- 1Department of Ocean Systems, NIOZ Royal Netherlands Institute for Sea Research, Utrecht University, Texel, Netherlands
- 2Department of Earth Sciences, Faculty of Geosciences, Utrecht University, Utrecht, Netherlands
The ratio of Mg to Ca in foraminiferal calcium carbonate (Mg/Cacc) is a popular tool to reconstruct past sea water temperatures. Its application is being complicated by other environmental factors affecting the Mg/Cacc, including salinity and the ratio between sea water [Mg2+] and [Ca2+]. Furthermore, there is considerable intra-specimen variability in Mg/Ca in the form of alternating high- and low-concentration bands. This banding has recently been linked to diurnal cyclicity, in which bands with relatively high Mg/Ca are precipitated during night time. Here we show that light not only impacts variability due to banding but also significantly affects average chamber Mg/Ca in the large benthic, symbiont-bearing foraminifer Amphistegina lessonii. These ratios are higher in foraminifera that calcify for a longer time in the dark, with a difference in Mg/Ca of 23 mmol/mol between carbonate formed completely in the dark vs. completely in the light. We propose that individual timing of chamber formation and thus presence or absence of light during calcification is an important driver of inter-specimen variability in foraminiferal Mg/Ca.
Introduction
Elements in foraminiferal calcite serve as tools to reconstruct past environments: Mg/Ca, for example, can be used to estimate paleo-temperatures (Nuernberg, 1995). However, such proxy-relationships are usually offset by other environmental variables: salinity and carbonate chemistry, for example, are known to impact Mg-incorporation (Lea et al., 1999; Dueñas-Bohórquez et al., 2011; Hönisch et al., 2013; Geerken et al., 2018), as does sea water Mg/Ca (Segev and Erez, 2006; de Nooijer et al., 2017b).
Light potentially impacts trace element incorporation in foraminiferal shells. In the planktonic species Orbulina universa (Spero et al., 2015; Holland et al., 2017) and Neogloboquadrina dutertrei (Fehrenbacher et al., 2017) bands of high element concentrations are associated to calcite formed in the dark. A potential mechanistic link between light and element incorporation was suggested by Al-Horani et al. (2003), who found light-dependent Ca2+-ATPase in corals. Erez (2003) speculated that similar mechanisms could promote calcification in foraminifera, which would make element incorporation in foraminiferal calcite light-dependent. This effect could be enhanced, for example by migration of planktonic species in the water column, hence affecting symbiont activity (Köhler-Rink and Kühl, 2000, 2005). Photosynthesis by these symbionts determines the pH and saturation state in the microenvironment of the foraminifera (Köhler-Rink and Kühl, 2000), as well as the oxygen levels near the foraminifera (Köhler-Rink and Kühl, 2005) which in turn, could affect the chemical speciation of trace and minor elements [e.g., S; (van Dijk et al., 2019)], and hence their uptake rates during calcification.
To quantify the relation between light and calcite chemistry, we tested the incorporation of Mg as a function of light exposure by culturing the symbiont bearing large benthic foraminifera Amphistegina lessonii under different durations of light exposure, while keeping all other factors constant.
Materials and Methods
Surface sediments were collected from the tropical reef aquarium at the Royal Burgers’ Zoo [Arnhem, Netherlands; (Ernst et al., 2011)] by scuba diving. Living specimens of A. lessonii > 250 μm were isolated and fed a solution of freeze dried Dunaliella salina with every exchange of culture medium. Diameters of the incubated specimens varied between ∼250 and ∼1000 μm, but they were distributed evenly over five groups of 10 specimens that were cultured at 6 different day/night regimes. Each of the 30 groups were placed in tissue bottles with 70 ml of culture medium that were placed in incubators with constant temperature (21°C), salinity (35.4) and light intensity (85 μmol photons/m2/s). The duration of light exposure per day varied for each treatment (4, 10, 12, 14, 20, and 24 h of light per day) and lasted 25 days in total.
The culture medium consisted of modified, filtered North Atlantic surface seawater with addition of 5 mg/L Calcein to stain carbonate formed during the experiment. Before and after addition of the Calcein, pH remained similar. Incorporation of this fluorescent marker is known not to impact trace metal incorporation (Dissard et al., 2009) and hence does not bias the interpretation of our results. Additionally, in four treatments (4, 10, 14, and 20 h of light exposure per day) the water chemistry was artificially altered when switching on or off the light. With the light switched on, low-Ba (∼4.4 μmol/mol) culture medium (ambient seawater + Calcein) was supplied. At the onset of every dark period, the culture medium was replaced by a second medium with a Ba/Ca ratio set at an elevated value of 19.0 μmol/mol (ambient seawater + Calcein + BaCl2), as a tracer for carbonate formed during the dark periods in contrast to the fluorescent dye which was incorporated during both light and dark periods. Both tracers were necessary, since using only Calcein does not allow to determine the timing of calcification. Using elevated Ba/Ca during night time without the additional use of Calcein would result in a bias, since chambers formed completely during day time would not be labeled and thus could not be differentiated from chambers formed before the onset of the experiment. Ba is a suitable tracer since its incorporation is mainly controlled by the concentration in the culture medium (Lea and Boyle, 1991; Lea and Spero, 1992; de Nooijer et al., 2017a). The medium with elevated Ba concentrations was produced by adding a BaCl2 solution to the low-Ba seawater. The two treatments of 12 h light exposure and 24 h light exposure per day did not involve enrichment of Ba and were only used to monitor the effect of light exposure on growth rate. When replacing the culture medium with the low Ba-seawater, culture bottles were rinsed three times thoroughly with low Ba culture medium to avoid contamination with seawater with a high Ba/Ca of the preceding light phase. After termination of the experiment, all specimens were then cleaned following procedures described by van Dijk et al. (2017) and subsequently examined for new, fluorescent chambers.
Individual chambers from 121 specimens of the enriched Ba treatments were analyzed for their Ba/Ca and Mg/Ca using LA-ICP-MS. A total of 57, 57, 40, and 42 laser ablation measurements of ultimate and penultimate chambers were conducted for treatments with 4, 10, 14, and 20 h light exposure, respectively. The first (oldest) chamber labeled with Calcein was not analyzed since the calcite of this chamber may (partly) reflect pre-experimental conditions (e.g., in case calcification would utilize a previously built internal calcium pool). In total 196 measurements were performed using 121 F and 71 F-1 chambers, if the F-1 chamber was not the first chamber labeled with Calcein. The laser system (NWR193UC, New Wave Research) at the NIOZ was used in combination with a two-volume sample cell (TV2), which allows detecting variability in El/Ca ratios within the foraminiferal chamber wall due to a short wash-out time of 1.8 s (van Dijk et al., 2017). A circular laser spot with a diameter of 80 μm was chosen to allow measurements in the center of the chamber. All specimens were ablated with an energy density of 1.0 ± 0.1 J/cm2 and a repetition rate of 6 Hz in a helium environment until the chamber walls were fully penetrated. A 0.7 L/m helium flow transported the resulting aerosol to an in-house-built smoothing device before entering the quadrupole ICP-MS (iCAP-Q, Thermo Fisher Scientific). Masses 7Li, 23Na, 24Mg, 25Mg, 43Ca, 44Ca, 55Mn, 88Sr, 137Ba, and 138Ba were measured, 44Ca served as an internal standard. The synthetic carbonate standard MACS-3 was used for calibration, in addition carbonate standards JCp-1, JCt-1, NFHS1 [NIOZ foraminifera house standard; (Mezger et al., 2016)] as well as glass standards SRM NIST610 and NIST612 were used for monitoring data quality.
Elemental concentrations of the culture media were determined with high resolution ICP-MS (Element2, Thermo Finnigan). For determining Ba and U, the culture medium samples were diluted to 10% with a 0.14M HNO3 solution, while samples for the analyses of Mg, Ca, and Sr were diluted to 1% with 0.14M HNO3. All samples were calibrated by matrix matched standard addition, with In as an internal standard and 1 and 10% diluted North Atlantic seawater was used for matrix matching. IAPSO standard water was used for reference. The trace metal composition of both culture media was very similar, except for the Ba content, which was served as a tracer for day- vs. night calcification. The medium’s Mg concentration varied only marginally (between 1.32 and 1.30 g/kg) between treatments. The relative standard deviation was less than 1% for Mg, Ba, and Ca.
Results
Foraminifera from most treatments grew between 0 and 9 chambers per individual, with an average of 2.1 added chambers (Figure 1). Only in the 24 h–light group, foraminifera grew significantly fewer new chambers than in all other treatments, on average less than 1 chamber was added per individual over the duration of the entire experiment (Figure 1). The majority of chambers formed across the different treatments were shaped and sized normally, without differences between treatments. Reproductions occurred once in both the 20 h light and the 14 h light treatment after 12 and 23 days, respectively.
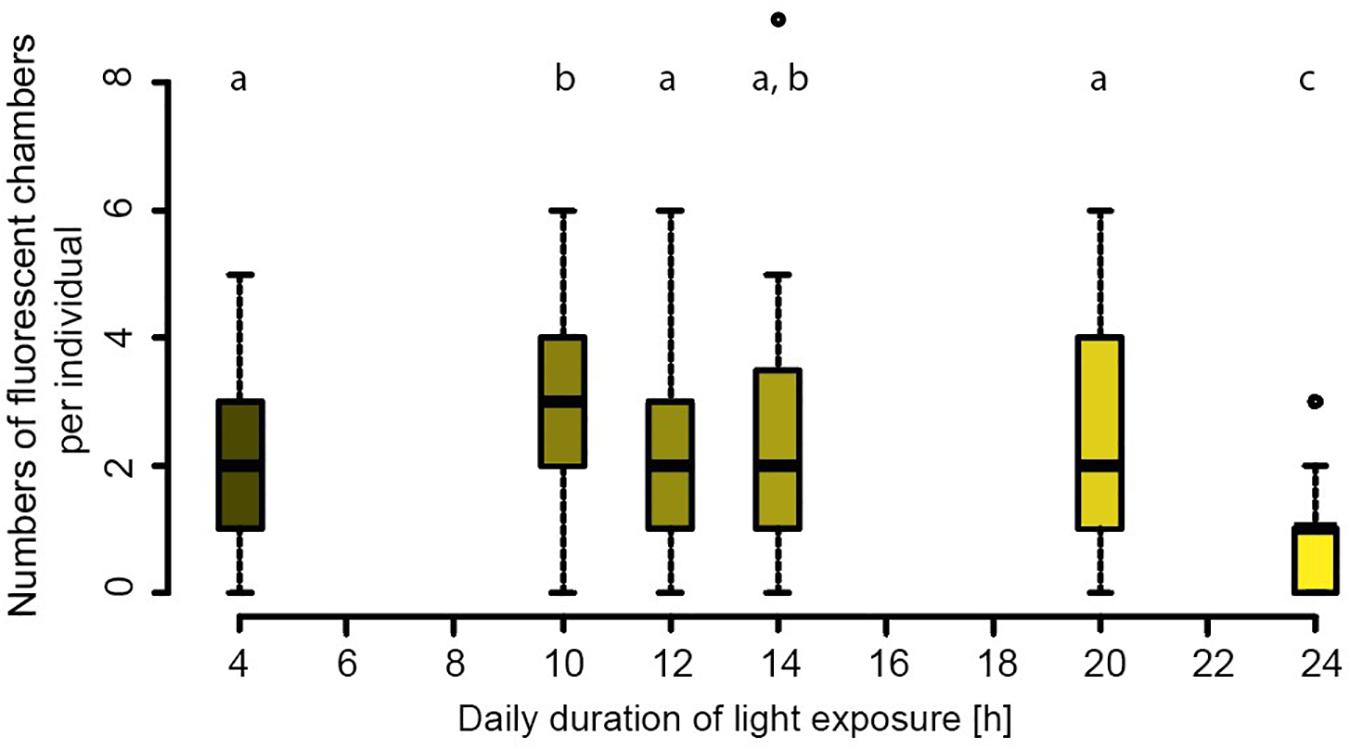
Figure 1. Newly formed chambers per individual for the six different treatments. Statistically significant groups (p > 0.05) are indicated by letters. Shading indicates day length.
Values for individual chamber measured Ba/Ca vary between 1.29 and 8.53 μmol/mol, with specimens from treatments that were exposed to high Ba for a longer time (and hence remained longer in the dark) showing higher Ba/Ca ratios (Figure 2). Values for Mg/Ca vary between 17.3 and 78.4 mmol/mol across treatments (Figure 3), whereas Mg incorporation does not vary significantly with light duration. Average elemental composition for specimens of each treatment is reported in Table 1.
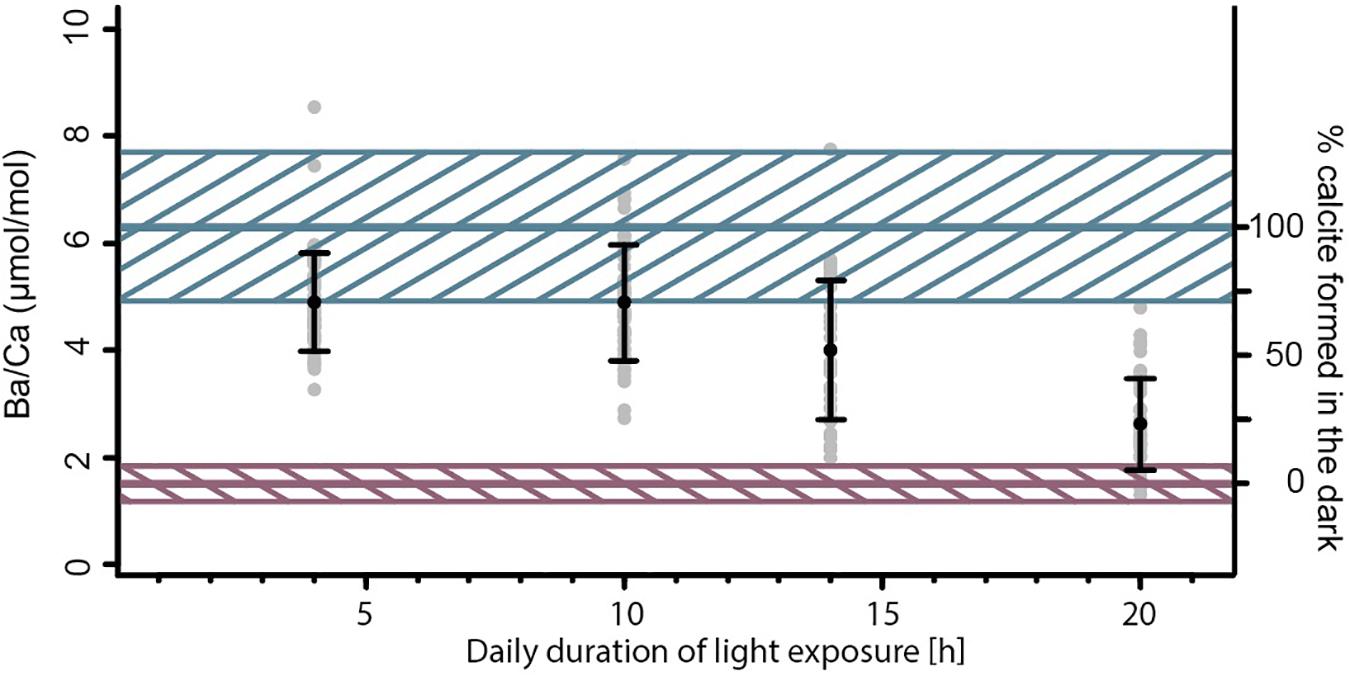
Figure 2. Ba/Ca ratio of the four different Ba-spiked treatments. Gray points represent single chamber measurements, black markers, and whiskers show the average ± standard deviation of a group. The blue and red line indicate upper and lower mixing model endmembers and their unceirtainties (hatched areas), based on the partition coefficient DBa and the Ba/CaSW.
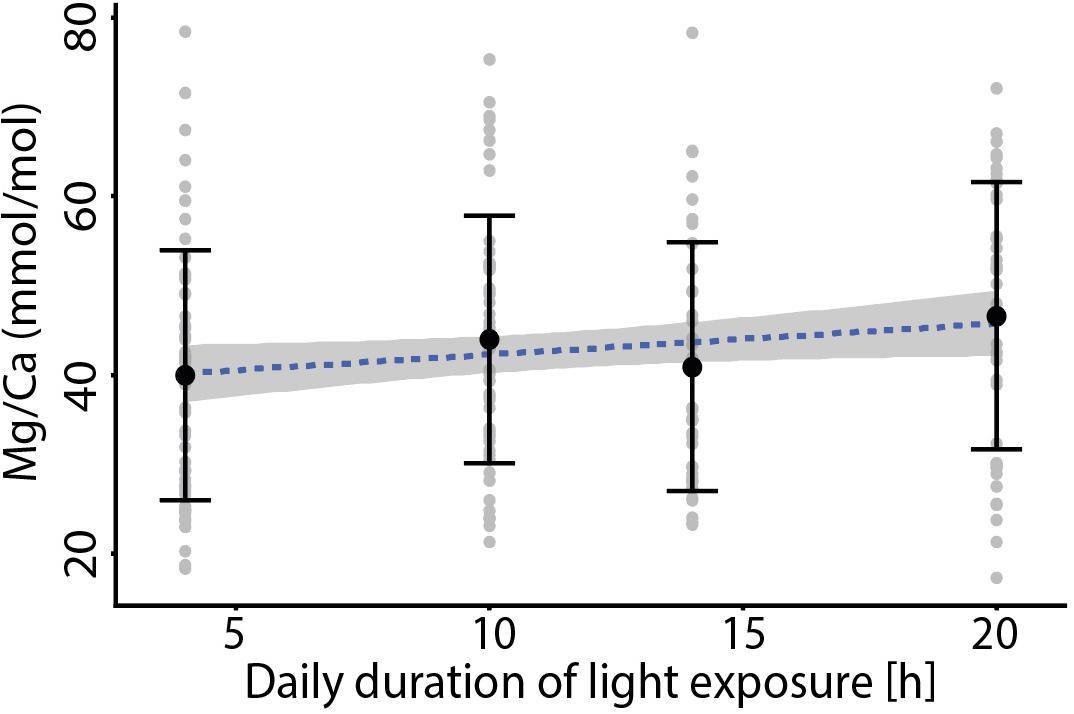
Figure 3. Foraminiferal Mg/Ca vs. the duration of light exposure per day for the four treatments that included increased Ba/CaSW during darkness, with no significant trend between the different treatments. Black markers indicate average values ± standard deviation. The specimens grown at 24 h light were not included since they produced very few new chambers compared to those of the other treatments.
Discussion
The Relation Between Darkness and Foraminiferal Growth
Chamber addition rates were similar for all day lengths (Figure 1), except for the longest day length (24 h light), with less growth and for the 10 h day length, where growth was slightly higher. Most conditions were thus sufficiently favorable for foraminifera to grow similarly, well and hence differences in elemental composition in these groups are not likely caused by stress. The fact that the 24 h of light exposure resulted in a strong reduction in chamber addition, suggests that darkness is necessary for calcification. This is in line to results presented by Nobes et al. (2008) who observed no significant difference in growth rates of Amphistegina spp. cultured under three different light intensities over the course of a 96 day incubation period, though in their experiment, mortality was highest amongst specimens cultured under highest light intensity. Nobes et al. (2008) concluded that light is not a limiting factor for the growth of symbiont bearing foraminifera and that for some species, less light can result in more growth.
Day vs. Night Calcification
Since foraminiferal Ba/CaCC correlates with sea water Ba/CaSW (Lea and Boyle, 1991; Lea and Spero, 1992; de Nooijer et al., 2017a) and is not significantly impacted by other environmental conditions (Lea and Spero, 1994; Hönisch et al., 2011), average test Ba/CaCC from our experiment reflect the relative amount of calcite produced during night- vs. day time. Endmember concentrations, assuming a specimen added calcite only during “day” or only during “night,” are calculated using the Ba concentrations from the culture media and the partition coefficient (DBa) for A. lessonii (0.33 ± 22%; de Nooijer et al., 2017a). Calcite precipitated during “day” should hence have a Ba/CaCC of 1.5 μmol/mol (±22%), while calcite precipitated during “night” should have a Ba/CaCC of 6.3 μmol/mol (±22%). These values are used as endmembers for our linear mixing model. Specimens exposed to sea water with elevated Ba for a longer time interval (i.e., longer “night”), have higher average Ba/CaCC and vice versa (Figure 2). These calculated endmember concentrations can be recognized by the plateaus of high or low Ba/Ca across the test wall in the laser ablation measurement profiles (Figure 4).
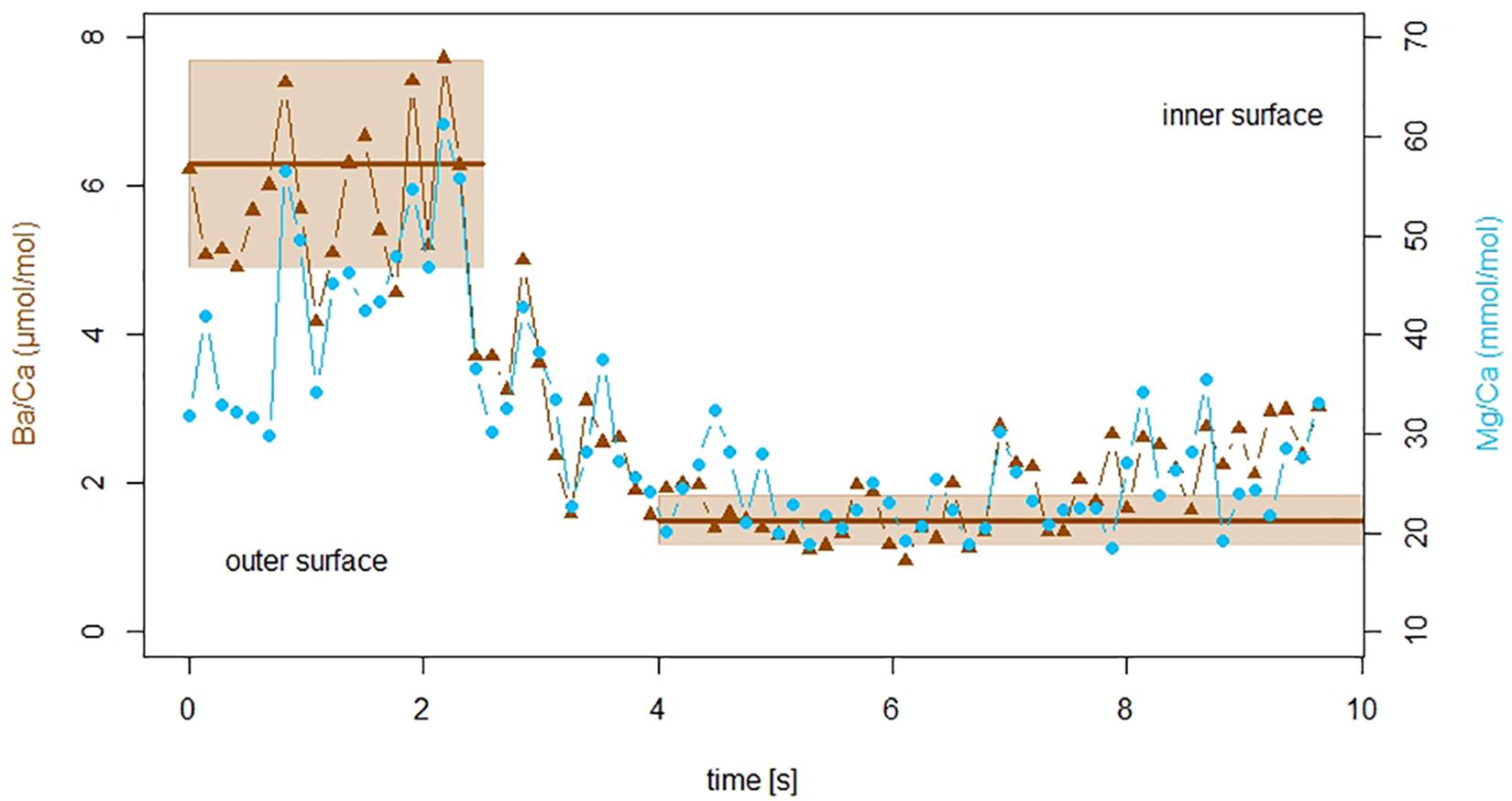
Figure 4. Laser ablation ICP-MS profile of a specimen of A. lessonii from the 10 h light treatment. Ba/Ca ratio of the F-chamber shown in brown triangles, blue circles indicate Mg/Ca ratios. The x-axis represents duration of the measurement, while the laser was moving fully through the test wall from the outermost layer (left) toward the inside (right) of the chamber. Ba/CaSW had been enhanced during night time, the two plateaus in the profile represent ratios expected based on the sea water concentration and the partition coefficient (±22% uncertainty) indicated by brown lines and hatched areas. The outer part of the shell with elevated Ba/Ca formed during high Ba/CaSW exposure (night time), while the lower plateau without elevated Ba/Ca ratios formed during low Ba/CaSW exposure (day time). Mg/CaSW was not manipulated, yet Mg/CaCC correlates with Ba/CaCC, showing that the incorporation of Mg varies between day and nighttime.
Based on Ba partitioning and this observation, the Ba/CaCC of each specimen was translated into the relative amount of calcite formed during “day” and “night.” The distribution of Ba shows that the majority of chambers formed partially during both periods, with only few chambers showing an average Ba/CaCC that matches one of the two endmembers (Figure 2). The distribution of single-chamber Ba/CaCC also shows that the “night” is somewhat overrepresented in the total population. The ratio of duration of darkness vs. light (day length) does not correspond 1:1 to the ratio of low-Ba-calcite to high-Ba-calcite. If the timing and duration of foraminiferal calcification would be fully random, however, high-Ba/low-Ba calcite would on average match the duration of night/day. Since this is not the case, the timing of the start of calcification and/or the duration of the chamber formation event are likely non-random. For example, Only few specimens in only one treatment (20 h of light exposure per day) finished forming a full chamber under low Ba conditions (i.e., during “day” time). While a large number of specimens formed chambers that consisted of 100% high-Ba-calcite, our results suggest that foraminifera tend to precipitate the majority of their calcite during darkness.
Production of an internal calcium pool (Anderson and Faber, 1984; Erez, 2003) and its utilization during chamber formation potentially biases our results. If the internal calcium pool was derived from seawater and therefore a source of the incorporated Ba, the measured Ba/Ca may reflect the Ba/Ca of the medium prior to the calcification event. However, even in the treatment with longest light exposure (20 h), specimens with Ba/CaCC indicating 100% daytime calcite are uncommon, but are present (Figure 2). If calcification uses a previously built internal calcium pool, the longevity of this pool is much shorter (<20 h) than previously suggested [2–3 days; (Erez, 2003)]. This discrepancy may be caused by inter-species differences in the size or contribution of this pool. Calcification in A. lessonii may be more similar to that of other species for which the absence of an internal calcium pool was shown (Nehrke et al., 2013). Furthermore, the observed sharp in- or decrease in the laser ablation profile of Ba/Ca in the F-chamber (Figure 4) does not support precipitation from a pool with a given Ba/Ca. In contrast, it more likely reflects Ba-uptake during chamber formation.
Elevated seawater Ba/Ca is suspected to promote Mg incorporation in large benthic foraminifera (de Nooijer et al., 2017a). To evaluate the impact of the day-night cycle on Mg incorporation it is therefore necessary to correct for the effect of increased Ba/Ca during the night time incubations. An increase of 1 μmol/mol in Ba/CaCC caused by increased Ba/CaSW is associated with an increase of 3.1 mmol/mol Mg/CaCC in A. lessonii under otherwise constant conditions (de Nooijer et al., 2017a). A similar relationship between Mg/CaCC and Ba/CaCC has been found for some planktonic foraminifera (Hathorne et al., 2009; Hori et al., 2018) but not for others (Hori et al., 2018), suggesting species-specific controls on Ba- and Mg-incorporation. Even though no causal explanation or potential mechanism for this effect is presently known, we corrected our data for this observed co-variation. To this end, the correlation between elevated Ba/Cacc and Mg/Cacc presented in de Nooijer et al. (2017a) was first corrected to the correct Mg concentrations based on the experimental temperatures used. The difference in Mg/Ca [mmol/mol] due to a 4°C temperature offset was calculated using a previously published Mg/Ca-temperature calibration for this species (van Dijk et al., 2019). In contrast, the Ba concentrations were not adjusted, since temperature is reported to have no effect on foraminiferal Ba/Cacc (Lea and Spero, 1994; Hönisch et al., 2011). The resulting linear regression of Mg/Cacc [mmol/mol] = 3.1 * Ba/Cacc [μmol/mol] – 10.36 (at 21°C) was applied to correct the potential impact of Ba on the Mg concentrations in this study. The temperature corrected regression was subtracted from the observed relationship between Ba/Ca and Mg/Ca [Mg/Cacc (mmol/mol) = 7.84 * Ba/Cacc (μmol/mol) + 5.03], to result in a final, Ba-effect corrected regression of Mg/Cacc [mmol/mol] = 4.74 * Ba/Cacc [μmol/mol] + 10.39. Explaining the entire observed correlation with Ba addition only would imply a 2.5 times higher sensitivity of Mg to Ba addition, which is very unlikely as we used the same species, same experimental set up and analytical approach. Overall the impact of Ba (in the micro mole range) on Mg (in the milli mole range) is likely very limited because of their very different relative contributions to the carbonate.
Impact of Day Length on Element Incorporation
Foraminiferal Mg/Ca is significantly correlated with the calculated portion of carbonate formed at “night” (Figure 5). Three treatments show very similar relationships, however, the 20 h light exposure group is offset from the others, although the slope of the regression with percentage of carbonate formed in the dark is similar [Figure 5 and Table 3a].
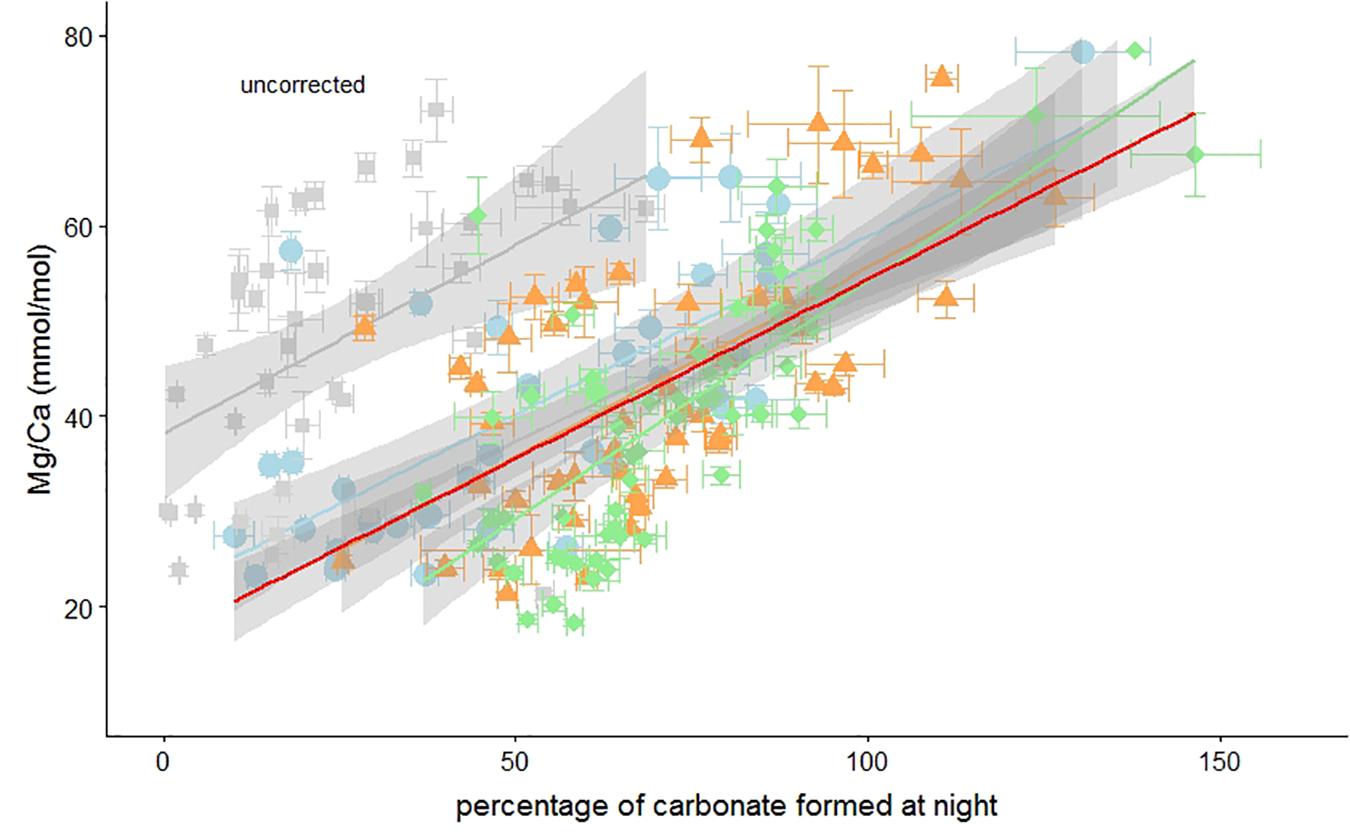
Figure 5. Uncorrected foraminiferal Mg/Ca vs. the percentage of carbonate formed during darkness. Elevated Ba concentrations in the culture medium at night has been used to calculate the percentage of carbonate formed during darkness (Figure 2). Individual measurements of specimens from the 4, 10, 14, and 20 h light per day treatment are indicated by green diamonds, orange triangles, blue circles, and gray squares, respectively. Horizontal and vertical error bars represent ± SEM on the Ba/Ca and Mg/Ca, respectively. Regressions can be found in Table 3a. Percentages over 100% are possible due to the uncertainty in the Ba/CaCC-Ba/CaSW regression (de Nooijer et al., 2017a).
The absence of darkness clearly reduced chamber addition rates and therefore short (i.e., 4 h) nights may have had a minor effect on calcification. We hypothesize that although chamber addition was not hampered (Figure 1), the 4-h night duration altered Mg incorporation in these foraminifera and offset the Mg/Ca-%calcite formed at night relationship (Figure 5). Even after correcting for a potential effect of elevated Ba on Mg incorporation, Mg/Ca in the foraminiferal calcite is significantly correlated with the percentage of calcite that was formed during darkness [Figure 6 and Table 3b].
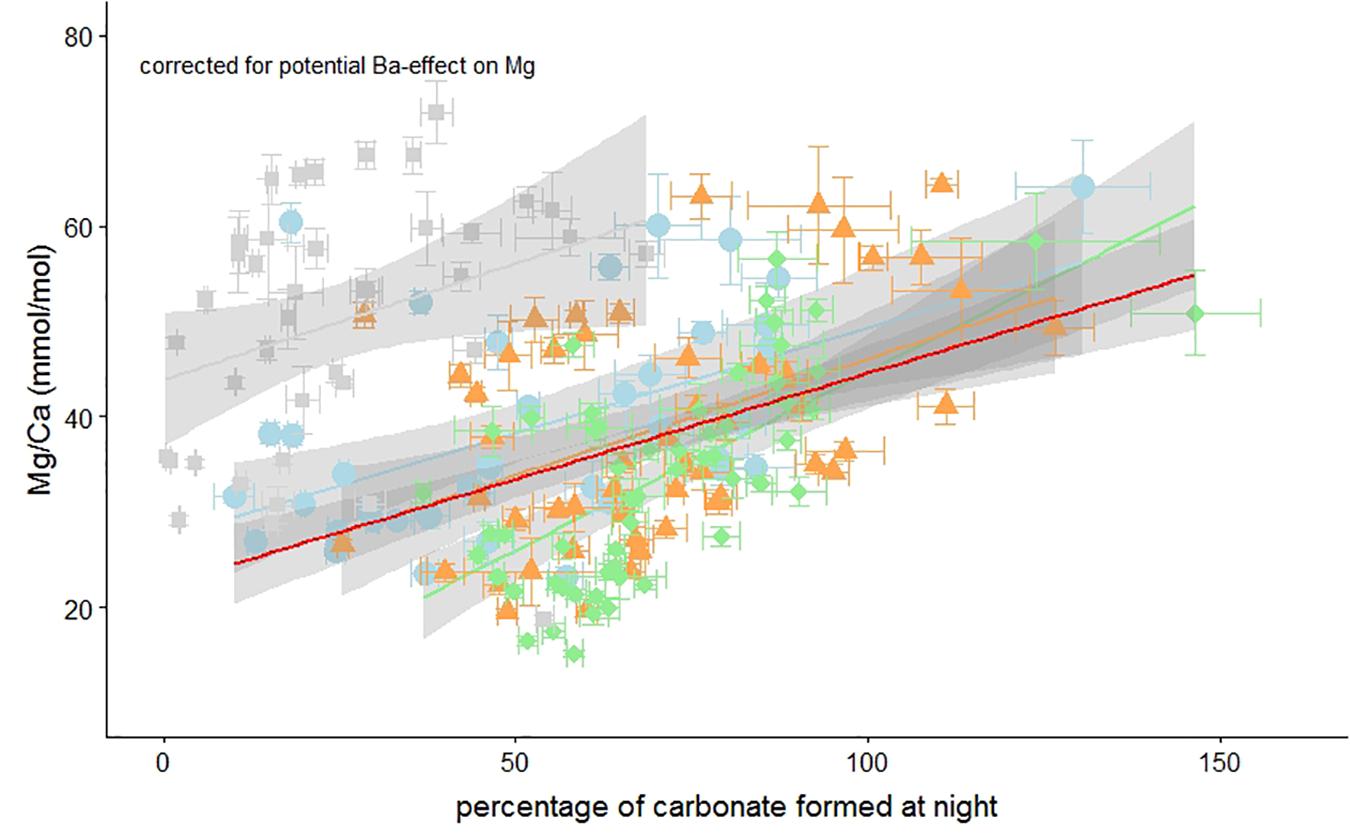
Figure 6. Foraminiferal Mg/Ca vs. the percentage of carbonate formed during darkness corrected for a potential impact of the Ba-label on the Mg-content. Individual measurements of specimens from the 4, 10, 14, and 20 h light per day treatment are indicated by green diamonds, orange triangles, blue circles, and gray squares, respectively. Horizontal and vertical error bars represent ± SEM on the Ba/Ca and Mg/Ca, respectively. Regressions can be found in Table 3a. Percentages over 100% are possible due to the uncertainty in the Ba/CaCC-Ba/CaSW regression (de Nooijer et al., 2017a).
Correlating the Mg/Ca to the portion of carbonate formed during “day” vs. the portion formed at “night” shows a relationship that, after correcting for a potential Ba-effect on Mg incorporation, can be described with the following equation:
Therefore the calcite end-member corresponding to only daylight (%dark_calcite = 0) would have a Mg/Cacc of 22.5 mmol/mol, while calcite formed completely during darkness (%dark_calcite = 100) has a much higher Mg/Cacc of 45.5 mmol/mol (Figure 6). The slope in Mg/Ca as a function of %dark_calcite is therefore an increase of about 2.3 mmol/mol Mg/Ca per 10% decrease of light exposure duration during calcification. Translating this to temperature reconstructions (van Dijk et al., 2019), implies an effect of Δ+1.36°C in A. lessonii per 10% increase in light exposure during calcification. Clearly such changes could be of major importance, especially when investigating temperatures at high latitudes where differences in day lengths are substantial, assuming that high latitude inhabitants respond similarly, to day/night cyclicity as A. lessonii. For A. lessonii the average Mg/Ca of the four different day length treatment is not significantly different (Figure 3), therefore temperature reconstructions do not have to be corrected for a latitudinal effect on day length and thus foraminiferal Mg/Ca. Due to potential species specific effects, this remains to be tested for other benthic as well as planktonic species.
Although the effect of light exposure on magnesium incorporation is evident, the underlying mechanism is not. Photosynthesis changes the pH and saturation state in the microenvironment of the foraminifera, pH can change from 8.03 under dark conditions to 8.12 during light exposure in A. lobifera (Köhler-Rink and Kühl, 2000). An increase of 0.1 pH unit has been shown by Lea et al. (1999) to lead to a decrease of 6% in the Mg/Ca in the tests of planktonic foraminifera such as O. universa and Globigerina bulloides although no correlation between the Mg content of the tests and light or dark exposure was found. Dependency of Mg incorporation on the inorganic carbon system has also been shown for large benthic foraminifera such as Operculina ammonoides (Evans et al., 2018), but no significant correlation between pCO2 (co-varying with pH) and Mg/Ca has been found for large benthic foraminifera Amphistegina gibbosa (van Dijk et al., 2017). The latter suggests that it is unlikely that pH is explains the here observed changes in Mg/Ca as a function of light exposure in. Although the calcification process may differ between (and within) planktonic and benthic genera, the sensitivity of planktonic Mg/Ca to seawater pH may indicate that the calcite chemistry of planktonic species may be even more vulnerable to the effect of day/night changes.
The large variability of elemental ratios between as well as within species, that is often observed in culture studies with controlled, constant environmental factors (e.g., Hathorne et al., 2009; de Nooijer et al., 2014; Geerken et al., 2018) appears to be at least partly caused by differences in the exposure of the different specimens to light during chamber formation. These differences, in turn, could be caused by differences in the timing of foraminiferal calcification such as duration or time of calcification onset. Segev and Erez (2006) as well as Hathorne et al. (2009) suggested that a large part of variation observed in foraminiferal Mg/Ca could be caused by physiological processes in addition to environmental impacts. Similar changes in Mg/Cacc occur in both symbiont bearing and -barren species, indicating that symbionts are not the driving factor for these variations.
Conclusion
In addition to previously known intra shell variations in Mg/Ca that correlated with a dark-light cycle (e.g., Spero et al., 2015; Fehrenbacher et al., 2017), here we show that also average chamber Mg/Ca values are strongly affected by light exposure. Though a population’s average Mg/Ca does not appear to be influenced by different light exposure caused by natural factors such as seasons as well as latitude, since the average of Mg/Ca in all four treatments with daily light exposure ranging from 4 to 20 h is very similar. Even though there are substantial effects on the individual foraminifera, this should not introduce a bias during application as a paleothermometer, when large numbers of specimens are analyzed. Individual timing of chamber formation, duration and time of beginning, though have a strong impact on an individual chamber’s Mg/Ca. An A. lessonii chamber completely formed in the dark is on average 23 mmol/mol increased in Mg/Ca compared to a chamber completely formed during light exposure. This factor explains a large part of inter-specimen variation previously observed but unexplained in culture studies (e.g., de Nooijer et al., 2014; Geerken et al., 2018).
Data Availability
The datasets generated for this study are available on request to the corresponding author.
Author Contributions
All authors designed the study. LD and LdN carried out the sampling and laboratory work. LD performed the measurements, carried out the data analysis, and drafted the manuscript. LdN and G-JR critically revised the manuscript. All authors approved the final version of the manuscript for publication.
Conflict of Interest Statement
The authors declare that the research was conducted in the absence of any commercial or financial relationships that could be construed as a potential conflict of interest.
Acknowledgments
We thank Max Janse (Burgers’ Zoo, Arnhem, The Netherlands) for providing foraminiferal specimens. Inge van Dijk helped with the preparations for this study, while Wim Boer and Bob Koster provided vital technical support. This work was carried out under the program of the Netherlands Earth System Science Centre (NESSC), financially supported by the Ministry of Education, Culture and Science (OCW) (grant no. 024.002.001).
References
Al-Horani, F. A., Al-Moghrabi, S. M., and De Beer, D. (2003). The mechanism of calcification and its relation to photosynthesis and respiration in the scleractinian coral Galaxea fascicularis. Mar. Biol. 142, 419–426. doi: 10.1007/s00227-002-0981-8
Anderson, O. R., and Faber, W. W. (1984). An estimation of calcium carbonate deposition rate in a planktonic foraminifer globigerinoides sacculifer using 45Ca as a tracer; a recommended procedure for improved accuracy. J. Foraminifer. Res. 14, 303–308. doi: 10.2113/gsjfr.14.4.303
de Nooijer, L., Brombacher, A., Mewes, A., Langer, G., Nehrke, G., Bijma, J., et al. (2017a). Ba incorporation in benthic foraminifera. Biogeosciences 14, 3387–3400. doi: 10.5194/bg-14-3387-2017
de Nooijer, L. J., van Dijk, I., Toyofuku, T., and Reichart, G. J. (2017b). The impacts of seawater mg/ca and temperature on element incorporation in benthic foraminiferal calcite. Geochem. Geophys. Geosyst. 18, 3617–3630. doi: 10.1002/2017GC007183
de Nooijer, L. J., Hathorne, E. C., Reichart, G. J., Langer, G., and Bijma, J. (2014). Variability in calcitic Mg/Ca and Sr/Ca ratios in clones of the benthic foraminifer Ammonia tepida. Mar. Micropaleontol. 107, 32–43. doi: 10.1016/j.marmicro.2014.02.002
Dissard, D., Nehrke, G., Reichart, G. J., Nouet, J., and Bijma, J. (2009). Effect of the fluorescent indicator calcein on Mg and Sr incorporation into foraminiferal calcite. Geochem. Geophys. Geosyst. 10, 1–13. doi: 10.1029/2009GC002417
Dueñas-Bohórquez, A., Raitzsch, M., de Nooijer, L. J., and Reichart, G.-J. (2011). Independent impacts of calcium and carbonate ion concentration on Mg and Sr incorporation in cultured benthic foraminifera. Mar. Micropaleontol. 81, 122–130. doi: 10.1016/j.marmicro.2011.08.002
Erez, J. (2003). The source of ions for biomineralization in foraminifera and their implications for paleoceanographic proxies (review). Rev. Mineral. Geochem. 54:115. doi: 10.2113/0540115
Ernst, S., Janse, M., Renema, W., Kouwenhoven, T., Goudeau, M.-L., and Reichart, G.-J. (2011). Benthic foraminifera in a large indo-pacific coral reef aquarium. J. Foraminifer. Res. 41, 101–113. doi: 10.2113/gsjfr.41.2.101
Evans, D., Müller, W., and Erez, J. (2018). Assessing foraminifera biomineralisation models through trace element data of cultures under variable seawater chemistry. Geochim. Cosmochim. Acta 236, 198–217. doi: 10.1016/j.gca.2018.02.048
Fehrenbacher, J. S., Russell, A. D., Davis, C. V., Gagnon, A. C., Spero, H. J., Cliff, J. B., et al. (2017). Link between light-triggered Mg-banding and chamber formation in the planktic foraminifera neogloboquadrina dutertrei. Nat. Commun. 8, 1–10. doi: 10.1038/ncomms15441
Geerken, E., de Nooijer, L. J., van Dijk, I., and Reichart, G. J. (2018). Impact of salinity on element incorporation in two benthic foraminiferal species with contrasting magnesium contents. Biogeosciences 15, 2205–2218. doi: 10.5194/bg-15-2205-2018
Hathorne, E. C., James, R. H., and Lampitt, R. S. (2009). Environmental versus biomineralization controls on the intratest variation in the trace element composition of the planktonic foraminifera G. inflata and G. scitula. Paleoceanography 24, 1–14. doi: 10.1029/2009PA001742
Holland, K., Eggins, S. M., Hönisch, B., Haynes, L. L., and Branson, O. (2017). Calcification rate and shell chemistry response of the planktic foraminifer Orbulina universa to changes in microenvironment seawater carbonate chemistry. Earth Planetar. Sci. Lett. 464, 124–134. doi: 10.1016/j.epsl.2017.02.018
Hönisch, B., Allen, K. A., Lea, D. W., Spero, H. J., Eggins, S. M., Arbuszewski, J., et al. (2013). The influence of salinity on Mg/Ca in planktic foraminifers - Evidence from cultures, core-top sediments and complementary δ18O. Geochim. Cosmochim. Acta 121, 196–213. doi: 10.1016/j.gca.2013.07.028
Hönisch, B., Allen, K. A., Russell, A. D., Eggins, S. M., Bijma, J., Spero, H. J., et al. (2011). Planktic foraminifers as recorders of seawater Ba/Ca. Mar. Micropaleontol. 79, 52–57. doi: 10.1016/j.marmicro.2011.01.003
Hori, M., Shirai, K., Kimoto, K., Kurasawa, A., Takagi, H., Ishida, A., et al. (2018). Chamber formation and trace element distribution in the calcite walls of laboratory cultured planktonic foraminifera (Globigerina bulloides and Globigerinoides ruber). Mar. Micropaleontol. 140, 46–55. doi: 10.1016/j.marmicro.2017.12.004
Köhler-Rink, S., and Kühl, M. (2000). Microsensor studies of photosynthesis and respiration in larger symbiotic foraminifera. I The physico-chemical microenviron- ment of Marginopora vertebralis, Amphistegina lobifera and Amphisorus hemprichii. Mar. Biol. 137, 473–486. doi: 10.1007/s002270000335
Köhler-Rink, S., and Kühl, M. (2005). The chemical microenvironment of the symbiotic planktonic foraminifer orbulina universa. Mar. Biol. Res. 1, 68–78. doi: 10.1080/17451000510019015
Lea, D. W., and Boyle, E. A. (1991). Barium in planktonic foraminifera. Geochim. Cosmochim. Acta. 55, 3321–3331. doi: 10.1016/0016-7037(91)90491-M
Lea, D. W., Mashiotta, T. A., and Spero, H. J. (1999). Controls on magnesium and strontium uptake in planktonic foraminifera determined by live culturing. Geochim. Cosmochim. Acta. 63, 2369–2379. doi: 10.1016/s0016-7037(99)00197-0
Lea, D. W., and Spero, H. J. (1992). Experimental determination of barium uptake in shells of the planktonic foraminifera Orbulina universa at 22°C. Geochim. Cosmochim. Acta 56, 2673–2680. doi: 10.1016/0016-7037(92)90352-J
Lea, D. W., and Spero, H. J. (1994). Assessing the reliability of paleochemical tracers: barium uptake in the shells of planktonic foraminifera. Paleoceanography 9, 445–452. doi: 10.1029/94PA00151
Mezger, E. M., de Nooijer, L. J., Boer, W., Brummer, G. J. A., and Reichart, G. J. (2016). Salinity controls on Na incorporation in Red Sea planktonic foraminifera. Paleoceanography 31, 1562–1582. doi: 10.1002/2016PA003052
Nehrke, G., Keul, N., Langer, G., de Nooijer, L. J., Bijma, J., and Meibom, A. (2013). A new model for biomineralization and trace-element signatures of Foraminifera tests. Biogeosciences 10, 6759–6767. doi: 10.5194/bg-10-6759-2013
Nobes, K., Uthicke, S., and Henderson, R. (2008). Is light the limiting factor for the distribution of benthic symbiont bearing foraminifera on the Great Barrier Reef? J. Exp. Mar. Biol. Ecol. 363, 48–57. doi: 10.1016/j.jembe.2008.06.015
Nuernberg, D. (1995). Magnesium in tests of neogloboquadrina pachyderma sinistral from high northern and southern latitudes. J. Foraminifer. Res. 25, 350–368. doi: 10.2113/gsjfr.25.4.350
Segev, E., and Erez, J. (2006). Effect of Mg/Ca ratio in seawater on shell composition in shallow benthic foraminifera. Geochemistr. Geophys. Geosyst. 7:Q02P09, doi: 10.1029/2005GC000969
Spero, H. J., Eggins, S. M., Russell, A. D., Vetter, L., Kilburn, M. R., and Hönisch, B. (2015). Timing and mechanism for intratest Mg/Ca variability in a living planktic foraminifer. Earth Planet. Sci. Lett. 409, 32–42. doi: 10.1016/j.epsl.2014.10.030
van Dijk, I., Barras, C., de Nooijer, L. J., Mouret, A., Geerken, E., Oron, S., et al. (2019). Coupled Ca and inorganic carbon uptake suggested by magnesium and sulfur incorporation in foraminiferal calcite. Biogeosci. Discuss. 16, 2115–2130. doi: 10.5194/bg-16-2115-2019
Keywords: Mg/Ca carbonates, foraminifera (benthic), light – dark, LA-IC-MS data, paleoclimate proxy
Citation: Dämmer LK, de Nooijer LJ and Reichart G-J (2019) Light Impacts Mg Incorporation in the Benthic Foraminifer Amphistegina lessonii. Front. Mar. Sci. 6:473. doi: 10.3389/fmars.2019.00473
Received: 08 April 2019; Accepted: 12 July 2019;
Published: 26 July 2019.
Edited by:
Benoit Thibodeau, The University of Hong Kong, Hong KongReviewed by:
Kaoru Kubota, Japan Agency for Marine-Earth Science and Technology, JapanDirk de Beer, Max-Planck-Gesellschaft (MPG), Germany
Copyright © 2019 Dämmer, de Nooijer and Reichart. This is an open-access article distributed under the terms of the Creative Commons Attribution License (CC BY). The use, distribution or reproduction in other forums is permitted, provided the original author(s) and the copyright owner(s) are credited and that the original publication in this journal is cited, in accordance with accepted academic practice. No use, distribution or reproduction is permitted which does not comply with these terms.
*Correspondence: Linda K. Dämmer, Linda.daemmer@NIOZ.nl