- 1Department of Biology, The University of North Carolina at Chapel Hill, Chapel Hill, NC, United States
- 2Environment, Ecology, and Energy Program, The University of North Carolina at Chapel Hill, Chapel Hill, NC, United States
- 3Centro Ecológico Akumal, Akumal, Mexico
Coral decline in the Caribbean is marked by the loss of habitat-forming corals, such as elkhorn coral (Acropora palmata). Elkhorn coral recovery has been isolated and patchy, but recently a “re-sheeting” phenomenon, in which elkhorn tissue grows over standing dead coral skeletons, was observed along the reefs in the Mexican Yucatán peninsula. Little is known about the ecological factors contributing to “re-sheeting,” but it is hypothesized that grazing from herbivores provides top-down control of algal growth and promotes coral recovery. The purpose of this study was to evaluate the status of elkhorn populations in Akumal, Mexico and determine if Diadema urchins or parrotfish populations are associated with higher elkhorn abundance and lower algal cover. To achieve this objective, we surveyed 12 spur and groove reef sites in Akumal, where re-sheeting was recently observed, and measured elkhorn coral and herbivore population metrics. We found that both herbivore groups are associated with increasing elkhorn coral presence and cover, and lower macroalgal cover. Additionally, we tested for sampling bias in counting Diadema urchins and found that a significant difference in urchins counts between paired day and night transects on shallow, high complexity reefs. Our results suggest that historically important herbivore groups may be contributing to the recovery of elkhorn coral in Akumal by facilitating tissue re-sheeting.
Introduction
Coral decline over the last few decades has greatly changed the structure and functioning of coral reef ecosystems. In the Caribbean, an important component of this decline was the loss of habitat-forming acroporid corals, particularly elkhorn coral (Acropora palmata). Elkhorn coral dominated shallow reef habitats until the 1980s when an outbreak of white-band disease decimated populations region-wide (Aronson and Precht, 2001). The recovery of elkhorn coral is a conservation priority because its unique branching structure provides habitat for countless other reef organisms, its critical role in reef accretion due to fast growth, and its role in buffering coastlines from wave action (Aronson and Precht, 2001; Bellwood et al., 2004; National Marine Fisheries Service, 2015). A second critical component of reef change in the Caribbean has been the decline of herbivore populations. Regionally, parrotfishes have been removed from reefs via overfishing (Hughes, 1994) and the long-spined sea urchin, Diadema antillarum, was nearly extirpated regionally by a pathogen outbreak in the 1980s (Lessios et al., 1984). The reduction of herbivory has led to an increased cover and biomass of macroalgae (Hughes, 1994; Aronson and Precht, 2000). This in turn, when extreme, can reduce coral settlement and recruitment and slow population recovery (Knowlton, 1992; Hughes, 1994; Edmunds and Carpenter, 2001; Mumby, 2009).
Despite the region-wide declines in major reef-building corals, there are some instances of patchy and isolated coral recovery documented in the Caribbean (Macintyre and Toscano, 2007; Zubillaga et al., 2008; Larson et al., 2014; Muller et al., 2014). Recently, a “re-sheeting” phenomenon, in which elkhorn tissue grows over relict coral skeletons (Bonito and Grober-Dunsmore, 2006), was observed along the reefs in the Mexican Yucatán peninsula (Bruno, 2014; Rodríguez-Martínez et al., 2014). Re-sheeting can be identified in the field (Figure 1) whereby thin, healthy tissue takes the shape of the substrate beneath it (in this case, usually the smoothed or flattened edges of relict skeletons) and the edge is not overgrown by another spatial competitor. Little is known about the ecological factors contributing to this re-sheeting phenomenon, and more generally, to the establishment of individual colonies and the regrowth of new populations (Graham et al., 2011). Coral recovery, in general, is complex and any number of ecological or environmental mechanisms can contribute to the recovery of coral species, including recruitment and post-settlement survival (Ritson-Williams et al., 2009), capacity to cope with additional stressors (Mora et al., 2016), interactions with predators and competitors (Grober-Dunsmore et al., 2006), or variation in geographic area and management status (Graham et al., 2011). However, we do know that grazing of benthic algae by herbivorous fishes and urchins is one mechanism of coral recovery which generally facilitates the survival and growth of juvenile or remnant corals (Edmunds and Carpenter, 2001; Hughes et al., 2007). This top-down control by herbivores suppresses the growth of macroalgae, enabling corals to settle and grow by reducing competition for space (Knowlton, 1992; Edmunds and Carpenter, 2001; Carpenter and Edmunds, 2006; Kuempel and Altieri, 2017). Increasing herbivore populations (Kramer et al., 2015; McField et al., 2018), and thus increased grazing activity, may be contributing significantly to the regrowth of elkhorn coral observed in Mexico, where it appears recovery is restricted to locations with numerous herbivores and little seaweed.
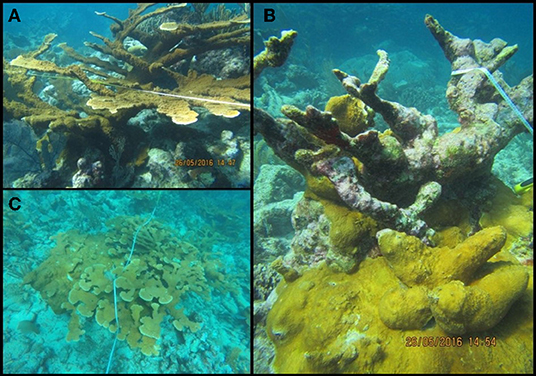
Figure 1. Examples of elkhorn coral (Acropora palmata) growth forms. (A) Typical branching colony growth form. (B) An example of re-sheeting growth over a relict elkhorn colony. (C) An elkhorn thicket, and in this case contains both growth forms, with re-sheeting regrowth on the left side of the thicket and more typical branching colonies on the right and upper side of the thicket.
Scientists have been calling for management initiatives that will aid in the recovery of herbivore populations as one way to boost coral recovery. In the Caribbean, much of this focus is placed on increasing the populations of herbivorous parrotfish species (Jackson et al., 2014; Adam et al., 2015a; Cramer et al., 2017). Understanding the impacts of multiple grazer populations is important for management, and an ongoing debate of the relative importance of parrotfish and urchin populations in controlling macroalgal growth, specifically, has not been resolved (Adam et al., 2015a; Russ et al., 2015; Bruno et al., 2019). Diadema populations remain low throughout the Caribbean, but locally high populations may contribute more grazing pressure to the top-down control of macroalgae. However, we may not have an accurate understanding of the contribution of Diadema to coral recovery on contemporary coral reefs. Diadema can be hard to detect on structurally complex reefs due to their nocturnal feeding behavior, as they are known to seek refuge from predators during the day (Ogden, 1976; Levitan and Genovese, 1989). If urchins are left out of management interventions, we may miss out on a critical component for coral reef recovery.
Akumal, Mexico, is a small coastal Caribbean town, located approximately 105 km south of Cancún, along the Riviera Maya in Quintana Roo. Patterns of coral ecosystem degradation in Akumal mirror past Caribbean region-wide decline of herbivores and corals from overfishing, hurricanes, and disease (Roy, 2004). Additionally, heavy coastal development and remarkable increases in tourism-based activities over the past decade are associated with deteriorating reef conditions in Akumal Bay, particularly in the backreef lagoon that is frequently visited by tourists (Gil et al., 2015; Figueroa-Zavala and Munoz Arroyo, 2018). Conservation efforts resulted in a fish sanctuary being announced in 2015 (Yucatan Times, 2019) and a larger (1,653 hectare) Marine Refuge for Protected Species in 2016 (Official Gazette of the Federation, 2016), yet formal protection and management measures are still being developed. Despite deteriorating conditions from coastal development, ecological monitoring along the Mesoamerican Barrier Reef indicated recent increases to elkhorn coral (Rodríguez-Martínez et al., 2014; McField et al., 2018) and herbivore populations (Kramer et al., 2015; McField et al., 2018). Yet, little is known about the abundance of elkhorn populations in Akumal and what factors may be contributing to the re-sheeting phenomenon observed on these spur-and-groove reefs.
The purpose of this study was to evaluate the status of elkhorn populations in forereef habitats of Akumal, Mexico and determine if historically important herbivore groups, namely Diadema urchins or parrotfishes, are associated with higher elkhorn abundance and less macroalgae. Specifically, we tested hypotheses that higher local density or biomass of herbivores corresponds to increased elkhorn presence, higher elkhorn live area index (LAI), and lower macroalgal percent cover. Additionally, we investigated the sampling bias of Diadema density by conducting paired day/night transects.
Materials and Methods
Research Site
We conducted this study on the coral reefs of Akumal, Mexico (Figure 2), where elkhorn re-sheeting was observed in recent years. There are three distinct sets of spur-and-groove reefs in Akumal, moving perpendicular from the shoreline. The shallowest spur and groove set has a depth range generally ≤11 m, the middle set is ~12–16 m deep, and the deepest set is >16 m deep. We sampled in the first two spur and groove sets (referred to as “shallow” and “deep” throughout), as the third deepest set is beyond the expected depth range of elkhorn coral. Survey locations were chosen based on published locations of previous elkhorn coral assessments (Rodríguez-Martínez et al., 2014) and monitorinig sites used by a local non-governmental organization, Centro Ecológico Akumal (CEA). Due to rarity of elkhorn coral presence across Akumal, we surveyed some locations known to have elkhorn coral to ensure that we would find spurs with elkhorn colonies, but there was no pre-determined surveying gradient of low-to-high abundance. Table 1 documents the total number of transects and which transects contained elkhorn colonies. We conducted 55 transect surveys from May to August 2016 at 12 reef sites, covering approximately 2,200 m2 of benthic habitat over a 10 km stretch of coastline. Transects were 20 m long, with all benthic information recorded within 1 m on each side and parrotfish observed within 2 m on either side.
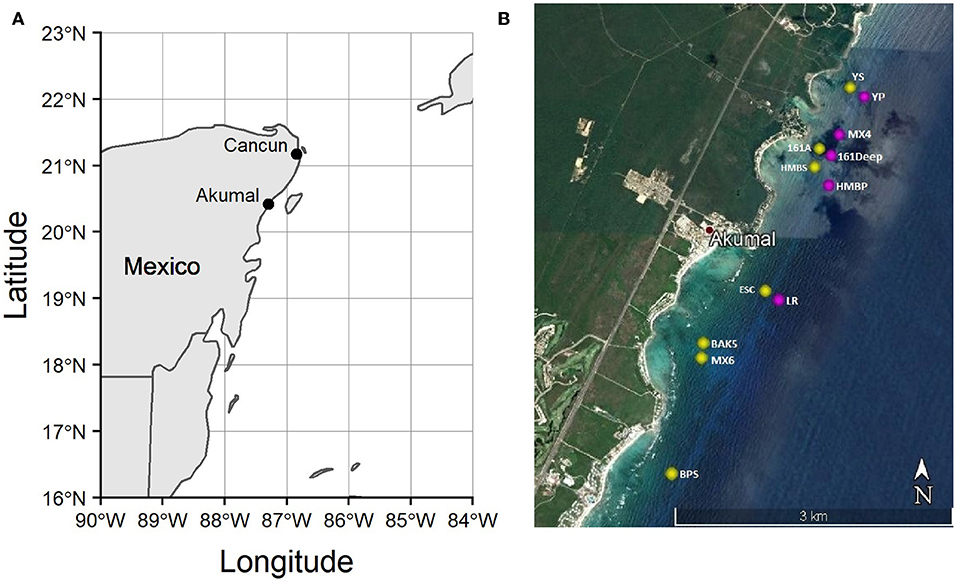
Figure 2. Map of the study region. (A) Location of Akumal, Mexico on the Yucatán Peninsula; (B) Survey site locations on Akumal coral reefs, made with Google Earth. Yellow dots indicate sites on the shallower spur-and-groove set (≤11 m), and pink dots indicate sites on the deeper set of spur-and-grooves (12–17 m).
Live Area Index of Elkhorn Coral
For each elkhorn colony or patch observed, we measured the colony size (length, width, and height in cm); estimated percent mortality; and documented observations of lesions from disease or predation, bleaching, fish bites, worms, and overgrowth. Colonies and patches were measured if the center or base of a colony was inside the 2 m belt. The calculation used for live area index (LAI) is: (W * L * % Live tissue)/100 in units cm2 (Larson et al., 2014). Elkhorn colonies and patches were grouped into three size classes (<60, 60–1,600, and >1,600 cm2) based on reproductive potential (Larson et al., 2014). For patches or thickets of elkhorn coral in which it was not possible to delineate individual colonies, the total width and length of the thicket was measured, and estimated percent coverage of live tissue documented (similar to the estimation of percent mortality).
Herbivore Abundance, Biomass, and Density
We recorded parrotfish species, number of individuals of each species, initial or terminal phase, and estimated size (cm). Parrotfish biomass was calculated from published length-weight ratios found on www.fishbase.org (see Table 2 for coefficient values used), using the equation: W = a × Lb (Froese and Pauly, 2000). In the equation, W represents weight (g), L represents length (cm) and the letters a and b represent standard coefficients based on the species. Parrotfish biomass for all species was pooled together for a biomass value for each transect (g/m2). Parrotfish species were assigned functional groups based on feeding strategy and the proportion of biomass and total individuals observed was calculated for each group (browser, scraper, and excavator). Browsers typically feed on fleshy macroalgae, grazers on turf algae, and excavators on endolithic algae but take large bites that usually excavate the reef framework (Adam et al., 2015b, 2018). The biomass (g/m2) and density (individuals per m2) of browser species was also calculated for each transect.
We counted all Diadema antillarum urchins observed within the 2 m belt, and density of Diadema was calculated for each transect (individuals/m2). We searched for urchins under crevices and included them in a count even if only the spines were visible. Transects were geolocated using surface GPS waypoints and starting points marked with flagging tape so we could return to the exact same spurs at night to recount Diadema urchins, for a total of 16 paired day-night Diadema surveys. On the paired transects only, reef topography was visually assessed by divers, whereas “complex” spurs had relatively higher reef relief and more available crevices or overhangs for organisms to seek refuge (Wilson et al., 2007).
Benthic Cover
Go-Pro cameras (Hero 4) in underwater housings were used to record benthic images along each transect, remaining ~25 cm above the benthos. A total of 30–35 images were collected per transect and uploaded to CoralNet for analysis (Beijbom et al., 2015). Ten points were randomly assigned to each image and we manually identified the benthic component for each tag. Identification was made to species level when possible and all ID tags were pooled into relevant functional groups (hard coral, soft coral, gorgonian, macroalgae, turf algae, crustose coralline algae (CCA), coralline-turf-bare (CTB), etc.). CTB refers to a combined category of coralline algae, turf algae, and bare substrate, and is used when the identification at the specific point cannot be resolved in the images.
Statistical Analysis
A two-step hurdle modeling approach was used to evaluate the relationship between elkhorn coral and six predictor variables: total parrotfish biomass, biomass of parrotfish browser species, density of parrotfish browser species, Diadema density, maximum depth, and site. Due to low sample size (n = 16), we could not include night time urchin density as a predictor in the models. This approach was used over other strategies to (1) aid in determining if either herbivore is associated with the presence/absence of elkhorn coral in addition to the amount of live tissue measured; (2) account for the existence of zeros in the response variable, as elkhorn coral is considered a rare species and was not observed on every transect. This is a well-accepted approach in ecological statistics for measuring the abundance of rare species (Welsh et al., 2002; Fletcher et al., 2005; Zuur et al., 2009). Prior to analysis, raw data were analyzed for normality, heteroscedasticity, outliers, and collinearity. Two outlier observations were removed prior to analysis. Total parrotfish density was not used as a predictor variable due to high collinearity with biomass and fish biomass is the more ecologically relevant variable for this study. Only Diadema density from day-time surveys was included in the models. Maximum depth, measured in meters, was used as the fixed effect because depth category of the spurs (deep or shallow) is confounding with site: meaning that sites were either deep or shallow, but not both. In all models, total parrotfish biomass, biomass of parrotfish browser species, density of parrotfish browser species, Diadema density, and depth were treated as fixed effects and site used as a random effect.
The first step tested the effect of these predictor variables on the presence of elkhorn colonies, using glmer generalized linear mixed-effects models from the lme4 package in R (Bates et al., 2015), with presence/absence of elkhorn colonies as a binomial response. In step two, for transects in which elkhorn colonies were present, we used mixed-effect lmer models from the lme4 package to test for the effect of these predictor variables on elkhorn LAI (a continuous response variable). Macroalgae was observed on every transect, so the step two mixed-effects structure was used to estimate the effect of the predictor variables on percent cover of macroalgae. All predictor variables were centered and scaled prior to modeling. Values are centered by subtracting variable means and scaled by standard deviations, using the center and scale functions, respectively, from base R. This results in standardized coefficients that can be compared directly. Chi-squared tests were used to evaluate if predictors could be dropped from the full model. Akaike information criterion (AIC) values were used to evaluate competing models. We used paired t-tests to evaluate the difference in Diadema density between day and night surveys. All statistical analyses were completed using R version 3.5.0.
Results
Description of Elkhorn and Herbivore Populations in Akumal
The heterogeneity of the spur and groove reef ecosystem in Akumal, Mexico, was indicated by the high variability in the presence and abundance of elkhorn coral and herbivores across sampling sites (Figure 3). Elkhorn coral was most common and had the highest live area index in shallow areas (Table 1) and was not found on the deepest transects (~15 m) at sites LR, YP, and HMBP (Figure 3, Table 1). We measured 85 elkhorn colonies or patches and found that a majority (n = 60) had a LAI >1,600 cm2 (Figure 4). Of those in the larger size class, we documented 15 colonies of a typical branching form, 25 observations of continuous re-sheeting over relict elkhorn skeleton, 12 observations of patchy re-sheeting colonies, and 8 thickets which contained a series of branching colonies (see Figure 1 for examples of form classification). Average percent cover of elkhorn coral was determined from the point-count image analysis and ranged from 0 to 6.5% (Table 1). At two sites, ESC and YS, elkhorn coral did not appear under the transect tape where images were taken for point-count analysis, but colonies did originate within the 2 m belt, which explains why there is a 0% cover but positive LAI value for these sites. The average percent cover of major benthic categories are: 32.56% for macroalgae, 23.5% for turf algae, 13.02% for CTB, and 11.65% for scleractinian corals (including elkhorn coral).
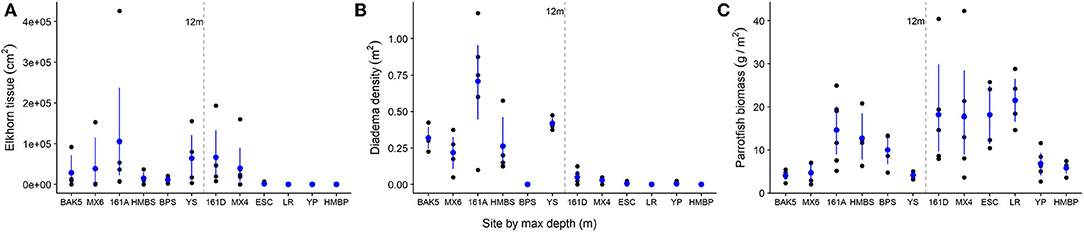
Figure 3. Variation in elkhorn and herbivore populations across survey sites in Akumal, Mexico. Each black dot represents one transect survey (or spur). Blue dots indicate average values at each site with bootstrapped standard errors. On the x-axis, sites are in order of increasing recorded maximum depth (m). The 12 m line indicates the separation between the “shallow” and “deep” sets of spur and groove reef areas. (A) Elkhorn tissue LAI (cm2); (B) Diadema urchin density (individuals/m2); (C) Parrotfish biomass (g/m2).
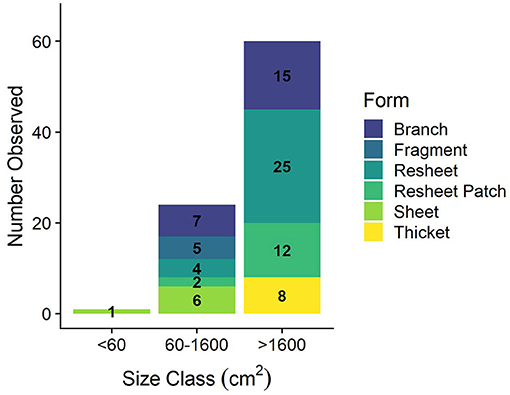
Figure 4. Elkhorn colony size class distribution. Definition of size classes from Larson et al. (2014) and classification of a colony or patch determined from LAI measurement (cm2).
The abundance and density of herbivores also varies by site and depth (Figure 3). Diadema density recorded in the day-time ranged from 0 to 1.2 individuals per m2, with an average of 0.18 individuals/m2 across all sites. Diadema urchins were more common in shallow sites (max density: 1.2/m2, mean density: 0.35/m2) and present but rare at sites deeper than 12 m (Figure 3, mean density: 0.017/m2). Diadema were not observed in belt transects in 3 out of our 12 sites (BPS, LR, HMBP).
We observed seven different species of parrotfishes, with species, average length (cm), and average weight (g) reported in Table 2. Parrotfish biomass ranged from ~2–43 g/m2. with an average of 12 g/m2. Parrotfish were observed in all transects but had higher average biomass in deeper sites (15 g/m2) compared to shallower sites (9 g/m2; Figure 3). Browser species, which typically feed on fleshy macroalgae, comprised the largest proportion of biomass and individuals observed (42 and 51%, respectively), although excavator species made up a comparable 39% of the total biomass (Figure 5).
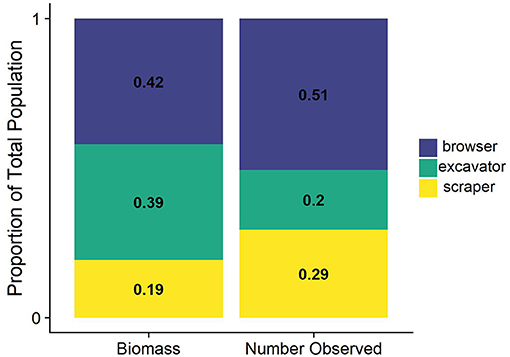
Figure 5. Proportional biomass and count of parrotfish functional groups. Species were classified as browser, scraper, or excavator based on previous analysis from Adam et al. (2018).
Herbivore Populations and the Presence of Elkhorn Coral
Herbivore population metrics of total parrotfish biomass and Diadema density have a positive statistical effect on the presence of elkhorn coral and accounted for 73.7% of variance explained in the model (Figures 6A,B and Table 3, marginal R2 = 0.737, conditional R2 = 0.929). The significance of Diadema density (estimate = 5.7, p = 0.023) was slightly higher, yet comparable to parrotfish biomass (estimate = 2.33, p = 0.046). Model selection revealed that browser density, browser biomass, and maximum depth were not significant predictors and were not included in the final model (Table 4).
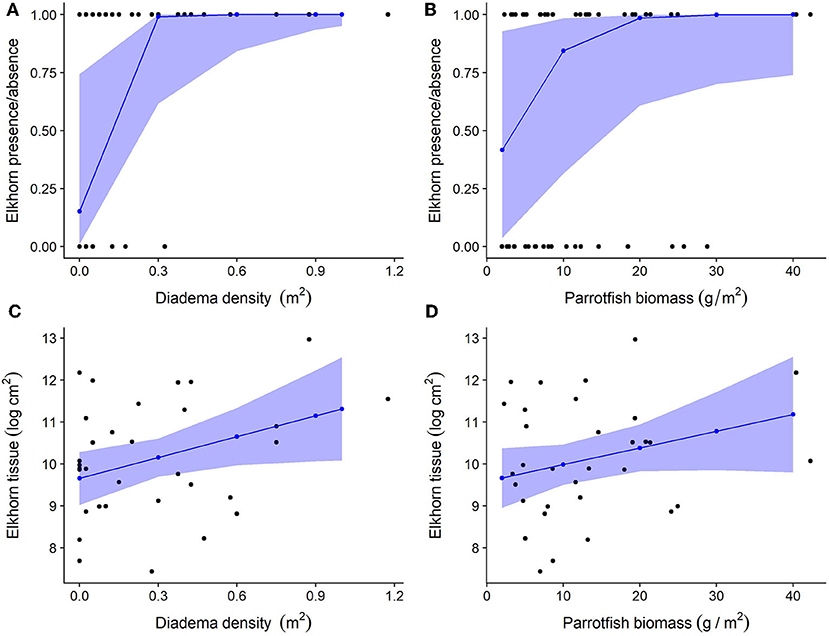
Figure 6. Relationship between herbivore groups and elkhorn coral. For all plots, black dots represent raw data points, blue dots, and lines represent unscaled model estimates and 95% confidence intervals from the final model. (A,B) Relationship between elkhorn coral presence and Diadema density and parrotfish biomass; (C,D) Relationship between elkhorn LAI and Diadema density and parrotfish biomass.
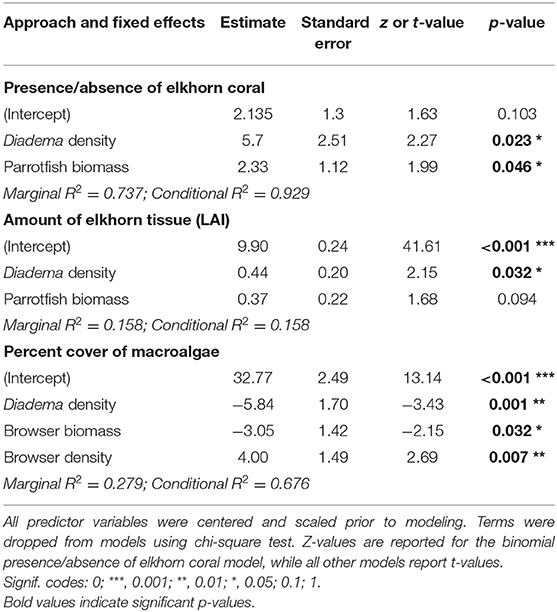
Table 3. Estimated regression parameters, standard error, z-value or t-value, and p-values from the final linear mixed-effects models.
Herbivore Populations and the Amount of Elkhorn Coral Tissue (LAI)
Linear mixed-effects models revealed that Diadema urchins have a statistically significant positive correlation on the amount of live elkhorn tissue (estimate = 1.66, p = 0.032) and total parrotfish biomass has a small, nearly statistically significant correlation (estimate = 0.04, p = 0.094; Table 3, Figures 6C,D). Browser density, browser biomass, and maximum depth were not significant terms and dropped from the final model. However, model comparison indicated that the final model had a lower AIC but was only marginally different from the null model (p = 0.06; Table 4), which only had site as a random effect. Even though Diadema density was a significant predictor of elkhorn LAI, fixed effects in the final model did not account for a substantial amount of the variance (Table 3; marginal R2 = 0.158).
Herbivore Populations and Macroalgal Cover
Diadema density (estimate = −5.84; p = 0.001) and browser species biomass (estimate = −3.95, p = 0.032) had statistically significant negative correlations with macroalgal cover. Browser species density had a significant positive correlation to macroalgal cover (estimate = 4.0, p = 0.007; Table 3, Figure 7). These predictors (fixed effects) accounted for 27.9% of the variance explained in the model (Table 3). Total parrotfish biomass and maximum depth were not significant terms and were dropped from the final model (Table 4). Significant temporal variance in Diadema urchin counts.
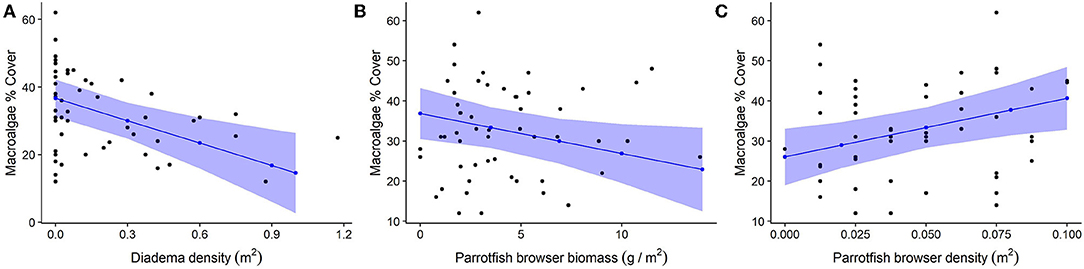
Figure 7. Relationship between herbivores and macroalgae cover. For all plots, black dots represent raw data points, blue dots and lines represent unscaled model estimates and 95% confidence intervals from the final model. (A) Negative association between Diadema density and macroalgae; (B) Negative association between parrotfish browser species biomass and macroalgae; (C) Positive association between parrotfish browser density and macroalgae.
Analysis of paired day and night transect surveys revealed there is a significantly higher number of Diadema urchins observed at night (Figure 8; df = 15, t = −3.36, p = 0.004). This difference is more pronounced in the shallower, higher relief reefs (df = 9, t = −4.6, p = 0.001). Diadema were present on deeper, lower relief sites, yet there was no difference between Diadema counts during day and night surveys (Figure 8). Because of the mixture of variables (depth, complexity) we cannot statistically attribute the differences in Diadema count due to any covariates other than time of day.
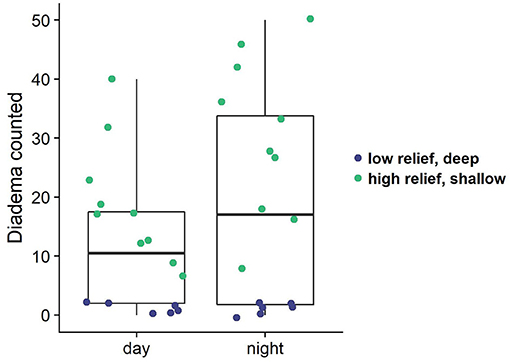
Figure 8. Diadema counts from paired day/night transects. Colored dots indicate the depth zone of the survey (shallow: ≤11 m, deep: 12–17 m), which also correspond to structural complexity in this case (shallow = high relief; deep = low relief).
Discussion
The goal of this study was to evaluate the status of elkhorn populations in Akumal, Mexico and determine if Diadema urchin or parrotfish populations are associated with higher elkhorn abundance and lower algal cover. The majority of elkhorn colonies observed were large in size (>1,600 cm2) and exhibited the re-sheeting growth form. We found that, overall, the presence of elkhorn coral and amount of live tissue increased with increasing herbivore density or biomass. Most strikingly, we found that both herbivore populations contributed significantly to the presence of elkhorn coral. Complementary linear mixed-effects models indicate that Diadema density was a better predictor than parrotfish biomass of total elkhorn tissue live area index, although the fixed effects only contributed to a small portion of the variance. We also found that higher Diadema density and parrotfish browser species biomass was correlated with lower macroalgal cover, even at low abundances. We observed higher Diadema density during our night surveys especially at shallower, high relief reef sites. While this result is not surprising because it corresponds to the expected nocturnal behavior of urchins (Carpenter, 1984), it suggests we may be underestimating population densities, and therefore grazing activity, of Diadema urchins when only considering day-time measurements. Our findings support the argument that recovering herbivore populations may be contributing significantly to controlling algal growth and facilitating high elkhorn abundance or resheeting on contemporary Caribbean shallow water forereef habitats (Morrison, 1988; Edmunds and Carpenter, 2001; Precht and Precht, 2015).
Top-down control of macroalgal growth is one ecological factor imperative for coral recovery, as this process provides physical space on the benthos for corals to settle or grow (Knowlton, 1992; Edmunds and Carpenter, 2001). We hypothesized that the “re-sheeting” phenomenon of elkhorn coral observed in Mexico was related to this top-down control of algal growth, since coral tissue is growing over bare surfaces of relic corals, not up into newly formed 3D structures. Of the 85 colonies surveyed, 43 were classified as re-sheeting and 37 of those colonies were in the largest size class (Figure 4); indicating that re-sheeting is contributing to a large portion of the total LAI and elkhorn cover on Akumal reefs.
Our study revealed that even at low biomass, parrotfish browser species, which feed specifically on macroalgae, have a significant negative correlation with macroalgal cover. Parrotfish biomass in the Mesoamerican Barrier Reef has been increasing slightly in the past few years, and the populations observed here in Akumal are comparable to country and region-wide parrotfish population sizes (Jackson et al., 2014; McField et al., 2018), indicating that recovering populations may be reaching a threshold in which they can exert effective top down control of algal growth (Williams et al., 2016). Even though total parrotfish biomass was not correlated to lower macroalgal cover, fish grazing activity from non-browser species may have other positive benefits such as contributing to increased coral calcification (Suchley and Alvarez-Filip, 2017) or overall reef accretion (Cramer et al., 2017). Conversely, we found increasing macroalgal cover with increasing browser species density. The high number of small bodied Redtail parrotfish species may be contributing to this trend (Table 2).
Our results also revealed increased coral presence and cover with increasing urchin populations and confirmed previous studies demonstrating that higher Diadema densities are associated with coral recovery (Edmunds and Carpenter, 2001; Carpenter and Edmunds, 2006; Myhre and Acevedo-Gutiérrez, 2007) and lower macroalgal cover (Carpenter and Edmunds, 2006; Myhre and Acevedo-Gutiérrez, 2007; Williams et al., 2016; Rodríguez-Barreras et al., 2018) in the Caribbean. This correlation may also be due to positive feedbacks associated with structural complexity and Diadema populations (Lee, 2006). The physical structure of the reef, and elkhorn skeletons in particular, may provide enough refuge to support larger Diadema populations, which results in increased grazing pressure. A 2013 study in Akumal Bay also demonstrated a positive relationship between habitat structural complexity and Diadema density (Lacey et al., 2013).
The spatial extent of grazing pressure can also influence effectiveness of top-down control of macroalgal growth. Previous evidence suggests that herbivory from urchins is more effective for promoting coral growth due to the high grazing intensity and spatially constrained grazing behavior of Diadema urchins (Carpenter, 1986; Sandin and McNamara, 2012), particularly in shallow-water reef zones (Morrison, 1988). Additionally, herbivorous fishes have larger foraging ranges (up to 0.5 hectares) so the grazing pressure from fish is more diffuse due to their roaming behavior (Carpenter, 1986; Sandin and McNamara, 2012; Adam et al., 2015a). This is one potential explanation for higher Diadema estimates in our models.
Implications for Local Management
Our results support recommendations to enhance herbivore populations as one mechanism to promote coral recovery and control macroalgal growth on degraded coral reefs (Adam et al., 2015a; Williams et al., 2016). Efforts to protect and potentially enhance fish populations are already underway through designation of a fish sanctuary and marine reserve in Akumal (Official Gazette of the Federation, 2016; Yucatan Times, 2019). Consideration of the full life-history of parrotfish species is also critical to ensure that ecological functions from all parrotfish groups (browsers, scrapers, and excavators) are maintained (Adam et al., 2015a). A robust parrotfish guild can provide complementary functions to promote reef recovery, including removal of different species of algae (macro vs. turf) and removal of dead coral which can provide space for new coral settlement or growth (Cardoso et al., 2009). We demonstrated that browser species specifically contribute to reduced macroalgae cover, and other studies have found that browser foraging behavior may result in higher rugosity reefs, as bites from browser species typically do not erode reef substrate. Population metrics and behaviors of excavator species, however, should be monitored to prevent excessive substrate erosion or corallivory, which could lead to coral mortality of other reef-building corals (Rotjan and Lewis, 2006; Cardoso et al., 2009; Burkepile, 2012; Bruno et al., 2019).
Strong associations between elkhorn abundance and Diadema populations in Akumal suggest that Diadema reintroduction may be an appropriate tool for local managers when developing holistic coral recovery or restoration plans (Adam et al., 2015a; Precht and Precht, 2015). Managers and researchers could work together toward finding and cultivating ideal site-specific Diadema population sizes: large enough to exhibit top-down control of macroalgal growth and promote coral recovery, but not so dense that urchin grazing harming coral recruitment by consuming coral spat or further eroding the reef substrate (Sammarco, 1980; Korzen et al., 2011; Sandin and McNamara, 2012). Diadema urchins are known bioeroders, meaning they can remove the hard carbonate substrate of the reef; therefore, benthic habitats with poor or no coral recruitment can also be flattened by grazing of extremely high-density urchin populations (Bak, 1994). The role of grazing by smaller urchins, namely Echinometra spp., has been noted in other Caribbean locations (Sangil and Guzman, 2016; Kuempel and Altieri, 2017); however, Echinometra spp. are not common on the exposed spur-and-groove forereef habitats in Akumal. These urchins are typically found in shallow, intertidal zones or protected leeward reefs (Brown-Saracino et al., 2007; McClanahan and Muthiga, 2007) and a 2013 study in Akumal documented variable populations densities of E. lucunter (0–12 individuals per m2) in a shallow, lagoon portion of Akumal Bay. However, the bay area in Akumal is a distinctly different reef structure than the spur-and-groove forereef. The habitat and spatial heterogeneity of both coral and Diadema populations demonstrates the need for location-specific information for evaluating associations between herbivory and coral recovery. To address the issue of inaccurate population estimates of Diadema urchins, day-time survey protocols should be supplemented with evening or night surveying, especially on high relief reefs where more urchins may be completely hidden during daylight hours. More robust information on herbivore population dynamics may also benefit in-water conservation projects, such as coral nurseries, as knowledge of local herbivore densities can provide guidance on geographic areas where outplanted coral fragments might have a higher competitive advantage due to grazing of macroalgae (Sandin and McNamara, 2012; Adam et al., 2015a; Precht and Precht, 2015). This study demonstrates the importance for considering Diadema population metrics in coral recovery efforts.
Local management interventions may be limited in the scope of problems that can be addressed; however, without mitigation of underlying causes of coral mortality such as ocean warming and coastal development, we should not expect herbivore protection or enhancement alone to increase coral resilience (Edmunds and Elahi, 2007; Adam et al., 2015a; Arias-González et al., 2017; Cox et al., 2017; Bruno et al., 2019).
Limitations and Other Considerations
Bottom-up forces, such as eutrophication from terrestrial runoff, which counter grazing pressure may also contribute to a shift toward algal dominance (Arias-González et al., 2017). This study did not test for bottom-up forcing from nutrients; although previous studies demonstrated a correlation between tourism, a proxy for nutrient influx, and declining reef condition in Akumal Bay (Gil et al., 2015; Renfro and Chadwick, 2017) and documented sources of anthropogenic nutrient input to Akumal waters (Hernández-Terrones et al., 2015).
While this study finds statistical correlations between Diadema density and parrotfish biomass with elkhorn live tissue cover, experimental manipulations of herbivore abundance and coral growth are needed to establish a causative effect. Herbivore exclusion studies have been conducted before (Lirman, 2001; Hughes et al., 2007; Sotka and Hay, 2009); however, in the Caribbean these particular studies involve “weedy” coral species that have different growth forms and rates and thus different ecological roles from “competitive” coral species, such as elkhorn coral (Bellwood et al., 2004; Darling et al., 2012). Additionally, we used a visual estimate for reef relief; however, studies quantifying rugosity and Diadema abundances would be more informative in quantifying the relationship not only between Diadema and reef complexity but also in developing predictions of accurate Diadema densities based on reef rugosity.
Lastly, even when elkhorn recovery is documented, tissue damage from predatory snails (e.g., Coralliophila abbreviata) and damselfish grazing (from the cultivation of turf algal “gardens”) may stifle the full potential for regrowth (Grober-Dunsmore et al., 2006). In Akumal, high damselfish density and turf algae cover has been observed the past few years (Figueroa-Zavala and Munoz Arroyo, 2018). These sources of tissue damage should be closely monitored.
Conclusion
In summary, our results suggest that Diadema urchins and parrotfishes are associated with the presence and higher abundance of elkhorn coral in Akumal, Mexico, likely through the top-down control of macroalgae growth. We found that even at low abundances, these herbivore groups are associated with less macroalgal cover, although experimental grazing studies are needed to quantify the magnitude of this effect. While gaps in our knowledge persist regarding the ecological factors contributing to coral recovery, results here suggest that both herbivore groups contribute to elkhorn abundance. Additionally, we highlight the importance of estimating accurate urchin counts using night-time surveys in order to fully understand the influence of Diadema grazing on macroalgal growth and coral recovery on contemporary Caribbean reefs.
Data Availability Statement
The datasets generated for this study are available on request to the corresponding author.
Author Contributions
LM and JB conceived of the study. LM, CA, JB, and BF-Z carried out the study and collected field data, made substantial contributions to the text, and interpretation of the final version of this manuscript. LM performed statistical analysis and wrote the first draft of the manuscript.
Funding
This work was supported by the Henry van Peters Wilson Memorial Fund from Department of Biology at UNC-Chapel Hill (LM), the Tinker Foundation via UNC Institute for the Study of the Americas (LM), and the Environment, Ecology and Energy Program (CA) at UNC-Chapel Hill.
Conflict of Interest
The authors declare that the research was conducted in the absence of any commercial or financial relationships that could be construed as a potential conflict of interest.
Acknowledgments
We would like to thank Jenny Hughes for her help in the first few days of conducting fieldwork in Akumal, the staff at CEA and Akumal Dive Centre for providing logistical support in the field, and Dr. James Umbanhowar for statistical assistance.
References
Adam, T. C., Burkepile, D. E., Ruttenberg, B. I., and Paddack, M. J. (2015a). Herbivory and the resilience of Caribbean coral reefs: knowledge gaps and implications for management. Mar. Ecol. Prog. Ser. 520, 1–20. doi: 10.3354/meps11170
Adam, T. C., Duran, A., Fuchs, C. E., Roycroft, M. V., Rojas, M. C., Ruttenberg, B. I., et al. (2018). Comparative analysis of foraging behavior and bite mechanics reveals complex functional diversity among Caribbean parrotfishes. Mar. Ecol. Prog. Ser. 597, 207–220. doi: 10.3354/meps12600
Adam, T. C., Kelley, M., Ruttenberg, B. I., and Burkepile, D. E. (2015b). Resource partitioning along multiple niche axes drives functional diversity in parrotfishes on Caribbean coral reefs. Oecologia 179, 1173–1185. doi: 10.1007/s00442-015-3406-3
Arias-González, J. E., Fung, T., Seymour, R. M., Garza-Pérez, J. R., Acosta-González, G., Bozec, Y. M., et al. (2017). A coral-algal phase shift in Mesoamerica not driven by changes in herbivorous fish abundance. PLoS ONE 12:e0174855. doi: 10.1371/journal.pone.0174855
Aronson, R. B., and Precht, W. F. (2000). Herbivory and algal dynamics on the coral reef at Discovery Bay, Jamaica. Limnol. Oceanogr. 45, 251–255. doi: 10.4319/lo.2000.45.1.0251
Aronson, R. B., and Precht, W. F. (2001). White-band disease and the changing face of Caribbean coral reefs. Hydrobiologia 460, 25–38. doi: 10.1023/A:1013103928980
Bak, R. P. M. (1994). Sea urchin bioerosion on coral reefs: place in the carbonate budget and relevant variables. Coral Reefs 13, 99–103. doi: 10.1007/BF00300768
Bates, D., Maechler, M., Bolker, B., and Walker, S. (2015). Fitting linear mixed-effects models using lme4. J. Stat. Softw. 67, 1–48. doi: 10.18637/jss.v067.i01
Beijbom, O., Edmunds, P. J., Roelfsema, C., Smith, J., Kline, D. I., Neal, B. P., et al. (2015). Towards automated annotation of benthic survey images: variability of human experts and operational modes of automation. PLoS ONE 10:e0130312. doi: 10.1371/journal.pone.0130312
Bellwood, D. R., Hughes, T. P., Folke, C., and Nystro, M. (2004). Confronting the coral reef crisis. Nature 429, 827–833. doi: 10.1038/nature02691
Bonito, V., and Grober-Dunsmore, R. (2006). Resheeting of relict Acropora palmata framework may promote fast growth but does it compromise the structural integrity of the colony? Coral Reefs 25:46. doi: 10.1007/s00338-005-0062-9
Brown-Saracino, J., Peckol, P., Allen Curran, H., and Robbart, M. L. (2007). Spatial variation in sea urchins, fish predators, and bioerosion rates on coral reefs of Belize. Coral Reefs 26, 71–78. doi: 10.1007/s00338-006-0159-9
Bruno, J. F., Cote, I. M., and Toth, L. T. (2019). Climate change, coral loss, and the curious case of the parrotfish paradigm: why don't marine protected areas improve reef resilience ? Ann. Rev. Mar. Sci. 11, 307–334. doi: 10.1146/annurev-marine-010318-095300
Burkepile, D. E. (2012). Context-dependent corallivory by parrotfishes in a Caribbean reef ecosystem. Coral Reefs 31, 111–120. doi: 10.1007/s00338-011-0824-5
Cardoso, S. C., Soares, M. C., Oxenford, H. A., and Côté, I. M. (2009). Interspecific differences in foraging behaviour and functional role of Caribbean parrotfish. Mar. Biodivers. Rec. 2:e148. doi: 10.1017/S1755267209990662
Carpenter, R. C. (1984). Predator and population density control of homing behavior in the Caribbean echinoid Diadema antillarum. Mar. Biol. 108, 101–108. doi: 10.1007/BF00392768
Carpenter, R. C. (1986). Partitioning herbivory and its effects on coral reef algal communities. Ecol. Monogr. 56, 345–363. doi: 10.2307/1942551
Carpenter, R. C., and Edmunds, P. J. (2006). Local and regional scale recovery of Diadema promotes recruitment of scleractinian corals. Ecol. Lett. 9, 268–277. doi: 10.1111/j.1461-0248.2005.00866.x
Cox, C., Valdivia, A., McField, M., Castillo, K., and Bruno, J. F. (2017). Establishment of marine protected areas alone does not restore coral reef communities in Belize. Mar. Ecol. Prog. Ser. 563, 65–79. doi: 10.3354/meps11984
Cramer, K. L., O'Dea, A., Clark, T. R., Zhao, J., and Norris, R. D. (2017). Prehistorical and historical declines in Caribbean coral reef accretion rates driven by loss of parrotfish. Nat. Commun. 8:14160. doi: 10.1038/ncomms14160
Darling, E. S., Alvarez-Filip, L., Oliver, T. A., Mcclanahan, T. R., and Cote, I. M. (2012). Evaluating life-history strategies of reef corals from species traits. Ecol. Lett. 15, 1378–1386. doi: 10.1111/j.1461-0248.2012.01861.x
Edmunds, P. J., and Carpenter, R. C. (2001). Recovery of Diadema antillarum reduces macroalgal cover and increases abundance of juvenile corals on a Caribbean reef. Proc. Natl. Acad. Sci. U.S.A. 98, 5067–5071. doi: 10.1073/pnas.071524598
Edmunds, P. J., and Elahi, R. (2007). The demographics of a 15-year decline in cover of the Caribbean reef coral Montastraea annularis. Ecol. Monogr. 77, 3–18. doi: 10.1890/05-1081
Figueroa-Zavala, B., and Munoz Arroyo, N.-H. (2018). Annual Report of Results From the Coastal Ecosystems Programme. Centro Ecológico Akumal. Internal Report.
Fletcher, D., MacKenzie, D., and Villouta, E. (2005). Modelling skewed data with many zeros: a simple approach combining ordinary and logistic regression. Environ. Ecol. Stat. 12, 45–54. doi: 10.1007/s10651-005-6817-1
Froese, R., and Pauly, D. (2000). FishBase. World Wide Web Electron. Publ. Available online at: http://www.fishbase.org (accessed July 21, 2016).
Gil, M. A., Renfro, B., Figueroa-Zavala, B., Penié, I., and Dunton, K. H. (2015). Rapid tourism growth and declining coral reefs in Akumal, Mexico. Mar. Biol. 162, 2225–2233. doi: 10.1007/s00227-015-2748-z
Graham, N. A. J., Nash, K. L., and Kool, J. T. (2011). Coral reef recovery dynamics in a changing world. Coral Reefs 30, 283–294. doi: 10.1007/s00338-010-0717-z
Grober-Dunsmore, R., Bonito, V., and Frazer, T. K. (2006). Potential inhibitors to recovery of Acropora palmata populations in St. John, US Virgin Islands. Mar. Ecol. Prog. Ser. 321, 123–132. doi: 10.3354/meps321123
Hernández-Terrones, L. M., Null, K. A., Ortega-Camacho, D., and Paytan, A. (2015). Water quality assessment in the Mexican Caribbean: impacts on the coastal ecosystem. Cont. Shelf Res. 102, 62–72. doi: 10.1016/j.csr.2015.04.015
Hughes, T. P. (1994). Catastrophes, phase shifts and large-scale degradation of a Caribbean Coral Reef. Science 265, 1547–1551. doi: 10.1126/science.265.5178.1547
Hughes, T. P., Rodrigues, M. J., Bellwood, D. R., Ceccarelli, D., Hoegh-Guldberg, O., McCook, L., et al. (2007). Phase shifts, herbivory, and the resilience of coral reefs to climate change. Curr. Biol. 17, 360–365. doi: 10.1016/j.cub.2006.12.049
Jackson, J., Donovan, M., Kramer, K., and Lam, W. (2014). Status and Trends of Caribbean Coral Reefs: 1970–2012. Global Coral Reef Monitoring Network, IUCN, Gland.
Knowlton, N. (1992). Thresholds and multiple stable states in coral reef community dynamics. Am. Zool. 32, 674–682. doi: 10.1093/icb/32.6.674
Korzen, L., Israel, A., and Abelson, A. (2011). Grazing effects of fish versus sea Urchins on turf algae and coral recruits: possible implications for coral reef resilience and restoration. J. Mar. Biol. 2011, 1–8. doi: 10.1155/2011/960207
Kramer, P., McField, M., Alvarez-Filip, L., Drysale, I., Rueda Flores, M., Giro, A., et al. (2015). 2015 Report Card for the Mesoamerican Reef. Available online at: http://www.healthyreefs.org (accessed January 4, 2016).
Kuempel, C. D., and Altieri, A. H. (2017). The emergent role of small-bodied herbivores in pre-empting phase shifts on degraded coral reefs. Sci. Rep. 7, 1–10. doi: 10.1038/srep39670
Lacey, E. A., Fourqurean, J. W., and Collado-Vides, L. (2013). Increased algal dominance despite presence of Diadema antillarum. Bull. Mar. Sci. 89, 1–18. doi: 10.5343/bms.2012.1015
Larson, E. A., Gilliam, D. S., Padierna, M. L., and Walker, B. K. (2014). Possible recovery of Acropora palmata (Scleractinia: Acroporidae) within the veracruz reef system, Gulf of Mexico : a survey of 24 reefs to assess the benthic communities. Rev. Biol. Trop. 62, 75–84. doi: 10.15517/rbt.v62i0.15903
Lee, S. C. (2006). Habitat complexity and consumer-mediated positive feedbacks on a Caribbean coral reef. Oikos 112, 442–447. doi: 10.1111/j.0030-1299.2006.14247.x
Lessios, H. A., Robertson, D. R., and Cubit, J. D. (1984). Spread of diadema mass mortality through the Caribbean. Science 226, 335–337. doi: 10.1126/science.226.4672.335
Levitan, D. R., and Genovese, S. J. (1989). Substratum-dependent predator-prey dynamics: patch reefs as refuges from gastropod predation. J. Exp. Mar. Bio. Ecol. 130, 111–118. doi: 10.1016/0022-0981(89)90198-6
Lirman, D. (2001). Competition between macroalgae and corals: effects of herbivore exclusion and increased algal biomass on coral survivorship and growth. Coral Reefs 19, 392–399. doi: 10.1007/s003380000125
Macintyre, I. G., and Toscano, M. A. (2007). The elkhorn coral Acropora palmata is coming back to the Belize Barrier Reef. Coral Reefs 26:757. doi: 10.1007/s00338-007-0268-0
McClanahan, T. R., and Muthiga, N. A. (2007). Ecology of Echinometra. Dev. Aquac. Fish. Sci. 37, 297–317. doi: 10.1016/S0167-9309(07)80079-2
McField, M., Kramer, P., Alvarez-Filip, L., Drysdale, I., Rueda Flores, M., Giro Peterson, A., et al. (2018). 2018 Report Card for the Mesoamerican Reef. Available online at: http://www.healthyreefs.org (accessed January 4, 2016).
Mora, C., Graham, N. A. J., and Nystrom, M. (2016). Ecological limitations to the resilience of coral reefs. Coral Reefs 35, 1271–1280. doi: 10.1007/s00338-016-1479-z
Morrison, D. (1988). Comparing fish and urchin grazing in shallow and deeper coral reef algal communities. Ecology 69, 1367–1382. doi: 10.2307/1941634
Muller, E. M., Rogers, C. S., and van Woesik, R. (2014). Early signs of recovery of Acropora palmata in St. John, US Virgin Islands. Mar. Biol. 161, 359–365. doi: 10.1007/s00227-013-2341-2
Mumby, P. J. (2009). Phase shifts and the stability of macroalgal communities on Caribbean coral reefs. Coral Reefs 28, 761–773. doi: 10.1007/s00338-009-0506-8
Myhre, S., and Acevedo-Gutiérrez, A. (2007). Recovery of sea urchin Diadema antillarum populations is correlated to increased coral and reduced macroalgal cover. Mar. Ecol. Prog. Ser. 329, 205–210. doi: 10.3354/meps329205
National Marine Fisheries Service (2015). Recovery Plan for Elkhorn (Acropora palmata) and Staghorn (A. cervicornis) Corals. Silver Spring, MD. National Oceanic and Atmospheric Administration, National Marine Fisheries Service, Southeast Regional Office.
Official Gazette of the Federation (2016). Agreement That Establishes With the Name of Bahía de Akumal the Refuge Area for the Protection of the Indicated Species, the Marine Portion Indicated in the State of Quintana Roo. Official Gazette of the Federation. Available online at: http://www.dof.gob.mx/nota_detalle.php?codigo=5428829&fecha=07/03/2016 (accessed May 13, 2019)
Ogden, J. C. (1976). Some aspects of herbivore-plant relationships on Caribbean reefs and seagrass beds. Aquat. Bot. 2, 103–116. doi: 10.1016/0304-3770(76)90013-9
Precht, L. L., and Precht, W. F. (2015). The sea urchin Diadema antillarum–keystone herbivore or redundant species? PeerJ. Prepr. 3, 1–43. doi: 10.7287/peerj.preprints.1565v2
Renfro, B., and Chadwick, N. E. (2017). Benthic community structure on coral reefs exposed to intensive recreational snorkeling. PLoS ONE 12:e0184175. doi: 10.1371/journal.pone.0184175
Ritson-Williams, R., Arnold, S., Fogarty, N., Steneck, R. S., Vermeij, M., and Paul, V. J. (2009). New perspectives on ecological mechanisms affecting coral recruitment on reefs. Smithson. Contrib. Mar. Sci. 38, 437–457. doi: 10.5479/si.01960768.38.437
Rodríguez-Barreras, R., Montañez-Acuña, A., Otaño-Cruz, A., and Ling, S. D. (2018). Apparent stability of a low-density Diadema antillarum regime for Puerto Rican coral reefs. ICES J. Mar. Sci. 75, 2193–2201. doi: 10.1093/icesjms/fsy093
Rodríguez-Martínez, R. E., Banaszak, A. T., McField, M. D., Beltrán-Torres, A. U., and Álvarez-Filip, L. (2014). Assessment of Acropora palmata in the mesoamerican reef system. PLoS ONE 9:e96140. doi: 10.1371/journal.pone.0096140
Rotjan, R. D., and Lewis, S. M. (2006). Parrotfish abundance and selective corallivory on a Belizean coral reef. J. Exp. Mar. Bio. Ecol. 335, 292–301. doi: 10.1016/j.jembe.2006.03.015
Roy, R. E. (2004). Akumal's reefs: stony coral communities along the developing Mexican Caribbean coastline. Rev. Biol. Trop. 52, 869–881.
Russ, G. R., Questel, S.-L. A., Rizzari, J. R., and Alcala, A. C. (2015). The parrotfish–coral relationship: refuting the ubiquity of a prevailing paradigm. Mar. Biol. 162, 2029–2045. doi: 10.1007/s00227-015-2728-3
Sammarco, P. W. (1980). Diadema and its relationship to coral spat mortality: grazing, competition, and biological disturbance. J. Exp. Mar. Bio. Ecol. 45, 245–272. doi: 10.1016/0022-0981(80)90061-1
Sandin, S. A., and McNamara, D. E. (2012). Spatial dynamics of benthic competition on coral reefs. Oecologia 168, 1079–1090. doi: 10.1007/s00442-011-2156-0
Sangil, C., and Guzman, H. M. (2016). Assessing the herbivore role of the sea-urchin Echinometra viridis: keys to determine the structure of communities in disturbed coral reefs. Mar. Environ. Res. 120, 202–213. doi: 10.1016/j.marenvres.2016.08.008
Sotka, E. E., and Hay, M. E. (2009). Effects of herbivores, nutrient enrichment, and their interactions on macroalgal proliferation and coral growth. Coral Reefs 28, 555–568. doi: 10.1007/s00338-009-0529-1
Suchley, A., and Alvarez-Filip, L. (2017). Herbivory facilitates growth of a key reef-building Caribbean coral. Ecol. Evol. 7, 11246–11256. doi: 10.1002/ece3.3620
Welsh, A. H., Cunningham, R. B., Donnelly, C. F., and Lindenmayer, D. B. (2002). Modelling the abundance of rare species: statistical models for counts with extra zeros. Ecol. Modell. 88, 297–308. doi: 10.1016/0304-3800(95)00113-1
Williams, S. M., Sanchez-Godinez, C., Newman, S. P., and Cortes, J. (2016). Ecological assessments of the coral reef communities in the Eastern Caribbean and the effects of herbivory in influencing coral juvenile density and algal cover. Mar. Ecol. 38, 1–10. doi: 10.1111/maec.12395
Wilson, S. K., Graham, N. A. J., and Polunin, N. V. C. (2007). Appraisal of visual assessments of habitat complexity and benthic composition on coral reefs. Mar. Biol. 151, 1069–1076. doi: 10.1007/s00227-006-0538-3
Yucatan Times (2019). Akumal: Officially Decreed by Government as Fish Refuge. Yucatan Times. Available online at: https://www.theyucatantimes.com/2015/04/akumal-officially-decreed-by-government-as-fish-refuge/ (accessed May 13, 2019)
Zubillaga, A. L., Márquez, L. M., Cróquer, A., and Bastidas, C. (2008). Ecological and genetic data indicate recovery of the endangered coral Acropora palmata in Los Roques, Southern Caribbean. Coral Reefs 27, 63–72. doi: 10.1007/s00338-007-0291-1
Keywords: Acropora palmata, Caribbean, Diadema antillarum, parrotfishes, herbivory
Citation: Mudge L, Alves C, Figueroa-Zavala B and Bruno J (2019) Assessment of Elkhorn Coral Populations and Associated Herbivores in Akumal, Mexico. Front. Mar. Sci. 6:683. doi: 10.3389/fmars.2019.00683
Received: 15 February 2019; Accepted: 22 October 2019;
Published: 12 November 2019.
Edited by:
Jesús Ernesto Arias González, Center for Research and Advanced Studies (CINVESTAV), MexicoReviewed by:
Juan Pablo Carricart-Ganivet, National Autonomous University of Mexico, MexicoLorenzo Alvarez-Filip, National Autonomous University of Mexico, Mexico
Copyright © 2019 Mudge, Alves, Figueroa-Zavala and Bruno. This is an open-access article distributed under the terms of the Creative Commons Attribution License (CC BY). The use, distribution or reproduction in other forums is permitted, provided the original author(s) and the copyright owner(s) are credited and that the original publication in this journal is cited, in accordance with accepted academic practice. No use, distribution or reproduction is permitted which does not comply with these terms.
*Correspondence: Laura Mudge, bG11ZGdlQGxpdmUudW5jLmVkdQ==