- 1Institute of Aquaculture, University of Stirling, Stirling, United Kingdom
- 2Institute of Biodiversity, Animal Health and Comparative Medicine, University of Glasgow, Glasgow, United Kingdom
- 3St Abbs Marine Station, St Abbs, United Kingdom
- 4Department of Biosciences, College of Science, Swansea University, Swansea, United Kingdom
Decapod crustaceans, such as those from the Homarus genus, are key benthic representatives that support very valuable fishing and aquaculture industries. Those commercially caught, such as the European lobster (H. gammarus) can be stored in live facilities for short (a few days) to long periods (up to 6 months) before being traded. Conditions in captivity are not standardized, including holding temperatures or feeding regimes. Herein, the physiological condition during long-term starvation (24 weeks) in H. gammarus was assessed at three temperatures (4, 8, and 12∘C). Our results indicate that, H. gammarus have the capacity to endure long-term starvation. Principal component analysis (PCA) of measured parameters showed two main components (Eigen value >1). Fasted animals kept at 12∘C, separated from all other experimental groups due to higher total phenoloxidase (PO) activity in the hemolymph and water content in the muscle, suggesting that keeping H. gammarus un-fed at this higher temperature is physiologically more demanding and detrimental. This was later confirmed by significant changes, particularly in this group, in the histology and lipid class composition of the hepatopancreas. These data call into question the suitability of current accepted in vivo condition markers (e.g., hemolymph protein concentration) to determine the physiological condition and welfare of decapods such as H. gammarus.
Introduction
Crustacean species such as those of the family Nephropidae (the clawed lobsters) experience starvation periods as part of their molt-dependent growth process. The capacity of these and other decapod species to cope with long periods of starvation has been widely reported in the literature (Stewart et al., 1972; Jones and Obst, 2000; Sacristan et al., 2016). For instance, Nephrops norvegicus males were shown to survive without feeding for over 6 months, without a reduction in body weight (Watts et al., 2014). This is in contrast to vertebrates where starvation is intrinsically linked with the loss of body weight (McCue, 2010). In decapod crustacean species, although long periods of starvation do not result in a reduction in body weight (usually since water content is modulated), the loss of specific organ mass such as the hepatopancreas (Watts et al., 2014), also referred to as the midgut gland, perigastric organ or digestive gland (Cervellione et al., 2017b), can occur. Changes in proximate composition in this gland have also been shown, with glycogen and lipids acting as key energy reserves (Ciaramella et al., 2014; Sacristan et al., 2017). The hepatopancreas, and in particular its lipid reserves, play a major role in the long-term survival of decapod crustaceans during starvation. This is an observation supported not only by the high percentage of lipids reported in the hepatopancreas of several species, but also by their decrease in starvation studies (Jones and Obst, 2000; Ciaramella et al., 2014; Watts et al., 2014; Sacristan et al., 2016). However, the sequence and rate in which energy reserves are mobilized from the hepatopancreas is highly variable among crustaceans (Sacristan et al., 2017). Moreover, the rate at which animals lose body or specific tissue mass will differ depending not only on how species prioritize the use of the different fuel resources during starvation but also on their overall energy requirements, which are inextricably linked to the animal’s body temperature. Populations of the European lobster Homarus gammarus are distributed widely - inhabiting sub-tidal eastern North Atlantic waters from the Lofoten Islands in northern Norway to Morocco, including the Mediterranean (Cobb and Castro, 2007), indicating a degree of thermo-tolerance. Furthermore, seasonal changes in food supply and temperature are to be expected, especially in shallow coastal areas where these animals live. In this case, a dependency between metabolic rate and temperature may be a beneficial strategy (reduced metabolic rate in winter when food availability is lower). In H. gammarus, the dependency between metabolic rate and temperature has been defined as “conservationist” as at low temperatures the metabolic rate is kept low even when food availability is high (Tully et al., 2000). In this scenario, one would expect that the effect(s) of starvation would be minimized. The role that temperature plays in starvation was first reported in Homarus americanus in 1972 (Stewart et al., 1972). From this initial study, it became clear that temperature is a key external factor when combined with the demands of starvation. Understanding, the interplay of these factors is important not only from a fundamental physiological perspective but also because the live holding of lobsters is a common practice, especially for H. americanus. Live storage of H. americanus accounts for 45–50% of >136,000 tons of lobsters landed in Canada and the United States (Thériault et al., 2013). Live holding of H. americanus normally occurs in customized facilities and is tightly regulated by HACCP legislation (Estrella, 1984; Food and Drug Administration, 2017). Live holding of this species takes place at low temperatures (3–5°C) controlled recirculation or through-flow systems, and without the need for feeding which is time-consuming and can adversely affect water quality (McLeese and Wilder, 1964). However, the EU legislation that regulates the holding of H. gammarus in the United Kingdom is less rigorous than that in North America, and covers only food safety, animal health and welfare, and fisheries management (Jacklin and Combes, 2007). United Kingdom legislation does not require customized facilities, and there is currently no stipulation of the feeding regime, holding temperature or a maximum length of confinement time. Moreover, various fishery advisory bodies in the United Kingdom have provided conflicting advice on the need to feed or not to feed (Beard and McGregor, 2004). Considering the relatively long periods of captivity over which H. gammarus are now being held (Jacklin and Combes, 2007), it has become important to understand the extent to which H. gammarus might be immunologically and/or physiologically compromised during long-term starvation at different temperatures. Furthermore, from a monitoring perspective there is a need to find suitable condition markers for this species. Several studies have proposed the use of serum protein concentration as a good indicator of the quality and physiological condition in H. americanus and H. gammarus (Hagerman, 1983; Ciaramella et al., 2014). This is mainly supported by the positive correlations found between serum protein concentration and hepatopancreas mass and its negative correlation to, for example, moisture content in the hepatopancreas (Wang and McGaw, 2014). However, both biochemical as well as cellular parameters in the hemolymph have been reported to be affected by starvation. Total hemocyte counts (THCs) for instance have been shown to decrease in H. americanus during starvation (Stewart et al., 1967), which could affect key immune parameters such as phenoloxidase (PO) activity, a response reported to change in other marine invertebrates (Matozzo et al., 2011; Coates et al., 2012), but which has not yet been measured in the Homarus genus. The circulating cells, i.e., hemocytes, of crustacean hemolymph are responsible for defense reactions to microbes and parasites, including phagocytosis, encapsulation and the release of immune factors (e.g., phenoloxidase). Decreased levels of hemocytes in the hemolymph can be attributed to the presence of a pathogen, or a consequence of environmental stress (Rosas et al., 2004; Matozzo et al., 2011; Coates et al., 2012; Johnson et al., 2016). A third reason could be the release of the enzyme, phenoloxidase, which causes the cells to undergo apoptosis and are eventually removed from circulation (thereby lowering hemocyte numbers). The release of phenoloxidase is a hallmark response of invertebrate innate immunity. Phenoloxidase catalyses phenols into pigment precursors and toxic by-products (oxidizing and nitrosative radicals). Eventually melanin is formed, and alongside the remaining hemocytes, phenoloxidase assists in microbial entrapment and/or wound healing (Coates and Nairn, 2013). Therefore, taking these straightforward measurements of THCs and PO provides insight into the immune readiness of lobsters in captivity. The recent study of Clark et al. (2017) illustrated how these and other diverse analytical techniques can increase the understanding of nutritional status in lobsters.
Therefore, this study has evaluated the effects of long-term starvation and temperature on the physiological condition of H. gammarus with the aim of defining best practices when holding H. gammarus in captivity. To this end, we recorded survival and body weight, and measured a diverse number of tissue, cellular and biochemical parameters in the hemolymph (protein content, total hemocyte counts “THCs,” total PO activity), hepatopancreas (hepatosomatic index “HSI,” histological examination, water content, glycogen, total lipid, and lipid class analysis) and muscle (water content and 1D-SDS analysis) of H. gammarus under different temperatures (4, 8, and 12°C). These temperatures mimic the seasonal range for United Kingdom coastal seawater (average 6–15°C; Morris et al., 2018) and represent recommended holding temperatures (4–10°C) for H. gammarus, according to United Kingdom codes of practice (Beard and McGregor, 2004; Jacklin and Combes, 2007). By analyzing this suite of traditional and more novel measures we have evaluated the suitability of well-accepted nutritional markers (i.e., protein concentration and HSI) to reflect the physiological condition of these animals.
Materials and Methods
Animal Husbandry and Experimental Conditions
Adult intermolt creel-caught male lobsters (H. gammarus, N = 72) were collected on arrival at the port, from a local shellfish merchant (Eyemouth, United Kingdom) in January 2014 (Table 1). Lobster claws were banded to avoid injuries and they were transported damp in polystyrene boxes to the St Abbs Marine Station (approximate transport time 1 h). On arrival, lobsters (in groups of 12) were placed non-selectively in six tanks (1.4 m2 floor area, 744 L; stocking density 4.82 kg/m2) and supplied with flow-through ambient temperature seawater (7–8°C). To mimic common industry practices, no dividers were placed in the tanks. A similar arrangement was used by D’Agaro et al. (2014). We considered this experimental set-up to be not only more industry relevant but also necessary to ensure the welfare of the animals over the 24-week experimental period. Recently, Espmark et al. (2017) highlighted the negative effects of keeping fish in smaller tanks, i.e., reduced food intake and growth. Once allocated, the temperature in each tank was adjusted over 72 h to one of three temperatures: 4, 8, and 12°C (two tanks per temperature) and maintained in a flow-through system at those temperatures, using an inbuilt heat exchange panel for the duration of the trial. Animals from all tanks were fed for 1 week to allow for acclimation. After this time, animals from one tank at each tested temperature were fed twice a week on a maintenance diet of cooked (de-shelled) blue mussel (Mytilus edulis) to satiation (shown by the presence of unconsumed food the following day), while the animals from the other tanks were unfed. The trial started on 14 January 2014 and lasted for 24 weeks (last sampling on 1 July 2014) and animals were kept under a natural photoperiod. On the final sampling day an additional six wild caught intermolt H. gammarus were sourced locally from a creel-caught vessel that was landing on that particular date to evaluate the effect of captivity conditions on the most temperature comparable group (fed group kept at 12°C). Water salinity was recorded weekly and ranged from 32.5 to 35 PSU over the duration of the trial. Other water quality parameters, including oxygen (Multiparameter waterproof meter, Hanna Instrument, Bedfordshire, United Kingdom), ammonia, nitrite, and nitrate levels were recorded and found to be within adequate levels (ammonia <0.5 ppm; nitrite ≤1 ppm, and nitrate <5 ppm) for the duration of the trial using commercial kits (Aquasonic, Wauchope, Australia). Experimental work was ethically reviewed and approved by the Divisional Ethics Committee and AWERB, University of Stirling, United Kingdom. All methods were performed in accordance to relevant guidelines and regulations by the University of Stirling.
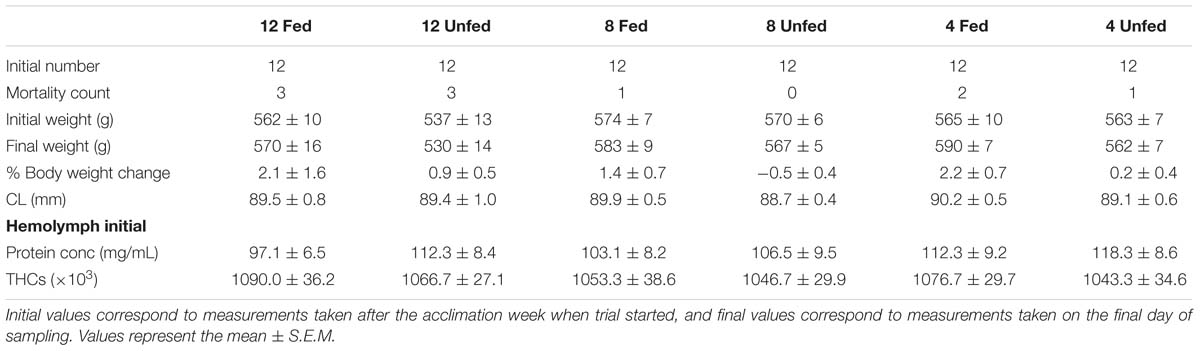
Table 1. Biophysical parameters measured in H. gammarus fed and unfed kept at different holding temperatures (4, 8, and 12°C).
Sampling Protocol
Initial Sampling
Lobsters were inspected daily and if mortalities occurred, these were recorded, and the dead animals were removed and checked macroscopically for clinical signs of disease. After the 1-week acclimatization, each lobster was tagged and sampled in vivo for baseline data. In this initial sampling, body weight (BB BD 523, Sartorius, Goettingen, Germany) and carapace length (CL) of each lobster were recorded. Hemolymph samples (0.6 mL) were drawn from the base of the fifth pereiopod using a 25G sterile hypodermic needle and used for the ex vivo determination of protein content and THCs.
Final Sampling
On the final sampling day, animals kept in captivity and those just creeled were sampled individually and their weight and CL were recorded, as in the initial sampling. Aliquots of the hemolymph samples were used for ex vivo protein determination and THCs, and the remainder was frozen at −80° C for later determination of total phenoloxidase (PO) activity. Animals were then killed by first destroying the dorsal supraesophageal ganglion and then by rapidly detaching the cephalothorax from the abdomen. After being sacrificed, post-mortem sampling was carried out by removing and weighing the hepatopancreas and by taking a sample of the deep abdominal muscle. The hepatosomatic index (HSI) was calculated using the following formula: HSI = [Weight of hepatopancreas (g)/weight of the animal (g)] × 100.
Cellular and Biochemical Properties of Hemolymph
Total Protein Content and Total Hemocyte Counts (THCs)
The total protein content of the hemolymph was estimated using an Eclipse (professional grade) handheld refractometer (Bellingham α Stanley, Tunbridge Wells, United Kingdom), adjusted for the refractive index of serum protein concentration (g dL−1). Ex vivo protein measurements using refractometry were validated with protein measurements carried out using the colorimetric Bradford Assay and Bovine Serum Albumin (BSA) protein standard curve (r2 = 0.94; Johnson et al., 2016).
For THCs, 50 μL of freshly withdrawn hemolymph was diluted 1:20 (v/v) with marine anticoagulant (3% NaCl, 100 mM dextrose, 50 mM citric acid, 10 mM EDTA, pH 4.6; Söderhäll and Smith, 1983) and stored on ice. THCs were performed in duplicate using an improved Neubauer hemocytometer.
Total Phenoloxidase Activity
Approximately 500 μL of fresh hemolymph was centrifuged at 1000 × g for 5 min to remove the cellular component. The supernatant was retained on ice and the cell pellet was discarded. Hemocyte-free hemolymph was diluted 1:20 in 100 mM Tris–HCl, pH 7.5 and assessed for total PO activities (including proPO and hemocyanin-derived phenoloxidase) as in Coates et al. (2012), Coates and Nairn (2013). The artificial enzyme activator, sodium dodecyl sulphate (SDS, 3.5 mM), was pre-incubated with the diluted hemolymph for 5 min prior to substrate addition. Dopamine hydrochloride (2 mM) was used as substrate in all assays (duplicates for each sample). An increase in absorbance at 475 nm indicated product (dopaminechrome) formation. Absorbance values were converted to μmol product formed per minute using the absorbance coefficient of 3600 M−1cm−1 for dopaminechrome at this wavelength. Total PO activities were corrected for hemolymph protein concentration – therefore values represent μmol/mg/min.
Compositional Analyses of Hepatopancreas
Histology
Cross sections of hepatopancreas (n = 4/group) fixed in 4% buffered neutral formaldehyde were embedded in paraffin, serially cut at 5 μm and stained with hematoxylin and eosin before standard examination under a light microscope. For each hepatopancreas, four regions (images) were randomly selected for image processing. Digital images were used to quantify total lumen area (TLA), and to determine the numbers and the areas of B-cell vacuoles (VBA), lipid droplets within R-cells (LDA) and F-cells (FCA) in each tubule and their ratios to total tissue area (TTA) as described in Cervellione et al. (2017a). In total twelve tubules from four lobsters, in each condition (=48 tubules per condition), were analyzed in this way using Image J software1.
Water Content
Subsamples of the hepatopancreas were freeze-dried for at least 24 h to a constant weight. Dry matter was calculated as the difference between the wet weight of the samples and their weight after freeze drying, and water content was calculated as the weight loss during this process.
Glycogen
Glycogen was analyzed as previously detailed (Albalat et al., 2010). Briefly, subsamples (∼150 mg) of frozen hepatopancreas were digested with 400 μL 30% KOH and boiled for 30 min. Afterward, pure ethanol (1:1.75 v:v) was added and the samples were left on ice for 2 h before being centrifuged at 14,000 rpm for 10 min. Pellets were resuspended with double distilled H2O and 50 μL (duplicates for each sample) was incubated at 95–100°C for 10 min with 1 mL of Anthrone reagent. Absorbance was measured in a spectrophotometer at 620 nm and total glycogen (glucosyl units) were determined according to Carroll et al. (1956) using a calibration curve constructed from standards of known concentrations of glucose (0.2–10 mM).
Total Lipid Extraction and Lipid Class Analysis
The total lipids from the hepatopancreas samples (duplicates for each sample) were extracted according to the Folch protocol (Folch et al., 1957). Extracted lipids were then re-suspended in chloroform: methanol (HPLC grade, Fisher Scientific, United Kingdom; 2:1, v/v) with butylated hydroxytoluene (BHT; 0.01%, w/v) to a final concentration of 10 mg mL−1 and stored at −25°C until further use.
Lipid class analyses (n = 3/group) were performed on total lipid samples using high-performance thin-layer chromatography (HPTLC) as described in Tocher and Harvie (1988). Lipid classes were visualized by charring at 160°C for 15 min after spraying with 3% (w/v) aqueous cupric acetate containing 8% of phosphoric acid and were quantified by densitometry using a CAMAG-3 TLC Scanner (Version Firmware 1.14.16; CAMAG, Muttenz, Switzerland) with winCATS Planar Chromatography Manager. Identification of individual classes was confirmed by comparing Rf values of known standards run alongside the samples.
Abdominal Muscle Analysis
Water Content
Subsamples of all muscle samples were freeze-dried to a constant weight. Dry matter was calculated as the difference between the wet weight of samples and their weight after freeze-drying, and water content was calculated as the weight loss during this process.
Protein Concentration and 1D-SDS-PAGE
Frozen muscle samples (n = 4) of fed and unfed lobsters held at 12°C (most extreme conditions) were homogenized and protein levels were analyzed using the bicinchoninic acid (BCA) assay (Thermo-Fischer, United States). To separate muscle proteins by molecular weight, 1-Dimensional Sodium Dodecyl Sulphate Polyacrylamide Gel Electrophoresis (1D-SDS-PAGE) was carried out using the Mini-Protean TGX® 12% pre-cast gels and Mini-PROTEAN® Tetra Cell System (Bio-Rad, United States). For estimation of molecular weight, protein markers were loaded in lane 1 of all gels (Precision Plus ProteinTM Standards, 10–250 kDa from Bio-Rad, United Kingdom). Gels were stained with InvitrogenTM SimplyBlueTM SafeStain (Thermo Fischer Scientific, United States) and de-stained overnight with dH2O.
SDS-PAGE images were acquired using an inGenius LHR Gel Imaging System (SynGene) and band densitometry data were obtained using GeneTools software version 4.3.8 (SynGene). Profile height data (band intensity) was imported into R and plotted using ggplot2 package.
Data Handling and Statistical Analyses
Principal component analysis and data representation were carried out using the R package (R function “prcomp” and “ggbiplot”). Ellipses shown in Figure 2 were generated using “ellipse.prob” (68%) for each group. Data were analyzed by 2-factor ANOVA, explanatory variables included “fed/unfed” condition and temperature (12, 8 or 4°C), both as categorical factors, while physiological and histology parameters were considered as response variables. When the overall test indicated a significant difference among feeding condition or holding temperature, pairwise comparisons (fed/fasted) and multiple comparisons (holding temperatures) were made with a T-test and Tukey’s post hoc tests, respectively. Changes in weight were analyzed by a 2-way repeated measures ANOVA, with the independent variable being time and the dependent variables being feeding and holding temperature. Differences were considered significant if p < 0.05 (SPSS, Statistics, version 23). Unless otherwise stated samples analyzed for each group correspond to the lobsters alive at the end of the trial (n = 9 for group kept at 12°C fed; n = 9 for group kept at 12°C unfed; n = 11 for group kept at 8°C fed; n = 12 for group kept at 8°C unfed; n = 10 for group kept at 4°C fed and n = 11 for group kept at 4°C unfed).
Results
Survival and Growth
Cumulative mortality over the 24-week period was between 0 and 25% under all conditions tested and no cause of death could be determined by macroscopic inspection. The highest cumulative mortality occurred in both the fed and unfed groups held at 12°C, with three mortalities recorded over the experimental period (Table 1), although given the size of the groups, these data should be considered observational. The claw length (CL), initial and final body weights and the percentage of change in body weight are shown in Table 1. A two-way repeated measures ANOVA indicated that body weight was significantly affected by feeding (Wilk’s Lambda; df = 1; F = 7.62; p = 0.008) but not holding temperature (Wilk’s Lambda; df = 2; F = 0.974; p = 0.384) or their interaction (feeding × temperature df = 2; F = 0.111; p = 0.895). No changes in CL were observed, as expected, since none of the lobsters molted during the experimental period.
Effect of Long-Term Starvation at Different Temperatures on Biometrics and Health Status Indicators
Due to the long period that H. gammarus were kept in captivity, data from the fed group kept at 12°C were compared to samples taken from wild lobsters captured on the final day of sampling (water temperature at this time of year was ∼12°C) to ascertain if fed animals kept in captivity had eaten appropriately and were in a similar condition to those that could be sampled from the wild at that time. Although this wild group had a significant larger CL, no significant differences in weight, HSI, water, glycogen and lipid contents in the hepatopancreas were observed when compared to the fed group kept at 12°C – thereby acting as a reference for the data observed in our captive fed animals (Table 2). In the context of lipid class analysis, the wild group showed significantly higher levels of wax/sterols compared to the captive fed group kept at 12°C, but no other differences were observed (Table 2).
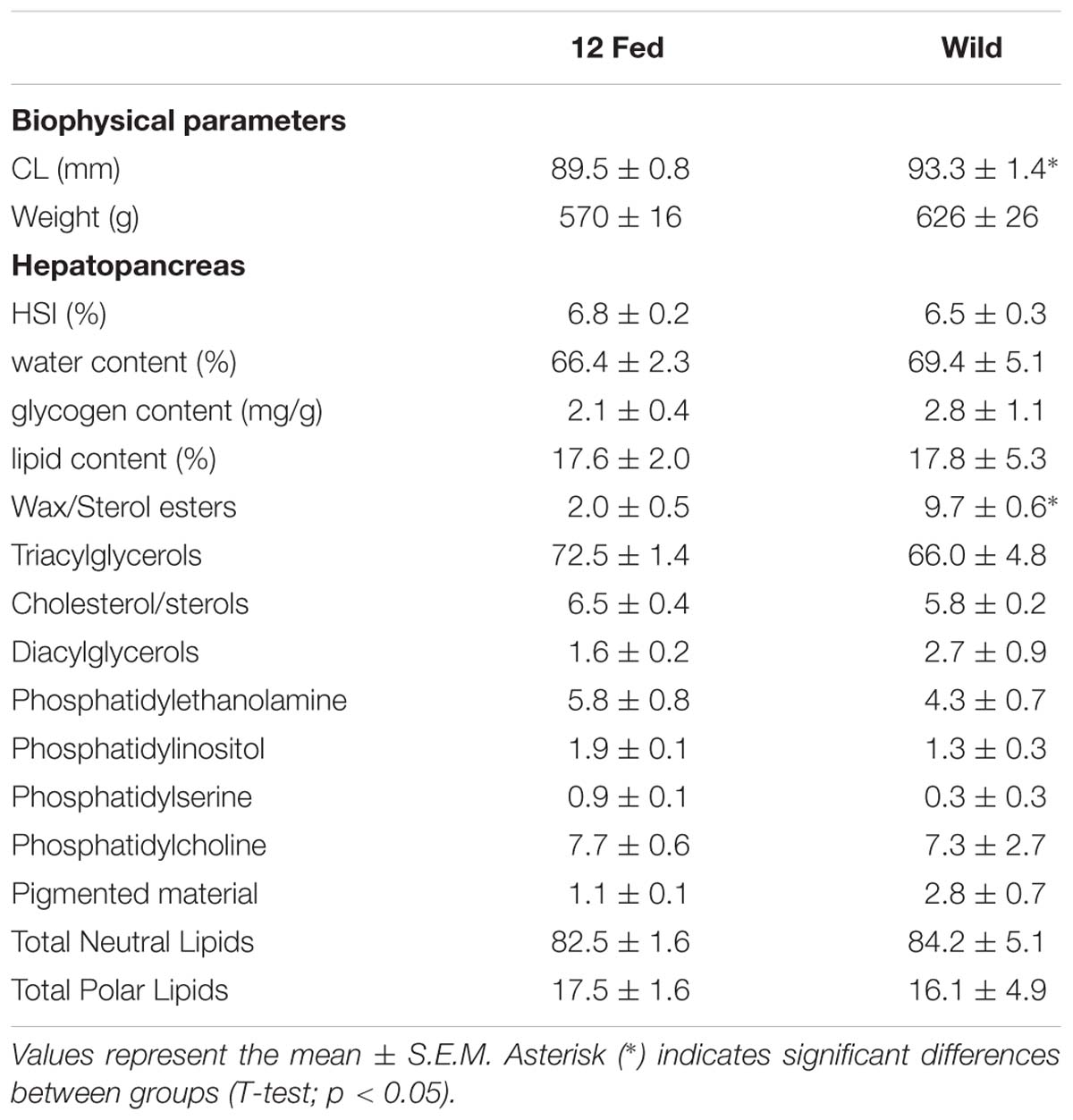
Table 2. Comparison of the experimental group fed and kept at 12°C (n = 9) with a reference group of H. gammarus captured from the wild on the final day of sampling (n = 6).
After 24 weeks in captivity, protein concentration in the hemolymph and hepatosomatic indices were significantly reduced in all fasted groups compared to their fed counterparts (Table 3). Moreover, our analyses indicated that the fasted lobsters kept at 12°C were physiologically more affected than the fasted groups at 4 and/or 8°C, not only because total PO activity was higher in the hemolymph, but also because this group showed significant compositional changes in the hepatopancreas with higher water and lower lipid contents (compared to fasted groups at 4 and 8°C; Table 3). Two-factor ANOVA indicated that most parameters were significantly affected by feeding (protein concentration and THCs in hemolymph; HSI, water content, glycogen and total lipid in hepatopancreas; water content in the muscle; all p < 0.05), while only total PO activity in hemolymph and water content in the muscle were affected by holding temperature (both p < 0.05; full details Table 4). When considering the interaction of feeding and holding temperature all factors considered were shown to be significant except for the protein concentration in the hemolymph (df = 2; F = 3.09; P = 0.053) and glycogen in the hepatopancreas (df = 2; F = 2.06; P = 0.133).
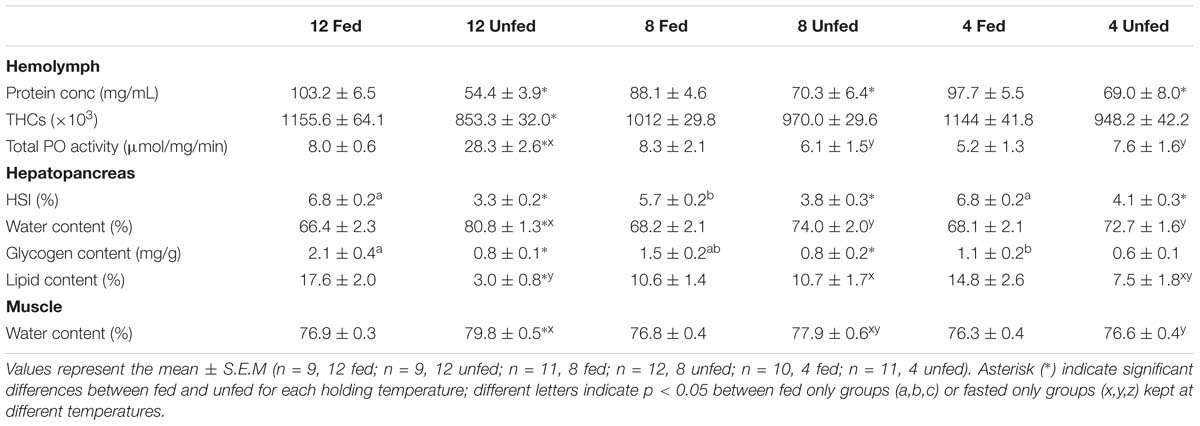
Table 3. Parameters measured in the hemolymph, hepatopancreas and muscle of H. gammarus fed and unfed kept at different holding temperatures (4, 8, 12°C) after being held in captivity for 24 weeks included in the PCA.
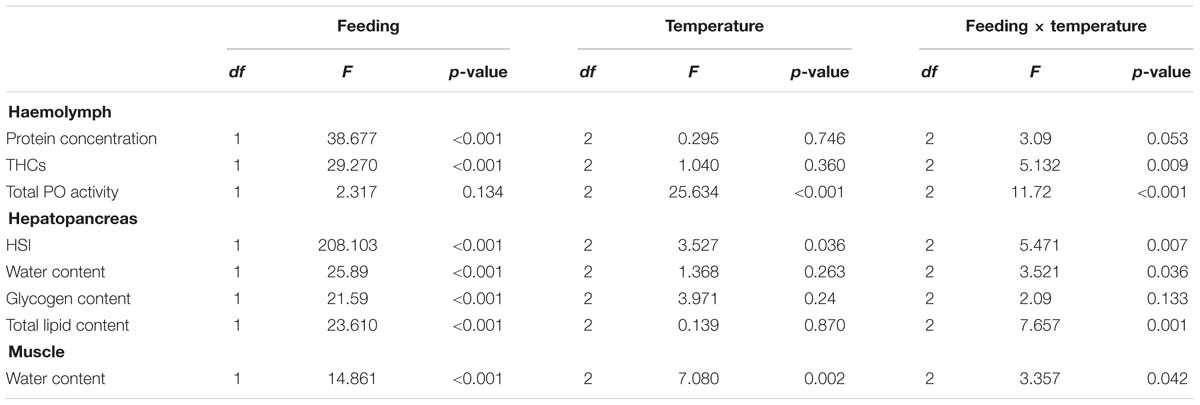
Table 4. Two-factor ANOVA on the parameters measured in the hemolymph, hepatopancreas and muscle of H. gammarus fed and unfed at different holding temperatures (4, 8, 12°C) after being held in captivity for 24 weeks included in the PCA.
When analyzing the lipid classes (Figure 1) decreases in triacylglycerols in all unfed groups, but particularly in the group kept at 12°C, were noted. Two-factor ANOVA indicated that while feeding significantly affected the composition of both neutral lipids (mainly driven by increases in sterol/esters and a decrease in triacylglycerols) and polar lipids (mainly driven by increases in phospholipids in the fasted condition). Only wax/sterol esters were affected by temperature alone (df = 2; F = 3.996; p = 0.047) and the interaction between feeding × temperature (df = 2; F = 5.728; p = 0.018), which also significantly affected phosphatidylethanolamine (df = 2; F = 5.134; p = 0.024; Figure 1; full details Table 5).
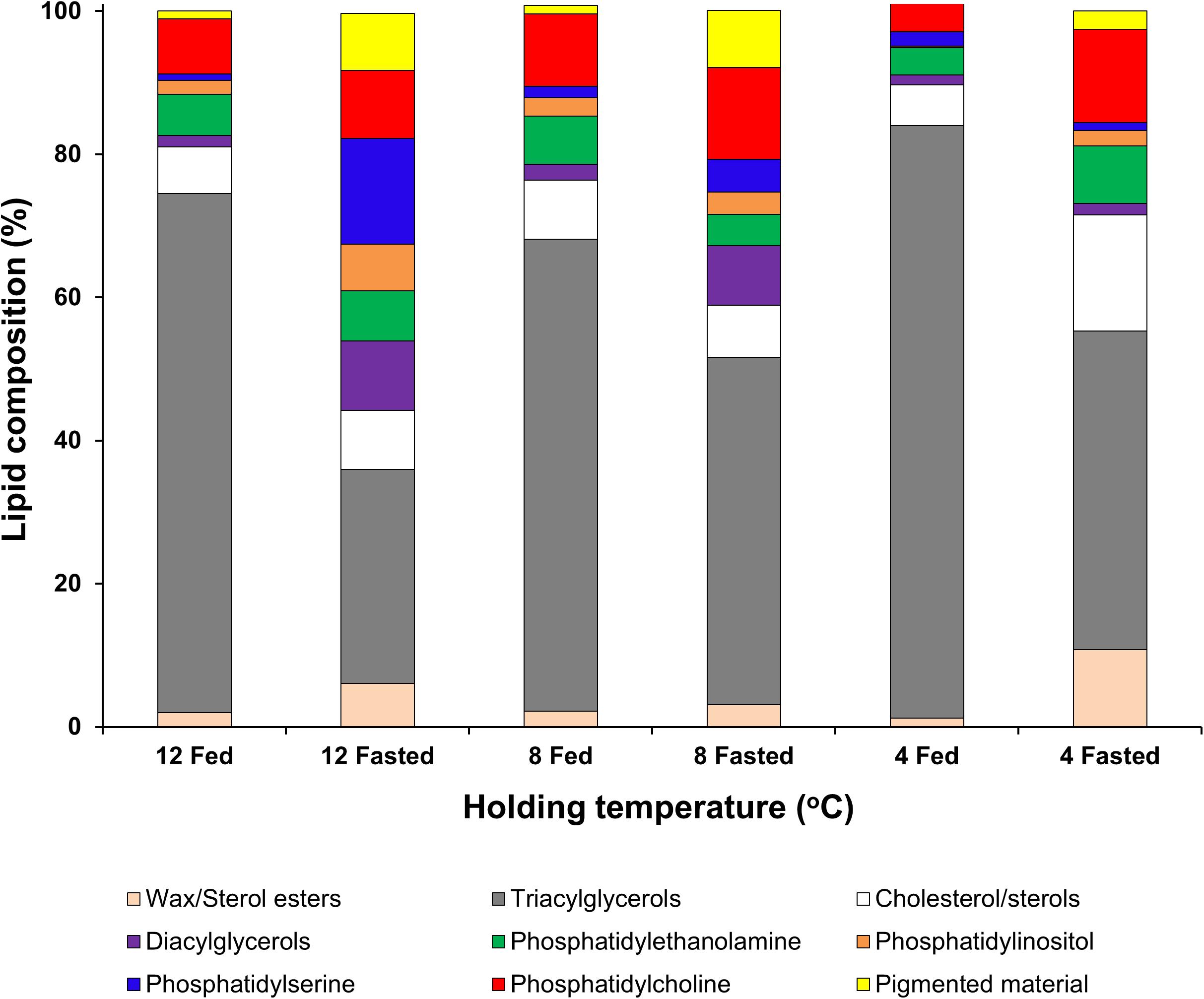
Figure 1. Lipid class analysis in lipid samples extracted from hepatopancreas tissue of H. gammarus after being held for 24 weeks under different conditions. Values represent the mean ± S.E.M (n = 4).
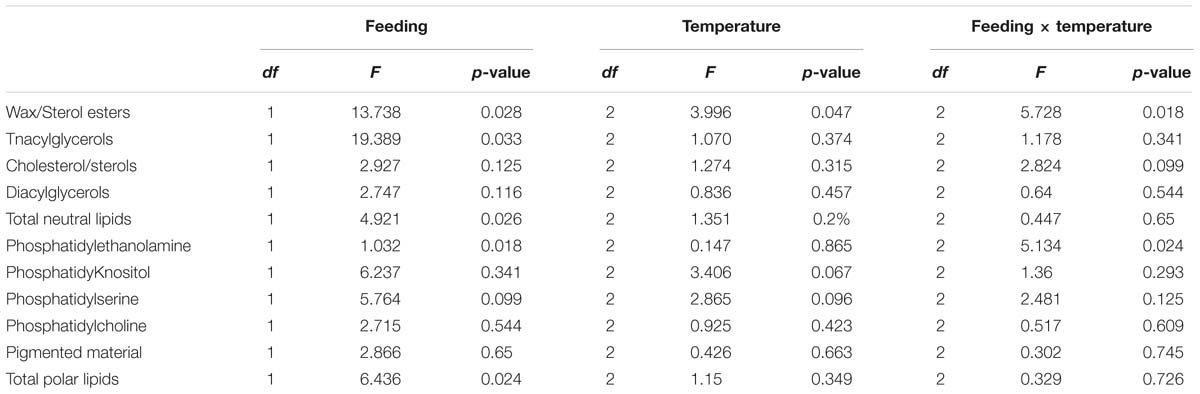
Table 5. Two-factor ANOVA on lipid class analysis of lipid samples extracted from hepatopancreas tissue from H. gammarus fed and unfed kept at different holding temperatures (4, 8, 12°C) after being held in captivity for 24 weeks.
To obtain an overall picture of the physiological condition of the animals at the end of this trial, the following data measured in the hemolymph (protein concentration, THCs, and total PO activity), hepatopancreas (HSI, water, glycogen and total lipid contents) and muscle (water content; data shown on Table 3) were subjected to PCA (Figure 2). Two PC with Eigen values >1 accounted for 61% of the variability (PC1 46.5% variability, Eigen value = 3.7192; PC2 14.6% variability, Eigen value = 1.169). From all parameters measured, protein concentration in the hemolymph (0.44), HSI (0.46) and water content in the hepatopancreas (−0.42) showed the highest loadings in the first component while total PO activity in the hemolymph (−0.76), glycogen in the hepatopancreas (−0.54) and water content in the muscle (−0.31) showed the highest loadings in the second component (Table 6). Highest correlation values were obtained between protein concentration in the hemolymph and water content in the hepatopancreas (−0.81) and protein concentration in the hemolymph with HSI (0.77; Table 7). The clearest separation was observed between the fasted group at 12°C and all the other groups including the fasted groups kept at lower temperatures (8 and 4°C), which were grouped within both principal components (Figure 2).
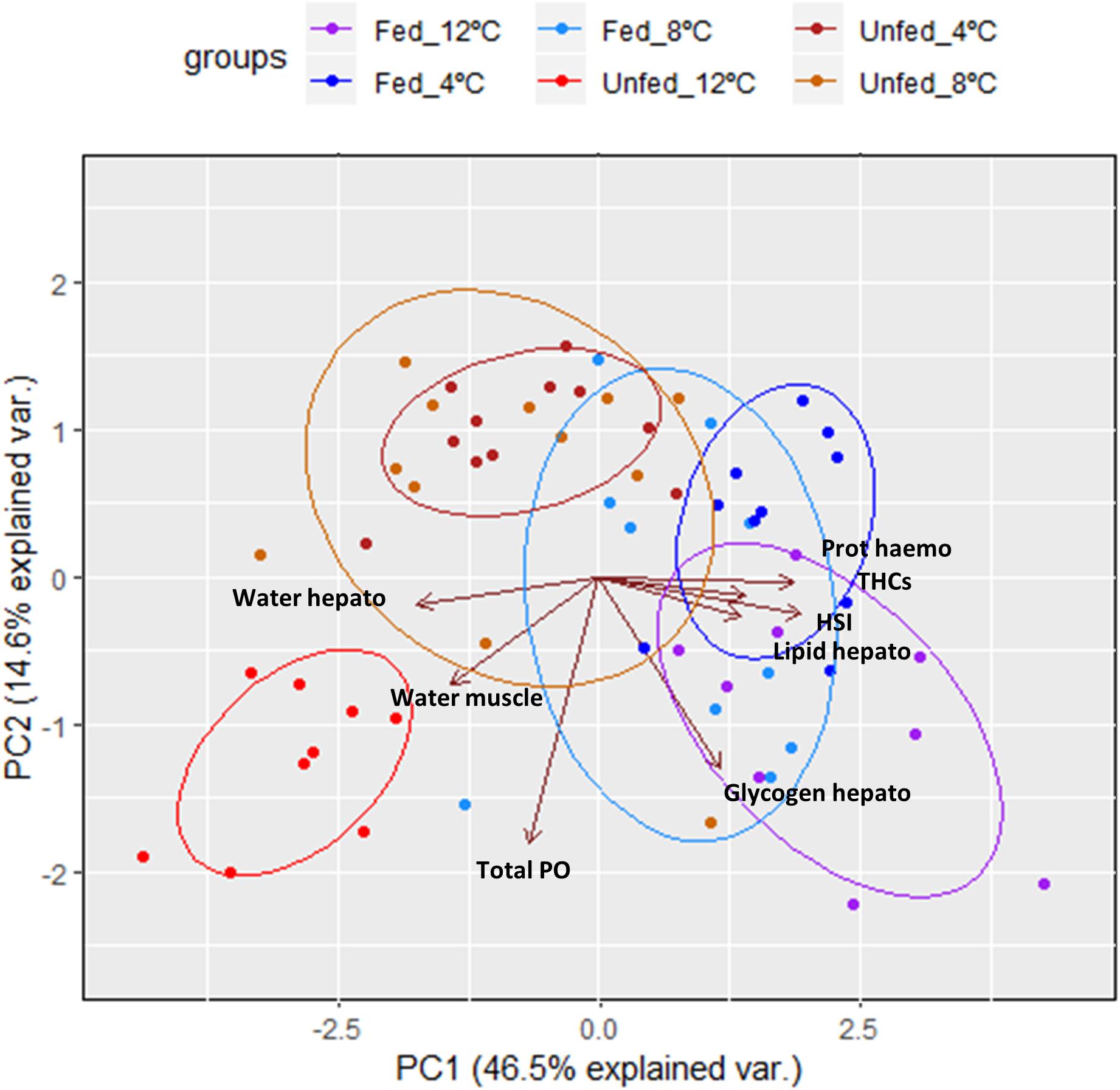
Figure 2. Principal component analysis of parameters measured in the hemolymph, hepatopancreas and muscle of H. gammarus after being held for 24 weeks under different conditions (n = 9, 12 fed; n = 9, 12 unfed; n = 10, 8 fed; n = 12, 8 unfed; n = 10, 4 fed; n = 11, 4 unfed).
Histological Changes in the Hepatopancreas in Response to Long-Term Starvation and Temperature
We examined the structural state of the hepatopancreas by histology and image analysis. The impact of fasting at 12°C was visually more pronounced than at 4°C (Figure 3), and is reflected in several morphometric parameters such as total tissue area (TTA) and relative lumen total area (LTA/TTA), lipid droplets within the R-cells (LDA), the number of F-cells and their total and relative areas (FCA and FCA/TTA; Table 8). When performing two-factor ANOVA, lumen and total tissue areas (LTA and TTA, respectively) did not change significantly across feeding conditions or holding temperatures. However, feeding did affect B-cell vacuolization in terms of both area (VBA df = 1; F = 8.61; p = 0.012) and their ratio to total tissue area (VBA/TTA df = 1; F = 12.03; p = 0.005), the number of F-cells (df = 1; F = 7.94; p = 0.016) and their total area (FCA df = 1; F = 11.97; p = 0.005). On the other hand, temperature alone affected VBA/TTA (df = 2; F = 4.32; p = 0.039). Again VBA/TTA (df = 2; F = 4.19; p = 0.042) was affected by the interaction of feeding × temperature in this case, together with the number of F-cells (df = 2; F = 11.04; p = 0.002), their area (FCA df = 2; F = 7.85; p = 0.007) and FCA/TTA (df = 2; F = 6.20; p = 0.014; Table 9). These data indicate that the main tissue features impacted were the area of the B-cell vacuoles and the number/area of F-cells.
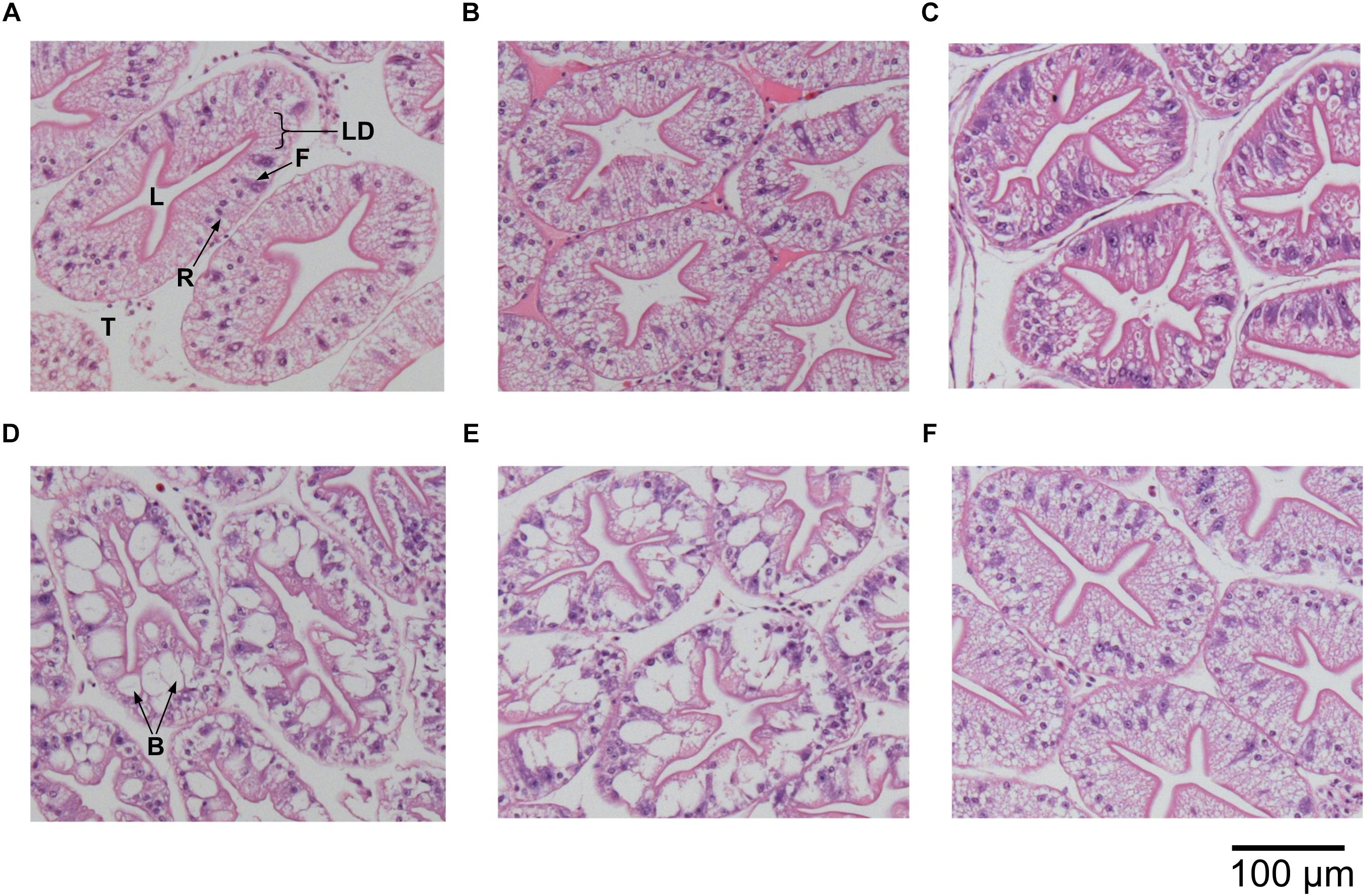
Figure 3. Histological cross sections of hepatopancreas tubules of H. gammarus (stained with hematoxylin and eosin) after being held for 24 weeks under different conditions. (A) 12 fed; (B) 8 fed; (C) 4 fed; (D) 12 fasted; (E) 8 fasted, and (F) 4 fasted. B, B-cell vacuole; F, F-cell; L, lumen; LD, lipid droplet; R, R-cell; T, tubule. Scale bar 100 μm.
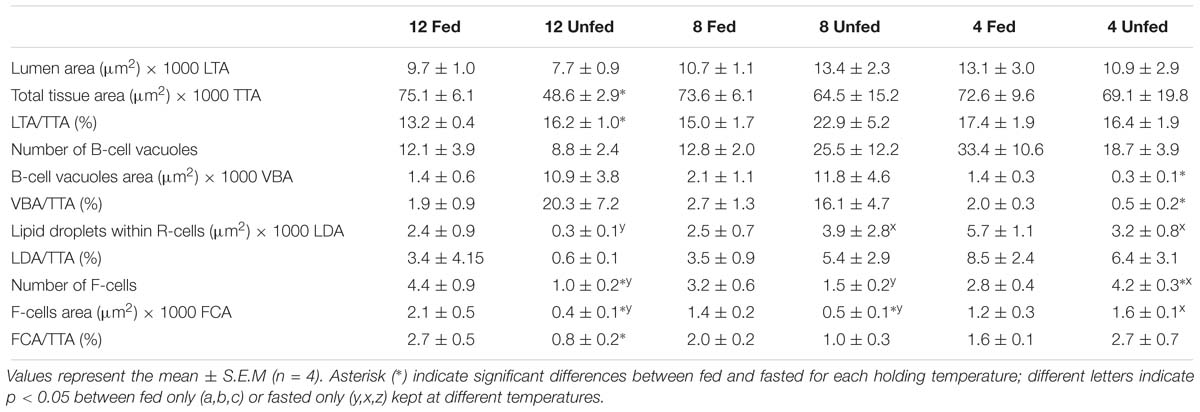
Table 8. Morphometric parameters measured on the hepatopancreas histology preparations of H. gammarus fed and unfed kept at different holding temperatures (4, 8, and 12°C) after being held in captivity for 24 weeks. In total 12 tubules from each of n = 4 lobsters in each condition (=48 tubules/condition) were analyzed.
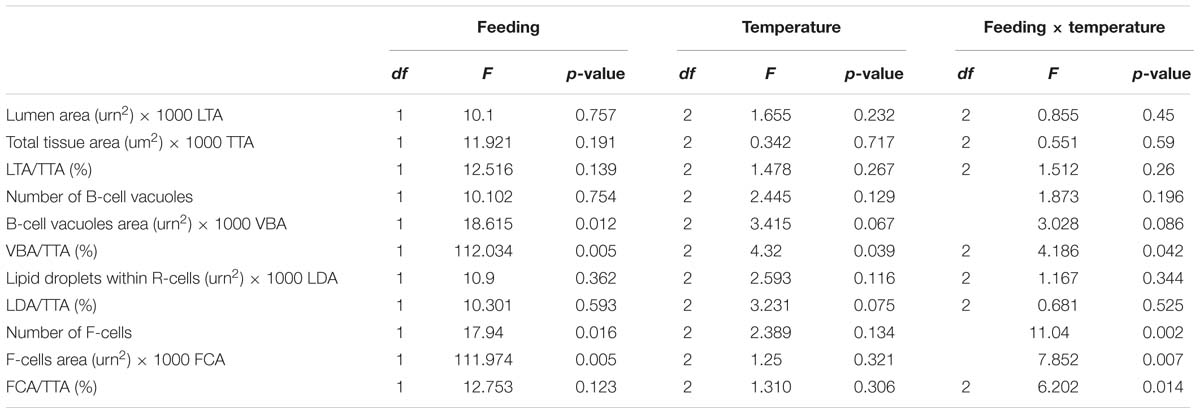
Table 9. t Two-factor ANOVA on the morphometric parameters measured on the hepatopancreas histology preparations of H. gammarus fed and unfed kept at different holding temperatures (4, 8, and 12°C) after being held in captivity for 24 weeks.
Physio-Chemical Properties of the Abdominal Muscle in Response to Long-Term Starvation and Temperature
As already reported, water content in the muscle increased significantly due to feeding conditions, temperature and the interaction between both factors (Table 4). In light of these results, proteins from the muscles of fed and unfed lobsters held at 12°C (the most extreme condition tested) were further examined using 1D-SDS-PAGE. Muscle protein in the fed group kept at 12°C had a concentration of 29.24 ± 3.20 mg/g compared to 23.50 ± 4.26 mg/g wet weight for the unfed group (same holding temperature), although these values were not significantly different (2-tailed; F = 1.25; df = 7; p = 0.339).
Examination of the muscle myofibrillar proteins in an SDS-PAGE gel (Figure 4) indicated no substantial differences between fed and unfed groups. Although the intensity of the myosin heavy chain band was low in both conditions, its intensity was significantly lower in the fasted group compared to the fed group (t-test; p = 0.01). The intensity of the actin band was also weaker in the fasted group although differences were not significant (t-test; p = 0.13). On the other hand, there was no evidence of additional lower MW bands indicative of an extensive degradation of the structural proteins (myosin and actin) due to starvation, at least under the conditions studied.
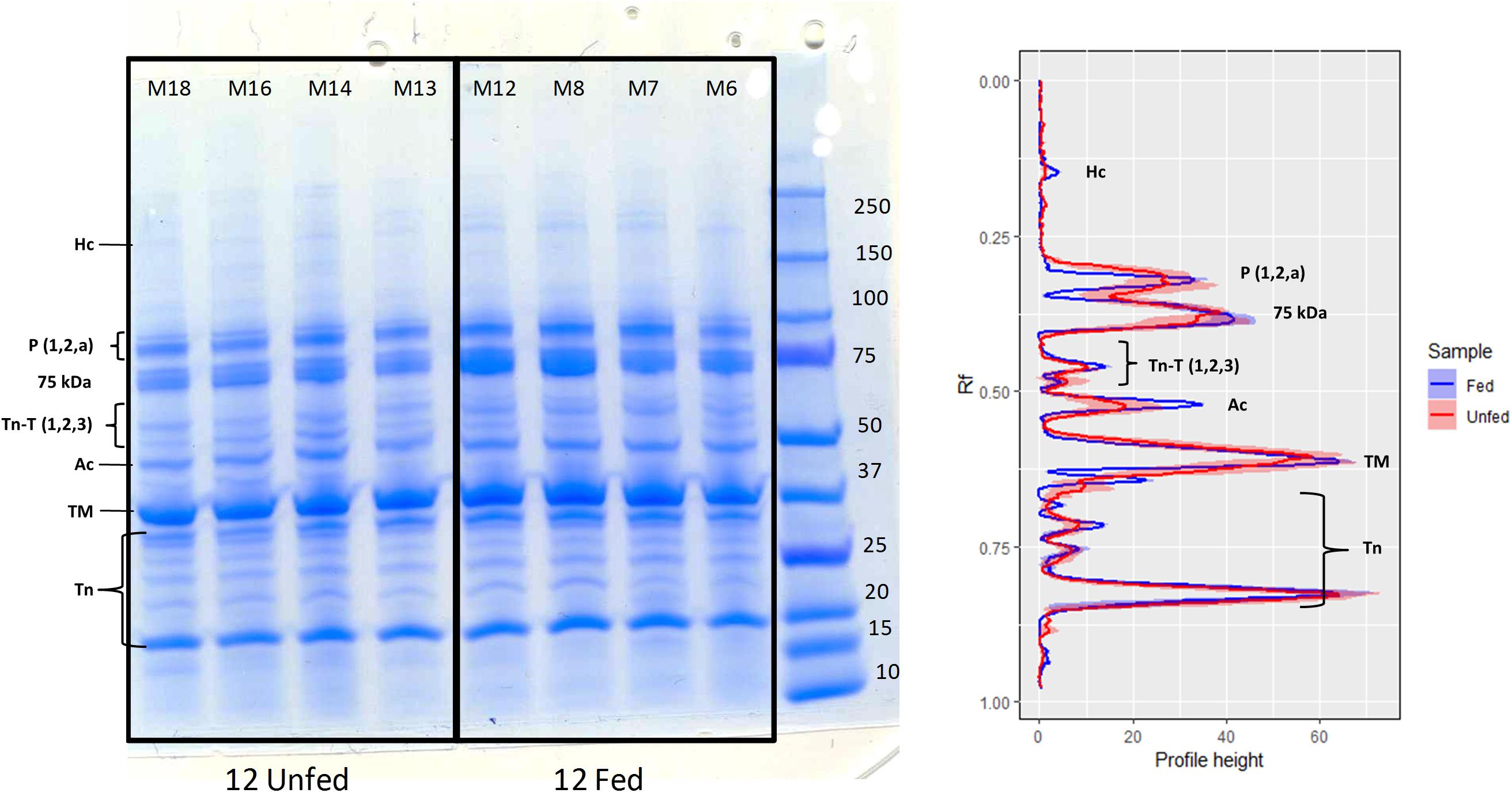
Figure 4. 1D-SDS-PAGE of muscle proteins extracted from H. gammarus fed and unfed kept at 12°C (n = 4/group). Band intensity measured as profile height to relative mobility (Rf) from 1D-SDS-PAGE gels. Lines represent the mean and colored areas represent S.E.M (fed shown in blue and fasted in red; n = 4). Ac, actin; Hc, myosin heavy chain; TM, tropomyosin; Tn, troponin (include troponin C and troponin I isoforms); TnT, troponin (three isoforms); P, paramyosin; 75 kDa unnamed fast muscle protein (Stentiford et al., 2000).
Discussion
From a strictly practical perspective, the most important aspects of live storage for shellfish merchants are survival, which can be compromised by infection and poor holding conditions, the total weight and the quality of the tail muscle of the lobsters at the point of sale. In the present study, mortality was low overall, although higher casualties were recorded when animals where held at 12°C than at 8°C and 4°C, regardless of feeding condition. Body weight was affected when animals were not fed, although the changes were small (ranging from −0.4 to 2.2% over the 24-week period), confirming the remarkable resilience of this crustacean species to sustain body weight over a long period of starvation, and in agreement with previous studies in H. americanus (Stewart et al., 1972) and N. norvegicus (20 and 30 weeks, respectively, Baden et al., 1994; Watts et al., 2014). This is in clear contrast to fish species, that can lose around 0.5% body weight per day (carp; Shimeno et al., 1990) and to avian species (dove; Ivacic and Labisky, 1973), which can lose up to 11% body weight per day (reviewed by McCue, 2010). Therefore, from a purely commercial perspective, given the small changes in weight, it is difficult to pinpoint the benefit of feeding versus not feeding at the temperatures tested. However, from a physiological and therein welfare perspective, some clear changes were observed in the hemolymph, hepatopancreas and muscles that highlight the particular impact of feeding and holding temperature on the condition of H. gammarus and possibly on the quality of the tail muscle (see later). Considering the PCA, it is apparent how lobsters fasted at 12°C do not cluster in the second component with those fasted at 8 and 4°C, indicating a further effect of holding temperature on the fasted animals. Fasted animals, regardless of holding temperature, show lower protein concentration in the hemolymph and lower HSI. As protein concentration in the hemolymph is highly correlated with HSI (0.770; factor loading PCA) and water content in the hepatopancreas (−0.810; factor loading PCA) it would indeed appear that protein concentration as an in vivo marker, is a good proxy reflecting the nutritional condition of H. gammarus. Under poor nutritional conditions, crustaceans tend to consume the main protein present in the hemolymph, namely hemocyanin, as a source of energy (Hagerman, 1983; Watts et al., 2014). Therefore, our results agree with previous work in which protein concentration has been shown to be a good indicator of nutritional condition (Ciaramella et al., 2014; Wang and McGaw, 2014).
However, other parameters in combination with protein concentration such as total PO activity give a more comprehensive picture of the lobster condition. Indeed, the increase in total PO in the hemolymph was not only highly significant but was also the main contributing factor to the separation of fasted H. gammarus at 12°C from those held at 8 and 4°C. This trend was observed in nicked (immune-challenged) edible crabs Cancer pagurus when held at 12°C (Johnson et al., 2016) suggesting that the combination of high temperature with another physiological stressor (nicking, or fasting as in the present study) can induce the release of hemocyte-bound PO and/or the synthesis of a new enzyme. Hypothetically, lower THCs, which were significantly reduced in the fasted group at 12°C (reduction of 23%), might reflect the release of the hemocyte-bound PO enzyme via cell rupture, which could be particularly relevant if a sub-type of hemocytes, such as granular cells are negatively affected by the biochemical changes that take place in the hemolymph (Matozzo et al., 2011). In this experiment, fasted lobsters kept at 12°C showed a significant decrease in THCs, suggesting that unfed lobsters kept at 12°C are immune-compromised. This drop in THCs in the unfed group kept at 12°C was accompanied by an increase in total PO activity suggesting a heightened response under higher temperatures (thermal stress). Whether the higher total PO levels provide the animal with an immediate and accessible defense mechanisms remains to be determined. In contrast to these changes in THCs and total PO activity, hemolymph protein levels were not significantly affected by the interaction between feeding condition and holding temperature, suggesting that this parameter alone does not completely capture lobster responses to multiple challenges (nutritional and thermal stresses).
Further confirmation that fasted animals held at 12°C were altered physiologically compared to those held at 8 and 4°C was found following further examination of the hepatopancreas. The hepatopancreas in decapod crustacean species is a key organ; responsible for the digestion and absorption of nutrients, storage of reserves, excretion of waste metabolites, and producer of immune factors (i.e., hemocyanin, lectins and ferritins; Roszer, 2014; Bowden, 2017). In this study, while glycogen in fed animals decreased when lobsters were held at lower temperatures reflecting a lower feed intake (data not shown), glycogen in all fasted groups was low irrespective of the holding temperature, possibly due to the long-term fasting nature of this trial. Other studies have reported that glycogen can decrease quickly in starved decapods (Sacristan et al., 2016) and therefore, other key components in the hepatopancreas such its lipid reserves, play a major role during long-term starvation (Watts et al., 2014). In this study, while HSI was significantly lower in all fasted groups, the fasted group held at 12°C showed significantly higher water content and lower lipid indicating that starvation at higher temperature might entail an increased energy expenditure that can only be met by oxidizing high energy lipids, resulting in substantial changes in proximate composition. Total lipid was particularly low in the unfed group kept at 12°C, which was accompanied with a marked reduction in triacylglycerols (from 72.5% fed 12°C to 27.5% unfed 12°C). Therefore, comparisons of starvation-induced losses based on mass, that do not consider changes in key tissue constituents, may be misleading (McCue, 2010). The structure of the H. gammarus hepatopancreas tubules provides evidence that partly corroborates these biochemical results. Four main types of cells in the hepatopancreas have been described in the literature, namely embryonic cells (E-cells), resorptive cells (R-cells) involved in the uptake and storage of nutrients, fibrillar cells (F-cells) involved in the release of digestive enzymes for extracellular digestions and the blister-like cells (B-cells) which contain a single large vacuole and are responsible for intracellular digestion (Barker and Gibson, 1977; Brunet and Mazza, 1994). Previous studies have reported that long periods of natural or imposed starvation induce changes particularly in the R-cells (fewer lipid droplets; Berillis et al., 2013; Cervellione et al., 2017a; Stathopoulou et al., 2017), a lower number of enzyme producing F-cells, which reduces the hepatopancreas digestive capacity (Papathanassiou and King, 1984; Icely and Nott, 1992) and hypertrophy of B-cells with enlarged vesicles (Sacristan et al., 2016). In this study, fasting produced a decrease in the number and the area of F-cells (release of digestive enzymes for extracellular digestion) and an increase in the vacuole area of B-cells (intracellular digestion). These parameters suggest that the hepatopancreas in fasted animals is in a more intrinsic catabolic state with reduced external digestive capacity. These parameters were also significantly affected by the interaction of feeding condition and holding temperature, indicating that the increased temperature under fasted conditions elicited further changes in the metabolic and digestive capacities of this organ. Reduction in the area of lipid droplets within the R-cells was significant only in the fasted group that was kept at 12°C, but not the fasted groups kept at lower temperatures. These results are consistent with the fasted group kept at 12°C, also displaying the highest decrease in HSI and total lipid percentage.
The suite of measures we have used to define the physiological condition of lobsters provides a comprehensive picture of the impact of holding conditions. While this is more informative than any individual measure alone, it nevertheless represents only a subset of the diverse analytical techniques that could be applied to define the nutritional status of lobsters. Other useful measures include the activities of various digestive enzymes, RNA/DNA ratios and the oxygen consumption of the animal (Rotllant et al., 2014a,b). Additionally, molecular gene expression analysis of the hepatopancreas can provide further information on the metabolic mechanisms utilized (Clark et al., 2017). If applied to the holding regimes we have used; these further measures could provide valuable insights into the varying energy demands of lobsters in relation to both feeding and temperature.
The consequences that the tested holding conditions could have on muscle quality were assessed by water content and by myofibrillar protein analysis (1D-SDS-PAGE). The fact that water content increased significantly in the fasted group kept at 12°C indicates that muscle quality could have been affected in this group. The main myofibrillar proteins detected in this study (myosin, tropomyosin, actin, paramyosin and different forms of troponins) were as detailed by Neil et al. (1993) and Stentiford et al. (2000), although the myosin heavy chain band was at a low intensity. Other authors have reported that in response to both fasting in H. americanus (D’Agaro et al., 2014) and post-mortem metabolism in N. norvegicus (Gornik et al., 2009), a number of fragments in the range 50–220 kDa appear, probably from actin and myosin (as indicated by the use of specific antibodies). Although in our study the band for the myosin heavy chain did have a lower intensity in the fasted group kept at 12°C, which is suggestive of some initial breakdown of the myofilaments, the more extensive degradation of myosin and actin observed by D’Agaro et al. (2014) in the tail muscle of H. americanus after only 4 weeks of starvation, was not found.
From a practical perspective, and considering the increased public attention on crustacean welfare, the results from the present study indicate that although H. gammarus can survive a prolonged period without food even at relatively high temperatures, keeping lobsters without food at temperatures greater than 8°C should be avoided as it clearly compromises their physiological condition and, as shown by the reduced number of THCs and increased PO activity, potentially impairs their immunological capacity. This may have consequences for the health status of animals (welfare perspective) and/or quality of the product (commercial perspective). We also highlight that nutritional markers such as the hemolymph protein level commonly used to assess lobster in vivo, when used alone, may fail to reflect the effect that additional stressors/challenges such as a higher temperature may have on their physiological and immune-capacity condition.
Ethics Statement
This study was carried out in European lobsters which are not included in the Animals (Scientific Procedures) Act. However, experimental work was ethically reviewed and approved by the Divisional Ethics Committee and AWERB, University of Stirling, United Kingdom. All methods were performed in accordance to relevant guidelines and regulations by the University of Stirling.
Author Contributions
AA, LJ, KT, and DN conceived the study. AA, LJ, CC, GD, FH, BM, JD, and DN contributed to formal analysis. AA, CC, KT, and DN performed the resources. AA wrote the original draft of the manuscript. CC and DN wrote, reviewed, and edited the manuscript.
Funding
The University of Glasgow, University of Stirling, Swansea University (College of Science Research Fund 2015/2016 awarded to CC and AA) and The Nesbitt-Cleland Trust (St Abbs Marine Station, United Kingdom) provided financial support.
Conflict of Interest Statement
LJ is currently employed by Todd Fish Tech, Dalgety Bay, United Kingdom. KT is currently the Research and Development Director of Todd Fish Tech, Dalgety Bay, United Kingdom.
The remaining authors declare that the research was conducted in the absence of any commercial or financial relationships that could be construed as a potential conflict of interest.
Acknowledgments
The authors are grateful to The Nesbitt-Cleland Trust (St Abbs Marine Station, United Kingdom).
Footnotes
References
Albalat, A., Sinclair, S., Laurie, J., Taylor, A., and Neil, D. (2010). Targeting the live market: recovery of Norway lobsters Nephrops norvegicus (L.) from trawl-capture as assessed by stress-related parameters and nucleotide breakdown. J. Exp. Mar. Biol. Ecol. 395, 206–214. doi: 10.1016/j.jembe.2010.09.002
Baden, S. P., Depledge, M. H., and Hagerman, L. (1994). Glycogen depletion and altered copper and manganese handling in Nephrops norvegicus following starvation and exposure to hypoxia. Mar. Ecol. Prog. Ser. 103, 65–72. doi: 10.3354/meps103065
Barker, P. L., and Gibson, R. (1977). Observations on feeding mechanism, structure of gut, and digestive physiology of European lobster Homarus gammarus (L) (Decapoda-Nephropidae). J. Exp. Mar. Biol. Ecol. 26, 297–324. doi: 10.1016/0022-0981(77)90089-2
Berillis, P., Simon, C., Mente, E., Sofos, F., and Karapanagiotidis, I. T. (2013). A novel image processing method to determine the nutritional condition of lobsters. Micron 45, 140–144. doi: 10.1016/j.micron.2012.10.014
Bowden, T. J. (2017). The humoral immune systems of the American lobster (Homarus americanus) and the European lobster (Homarus gammarus). Fish. Res. 186, 367–371. doi: 10.1016/j.fishres.2016.07.023
Brunet, M. A., and Mazza, J. (1994). Gut structure and digestive cellular processes in marine Crustacea. Oceanogr. Mar. Biol. 32, 335–367.
Carroll, N. V., Longley, R. W., and Roe, J. H. (1956). Determination of glycogen in liver and muscle by use of anthrone reagent. J. Biol. Chem. 220, 583–593.
Cervellione, F., McGurk, C., and Van den Broeck, W. (2017b). “Perigastric organ”: a replacement name for the “hepatopancreas” of Decapoda. J. Crustacean Biol. 37, 353–355. doi: 10.1093/jcbiol/rux020
Cervellione, F., McGurk, C., Eriksen, T. B., and Van den Broeck, W. (2017a). Effect of starvation and refeeding on the hepatopancreas of whiteleg shrimp Penaeus vannamei (Boone) using computer-assisted image analysis. J. Fish Dis. 40, 1707–1715. doi: 10.1111/jfd.12639
Ciaramella, M. A., Battison, A. L., and Horney, B. (2014). Measurement of tissue lipid reserves in the American lobster (Homarus americanus): hemolymph metabolites as potential biomarkers of nutritional status. J. Crustacean Biol. 34, 629–638. doi: 10.1163/1937240x-00002262
Clark, K. F., Yang, J., Acorn, A. R., Garland, J. J., Stewart-Clark, S. E., and Greenwood, S. J. (2017). The impact of harvesting location on the physiological indicators of the American lobster (Homarus americanus H. Milne Edwards, 1837) (Decapoda: Nephropidae) during live storage. J. Crustacean Biol. 37, 303–314. doi: 10.1093/jcbiol/rux033
Coates, C. J., Bradford, E. L., Krome, C. A., and Nairn, J. (2012). Effect of temperature on biochemical and cellular properties of captive Limulus polyphemus. Aquaculture 334, 30–38. doi: 10.1016/j.aquaculture.2011.12.029
Coates, C. J., and Nairn, J. (2013). Hemocyanin-derived phenoloxidase activity: a contributing factor to hyperpigmentation in Nephrops norvegicus. Food Chem. 140, 361–369. doi: 10.1016/j.foodchem.2013.02.072
Cobb, J. S., and Castro, K. M. (2007). “Homarus species,” in Lobsters: Biology, management, Aquaculture and Fisheries, ed. B. F. Phillips (Hoboken: Blackwell publishing), 310–332.
D’Agaro, E., Sabbioni, V., Messina, M., Tibaldi, E., Bongiorno, T., Tulli, F., et al. (2014). Effect of confinement and starvation on stress parameters in the American lobster (Homarus americanus). Ital. J. Anim. Sci. 13:3530. doi: 10.4081/ijas.2014.3530
Espmark, ÅM., Kolareviç, J., Åsgård, T., and Terjesen, B. F. (2017). Tank size and fish management history matters in experimental design. Aquac. Res. 48, 2876–2894. doi: 10.1111/are.13121
Estrella, B. T. (1984). Techniques for Live Storage & Shipping of American Lobster. Boston: Commonwealth of Massachusetts.
Folch, J., Lees, M., and Stanley, G. H. S. (1957). A simple method for the isolation and purification of total lipids from animal tissues. J. Biol. Chem. 226, 497–509.
Food and Drug Administration. (2017). Procedures for the Safe and Sanitary Processing and Importing of Fish and Fishery Products Wildlife and Fisheries. Washington, D.C: U.S. Government Publishing Office.
Gornik, S. G., Albalat, A., Atkinson, R. J. A., and Neil, D. M. (2009). Biochemical investigations into the absence of rigor mortis in the Norway lobster Nephrops norvegicus. J. Exp. Mar. Biol. Ecol. 373, 58–65. doi: 10.1016/j.jembe.2009.03.004
Hagerman, L. (1983). Hemocyanin concentration of juvenile lobsters (Homarus gammarus) in relation to molting cycle and feeding conditions. Mar. Biol. 77, 11–17. doi: 10.1007/bf00393205
Icely, J. D., and Nott, J. A. (1992). “Digestion and absorption: digestive system and associated organs,” in Microscopic Analtomy of Invertebrates, Decapod Custacea, eds F. W. Harrison and A. G. Humes (New York: Wiley-Liss), 147–201.
Ivacic, D. L., and Labisky, R. F. (1973). Metabolic responses of mourning doves to short-term food and temperature stresses in winter. Wilson Bull. 85, 182–196.
Jacklin, M., and Combes, J. (2007). The Good Practice Guide to Handling and Storing Live Crustacea. United Kingdom: Seafish Publications.
Johnson, L., Coates, C. J., Albalat, A., Todd, K., and Neil, D. (2016). Temperature-dependent morbidity of ‘nicked’ edible crab. Cancer pagurus. Fish. Res. 175, 127–131. doi: 10.1016/j.fishres.2015.11.024
Jones, P. L., and Obst, J. H. (2000). Effects of starvation and subsequent refeeding on the size and nutrient content of the hepatopancreas of Cherax destructor (Decapoda : Parastacidae). J. Crustacean Biol. 20, 431–441. doi: 10.1163/20021975-99990057
Matozzo, V., Gallo, C., and Marin, M. G. (2011). Effects of temperature on cellular and biochemical parameters in the crab Carcinus aestuarii (Crustacea. Decapoda). Mar. Env. Res. 71, 351–356. doi: 10.1016/j.marenvres.2011.04.001
McCue, M. D. (2010). Starvation physiology: reviewing the different strategies animals use to survive a common challenge. Comp. Biochem. Physiol. A Mol. Integr. Physiol. 156, 1–18. doi: 10.1016/j.cbpa.2010.01.002
McLeese, D. W., and Wilder, D. G. (1964). Lobster storage and shipment. Fish. Res. Board Can. Bull. 147, 1–69.
Morris, D. J., Pinnegar, J. K., Maxwell, D. L., Dye, S. R., Fernand, L. J., Flatman, S., et al. (2018). Over 10 million seawater temperature records for the United Kingdom continental shelf between 1880 and 2014 from 17 cefas (United Kingdom government) marine data systems. Earth Syst. Sci. Data 10, 27–51. doi: 10.5194/essd-10-27-2018
Neil, D. M., Fowler, W. S., and Tobasnick, G. (1993). Myofibrillar protein composition correlates with histochemistry in fibres of the abdominal flexor muscles of the Norway lobster (Nephrops norvegicus). J. Exp. Biol. 183, 185–201.
Papathanassiou, E., and King, P. E. (1984). Effects of starvation on the fine-structure of the hepatopancreas in the common prawn Palaemon serratus (Pennant). Comp. Biochem. Physiol. A Physiol. 77, 243–249. doi: 10.1016/0300-9629(84)90055-0
Rosas, C., Cooper, E. L., Pascual, C., Brito, R., Gelabert, R., Moreno, T., et al. (2004). Indicators of physiological and immunological status of Litopenaeus setiferus wild populations (Crustacea. Penaeidae). Marine Biol. 145, 401–413.
Roszer, T. (2014). The invertebrate midintestinal gland (“hepatopancreas”) is an evolutionary forerunner in the integration of immunity and metabolism. Cell Tissue Res. 358, 685–695. doi: 10.1007/s00441-014-1985-7
Rotllant, G., Mente, E., Gisbert, E., and Karapanagiotidis, I. T. (2014b). Effects of different diets on the digestive physiology of adult norway lobster Nephrops norvegicus. J. Shellfish Res. 33, 1–9. doi: 10.2983/035.033.0101
Rotllant, G., Company, J. B., Alvarez-Fernández, I. J., García, A., Aguzzi, J., and Durfort, M. (2014a). The effects of seasonal variation on the nutritional condition of Nephrops norvegicus (Astacidea: Nephropidae) from wild populations in the western Mediterranean. J. Mar. Biol. Ass. UK. 94, 763–773. doi: 10.1017/s0025315414000022
Sacristan, H. J., Ansaldo, M., Franco-Tadic, L. M., Gimenez, A. V. F., and Greco, L. S. L. (2016). Long-term starvation and posterior feeding effects on biochemical and physiological responses of midgut gland of Cherax quadricarinatus juveniles (Parastacidae). PLoS One 11:e0150854. doi: 10.1371/journal.pone.0150854
Sacristan, H. J., Rodríguez, Y. E., Pereira, N. A., López Greco, L. S., Lovrich, G. A., and Fernández Gimenez, A. V. (2017). Energy reserves mobilization: strategies of three decapod species. PLoS One 12:e0184060. doi: 10.1371/journal.pone.0184060
Shimeno, S., Kheyyali, D., and Takeda, M. (1990). Metabolic adaptation to prolonged starvation in carp. Nippon Suisan Gakk. 56, 35–41. doi: 10.2331/suisan.56.35
Söderhäll, K., and Smith, V. J. (1983). Separation of the hemocyte populations of Carcinus maenas and other marine decapods, and prophenoloxidase distribution. Dev. Comp. Immunol. 7, 229–239. doi: 10.1016/0145-305x(83)90004-6
Stathopoulou, E., Apostologamvrou, C., Vafidis, D., Mente, E., and Berillis, P. (2017). Sex differentiation in the histology of the digestive gland of Nephrops norvegicus from Pagasitikos Gulf during spring. Aquat. Living Resour. 30, 28–35.
Stentiford, G. D., Neil, D. M., and Coombs, G. H. (2000). Alterations in the biochemistry and ultrastructure of the deep abdominal flexor muscle of the Norway lobster Nephrops norvegicus during infection by a parasitic dinoflagellate of the genus Hematodinium. Dis. Aquat. Org. 42, 133–141. doi: 10.3354/dao042133
Stewart, J. E., Arie, B., and Horner, G. W. (1972). Effects of temperature, food, and starvation on several physiological parameters of lobster Homarus americanus. J. Fish. Res. Board Can. 29, 439–442. doi: 10.1139/f72-072
Stewart, J. E., Cornick, J. W., Foley, D. M., Li, M. F., and Bishop, C. M. (1967). Muscle weight relationship to serum proteins hemocytes and hepatopancreas in lobster Homarus americanus. J. Fish. Res. Board Can. 24, 2339–2354. doi: 10.1139/f67-189
Thériault, G., Hanlon, J., and Creed, L. (2013). Report of the Maritime Lobster Panel. Available at https://novascotia.ca/fish/documents/Maritime-Lobster-Panel-Report-NOV1.pdf (accessed October 11, 2018).
Tocher, D. R., and Harvie, D. G. (1988). Fatty acid compositions of the major phosphoglycerides from fish neural tissues - (n-3) and (n-6) poly-unsaturated fatty-acids in rainbow-trout (Salmo gairdneri) and Cod (Gadus morhua) brains and retinas. Fish Physiol. Biochem. 5, 229–239. doi: 10.1007/bf01874800
Tully, O., O’Donovan, V., and Fletcher, D. (2000). Metabolic rate and lipofuscin accumulation in juvenile European lobster (Homarus gammarus) in relation to simulated seasonal changes in temperature. Mar. Biol. 137, 1031–1040. doi: 10.1007/s002270000410
Wang, G. Q., and McGaw, I. J. (2014). Use of serum protein concentration as an indicator of quality and physiological condition in the lobster Homarus americanus (Milne-Edwards, 1837). J. Shellfish Res. 33, 805–813. doi: 10.2983/035.033.0315
Keywords: Homarus gammarus, physiology, starvation, holding temperature, condition markers, hemolymph protein concentration
Citation: Albalat A, Johnson L, Coates CJ, Dykes GC, Hitte F, Morro B, Dick J, Todd K and Neil DM (2019) The Effect of Temperature on the Physiological Condition and Immune-Capacity of European Lobsters (Homarus gammarus) During Long-Term Starvation. Front. Mar. Sci. 6:281. doi: 10.3389/fmars.2019.00281
Received: 27 November 2018; Accepted: 13 May 2019;
Published: 29 May 2019.
Edited by:
Gionata De Vico, University of Naples Federico II, ItalyReviewed by:
Josefina Blasco, University of Barcelona, SpainClaudio Agnisola, University of Naples Federico II, Italy
Copyright © 2019 Albalat, Johnson, Coates, Dykes, Hitte, Morro, Dick, Todd and Neil. This is an open-access article distributed under the terms of the Creative Commons Attribution License (CC BY). The use, distribution or reproduction in other forums is permitted, provided the original author(s) and the copyright owner(s) are credited and that the original publication in this journal is cited, in accordance with accepted academic practice. No use, distribution or reproduction is permitted which does not comply with these terms.
*Correspondence: Amaya Albalat, YW1heWEuYWxiYWxhdEBzdGlyLmFjLnVr
†Present address: Laura Johnson and Keith Todd, Unit 4 & 5, Todd Fish Tech, Dalgety Bay, United Kingdom