- 1Marine Research Centre, Finnish Environment Institute, Helsinki, Finland
- 2Dipartimento Di Scienze Della Vita E Dell'Ambiente, Università Politecnica delle Marche, Ancona, Italy
- 3Finnish Environment Institute, Jyväskylä Office, Jyväskylä, Finland
Contamination status of coastal areas of Finland (northern Baltic Sea) markedly affected by anthropogenic activities (harbors, shipyards and maritime activity, industry, municipal and agricultural inputs, legacy contamination) was assessed for the first time using the weight of evidence (WOE) approach. The key element of the study was the caging (transplantation) of Baltic mussels (Mytilus trossulus) for the measurement of tissue accumulation of polycyclic aromatic hydrocarbons (PAHs) and applying a suite of biomarkers of biological effects of contaminants. Additional variables included in the assessment were trace metals in seawater, macrozoobenthos, near-bottom oxygen levels and eutrophication indicators. The chemical parameters were supported by passive sampling of PAHs and organotins at the study sites. The integrated approach combining all the line of evidence (LOE) variables into the WOE showed separation of some sites as more affected by hazardous substances than others, with the most contaminated areas found around harbor and ship yard areas. The contaminant levels measured in the different matrices were not alarmingly high at none of the areas compared to many other areas within or outside the Baltic Sea under more heavy anthropogenic impact, rarely exceeding any given threshold values for Good Environmental Status of the EU Marine Strategy Framework Directive. However, significant biological effects were recorded in mussels in the most contaminated sites, signifying that the combined effects caused by the contaminants and other environmental factors are disturbing the health of marine organisms in the area. The results of this successful combined application based on the mussel transplantation method and the WOE approach are highly encouraging for further trials in developing the monitoring of chemical contamination in the Baltic Sea.
Introduction
Mussels belonging to the genus Mytilus are globally used bioindicator organisms in marine pollution monitoring (see e.g., recent reviews by Beyer et al., 2017; Krishnakumar et al., 2018, and references within). By efficiently filtering large amounts of water and using waterborne particles as food they become exposed to extensive amounts of various types of hazardous substances in the dissolved form or as bound to particles (Viarengo and Canesi, 1991). In the low-diversity brackish-water ecosystem of the Baltic Sea the Baltic mussel (Mytilus trossulus) is an ecological key species (Koivisto and Westerbom, 2010; Väinölä and Strelkov, 2011), which has been used in biomonitoring and case studies concerning chemical contamination (e.g., Baršienė et al., 2006; Kopecka et al., 2006; Lehtonen et al., 2006a; Schiedek et al., 2006). By applying the caging approach, i.e., transplantation of bioindicator organisms, it is possible to expose them in sites of interest along a pollution gradient or hot spots; this approach has been successfully undertaken in various sea areas (e.g., Andral et al., 2004, 2011; Regoli et al., 2004; Smolders et al., 2004; Damiens et al., 2007; Tsangaris et al., 2010; Serafim et al., 2011; Marigómez et al., 2013; Lekube et al., 2014; Moschino et al., 2016), and also in the Baltic Sea (Rank et al., 2007; Dabrowska et al., 2013; Turja et al., 2013, 2014, 2015; Lehtonen et al., 2016).
In the current study, mussels were transplanted in 2016 and 2017 in cages along the Baltic Sea coast of Finland outside 10 main cities with significant ports to assess the contamination status of the areas. The cages were deployed for ca. two months and the mussels were examined for selected biomarker responses [antioxidative defense system (ADS), biotransformation, and neurotoxicity], and for the accumulation of polycyclic aromatic hydrocarbons (PAH) and organotin compounds. In addition, passive samplers were deployed in the cages for the detection of the aforementioned groups of compounds. As biomarkers of the ADS response and damage caused by oxidative stress enzymatic activities of catalase (CAT) and glutathione reductase (GR), and the level of lipid peroxidation (LPO) were measured in the digestive gland of mussels. Phase II biotransformation enzyme glutathione S-transferase (GST), also involved in ADS, was determined in the same tissue. Activity of acetylcholinesterase (AChE), an indicator of neurotoxic effects, was measured in the gill tissue. A morphometric body condition index (CI) was determined as a background parameter for the nutritional status of the mussels.
Polymer based passive samplers are increasingly used in studying harmful substances in different environmental matrices (Smedes et al., 2009; Rusina et al., 2010; Tucca et al., 2014; Pintado-Herrera et al., 2016). They enable the determination of low concentrations of hydrophobic substances which in grab water samples remain below the detection limit. The samplers measure only freely dissolved chemical concentration which can penetrate through cell walls and be harmful to organisms (Mayer et al., 2003; Alvarez et al., 2004; Vrana et al., 2005). In this study, passive samplers were deployed at half of the mussel caging sites for the additional detection of PAH and organotin compounds.
A classical weight of evidence (WOE) elaboration was applied to integrate all the data for a holistic assessment of the health status of the study sites. The WOE approach combines data from different typologies of investigations, or lines of evidence (LOEs), and typically integrates chemical results with assessment of various biological effects (Piva et al., 2011; Benedetti et al., 2012). Individual LOEs are independently elaborated to summarize specific Hazard Quotients (HQs) before their final integration when a different weight is given according to their ecological relevance. Among the advantages of a similar approach is the possibility to discriminate differences between evaluations from various typologies of studies. Despite the choice of the most appropriate LOEs depends on local objectives and specificities, WOE studies have been increasingly adopted for environmental quality characterization and as a fundamental component within Ecological Risk Assessment (ERA) strategies and recommended within recent European directives for evaluation of ecological status through multiple quality indicators (Benedetti et al., 2012).
In the present work, a WOE investigation was applied integrating chemical characterization of water (LOE1), bioaccumulation (LOE2), and biomarkers (LOE3) in caged mussels, benthic community status (LOE4). Data on oxygen level and eutrophication conditions were further considered and integrated as additional LOEs (LOE5 and LOE6) in the final WOE elaboration.
Materials and Methods
Study Sites, Collection of Mussels, and the Caging Set-Up
Mussels for the caging experiment were collected by scuba diving in Hanko, Finland (Figure 1) in May in 2016 and 2017 and transported in ambient seawater in thermo-insulated boxes to the Marine Research Laboratory of the Finnish Environment Institute (SYKE) in Helsinki. Mussels with shell length of 25–30 mm were selected and epibionts were removed from the shell surfaces. The mussels were kept at 10°C for 2 days in aerated water collected from the sampling site. In 2016, the cagings were done at five sites outside the cities of Kotka (site depth: 21 m), Helsinki (21 m), Parainen (38 m), Pori (23 m), and Vaasa (24 m) (five sites), and in 2017 at five sites at Porvoo (17 m), Hanko (28 m), Naantali (15 m), Uusikaupunki (18 m), and Rauma (13 m) (Table 1). The deployment sites covered the whole area of natural occurrence of the species in Finnish coastal waters with variability in some environmental factors. Salinity ranged from around 4.5 (Kotka) to 6.3 (Hanko) at the Gulf of Finland sites, from 4.7 to 5.2 at the Archipelago Sea sites, and from 5.8 (Uusikaupunki) to 5.0 (Vaasa) at the Bothnian Sea sites.
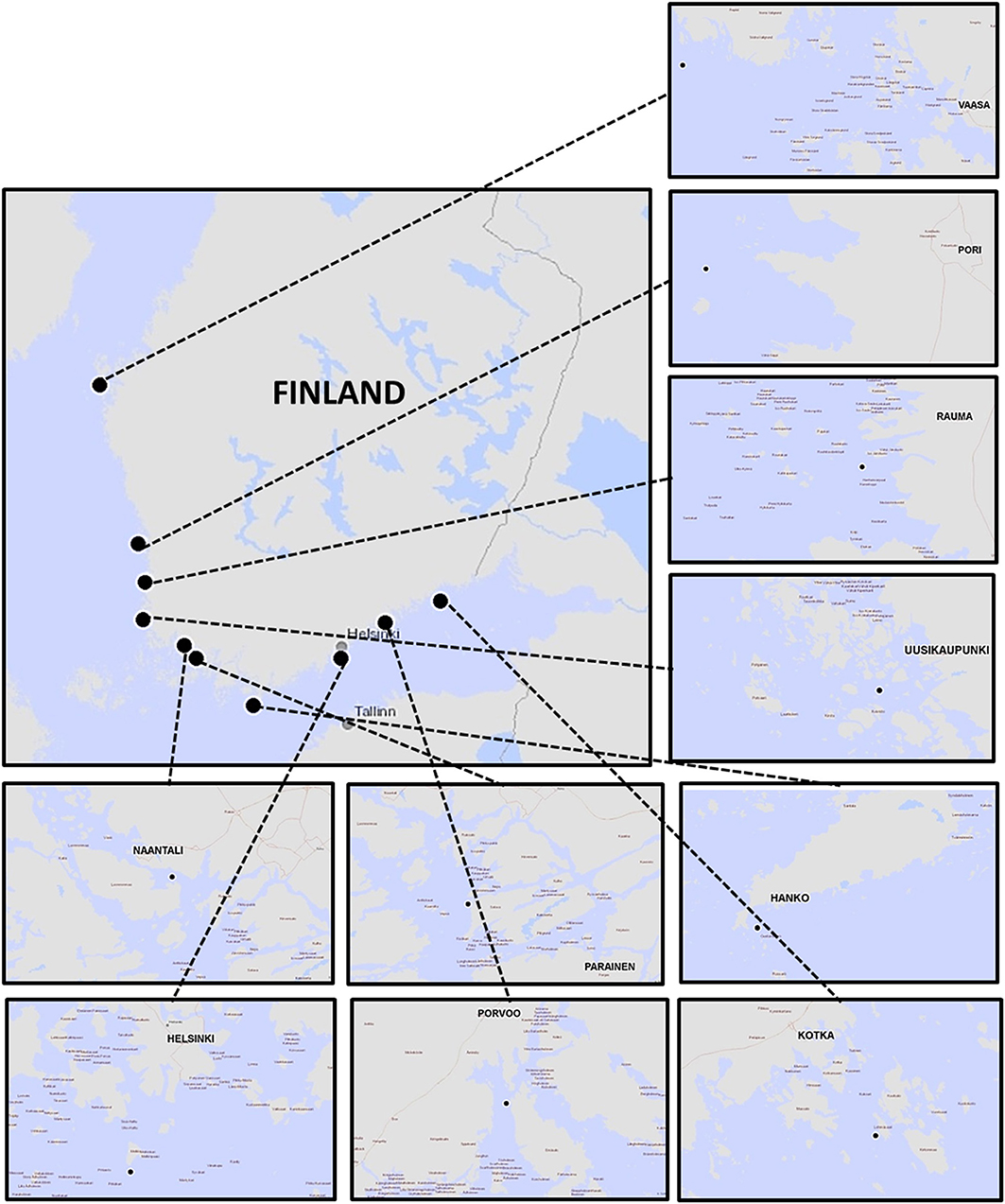
Figure 1. Study sites along the coast of Finland. The black dot in each site map represent the exact site of the mussel cage.
Mussels were placed inside the cages for ca. 8–9 weeks until recovery in late July. In this sea area the major spawning period of mussels is early summer (Benito et al., 2019). Although the general seasonal reproduction pattern is quite clear, the exact timing is dependent on various environmental factors (e.g., nutrition and temperature) that differ between locations, even on a small scale. In addition, even within a population it is common to observe individuals in a different spawning stage. Thus, by the end of the exposure period the mussels at all the study sites had most likely recovered from spawning stress.
The mussel cages were constructed of stainless steel and had a rectangular shape (height 60 cm, width 40 × 40 cm). Within the frame structure there were five easily removable steel net boxes (mesh size of 10 × 10 mm) in which the mussels (ca. 200 individuals per cage) were randomly distributed. The cages were deployed at the water depth of 7–8 m, anchored to the bottom with a rope attached to an object weighing ca. 70 kg, and held in a stable vertical position by submerged buoys. For maritime safety, each cage was marked with a 4 m ODAS buoy equipped with radar reflectors and a light blinking at 20 s intervals.
After the recovery of the cages the mussels were immediately dissected aboard the vessel for biomarker and chemical analyses. Digestive gland and gill tissues were taken for the various enzymatic biomarker analyses (n = 15 individuals), snap-frozen in liquid nitrogen and later stored at −80°C until analysis. Shell length of individual mussels was measured for the morphometric condition index (CI) determination (n = 15). For chemical contamination measurements the whole soft tissue was dissected from 60 to 120 individuals after a depuration period of 4–5 h. These samples were pooled (2–4 pools) and stored in glass vials in a −20°C freezer.
Biomarker Measurements
ADS, Biotransformation Phase II Activity, and Neurotoxicity
The ADS response was assessed by measuring the enzymatic activity of CAT, GR, and SOD as well as the level of lipid peroxidation (LPO). GST was measured for biotransformation Phase II activity, and acetylcholinesterase activity (AChE) was measured to assess neurotoxicity. For the enzymatic assays, digestive glands of mussels (n = 15) were homogenized in potassium phosphate buffer (100 mM, pH 7.4) and gills (n = 15) in sodium phosphate buffer (200 mM, pH 7.0) containing 0.1% Triton-X.
GST activity was estimated by measuring the formation rate of the conjugated substrate [chlorodinitrobenzene (CNDB)-glutathione (GSH)] at 340 nm (Habig et al., 1974). Final concentrations of 1 mM CNDB (Sigma 237329) and 1 mM GSH (Sigma G6529) in potassium phosphate buffer (100 mM, pH 7.0) were used in the reaction. CAT activity was measured as CAT mediated degradation of hydrogen peroxide (H2O2) at 240 nm (Claiborne, 1985). The reaction mixture contained 4.3 μM H2O2 (Fluka 95302) in potassium phosphate buffer (100 mM, pH 7.0). GR activity was measured indirectly as consumption of NADPH in the reduction of oxidized glutathione (GSSG) (Carlberg and Mannervik, 1975). The reaction mixture contained 2 mM EDTA (Sigma E5134), 0.5 mM GSSG (Sigma G4376), and 0.1 mM NADPH (Sigma N7505) in potassium phosphate buffer (100 mM, pH 7.5). Levels of LPO were measured as the generation of thiobarbituric acid reactive substances (TBARS) (Ohkawa et al., 1979). The reaction mixture contained 0.24 M trichloroacetic acid (Riedel de Haën 33731), 60 mM Tris-HCl with 0.1 mM DTPA and 16 mM 2-thiobarbituric acid (Sigma T5500). The amount of TBARS was measured by reading absorbance at 535 nm. Analyses of AChE activity were performed from gill samples as described in Bocquené and Galgani (1998) with modifications as in Leiniö and Lehtonen (2005).
All the enzymatic assays, LPO, and protein content (Bradford, 1976) used for the calculation of specific enzymatic activities were measured in 96-well microplates using the TECAN Infinite 200 (TECAN) spectrophotometer with Magellan software.
Morphometric Condition Index
The CI determined in mussels (n = 15) using the formula CI = (soft tissue dry weight [mg]/shell length [mm]2) * 100.
Contaminant Measurements in Soft Tissues of Mussels
PAH Compounds
Pooled samples of mussel soft tissue were homogenized and 5–10 g was taken for the extraction. Internal (Fluoro-PAHs, Chiron) and yield PAH standards were added to the homogenate. Five milliliter of water and 10 ml of ethylacetate were added and the samples were shaken for 1 min. A salt mixture consisting of 4 g MgSO4 and 2 g NaCl was added. The samples were again shaken for 1 min and then centrifuged for 10 min. Five milliliters of the ethylacetate extract supernatant was taken and 200 μl of isooctane added to the extract. Ethylacetate was evaporated under a nitrogen flow and 1 ml of hexane was added. The extract was purified in a column containing glass wool, Na2SO4, and silica. The PAH compounds, now in hexane, were eluted with hexane/dichloromethane (3:1, v/v). After elution 0.5 ml isooctane was added as solvent keeper and the solvent was evaporated under a gentle flow of nitrogen until a final volume of 0.5 ml was reached. Ten microliters of injection standards (deuterated PAHs, Dr. Ehrenstorfer) were added and the sample was analyzed with a Thermo GC-MS/MS (Trace 1310 GC Ultra gas chromatograph and TSQ Quantum XLS ultra mass spectrometer). Measurements were done with selected reaction monitoring (SRM) mode. Identification of the PAH compounds was done by selecting two typical fragment ions.
Organotins
From samples collected in 2017, organotins were extracted with acetic acid from freeze-dried homogenized mussel tissue samples to ether-hexane mixture tropolone for complexation. The tissues were broken down with tetramethylammoniumhydroxide prior to extraction. Ethyl derivatives of the compounds were formed using Na-tetraethylborate. The derivatized extracts were cleaned in columns containing activated aluminum oxide using ether-hexane solution as the eluent. The compounds determined were monobutyltin (MTB), DBT, TBT, monophenyltin (MPhT), DThT, triphenyltin (TPhT), and dioctyltin (DOT). For quantitation, the perdeuterated analog of each compound was used as an internal standard, and added to the samples at the beginning of the procedure except for DOT for which perdeuterated DPhT was used. Calibration samples, two zero samples and two control samples treated similarly to the actual samples were analyzed for each sample series. The compounds were determined by using gas chromatography (Hewlett Packard 6890) with high resolution mass spectrometer (Autospec Ultima) using HP-1 capillary capillary column (J&W Scientific: 12 m, i.d. 0.20 mm, 0.33 μm). The detection limits of the method are 0.1–1.1 ng g wet wt.−1 depending on the compound. The method is accredited.
Contaminant Measurements Using Passive Samplers
Again only in 2017, polydimethylsiloxane (PDMS) passive samplers (SSP-M823-010, area 90 × 55 mm, thickness 250 μm) were attached to the cages placed at five sites to assess the concentrations of waterborne PAHs and organotins. Two samplers were applied at each caging site. At the time of retrieval the samplers were rinsed with ultrapure water, wrapped in aluminum foil and transferred to the laboratory in a tightly closed container. The samplers were stored in a freezer (−20°C) until extraction. After deployment the organotins were extracted from the samplers with acetic acid to ether-hexane which contained tropolone. The compounds were ethylated with sodium tetraethyl borate, cleaned with activated aluminum oxide column and eluted with ether-hexane. Organotins were analyzed with GC-MS/MS (Agilent 7010, column J&W Scientific, length 12 m, i.d. 0.20 mm, film thickness 0.33 μm) using multiple reaction monitoring (MRM).
For PAH analyses the samplers were shaken in methanol for 24 h and the extract was evaporated to a smaller volume. The extract was cleaned with EPH cartridge (Biotage) and the PAH compounds were eluted with dichloromethane, which was again evaporated to a smaller volume and exchanged to cyclohexane and analyzed with GC/MS. The PDMS samplers are deployed until the equilibrium between the sampler and the surrounding medium has been reached (Smedes et al., 2009). The average concentration of studied chemical in seawater during the deployment can be calculated if the sampler-water partition (Ks, w) coefficient is known. Ks, w between PMDS passive sampler and TBT, diphenyltin (DPhT) and dibutyltin (DBT) has been determined in a laboratory experiment (Ahkola et al., 2018).
Determination of Trace Metals in Water
The seawater samples were preserved with concentrated nitric acid (super purity grade) as 150 μl per 30 ml sample. The samples were diluted 5x before the measurement. The internal standard elements (Rh, Ir) were added on-line using the ICP-MS peristaltic pump. To remove spectral interferences ICP-MS measurement was performed in KED (Kinetic Energy Discrimination) mode using helium as collision gas. Concentrations of the following trace metals are reported here: arsene (As), cadmium (Cd), cobalt (Co), chromium (Cr), copper (Cu), nickel (Ni), lead (Pb), and zinc (Zn).
Additional Data
The HERTTA open environmental database of SYKE containing the national monitoring data was utilized to acquire data on macrozoobenthos in areas in the vicinity of the mussel caging sites. Data from sampling stations in these areas (1–12, depending on site) were elaborated to the brackish water benthic index (BBI; Perus et al., 2007) which offers a salinity-corrected tool for classification of the soft-bottom zoobenthos under the demands of the European Union Water Framework Directive (EU WFD). Near-bottom (1 m above the seafloor) oxygen concentrations were also collected from the HERTTA database. Status classifications of nitrogen (N), phosphorus (P) and chlorophyll a (chla) in the water column were taken from the EU WFD assessments to represent the eutrophication status of the caging areas. As the status classification (“Bad,” “Poor,” “Moderate,” “Good,” “High”) is calculated for a limited coastal waterbody area from several stations and based on 6-year means of concentrations in the upper 5-m surface waters in the summer months, the obtained assessment status is only a robust estimate over these highly dynamic variables.
Statistical Analysis
The SYSTAT™ software were used to calculate the statistics. All data were tested for the normality and homogeneity of variance with Bartlett's test. One-way ANOVA followed by Tukey's post hoc HSD multiple comparisons. Principal Component Analysis (PCA) was used for exploratory analysis of interactions between individual PAH compounds measured in the tissues of mussels and the biomarkers applied.
The WOE Approach
Data on water chemistry, bioaccumulation and variations of biomarkers in caged mussels as well as benthic community status were elaborated within the WOE model, which summarizes hazard indices for various LOEs in specific modules before their overall integration. The computation rules of the WOE model have been successfully applied to classify environmental hazards in different conditions of origin, typology, and intensity of pollution, including highly and moderately contaminated sites from industrial areas, harbors, brackish environments, and the Costa Concordia shipwreck on the Italian coast (Piva et al., 2011; Benedetti et al., 2012, 2014; Regoli et al., 2014; Bebianno et al., 2015), and most recently in the assessment of environmental effects of offshore oil platforms in the Adriatic Sea (Regoli et al., 2019) In the latter paper, full and updated descriptions of the LOE/WOE calculation methodologies are presented.
Briefly, the evaluation of chemical hazard in LOE1 is initially based on the calculation for each pollutant of Ratio to Reference (RTR), i.e., the ratio between concentration measured in seawater and relative threshold values which included lower EAC and EC from OSPAR (2004) and Danish EPA 1182/2007 levels. From the calculated ratio to reference, a RTRw is obtained by the application of a correction factor (w) which, depending to the typology of chemicals, ranges from 1 to 1.3 for “non priority” (w = 1), “priority” (w = 1.1) or “priority and hazardous” pollutants (w = 1.3) according to EC Directive 2008/105 (EC, 2008). In the calculation of the specific HQ for chemistry (HQC), an average RTRw is obtained for all of the parameters with RTR <1 (i.e., values below the SQG), while for those with RTR >1, the RTRw are individually added into the summation Σ:
The values of HQC are assigned to one class of chemical hazard (absent or negligible, slight, moderate, major, severe) depending on the number, typology and magnitude of exceeding chemicals (see Piva et al., 2011 for detailed thresholds levels).
For the elaboration of chemical results in caged mussels (LOE2), the RTRw is calculated for each parameter as the increase of concentration compared to control specimens, corrected for the typology of pollutant and the statistical significance of the difference, and assigned to one of five classes of effect. The cumulative HQ for bioavailability (HQBA) does not consider parameters with RTRw <1.3 (concentrations ≤ control value for a priority and hazardous pollutants), calculates the average for those with RTRw ranging between 1.3 and 2.6 (i.e., up to 2-fold increase compared to controls for a priority and hazardous pollutant), and adds the summation (Σ) of all those with RTRw ≥2.6):
The level of cumulative HQBA is summarized in one class of hazard for bioavailability, from “Absent” to “Severe,” depending on the distribution of analyzed chemicals within the different classes of effect (Regoli et al., 2014).
Results on biomarkers are elaborated (LOE3) considering a “weight” based on the toxicological relevance of each biological endpoint, and a “threshold” indicating the minimum variation considered of biological significance for various responses. For every analyzed biomarker, the measured variation is compared to the specific threshold, then corrected for the weight of the response and the statistical significance of the difference compared to controls. Each biomarker response is assigned by the model to 1 of 5 classes of effect (from “Absent” to “Severe”); the calculation of the HQ for biomarkers (HQBM) does not consider the contribution of responses with an effect lower or equal to threshold (“Absent” or “Slight”), calculates the average for those with an effect up to 2-fold compared to the threshold (“Moderate”) and adds the summation (Σ) for the responses more than 2-fold greater than the respective threshold, i.e., “Major” or “Severe” (Regoli et al., 2014):
According to variations measured for various biomarkers the model summarizes the level of cumulative HQBM in one of five classes of hazard for biomarkers, from “Absent” to “Severe” (Regoli et al., 2014).
For benthic communities (LOE4), using the approach of Perus et al. (2007) for the classification of BBI, the interval of values between 0 and the threshold “Not-good status/Good status” has been divided in two equal classes, respectively assigned to level of hazard “Major” and “Severe.” Similarly, the interval of values ranging between the threshold “Not-good status/Good status” and one has been divided in three classes corresponding to a level of hazard “Moderate” (with BBI ranging within the first 30% of the interval threshold-to-1), “Slight” (in the following 35% of the interval threshold-to-1) and “Absent” (in the last 35% of the interval threshold-to-1). The samples of each site were initially processed individually to calculate the HQ and level of hazard: the HQ of single samples were then averaged to obtain the mean hazard level for each site.
For the LOE on oxygen levels (LOE5), considering the values indicated as the threshold “Good”-“Moderate” (4 mg l−1), threshold “Moderate”-“Bad” (2 mg l−1), the minimum oxygen level (0 mg l−1) and typically higher level of oxygen in investigated sites (10 mg l−1), the following classes of hazard have been considered for hypoxic conditions: 0–1 mg/L, HQ “Severe”; 1–2 mg l−1, HQ “Major”; 2–4 mg l−1, HQ “Moderate”; 4–7 mg l−1, HQ “Slight”; 7– ≥10 mg l−1, HQ “Absent”.
A “eutrophication index” (LOE6) was calculated combining and differently weighting the evaluations of “chla status” (weight 40%), “P status” (30%) and “N status” (30%).
The elaborations of results from individual water chemistry, bioaccumulation, and biomarkers in caged mussels, benthic communities, oxygen levels, and eutrophication status were finally integrated in the WOE assessment, giving a different weight to various lines of evidence, to summarize an overall WOE index, finally assigned to one of five classes of risk from “Absent” to “Severe” (Piva et al., 2011).
Results
Trace Metals in Seawater
Concentrations of As, Cd, Co, Cr, Cu, Ni, Pb, and Zn at the caging sites are presented in Table 2. Only values of Zn (five stations) and Cu (1) exceeded the water quality threshold values derived from literature (Table 2). However, below these threshold levels significant variability was recorded, between the sites, especially in regard to Co (up to ca. 20 times differences), Cr (up to 100 times) and Pb (up to ca. 40 times). When these data were elaborated according to weighted criteria, the summarized level of hazard was “Absent” for Kotka, Porvoo, Hanko and Pori, “Slight” in Helsinki, Parainen, Uusikaupunki and Vaasa, “Moderate” in Naantali and Rauma. At all these stations the parameter contributing most to the calculated HQ was Zn (Table 3).
PAHs and Organotins in Mussel Tissues
Uusikaupunki, Pori and Vaasa emerged as the sites with clearly lower total PAH tissue levels compared to the others (9.5–29.7 μg kg−1, Figure 2). Mussels in Kotka showed the highest total tissue concentrations of PAHs (96.6 μg kg−1) with Hanko and Naantali coming up next (88.5 and 86.0 μg kg−1, respectively). Mussels in Kotka and Parainen showed a quite different PAH compound profile compared to the others with proportionately higher share of benzo compounds (61 and 53% of total, respectively) while these were not recorded at all at stations Porvoo, Hanko, Naantali, Uusikaupunki, and Pori. Another notable distinction was the presence of methylated naphthalenes, which were very prominent at the sites characterized by low PAH tissue levels, namely Pori (100% of total) and Vaasa (49%) but completely absent at Porvoo, Naantali, Uusikaupunki, and Rauma.
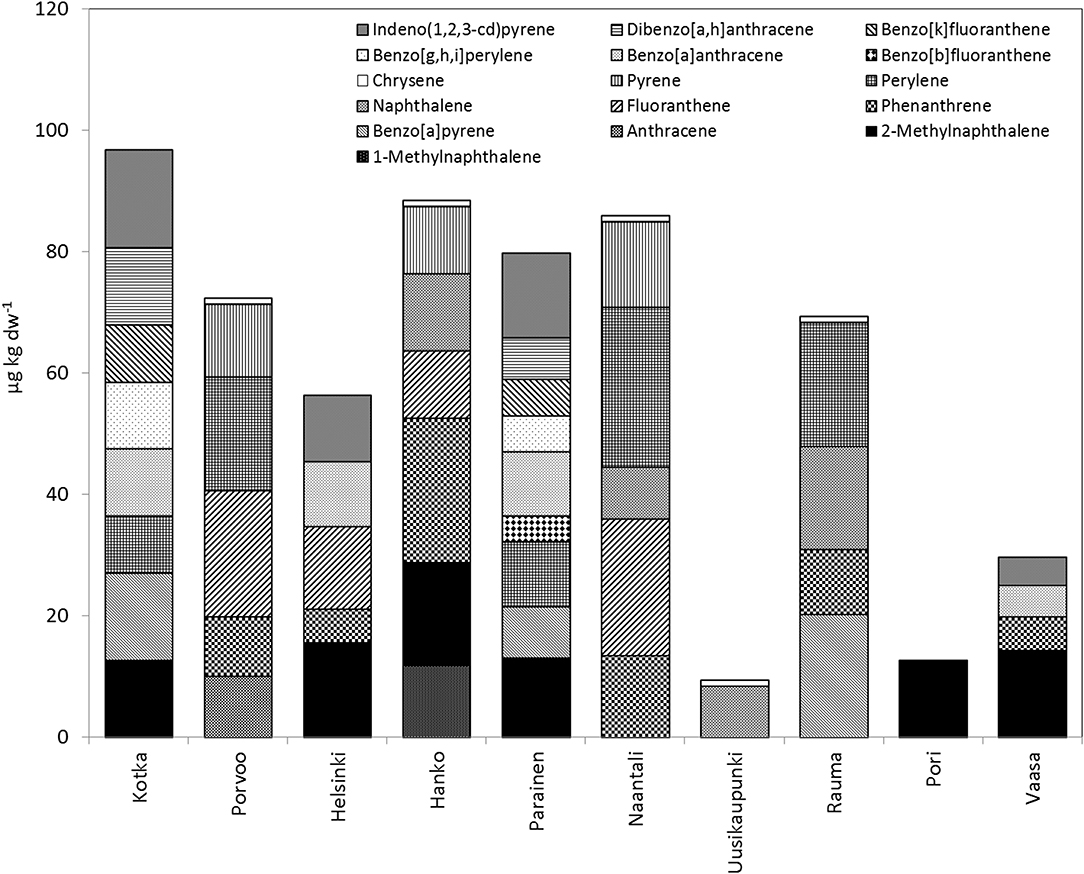
Figure 2. PAH compounds (μg kg dry wt.−1) in soft tissues of caged mussels from the study sites. Fluorene, acenaphthene, acenaphthylene, and benzo[e]pyrene were not detected in any of the samples.
The levels of organotins were measured only in mussels caged in 2017 at five stations, being clearly highest in mussels caged in Naantali with 223 μg kg dry wt.−1 of total organotins, which of 80% consisting of TBT (Figure 3). For the rest of the sites the organotin levels were between 18.2 (Hanko) and 54.3 μg kg dry wt.−1 (Porvoo). TPhT was recorded at every site with the levels ranging from 4.3 (Rauma) to 18.3 μg kg dry wt.−1 (Porvoo). DBT was measured only in mussels caged at the Porvoo site (16.6 μg kg dry wt.−1).
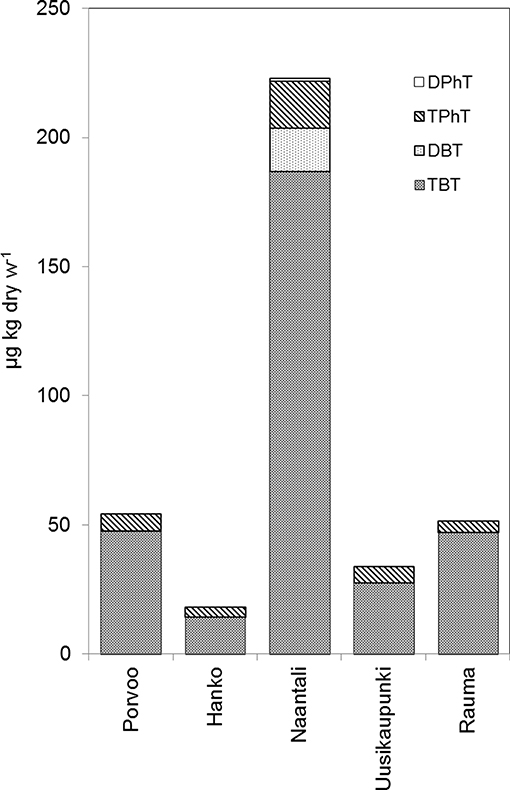
Figure 3. Organotins (μg kg dry wt.−1) in soft tissues of caged mussels from five study sites in 2017. MBT, MPhT, and DOT were not detected in any of the samples.
The weighted elaboration of bioaccumulation data provided a clear discrimination between the sampling areas with a level of hazard ranging from “Absent”/“Slight” in Pori, Vaasa, Uusikaupunki, and Helsinki to “Moderate” in Hanko and “Major” for mussels caged in Kotka, Porvoo, Parainen, Naantali, and Rauma (Table 3). Perylene, methylnaphatalenes, benzo[a]pyrene (B[a]P), and organotins were the compounds contributing most to the “Major” bioavailability hazard.
PAHs and Organotins in Passive Samplers
Concentrations of PAHs and organotins in PDMS passive samplers were measured only in 2017 at five caging sites. The total concentration patterns of both groups of compounds coincided generally well with those measured in mussel tissues (data not shown). The μg kg sampler−1 concentrations can be converted to ng l−1 or pg l−1 when the sampler-water partition coefficient Ks, w is known for the used chemical and the PDMS sampler type. The partition coefficients for certain PAHs (anthracene, acenaphthene, B[a]P, chrysene, fluoranthene, fluorene, naphthalene, phenanthrene, pyrene), and organotins (TBT, TPhT, DBT) are available from the literature (Smedes et al., 2009; Ahkola et al., 2018). As the partition coefficient depends on the characteristics of the studied compound the average concentrations during the sampling period differ from the μg kg sampler−1 ones. With PAHs the partition factors differ between 3.10 and 5.92 (naphthalene–B[a]P), which means that naphthalene prefers to water phase more than B[a]P. High concentrations of naphthalene were observed at all study sites despite it did not stand out when the μg kg sampler−1 amounts were studied. The highest concentrations of PAHs were found at Porvoo and Uusikaupunki but due to the lack of the Ks, w all the detected compounds are not included in this calculation (Figure 4). The results suggest that the dissolved fraction collected by passive samplers differs markedly from the one bioaccumulated by mussels.
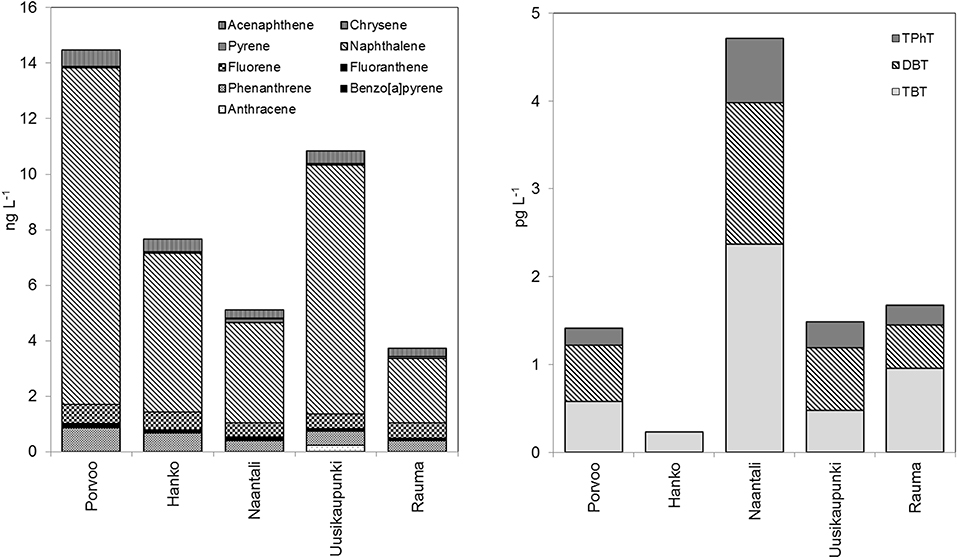
Figure 4. Seawater concentrations of PAHs (ng L−1) and organotins (pg L−1) calculated from accumulated amounts in passive samplers depolyed at five mussel caging sites in 2017.
The profile of total organotin concentrations was quite alike to that measured in mussels, implying that the bioaccumulation of organotins to mussels and PDMS samplers is more similar than in case of PAHs. Similar to the mussel tissue samples the peak concentrations of total organotins in passive samplers were measured in Naantali and the lowest ones in Hanko. TBT was discovered at all five sampling sites and TPhT and DBT from four sites except in Hanko, which also showed a “Moderate” bioavailability hazard level (Table 3). TBT was again clearly the prominent organotin compound.
Biomarker Responses
Marked differences were observed in the measured biomarkers (Figure 5), some coinciding with higher concentrations of the measured compounds in mussel tissues and passive samplers. ANOVA and Tukey post hoc statistics on differences between biomarker responses between the study stations are presented in Table 4, showing significant differences between the sites.
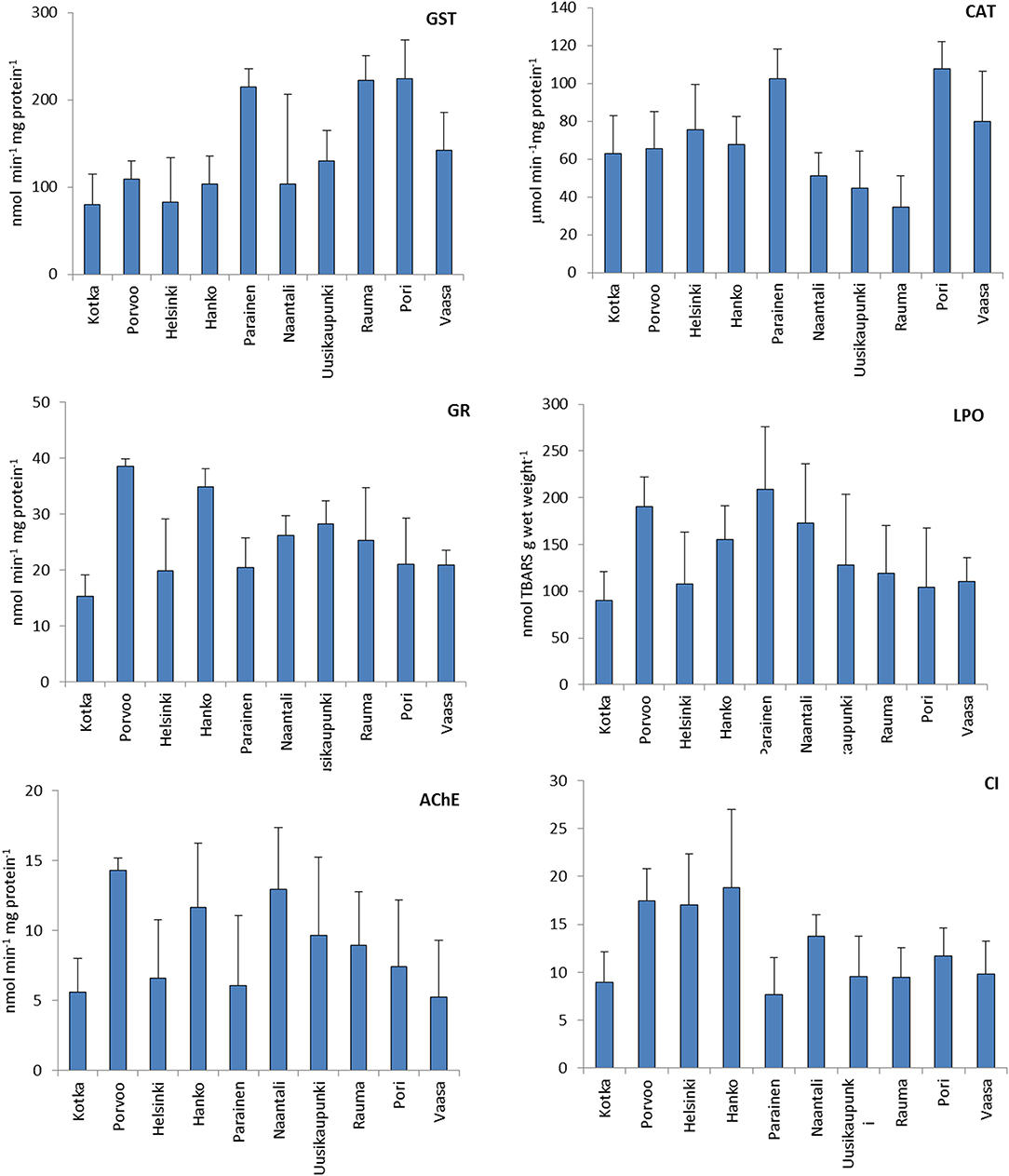
Figure 5. Biomarkers (mean ± SD) measured in caged mussels from the study sites. GST, glutathione S-transferase; CAT, catalase (CAT); GR, glutathione reductase; LPO, lipid peroxidation; AChE, acetylcholinesterase; CI, condition index. Statistical significances: see Table 3.
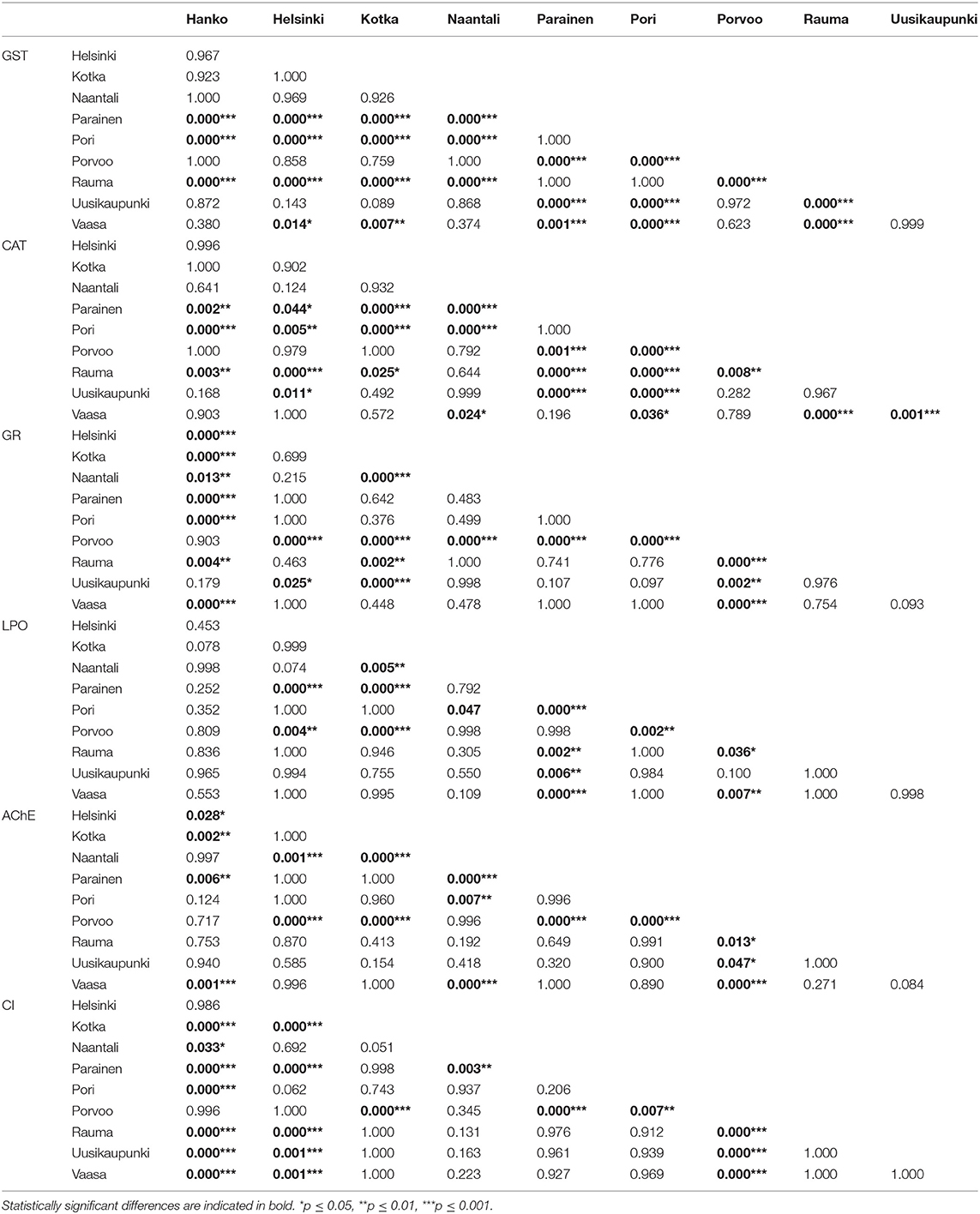
Table 4. ANOVA performed on each biomarker at the different study locations with post hoc Tukey's HSD multiple comparisons.
An exploratory PCA examining the connections between the bioaccumulation of the different PAHs and biomarker responses are presented in Figure 6. The results show clear connections between the tissue levels of certain groups of PAH compound types (methylated naphthalanes, benzo compounds, and “classic” parent PAHs), and the biomarker responses.
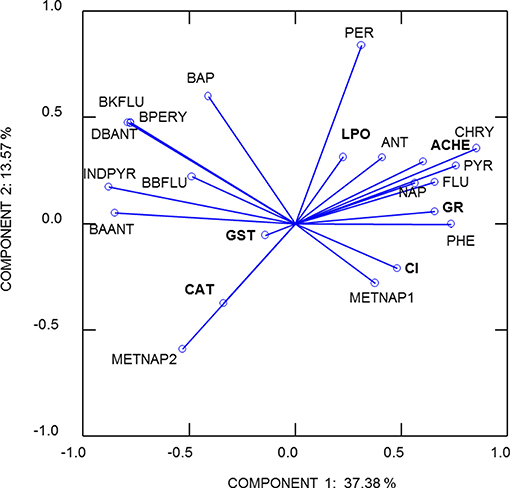
Figure 6. Principal Component Analysis (PCA) on biomarkers and PAH compounds. GST, glutathione S-transferase; CAT, catalase; GR, glutathione reductase; LPO, lipid peroxidation; AChE, acetylcholinesterase; CI, condition index; METNAP1, 1-methylnaphthalene; METNAP2, 2-methylnaphthalene; ANT, anthracene; BAP, benzo[a]pyrene; PHE, phenanthrene; FLU, fluoranthene; NAP, naphthalene; PER, perylene; PYR, pyrene; CHRY, chrysene; BBFLU, benzo[b]fluoranthene; BAANT, benzo[a]anthracene; BPERY, benzo[g,h,i]perylene; BKFLU, benzo[k]fluoranthene; DBANT, dibenzo[a,h]anthracene; INDPYR, indeno(1,2,3-cd)pyrene.
According to the toxicological relevance and the magnitude of variations observed for each biomarker, the overall significance of observed variations was summarized in a HQ “Absent” or “Slight” for Pori, Vaasa, Rauma and Parainen, and “Moderate” for all the other stations (Table 3).
Supporting Data: Macrozoobenthos, Near-Bottom Oxygen, and Eutrophication Status
From the obtained BBI values the level of hazard elaborated for benthic communities was “Absent” or “Slight” for Uusikaupunki, Rauma, Helsinki, “Moderate” for Porvoo, Hanko, Parainen, Naantali, and “Major” for Kotka (Table 3). The oxygen levels corresponded to an hypoxic hazard level summarized as “Absent” for all the stations except for Porvoo and Uusikaupunki (“Slight”). Eutrophication hazard obtained from the results on P, N, and chla ranged from “Slight” (Pori and Vaasa), “Moderate” (Uusikaupunki, Rauma, and Hanko) to “Major” (all the other sites, Table 3).
Application of the WOE Approach
In the conclusive WOE elaboration, the HQs obtained from various LOEs were normalized to a common scale and integrated giving them a different weight according to the ecological relevance of each typology of analyses. The weights assigned in this study to various LOEs were 0.9 for trace metal analyses of seawater, 1.2 for bioavailability in transplanted mussels, 1.0 for biomarkers, 1.3 for benthic communities, 0.5 for oxygen, and 0.5 for eutrophication. A large number of heterogeneous analytical results were summarized in a WOE evaluation, which discriminated various sites increasing from “Slight” (Pori, Uusikaupunki, Vaasa, Helsinki, Hanko) to “Moderate” (Parainen, Rauma, Porvoo, Naantali, and Kotka) (Table 3). The overall class derived from a combination of specific HQs ranging from “Absent”/“Slight” to “Moderate” (WOE “Slight”) or from “Absent”/ “Slight” to “Major” (WOE “Moderate”).
Discussion
Holistic interpretation of data on contaminant concentrations, biomarker responses, and various environmental conditions is typically highly complex. This study shows that the WOE approach provides robust support for the understanding and communicating the results. In combination with the presenting of the actual results it also provides transparency of the basis of the interpretation.
Site-Specific Differences in Chemical Hazard and Bioaccumulation
Examining the map, different types of coastal environments where the cagings were performed can be seen, showing open sea sites with less coastal anthropogenic impacts, sites deep inside a dense archipelago close to major harbors, maritime and near-shore industrial activities, and something between these. The first category includes Hanko, Uusikaupunki, Pori and Vaasa, while the rest belong to mainly to the second one. It should also be understood that the exact placing of the cages in the study areas has an obvious effect on the results since there are specific point sources and various environmental gradients in each area. For a comprehensive and detailed study, the number of caging units per area should be manifold to be able to characterize the area in a smaller scale. In addition, in some cases the deployment of the cages exactly to the locations foreseen as optimal in regard to the assessment of local pollution was not possible due to factors such as the proximity of major ship routes, shallowness, and the presence of underwater rocks dangerous to surface navigation of the research vessel. However, since mussels filter seawater very effectively and the it is moving along horizontal and vertical currents (considering also strong resuspension in shallow coastal areas especially impacted by intensive ship traffic) the mussels in a caging unit actually represent the average conditions within a quite large area. Nevertheless, the results from this study should not be taken as exact indicators of local conditions especially in the more open areas that are obviously less impacted by near-coast activities.
Chemical characterization using the concentrations of trace metals in seawater did not show any extreme situations in any of the study areas. Regarding Zn, values exceeding the given threshold of 1.1 μg l−1 were observed at half (5) of the sites. The highest values above the threshold by five times was recorded at Rauma and is probably related to high resuspension of sediments mainly due to ongoing dredging operations in the harbor area during the time of caging; this may also be reason for the occurrence of some other contaminants in passive samplers and in mussel tissues. Rauma was also the only site where the threshold for Cu was exceeded. For Zn, the second highest value (2.8 μg l−1) recorded in Naantali could be associated also to a constantly high sediment resuspension due to the extensive maritime traffic in the area, consisting of oil tankers to the large oil terminal as well as daily passenger ferries between Finland and Sweden. The nearby Parainen, also within the same dense achipelago with limited water exchange, is also strongly affected by ferry traffic and the resulting sediment resuspension; these two sites are characterized by higher concentrations of most of the measured trace metals in seawater which are likely derived from the resuspended sediments. Earlier studies on mussels, both native (Lehtonen et al., 2006a) and caged mussels (Lehtonen et al., 2016) in the area have shown a contamination gradient in the region with the inner part being more polluted. Obviously, there is a large number of other groups of contaminants that are also released from sediments during resuspension; if the trace metals indeed originate from this matrix they act as a proxy for the overall exposure to other contaminants as well. One of the groups is organotins (mainly TBT), which in Naantali showed by far the highest concentrations both in mussel tissues (>4-fold higher levels compared to Rauma and Porvoo) as well as in passive samplers (3-fold). In this study, sediment particles introduced to the water phase by resuspension appear to be the most likely source of organotins detected both in caged mussels and passive samplers.
In general, the total PAH levels measured in this study, ranging from 9.5 to 96.9 ng g−1 dry wt., coincide very well with the earlier studies in coastal areas of Finland (Turja et al., 2013, 2014, 2015; Lehtonen et al., 2016). However, they are significantly lower than those measured e.g., in the Northern Atlantic (∑PAH16 72–2217 ng g−1 dry wt; EU H2020 GRACE project, unpublished data). Examining the bioaccumulation of PAH compounds in the caged mussels, the cities of Vaasa, Pori and Uusikaupunki, all along the Bothnia Sea coast, stand out as the least polluted by these compounds. Between Pori and Uusikaupunki there is Rauma, where the resuspension related to the dredging activities mentioned above may have caused the elevated tissue levels not observed at the other Bothnian Sea sites, with high-molecular weight compounds B[a]P and perylene comprising an unusually high share of 59% of total PAHs. Mussels caged in Rauma contained no detectable levels of methylated naphthalenes, which were in turn commonly observed at the other sites and at relatively marked shares of total PAHs, e.g., in the nearby Pori 2-methylnaphthalenes formed 100% of the measured PAHs.
Notably, mussels caged near the large oil terminals in Porvoo and Naantali did not contain methylated naphthalenes, which are often connected to oil. Instead, phenanthrene, fluoranthene, perylene, and pyrene formed 61 and 76% of total PAHs at these sites (respectively), much more than in the other areas. With the exception of anthracene (in Porvoo) and naphthalene (in Naantali) the PAH profiles were identical and this is quite likely connected to the nearby main environmental stressors, identified as oil industry and transport, and maritime traffic.
The PAH profiles at Kotka and Parainen are quite different compared to other sites. The total levels are similar to some other sites but the share of benzo compounds reaches 61 and 53%, respectively. In comparison, no benzo compounds were detected in mussels at half (5) of the study sites. Compared to the three other sites in the Gulf of Finland (Porvoo, Helsinki and Hanko) no bioaccumlation of phenanthrene and fluoranthene, both relatively abundant at all these sites, could be detected in mussels. The Kotka region under the influence of the large Kymijoki is notoriously known for its high contamination by the abundant pulp mill industry and one of the rivers is one of the hotspots for dioxins (HELCOM, 2010). The riverborne contaminants have greatly polluted also the coastal sediments with various types of substances, including PAHs. The highly different PAH profile especially compared to the other Gulf of Finland caging sites suggest a different exposure scheme in the Kotka sea region, possibly related to the high industrial activities of the past.
The caging site at Hanko is next to the open sea and affected mainly by maritime traffic. Methylated naphthalenes consisted a significant share (33%) of the bioaccumulated PAH compounds in mussels. Mussels caged near Helsinki, another open-sea site greatly affected by traffic but also by other sources related to the metropolitan area, contained 28% of these compounds. Taking in account that in Pori and Vaasa, the sites with the lowest PAH contamination, the shares of methylated naphthalenes was largest (up to 100%) it may be considered that these sites are significantly less affected by other types of PAHs, e.g., those released to the water column via intensive resuspension of highly contaminated sediments such as found in Naantali, Parainen, and Kotka.
High tissue concentrations (on a regional scale) of phenanthrene in mussels collected from the Hanko region have been recorded also in other studies (Turja et al., 2014; Lehtonen et al., 2016; Turja et al. submitted manuscript). Also, elevated concentrations of methyl phenanthrenes (not measured here) have been observed (Turja et al., submitted manuscript), possibly connected to the anomalous concentrations of methyl naphthalenes measured in the present study. Thus, although the overall assessment of the Hanko site does not indicate any great environmental concern, these two single indicators show that further studies are needed in this area, especially since the observations already cover a period of over 10 years.
The PDMS passive samplers measure only freely dissolved chemical fraction so the concentrations determined with field deployed passive sampler are lower than the total ones. The average concentrations could be determined only to certain PAHs and organotins for which sampler water partition coefficients exist. The environmental quality standard (EQS) TBT in surface water is very low (0.2 ng L−1) and in most cases near the laboratory detection limit (EC, 2008). With PDMS passive sampler the presence of TBT in aquatic environment can be detected in much lower concentrations than before, which further enables a more reliable risk assessment.
Site-Specific Differences in Biomarkers
When examining the results on biomarker responses it is of key importance to take into account and understand the effects of factors not related to chemical contamination such as salinity and food availability (eutrophication status) that vary greatly between the study areas. Both the Gulf of Finland and the Archipelago Sea are considered highly eutrophicated areas while the Bothnian Sea suffers much less from these problems (HELCOM, 2018).
In this part of the Baltic Sea mussels live at the absolute limits of salinity tolerance (e.g., Westerbom et al., 2002); this has crucial implications on their growth and general physiology, which in turn may, at some point, lead to increased susceptibility to pollution insults or the ability to respond to pollution with protective cellular mechanisms. Although the salinity differences might appear small (2–3 per mille) compared to full-marine areas, they are crucially important at this critical range. In addition, salinity conditions in the Baltic Sea are stable, e.g., estuarine areas are naturally lower in salinity and devoid of any marine-originated brackish water adapted biota, and seasonal variations through ice melt and river discharge are low. In case of mussels transplanted in the most low-saline sites in the eastern Gulf of Finland (Kotka, Porvoo, Helsinki) and Bothnian Sea (Pori, Vaasa) the effects of extreme salinity conditions and energy-demanding osmotic stress can affect some of the biomarkers.
While high eutrophication typically is a serious environmental problem, in the case of caged mussels in this short-term exposure it can be considered as a positive growth-enhancing factor. In the present study the difference in eutrophication status is reflected in the significantly higher CI values of mussels transplanted in the Gulf of Finland (Porvoo, Helsinki, Hanko) compared to the Bothnian Sea (axis Uusikaupunki-Vaasa), indicating poorer growth opportunities in the latter area. The observation that most of the Bothnian Sea sites were less contaminated with PAHs (with the exception of Rauma discussed earlier) compared with the whole Gulf of Finland the low CI is indeed caused by nutrition or salinity stress. The abundant food conditions in the Gulf of Finland “balance out” the higher contaminant stress in the region so that the mussels are able to grow and attain energy needed for the defense mechanisms; however, in the case of Kotka it appears that not even the good food conditions are sufficient to counteract the combined effects of elevated contamination levels and low salinity. At Parainen and Naantali in the inner Archipelago Sea food is abundant and also salinity is more tolerable, and the markedly lower CI values compared to Porvoo, Helsinki and Hanko are likely due to contaminant stress.
Despite of the use of AChE activity as a specific indicator of exposure to some pesticicides (e.g., Bocquené and Galgani, 1998) it has been recently used also as an indicator of non-specific stress since it responds to a variety of chemicals and other factors such as trace metals, hydrocarbons, detergents, and algal toxins (Zinkl et al., 1991; Payne et al., 1996; Guilhermino et al., 1998; Lehtonen et al., 2003, respectively). The lowest values are measured at the low-salinity ends, Kotka and Vaasa, the former considered polluted and the latter among the cleanest ones of the study sites. Thus, salinity obviously affects mussels in these low-salinity areas. The PCA carried out on PAHs and biomarkers shows clear separation of groups of bioaccumulated PAH compounds and their interrelationships with selected biomarker responses. An interesting result is that at the sites where the mussels were characterized by zero bioaccumulation of benzo compounds (Porvoo, Hanko, and Naantali) they also had a significantly higher AChE activity. The most relevant pairwise comparison can be made with Naantali and Parainen, relatively close by and similar environmental conditions, with mussels containing no benzo compounds at the former site having a significantly higher AChE activity compared to the those at the latter having a share of 42% of their PAHs as these types. The total tissue PAH concentrations were similar at both sites, 79.9 and 86 μg kg dry wt.−1, respectively for Parainen and Naantali. An important observation is also that at the low-salinity Kotka site the mussels showed high levels of benzo compounds and a very low AChE activity.
Still focusing on the sites with high benzo compound bioaccumulation and especially the Archipelago Sea site pair Naantali and Parainen, the activity of GST is 2-fold higher at the latter site characterized by the high content and share of benzo compounds. This explicitly indicates an elevated detoxification and phase II biotransformation of these compounds. In Kotka the bioaccumulation of benzo compounds was also high but as can be depicted from a very low AChE activity the mussels are apparently unable to respond to the challenge since GST activity is also very low. Conclusively, the contaminant load jointly with threshold-level salinity presents the mussels caged at the Kotka site a too challenging situation to cope with. The results obtained here obviously call for more detailed investigations on the relationships of the bioaccumulation of different PAH compounds and biomarkers measured in mussels under field exposure conditions.
WOE Elaboration
A typical challenge in multidisciplinary biomonitoring is the difficulty to summarize the overall significance of heterogeneous results to better evaluate and quantify environmental impacts and risks on both geographical and temporal scales. This work corroborates previous findings that the criteria of a quantitative WOE approach reflect an important advancement in such risk assessment procedures. Various typologies of results (i.e., the LOEs) are independently elaborated through logical flowcharts and mathematical algorithms, which provide synthetic indices of hazard for each of the considered LOEs, before their final integration in a quantitative WOE evaluation (Piva et al., 2011). The hazards for water chemistry and bioavailability in caged mussels were based on the number, magnitude and potential toxicity of chemicals exceeding accepted quality guidelines or natural concentrations in reference organisms, while the evaluation of the biomarker considered, for each parameter, is based on the toxicological relevance of the measured endpoint and the magnitude of variations compared to specific thresholds. Benthic community data were elaborated to provide a commonly accepted ecological descriptor, and additional indices on oxygen and eutrophication were included in the overall integration. Among the advantages of a similar WOE approach is the possibility to discriminate differences between evaluations from various typologies of studies: elevated chemical loads in environmental matrices are not necessarily bioavailable and, at the same time, unexpected biological effects are caused by low levels of pollutants acting through synergistic mechanisms. Another relevant feature of this WOE approach is the weighted elaboration of data, allowing to abandon the “pass-to-fail” approach where even a single parameter slightly below or above a threshold would determine the chemical classification. Similarly, biological evaluations are not based on variations of individual responses, thus providing a more integrated assessment of the ecotoxicological or ecological hazards. As recently shown in a complex monitoring scenario of off-shore platforms (Regoli et al., 2019), this study confirmed the practical importance of a transparent procedure combining a scientifically sound approach with the possibility to synthesize the overall significance of the results obtained. Concerning the different typologies of the results obtained in the present study on the coastline of Finland the WOE integration summarized a “Slight” environmental impact in half of the study areas (Vaasa, Pori, Uusikaupunki, Hanko, and Helsinki) and “Moderate” in the other ones, none of the sites showing “Major” or “Severe” impacts. Conclusively, the approach provides elaborated hazard indices in a user-friendly format useful to support a more comprehensive process of risk assessment and “site-oriented” management decisions.
Conclusions
The key message of this study is that the use of mussel caging and WOE is an effective and practical method for evaluating the contamination status of marine coastal areas in the Baltic Sea. Both are novel methods in this region; caging of bivalves has so far been tested in research projects only (e.g., Rank et al., 2007; Dabrowska et al., 2013; Turja et al., 2013, 2014, 2015; Lehtonen et al., 2016; Kholodkevich et al., 2017) but it has not been included in regular monitoring programmes in any of the surrounding countries. The current study was targeted at developing the caging methodology and assessing its efficiency in the coastal waters of Finland and the results show convincingly that a fully integrated field sampling methodology including simultaneous chemical and biological effects parameters (biomarkers) is a cost-efficient and effective methodology. The application of biomarkers and other biological effects methods has gained more ground in the monitoring of marine pollution (Cajaraville et al., 2000; Hagger et al., 2009; Lam, 2009) and has been recommended based on the results of large research programmes carried out also in the Baltic Sea (Lehtonen et al., 2006b, 2014). Combined with the WOE approach an integrated assessment on the status of chemical contamination can be obtained and also linked with other environmental indicators (community analyses, eutrophication, hydrography) a more holistic assessment can be achieved, showing more clearly the linkages between contamination between hazardous substances and other stressors, both natural and anthropogenic. An integrated chemical-biological monitoring yields significantly more information on the contamination status of aquatic environments compared to the chemistry-only based approach, presenting early warning signals of biological effects and also mixture/multistressor effects that would remain undiscovered without using biomarkers. However, due to the complex biological and physico-chemical interactions biomarker results measured in field populations (also caged organisms) should always be carefully examined to ensure correct data interpretation.
Data Availability Statement
The datasets generated for this study are available on request to the corresponding author.
Author Contributions
KL, HA, TK, and AL contributed conception and design of the study. All authors contributed to the organization of the database, wrote sections of the manuscript, and contributed to manuscript revision, read and approved the submitted version. KL, GE, and FR performed the statistical analysis. KL wrote the first draft of the manuscript.
Conflict of Interest
The authors declare that the research was conducted in the absence of any commercial or financial relationships that could be construed as a potential conflict of interest.
Acknowledgments
The authors are grateful to the crew of r/v Aranda and technical staff of SYKE for highly professional work performances during the deployment and recovery of the mussel cages. Jari Nuutinen and Timo Sara-Aho (SYKE) performed the PAH and trace metal analyses, respectively. Thanks are also directed to Jaakko Mannio, Katri Siimes, and Emmi Vähä for management, help, and expertize during the projects leading to this paper and its preparation. The projects MerPAH and UuPri during which the data was collected were funded by the Ministry of the Environment of Finland.
References
Ahkola, H., Siimes, K., and Rantakokko, P. (2018). “Suitability of silicone passive sampler for monitoring TBT concentrations in surface water,” in 2nd Water JPI Conference, Emerging Pollutants in Freshwater Ecosystems (Helsinki: Poster).
Alvarez, D. A., Petty, J. D., Huckins, J. N., Jones-Lepp, T. L., Getting, D. T., and Goddard, J. P. (2004). Development of a passive, in situ, integrative sampler for hydrophilic organic contaminants in aquatic environments. Environ. Toxicol. Chem. 23, 1640–1648. doi: 10.1897/03-603
Andral, B., Galgani, F., Tomasino, C., Bouchoucha, M., Blottiere, C., Scarpato, A., et al. (2011). Chemical contamination baseline in the Western Basin of the Mediterranean Sea based on transplanted mussels. Arch. Environ. Contam. Toxicol. 61, 261–271. doi: 10.1007/s00244-010-9599-x
Andral, B., Stanisiere, J. Y., Sauzade, D., Damier, E., Thebault, H., Galgani, F., et al. (2004). Monitoring chemical contamination levels in the Mediterranean based on the use of mussel caging. Mar. Pollut. Bull. 49, 704–712. doi: 10.1016/j.marpolbul.2004.05.008
Baršienė, J., Lehtonen, K. K., Koehler, A., Broeg, K., Vuorinen, P. J., Lang, T., et al. (2006). Biomarker responses in flounder (Platichthys flesus) and mussel (Mytilus edulis) in the Klaipeda-Butinge area (Baltic Sea). Mar. Pollut. Bull. 53, 422–436. doi: 10.1016/j.marpolbul.2006.03.009
Bebianno, M. J., Pereira, C. G., Rey, F., Cravo, A., Duarte, D., D'Errico, G., et al. (2015). Integrated approach to assess ecosystem health in harbor areas. Sci. Total Env. 514, 92–107. doi: 10.1016/j.scitotenv.2015.01.050
Benedetti, M., Ciaprini, F., Piva, F., Onorati, F., Fattorini, D., Notti, A., et al. (2012). A multidisciplinary weight of evidence approach toward polluted sediments: integrating sediment chemistry, bioavailability, biomarkers responses and bioassays. Environ. Int. 38, 17–28. doi: 10.1016/j.envint.2011.08.003
Benedetti, M., Gorbi, S., Fattorini, D., Pacitti, D., d'Errico, G., Piva, F., et al. (2014). Environmental hazards from natural seepage: integrated classification of risk from sediment In review chemistry, bioavailability and biomarkers responses 512 in sentinel species. Environ. Pollut. 185, 116–126. doi: 10.1016/j.envpol.2013.10.023
Benito, D., Ahvo, A., Nuutinen, J., Bilbao, D., Saenz, J., Etxebarria, N., et al. (2019). Influence of season-depeding ecological variables on biomarker baseline levels in mussels Mytilus trossulus from two Baltic Sea subregions. Sci. Total Env. 689, 1087–1103. doi: 10.1016/j.scitotenv.2019.06.412
Beyer, J., Green, N. W., Brooks, S., Allan, I. J., Ruus, A., Gomes, T., et al. (2017). Blue mussels (Mytilus edulis spp.) as sentinel organisms in coastal pollution monitoring: a review. Mar. Environ. Res. 130, 338–365. doi: 10.1016/j.marenvres.2017.07.024
Bocquené, G., and Galgani, F. (1998). Biological effects of contaminants: cholinesterase inhibition by organophosphate and carbamate compounds. ICES Tech. Mar. Environ. Sci. 22:1–12.
Bradford, M. M. (1976). A rapid and sensitive method for quantitation of microgram quantities of protein utilizing the principle of protein dye binding. Anal. Biochem. 72, 248–254. doi: 10.1006/abio.1976.9999
Cajaraville, M. P., Bebianno, M. J., Blasco, J., Porte, C., Sarasquete, C., and Viarengo, A. (2000). The use of biomarkers to assess the impact of pollution in coastal environments of the Iberian Peninsula: a practical approach. Sci. Total Environ. 247, 295–311. doi: 10.1016/S0048-9697(99)00499-4
Carlberg, I., and Mannervik, B. (1975). Purification and characterization of the flavoenzyme glutathione reductase from rat liver. J. Biol. Chem. 250, 5475–5480.
Claiborne, A. (1985). “Catalase activity,” in Handbook of Methods for Oxygen Radical Research. ed R. A. Greenwald (Boca Raton, FL: CRC Press, 283–284.
Dabrowska, H., Kopko, O., Turja, R., Lehtonen, K. K., Gora, A., and Polak-Juszczak, L. (2013). Sediment contaminants and contaminant levels and biomarkers in caged mussels (Mytilus trossulus) in the southern Baltic Sea. Mar. Environ. Res. 84, 1–9. doi: 10.1016/j.marenvres.2012.11.001
Damiens, G., Gnassia-Barelli, M., Loques, F., Romeo, M., and Salbert, V. (2007). Integrated biomarker response index as a useful tool for environmental assessment evaluated using transplanted mussels. Chemosphere 66, 574–583. doi: 10.1016/j.chemosphere.2006.05.032
EC (2008). Directive 2008/105/EC of the European Parliament and the Council of 16 December 2008 on environmental quality standards in the field of water policy. Off. J. Euro. Union 348, 84–97. Available online at: http://data.europa.eu/eli/dir/2008/105/oj
Guilhermino, L., Barros, B., Silva, M. C., and Soares, A. M. V. M. (1998). Should the use of inhibition of cholinesterase as a specific biomarker for organophosphate and carbamate pesticides be questioned? Biomarkers 3, 157–163. doi: 10.1080/135475098231318
Habig, W. H., Pabst, M. J., and Jakoby, B. (1974). Glutathione-S-transferase. The first enzymatic step in mercapturic acid formation. J. Biol. Chem. 249, 7130–7139.
Hagger, J. A., Galloway, T. S., Langston, W. J., and Jones, M. B. (2009). Application of biomarkers to assess the condition of European marine sites. Environ. Pollut. 157, 2003–2010. doi: 10.1016/j.envpol.2009.02.038
HELCOM (2010). “Hazardous substances in the Baltic Sea–an integrated thematic assessment of hazardous substances in the Baltic Sea,” in Baltic Sea Environment Proceedings (Helsinki), 120B.
HELCOM (2018). “State of the Baltic Sea–second HELCOM holistic assessment 2011-2016,” in Baltic Sea Environment Proceedings (Helsinki), 155.
Kholodkevich, S. V., Kuznetsova, T. V., Sharov, A. N., Kurakin, A. S., Lips, U., Kolesova, N., et al. (2017). Applicability of a bioelectronic cardiac monitoring system for the determination of biological effects of pollution in bioindicator species in the Gulf of Finland. J. Mar. Sys. 171, 8151–8158. doi: 10.1016/j.jmarsys.2016.12.005
Koivisto, M. E., and Westerbom, M. (2010). Habitat structure and complexity as determinants of biodiversity in blue mussel beds on sublittoral rocky shores. Mar. Biol. 157, 1463–1474. doi: 10.1007/s00227-010-1421-9
Kopecka, J., Lehtonen, K. K., Baršienė, J., Broeg, K., Vuorinen, P. J., Gercken, J., et al. (2006). Measurements of biomarker levels in flounder (Platichthys flesus) and blue mussel (Mytilus trossulus) from the Gulf of Gdansk (southern Baltic). Mar. Pollut. Bull. 53, 406–421. doi: 10.1016/j.marpolbul.2006.03.008
Krishnakumar, P., Ali Qurban, M., and Sasikumar, G. (2018). “Biomonitoring of trace metals in the coastal waters using bivalve molluscs,” in Trace Elements–Human Health and Environment. ed H. Saled (InTech Open),153–176.
Lam, P. K. S. (2009). Use of biomarkers in environmental monitoring. Ocean Coast. Manag. 52, 348–354. doi: 10.1016/j.ocecoaman.2009.04.010
Lehtonen, K. K., Kankaanpä, H., Leiniö, S., Sipiä, V. O., Pflugmacher, S., and Sandberg-Kilpi, E. (2003). Accumulation of nodularin-like compounds from the cyanobacterium Nodularia spumigena and changes in acetylcholinesterase activity in the clam Macoma balthica during short-term laboratory exposure. Aquat. Toxicol. 64, 461–476. doi: 10.1016/S0166-445X(03)00101-2
Lehtonen, K. K., Leiniö, S., Schneider, R., and Leivuori, M. (2006a). Biomarkers of pollution effects in the bivalves Mytilus edulis and Macoma balthica collected from the southern coast of Finland (Baltic Sea). Mar. Ecol. Prog. Ser. 322, 155–168. doi: 10.3354/meps322155
Lehtonen, K. K., Schiedek, D., Koehler, A., Lang, T., Vuorinen, P. J., Förlin, L., et al. (2006b). The BEEP project in the Baltic Sea: overview of results and outline for a regional biological effects monitoring strategy. Mar. Pollut. Bull. 53, 523–537. doi: 10.1016/j.marpolbul.2006.02.008
Lehtonen, K. K., Sundelin, B., Lang, T., and Strand, J. (2014). Development of tools for integrated monitoring and assessment of hazardous substances and their biological effects in the Baltic Sea. Ambio 43, 69–81. doi: 10.1007/s13280-013-0478-3
Lehtonen, K. K., Turja, R., Budzinski, H., and Devier, M.-H. (2016). An integrated chemical-biological study using caged mussels (Mytilus trossulus) along a pollution gradient in the Archipelago Sea (SW Finland, Baltic Sea). Mar. Environ. Res.119, 207–221. doi: 10.1016/j.marenvres.2016.06.003
Leiniö, S., and Lehtonen, K. K. (2005). Seasonal variability in biomarkers in the bivalves Mytilus edulis and Macoma balthica from the northern Baltic Sea. Comp. Biochem. Physiol. C 140, 408–421. doi: 10.1016/j.cca.2005.04.005
Lekube, X., Izagirre, U., Soto, M., and Marigomez, I. (2014). Lysosomal and tissue-level biomarkers in mussels cross-transplanted among four estuaries with different pollution levels. Sci. Total Environ. 472, 36–48. doi: 10.1016/j.scitotenv.2013.10.075
Marigómez, I., Zorita, I., Izagirre, U., Ortiz-Zarragoitia, M., Navarro, P., Etxebarria, N., et al. (2013). Combined use of native and caged mussels to assess biological effects of pollution through the integrative biomarker approach. Aquat. Toxicol. 136, 32–48. doi: 10.1016/j.aquatox.2013.03.008
Mayer, P., Tolls, J., Hermens, L., and Mackay, D. (2003). Equilibrium sampling devices. Environ. Sci. Technol. 37, 184–191. doi: 10.1021/es032433i
Moschino, V., Del Negro, P., De Vittor, C., and Da Ros, L. (2016). Biomonitoring of a polluted coastal area (Bay of Muggia, Northern Adriatic Sea): a five-year study using transplanted mussels. Ecotoxicol. Environ. Saf. 128, 1–10. doi: 10.1016/j.ecoenv.2016.02.006
Ohkawa, H., Ohishi, N., and Yagi, K. (1979). Assay for lipid peroxides in animal tissues by thiobarbituric acid reaction. Anal. Biochem. 95, 351–358. doi: 10.1016/0003-2697(79)90738-3
Payne, J. F., Mathieu, A., Melvin, W., and Fancey, L. L. (1996). Acetylcholinesterase, an old biomarker with a new future? Field trials in association with two urban rivers and a paper mill in Newfoundland. Mar. Pollut. Bull. 32, 225–231 doi: 10.1016/0025-326X(95)00112-Z
Perus, J., Bonsdorff, E., Bäck, S., Lax, H. G., Villnäs, A., and Westberg, V. (2007). Zoobenthos as indicators of ecological status in coastal brackish waters: a comparative study from the Baltic Sea. Ambio 36, 250–256. doi: 10.1579/0044-7447(2007)36[250:ZAIOES]2.0.CO;2
Pintado-Herrera, M. G., Lara-Martin, P. A., Gonzalez-Mazo, E., and Allan, I. J. (2016). Determination of silicone rubber and low-density polyethylene diffusion and polymer/water partition coefficients for emerging contaminants. Environ. Toxicol. Chem. 35, 2162–2172. doi: 10.1002/etc.3390
Piva, F., Ciaprini, F., Onorati, F., Benedetti, M., Fattorini, D., Ausili, A., et al. (2011). Assessing sediment hazard through a Weight of Evidence approach with bioindicator organisms: a practical model to elaborate data from sediment chemistry, bioavailability, biomarkers and ecotoxicological bioassays. Chemosphere 83, 475–485 doi: 10.1016/j.chemosphere.2010.12.064
Rank, J., Lehtonen, K. K., Strand, J., and Laursen, M. (2007). DNA damage, acetylcholinesterase activity and lysosomal stability in native and transplanted mussels (Mytilus edulis) in areas close to coastal chemical dumping sites in Denmark. Aquat. Toxicol. 84, 50–61 doi: 10.1016/j.aquatox.2007.05.013
Regoli, F., d'Errico, G., Nardi, A., Mezzelani, M., Fattorini, D., Benedetti, M., et al. (2019). Application of a weight of evidence approach for monitoring complex environmental scenarios: the case-study of off-Shore platforms. Front. Mar. Sci. 6:377. doi: 10.3389/fmars.2019.00377
Regoli, F., Frenzilli, G., Bocchetti, R., Annarumma, F., Scarcelli, V., Fattorini, D., et al. (2004). Time-course variations of oxyradical metabolism, DNA integrity and lysosomal stability in mussels, Mytilus galloprovincialis, during a field translocation experiment. Aquat. Toxicol. 68, 167–178. doi: 10.1016/j.aquatox.2004.03.011
Regoli, F., Pellegrini, D., Cicero, A. M., Nigro, N., Benedetti, M., Gorbi, S., et al. (2014). A multidisciplinary weight of evidence approach for environmental risk assessment at the Costa Concordia wreck: integrative indices from Mussel Watch. Mar. Environ. Res. 96, 92–104. doi: 10.1016/j.marenvres.2013.09.016
Rusina, T. P., Smedes, F., and Klanova, J. (2010). Diffusion coefficients of polychlorinated biphenyls and polycyclic aromatic hydrocarbons in polydimethylsiloxane and low-density polylethylene polymers. J. Appl. Polym. Sci. 116, 1803–1810. doi: 10.1002/app.31704
Schiedek, D., Broeg, K., Baršienė, J., Lehtonen, K. K., Gercken, J., Pfeifer, S., et al. (2006). Biomarker responses and indication of contaminant effects in blue mussel (Mytilus edulis) and female eelpout (Zoarces viviparus) from the western Baltic Sea. Mar. Pollut. Bull. 53, 387–405. doi: 10.1016/j.marpolbul.2005.11.013
Serafim, A., Lopes, B., Company, R., Cravo, A., Gomes, T., Sousa, V., et al. (2011). A multi-biomarker approach in cross-transplanted mussels Mytilus galloprovincialis. Ecotoxicology 20, 1959–1974. doi: 10.1007/s10646-011-0737-7
Smedes, F., Geertsma, R. W., Zande, T. V. D., and Booij, K. (2009). Polymer-water partition coefficients of hydrophobic compounds for passive sampling: application of cosolvent models for validation. Environ. Sci. Technol. 43, 7047–7054. doi: 10.1021/es9009376
Smolders, R., Bervoets, L., De Coen, W., and Blust, R. (2004). Cellular energy allocation in zebra mussels exposed along a pollution gradient: linking cellular effects to higher levels of biological organization. Environ. Pollut. 129, 99–112. doi: 10.1016/j.envpol.2003.09.027
Tsangaris, C., Kormas, K., Strogyloudi, E., Hatzianestis, I., Neofitou, C., Andral, B., et al. (2010). Multiple biomarkers of pollution effects in caged mussels on the Greek coastline. Comp. Biochem. Physiol. C Toxicol. Pharmacol. 151, 369–378. doi: 10.1016/j.cbpc.2009.12.009
Tucca, F., Moya, H., and Barra, R. (2014). Ethylene vinyl acetate polymer as a tool for passive sampling monitoring of hydrophobic chemicals in the salmon farm industry. Mar. Poll. Bull. 88, 174–179. doi: 10.1016/j.marpolbul.2014.09.009
Turja, R., Höher, N., Snoeijs, P., Baršienė, J., Butrimavičienė, L., Kuznetsova, T., et al. (2014). A multibiomarker approach for the assessment of pollution impacts in two areas in the Swedish Baltic Sea coast using caged mussels (Mytilus trossulus). Sci. Total Environ. 473–474, 398–409. doi: 10.1016/j.scitotenv.2013.12.038
Turja, R., Lehtonen, K. K., Meierjohann, A., Brozinski, J., Vahtera, E., Soirinsuo, A., et al. (2015). The mussel caging approach in assessing biological effects of wastewater treatment plant discharges in the Gulf of Finland (Baltic Sea). Mar. Pollut. Bull. 97, 135–149. doi: 10.1016/j.marpolbul.2015.06.024
Turja, R., Soirinsuo, A., Budzinski, H., Devier, M.-H., and Lehtonen, K. K. (2013). Biomarker responses and accumulation of hazardous substances in mussels (Mytilus trossulus) transplanted along a pollution gradient close to an oil terminal in the Gulf of Finland (Baltic Sea). Comp. Biochem. Physiol. C 157, 80–92. doi: 10.1016/j.cbpc.2012.09.006
Väinölä, R., and Strelkov, P. (2011). Mytilus trossulus in Northern Europe. Mar. Biol. 158, 817–833. doi: 10.1007/s00227-010-1609-z
Viarengo, A., and Canesi, L. (1991). Mussels as biological indicators of pollution. Aquaculture 94, 225–243. doi: 10.1016/0044-8486(91)90120-V
Vrana, B., Mills, G. A., Allan, I. J., Dominiak, E., Svensson, K., Knutsson, J., et al. (2005). Passive sampling techniques for monitoring pollutants in water. Trends Anal. Chem. 24, 845–868. doi: 10.1016/j.trac.2005.06.006
Westerbom, M., Kilpi, M., and Mustonen, O. (2002). Blue mussels, Mytilus edulis, at the edge of the range: population structure, growth and biomass along a salinity gradient in the north-eastern Baltic Sea. Mar. Biol. 140, 991–999. doi: 10.1007/s00227-001-0765-6
Keywords: Baltic Sea, biomonitoring, marine pollution, mussel caging, biomarkers, weight of evidence, integrated assessment
Citation: Lehtonen KK, d'Errico G, Korpinen S, Regoli F, Ahkola H, Kinnunen T and Lastumäki A (2019) Mussel Caging and the Weight of Evidence Approach in the Assessment of Chemical Contamination in Coastal Waters of Finland (Baltic Sea). Front. Mar. Sci. 6:688. doi: 10.3389/fmars.2019.00688
Received: 28 February 2019; Accepted: 25 October 2019;
Published: 15 November 2019.
Edited by:
Francois Galgani, Institut Français de Recherche pour l'Exploitation de la Mer (IFREMER), FranceReviewed by:
Periyadan K. Krishnakumar, King Fahd University of Petroleum and Minerals, Saudi ArabiaHalldór Pálmar Halldórsson, University of Iceland, Iceland
Copyright © 2019 Lehtonen, d'Errico, Korpinen, Regoli, Ahkola, Kinnunen and Lastumäki. This is an open-access article distributed under the terms of the Creative Commons Attribution License (CC BY). The use, distribution or reproduction in other forums is permitted, provided the original author(s) and the copyright owner(s) are credited and that the original publication in this journal is cited, in accordance with accepted academic practice. No use, distribution or reproduction is permitted which does not comply with these terms.
*Correspondence: Kari K. Lehtonen, a2FyaS5sZWh0b25lbkB5bXBhcmlzdG8uZmk=