- 1Faculty of Science and Technology, Life and Environmental Sciences, Bournemouth University, Poole, United Kingdom
- 2Artecology Ltd., Sandown, United Kingdom
Coastal squeeze caused by sea level rise threatens the size, type, and quality of intertidal habitats. Along coastlines protected by hard defenses, there is a risk that natural rocky shore habitats will be lost, with the remaining assemblages, characteristic of hard substrata, confined to sea walls and breakwaters. These assemblages are likely to be less diverse and different to those found on natural shores, as these structures lack features that provide moist refugia required by many organisms at low tide, such as pools and crevices. Engineering solutions can help mitigate the impact of sea level rise by creating habitats that retain water on existing structures. However, as experimental trials are strongly affected by local conditions and motivations, the development of new techniques and solutions are important to meet the needs of local communities and developers. Following a small-scale community project, a feasibility study retrofitted five concrete-cast artificial rock pools (“Vertipools”) on a vertical seawall on the south coast of England. After 5 years, the artificial pools increased the species richness of the sea wall and attracted mobile fauna previously absent, including fish and crabs. The Vertipools had assemblages which supported several functional groups including predators and grazers. Although disturbance of algal assemblages on the seawall from the retrofitting process was still evident after 3 years, succession to full canopy cover was underway. Collaboration between policy makers, ecologists, children and artists produced an ecologically sensitive design that delivered substantial benefits for biodiversity, which can be adapted and scaled-up to both mitigate habitat loss and enhance coastal recreational amenity.
Introduction
Climate change and biodiversity loss are two of the greatest threats to coastal areas around the world (IUCN, 2016; Committee on Climate Change UK, 2018; WWF, 2018). Not only do coastal areas support important biodiversity, act as carbon sinks and provide a buffer in front of sea defenses, they also have an important role in tourism and the provision of cultural services (Committee on Climate Change UK, 2018).
Coastal protection provided by seawalls forms a barrier between the land and sea, preventing the natural migration of the coastline. These barriers, coupled with increased sea levels, are resulting in “coastal squeeze,” which occurs when the high water mark is fixed by a defense structure and the low water mark is moving landward due to sea level rise, resulting in substantial losses of intertidal habitats (Pontee, 2011). The construction of coastal defense structures results in the steepening of the shore profile (Jackson and McIlvenny, 2011; Committee on Climate Change UK, 2018) which creates less space for colonization and compressed species zonation (Kendall et al., 2004). This, coupled with the lack of water retention which would naturally occur in crevices and pools (Firth et al., 2013), results in a poor quality habitat. Habitat heterogeneity is also generally absent on most artificial structures; in contrast, natural rocky shores have a high variety of surface textures, crevices, overhangs and pools, which provide suitable refugia and habitats for a diverse range of species (Connell, 1972; Underwood et al., 2008).
Water retention is important on a rocky shore as it creates refugia from desiccation stress and predation during periods of low tide (Firth et al., 2013; White et al., 2014). Although the physico-chemical composition of rock pools is known to fluctuate diurnally and seasonally with changes in temperature, pH, salinity and oxygen saturation, these fluctuations are not as extreme as on the emergent rock surfaces (Daniel and Boyden, 1975; Metaxas and Scheibling, 1993). Rock pools are known to extend the limits of distribution for intertidal species, including larger brown algae (Fucus spp.), limpets and mussels (Green, 1971). Photosynthesis of algae within the pools can influence oxygen and carbon dioxide levels, which in turn affect the pH of the water (Metaxas and Scheibling, 1993; Björk et al., 2004). Intertidal fish also use rock pools as habitats, but rock pools decline in abundance as the shore height increases (Bennett and Griffiths, 1984; Zander et al., 1999). White et al. (2014) found that more complex rock pools with ledges and algal cover resulted in higher abundances and diversity of intertidal fish.
Ecological enhancement schemes that integrate ecology with engineering can create potential solutions to mitigate low habitat heterogeneity and produce multifunctional structures that provide coastal protection and also incorporate suitable habitats for marine species (Firth et al., 2013; Dafforn et al., 2015; Evans et al., 2015; Morris et al., 2016). Trials have indicated that creating artificial features which increase water retention and habitat heterogeneity can provide opportunities for colonization by a variety of species (Chapman and Blockley, 2009; Browne and Chapman, 2011; Firth et al., 2014a; Evans et al., 2015). There have been several examples of trials including the use of artificial panels (Moschella et al., 2005; Borsje et al., 2011; Loke and Todd, 2016), manipulations of concrete (Chapman and Underwood, 2011; Firth et al., 2016a), drilling holes into existing artificial structures (Martins et al., 2010; Firth et al., 2014b; Evans et al., 2015; Hall et al., 2018) and by creating pre-cast concrete units [See Firth et al. (2016b) for review]. Browne and Chapman (2011, 2014) deployed pre-cast flowerpots at different tidal heights on a seawall in Sydney to mimic natural rock pools; although some flowerpots were dislodged by waves, the remaining ones increased the biodiversity of the seawall by attracting novel sessile species. Morris et al. (2017) investigated the larger scale effects of the flowerpots by studying the mobile communities. In addition, they added artificial turf to some of the pots in order to see the effects on the native and non-native sessile communities. The study found higher densities of mobile and sessile species in pots without artificial turf, implying that the turf prevented particular species from colonizing the pots. The outcome of experimental trials are strongly affected by local conditions and motivations (Airoldi et al., 2005), therefore development of new techniques and solutions are important to meet the needs of local communities and developers.
Shelving the Coast Project
As part of the community science project “Shelving the Coast” on the Isle of Wight in southern England, artists, school children and ecologists designed and created a series of structures subsequently named and referred to here as “Vertipools.” These pre-cast concrete artificial rock pools aimed to vertically extend the intertidal zone to mitigate the effects of sea level rise, which could result in the loss of the existing intertidal rock pools. The Vertipools were designed to be attached to coastal structures, such as vertical seawalls and groynes. The exterior patterns were designed by local primary school children aged between 5 and 7, as part of an educational project on coastal squeeze. The V-shape was inspired by the bow of a ship to deflect wave energy and the hollow insides allow for water retention at low tide (Supplementary Figures S1A–F). The educational program involved three activities; an animation project, song writing and recording exercise and designing the Vertipools exterior surfaces.
The main purpose of this study was to monitor the growth of the assemblages on and within the Vertipools and to determine whether the installation would improve the species diversity of assemblages living on the seawall within the study area. Studies on colonization of hard substrata have shown changes in assemblages and successional processes with an increased number of species and functional groups over time (Benedetti-Cecchi, 2000; Jenkins and Martins, 2010; Herbert et al., 2017). Our general hypothesis is that there will be a community succession over time in the artificial pools and the disturbed areas of the sea wall. The Vertipools were surveyed seasonally in year 2 and 3 to determine the early community succession and then again in year 5 to compare the species richness with the seawall. The following hypotheses were tested:
(1) Vertipools will support a higher species richness than the seawall after 5 years;
(2) Season and year will have a significant effect on the community succession and in turn the species richness and functional groups of the assemblages within the Vertipools during 2015 and 2016;
In order to assess the impact of Vertipool construction on existing assemblages on the seawall the following hypothesis was tested:
(3) The disturbed area of the seawall will have a similar percentage cover of algae to the undisturbed seawall after 3 years.
Materials and Methods
Study Site
The site at Bouldnor is located on the south coast of England approximately 5 km east of Yarmouth on the north west coast of the Isle of Wight (50°42′27.5″N 1°28′57.1″W) (Figure 1). The shore is moderately sheltered with a north facing aspect and a mean tidal range of 2 m. This stretch of coast has been heavily modified and includes a vertical concrete seawall constructed in 1985 (Figure 1). The seawall has well-established zones of marine algae dominated by the brown seaweeds Ascophyllum nodosum and Fucus spiralis. Below the sea wall, the shore is dynamic and truncated, consisting of limestone boulders surrounded by mobile mixed sediments. Natural rock pools which are approximately 30 cm in diameter and 10 cm deep, appear when beach levels are low but are often smothered by sediment. Other natural rock pools occur on nearby protected areas. This site was chosen for the trial due to the low risk of public interference and ease of access for installation and monitoring.
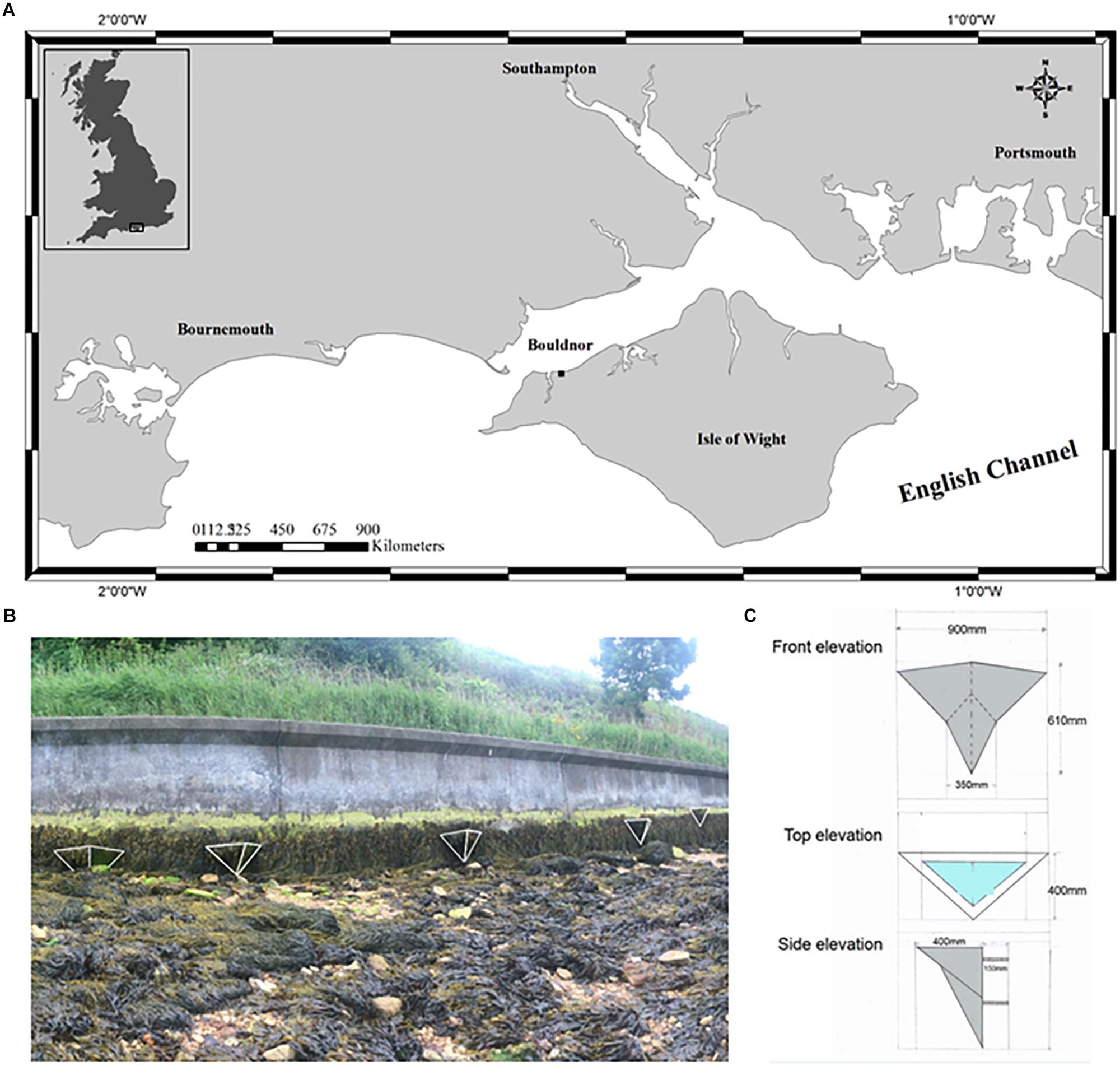
Figure 1. (A) Map illustrating the location of Vertipool test site at Bouldnor, Isle of Wight, (B) Location of the seawall and Vertipools on the seawall, and (C) dimensions of the Vertipools.
Vertipool Description
During September 2013, five concrete wooden-cast Vertipools were installed between Mean Tide Level (MTL) and High Water Neaps (HWN) on the vertical concrete seawall. The outside of each Vertipool was hand sculptured using wet cement to incorporate the design of the school children (Supplementary Figure S1A). The Vertipools weigh 50 to 70 kg and are 900 mm at their widest, 610 mm in height, protrude a maximum 400 mm from the seawall and have an undulating pool depth of 10–200 mm (Figure 1 and Supplementary Figure S1). Three 20 mm diameter M20 stainless steel coach bars attached to a T-shape steel plate were cast into the back of the Vertipools for attachment to the seawall. Three holes were made in the seawall using a 25 mm diameter SDS drill bit to a depth of 150 mm and filled with a marine grade resin bonding agent (Fischer Resin Mortar), before attaching the Vertipools.
Assemblage Monitoring
Seasonal variation in assemblages in the Vertipools was recorded in spring, summer, autumn and winter of the second and third year after installation (2015 and 2016) and long-term changes were recorded in the fifth year after installation (2018). In addition, the effects of retrofitting on the seawall were monitored in the third year after installation (2016), after which the beach level had risen too much to access some of the control quadrats, so sampling was stopped. All fauna and macroalgae were surveyed thoroughly in each habitat using visual in situ non-destructive sampling techniques and organisms were identified to the lowest taxonomic level possible (Evans et al., 2015). Care was taken to survey both the canopy and understory communities.
The Vertipools were sampled seasonally (spring, summer, autumn, and winter) during 2015 and 2016 in order to detect any variation in assemblages. Percentage cover of macroalgae and counts of sessile and mobile fauna were recorded on both the inside and outside of each Vertipool separately. The internal and external surface area of the pool were each approximately 0.25 m2. In addition, 5 years post installation, the Vertipools were surveyed again once in September 2018 and the communities were compared to the adjacent seawall.
The undisturbed seawall adjacent to the Vertipools was sampled using five 0.25 m2 quadrats to record the percentage cover of algae and counts of sessile and mobile fauna at a comparable tidal height (>2 m distant) adjacent to the Vertipools. This data was compared with the data collected in the Vertipools after 5 years.
To measure the effect of retrofitting the Vertipools on the existing algal assemblage on the vertical sea wall, areas scraped and cleared at the time of installation (referred to as “Disturbed Seawall”) were monitored in 2016 (3 years post installation). The limited space available necessitated use of smaller 25 cm × 25 cm quadrats placed either side of each of the five Vertipools to record percentage cover of flora (N = 10).
Statistical Analyses
All data were tested for normality and equal variances and all t-tests and Generalized Linear Mixed Models (GLMMs) were run using R v3.5.1 (R Core Team, 2018), the GLMMs were run using package “lme4” version 1.1-18-1. Species richness (S) were calculated for each Vertipool using the DIVERSE function, which calculates diversity indices, in PRIMER-e V6 (Clarke and Gorley, 2006). To test hypothesis 1, that Vertipools will support greater species richness than the seawall after 5 years, the data recorded from the inside and outside habitats on each Vertipool were combined and averaged (N = 5). A two-sample t-test was used to test for differences in mean species richness between habitats (Vertipool and control seawall).
To test hypothesis 2, whether species richness and abundance of algae in the Vertipools were significantly different between seasons (spring, summer, autumn, and winter) and years (2015 and 2016), a Poisson generalized linear mixed model was used (R Core Team, 2018). The Poisson GLMM included the individual Vertipool as a random variable within the model. The parameters were fitted by maximum likelihood (Bolker et al., 2009).
To test if seasonal variation in assemblages and functional groups were observed in Vertipools during 2015 and 2016, PRIMER-E was used on presence/absence data to create a Jaccard similarity matrix. Assemblages were presented visually using Multidimensional Scaling (MDS) and ANOSIM (analysis of similarity) was used to test for differences in assemblages between seasons and years. All species were classified into the morph-functional groups that were present at the site: Canopy algae, Sub-canopy algae, Filter feeders, Grazers, and Predators (Arenas et al., 2006; Firth et al., 2014a). The five functional groups were used to create a Jaccard similarity matrix on presence/absence data. ANOSIM was used to highlight the variation in functional groups between Season and Year and significant results were explored further using SIMPER (similarity of percentages).
To test hypothesis 3, that the disturbed area of the seawall had similar percentage cover of algae to the undisturbed seawall after 3 years, a beta regression GLM (“betareg” version 3.1–2) was used to test the differences in mean percentage cover of algae between habitats (Disturbed seawall and Control seawall). To assess the similarity of communities, a Jaccard similarity matrix on presence/absence data was used perform an ANOSIM and SIMPER to highlight the variation in species abundance.
Results
Over the 5 years, a total of 24 species were recorded on the inside of the Vertipools, 15 species were found on the outside of the Vertipools, 12 species on the control seawall and eight species on the disturbed seawall (Table 1). During the study, the algal cover on the exterior of the Vertipools developed from a community dominated by opportunistic green algae (Ulva spp.) into a dense over-hanging fucoid canopy (F. spiralis). The algal assemblages inside the Vertipools comprised of fucoids, filamentous and branching algae. Mobile species observed inside the Vertipools included fish (Lipophrys pholis), crabs (Carcinus maenas, Porcellana platycheles) and gastropods Patella vulgata and Littorina obtusata. The shore crab C. maenas was observed inside the Vertipools at various life stages including juvenile, adult and when freshly molted along with the discarded exoskeleton indicating recent ecdysis. The broad clawed porcelain crab (P. platycheles) was recorded in the Vertipool located at the highest tidal height, a location in which it would previously not have been able to survive without the refuge provided by the Vertipool. The brown algae Sargassum muticum found inside the pools and the barnacle Austrominius modestus on the exterior surfaces were the only two non-native species observed.
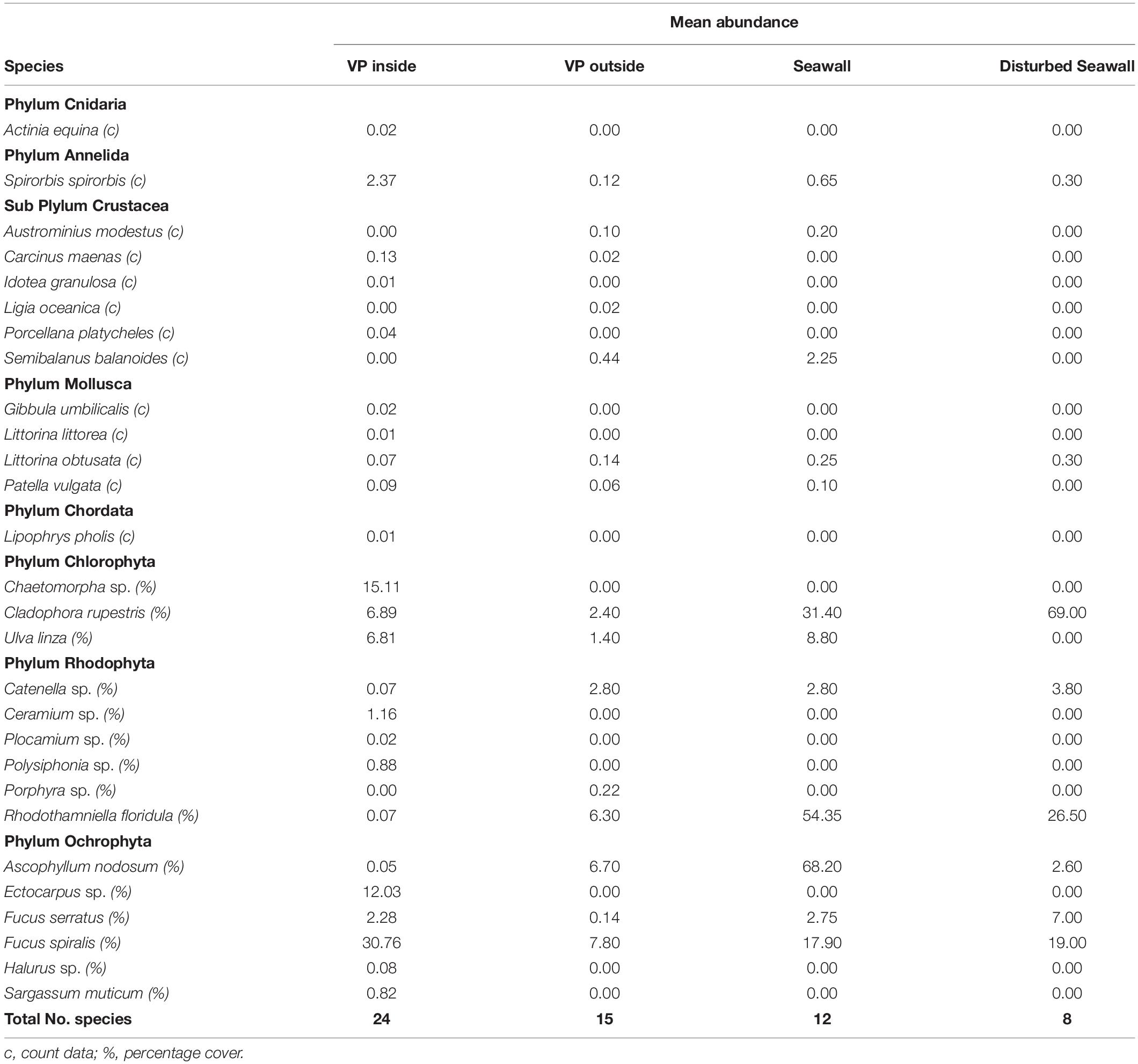
Table 1. Mean abundance of all species found in the four habitats studied; VP Inside (2015, 2016, 2018 n = 50), VP Outside (2015, 2016, 2018 n = 50), Seawall (2016, 2018 n = 20), Disturbed Seawall (2016 n = 10).
Vertipool vs. Seawall
After 5 years the Vertipools showed a significantly greater species richness (mean = 9.6) compared to the seawall (mean = 6.6) (t-test8 = −3.32, P = 0.01) (Figure 2). Ten species were found to be unique to the Vertipools; these were algae Chaetomorpha sp. Ulva lactuca, Ectocarpus sp., F. serratus and Polysiphonia sp., benthic invertebrates Actinia equina and A. modestus and mobile species L. obtusata, C. maenas, and P. platycheles (Table 1). Additionally, the shanny fish L. pholis was found in the pools in March 2015. The barnacle A. modestus was recorded on the exterior rough surface of the Vertipool, but not found on the sea wall. Fronds of the alga F. spiralis attained a greater tidal elevation on the Vertipool compared to the sea wall (Supplementary Figure S1F).
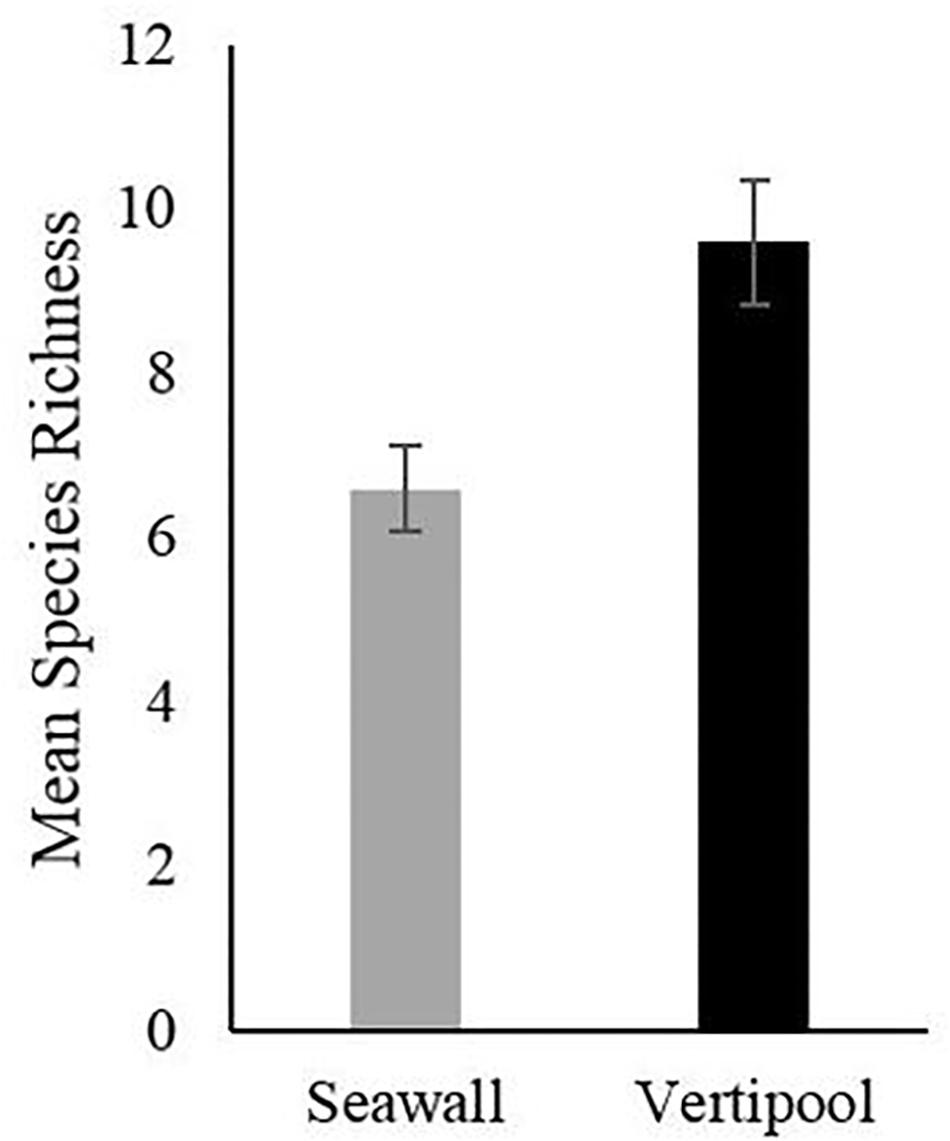
Figure 2. Mean species richness after 5 years recorded in Vertipool and Seawall (Control) (+/– SE., N = 5).
Vertipools
There was a significant difference in species richness between seasons (Table 2) with winter having the highest mean species richness and spring having the lowest mean species richness. There was no significant difference in species richness between years and no significant interaction between seasons and year (Table 2). There was a significant difference in total abundance of algae between both season and year (Table 2), with 2016 having a higher abundance of algae than 2015 and spring having the lowest abundance of algae across all seasons (Table 2).
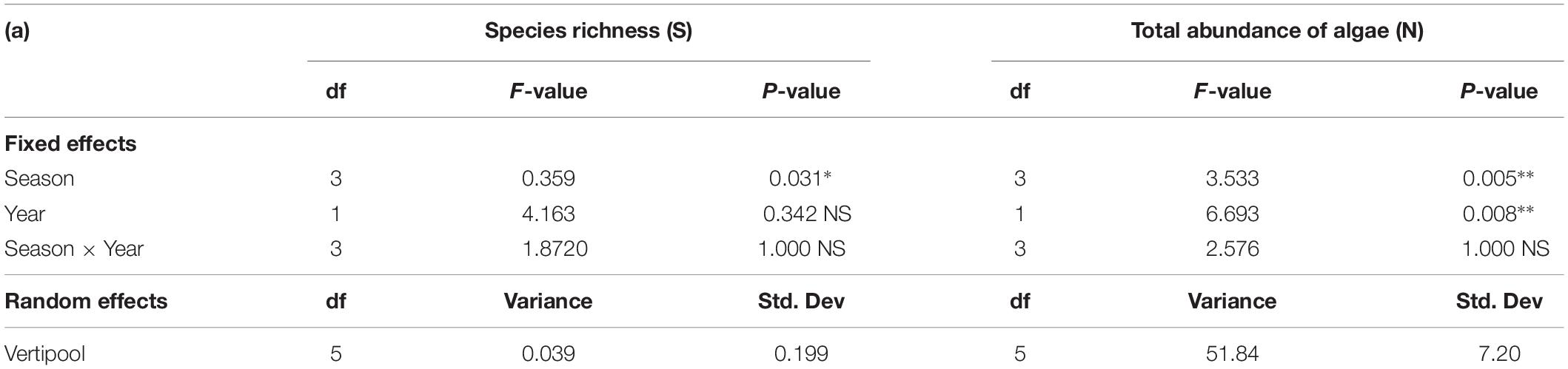
Table 2. Generalized Linear mixed effect model results for Species Richness (S) (all fauna and flora) and Total abundance of algae (N) between season, year and season×year with Vertipool included as a random effect for 2015 and 2016 (∗∗∗P < 0.001, ∗∗P < 0.01,*P < 0.05, NS, Not significant).
There was a significant difference in assemblage composition among seasons (Global R = 0.143, P = 0.0002) and between years (Global R = 0.117, P = 0.001, Figure 3). The MDS showed that the assemblages in 2015 and 2016 are separated with slight overlap and the spring samples are clustered together (Figure 3).
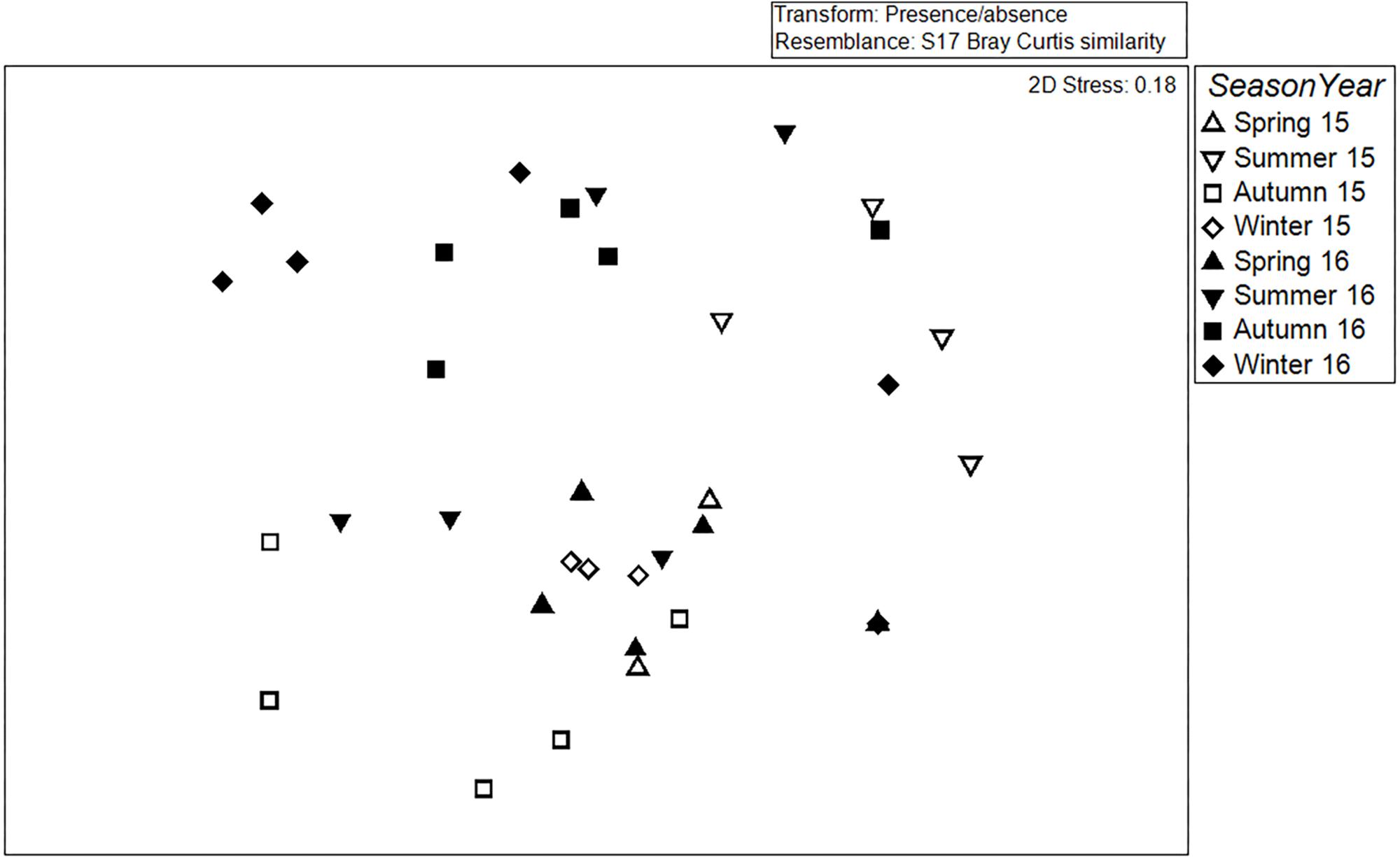
Figure 3. Multidimensional Scaling (MDS) plot comparing assemblages inside the Vertipools in Spring 2015–Winter 2016.
The functional groups of species inside the Vertipools were also significantly different between years (ANOSIM, Global R = 0.083, P = 0.026), but not seasons (ANOSIM, Global R = 0.012, P = 0.336, Figure 4). The Vertipools were initially dominated by sub-canopy algae, yet after spring 2016 it was transformed into canopy-algae dominated community. Spring 2016 heralded the arrival of filter feeders and predators in the Vertipools (Figure 4). Table 3 details the average abundance of functional groups for each year; canopy algae, filter feeders, predators and grazers were all more abundant in 2016.
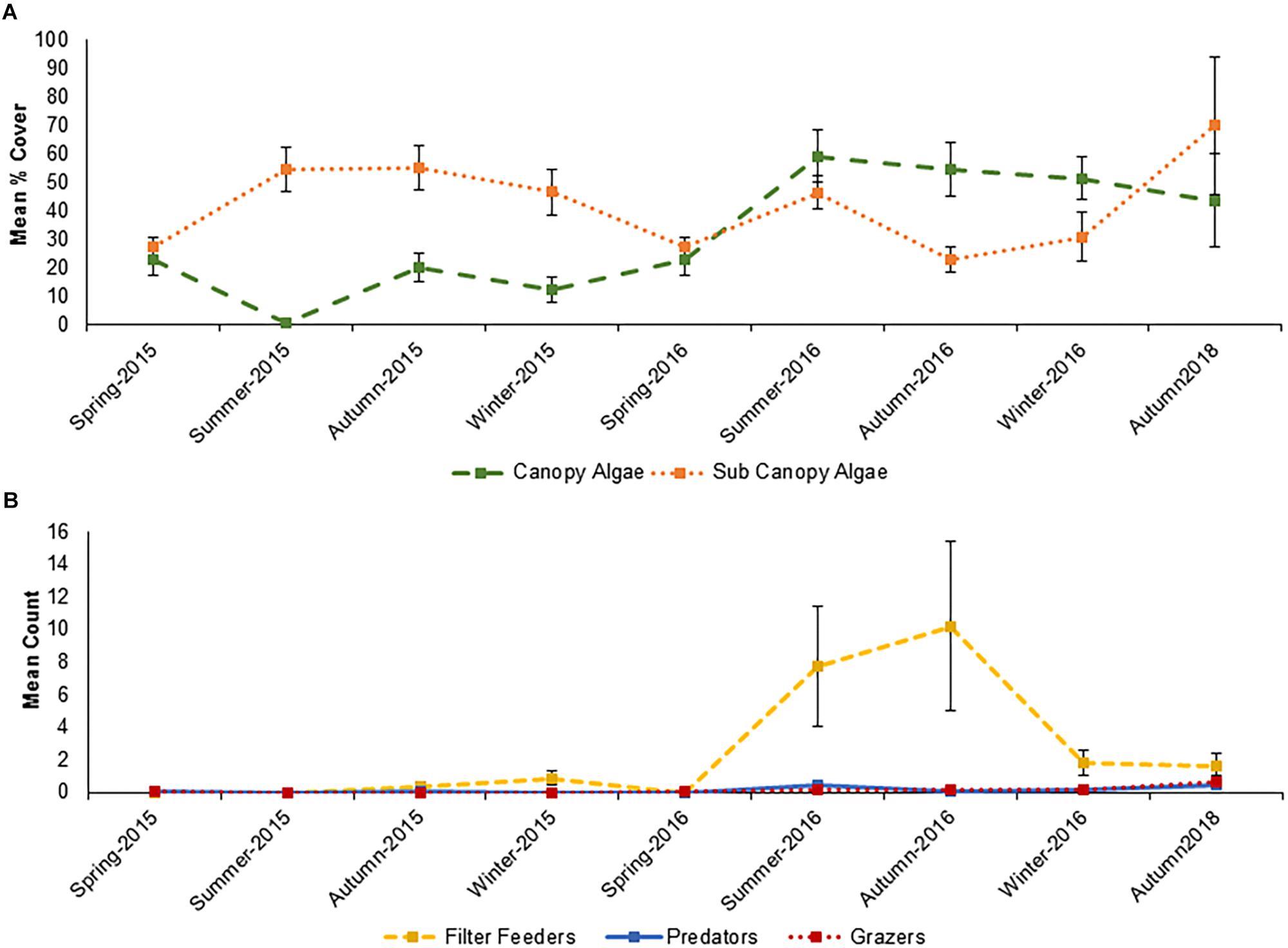
Figure 4. Seasonal variation in (A) mean % cover and (B) count of main functional groups inside the Vertipools between Spring 2015 and Autumn 2018 (+/–SE., N = 5). The rock pools were fitted to the wall in September 2013.

Table 3. SIMPER analysis run using Jaccard similarity matrix indicating average abundance of functional groups per Vertipool in 2015 and 2016 (Av. Abund = mean abundance, Av. Diss = Average dissimilarity, Diss/SD = SD of dissimilarity, Contrib% = contribution %, Cum% = cumulative percentage).
Disturbed Seawall vs. Undisturbed Seawall
After 3 years, the disturbed seawall communities had not recovered algal percentage cover similar to that of the undisturbed sea wall (beta regression GLM, F = 47.87, P < 0.001, pseudo r-squared = 0.66, Figure 5). Yet there was a significant difference in assemblage composition between the disturbed and undisturbed seawall (ANOSIM, Global R = 0.193, P = 0.18). 78.03% of the overall 33.69% dissimilarity between habitats was due to four algal species; A. nodosum, F. spiralis, F. serratus, and Catenella sp. A. nodosum was most abundant on the seawall, whereas F. spiralis, F. serratus, and Catenella sp. were most abundant on the disturbed seawall (Table 4).
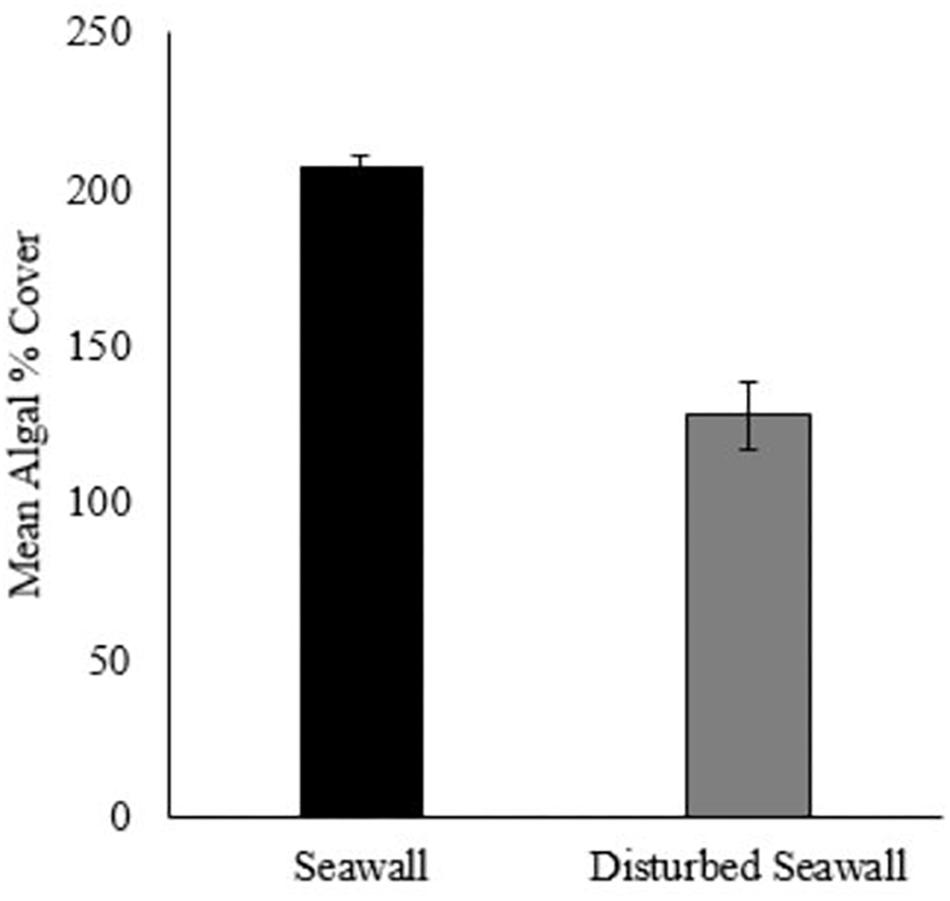
Figure 5. Mean % cover of algae recorded on the disturbed seawall and the control seawall after 3 years (+/– SE, N = 10, note difference in quadrat size between habitats- disturbed seawall 25 × 25 cm, control seawall 50 × 50 cm).
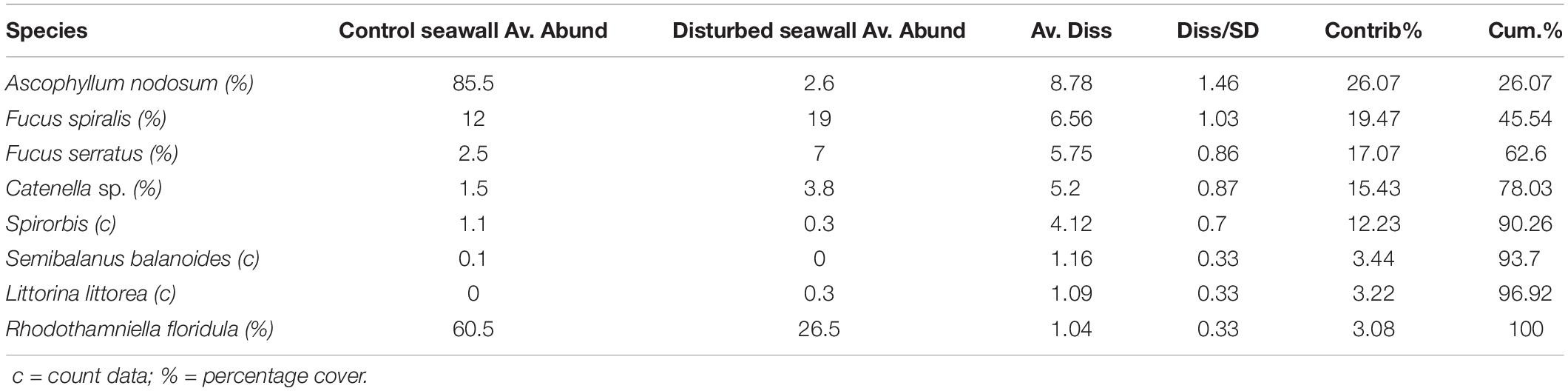
Table 4. SIMPER analysis run using Jaccard similarity matrix on disturbed vs. control seawall after 3 years, mean abundance (%) of species contributing to dissimilarity between habitats SIMPER, Average dissimilarity = 39.63% (Av. Abund = mean abundance, Av. Diss = Average dissimilarity, Diss/SD = SD of dissimilarity, Contrib% = contribution %, Cum% = cumulative percentage).
Discussion
Although a small-scale study, compared to previous studies (Browne and Chapman, 2014; Evans et al., 2015; Firth et al., 2016a) this currently represents the longest time-series of species richness and assemblage compositions in artificial rock pools. The water retention and increased surface texture provided by the Vertipools created a habitat which was absent from the existing sea wall, enabling a variety of different rock pool species, including fish, to inhabit the structure. Within the study area, the Vertipools increased the species richness on the seawall, supporting a wider range of taxa that is more characteristic of natural shores (Crisp and Southward, 1958; Martins et al., 2007; Firth et al., 2014a). All of the Vertipools remained attached to the seawall with no visible signs of damage; destruction of enhancement devices by waves has been a problem in previous studies (Browne and Chapman, 2014). The Vertipools were designed to deflect wave energy and the strong internal and external fixings ensured that no damage was caused to the Vertipools or the seawall. One of the greatest concerns with retrofitting enhancement devices is the potential to damage the structural integrity of the coastal assets. As sea levels rise and coastal squeeze becomes more severe (Pontee, 2011; Committee on Climate Change UK, 2018), limiting the refugia provided by natural habitats (Jackson and McIlvenny, 2011), it is probable that the Vertipools will become more important to species currently surviving in natural pools at lower tidal levels. Without the refuge provided by the Vertipools, many of the mobile species recorded would not be able to survive at such a high tidal height (Pallas et al., 2006).
Initially, the Vertipools were colonized by opportunistic green algae (Ulva spp.), followed by a shift to fucoid algae on the exterior and branching/filamentous algae on the interior. This follows typical succession on a rocky shore (Benedetti-Cecchi and Cinelli, 1996; Benedetti-Cecchi, 2000; Martins et al., 2007; Viejo et al., 2008), although a longer monitoring period is required to establish whether the assemblages have stabilized (Browne and Chapman, 2014). An increased number of functional groups found within the Vertipools in 2016, suggest that communities were still developing; in 2015 the assemblage was dominated by sub-canopy algae, yet in spring 2016 the assemblage changed and became characterized by canopy algae with an increased number of filter feeders, predators and grazers.
Seasonal variation in assemblages has been observed in the Vertipools, with red filamentous algae appearing in the summer months (Christie et al., 2009) and barnacles recruiting in the spring (Jenkins et al., 2000). The close proximity to natural habitat and propagule supply may facilitate colonization at this site and locations with less spatial connectivity may take longer to colonize (Cowen and Sponaugle, 2009; Herbert et al., 2017). The largest change in the community was noted when grazers, particularly limpets, moved onto the Vertipools, removing the fucoids from the exterior of the Vertipools.
The Vertipool located at the greatest height on the seawall took longest to colonize, with the interior community predominately consisting of opportunistic algae (Ulva spp.), whereas the exterior was colonized by F. spiralis. Previously, the reduced number of organisms in high shore ecological enhancements has been linked to low recruitment levels (Browne and Chapman, 2014). Over the duration of the study it was noticed that the elevation of fucoids on the exterior of the Vertipool increased to a height above that of the fucoids growing on the seawall, possibly due to the damper, shaded “overhang” effect created by the Vertipools.
Enhancement of Seawall
Given predicted sea level rise and truncation of the intertidal zone, the provision of suitable habitats on artificial structures is necessary to prevent further biodiversity loss. As with natural rock pools found on the upper shore, the distribution of intertidal species extended higher up the shore due to the installation of the Vertipools. Mobile fauna such as crabs (C. maenas, P. platycheles) and periwinkles (L. obtusata), previously absent from the seawall, were found inside the Vertipools on multiple occasions. Studies have shown that rock pools support a more diverse community than adjacent rock faces (Firth et al., 2013, 2014a) on both natural shores (Firth et al., 2014a) and artificial structures (Chapman and Blockley, 2009; Browne and Chapman, 2014; Evans et al., 2015). As this study was a small-scale trial project, the five Vertipools were only installed at one site, resulting in low spatial replication. Future studies will need to include trials at multiple sheltered and exposed sites with increased replication to determine wider scale benefits and impacts. Shore height, pool volume, surface area, depth, shading and drainage are known to impact the physico-chemical composition of rock pools (Daniel and Boyden, 1975; Metaxas and Scheibling, 1993; White et al., 2014), therefore, the installation of artificial pools of different sizes and depths and at a variety of heights would be beneficial. The elevated presence of the alga F. spiralis on the exterior base of the Vertipools could indicate reduced desiccation stress for the species at this height. Therefore, compared to the seawall, the establishment of Vertipools, constructed of roughened concrete, creates a more heterogeneous habitat providing water retention and damp refugia. These features enable species to occupy levels that are elevated above current zones on the seashore. Natural rock pools are not permanent features of this particular study site as the shore is mobile and prone to periodic smothering by sediments. However, rock pools harboring protected species at similar tidal levels do occur on the island 5 km to the west, so the installation of artificial pools on sea walls may be regionally beneficial to the conservation of these species should they colonize in the longer term.
Impacts of Retrofitting
In the present study, the shoreline and seawall were dominated by A. nodosum which is slow to recover after disturbance events (Jenkins et al., 2004), due to poor growth and recruitment mortality (Stengel and Dring, 1997). This study monitored how the retrofitting process affected the existing algal cover on the seawall. After 3 years the disturbed areas were recolonized by F. spiralis (19.00% cover) and A. nodosum (2.60% cover), with an understory of R. floridula (26.50% cover) and C. rupestris sp. (69% cover). However, as shown by Jenkins et al. (2004), A. nodosum took longer to grow than F. spiralis. Overall, the early recolonization of algae indicates that the retrofitting process is unlikely to have any long-term impact on these assemblages, although A. nodosum does need continued monitoring. As the disturbed patches of the sea wall were limited in area, a smaller sized quadrat was necessary for sampling, so comparative estimates of algal cover areas should be considered with caution.
One criticism of retrofitted objects is that they might reduce the structural integrity of the seawall (French, 2001). To date, however, no visible signs of damage or weakening have been observed, although this will continue to be monitored over time. Moreover, cross disciplinary work needs to be conducted between engineers and ecologists to create multifunctional structures for the future (Dafforn et al., 2015; Firth et al., 2016a). Evidence suggests that if ecological enhancement devices are aesthetically pleasing, the general public and coastal managers are more supportive of their use (Morris et al., 2016). Incorporating education and public engagement into habitat creation schemes is an excellent way to connect and educate the general public and school children on important issues such as coastal squeeze and sea level rise. The use of art to activate and engage the students on a complex topic worked well in designing the Vertipools exterior patterns and is recommended for future projects.
Conclusion
Extending the intertidal zone vertically by creating bioreceptive artificial rock pools for marine life to inhabit has been successful in this feasibility study. Vertipools could be installed and retrofitted on a variety of different coastal structures including seawalls and groynes to produce biologically favorable built environments. If replicated more widely, these features have the potential to mitigate the impact of coastal squeeze and other physical disturbances that limit the size of the intertidal zone, such as coastal development. Combined with other interventions, such as the creation of holes and grooves to create refugia at different scales, increased habitat heterogeneity on these structures will improve species and functional diversity.
Author Contributions
AH, RH, JB, IB, and NG contributed to the conception and design of the study. AH conducted the data collection and statistical analysis. AH wrote the main sections of the manuscript with inputs from RH, IB, NG, and JB. All authors contributed to manuscript revision and read and approved the submitted version.
Conflict of Interest Statement
IB and NG were employed by the company Artecology Ltd.
The remaining authors declare that the research was conducted in the absence of any commercial or financial relationships that could be construed as a potential conflict of interest.
Funding
The Shelving the Coast project was funded by the Artists Project Earth (APE), Solent Forum, EU Coastal Communities Adapting to Change (CCATCH), and the Isle of Wight Area of Outstanding Natural Beauty (AONB) project. This work was supported by the Bournemouth University from the Open Access Publication Fund.
Acknowledgments
We are grateful to the Isle of Wight Council Principal Engineer Peter Marsden for permission to undertake this research and for the help of the Island 2000 Trust and Arc Consulting for initiating and developing Shelving the Coast. We thank the Artecology Ltd. and the Eccleston George Collective for the design, construction, and installation of the Vertipools. We also thank the two reviewers for their comments which improved the manuscript.
Supplementary Material
The Supplementary Material for this article can be found online at: https://www.frontiersin.org/articles/10.3389/fmars.2019.00456/full#supplementary-material
References
Airoldi, L., Abbiati, M., Beck, M., Hawkins, S., Jonsson, P., Martin, D., et al. (2005). An ecological perspective on the deployment and design of low-crested and other hard coastal defence structures. Coast. Eng. 52, 1073–1087 doi: 10.1016/j.coastaleng.2005.09.007
Arenas, F., Sánchez, I., Hawkins, S. J., and Jenkins, S. R. (2006). The invasibility of marine algal assemblahes: role of functional diversity and identity. Ecology 87, 2851–2861. doi: 10.1890/0012-9658(2006)87%5B2851:tiomaa%5D2.0.co;2
Benedetti-Cecchi, L. (2000). Predicting direct and indirect interaction during succession in a mid-littoral rocky shore assemblage. Ecol. Monogr. 70, 45–72. doi: 10.1890/0012-9615(2000)070%5B0045:pdaiid%5D2.0.co;2
Benedetti-Cecchi, L., and Cinelli, F. (1996). Patterns of disturbance and recovery in littoral rock pools: nonhierarchical competition and spatial variability in secondary succession. Mar. Ecol. Prog. Ser. 135, 145–161. doi: 10.3354/meps135145
Bennett, B. A., and Griffiths, C. L. (1984). Factors affecting the distribution, abundance and diversity of rock-pool fishes on the Cape Peninsula, South Africa. S. Afr. J. Zool. 19, 97–104. doi: 10.1080/02541858.1984.11447865
Björk, M., Axelsson, L., and Beer, S. (2004). Why is Ulva intestinalis the only macroalga inhabiting isolated rockpools along the Swedish Atlantic coast? Mar. Ecol. Prog. Ser. 284, 109–116. doi: 10.3354/meps284109
Bolker, B. M., Brooks, M. E., Clark, C. J., Geange, S. W., Poulsen, J. R., Stevens, M. H. H., et al. (2009). Generalized linear mixed models: a practical guide for ecology and evolution. Trends Ecol. Evol. 24, 127–135. doi: 10.1016/j.tree.2008.10.008
Borsje, B. W., van Wesenbeeck, B. K., Dekker, F., Paalvast, P., Bouma, T. J., van Katwijk, M. M., et al. (2011). How ecological engineering can serve in coastal protection. Ecol. Eng. 37, 113–122. doi: 10.1016/j.ecoleng.2010.11.027
Browne, M. A., and Chapman, M. G. (2011). Ecologically informed engineering reduces loss of intertidal biodiversity on artificial shorelines. Environ. Sci. Technol. 45, 8204–8207. doi: 10.1021/es201924b
Browne, M. A., and Chapman, M. G. (2014). Mitigating against the loss of species by adding artificial intertidal pools to existing seawalls. Mar. Ecol. Prog. Ser. 497, 119–129. doi: 10.3354/meps10596
Chapman, M. G., and Blockley, D. J. (2009). Engineering novel habitats on urban infrastructure to increase intertidal biodiversity. Oecologia 161, 625–635. doi: 10.1007/s00442-009-1393-y
Chapman, M. G., and Underwood, A. J. (2011). Evaluation of ecological engineering of “armoured” shorelines to improve their value as habitat. J. Exp. Mar. Biol. Ecol. 400, 302–313. doi: 10.1016/j.jembe.2011.02.025
Christie, H., Norderhaug, K. M., and Fredriksen, S. (2009). Macrophytes as habitat for fauna. Mar. Ecol. Prog. Ser. 396, 221–233. doi: 10.3354/meps08351
Committee on Climate Change UK (2018). Managing the Coast in a Changing Climate Committee on Climate Change. Available at: www.theccc.org.uk/publications (accessed October 31, 2018).
Connell, J. H. (1972). Interactions on marine rocky intertidal shores. Annu. Rev. Ecol. Syst. 3, 169–192. doi: 10.1146/annurev.es.03.110172.001125
Cowen, R. K., and Sponaugle, S. (2009). Larval dispersal and marine population connectivity. Annu. Rev Mar. Sci. 1, 443–466. doi: 10.1146/annurev.marine.010908.163757
Crisp, D. J., and Southward, A. J. (1958). The distribution of intertidal organisms along the coasts of the English Channel. J. Mar. Biol. Assoc. U.K. 37, 157–208. doi: 10.1017/S0025315400014909
Dafforn, K. A., Glasby, T. M., Airoldi, L., Rivero, N. K., Mayer-Pinto, M., and Johnston, E. L. (2015). Marine urbanization: an ecological framework for designing multifunctional artificial structures. Front. Ecol. Environ. 13, 82–90. doi: 10.1890/140050
Daniel, M., and Boyden, C. (1975). Diurnal variations in physio-chemical conditions within intertidal rockpools. Field Stud. 4, 161–176.
Evans, A. J., Firth, L. B., Hawkins, S. J., Morris, E. S., Goudge, H., and Moore, P. J. (2015). Drill-cored rock pools: an effective method of ecological enhancement on artificial structures. Mar. Freshw. Res. 67, 123–130. doi: 10.1071/MF14244.
Firth, L., Schofield, M., White, F. J., Skov, M. W., and Hawkins, S. J. (2014a). Biodiversity in intertidal rock pools: informing engineering criteria for artificial habitat enhancement in the built environment. Mar. Environ. Res. 102, 122–130. doi: 10.1016/j.marenvres.2014.03.016
Firth, L., Thompson, R. C., Bohn, K., Abbiati, M., Airoldi, L., Bouma, T. J., et al. (2014b). Between a rock and a hard place: environmental and engineering considerations when designing coastal defence structures. Coast. Eng. 87, 122–135. doi: 10.1016/j.coastaleng.2013.10.015
Firth, L. B., Browne, K. A., Knights, A. M., Hawkins, S. J., and Nash, R. (2016a). Eco-engineered rock pools: a concrete solution to biodiversity loss and urban sprawl in the marine environment. Environ. Res. Lett. 11:094015. doi: 10.1088/1748-9326/11/9/094015
Firth, L. B., Knights, A. M., Bridger, D., Evans, A. J., Mieszkowska, N., Moore, P. J., et al. (2016b). Ocean sprawl: challenges and opportunities for biodiversity management in a changing world. Oceanogr. Mar. Biol. 54, 193–269.
Firth, L. B., Thompson, R. C., White, F. J., Schofield, M., Skov, M. W., Hoggart, S. P. G., et al. (2013). The importance of water-retaining features for biodiversity on artificial intertidal coastal defence structures. Divers. Distrib. 19, 1275–1283. doi: 10.1111/ddi.12079
French, P. (2001). Coastal Defences: Processes, Problems and Solutions. First Edit. London: Routledge.
Green, J. M. (1971). Local distribution of Oligocottus masculosus girard and other tidepool cottids of west coast of Vancouver Island, British Columbia. Can. J. Zool. 49, 1111–1128 doi: 10.1139/z71-172
Hall, A. E., Herbert, R. J. H., Britton, J. R., and Hull, S. L. (2018). Ecological enhancement techniques to improve habitat heterogeneity on coastal defence structures. Estuar. Coast. Shelf Sci. 210, 68–78. doi: 10.1016/j.ecss.2018.05.025
Herbert, R. J., Collins, K., Mallinson, J., Hall, A. E., Pegg, J., Ross, K., et al. (2017). Epibenthic and mobile species colonisation of a geotextile artificial surf reef on the south coast of England. PLoS One 12:e0184100. doi: 10.1371/journal.pone.0184100
IUCN (2016). Explaining Ocean Warming: Causes, Scale, Effects and Consequences. eds D. Laffoley, and J. M. Baxter, Gland: IUCN.
Jackson, A. C., and McIlvenny, J. (2011). Coastal squeeze on rocky shores in northern Scotland and some possible ecological impacts. J. Exp. Mar. Biol. Ecol. 400, 314–321. doi: 10.1016/j.jembe.2011.02.012
Jenkins, S. R., Åberg, P., Cervin, G., Coleman, R. A., Delany, J., Della Santina, P., et al. (2000). Spatial and temporal variation in settlement and recruitment of the intertidal barnacle Semibalanus balanoides (L.) (Crustacea: Cirripedia) over a European scale. J. Exp. Mar. Biol. Ecol. 243, 209–225. doi: 10.1016/S0022-0981(99)00121-5
Jenkins, S. R., and Martins, G. M. (2010). “Succession on Hard Substrata,” in Biofouling. S. Dürr, J. C. Thomason. (Oxford: Wiley-Blackwell).
Jenkins, S. R., Norton, T. A., and Hawkins, S. J. (2004). Long term effects of Ascophyllum nodosum canopy removal on mid shore community structure. J. Mar. Biol. Assoc. 84, 327–329. doi: 10.1017/S0025315404009221h
Kendall, M. A., Burrows, M. T., Southward, A. J., and Hawkins, S. J. (2004). Predicting the effects of marine climate change on the invertebrate prey of the birds of rocky shores. IBIS 146, 40–47. doi: 10.1111/j.1474-919X.2004.00326.x
Loke, L. H. L., and Todd, P. A. (2016). Structural complexity and component type increase intertidal biodiversity independently of area. Ecology 97, 383–393. doi: 10.1890/15-0257.1
Martins, G. M., Hawkins, S. J., Thompson, R. C., and Jenkins, S. R. (2007). Community structure and functioning in intertidal rock pools: effects of pool size and shore height at different successional stages. Mar. Ecol. Prog. Ser. 329, 43–55. doi: 10.3354/meps329043
Martins, G. M., Thompson, R. C., Neto, A. I., Hawkins, S. J., and Jenkins, S. R. (2010). Enhancing stocks of the exploited limpet Patella candei d’Orbigny via modifications in coastal engineering. Biol. Conserv. 143, 203–211. doi: 10.1016/j.biocon.2009.10.004
Metaxas, A., and Scheibling, R. (1993). Community structure and organization of tidepools. Mar. Ecol. Prog. Ser. 98, 187–198. doi: 10.3354/meps098187
Morris, R. L., Deavin, G., Hemelryk Donald, S., and Coleman, R. A. (2016). Eco-engineering in urbanised coastal systems: consideration of social values. Ecol. Manag. Restor. 17, 33–39. doi: 10.1111/emr.12200
Morris, R. L., Golding, S., Dafforn, K. A., and Coleman, R. A. (2017). Can coir increase native biodiversity and reduce colonisation of non-indigenous species in eco-engineered rock pools? Ecol. Eng. 120, 622–630. doi: 10.1016/j.ecoleng.2017.06.038
Moschella, P. S., Abbiati, M., Åberg, P., Airoldi, L., Anderson, J. M., Bacchiocchi, F., et al. (2005). Low-crested coastal defence structures as artificial habitats for marine life: using ecological criteria in design. Coast. Eng. 52, 1053–1071. doi: 10.1016/j.coastaleng.2005.09.014
Pallas, A., Garcia-Calvo, B., Corgos, A., Bernardez, C., and Freire, J. (2006). Distribution and habitat use patterns of benthic decapod crustaceans in shallow waters: a comparative approach. Mar. Ecol. Prog. Ser. 324, 173–184. doi: 10.3354/meps324173
Pontee, N. I. (2011). Reappraising coastal squeeze: a case study from north-west England. Mar. Eng. 164, 127–138. doi: 10.1680/maen.2011.164.3.127.
R Core Team (2018). R: A Language and Environment for Statistical Computing. Vienna: R Foundation for Statistical Computing.
Stengel, D. B., and Dring, M. J. (1997). Morphology and in situ growth rates of plants of Ascophyllum nodosum (Phaeophyta) from different shore levels and responses of plants to vertical transplantation. Eur. J. Phycol. 32, 193–202. doi: 10.1017/S0967026297001200
Underwood, A. J., Chapman, M. G., Cole, V. J., and Palomo, M. G. (2008). Numbers and density of species as measures of biodiversity on rocky shores along the coast of New South Wales. J. Exp. Mar. Biol. Ecol. 366, 175–183. doi: 10.1016/j.jembe.2008.07.022
Viejo, R. M., Arenas, F., Fernández, C., and Gómez, M. (2008). Mechanisms of succession along the emersion gradient in intertidal rocky shore assemblages. Oikos 117, 376–389. doi: 10.1111/j.2007.0030-1299.16206.x
White, G. E., Hose, G. C., and Brown, C. (2014). Influence of rock-pool characteristics on the distribution and abundance of inter-tidal fishes. Mar. Ecol. 36, 1332–1344. doi: 10.1111/maec.12232
Keywords: ecological enhancement, climate change, sea level rise, seawall, habitat creation, ocean sprawl, ecological engineering
Citation: Hall AE, Herbert RJH, Britton JR, Boyd IM and George NC (2019) Shelving the Coast With Vertipools: Retrofitting Artificial Rock Pools on Coastal Structures as Mitigation for Coastal Squeeze. Front. Mar. Sci. 6:456. doi: 10.3389/fmars.2019.00456
Received: 06 November 2018; Accepted: 08 July 2019;
Published: 30 July 2019.
Edited by:
Edward Jeremy Hind-Ozan, Department for Environment, Food and Rural Affairs, United KingdomReviewed by:
Jose M. Fariñas-Franco, National University of Ireland Galway, IrelandJuniper Simonis, DAPPER Stats, Portland, OR, United States
Copyright © 2019 Hall, Herbert, Britton, Boyd and George. This is an open-access article distributed under the terms of the Creative Commons Attribution License (CC BY). The use, distribution or reproduction in other forums is permitted, provided the original author(s) and the copyright owner(s) are credited and that the original publication in this journal is cited, in accordance with accepted academic practice. No use, distribution or reproduction is permitted which does not comply with these terms.
*Correspondence: Alice E. Hall, ahall@bournemouth.ac.uk