits in the shallower part of their bathymetric range, strongly threatening their survival and influencing their distribution (Gori et al., 2014; Maier et al., 2019).
Global change influences several environmental drivers of paramount importance for temperate corals. Ocean acidification is thought to be a major threat to coral ecosystems, reducing the amount of carbonate ions [CO32–] available in seawater. Predictions anticipate that ca. 70% of known CWCs reefs will be exposed to waters corrosive to aragonite before the end of 21st century (Tittensor et al., 2010) up to evoking negative to counteractive scenarios for their eventual survival (Maier et al., 2011; McCulloch et al., 2012; Gammon et al., 2018). However, some studies reveal that net calcification rates, as well as dissolution rates of exposed skeleton and respiration rates of different deep-sea coral species, do not significantly change when exposed to high seawater pCO2, with D. cornigera showing no physiological alterations (Movilla et al., 2014; Rodolfo-Metalpa et al., 2015; Reynaud and Ferrier-Pagès, 2019).
Climate change may also affect nutrient concentrations and food sources, which are of primarily importance for CWCs distribution and survival (Lionello and Scarascia, 2018). The Mediterranean Sea is markedly oligotrophic and characterized by a strong stratification of the water column during summer, resulting in high temperatures, and low food availability (e.g., Zabala and Ballesteros, 1989). In deep-sea habitats, where food availability may be extremely heterogeneous in time and space (Mienis et al., 2009), benthic ecosystems are under strong nutritional forcing, leading to a “summer dormancy” in many benthic suspension feeders (Lejeusne et al., 2010). Global warming is intensifying water column stratification, producing a 40% lengthening of summer conditions (Coma et al., 2009) and influencing the fundamental biological processes that require high-energy investments, such as coral growth and reproduction. Opportunistic trophic behavior has been observed in D. pertusum (Mueller et al., 2014) and recent studies demonstrated a high trophic plasticity of D. cornigera, which favors the wide distribution of this coral in areas with variable food availability (Gori et al., 2018).
However, the big question remains: will the yellow coral D. cornigera have an edge in the race against global ocean warming? Our results suggest that the natural thermal tolerance of the mesophotic-bathyal coral D. cornigera will likely prove to be an advantage in the survival struggle in a progressively warming ocean. We anticipate that D. cornigera could experiment in principle an increase of its horizontal and vertical distribution range, perhaps even taking advantage in the long term of a concomitant decline of more thermal-sensitive reef-forming calcareous organisms.
Data Availability Statement
The raw data supporting the conclusions of this manuscript will be made available by the authors, without undue reservation, to any qualified researcher.
Author Contributions
GC analyzed the data and wrote the manuscript together with MT, LA, and PM. All authors contributed to the discussion and preparation of the manuscript.
Funding
This work was partly supported by the EU F.P. VII Projects HERMIONE (contract no. 226354), CoCoNet (contract no. 287844), MISTRALS/PALEOMEX/COFIMED and Convenzione MATTM-CNR per i Programmi di Monitoraggio per la Direttiva sulla Strategia Marina (MSFD, Art. 11, Dir. 2008/56/CE), and is part of the DG Environment programme IDEM (grant agreement no. 11.0661/2017/750680/SUB/EN V.C2) and the MIUR-PRIN GLIDE.
Conflict of Interest
The authors declare that the research was conducted in the absence of any commercial or financial relationships that could be construed as a potential conflict of interest.
Acknowledgments
We thank captain, crew, and scientific staff of R/V Urania and Minerva Uno cruises for their skillful and efficient cooperation during operations at sea (ISMAR-Bologna scientific contribution no. 2000).
Supplementary Material
The Supplementary Material for this article can be found online at: https://www.frontiersin.org/articles/10.3389/fmars.2019.00692/full#supplementary-material
References
Addamo, A. M., Vertino, A., Stolarski, J., García-Jiménez, R., Taviani, M., and Machordom, A. (2016). Merging scleractinian genera: the overwhelming genetic similarity between solitary Desmophyllum and colonial Lophelia. BMC Evol. Biol. 16:108. doi: 10.1186/s12862-016-0654-8
Altuna, A., and Poliseno, A. (2019). “Taxonomy, genetics and biodiversity of Mediterranean deep-sea corals and cold-water corals,” in Mediterranean Cold-Water Corals: Past, Present and Future, eds C. Orejas and C. Jiménez (Cham, CH: Springer International Publishing), 121–156. doi: 10.1007/978-3-319-91608-8_14
Angeletti, L., Taviani, M., Canese, S., Foglini, F., Mastrototaro, F., Argnani, A., et al. (2014). New deep-water cnidarian sites in the southern Adriatic Sea. Mediterr. Mar. Sci. 15, 263–273. doi: 10.12681/mms.558
Aymà, A., Aguzzi, J., Canals, M., Company, J. B., Lastras, G., Mecho, A., et al. (2019). “Occurrence of living cold-water corals at large depths within submarine canyons of the northwestern Mediterranean Sea,” in Mediterranean Cold-Water Corals: Past, Present and Future, eds C. Orejas and C. Jiménez (Cham, CH: Springer International Publishing), 271–284. doi: 10.1007/978-3-319-91608-8-26
Brooke, S., Ross, S. W., Bane, J. M., Seim, H. E., and Young, C. M. (2013). Temperature tolerance of the deep-sea coral Lophelia pertusa from the southeastern United States. Deep Sea Res. Part II 92, 240–248. doi: 10.1016/j.dsr2.2012.12.001
Chimienti, G., Bo, M., Taviani, M., and Mastrototaro, F. (2019). “Occurrence and biogeography of Mediterranean cold-water corals,” in Mediterranean Cold-Water Corals: Past, Present and Future, eds C. Orejas and C. Jiménez (Cham, CH: Springer International Publishing), 213–243. doi: 10.1007/978-3-319-91608-8_19
Coma, R., Ribes, M., Serrano, E., Jiménez, E., Salat, J., and Pascual, J. (2009). Global warming-enhanced stratification and mass mortality events in the Mediterranean. PNAS 106, 6176–6181. doi: 10.1073/pnas.0805801106
Corriero, G., Pierri, C., Mercurio, M., Marzano, C. N., Tarantini, S. O., Gravina, M. F., et al. (2019). A Mediterranean mesophotic coral reef built by non-symbiotic scleractinians. Sci. Rep. 9:3601. doi: 10.1038/s41598-019-40284-4
Gammon, M. J., Tracey, D. M., Marriott, P. M., Cummings, V. J., and Davy, S. K. (2018). The physiological response of the deep-sea coral Solenosmilia variabilis to ocean acidification. PeerJ 6:e5236. doi: 10.7717/peerj.5236
Gattuso, J. -P., Magnan, A., Billé, R., Cheung, W. W. L., Howes, E. L., Joos, F., et al. (2015). Contrasting futures for ocean and society from different anthropogenic CO2 emissions scenarios. Science 349:4722. doi: 10.1126/science.aac4722
Gori, A., Reynaud, S., Orejas, C., Gili, J. -M., and Ferrier-Pagès, C. (2014). Physiological performance of the cold-water coral Dendrophyllia cornigera reveals its preference for temperate environments. Coral Reefs 33, 665–674. doi: 10.1007/s00338-014-1167-9
Gori, A., Tolosa, I., Orejas, C., Rueda, L., Viladrich, N., Grinyó, J., et al. (2018). Biochemical composition of the cold-water coral Dendrophyllia cornigera under contrasting productivity regimes: insights from lipid biomarkers and compound-specific isotopes. Deep Sea Res. I 141, 106–117. doi: 10.1016/j.dsr.2018.08.010
Hoegh-Guldberg, O., Poloczanska, E. S., Skirving, W., and Dove, S. (2017). Coral reef ecosystems under climate change and ocean acidification. Front. Mar. Sci. 4:158. doi: 10.3389/fmars.2017.00158
Hoogenboom, M., Rodolfo-Metalpa, R., and Ferrier-Pagès, C. (2010). Co-variation between autotrophy and heterotrophy in the Mediterranean coral Cladocora caespitosa. J. Exp. Biol. 213, 2399–2409. doi: 10.1242/jeb.040147
Hooidonk, R. V., Maynard, J. A., Manzello, D., and Planes, S. (2014). Opposite latitudinal gradients in projected ocean acidification and bleaching impacts on coral reefs. Glob. Chang. Biol. 20, 103–112. doi: 10.1111/gcb.12394
Hughes, T. P., Kerry, J. T., Baird, A. H., Connolly, S. R., Dietzel, A., Eakin, C. M., et al. (2018). Global warming transforms coral reef assemblages. Nature 556, 492–496. doi: 10.1038/s41586-018-0041-2
Kersting, D. K., Bensoussan, N., and Linares, C. (2013). Long-term responses of the endemic reef- builder Cladocora caespitosa to Mediterranean warming. PLoS One 8:e70820. doi: 10.1371/journal.pone.0070820
Kružić, P., Zibrowius, H., and Pozar-Domac, A. (2002). Actiniaria and Scleractinia (Cnidaria, Anthozoa) from the Adriatic Sea (Croatia): first records, confirmed occurrences and significant range extensions of certain species. Ital. J. Zool. 69, 345–353. doi: 10.1080/11250000209356480
Lejeusne, C., Chevaldonné, P., Pergent-Martini, C., Boudouresque, C. F., and Pérez, T. (2010). Climate change effects on a miniature ocean: the highly diverse, highly impacted Mediterranean Sea. Trends Ecol. Evol. 25, 250–260. doi: 10.1016/j.tree.2009.10.009
Levin, L. A., and Bris, N. L. (2015). The deep ocean under climate change. Science 350, 766–768. doi: 10.1126/science.aad0126
Lionello, P., and Scarascia, L. (2018). The relation between climate change in the Mediterranean region and global warming. Reg. Environ. Chang. 18, 1481–1493. doi: 10.1007/s10113-018-1290-1
Locarnini, R. A., Mishonov, A. V., Antonov, J. I., Boyer, T. P., Garcia, H. E., Baranova, O. K., et al. (2013). World Ocean Atlas 2013, Volume 1: Temperature. Washington D.C: National Oceanic and Atmospheric Administration (NOAA). doi: 10.1007/s10113-018-1290-1
Lough, J. M., Anderson, K. D., and Hughes, T. P. (2018). Increasing thermal stress for tropical coral reefs: 1871–2017. Sci. Rep. 8:6079. doi: 10.1038/s41598-018-24530-9
Loya, Y., Puglise, K. A., and Bridge, T. C. L. (2018). Mesophotic Coral Ecosystems. Cham, CH: Springer International Publishing. doi: 10.1007/978-3-319-92735-0
Maier, C., Watremez, P., Taviani, M., Weinbauer, M. G., and Gattuso, J. P. (2011). Calcification rates and the effect of ocean acidification on Mediterranean cold-water corals. Proc. R. Soc. B Biol. Sci. 279, 1716–1723. doi: 10.1098/rspb.2011.1763
Maier, C., Weinbauer, M. G., and Gattuso, J.-P. (2019). “Fate of Mediterranean scleractinian cold-water corals as a result of global climate change. a synthesis,” in Mediterranean Cold-Water Corals: Past, Present and Future, eds C. Orejas and C. Jiménez (Cham, CH: Springer International Publishing), 517–529. doi: 10.1007/978-3-319-91608-8-44
Mastrototaro, F., D’Onghia, G., Corriero, G., Matarrese, A., Maiorano, P., Panetta, P., et al. (2010). Biodiversity of the white coral bank off Cape Santa Maria di Leuca (Mediterranean Sea): an update. Deep Sea Res. II 57, 412–430. doi: 10.1016/j.dsr2.2009.08.021
McCulloch, M., Falter, J., Trotter, J., and Montagna, P. (2012). Coral resilience to ocean acidification and global warming through pH up-regulation. Nat. Clim. Chang. 2, 623–627. doi: 10.1038/nclimate1473
Mienis, F., de Stigter, H. C., de Haas, H., and van Weering, T. C. E. (2009). Near-bed particle deposition and resuspension in a cold-water coral mound area at the Southwest Rockall Trough margin, NE Atlantic. Deep Sea Res. I 56, 1026–1038. doi: 10.1016/j.dsr.2009.01.006
Montagna, P., McCulloch, M., Mazzoli, C., Silenzi, S., and Odorico, R. (2007). The non-tropical coral Cladocora caespitosa as the new climate archive for the Mediterranean Sea: high-resolution (˜ weekly) trace element systematics. Quat. Sci. Rev. 26, 441–462. doi: 10.1016/j.quascirev.2006.09.008
Movilla, J., Orejas, C., Calvo, E., Gori, A., Lopez-Sanz, A., Grinyo, J., et al. (2014). Differential response of two Mediterranean cold-water coral species to ocean acidification. Coral Reefs 33, 675–686. doi: 10.1007/s00338-014-1159-9
Movilla, J. (2019). “A case study: variability in the calcification response of Mediterranean cold-water corals to ocean acidification,” in Mediterranean Cold-Water Corals: Past, Present and Future, eds C. Orejas and C. Jiménez (Cham, CH: Springer International Publishing), 531–533. doi: 10.1007/978-3-319-91608-8_45
Mueller, C. E., Larsson, A. I., Veuger, B., Middelburg, J. J., and Van Oevelen, D. (2014). Opportunistic feeding on various organic food sources by the cold-water coral Lophelia pertusa. Biogeosciences 11, 123–133. doi: 10.5194/bg-11-123-2014
Orejas, C., Ferrier-Pagès, C., Reynaud, S., Gori, A., Beraud, E., Tsounis, G., et al. (2011). Long-term growth rates of four Mediterranean cold-water coral species maintained in aquaria. Mar. Ecol. Prog. Ser. 429, 57–65. doi: 10.3354/meps09104
Orejas, C., Gori, A., Jiménez, C., Riviera, J., Lo Iacono, C., Hadjioannou, L., et al. (2017). First in situ documentation of a population of the coral Dendrophyllia ramea off cyprus (Levantine Sea) and evidence of human impacts. Galaxea CRS 19, 15–16. doi: 10.3755/galaxea.19.1_15
Orejas, C., Taviani, M., Ambroso, S., Andreou, V., Bilan, M., Bo, M., et al. (2019). “Cold-water corals in aquaria: advances and challenges. A focus on the Mediterranean,” in Mediterranean Cold-Water Corals: Past, Present and Future, eds C. Orejas and C. Jiménez (Cham, CH: Springer International Publishing), 435–471. doi: 10.1007/978-3-319-91608-8-38
Ozer, T., Gertman, I., Kress, N., Silverman, J., and Herut, B. (2017). Interannual thermohaline (1979–2014) and nutrient (2002–2014) dynamics in the Levantine surface and intermediate water masses, SE Mediterranean Sea. Glob. Planet. Chang. 151, 60–67. doi: 10.1016/j.gloplacha.2016.04.001
Pachauri, R. K., Allen, M. R., Barros, V. R., Broome, J., Cramer, W., Christ, R., et al. (2014). Climate Change 2014: Synthesis Report. Contribution of Working Groups I, II and III to the Fifth Assessment Report of the Intergovernmental Panel on Climate Change. Geneva: IPCC.
Poloczanska, E. S., Brown, C. J., Sydeman, W. J., Kiessling, W., Schoeman, D. S., Moore, P. J., et al. (2013). Global imprint of climate change on marine life. Nat. Clim. Chang. 3, 919–925. doi: 10.1038/nclimate1958
Puig, P., and Gili, J.-M. (2019). “Submarine canyons in the Mediterranean: a shelter for cold-water corals,” in Mediterranean Cold-Water Corals: Past, Present and Future, eds C. Orejas and C. Jiménez (Cham, CH: Springer International Publishing), 285–289. doi: 10.1007/978-3-319-91608-8_27
Reynaud, S., and Ferrier-Pagès, C. (2019). “Biology and ecophysiology of Mediterranean cold–water corals,” in Mediterranean Cold-Water Corals: Past, Present and Future, eds C. Orejas and C. Jiménez (Cham, CH: Springer International Publishing), 391–404. doi: 10.1007/978-3-319-91608-8-35
Roberts, J. M., Wheeler, A., Freiwald, A., and Cairns, S. (2009). Cold-Water Corals: The Biology and Geology of Deep-Sea Coral Habitats. Cambridge: Cambridge University Press.
Roberts, J. M., Wheeler, A. J., and Freiwald, A. (2006). Reefs of the deep: the biology and geology of cold-water coral ecosystems. Science 312, 543–547. doi: 10.1126/science.1119861
Roder, C., Berumen, M. L., Bouwmeester, J., Papathanassiou, E., Al-Suwailem, A., and Voolstra, C. R. (2013). First biological measurements of deep-sea corals from the Red Sea. Sci. Rep. 3:2802. doi: 10.1038/srep02802
Rodolfo-Metalpa, R., Montagna, P., Aliani, S., Borghini, M., Canese, S., Hall-Spencer, J. M., et al. (2015). Calcification is not the Achilles’ heel of cold-water corals in an acidifying ocean. Glob. Chang. Biol. 21, 2238–2248. doi: 10.1111/gcb.12867
Rodolfo-Metalpa, R., Peirano, A., Houlbrèque, F., Abbate, M., and Ferrier-Pagès, C. (2008). Effects of temperature, light and heterotrophy on the growth rate and budding of the temperate coral Cladocora caespitosa. Coral Reefs 27, 17–25. doi: 10.1007/s00338-007-0283-1
Rodolfo-Metalpa, R., Richard, C., Allemand, D., and Ferrier-Pagès, C. (2006). Growth and photosynthesis of two Mediterranean corals, Cladocora caespitosa and Oculina patagonica, under normal and elevated temperatures. J. Exp. Biol. 209, 4546–4556. doi: 10.1242/jeb.02550
Rueda, J. L., Urra, J., Aguilar, R., Angeletti, L., Bo, M., García-Ruiz, C., et al. (2019). “Cold-water coral associated fauna in the Mediterranean Sea and adjacent areas,” in Mediterranean Cold-Water Corals: Past, Present and Future, eds C. Orejas and C. Jiménez (Cham, CH: Springer International Publishing), 295–333. doi: 10.1007/978-3-319-91608-8_29
Salomidi, M., Zibrowius, H., Issaris, Y., and Milionis, K. (2010). Dendrophyllia in Greek waters, Mediterranean Sea, with the first record of D. ramea (Cnidaria, Scleractinia) from the area. Mediterr. Mar. Sci. 11, 189–194. doi: 10.12681/mms.102
Shaltout, M., and Omstedt, A. (2014). Recent sea surface temperature trends and future scenarios for the Mediterranean Sea. Oceanologia 56, 411–443. doi: 10.5697/oc.56-3.411
Taviani, M., Angeletti, L., Canese, S., Cannas, R., Cardone, F., Cau, A., et al. (2017). The “Sardinian cold-water coral province” in the context of the Mediterranean coral ecosystems. Deep Sea Res. II 145, 61–78. doi: 10.1016/j.dsr2.2015.12.008
Tittensor, D. P., Baco, A. R., Hall-Spencer, J. M., Orr, J. C., and Rogers, A. D. (2010). Seamounts as refugia from ocean acidification for cold-water stony corals. Mar. Ecol. 31, 212–225. doi: 10.1111/j.1439-0485.2010.00393.x
Vargas-Yáñez, M., Jesús García, M., Salat, J., García-Martínez, M. C., Pascual, J., and Moya, F. (2008). Warming trends and decadal variability in the Western Mediterranean shelf. Glob. Plan. Chang. 63, 177–184. doi: 10.1016/j.gloplacha.2007.09.001
Vertino, A., Stolarski, J., Bosellini, F. R., and Taviani, M. (2014). “Mediterranean corals through time: from miocene to present,” in The Mediterranean Sea: Its History and Present Challenges, eds S. Goffredo and Z. Dubinsky (Dordrecht: Springer), 257–274. doi: 10.1007/978-94-007-6704-1-14
Vertino, A., Taviani, M., and Corselli, C. (2019). “Spatio-temporal distribution of Mediterranean cold-water corals,” in Mediterranean Cold-Water Corals: Past, Present and Futureed, eds C. Orejas and C. Jiménez (Cham, CH: Springer International Publishing), 67–83. doi: 10.1007/978-3-319-91608-8_9
Keywords: coral ecosystems, Dendrophyllia cornigera, mesophotic zone, global change, coral survival, future ocean
Citation: Castellan G, Angeletti L, Taviani M and Montagna P (2019) The Yellow Coral Dendrophyllia cornigera in a Warming Ocean. Front. Mar. Sci. 6:692. doi: 10.3389/fmars.2019.00692
Received: 02 September 2019; Accepted: 28 October 2019;
Published: 08 November 2019.
Edited by:
Roberto Sandulli, Università degli Studi di Napoli Parthenope, ItalyReviewed by:
Christine Ferrier-Pagès, Scientific Centre of Monaco, MonacoGiorgio Bavestrello, University of Genoa, Italy
Copyright © 2019 Castellan, Angeletti, Taviani and Montagna. This is an open-access article distributed under the terms of the Creative Commons Attribution License (CC BY). The use, distribution or reproduction in other forums is permitted, provided the original author(s) and the copyright owner(s) are credited and that the original publication in this journal is cited, in accordance with accepted academic practice. No use, distribution or reproduction is permitted which does not comply with these terms.
*Correspondence: Lorenzo Angeletti, lorenzo.angeletti@bo.ismar.cnr.it